- Department of Endangered Species Management, Wildlife Institute of India, Dehradun, Uttrakhand, India
Introduction: Terrestrial mammals play a pivotal role in tropical forest ecosystems, representing a rich and functionally diverse component of these biomes. However, they confront formidable threats globally, such as hunting, habitat loss, and fragmentation, amidst the expansion of human-altered landscapes in tropical regions. Understanding the impacts of these changes on mammal communities is crucial for designing effective conservation interventions. Northeast India's tropical forests, a biodiversity hotspot, host a diverse array of mammalian fauna. The primary challenge faced by these species involves anthropogenic activities leading to the shrinkage and fragmentation of forested habitats.
Methodology: In this study, we conducted systematic camera trapping in Mehao Wildlife Sanctuary (WLS) to assess mammal status and distribution. Employing single-season occupancy models, we evaluated factors influencing mammalian species' detection probability and spatial utilization within the landscape.
Results: The study documented 27 mammalian species, with 12 identified as "threatened" on the IUCN Red List. Species like clouded leopard, marbled cat, Gongshan muntjac, red goral, Mishmi takin, and sambar were recorded but observed infrequently. Notably, we reported the Asiatic brush-tailed porcupine's first sighting in Mehao. Our findings revealed that undisturbed forest habitats were preferred by all species. Mainland serow dominated most of the sanctuary's area, followed by northern red muntjac, yellow-throated marten, and masked palm civet.
Discussion: The response of mammalian species to ecological and anthropogenic covariates varied significantly, underscoring the necessity for species-specific management strategies alongside a landscape-scale conservation approach. These outcomes emphasize the urgency of managing and safeguarding rare and elusive mammalian species within and around Mehao WLS.
1 Introduction
Though tropical forests cover less than 10% of the Earth’s land surface (Bradshaw et al., 2009), they are incredibly diverse and support at least two-thirds of the world’s biodiversity (Raven, 1988). Current research estimates that tropical forests will have one of the highest rates of natural vegetation loss in the near future (Smith, et al., 2023). Protecting tropical forests is the central thrust of several national and international biodiversity conservation strategies (CBD, 2015; Panfil & Harvey, 2015). Tropical forest provides substantial local, regional, and global benefits through economic goods and ecosystem services. As they have greater species diversity than other ecosystems, tropical forest biodiversity losses are likely greater (Rosenzweig, 1992). Mammals in tropical forests are a priority group as they represent a rich and functionally diverse component of this biome even as they face threats such as hunting, habitat loss, and habitat fragmentation (Ceballos et al., 2005; Schipper et al., 2008; Visconti et al., 2011; Ahumada et al., 2011). Tropical mammals are among the most threatened species globally, yet their status remains challenging to establish due to the inherent difficulty in their observation (Linkie et al., 2007). It is important to consider that their long-term trends may not be well-established, and the ‘most threatened’ designation assumes certain knowledge about their status. Information on distribution, habitat utilization, and threat response are critical to developing conservation strategies (Espartosa et al., 2011).
Mammals play a crucial role in maintaining ecosystem functions, including forest structures, nutrient cycling, carbon storage, seed dispersal and trophic management (Brodie et al., 2009; Jansen et al., 2010; Estes et al., 2011; Sobral et al., 2017). However, the secretive and cryptic nature of forest-dwelling mammals hinders data collection and the development of conservation measures. Statistical techniques, such as occupancy modeling, which considers and corrects imperfect detection, are essential to generate evidence for environmental policies (MacKenzie et al., 2002; Brodie et al., 2015; Guillera-Arroita, 2017). Track and sign surveys, direct observations, camera trapping, and interviews with local communities and hunters currently detect mammalian fauna (Ramesh and Downs, 2014; De Bondi et al., 2010; Aiyudurai et al., 2010). Numerous studies indicate that camera traps are an effective method to survey mammals, including rare, elusive, and nocturnal species (Silveira et al., 2003; Tobler et al., 2008; Harmsen et al., 2010; Espartosa et al., 2011; O’Connell et al., 2011). The use of camera traps to collect data on the distribution and abundance of mammals can provide a wealth of information (Rowcliffe and Carbone, 2008; Tobler et al., 2009; Pettorelli et al., 2010; Kinnaird and O’Brien, 2012; Ramesh et al., 2012; Ramesh et al., 2013). Sign surveys (Carrillo et al., 2000; Naughton-Treves et al., 2003; Harvey et al., 2006; Bali et al., 2007; Parry et al., 2007; Norris et al., 2008) are increasingly being used to survey tropical forest mammals. In tropical forests, suitable substrates for footprint impressions are not always present, and weather conditions lead to biases in sign surveys (Dirzo and Miranda, 1990; Norris et al., 2008).
To reconcile conservation and development, it is increasingly important to comprehend how human-modified landscapes in the tropics affect mammal communities (Boron et al., 2019). In tropical rainforests, habitat loss, hunting, land-use change, and deforestation threaten biodiversity conservation (Schipper et al., 2008; Visconti et al., 2011; Barlow et al., 2016; Hansen et al., 2008; Laurance and Luarance, 1999). Agriculture and tree plantations are rapidly increasing across the tropics to meet rising human demand for food, timber, and fiber (Hansen et al., 2013; Abood et al., 2015; Haddad et al., 2015; Riitters et al., 2016). These developments are lowering the area of forests and fragmenting or reducing wildlife habitat (Laurance et al., 2002; Fischer and Lindenmayer, 2007; Laurance et al., 2011). In addition, the problem of hunting has worsened due to expanding human populations, easier access to remote forests, and the use of modern hunting techniques and weapons. According to recent estimates, hunting pressure and land-use changes have caused mammalian populations to decline by more than 80% and 30%, respectively (Almeida-Rocha et al., 2017; Benítez-López et al., 2017). The native populations of local communities in northeast India have more in common with Southeast Asian cultures than peninsular India, and hunting is common (Datta, 2007). Hunting is common in Arunachal Pradesh, and it has decimated wildlife populations in the state (Datta, 2002; Hilaluddin and Ghose, 2005; Mishra et al., 2006).
Several surveys and documentation have been carried out in Arunachal Pradesh on large and small mammals (Athreya et al., 1997; Chowdhury, 1997; Datta, 1998; Datta et al., 2008a; Mishra et al., 2006; Gopi et al., 2010; Gopi et al., 2012; Selvan, 2013; Dasgupta et al., 2014; Selvan et al., 2014b; Dasgupta et al., 2015; Adhikarimayum and Gopi, 2018), Malayan sun bear (Sethy and Chauhan, 2012) and red panda (Kakati, 1996). Most wildlife surveys in Arunachal have been restricted to low and mid-elevation forests and have focused on rare species (Katti et al., 1990; Athreya and Johnsingh, 1995; Selvan et al., 2013; Roy et al., 2015). Very few surveys or ecological research in the state have produced significant discoveries and information (Datta and Goyal, 1997; Selvan et al., 2014a) of new species like leaf deer (Datta et al., 2003), Chinese goral (Mishra et al., 2004) and Arunachal macaque (Sinha et al., 2005).
In this context, we initiated the first systematic camera trapping study to evaluate the assemblage of terrestrial mammals in Mehao WLS and to understand factors affecting detection probability and occupancy. Mehao WLS is located in Arunachal Pradesh in the eastern Himalayas, which has been recognized as a global biodiversity hotspot (Myers et al., 2000). The rugged terrain and dense forest cover of Mehao WLS harbor 60 species of mammals (Chakraborty and Sen, 1991). Idu Mishmi and Adi are the main communities living around Mehao WLS. The Idu Mishmi community has social taboos restricting hunting and forest use, which may have contributed to protecting biodiversity in the area (Nijhawan and Mihu, 2020). Few studies have been conducted on mammals in Mehao WLS (Katti et al., 1990; Chakraborty and Sen, 1991; Sarma et al., 2015; Ahmad et al., 2023). The ones that have been carried out focus primarily on the status and distribution of hoolock gibbon (Chetry et al., 2010; Roy et al., 2015), while a write-up on Mehao WLS was published by Sinha (1984).
Our specific objectives for this research were (1) To determine the species richness and relative detection rate of mammals in and around Mehao WLS and (2) To investigate the factors influencing the detection probability and occupancy of mammalian fauna. The findings of this study are of paramount importance for managing and conserving rare and elusive species in and around Mehao WLS.
2 Study area
The current study was conducted in Mehao WLS, Lower Dibang Valley district of Arunachal Pradesh (Figure 1). The sanctuary was established in 1980 and is home to numerous species of wildlife, including tigers, leopards, hoolock gibbons, tree shrews, wild dogs, and clouded leopards. Mehao WLS lies between 28˚ 05’ and 28˚ 15’ north latitude and 93˚ 30’ and 95˚ 45’ east longitudes and is spread over an area of 281.5 sq km. The terrain in Mehao WLS is plain in the southern section and hilly in the northern sections, with an elevation range of 400-3,568m above mean sea level. The valleys are narrow around river courses. The sanctuary is dotted with small flat areas at various elevations that provide habitat for various wildlife species. All the streams flow into the Dibang River, which later empties into the Lohit River—an important tributary of the Brahmaputra River. Several seasonal streams drain into these rivers, which flow rapidly during the monsoon.
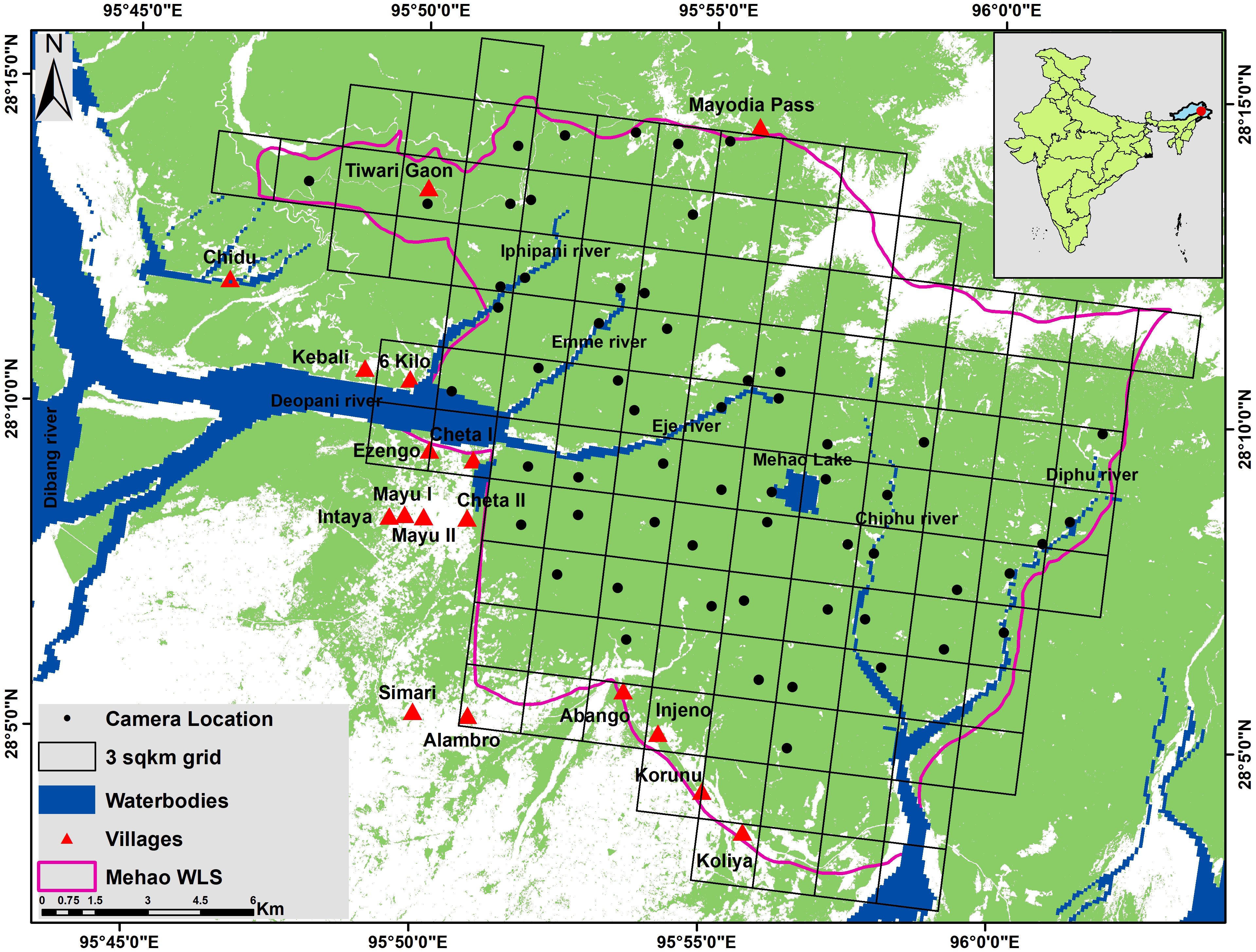
Figure 1 Map showing the location of camera trap, grids, and forest cover (green) in Mehao Wildlife Sanctuary.
Champion and Seth (1968) state that the study area falls within 8B/CI East Himalayan Sub-tropical Wet Hill Forests. The forest type changes with altitude in Mehao WLS. It includes tropical evergreen forest (900m amsl), subtropical and temperate forest (900m-1,800m amsl), temperate broad leave forest (1,800m-2,800m amsl), and temperate conifer forest (2,800m-3,500m amsl). The forest is dominated by Terminalia myriocarpa, Messua ferrea, Dillenia indica, Castanopsis indica, and Albizia lucida while primary shrub and herb species include Clerodendrum viscosum, Tephrosia candida, Maesa indica, and Solanum khasianum. Bamboo species in the area include Dendrocalamus giganteus, Phyllostachys bambusoides, Bambusa pallida, Dendrocalamus hamiltonii, and Melocanna baccifera. Mehao WLS is also home to Mishmi teeta (Coptis teeta), an extremely valuable medicinal herb and threatened holoparasitic plant, Sapria himalayana (Ahmad et al., 2020). The sanctuary is warmer at lower altitudes and cooler at higher altitudes. In the summer, the average temperature at a lower altitude is around 38°C and falls to 4°C- 12°C in the winter. At higher altitudes, the summer temperature is around 12°C, and it experiences snowfall in the winter with temperatures around -3°C.
Numerous Idu Mishmi villages along the sanctuary’s periphery include Koronu, Injuno, Abango, Balek, Simari, and Tiwarigaon. Strong taboos exist among the Idu Mishmi, prohibiting hunting gibbons and other wild animals. This has probably contributed to the abundance of wildlife in the region.
3 Methodology and data analysis
The field survey was conducted between November 2017 to February 2018. Reconnaissance was carried out during the initial months of the study period to develop adequate knowledge and understanding of the area while also collecting baseline data for further intensive sampling. An effort was made to deploy camera trap units randomly throughout the study area. A total of 58 camera traps were deployed with only one per 3 sq km grid, thus covering an area of 174 sq km. Cuddeback C1 camera traps were used. They have a trigger speed of 1.4 seconds, a detection range of 100 feet and were programmed to recover as fast as possible (FAP) after being triggered. Along animal trails, cameras were placed 20 cm above the ground and left active for 24 hours to capture images of all mammalian species encountered. Each grid contained one camera trap site with an average distance of 1.2 to 1.5 km between each trap. Then, we calculated Global Moran’s I (Moran, 1950) using the Spatial Autocorrelation tool in ArcGIS (version 10.3) to determine whether the spatially rarified occurrence data assumed the random distribution after applying spatial filtering (version 10.3). Each camera was installed for 50 days. Thus, there were a total of 2,900 trap nights. Trap sites were placed throughout the study area.
Visual encounters or sightings are rare in the study area due to many species’ elusive and nocturnal nature. In addition, several species found in the study area cannot be studied using standard photographic images for capture-recapture estimation techniques as individuals lack unique identifiable features. In the monsoon season, bridges often get washed away, and frequent landslides impede field research. Droppings, scats, and pellets are often degraded due to incessant rain in the monsoon. Thus, there is limited scope for conventional research methods in this landscape to monitor the abundance of mammals.
During this research, we could not inspect some camera units during the winter and pre-monsoon periods, as the access routes were inaccessible after heavy snowfall and rainfall. Extensive fieldwork could not be conducted in the northern part of Mehao WLS as the area remained inaccessible.
3.1 Relative detection rate
The relative detection rate (RDR) was obtained by dividing the total number of independent photographs for each species by the total number of trap nights and multiplying by 100 (Carbone et al., 2001). Multiple photographs of single individuals within 30 minutes were recorded as one effective photograph (O’Brien et al., 2003).
3.2 Species accumulation curve
The species accumulation curve of mammals in Mehao WLS was calculated using rarefaction. It estimates the species richness or the assemblage for a particular level of ‘camera effort’ (Magurran, 2021). After repeated re-sampling, it is estimated by calculating the mean of all pooled individuals or samples (Gotelli and Colwell, 2001). Thus, the sample-based rarefaction curves can account for natural sampling heterogeneity in data. The rarefaction curve of the mammalian species in Mehao WLS was calculated using the “vegan” package of R software 3.4.0.
3.3 Occupancy modeling
We documented the occupancy and relative detection rate of mammals in the sanctuary through a camera trap survey. Since estimating the abundance or density of many species is difficult, Relative detection rate (RDR) is the primary measure of species diversity. Occupancy can be determined by the presence/absence of a species at camera trap sites during a sampling session. For each camera location, we created a mammalian species detection history (1100100), with ‘1’ indicating species detection during the sampling event and ‘0’ indicating non-detection (Otis et al., 1978). In some instances, certain individuals were recorded multiple times at a camera station within a short time frame (≤30 minutes). To prevent counting these repeated observations as multiple detections and thereby avoiding the issue of pseudoreplication, we treated the initial capture of the animal within that time frame as a distinct and independent record or detection, following the guidance provided (O’Brien et al., 2003). Site occupancy is defined as the proportion of an area or sites occupied by a species (MacKenzie et al., 2018). Naive occupancy is the ratio of sites where it is detected compared to the total number of sites surveyed without accounting for imperfect detection (MacKenzie et al., 2018). The detection probability is detecting a species through repeated site surveys, which permits an unbiased estimation of site occupancy (MacKenzie et al., 2018).
The main advantage of occupancy models is that they explicitly account for imperfect detection or the probability of missing a species present in the area. In wildlife research, especially ones using camera trap surveys, imperfect detection is unavoidable. A failure to account for imperfect detection can substantially affect model results and bias inferences (Gu and Swihart, 2004; MacKenzie, 2005; Sollmann et al., 2013).
We calculated each camera site’s independent photographic detection rate for Mithun (Bos frontalis) and humans per 100 trap nights. For large-scale monitoring of several species, site occupancy gives an impartial measure of species status and is cost-effective (Sarmento et al., 2011; Kinnaird and O’Brien, 2012). The camera trapping period was limited to 50 days to reduce the probability of changes in occupancy. The single-season occupancy model (MacKenzie et al., 2018) was used to predict the likelihood of a species occupying a specified site and the detection probability (p). However, it identifies ecological and anthropological factors underlying mammal distributions.
3.4 Covariates
To improve model convergence, we z-standardized all continuous covariates using the formula z = (x-x’)/SD, where x is the individual value, x’ is the factor mean, and SD is the standard deviation (Sunarto et al., 2012). Correlations between independent variables were calculated to eliminate multicollinearity issues (Graham, 2003). First, a Variance Inflation Factor (VIF) was calculated for each covariate to assess multicollinearity among variables; those with a VIF<3 were included in the model. Then, Pearson correlation tests were conducted, and correlated variables (Pearson correlation coefficient>0.70) were excluded from the model (Supplementary Figure 1). Using the “camtrapR package”, we created species-specific detection matrices for several mammal species (version 1.2.3) (Niedballa et al., 2016). We used the “unmarked package” to model occupancy (version 0.12-2) (Fiske et al., 2017).
We investigated several natural and anthropogenic covariates that could influence the space utilization of mammals in the study area (Table 1). Based on previous research (Hebblewhite et al., 2014; Li et al., 2017; Wang et al., 2018; Xiao et al., 2018; Yang et al., 2019), we investigated variables such as vegetation, ruggedness, elevation, Mithun encounter rates, and distance to a water body, as well as settlements and roads, which may influence species occupancy. We calculated the distance (m) from each camera to settlements, roads, and rivers using ArcToolbox in ArcGIS 10.3 (ESRI Inc.).
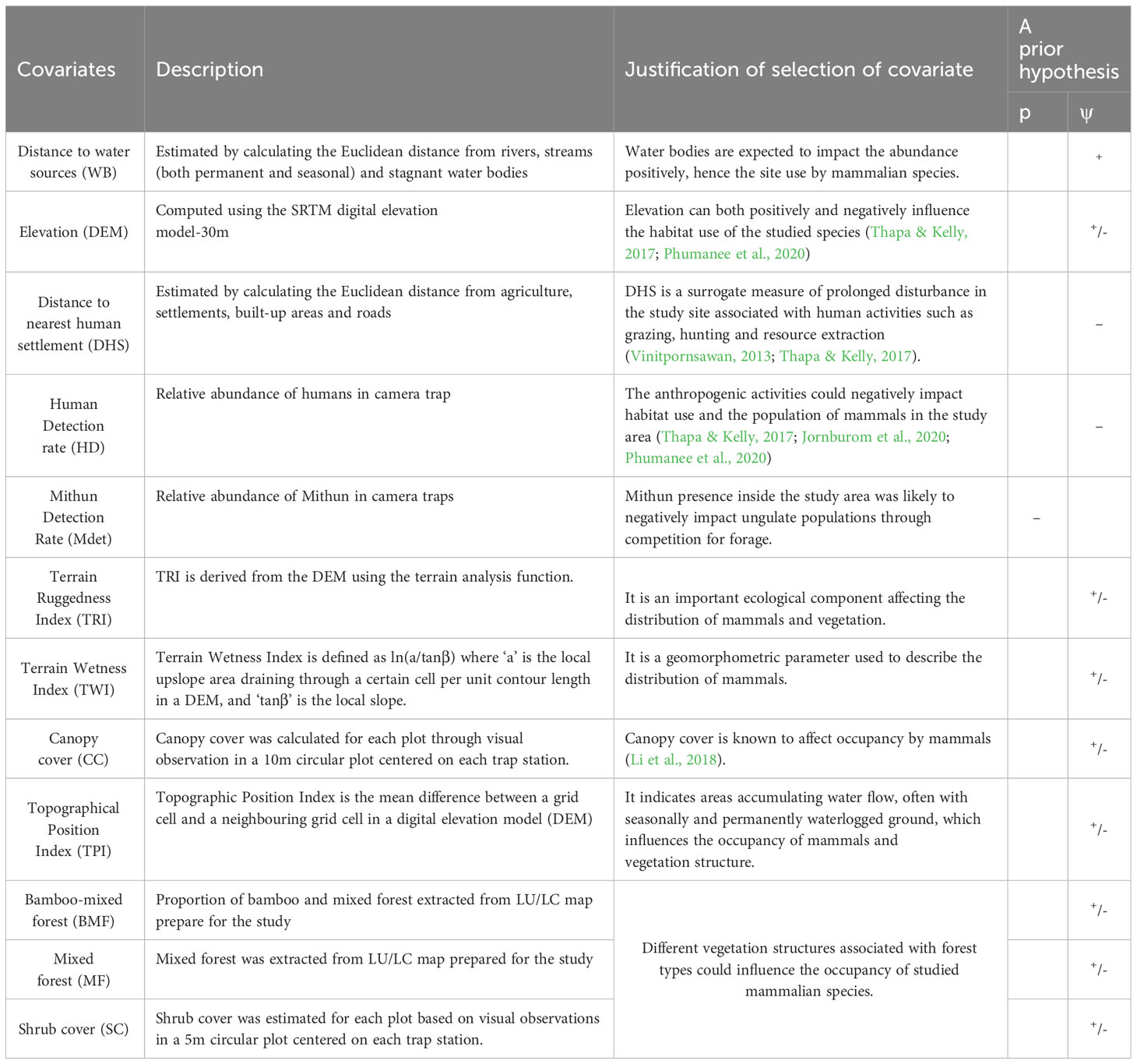
Table 1 Factors hypothesized to influence patterns of occupancy (ψ) and detection probability in Mehao WLS.
We used portable GPS receivers to determine the elevation of each location. We determined ruggedness using a one-kilometre radius and a topographic position index derived from the Shuttle Radar Topography Mission (SRTM) 30-m digital elevation model. Mithun and human presence are assumed to influence the animal’s detection rate and 30 minutes of independence for each record. Mithun and human encounter rates for each camera station were calculated as the number of detections per 100 camera trap days over the entire sampling period (O’Brien et al., 2003). We created a 10 sq m circular plot around each camera location and recorded covariates such as elevation, habitat type, forest type, and tree/vegetation cover percentage. The habitat characteristics for each camera location were also assessed (including ground cover, shrub cover, and canopy cover visual estimation) (Kushwaha et al., 2004; Ramesh et al., 2013).
4 Result
4.1 Relative detection rate, sampling effort and trap nights
We carried out 50 camera-trapping days at 58 sites (n=2,900 trap nights) and recorded 27 wild mammals, Mithun (semi-domesticated) species, and humans. We focused our on-site occupancy analyses on 10 of the 27 species for which we had sufficient data. However, we analyzed the detection rate for all terrestrial mammals.
The 27 species of mammals recorded in the camera trap survey included 12 carnivore species (four Felids, three Viverrids, two Mustelidae, two Canids, and one species each from the Ursidae and Prionodontidae families) and seven herbivore species (four Bovidae, two Cervidae, and one Suidae) species. In the carnivore guild, we recorded Indo-Chinese clouded leopard (Neofelis nebulosa), marbled cat (Pardofelis marmorata), leopard cat (Prionailurus bengalensis), Asiatic golden cat (Catopuma temminckii), masked palm civet (Paguma larvata), common palm civet (Paradoxurus hermaphroditus), large Indian civet (Viverra zibetha), wild dog (Cuon alpinus), spotted linsang (Prionodon pardicolor), Asiatic black bear (Ursus thibetanus), golden jackal (Canis aureus), and yellow-throated marten (Martes flavigula). In the herbivore guild, we recorded Mishmi takin (Budorcas taxicolor), red goral (Naemorhedus baileyi), mainland serow (Capricornis sumatraensis), mithun (Bos frontalis), Indian muntjac (Muntiacus vaginalis), sambar (Rusa unicolor), and Indian wild pig (Sus scrofa). We also recorded seven other species (two Hystricidae, three Sicuridae and a species each from Hylobatidae, Herpestidae and Cercopithecidae). This included Malayan porcupine (Hystrix brachyura), Asiatic brush-tailed porcupine (Atherurus macrourus), black giant squirrel (Ratufa bicolor), Himalayan striped squirrel (Tamiops macclellandi), Pallas’s squirrel (Callosciurus erythraeus), crab-eating mongoose (Herpestes urva), Western hoolock gibbon (Hoolock hoolock), and northern pig-tailed macaque (Macaca leonina). Through sign surveys, direct sightings, questionnaire surveys, and camera trappings, we identified 27 species present in Mehao WLS. Twelve of the 27 species recorded are of high global conservation importance in the IUCN Red List, and the conservation status of species is listed as Endangered (2), Vulnerable (9) and Near Threatened (3) Least Concern (11) (IUCN 2010) (Supplementary Table 1).
The Relative detection rate was calculated for Asiatic brush-tailed porcupine (RDR=4 ± 3.6 SE), followed by Mainland serow (RDR=3.21 ± 0.77 SE), northern red muntjac (RDR=3.06 ± 0.97 SE), Mishmi takin (RDR=1.34 ± 1.25 SE), mithun (RDR=1.28 ± 0.51 SE), Indian wild pig (RDR=0.70 ± 0.36 SE), yellow-throated marten (RDR=0.56 ± 0.16 SE), masked palm civet (RDR=0.47 ± 0.18 SE), Himalayan black bear (RDR=0.24 ± 0.11 SE), Asian golden cat (RDR=0.17 ± 0.09 SE) and leopard cat (RDR=0.17 ± 0.08 SE). According to RDR estimates, the most abundant mammalian species was the Asiatic brush-tailed porcupine (Figure 2). Yellow-throated marten was the most abundant carnivore. According to RDR value, yellow-throated marten, masked palm civet, large Indian civet, leopard cat, marbled cat, Asiatic Golden Cat, clouded leopard, wild dog, and Asiatic black bear are the major predators in Mehao WLS. Figure 3 represents the mainland serow, Northern red muntjac and Yellow-throated marten distributions across the study area. Asiatic brush-tailed porcupine was present in the southern part of the sanctuary.
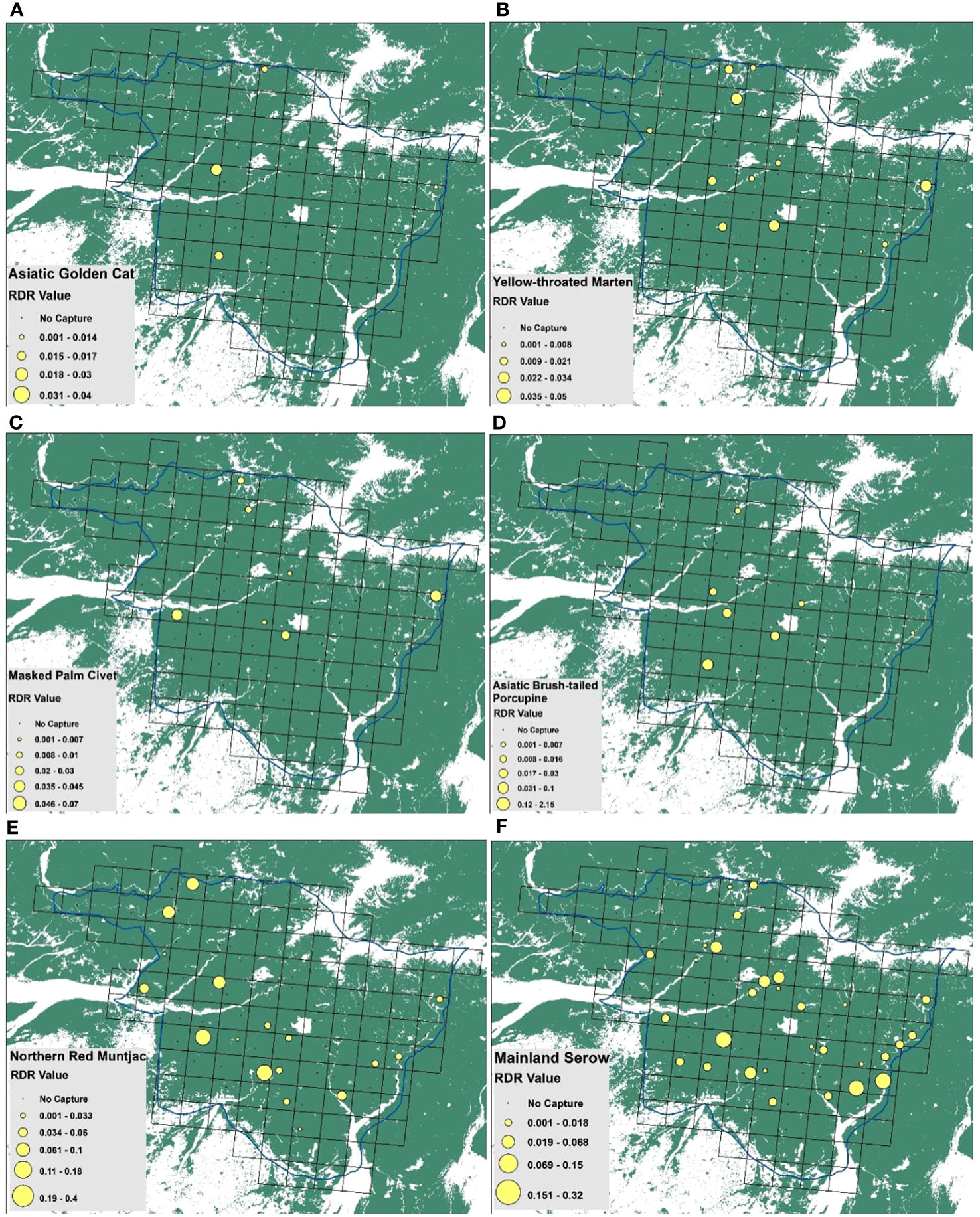
Figure 3 Spatial distribution maps of (A) Asian golden cat, (B) yellow-throated marten, (C) masked palm civet, (D) Asiatic brush-tailed porcupine, (E) northern red muntjac and (F) mainland serow in Mehao WLS.
4.2 Species accumulation curve
The Species Accumulation Curve shows that species richness for camera traps in Mehao WLS has not reached an asymptote after 80 trap nights (Figure 4). This insight highlights the need for additional survey efforts to obtain a more comprehensive understanding of the species present in the area.
4.3 Occupancy
Table 2 lists the site occupancy and detection probability of selected mammalian species. Naive occupancy was highest for mainland serow (0.48) followed by northern red muntjac (0.28), Asiatic brush-tailed porcupine (0.25), Asiatic golden cat (0.25), yellow-throated marten (0.21), masked palm civet (0.19), and lowest for Asiatic black bear (0.09), leopard cat (0.07), and Mishmi takin (0.05).
4.4 Estimates of occupancy
Estimates of site occupancy for all species with standard errors ranged from 0.07 ± 0.04 to 0.58 ± 0.08. Mainland serow has the highest probability of occupancy [ψ(.)SE ψ(.): 0.58 ± 0.08] followed by northern red muntjac [ψ(.) SE ψ(.): 0.30 ± 0.06], brush-tailed porcupine [ψ(.) SE ψ(.): 0.26 ± 0.06], Asian golden cat [ψ(.) SE ψ(.): 0.26 ± 0.06], yellow-throated marten [ψ(.)SE ψ(.): 0.25 ± 0.07], masked palm civet [ψ(.) SE ψ(.): 0.21 ± 0.09], and leopard cat [ψ(.) SE ψ(.): 0.184 ± 0.161], Mishmi takin [ψ(.) SE ψ(.): 0.07 ± 0.04], Asiatic black bear [ψ(.) SE ψ(.): 0.15 ± 0.08] and wild boar [ψ(.) SE ψ(.): 0.14 ± 0.08] have the lowest occupancy probability (Table 2). The estimated occupancy probability for all these species is larger than the naïve estimates.
4.5 Detection probability
The estimates for the probability of detection with standard error range from 0.03 ± 0.02 to 0.24 ± 0.03. The probability of detection was highest for brush-tailed porcupine (ρ = 0.24 ± 0.03), followed by northern red muntjac (ρ = 0.18 ± 0.02), mainland serow (ρ = 0.11 ± 0.02), masked palm civet (ρ = 0.17 ± 0.03), Asian golden cat (ρ = 0.11 ± 0.01), yellow-throated marten (ρ = 0.11 ± 0.02), and Mishmi takin (ρ =0.10 ± 0.04). The leopard cat, wild boar, and Asiatic black bear have the lowest (< 0.1) probability of detection. The results of the top four models are summarized here, while a more comprehensive overview of the model selection process can be found in Table 3 of the Supplementary Materials (Supplementary Table 2). In Table 3, the site occupancy models for species such as the Asian golden cat and the masked palm civet, we used a constant detection p(.) probability. In the case of northern red muntjac, the presence of mithun had a positive impact on detection (Mdet, ρ = 0.25 ± 0.09) (Table 4). Figure 5 summarizes the influence of mithun on the northern red muntjac detection probability.
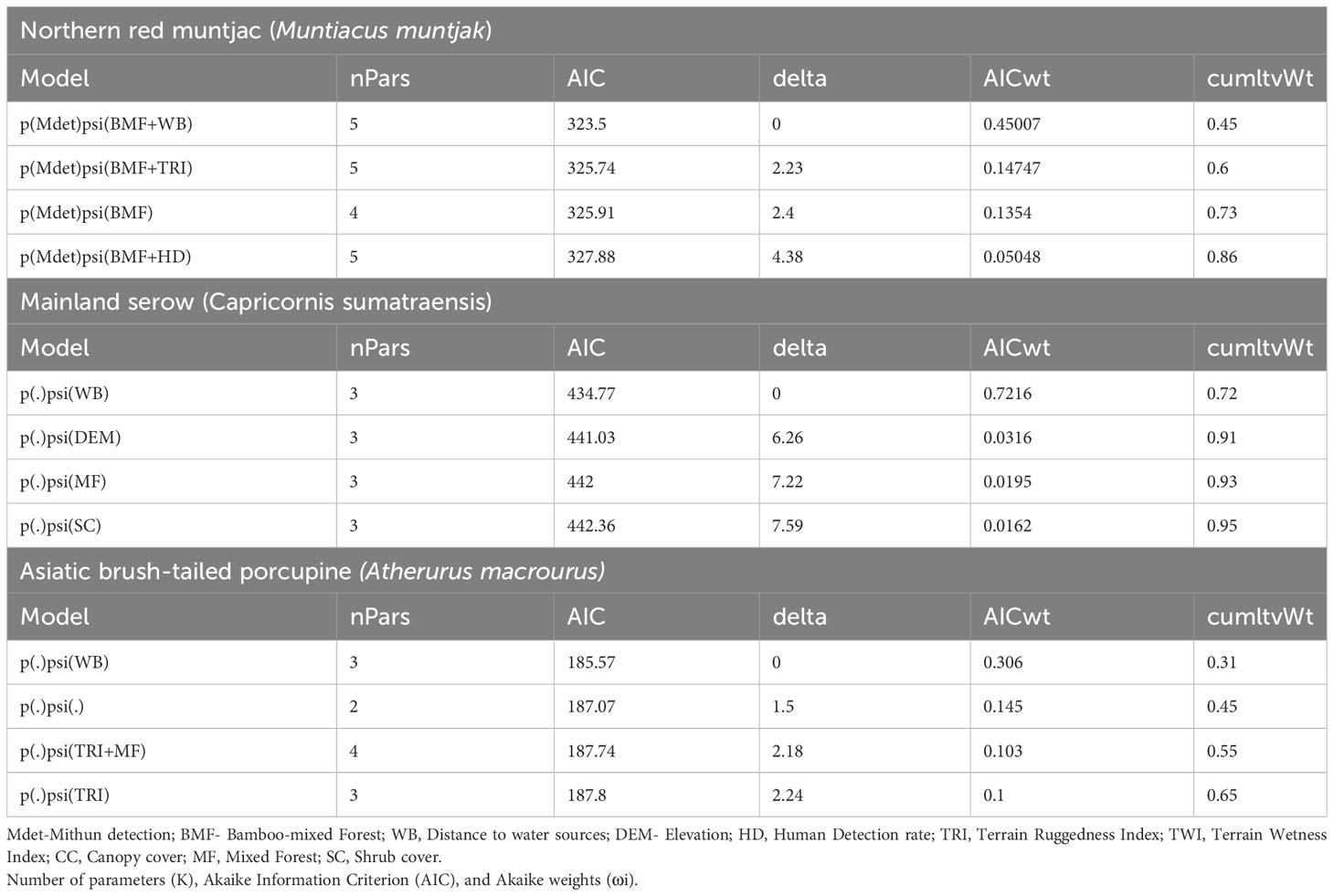
Table 3 Results of model selection to determine ecological and anthropogenic covariates that influence the probability of habitat use by mammals in Mehao Wildlife Sanctuary, Arunachal Pradesh.
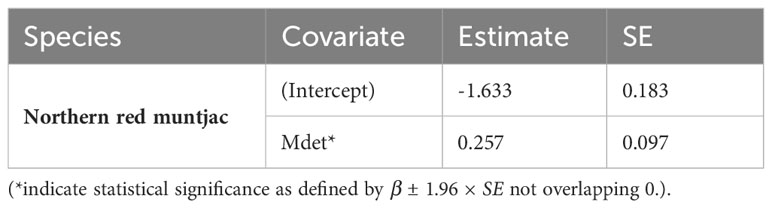
Table 4 Estimates of β coefficient values (standard errors, SE) of northern red muntjac detection probability for different covariates based on the models Δ AIC < 2.
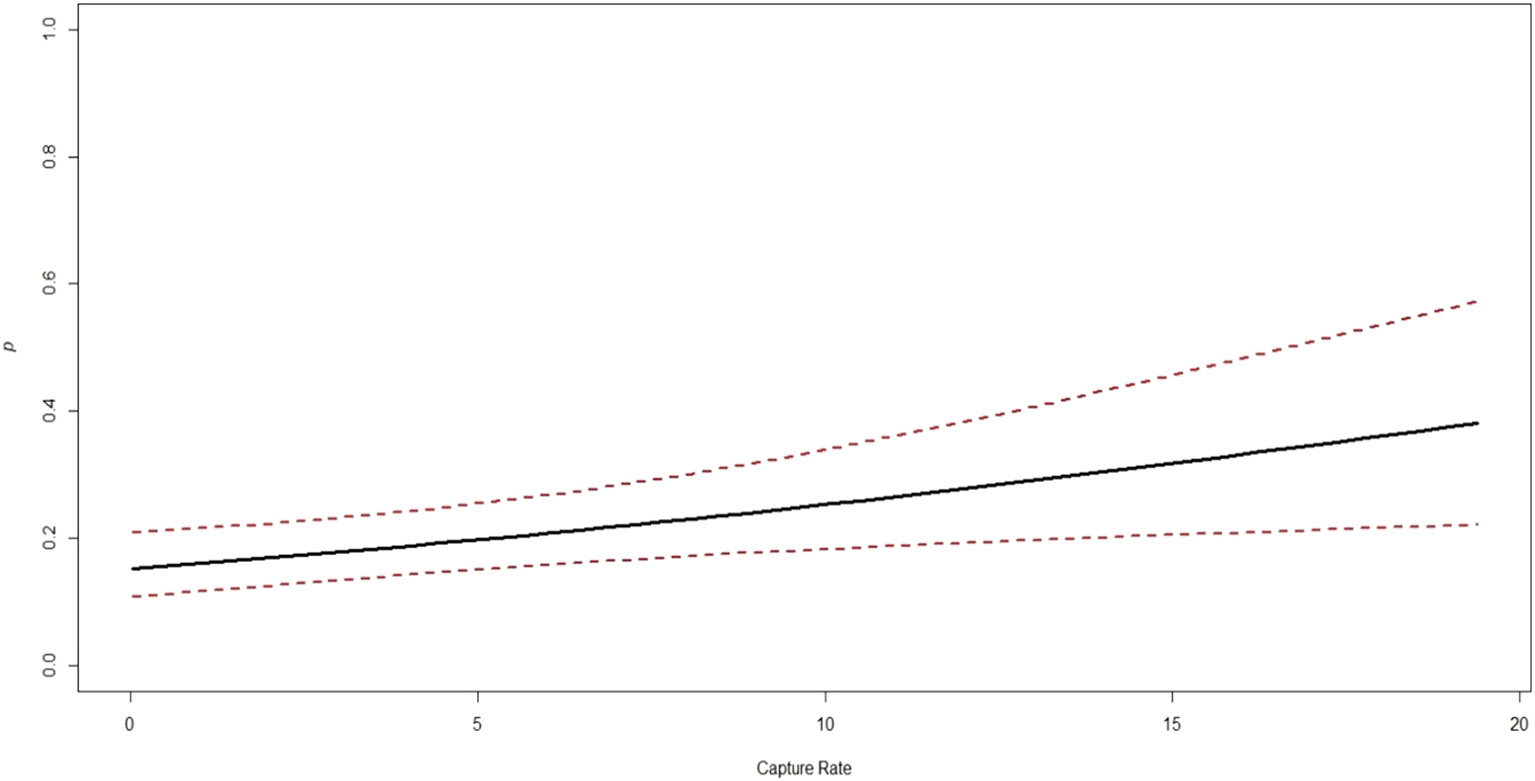
Figure 5 Association between the highly influential covariates (based on estimates of regression parameters (β) and 95% CI from the best models) and the probability of northern red muntjac occupancy in Mehao wildlife sanctuary, Arunachal Pradesh.
4.6 Influence of covariates on occupancy probability
The site use probability was modeled using an information-theoretic approach and the detection probability model that best fit the data. Table 5 summarizes the best predictive models for species occupancy, including a complete set of models and regression coefficient estimates for each model. Figure 6 provides an overview of the influence of covariates on the studied species’ occupancy probability. The best model for northern red muntjac revealed that bamboo-mixed forest (BMF) (βestimate: 1.08 ± 0.48) and distance from waterbody (WB) (βestimate: 0.65 ± 0.35) had a positive influence on occurrence probability. The strongest ecological correlate for mainland serow was a negative response to distance from WB (βestimate: -1.85 ± 0.78). The Asiatic brush-tailed porcupine is their best model with 31% AIC weight. The response to distance from a WB was the strongest ecological correlation (βestimate: 0.6 ± 0.32).
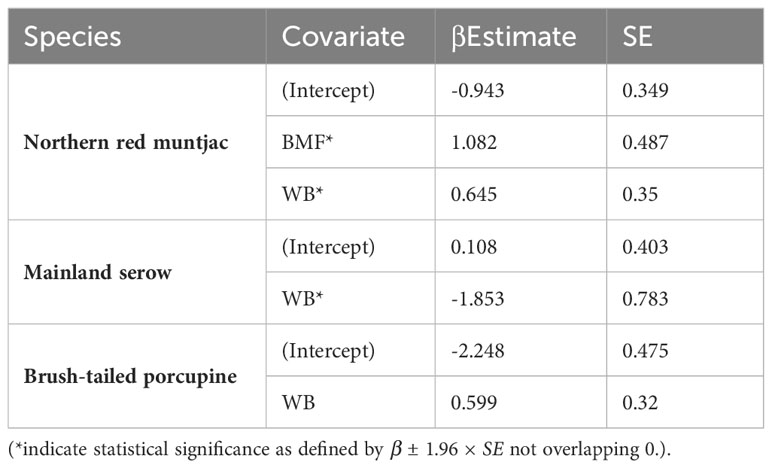
Table 5 Estimates of β coefficient values (standard errors, SE) of mammals’ site-use probability for different individual covariates based on the models Δ AIC < 2.
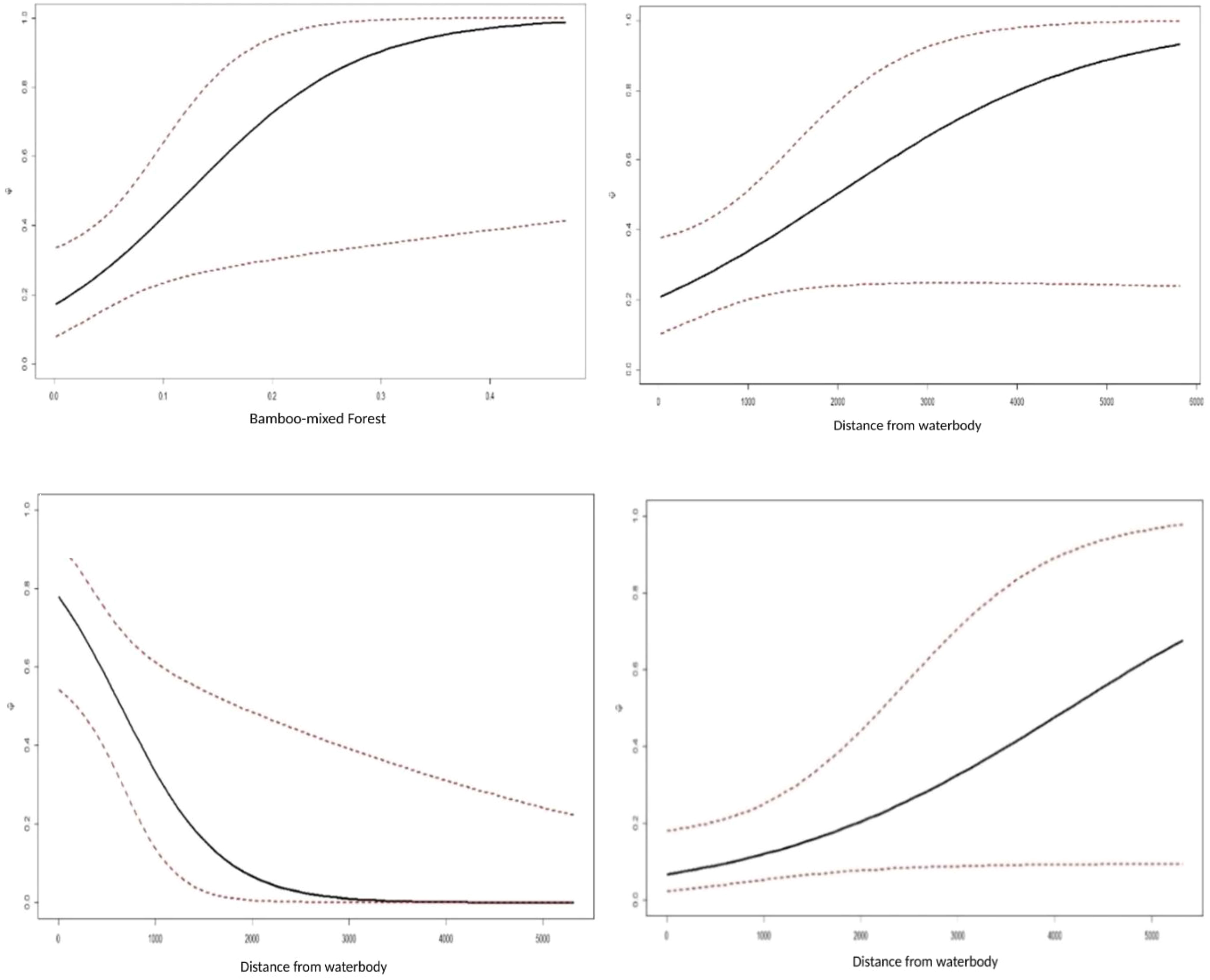
Figure 6 Association between probability and highly influential covariates (based on estimates of regression parameters (β) and 95% CI from the best models of northern red muntjac (top left, top right), mainland serow (bottom left), and Asiatic brush-tailed porcupine (bottom right) occupancy in Mehao Wildlife Sanctuary, Arunachal Pradesh.
5 Discussion
This study documented several rare and elusive species in Mehao WLS (Supplementary Figure 2). During the camera trap survey and direct sightings, 27 species of mammals were recorded. However, our analysis of six distinct mammalian species revealed novel information with regard to the conservation value of the study area. We documented that Mehao WLS supports rare and threatened species such as clouded leopard, spotted linsang, marbled cat, hoolock gibbon, sambar, mainland serow, etc. The analysis revealed that mammalian fauna in this area responded to ecological and anthropogenic factors in various ways, but the responses were species-specific, as predicted. Species responded differently to different covariates, implying that management actions must be prioritized based on the relative importance of species in terms of conservation needs. Habitat and anthropogenic disturbance include habitat restoration and protection, invasive species control, and biodiversity conservation. For anthropogenic disturbance, mitigation efforts may focus on reducing human-wildlife conflicts, sustainable land use planning, wildlife-friendly infrastructure design, and regulatory measures to limit harmful activities. These management actions can help mitigate the negative impacts of habitat loss and anthropogenic disturbance on wildlife species and promote ecosystem conservation and sustainable management. We did not conduct sampling outside the sanctuary, but the species in this study may also be present in agricultural and human-occupied landscapes. --
Including species in ecological models with covariates relies significantly on their detection rates in camera trap data, reflecting their presence and behavior within a studied habitat. In our analysis, the Asiatic golden cat, leopard cat, Himalayan black bear, yellow-throated marten, masked palm civet, Mishmi takin, and wild boar were regrettably omitted due to their notably lower capture rates. While these species undoubtedly contribute to the region’s biodiversity, their infrequent appearance in camera trap records posed a challenge in establishing reliable relationships with the covariates under investigation. Focusing on species with higher capture rates ensures robustness in our model, allowing for a more comprehensive understanding of the ecological dynamics between covariates and the more frequently observed wildlife within this ecosystem.
Given our predictions, the detection probability of northern red muntjac showed positive significance to the presence of mithun as compared to mainland serow, as the former is more of generalist species (Paudel & Kindlmann, 2012). However, this study has observed that northern red muntjac are close to human settlement areas with gentle slopes (Paudel and Kindlmann, 2012), and mithun is a semi-domesticated bovid species that prefers to live near human settlements. Our occupancy survey revealed that northern red muntjac primarily choose dense forests with low-growing vegetation, such as bamboo-mixed forest for foraging and rest (Teng et al., 2004; Odden and Wegge, 2007).
Mainland serow showed a significant association with water bodies. Due to the absence of dense understory vegetation on steep slopes, the serow is primarily found in the mountain’s deeply dissected rocky gorges and ravines, which also provide shelter (Wu and Zhang, 2004). There are steep hills and deep valleys with multiple rocky streams and some perennial rivers surrounded by dense forests and patches of grass. This provides a suitable habitat for serow, which feeds on vegetation growing near water bodies and provides cover from predators such as black bears and humans. Serows also use dense forests near water habitats to maintain their body temperature (Aryal, 2009).
The Asiatic brush-tailed porcupine is a lesser-known rodent species in northeast India (Talukdar et al., 2019; Molur, 2020). This is the first record of this species from Mehao WLS. They prefer the plain area with a gentle slope in the dense forest of Mehao WLS. They have moderate temperature tolerance, and the dense forest near waterbodies provides cover and moisture to maintain their body temperature. The species was recorded from other forests in Arunachal Pradesh, including Namdapha NP, Pakke WLS, and Changlang district (Agarwal, 2000; Datta et al., 2008a, Datta et al., 2008b).
However, the Asiatic golden cat is one the least studied species in tropical Asia (Yongdrup et al., 2019), and it is thought to be rare due to limited sightings (Grassman et al., 2005). It is a solitary hunter and forest-dependent species whose range is threatened by significant habitat loss and fragmentation (McCarthy et al., 2015). Over time, land use has changed forest cover, and human pressure on natural resources has increased at lower elevations. The species has also been recorded in the high-altitude ranges from India to Bhutan (Bashir et al., 2011; Jigme, 2011; Dhendup, 2016). During the survey, we captured four different morphs of the Asiatic golden cat in Mehao WLS.
We found that yellow-throated marten prefers elevated areas. Forest habitat quality is correlated with elevation, especially since there is relatively less anthropogenic disturbance at higher altitudes, as urban areas, agricultural fields, and orchards are generally found at lower altitudes (Lee et al., 2021). The presence of yellow-throated martens decreased in areas with rugged terrain because they tend to avoid shrub-covered regions, given their preference for an arboreal lifestyle (Duckworth, 1997; Appel and Khatiwada, 2014). The masked palm civet prefers forest habitats in lowland areas with ample amounts of water, where they feed on berries, seeds, molluscs, small mammals, amphibians, insects, and arthropods (Zhou et al., 2008; Matsuo and Ochiai, 2009; Belden et al., 2014). Though there is anthropogenic pressure, this species still survives in lowland areas as it has a degree of tolerance to habitat alteration (Zhou et al., 2008; Semiadi et al., 2016).
The Idu Mishmi community has intricate animistic beliefs linked to the forests and the landscape, which ensures prudent resource management. The practice of social taboos by local people can be harnessed by conservation projects so that local people appreciate and willingly engage in community-based conservation projects. Current scientific literature reveals that habitat fragmentation and loss of habitat due to anthropogenic pressure (like expansion of agricultural land, built-up area, and construction of highways) leads to local threats to these species. Such anthropogenic pressures may also affect species distribution and abundance in the landscape (Aiyadurai et al., 2010).
6 Conservation implications
Mehao WLS is an important landscape for mammalian species due to its diverse topography, vegetation, and climatic conditions. Our study provides quantitative insights for multi-species conservation decision-making as it accounts for significant ecological variables. The modeling of multi-species occupancy provided data to predict mammalian responses to land-use changes. These insights are important for decision-making by forest officials. This would benefit various mammalian species and help formulate an informed land-use policy.
This study also provides crucial ecological data for various mammalian species. It exemplifies a simple method to estimate the distribution and habitat occupancy of rare and elusive mammals in Mehao WLS. Due to the varied nature of species targeted in our study, we recognize that the data available for analysis may differ significantly. This disparity can introduce limitations to the comprehensiveness of our study, as it may not provide sufficient insights into the behaviours and patterns of certain species. To overcome this limitation, it is essential to conduct additional sampling efforts across multiple seasons. This approach is necessary to ensure a more comprehensive and robust analysis, particularly when surveying rare and elusive species. However, microhabitats are essential for managing and conserving mammalian species. Such data is crucial for designing and implementing species-specific conservation initiatives and effectively managing protected areas. To date, there has been little supporting information on mammalian species in Mehao WLS (Ahmad et al., 2023), and it is important to carry out a long-term study on mammals in the landscape (Katti et al., 1990).
The effectiveness of patrolling along with other management and conservation interventions will be effective with continued monitoring that can reveal the presence of various species, identify threats, and monitor the prevalence of invasive species. The cameras deployed in this study remained operational throughout the day, and the data indicates that the arboreal nature of certain species may limit the number of photographic records. Despite this possible limitation, it is important to initiate conservation efforts for various species according to available data, which indicates that the species occur in small numbers and require greater protection. Data analysis of mammalian species throughout their range is critical for effectively and efficiently conserving these species in a continuously changing landscape.
Data availability statement
The original contributions presented in the study are included in the article/Supplementary Material. Further inquiries can be directed to the corresponding author.
Ethics statement
The animal study was approved by Wildlife Institute of India, Dehradun. The study was conducted in accordance with the local legislation and institutional requirements.
Author contributions
AA carried out field work, analyzed the data, and led the writing of the manuscript. GG conceived ideas and designed methodology. Both authors contributed to the article and approved the submitted version.
Funding
The author(s) declare financial support was received for the research, authorship, and/or publication of this article. Funding support was received from Department of Science and Technology (DST), Government of India.
Acknowledgments
The authors thank the Director and Dean of Wildlife Institute of India, Dehradun, for institutional support, the Forest Department of Arunachal Pradesh for providing the necessary field permission and the Department of Science and Technology. We also thank Mr. Kenjum Rina (DFO-Roing) and Mr. Ipra Mekola (Member of Arunachal Pradesh State Board of Wildlife) for their kind support in facilitating our fieldwork. We thank our field assistants, Mr Kiran Pulu, Mr Ashok Sherpa, and Mr Rajan Lingi, for their hard work and rigorous efforts during field data collection. In addition, the first author thanks Dr. Anukul Nath for comments and suggestions on an earlier manuscript draft.
Conflict of interest
The authors declare that the research was conducted in the absence of any commercial or financial relationships that could be construed as a potential conflict of interest.
Publisher’s note
All claims expressed in this article are solely those of the authors and do not necessarily represent those of their affiliated organizations, or those of the publisher, the editors and the reviewers. Any product that may be evaluated in this article, or claim that may be made by its manufacturer, is not guaranteed or endorsed by the publisher.
Supplementary material
The Supplementary Material for this article can be found online at: https://www.frontiersin.org/articles/10.3389/fevo.2023.1106329/full#supplementary-material
References
Abood S. A., Lee J. S. H., Burivalova Z., Garcia Ulloa J., Koh L. P. (2015). Relative contributions of the logging, fiber, oil palm, and mining industries to forest loss in Indonesia. Conserv. Lett. 8 (1), 58–67. doi: 10.1111/conl.12103
Adhikarimayum A., Gopi. G. V. (2018). First photographic record of tiger presence at higher elevations of the Mishmi Hills in the Eastern Himalayan Biodiversity Hotspot, Arunachal Pradesh, India. J. Threatened Taxa. 12833-12836. 10.11609/jott. 10, 12833–12836. doi: 10.11609/jott.4381.10.13.12833-12836
Agarwal V. C. (2000). Taxonomic studies of Indian muridae and hystricidae mammalia: rodentia). Records Zoological Survey India Occassional Paper 180, 177. https://cir.nii.ac.jp/crid/1130000797432467840
Ahmad A., Kanagaraj R., Gopi G. V. (2023). Wildlife habitat mapping using Sentinel-2 imagery of Mehao Wildlife Sanctuary, Arunachal Pradesh, India. Heliyon 9 (3). doi: 10.1016/j.heliyon.2023.e13799
Ahmad A., Kumar A., Rawat G. S., Gopi G. V. (2020). Recent record of a threatened holoparasitic plant Sapria himalayana Griff. in Mehao Wildlife Sanctuary, Arunachal Pradesh, India. J. Threat. Taxa 12 (10):16399–401. doi: 10.11609/jot.5168.12.10.16399-16401
Ahumada J. A., Silva C. E., Gajapersad K., Hallam C., Hurtado J., Martin E., et al. (2011). Community structure and diversity of tropical forest mammals: data from a global camera trap network. Philos. Trans. R. Soc. B: Biol. Sci. 366 (1578), 2703–2711. doi: 10.1098/rstb.2011.0115
Aiyadurai A., Singh N., Milner-Gulland E. (2010). Wildlife hunting by indigenous tribes: A case study from Arunachal Pradesh, north-east India. Oryx 44 (4), 564–572. doi: 10.1017/S0030605309990937
Almeida-Rocha J. M., Peres C. A., Oliveira L. C. (2017). Primate responses to anthropogenic habitat disturbance: a pantropical meta-analysis. Biol. Conserv. 215, 30–38. doi: 10.1111/mec.15688
Appel A., Khatiwada A. P. (2014). Yellow-throated martens martes flavigula in the kanchenjunga conservation area, Nepal. Small Carnivore Conserv. 50, 14–19.
Aryal A. (2009). Habitat ecology of Himalayan serow (Capricornis sumatraensis ssp. thar) in Annapurna Conservation Area of Nepal. Tiger paper 34 (4), 12–20. https://www.fao.org/3/ak851e/ak851e00.pdf#page=14.
Athreya R. M., Captain A. S., Athreya V. R. (1997). A faunal survey of Namdapha Tiger Reserve, Arunachal Pradesh, India. Unpublished Rep.
Athreya V. R., Johnsingh A. J. T. (1995). Survey of the clouded leopard (Neofelis nebulosa) in North-east India (Dehradun, India: Unpublished report, Wildlife Institute of India).
Bali A., Kumar A., Krishnaswamy J. (2007). The mammalian communities in coffee plantations around a protected area in the Western Ghats, India. Biol. Conserv. 139, 93–102. doi: 10.1016/j.biocon.2007.06.017
Barlow J., Lennox G. D., Ferreira J., Berenguer E., Lees A. C., Mac, Nally R., et al. (2016). Anthropogenic disturbance in tropical forests can double biodiversity loss from deforestation. Nature 535, 144. doi: 10.1038/nature18326
Bashir T., Bhattacharya T., Poudyal K., Sathyakumar S. (2011). Notable observations on the melanistic Asiatic Golden cat (Pardofelis temminckii) of Sikkim, India. NeBIO 2 (1), 1–4.
Bradshaw C. J., Sodhi N. S., Brook B. W. (2009). Tropical turmoil: a biodiversity tragedy in progress. Front. Ecol. Environ. 7 (2), 79–87. doi: 10.1890/070193
Belden G., Jukie A., Megom N., Unggang J. (2014). Lowland records of masked palm civet Paguma larvata from Sarawak, Malaysian Borneo. Small Carnivore Conserv. 51, 56–58.
Benítez-López A., Alkemade R., Schipper A. M., Ingram D. J., Verweij P. A., Eikelboom J. A. J., et al. (2017). The impact of hunting on tropical mammal and bird populations. Science 356 (6334), 180–183.
Boron V., Deere N. J., Xofis P., Link A., Quiñones-Guerrero A., Payan E., et al. (2019). Richness, diversity, and factors influencing occupancy of mammal communities across human-modified landscapes in Colombia. Biol. Conserv. 232, 108–116. doi: 10.1016/j.biocon.2019.01.030
Brodie J. F., Giordano A. J., Ambu L. (2015). Differential responses of large mammals to logging and edge effects. Mamm. Biol. 80, 7–13. doi: 10.1016/j.mambio.2014.06.001
Brodie J. F., Helmy O. E., Brockelman W. Y., Maron J. L. (2009). Bushmeat poaching reduces the seed dispersal and population growth rate of a mammal-dispersed tree. Ecol. Appl. 19 (4), 854–863. doi: 10.1890/08-0955.1
Carbone C., Christie S., Conforti K., Coulson T., Franklin N., Ginsberg J. R., et al. (2001). The use of photographic rates to estimate densities of tigers and other cryptic mammals. Anim. Conserv. 4, 75–79. doi: 10.1017/S1367943001001081
Carrillo E., Wong G., Cuaro´n A. D. (2000). Monitoring mammal population in Costa Rican protected areas under different hunting restrictions. Conserv. Biol. 24, 1580–1591. doi: 10.1111/j.1523-1739.2000.99103.x
CBD (2015). Report of the Ad Hoc Technical Expert Group on Indicators for the strategic Plan for Biodiversity 2011–2020 (Montreal, Canada: UNEP). Available at: http://www.cbd.int/doc/meetings/ind/id-ahteg-2015-01/official/id-ahteg-2015-01-03-en.pdf.
Ceballos G., Ehrlich P. R., Soberón J., Salazar I., Fay J. P. (2005). Global mammal conservation: what must we manage? Science 309 (5734), 603–607. doi: 10.1126/science.1114015
Chakraborty S., Sen A. K. (1991). Mammals of the Mehao Wildlife Sanctuary (Dibang Valley District, Arunachal Pradesh) with remarks on their status. Zoological Survey India 88 (3and4) 263-, 285. doi: 10.26515/rzsi/v88/i3-4/1991/161022
Champion H. G., Seth S. K. (1968). A revised survey of the forest types of India. (Manager of publications).
Chetry D., Chetry R., Ghosh K., Singh A. K. (2010). Status and distribution of the eastern hoolock gibbon (Hoolock leuconedys) in Mehao Wildlife Sanctuary, Arunachal Pradesh, India. Primate Conserv. 2010 (25), 87–94. doi: 10.1896/052.025.0113
Choudhury A. (1997). The status of the Sumatran rhinoceros in north-eastern India. Oryx 31 (2), 151–152. doi: 10.1046/j.1365-3008.1997.d01-9.x
Dasgupta S., Choudhury P., Ashraf N. V. K., Bhattacharjee P. C., Kyarong S. (2015). Food preference of rehabilitated Asiatic black bear cubs in lowland tropical forests of northeast India. Asian J. Conserv. Biol. 4 (1), 20–25. https://www.ajcb.in/journals/full_papers_july_2015/AJCB-Vol4-No1-Dasgupta%20et%20al.pdf
Dasgupta S., Choudhury P., Bhattacharjee B. C. (2014). Activity pattern of the orphaned Asiatic Black Bear Ursus thibetanus (Mammalia: Carnivora: Ursidae) cubs during rehabilitation processes. J. Threatened Taxa 6 (10), 6371–6375. doi: JoTT.o3887.6371-5
Datta A. (1998). Evidence of clouded leopard Neofelis nebulosa in Pakhui Wildlife Sanctuary, Arunachal Pradesh. J. Bombay Natural History Soc. 95, 498–499. https://www.biodiversitylibrary.org/page/48605048#page/542/mode/1up
Datta A. (2002). Status of hornbills and hunting among indigenous Communities in Eastern Arunachal Pradesh (Bangalore, India: Unpublished Report. Wildlife Conservation Society and WCS-India Programme).
Datta A. (2007). “Protecting with people in Namdapha: threatened forests, forgotten people,” in Making Conservation Work: Securing Biodiversity in This New Century. Eds. Shahabuddin G., Rangarajan M. (New Delhi, India: Permanent Black), 165–209.
Datta A., Anand M. O., Naniwadekar R. (2008a). Empty forests: Large carnivore and prey abundance in Namdapha National Park, north-east India. Biol. Conserv. 141 (5), 1429–1435. doi: 10.1016/j.biocon.2008.02.022
Datta A., Goyal S. P. (1997). Responses of arboreal mammals to selective logging in Arunachal Pradesh (Dehradun: Final report, Wildlife Institute of India).
Datta A., Naniwadekar R., Anand M. O. (2008b). Hornbills, hoolocks and hog badgers: long-term monitoring of threatened wildlife with local communities in Arunachal Pradesh, north-east India. Final report to the Rufford Small Grants Program (UK). Nat. Conserv. Foundation Mysore India 80.
Datta A., Pansa J., MadhuSudan M. D., Mishra C. (2003). Discovery of the leaf deer Muntiacus putaoensis in Arunachal Pradesh: an addition to the large mammals of India. Curr. Sci. 84, 101–103. https://www.jstor.org/stable/24107434
De Bondi N., White J. G., Stevens M., Cooke R. (2010). A comparison of the effectiveness of camera trapping and live trapping for sampling terrestrial small-mammal communities. Wildlife Res. 37 (6), 456–465. doi: 10.1071/WR10046
Dhendup T. (2016). Status of asiatic golden cat catopuma temminckii vigors & Horsfiel(Carnivora: felidae) in Bhutan. J. Threatened Taxa 8 (4), 8698–8702. doi: 10.11609/jott.2560.8.4.8698-8702
Dirzo R., Miranda A. (1990). Contemporary Neotropical defaunation and the forest structure, function, and diversity—a sequel to John Terborgh. Conserv. Biol. 4, 444–447. doi: 10.1111/j.1523-1739.1990.tb00320.x
Duckworth J. W. (1997). Small carnivores in Laos: a status review with notes on ecology, behaviour and conservation. Small Carnivore Conserv. 16 (1), 21.
Espartosa K. D., Pinotti B. T., Pardini R. (2011). Performance of camera trapping and track counts for surveying large mammals in rainforest remnants. Biodiversity Conserv. 20, 2815. doi: 10.1007/s10531-011-0110-4
Estes J. A., Terborgh J., Brashares J. S., Power M. E., Berger J., Bond W. J., et al. (2011). Trophic downgrading of planet Earth. Science 333 (6040), 301–306. doi: 10.1126/science.1205106
Fischer J., Lindenmayer D. B. (2007). Landscape modification and habitat fragmentation: a synthesis. Global Ecol. Biogeography 16 (3), 265–280. doi: 10.1111/j.1466-8238.2007.00287.x
Fiske I., Chandler R., Miller D., Royle A., Kéry M., Hostetler J., et al. (2017). Package ‘unmarked’: models for data from unmarked animals. Version 0.12-2.
Gopi G. V., Habib B., Selvan K. M., Lyngdoh S. (2012). Conservation of the endangered Asiatic wild dog (Cuon alpinus) in Western Arunachal Pradesh: linking ecology, ethics and economics to foster better coexistence (Dehradun: Wildlife Institute of India), 137.
Gopi G. V., Lyngdoh S., Selvan K. M. (2010). Conserving the endangered Asiatic wild dog Cuon alpinus in western Arunachal Pradesh: fostering better coexistence for conservation (London, U.K: Final Technical Report to Rufford Small Grant Programme).
Gotelli N. J., Colwell R. K. (2001). Quantifying biodiversity: procedures and pitfalls in the measurement and comparison of species richness. Ecol. letters. 4, 379–391. doi: 10.1046/j.1461-0248.2001.00230.x
Graham M. H. (2003). Confronting multicollinearity in ecological multiple regression. Ecology 84 (11), 2809–2815. doi: 10.1890/02-3114
Grassman L. I., Tewes M. E., Silvy N. J., Kreetiyutanont K. (2005). Ecology of three sympatric felids in a mixed evergreen forest in north-central Thailand. J. mammalogy 86 (1), 29–38. doi: 10.1644/1545-1542(2005)086<0029:EOTSFI>2.0.CO;2
Gu W., Swihart R. K. (2004). Absent or undetected? Effects of non-detection of species occurrence on wildlife–habitat models. Biol. Conserv. 116, 195–203. doi: 10.1016/S0006-3207(03)00190-3
Guillera-Arroita G. (2017). Modelling of species distributions, range dynamics and communities under imperfect detection: advances, challenges and opportunities. Ecography 40 (2), 281–295. doi: 10.1111/ecog.02445
Haddad N. M., Brudvig L. A., Clobert J., Davies K. F., Gonzalez A., Holt R. D., et al. (2015). Habitat fragmentation and its lasting impact on Earth’s ecosystems. Sci. Adv. 1 (2), e1500052. doi: 10.1126/sciadv.1500052
Hansen M. C., Roy D. P., Lindquist E., Adusei B., Justice C. O., Altstatt A. (2008). A method for integrating MODIS and Landsat data for systematic monitoring of forest cover and change in the Congo Basin. Remote Sens. Environ. 112 (5), 2495–2513. doi: 10.1016/j.rse.2007.11.012
Hansen M. C., Potapov P. V., Moore R., Hancher M., Turubanova S. A., Tyukavina A., et al. (2013). High-resolution global maps of 21st- century forest cover change. Science 342 (6160), 850–853. doi: 10.1126/science.1244693
Harmsen B. J., Foster R. J., Silver S., Ostro L., Doncaster C. P. (2010). Differential use of trails by forest mammals and the implications for camera-trap studies: A case study from Belize. Biotropica 42 (1), 126–133. doi: 10.1111/j.1744-7429.2009.00544.x
Harvey C. A., Gonzalez J., Somarriba E. (2006). Dung beetle and terrestrial mammal diversity in forests, indigenous agroforestry systems and plantain monocultures in Talamanca, Costa Rica. Biodiversity Conserv. 15, 555–585. doi: 10.1007/s10531-005-2088-2
Hebblewhite M., Miquelle D. G., Robinson H., Pikunov D. G., Dunishenko Y. M., Aramilev V. V., et al. (2014). and murzin, A Including biotic interactions with ungulate prey and humans improves habitat conservation modeling for endangered Amur tigers in the Russian Far East. A Biol. Conserv. 178, 50–64. doi: 10.1016/j.biocon.2014.07.013
Hilaluddin K. R., Ghose D. (2005). Conservation implications of wild animal biomass extractions in Northeast India. Anim. Biodiversity Conserv. 28 (2), 169–179. doi: 10.32800/abc.2005.28.0169
Jansen P. A., Muller-Landau H. C., Wright S. J. (2010). Bushmeat hunting and climate: an indirect link. Science 327 (5961), 30–30.
Jigme K. (2011). Four colour morphs of and the altitudinal record of Asiatic Golden Cat in Bhutan. Cat News 55, 12–13.
Jornburom P., Duangchantrasiri S., Jinamoy S., Pattanavibool A., Hines J. E., Arnold T. W., et al. (2020). Habitat use by tiger prey in Thailand’s Western Forest Complex: What will it take to fill a half-full tiger landscape? J. Nat. Conserv. 58, 15896. doi: 10.1016/j.jnc.2020.125896
Katti K. V., Manrekar N., Mukherjee S., Sharma D. (1990). A report on wildlife survey in Arunachal Pradesh with special reference to Takin (Wildlife Institute of India).
Kakati K. (1996). Survey of the Red Panda in Eagle Nest Wildlife Sanctuary, Arunachal Pradesh - a report (Dehradun: Wildlife Institute of India).
Katti M., Manjrekar N., Mukherjee S., Sharma D. (1990). Wildlife survey in Arunachal Pradesh with special reference to takin (Dehradun: Wildlife Institute of India).
Kinnaird M. F., O’Brien T. G. O. (2012). Effects of private-land use, livestock management, and human tolerance on diversity, distribution, and abundance of large African mammals. Conserv. Biol. 26, 1026–1039. doi: 10.1111/j.1523-1739.2012.01942.x
Kushwaha S. P. S., Khan A., Habib B., Quadri A., Singh A. (2004). Evaluation of sambar and muntjak habitats using geostatistical modelling. Curr. Sci., 1390–1400.
Laurance S. G., laurance W. F. (1999). Tropical wildlife corridors: use of linear rainforest remnants by arboreal mammals. Biol. Conserv. 91 (2-3), 231–239.
Laurance W. F., Lovejoy T. E., Vasconcelos H. L., Bruna E. M., Didham R. K., Stouffer P. C., et al. (2002). Ecosystem decay of Amazonian Forest fragments: a 22-year investigation. Conserv. Biol. 16 (3), 605–618.
Laurance W. F., Useche D. C., Shoo L. P., Herzog S. K., Kessler M., Escobar F., et al. (2011). Global warming, elevational ranges and the vulnerability of tropical biota. Biol. Conserv. 144 (1), 548–557.
Lee H. J., Lee O. S., Woo D. G., Kim H. N., Wallace M. C., Jo Y. S. (2021). Current distribution and habitat models of the yellow-throated marten, Martes flavigula, in South Korea. Mammal Res. 66 (3), 429–441. doi: 10.1007/s13364-021-00567-6
Li Z., Kang A., Gu J., Xue Y., Ren Y. I., Zhu Z., et al. (2017). Effects of human disturbance on vegetation, prey and Amur tigers in Hunchun Nature Reserve, China. Ecol. Model. 353, 28–36. doi: 10.1016/j.ecolmodel.2016.08.0
Li X., Bleisch W. V., Jiang X. (2018). Using large spatial scale camera trap data and hierarchical occupancy models to evaluate species richness and occupancy of rare and elusive wildlife communities in southwest China. Diversity Distributions 24 (11), 1560–1572. doi: 10.1111/ddi.12792
Linkie M., Dinata Y., Nugroho A., Hadir I. A. (2007). Estimating occupancy of a data deficient mammalian species living in tropical rainforests: sun bears in Kerinci Seblat region, Sumatra. Biol. Conserv. 137, 27. doi: 10.1016/j.biocon.2007.01.016
MacKenzie D. I. (2005). Was it there? Dealing with imperfect detection for species presence/absence data. Aust. New Z. J. Stat 47, 65–74. doi: 10.1111/j.1467-842X.2005.00372.x
MacKenzie D. I., Nichols J. D., Lachman G. B., Droege S., Andrew Royle J., Langtimm C. A. (2002). Estimating site occupancy rates when detection probabilities are less than one. Ecology 83 (8), 2248–2255. doi: 10.1890/0012-9658(2002)083[2248:ESORWD]2.0.CO;2
MacKenzie D. I., Nichols J. D., Royle J. A., Pollock K. H., Bailey L., et al. (2018). Occupancy estimation and modeling: Inferring patterns and dynamics of species occurrence, 2nd edition (San Diego, CA: Academic Press), 3-26.
Magurran A. E. (2021). Measuring biological diversity. Curr. Biol. 31 (19), R1174–R1177. doi: 10.1016/j.cub.2021.07.049
Matsuo R., Ochiai K. (2009). Dietary overlap among two introduced and one native sympatric carnivore species, the raccoon, the masked palm civet, and the raccoon dog, in Chiba Prefecture, Japan. Mammal Study 34 (4), 187–194. doi: 10.3106/041.034.0402
McCarthy J., Dahal S., Dhendup T., Gray T. N. E., Mukherjee S., Rahman H., et al. (2015). Catopuma temminckii. IUCN Red List Threatened Species 2015.
Mishra C., Datta A., MadhuSudan M. D. (2004). “The high altitude wildlife of Western Arunachal Pradesh: a survey report. Unpublished Report,” in Nature conservation Foundation, International Snow Leopard Trust, and Wildlife Conservation Society (India Program)(Mysore, India).
Mishra C., MadhuSudan M. D., Datta A. (2006). Mammals of the high altitudes of western Arunachal Pradesh, eastern Himalaya: an assessment of threats and conservation needs. Oryx 40, 29–35. doi: 10.1017/S0030605306000032
Molur S. (2020). Atherurus macrourus. (amended version of 2016 assessment) The IUCN Red List of Threatened Species 2020.
Moran P. A. (1950). Notes on continuous stochastic phenomena. Biometrika 37 (1/2), 17–23. doi: 10.1093/biomet/37.1-2.17
Myers N., Mittermeier R. A., Mittermeier C. G., Da Fonseca G. A., Kent J. (2000). Biodiversity hotspots for conservation priorities. Nature 403 (6772), 853–858. doi: 10.1038/35002501
Naughton-Treves L., Mena J. L., Treves A., Alvarez N., Radeloff V. C. (2003). Wildlife survival beyond park boundaries: the impact of slash-and-burn agriculture and hunting on mammals in Tambopata, Peru. Conserv. Biol. 17, 1106–1117. doi: 10.1046/j.1523-1739.2003.02045.x
Niedballa J., Sollmann R., Courtiol A., Wilting A. (2016). camtrapR: an R package for efficient camera trap data management. Methods Ecol. Evol. 7 (12), 1457–1462. doi: 10.1111/2041-210X.12600
Nijhawan S., Mihu A. (2020). Relations of blood: hunting taboos and wildlife conservation in the Idu Mishmi of Northeast India. J. Ethnobiology 40 (2), 149–166. doi: 10.2993/0278-0771-40.2.149
Norris D., Peres C. A., Michalski F., Hinchsliffe K. (2008). Terrestrial mammal responses to edges in Amazonian Forest patches: a study based on track stations. Mammalia 72, 15–23. doi: 10.1515/MAMM.2008.002
O’Brien T. G., Kinnaird M. F., Wibisono H. T. (2003). Crouching tigers, hidden prey: Sumatran tiger and prey populations in a tropical forest landscape. Anim. Conserv. 6, 131–139.
O’Connell A. F., Nichols J. D., Karanth K. U. (2011). “Introduction,” in Camera Traps in Animal Ecology. Eds. O’Connell A. F., Nichols J. D., Karanth K. U. (Tokyo: Springer). doi: 10.1007/978-4-431-99495-4_1
Odden M., Wegge P. (2007). Predicting spacing behavior and mating systems of solitary cervids: a study of hog deer and Indian muntjac. Zoology 110, 270.
Otis D. L., Burnham K. P., White G. C., Anderson D. R. (1978). Statistical inference from capture data on closed animal populations. Wildlife Monograph 62, 1–135.
Parry L., Barlow J., Peres C. A. (2007). Large-vertebrate assemblages of primary and secondary forests in the Brazilian Amazon. J. Trop. Ecology. 23, 653–662.
Panfil S. N., Harvey C. A. (2015). REDD+ and biodiversity conservation: A review of the biodiversity goals, monitoring methods, and impacts of 80 REDD+ projects. Conserv. Lett. 9 (2), 143–150. doi: 10.1111/conl.12188
Paudel P. K., Kindlmann P. (2012). Human disturbance is a major determinant of wildlife distribution in Himalayan midhill landscapes of Nepal. Anim. Conserv. 15 (3), 283–293. doi: 10.1111/j.1469-1795.2011.00514.x
Pettorelli N., Lobora A. L., Msuha M. J., Foley C., Durant S. M. (2010). Carnivore biodiversity in Tanzania: Revealing the distribution patterns of secretive mammals using camera traps. Anim. Conserv. 13, 131–139. doi: 10.1111/j.1469-1795.2009.00309.x
Phumanee W., Steinmetz R., Phoonjampa R., Bejraburnin T., Grainger M., Savini T. (2020). Occupancy-based monitoring of ungulate prey species in Thailand indicates population stability, but limited recovery. Ecosphere 11 (9), e03208. doi: 10.1002/ecs2.3208
Ramesh T., Downs C. T. (2014). Land use factors determining occurrence of Red-necked Spurfowl (Pternistis afer) in the Drakensberg Midlands, South Africa. J. Ornithology. 155, 471–480. doi: 10.1007/s10336-013-1028-2
Ramesh T., Kalle R., Sankar K., Qureshi Q. (2012). Spatio-temporal partition-ing among large carnivores in relation to major prey species in Western Ghats. J. Zoology 287, 269–275. doi: 10.1111/j.1469-7998.2012.00908.x
Ramesh T., Kalle R., Sankar K., Qureshi Q. (2013). Dry season factors determining habitat use and distribution of mouse deer (Moschiola indica) in the western ghats. Eur. J. Wildlife Res. 59, 271–280. doi: 10.1007/s10344-012-0676-5
Riitters K., Wickham J., Costanza J. K., Vogt P. (2016). A global evaluation of forest interior area dynamics using tree cover data from 2000 to 2012. Landscape Ecol. 31 (1), 137–148. doi: 10.1007/s10980-015-0270-9
Rowcliffe J. M., Carbone C. (2008). Surveys using camera traps: Are we looking to a brighter future? Anim. Conserv. 11, 185–186. doi: 10.1111/j.1469-1795.2008.00180.x
Roy K., Nautiyal H., Dasgupta S. (2015). A preliminary study on the activity budget of post-released Eastern Hoolock Gibbon Hoolock leuconedys (Mammalia: Primates: Hylobatidae) in Mehao Wildlife Sanctuary, Arunachal Pradesh, India. J. Threatened Taxa 7 (12), 7862–7869. doi: 10.11609/JoTT.o3869.7862–7869
Rosenzweig M. L. (1992). Species diversity gradients: we know more and less than we thought. J. mammalogy 73 (4), 715–730. doi: 10.2307/1382191
Sarma K., Krishna M., Kumar A. (2015). Fragmented populations of the Vulnerable eastern hoolock gibbon Hoolock leuconedys in the Lower Dibang Valley district, Arunachal Pradesh, India. Oryx 49 (1), 133–139. doi: 10.1017/S0030605312001299
Sarmento P. B., Cruz J., Eira C., Fonseca C. (2011). Modeling the occupancy of sympatric carnivorans in a Mediterranean ecosystem. Eur. J. Wildlife Res. 57, 119–131. doi: 10.1007/s10344-010-0405-x
Schipper J., Chanson J. S., Chiozza F., Al E. (2008). The status of the World’s land and marine mammals: diversity, threat, and knowledge. Science 322, 225–230. doi: 10.1126/science.1165115
Selvan M., Lyngdoh S., Gopi G. V., Habib B. (2014a). Density estimation of leopard cat Prionailurus bengalensis using capture-recaptures sampling in lowland forest of Pakke Tiger Reserve, Arunachal Pradesh, India. Mammalia 78 (4), 555–559. doi: 10.1515/mammalia-2013-0084
Selvan K. M., Lyngdoh S., Habib B., Gopi G. V. (2014b). Population density and abundance of sympatric large carnivores in the lowland tropical evergreen forest of Indian Eastern Himalayas. Mamm. Biol. 79 (4), 254–258. doi: 10.1016/j.mambio.2014.03.002
Selvan K. M., Veeraswami G. G., Lyngdoh S., Habib B., Hussain S. A. (2013). Prey selection and food habits of three sympatric large carnivores in a tropical lowland forest of the Eastern Himalayan Biodiversity Hotspot. Mamm. Biol. 78 (4), 296–303. doi: 10.1016/j.mambio.2012.11.009
Semiadi G., Ross J., Hearn A. J., Macdonald D. W., Mathai J., Augeri D. M., et al. (2016). Predicted distribution of the masked palm civet Paguma larvata (Mammalia: Carnivora: Viverridae) on Borneo. Raffles Bull. Zoology 2016 (S33), 89-95.
Sethy J., Chauhan N. P. S. (2012). Conservation status of Sun bear (Helarctos malayanus) in Nagaland State, North-East India. Asian J. Conserv. Biol. 1 (2), 103–109. Available at: https://www.ajcb.in/journals/full_papers_dec_12/8_Sethy_Chauhan%20_AJCB_1_2_103-109.pdf.
Silveira L., Jácomo A. T., Diniz-Filho J. A. F. (2003). Camera trap, line transect census and track surveys: a comparative evaluation. Biol. Conserv. 114 (3), 351–355. doi: 10.1016/S0006-3207(03)00063-6
Sinha B. C. (1984). A write-up on mehao wildlife sanctuary. Status Himalayas. New Delhi Br. Council. Unpublished Rep., 43–52.
Sinha A., Datta A., MadhuSudan M. D., Mishra C. (2005). Macaca munzala: a new species from western Arunachal Pradesh, northeastern India. International. J. Primatology 26 (4), 977.
Smith C., Baker J. C. A., Spracklen D. V. (2023). Tropical deforestation causes large reductions in observed precipitation (615(7951: Nature). doi: 10.1038/s41586-022-05690-1
Sobral M., Silvius K. M., Overman H., Oliveira L. F., Raab T. K., Fragoso J. (2017). Mammal diversity influences the carbon cycle through trophic interactions in the Amazon. Nat. Ecol. Evol. 1 (11), 1670–1676.
Sollmann R., Modhamed A., Samejima H., Wilting A. (2013). Risky business or simple solution—relative abundance indices from camera-trapping. Biol. Conserv. 159, 405–412.
Sunarto S., Kelly M. J., Parakkasi K., Klenzendorf S., Septayuda E., Kurniawan H. (2012). Tigers need cover: Multi-scale occupancy study of the big cat in Sumatran forest and plantation landscapes. PloS One 7 (1), e30859. doi: 10.1371/journal.pone.0030859
Talukdar N. R., Choudhury P., Singh B. (2019). Current records of porcupine in northeast India: distribution, habitat preference and conservation. Trop. Ecol. 60 (1), 41–51. doi: 10.1007/s42965-019-00005-4
Teng L., Song Y. L., Zeng Z. (2004). Forage and bed site characteristics of Indian muntjac (Muntiacus muntjak) in Hainan Island, China. Ecol. Res. 19675–, 681. doi: 10.1111/j.1440-1703.2004.00683.x
Thapa K., Kelly M. J. (2017). Prey and tigers on the forgotten trail: high prey occupancy and tiger habitat use reveal the importance of the understudied Churia habitat of Nepal. Biodiversity Conserv. 26, 593–616. doi: 10.1007/s10531-016-1260-1
Tobler M. W., Carrillo-Percastegui S. E., Leite Pitman R., Mares R., Powell G. (2008). An evaluation of camera traps for inventorying large- and medium-sized terrestrial rainforest mammals. Anim. Conserv. 11 (3), 169–178. doi: 10.1111/j.1469-1795.2008.00169.x
Tobler M. W., Carrillo-Percastegui S. E., Powell G. (2009). Habitat use, activity patterns and use of mineral licks by five species of ungulate in south-eastern Peru. J. Trop. Ecol. 25, 261–270. doi: 10.1017/S0266467409005896
Vinitpornsawan S. (2013). Population and spatial ecology of Tigers and Leopards relative to prey availability and human activity in Thung Yai Naresuan (East) Wildlife Sanctuary, Thailand. Dissertation Doctor Philosophy. doi: 10.7275/sbtd-sz56
Visconti P., Pressey R. L., Giorgini D., Maiorano L., Bakkenes M., Boitani L., et al. (2011). Future hotspots of terrestrial mammal loss. Philos. Trans. R. Soc. B: Biol. Sci. 366, 2693–2702. doi: 10.1098/rstb.2011.0105
Wang T., Andrew Royle J., Smith J. L. D., Zou L., Lü X., Li T., et al. (2018). Living on the edge: Opportunities for Amur tiger recovery in China. Biol. Conserv. 217, 269–279. doi: 10.1016/j.biocon.2017.11.008
Wu P., Zhang E. (2004). Habitat selection and its seasonal change of serow () in Cibagou Nature Reserve, Tibet. Acta Theriologica Sin. 24 (01), 6–12.
Xiao W., Hebblewhite M., Robinson H., Feng L., Zhou B. O., Mou P. U., et al. (2018). Relationships between humans and ungulate prey shape Amur tiger occurrence in a core protected area along the Sino-Russian border. Ecol. Evol. 8, 11677–11693. doi: 10.1002/ece3.4620
Yang H., Han S., Xie B., Mou P., Kou X., Wang T., et al. (2019). Do prey availability, human disturbance, and habitat structure drive the daily activity patterns of Amur tigers (Panthera tigris altaica)? J. Zoology 307, 131–140. doi: 10.1111/jzo.12622
Yongdrup P., Sherub K., Tshering U., Chaida L. (2019). Abundance and Distribution of Asiatic Golden Cat (Catopumma teminckii Vigors and Horsfield) and Clouded Leopard (Neofelis nebulosa Griffith) in Jomotsangkha Wildlife Sanctuary, Bhutan. Bhutan J. Natural Resour. Dev. 6 (1), 8–8.
Keywords: tropical forest, biodiversity hotspot, mammalian species, occupancy model, Mehao WLS, camera trapping
Citation: Ahmad A and Gopi GV (2024) Site occupancy of select mammals in the tropical forest of Eastern Himalaya. Front. Ecol. Evol. 11:1106329. doi: 10.3389/fevo.2023.1106329
Received: 23 November 2022; Accepted: 30 November 2023;
Published: 27 February 2024.
Edited by:
David Jack Coates, Conservation and Attractions (DBCA), AustraliaReviewed by:
Jaime A. Collazo, North Carolina State University, United StatesMark Cowan, Curtin University, Australia
Copyright © 2024 Ahmad and Gopi. This is an open-access article distributed under the terms of the Creative Commons Attribution License (CC BY). The use, distribution or reproduction in other forums is permitted, provided the original author(s) and the copyright owner(s) are credited and that the original publication in this journal is cited, in accordance with accepted academic practice. No use, distribution or reproduction is permitted which does not comply with these terms.
*Correspondence: Govindan Veeraswami Gopi, Z29waWd2QHdpaS5nb3YuaW4=