- 1Sichuan Zoige Alpine Wetland Ecosystem National Observation and Research Station, Institute of Qinghai-Tibetan Plateau, Southwest Minzu University, Chengdu, China
- 2Sichuan Academy of Grassland Sciences, Chengdu, China
- 3Sichuan Zoige Alpine Wetland Ecosystem National Observation and Research Station & Key Laboratory for Bio-Resource and Eco-Environment of Ministry of Education, College of Life Sciences, Sichuan University, Chengdu, China
Heavy metals elements are not only related to the functions of ecosystems but also affect human health. To understand the characteristics of heavy metals in the topsoil of the Zoige alpine basin, a total of 252 grass and topsoil samples were collected in May and September 2014. The results showed that only Cd and Pb highly exceeded their background values (BV); in May and September, Cd was 2.02- and 1.55-fold higher than its BV, respectively, and Pb was 2.35- and 2.17-fold above its BV, respectively. The sources of Cd and Pb were homologous. In addition, the comprehensive potential ecological risk index was less than 150, indicating that heavy metal pollution in the study area is currently low. The spatial interpolation indicated that Cd and Pb pollution might be related to tourism and transportation, but the low biological absorption coefficient for all heavy metals showed that heavy metal absorption ability of forage was low and would not impact yak breeding. Finally, the soil was lightly contaminated by Cd and Pb due to the rapid development of the animal husbandry and tourism. The spatial variation of heavy metal in the basin is dominated by structural factors, and the random factors also have an effect on spatial distribution of As, Cd, Cu and Ni. The random factors such as overgrazing can exert an influence on physical structure and the circulation of nutrient substances of meadow soil through livestock grazing and trampling, ultimately affecting the content and distribution of soil heavy metals.
Introduction
Heavy metal can be stored in the soil which can be transferred into groundwater or plants and ultimately harm human health by passing through the food chain (Sheng et al., 2012). The content and distribution of soil heavy metals are mainly affected by the soil parent material as well as human activities during the process of soil development. In addition, the contents and distribution of soil heavy metals are also influenced by factors such as soil physicochemical properties, the crop cultivation environment, atmospheric deposition and the interactions between different heavy metal elements (Intawongse and Dean, 2006). Because the characteristics of soil heavy metal pollution are concealment, hysteresis, accumulation, irreversibility, poisonousness and persistence, soil heavy metals are popular research objects and play an important role in the quantitative evaluation of the soil environment (Andersen et al., 2002; Li et al., 2008; Sun et al., 2010; Olawoyin et al., 2012; Oluseye and Fatoba, 2013; Li et al., 2015; Jin et al., 2017).
Grassland has more than a third of earth’s terrestrial surface, supporting a large number of wild and domesticated ungulates (Frank et al., 2018). The Qinghai-Tibetan Plateau (QTP) covers an area of approximately 2.5 million km2, has an average elevation of 4,000 m, and also has the world’s largest pastoral alpine grassland (Mipam et al., 2019), which provides many of animal husbandry products for the people living in and adjacent this area. This area is well-known and only slightly affected by human activities, such as exploration and use of its natural resources, transportation, tourism, urban construction and increasing human population, due to its unique geographic location and for historical reasons, the exploitation of natural resources in this area has remained at a very low level (Xie et al., 2014; Yang et al., 2017). However, with the rapid development of the economy and tourism in recent years, the ecological environment of this area is increasingly threatened by human activities leading to its vulnerable to the global pollutants (Huang et al., 2012).
The Zoige alpine basin, the world’s largest alpine wetland and peatland, is located on the northeast edge of the QTP. The economy of this area mainly depends on animal husbandry in which yak breeding dominates. Nomadism is the main grazing practice in the warm season, while in the cold season grazing is around the settlements. The nomadic areas are located in the vicinity of the road or highways in order to facilitate the management and trade of animal by-products. To date, there are several studies on the geology, vegetation, climate and soil environment in the Zoige basin. However, all the studies about soil in this area have mainly focused on the distribution of soil organic carbon (Tian et al., 2003; Luan et al., 2014; Ma et al., 2016), the characteristics of wetland soil moisture and nutrients (Tian et al., 2005; Gao et al., 2011), wetland degradation (Li et al., 2011; Huo et al., 2013; Hu et al., 2015), and soil microbial community (Niu et al., 2011; Tian et al., 2012; Yun et al., 2012; Wu et al., 2016). Till now, only a little research has concentrated on the heavy metals in soils from the Zoige alpine basin (Chen et al., 2021). For example, the heavy metal contents of the Zoige basin were determined by hyperspectral remote sensing technology (Yuan et al., 2016). According to statistics, from 1989 to 2009, when the Zoige meadow grazing overloading rate reached 99%, and is still rising (Wang et al., 2015). The animal manure has greatly increased following the development of animal husbandry. As a result, it will influence the content and distribution of top soil heavy metals in the meadow (Wang et al., 2013).
Therefore, in order to explore the content and spatial distribution of soil heavy metal in the warm season, and to understand the level of pollution and the concentration of heavy metals in this area, we collected surface soil and grass samples in two sampling months from the Zoige alpine basin to determine the concentration of seven heavy metal elements to explore the possible source, assess the potential ecological risk of the metals, and analyze the spatial heterogeneity and distribution of heavy metals. This work will provide a deep insight into the mechanisms of ecological risks of heavy metals and grassland managements.
Materials and methods
Study area
The Zoige alpine basin lies at 32°56′-34°19’N and 102°08′-103°39′E at an altitude of 3,400–3,900 m, and it has an area of 22,716 km2. The average annual precipitation is approximately 648.5 mm; the annual evaporation is 943–1,031 mm; and the annual average relative humidity is 70.4%. The climate is semi-humid continental monsoon, and the mean annual temperature is 1.1–1.5°C, the extreme minimum temperature is −33.7°C, the warm season lasts from April to September each year, the cold season lasts from October each year to March of the following year, and the annual permafrost lasts for 6 months (Hou et al., 2020).
The area is covered by two different types of vegetation, marsh and meadow. The dominant species in the marsh vegetation are Carex muliensis, Carex lasiocarpa, Carex meyeriana and Kobresia tibetica, while the meadow vegetation is dominated by Kobresia spp., Polygonum spp., and Gramineae (Huo et al., 2013). The soil is mainly composed of subalpine meadow soil, swamp soil, brown soil, and plateau cinnamon soil (Chai, 1965). The study area was located in Zoige National Nature Reserve and did not have any large-scale industry. The China national highway G213 runs through the basin from the east to the north and around a wetland reserve, which is near the route of the S209 provincial highway.
Sample collection and preparation
In May and September 2014, a total of 63 sample points were set up within the study area that was divided into 21 grid cells (approx. 20 km2 each), and each grid cell includes 3 sample points (Figure 1). Two grass and topsoil samples (0–5 cm) were collected from each sample point and a total of 126 grass samples and 126 topsoil samples were collected. We collected aboveground grass samples from a 1 × 1 m quadrat at each sampling point, and we collected topsoil samples using a plastic soil auger after the grass removed. Soil samples were air dried at room temperature about two weeks and then remove the impurities. After that the samples were sieved with a 0.15 mm mesh sieve for further analysis. The impurities, like litter, dead biomass and small stones, were removed by hand from grass samples, and dried to constant weight in an oven at 65°C, crushed with a stainless steel pulverizer, sieved with a 0.15 mm mesh sieve and stored at room temperature for subsequent analysis.
Chemical analysis
Soil samples (0.2 g) were digested with a mixture of ultrapure 2 ml HCl 37% and 6 ml HNO3 68% in microwave digestors (CEM MARS6, United States PyNN Inc.). The digestion solution was then analyzed by inductively coupled plasma optical emission spectroscopy (ICP-OES) using an iCAP6000 series instrument (United States Thermo Fisher Scientific Inc.). Grass samples were pre-digested at room temperature for half an hour with a mixture of 4 ml HNO3 68% and 2 ml H2O2 30%, then were added 4 ml HNO3 68% in the digestor and digested at 180°C in a microwave digestion instrument (CEM MARS6). The concentrations of seven elements of heavy metals (As, Cd, Cr, Cu, Co, Ni and Pb) in grass samples were also determined by ICP-OES. Each sample was repeatedly determined three times. The standard reference materials were included for quality assurance and control. The percentage recovery of the seven elements ranged from 80 to 110%.
Data analysis
Statistical analysis
Normal distribution for each collective was tested using the Kolmogorov–Smirnov test. A t-test was employed to compare the differences in the means of two parameters. Pearson correlation analysis was performed in SPSS 21.0 software (IBM Inc., United States) to analyze the relationships among the concentrations of heavy metals.
Assessment of potential ecological risk
The potential ecological risk index (RI) is commonly used to evaluate the degree of heavy metal contamination. According to Hakanson (1980), the calculation formulas for RI of heavy metal elements are as follows:
where is the concentration of heavy metal element in the soil; is the background value (BV) of element ; is the potential ecological risk factor of element ; and is the toxic factor of element . The BV of the seven elements of heavy metals are 0.097 mg kg−1 for Cd, 11.20 mg kg−1 for As; 22.60 mg kg−1 for Cu; 12.70 mg kg−1 for Co; 26.00 mg kg−1 for Pb; 26.90 mg kg−1 for Ni; and 61.00 mg kg−1 for Cr, all of which were determined in a soil investigation in China (Wei et al., 1991). The toxic factors of Cd, As, Cu, Co, Pb, Ni, and Cr are 30, 10, 5, 5, 5, 5 and 2, respectively (Hakanson, 1980; Jin et al., 2017). The grading evaluation criteria for was low (<40), moderate (40–80), considerable (80–160), high (160–320), very high (>320) and for RI was low (<150), moderate (150–300), considerable (300–600), high (>600).
Calculation of biological absorption coefficient
The biological absorption coefficient (BAC) is employed to evaluate the ability of a plant to transport and enrich heavy metals from its belowground to aboveground parts, and a high value of BAC indicates that the plant has a strong ability of transporting heavy metals. According to Fuente et al. (2010), BAC is calculated as follows:
where is the content of a metal in the aboveground parts of plants, and is the content of this metal in the soil (Kabata-Pendias, 2001). Elements are enriched in the organisms when BAC > 1, and plants can transport most of the heavy metals from belowground to the aboveground parts if BAC > 0.5.
Geostatistical analysis
Geostatistical analysis is a powerful tool for distinguishing the sources of observed contamination, and geostatistical methods, i.e., Semi-variogram and Ordinary Kriging, were applied to estimate the spatial distribution of seven heavy metals in topsoil. To understand the spatial autocorrelations, several theoretical models, such as linear, exponential, Gaussian and spherical models, were fitted to the semi-variance functions. The composition and variation in the characteristics of soil heavy metal elements directly reflect the consequences of soil development and effects of human activities (Munoz-Barbosa et al., 2004). To understand the spatial correlation and variability of the seven heavy metals, a semi-variance analysis was employed, which reflects the differences between two observed values at different distances (Kelly et al., 1996). Before spatial analysis and the calculation of variation function, the normality distribution of the spatial data was tested (Rossi and Franz, 1992). The semi-variance analysis software GS+ version 9.0 was used to calculate the semi-variance parameters, and the spatial distribution map of heavy metals was constructed with Surfer software version 8.0 (Golden software, Inc., United States).
Results and discussion
Concentrations of heavy metals in soil and grass
The concentrations of As, Cd, Cr, Cu, Co, Ni and Pb in the soil at different sampling sites and in May and September, as well as their BVs, are shown in Table 1. The mean contents of Cd and Pb in May and September and the mean contents of As and Cu in May were higher than their BVs. In particular, the concentrations of Cd and Pb greatly exceeded their BVs. In May and September, the mean concentrations of Cd were 0.17 mg·kg−1 and 0.13 mg·kg−1, respectively; and the mean concentrations of Pb were 52.58 mg·kg−1 and 48.51 mg·kg−1, respectively. The results indicate a serious contamination of Cd and Pb in Zoige basin. The pollution of soil Pb is mainly produced by emissions of vehicle exhaust and domestic waste (McClintock, 2012) due to the fact that the main anti-knocking agent in Chinese motor fuels was tetraethyl-lead before 2000. Thus, the pollution of Cd may be related to road dust (Wang et al., 2007).
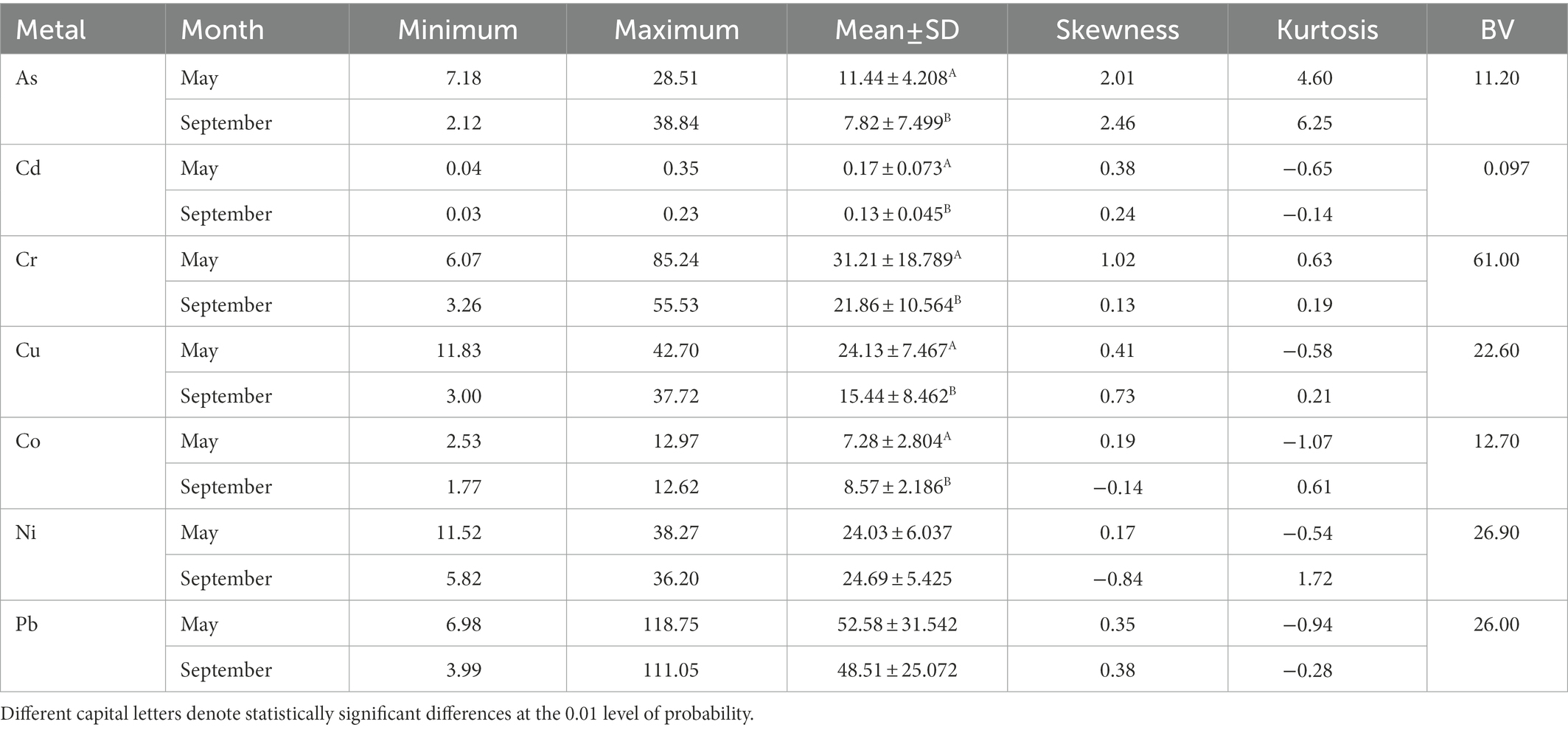
Table 1. Concentrations of heavy metals (mg·kg−1) in the topsoil of Zoige basin in May and September.
In addition, the contents of most heavy metals in May were generally higher than September, which may be related to the eluviation and plants absorption in summer (Panichayapichet et al., 2007). Specifically, the concentrations of As, Cd, Cr, Cu and Pb in May were 1.46, 1.31, 1.43, 1.56, and 1.08 times the values measured in September, respectively. On the contrary, the contents of Co and Ni in May were lower than those in September, which may be related to the poor absorption of these two elements by meadow plants (Cai et al., 2010).
The grass concentrations of As, Cd, Cr, Cu, Co, Ni and Pb were also measured in different sampling months (Table 2), and the heavy metal contents of grass in May were higher than those in September. In the Zoige basin, the vegetation is covered by accumulated snow lasting from October to the coming April. To avoid frost damage, physiological activities of plants become dormant in the winter. Probably because of few photosynthetic products and increased consuming of organic matters in winter, heavy metals were enriched in the grass and had a relatively higher concentration in May than September. In addition, the concentration of each heavy metal in grass was far lower than its content in the soil in two sampling months, which may be connected with the low enrichment capacity of vegetation for heavy metals in soil.
Correlation analysis of heavy metals
A correlation analysis can detect the source similarities between heavy metal elements (Zou et al., 2015). As displayed in Table 3, there were significant correlations among the contents of Cd, Cr, Cu, Co, Ni and Pb in both May and September, with correlation coefficients between 0.275 and 0.931. In particular, the correlation coefficients among Cd, Co and Pb were greater than 0.850 and highly significant, suggesting that the sources of Cd, Co and Pb were homologous and closely related. However, the concentration of topsoil As was not significantly correlated with the other heavy metals, indicating unique sources of As that require further studies.
Risk assessment of heavy metals
We used the Hakanson RI method to evaluate the potentially ecological risk of seven heavy metals in the topsoil (Table 4). The RI and values for May and September were listed in the following order: Cd > As>Pb > Cu > Ni > Co > Cr and Cd > Pb > As>Ni > Cu > Co > Cr, respectively. Further, the Eri values for Cd in both months were higher than those of the other metals. The ecological risk of Cd contamination was moderate, while the risk levels of other heavy metals contamination were low. In addition, both RI values for the 2 months were less than 150 (86.604 for May and 68.606 for September), demonstrating a low ecological risk of heavy metals in general. However, the level of Cd contamination in the topsoil deserves attention.
Considering the relatively higher ecological risk of Cd contamination, we further analyzed the spatial distribution of topsoil Cd by conducting an ordinary kriging interpolation using the Cd values (Figure 2). The areas with low potential Cd pollution levels in May and September were mainly located in the middle and at the mouth of the basin. It is worth noting that the areas with high EI values were in the Zoige County which is adjacent to the G213 national highway in the northeast and the S209 provincial highway in the southeast. Thus, we speculate that the source of Cd pollution may come from human waste and vehicle emissions on the highway.
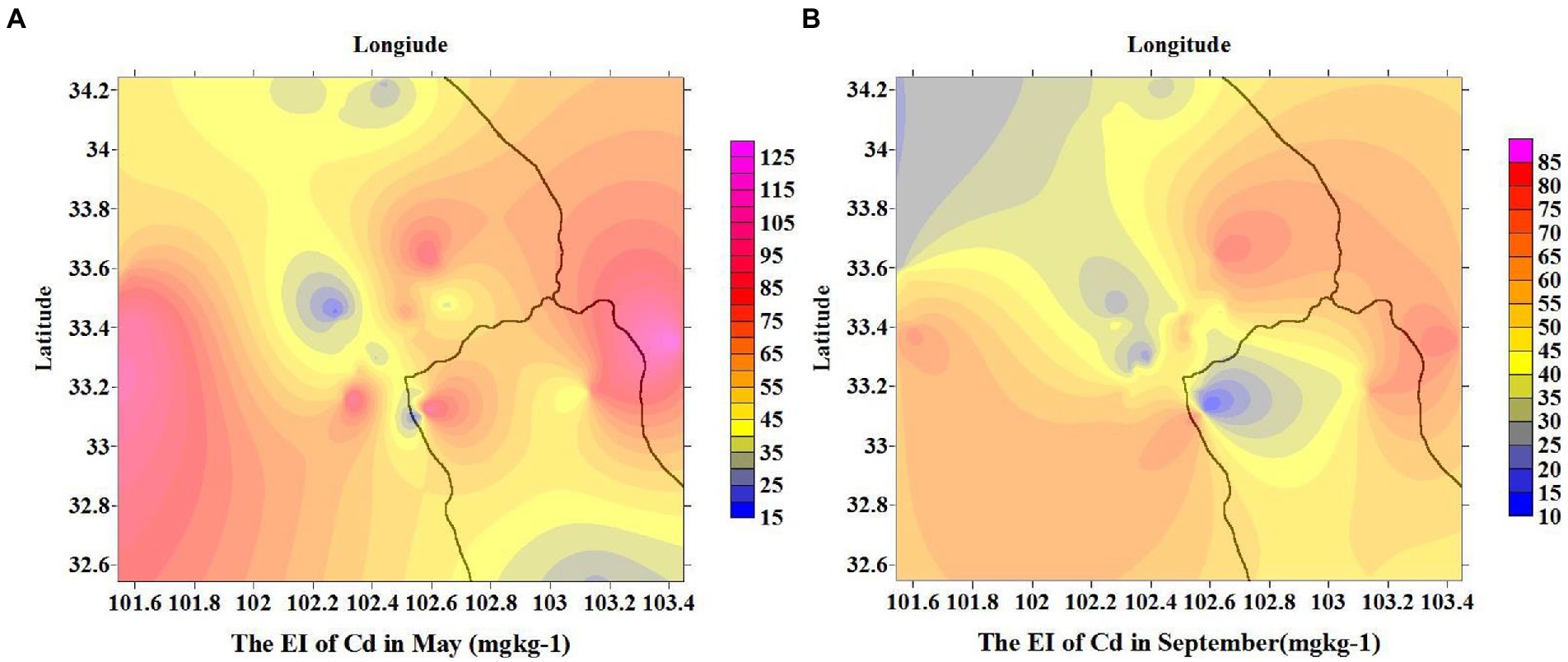
Figure 2. Spatial distribution of the potential ecological risk of Cd in May (A) and September (B) in the topsoil. (The blue areas indicate lower potential ecological risk; the yellow area denotes a moderate risk level; and the red area represents considerable risk).
Biological absorption of heavy metals
We applied BAC to indicate the ability of grass accumulating heavy metal from soil (D’Souza et al., 2013). Higher values of BAC indicate that the mobility ability of elements from soil to grass is greater. In our study, the BAC values (%) decreased in the sequence of Cr(0.179) > Cu(0.174) > As(0.153) > Cd(0.118) > Ni(0.086) > Pb(0.051) > Co(0.048) in May, and sequence of Cu(0.260) > Cr(0.223) > As(0.124) > Cd(0.077) > Ni(0.074) > Pb(0.037) > Co(0.027) in September. Overall, the mean BAC values of Cr and Cu were higher than those of other heavy metals, but all values were lower than 0.5. Therefore, the heavy metal absorption ability of forages in Zoige basin was low. Besides, the biological absorption coefficient of heavy metals in May was greater than September, except for Cr and Cu, and may because May and September are the beginning and last month of the warm season.
Spatial distribution of heavy metals
Theoretically, different Co/(Co + C) values can represent the variability in the spatial correlation, in which higher values indicate that stronger spatial correlation (Bernardi et al., 2016). The Co/(Co + C) values of Cr, Co, and Pb in May and all of the heavy metals in September were less than 25%, indicating strong spatial correlation of heavy metals distributions in topsoil (Table 5). Therefore, the spatial variation in these heavy metals was mainly affected by structural factors but not that nomadic overgrazing has a significant impact on the spatial pattern of heavy metal in meadow soil. In particular, the Co/(Co + C) values of As, Cd, Cu and Ni in May were between 25 and 75%, suggesting a moderate spatial correlation and random factor such as grazing also contribute to the spatial variation of heavy metals.
The kriging interpolation maps of the seven heavy metal concentrations are presented in Figure 3. The figure shows that the areas with high concentrations of As, Cd and Pb (above BV) were larger than other heavy metals, which verified the above results and indicated that a potential pollution from these metals may exist. Moreover, it is similar to the results of the potential ecological risk interpolation (see Figure 2), the areas with high Pb and Cd concentrations in the topsoil were also distributed near the G213 and S209 highways.
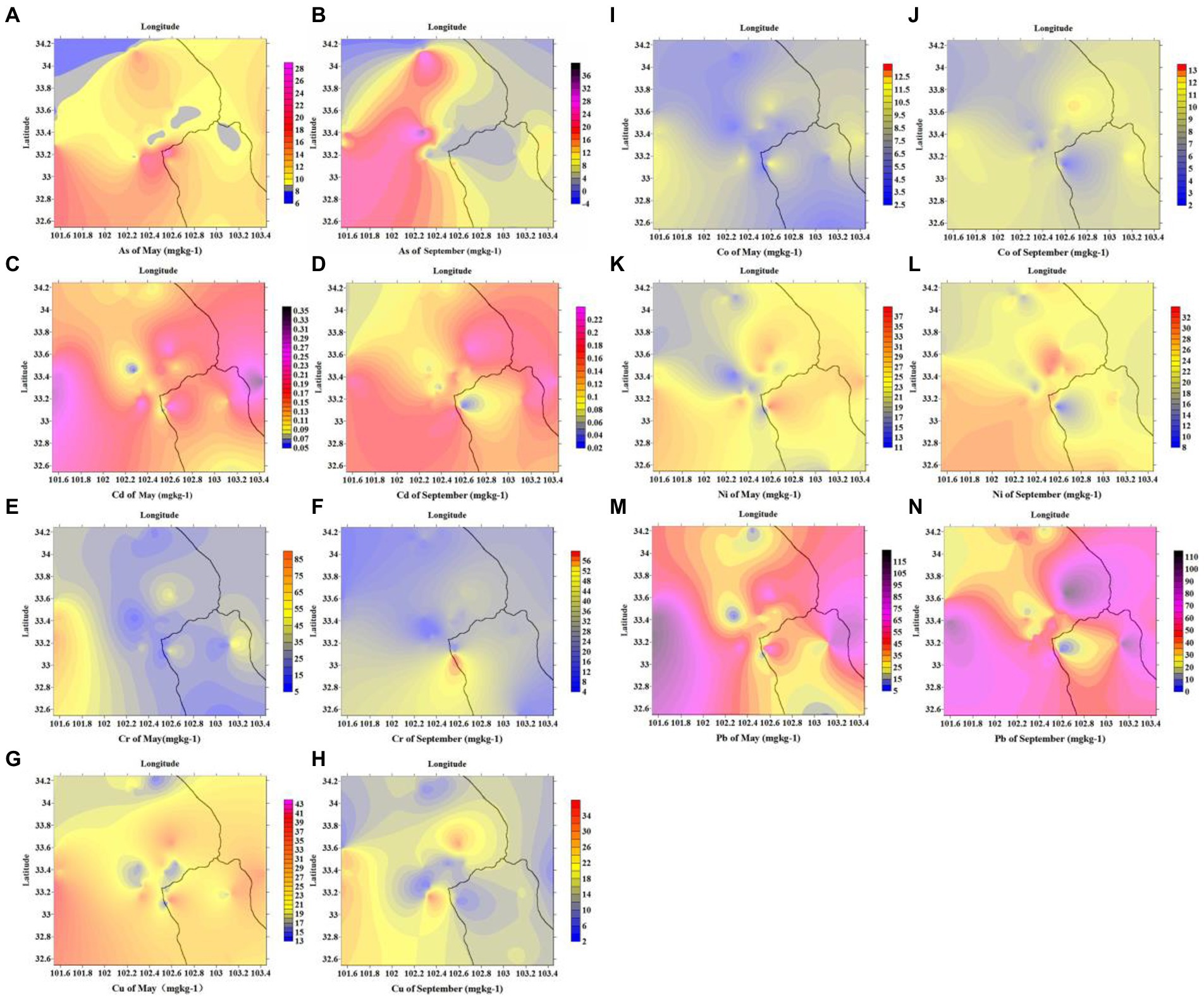
Figure 3. Spatial distribution of the topsoil heavy metals of As (A,B), Cd (C,D), Cr (E,F), Cu (G,H), Co (I,J), Ni (K,L) and Pb (M,N). (The blue and yellow areas indicate that the metal concentrations were lower than the background values (BV); the red area denotes concentrations between 1BV and 2BV; the purple areas indicate concentrations between 2BV and 3BV; and the black area represents concentrations equal to or greater than 3BV).
According to the results of variogram analysis, the spatial variation of soil As, Cd, Cu and Ni were influenced by random and structural factors. Therefore, we can confirm that the Cd contamination partly originates from human activities such as transportation and livestock farming. The spatial pattern variation of heavy metal in Zoige basin is related to the overgrazing area in the warm season. More specifically, livestock mainly affect the physical structure of meadow soil through grazing and trampling (Dakhah and Gifford, 1980). At the same time, it affects the circulation of nutrient substances of the grassland and finally causes changes in the chemical composition of grassland soil (Haynes and Williams, 1993). Heavy grazing can lead to a relative increase of soil organic matter content (Haynes and Williams, 1993). Studies have shown that plants can transfer more C to the belowground in response to the reduced forage yields under heavy grazing disturbance (Derner et al., 1997). In addition, livestock trampling benefits the breakup of litters and mix of litter with soil particles, contributing to the litter decomposition and transfer of C and mineral elements into the soil (Naeth et al., 1991). Besides, the contents of most mineral elements in soil are positively correlated with soil organic carbon and total nitrogen (Stevenson, 1984; Rahman et al., 1996), suggesting that heavy metal deposition in soil may be related to the accumulation of soil organic matters. Therefore, heavy grazing can affect the spatial pattern of heavy metals via the changes of soil organic matters under grazing disturbance.
Conclusion
In summary, this study showed that there is only a very slightly heavy metal pollution in the Zoige alpine basin by Cd and Pb contamination. The potential ecological risk assessment indicated that the evaluation criteria grade of Cd is moderate which should be highlighted in further investigation. Furthermore, there is almost no heavy metals enrichment in the grass, which can provide evidences that no heavy metal pollution exists in animal husbandry of this area. The spatial variation in these heavy metals is mainly affected by structural factors but not by nomadic heavy grazing by animals.
Data availability statement
The raw data supporting the conclusions of this article will be made available by the authors upon request.
Author contributions
TM and YW conceived and designed the study. TG, HZ, YA, SZ, LT, and TM collected the data. TG, HZ, and YA analyzed the data. TM wrote the first draft with input from LT. All authors provided critical feedback on manuscript draft. All authors contributed to the article and approved the submitted version.
Funding
This work was supported by the National Natural Science Foundation of China (32271628), Sichuan Science and Technology Program (2022YFN0037, 2023NSFSC0201), Fundamental Research Funds for the Central Universities (2021SCU12100), Qinghai Science and Technology Major Program (2021-NK-A5), the Fundamental Research Funds for the Central Universities of Southwest Minzu University (ZYN2023001) and Open Foundation of Lanzhou University State Key Laboratory of Grassland Agro-ecosystems.
Conflict of interest
The authors declare that the research was conducted in the absence of any commercial or financial relationships that could be construed as a potential conflict of interest.
Publisher’s note
All claims expressed in this article are solely those of the authors and do not necessarily represent those of their affiliated organizations, or those of the publisher, the editors and the reviewers. Any product that may be evaluated in this article, or claim that may be made by its manufacturer, is not guaranteed or endorsed by the publisher.
References
Andersen, M. K., Raulund, R. K., Hansen, H. C. B., and Strobel, B. W. (2002). Distribution and fractionation of heavy metals in pairs of arable and afforested soils in Denmark. Euro. J. Soil Sci. 53, 491–502. doi: 10.1046/j.1365-2389.2002.00467.x
Bernardi, A. C. C., Bettiol, G. M., Ferreira, R. P., Santos, K. E. L., Rabello, L. M., and Inamasu, R. Y. (2016). Spatial variability of soil properties and yield of a grazed alfalfa pasture in Brazil. Precis. Agric. 17, 737–752. doi: 10.1007/s11119-016-9446-9
Cai, L., Huang, L., Zhou, Y., Xu, Z., Peng, X., Yao, L., et al. (2010). Heavy metal concentrations of agricultural soils and vegetables from Dongguan. Guangdong. J. Geogr. Sci. 20, 121–134. doi: 10.1007/s11442-010-0121-1
Chen, X., Su, Q., Chen, H., and Xue, D. (2021). A high-resolution accumulation record of arsenic and mercury after the first industrial revolution from a peatland in Zoige, Qinghai-Tibet plateau. Land 10:1241. doi: 10.3390/land10111241
D’Souza, R., Varun, M., Pratas, J., and Paul, M. S. (2013). Spatial distribution of heavy metals in soil and flora associated with the glass industry in north Central India: implications for phytoremediation. Soil Sediment Contam. 22, 1–20. doi: 10.1080/15320383.2012.697936
Dakhah, M., and Gifford, G. F. (1980). Influence of vegetation, rock cover, and trampling on infiltration rates and sediment production. J. Am. Water Resour. As. 16, 979–986. doi: 10.1111/j.1752-1688.1980.tb02537.x
Derner, J. D., Briske, D. D., and Boutton, T. W. (1997). Does grazing mediate soil carbon and nitrogen accumulation beneath C4, perennial grasses along an environmental gradient? Plant Soil 191, 147–156. doi: 10.1023/A:1004298907778
Frank, D. A., Wallen, R. L., Hamilton, E. W. III, White, P. J., and Fridley, J. D. (2018). Manipulating the system: how large herbivores control bottom-up regulation of grasslands. J. Ecol. 106, 434–443. doi: 10.1111/1365-2745.12884
Fuente, V. D. A., Rufo, L., Rodríguez, N., Amils, R., and Zulugag, J. (2010). Metal accumulation screening of the Río Tinto Flora (Huelva, Spain). Biol. Trace Elem. Res. 134, 318–341. doi: 10.1007/s12011-009-8471-1
Gao, J., Ouyang, H., Lei, G., Xu, X., and Zhang, M. (2011). Temperature and soil moisture interactively affect soil carbon mineralization in Zoige alpine wetlands. Chinese Geogr. Sci. 21, 27–35. doi: 10.1007/s11769-011-0439-3
Hakanson, L. (1980). An ecological risk index for aquatic pollution control.a sedimentological approach. Water Res. 14, 975–1001. doi: 10.1016/0043-1354(80)90143-8
Haynes, R. J., and Williams, P. H. (1993). Nutrient cycling and soil fertility in the grazed pasture ecosystem. Adv. Agron. 49, 119–199. doi: 10.1016/S0065-2113(08)60794-4
Hou, M., Ge, J., Gao, J., Meng, B., Li, Y., Yin, J., et al. (2020). Ecological risk assessment and impact factor analysis of alpine wetland ecosystem based on LUCC and boosted regression tree on the Zoige plateau, China. Remote Sens. 12:368. doi: 10.3390/rs12030368
Hu, G., Dong, Z., Lu, J., and Yan, C. (2015). The developmental trend and influencing factors of aeolian desertification in the Zoige Basin, eastern Qinghai-Tibet plateau. Aeolian Res. 19, 275–281. doi: 10.1016/j.aeolia.2015.02.002
Huang, J., Kang, S., Zhang, Q., and Yan, H. (2012). Wet deposition of mercury at a remote site in the Tibetan plateau: concentrations, speciation, and fluxes. Atmos. Environ. 62, 540–550. doi: 10.1016/j.atmosenv.2012.09.003
Huo, L., Chen, Z., Zou, Y., Lu, X., Guo, J., and Tang, X. (2013). Effect of Zoige alpine wetland degradation on the density and fractions of soil organic carbon. Ecol. Eng. 51, 287–295. doi: 10.1016/j.ecoleng.2012.12.020
Intawongse, M., and Dean, J. R. (2006). Uptake of heavy metals by vegetable plants grown on contaminated soil and their bioavailability in the human gastrointestinal tract. Food Addit. Contam. 23, 36–48. doi: 10.1080/02652030500387554
Jin, A., Yang, L., Chen, X., Loh, P., Lou, Z., Liu, G., et al. (2017). Ecological risk and contamination history of heavy metals in the Andong tidal flat, Hangzhou Bay, China. Hum. Ecol. Risk. Assess. 23, 617–640. doi: 10.1080/10807039.2016.1263541
Kelly, J., Thornton, I., and Simpson, P. R. (1996). Urban geochemistry: a study of the influence of anthropogenic activity on the heavy metal content of soils in traditionally industrial and non-industrial areas of Britain. Appl. Geochem. 11, 363–370. doi: 10.1016/0883-2927(95)00084-4
Li, C., Kang, S., Wang, X., Ajmone-Marsan, F., and Zhang, Q. (2008). Heavy metals and rare earth elements (REEs) in soil from the Nam Co Basin, Tibetan Plateau. Environ. Geol. 53, 1433–1440. doi: 10.1007/s00254-007-0752-4
Li, P., Lin, C., Cheng, H., Duan, X., and Lei, K. (2015). Contamination and health risks of soil heavy metals around a lead/zinc smelter in southwestern China. Ecotox. Environ. Safe. 113, 391–399. doi: 10.1016/j.ecoenv.2014.12.025
Li, K., Yang, Y., Yang, Y., and Han, D. (2011). Characteristics and influence factors of the swamp degradation under the stress of grazing in the Zoige plateau. Acta Ecol. Sin. 115, 362–367.
Luan, J., Cui, L., Xiang, C., Wu, J., Song, H., and Ma, Q. (2014). Soil carbon stocks and quality across intact and degraded alpine wetlands in Zoige, East Qinghai-Tibet plateau. Wetl. Ecol. Manag. 22, 427–438. doi: 10.1007/s11273-014-9344-8
Ma, K., Liu, J., Balkoviè, J., Skalsky, R., Azevedo, L. B., and Kraxner, F. (2016). Changes in soil organic carbon stocks of wetlands on China's Zoige plateau from 1980 to 2010. Ecol. Model. 327, 18–28. doi: 10.1016/j.ecolmodel.2016.01.009
McClintock, N. (2012). Assessing soil lead contamination at multiple scales in Oakland, California: implications for urban agriculture and environmental justice. Appl. Geogr. 35, 460–473. doi: 10.1016/j.apgeog.2012.10.001
Mipam, T. D., Zhong, L., Liu, J., Miehe, G., and Tian, L. (2019). Productive overcompensation of alpine meadows in response to yak grazing in the eastern Qinghai-Tibet plateau. Front. Plant Sci. 10:925. doi: 10.3389/fpls.2019.00925
Munoz-Barbosa, A., Cutiérrez-Galindo, E. A., Segovia-Zavala, J. A., Delgadillo-Hinojosa, F., and Sandoval-Salazar, G. (2004). Trace metal enrichment in surficial sediments of the northwest coast of Baja California, Mexico. Mar. Pollut. Bull. 48, 596–603. doi: 10.1016/j.marpolbul.2003.11.019
Naeth, M. A., Bailey, A. W., Pluth, D. J., Chanasyk, D. S., and Hardin, R. T. (1991). Grazing impacts on litter and soil organic matter in mixed prairie and fescue grassland ecosystems of Alberta. J. Range Manag. 44, 7–12. doi: 10.2307/4002629
Niu, J., Zhou, X., Jiang, N., and Wang, Y. (2011). Characteristics of soil microbial communities under dry and wet condition in Zoige alpine wetland. Acta Ecol. Sin. 31, 474–482.
Olawoyin, R., Oyewole, S. A., and Grayson, R. L. (2012). Potential risk effect from elevated levels of soil heavy metals on human health in the Niger delta. Ecotox. Environ. Safe. 85, 120–130. doi: 10.1016/j.ecoenv.2012.08.004
Oluseye, O. C., and Fatoba, P. O. (2013). Pollution loads and the ecological risk assessment of soil heavy metals around a mega cement factory in Southwest Nigeria. Pol. J. Environ. Stud. 22, 487–493.
Panichayapichet, P., Nitisoravut, S., and Simachaya, W. (2007). Spatial distribution and transport of heavy metals in soil, ponded-surface water and grass in a pb-contaminated watershed as related to land-use practices. Environ. Monit. Assess. 135, 181–193. doi: 10.1007/s10661-007-9642-1
Rahman, S., Takaki, H., Tamai, M., and Nagatomo, Y. (1996). Distribution of zinc, manganese, copper, cobalt, and nickel in andosols profiles. Soil Sci. Plant Nutr. 42, 881–891. doi: 10.1080/00380768.1996.10416635
Rossi, R., and Franz, E. (1992). Geostatistical tools for modeling and interpreting ecological spatial dependence. Ecol. Monogr. 62, 277–314. doi: 10.2307/2937096
Sheng, J. J., Wang, X. P., Gong, P., Tian, L. D., and Yao, T. D. (2012). Heavy metals of the Tibetan top soils. Environ. Sci. Pollut. R. 19, 3362–3370. doi: 10.1007/s11356-012-0857-5
Stevenson, F. J. (1984). Humus chemistry: Genesis, composition, reactions. New York: John Wiley and Sons Press.
Sun, Y. B., Zhou, Q. X., Xie, X. K., and Liu, R. (2010). Spatial, sources and risk assessment of heavy metal contamination of urban soils in typical regions of Shenyang, China. J. Hazard. Mater. 174, 455–462. doi: 10.1016/j.jhazmat.2009.09.074
Tian, Y. B., Xiong, M. B., and Song, G. Y. (2005). Restoration succession of wetland soils and their changes of water and nutrient in Ruoergai plateau. Chinese J. Ecol. 24, 21–25.
Tian, Y. B., Xiong, M. B., Xiong, X. S., and Song, G. Y. (2003). The organic carbon distribution and flow in wetland soil-plant system in Ruoergai plateau. Chinese J. Plant Ecol. 27, 490–495. doi: 10.17521/cjpe.2003.0071
Tian, J., Zhu, Y., Kang, X., Dong, X., Li, W., Chen, H., et al. (2012). Effects of drought on the archaeal community in soil of the Zoige wetlands of the Qinghai-Tibetan plateau. Eur. J. Soil Biol. 52, 84–90. doi: 10.1016/j.ejsobi.2012.07.003
Wang, C., Chen, Z., Wang, J., Zhou, N., and Xu, S. (2007). Pollution of lead and cadmium in soil and vegetable on sides of the main roads of chongming island. J. Agro-Environ. Sci. 26, 634–638.
Wang, H., Dong, Y. H., Yang, Y. Y., Toor, G., and Zhang, X. M. (2013). Changes in heavy metal contents in animal feeds and manures in an intensive animal production region of China. J. Environ. Sci. 25, 2435–2442. doi: 10.1016/S1001-0742(13)60473-8
Wang, X. F., He, P., and Kang, W. X. (2015). Analysis on factors in the formation of grassland desertification in Ruoergai plateau. J. Cent. South Univ. Forest. Technol. 35, 100–106.
Wei, F., Zheng, C. J., Chen, J., and Wu, Y. (1991). Study on the background contents on 61 elements of soils in China. Chinese J. Environ. Sci. 12, 12–19.
Wu, L., Nie, Y., Yang, Z., and Zhang, J. (2016). Responses of soil inhabiting nitrogen-cycling microbial communities to wetland degradation on the Zoige plateau, China. J. Mt. Sci. 13, 2192–2204. doi: 10.1007/s11629-016-4004-5
Xie, H., Li, J., Zhang, C., Tian, Z., Liu, X., Tang, C., et al. (2014). Assessment of heavy metal contents in surface soil in the Lhasa-Shigatse-Nam co area of the Tibetan plateau, China. Bull. Environ. Contam. Tox. 93, 192–198. doi: 10.1007/s00128-014-1288-4
Yang, G., Peng, C., Chen, H., Dong, F., Wu, N., Yang, Y., et al. (2017). Qinghai-Tibetan plateau peatland sustainable utilization under anthropogenic disturbances and climate change. Ecosyst. Health Sust. 3:e01263. doi: 10.1002/ehs2.1263
Yuan, Z. Q., Cao, C. X., Bao, D. M., Chen, W., Tian, R., Er, G., et al. (2016). Inversion on contents of heavy metals in soil of wetlands in Zoige plateau based on remote sensing data. Wetl. Sci. 14, 113–116.
Yun, J., Zhuang, G., Ma, A., Guo, H., Wang, Y., and Zhang, H. (2012). Community structure, abundance, and activity of methanotrophs in the Zoige wetland of the Tibetan plateau. Microb. Ecol. 63, 835–843. doi: 10.1007/s00248-011-9981-x
Keywords: heavy metals, potential ecological risk, biological absorption coefficient, kriging, grassland
Citation: Gan T, Zhao H, Ai Y, Zhang S, Wen Y, Tian L and Mipam TD (2023) Spatial distribution and ecological risk assessment of heavy metals in alpine grasslands of the Zoige Basin, China. Front. Ecol. Evol. 11:1093823. doi: 10.3389/fevo.2023.1093823
Edited by:
Daniel de Paiva Silva, Goiano Federal Institute (IFGOIANO), BrazilReviewed by:
Raquel Fernanda Salla, Federal University of Sao Carlos, BrazilJosino Moreira, Oswaldo Cruz Foundation (Fiocruz), Brazil
Copyright © 2023 Gan, Zhao, Ai, Zhang, Wen, Tian and Mipam. This is an open-access article distributed under the terms of the Creative Commons Attribution License (CC BY). The use, distribution or reproduction in other forums is permitted, provided the original author(s) and the copyright owner(s) are credited and that the original publication in this journal is cited, in accordance with accepted academic practice. No use, distribution or reproduction is permitted which does not comply with these terms.
*Correspondence: Tserang Donko Mipam, ✉ dGRtaXBhbUAxNjMuY29t
†These authors have contributed equally to this work