- 1Inner Mongolia Key Laboratory of Soil Quality and Nutrient Resources, Key Laboratory of Agricultural Ecological Security and Green Development, College of Grassland, Resources and Environment, Inner Mongolia Agricultural University, Hohhot, China
- 2College of Resources and Environmental Sciences, China Agricultural University, Beijing, China
Enhanced atmospheric nitrogen (N) deposition is threating species diversity in the desert steppe ecoregions. Needlegrass (Stipa breviflora) is the dominant specie in the desert steppe grasslands of China and southern Mongolia, and the response of S. brevifolia to N deposition is not well known. In this study, we conducted an experiment to determine the growth and N uptake of S. breviflora in response to several N addition rates. The results showed that N addition did not change plant growth, emergence rate, plant height, or biomass of S. breviflora, even at a N addition rate of 50 kg N ha−1 yr.−1 with sufficient soil moisture during a 120-day growth period. The absence of a N effect was due to the fact that N uptake in S. breviflora was not improved by N addition. These results indicated that S. breviflora is very conservative with respect to N utilization, which could possibly help it resist enhanced atmospheric N deposition. Moreover, conservative N utilization also enables S. breviflora to survive in N-limiting soils.
1. Introduction
Atmospheric nitrogen (N) deposition has become an important driving factor in grassland ecological systems, and is due mainly to the excessive use of fossil fuels and N fertilizer in the past century (Fowler et al., 2013; IPCC, 2013; Gao et al., 2014). N deposition increases from 34 Tg N yr.−1 in 1860 to 109 Tg N yr.−1 in 2010 globally and is expected to reach 270 Tg N yr.−1 in 2050 (Galloway et al., 2004; Fowler et al., 2013). In China, atmospheric N deposition increases from 13.2 Tg N in 1980 to 21.1 Tg N in 2010 with a rate of increase of 8 Tg N yr.−1 (Liu et al., 2013). Although N deposition generally promotes plant growth (Liu et al., 2010, 2011), many studies have shown that it also has a significant negative impact on grassland ecosystems, in that it reduces species diversity (Bobbink et al., 2010; Duprè et al., 2010; Lan and Bai, 2012) and accelerates soil acidification (Richter and Markewitz, 2001). Researchers (Bobbink, 2004; Bai et al., 2010; Liu et al., 2011) defined the threshold of N deposition to grasslands, which is the value above which aboveground biomass and species diversity significantly decrease (Wang et al., 2019). The thresholds are 4.6 N·m−2 yr.−1 for alpine grasslands in the United States and 7.8 g N·m−2 yr.−1 for acid grassland in England (Bowman et al., 2012; Tipping et al., 2013). The study showed that each 2.5 kg N ha−1 yr.−1 of N deposition results in the loss of one specie per 4 m2 quadrat (Stevens et al., 2004). In China, the thresholds are higher, 9.17 g N·m−2 yr.−1 for semi-arid grasslands and 10.5 g N·m−2 yr.−1 for typical steppe (Bai et al., 2015; Chen et al., 2016). N deposition affects plant communities through direct toxicity, soil acidification, nutrient imbalance and altered interspecific competition (Clark and Tilman, 2008; Bobbink et al., 2010; Tian et al., 2016; Payne et al., 2017). For example, N deposition reduces species richness in acid grasslands as a result of soil acidification (Stevens et al., 2010). In addition, the accumulation of deposited N changes the interspecific relationships of plants, resulting in the gradual elimination of less competitive species (Clark and Tilman, 2008; Bai et al., 2010).
In China, 25% of grasslands are desert steppe (Kang et al., 2007), which are more vulnerable than other grasslands. The current annual N deposition in desert steppe reaches to 14.7 kg N ha−1 yr.−1 (Zhang et al., 2017). Previous studies have found that N deposition increases the aboveground biomass in desert steppe during wet years and reduces the species richness and stability of communities (Su et al., 2012; Wu et al., 2020; Yu et al., 2021). However, most of these studies focus on the effects of N deposition on grassland ecosystem and plant communities. Very few studies have investigated the response of individual plants to N deposition.
Specie in the genus Stipa (Poaceae) are widely distributed across Eurasia (Coupland, 1993) and many are xerophytes (Lu and Wu, 1996). S. breviflora Griseb. (needlegrass) is a common species that is mainly found in the dry regions of China, especially in Inner Mongolia, Xinjiang, and Ningxia as the dominant species of desert steppe grassland. S. breviflora is an excellent pasture grass in the desert steppe that produces early green shoots with fine palatability and other advantages (Yan et al., 2020). A long-term experiment in the desert steppe showed that S. breviflora biomass production do not respond to N deposition (Wu et al., 2020). This is consistent with a previous study showing that C4 plants are almost insensitive to N addition, while C3 plants are highly sensitive (Zhong et al., 2019). However, the reason for this have yet to be clarified. It could be due to a N-soil moisture interaction or conservative N utilization. Drought is the main climatic factor that limits plant growth and distribution in arid regions of the world (Knapp et al., 2017). If drought intensity exceeded N deposition, plants would not respond to N deposition. Conservative N utilization could also make plants insensitive to N deposition.
Because S. breviflora cannot respond to additional N even in wet years, we assumed that soil moisture should not be involved in the interaction between S. breviflora and added N. Therefore, we hypothesized that S. breviflora can resist atmospheric N deposition through conservative N utilization. To test this hypothesis, we investigated growth and N uptake in S. breviflora plants at several different level of added N. In order to avoid interference from soil moisture levels, plants were well irrigated during the entire growth period.
2. Materials and methods
2.1. Study site and experimental design
The experiment was conducted in a phytotron at Inner Mongolia Agricultural University, Hohhot, China, with the following controlled climatic conditions: 12 h light/12 h dark photoperiod, 40% day/40% night relative humidity and 25°C average indoor temperature. The seeds of S. breviflora were collected from the desert steppe located in Siziwang Banner, Wulanchabu city, Inner Mongolia autonomous region, China (41°46′43.6″N, 111°53′41.7″E). In order to eliminate errors caused by seed size, uniform seeds were selected by weighing based on the average size of each batch (0.31 g per 100 seeds). The studied soil was the topsoil (0–20 cm) collected from the same site. After air-drying, soil properties were determined. The soil type was chestnut soil, with a pH of 8.29 and a field capacity of 23%, Other soil properties were shown in Supplementary Table S1. The soil was passed through a 2 mm sieve and used to fill pots of 15 cm in diameter and 12 cm in height that can hold 1.5 kg of soil. The seeds were sterilized in 10% H2O2 for 30 min and germinated in Petri dishes (120 mm in diameter) on wet filter papers.
Five N addition treatments (0, 7.69, 15.38, 23.08, and 38.46 mg N kg−1 soil) with four replicates each were used in this study. The rates were calculated to equal atmospheric N deposition rates of 0, 10, 20, 30, and 50 kg N ha−1 yr.−1, respectively (Supplementary Figure S1). Based on the ratios of organic N:inorganic N of deposition (30%:70%) and NH4+-N:NO3−-N (1.63:1.00) in desert steppe (data from our N deposition monitor net), we designed the N fertilizer mixture to be CO(NH2)2:NH4HCO3:Ca(NO3)2 = 7.41:27.73:17.96 in order to accurately mimic the N forms in deposited N.
All of the N fertilizers were dissolved in deionized and were then added to the 1.5 kg of soil in the pot. After air-drying, the N and soil were mixed thoroughly. Germinated seeds were sown in the pots at a rate of 11 seeds per pot. Soil moisture in each pot was maintained at 70% of field capacity by weighing every 2 days. When the plants were 5–6 cm high, they were thinned to three plants per pot.
2.2. Plants, soil sampling, and analysis
The number of seeds emerging is recorded at 5 days after sowing (DAS) to calculate the emergence rate. The height of S. breviflora was measured with a standard ruler at 30, 60, 90, and 120 DAS. The number of S. breviflora leaves was also counted by forceps at the same time. At harvest, shoots were cut at soil surface. Roots were lifted out of soil and washed by deionized water carefully. All the plant materials were dried at 70°C for 3 days and weighed.
The fresh soil in the pots was collected and separated into two parts, one for soil total N analysis and the other for soil inorganic N measurement. The NH4+-N and NO3−-N in the fresh soil were extracted using KCl solution and the concentrations were determined using a continuous flow analyzer (SEAL, Germany). The soil inorganic N concentration (SIN)was the sum of the ammonium and nitrate concentrations. Plant materials were ground with a ball mill (Retsch MM 400, Germany) and air-dried soil samples were digested in a mixture of concentrated H2SO4 and H2O2, and the N concentration was then determined using the micro-Kjeldahl procedure (Gallaher et al., 1976). The N content was calculated by multiplying plant N concentration and biomass (Li et al., 2010). Soil pH was measured with a pH meter (Leici, China) at a soil:water ratio of 1:2.5. The equations for calculating plant N uptake rate and soil residual N rate were listed as follows:
2.3. Statistical analysis
SPSS software version 24.0 (IBM, US) was used for one-way analysis of variance (ANOVA) to determine the significance of plant height, leaf number, biomass, soil inorganic N concentration, soil pH, plant and soil total N, and N content of the plants (LSD, α = 0.05). Average values are reported as the arithmetic mean. Origin 2021 software (OrginLab, United States) was used to create the figures.
3. Results
3.1. Plant growth
Compared with the control without N addition, N addition did not increase the emergence rate and plant height of S. breviflora plants during 120 days of growth irrespective of the amount of N added (Figure 1; Supplementary Figure S1). The average plant height was 18.1 cm at 30 DAS. Plant height increased by 45.4% at 60 DAS compared with the height at 30 DAS. After 60 DAS, the plants cannot grow higher any more, and maintained at 26.5 cm on average.
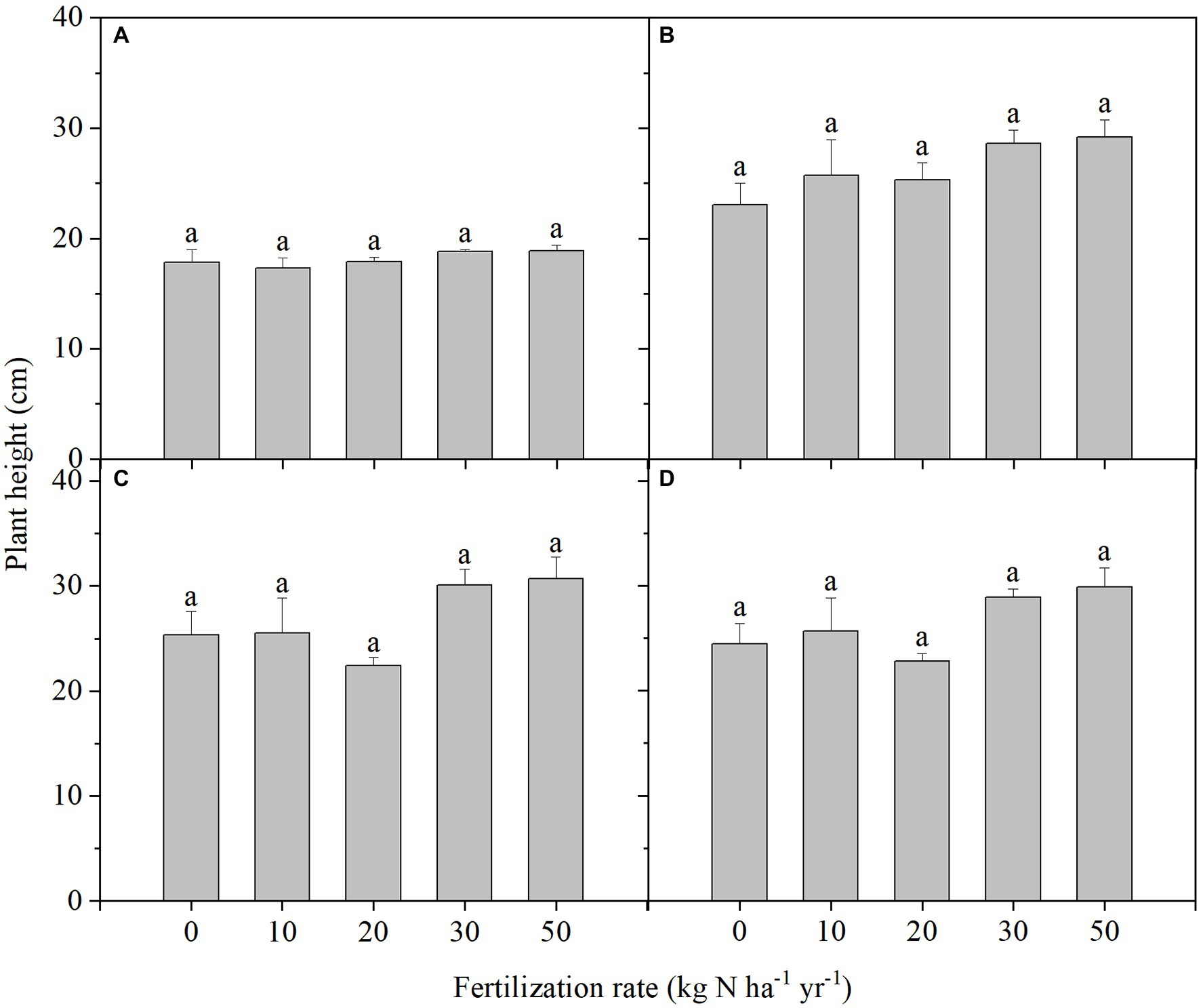
Figure 1. Plant heights in Stipa breviflora plants grown under different N addition rates at 30 days after sowing (DAS) (A), 60 DAS (B), 90 DAS (C), and 120 DAS (D). Each value is the mean of four replicates (+SD). Different lowercase letters indicate significant differences among the treatments at p < 0.05.
Added N significantly suppressed leaf emergence when N addition rates were 30 and 50 kg ha−1 yr.−1 at 30 DAS compared with the control (Supplementary Figure S2). There were six leaves on the S. breviflora plants on average in these two treatments, and this was 27.1% lower than the number in the other treatments. This discrepancy was amplified at 60 DAS when the average leaf number was nine. However, leaf numbers in all the treatments were similar at 90 and 120 DAS. From 90 to 120 DAS, leaf number increased by 42.4%, and reached to 99 leaves (including growing leaves). The leaf emergence rate was much higher from 90 to 120 DAS compared to the other growth periods.
Similar to the situation with plant height, N addition also did not change plant biomass including shoot and root biomass (Figure 2). The shoot and root biomass of each pot averaged 2.04 g pot−1 and 2.40 g pot−1 at 120 DAS, respectively. The ratio of root:shoot biomass of the plants was ~1.
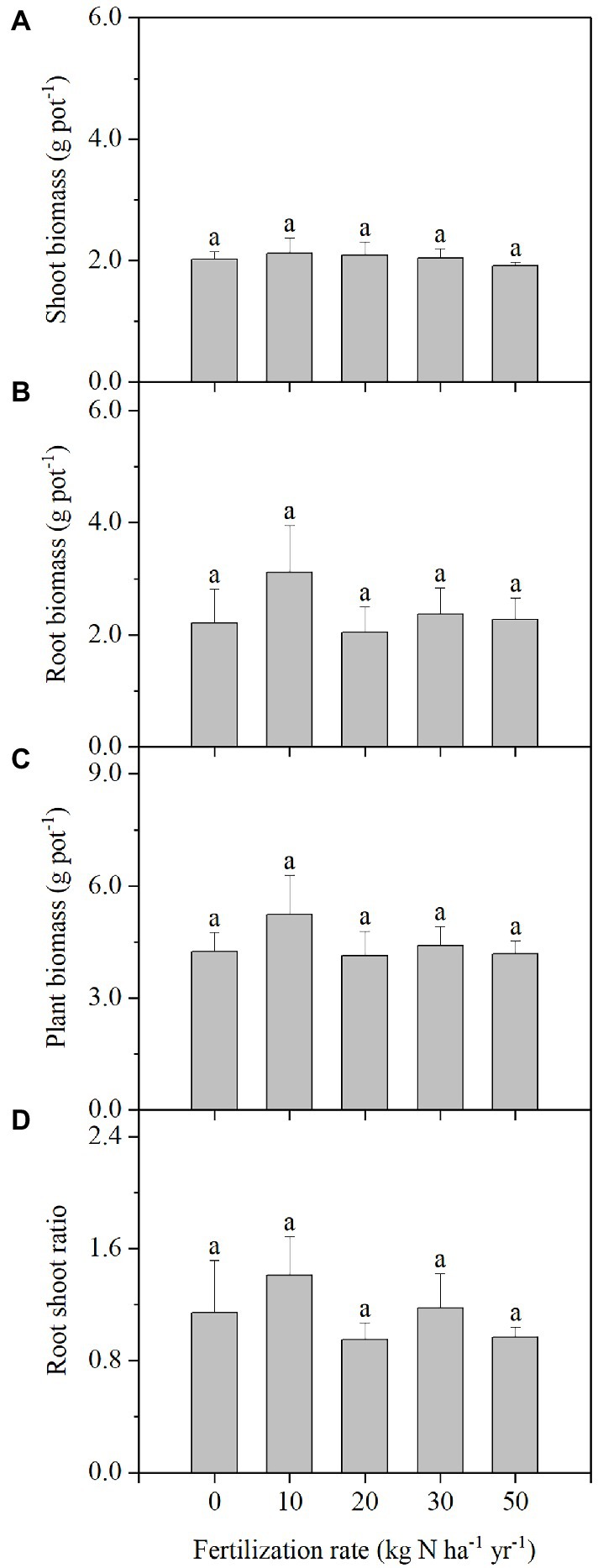
Figure 2. Shoot biomass (A), root biomass (B), plant biomass (C), and root-shoot ratios (D) in Stipa breviflora plants grown under different N addition rates at120 DAS. Each value is the mean of four replicates (+SD). Different lowercase letters indicate significant differences among the treatments at p < 0.05.
3.2. N uptake
Added N did not significantly improve shoot and root N concentrations relative to the control at harvest. Shoot N concentration was 2.33%, and was 2.01 times higher than the average root N concentration of 1.16% (Supplementary Figure S3). The shoot N contents were similar among all treatments, which were 47.3 mg pot−1 on average. The same trend was found in root N content and plant total N content, which averaged 30.6 and 77.8 mg pot−1, respectively. Shoot N content was 54.6% higher than root N content (Figure 3).
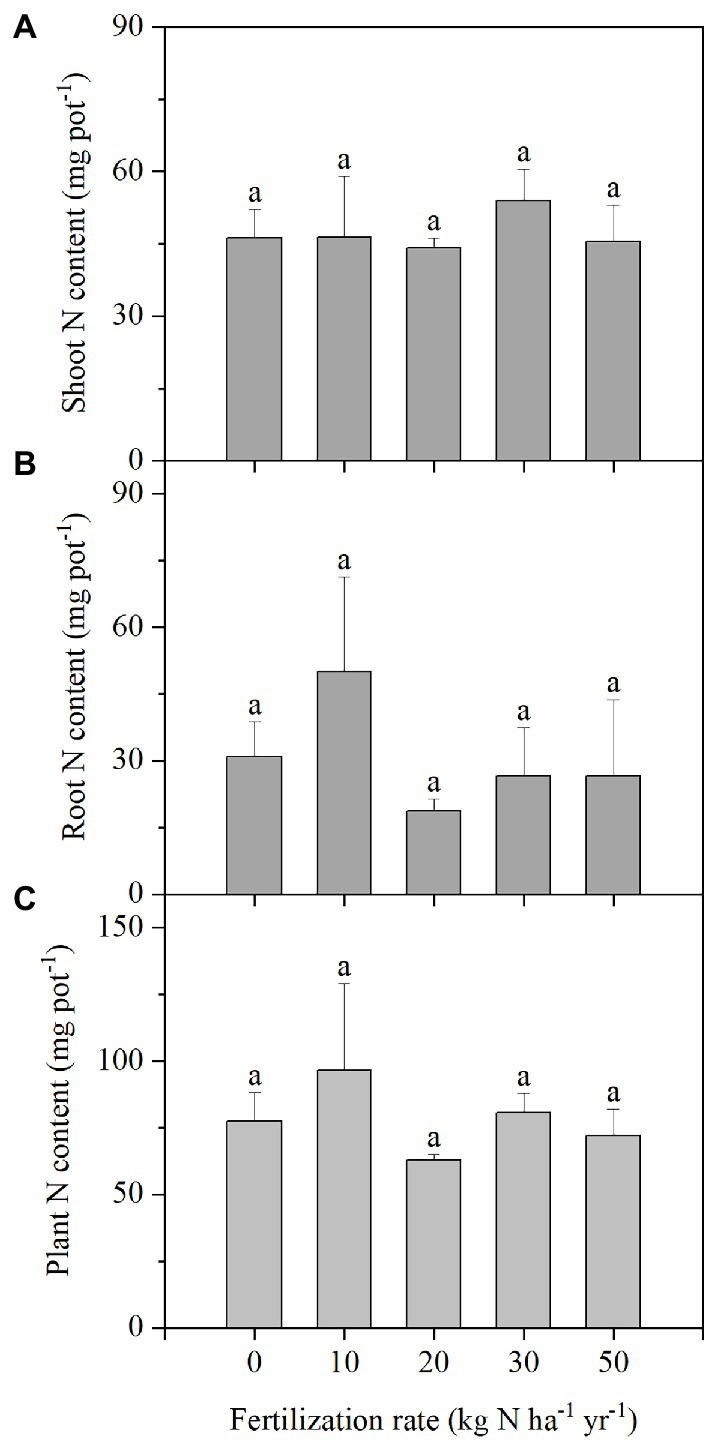
Figure 3. N content of the shoots (A), roots (B), and whole plants (C) in Stipa breviflora plants grown under different N addition rates at 120 DAS. Each value is the mean of four replicates (+SD). Different lowercase letters indicate significant differences among the treatments at p < 0.05.
3.3. Soil N and soil pH
At harvest, no significant changes were found for soil NH4+ concentration in any of the N addition treatments relative to the control (Figure 4). However, N addition increased soil NO3− concentration by 380.2% compared with the control when the addition rate was 50 kg ha−1 yr.−1 (Figure 4). In contrast, soil NO3− concentration in the other treatments with relatively low N addition rates were not significantly different than the control. No significant changes in soil total N were measured for the different N addition rates (Figure 4). Compared to the control, the soil pH decreased significantly by 0.15 pH units only when the N addition rate reach to 50 kg ha−1 yr.−1, and there were no significant differences in the other treatments (Supplementary Figure S4).
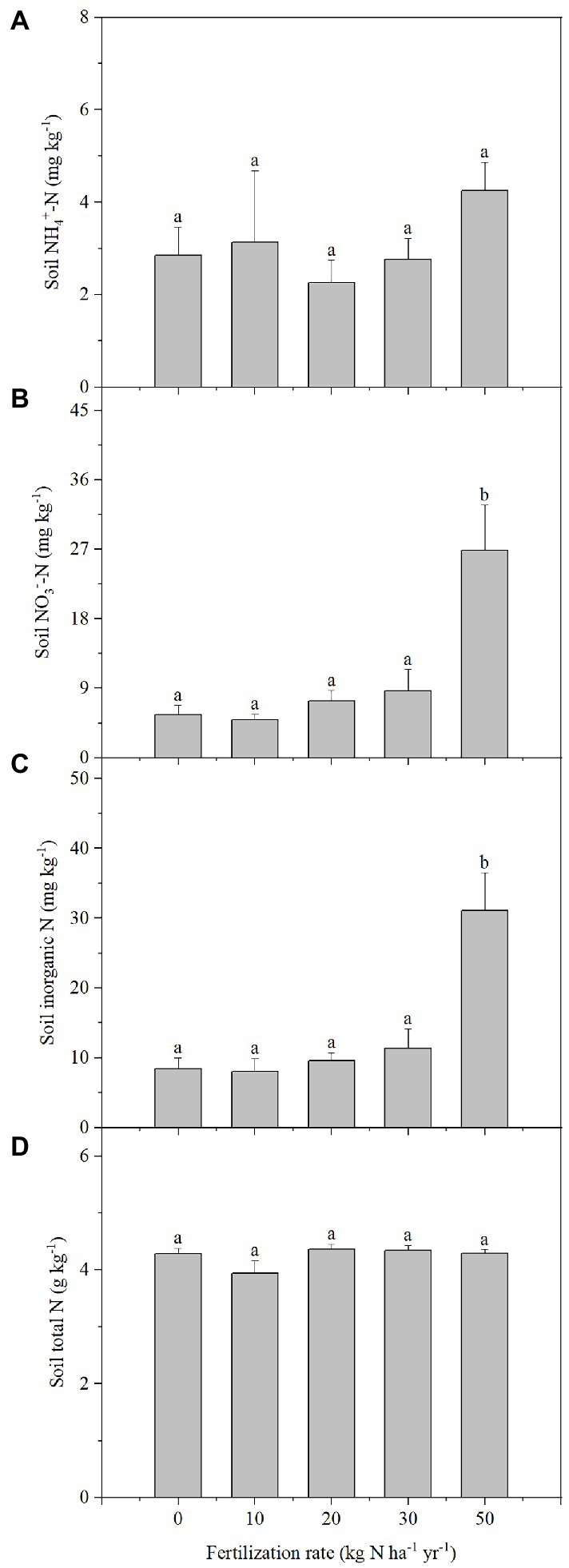
Figure 4. NH4+(A), NO3−(B), inorganic N (C), and total N (D) concentration of pot soils in which Stipa breviflora plants were grown under different N addition rates at 120 DAS. Each value is the mean of four replicates (+SD). Different lowercase letters indicate significant differences among the treatments at p < 0.05.
4. Discussion
4.1. Plant growth
Added N did not change plant height, leaf number, and biomass, although there were changes in leaf number before 60 DAS at N addition rates of 30 and 50 kg ha−1 yr.−1. This indicates that the growth of S. breviflora plants did not respond to N addition, as we hypothesized, and is consistent with the result of Wu et al.,who show that continuous N addition did not change the growth of S. breviflora in natural system, even at 100 kg ha−1 yr.−1 in an experiment spanning 16 years (Wu et al., 2020). In many studies, N addition rate can be as high as 200 kg N ha−1 yr.−1,which results in a change in the growth of some plants (Kazanski et al., 2019). It is possible that the same things could occur in S. breviflora if the plants were exposed to a much higher N addition rate than we used. However, it is difficult to achieve such high N deposition rates in the desert steppe.
In a previous study conducted in the desert steppe, N addition also fails to increase the plant height and biomass in Alhagi sparsifolia and Lycium ruthenicum (Li et al., 2022). In contrast, Wan et al. (2019) and Ren et al. (2020) found that N addition promotes plant height and biomass accumulation in Solidago canadensis, Artemesia argyi, and Pterocypsela laciniata. Furthermore, the sensitivities of plants to N addition in the same grassland system can be different. The two dominant species in the desert steppe have obvious differences in sensitivity to added N; a positive effect is observed in Artemisia capillaris but not in Stipa tianschanica when the N addition rate is 100 kg N ha−1 yr.−1. The results of our study leave no doubt that S. breviflora is insensitive to N addition. In terms of individual plants, N addition is beneficial to nitrophilic species (Bobbink et al., 1998), but not to plants with a conservative N use strategy (Suding et al., 2005; Bobbink et al., 2010; Lü et al., 2020).
4.2. N uptake
In plants that grows in the desert steppe, leaf N concentration stay between 19.0 and 30.0 mg g−1, while root N concentration is lower, and ranges from 7.0 to19.0 mg g−1 (Li, 2014); this includes S. breviflora, Cleistogenes songorica, Convolvulus argenteum, Artemisia frigida, and Kochia prostrata. The ranges are stable even though the geographical locations are different (Ma et al., 2019; Li et al., 2022; Liu et al., 2022). Leaf and root N concentrations in S. breviflora in the desert steppe are determined to be 19.6 and 7.2 mg g−1, respectively, in a previous study conducted in natural conditions (Li, 2018). In this study, the leaf and root N concentration of S. breviflora plants were 23.3 and 11.6 mg g−1 respectively, which were slightly higher than the result of the previous study. This discrepancy could be due to the better growth condition in our study. Compared with the other plant species that grow in the desert steppe, S. breviflora has the highest biomass with the lowest N concentration (Li, 2018). Therefore, the efficient N utilization of S. breviflora is one of the reasons that it became a constructive species.
In two previous studies, N addition increases the N concentration of plants (Yuan and Chen, 2015; Huang et al., 2018), however, the N concentration of S. breviflora plants was not affected by added N in our study. Similar results are found in the desert steppes for Lycium ruthenicum Murr and Alhagi sparsifolia Shap as constructive species. Plants that adopt a conservative strategy for N utilization can provide a guarantee for maintaining ecosystem stability and effectively reduce the disturbance caused by N addition to grassland ecosystems.
4.3. Soil N concentration
At N addition rates equaled to or less than 30 kg N ha−1 yr.−1, SIN did not show a significant change. The budget between input and output determines the change in soil inorganic N concentration. Added N was the only source of input N in this study because the effects of N deposition can be ignored. Although 30% of the N added to the soil is urea, it is unlikely to maintain this form at harvest because urea is converted into NO3−-N in calcareous soil within 1 week after addition (Tong et al., 1992). N output occurs through plant uptake, NO3− leaching, and NH3 emission. In the N addition treatments, the uptake of S. breviflora plants was not greater than in the control, which was due to the same root N uptake rate (Supplementary Figure S6A). Also, no N leaching occurred during plant growth due to the use of weighing irrigation. The NH3 emission were not measured, but it can be assumed to be <20% according to a previous study (Stumpe et al., 1984). Therefore, this suggests that most N added to the soil is absorbed by soil microorganisms. The study in the desert steppe also showed that N addition significantly increased the amount of microbial biomass N in soil when there was sufficient water (Zhu et al., 2022). It is also confirmed by a mate-analysis that N addition leads to a 10% increase of soil microbial N (Zhou et al., 2017). Soil inorganic N concentration in the 50 kg N ha−1 yr.−1 treatment was higher than that in the 30 kg N ha−1 treatment yr.−1 by 19.67 mg kg−1, which is close to the difference in N addition rate between the two treatments. Soil residual N rate was 58.9% in the 50 kg N ha−1 yr.−1 treatment, suggesting 29.5 kg N ha−1 yr.−1 cannot be used by plants and soil microorganisms during growth period (Supplementary Figure S6B). This indicates that the soil is N-limiting, and 30 kg N ha−1 yr.−1 is a key change-point between N-limiting and non-limiting. However, it should be clarified that a short-term pot experiment cannot fully mimic natural conditions for soil microorganisms. This value can only be a reference which could possibly be modified under natural conditions.
5. Conclusion
The growth of S. breviflora plants did not respond to N addition, even when the rate was 50 kg N ha−1 yr.−1 and the soil moisture level was sufficient. This was due to the fact that additional N cannot increase N uptake in S. breviflora. The results of our study indicate that S. breviflora is very conservative with respect to N utilization, which can help the plants to resist the effects of increased atmospheric N deposition. Moreover, conservative N utilization also allow S. breviflora to survive in N-limiting soils.
Data availability statement
The raw data supporting the conclusions of this article will be made available by the authors, without undue reservation.
Author contributions
KZ and HL conceived the study. KZ wrote the majority of the manuscript with input from HL and HG. ZS participated in the experiment and provided the laboratory space.
Funding
The study was funded by the Double First-Class Financial Capital in China (Grant number: NDYB2018-4/NDYB2019-4), the Programs for Key Science and Technology Development of Inner Mongolia in 2021 (2021GG0076), the Scientific Research Start-up Fund of the Autonomous Region Human Resources and Social Security Department in 2018 (for HL) and the Project of Grassland Talent (for HL).
Acknowledgments
We thank Guodong Han and Fei Li for providing advices.
Conflict of interest
The authors declare that the research was conducted in the absence of any commercial or financial relationships that could be construed as a potential conflict of interest.
Publisher’s note
All claims expressed in this article are solely those of the authors and do not necessarily represent those of their affiliated organizations, or those of the publisher, the editors and the reviewers. Any product that may be evaluated in this article, or claim that may be made by its manufacturer, is not guaranteed or endorsed by the publisher.
Supplementary material
The Supplementary material for this article can be found online at: https://www.frontiersin.org/articles/10.3389/fevo.2023.1106089/full#supplementary-material
References
Bai, W., Guo, D., Tian, Q., Liu, N., Cheng, W., Li, L., et al. (2015). Differential responses of grasses and forbs led to marked reduction in below-ground productivity in temperate steppe following chronic N deposition. J. Ecol. 103, 1570–1579. doi: 10.1111/1365-2745.12468
Bai, Y. F., Wu, J. G., Clark, C. M., Naeem, S., Pan, Q. M., Huang, J. H., et al. (2010). Tradeoffs and thresholds in the effects of nitrogen addition on biodiversity and ecosystem functioning: evidence from inner Mongolia grasslands. Glob. Chang. Biol. 16, 358–372. doi: 10.1111/j.1365-2486.2009.01950.x
Bobbink, R. (2004). Plant species richness and the exceedance of empirical nitrogen critical loads: An inventory. Utrecht: Utrecht University.
Bobbink, R., Hicks, K., Galloway, J., Spranger, T., Alkemade, R., Ashmore, M., et al. (2010). Global assessment of nitrogen deposition effects on terrestrial plant diversity: a synthesis. Ecol. Appl. 20, 30–59. doi: 10.1890/08-1140.1
Bobbink, R., Hornung, M., and Roelofs, J. G. (1998). The effects of air-borne nitrogen pollutants on species diversity in natural and semi-natural European vegetation. J. Ecol. 86, 717–738. doi: 10.1046/j.1365-2745.1998.8650717.x
Bowman, W. D., Murgel, J., Blett, T., and Porter, E. (2012). Nitrogen critical loads for alpine vegetation and soils in Rocky Mountain National Park. J. Environ. Manag. 103, 165–171. doi: 10.1016/j.jenvman.2012.03.002
Chen, W. Q., Zhang, Y. J., Mai, X. H., and Shen, Y. (2016). Multiple mechanisms contributed to the reduced stability of Inner Mongolia grassland ecosystem following nitrogen enrichment. Plant Soil 409, 283–296. doi: 10.1007/s11104-016-2967-1
Clark, C. M., and Tilman, D. (2008). Loss of plant species after chronic low-level nitrogen deposition to prairie grasslands. Nature 451, 712–715. doi: 10.1038/nature06503
Duprè, C., Stevens, C. J., Ranke, T., Bleeker, A., Peppler, L. C., Gowing, D. J., et al. (2010). Changes in species richness and composition in European acidic grasslands over the past 70 years: the contribution of cumulative atmospheric nitrogen deposition. Glob. Chang. Biol. 16, 344–357. doi: 10.1111/j.1365-2486.2009.01982.x
Fowler, D., Coyle, M., Skiba, U., Sutton, M. A., Cape, J. N., Reis, S., et al. (2013). The global nitrogen cycle in the twenty-first century. Philos. Trans. R. Soc. B Biol. Sci. 368:20130164. doi: 10.1098/rstb.2013.0164
Gallaher, R., Weldon, C., and Boswell, F. (1976). A semiautomated procedure for total nitrogen in plant and soil samples. Soil Sci. Soc. Am. J. 40, 887–889. doi: 10.2136/sssaj1976.03615995004000060026x
Galloway, J. N., Dentener, F. J., Capone, D. G., Boyer, E. W., Howarth, R. W., Seitzinger, S. P., et al. (2004). Nitrogen cycles: past, present, and future. Biogeochemistry 70, 153–226. doi: 10.1007/S10533-004-0370-0
Gao, Y., He, N. P., and Zhang, X. Y. (2014). Effects of reactive nitrogen deposition on terrestrial and aquatic ecosystems. Ecol. Eng. 70, 312–318. doi: 10.1016/j.ecoleng.2014.06.027
Huang, J., Wang, P., Niu, Y., Yu, H., Ma, F., Xiao, G., et al. (2018). Changes in C:N:P stoichiometry modify N and P conservation strategies of a desert steppe species Glycyrrhiza uralensis. Sci. Rep. 8:12668. doi: 10.1038/s41598-018-30324-w
IPCC. (2013). The physical science basis. Contribution of working group I to the fifth assessment report of the intergovernmental panel on climate change. Cambridge: Cambridge University Press.
Kang, L., Han, X., Zhang, Z., and Sun, O. J. (2007). Grassland ecosystems in China: review of current knowledge and research advancement. Philos. Trans. R. Soc. B Biol. Sci. 362, 997–1008. doi: 10.1098/rstb.2007.2029
Kazanski, C. E., Riggs, C. E., Reich, P. B., and Hobbie, S. E. (2019). Long-term nitrogen addition does not increase soil carbon storage or cycling across eight temperate Forest and grassland sites on a Sandy outwash plain. Ecosystems 22, 1592–1605. doi: 10.1007/s10021-019-00357-x
Knapp, A. K., Ciais, P., and Smith, M. D. (2017). Reconciling inconsistencies in precipitation-productivity relationships: implications for climate change. New Phytol. 214, 41–47. doi: 10.1111/nph.14381
Lan, Z. C., and Bai, Y. F. (2012). Testing mechanisms of N-enrichment-induced species loss in a semiarid Inner Mongolia grassland: critical thresholds and implications for long-term ecosystem responses. Philos. Trans. R. Soc. B Biol. Sci. 367, 3125–3134. doi: 10.1098/rstb.2011.0352
Li, Y. H. (2014) Responses of plant community structure and function to warming and nitrogen addition in desert steppe of Inner Mongolia. [dissertation’s thesis]. [Hohhot]: Inner Mongolia Agricultural University.
Li, J. W. (2018) Relationship between plant functional traits and functional diversity in a Stipa breviflora desert steppe under long-term grazing with different stocking rates. dissertation’s thesis [Hohhot]: Inner Mongolia Agricultural University.
Li, Q. Z., Sun, J. H., Wei, X. J., Christie, P., Zhang, F. S., and Li, L. (2010). Overyielding and interspecific interactions mediated by nitrogen fertilization in strip intercropping of maize with faba bean, wheat and barley. Plant Soil 339, 147–161. doi: 10.1007/s11104-010-0561-5
Li, M. R., Wang, L. L., Li, J. J., Peng, Z. L., Wang, L., Zhang, X. F., et al. (2022). Grazing exclusion had greater effects than nitrogen addition on soil and plant community in a desert steppe, northwest of China. BMC Plant Biol. 22:60. doi: 10.1186/s12870-021-03400-z
Liu, Q., Chen, W., Gao, H., Sun, Z., Wang, Y., and Li, H. (2022). Nutrient budgets drive the changes in shoot N and P concentrations of plants in Inner Mongolia's grasslands over the past 40 years. Sci. Total Environ. 838:156374. doi: 10.1016/j.scitotenv.2022.156374
Liu, X., Duan, L., Mo, J., Du, E., Shen, J., Lu, X., et al. (2011). Nitrogen deposition and its ecological impact in China: an overview. Environ. Pollut. 159, 2251–2264. doi: 10.1016/j.envpol.2010.08.002
Liu, X., Song, L., He, C., and Zhang, F. (2010). Nitrogen deposition as an important nutrient from the environment and its impact on ecosystems in China. J. Arid. Land 2, 137–143. doi: 10.3724/sp.J.1227.2010.00137
Liu, X. J., Zhang, Y., Han, W. X., Tang, A. H., Shen, J. L., Cui, Z. L., et al. (2013). Enhanced nitrogen deposition over China. Nature 494, 459–462. doi: 10.1038/nature11917
Lü, X. T., Hou, S. L., Reed, S., Yin, J. X., Hu, Y. Y., Wei, H. W., et al. (2020). Nitrogen enrichment reduces nitrogen and phosphorus resorption through changes to species resorption and plant community composition. Ecosystems 24, 602–612. doi: 10.1007/s10021-020-00537-0
Lu, S. L., and Wu, Z. L. (1996). On geographical distribution of the genus Stipa L. in China. J. Syst. Evol. 34, 242–253.
Ma, Q., Liu, X., Li, Y., Li, L., Yu, H., Qi, M., et al. (2019). Nitrogen deposition magnifies the sensitivity of desert steppe plant communities to large changes in precipitation. J. Ecol. 108, 598–610. doi: 10.1111/1365-2745.13264
Payne, R. J., Dise, N. B., Field, C. D., Dore, A. J., Caporn, S. J., and Stevens, C. J. (2017). Nitrogen deposition and plant biodiversity: past, present, and future. Front. Ecol. Environ. 15, 431–436. doi: 10.1002/fee.1528
Ren, G. Q., Yang, H. Y., Li, J., Prabakaran, K., Dai, Z. C., Wang, X. P., et al. (2020). The effect of nitrogen and temperature changes on Solidago canadensis phenotypic plasticity and fitness. Plant Spec. Biol. 35, 283–299. doi: 10.1111/1442-1984.12280
Richter, D. D., and Markewitz, D. (2001). Understanding soil change: Soil sustainability over millennia, centuries, and decades. Cambridge: Cambridge University Press.
Stevens, C. J., Dise, N. B., Mountford, J. O., and Gowing, D. J. (2004). Impact of nitrogen deposition on the species richness of grasslands. Science 303, 1876–1879. doi: 10.1126/science.1094678
Stevens, C. J., Dupre, C., Dorland, E., Gaudnik, C., Gowing, D. J., Bleeker, A., et al. (2010). Nitrogen deposition threatens species richness of grasslands across Europe. Environ. Pollut. 158, 2940–2945. doi: 10.1016/j.envpol.2010.06.006
Stumpe, J. M., Vlek, P. L. G., and Lindsay, W. L. (1984). Ammonia volatilization from urea and urea phosphates in calcareous soils. Soil Sci. Soc. Am. J. 48, 921–927. doi: 10.2136/sssaj1984.03615995004800040044x
Su, J., Li, X., Li, X., and Feng, L. (2012). Effects of additional N on herbaceous species of desertified steppe in arid regions of China: a four-year field study. Ecol. Res. 28, 21–28. doi: 10.1007/s11284-012-0994-9
Suding, K. N., Collins, S. L., Gough, L., Clark, C., Cleland, E. E., Gross, K. L., et al. (2005). Functional-and abundance-based mechanisms explain diversity loss due to N fertilization. Proc. Natl. Acad. Sci. U. S. A. 102, 4387–4392. doi: 10.1073/pnas.0408648102
Tian, Q., Liu, N., Bai, W., Li, L., Chen, J., Reich, P. B., et al. (2016). A novel soil manganese mechanism drives plant species loss with increased nitrogen deposition in a temperate steppe. Ecology 97, 65–74. doi: 10.1890/15-0917.1
Tipping, E., Henrys, P. A., Maskell, L. C., and Smart, S. M. (2013). Nitrogen deposition effects on plant species diversity; threshold loads from field data. Environ. Pollut. 179, 218–223. doi: 10.1016/j.envpol.2013.04.008
Tong, Y. A., Wen, H. S., and Deng, J. L. (1992). Decomposition and transformation of urea in different soil types. Shaanxi journal of. Agric. Sci. 1, 15–16.
Wan, L. Y., Qi, S. S., Zou, C. B., Dai, Z. C., Ren, G. Q., Chen, Q., et al. (2019). Elevated nitrogen deposition may advance invasive weed, Solidago canadensis, in calcareous soils. J. Plant Ecol. 12, 846–856. doi: 10.1093/jpe/rtz019
Wang, J., Gao, Y. Z., Zhang, Y. H., Yang, J. J., and Han, X. G. (2019). Asymmetry in aboveand belowground productivity responses to N addition in a semiarid temperate steppe. Glob. Chang. Biol. 25, 2958–2969. doi: 10.1111/gcb.14719
Wu, Q., Ren, H. Y., Wang, Z. W., Li, Z. G., Liu, Y. H., Wang, Z., et al. (2020). Additive negative effects of decadal warming and nitrogen addition on grassland community stability. J. Ecol. 108, 1442–1452. doi: 10.1111/1365-2745.13363
Yan, D. Q., Ren, J., Liu, J. M., Ding, Y., and Niu, J. M. (2020). De novo assembly, annotation, marker discovery, and genetic diversity of the Stipa breviflora Griseb. (Poaceae) response to grazing. PLoS One 15:e0244222. doi: 10.1371/journal.pone.0244222
Yu, H., Ma, Q., Liu, X., Li, Y., Li, L., Qi, M., et al. (2021). Resistance, recovery, and resilience of desert steppe to precipitation alterations with nitrogen deposition. J. Clean. Prod. 317:128434. doi: 10.1016/j.jclepro.2021.128434
Yuan, Z., and Chen, H. Y. (2015). Negative effects of fertilization on plant nutrient resorption. Ecology 96, 373–380. doi: 10.1890/14-0140.1
Zhang, Y., Xu, W., Wen, Z., Wang, D., Hao, T., Tang, A., et al. (2017). Atmospheric deposition of inorganic nitrogen in a semi-arid grassland of Inner Mongolia, China. J. Arid. Land 9, 810–822. doi: 10.1007/s40333-017-0071-x
Zhong, S. Z., Xu, Y. Q., Meng, B., Loik, M. E., Ma, J. Y., and Sun, W. (2019). Nitrogen addition increases the sensitivity of photosynthesis to drought and re-watering differentially in C3 versus C4 grass species. Front. Plant Sci. 10:815. doi: 10.3389/fpls.2019.00815
Zhou, Z., Wang, C., Zheng, M., Jiang, L., and Luo, Y. (2017). Patterns and mechanisms of responses by soil microbial communities to nitrogen addition. Soil Biol. Biochem. 115, 433–441. doi: 10.1016/j.soilbio.2017.09.015
Keywords: Stipa breviflora, desert steppe, N addition, plant growth, N uptake
Citation: Zhao K, Gao H, Sun Z, Zhang J and Li H (2023) Growth of Stipa breviflora does not respond to nitrogen addition because of its conservative nitrogen utilization. Front. Ecol. Evol. 11:1106089. doi: 10.3389/fevo.2023.1106089
Edited by:
Bayartungalag Batsaikhan, Mongolian Academy of Sciences (MAS), MongoliaReviewed by:
Tao Huang, Nanjing Normal University, ChinaShaokun Wang, Northwest Institute of Eco-Environment and Resources (CAS), China
Copyright © 2023 Zhao, Gao, Sun, Zhang and Li. This is an open-access article distributed under the terms of the Creative Commons Attribution License (CC BY). The use, distribution or reproduction in other forums is permitted, provided the original author(s) and the copyright owner(s) are credited and that the original publication in this journal is cited, in accordance with accepted academic practice. No use, distribution or reproduction is permitted which does not comply with these terms.
*Correspondence: Haigang Li, aGFpZ2FuZ2xpQGltYXUuZWR1LmNu