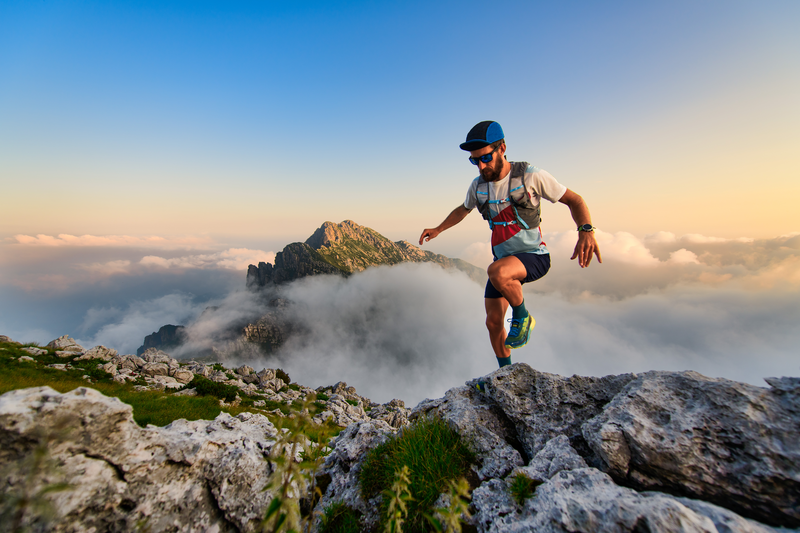
95% of researchers rate our articles as excellent or good
Learn more about the work of our research integrity team to safeguard the quality of each article we publish.
Find out more
ORIGINAL RESEARCH article
Front. Ecol. Evol. , 05 May 2023
Sec. Behavioral and Evolutionary Ecology
Volume 11 - 2023 | https://doi.org/10.3389/fevo.2023.1091395
This article is part of the Research Topic Advances in the Evolutionary Ecology of Termites, Volume II View all 10 articles
A colony of subterranean termites contains different castes such as workers and soldiers that perform various tasks. Foraging activity is vital among many tasks of a colony and ~20% of the worker population in a colony of Coptotermes formosanus engage in foraging. Although flexibility in task allocation plays a crucial role in maintaining colony function in fluctuating environments, such flexibility is barely understood in subterranean termites. Here, we investigated regulations of foraging task allocation in response to different soil types at the nest and the foraging site. At the nest and foraging site, either nitrogen-rich organic soil or nitrogen-poor sand, which differed in carbon and nitrogen ratio, was provided and the proportions of workers and soldiers at the foraging site were determined. Our results showed that the foraging worker proportion and per-capita wood consumption significantly differed depending on the soil type at the nest but not the soil type at the foraging site. When the colony had access to organic soil at the nest, the proportion of workers at the foraging site and per-capita wood consumption was much smaller than those with sand at the nest. Conversely, the proportion of soldiers at the foraging site remained the same regardless of the soil type at the nest and the foraging site. In brief, the current study showed flexible regulation of foraging task allocation in C. formosanus and demonstrated that perturbation of soil type alters the allocation of workers, but not soldiers, in the colony.
Social insects are one of the most successful organisms in various terrestrial ecosystems and their success is often explained by an elaborate task division system in the colony (Hölldobler and Wilson, 2009). Division of labor in insect societies allows the colony members to perform several tasks simultaneously, such as reproduction, foraging, brood care, and defense with highly specialized individuals (Oster and Wilson, 1978; Porter and Tschinkel, 1985; Jeanne, 1986; Robinson, 1992; Beshers and Fewell, 2001). A total of two major factors are critical for individual task determination: age (age polyethism) and morphology (morphological polyethism) (Oster and Wilson, 1978; Robinson, 1992). Age polyethism is observed across various social insects (Free, 1964; Oster and Wilson, 1978; McMahan, 1979; Gerber et al., 1988; Moritz, 1988; Noirot, 1989; Hölldobler and Wilson, 1990; Seeley and Kolmes, 1991; Robinson, 1992; Beshers and Fewell, 2001), whereas morphological polyethism is not as common as age polyethism and is found only in a few ant species and nearly all termites (Oster and Wilson, 1978; Wheeler, 1986; Noirot, 1989; Hölldobler and Wilson, 1990).
Foraging is an important task for survival and maintaining a colony, but it is a costly and risky task (Fewell, 1988; Korb and Linsenmair, 2002; Rytter and Shik, 2016; Pyke and Starr, 2021). Individuals are required to leave the safety of the nest in search of food resources, and they need to bring back food to provision the entire colony. In many social insects, foraging activities are performed by older individuals and only a small portion of colony members is often allocated to the foraging task (Golley and Gentry, 1964; Erickson, 1972; Rogers et al., 1972; Lewis et al., 1974; Bruin et al., 1977; Porter and Jorgensen, 1981; MacKay, 1983; Beekman et al., 2004; Du et al., 2017a; Su et al., 2017; Lee et al., 2022). If the environments are stable (i.e., no disturbances), the colony would maintain a certain proportion of individuals in the foraging task. By doing so, the colony may be able to achieve high efficiency in foraging with specialized individuals. However, the task allocation in the colony of social insects is known to be flexible, and the colony is capable to adjust the proportion of individuals in foraging task in response to changes in internal demand and external conditions (Robinson, 1992; Gordon, 1996, 2016; Beshers and Fewell, 2001; Loftus et al., 2021). Therefore, flexibility in task allocation plays a crucial role in the colony to maintain its function against such changes (Middleton and Latty, 2016).
Among many factors that could affect foraging task allocation, food quality has been widely explored in diverse social insects because optimal foraging theories predict that it would be advantageous if the colony consumed nutritious food while expending the same amount of energy in foraging (Kay, 2002; Pyke and Starr, 2021). When the food quality is not suitable or food becomes unavailable, foragers in a honeybee colony cease foraging activities (Von Frisch, 1967). On the other hands, several ant species increase the number of foraging individuals once they have access to food that has a high concentration of sucrose (Wilson, 1962; Szlep and Jacobi, 1967; Hangartner, 1969, 1970; Breed et al., 1987; De Biseau et al., 1991; Beckers et al., 1993), suggesting that food quality could play a vital role in regulating the number of foragers in ant colonies. Assuming the colony size does not change drastically in a short period of time, an increased or decreased number of foragers could indicate flexible foraging task allocation depending on food quality. In addition to food quality, the amount of food stored in the colony also affects foraging activities in honeybees. For instance, bee foragers make a decision whether to remain inside the hive or forage out based on the amount of nectar stored (Seeley, 1989; Seeley et al., 1991). However, it is elusive as to how the colony of subterranean termites adjusts the proportion of foraging workers in response to different food sources.
Contrary to other social insects, the impact of food quality on the proportion of foragers in termites is a challenging subject to study. This is largely because subterranean termites do not have many food options since they primarily feed on wood, either partially decayed by wood-decaying fungi (e.g., Reticulitermes) or living trees (e.g., Coptotermes) (Lenz, 1994). Wood is naturally very poor in terms of nutrition as the majority of nutrients in this diet are carbon with an extremely low amount of nitrogen (Matsumoto, 1976; La Fage and Nutting, 1978). However, it has been suggested that carbon and nitrogen balance is an important factor in determining colony size, and species with a better carbon–nitrogen balance tend to grow larger than those with a poor balance (Higashi et al., 1992). The Formosan subterranean termite, Coptotermes formosanus Shiraki, is known to form large colonies, with some mature colonies having millions of individuals (Su and Scheffrahn, 1988) and grow rapidly in a few years (Chouvenc and Su, 2014). The large colony size of this species implies that it has a better source of dietary nitrogen. Along with this speculation, a recent study on the dietary nitrogen of C. formosanus showed that colonies that have access to nitrogen-rich organic soil were able to grow eight times larger than colonies reared in nitrogen-poor sand (Mullins et al., 2021).
Subterranean termites such as C. formosanus build nests below ground or inside trees and forage for multiple food resources simultaneously over long distances through underground tunnels (King and Spink, 1969; Su and Scheffrahn, 1988). Mature colonies of Coptotermes formosanus maintain foraging abilities over decades even with the change in demographic compositions and breeding structures (Chouvenc et al., 2022). In C. formosanus, it has been reported that 10% to 20% of old workers in the colony perform foraging task and these foragers displayed task division (Du et al., 2017b; Lee et al., 2021, 2022). The foraging task allocation in the colony of C. formosanus showed resiliency against disturbances, and the colony maintained ~20% of foraging workers despite the change in colony size and demographic composition due to the periodical removal of foragers (Lee et al., 2022).
In a termite colony, soldiers mainly perform defense regardless of evolutionary history from basal to derived, to protect nestmates from predators, and soldiers in termites have highly modified mandibles for defensive purposes (Tian and Zhou, 2014). While recent studies have shown soldiers in some termite species also act as scouts and actively participate in the foraging process (Casarin et al., 2008; Almeida et al., 2016; Sacramento et al., 2020). Different termite species have a specific soldier ratio in the colony which has evolved through the selection process and colonies maintain it through physiological constraints (Haverty, 1977). Workers are accompanied by soldiers when forage and ~10% of individuals in a colony of C. formosanus are soldiers (Haverty, 1977). However, it is poorly understood how the colony of termites regulates the proportion of soldiers in a foraging group. If a high proportion of soldiers involves in foraging activity, it can be a burden to workers at the foraging site because soldiers must be fed by workers (Haverty, 1977, 1979; Haverty and Howard, 1981).
In this study, we investigated how colonies of C. formosanus regulate the proportion of workers and soldiers at the foraging site in response to different soil types at the nest and the foraging site. We prepared two distinct types of nest and foraging site, each filled with either nitrogen-rich organic soil or nitrogen-poor sand, yielding four different combinations. We hypothesized that (1) foraging worker proportion will be higher in colonies that have access to nitrogen-rich organic soil at the foraging site than those of colonies that have access to the sand foraging site. However, (2) soldier proportion at the foraging site will be stable regardless of soil type at the foraging site and the nest.
Several hundred winged images (i.e., alates) of C. formosanus were collected in Broward County, FL, USA, during the dispersal flight season (May 17th, 2016), using a light trap. Collected termites were immediately brought back to the laboratory to morphologically identify sexes. At the same time, hundreds of rearing units (8 × 2.5 cm, height × diameter, IntraPac, Plattsburgh, New York, United States) were prepared which contain 6 g of moistened organic soil at the bottom (3 cm high) and four pieces of wood (5 × 0.5 × 0.5 cm3, Picea sp.) on top of the soil, and an agar solution (3%) was poured over the top of soil and wood pieces in each rearing unit. After 2 h, once agar solidified, a pair of reproductives was introduced into the vials and kept at 28 ± 1 °C. After 6–8 months, hundreds of surviving colonies were transferred to large vials (6.3 × 4.6 cm, height × diameter, IntraPac, Plattsburgh, New York, United States), containing the same components but in greater amounts.
One year after colony foundation, surviving colonies were further transferred to a container box (1.5 L, 17 × 12 × 7 cm, Pioneer Plastics, Dixon, Kentucky, United States), which contained moistened organic soil at the bottom (3–4 cm high) and a piece of wood (14.5 × 4 × 1 cm, Picea sp.), to accommodate colony growth. Prior to placing the vials in the box, each vial was equipped with a modified reproductive excluder as a lid, which allowed only workers and soldiers to pass through (Lee et al., 2019). The vial was, then, placed horizontally at the bottom corner of the container box. Weekly inspections were performed on colonies, and water and wood were replenished as needed. All colonies were maintained at 28 ± 1°C and 80 ± 2% humidity.
Prior to starting experiments (2 months before), the 2-year-old C. formosanus colonies were processed to count the number of termites in the colony, in order to choose similar-sized colonies and verify the presence of reproductives. We, then, prepared an artificial nest (hereafter: nest) using a container box (1.5 L, 17 × 12 × 7 cm, Pioneer Plastics, Dixon, Kentucky, United States) filled with either nitrogen-rich organic soil (Nature's Care, organic & natural potting mix, Scotts Miracle-Gro, Marysville, Ohio, United States) or nitrogen-poor sand. Both organic soil and sand were filled up to 5 cm deep and moistened with deionized water. A hole was drilled in the side of the container.
A piece of wood (14.5 × 4 × 1 cm, Picea sp.) was provided as food for 2 weeks prior to experiments. In the meantime, an artificial foraging site (hereafter: foraging site) was also prepared in the same manner as nests (described above). A piece of wood was dried in the oven at 60°C for 48 h and dry weight was measured. Then, it was soaked in the water for 3 days and placed at the foraging site on top of either organic soil or sand. After preparing the foraging site, the wood at the nest was removed, and two container boxes were connected by plastic tubes (3 m in length) (Figure 1). In the current study, a total of four different combinations were tested as follows: (1) organic soil nest and sand foraging site (OS–S) (Figure 1A), (2) organic soil nest and organic soil foraging site (OS–OS) (Figure 1B), (3) sand nest and sand foraging site (S–S) (Figure 1C), and (4) sand nest and organic soil foraging site (S–OS) (Figure 1D).
Figure 1. Schematic drawings of different nutrition availabilities at the nest and the foraging site. The left and right containers are defined as the nest and the foraging site, respectively. Light black and gray colors indicate nitrogen-rich organic soil (OS) and nitrogen-poor sand (S), respectively. The nest and foraging site were connected by a 3 m plastic tube, and a colony of Coptotermes formosanus was introduced into the nest. In total, four different conditions were examined, and 14 colonies were used as follows: (A) organic soil at both the nest and foraging site (OS–OS; three colonies), (B) organic soil at the nest and sand in the foraging site (OS–S; three colonies), (C) sand at the nest and organic soil in the foraging site (S–OS; four colonies), and (D) sand at both nest and foraging site (S–S; four colonies). As a food source, a piece of wood of the same size was provided for all experiments.
We observed that all colonies had reached the foraging site and started feeding on wood within a week. Then, the pieces of wood at the foraging site were removed and replaced with another piece of wood (14.5 × 4 × 1 cm, Picea sp.), prepared using the same methods described above. All experimental units (in total: 14 colonies) units were covered with black plastic throughout the entire span of the experiment to prevent any disturbance, and temperature and humidity were kept at 28 ± 1°C and 80 ± 2%, respectively.
The carbon and nitrogen ratio (C/N ratio) of sand and organic soil was measured using an elemental analyzer (LECO, CN628, MI, United States). Both sand and organic soil were dried in the oven at 60°C for a week, and a standard reference for the calibration was conducted using Ethylenediaminetetraacetic acid (EDTA) calibrator prior to the measurement and in between measurements of sand and organic soil.
The number of workers and soldiers at the foraging site was recorded every 3 days (i.e., 72 h interval). To count the number of termites at the foraging site, the connecting tubes between the nest and foraging site were clipped at the distal end of the foraging site. After counting, termites were returned to the foraging site. In total, we counted the number of termites at the foraging site five times. After the fifth count (i.e., 15 days), colonies were processed to determine the total number of termites in the colony and calculate the proportion of foraging workers and the proportion of soldiers at the foraging site. Larvae (the first and second instar) and pre-soldiers were counted as workers and soldiers, respectively. Wood at the foraging site was washed with water to remove debris and dried in the oven at 60°C for 48 h, and it was weighed to determine the amount of wood consumption.
The foraging worker proportion was calculated by the number of workers at the foraging site divided by the total number of workers in the colony (number of workers at the foraging site and the nest). Soldier proportion at the foraging site was determined in the same manner, with the number of soldiers at the foraging site divided by the total number of soldiers in the colony. Per-capita wood consumption (mg of wood consumed/number of termites) was also calculated. For the calculation of wood consumption, the amount of wood consumption (mg) was divided by the total number of termites in the colony.
In total, 14 colonies were examined [three colonies for OS–S (nest–foraging site), three colonies for OS–OS, four colonies for S–S, and four colonies for S–OS], and the number of termites in colonies in each combination was subjected to Kruskal–Wallis test (P < 0.05), to confirm no difference on colony size. In addition to the colony size, the carbon and nitrogen ratio of the sand (n = 5) and the organic soil (n = 5) was compared with a t-test (P < 0.05). Foraging worker proportion and soldier proportion at the foraging site were fitted to linear mixed models (LMMs), with the soil type (i.e., sand or organic soil) at the nest and foraging site treated as fixed effects, and both sampling date and colony as random effects to account for repeated measurements. In the LMMs, the fixed effect was treated as a simple main effect. Then, type II analysis of variance (ANOVA) using the Wald chi-square test was performed for foraging worker proportion and proportion of soldiers at the foraging site to determine the statistical significance of each explanatory variable. We also calculated the confidence interval of the foraging worker proportion and soldier proportion at the foraging site depending on the soil type at the nest and the foraging site. Per-capita wood consumption was subjected to a two-way analysis of variance (ANOVA) with soil type at the nest and foraging site as factors. All statistical analyses were performed in R 4.1.3 (Team, 2022).
In the current study, we used colonies with no statistical differences in colony size (i.e., number of termites in the colony) to exclude any effect from colony size (W = 0.329, df = 3, P = 0.9546, Figure 2), which allowed us to solely focus on the proportion of termites at the foraging site depending on soil type at the nest and foraging site. A comparison of carbon and nitrogen percentage showed that organic soil contained significantly more carbon and nitrogen than sand [Table 1; carbon (%): t = −16.935, df = 10, P < 0.01; nitrogen (%): t = −18.482, df = 10, P < 0.01].
Figure 2. The average number of termites in colonies of C. formosanus used in this study. In total, four different conditions were tested using 14 different colonies. OS (organic soil) and S (sand) indicate the soil type of either the nest or the foraging site, from left (nest) to right (foraging site). The number of termites in each condition was compared with the Kruskal–Wallis test and showed no significant differences.
Table 1. Average percentages (mean ± sd) of carbon and nitrogen in organic soil and sand used in this study.
Foraging worker proportions were significantly different between soil types at the nest (χ2 = 14.217, df = 1, P < 0.01; Figure 3A) but not at the foraging site (χ2 = 0.003, df = 1, P = 0.954; Figure 3B). When we a calculated 95% confidence interval (CI) based on the soil type at the nest, the CI of foraging worker proportion at the organic soil nest was 0.08 ± 0.03 (mean ± CI) and at the sand nest was 0.17 ± 0.03 (mean ± CI). Based on the soil type at the foraging site, the CI of foraging worker proportion at the sand foraging site was 0.13 ± 0.06 (mean ± CI) and that at the organic soil foraging site was 0.13 ± 0.01 (mean ± CI). The foraging worker proportion was the greatest when both the nest and foraging site had sand (20.42 ± 2.60%, mean ± s.d), whereas the lowest foraging worker proportion was observed in the combination of the organic soil nest and the sand foraging site (3.94 ± 0.72%, mean ± s.d).
Figure 3. Box plots of the proportion of workers (A, B) and soldiers (C, D) at the foraging site in different nutrition availabilities. In total, 14 colonies of C. formosanus were tested [three colonies: OS (organic soil)–S (sand), from left to right, nest and foraging site, respectively; three colonies: OS–OS; four colonies: S–S; four colonies: S–OS]. The proportion of workers at the foraging site was calculated by the number of workers at the foraging site divided by the total number of workers in the colony. The proportion of soldiers at the foraging site was calculated in the same manner. Black dots indicate each collected data points from each sampling, and the red cross represent the mean and thick lines inside boxes indicate the median value of the data.
Contrary to foraging worker proportion, we found no significant fixed effects to explain soldier proportion at the foraging site according to the LMMs. It showed that the soil type at the nest (χ2 = 3.505, df =1, P = 0.061; Figure 3C) and at the foraging site (χ2 = 0.960, df =1, P = 0.327; Figure 3D) did not affect soldier proportion at the foraging site. The CI of soldier proportion at the foraging site overlapped, and it was 0.17 ± 0.05 (mean ± CI) at the organic soil nest and 0.24 ± 0.05 (mean ± CI) at the sand nest. When we calculated based on soil type at the foraging site, the CI of soldier proportion was 0.19 ± 0.06 at the sand foraging site and 0.25 ± 0.05 at the organic soil foraging site.
Similar to the proportion of workers at the foraging site, per-capita wood consumption showed that soil type at the nest was a significant factor (F = 22.794, df = 1, 10, P < 0.01), and the interaction of nest and foraging site was also significant (F = 6.675, df = 1, 10, P < 0.05). However, soil type at the foraging site did not affect per-capita wood consumption (F = 1.843, df = 1, 10, P = 0.204; Figure 4). Termites consumed the greatest amount of wood when both nest and foraging site contained sand, which was approximately three times greater than that consumed in organic soil nest and sand foraging site (Figure 4).
Figure 4. Per-capita wood consumption (mg of wood consumption/number of termites) of C. formosanus in different combinations during experiments. The amount of wood consumption was calculated by subtracting the weight of wood pre and post experiments: (A) nest, (B) foraging site. The number of termites was determined after the experiment by counting all individuals in each colony. Total of 14 colonies of C. formosanus were used. OS and S indicate organic soil and sand, respectively. Cross with red color and thick lines inside boxes represents the mean and median values of the data.
Task allocation is an important mechanism in insect societies to maintain colony function in response to any change inside and outside the colonies, and flexible regulation of task allocation in the colony organized by individual's collective behaviors without hierarchical control plays an important role in the resiliency of the colony (Gordon, 1996; Middleton and Latty, 2016). Our current study found two important results about task allocation in the Formosan subterranean termite, Coptotermes formosanus. First, a colony of C. formosanus is capable of adjusting the proportion of foraging workers in response to the soil type at the nest. Contrary to the foraging worker proportion, the soldier proportion at the foraging site, however, was maintained at 10–20% regardless of nutrition availabilities at both the nest and foraging site. In this study, it was assumed that the food requirements of colonies remained the same, and colony demography is likely similar across colonies since we used colonies with no statistical difference in colony size and age (Figure 2).
When the colony had organic soil at the nest, the foraging worker proportion was significantly smaller than those of sand (Figure 3A). A recent study on colony growth in C. formosanus revealed a colony with access to organic soil was approximately three times larger in biomass and eight times larger in population size than colonies that were reared in the sand, suggesting that C. formosanus utilized organic matter from the organic soil (Mullins et al., 2021). Assuming similar-sized colonies require the same amount of food to provision all colony members (Patel et al., 2020), the low foraging worker proportion found in colonies with organic soil nest implies that these colonies were able to provision colony members without sending out a large proportion of workers to forage for food in comparison to colonies with the sand nest (Figure 3). This result was further supported by our wood consumption analysis that the per-capita wood consumption of colonies with organic soil nest was significantly lower than those of colonies with sand nest (Figure 4). Allocating many workers to risky foraging task may be less advantageous if they have any available resources near the nest, as they could potentially encounter predators such as ants while foraging.
Contrary to the nest, the soil type at the foraging site did not significantly affect the proportion of foraging workers (Figure 3). This result suggested that once the colony started the foraging process due to the potential depletion of food resources near the nest, colonies will maintain a 10–20% proportion of foraging workers in the colony. It has been reported that colonies of C. formosanus could reduce the risk of worker loss caused by natural disturbances or anthropogenic events such as pesticide applications by allocating up to 20% of their workers to foraging task, while also achieving high foraging efficiency within their worker force (Lee et al., 2022). In addition, per-capita wood consumption found in the present study further showed no statistical differences when colonies had access to either organic or sand foraging site (Figure 4). In the case of sand foraging site and nest, the colony has to be entirely reliant on a piece of wood for food by sending out the maximum proportion of forging workers, whereas the colony with sand nest and organic soil foraging site might be able to utilize the organic matter from the foraging site. However, our results showed that both colonies did not differ in per-capita wood consumption (Figure 4), indicating colonies that provided either organic soil or sand at the foraging site consumed a similar amount of wood.
Because subterranean termites such as Coptotermes and Reticulitermes mainly feed on woody materials including living trees and decayed wood, the impact of food quality and quantity on foraging in a colony of subterranean termites is not well understood. However, a handful of termites in the family of Termitidae has shown that resource density and suitability affect their foraging (Araújo et al., 2011; Almeida et al., 2018). When Nasutitermes aff. coxipoensis colonies had access to low-resource density, termites constructed longer and more foraging trails, suggesting increased searching efforts (Almeida et al., 2018). On the other hand, when termites had a high density of food resource, shorter and less trails were observed and showed optimization of the tunnel in response to resource density (Almeida et al., 2018). Similarly, Cornitermes cumulans (Kollar) excavated tunnels intensively when resource suitability is low, whereas tunnel length, number, and tunneling speed decreased when the resource is abundant (Araújo et al., 2011). Although the impact of resource suitability and density on tunnel formation in subterranean termites is poorly understood, several optimizations on tunnel geometry and behaviors during tunnel constructions have been reported in C. formosanus (Lee et al., 2006, 2020; Michael et al., 2023).
This observation raises a question of why C. formosanus did not increase their foraging worker proportion when they have the sand nest and the organic soil foraging site to maximize cost and benefit in foraging. Coptotermes formosanus is known to construct underground nests, and they could inhabit multiple pieces of wood over long distance (King and Spink, 1969; Su and Scheffrahn, 1988). Therefore, C. formosanus continuously encounters soil near the nest and during underground tunnel excavations. Despite ubiquitous soil, it is possible that the acquisition of organic matters from soil may only occur near the nest, and foraging workers may focus on finding and feeding on pieces of wood at a remote feeding site to provision the colony. Since older workers in the colony of C. formosanus are distributed farther away from the nest for foraging (Su et al., 2017), young workers remain near the nest, and they could participate in organic matter acquisition from the soil near the nest. However, future study is warranted to study such possibilities.
Compared to workers, we found different patterns from soldiers in C. formosanus. Soil type at the nest and foraging site did not have any impact on soldier proportion at the foraging site. Soldiers in termites, excluding soldierless termites in the family of Termitidae, are morphologically specialized for the defense task (Eggleton, 2010). In some termite species, soldiers also perform as scouts in the foraging group (Traniello, 1981; Traniello and Leuthold, 2000; Casarin et al., 2008; Almeida et al., 2016; Sacramento et al., 2020) and could help the tunneling process (Janowiecki and Vargo, 2022). Acting as a scout and helping with tunneling behavior could reduce predation risk in the early stage of foraging (Traniello, 1981). Soldiers in termites are fully dependent on workers for nutrition because of modified mandibles for a defensive purpose (Noirot and Darlington, 2000; Eggleton, 2010). Under normal conditions (i.e., no predation risk), a high number of soldiers at the foraging site will impose a burden on workers as they need to provide food to soldiers. A higher number or proportion of soldiers could reduce the potential predation risk, preventing the cost of foraging during the early phase (Almeida et al., 2016) and may improve the decision of foraging (Sacramento et al., 2020). Despite the aforementioned studies reported the effect of soldiers in foraging, a recent study, however, showed that the presence of soldiers and different proportions of soldiers in C. formosanus did not affect the tunneling behaviors of workers (McCarthy et al., 2023). Therefore, it is possible that the proportion of soldiers in the foraging group could be set intrinsically at 10–20%, to maximize the protection of foraging workers from predation risk while limiting the burden on workers. As predicted, morphological polyethism could be less flexible to environmental factors compared to age polyethism (Oster and Wilson, 1978).
In conclusion, the current study showed the same perturbation could lead to different responses depending on castes in termites. Workers need to forage for food to provision their nestmates including the queen, king, younger broods, and soldiers, while soldiers are in charge of protecting their nestmates at the foraging site. Therefore, foraging worker proportion varied in different nutrition availability at the nest, whereas soldier proportions at the foraging site were maintained stable regardless of soil type in C. formosanus.
The raw data supporting the conclusions of this article will be made available by the authors, without undue reservation.
S-BL and N-YS conceptualized and designed the study, interpreted the results together, and wrote the first draft. S-BL carried out experiments, data collection, and data analysis. Both authors contributed to the article and approved the submitted version.
This study was supported by the USDA National Institute of Food and Agriculture, Hatch project number FLA-FTL-005865 and FLA-FTL-005660.
We thank Alina Lorenzo, Joseph Velenovsky, and Thomas Chouvenc (University of Florida) for proofreading the first draft and providing constructive comments.
The authors declare that the research was conducted in the absence of any commercial or financial relationships that could be construed as a potential conflict of interest.
All claims expressed in this article are solely those of the authors and do not necessarily represent those of their affiliated organizations, or those of the publisher, the editors and the reviewers. Any product that may be evaluated in this article, or claim that may be made by its manufacturer, is not guaranteed or endorsed by the publisher.
Almeida, C. S., Cristaldo, P. F., Desouza, O. G., Bacci, L., Florencio, D. F., Cruz, N. G., et al. (2018). Resource density regulates the foraging investment in higher termite species. Ecol. Entomol. 43, 371–378. doi: 10.1111/een.12508
Almeida, C. S., Cristaldo, P. F., Florencio, D. F., Cruz, N. G., Santos, A. A., Oliveira, A. P., et al. (2016). Combined foraging strategies and soldier behaviour in Nasutitermes aff. coxipoensis (Blattodea: Termitoidea: Termitidae). Behav. Processes 126, 76–81. doi: 10.1016/j.beproc.2016.03.006
Araújo, A. P. A., Araújo, F. S., and Desouza, O. (2011). Resource suitability affecting foraging area extension in termites (Insecta, Isoptera). Sociobiology 57, 271–283.
Beckers, R., Deneubourg, J-. L., and Goss, S. (1993). Modulation of trail laying in the ant Lasius niger (Hymenoptera: Formicidae) and its role in the collective selection of a food source. J. Insect Behav. 6, 751–759. doi: 10.1007/BF01201674
Beekman, M., Sumpter, D. J. T., Seraphides, N., and Ratnieks, F. L. W. (2004). Comparing foraging behaviour of small and large honey-bee colonies by decoding waggle dances made by foragers. Funct. Ecol. 18, 829–835. doi: 10.1111/j.0269-8463.2004.00924.x
Beshers, S. N., and Fewell, J. H. (2001). Models of division of labor in social insects. Annu. Rev. Entomol. 46, 413–40. doi: 10.1146/annurev.ento.46.1.413
Breed, M. D., Fewell, J. H., Moore, A. J., and Williams, K. R. (1987). Graded recruitment in a ponerine ant. Behav. Ecol. Sociobiol. 20, 407–411. doi: 10.1007/BF00302983
Bruin, M. K-. D., Rost, L. C. M., and Draisma, F. G. A. M. (1977). Estimates of the number of foraging ants with the Lincoln-index method in relation to the colony size of Formica polyctena. J. Anim. Ecol. 457–470. doi: 10.2307/3823
Casarin, F. E., Costa-Leonardo, A. M., and Arab, A. (2008). Soldiers initiate foraging activities in the subterranean termite, Heterotermes tenuis. J. Insect Sci. 8, 2. doi: 10.1673/031.008.0201
Chouvenc, T., Ban, P. M., and Su, N-. Y. (2022). Life and death of termite colonies, a decades-long age demography perspective. Front. Ecol. Evol. 10. doi: 10.3389/fevo.2022.911042
Chouvenc, T., and Su, N-.Y. (2014). Colony age-dependent pathway in caste development of Coptotermes formosanus Shiraki. Insectes Soc. 61, 171–182. doi: 10.1007/s00040-014-0343-9
De Biseau, J. C., Deneubourg, J. L., and Pasteels, J. M. (1991). Collective flexibility during mass recruitment in the ant Myrmica sabuleti (Hymenoptera: Formicidae). Psyche 98, 323–336. doi: 10.1155/1991/38402
Du, H., Chouvenc, T., Osbrink, W. L. A., and Su, N. Y. (2017a). Heterogeneous distribution of castes/instars and behaviors in the nest of Coptotermes formosanus Shiraki. Insectes Soc. 64, 103–112. doi: 10.1007/s00040-016-0520-0
Du, H., Chouvenc, T., and Su, N-. Y. (2017b). Development of age polyethism with colony maturity in Coptotermes formosanus (isoptera: Rhinotermitidae). Environ. Entomol. 46, 311–318. doi: 10.1093/ee/nvw162
Eggleton, P. (2010). “An introduction to termites: biology, taxonomy and functional morphology,” in Biology of termites: a modern synthesis (Berlin: Springer), p. 1–26. doi: 10.1007/978-90-481-3977-4_1
Erickson, J. M. (1972). Mark-recapture techniques for population estimates of Pogonomyrmex ant colonies: an evaluation of the 32P technique. Ann. Entomol. Soc. Am. 65, 57–61. doi: 10.1093/aesa/65.1.57
Fewell, J. H. (1988). Energetic and time costs of foraging in harvester ants, Pogonomyrmex occidentalis. Behav. Ecol. Sociobiol. 22, 401–408. doi: 10.1007/BF00294977
Free, J. B. (1964). The allocation of duties among worker honeybees. Anim. Behav. 12, 389–390. doi: 10.1016/0003-3472(64)90032-6
Gerber, C., Badertscher, S., and Leuthold, R. H. (1988). Polyethism in Macrotermes bellicosus (Isoptera). Insectes Soc. 35, 226–240. doi: 10.1007/BF02224056
Golley, F. B., and Gentry, J. B. (1964). Bioenergetics of the southern harvester ant, Pogonomyrmex badius. Ecology 45, 217–225. doi: 10.2307/1933834
Gordon, D. M. (1996). The organization of work in social insect colonies. Nature 380, 121–124. doi: 10.1038/380121a0
Gordon, D. M. (2016). From division of labor to the collective behavior of social insects. Behav. Ecol. Sociobiol. 70, 1101–1108. doi: 10.1007/s00265-015-2045-3
Hangartner, W. (1969). Structure and variability of the individual odor trail in Solenopsis geminata Fabr. (Hymenoptera, Formicidae). Z. Vgl. Physiol. 62, 111–120. doi: 10.1007/BF00298046
Hangartner, W. (1970). Control of pheromone quantity in odor trails of the Antacanthomyops interjectus MAYR. Experientia 26, 664–665. doi: 10.1007/BF01898753
Haverty, M. I. (1977). The proportion of soldiers in termite colonies: a list and a bibliography. Sociobiology 2, 199–216.
Haverty, M. I. (1979). Soldier production and maintenance of soldier proportions in laboratory experimental groups of Coptotermes formosanus Shiraki. Insectes Soc. 26, 69–84. doi: 10.1007/BF02283914
Haverty, M. I., and Howard, R. W. (1981). Production of soldiers and maintenance of soldier proportions by laboratory experimental groups of Reticulitermes flavipes (Kollar) and Reticulitermes virginicus (Banks) (Isoptera: Rhinotermitidae). Insectes Soc. 28, 32–39. doi: 10.1007/BF02223620
Higashi, M., Abe, T., and Burns, T. P. (1992). Carbon—nitrogen balance and termite ecology. Proc. R. Soc. Lond. B,. Biol. Sci. 249, 303–308. doi: 10.1098/rspb.1992.0119
Hölldobler, B., and Wilson, E. O. (1990). The Ants. Cambridge, MA: Harvard University Press. doi: 10.1007/978-3-662-10306-7
Hölldobler, B., and Wilson, E. O. (2009). The Superorganism: The Beauty, Elegance, and Strangeness of Insect Societies. New York, NY: WW Norton and Company.
Janowiecki, M. A., and Vargo, E. L. (2022). Effect of soldiers on collective tunneling behavior in three species of Reticulitermes (Blattodea: Rhinotermitidae). Insectes Soc. 69, 237–245. doi: 10.1007/s00040-022-00864-6
Jeanne, R. L. (1986). The evolution of the organization of work in social insects. Monitore Zoologico Italiano-Italian J. Zool. 20, 119–133.
Kay, A. (2002). Applying optimal foraging theory to assess nutrient availability ratios for ants. Ecology 83, 1935–1944. doi: 10.1890/0012-9658(2002)0831935:AOFTTA2.0.CO
King, E. G., and Spink, W. T. (1969). Foraging galleries of the Formosan subterranean termite, Coptotermes formosanus, in Louisiana. Ann. Entomol. Soc. Am. 62, 536–542. doi: 10.1093/aesa/62.3.536
Korb, J., and Linsenmair, K. E. (2002). Evaluation of predation risk in the collectively foraging termite Macrotermes bellicosus. Insectes Soc. 49, 264–269. doi: 10.1007/s00040-002-8312-0
La Fage, J. P., and Nutting, W. L. (1978). “Nutrient dynamics of termites,” in Brian MV, eds Production Ecology of Ants and Termites (Cambridge, UK: Cambridge University Press), p. 165–232.
Lee, S-.B., Chouvenc, T., Mizumoto, N., Mullins, A., and Su, N-.Y. (2022). Age-based spatial distribution of workers is resilient to worker loss in a subterranean termite. Sci. Rep. 12, 1–8. doi: 10.1038/s41598-022-11512-1
Lee, S-.B., Chouvenc, T., and Su, N-.Y. (2019). A reproductives excluder for subterranean termites in laboratory experiments. J. Econ. Entomol. 112, 2882–2887. doi: 10.1093/jee/toz211
Lee, S-.B., Chouvenc, T., and Su, N-.Y. (2021). Differential time allocation of foraging workers in the subterranean termite. Front. Zool. 18, 1–8. doi: 10.1186/s12983-021-00446-5
Lee, S-.B., Su, N-.Y., Song, H-.S., and Lee, S.-H. (2020). Minimizing moving distance in deposition behavior of the subterranean termite. Ecol. Evol. 10, 2145–52. doi: 10.1002/ece3.6051
Lee, S.-H., Bardunias, P., and Su, N.-Y. (2006). Food encounter rates of simulated termite tunnels with variable food size/distribution pattern and tunnel branch length. J. Theor. Biol. 243, 493–500. doi: 10.1016/j.jtbi.2006.07.026
Lenz, M. (1994). “Food resources, colony growth and caste development in wood-feeding termites,” in Hunt, J., and Nalepa, C. A. Nourishment and Evolution in Insect Societies (Westview Press, Boulder. CO, USA: Westview Press), p. 159–210.
Lewis, T., Pollard, G. V., and Dibley, G. C. (1974). Rhythmic foraging in the leaf-cutting ant Atta cephalotes (L.)(Formicidae: Attini). J. Anim. Ecol. 129–141. doi: 10.2307/3162
Loftus, J. C., Perez, A. A., and Sih, A. (2021). Task syndromes: linking personality and task allocation in social animal groups. Behavioral Ecol. 32, 1–17. doi: 10.1093/beheco/araa083
MacKay, W. P. (1983). Stratification of workers in harvester ant nests (Hymenoptera: Formicidae). J. Kans. Entomol. Soc. 56, 538–542.
Matsumoto, T. (1976). The role of termites in an equatorial rain forest ecosystem of West Malaysia. I. Population density, biomass, carbon, nitrogen and calorific content and respiration rate. Oecologia 22, 153–178. doi: 10.1007/BF00344714
McCarthy, J., Khadka, A., Hakanoglu, H., and Sun, Q. (2023). Influence of soldiers on exploratory foraging behavior in the formosan subterranean termite, Coptotermes formosanus (Blattodea: Rhinotermitidae). Insects 14, 198. doi: 10.3390/insects14020198
Michael, Z., Chouvenc, T., Su, N-.Y., and Lee, S.-B. (2023). Finding shortcuts through collective tunnel excavations in a subterranean termite. Behav. Ecol. arad007. doi: 10.1093/beheco/arad007
Middleton, E. J. T., and Latty, T. (2016). Resilience in social insect infrastructure systems. J. R. Soc. Interface 13, 20151022. doi: 10.1098/rsif.2015.1022
Moritz, R. (1988). The biology of the honey bee. Anim. Behav. 36, 952–953. doi: 10.1016/S0003-3472(88)80187-8
Mullins, A., Chouvenc, T., and Su, N-.Y. (2021). Soil organic matter is essential for colony growth in subterranean termites. Sci. Rep. 11, 1–11. doi: 10.1038/s41598-021-00674-z
Noirot, C. (1989). Social structure in termite societies. Ethol. Ecol. Evol. 1, 1–17. doi: 10.1080/08927014.1989.9525528
Noirot, C., and Darlington, J. P. E. C. (2000). “Termite nests: architecture, regulation and defence,” in Termites: evolution, sociality, symbioses, ecology (Berlin: Springer), p. 121–139. doi: 10.1007/978-94-017-3223-9_6
Oster, G. F., and Wilson, E. O. (1978). Caste and Ecology in the Social Insects. Princeton: Princeton University Press.
Patel, J. S., Lee, S-.B., Chouvenc, T., and Su, N-.Y. (2020). Inferring termite colony size using wood consumption in subterranean termites (Blattodea: Rhinotermitidae) in laboratory-rearing conditions. J. Econ. Entomol. 113, 905–910. doi: 10.1093/jee/toz353
Porter, S. D., and Jorgensen, C. D. (1981). Foragers of the harvester ant, Pogonomyrmex owyheei: a disposable caste? Behav. Ecol. Sociobiol. 9, 247–256. doi: 10.1007/BF00299879
Porter, S. D., and Tschinkel, W. R. (1985). Fire ant polymorphism: the ergonomics of brood production. Behav. Ecol. Sociobiol. 16, 323–336. doi: 10.1007/BF00295545
Pyke, G. H., and Starr, C. K. (2021). “Optimal foraging theory,” in Encyclopedia of Social Insects (Berlin: Springer), p. 677–685. doi: 10.1007/978-3-030-28102-1_90
Robinson, G. E. (1992). Regulation of division of labor in insect societies. Annu. Rev. Entomol. 37, 637–665. doi: 10.1146/annurev.en.37.010192.003225
Rogers, L., Lavigne, R., and Miller, J. L. (1972). Bioenergetics of the western harvester ant in the shortgrass plains ecosystem. Environ. Entomol. 1, 763–768. doi: 10.1093/ee/1.6.763
Rytter, W., and Shik, J. Z. (2016). Liquid foraging behaviour in leafcutting ants: the lunchbox hypothesis. Anim. Behav. 117, 179–186. doi: 10.1016/j.anbehav.2016.04.022
Sacramento, J. J. M., Cristaldo, P. F., Santana, D. L., Cruz, J. S., dos Santos Oliveira, B. V., Dos Santos, A. T., et al. (2020). Soldiers of the termite Nasutitermes corniger (Termitidae: Nasutitermitinae) increase the ability to exploit food resources. Behav Processes. 181, 104272 doi: 10.1016/j.beproc.2020.104272
Seeley, T. D. (1989). Social foraging in honey bees: how nectar foragers assess their colony's nutritional status. Behav. Ecol. Sociobiol. 24, 181–199. doi: 10.1007/BF00292101
Seeley, T. D., Camazine, S., and Sneyd, J. (1991). Collective decision-making in honey bees: how colonies choose among nectar sources. Behav. Ecol. Sociobiol. 28, 277–290. doi: 10.1007/BF00175101
Seeley, T. D., and Kolmes, S. A. (1991). Age polyethism for hive duties in honey bees—illusion or reality? Ethology 87, 284–297. doi: 10.1111/j.1439-0310.1991.tb00253.x
Su, N-.Y., Osbrink, W., Kakkar, G., Mullins, A., and Chouvenc, T. (2017). Foraging distance and population size of juvenile colonies of the formosan subterranean termite (Isoptera: Rhinotermitidae) in laboratory extended arenas. J. Econ. Entomol. 110, 1728–1735. doi: 10.1093/jee/tox153
Su, N-.Y., and Scheffrahn, R. H. (1988). Foraging population and territory of the Formosan Subterranean Termite (Isoptera, Rhinotermitidae) in an urban-environment. Sociobiology 14, 353–360.
Szlep, R., and Jacobi, T. (1967). The mechanism of recruitment to mass foraging in colonies of Monomorium venustum Smith, M. subopacum ssp. Phœnicium Em., Tapinoma israelis For. and T. Simothi v. Phœnicium Em. Insectes Soc 14, 25–40. doi: 10.1007/BF02222750
Tian, L., and Zhou, X. (2014). The soldiers in societies: defense, regulation, and evolution. Int. J. Biol. Sci. 10, 296. doi: 10.7150/ijbs.6847
Traniello, J. F. A. (1981). Enemy deterrence in the recruitment strategy of a termite: soldier-organized foraging in Nasutitermes costalis. Proc. Nat. Acad. Sci. 78, 1976–1979. doi: 10.1073/pnas.78.3.1976
Traniello, J. F. A., and Leuthold, R. H. (2000). “Behavior and ecology of foraging in termites,” in Termites: evolution, sociality, symbioses, ecology (Berlin: Springer), p. 141–168. doi: 10.1007/978-94-017-3223-9_7
Von Frisch, K. (1967). The Dance Language and Orientation of Bees. Cambridge, MA: Harvard University Press.
Wheeler, D. E. (1986). Developmental and physiological determinants of caste in social Hymenoptera: evolutionary implications. Am. Nat. 128, 13–34. doi: 10.1086/284536
Keywords: task allocation, C. formosanus, subterranean termite, flexibility, foraging
Citation: Lee S-B and Su N-Y (2023) Foraging proportion of the Formosan subterranean termite workers and soldiers in relation to soil type. Front. Ecol. Evol. 11:1091395. doi: 10.3389/fevo.2023.1091395
Received: 07 November 2022; Accepted: 07 April 2023;
Published: 05 May 2023.
Edited by:
Alberto Arab, Federal University of ABC, BrazilReviewed by:
Fabiana E. Casarin, Federal University of São Paulo, BrazilCopyright © 2023 Lee and Su. This is an open-access article distributed under the terms of the Creative Commons Attribution License (CC BY). The use, distribution or reproduction in other forums is permitted, provided the original author(s) and the copyright owner(s) are credited and that the original publication in this journal is cited, in accordance with accepted academic practice. No use, distribution or reproduction is permitted which does not comply with these terms.
*Correspondence: Sang-Bin Lee, bHNiNTE2MkB1ZmwuZWR1
†ORCID: Sang-Bin Lee orcid.org/0000-0001-7982-0842
Nan-Yao Su orcid.org/0000-0002-0431-5005
Disclaimer: All claims expressed in this article are solely those of the authors and do not necessarily represent those of their affiliated organizations, or those of the publisher, the editors and the reviewers. Any product that may be evaluated in this article or claim that may be made by its manufacturer is not guaranteed or endorsed by the publisher.
Research integrity at Frontiers
Learn more about the work of our research integrity team to safeguard the quality of each article we publish.