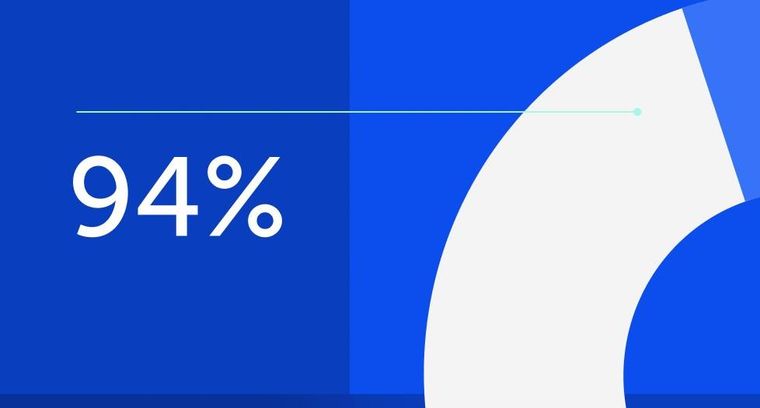
94% of researchers rate our articles as excellent or good
Learn more about the work of our research integrity team to safeguard the quality of each article we publish.
Find out more
ORIGINAL RESEARCH article
Front. Ecol. Evol., 27 March 2023
Sec. Conservation and Restoration Ecology
Volume 11 - 2023 | https://doi.org/10.3389/fevo.2023.1094152
Due to consistent population declines across the continent, grassland birds have become a guild of high conservation and management interest. Despite a large number of studies investigating grassland bird habitat associations, we know relatively little about the mechanisms through which habitat characteristics may impact grassland birds, as these mechanisms are often assumed rather than directly tested. For this study, we estimated whether the effects of habitat structure on breeding Field Sparrows are mediated through changes in predator (snake and raccoon) abundance, alternative prey availability, or arthropod biomass using structural equation models. We found no evidence of nest survival or nest density of Field Sparrows being directly influenced by nest predator abundance, alternative prey, or arthropod biomass, although habitat characteristics associated with increased nest survival were also associated with greater arthropod biomass and reduced predator abundance. We suggest that habitat structure in our study area primarily impacts breeding Field Sparrows through direct means, such as influencing nest concealment or foraging efficiency. Our results also suggest that nest success and nest density are decoupled in our study area, so Field Sparrows may be preferentially selecting nest sites with structural characteristics that do not increase nest survival. Ultimately, our findings from this study indicate that while predator avoidance and food provisioning likely play an important role in determining nest survival for grassland birds, predator abundance and arthropod biomass may not necessarily predict predation risk and foraging efficiency to the extent that is often assumed.
Grassland birds are the most rapidly declining bird guild in North America (Rosenberg et al., 2019), due in large part to extensive habitat loss and fragmentation resulting from the spread of agriculture and other human-dominated landscapes (Brennan and Kuvlesky, 2005; Hill et al., 2014; Stanton et al., 2018). Although the plight of grassland birds has been well known for several decades (Robbins et al., 1986; Herkert, 1994), declines of many species continue to the present day (Rosenberg et al., 2019). An important factor contributing to the continued decline of grassland birds is our limited understanding of the mechanisms through which habitat structure affects bird populations, which can reduce the effectiveness of management actions and result in inconsistent patterns of bird-habitat relationships among studies. For example, Grasshopper Sparrows (Ammodramus savannarum) have been documented preferentially selecting grasslands dominated by native bunch grasses (Whitmore, 1981) and grasslands primarily composed of exotic rhizomatous grass (Johnson and Schwartz, 1993; Madden et al., 2000). Similarly, patch size, edge proximity, and landscape composition have varying effects on nest survival of grassland birds (Winter et al., 2006; Benson et al., 2013), despite generalized assumptions that such effects are consistent. Whereas studies documenting covariance between habitat variables and metrics of bird populations are common (see Dechant et al., 2002; Hull, 2002; Herkert, 2002, and citations therein), the mechanisms through which habitat structure affects grassland birds remain understudied (but see Ellison et al., 2013; Lyons et al., 2015). Increasing our understanding of the direct and indirect effects of habitat structure on grassland birds may clarify the inconsistencies of previous bird-habitat studies, and represents a critical step toward improving management actions for declining species.
Habitat structure may directly affect birds in several ways, including providing nesting and perching substrates, contributing to nest concealment (Nelson and Martin, 1999; Davis, 2005; Warren and Anderson, 2005), and influencing foraging efficiency (Bradbury et al., 2005) and nest microclimate (Martin, 1998; Nelson and Martin, 1999; Hoekman et al., 2002). Additionally, habitat characteristics across multiple spatial scales may impact birds indirectly by influencing community interactions with avian predators or prey via bottom-up processes (Karr et al., 1992; Feeley and Terborgh, 2008; Klug et al., 2010). Consequentially, the impacts of habitat characteristics on birds are frequently assumed to be at least partially due to changes in predator abundance (Thompson et al., 2002; Davis, 2005; Barding and Nelson, 2008; Hirsch-Jacobson et al., 2012) or prey availability in the form of arthropod biomass (McIntyre and Thompson, 2003; Shochat et al., 2005; Hamer et al., 2006; Hickman et al., 2006; Loss and Blair, 2011; Londe et al., 2021). Predation is the leading cause of avian nest failure (Ricklefs, 1969; Martin, 1993), and birds face selective pressure to establish nest sites in areas that minimize predation risk (Martin, 1993, 1998). In eastern North American grasslands, prolific nest predators include snakes (Weatherhead and Blouin-Demers, 2004; Ellison and Ribic, 2012; DeGregorio et al., 2014) and meso-mammals such as raccoons (Nelson, 2001; Schmidt, 2002; Lyons et al., 2015); thus, habitat characteristics may indirectly impact bird communities via changes in the activity or abundance of these predator guilds. For instance, Klug et al. (2010) found that shrub cover in grasslands indirectly decreases avian nest success by increasing snake abundance, and the negative impact of wooded edges on many grassland birds is understood to be at least partially caused by an increase in the activity of generalist nest predators (Johnson and Temple, 1990; Winter et al., 2000; Galligan et al., 2006) and brown-headed cowbirds (Jensen and Finck, 2004; Benson et al., 2013). Similarly, one of the mechanisms through which habitat fragmentation negatively affects grassland bird populations may be an increase in nest predator density in smaller, more fragmented patches compared to large contiguous grasslands (Chalfoun et al., 2002; Ribic et al., 2009).
Predation risk is not necessarily determined solely by predator abundance, rather it can be influenced by multiple factors. For instance, alternative prey availability can affect predator behavior by determining the extent to which they target bird nests as a food source (Ackerman, 2002; Schmidt et al., 2008; Nordberg and Schwarzkopf, 2019), as predators may change prey preference according to relative abundance or availability of different prey (Murdoch, 1969). Small mammals are a common alternative prey guild for birds in tallgrass prairies, as small mammals are also preyed upon by snakes and generalist meso-mammals. Therefore, if small mammal abundance is low in a grassland patch and bird nests represent a more easily available prey item, nest predation rates may rise independently of predator abundance. Additionally, if alternative prey are overabundant, they may attract more nest predators which can also increase nest predation rates (Haffele et al., 2013). Arthropods may also act as alternative prey for grassland birds in systems where snakes such as North American Racers (Coluber constrictor), which frequently feed on arthropods (Klimstra, 1959), are a dominant nest predator.
Arthropods are an important food source for grassland birds, particularly nestlings (Best, 1977; Kaspari and Joern, 1993; McIntyre and Thompson, 2003), thus avian abundance and nest density may be positively influenced by arthropod biomass. Additionally, an increase in arthropod biomass may improve nest success by allowing nestlings to grow and fledge faster, and by reducing the number of foraging trips by adults, which can alert predators of the nest location (Eichholz and Koenig, 1992; Eggers et al., 2005). Data from Shochat et al. (2005) suggests that birds preferentially nested in managed (burned and grazed) grassland plots because these plots supported higher arthropod biomass than unmanaged grasslands. Similar conclusions were reached by Hickman et al. (2006), who found that higher bird abundance and species richness in native versus exotic grasslands in Kansas may be explained by greater arthropod biomass in native grasslands. Grassland bird species richness has also been positively related to arthropod richness in shortgrass prairies of Wyoming (Hamer et al., 2006).
For this study, we combined multiple datasets from Burning Star Wildlife Management Area in Jackson County, IL, to estimate the extent to which the effects of habitat structure on grassland birds during the breeding season are mediated through arthropod biomass, nest predator abundance, and alternative prey availability. We chose Field Sparrows (Spizella pusilla) as our focal bird species, as they are the most common grassland bird nesting in our study area. The Field Sparrow is a facultative grassland species that can successfully inhabit a variety of open habitats, including old fields and woodland openings (Herkert, 1991; Vickery et al., 1994; Dechant et al., 2002). Though common, Field Sparrows have experienced population declines throughout the Midwest due to habitat loss and their avoidance of human-dominated areas (Herkert, 1995; Sauer et al., 2020). We used structural equation modeling to evaluate how the effects of habitat structure on Field Sparrow nest survival and nest density may be mediated through changes in other components of the grassland community. We considered both nest survival and nest density because using both metrics presents a more accurate assessment of avian habitat quality than nest survival alone (Johnson, 2007; Monroe et al., 2016; Pulliam et al., 2021). We tested four non-exclusive predictions: (1) habitat structure affects Field Sparrow nest survival and density by influencing arthropod biomass; (2) habitat structure affects Field Sparrow nest survival and density by influencing the abundance of nest predators, specifically snakes and raccoons; (3) habitat structure affects Field Sparrows via alterative prey such as small mammals or arthropods; (4) our ecological null hypothesis: the effects of habitat structure on Field Sparrows are primarily direct. We expected nest survival and nest density to be differentially influenced by grassland habitat and community characteristics, as factors affecting nest survival and nest density or adult abundance are frequently decoupled (Chalfoun and Schmidt, 2012).
Our study took place at Burning Star State Fish and Wildlife Area (37°52’N, 89°12’W, hereafter “Burning Star”), a former surface coal mine composed of 1,824 hectares (ha) of both reclaimed and undisturbed land in northeast Jackson County, Illinois (Figure 1). Landcover types present at Burning Star include early- to late-successional forest (936 ha), agriculture (456 ha), wetland (66 ha), and restored tallgrass prairie (110 ha), as well as several freshwater lakes (223 ha). Fieldwork occurred on 10 restored prairie patches (hereafter “sites”) within Burning Star, which ranged in size from 1.8 to 35.9 ha (mean ± SD = 10.99 ± 10.57 ha). We conducted fieldwork in 2019, 2020, and 2021, from early May to late July of each year. Common grasses on prairie sites included warm-season natives such as big bluestem (Andropogon gerardii), Indian grass (Sorghastrum nutans), and switchgrass (Panicum virgatum), as well as non-native grasses such as smooth brome (Bromus inermis), Kentucky bluegrass (Poa pratensis), and foxtail (Setaria spp.). Common forbs included Canada goldenrod (Solidago canadensis), annual ragweed (Ambrosia artemisiifolia), and non-natives such as sericea lespedeza (Lespedeza cuneata) and sweet clover (Melilotus spp.). Management actions such as prescribed fire have been minimal at these sites since restoration was conducted shortly after 1992, resulting in the establishment and encroachment of woody shrubs such as eastern red cedar (Juniperus virginiana), blackberry (Rubus sp.), honey locust (Gleditsia triacanthos), and non-native autumn olive (Elaeagnus umbellata). Nest predators such as meso-mammals and snakes are common in our study area, and nest cameras deployed at Burning Star have identified North American racers (Coluber constrictor), prairie kingsnakes (Lampropeltis calligaster), and racoons (Procyon lotor) as the most common nest predators (Supplementary Table S1).
Figure 1. Map of Burning Star State Fish and Wildlife Area in Jackson County, IL, United States, where fieldwork for this study occurred. Inset on the top right shows the location of Burning Star in the state of Illinois.
We performed two rounds of vegetation structure surveys each field season from 2019 to 2021. The first round of surveys took place in mid-May, and the second round was in mid-July. For a full description of the vegetation survey methodology used in this study, see Glass and Eichholz (2021). Briefly, vegetation surveys were conducted along a series of sampling points in each grassland site. At each sampling point, we measured vegetation density and height using a Robel pole (Robel et al., 1970). We took Robel Pole readings at every sampling point from each cardinal direction, at a distance of 4 meters and a height of 1 meter (Fisher and Davis, 2010), and the four readings were averaged into one value to represent the sampling point. Vegetation height was considered the point of highest contact of vegetation on the Robel pole, while vegetation density was estimated by recording the lowest point on the Robel pole that could be seen through the vegetation. We also estimated the percent cover of woody vegetation within a 20 × 50 cm quadrat.
We searched for Field Sparrow nests at each site 10 times per field season (2019–2021), with every site being nest searched approximately once a week between 6 am and 10 am. A designated nest search area of approximately 3 ha was delineated within each site. For sites that were smaller than 3 ha, the entire site was the nest search area. We searched for nests using a modified version of the rope dragging method (Winter et al., 2003), where a rope is dragged between two observers, with a third observer walking approximately 5 meters behind the rope. Adult birds may flush off their nests due to disturbance from the rope, which can lead to their nests being discovered by observers. For our modified version, we used a looped rope so that two disturbances were caused in quick succession, to allow for greater nest detection. When rope dragging, we took care to avoid known nests, to minimize the number of times they were disturbed. Found nests were monitored every three to four days until they either fledged or failed. We determined nest age by floating eggs in a cup of water to estimate developmental stage (Westerskov, 1950). If nests were found in the nestling stage, we aged nestlings based on appearance (e.g., Jonsomjit et al., 2007, pp.9–14) and assumed a 12-day incubation length (Best, 1978) to determine when the nest was initiated.
We calculated the daily survival rate (DSR; Mayfield, 1975) of each Field Sparrow nest using the nest survival module in the RMark package (Laake, 2013) in Program R, which interfaces with Program Mark (White and Burnham, 1999). This module estimates DSR using methods described in Dinsmore et al. (2002). To calculate nest density, we used the following equation derived from Arnold et al. (2007):
where N = the number of Field Sparrow nests found in the nest search area, HA = the size of the nest search area (ha), DSR = the average DSR for all nests in the site, and d = the average nest age at first discovery. The DSRd term in the equation allowed us to account for nests that failed before they could be discovered by our field crews (McPherson et al., 2003; Arnold et al., 2007). However, this equation does not completely remove sources of bias, as the probability of flushing off a nest may vary among individual Field Sparrows. Nest density estimates were log-transformed prior to modeling, to improve normality and homogeneity of variance.
We estimated relative snake abundance among grassland sites using grids of coverboards that were checked once a week for the duration of each field season. We used 0.6 m2 plywood sheets for our coverboards, arranged in a grid of four parallel rows of five boards each, for a total of 20 boards per site. Rows were spaced 50 meters apart, and boards within rows were placed at 15-meter intervals. Snakes encountered under coverboards were identified to species. As the interest of snakes in this study is confined to their role as nest predators, we only considered snake species that are known predators of bird nests. In our study area, such species include the North American Racer (Coluber constrictor), Prairie Kingsnake (Lampropeltis calligaster), Black Kingsnake (Lampropeltis nigra), Common Garter Snake (Thamnophis sirtalis), and Black Ratsnake (Pantherophis obsoletus). We calculated a relative abundance index of snakes for each site by dividing the number of snakes encountered (all nest predator species combined) by the number of boards checked (Klug et al., 2009; Glass and Eichholz, 2022).
We estimated relative raccoon abundance among grassland sites using camera traps consisting of infra-red motion-triggered trail cameras (Cuddeback Long Range IR, model E2; Non Typical Inc., De Pere, WI, United States) placed approximately 0.5 m off the ground, and baited with a fatty acid scent disk (USDA APHIS Potacello Supply Depot, Potacello, ID, United States) and a can of sardines. We programmed cameras to operate day and night, taking two photos per trigger, with a one-minute pause between consecutive triggers. We stocked camera traps in each grassland site at a rate of one trap per 10 ha to minimize overlap between raccoon home ranges. A study that took place in a similar landscape and seasonal timeframe as ours (Barding and Nelson, 2008) estimated the core area of a raccoon home range at around 8 ha, though raccoon home ranges can vary by season, individual fitness, and landscape composition (Gehrt, 2003; Beasley et al., 2007). We placed the camera traps at random points within each site, generated using ArcMap 10.6 (ESRI, 2018). Camera trap locations were separated from each other by at least 300 meters. The tall and dense herbaceous vegetation in our grassland sites would have obscured raccoons from the camera viewshed and caused numerous false triggers, so we trimmed a 2 m2 area of vegetation to an approximately 5 cm uniform height in front of each camera to make raccoon detection possible. We then baited the center of each trimmed area to increase encounter probability within the narrow viewshed of the camera (43.6° angle, 2 m range). We moved the locations of the camera traps every two weeks to ensure even sampling throughout the site and prevent raccoons from adapting to the locations of the bait stations (DeVault et al., 2011), resulting in six different locations for each individual camera throughout the 12-week field season.
We calculated a relative abundance index of raccoons for each site using the number of raccoon occurrences per 24-h period that the camera was operating (O’Brien et al., 2003; Rovero and Marshall, 2009; O’Brien, 2011). Photos taken at the same camera within one hour were considered the same occurrence (Lucherini et al., 2009; Rovero and Marshall, 2009). For this index to be a valid indicator of abundance, detection probability among camera traps cannot vary across cameras or sites. Potential sources of variation in detection probability include using different camera setups, considering multiple species, and variation in environmental covariates relevant to the camera viewshed (Hofmeester et al., 2019). By keeping camera models and settings constant throughout the study and creating similar microenvironments for each camera trap (2 m2 trimmed patch of grassland in a randomly selected location), we were able to minimize variation in detection probability among cameras.
For a full description of small mammal trapping techniques and abundance estimates used in this study, see Glass and Eichholz (2021). Briefly, small mammals were captured at each site using a 10 × 10 grid of 100 Sherman traps (7.6 × 8.9 × 22.9 cm; H. B. Sherman Traps, Tallahassee, Florida), with traps evenly spaced at 10-meter intervals. Traps were baited with oats and supplied with a ball of polyester stuffing. Two trapping sessions occurred per year at each field site. Each session of trapping consisted of three consecutive trap nights, and traps were checked periodically throughout the day. Captured individuals were identified to species when possible, otherwise to genus, and marked with a unique ear tag.
We used Huggins P and C models in Program Mark (Huggins, 1989; White and Burnham, 1999) to estimate capture probabilities (p) and recapture probabilities (c) specific to each of the six trapping sessions (two sessions each for three years), to account for varying detection probability (= p in the context of our study). We then estimated small mammal abundance for each trapping session by dividing the number of individuals caught at each site by p specific to that session (Weldy et al., 2019). Small mammal abundance estimates for each year were calculated as the average abundance over the two trapping sessions. Abundance estimates were log-transformed prior to modeling, to meet the assumptions of linearity and normality.
Arthropod samples were collected at field sites once each month, for a total of three sampling events per field season. At each site, we set out two transects, each consisting of 10 arthropod pan traps (e.g., Lovell et al., 2007; Spafford and Lortie, 2013). Pan traps consisted of white, blue, yellow, or red 590-mL plastic bowls (diameter = 14.75 cm) that were filled halfway with water and a few drops of dish soap to reduce surface tension. Each pair of transects at a site (20 bowls total) contained five bowls of each color. Pan traps were placed on the ground at 10 m intervals within a transect, and transects were placed 10 m apart, running parallel to each other. Transects began at randomly selected locations at least 30 meters from an edge and were oriented parallel to the longest length of the site. Transect locations at each site remained constant across samples and years. Pan traps were left undisturbed for 24 h, after which arthropods were collected. Arthropod samples were stored in 91% ethanol until the conclusion of the field season. Arthropods were then individually identified to taxonomic order, dried at 50° Celsius for 48 h, and weighed (accuracy = 0.001 g) to determine dry biomass (g). For each site, we pooled all three samples within a year to determine total arthropod biomass. Before analysis, we truncated our arthropod dataset to include only orders that are present in Field Sparrow diets: Orthoptera, Lepidoptera, Araneae, Hemiptera, Coleoptera, Diptera, Odonata, and Hymenoptera (Evans, 1964; Best, 1977).
We used structural equation models (SEMs), a form of path analysis, to estimate how the effects of habitat structure on nest survival and nest density may be mediated through predator activity or arthropod biomass. SEMs are probabilistic models that combine multiple predictor and response variables into a single causal network (Grace et al., 2012; Lefcheck, 2016). This approach allows researchers to test models representing hypothetical direct and indirect relationships in a natural system. Relationships between variables in our SEMs are represented as unidirectional paths and are assumed to be causal, a standard practice for structural equation modeling (Grace et al., 2012; Lefcheck, 2016). SEMs must additionally meet the same assumptions inherent in traditional linear modeling approaches, such as normality, homogeneity of variance, and low kurtosis (Grace, 2008; Grace et al., 2012). The primary limitation associated with SEMs is that a large amount of data is needed compared to traditional linear models, especially as the number of causal relationships represented in an SEM increase. A small sample size both limits the ability of an SEM to find evidence for ecological relationships, and may limit the ability of SEM software to correctly identify adequate model fit (Lefcheck, 2016).
Five variables were used as predictors of Field Sparrow nest survival and nest density in our SEMs: snake abundance, raccoon abundance, small mammal abundance, arthropod biomass, and a composite habitat variable representing relevant Field Sparrow habitat characteristics across multiple spatial scales. Additionally, small mammal abundance was used as a predictor of nest predator abundance. We represented habitat as a composite variable (Grace and Bollen, 2008) because it allows us to represent the collective effects of multiple parameters as a single variable. Composite variables are often used in SEMs of natural systems to represent an aggregate or multi-faceted concept (e.g., Hopcraft et al., 2012; Lehmann et al., 2014; Blüthgen et al., 2016), such as wildlife habitat associations (Grace, 2008: Figure 5). We constructed two composite habitat variables, one each for the nest survival and nest density SEMs, using a linear combination of causal indicator variables representing habitat characteristics across multiple spatial scales that were demonstrated in a previous analysis of Field Sparrows at Burning Star (Supplementary Table S2) to be associated with Field Sparrow nest survival or nest density. The previous analysis considered habitat variables representing four spatial scales: nest site vegetation characteristics, within-patch vegetation characteristics, abiotic patch structure (patch size, shape, edge composition), and surrounding landscape composition (Supplementary Table S3). Nest-site scale variables present in the previous nest survival analysis were not considered for this study, as there is no a priori reason to suspect that nest-site characteristics influence patch-level predator abundance or arthropod biomass. Loadings for each indicator variable were based on standardized coefficients of each indicator’s association with nest density (for the nest density model) or DSR (for the nest survival model), resulting in composite variables with zero error variance (Grace and Bollen, 2005, 2008). The lack of error variance in composite variables is appropriate given that they are assumed to have no further error variance beyond the collective effects of their indicator components (Grace and Bollen, 2008; Blüthgen et al., 2016). The composite variable for the nest survival model (hereafter composite nest survival habitat) included grass cover, woody cover, and bare ground at the within-patch scale, and the proportion of road edge and agriculture edge around a site. The composite variable for the nest density model (hereafter composite nest density habitat) included woody cover at the within-patch scale and the proportion of tree edge around a site.
We built our SEMs using the piecewiseSEM package (Lefcheck, 2016) in RStudio version 2022.2.3.492 (RStudio Team, 2022). In piecewiseSEM, linear models representing different pathways are fitted individually, instead of estimating relationships between all variables simultaneously, which allows greater flexibility for individual model structures and more robust parameter estimates for limited datasets. For both Field Sparrow response variables (nest density and DSR), we began with a global model that contained all predicted relationships (Figure 2). These included a direct effect of habitat on Field Sparrows, arthropods, small mammals, snakes, and raccoons; a direct effect of arthropods on snakes; a direct effect of small mammals on snakes and raccoons; and a direct effect of arthropods, small mammals, snakes, and raccoons on Field Sparrows. We removed nonsignificant (p > 0.1) paths, then compared nested models using Akaike’s Information Criterion (AIC; Burnham and Anderson, 2002) to determine the best supported model for both nest density and nest survival. The paths in our SEMs consisted of generalized linear mixed models (GLMMs) created using the lme4 package (Bates et al., 2015) in RStudio. All paths included year as a random variable to account for unmodeled temporal variability in our data, and all paths except those involving nest survival used a Gaussian distribution and identity link. GLMMs with nest survival as the response variable used a binomial distribution and logit-link function. We assessed model fit using a Chi-square GOF test, with p > 0.1 indicating good model fit. The effect sizes of relationships among variables in SEMs were estimated using standardized regression coefficients (β) and are reported as the estimate ± standard error.
Figure 2. Global structural equation models containing all hypothesized direct relationships among variables that may affect nest survival (A) and nest density (B) of Field Sparrows (Spizella pusilla). Nest survival habitat (A) and nest density habitat (B) are composite variables comprised of habitat characteristics previously demonstrated to be associated with nest survival and nest density. The sign (+ or −) under each habitat characteristic represents the directional effect it has on the composite variable.
We found and monitored 97 Field Sparrow nests across three field seasons and 10 grassland sites. DSR for all Field Sparrow nests in our study was 0.9 ± 0.01 SE. Predation was the cause of all but two nest failures in this study. One nest was destroyed by a storm, and one other was abandoned after being partially depredated. Mean nest density was 3.23 ± 0.79 nests/ha, with values ranging from 0 to 17.18. We encountered 96 nest predator snakes of five different species during coverboard surveys, including 39 North American racers, 23 prairie kingsnakes, 17 black kingsnakes, 15 common garter snakes, and 2 black ratsnakes. Relative abundance indices for snakes (snakes/board) ranged from 0 to 0.4 (mean = 0.16 ± 0.02 SE). For our raccoon surveys, we deployed camera traps for 78 days in 2019, 81 days in 2020, and 80 days in 2021, and documented a total of 418 raccoon occurrences. Relative abundance indices for raccoons (raccoon occurrences/day) ranged from 0.01 to 0.26 (mean = 0.13 ± 0.01 SE). During the three years of this study, we collected 13,843 arthropods among the eight orders we considered (Supplementary Table S4). The total dry weight of arthropods collected was 42.448 grams. Hymenoptera was the most highly represented order (45% of total biomass), followed by Araneae (24% of total biomass). Arthropod biomass estimates for each site ranged from 0.526 to 2.931 g (mean = 1.41 ± 0.116 SE).
Our small mammal trapping efforts from 2019 to 2021 yielded 1,181 captures of 827 individuals, including 472 Peromyscus mice, 321 Microtus voles, 18 marsh rice rats (Oryzomys palustris), 6 Northern short-tailed shrews (Blarina brevicauda), 2 Eastern chipmunks (Tamias striatus), 2 meadow jumping mice (Zapus hudsonius), and 1 house mouse (Mus musculus). Capture estimates for two of the six trapping sessions (Session 2 in 2019 and Session 1 in 2020, see Supplementary Table S5 in Supplementary material) were highly imprecise, and resulted in unrealistic abundance estimates. We therefore disregarded abundance estimates from these trapping sessions for our SEMs. Instead, small mammal abundance values for 2019 were estimates from the first trapping session of that year, and abundance values for 2020 came from the second trapping session. Small mammal abundance values for 2021 were the average of abundance estimates between the first and second trapping sessions. Abundance estimates for our field sites ranged from 0.45 to 172.84 small mammals per ha (mean = 34.44 ± 7.91 SE).
The global model for nest density adequately fit our data (Fisher’s C = 5.53, p = 0.7, DF = 8). The nest survival global model was initially a poor fit (Fisher’s C = 15.058, p = 0.058, DF = 8), but model fit improved when the least informative variable, small mammal abundance, was dropped (Fisher’s C = 9.834, p = 0.132, DF = 6). For nest survival and nest density SEMs, the most supported model contained only the path between the composite habitat variable and the Field Sparrow response variable, indicating that the influences of habitat structure on Field Sparrow nest survival and nest density were primarily direct, and not mediated by arthropod biomass or predator abundance. The composite habitat variables for nest survival and nest density were significantly and positively related to Field Sparrow DSR (p = 0.045, β = 0.98 ± 0.49) and nest density (p < 0.01, β = 0.94 ± 0.26), respectively. Reducing the nest survival global model down to only significant paths (p < 0.1) revealed that composite nest survival habitat was negatively associated with snake abundance (p = 0.092, β = −0.35 ± 0.2) and raccoon abundance (p < 0.01, β = −0.54 ± 0.18), and positively associated with arthropod biomass (p = 0.015, β = 0.31 ± 0.13), though we detected no significant relationship between DSR and nest predator abundance or arthropod biomass (Figure 3). Our nest density SEM estimated a significant and positive relationship between composite nest density habitat and raccoon abundance (p = 0.076, β = 0.64 ± 0.34), but there was no significant relationship between raccoon abundance and Field Sparrow nest density (Figure 4).
Figure 3. Structural equation model comprised only of significant (p < 0.1) paths, examining direct and indirect effects of habitat characteristics on Field Sparrow daily nest survival. The regression coefficient (β) and marginal R2 value are shown for each path. The loading for each component of the composite habitat variable is listed above the component.
Figure 4. Structural equation model comprised only of significant (p < 0.1) paths, examining direct and indirect effects of habitat characteristics on Field Sparrow nest density. The regression coefficient (β) and marginal R2 value are shown for each path. The loading for each component of the composite habitat variable is listed above the component.
We sought with this study to examine whether the effects of habitat structure on Field Sparrows were mediated through predator abundance, alternative prey availability, or arthropod biomass. Field Sparrow nest density and survival rates at Burning Star were consistent with other studies on Field Sparrows from our region (McCoy et al., 2001; Galligan et al., 2006; Herakovich et al., 2021), with nest density generally being higher in smaller, shrubbier grasslands and lower in larger grasslands with a reduced shrub component. Of the predictions we tested, our results best support the concept of habitat structure primarily influencing Field Sparrow nest survival and nest density directly, as we found no evidence for an effect any predictor variable besides composite habitat on our Field Sparrow response variables. Composite nest survival habitat was associated with reduced abundance of both raccoons and snakes, and an increase in arthropod biomass, although our models did not detect a relationship between nest survival and predator abundance or arthropod biomass. It is possible that these relationships exist but the relatively small sample size of our dataset lacked the power to detect them. Alternatively, the precision in our arthropod and predator estimates is likely much lower than that of our habitat variable estimates, and may have been inadequate for our models to predict a relationship between arthropods or predators and nest survival. There are also other ways that habitat characteristics may indirectly affect avian nest survival and density that were not examined in this study, such as the abundance of competitors (Fretwell and Lucas, 1970) and conspecifics (Ahlering and Faaborg, 2006; Andrews et al., 2015). Nest predation may be density dependent in some systems, where predation rates are positively associated with nest density due to increased predator activity (e.g., Shitikov et al., 2018). We did not examine a relationship between nest density and nest survival in this study because we use DSR to calculate nest density, so the two metrics are not independent.
It is well established that avoiding predation and finding food are important aspects of nest survival for grassland birds, and several studies have linked various metrics of avian nest success to arthropod biomass (Duguay et al., 2000; Boulton et al., 2008; Richmond et al., 2011) and predator abundance (Rogers and Caro, 1998; Patten and Bolger, 2003; Beja et al., 2013). However, the relationships between predators, arthropods, and birds are complex and can vary among bird species and geographic regions (Chalfoun et al., 2002; Kelly et al., 2017). In many cases, micro and macro habitat characteristics, as well as adult bird behavior, may have a greater impact on predator avoidance and food provisioning than predator and arthropod abundance. For example, habitat characteristics such as vegetation density and the amount of bare ground may influence foraging efficiency for birds (Whittingham and Markland, 2002; Butler and Gillings, 2004), which can have a stronger impact on food provisioning rates than arthropod biomass or abundance (Tremblay et al., 2005; Zalik and Strong, 2008). Bare ground was a positive contributor to the nest survival composite habitat variable, thus increased foraging efficiency resulting from habitat structure may be a relevant contributor to nest survival for Field Sparrows in our study. Additionally, adult birds can often compensate for any reductions in local food availability by increasing foraging effort (Adams et al., 1994; Zalik and Strong, 2008), which minimizes the impact that variation in arthropod biomass can have on nest success.
Just as foraging success may not covary with arthropod abundance, nest predation rates may not directly relate to predator abundance, despite the widespread assumption that this is the case. Results from studies examining a relationship between predator abundance and nest survival are inconsistent, as multiple studies across various habitat types and bird taxa found no evidence for such a relationship (Schwemm and Martin, 2005; Boulton et al., 2008; Richmond et al., 2011; Hollander et al., 2015). Instead, predation rates for these systems may primarily be influenced by other factors, such as adult behavior (Fontaine and Martin, 2006; Lima, 2009), or seasonal changes in predator behavior (Sperry et al., 2008; Benson et al., 2010). Alternative prey availability can have a strong impact on nest predation (Ackerman, 2002; Schmidt et al., 2008; Nordberg and Schwarzkopf, 2019), though our results suggest that small mammals are not a major driver of nest survival in this system. Habitat structure around the nest site directly affects nest survival and predation rates by influencing visual and olfactory nest concealment from predators, as well as determining nest microclimate (Farnsworth and Simons, 1999; Flaspohler et al., 2000; Lomas et al., 2014; Grisham et al., 2016). The habitat characteristics that increased nest survival in this study were associated with fewer predators and more arthropods, even though predator abundance and arthropod biomass did not in turn influence nest survival. This may indicate that predators and arthropods are important aspects of nest survival at Burning Star, but nest survival does not directly respond to predator abundance and arthropod biomass per se. Instead, nest survival may be impacted by other measures of predator activity and prey availability that relate weakly to predator abundance and arthropod biomass but were not measured in this study, such as predator nest-searching effort or efficiency of arthropod foraging by adult birds.
Our results were consistent with our prediction that the factors affecting nest density and nest success would be decoupled in our study area. Although the nest survival and nest density model results both suggested a primarily direct effect of habitat structure, composite nest density habitat was positively, instead of negatively, associated with raccoon abundance. Additionally, the characteristics associated with composite nest density habitat were different from those associated with composite nest success habitat. Because nest site selection for birds is an adaptive process (Martin, 1993, 1998), birds should generally have evolved to select nest sites with characteristics that promote greater nest survival, such as areas where nest predators are less abundant (Morton, 2005; Fontaine and Martin, 2006; Eichholz et al., 2012; Bonnington et al., 2015). However, several factors can lead to a decoupling of nest survival and nest density, including perceived predation risk not being reflective of true predation risk (e.g., Renfrew et al., 2005; Vernouillet et al., 2020), and dominant individuals forcing others into more marginal habitat (Fretwell and Lucas, 1970; Van Horne, 1983; Pulliam, 1988). Behavioral dominance and interspecific competition are high in some Field Sparrow populations (Fretwell, 1968), so dominant individuals may have prevented others from establishing territories in high quality nesting habitats at Burning Star, resulting in nest density increasing in less preferred habitat, such as that associated with greater predator activity. The two components that comprise composite nest density habitat, woody cover and tree edge, are associated with nest predators such as meso-mammals (Beasley et al., 2007; Barding and Nelson, 2008; Beatty et al., 2014) and snakes (Richardson et al., 2006; Klug et al., 2010; Martino et al., 2012). However, Field Sparrow associations with woody cover and forest edges are consistent with previous research (Lanyon, 1981; Herkert, 1991; Dechant et al., 2002), as Field Sparrows frequently use shrubs and saplings for nesting substrates, as well as song perches and roosting areas. Preferential selection of these habitat characteristics suggests that they were once advantageous for nest survival (Chalfoun and Schmidt, 2012). However, the midwestern landscape has changed dramatically in the past century (Herkert, 1994; Briggs et al., 2005), resulting in a rapid increase of raccoon activity in Illinois grasslands (Gehrt et al., 2002; Schmidt, 2002). Field Sparrows in our area may therefore be preferentially nesting in patches with habitat characteristics that no longer promote nest survival. The possibility that some midwestern grasslands may become ecological traps for certain bird species due to changes in landscape and grassland structure has been a concern of ecologists for several decades (Herkert, 1994; Stallman and Best, 1996; Heske et al., 1999; Shochat et al., 2005). Whether Burning Star is acting as an ecological trap for Field Sparrows cannot be fully determined with this study, as factors other than nest survival, such as fledgling survival and female fecundity, also influence the extent to which an area acts as an ecological trap (Demeyrier et al., 2016; Hale and Swearer, 2016).
Ultimately, we found no evidence that the impacts of habitat structure on Field Sparrows are mediated by predator abundance or arthropod biomass in our study area. Instead, habitat characteristics may primarily impact nest survival rates through other means, such as determining nest concealment and microclimate, and influencing foraging efficiency. Land managers and wildlife conservationists working to improve habitat for bird communities should not assume that quality bird habitat is directly related to arthropod biomass or predator abundance, but should instead determine which drivers are impacting nest survival and nest density in their system. However, in the event that such information is lacking, encouraging habitat characteristics that are associated with reduced predator abundance and greater arthropod biomass may improve avian nest survival even when predator abundance and arthropod biomass are not the primary determinants of predation risk and food availability, as was the case here. The influential habitat characteristics that we identified here are not universal, as they may depend on the management history, wildlife communities, spatial mosaic patterns, and regional location of a given grassland system. We therefore encourage managers and decision-makers to be familiar with habitat characteristics associated with grassland nest predators and arthropods in their area. Our study also provides an example of nest survival and nest density responding to different habitat characteristics, so managers and researchers should avoid determining habitat quality by nest density or bird abundance alone. To address some of the caveats present in this study, we suggest that future research into this topic employ a larger dataset and consider other mechanisms through which habitat structure may indirectly affect bird communities, such as the presence or abundance of interspecific competitors and conspecifics, and density dependent nest success.
The raw data supporting the conclusions of this article will be made available by the authors, without undue reservation.
The animal study was reviewed and approved by Southern Illinois University Carbondale Institutional Animal Care and Use Committee.
AG contributed to study design, gathered field data, conducted statistical analyses, and wrote the manuscript. ME contributed to study design, edited the manuscript, and secured research funding. All authors contributed to the article and approved the submitted version.
This research was funded by the Illinois Department of Natural Resources via Federal Wildlife Aid Grant W-106-R, and by the Illinois Ornithological Society Grants Program.
The authors would like to thank C. Crawford, B. Baum, and the many field technicians who have assisted with research at Burning Star, for the immense amount of help they provided in the field.
The authors declare that the research was conducted in the absence of any commercial or financial relationships that could be construed as a potential conflict of interest.
All claims expressed in this article are solely those of the authors and do not necessarily represent those of their affiliated organizations, or those of the publisher, the editors and the reviewers. Any product that may be evaluated in this article, or claim that may be made by its manufacturer, is not guaranteed or endorsed by the publisher.
The Supplementary material for this article can be found online at: https://www.frontiersin.org/articles/10.3389/fevo.2023.1094152/full#supplementary-material
Ackerman, J. T. (2002). Of mice and mallards: positive indirect effects of coexisting prey on waterfowl nest success. Oikos 99, 469–480. doi: 10.1034/j.1600-0706.2002.11802.x
Adams, J. S., Knight, R. L., McEwen, L. C., and George, T. L. (1994). Survival and growth of nestling vesper sparrows exposed to experimental food reductions. Condor 96, 739–748. doi: 10.2307/1369477
Ahlering, M. A., and Faaborg, J. (2006). Avian habitat management meets conspecific attraction: if you build it, will they come? Auk 123, 301–312. doi: 10.1093/auk/123.2.301
Andrews, J. E., Brawn, J. D., and Ward, M. P. (2015). When to use social cues: conspecific attraction at newly created grasslands. Condor 117, 297–305. doi: 10.1650/CONDOR-14-172.1
Arnold, T. W., Craig-Moore, L. M., Armstrong, L. M., Howerter, D. W., Devries, J. H., Joynt, B. L., et al. (2007). Waterfowl use of dense nesting cover in the Canadian parklands. J. Wildl. Manag. 71, 2542–2549. doi: 10.2193/2005-568
Barding, E. E., and Nelson, T. A. (2008). Raccoons use habitat edges in northern Illinois. Am. Midl. Nat. 159, 394–402. doi: 10.1674/0003-0031(2008)159[394:RUHEIN]2.0.CO;2
Bates, D., Maechler, M., Bolker, B., and Walker, S. (2015). Fitting linear mixed-effects models using lme4. J. Stat. Softw. 67, 1–48. doi: 10.18637/jss.v067.i01
Beasley, J. C., DeVault, T. L., and Rhodes, O. E. Jr. (2007). Home-range attributes of raccoons in a fragmented agricultural region of northern Indiana. J. Wildl. Manag. 71, 844–850. doi: 10.2193/2006-022
Beatty, W. S., Beasley, J. C., and Rhodes, O. E. Jr. (2014). Habitat selection by a generalist mesopredator near its historical range boundary. Can. J. Zool. 92, 41–48. doi: 10.1139/cjz-2013-0225
Beja, P., Schindler, S., Santana, J., Porto, M., Morgado, R., Moreira, F., et al. (2013). Predators and livestock reduce bird nest survival in intensive Mediterranean farmland. Eur. J. Wildl. Res. 60, 249–258. doi: 10.1007/s10344-013-0773-0
Benson, T. J., Brown, J. D., and Bednarz, J. C. (2010). Identifying predators clarifies predictors of nest success in a temperate passerine. J. Anim. Ecol. 79, 225–234. doi: 10.1111/j.1365-2656.2009.01604.x
Benson, T. J., Chiavacci, S. J., and Ward, M. P. (2013). Patch size and edge proximity are useful predictors of brood parasitism but not nest survival of grassland birds. Ecol. Appl. 23, 879–887. doi: 10.1890/12-1101.1
Best, L. B. (1977). Nestling biology of the field sparrow. Auk 94, 308–319. doi: 10.1093/auk/94.2.308
Best, L. B. (1978). Field sparrow reproductive success and nesting ecology. Auk 95, 9–22. doi: 10.2307/4085491
Blüthgen, N., Simons, N. K., Jung, K., Prati, D., Renner, S. C., Boch, S., et al. (2016). Land use imperils plant and animal community stability through changes in asynchrony rather than diversity. Nat. Commun. 7:10697. doi: 10.1038/ncomms10697
Bonnington, C., Gaston, K. J., and Evans, K. L. (2015). Ecological traps and behavioural adjustments of urban songbirds to fine-scale spatial variation in predator activity. Anim. Conserv. 18, 529–538. doi: 10.1111/acv.12206
Boulton, R. L., Richard, Y., and Armstrong, D. P. (2008). Influence of food availability, predator density, and forest fragmentation on nest survival of New Zealand robins. Biol. Conserv. 141, 580–589. doi: 10.1016/j.biocon.2007.12.007
Bradbury, R. B., Hill, R. A., Mason, D. C., Hinsley, S. A., Wilson, J. D., Balzter, H., et al. (2005). Modelling relationships between birds and vegetation structure using airborne LiDAR data: a review with case studies from agricultural and woodland environments. Ibis 147, 443–452. doi: 10.1111/j.1474-919x.2005.00438.x
Brennan, L. A., and Kuvlesky, W. P. Jr. (2005). North American grassland birds: an unfolding conservation crisis? J. Wildl. Manag. 69, 1–13. doi: 10.2193/0022-541X(2005)069<0001:NAGBAU>2.0.CO;2
Briggs, J. M., Knapp, A. K., Blair, J. M., Heisler, J. L., Hoch, G. A., Lett, M. S., et al. (2005). An ecosystem in transition: causes and consequences of the conversion of Mesic grassland to shrubland. Bioscience 55, 243–254. doi: 10.1641/0006-3568(2005)055[0243:AEITCA]2.0.CO;2
Burnham, K. P., and Anderson, D. R. (2002). Model Selection and Multimodel Inference: A Practical Information-Theoretic Approach. 2nd Edn. New York: Springer.
Butler, S. J., and Gillings, S. (2004). Quantifying the effects of habitat structure on prey detectability and accessibility to farmland birds. Ibis 146, 123–130. doi: 10.1111/j.1474-919X.2004.00352.x
Chalfoun, A. D., and Schmidt, K. A. (2012). Adaptive breeding-habitat selection: is it for the birds? Auk 129, 589–599. doi: 10.1525/auk.2012.129.4.589
Chalfoun, A. D., Thompson, F. R. III, and Ratnaswamy, M. J. (2002). Nest predators and fragmentation: a review and meta-analysis. Conserv. Biol. 16, 306–318. doi: 10.1046/j.1523-1739.2002.00308.x
Davis, S. K. (2005). Nest-site selection patterns and the influence of vegetation on nest survival of mixed-grass prairie passerines. Condor 107, 605–616. doi: 10.1093/condor/107.3.605
Dechant, J. A., Sondreal, M. L., Johnson, D. H., Igl, L. D., Goldade, C. M., Parkin, B. D., et al. (2002). Effects of Management Practices on Grassland Birds: Field Sparrow. USGS Northern Prairie Wildlife Research Center: North Dakota. 148.
DeGregorio, B. A., Chiavacci, S. J., Weatherhead, P. J., Willson, J. D., Benson, T. J., and Sperry, J. H. (2014). Snake predation on north American bird nests: culprits, patterns, and future directions. J. Avian Biol. 45, 325–333. doi: 10.1111/jav.00364
Demeyrier, V., Lambrechts, M. M., Perret, P., and Grégoire, A. (2016). Experimental demonstration of an ecological trap for a wild bird in a human-transformed environment. Anim. Behav. 118, 181–190. doi: 10.1016/j.anbehav.2016.06.007
DeVault, T. L., Olson, Z. H., Beasley, J. C., and Rhodes, O. E. Jr. (2011). Mesopredators dominate competition for carrion in an agricultural landscape. Basic Appl. Ecol. 12, 268–274. doi: 10.1016/j.baae.2011.02.008
Dinsmore, S. J., White, G. C., and Knopf, F. L. (2002). Advanced techniques for modeling avian nest survival. Ecology 83, 3476–3488. doi: 10.1890/0012-9658(2002)083[3476:ATFMAN]2.0.CO;2
Duguay, J. P., Wood, P. B., and Miller, G. W. (2000). Effects of timber harvests on invertebrate biomass and avian nest success. Wildlife Soc. B. 28, 1123–1131.
Eggers, S., Griesser, M., Andersson, T., and Ekman, J. (2005). Nest predation and habitat change interact to influence Siberian jay numbers. Oikos 111, 150–158. doi: 10.1111/j.0030-1299.2005.13802.x
Eichholz, M. W., Dassow, J. A., Weatherhead, P. J., and Stafford, J. D. (2012). Experimental evidence that nesting ducks use mammalian urine to assess predator abundance. Auk 129, 638–644. doi: 10.1525/auk.2012.12040
Eichholz, M. W., and Koenig, W. (1992). Gopher snake attraction to birds' nests. Southwest. Nat. 37, 293–299. doi: 10.2307/3671872
Ellison, K. S., and Ribic, C. A. (2012). Nest Defense- Grassland Bird Responses to Snakes. North Dakota: USGS Northern Prairie Wildlife Research Center. 252.
Ellison, K. S., Ribic, C. A., Sample, D. W., Fawcett, M. J., and Dadisman, J. D. (2013). Impacts of tree rows on grassland birds and potential nest predators: a removal experiment. PLoS One 8:e59151. doi: 10.1371/journal.pone.0059151
Evans, F. C. (1964). The food of vesper, field and chipping sparrows nesting in an abandoned field in southeastern Michigan. Am. Midl. Nat. 72, 57–75. doi: 10.2307/2423210
Farnsworth, G. L., and Simons, T. R. (1999). Factors affecting nesting success of wood thrushes in great Smokey Mountains National Park. Auk 116, 1075–1082. doi: 10.2307/4089686
Feeley, K. J., and Terborgh, J. W. (2008). Direct versus indirect effects of habitat reduction on the loss of avian species from tropical forest fragments. Anim. Conserv. 11, 353–360. doi: 10.1111/j.1469-1795.2008.00182.x
Fisher, R. J., and Davis, S. K. (2010). From Wiens to Robel: a review of grassland bird habitat selection. J. Wildl. Manag. 74, 265–273. doi: 10.2193/2009-020
Flaspohler, D. J., Temple, S. A., and Rosenfield, R. N. (2000). Relationship between nest success and concealment in two ground-nesting passerines. J. Field Ornithol. 71, 736–747. doi: 10.1648/0273-8570-71.4.736
Fontaine, J. J., and Martin, T. E. (2006). Habitat selection responses of parents to offspring predation risk: an experimental test. Am. Nat. 168, 811–818. doi: 10.1086/508297
Fretwell, S. D. (1968). Habitat distribution and survival in the field sparrow (Spizella pusilla). Bird-Banding 39, 293–306. doi: 10.2307/4511515
Fretwell, S. D., and Lucas, H. L. Jr. (1970). On territorial behavior and other factors influencing habitat distributions in birds. Acta Biotheor. 19, 16–36. doi: 10.1007/BF01601953
Galligan, E. W., DeVault, T. L., and Lima, S. L. (2006). Nesting success of grassland and savanna birds on reclaimed surface coal mines of the midwestern United States. Wilson J. Ornithol. 118, 537–546. doi: 10.1676/05-086.1
Gehrt, S. D. (2003). “Raccoon” in Wild Mammals of North America: Biology, Management, and Conservation. eds. G. A. Feldhamer, B. C. Thompson, and J. A. Chapman (Baltimore, MD: Johns Hopkins University Press), 611–634.
Gehrt, S. D., Hubert, G. F., and Ellis, J. A. (2002). Long-term population trends of raccoons in Illinois. Wildl. Soc. Bull. 30, 457–463.
Glass, A., and Eichholz, M. W. (2021). Habitat associations of small mammal communities in a restored prairie system in southern Illinois. J. Mammal. 102, 789–801. doi: 10.1093/jmammal/gyab002
Glass, A., and Eichholz, M. W. (2022). Snakes on the plains: the impacts of habitat structure on snake communities in Illinois grasslands. Wildl. Soc. Bull. 46:1366. doi: 10.1002/wsb.1366
Grace, J. B. (2008). Structural equation modeling for observational studies. J. Wildl. Manag. 72, 14–22. doi: 10.2193/2007-307
Grace, J. B., and Bollen, K. A. (2005). Interpreting the results from multiple regression and structural equation models. Bull. Ecol. Soc. Am. 86, 283–295. doi: 10.1890/0012-9623(2005)86[283:ITRFMR]2.0.CO;2
Grace, J. B., and Bollen, K. A. (2008). Representing general theoretical concepts in structural equation models: the role of composite variables. Environ. Ecol. Stat. 15, 191–213. doi: 10.1007/s10651-007-0047-7
Grace, J. B., Schoolmaster, D. R. Jr., Guntenspergen, G. R., Little, A. M., Mitchell, B. R., Miller, K. M., et al. (2012). Guidelines for a graph-theoretic implementation of structural equation modeling. Ecosphere 3:art73. doi: 10.1890/ES12-00048.1
Grisham, B. A., Godar, A. J., Boal, C. W., and Haukos, D. A. (2016). Interactive effects between nest microclimate and nest vegetation structure confirm microclimate thresholds for lesser prairie-chicken nest survival. Condor 118, 728–746. doi: 10.1650/CONDOR-16-38.1
Haffele, R. D., Eichholz, M. W., and Dixon, C. S. (2013). Duck productivity in restored species-rich native and species-poor non-native plantings. PLoS One 8:e68603. doi: 10.1371/journal.pone.0068603
Hale, R., and Swearer, S. E. (2016). Ecological traps: current evidence and future directions. Proc. Royal Soc. B 283:20152647. doi: 10.1098/rspb.2015.2647
Hamer, T. L., Flather, C. H., and Noon, B. R. (2006). Factors associated with grassland bird species richness: the relative roles of grassland area, landscape structure, and prey. Landsc. Ecol. 21, 569–583. doi: 10.1007/s10980-005-2167-5
Herakovich, H., Whelan, C. J., Barber, N. A., and Jones, H. P. (2021). Impacts of a recent bison reintroduction on grassland bird nests and potential mechanisms for these effects. Nat. Areas J. 41, 93–103. doi: 10.3375/043.041.0204
Herkert, J. R. (1991). An ecological study of the breeding birds of grassland habitats within Illinois. [dissertation]. Urbana (IL): University of Illinois.
Herkert, J. R. (1994). The effects of habitat fragmentation on midwestern bird communities. Ecol. Appl. 4, 461–471. doi: 10.2307/1941950
Herkert, J. R. (1995). An analysis of Midwestern breeding bird population trends: 1966-1993. Am. Midl. Nat. 134, 41–50. doi: 10.2307/2426481
Herkert, J. R. (2002). Effects of Management Practices on Grassland Birds: Henslow’s Sparrow. Northern Prairie Wildlife Research Center: Jamestown, ND.
Heske, E. J., Robinson, S. K., and Brawn, J. D. (1999). Predator activity and predation on songbird nests on forest-field edges in east-central Illinois. Landsc. Ecol. 14, 345–354. doi: 10.1023/A:1008033603827
Hickman, K. R., Farley, G. H., Channell, R., and Steier, J. E. (2006). Effects of Old World bluestem (Bothriochloa ischaemum) on food availability and avian community composition within the mixed-grass prairie. Southwest. Nat. 51, 524–530. doi: 10.1894/0038-4909(2006)51[524:EOOWBB]2.0.CO;2
Hill, J. M., Egan, J. F., Stauffer, G. E., and Diefenbach, D. R. (2014). Habitat availability is a more plausible explanation than insecticide acute toxicity for U. S. Grassland bird species declines. PLoS One 9:e98064. doi: 10.1371/journal.pone.0098064
Hirsch-Jacobson, R., Cox, W. A., Tewes, E. E., Thompson, F. R. III, and Faaborg, J. (2012). Parents or predators: examining intraseasonal variation in nest survival for a migratory passerine. Condor 114, 358–364. doi: 10.1525/cond.2012.110057
Hoekman, S. T., Ball, I. J., and Fondell, T. F. (2002). Grassland birds orient nests relative to nearby vegetation. Wilson Bull. 114, 450–456. doi: 10.1676/0043-5643(2002)114[0450:GBONRT]2.0.CO;2
Hofmeester, T. R., Cromsigt, J. P. G. M., Odden, J., Andren, H., Kindberg, J., and Linnell, J. D. C. (2019). Framing pictures: a conceptual framework to identify and correct for biases in detection probability of camera traps enabling multi-species comparison. Ecol. Evol. 9, 2320–2336. doi: 10.1002/ece3.4878
Hollander, F. A., van Dyck, H., San Martin, G., and Titeux, N. (2015). Nest predation deviates from nest predator abundance in an ecologically trapped bird. PLoS One 10:e0144098. doi: 10.1371/journal.pone.0144098
Hopcraft, J. G. C., Anderson, T. M., Pérez-Vila, S., Mayemba, E., and Olff, H. (2012). Body size and the division of niche space: food and predation differentially shape the distribution of Serengeti grazers. J. Anim. Ecol. 81, 201–213. doi: 10.1111/j.1365-2656.2011.01885.x
Huggins, R. M. (1989). On the statistical analysis of capture experiments. Biometrika 76, 133–140. doi: 10.1093/biomet/76.1.133
Hull, S. D. (2002). Effects of Management Practices on Grassland Birds: Eastern Meadowlark. Northern Prairie Wildlife Research Center: Jamestown, ND.
Jensen, W. E., and Finck, E. J. (2004). Edge effects on nesting Dickcissels (Spiza americana) in relation to edge type of remnant tallgrass prairie in Kansas. Am. Midl. Nat. 151, 192–199. doi: 10.1674/0003-0031(2004)151[0192:EEONDS]2.0.CO;2
Johnson, M. D. (2007). Measuring habitat quality: a review. Condor 109, 489–504. doi: 10.1093/condor/109.3.489
Johnson, D. H., and Schwartz, M. D. (1993). The conservation reserve program and grassland birds. Conserv. Biol. 7, 934–937. doi: 10.1046/j.1523-1739.1993.740934.x
Johnson, R. G., and Temple, S. A. (1990). Nest predation and brood parasitism of tallgrass prairie birds. J. Wildl. Manag. 54, 106–111. doi: 10.2307/3808909
Jonsomjit, D. A., Jones, S. L., Gardali, T., Geupel, G. R., and Gouse, P. J. (2007). A Guide to Nestling Development and Aging in Altricial Passerines: Biological Technical Publication. U.S Department of Interior Fish and Wildlife Service, Washington.
Karr, J. R., Dionne, M., and Schlosser, I. J. (1992). “Bottom-up vs. top-down regulation of vertebrate populations: lessons from birds and fish” in Effects of resource distribution on animal-plant interactions. eds. M. D. Hunter, T. Ohgushi, and P. W. Price (San Diego, CA: Academic Press), 243–286.
Kaspari, M., and Joern, A. (1993). Prey choice by three insectivorous grassland birds: reevaluation opportunism. Oikos 68, 414–430. doi: 10.2307/3544909
Kelly, J. K., Schmidt, K. A., and Ostfeld, R. S. (2017). Not all nesting guild members are alike: nest predators and conspecific abundance differentially influence nest survival in the ground-nesting ovenbird (Seiurus aurocapilla) and Veery (Catharus fuscescens). Wilson J. Ornithol. 129, 112–121. doi: 10.1676/1559-4491-129.1.112
Klimstra, W. D. (1959). Foods of the racer, Coluber constrictor, in southern Illinois. Copeia 1959, 210–214. doi: 10.2307/1440390
Klug, P. E., Jackrel, S. L., and With, K. A. (2010). Linking snake habitat use to nest predation risk in grassland birds: the dangers of shrub cover. Oecologia 162, 803–813. doi: 10.1007/s00442-009-1549-9
Klug, P. E., Wolfenbarger, L. L., and McCarty, J. P. (2009). The nest predator community of grassland birds responds to agroecosystem habitat at multiple scales. Ecography 32, 973–982. doi: 10.1111/j.1600-0587.2009.05857.x
Laake, J. L. (2013). RMark: An R Interface for Analysis of Capture-Recapture Data with MARK. AFSC Processed Rep 2013–01. Seattle, WA: Alaska Fisheries Science Center, NOAA, National Marine Fisheries Service.
Lanyon, W. E. (1981). Breeding birds and old field succession on fallow Long Island farmland. Bull. Am. Mus. Nat. Hist. 168, 1–60.
Lefcheck, J. S. (2016). piecewiseSEM: piecewise structural equation modeling in R for ecology, evolution, and systematics. Methods Ecol. Evol. 7, 573–579. doi: 10.1111/2041-210X.12512
Lehmann, C. E. R., Anderson, T. M., Sankaran, M., Higgins, S. I., Archibald, S., Hoffmann, W. A., et al. (2014). Savanna vegetation-fire-climate relationships differ among continents. Science 343, 548–552. doi: 10.1126/science.1247355
Lima, S. L. (2009). Predators and the breeding bird: behavioral and reproductive flexibility under the risk of predation. Biol. Rev. 84, 485–513. doi: 10.1111/j.1469-185X.2009.00085.x
Lomas, S. C., Whisson, D. A., Maguire, G. S., Tan, L. X., Guay, P.-J., and Weston, M. A. (2014). The influence of cover on nesting red-capped plovers: a trade-off between thermoregulation and predation risk? Vic. Nat. 131, 115–127.
Londe, D. W., Elmore, R. D., Davis, C. A., Fuhlendorf, S. D., Hovick, T. J., Lutbeg, B., et al. (2021). Fine-scale habitat selection limits trade-offs between foraging and temperature in a grassland bird. Behav. Ecol. 32, 625–637. doi: 10.1093/beheco/arab012
Loss, S. R., and Blair, R. B. (2011). Reduced density and nest survival of ground-nesting songbirds relative to earthworm invasions in northern hardwood forests. Conserv. Biol. 25, 983–992. doi: 10.1111/j.1523-1739.2011.01719.x
Lovell, S., Hamer, M., Slotow, R., and Herbert, D. (2007). Assessment of congruency across arthropod taxa and taxonomic levels to identify potential surrogates. Biol. Conserv. 139, 113–125. doi: 10.1016/j.biocon.2007.06.008
Lucherini, M., Reppucci, J. I., Walker, R. S., Villalba, M. L., Wurstten, A., Gallardo, G., et al. (2009). Activity pattern segregation of carnivores in the high Andes. J. Mammal. 90, 1404–1409. doi: 10.1644/09-MAMM-A-002R.1
Lyons, T. P., Miller, J. R., Debinski, D. M., and Engle, D. M. (2015). Predator identity influences the effect of habitat management on nest predation. Ecol. Appl. 25, 1596–1605. doi: 10.1890/14-1641.1
Madden, E. M., Murphy, R. K., Hansen, A. J., and Murray, L. (2000). Models for guiding management of prairie bird habitat in northwestern North Dakota. Am. Midl. Nat. 144, 377–392. doi: 10.1674/0003-0031(2000)144[0377:MFGMOP]2.0.CO;2
Martin, T. E. (1998). Are microhabitat preferences of coexisting species under selection and adaptive? Ecology 79, 656–670. doi: 10.1890/0012-9658(1998)079[0656:AMPOCS]2.0.CO;2
Martino, J. A., Poulin, R. G., Parker, D. L., and Somers, C. M. (2012). Habitat selection by grassland snakes at northern range limits: implications for conservation. J. Wildl. Manag. 76, 759–767. doi: 10.1002/jwmg.313
McCoy, T. D., Ryan, M. R., and Burger, L. W. (2001). Grassland bird conservation: CP1 vs. CP2 plantings in conservation reserve program fields in Missouri. Am. Midl. Nat. 145, 1–17. doi: 10.1674/0003-0031(2001)145[0001:GBCCVC]2.0.CO;2
McIntyre, N. E., and Thompson, T. R. (2003). A comparison of conservation reserve program habitat plantings with respect to arthropod prey for grassland birds. Am. Midl. Nat. 150, 291–301. doi: 10.1674/0003-0031(2003)150[0291:ACOCRP]2.0.CO;2
McPherson, R. J., Arnold, T. W., Armstrong, L. M., and Schwarz, C. J. (2003). Estimating the nest-success rate and the number of nests initiated by radiomarked mallards. J. Wildl. Manag. 67, 843–851. doi: 10.2307/3802691
Monroe, A. P., Chandler, R. B., Burger, L. W. Jr., and Martin, J. A. (2016). Converting exotic forages to native warm-season grass can increase avian productivity in beef production systems. Agric. Ecosyst. Environ. 233, 85–93. doi: 10.1016/j.agee.2016.08.024
Morton, E. S. (2005). Predation and variation in breeding habitat use in the ovenbird, with special reference to breeding habitat selection in northwestern Pennsylvania. Wilson Bull. 117, 327–335. doi: 10.1676/04-135.1
Murdoch, W. W. (1969). Switching in general predators: experiments on predator specificity and stability of prey populations. Ecol. Monogr. 39, 335–354. doi: 10.2307/1942352
Nelson, H. (2001). Impact of predation on avian recruitment: an introduction. Wildl. Soc. Bull. 9, 2–5.
Nelson, K. J., and Martin, K. (1999). Thermal aspects of nest-site location for vesper sparrows and horned larks in British Columbia. Stud. Avian Biol. 19, 137–143.
Nordberg, E. J., and Schwarzkopf, L. (2019). Predation risk is a function of alternative prey availability rather than predator abundance in a tropical savanna woodland ecosystem. Sci. Rep. 9:7718. doi: 10.1038/s41598-019-44159-6
O’Brien, T. G. (2011). “Abundance, density, and relative abundance: a conceptual framework” in Camera Traps in Animal Ecology. eds. A. F. O’Connell, J. D. Nichols, and K. U. Karanth (New York: Springer), 71–96.
O’Brien, T. G., Kinniard, M. F., and Wibisono, H. T. (2003). Crouching tigers, hidden prey: Sumatran tiger and prey populations in a tropical forest landscape. Anim. Conserv. 6, 131–139. doi: 10.1017/S1367943003003172
Patten, M., and Bolger, D. T. (2003). Variation in top-down control of avian reproductive success across a fragmentation gradient. Oikos 101, 479–488. doi: 10.1034/j.1600-0706.2003.12515.x
Pulliam, H. R. (1988). Sources, sinks, and population regulation. Am. Nat. 132, 652–661. doi: 10.1086/284880
Pulliam, J. P., Somershoe, S., Sather, M., and McNew, L. B. (2021). Nest density drives productivity in chestnut-collared longspurs: implications for grassland bird conservation. PLoS One 16:e0256346. doi: 10.1371/journal.pone.0256346
Renfrew, R. B., Ribic, C. A., and Nack, J. L. (2005). Edge avoidance by nesting grassland birds: a futile strategy in a fragmented landscape. Auk 122, 618–636. doi: 10.1093/auk/122.2.618
Ribic, C. A., Koford, R. R., Herkert, J. R., Johnson, D. H., Niemuth, N. D., Naugle, D. E., et al. (2009). Area sensitivity in north American grassland birds: patterns and processes. Auk 126, 233–244. doi: 10.1525/auk.2009.1409
Richardson, M. L., Weatherhead, P. J., and Brawn, J. D. (2006). Habitat use and activity of prairie kingsnakes (Lampropeltis calligaster calligaster) in Illinois. J. Herpetol. 40, 423–428. doi: 10.1670/0022-1511(2006)40[423:HUAAOP]2.0.CO;2
Richmond, S., Nol, E., and Burke, D. (2011). Avian nest success, mammalian nest predator abundance, and invertebrate brey availability in a fragmented landscape. Can. J. Zool. 89, 517–528. doi: 10.1139/z11-017
Ricklefs, R. E. (1969). An analysis of nesting mortality in birds. Smithson. Contrib. Zool. 9, 1–48. doi: 10.5479/si.00810282.9
Robbins, C. S., Bystrak, D., and Geiggler, P. H. (1986). The Breeding Bird Survey: Its First Fifteen Years, 1965–1979. Washington, DC. Fish and Wildlife Service Resource Publication 157.
Robel, R. J., Briggs, J. N., Dayton, A. D., and Hulbert, L. C. (1970). Relationships between visual obstruction measurement and weight of grassland vegetation. J. Range Manag. 23, 295–297. doi: 10.2307/3896225
Rogers, C. M., and Caro, M. J. (1998). Song sparrows, top carnivores and nest predation: a test of the mesopredator release hypothesis. Oecologia 116, 227–233. doi: 10.1007/s004420050583
Rosenberg, K. V., Dokter, A. M., Blancher, P. J., Sauer, J. R., Smith, A. C., Smith, P. A., et al. (2019). Decline of the north American avifauna. Science 366, 120–124. doi: 10.1126/science.aaw1313
Rovero, F., and Marshall, A. R. (2009). Camera trapping photographic rate as an index of density in forest ungulates. J. Appl. Ecol. 46, 1011–1017. doi: 10.1111/j.1365-2664.2009.01705.x
Sauer, J. R., Hines, J. E., and Fallon, J. (2020). The North American Breeding Bird Survey, Results and Analysis 1966–2019. Virginia: U.S. Geological Survey Data Release.
Schmidt, K. A. (2002). Nest predation and population declines in Illinois songbirds: a case for mesopredator effects. Conserv. Biol. 17, 1141–1150. doi: 10.1046/j.1523-1739.2003.02316.x
Schmidt, K. A., Rush, S. A., and Ostfeld, R. S. (2008). Wood thrush nest success and post-fledging survival across a temporal pulse of small mammal abundance in an oak forest. J. Anim. Ecol. 77, 830–837. doi: 10.1111/j.1365-2656.2008.01378.x
Schwemm, C. A., and Martin, P. L. (2005). “Response of nest success of Xantus’s Murrelets (Synthliboramphus hypoleucus) to native predator abundance, Santa Barbara Island, California,” in Proceedings of the Sixth California Island Symposium, eds. D. K. Garcelon and C. A. Schwemm Ventura, CA, 373–384.
Shitikov, D., Vaytina, T., Makarova, T., Fedotova, S., Volkova, V., and Samsonov, S. (2018). Species-specific nest predation depends on the total passerine nest density in open-nesting passerines. J. Ornithol. 159, 483–491. doi: 10.1007/s10336-017-1526-8
Shochat, E., Patten, M. A., Moris, D. W., Reinking, D. L., Wolfe, D. H., and Sherrod, S. K. (2005). Ecological traps in isodars: effects of tallgrass prairie management on bird nest success. Oikos 111, 159–169. doi: 10.1111/j.0030-1299.2005.13907.x
Spafford, R. D., and Lortie, C. J. (2013). Sweeping beauty: is grassland arthropod community composition effectively estimated by sweep netting? Ecol. Evol. 3, 3347–3358. doi: 10.1002/ece3.688
Sperry, J. H., Peak, R. G., Cimprich, D. A., and Weatherhead, P. J. (2008). Snake activity affects seasonal variation in nest predation risk for birds. J. Avian Biol. 39, 379–383. doi: 10.1111/j.0908-8857.2008.04451.x
Stallman, H. R., and Best, L. B. (1996). Bird use of an experimental strip intercropping system in Northeast Iowa. J. Wildl. Manag. 60, 354–362. doi: 10.2307/3802235
Stanton, R. L., Morrissey, C. A., and Clark, R. G. (2018). Analysis of trends and agricultural drivers of farmland bird declines in North America: a review. Agric. Ecosyst. Environ. 254, 244–254. doi: 10.1016/j.agee.2017.11.028
Thompson, F. R. III, Donovan, T. M., DeGraaf, R. M., Faaborg, J., and Robinson, S. K. (2002). A multi-scale perspective of the effects of forest fragmentation on birds in eastern forests. Stud. Avian Biol. 25, 8–19.
Tremblay, I., Thomas, D., Blondel, J., Perret, P., and Lambrechts, M. M. (2005). The effect of habitat quality on foraging patterns, provisioning rate and nestling growth in Corsican blue tits Parus caeruleus. Ibis 147, 17–24. doi: 10.1111/j.1474-919x.2004.00312.x
Van Horne, B. (1983). Density as a misleading indicator of habitat quality. J. Wildl. Manag. 47, 893–901. doi: 10.2307/3808148
Vernouillet, A., Fortin, M.-J., Fiola, M.-L., and Villard, M.-A. (2020). Do female songbirds avoid a mammalian nest predator when selecting their nest site? Front. Ecol. Evol. 8:2020. doi: 10.3389/fevo.2020.571456
Vickery, P. D., Hunter, M. L., and Melvin, S. M. (1994). Effects of habitat area on the distribution of grassland birds in Maine. Conserv. Biol. 8, 1087–1097. doi: 10.1046/j.1523-1739.1994.08041087.x
Warren, K. A., and Anderson, J. T. (2005). Grassland songbird nest-site selection and response to mowing in West Virginia. Wildl. Soc. Bull. 33, 285–292. doi: 10.2193/0091-7648(2005)33[285:GSNSAR]2.0.CO;2
Weatherhead, P. J., and Blouin-Demers, G. (2004). Understanding avian nest predation: why ornithologists should study snakes. J. Avian Biol. 35, 185–190. doi: 10.1111/j.0908-8857.2004.03336.x
Weldy, M. J., Epps, C. W., Lesmeister, D. B., Manning, T., Linnell, M. A., and Forsman, E. D. (2019). Abundance and ecological associations of small mammals. J. Wildl. Manag. 83, 902–915. doi: 10.1002/jwmg.21641
Westerskov, K. (1950). Methods for determining the age of game bird eggs. J. Wildl. Manag. 14, 56–67. doi: 10.2307/3795978
White, G. C., and Burnham, K. P. (1999). Program MARK: survival estimation from populations of marked animals. Bird Study 46, S120–S139. doi: 10.1080/00063659909477239
Whitmore, R. C. (1981). Structural characteristics of grasshopper sparrow habitat. J. Wildl. Manag. 45, 811–815. doi: 10.2307/3808729
Whittingham, M. J., and Markland, H. M. (2002). The influence of substrate on the functional response of an avian granivore and its implications for farmland bird conservation. Oecologia 130, 637–644. doi: 10.1007/s00442-001-0850-z
Winter, M., Hawks, S., Shaffer, J. A., and Johnson, D. H. (2003). Guidelines for finding nests of passerine birds in tallgrass prairie. Prairie Nat. 35, 197–211.
Winter, M., Johnson, D. H., and Faaborg, J. (2000). Evidence for edge effects on multiple levels in tallgrass prairie. Condor 102, 256–266. doi: 10.1093/condor/102.2.256
Winter, M., Johnson, D. H., Shaffer, J. A., Donovan, T. M., and Svedarsky, W. D. (2006). Patch size and landscape effects on density and nesting success of grassland birds. J. Wildl. Manag. 70, 158–172. doi: 10.2193/0022-541X(2006)70[158:PSALEO]2.0.CO;2
Keywords: field sparrow, habitat structure, nest density, nest survival, Spizella pusilla, structural equation models
Citation: Glass A and Eichholz MW (2023) Estimating direct and indirect effects of habitat structure on nesting field sparrows (Spizella pusilla) using structural equation models. Front. Ecol. Evol. 11:1094152. doi: 10.3389/fevo.2023.1094152
Received: 09 November 2022; Accepted: 10 March 2023;
Published: 27 March 2023.
Edited by:
Binbin Li, Duke Kunshan University, ChinaReviewed by:
Kendra B. Sewall, Virginia Tech, United StatesCopyright © 2023 Glass and Eichholz. This is an open-access article distributed under the terms of the Creative Commons Attribution License (CC BY). The use, distribution or reproduction in other forums is permitted, provided the original author(s) and the copyright owner(s) are credited and that the original publication in this journal is cited, in accordance with accepted academic practice. No use, distribution or reproduction is permitted which does not comply with these terms.
*Correspondence: Alex Glass, YWxleC5nbGFzc0BiaXJkcGFydG5lcnMub3Jn
Disclaimer: All claims expressed in this article are solely those of the authors and do not necessarily represent those of their affiliated organizations, or those of the publisher, the editors and the reviewers. Any product that may be evaluated in this article or claim that may be made by its manufacturer is not guaranteed or endorsed by the publisher.
Research integrity at Frontiers
Learn more about the work of our research integrity team to safeguard the quality of each article we publish.