- 1Zhejiang Provincial Key Laboratory of Plant Evolutionary Ecology and Conservation, School of Life Sciences, Taizhou, Zhejiang, China
- 2Linhai Station of Zhejiang Provincial Forest Ecological Research, Taizhou University, Taizhou, Zhejiang, China
- 3Taizhou Key Laboratory of Mountain Ecological Restoration and Special Industry Cultivation, Taizhou University, Taizhou, Zhejiang, China
Introduction: Forest streams reserve more than 90% of the organic carbon (OC) in sediments, thus playing crucial roles in the global carbon cycle. Although forest streams are widely distributed across various forest regions, seasonal dynamics of OC in forest sediments have not been fully investigated.
Methods: Here, we sampled soils (0–5 cm) in 15 representative forest stream sediments for 5 critical periods (snowmelt season, early growing season, growing season, late growing season, and seasonal snow cover season) during a one-year investigation in a geologically fragile subalpine coniferous forest catchment in the upper reaches of the Yangtze River in Sichuan province and the OC concentrations were measured by potassium dichromate external heating method.
Results: The OC concentration of stream sediments ranged from 6.39 to 458.93 g kg−1, and the average was 84.56 g kg−1 for 15 streams in 5 critical periods during a 1-year investigation. Correspondingly, their stocks ranged from 2.05 to 310.56 kg m−2, and the average was 46.03 kg m−2. The maximum and minimum OC stocks were consistently observed during the late growing season and the snowmelt season, respectively. Otherwise, the OC stocks ranged from 1.31 to 218.05, 1.29 to 182.64, and 0.99 to 190.38 kg m−2 for the upstream, midstream, and downstream sediments, and the average was 39.36, 36.58, and 37.93 kg m−2, respectively. The average ratios of the OC stocks of the upstream and downstream ranged from 0.10–6.31, with an average of 1.43, during 5 critical periods, which indicated that the forest stream sediments may play crucial roles as carbon sources.
Discussion: Furthermore, based on regression analysis, we found that the seasonal dynamics of OC concentrations and stocks were mainly regulated by precipitation, temperature, sediment depth, and litter carbon input to the streams together. These findings demonstrate that forest stream sediments may play crucial roles in the carbon biogeochemical cycle of subalpine forests and adjoining streams.
1. Introduction
Sediments capture and store a significant amount of organic carbon (OC), and more than 10% of the terrestrially derived carbon is stored in sediments transported through inland waters (Nellemann et al., 2009; Donato et al., 2011; Marttila et al., 2012). Forest streams are widely distributed in forest ecosystems and connect the terrestrial–aquatic biogeochemical cycle by acting as active zones for material exchange between land and water, especially sediment exchange (Figure 1; Sutherland et al., 2002; Öquist et al., 2009). Since terrestrial OC, especially for the sediments of forest streams, is an important component of the global carbon pool, exploring the sources and dynamics of forest sediments OC can help us better use forest stream sediments to capture carbon and enhance terrestrial carbon sinking (Graça et al., 2015). Although some researchers have quantitatively compared the organic carbon sources in litter and water bodies from different forest streams (Zhang et al., 2017; Hou et al., 2021, 2022), more attention should be concentrated on the dynamics of OC among different growing season and different area of stream in forest stream sediments.
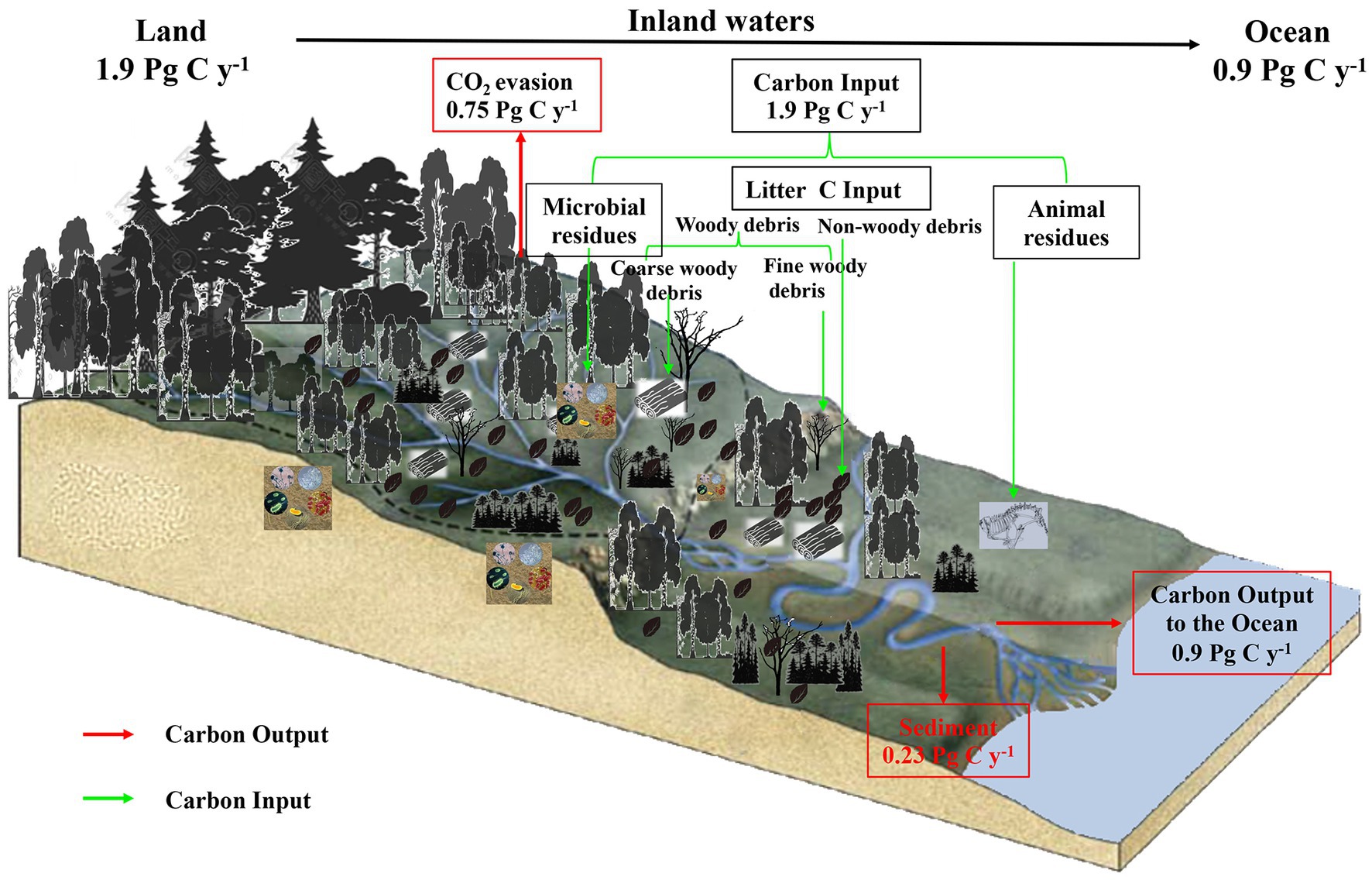
Figure 1. Simplified schematic of the role of inland aquatic systems in the global carbon balance. Inland aquatic systems are active components of the global carbon cycle that store terrestrially derived carbon in sediments and lose CO2 as emissions to the atmosphere in addition to transporting it to the oceans.
Inner waters provide a major output pathway for energy and material to be transported from upstream to downstream areas; thus, the organic carbon stored in their waters is important at regional and even global scales (Wallace et al., 1997; Graça et al., 2015; Yue et al., 2016; Hou et al., 2021), especially that stored in their sediments, because they are significant depositional environments that contain a large amount of organic carbon (Cole et al., 2007). Globally, the storage of organic carbon in the sediments of the global oceans is approximately 120–240 Tg C a−1 (Duarte et al., 2004), and the burial of organic carbon in the sediments of natural lakes has been estimated to be in the range of 30 to 70 Tg C a−1 (Dean and Gorham, 1998; Einsele et al., 2001). Compared with lakes, organic carbon burial in impoundments is much greater, ranging from 150 to 220 Tg C a−1 (Mulholland and Stallard, 1998; Tranvik et al., 2009). In the geosphere, streams cover less than 3% of the forest catchment area but function as bridges that link terrestrial–aquatic biogeochemical cycles (Hou et al., 2021). Although contemporary studies of organic carbon flux measurements for different kinds of sediments are increasing, the actual organic carbon stock currently stored in forest stream sediments remains largely unknown. Previous studies have also examined the influencing mechanisms for the transport of organic particulate carbon along river continua; these studies have focused only on large rivers and floodplains but not on stream and bed sediments or streams in subalpine forest regions (Depetris and Kempe, 1993). Therefore, a thorough investigation of organic carbon dynamics in forest stream sediments can help elucidate the biogeochemical links between forests and aquatic ecosystems and provide a scientific basis for managing forest catchments and predicting the global carbon cycle.
The OC buried in sediments represents short–to long-term sequestration of atmospheric CO2 or CH4 (Qin et al., 2015), which is mainly regulated by both input and output. Carbon in water sediments mainly includes autochthonous OC from benthic organisms and phytoplankton and allochthonous OC from stream inputs (Yue et al., 2016; Hou et al., 2021; Yang and Wu, 2021). Theoretically, forests and riparian zones import different kinds of organic matter into adjacent streams and rivers through plant residues (woody and nonwoody debris; Wallace et al., 1997; Zhong and Wang, 2008; Yue et al., 2016; Hou et al., 2021), surface runoff (Ågren et al., 2007; Gomes et al., 2019), and percolating water (Cleveland et al., 2014; Hou et al., 2021). All these inputs are regulated by the multiple functions of different biological and abiotic variables, which vary over different critical periods. First, for OC, the ultimate source is past and present primary production, either in a watershed or in the water body. Therefore, different types of adjoining stream vegetation have different litter biomass and different influences on the OC quantity and quality that inputs to the sediments (Qin et al., 2015; Mao et al., 2021; Guan, 2022). Second, plant species and their growth rhythms change with climatic factors (temperature, precipitation, etc.) during different critical periods, which influences the carbon input to stream sediments in the form of litterfall or dissolved carbon (Yue et al., 2016; Zhang et al., 2017; Hou et al., 2021). Third, precipitation (rainfall and snowfall) is also the major regulator of OC stocks. For example, the increase in runoff and percolating water caused by precipitation can consistently influence OC input and output driven by unsteady water flow (Xie et al., 2016), which affects the OC stocks buried in the sediments. Furthermore, stream characteristics, such as length and width, sediment depth, and flow velocity, are the main regulators of carbon dynamics in subalpine forest streams (Qin et al., 2015). However, less research focused on the relationship between OC stocks in forest stream sediments and climatic factors and stream characteristics, particularly in the headwaters of mountainous subalpine forest regions.
There are three main pathways for OC lost from sediments during transport: mineralization followed by release into the atmosphere, transference by water flow, and sequestration of C in sediments. Thus, less than half of this carbon (C) reaches the ocean (Cole et al., 2007; Battin et al., 2009). These processes vary greatly with climatic factors (e.g., precipitation and temperature), plant rhythms, vegetation types and characteristics along streams (Frost et al., 2006; Ågren et al., 2014; Winterdahl et al., 2014). All these pathways vary over different critical periods in forest streams (Yang and Wu, 2021). First, stream OC deposited at sediment interfaces is mineralized to CO2 or CH4 by microbial activity, which is mainly controlled by climate factors. For example, temperature, precipitation, and oxygen exposure have been identified as important regulators of OC burial efficiency in water sediments (Sobek et al., 2009; Gudasz et al., 2010, 2012; Fenner and Freeman, 2013). These factors are the main regulators of microbial metabolism, controlling the mineralization rates for OC in sediments. Second, the output of OC from streams is altered by a variety of processes, including primary production, microbial breakdown, sorption to particles, and photodegradation within aquatic systems, which are mainly regulated by climatic factors (Wallin et al., 2013). Third, OC export from forest streams typically depends upon stream characteristics (length, width, flow velocity, etc.) and often varies with downstream distance (Mattsson et al., 2003). For example, streams with larger areas and lower flow rates store more carbon in their sediments than streams with smaller areas and faster flow rates (Zhang et al., 2017). In general, compared with stationary water bodies, such as reservoirs and lakes, forest streams are more active and respond more quickly to changing environmental factors, such as temperature and precipitation. Therefore, it is important to study the seasonal dynamics of OC concentration and storage in forest stream sediments, and comprehensively investigate the effects of these factors on the dynamics of OC concentration, storage, and export.
The subalpine coniferous forests located at the eastern margin of the Qinghai-Tibet Plateau and the upper reaches of the Yangtze River are the most important “water towers” in the Yangtze River basin, and they are known as “natural regulatory reservoirs.” They play important and irreplaceable roles in water conservation, as global climate change indicators and in biodiversity conservation (Wu et al., 2014; Yue et al., 2016; Hou et al., 2021). Studies have also shown that in this forest ecosystem, forest streams not only participate directly as important components of the biological micro–cycle but also serve as indispensable links between the forest ecosystem and its adjoining aquatic ecosystem (such as rivers, rivers, and lakes). Although previous investigations have paid much attention to the concentration and storage characteristics of woody and nonwoody carbon debris in forest streams (Yue et al., 2016; Zhang et al., 2017; Hou et al., 2021), less attention has been given to the storage characteristics of OC in subalpine forest stream sediments. The objective of this study was to further elucidate the dynamic changes in OC in subalpine forest stream sediments. We hypothesized that (1) the OC concentration and stocks may show different dynamic patterns among different critical periods, such as the growing period, snow-covered period, and snowmelt period; (2) the maximum and minimum values of the indices mentioned above would appear during the litterfall and snowmelt periods, respectively; and (3) the OC stocks in the upstream sediments would be greater than those in the downstream sediments of these forest streams.
2. Materials and methods
2.1. Site description
This study was conducted at the long-term research station of the subalpine forest ecosystem in the Bipenggou Valley (31°14′ ~ 31°19′N, 102°53′ ~ 102°57′E, 2458 ~ 4,619 m.a.s.l.), Li County, Southwest China, located at the eastern margin of the Qinghai-Tibet Plateau and the transition of the Sichuan Basin alpine valley zone. Frequent geological breaks, clear seasonal snow cover (the maximum snow depth was approximately 35 cm), and frequent freeze–thaw cycles are common in this region (Hou et al., 2021). The highest and lowest temperatures are 23°C (July) and − 18°C (January), respectively. The frozen season lasts from November to April and ends in late April (Zhu et al., 2013). The detailed characteristics of this catchment are shown in our previous research (Hou et al., 2022).
2.2. Experimental design and sampling
Based on previous investigations, 15 permanent streams representative of the forest catchment were selected at altitudes of 3,600 ~ 3,700 m in this typical subalpine forest catchment [detailed characteristics of these streams are shown in Hou et al. (2021)]. The total investigated area was 4.3 km2. We chose three sampling sites at the upper portion, middle, and end of each stream for collecting water samples and measured the length, width, sediment depth, and discharge velocity (stream characteristics) of each stream at each sampling time. We measured the length, width, and width with a flexible ruler for each stream at every sampling site. The length was measured from the source to the estuary along the banks, and the width was measured horizontally from one bank to the other bank. Stream depth was measured vertically. All these indices were measured three times, and then the average was taken. In addition, we measured the discharge velocity at intervals of 30 min with a flowmeter (Martin Marten Z30, Current-metre, Barcelona, Spain), recorded the temperature every 2 h with a button thermometer (iButton DS1923-F5, Maxim/Dallas Semiconductor, Sunnyvale, United States), and measured the precipitation for real-time monitoring by a rainfall monitor (ZXCAWS600, Weather, Beijing, China) at every site.
We divided a year into five different periods–the snowmelt season (SMS: April to May), early growing season (EGS: May to June), growing season (GS: July to August), late growing season (LGS: September to October) and seasonal snow cover (SSC: November to April of the following year)–based on phenological changes, seasonal precipitation, and the temperature dynamics of the study area (Yang and Wu, 2021). Water samples were collected nine times during the growing seasons and four times during the nongrowing seasons. Specifically, water samples were collected during the LGS at approximately 15 days intervals. Therefore, the DC stock and concentration during each period for the 15 streams were treated as 15 replicates during data analysis.
Three sampling sites for collecting sediment samples were set up in the upper portion, middle, and end of each stream, respectively. The sampling sites were located directly below the water sampling points. Five surface sediment samples (0–5 cm) were collected vertically from bottom to top with a stainless-steel column sampler, then mixed as one sample for each sample site (length × width, 1 m × 1 m; Liu et al., 2016; Zhang et al., 2020). Three samples were collected for each site. Before each sampling, all sampling tools were thoroughly cleaned to avoid cross-contamination. All samples were sealed in polyethylene bags with air excluded and recorded (time, place, and description of the stream characteristics). Then, they were stored under cryogenic conditions and treated and analyzed as soon as possible. The sediments were dried at 65°C to a constant weight, impurities such as gravel (without OC) were removed by examining the samples on a hard white paper board, and the samples were dispersed with glass bars under equal pressure, passed through a 60-mesh sieve and sealed. All samples were transported to the laboratory within 72 h (Mei et al., 2022).
The OC content of the sediments was measured by the potassium dichromate oxidation-external heating method (Lu, 1999). First, approximately 0.01 g of air-dried soil samples were sieved with 100-mesh sieves, and then they were placed at the bottom of dissolving tubes. Then, 5 mL potassium dichromate solution and 5 mL sulfuric acid were added to the tubes, and the tubes were shaken well. Next, the tubes were boiled for 15 min on an electric stove at 220–230°C. Finally, the liquid in each desiccation tube was transferred to a conical flask with 4 drops of phenanthroline indicator, and the remaining potassium dichromatic was titrated with ferrous sulfate after cooling. The solution changed from orange-yellow to gray-green and then to brown-red. When the volume of ferrous sulfate used in the sample titration was less than 1/3rd of the volume of ferrous sulfate used in the blank calibration, the weight of the soil sample was reduced.
2.3. Analytical methods and calculations
Each stream was approximated to be a cube, so the carbon stock per square in sediment Cs was estimated to be.
Where Cs is the carbon stock per square in sediment, kg m−2; C is the OC concentration, g kg−1; V is the volume of stream sediment cube, m3; is the soil bulk density, g m−3; and S is the area of the stream, m2.
The V (volume of the stream sediment cube, m3) was calculated as follows:
where Ls is the length of the stream, m; Ws is the width of the stream, m; and Ds is the thickness of the sediment, m.
The bulk density of the sediment (g m−3) was calculated as follows:
where m is the dry weight of the sediment, g; Mc is the water content of the sediment; and Vc is the volume of the collector for the sediment, m3.
The water content of sediment Mc was calculated by the formula:
where m1 is the wet weight of the sediment, g, and m2 is the dry weight of the sediment, g.
Linear mixed-effect models were used to analyze the relationships between the OC stock and export of stream sediments and environmental factors (temperature, precipitation) and stream characteristics in these streams among different sampling periods. First, the normality of the residuals, the homoscedasticity of the errors, and the independence of the errors for our data were tested to determine whether they met the assumptions for analyzes. Second, the sampling period was treated as a fixed effect, and the OC stock and export of the 15 streams during each period were treated as repetitions. Then, we conducted repeated measures by analysis of variance (ANOVA) and least significant difference (LSD) to examine the variability among different critical periods. Third, to better illustrate the correlations of the relative indices of the OC stock and export with the explanatory variables (precipitation, temperature, sediment depth, discharge rate, litter carbon input), the variables were treated as fixed factors, and the stream was included as a random factor. We used linear and quadratic models to fit the relationships of these indices of OC with changes in the various explanatory variables. All analyzes were conducted in R using the LME4 package (Bates et al., 2015).
3. Results
3.1. The organic carbon concentration of sediments in forest streams
In general, the dynamics of the organic carbon concentration of sediments in the 15 forest streams varied with different critical periods. According to stream characteristics, the organic carbon concentration of the sediments in the 15 subalpine forest streams ranged from 6.39 to 458.93 g kg−1, and the average value was 84.56 g kg−1 (Supplementary Figure S1). And the maximum value (115.98 g kg−1) was observed during the late growing season, the value sharply decreased during the snow-covered season (66.59 g kg−1), it gradually decreased during the snowmelt season (65.44 g kg−1) and the early growing season (61.10 g kg−1) and then it gradually increased to 61.98 g kg−1 during the growing season. There was no significant difference between the SCS and GS values during our one-year investigation (Figure 2).
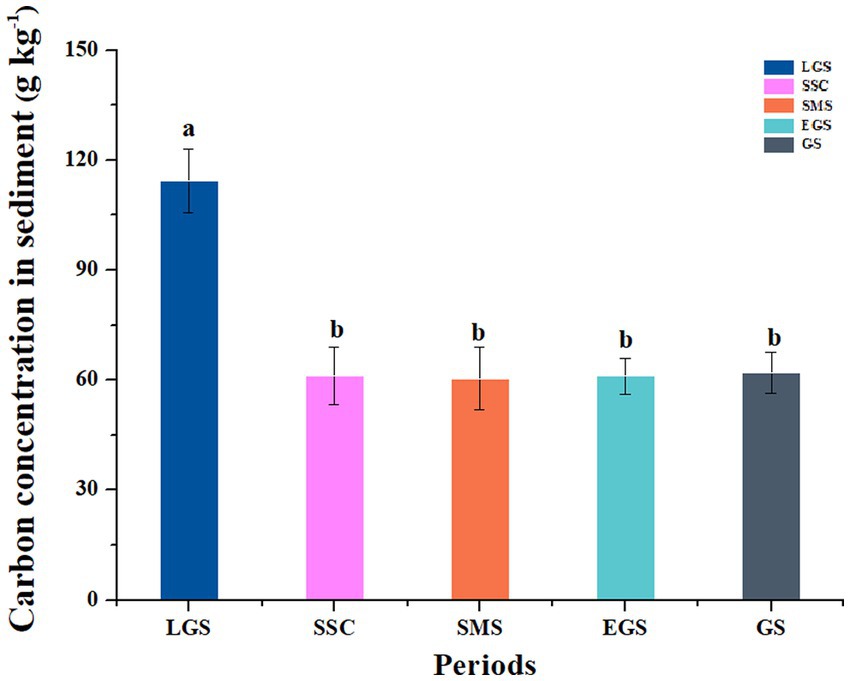
Figure 2. Dynamics of organic carbon concentration in the sediments of the subalpine forest streams in the upper reaches of the Yangtze River. LGS, SSC, SMS, EGS, and GS indicate the sampling periods, i.e., the late growing season (LGS: September to October), seasonal snow cover (SSC: November to April of the following year), snowmelt season (SMS: April to May), early growing season (EGS: May to June), and growing season (GS: July to August). The values of the vertical coordinates are the accumulated values from a total of 15 streams during this period. Different lowercase letters indicate significant differences among the different periods (p < 0.05), while the same letter indicates no significant difference among each.
3.2. Organic carbon stock of sediments in forest streams
The average total OC stock for the sediments in the forest streams ranged from 20.92–76.59 kg m−2, and the average value was 43.80 kg m−2 during different key periods (Figure 3). In general, the dynamics of the OC stock of sediments in forest streams showed decreasing patterns, which was similar to the dynamics of the carbon concentration of the sediments. The highest value was 76.59 kg m−2, which was reached during the late growing season, and the value sharply decreased during the snow-covered season (28.54 kg m−2). Then, there was a gradual decrease in the value during the snowmelt season (27.73 kg m−2), the early growing season (24.46 kg m−2), and the growing season (20.92 kg m−2), while there was no significant difference between the SCS and GS values during our one-year investigation (Figure 3).
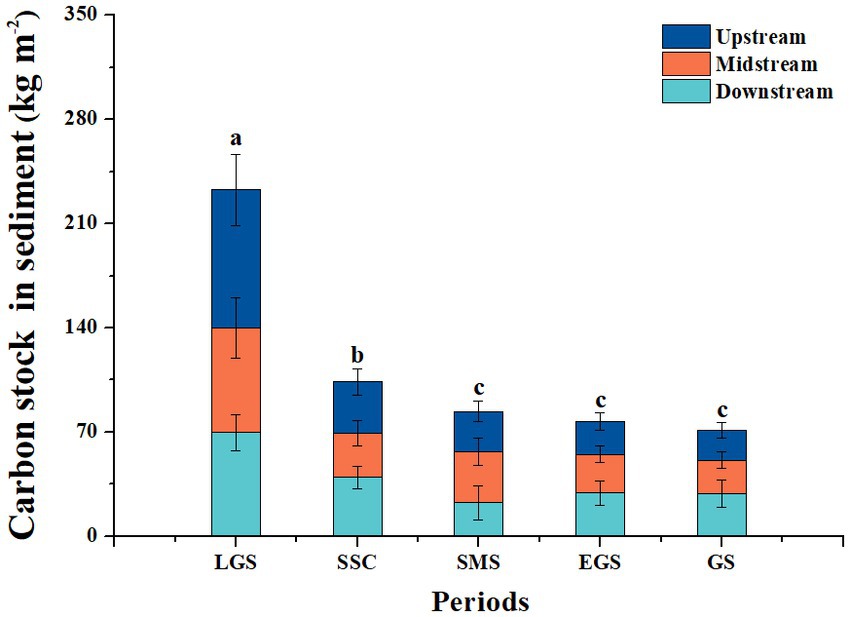
Figure 3. Dynamics of organic carbon stocks in the sediments of the subalpine forest streams in the upper reaches of the Yangtze River. LGS, SSC, SMS, EGS, and GS indicate the sampling periods, i.e., the late growing season (LGS: September to October), seasonal snow cover (SSC: November to April of the following year), snowmelt season (SMS: April to May), early growing season (EGS: May to June), and growing season (GS: July to August). The values of the vertical coordinates are the averaged values for the 15 streams during this period. Different lowercase letters indicate significant differences among different periods (p < 0.05), while the same letter indicates no significant difference among each.
The OC stock in the upstream, midstream and downstream sediments of the 15 forest streams generally showed common patterns similar to the OC concentration of the sediments (Figure 3). The carbon stock of the sediments reached maximum values (92.69, 70.34 and 69.73 kg m−2) during the late growing season and then decreased during the growing season (20.30, 22.59, 28.60 kg m−2), and the average was 39.36, 36.58, and 37.93 kg m−2 for the upstream, midstream, and downstream regions, respectively.
In contrast, according to stream characteristics, the total OC stock of the sediments of the 15 subalpine forest streams ranged from 2.05 to 310.56 kg m−2, and the average value was 46.03 kg m−2 (Figure 4). The OC stock of the sediments ranged from 1.31 to 218.05, 1.29 to 182.64, and 0.99 to 190.38 kg m−2 in the upstream, midstream, and downstream regions, respectively. The average values were 39.36, 36.58, and 37.93 kg m−2 for the upstream, midstream, and downstream regions, respectively.
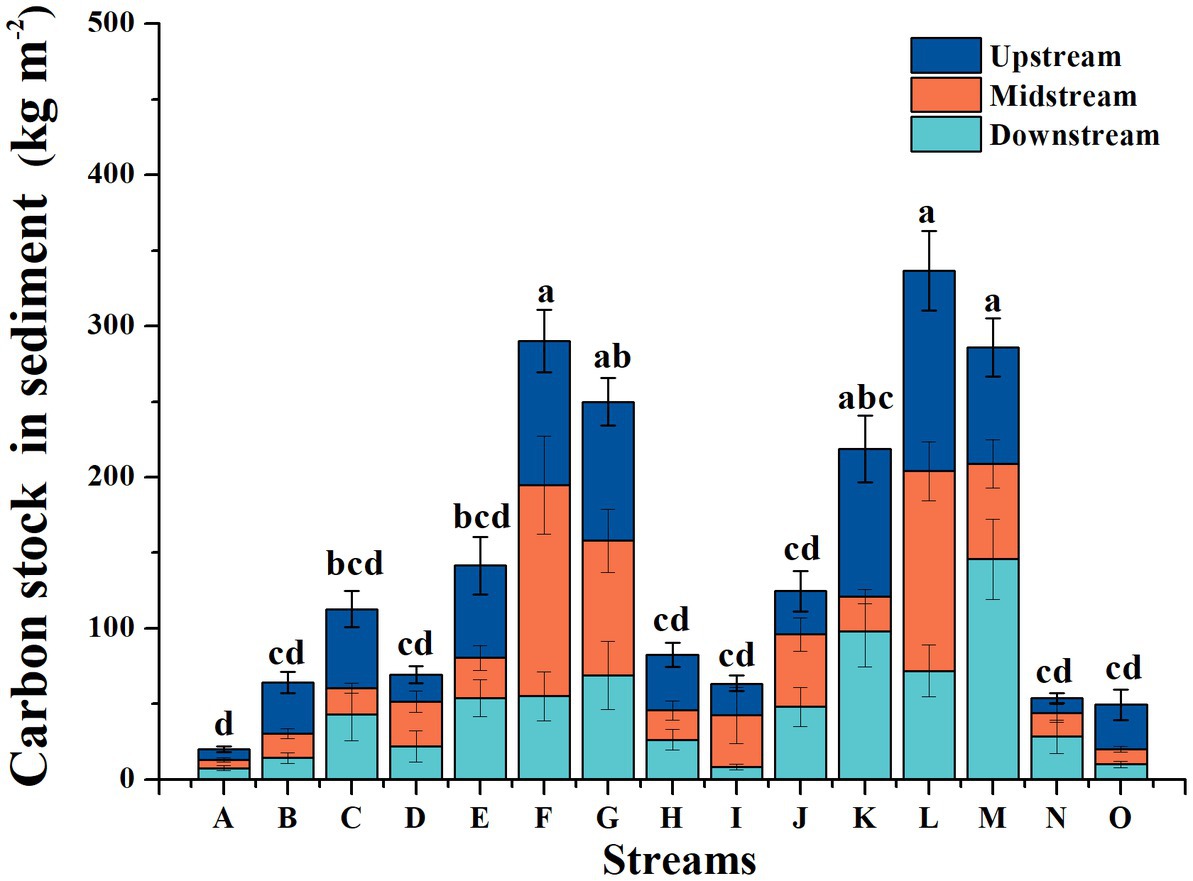
Figure 4. Dynamics of organic carbon stocks in the sediments of the subalpine forest streams in the upper reaches of the Yangtze River. Each bar is the average of 13 sampling times for each forest stream. Different lowercase letters indicate significant differences among different periods (p < 0.05), while the same letter indicates no significant difference among each. *A-O are the sampled streams in the study.
3.3. Organic carbon stock upstream to downstream sediment ratios
The average ratios of the OC stock of upstream to downstream sediments in the forest streams ranged from 0.10–6.31, with an average of 1.43 (Figure 5), during different key periods. Except for some streams that had ratios <1, the ratios of most of the streams were > 1 (Supplementary Figure S2). In general, like the trends in the OC stock, all of them showed similar patterns. The highest value, 1.79, was reached during the late growing season, and the value sharply decreased during the snow-covered season (1.37). Then, it showed a gradual decrease during the snowmelt season (1.22) and the early growing season (1.32) and gradually increased to 1.43 during the growing season, and there was no significant difference between the SCS and GS values during our one-year investigation (Figure 5).
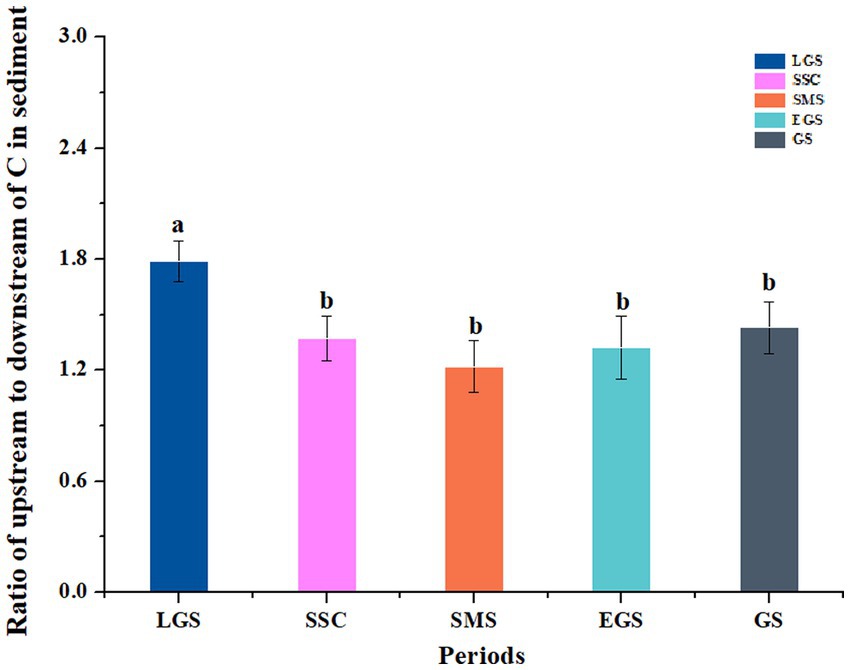
Figure 5. The ratios of organic carbon stocks in upstream and downstream sediments of the subalpine forest streams in the upper reaches of the Yangtze River from July 11, 2015, to August 02, 2016. LGS, SSC, SMS, EGS, and GS indicate the sampling periods, i.e., the later growing season (LGS: September to October), seasonal snow cover (SSC: November to April of the following year), snowmelt season (SMS: April to May), early growing season (EGS: May to June), and growing season (GS: July to August), respectively. The values of the vertical coordinates are the averaged values for the 15 streams during this period. Different lowercase letters indicate significant differences among different periods (p < 0.05), while the same letter indicates no significant difference from each other.
3.4. Relationships between the indices for organic carbon and relative variables
The organic carbon concentration was slightly and positively correlated with stream width, length, dissolved carbon output from water, sediment depth, and litter carbon input. It was also slightly and negatively correlated with litter carbon output from the streams (Table 1).
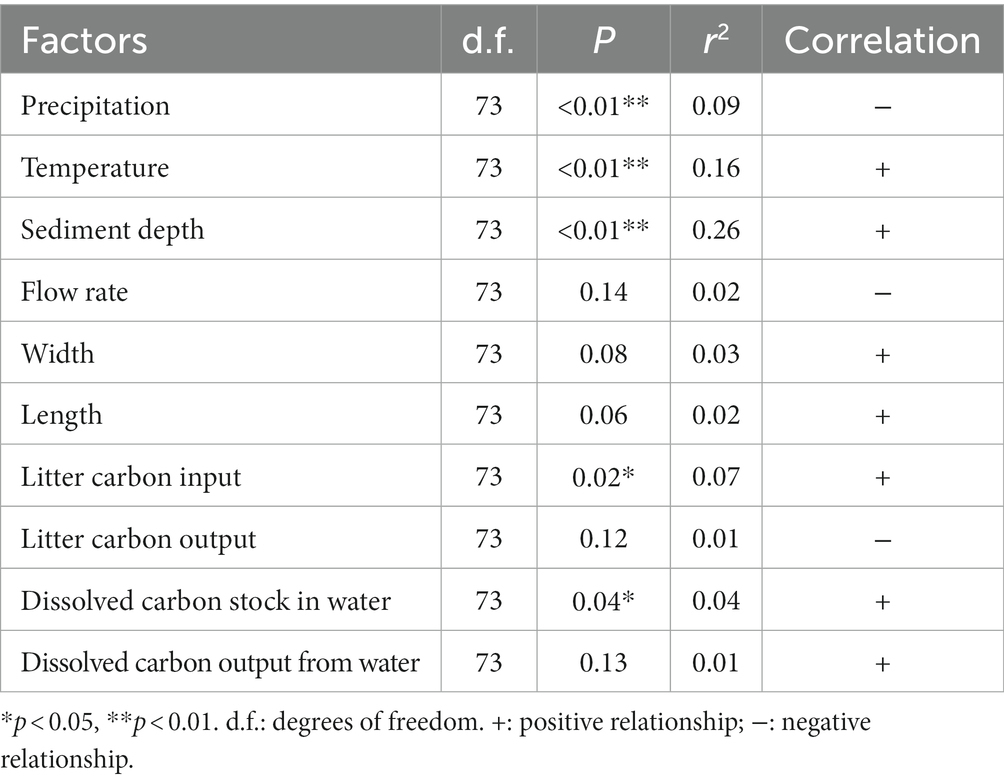
Table 1. Relationship between organic carbon concentration in the sediments and the investigated stream characteristics of the subalpine forest catchment.
In addition, the OC stock in the sediments was significantly and positively correlated with temperature, sediment depth, litter carbon input, and the dissolved carbon stock of the water but was significantly and negatively correlated with precipitation and flow rate, respectively. However, the OC stock was slightly and positively correlated with dissolved carbon from water but was slightly and negatively correlated with litter carbon from the streams, and the correlations were not significant (Table 2).
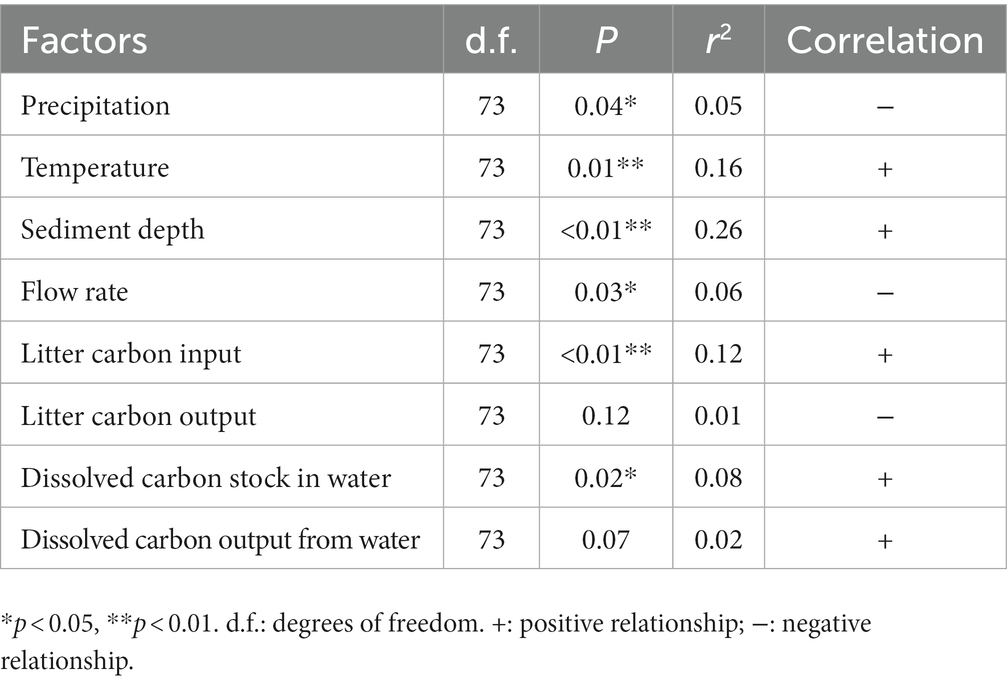
Table 2. Relationships between organic carbon stocks in the sediments and the investigated stream characteristics of the subalpine forest catchments.
4. Discussion
Our results indicated that the maximum values for the concentration and stock of the OC in the 15 forest stream sediments were consistently observed during the late growing period, while there was no significant difference among the other four periods, which was inconsistent with our first and second hypotheses. Climatic factors (temperature and precipitation) and stream characteristics (sediment depth, flow rate, litter carbon input, and dissolved carbon stock) drove the seasonal sink–source patterns for OC in the subalpine forest streams. Furthermore, the results showed that the OC stock in the stream sources was higher than that at the ends of the streams in the forest–stream meta-ecosystem, which was consistent with our third hypothesis, implying that subalpine forest streams may play an important role as carbon sources for subalpine forest regions.
4.1. Dynamics of OC concentration and stock in sediments
The average OC concentration was 84.56 g kg−1 for the 15 forest streams during this one-year investigation (Figure 2), which was not only larger than the average value (39.4 g kg−1) for impoundment sediments in the glacial plains of the midwestern United States (Pittman et al., 2013) but also larger than the average value (26.0 g kg−1) of 573 globally distributed fjord sediments (Smith et al., 2015; Table 3). The relatively large amount of OC buried as sediments in forest streams might be caused by the following reason. Compared with other aquatic ecosystems, this subalpine forest has larger primary productivity (Yue et al., 2016), which is a primary regulator of OC stocks in sediments. As the results of this study, more than 95% of the total OC was stored in the sediments of the 15 forest streams, which was much greater than that stored in litter and water (Table 4). Studies by Mendonça et al. (2012) and Clow et al. (2015) also showed that OC burial rates were proportional to the crop planting area adjoining them.
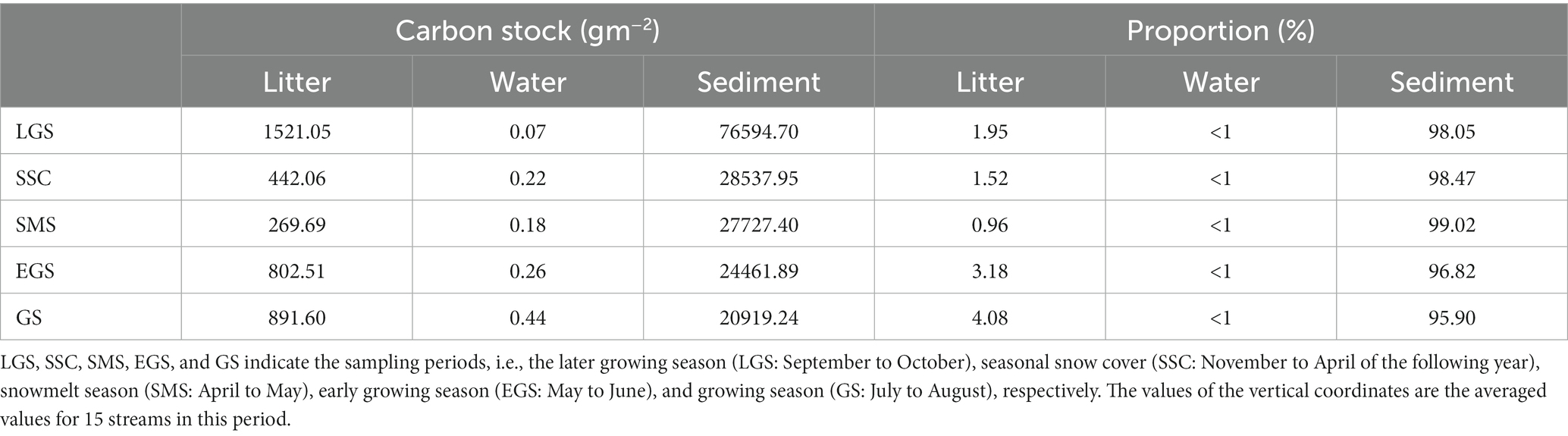
Table 4. Proportion of organic carbon in the forest-steam-river meta-ecosystems of the subalpine forest catchments.
In addition, the OC stocks fluctuated widely for the 15 forest streams in this study (Figure 5), and the average OC stock was 48.02 kg m−2, which was larger than the value reported by Collins and Walling (2007) (1.58 g kg−1 in the river Piddle’s sediment, England) and in the Frome River, England (the maximum OC stock reached 23.5 kg m−2; Heppell et al., 2009), lengths of forest streams might be the main factors. We found that the lowest OC stock was observed in river A, which had the longest length, while it was the highest in river L, which had the shortest length. A possible reason is that large streams experience less frequent water disturbances, which allows for less OC to be buried in the sediments during high-flow events than in smaller streams (Marttila and Kløve, 2014). The OC stock was significantly and negatively related to the flow rate of the forest streams, which provided evidence for this inference (Table 2). The results in this study all showed that small forest streams (less than 2 m wide) might act as carbon sinks via sediment OC storage, which has previously been overlooked and is consistent with the results of a study by Marttila and Kløve, 2014.
The OC concentration and stocks of the stream sediments fluctuated widely over time, and the most significant values consistently occurred after or during high flow events during the late growing period coinciding with the autumn rains (Figure 2), which was influenced by the temperature, precipitation, and stream characteristics along the streams during this one-year investigation (Table 1). The residues of plants, microbes, and dead animals buried at the bottom of the streams and organic matter released from the stream bottom sediments were the main sources of OC in the forest stream sediments (Qin et al., 2015). In particular, plant matter at the water surface plays important roles as C input to forest riparian zones (Yue et al., 2016; Hou et al., 2021), which is regulated by climatic factors. Compared with other periods, more litter entered the streams during the late growing season because it was the peak period for litterfall caused by low temperatures. Low temperatures can decelerate the mineralization rates for sediment OC, and the increased litter burial caused more OC to be stored in the sediments (Gudasz et al., 2010; Mendonça et al., 2012). Additionally, the high precipitation during this period can lead to more exogenous inputs, mainly from soil organic matter carried by surface runoff and underground runoff (Zhang et al., 2017; Hou et al., 2021). Both factors led to the greatest amount of OC being buried in the sediments during this period (Qin et al., 2015). The significant relationships between precipitation, temperature, and the OC stock provided positive evidence.
On the other hand, stream characteristics such as channel morphology, structure and texture, water velocity and depth, and macrophytes can affect sediment C storage conditions, which also vary with seasonal changes (Marttila et al., 2012; Hou et al., 2022). For example, some studies have shown that there is a significant negative correlation between the burial rate of OC in sediments and the water surface area (Downing et al., 2008). Mendonça et al. (2012) found that the burial rate of OC in sediments is significantly and positively correlated with the annual average runoff. In this study, we also found that sediment depth most significantly accounted for the variation in the sediment OC stock. During this one-year investigation, these effects were especially apparent during the late growing period, when the greatest amount of OC storage was observed due to high precipitation. Adams and Beschta (1980) also found that the maximum value of OC storage occurred during summer in Oregon, United States, which coincided with the period of greatest precipitation. Similar phenomena were also observed in studies by Walling et al. (2003) and Collins and Walling (2007). In contrast, the highest OC sediment stocks were reported during the winter months at some sites (Walling and Amos, 1999), which might be caused by the dense aquatic vegetation appeared in the channel of the Upper Piddle at many locations during the summer months, while the decay of this vegetation during the autumn and winter would produce substantial quantities of organic detritus but “grabbed” in the sediments due to frozen. Briefly, the seasonal dynamics of OC stocks in the sediments of forest streams are affected by seasonal climatic change, stream characteristics and their interactions.
4.2. Dynamics of the ratios of organic carbon upstream and downstream sediments
As our results noted, the organic carbon stocks of 15 forest stream sediments were highest in the upstream regions, and the ratios of the OC stocks in the upstream and downstream sediments were all above 1 during the five different critical periods. All the patterns appeared to be consistent with the variational periods; the maximum values were observed during the late growing season, and the minimum values were observed during the snowmelt periods (Figure 5). This was similar to observations by Marttila and Kløve (2014), who showed that the proportion of specific OC storage in sediments increased from downstream to upstream reaches. In contrast, Collins and Walling (2007) and Lopez-Tarazon et al. (2011) observed an increase in specific sediment OC storage from the upstream to downstream regions and attributed this to the substantial increase in channel area with increasing distance from the source. On the one hand, the decreasing trend found in this study may be because the sediment OC inputs from adjoined uplands stored in the headwater regions cannot be fully transported to downstream regions(Zhang et al., 2017). On the other hand, this might be caused by the characteristics of the OC stored upstream, which consists of more large eroded and undecomposed particulate organic matter that is transported from the catchment area, which is hard to be transported to the down steams (Marttila and Kløve, 2008, 2010), while the proportion of particulate OC in large rivers is typically small (less than <5%; Mattsson et al., 2003). Another possible reason for the downstream decrease in OC included changing hydrological pathways through soils and increasing proportions of postglacial sediments deposited in the lower-lying parts of catchments that reduced export because of OC adsorption to mineral surfaces(Zhang et al., 2017).
Based on this study, the proportion of OC upstream of small forest streams is larger than previously assumed. A study by Kortelainen et al. (2004) concluded that in Finland, aquatic ecosystems (lakes) contain the second-largest areal C stocks (19 kg C m−2) after peatlands (72 kg C m−2), but the present study showed that forest stream sediments, especially the sediments of upstream sections (the average OC stock upstream was 41.19 kg C m−2), can be a more important site for carbon storage and transport than lakes. Additionally, the stream bed sediments are not stable because resuspension can occur, thereby affecting the carbon cycle over shorter periods relative to lake deposits. While it was not possible to evaluate the residence times for organic matter in the headwaters of the streams in this study, the results clearly show that even though some seasonal and temporal variation occurred in stream bed storage, it was still quite stable under headwater conditions, although the amount of storage fluctuated. The sampling procedure did not include deeper river pool areas, which might represent more stable carbon pools within the main channel area. Therefore, in-depth investigations of sediment OC are important for developing the models and databases needed to fully understand the role of forest streams in the carbon cycle within a forest-stream ecosystem.
5. Conclusion
The total OC concentration and stocks of subalpine forest stream sediments showed different patterns during critical periods, and these were affected by seasonal changes in temperature and precipitation, meanwhile stream characteristics also played important roles in regulating the seasonal dynamics of OC in sediments. Seasonal precipitation and temperature patterns, stream sediment depth, and litter carbon input dominated the dynamics of OC stocks in the stream sediments. Additionally, sediments in the up reach reserved more OC than that in the downstream sections for the forest–stream meta-ecosystem, indicating that subalpine forest streams sediments may act as main carbon sources and play a critical role in linking the carbon biogeochemical cycles between subalpine forests and adjoining rivers. Vegetation species composition, nutrient availability, topography, fire, harvesting, and soil type all potentially influence the dynamics of the OC stocks in the watershed sediments of subalpine forests. However, due to the experimental conditions, these factors were not taken into account in this study, thus a more comprehensive examination of their relative influences could improve OC estimation and modeling.
Data availability statement
The original contributions presented in the study are included in the article/Supplementary material, further inquiries can be directed to the corresponding author.
Author contributions
WY and JH: conceptualization, methodology, validation, writing review and editing, and data curation. JH and FL: software. JH: formal analysis, writing–original draft preparation, and visualization. JH, FL, ZW, and XL: investigation. WY: resources, supervision, and project administration. WY and FL: funding acquisition. All authors have read and agreed to the published version of the manuscript.
Funding
This study was supported by the National Natural Science Foundation of China (32071554, 32001139) and the Taizhou Bureau of Science and Technology (22nya11).
Acknowledgments
The authors of this study would like to thank all the people who were involved in the initial sampling assignments.
Conflict of interest
The authors declare that the research was conducted in the absence of any commercial or financial relationships that could be construed as a potential conflict of interest.
Publisher’s note
All claims expressed in this article are solely those of the authors and do not necessarily represent those of their affiliated organizations, or those of the publisher, the editors and the reviewers. Any product that may be evaluated in this article, or claim that may be made by its manufacturer, is not guaranteed or endorsed by the publisher.
Supplementary material
The Supplementary material for this article can be found online at: https://www.frontiersin.org/articles/10.3389/fevo.2023.1093448/full#supplementary-material
References
Adams, J. N., and Beschta, R. L. (1980). Gravel bed composition in Oregon coastal streams. Can. J. Fish. Aquat. Sci. 37, 1514–1521. doi: 10.1139/f80-196
Ågren, A. M., Buffam, I., Cooper, D. M., Tiwari, T., Evans, C. D., and Laudon, H. (2014). Can the heterogeneity in-stream dissolved organic carbon be explained by contributing landscape elements? Biogeosciences 11, 1199–1213. doi: 10.5194/bg-11-1199-2014
Ågren, A. M., Buffam, I., and Jansson, M. (2007). Importance of seasonality and small streams for the landscape regulation of dissolved organic carbon export. J. Geophys. Res. Biogeosci. 112:381. doi: 10.1029/2006JG000381
Bates, D., Maechler, M., Bolker, B., and Walker, S. (2015). Fitting linear mixed-effects models using lme4. J. Stat. Softw. 67, 1–48. doi: 10.18637/jss.v067.i01
Battin, T. J., Luyssaert, S., Kaplan, L. A., Aufdenkempe, A. K., Richter, A., and Tranvik, L. J. (2009). The boundless carbon cycles. Nat. Geosci. 2, 598–600. doi: 10.1038/ngeo618
Cleveland, C. C., Reed, S. C., Keller, A. B., Nemergut, D. R., O’Neill, S. P., Ostertag, R., et al. (2014). Litter quality versus soilmicrobial community controls over decomposition: a quantitative analysis. Oecologia 174, 283–294. doi: 10.1007/s00442-013-2758-9
Clow, D. W., Stackpoole, S. M., Verdin, K. L., Butman, D. E., Zhu, Z., Krabbenhof, D. P., et al. (2015). Organic carbon burial in lakes and reservoirs of the conterminous United States. Environ. Sci. Technol. 49, 7614–7622. doi: 10.1021/acs.est.5b05076
Cole, J. J., Prairie, Y. T., Caraco, N. F., McDowell, W. H., Tranvik, L. J., Striegl, R. G., et al. (2007). Plumbing the global carbon cycle: integrating inland waters into the terrestrial carbon budget. Ecosystems 10, 172–185. doi: 10.1007/s10021-006-9013-8
Collins, A. L., and Walling, D. E. (2007). Fine-grained bed sediment storage within the main channel systems of the Frome and piddle catchments, Dorset, UK. Hydrol. Process. 21, 1448–1459. doi: 10.1002/hyp.6269
Dean, W. E., and Gorham, E. (1998). Magnitude and significance of carbon burial in lakes, reservoirs, and peatlands. Geology 26, 535–538. doi: 10.3133/fs05899
Depetris, P. A., and Kempe, S. (1993). Carbon dynamics and sources in the Parana River. Limnol. Oceanogr 38, 382–395.
Donato, D. C., Boone, K. J., Murdiyarso, D., Kurnianto, S., Stidham, M., and Kanninen, M. (2011). Mangroves among the most carbon-rich forests in the tropics. Nat. Geosci. 4, 293–297. doi: 10.1038/ngeo1123
Downing, J. A., Cole, J. J., Middelburg, J. J., Striegl, R. G., Duarte, C. M., Kortelainen, P., et al. (2008). Sediment organic carbon burial in agriculturally eutrophic impoundments over the last century. Global Biogeochem. Cycles 22:GB1018. doi: 10.1029/2006GB002854
Duarte, C. M., Middelburg, J. J., and Caraco, N. (2004). Major role of marine vegetation on the oceanic carbon cycle. Biogeosci. Discuss. 2, 1–8. doi: 10.5194/bg-2-1-20052005
Einsele, G., Yan, J. P., and Hinderer, M. (2001). Atmospheric carbon burial in modern lake basins and its significance for the global carbon budget. Glob. Planet. Change 30, 167–195. doi: 10.1016/S0921-8181(01)00105-9
Fenner, N., and Freeman, C. (2013). Carbon preservation in humic lakes; a hierarchical regulatory pathway. Glob. Chang. Biol. 19, 775–784. doi: 10.1111/gcb.12066
Ferland, M. E., Giorgio, P. A. D., Teodor, C. R., and Prairie, Y. T. (2012). Long-term C accumulation and total C stocks in boreal lakes in northern Québec. Global Biogeochem. Cycles 26:GBOE04. doi: 10.1029/2011GB004241
Frost, P. C., Benstead, J. P., Cross, W. F., Hillebrand, H., Larson, J. H., Xenopoulos, M. A., et al. (2006). Threshold elemental ratios of carbon and phosphorus in aquatic consumers. Ecol. Lett. 9, 774–779. doi: 10.1111/j.1461-0248.2006.00919.x
Gomes, T. F., Broek, M. V., Govers, G., Silva, R. W. C., Moraes, J. M., Camargo, P. B., et al. (2019). Runoff, soil loss, and sources of particulate organic carbon delivered to streams by sugarcane and riparian areas: an isotopic approach. Catena 181, 104083–104089. doi: 10.1016/j.catena.2019.104083
Graça, M. A., Ferreira, V., Canhoto, C., Encalada, A. C., Guerrero-Bolaño, F., Wantzen, K. M., et al. (2015). Conceptual model of litter breakdown in low order streams. Int. Rev. Hydrobiol. 100, 1–12. doi: 10.1002/iroh.201401757
Guan, Q. S. (2022). Physicochemical properties of mangrove sediments in Jiulong River, Estuary. Forest Sci. Technol. 12, 809–823. doi: 10.4236/jep.2021.1211048
Gudasz, C., Bastviken, D., Premke, K., Steger, K., and Tranvik, L. J. (2012). Constrained microbial processing of allochthonous organic carbon in boreal lake sediments. Limnol. Oceanogr. 57, 163–175. doi: 10.4319/lo.2012.57.1.0163
Gudasz, C., Bastviken, D., Streger, K., Premke, K., Sobek, S., and Tranvik, L. J. (2010). Temperature-controlled organic carbon mineralisation in lake sediments. Nature 466, 478–481. doi: 10.1038/nature09186
Heppell, C. M., Wharton, G., Cotton, J. A. C., Bass, J. A. B., and Roberts, S. E. (2009). Sediment storage in the shallow hyporheic of lowland vegetated reaches. Hydrol. Process. 23, 2239–2251. doi: 10.1002/hyp.7283
Hou, J. F., Li, F., Wang, Z. H., Li, X. Q., Cao, R., and Yang, W. Q. (2022). Dynamics of dissolved carbon in subalpine Forest streams. Forests 13:795. doi: 10.3390/f13050795
Hou, J. F., Li, F., Wang, Z. H., Li, X. Q., and Yang, W. Q. (2021). Budget of plant litter and litter. Carbon in the subalpine forest streams. Forests 12:1764. doi: 10.3390/f12121764
Kortelainen, P., Pajunen, H., Rantakari, M., and Saarnisto, M. (2004). A large carbon pool and small sink in boreal Holocene Lake sediments. Glob. Chang. Biol. 10, 1648–1653. doi: 10.1002/hyp.7283
Kunz, M. J., Anselmetti, F., Wüest, A., Wehrli, B., Vollenweider, A., Thüring, S., et al. (2011). Sediment accumulation and carbon, nitrogen, and phosphorus deposition in the large tropical reservoir Lake Kariba (Zambia/Zimbabwe). J. Geophys. Res. Biogeosci. 116:G0300. doi: 10.1029/2010JG001538
Liu, J. W., Yu, X. L., Zhang, J. B., Yao, Q. C., and Hu, B. Q. (2016). Analysis on carbon, nitrogen and phosphorus enrichment characteristics of sediments in urban parts along uprivers to downriver of a typical river bounding for sea in Beibu gulf, Guangxi. Environ. Pollut. Control 38, 36–41. doi: 10.15985/j.cnki.1001-3865.2016.07.007
Lopez-Tarazon, J. A., Batalla, R. J., and Vericat, D. (2011). In-channel sediment storage in a highly erodible catchment: the river Isabela (Ebro Basin, southern Pyrenees). Z. Geomorphol. 55, 365–382. doi: 10.1127/0372-8854/2011/0045
Lu, R. K.. (1999). Soil agrochemical analysis methods. China Agricultural Science and Technology Press: Beijing, China.
Luo, Z., Ma, J. M., Zheng, S. L., Nan, C. Z., and Nie, L. M. (2015). Different hydrodynamic conditions on the deposition of organic carbon in sediment of two reservoirs. Hydrobiologia 765, 15–26. doi: 10.1007/s10750-015-2410-2
Mao, Y., Ma, Q., Lin, J., Chen, Y., and Shu, Q. (2021). Distribution and sources of organic carbon in surface intertidal sediments of the Rudong coast, Jiangsu Province, China. J. Mar. Sci. Eng. 9, 1–14. doi: 10.3390/jmse9090992
Marttila, H., and Kløve, B. (2008). Erosion, and delivery of deposited peat sediment. Water Resour. Res. 44:W06406. doi: 10.1029/2007WR006486
Marttila, H., and Kløve, B. (2010). Dynamics of erosion and suspended sediment transport from drained peatland forestry. J. Hydrol. 388, 414–425. doi: 10.1016/j.jhydrol.2010.05.026
Marttila, K., and Kløve, B. (2014). Storage, properties, and seasonal variations in fine grained bed sediment within the main channel and headwaters of the river Sanginjoki, Finland. Hydrol. Process. 28, 4756–4765. doi: 10.1002/hyp.9953
Marttila, H., Tammela, S., and Kløve, B. (2012). Hydraulic geometry, hydraulics and sediment properties of Forest brooks after extensive Erosion from upland Peatland drainage. Open J. Mod. Hydrol. 2, 59–69. doi: 10.4236/ojmh.2012.23008
Mattsson, T., Finer, L., Kortelainen, P., and Sallantaus, T. (2003). Brook water quality and background leaching from unmanaged forested catchments in Finland. Water Air Soil Pollut. 147, 275–298. doi: 10.1023/A:1024525328220
Mei, J. F., Jia, J. P., Bi, C. J., Liu, J. P., Zeng, Y. S., and Chen, Z. L. (2022). Effects of human enclosure and farming activities on heavy metals in sediments/soils of the coastal reclamation areas in the Yangtze estuary. J. Soils Sediments 22, 2435–2447. doi: 10.1007/s11368-022-03248-2
Mendonça, R., Kosten, S., Sobek, S., Barros, N., Cole, J. J., Tranvik, L., et al. (2012). Hydroelectric carbon sequestration. Nat. Geosci. 5, 838–840. doi: 10.1038/ngeo1705
Mendonça, R., Kosten, S., Sobek, S., Cole, J. J., Bastos, A. C., Albuquerque, A. L., et al. (2014). Carbon sequestration in a large hydroelectric reservoir: an integrative seismic approach. Ecosystems 17, 430–441. doi: 10.1007/s00442-013-2758-9
Mulholland, P. J., and Stallard, R. F. (1998). Terrestrial sedimentation and the carbon cycle: coupling weathering and erosion to carbon burial. Global Biogeochem. Cycles 12, 231–257. doi: 10.1029/98GB00741
Nellemann, C., Corcoran, E., Duarte, C. M., Valdes, L., De, Y. C., Fonseca, L., et al. (2009). Blue carbon: The role of healthy in binding carbon: A rapid response assessment ; United Nations Environment Programme, GRID-Arenal: Arendal, Norway.
Öquist, M. G., Wallin, M., Seibert, J., Bishop, K., and Laudon, H. (2009). Dissolved inorganic. Carbon export across the soil/stream interface and its fate in a boreal headwater stream. Environ. Technol. 43, 7364–7369. doi: 10.1021/es900416h
Pittman, B., Jones, J. R., Millspaugh, J. J., Kremer, R. J., and Downing, J. A. (2013). Sediment organic carbon distribution in 4 small northern Missouri impoundments: implications for sampling and carbon sequestration. Inland Waters. 3, 39–46. doi: 10.5268/IW-3.1.507
Qin, J., Yang, H., Zhang, M. L., Xie, B., Li, J., Sun, P. P., et al. (2015). Sources of organic carbon in sediments of Baoxanghe reservoir in Dianchi Basin. Geogr. Res. 34, 53–64. doi: 10.11821/dlyj201501005
Smith, R. W., Bianchi, T. B., Allison, M., Savage, C., and Galy, V. (2015). High rates of organic carbon burial in fjord sediments globally. Nat. Geosci. 8, 450–453. doi: 10.1038/ngeo2421
Sobek, S., Durisch-Kaiser, E., Zurbrü, R., Wongfun, N., Woessels, M., Pasche, N., et al. (2009). Organic carbon burial efficiency in lake sediments controlled by oxygen exposure time and sediment source. Limnol. Oceanogr. 54, 2243–2254. doi: 10.1038/ngeo2421
Sutherland, A. B., Meyer, J. L., and Gardiner, E. P. (2002). Effects of land cover on sediment regime and fish assemblage structure in four southern Appalachian streams. Freshw. Biol. 47, 1791–1805. doi: 10.1046/j.1365-2427.2002.00927.x
Tranvik, L. J., Downing, J. A., Cotner, J. B., Loiselle, S. A., Striegl, R. G., Ballatore, T. J., et al. (2009). Lakes and impoundments as regulators of carbon cycling and climate. Limnol. Oceanogr. 54, 2298–2314. doi: 10.4319/lo.2009.54.6_part_2.2298
Wallace, J. B., Eggert, S. L., Meyer, J. L., and Webster, J. R. (1997). Multiple trophic levels of a forest stream are linked to terrestrial litter inputs. Science 277, 102–104. doi: 10.1126/science.277.5322.102
Walling, D. E., and Amos, C. M. (1999). Source, storage, and mobilization of fine sediment in a chalk stream system. Hydrol. Process. 13, 323–340. doi: 10.1002/(SICI)1099-1085(19990228)13:3<323::AID-HYP741>3.0.CO;2-K
Walling, D. E., Owens, P. N., Carter, J., Leeks, G. J. L., Lewis, S., Meharg, A. A., et al. (2003). Storage of sediment-associated nutrients and contaminants in river channels and floodplain systems. Appl. Geochem. 18, 195–220. doi: 10.1016/S0883-2927(02)00121-X
Wallin, M. B., Grabs, T., and Buffam, I. (2013). Evasion of CO2 from streams–the dominant component of the carbon export through the aquatic conduit in a boreal landscape. Glob. Chang. Biol. 19, 785–797. doi: 10.1111/gcb.12083
Wang, F. S., Lang, Y. C., Liu, C. Q., Qin, Y., Yu, N. X., and Wang, B. L. (2019). Flux of organic carbon burial and carbon emission from a large reservoir: implications for the cleanliness assessment of hydropower. Chin. Sci. Bull. 64, 603–611. doi: 10.1016/j.scib.2019.03.034
Winterdahl, M., Erlandsson, M., Futter, M. N., Weyhenmeyer, G. A., and Bishop, K. (2014). Intra-annual variability of organic carbon concentrations in running waters: drivers along a climatic gradient. Global Biogeochem. Cycles 28, 451–464. doi: 10.1002/2013GB004770
Wu, F. Z., Peng, C. H., Zhu, J., Zhang, J., Tan, B., and Yang, W. Q. (2014). Impact of freezing and. Thawing dynamics on foliar litter carbon release in alpine/subalpine forests along an altitudinal gradient in the eastern Tibetan plateau. Biogeosciences 11, 6471–6481. doi: 10.5194/bgd-11-9539-2014
Xie, M. J., Mladenov, N., Williams, M. W., Neff, J. C., Wasswa, J., and Hannigan, M. P. (2016). Water-soluble organic aerosols in the Colorado Rocky Mountains, USA: composition, sources and optical properties. Sci. Rep. 6:39339. doi: 10.1038/srep39339
Yang, W. Q., and Wu, F. Z.. (2021). Ecosystem processes and management of subalpine coniferous forest in the upper reaches of the Yangtze River. Science Press. Beijing.
Yue, K., Yang, W., and Peng, C. (2016). Foliar litter decomposition in an alpine forest meta-ecosystem on the eastern Tibetan plateau. Sci. Total Environ. 566–567, 279–287. doi: 10.1016/j.scitotenv.2016.05.081
Zhang, Z. B., Liu, T., Cao, Q. M., Zhang, J., and Deng, H. G. (2020). Spatial distribution and stoichiometric characteristics of C, N, P in the sediment-Potamogeton crispus system in Dongping Lake. Environ. Chem. 39, 2263–2271. doi: 10.7524/j.issn.0254-6108.2019061401
Zhang, Y., Yang, W. Q., Tan, B., Liang, Z. Y., and Wu, F. Z. (2017). Contents and output characteristics of dissolved carbon in the water body of alpine forest headwater streams in rainfall season. J. Univ. Chin. Acad. Sci. 34, 515–520. doi: 10.7523/j.issn.2095-6134.2017.04.014
Zhong, H., and Wang, W. X. (2008). Effects of sediment composition on inorganic. Mercury partitioning, speciation, and bioavailability in oxic surficial sediments. Environ. Pollut. 151, 222–230. doi: 10.1016/j.envpol.2007.01.049
Keywords: forest stream, organic carbon, sediment carbon, stream characteristics, carbon stocks
Citation: Hou J, Li F, Wang Z, Li X, Cao R and Yang W (2023) Seasonal dynamics of sediment organic carbon storage for the upper streams of the Yangtze River. Front. Ecol. Evol. 11:1093448. doi: 10.3389/fevo.2023.1093448
Edited by:
Junjie Lin, Chongqing Three Gorges University, ChinaReviewed by:
Muhammad Faheem, Nanjing University of Information Science and Technology, ChinaLiping He, Chongqing Three Gorges University, China
Yanzhong Li, Nanjing University of Information Science and Technology, China
Copyright © 2023 Hou, Li, Wang, Li, Cao and Yang. This is an open-access article distributed under the terms of the Creative Commons Attribution License (CC BY). The use, distribution or reproduction in other forums is permitted, provided the original author(s) and the copyright owner(s) are credited and that the original publication in this journal is cited, in accordance with accepted academic practice. No use, distribution or reproduction is permitted which does not comply with these terms.
*Correspondence: Wanqin Yang, c2N5YW5nd3FAMTYzLmNvbQ==