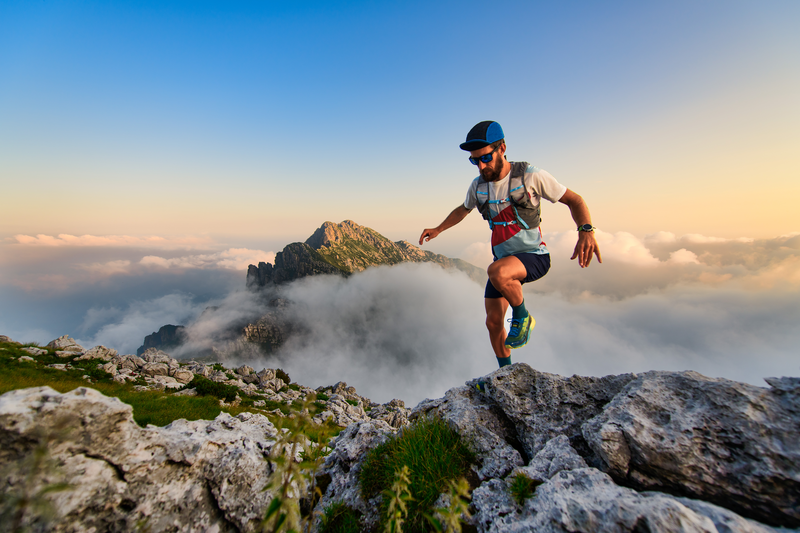
94% of researchers rate our articles as excellent or good
Learn more about the work of our research integrity team to safeguard the quality of each article we publish.
Find out more
ORIGINAL RESEARCH article
Front. Ecol. Evol. , 12 May 2023
Sec. Ecophysiology
Volume 11 - 2023 | https://doi.org/10.3389/fevo.2023.1106635
This article is part of the Research Topic Applications of Conservation Physiology to Wildlife Fitness and Population Health View all 10 articles
In species where offspring survival is highly variable relative to adult survival, such as bighorn sheep (Ovis canadensis), physiological indicators of maternal investment could clarify the functional mechanisms of life history trade-offs and serve as important predictors of population dynamics. From a management perspective, simple predictors of juvenile survival measured non-lethally from maternal samples could aid in identifying at-risk populations or individuals before significant mortality occurs. Blood biochemical parameters can offer low-cost insights into animal health and physiology, therefore we sought to develop a simple biochemical predictor of juvenile survival based on maternal blood samples. We measured biochemical indicators of energy balance in adult bighorn sheep at a single time point in January or February, and then monitored survival through August of the same year to assess how those measures related to survival of individual adults and their juvenile offspring. Juvenile survival was lower over the subsequent spring and summer when maternal adult serum beta-hydroxybutyric acid (β-HBA) concentration was high, indicating a negative energy balance in the mothers. However, serum β-HBA did not correlate with adult survival over the same period. Our findings suggest that even when maternal body condition is high, short-term caloric deficit may be sufficient trigger to decrease investment in offspring survival. This mechanism could protect adult females from investing heavily in juvenile survival when resources become too limited to support population growth. Our study suggests that β-HBA could be a powerful monitoring tool for bighorn sheep and other threatened ruminant populations under resource limitation.
The relative influence of adult versus juvenile survival on population dynamics varies strongly across species depending on whether they are k-selected, i.e., having fewer young with greater parental investment, or r-selected, i.e., having more young with reduced parental investment (Mac Arthur and Wilson, 1967). For k-selected species such as large terrestrial herbivores, which often exist near the carrying capacity of their environment, annual adult survival tends to be relatively high with little variation (Mac Arthur and Wilson, 1967). In contrast, juvenile survival tends to be variable, and therefore population growth is more sensitive to juvenile survival parameters (Gaillard et al., 2000). The trade-off of investing in adult versus juvenile survival has been documented across numerous species, including large terrestrial herbivores. However, the physiological mechanisms underlying these trade-offs remain unclear due to the challenges of obtaining long-term measures of survival and linking them to function (Ricklefs and Wikelski, 2002). Mechanistic understanding of life history choices could contribute to improved understanding of demographic trends in natural wildlife populations.
Reproductive success depends on the physiological propensity of an animal to breed, and subsequently to invest in the survival of its offspring (Wasser and Barash, 1983). In k-selected species that evolved near carrying capacity, we would expect reduced probability of breeding and investment in the survival of fewer offspring when resources become limited (Pianka, 1970). In capital breeders that rely on stored endogenous resources for reproduction and offspring rearing, fertility is heavily dependent on maternal energy balance (Boyd, 2000). This pattern is likely mediated by hormonal regulation (Garcia-Garcia, 2012; Clarke, 2014). Postpartum maternal expenditure is also dependent on resource availability. For example, in bighorn sheep, maternal expenditure decreases when competition for resources increases (Festa-Bianchet et al., 1997). Reduced fertility and maternal expenditure under resource limitation reflects a conservative reproductive strategy that favors maternal rather than offspring survival (Martin and Festa-Bianchet, 2010). While reproductive hormones mediate the relationship between energy balance and fertility, they do not explain the expected trade-off between maternal energy balance and offspring survival. In large mammals, lactation is the costliest part of raising offspring (Clutton-Brock et al., 1989), therefore reducing the quantity or quality of lactation as soon as resources become limited could significantly reduce energy expenditure and enhance maternal survival at the cost of juvenile survival. In k-selected species with high adult survival and variable juvenile survival, we would therefore expect reduced investment in lactation as soon as resources become limited and before significant loss of maternal body condition occurs. Biochemical indicators of current maternal energy balance are therefore likely to be good predictors of investment in offspring survival, because they reflect current resource availability and may be a proxy for lactation quality (Adewuyi et al., 2005).
In species where offspring survival is highly variable relative to adult survival, indicators of maternal investment could be important predictors of population dynamics. In populations of conservation concern, including a biochemistry profile of serum or plasma samples during routine monitoring could provide a low-cost and informative tool for managers to identify at-risk populations or individuals before juvenile mortality occurs. The utility of blood biochemical parameters for monitoring large ungulates has been demonstrated by Milner et al. (2003), who found that parameters reflecting energy balance and body condition were related to subsequent survival and successful calving in Svalbard reindeer. The relationships between blood biochemical parameters and offspring survival have not been evaluated in ungulates from temperate environments or that are subjected to predation, but it is reasonable to expect that energy balance would correlate to survival and reproduction in any resource-limited population.
Biochemical parameters that reflect energy balance in ruminants include serum concentrations of glucose, triglycerides, non-esterified fatty acids (NEFAs), and beta-hydroxybutyric acid (β-HBA). Blood glucose is an important source of fuel for glycolytic tissues, including nerves and muscles, and may decrease when energy stores become depleted (Chowdhury and Ørskov, 1994). However, blood glucose is also highly sensitive to stress and therefore may not accurately reflect energy balance in animals that are captured for sampling (Marco et al., 1997). Adipose tissue in mammals is composed of triglycerides which are broken down, in a process known as lipolysis, to form NEFAs during periods of negative energy balance (Herdt, 2000). NEFA concentrations in the blood could therefore indicate negative energy balance. β-HBA is a type of ketone made in response to negative energy balance in all mammals, but is most clinically apparent in lactating ruminants (Herdt, 2000). In addition to fueling tissues during energy restriction, β-HBA is a precursor to and regulator of milk fat in ruminants (Zhang et al., 2015). β-HBA is much less sensitive to acute stress than glucose, and is therefore unlikely to be affected by capture stress (Koeslag et al., 1980). Because β-HBA directly links maternal energy balance with milk quality in ruminants, this metabolite could accurately indicate when a lactating female is conserving energy versus investing in offspring survival. β-HBA levels in wild ruminant species could therefore represent an important physiological mechanism for life history trade-offs under resource limitation in wild ruminant species.
In this study, we assessed the utility of blood biochemical parameters (Table 1), including measurements of energy balance, organ health, and immune function for predicting adult and juvenile survival in bighorn sheep (Ovis canadensis) in southeastern Oregon and northern Nevada. Bighorn sheep are a large herbivore species historically distributed across the North American west, but now existing mainly in fragmented populations which are vulnerable to local extinction (Whittaker et al., 2004). In this ecosystem, bighorn sheep feed on grasses and shrubs, with diet selection varying by season and by reproductive status (Van Dyke et al., 1983). Adult survival is relatively stable between years, but juvenile survival varies significantly as is typical for k-selected species (Spaan, 2022). Persistence of local populations is therefore highly dependent on juvenile survival rates, and monitoring and predicting juvenile survival is critical for effective management. During our study period, annual pregnancy rates in adult females exceeded 90 and 78% were seen with juveniles (Spaan et al., 2021). Juvenile survival is influenced by many factors including the presence of the respiratory bacterial pathogen Mycoplasma ovipneumoniae and forage quality (Spaan et al., 2021), but the role of maternal health has not been investigated in this region. Maternal body condition, a long-term measure of nutritional status, is known to influence juvenile survival in other bighorn sheep populations (Festa-Bianchet et al., 2019), but the effect of short-term maternal energy balance has not been explored. We hypothesized that parameters reflecting maternal energy balance would predict offspring survival from late gestation through the first 4 months of life, a period when juvenile mortality tends to be particularly high (Spaan et al., 2021). We also hypothesized that these parameters would predict adult survival during the same time period, but to a lesser extent because adult survival rates tend to be relatively stable. We did not expect measures of organ function to predict survival because organ failure is rare in wildlife except in instances of severe malnutrition or disease. Immune function is highly dependent on energy balance (Van Noordwijk and de Jong, 1986), therefore we did not expect immune-related parameters to improve upon survival predictions derived from indicators of energy balance.
To evaluate our hypotheses, we collected a blood sample from each individual in the study population in January or February of each study year and then monitored adult and juvenile survival through August. Our study design was made possible by an intensive monitoring program carried out by the Oregon Department of Fish and Wildlife (ODFW) and Nevada Department of Wildlife (NDOW), but this type of monitoring is not feasible in all bighorn sheep populations. However, periodic capture and blood sampling of individuals is a common management practice even in populations that are not intensively monitored. A simple biochemical indicator of adult and juvenile survival probability could therefore provide a useful tool for large-scale monitoring of bighorn sheep in populations where intensive, long-term monitoring is not possible.
Our study encompassed seven bighorn sheep populations, all located in southeastern Oregon and northern Nevada, United States (Figure 1). The populations included the Blue Mountain (BSP), Trout Creek (TCP), Bowden Hills (BHP), Rattlesnakes (RSP), Ten Mile (TMP), Santa Rosa Population (SRP), and the Upper Owyhee (UOP) populations in Oregon and Nevada. Elevation across the study area ranged from approximately 1,050 m in the Owyhee Canyon to 2,957 m in the Santa Rosa Mountains. Mean precipitation for the study area was approximately 22.5–35.0 cm per annum (Omernik and Griffith, 2014). The study area terrain types included elevated plateaus, sheer-walled canyons with intermittent lakes and ephemeral streams, and mountains of low to mid-elevation with primarily steep slopes and ephemeral or perennial streams (Omernik and Griffith, 2014). For more detailed information on geology, vegetation, wildlife, and land use practices, please refer to Spaan (2022).
Figure 1. Bighorn sheep in this study were from 7 populations in southeastern Oregon and Northern Nevada. Polygons shown here for each population indicate female bighorn sheep 95% summer utilization distributions from Spaan (2022). Abbreviations are: Blue Mountain (BSP), Trout Creek (TCP), Bowden Hills (BHP), Rattlesnakes (RSP), Ten Mile (TMP), Santa Rosa Population (SRP), and the Upper Owyhee (UOP) populations.
All capture, handling, and disease testing were conducted by Oregon Department of Fisheries and Wildlife (ODFW) and Nevada Department of Wildlife (NDOW). Capture methodology followed the recommendations of Foster (2004) and the American Society of Mammalogists (Sikes and the Animal Care and Use Committee of the American Society of Mammalogists, 2016). ODFW and NDOW captured, collared, and sampled adult bighorn sheep across the 7 populations between January 2016 and February 2018. Captures were conducted using a net gun fired from a helicopter, with individual bighorn sheep blindfolded and hobbled once captured (Krausman et al., 1985). Bighorn sheep were brought to a centralized area at the base of their range to be fitted with a telemetry collar and to collect biological samples, except where capture location was too far from basecamp to transport them quickly, in which case they were field processed, at the capture location.
At capture, each adult female was fitted with a Vertex Survey Globalstar collar (Vectronic Aerospace, Berlin, Germany) and each adult male was fitted with one of two collars (n = 41 Vertex Survey Globalstar collars; n = 5 with Telonics Globalstar collars). The age of each adult was estimated from horn growth rings (Geist, 1966; Hoefs and Konig, 1984). Blood was obtained via jugular venipuncture into a vacutainer tube with no additive and placed on ice for transport back to the mobile laboratory within 12 h.
At the mobile laboratory the no-additive tubes containing whole blood were spun at 5000xg for 10 min to separate the serum. The serum was pipetted into sterile cryotubes and frozen in liquid nitrogen for transport back to Oregon State University. The large animal metabolic chemistry panels were run at the Veterinary Diagnostic Laboratory of the Carlson College of Veterinary Medicine at Oregon State University1 on an automated analyzer from the frozen serum in one batch each year to detect all analytes shown in Table 1.
Population-level M. ovipneumoniae presence was determined as described in Spaan et al. (2021). Briefly, testing of adult sheep was performed at Washington Animal Disease Diagnostic Laboratory (WADDL) using PCR (Manlove et al., 2019) and cELISA (Ziegler et al., 2014). Testing of juvenile sheep was done opportunistically, where dead juveniles were located in a population and samples (including either the entire corpse, heart, liver, lungs, the head, nasal and ear swabs, and/or tissue samples depending on the state of decomposition) were submitted to WADDL for gross-and histo-pathology on lung tissue sample and PCR tests on swabs. Similarly to Spaan et al. (2021), for survival analyses, each juvenile bighorn sheep was encoded as M. ovipneumoniae-exposed if it came from a population with dead M. ovipneumoniae juveniles in the same year.
The GPS collars fitted to the adults provide a GPS location every 13 h and were set to report a mortality if stationary for 12 h, allowing us to accurately determine mortality date for each adult. Each collar also transmitted its own unique VHF frequency, with the occasional duplicate placed on individuals in different populations between which dispersal was deemed unlikely. The collars were also fitted with colored tags with unique numbers, allowing for identification of individuals observed in the field.
From 2016 to 2018, as described in Spaan et al. (2021), we conducted semi-monthly observations of all collared adult females between April 1 and August 31 to assess the survival of their juvenile. Juvenile identification was determined via observation of physical contact between adult females and juveniles, such as nursing or bedding down together. Juveniles are weaned at approximately 4 months of age (Festa-Bianchet, 1988); thus, our observation period was intended to cover birth through weaning. We located adult females for observation using an R-1000 telemetry receiver fitted with an RA-23 K VHF directional antenna (Telonics, Inc., Mesa, AZ). We conducted observations with Kowa TSN-601 spotting scopes fitted with a 20–60x magnification mounted on tripods. Once an adult female was confirmed to not or no longer have a juvenile, i.e., two consecutive observations where the adult female was observed without a juvenile, we stopped tracking that individual adult female (e.g., Cassirer and Sinclair, 2007).
We conducted the following analyses separately for adult bighorn sheep and juveniles. We estimated survival functions of adults and juveniles using Kaplan–Meier survival estimates (Kaplan and Meier, 1958) using the R package survival (Therneau, 2021). For an initial selection of covariates for model selection, we used extreme gradient boosting (Chen and Guestrin, 2016) in the package xgboost (Chen et al., 2021) to estimate the relative importance of multiple physiological parameters (Supplementary Appendix I) in predicting binary outcomes of known deaths vs. survival or unknown fates. We chose covariates with a relative importance greater than 0.05 to use in the model selection process for Cox proportional hazards models (Cox, 1972) using right-censored data to estimate correlates of selected covariates with survival. We calculated the pairwise Pearson’s correlation coefficient for all selected covariates, and using a correlation threshold of 0.5, we chose one of two correlated variables for the next model selection step. Using an exhaustive model selection process, we compared the Akaike’s information criteria (AIC) score for each model that included sex, population, and age for adult survival, and population and adult female’s age for juvenile survival.
With the most parsimonious models for adult and juvenile survival, we used the Cox proportional hazards (hereafter Coxph) model to investigate the association between survival time and predictor covariates identified in the previous analysis. Only complete cases (i.e., no missing data) were used in the Coxph models. We used survival data for eight observation periods, each lasting approximately 2 weeks. We used an alpha value of 0.05 for thresholds of model and parameter significance, and after Bonferroni correction for multiple comparisons using the same data (two tests), we used an alpha value of 0.025. For visualization of variation in hazard ratio in relation to individual continuous covariates, we used the R packages rankhazard (Karvanen and Koski, 2016) and smoothHR (Araújo and Meira-Machado, 2022) to examine relationships between individual covariates and survival. All analyses were performed in R version 3.6.3 (R Core Team, 2020).
We observed the survival outcomes of 129 adult sheep (82 female, 47 male) and 61 juveniles across seven populations during the study period (Figure 2). Adult estimates of survival over the study period were high (0.92, Figure 3) while juvenile estimates of survival were relatively low (0.44, Figure 3).
Figure 2. Sample sizes and demographic distribution of Bighorn Sheep (Ovis canadensis) in seven populations in Nevada and Oregon, United States. Sample sizes for juveniles are reflected in “F with lamb” categories; i.e., each pink point represents two individuals used in this study (a female and its juvenile), blue points represent one female without a juvenile, and yellow points represent one male.
Figure 3. Kaplan–Meier survival estimates and sample sizes for adult bighorn sheep and juveniles across 8 observation periods. Each observation period lasted approximately two weeks.
Covariates considered are listed in Table 1. The covariates that were important in predicting survival in the extreme gradient boosting analysis (Supplementary Appendix I) were age, P (phosphate), total CO2, creatinine, and β-HBA, cholesterol and Cl (chloride). P and TCO2 were correlated with each other (Supplementary Appendix VII) so we did not include both in the Coxph model. We chose to use P, because the distribution of TCO2 data for bighorn sheep with different survival outcomes was highly variable (Supplementary Appendix II). However, we reported results from models including TCO2 to inform future studies (Supplementary Appendix V). Cholesterol and the total number of monocytes had >0.05 relative importance in predicting binary sheep survival (Supplementary Appendix I), but inclusion of these covariates substantially lowered sample size of full cases (n = 125 to 95), therefore we left them out of the Coxph model selection step. The most parsimonious Coxph model included P, creatinine, and β-HBA (Supplementary Appendix III). We observed a significant and positive correlation between P and bighorn sheep mortality (Table 2; Figure 4). Age was also significantly and positively correlated with bighorn sheep mortality (Table 2). The effect size of age on adult mortality was 1.62 (95% CI 1.12–2.33), indicating that a one-year increase in age was associated with a 1.62 increase in the chances of mortality. The effect size of P on adult mortality was 2.29 (95% CI 1.05–4.98), indicating that a 1 mg/dL increase in P was associated with a 2.29 increase in the chances of mortality.
Figure 4. Violin plots overlaid with box plots of phosphate (P) in adult bighorn sheep, grouped by survival outcome (A), and corresponding smoothed hazard functions of the same variables with 95% confidence intervals for adults (B). Minimum values refer to values of x when the natural log of hazard ratio is 0. Violin plots indicate probability density of the raw phosphate data points, and boxplots indicate median and interquartile range of the same data points.
Covariates considered are listed in Table 1. The covariates that were important in predicting juvenile survival in the extreme gradient boosting analysis (Supplementary Appendix 1) were the presence/absence of M. ovipneumoniae, adult female β-HBA, adult female K (potassium), total number of adult female monocytes and total number of adult female neutrophils. None of these covariates were correlated with each other (Supplementary Appendix VII). The total number of adult female monocytes and neutrophils had >0.05 relative importance in predicting binary juvenile survival (Supplementary Appendix I), but inclusion of these covariates substantially lowered sample size of full cases (N = 59 to 46) for juveniles, therefore we left them out of the Coxph model selection step. The most parsimonious Coxph model included all three covariates (M. ovipneumoniae, adult female’s β-HBA and K, Supplementary Appendix IV). We observed a significant and positive correlation with adult females’ β-HBA and juvenile mortality (Table 3; Figure 5). When the β-HBA outlier was removed, the overall result remained unchanged (Supplementary Appendix VI). With the full dataset, the effect size of β-HBA was 1.83 (95% CI 1.02–3.28), indicating that a 1 mg/dL increase in maternal β-HBA was associated with a 1.83 increase in the chances of juvenile mortality.
Table 3. Results of Cox proportional hazard models for juvenile bighorn sheep (Ovis canadensis, Likelihood ratio test = 26.66, DF = 9, p = 0.002).
Figure 5. Violin plots overlaid with box plots of beta-hydroxybutyric acid (β-HBA) in adult bighorn sheep (A) and maternal β-HBA in juveniles (B), grouped by survival outcome. Corresponding smoothed hazard functions of the same variables with 95% confidence intervals for adults (C) and juveniles (D). Minimum values refer to values of x when the natural log of hazard ratio is 0. Violin plots indicate probability density of the raw β-HBA data points, and boxplots indicate median and interquartile range of the same data points.
Maternal β-HBA, an indicator of negative energy balance in ruminants, was a significant predictor of bighorn sheep juvenile mortality during the 16 weeks after sampling in our study. Because β-HBA supplies energy to tissues during periods of caloric deficit and is a regulator of milk fat production (Zhang et al., 2015), this metabolite may be an important biochemical mediator of the trade-off between adult versus offspring survival in k-selected ruminant species. Our findings align with previous work in Svalbard reindeer, where maternal β-HBA concentration was also associated with reduced juvenile survival (Milner et al., 2003). Together, results from these studies suggest that maternal β-HBA could be a consistent indicator of stage-specific survival probability in capital breeders. In species where population dynamics are largely determined by juvenile survival rates, the ability to predict offspring survival rates from a simple biochemical indicator could contribute to large-scale monitoring efforts and facilitate targeted conservation actions.
In contrast to juvenile survival, serum concentration of β-HBA was not a significant predictor of adult survival, possibly because it is an indicator of current energy balance and does not directly correspond to total energy stores. Body mass and fat percentage, which are more direct measures of stored energy, have previously been found to correlate with overwinter survival of bighorn adult females (Festa-Bianchet et al., 1997; Denryter et al., 2022). However, we only monitored adult survival from January or February through August, so it is unknown how the short-term measures of energy balance in our study might correlate with overwinter survival. Among the biochemical parameters that we measured, serum phosphate concentration was the only significant predictor of adult survival. Adult ruminants excrete most of their phosphorus as phosphate in saliva to support rumen microorganisms and to buffer rumen pH (Grünberg, 2014), therefore increased serum phosphate could indicate decreased saliva production due to dehydration. Dehydration could reflect a number of factors such as capture stress, underlying disease, or unknown environmental stressors that decrease probability of survival in adults.
Survival probability for juveniles was only 44% over the 16 week monitoring period of our study, compared with 92% survival in adults. This highlights the extreme vulnerability of bighorn sheep juveniles and the important role of juvenile mortality rates in driving population dynamics. In addition to maternal energy balance, juvenile mortality is known to be related to M. ovipneumoniae infection in our study system. Using data from the same populations, Spaan et al. (2021) found that the populations without M. ovipneumoniae have juvenile survival rates that are 20 times higher than populations with M. ovipneumoniae. Similarly, our analysis found that M. ovipneumoniae presence in the population was important in predicting binary outcomes of juvenile survival, although not statistically significant in Cox survival models once we controlled for population (due to reduced sample sizes and population structure compared to Spaan et al., 2021). However, it is important to consider the effects of maternal energy balance in the context of disease, predation, and other environmental stressors on juvenile survival.
Our study found that maternal β-HBA, a simple biochemical indicator of negative energy balance, significantly predicted juvenile survival from late pregnancy through the first 4 months post-birth, but did not predict adult survival during the same period. This finding may represent a snapshot of the physiological processes involved in life history trade-offs of a k-selected herbivore species. Even when maternal body condition is high, short-term caloric deficit may be a sufficient trigger to decrease investment in offspring survival. This mechanism could protect adult females from investing heavily in juvenile survival when resources become too limited to support population growth. Our study suggests that β-HBA could be a powerful monitoring tool for bighorn sheep and other threatened capital breeding populations under resource limitation. However, maternal energy balance is only one factor that affects juvenile survival, and it must be interpreted in the context of environmental and disease factors that vary over time and among populations. For example, although we did not detect an interaction effect of M. ovipneumoniae and β-HBA on lamb survival in our study, such a relationship could manifest in other ecosystems or on different time scales. Additionally, the drivers of maternal energy balance in bighorn sheep are not fully understood. Previous research found that forage availability did not strongly influence juvenile survival, suggesting that competition or other behavioral traits might underlie the differences in maternal energy balance we observed in our study. Future research should seek to determine the relative importance of maternal energy balance, disease, and other variables for predicting juvenile survival, as well as the underlying causes of variation in maternal energy balance.
The datasets presented in this study can be found in online repositories. The names of the repository/repositories and accession number(s) can be found at: https://github.com/devansong/BHS.
The animal study was reviewed and approved by Oregon State University Institutional Animal Care and Use Committee.
CL helped with data analysis design, interpreted finding, and led the writing. MG helped with early data analysis and participated in editing of drafts. AD-S performed the data analysis. JB, CC, and RS helped with data collection and interpretation. CE and BB advised students on the project, helped with data collection, and conceived of the project. All authors contributed to the article and approved the submitted version.
This work was supported by the United States Department of Interior and Oregon Department of Fish and Wildlife (nos. F15AF01356 and F19AF00469), Oregon Foundation of North American Wild Sheep, Nevada Bighorn Unlimited—Midas, Nevada Bighorn Unlimited—Reno, and Nevada Muleys, NSF-NIH-NIFA Ecology and Evolution of Infectious Disease as grant number DEB 1911994 and UK Biotechnology and Biological Sciences Research Council as grant number BB/T011416/1. The funders had no role in study design, data collection and analysis, decision to publish, or preparation of the manuscript.
Captures, collaring, and sampling of bighorn sheep were conducted by Oregon Department of Fish and Wildlife (ODFW) and Nevada Department of Wildlife (NDOW). We thank Phillip Milburn, Scott Torland, and Autumn Larkins of ODFW for helping to initiate this project. We also thank Ed Partee of NDOW for support when working with the bighorn sheep that were located on the Nevada side of the population. We thank the veterinarian involved from NDOW, Peregrine Wolff (NDOW), and all of the support staff at ODFW and NDOW. Field technicians Geoff Gerdes, Logan Gmuender, Lindsey Howard, and Colton Padilla were essential in the collection of data. Dr. Jennifer Johns, a veterinary clinical pathologist, assisted in the interpretation of our findings. Dana Tsuchida, a veterinary student, helped with early versions of analysis and discussion that led to this paper.
The authors declare that the research was conducted in the absence of any commercial or financial relationships that could be construed as a potential conflict of interest.
All claims expressed in this article are solely those of the authors and do not necessarily represent those of their affiliated organizations, or those of the publisher, the editors and the reviewers. Any product that may be evaluated in this article, or claim that may be made by its manufacturer, is not guaranteed or endorsed by the publisher.
The Supplementary material for this article can be found online at: https://www.frontiersin.org/articles/10.3389/fevo.2023.1106635/full#supplementary-material
Adewuyi, A. A., Gruys, E., and van Eerdenburg, F. J. (2005). Non esterified fatty acids (NEFA) in dairy cattle. Review. Vet. Q. 27, 117–126. doi: 10.1080/01652176.2005.9695192
Araújo, A., and Meira-Machado, L. (2022). Smooth HR: smooth hazard ratio curves taking a reference value. R package version 1.0.4 Available at: https://CRAN.R-project.org/package=smoothHR
Boyd, I. L. (2000). State-dependent fertility in pinnipeds: contrasting capital and income breeders. Funct. Ecol. 14, 623–630. doi: 10.1046/j.1365-2435.2000.t01-1-00463.x
Cassirer, E. F., and Sinclair, A. R. E. (2007). Dynamics of pneumonia in a bighorn sheep metapopulation. J. Wildl. Manag. 71, 1080–1088. doi: 10.2193/2006-002
Chen, T., and Guestrin, C., (2016). Xgboost: a scalable tree boosting system. In Proceedings of the 22nd ACM SIGKDD international conference on knowledge discovery and data mining (pp. 785–794)
Chen, Tianqi, He, Tong, Benesty, Michael, Khotilovich, Vadim, Tang, Yuan, Cho, Hyunsu, et al. (2021). Xgboost: Extreme gradient boosting. R package version 1.4.1.1. Available at: https://CRAN.R-project.org/package=xgboost
Chowdhury, S. A., and Ørskov, E. R. (1994). Implications of fasting on the energy metabolism and feed evaluation in ruminants. J. Anim. Feed Sci. 3, 161–169. doi: 10.22358/jafs/69830/1994
Clarke, I. J. (2014). Interface between metabolic balance and reproduction in ruminants: focus on the hypothalamus and pituitary. Horm. Behav. 66, 15–40, ISSN 0018-506X. doi: 10.1016/j.yhbeh.2014.02.005
Clutton-Brock, T., Albon, S., and Guinness, F. (1989). Fitness costs of gestation and lactation in wild mammals. Nature 337, 260–262. doi: 10.1038/337260a0
Cox, D. R. (1972). Regression models and life-tables. J. R. Stat. Soc. B 34, 187–202. doi: 10.1111/j.2517-6161.1972.tb00899.x
Denryter, K., Conner, M., Stephenson, T., German, D., and Monteith, K. (2022). Survival of the fattest: how body fat and migration influence survival in highly seasonal environments. Funct. Ecol. 36, 2569–2579. doi: 10.1111/1365-2435.14151
Festa-Bianchet, M. (1988). Nursing behavior of bighorn sheep: correlates of ewe age, parasitism, lamb age, birthdate and sex. Anim. Behav. 36, 1445–1454. doi: 10.1016/S0003-3472(88)80215-X
Festa-Bianchet, M., Côté, S. D., Hamel, S., and Pelletier, F. (2019). Long-term studies of bighorn sheep and mountain goats reveal fitness costs of reproduction. J. Anim. Ecol. 88, 1118–1133. doi: 10.1111/1365-2656.13002
Festa-Bianchet, M., and Jorgenson, J. T. (1998). Selfish mothers: reproductive expenditure and resource availability in bighorn ewes. Behav. Ecol. 9, 144–150.
Festa-Bianchet, M., Jorgenson, J. T., Bérubé, C. H., Portier, C., and Wishart, W. D. (1997). Body mass and survival of bighorn sheep. Can. J. Zool. 75, 1372–1379. doi: 10.1139/z97-763
Gaillard, J.-M., Festa-Bianchet, M., Yoccoz, N. G., Loison, A., and Toïgo, C. (2000). Temporal variation in fitness components and population dynamics of large herbivores. Annu. Rev. Ecol. Syst. 31, 367–393. doi: 10.1146/annurev.ecolsys.31.1.367
Garcia-Garcia, R. M. (2012). Integrative control of energy balance and reproduction in females. ISRN Vet. Sci. 2012:121389. doi: 10.5402/2012/121389
Geist, V. (1966). Validity of horn segment counts in aging bighorn sheep. J. Wildl. Manag. 30, 634–635. doi: 10.2307/3798763
Grünberg, W. (2014). Treatment of phosphorus balance disorders. Vet. Clin. North Am. Food Anim. Pract. 30, 383–408. doi: 10.1016/j.cvfa.2014.03.002
Herdt, T. H. (2000). Ruminant adaptation to negative energy balance: influences on the etiology of ketosis and fatty liver. Vet. Clin. North Am. Food Anim. Pract. 16, 215–230, ISSN 0749-0720. doi: 10.1016/S0749-0720(15)30102-X
Hoefs, M., and Konig, R. (1984). Reliability of aging old dall sheep ewes by the horn annulus technique. J. Wildl. Manag. 48:980. doi: 10.2307/3801451
Kaplan, E. L., and Meier, P. (1958). Nonparametric estimation from incomplete observations. J. Am. Stat. Assoc. 53, 457–481. doi: 10.1080/01621459.1958.10501452
Karvanen, J., and Koski, N. (2016). rankhazard: rank-hazard plots. R package version 1.1.0. Available at: https://CRAN.R-project.org/package=rankhazard
Koeslag, J. H., Noakes, T. D., and Sloan, A. W. (1980). Post-exercise ketosis. J. Physiol. 301, 79–90. doi: 10.1113/jphysiol.1980.sp013190
Krausman, P. R., Hervert, J. J., and Ordway, L. L. (1985). Capturing deer and mountain sheep with a net-gun. Wildl. Soc. Bull. 13, 71–73.
Mac Arthur, R. H., and Wilson, E. O. (1967). The theory of island biogeography. Princeton, N.J.: Princeton University Press, p. 203.
Manlove, K., Branan, M., Baker, K., Bradway, D., Cassirer, E. F., Marshall, K. L., et al. (2019). Risk factors and productivity losses associated with Mycoplasma ovipneumoniae infection in United States domestic sheep operations. Prev. Vet. Med. 168, 30–38. doi: 10.1016/j.prevetmed.2019.04.006
Marco, I., Viñas, L., Velarde, R., Pastor, J., and Lavin, S. (1997). Effects of capture and transport on blood parameters in free-ranging mouflon (Ovis ammon). J. Zoo Wildl. Med. 28, 428–433.
Martin, J. G. A., and Festa-Bianchet, M. (2010). Bighorn ewes transfer the costs of reproduction to their lambs. Am. Nat. 176, 414–423. doi: 10.1086/656267
Milner, J. M., Stien, A., Irvine, R. J., Albon, S. D., Langvatn, R., and Ropstad, E. (2003). Body condition in svalbard reindeer and the use of blood parameters as indicators of condition and fitness. Can. J. Zool. 81, 1566–1578. doi: 10.1139/z03-152
Omernik, J. M., and Griffith, G. E. (2014). Ecoregions of the conterminous United States: evolution of a hierarchical spatial framework. Environ. Manag. 54, 1249–1266. doi: 10.1007/s00267-014-0364-1
R Core Team. (2020). R: a language and environment for statistical computing. R Foundation for Statistical Computing, Vienna, Austria. Available at: https://www.R-project.org/
Ricklefs, R. E., and Wikelski, M. (2002). The physiology/life-history nexus. Trends Ecol. Evol. 17, 462–468, ISSN 0169-5347. doi: 10.1016/S0169-5347(02)02578-8
Sikes, R. S., the Animal Care and Use Committee of the American Society of Mammalogists (2016). 2016 guidelines of the American Society of Mammalogists for the use of wild mammals in research and education. J. Mammal. 97, 663–688.
Spaan, R. S. (2022). Characterizing the spread and consequences of Mycoplasma ovipneumoniae on Bighorn sheep (Ovis canadensis). Northern Basin Range Ecosystem. doi: 10.13140/RG.2.2.30060.85121
Spaan, R. S., Epps, C. W., Crowhurst, R., Whittaker, D., Cox, M., and Duarte, A. (2021). Impact of Mycoplasma ovipneumoniae on juvenile bighorn sheep (Ovis canadensis) survival in the northern basin and range ecosystem. Peer J. 9:e10710. doi: 10.7717/peerj.10710
Therneau, T, (2021). A package for survival analysis in R. R packageversion 3.2-13 Available at: https://CRAN.R-project.org/package=survival
Van Dyke, W., Sands, A., Yoakum, J., Polenz, A., and Blaisdell, J. (1983). Wildlife habitats in managed rangelands the Great Basin of southeastern Oregon. Gen. Tech. Rep. PNW-GTR-159. Portland, OR: U.S. Department of Agriculture, Forest Service, Pacific northwest Forest and range Experiment Station, p. 36
Van Noordwijk, A. J., and de Jong, G. (1986). Acquisition and allocation of resources: their influence on variation in life history tactics. Am. Natural. 128, 137–142. doi: 10.1086/284547
Wasser, S. K., and Barash, D. P. (1983). Reproductive suppression among female mammals: implications for biomedicine and sexual selection theory. Q. Rev. Biol. 58, 513–538. doi: 10.1086/413545.
Whittaker, D. G., et al. (2004). Genetic variability of reintroduced California Bighorn sheep in Oregon. J. Wildl. Manag. 68, 850–859.
Zhang, M., Zhang, S., Hui, Q., Lei, L., Du, X., Gao, W., et al. (2015). β-Hydroxybutyrate facilitates fatty acids synthesis mediated by sterol regulatory element-binding protein1 in bovine mammary epithelial cells. Cell. Physiol. Biochem. 37, 2115–2124. doi: 10.1159/000438569
Keywords: bighorn sheep, energy balance, maternal effects, body condition, wildlife health monitoring, plasma biochemistry profile
Citation: Laliberte C, Devan-Song A, Burco JD, Couch CE, Gentzkow MF, Spaan RS, Epps CW and Beechler BR (2023) A simple biochemical plasma test as an indicator of maternal energy balance predicts offspring survival in bighorn sheep. Front. Ecol. Evol. 11:1106635. doi: 10.3389/fevo.2023.1106635
Received: 24 November 2022; Accepted: 25 April 2023;
Published: 12 May 2023.
Edited by:
Terri Maness, Louisiana Tech University, United StatesReviewed by:
Thomas Stephenson, California Department of Fish and Wildlife, United StatesCopyright © 2023 Laliberte, Devan-Song, Burco, Couch, Gentzkow, Spaan, Epps and Beechler. This is an open-access article distributed under the terms of the Creative Commons Attribution License (CC BY). The use, distribution or reproduction in other forums is permitted, provided the original author(s) and the copyright owner(s) are credited and that the original publication in this journal is cited, in accordance with accepted academic practice. No use, distribution or reproduction is permitted which does not comply with these terms.
*Correspondence: Brianna R. Beechler, QnJpYW5uYS5iZWVjaGxlckBvcmVnb25zdGF0ZS5lZHU=
Disclaimer: All claims expressed in this article are solely those of the authors and do not necessarily represent those of their affiliated organizations, or those of the publisher, the editors and the reviewers. Any product that may be evaluated in this article or claim that may be made by its manufacturer is not guaranteed or endorsed by the publisher.
Research integrity at Frontiers
Learn more about the work of our research integrity team to safeguard the quality of each article we publish.