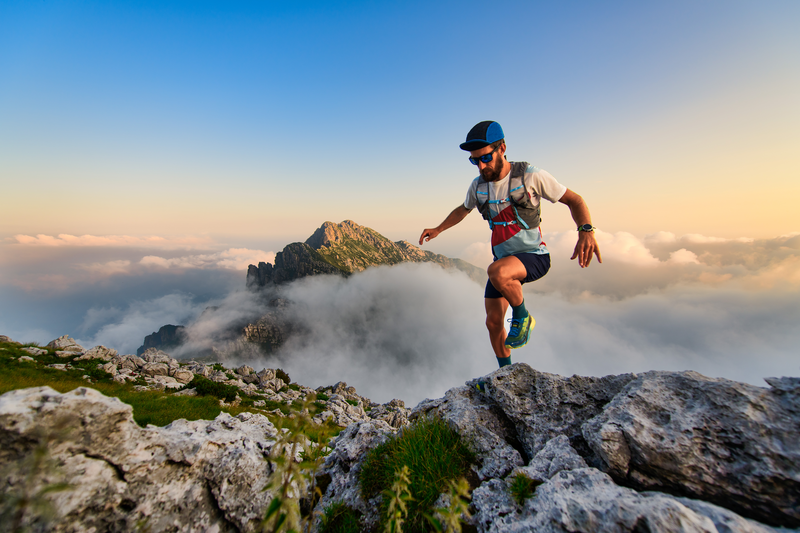
94% of researchers rate our articles as excellent or good
Learn more about the work of our research integrity team to safeguard the quality of each article we publish.
Find out more
ORIGINAL RESEARCH article
Front. Ecol. Evol. , 17 April 2023
Sec. Biogeography and Macroecology
Volume 11 - 2023 | https://doi.org/10.3389/fevo.2023.1092840
This article is part of the Research Topic Ecosystem Nutrients Stoichiometry From Local to Global Scales View all 8 articles
Introduction: The application of phosphorus (P) fertilizer can promote photosynthesis in forage grasses and accelerate their establishment.
Methods: To improve the utilization efficiency of P fertilizer for bluegrass (Poa L.) in alpine regions, the effects of P fertilizer on their growth, and carbon (C), nitrogen (N) and P distribution in their different organs of them are tested at six P fertilization levels (3, 6, 9, 12, 15, 18 g·m−2).
Results: (1) The nutrient content in each organ of bluegrass varies during different growth stages, with the lowest nutrient content occurring in the wilt stage. (2) The response of the nutrient content and ratio of each organ of bluegrass to different P fertilization levels varies. When the P application rate was 15 g·m−2, the contents of N and P in roots were the highest, and their C/N and C/P ratios were the lowest. When the P application rate was 12 g·m−2, the contents of N and P in the stems were the highest, and their C/N and C/P ratios were the lowest. When the P application rate was 9 g·m−2, the contents of N and P in leaves were the highest, and their C/N and C/P ratios were the lowest. When the P application rate was 6 g·m−2 the contents of N and P in the panicle were the highest, and their C/N and C/P ratios were the lowest.
Discussion: These results provide a better understanding of the effect of P fertilization in the nutrient partitioning pattern of perennial forage plant organs in alpine regions. The information from this study can support a more reasonable P fertilization for the establishment of early grassland. For example, in artificial forage grassland, the application of low-concentration P fertilizer (6~9 g·m−2) can promote the nutrient content in spikes and stems of forage grass; for ecological management, the application of high-concentration P fertilizer (15 g·m−2) can promote the nutrient content in roots and enhance the ecological benefits of forage grassland.
Ecological stoichiometry observes the balance between energy and multiple chemical elements (e.g., C, N, and P) at different levels of a biosphere; thus, it can be used to explore physiological and biochemical processes/interactions of multiple elements in plants, and it has been widely used in terrestrial ecosystems studies and other applications (They et al., 2017; Zheng et al., 2018; Moody and Wilkinson, 2019; Sterner and Elser, 2022). Carbon (C) is the substrate and energy source for many physiological and biochemical processes in plants. Nitrogen (N) and phosphorus (P) are important components of various proteins and genetic materials and the main limiting factors in terrestrial ecosystems (Elser et al., 2007; Su et al., 2019; Wang et al., 2022). Elemental ratios are a useful tool for understanding elemental biogeochemical cycling, as well as connecting different levels of plant biology from organs to ecosystems (Li et al., 2018; Wang et al., 2019). For example, C/N and C/P ratios represent the ability of plants to assimilate C, the plant growth rate, and the nutrient-using efficiency during nutrient uptake (Ågren, 2004). N/P is a key indicator to determine community structure and function and can be used to indicate nutrient elements that limit productivity (Sardans et al., 2021). Therefore, understanding the relationship between the C, N, and pin plants can improve our understanding of plant growth and development as well as their strategies for adapting.
The alpine grassland is one of the dominant grassland types on the Qinghai-Tibet Plateau, and it is particularly sensitive to changes in environmental conditions (Lin et al., 2015; Wei et al., 2020). The genus of bluegrass is distributed widely across the alpine grassland of the Qinghai-Tibet Plateau. Fertilizer application is an effective management practice to enhance the productivity of grassland communities in seminatural and artificial grasslands (Stevens et al., 2015; Soons et al., 2017). Plants promote their growth by converting P into nucleic acids (Reich et al., 2003), and P plays an important role in regulating the metabolism and energy conversion in living organisms; thus, P was considered one of the common limiting factors in the primary productivity of terrestrial ecosystems (Elser et al., 2009; Hidaka and Kitayama, 2009; Lu and Tian, 2017). Therefore, it is imperative to maintain a sustainable C, N, and P stoichiometric balance in the soil ecosystem, and stoichiometric balance could facilitate and maximize agricultural productivity (Yuan et al., 2019). The addition of P fertilizer to the soil can increase C sequestration (Mori et al., 2010; He and Dijkstra, 2015), which, in turn, can disrupt the balance between C, N, and P in a plant; consequently, C/N, C/P, and N/P ratios are also markedly changed (Peñuelas et al., 2013). Previous studies have found that adding P fertilization can directly increase N and P concentrations in plant tissues, thus, reducing C/N, C/P, and N/P (Ostertag, 2010; Mao et al., 2016). However, few studies have found that the effect of additional P in nutrient concentrations in plants varies with different growth stages and organ tissues. Different organs of plants have different physiological functions, and these differences also lead to differences in nutrient allocation trade-offs among organs (Minden et al., 2014; Luo et al., 2021). In addition, the difference in genetic backgrounds in plants affects their survivability and reproductivity. Plants constantly adjust the distribution of their nutrient content to adapt to environmental changes at different growth stages, which results in differences in nutrient content and stoichiometric ratios in plants at different reproductive stages (Gao et al., 2019; Lu et al., 2019; Wang et al., 2020a). The C:N:P stoichiometric ratio of plants is greatly affected by nutrient availability, which can reflect the chemical element cycle between the environment and plants. For a plant organ in a given growth stage, its dominant limiting nutrient varies with different stoichiometric ratios. For example, N is the dominant limited nutrient when the N/P ratio is less than 14; similarly, P becomes the dominant limited nutrient when the N/P ratio is more than 16 (Verhoeven et al., 1996). Therefore, changes in C, N, and P contents and their stoichiometric ratios in plants could expand our understanding of the nutrient status of plants during the growth process and the utilization of nutrients by plants after adding P fertilizer. Bluegrass is an edible forge in alpine grassland, and its vigorously expanding root systems have crucial roles in the restoration of degraded grassland and the maintenance of the ecological functions of the grassland ecosystem. Therefore, according to different production uses, reasonable and efficient cultivation measures help to improve production efficiency and effectiveness. Based on these studies (Mao et al., 2016; Gong et al., 2022), plants show more sensitivity to P addition; therefore, we hypothesized that P addition has a significant effect on the stoichiometric characteristics of newly developed grasslands at different growth stages. Stoichiometric traits and allocating patterns among organs for nutrient contents in bluegrass were examined at different reproductive stages. These hypotheses were clarified as follows: (1) the addition of P significantly increased the nutrient content of each organ and increased the yield of forage grass and (2) the nutrient distribution among different organs should be different to achieve an improvement in the efficiency of nutrient utilization of plants.
The study site is located in the National Forage Germplasm Resource Park in Xihai town, Haibei Prefecture, Qinghai Province (36°59′18″ N, 100°53′16″ E; elevation: 3156 m), with a highland continental climate, a chestnut-calcium soil texture, mean annual precipitation (MAP) of approximately 400 mm, and mean annual temperature (MAT) of 0.9°C. The relative nutrient concentrations in the 0 ~ 30 cm depth soil layer were: organic matter (OM) 34.46 g kg−1, total nitrogen (TN) 1.47 g kg−1, total phosphorus (TP) 1.42 g kg−1, alkaline nitrogen (AN) 84.7 mg kg−1, available phosphorus (AP) 2.4 mg kg−1, available potassium (AK) 147.2 mg kg−1, and acidity of (pH) 8.16.
In this study, six P fertilizer gradients and a control group were designed for the artificial grass of Poa pratensis var. anceps Gaud cv. Qinghai (strip sown at a density of 0.75 g·m−2, depth 2–3 cm, row spacing 30 cm, and preceding crop of Avena sativa L.), namely CK (control), P1 (3 g·m−2), P2 (6 g·m−2), P3 (9 g·m−2), P4 (12 g·m−2), P5 (15 g·m−2), and P6 (18 g·m−2). In addition, calcium superphosphate (P2O5, 12%) was also used as fertilizer. The experiment was conducted with a completely randomized group design with three replications and a plot area of 3 m × 9 m at an interval of 1 m. Fertilizer (and supplemental fertilizer) was uniformly spread in the test plot once in July 2019.
Sampling was conducted in August (growth stage), September (mature stage), and October (wilt stage) 2019. A 50 cm × 50 cm sample square was randomly selected in each treatment plot, and the aboveground organs, including the stem and leaves, were harvested and retained with 0.2 cm stubble height; meanwhile, the 0 to 30 cm root system was collected through a root auger with 10 cm diameter. Root samples were carefully collected by buffering the inter-root soil with water. According to the different organs of plants, spikes, leaves, stems, and roots were stored in different labeled envelope bags, and these samples were dried at 75°C until they had a constant weight, and they were weighed separately. Healthy stems, leaves, spikes, and roots were crushed with a grinder and passed through a 1 mm sieve; 0.20 g grind samples were weighed to determine the contents of organic carbon (OC), total nitrogen (TN), and total phosphorus (TP).
Plant organs were grounded with a ball mill to allow for the measurement of C, N, and P concentrations for each organ at each growth stage. The OC content was determined by the potassium dichromate volumetric method; TN content was determined by the concentrated sulfuric acid digestion semiautomatic Kjeldahl method; TP content was determined by the triacid digestion molybdenum antimony anti-colorimetric method (Luo et al., 2021). The C, N, and P concentrations were expressed as mass fractions. C/P, C/N, and N/P were expressed as mass fraction ratios.
The allocation of C, N, and P to organs at the end of each growth stage was calculated as follows:
where Er is the C, N, and P allocation ratio, Ei is the C, N, and P concentrations (mg/g); Bi is the organ dry biomass (g); Ti is the total plant content of C, N, and P (mg).
One-way ANOVA was used to determine the effects of P fertilizer gradients on the C, N, and P concentrations and stoichiometry ratios, and the effects of P fertilizer gradients on the organ dry biomass were also determined. Two-way ANOVA was used to analyze the effects of growth stages and increased P on the C, N, and P stoichiometric ratios and the dry biomass of each organ. Data analyses were performed using SPSS 26.0 software, and figures were generated using Origin 9.0 software.
There were differences in the C, N, and P concentrations at different growth stages. In the control group, the plant’s C, N, and P contents were highest in the growth stage, then gradually decreased with plant growth, and reached their lowest levels in the wilt stage. The P content of roots and stems was significantly reduced at the wilt stage, and the N content of leaves and spikes was significantly reduced at the wilt stage (Figure 1). In the control group, the plant’s C/N and C/P ratios were highest in the wilt stage and lowest in the growth stage. The roots and stems’ N/P ratios were highest in the wilt stage and lowest in the mature stage. The leaves and spikes’ N/P ratios were highest in the mature stage and lowest in the wilt stage (Figure 2).
Figure 1. The effect of P fertilizer gradient addition on C, N, and P concentrations in different organs (A, root; B, stem; C, leaf; D, spike) at different growth stages of bluegrass. Different lowercase letters indicate significant differences among different phosphorus additions at p < 0.05.
Figure 2. The effect of P fertilizer gradient addition on C/N, C/N, and N/P in different organs (A, root; B, stem; C, leaf; D, spike) at different growth stages of bluegrass. Different lowercase letters indicate significant differences among different phosphorus additions at p < 0.05.
There were significant differences in the effects of increased P fertilization on the C, N, and P concentrations in plant organs (Figure 1). When the fertilizer applied was less than 9 g·m−2, the roots’ C concentration increased with an increasing P concentration at different fertility levels. When the fertilizer applied was greater than 9 g·m−2, the roots’ C concentration decreased slowly during the growth period, while the roots’ C concentration decreased significantly during the mature period, and a decrease in the C concentration during the wilting period occurred when the fertilizer application was greater than 12 g·m−2. The N and P concentrations of roots were the highest when the fertilizer applied was at 15 g·m−2 and decreased significantly when the fertilizer application was greater than 15 g·m−2 (Figure 1A). The C, N, and P concentrations of the stems were the highest at different fertility stages at a fertilizer application rate of 12 g·m−2 and significantly decreased at a fertilizer application rate greater than 12 g·m−2. The P concentration of the stems was significantly lower in the wilt stage than in the growth and mature stages at all P treatment concentrations (Figure 1B). The leaves’ C concentrations were the highest at a fertilizer application rate of 12 g·m−2, and the N and P concentrations were the highest at a fertilizer application rate of 9 g·m−2, with the leaves’ N concentrations being significantly lower in the wilt stage than in the growth and mature stages at all P application rates (Figure 1C). The C concentration of the spikes reached its highest value at a fertilizer application rate of 12 g·m−2, and the N and P concentrations reached their highest value at a fertilizer application rate of 6 g·m−2 (Figure 1D).
There were differences in the effects of increased P fertilization on the C/N, C/P, and N/P ratios of plant organs (Figure 2). P fertilizer gradients significantly reduced the C/N and C/P in roots, with both of them reaching the lowest value at a fertilizer application rate of 15 g·m−2, and these ratios were highest at the wilt stage. The N/P ratio in the roots first decreased, then showed an increasing trend at the wilt stage (Figure 2A). The C/N and C/P ratios of stems were the lowest at a fertilizer application rate of 12 g·m−2, and the C/P and N/P ratios of stems were significantly higher at the wilt stage than at the mature and other growth stages (Figure 2B). The C/N, C/P, and N/P ratios of leaves were the lowest under the fertilizer application rate of 9 g·m−2 and showed a decreasing and then an increasing trend through different fertility stages. The C/N ratio of leaves was significantly higher at the wilt stage than at the mature and other growth stages (Figure 2C). The C/N and C/P ratios of the spikes were the lowest at a fertilizer application rate of 6 g·m−2 and the highest at a fertilizer application rate of 12 g·m−2. The C/N ratio of the spikes was significantly higher at the wilt stage than at the mature and other growth stages (Figure 2D).
According to the two-way ANOVA results, the effects of increased P fertilization and life stages, and their interaction on the C, N, and P concentrations and ratios were different. P fertilizer gradients and life stages had significant effects on the stoichiometric characteristics and ratios of all organs, with no significant effects of P fertilizer gradients and life stages on the stems and roots’ N/P ratio, respectively. The indicators that were affected by the interaction between the P fertilizer gradients and life stages were the roots’ N and P stoichiometry and C/N and N/P ratios; stems’ N and P stoichiometry and C/P ratio; leaves’ C/N and N/P ratios; spikes’ C/N and N/P ratios (Table 1).
Table 1. Two-way ANOVA (F value) of the effect of increased phosphorus and life stages, and their interaction on organs’ C, N, and P stoichiometry in bluegrass.
The dry biomass of plants was influenced by P and the plants’ life stages. In particular, at the different growth stages, the leaf, stem, root, and spike dry biomass and total biomass were significantly higher under the P fertilizer gradient treatments than under the control treatment. This result showed that plant organ dry biomass significantly increased with increased P at the different growth stages. With increased P fertilization, the roots’ C, N, and P contents decreased and then increased; the stems, leaves, and spikes’ C, N, and P contents increased and then decreased (Figure 3). The Roots’ C, N, and P contents reached their maximum values at the fertilizer application rate of 18 g·m−2 and increased by 3.57, 16.03, and 5.71%, respectively, compared to the control treatment; the stems’ C, N, and P contents reached their maximum values at the fertilizer application rate of 12 g·m−2 and increased by 54.46, 52.80, and 71.08%, respectively, compared to the control treatment; the leaves’ C, N, and P contents reached their maximum values at the fertilizer application rate of 9 g·m−2 and increased by 46.96, 23.37, and 80.10%, respectively, compared to the control treatment; the spikes’ C, N, and P contents reached their maximum values at the fertilizer application rate of 6 g·m−2 and increased by 55.53, 53.70, and 46.58%, respectively, compared to the control treatment. This result indicates that under a low application rate of exogenous P, plants preferentially allocate nutrients to aboveground parts during the growth period.
Figure 3. The effect of P fertilizer gradients on the distribution of C, N, and P contents in different organs at the different growth stages of bluegrass.
Scatter plots of N and P contents and the N/P ratio of each organ were analyzed to obtain the relationship between N/P as a function of N(y) and P (x) for each organ (Figure 4). N/P was significantly positively correlated with the N content and significantly negatively correlated with the P content. The linear equations of root N/P with N and root N/P with P were y = 0.119x + 0.314 and y = −0.110x + 1.416, respectively; the linear equations of stem N/P with N and stem N/P with P were y = 0.063x + 0.672 and y = −0.161x + 1.741, respectively; the linear equations of leaf N/P with N and leaf N/P with P were y = 0.115x + 1.047 and y = −0.226x + 3.467, respectively; the linear equations of spike N/P with N and spike N/P with P were y = 0.136x + 0.689 and y = −0.266x + 3.914, respectively. Scatter plots of the N/P ratio and N and P contents of each organ showed significant trends, and the correlation coefficients of the equations were all greater than 0.6, indicating a high correlation and reaching a highly significant level.
Figure 4. Scatter plots and best-fit lines of N/P ratio and N and P contents in different organs of bluegrass.
The nutrient-distributing patterns of each organ within plants are largely determined by exogenous nutrient supply, life history, and physiological traits for some specific plants, which is also known as the outcome of plant adaption for survival conditions (Kerkhoff et al., 2006; Mao et al., 2016; Wang et al., 2020b). Our results indicated that the highest C, N, and P contents were detected in varied organs of bluegrass during the flowering reproductive stage, while these contents sharply decreased in the reproductive stage. These findings which were consistent with the results of a study on Eremopyrum distans suggested that the concentrations of C, N, and P varied in different organs of E. distans in different growth stages (Zhang et al., 2022). The higher nutrient content at the flowering reproductive stage is because plants may invest more nutrients at the flowering reproductive stage to build more efficient reproductive organs (e.g., seeds and rhizomes) (Sun and Chen, 2001). However, our results are inconsistent with the outcome of the N and P concentrations in the leaves of Centaurea pulchella, in which N and P concentrations increased in reproductive growth stages (Xiao et al., 2014). The possible reason is related to its life history because more than one-third of its growth period is occupied by reproduction; consequently, a large amount of N and P nutrients were absorbed and allocated to the reproductive organ to ensure the offspring’s reproduction.
The stoichiometric traits of different organs in the plant varied in different species; our results demonstrated that the nutrient contents in the various organs of bluegrass were different. For example, N content in the leaves was higher than in the roots and stems, which was consistent with previous studies (Li et al., 2013). Different plant organs bear different physiological attribute functions, leading to significant differences in the element content and ratio of each organ (Tong et al., 2018). The addition of exogenous P was considered an effective way to enhance the plant’s adaptive ability to an adverse environment because P has the potential to regulate nutrient requirements and adjust the relative abundance of elements in the plant (Yan et al., 2016). Meanwhile, exogenous P was a form of available phosphorus, which could improve the nutrient utilization ability of plants, consequently, regulating the concentration and distribution of elements in plant tissues (Cusell et al., 2014; Peñuelas et al., 2015; Bracken et al., 2016). The leaves were regarded as an important organ for photosynthesis; the N and P are elemental components during the process of photosynthesis; consequently, the content of N and P was higher in leaves compared with other organs of the plant. Consistent with our hypothesis, the addition of P fertilizer significantly affected the C, N, and P concentrations of all organs, and these changes were largely dependent on the amount of P fertilizer. Furthermore, the C, N, and P contents of all organs during the fertility period were highest in different growth stages and gradually decreased with plant growth, reaching the lowest values at the wilt stage. The C, N, and P concentrations of all organs were the highest upon P3 application, while they decreased when the P amount was beyond the P3 level (De et al., 2014). The addition of P fertilizer promotes photosynthesis in plants; in turn, effective photosynthesis improves the accumulation of C, N, and P contents in plants. In addition, excessive P fertilizer could accelerate the progress of soil acidification, resulting in a decrease in C, N, and P contents in plants.
Stoichiometry mainly reflected the utilizing capacity of C, N, and P in plants, which is largely affected by environmental conditions. P application is an indispensable practice in agricultural crop production; P fertilizers are used to increase the accumulation of nutrients in the soil to improve the soil’s ability to supply nutrients to plants (Liu et al., 2021). Prior studies have found that a reserve of N and P concentrations in soil and plant increased through exogenous P application (Chen et al., 2015). Our findings support this hypothesis. Exogenous P addition has a role in regulating the nutrient contents of bluegrass organs. The C content of the root was highest after applying 9 g·m−2 P fertilizer, while the C contents of the stem, leaf, and spike were at the maximum after applying 12 g·m−2 P fertilizer. Our findings indicated that the aboveground organs of the plant absorbed more P and C compared to the root system. C is a crucial product for plant photosynthesis, and P fertilizer can promote the plant photosynthetic process; therefore, aboveground organs might require more P than roots. The N and P contents in roots, stems, leaves, and spikes were maximized at the fertilizer application rates of 15 g·m−2, 12 g·m−2, 9 g·m−2, and 6 g·m−2, respectively, which indicated that the P requirement of each organ was different; the N and P contents accumulation in different organs is ranked as follows: root > stem > leaf > spike. This may be related to nutrient transportation during plant growth because the root system was considered the origin for absorbing potential nutrients from the soil; meanwhile, the root is also regarded as a vital bridge to transplant these nutrients into aboveground organs. Therefore, exogenous P fertilizer not only accelerated the growth and expansion of root systems but also facilitated sufficient nutrients in transferring and distributing to aboveground organs (Lin and Sternberg, 2007). This may also be related to plant nutrient allocation; to ensure reproduction and effective photosynthesis, the plant preferentially allocated more nutrients to spikes and leaves when the soil condition is relatively barren. The above research results support our second hypothesis and prove that there is a different degree of heterogeneity in nutrient allocation in the various organs of bluegrass, which is also consistent with the results of previous studies (Minden et al., 2014).
Adjusting the nutrient and stoichiometric ratios of the plant was the crucial strategy to adapt to environmental changes, and these adjustments were made with the growth of plants (Drenovsky and Richards, 2005). The C/N and C/P ratios were regarded as key indicators to reflect relative plant growth rates; C is assimilated by the photosynthesis pathway, and its limited effect on plant growth was neglected; conversely, N and P were the main limited elements for plant growth in terrestrial ecosystems (Elser et al., 2007; Wang et al., 2014). An increased P fertilization had a profound effect on the C, N, and P ratios of the plant organs. For example, C/N and C/P of roots, stems, leaves, and spikes were lowest at 15 g·m−2, 12 g·m−2, 9 g·m−2, and 6 g·m−2 fertilizer levels, respectively, while N and P contents of each organ were highest, indicating that P fertilizer could have a positive effect in accumulating the content of N and P in each organ. Consequently, the ratio of C/N and C/P decreased. Prior studies indicated that P application could enhance the concentrations of N and P in plants, thus, reducing the ratio of C/N, C/P, and N/P (Mao et al., 2016). In addition, some studies also indicated that fertilization could alleviate environmental stresses and improve plant uptake of N and P (Lu et al., 2022).
N/P was employed as an indicator in evaluating nutrient availability for plant growth. N was regarded as the dominant nutrient when the N/P ratio was less than 14; similarly, P was the dominant nutrient when the N/P ratio was more than 16 (Reich and Olkesyn, 2004). Our results demonstrated that N/P was less than 14 in different organs; therefore, N was the dominant limiting nutrient in bluegrass. The N/P of all organs at different growth stages first decreased and then increased with increasing P fertilizer, indicating that low P application could aggravate the N restriction of plants and alleviate the N restriction in bluegrass. In nutrient-poor soil conditions, exogenous P addition accelerated the recycle time of nutrients in the plant and improved the nutrient use efficiency of plants, consequently. Regulating the nutrient level of plants was an effective strategy for plants to adapt to the barren soil condition (Crous et al., 2019). For example, some studies indicated that sufficient precipitation could alleviate the water limitation in plant growth. Plants do not allocate large amounts of N to green leaves in improving the efficiency of water use in desert steppes; consequently, N concentration and N/P in green leaves are significantly reduced by increased precipitation (Huang et al., 2018). N/P first decreases and then increases with the increase of P fertilizer; the probable reason is that exogenous P addition could alleviate the negative effects of limited P in the soil (Gong et al., 2022); the increase in P in plants promotes their N utilization and then the N/P in their tissues decreases. When P application exceeds a certain level, plant physiological and biochemical processes are inhibited, resulting in a reduced nitrogen use efficiency and an increased N/P.
P fertilizers promote chlorophyll synthesis and facilitate plant photosynthesis. Some studies have shown that P application can promote grass root growth and accelerate grassland establishment; however, neutral or negative effects were observed with excessive P application (Frank et al., 2022). P fertilizer was regarded as an effective management practice to improve grass productivity in artificial grasslands. To conclude on the efficiency and rationale of the P fertilization practice, the effect of P fertilizer on the C, N, and P contents in the different organs of bluegrass was examined in this study. The C content of roots was highest at 9 g·m−2 P fertilizer level, while the C contents of stems, leaves, and spikes were maximum at 12 g·m−2 P fertilizer level. Furthermore, N and P contents of roots, stems, leaves, and spikes were highest at 15 g·m−2, 12 g·m−2, 9 g·m−2, and 6 g·m−2 fertilizer application levels, respectively. Therefore, our findings suggested that P fertilizer should be comprehensively considered as a trade-off between productivity and fertilizer utilization efficiency when applying P fertilizer to artificial bluegrass grasslands.
The effects of P addition on the stoichiometric characteristics of bluegrass organs in alpine regions were studied. The growth of bluegrass was vigorous, and the nutrient content of each organ was high in the growing period. The addition of P fertilizer significantly increased the nutrient content of each organ, but the excessive addition of P reduced the nutrient content. The contents and ratios of C, N, and P in each organ of bluegrass were different in response to the P supplementation rate. The contents of N and P in the roots, stems, leaves, and spikes were the highest at fertilization rates of 15 g·m−2, 12 g·m−2, 9 g·m−2, and 6 g·m−2, respectively, while the C/N and C/P ratios in roots, stems, leaves, and spikes were the lowest at fertilization rates of 15 g·m−2, 12 g·m−2, 9 g·m−2, and 6 g·m−2, respectively. The N/P ratio of all organs was less than 14 and showed a trend of decreasing first and then increasing with P addition, indicating that a low P application increased N restriction, while a high P application alleviated N restriction in this area. Therefore, the utilization of P to plants according to the stoichiometric characteristics and ratio changes of various organs enables a more reasonable and more efficient application of P fertilizer for bluegrass, improving its beneficial and control effect.
The original contributions presented in the study are included in the article/supplementary material, further inquiries can be directed to the corresponding author/s.
JW and XW conceived and designed the study. JW, KH, LH, and HX performed most of the experiments. MS and LJ analyzed the data. XW was responsible for conceptualization, funding acquisition, and writing–review and editing. GB conceived the idea, made a great contribution to the analysis of manuscript data, and revised and reviewed important manuscript content. All authors contributed to the article and approved the submitted version.
This study was funded by the Qinghai Province science and technology basic condition platform (2021-ZJ-T01); Qinghai Province Kunlun Talent Program; National Science Foundation of China (31760711, Grants 32060398 and U21A20239); Western Light Talent Culture Project (2021-XBZG-12); Demonstration of “grass-herb-shrub” technology for sandy land control in Qinghai Lake Basin (QSCZ-2022-001); Opening Foundation of State Key Laboratory of Plateau Ecology and Agriculture (2021-KF-07).
The authors thank the Key Laboratory of Superior Forage Germplasm in the Qinghai-Tibetan Plateau for providing a platform for this research, the team of teachers for their suggestions on this article, and the team of students for their help in sample collection, data analysis, etc.
The authors declare that they have no known competing financial interests or personal relationships that could have appeared to influence the work reported in this paper.
All claims expressed in this article are solely those of the authors and do not necessarily represent those of their affiliated organizations, or those of the publisher, the editors and the reviewers. Any product that may be evaluated in this article, or claim that may be made by its manufacturer, is not guaranteed or endorsed by the publisher.
Ågren, G. (2004). The C:N:P stoichiometry of autotrophs: theory and observations. Ecol. Lett. 7, 185–191. doi: 10.1111/J.1461-0248.2004.00567.X
Bracken, M. E. S., Hillebrand, H., Borer, E. T., Seabloom, E. W., Cebrian, J., Cleland, E. E., et al. (2016). Signatures of nutrient limitation and co-limitation: responses of autotroph internal nutrient concentrations to nitrogen and phosphorus additions. Oikos J. Ecol. 124, 113–121. doi: 10.1111/oik.01215
Chen, F. S., Niklas, K. J., Liu, Y., Fang, X. M., Wan, S. Z., and Wang, H. (2015). Nitrogen and phosphorus additions alter nutrient dynamics but not resorption efficiencies of Chinese fir leaves and twigs differing in age. Tree Physiol. 35, 1106–1117. doi: 10.1093/treephys/tpv076
Crous, K. Y., Wujeska-Klause, A., Jiang, M., Medlyn, B. E., and Ellsworth, D. S. (2019). Nitrogen and phosphorus retranslocation of leaves and stemwood in a mature eucalyptus forest exposed to 5 years of elevated CO2. Front. Plant Sci. 10:664. doi: 10.3389/fpls.2019.00664
Cusell, C., Kooijman, A., and Lamers, L. P. M. (2014). Nitrogen or phosphorus limitation in rich fens? Edaphic differences explain contrasting results in vegetation development after fertilization. Plant Soil 384, 153–168. doi: 10.1007/s11104-014-2193-7
De, K. J., Zhang, D. G., Wang, W., Xu, C. T., Zhang, M., and Wei, X. J. (2014). Effects of fertilizers on the alpine meadow plant and soil N, P, K contents. Acta Agrestia Sinica 22, 299–305. In Chinese. doi: 10.11733/j.issn.1007-0435.2014.02.014
Drenovsky, R. E., and Richards, J. H. (2005). Nitrogen addition increases fecundity in the desert shrub Sarcobatus vermiculatus. Oecologia 143, 349–356. doi: 10.1007/s00442-004-1821-y
Elser, J. J., Andersen, T., Baron, J. S., Bergstrom, A. K., Jansson, M., Kyle, M., et al. (2009). Shifts in lake N:P stoichiometry and nutrient limitation driven by atmospheric nitrogen deposition. Science 326, 835–837. doi: 10.1126/science.1176199
Elser, J. J., Bracken, M. E. S., and Cleland, E. E. (2007). Global analysis of nitrogen and phosphorus limitation of primary producers in freshwater, marine and terrestrial ecosystems. Ecol. Lett. 10, 1135–1142. doi: 10.1111/j.1461-0248.2007.01113.x
Frank, K. W., Caussoin, R. E., Fry, J. D., Frost, M. D., and Baird, J. H. (2022). Nitrogen, phosphorus and potassium effects on seeded buffalograss establishment. HortScience 37, 371–373. doi: 10.21273/HORTSCI.37.2.371
Gao, J., Song, Z., and Liu, Y. (2019). Response mechanisms of leaf nutrients of endangered plant (Acer catalpifolium) to environmental factors varied at different growth stages. Glob Ecol Conserv 17:e00521. doi: 10.1016/j.gecco.2019.e00521
Gong, J. R., Zhang, Z. H., Zhu, C. C., Shi, J. Y., Zhang, W. Y., Song, L. Y., et al. (2022). The response of litter decomposition to phosphorus addition in typical temperate grassland in Inner Mongolia. J. Arid Environ. 197:104677. doi: 10.1016/j.jaridenv.2021.104677
He, M. Z., and Dijkstra, F. A. (2015). Phosphorus addition enhances loss of nitrogen in a phosphorus-poor soil. Soil Biol. Biochem. 82, 99–106. doi: 10.1016/j.soilbio.2014.12.015
Hidaka, A., and Kitayama, K. (2009). Divergent patterns of photosynthetic phosphorus-use efficiency versus nitrogen-use efficiency of tree leaves along nutrient-availability gradients. J. Ecol. 97, 984–991. doi: 10.1111/j.1365-2745.2009.01540.x
Huang, J. Y., Yu, H. L., Liu, J. L., Ma, F., and Han, L. (2018). Effects of precipitation levels on the C:N:P stoichiometry in plants, microbes and soils in a desert steppe in China. Acta Ecol. Sin. 38, 5362–5373. In Chinese. doi: 10.5846/stxb.201703200470
Kerkhoff, A., Fagan, W., Elser, J., and Enquist, B. (2006). Phylogenetic and growth form variation in the scaling of nitrogen and phosphorus in the seed plants. Am. Nat. 168, E103–E122. doi: 10.1086/507879
Li, F., Hu, J. Y., Xie, Y. H., Yang, G. S., Hu, C., Chen, X. S., et al. (2018). Foliar stoichiometry of carbon, nitrogen, and phosphorus in wetland sedge Carex brevicuspis along a small-scale elevation gradient. Ecol. Indic. 92, 322–329. doi: 10.1016/j.ecolind.2017.04.059
Li, H., Li, J., He, Y. L., Li, S. J., Liang, Z. S., Peng, C. H., et al. (2013). Changes in carbon, nutrients and stoichiometric relations under different soil depths, plant tissues and ages in black locust plantations. Acta Physiol. Plant. 35, 2951–2964. doi: 10.1007/s11738-013-1326-6
Lin, L., Li, Y. K., Xu, X. L., Zhang, F. W., Du, Y. G., Liu, S. L., et al. (2015). Predicting parameters of degradation succession processes of Tibetan Kobresia grasslands. Solid Earth. 6, 1237–1246. doi: 10.5194/se-6-1237-2015
Lin, Y., and Sternberg, L. D. L. (2007). Nitrogen and phosphorus dynamics and nutrient resorption of Rhizophora mangle leaves in South Florida, USA. Bull. Mar. Sci. 50, 291–296. doi: 10.1006/ecss.1999.0558
Liu, Q., Xu, H., and Yi, H. (2021). Impact of fertilizer on crop yield and C:N:P stoichiometry in arid and semi-arid soil. Int. J. Environ. Res. Public Health 18:4341. doi: 10.3390/IJERPH18084341
Lu, C. Q., and Tian, H. Q. (2017). Global nitrogen and phosphorus fertilizer use for agriculture production in the past half century: shifted hot spots and nutrient imbalance. Earth Syst Sci Data 9, 181–192. doi: 10.5194/essd-9-181-2017
Lu, Z. Y., Wang, Y. Y., Degryse, F., Huang, C. D., Hou, C. H., Wu, L. Q., et al. (2022). Magnesium-fortified phosphate fertilizers improve nutrient uptake and plant growth without reducing phosphorus availability. Pedosphere 32, 744–751. doi: 10.1016/j.pedsph.2022.06.010
Lu, J. Y., Yang, M., Liu, M. G., Wang, Y. Y., and Yang, H. M. (2019). Leaf stoichiometry and resorption of N and P in Lucerne at different growth stages under different water supplies. J. Plant Nutr. 42, 501–511. doi: 10.1080/01904167.2019.1567776
Luo, Y., Luo, Y., Peng, Q., Li, K., Gong, Y., and Han, W. (2021). Patterns of nitrogen and phosphorus stoichiometry among leaf, stem and root of desert plants and responses to climate and soil factors in Xinjiang, China. Catena 199:e105110:105100. doi: 10.1016/j.catena.2020.105100
Mao, R., Chen, H. M., Zhang, X. H., Shi, F. X., and Song, C. C. (2016). Effects of P addition on plant C: N: P stoichiometry in an N-limited temperate wetland of Northeast China. Sci. Total Environ. 559, 1–6. doi: 10.1016/j.scitotenv.2016.03.158
Minden, V., Kleyer, M., and Byers, D. (2014). Internal and external regulation of plant organ stoichiometry. Plant Biol. 16, 897–907. doi: 10.1111/plb.12155
Moody, E. K., and Wilkinson, G. M. (2019). Functional shifts in lake zooplankton communities with hypereutrophication. Freshw. Biol. 64, 608–616. doi: 10.1111/fwb.13246
Mori, T., Ohta, S., Ishizuka, S., Konda, R., Wicaksono, A., Heriyanto, J., et al. (2010). Effects of phosphorus addition on N2O and NO emissions from soils of an Acacia mangium plantation. Soil Sci Plant Nutr 56, 782–788. doi: 10.1111/j.1747-0765.2010.00501.x
Ostertag, R. (2010). Foliar nitrogen and phosphorus accumulation responses after fertilization: an example from nutrient-limited Hawaiian forests. Plant Soil 334, 85–98. doi: 10.1007/s11104-010-0281-x
Peñuelas, J., Poulter, B., Sardans, J., Ciais, P., van der Velde, M., Bopp, L., et al. (2013). Human-induced nitrogen-phosphorus imbalances alter natural and managed ecosystems across the globe. Nat. Commun. 4:2934. doi: 10.1038/ncomms3934
Peñuelas, J., Sardans, J., Rivas-Ubach, A., and Janssens, I. A. (2015). The human-induced imbalance between C, N and P in earth's life system. Glob. Chang. Biol. 18, 3–6. doi: 10.1111/j.1365-2486.2011.02568.x
Reich, P. B., and Olkesyn, J. (2004). Global patterns of plant leaf N and PR in relation to temperature and latitude. Proce Natl Acad Sci U. S. A. 101, 11001–11006. doi: 10.1073/pnas.0403588101
Reich, P. B., Wright, I. J., Bares, J. C., Craine, J. M., Oleksyn, J., Westoby, M., et al. (2003). The evolution of plant functional variation: traits, spectra, and strategies. Evolution 164, S143–S164. doi: 10.1086/374368
Sardans, J., Janssens, I. A., Ciais, P., Obersteiner, M., and Peñuelas, J. (2021). Recent advances and future research in ecological stoichiometry. Perspect Plant Ecol Evol Syst 50, 125611–128319. doi: 10.1016/j.ppees.2021.125611
Soons, M. B., Hefting, M. M., Dorland, E., Lamers, L. P., Versteeg, C., and Bobbink, R. (2017). Nitrogen effects on plant species richness in herbaceous communities are more widespread and stronger than those of phosphorus. Biol. Conserv. 212, 390–397. doi: 10.1016/j.biocon.2016.12.006
Sterner, R. W., and Elser, J. J. (2022). Ecological stoichiometry: the biology of elements from molecules to the biosphere. Princeton Univ Press 78:501. doi: 10.1086/382444
Stevens, C. J., Lind, E. M., Hautier, Y., Harpole, W. S., Borer, E. T., Hobbie, S., et al. (2015). Anthropogenic nitrogen deposition predicts local grassland primary production worldwide. Ecology 96, 1459–1465. doi: 10.1890/14-1902.1
Su, H. J., Wu, Y., Xia, W. L., Yang, L., Chen, J. F., Han, W. X., et al. (2019). Stoichiometric mechanisms of regime shifts in freshwater ecosystem. Water Res. 149, 302–310. doi: 10.1016/j.watres.2018.11.024
Sun, S. C., and Chen, L. Z. (2001). Leaf nutrient dynamics and resorption efficiency of Quercus liaotungensis in the Dongling mountain region. Chin J Plant Ecol 25, 76–82. In Chinese. doi: 10.3321/j.issn:1005-264X.2001.01.013
They, N. H., Amado, A. M., and Cotner, J. B. (2017). Redfield ratios in inland waters: higher biological control of C:N:P ratios in tropical semi-arid high water residence Time Lakes. Front. Microbiol. 8:1505. doi: 10.3389/fmicb.2017.01505
Tong, Y. D., Qiao, Z., Wang, X. J., Liu, X. Y., Chen, G. Y., Zhang, W., et al. (2018). Human activities altered water N:P ratios in the populated regions of China. Chemosphere 2018, 1070–1081. doi: 10.1016/j.chemosphere.2018.07.108
Verhoeven, J. T., Koerselman, W., and Meuleman, A. F. (1996). Nitrogen- or phosphorus-limited growth in herbaceous, wet vegetation: relations with atmospheric inputs and management regimes. Trends Ecol Evol 11, 494–497. doi: 10.1016/s0169-5347(96)10055-0
Wang, Z. Q., Bu, H. Y., Wang, M. C., Huang, H., and Niklas, K. J. (2020a). Allocation strategies for seed nitrogen and phosphorus in an alpine meadow along an altitudinal gradient on the Tibetan plateau. Front. Plant Sci. 11:614644. doi: 10.3389/fpls.2020.614644
Wang, Z. Q., Gong, H. Y., Sardans, J., Zhou, Q. P., Deng, J. M., Niklas, K. J., et al. (2022). Divergent nitrogen and phosphorus allocation strategies in terrestrial plant leaves and fine roots: a global meta-analysis. J. Ecol. 110, 2745–2758. doi: 10.1111/1365-2745.13985
Wang, X. G., Lü, X. T., and Han, X. G. (2014). Responses of nutrient concentrations and stoichiometry of senesced leaves in dominant plants to nitrogen addition and prescribed burning in a temperate steppe. Ecol. Eng. 70, 154–161. doi: 10.1016/j.ecoleng.2014.05.015
Wang, Z. Q., Lv, S. Q., Song, H., Wang, M. C., Zhao, Q., Hung, H., et al. (2020b). Plant type dominates fine-root C:N:P stoichiometry across China: a meta-analysis. J. Biogeogr. 47, 1019–1029. doi: 10.1111/jbi.13791
Wang, Z. Q., Yu, K. L., Lv, S. Q., Niklas, K. J., Mipam, T. D., Crowther, T. W., et al. (2019). The scaling of fine root nitrogen versus phosphorus in terrestrial plants: a global synthesis. Funct. Ecol. 33, 2081–2094. doi: 10.1016/j.soilbio.2022.108714
Wei, L. N., Zhang, C. P., Dong, Q. M., Yang, Z. Z., Chu, H., Yu, Y., et al. (2020). Effects of temperature and water potential on seed germination of 13 Poa L. species in the Qinghai-Tibetan plateau. Glob Ecol Conserv 25:e01442. doi: 10.1016/j.gecco.2020.e01442
Xiao, Y., Tao, Y., and Hang, Y. M. (2014). Biomass allocation and leaf stoichiometric characteristics in four desert herbaceous plants during different growth periods in the Gurbantünggüt Desert, China. Chin J Plant Ecol 38, 929–940. In Chinese. doi: 10.3724/SP.J.1258.2014.00087
Yan, Z., Han, W., Peuelas, J., Sardans, J., Elser, J. J., and Du, E. (2016). Phosphorus accumulates faster than nitrogen globally in freshwater ecosystems under anthropogenic impacts. Ecol. Lett. 19, 1237–1246. doi: 10.1111/ele.12658
Yuan, H. Z., Liu, S. L., Razavi, B. S., Zhran, M., Wang, J., Zhu, Z. K., et al. (2019). Differentiated response of plant and microbial C: N: P stoichiometries to phosphorus application in phosphorus-limited paddy soil. Eur. J. Soil Biol. 95:103122. doi: 10.1016/j.ejsobi.2019.103122
Zhang, L., Liu, H. L., Zhang, L. W., Chen, Y. F., and Baskin, C. C. (2022). Effects of increased precipitation on C, N and P stoichiometry at different growth stages of a cold desert annual. Glob Ecol Conserv 37, e02158–e09894. doi: 10.1016/j.gecco.2022.e02158
Keywords: stoichiometry, P fertilizer gradients, bluegrass, different organs, Qinghai-Tibetan Plateau
Citation: Wang J, He K, Bao G, He L, Xu H, Song M, Jin L and Wei X (2023) Effects of increased phosphorus fertilizer on C, N, and P stoichiometry in different organs of bluegrass (Poa L.) at different growth stages. Front. Ecol. Evol. 11:1092840. doi: 10.3389/fevo.2023.1092840
Received: 08 November 2022; Accepted: 20 March 2023;
Published: 17 April 2023.
Edited by:
Tserang Donko Mipam, Southwest Minzu University, ChinaReviewed by:
Yongheng Gao, Chengdu Institute of Biology (CAS), ChinaCopyright © 2023 Wang, He, Bao, He, Xu, Song, Jin and Wei. This is an open-access article distributed under the terms of the Creative Commons Attribution License (CC BY). The use, distribution or reproduction in other forums is permitted, provided the original author(s) and the copyright owner(s) are credited and that the original publication in this journal is cited, in accordance with accepted academic practice. No use, distribution or reproduction is permitted which does not comply with these terms.
*Correspondence: Xiaoxing Wei, d3Vpa29AMTYzLmNvbQ==
Disclaimer: All claims expressed in this article are solely those of the authors and do not necessarily represent those of their affiliated organizations, or those of the publisher, the editors and the reviewers. Any product that may be evaluated in this article or claim that may be made by its manufacturer is not guaranteed or endorsed by the publisher.
Research integrity at Frontiers
Learn more about the work of our research integrity team to safeguard the quality of each article we publish.