- 1Department of Plant and Wildlife Science, Brigham Young University, Provo, UT, United States
- 2Utah Division of Wildlife Resources, Salt Lake City, UT, United States
- 3Clark Family School of Environment and Sustainability, Western Colorado University, Gunnison, CO, United States
Maternal effects are the influence of maternal phenotype and the maternally-provided environment on the phenotype (i.e., expression of traits) of offspring. Frequently, maternal effects are manifest both before and after parturition. Pre-parturition effects are primarily direct allocation of energy to the offspring that is in utero. Post-parturition effects can include direct (e.g., nursing and defending offspring) and indirect (e.g., selection of habitat that is relatively safe or has high nutritional value) influences. While both direct and indirect effects are often discussed, there is a paucity of information on the relative importance of each type on offspring due to the difficulty in monitoring mothers prior to parturition and mother-offspring relationships after parturition in free-ranging animals. Our objective was to determine the importance of direct maternal effects on birth weight, growth rates, and survival of mule deer (Odocoileus hemionus) fawns from birth through the first 18 months of life. We determined the effect of nutritional condition of the dam (mother) on birth weight (pre-parturition direct effect). We also examined the post-parturition direct effect of dam nutritional condition on growth rates and survival of fawns. Direct maternal effects were evident both before and after parturition; dams in better nutritional condition produced offspring with greater mass at birth, higher rates of growth, and increased survival. Our findings demonstrate that maternal nutritional condition influences fawn health from gestation through recruitment. These links highlight the importance of considering direct maternal effects when examining population dynamics and reproductive success in long-lived mammals. Management plans for ungulates should include assessment of nutritional condition of adult females to maximize likelihood of effective conservation.
1. Introduction
Maternal effects are the influence of maternal phenotype and “the maternally provided environment” on the phenotype (i.e., expression of traits) of offspring (Bernardo, 1996; Wolf and Wade, 2009). Life history theory posits that females must balance their own needs and probability of survival with current and future reproductive events (Festa-Bianchet et al., 1998; Marshall and Uller, 2007). When resources are abundant, females often produce larger or more offspring by allocating more energy toward current reproduction (Haywood and Perrins, 1992; Bardsen et al., 2008). However, when resources are scarce, females often allocate less energy to current reproduction (e.g., produce smaller and fewer offspring) to maximize lifetime fitness (Smith and Fretwell, 1974; Einum and Fleming, 2000). Producing smaller offspring potentially decreases current maternal reproductive success in exchange for enhancing survival to a future reproductive bout. Therefore, maternal effects may have a positive or negative influence on the fitness potential of offspring (Kirkpatrick and Lande, 1989; Marshall and Uller, 2007; Freeman et al., 2013). Because of this maternal influence on fitness and production, effective conservation can only be achieved with an understanding maternal effects.
Identifying the life-history traits of offspring that are influenced by maternal effects can be difficult, as there are numerous mechanisms driving both life-history traits and maternal effects (Kirkpatrick and Lande, 1989; Benton et al., 2001). Maternal nutritional condition and subsequent energy allocation have been shown to influence offspring traits, including size at birth (Feiner et al., 2016), growth rate (Haywood and Perrins, 1992), survival (Duquette et al., 2014), age at first reproduction, and fecundity. Nonetheless, maternal effects may disappear after the period of maternal care (Gendreau et al., 2005) or be masked by the influence of environmental conditions in long-lived species (Hewison and Gaillard, 1999). Further, relatively little is known about the importance of maternal effects in large, long-lived species due to the difficulty of studying maternal-offspring relationships in free-ranging mammals. Therefore, determining the duration of maternal effects can be challenging but is critically important for understanding life-history, and can have important implications for conservation.
Ungulates provide a model system to study the duration of maternal effects because they are iteroparous and long-lived (Freeman et al., 2013). Short-term maternal effects, from birth to weaning and during early-life growth, exist in some ungulate species (Kojola, 1993; Wauters et al., 1995; Festa-Bianchet and Jorgenson, 1998). Long-lasting maternal effects may also be present. An intergenerational maternal effect exists on growth and size at maturity in white-tailed deer (Odocoileus virginianus; Monteith et al., 2009). Additionally, maternal effects last into adulthood for antler growth in elk (Cervus canadensis) and mule deer (Odocoileus hemionus), a sexually selected trait that influences fitness potential (Freeman et al., 2013). Despite some evidence for both short- and long-term maternal effects, maternal effects among offspring of ungulate species from early life through recruitment are not well understood.
Maternal effects can manifest both before and after parturition (Bernardo, 1996). However, there has been little differentiation between effects in utero or during rearing of offspring. Pre-parturition effects are primarily direct allocation of energy to the offspring that is in utero (Robbins and Robbins, 1979). Nevertheless, both direct and indirect maternal effects can influence offspring post-parturition. For example, direct effects include energy and nutrient allocation such as milk to nursing young and indirect effects include protection of young (Wolf and Wade, 2009), habitat selection, and maternal care other than nursing (Bernardo, 1996).
Here we focus on direct maternal effects in mule deer fawns. Our objective was to determine the importance of direct maternal effects on birth weight, growth rates, and survival of neonates through 18 months of life. More specifically, we examined the influence of nutritional condition of dams on birth weight, growth rates, and survival of offspring. We hypothesized that maternal nutritional condition would influence offspring both while in utero (birth weight) and throughout the lactation period (growth rate and survival). We predicted that greater maternal condition would be correlated with increased birth weight, growth, and survival of fawns. We predicted that greater maternal condition would lead to larger body sizes for fawns at 6 months of age and that larger fawns would have a greater likelihood of survival through their first winter to recruitment at 18 months of age.
2. Materials and methods
2.1. Study area
We conducted this study on the Cache Management Area in northern Utah, United States. This area comprised a portion of the Bear River Range and consisted of federal, state and private lands. Land uses in this area included crop cultivation, agricultural grazing, forest recreational use, and private residences. The topography included steep mountains, deep canyons and high mountain valleys with elevation that ranged from 1,300 to 3,000 meters. The area was comprised of high elevation coniferous forest and lower elevation shrub steppe habitats. High elevation forests were predominantly comprised of lodgepole pine (Pinus contorta), Engelmann spruce (Picea englemannii), and white fir (Abies concolor). Low elevation shrub steppe was dominated by sagebrush (Artemisia tridentate), bitterbrush (Purshia tridentate), snowberry (Symphoricarpos albus), chokecherry (Prunus virginiana), and aspen (Populus tremuloides). Mule deer in this system typically migrated from relatively high elevations to low elevation each fall (e.g., November) and returned to high elevations each spring (e.g., April–May). Potential competitors of mule deer included elk (Cervus canadensis), pronghorn (Antilocapra americana), moose (Alces alces), domestic cattle (Bos taurus), and domestic sheep (Ovis aries). Primary predators of mule deer included coyote (Canis latrans) and mountain lion (Puma concolor). Average annual temperature ranged from 7 to 27° C during the summer and from −10 to 2° C during the winter, with average annual precipitation of 80 cm per year, the majority of which occurred during winter (PRISM Climate Group, Oregon State University, https://prism.oregonstate.edu, accessed 15 Jan 2021).
2.2. Capture of adult female mule deer
During March of 2018–2020, we captured adult female deer via helicopter net-gunning (Barrett et al., 1982; Krausman et al., 1985; White and Bartmann, 1994). Individuals were hobbled, blindfolded, and transported to one of four processing stations in the study area. We recorded weight, body size (hind foot length, chest and neck girth), nutritional condition, and age (estimation based on tooth wear; Severinghaus, 1949; Robinette et al., 1957) of each captured female prior to release. We estimated nutritional condition by estimating ingesta-free body fat using ultrasonography measures of rump fat and loin muscle thickness, body weight, and a palpation score (Cook et al., 2004, 2010). We determined pregnancy via transabdominal ultrasonography (E.I. Medical Imaging portable ulstrasound; Smith and Lindzey, 1982). Pregnant females were fitted with GPS collars equipped with Neolink technology and a vaginal implant transmitter (VIT; Model M3930U, Advanced Telemetry Systems Inc., Isanti, MN, United States) with a temperature and light sensitive switch (Bishop et al., 2007). Neolink radio-pairing technology in the GPS collar monitors the status of the VIT and allows remote monitoring of both VITs and neonate collars via the mother’s collar. When VITs were expelled from deer during parturition and the VIT detected light or a temperature below 32°C, the VIT broadcast a birth message to the GPS collar. The birth message sent to the GPS collar triggered an email alerting us of the birthing event. In addition, the very high frequency (VHF) beep pattern of the VIT also changed from a 30 ppm pattern to a 60 ppm pattern. Because we were interested in maternal effects through early life, only those females that lived through December were incorporated in the study.
2.3. Neonate capture and monitoring
When an email alert of a birthing event was received, we waited at least 6 h to allow time for bonding between adult and offspring before beginning our search for neonates (Heffelfinger et al., 2018). We used a combination of the GPS location, from the adult collar at the time the VIT was expelled, and radio-telemetry to locate the VIT and parturition site. Once the VIT was located, we performed a systematic search to locate neonatal fawn(s). Upon discovery of neonates, we fitted them with a Neolink series VHF, mortality-sensing, drop-off radio collar (Model M4230BU, Advanced Telemetry Systems, Isanti, MN, United States). We recorded several measurements including weight, chest girth, hind foot length, and new hoof growth. We measured new hoof growth as a secondary measure of neonate age because the birth of some fawns was delayed from the time the VIT was expelled (to estimate the age of the neonate(s); Haugen and Speake, 1958; Robinette et al., 1973; Sams et al., 1996; Lomas and Bender, 2007). We handled neonates with nitrile gloves, kept handling time to a minimum, and replaced them at site of capture in order to reduce the transfer of human scent and the likelihood of maternal abandonment.
If the neonate collar remained motionless for 8 h, a mortality warning was also sent and the VHF beep-pattern increased from 30 to 60 ppm. We attempted to locate neonates within 24 h of a mortality notification in order to determine cause of mortality. After locating a mortality, we searched for the fawn carcass and examined evidence found at the mortality site. We used field or lab necropsies to determine cause of death and classified mortalities into the following causes using criteria from the literature: bobcat predation, cougar predation, coyote predation, unknown predation, malnutrition/disease, accident, capture related, and unknown (White, 1973; Gese and Grothe, 1995; Stonehouse et al., 2016). We did not analyze cause of death relative to nutritional condition of the dam because sample size was too small to determine relationships between nutritional condition and the many causes of death. All handling of animals was approved by the Institutional Animal Care and Use Committee at Brigham Young University, and was in accordance with guidelines of the American Society of Mammologists (Sikes, 2016).
Growth rate was determined by subtracting birth weight from weight at 6 months of age and dividing by the number of days between captures. To bolster our sample size of fawns monitored from 6 months of age to 18 months of age, we captured uncollared fawns each December. These new animals were captured at the same time we captured collared fawns to determine body weight and growth rate of neonates when they had reached 6 months of age. The data collected on new fawns that were captured for the first time during December each year included sex and body weight. These new animals were only included in our analysis of the relationship between body weight and the likelihood of survival from 6 months to 18 months of age.
2.4. Statistical analyzes
We used generalized linear models and model selection in program R (version 4.0.2) to determine the influence of maternal effects on birth weight and growth rate of neonates, and body size at 6 months. In addition to the base program, we used the MuMIn package to rank models and the ggplot2 package to produce figures (Wickham, 2016; Barton, 2020). We used the explanatory variables maternal age and condition, neonate birth weight, sex, presence of twin, hoof growth, capture date, year of birth, and fawn growth. We formulated a list of a priori models for each of the response variables including birth weight, growth rate, and body size at 6 months of age. Prior to construction of models, we evaluated variables for multicollinearity and excluded explanatory variables that were correlated (r > |0.50|) in the same model. We evaluated and ranked a priori models using Akaike’s Information Criteria adjusted for small sample sizes (AICc) and AICc weights (Akaike, 1973; Anderson and Burnham, 2002). We considered strongly competing models to be those with ΔAICc <2. In the event of competing models, we averaged β coefficients from models that carried >5% AICc weight.
We evaluated survival from birth to 6 months of age, and from 6 months of age to 18 months of age using Cox Proportional Hazard (CPH) models using the s package in program R (Therneau, 2015). CPH models allow for estimates of survival for each individual, based on sampled variables and varying time components (Cox, 1972; Fox and Weisberg, 2002). Time components for survival to 6 months included monthly survival from zero to 6 months of age, and a comparison of survival between the first month of life and months two through six following parturition. Potential explanatory variables included maternal nutritional condition, neonate sex, birth weight, presence of a twin, and day relative to mean date of parturition. We modeled survival to 18 months of age as a daily time step. Because some of the additional juveniles sampled had unknown parentage, we limited variables included in this model to sex, year, and capture weight. We determined the most influential factors associated with neonate survival to 6 months and juvenile survival to 18 months. Similar to the generalized linear models, we first formulated a priori models and then ranked them based on minimization of Akaike’s Information Criteria adjusted for small sample sizes (AICc) and AICc weights (Akaike, 1973; Anderson and Burnham, 2002).
3. Results
Between March of 2018 and March of 2020 we captured and marked 89 female mule deer with GPS collars. Of these adults, 22 individuals were captured in two consecutive years and one individual was captured all 3 years for a total of 112 capture events. Average ingesta-free body fat (IFBF) for adult females was 6.06% in 2018, 4.34% in 2019, and 6.26% in 2020. Ultrasonography revealed that 106 of 112 (95%) captured animals were pregnant. We captured neonates from 61 collared adult females. In three fawning seasons we located 104 neonates and captured and collared all 98 individuals (6 were stillborn). Over the course of the study we observed 39 sets of twins, and 20 singletons. Of neonates captured, 49 were male and 49 were female. Parturition dates ranged from 28 May to 29 June. Mean date of parturition was June 8th, 14th, and 9th during 2018–2020, respectively. Between December of 2018 and December of 2020, we captured and collared 59 mule deer fawns at approximately 6 months of age. Of the 59 juveniles captured, 27 were individuals originally captured as neonates. Average weight was 30.5 kg in 2018 (SE = 1.2), 31.0 kg in 2019 (SE = 0.8), and 33.6 kg in 2020 (SE = 1.0).
We examined the influence of maternal IFBF on birth weight for 98 neonates. Explanatory variables associated with timing of parturition (mean, median, onset) were highly correlated (r > |0.50|) along with onset and hoof growth. Thus, we did not include these variables together in the same model, but did allow them to occur across models for evaluation. Out of 23 candidate models, the top model examining neonate birth weight accounted for 78.4% of the AICc weight compared to 15.4% for the second-ranked model (no competing models, ΔAICc<2; Table 1). The most supported model included influence of twin, sex, hoof growth, IFBF, and maternal age. As predicted, birth weight of neonates was positively related to IFBF. On average for females with the lowest IFBF, neonates weighed 3.0 kg at birth (SE = 0.1). For females with higher IFBF, neonates averaged 3.5 kg at birth (SE = 0.1; Figure 1). Presence of a twin was associated with lower birth weight. On average, neonates associated with a twin weighed 0.2 kg less than those that were singletons. Age was in the top model but confidence intervals around the estimate overlapped zero. Hoof growth was positively associated with birth weight, but of limited relevance. Measurement of hoof growth is used to determine age of neonate at capture, and older neonates would be heavier than those captured closer to parturition. Males were also associated with greater birth weight (Table 1).
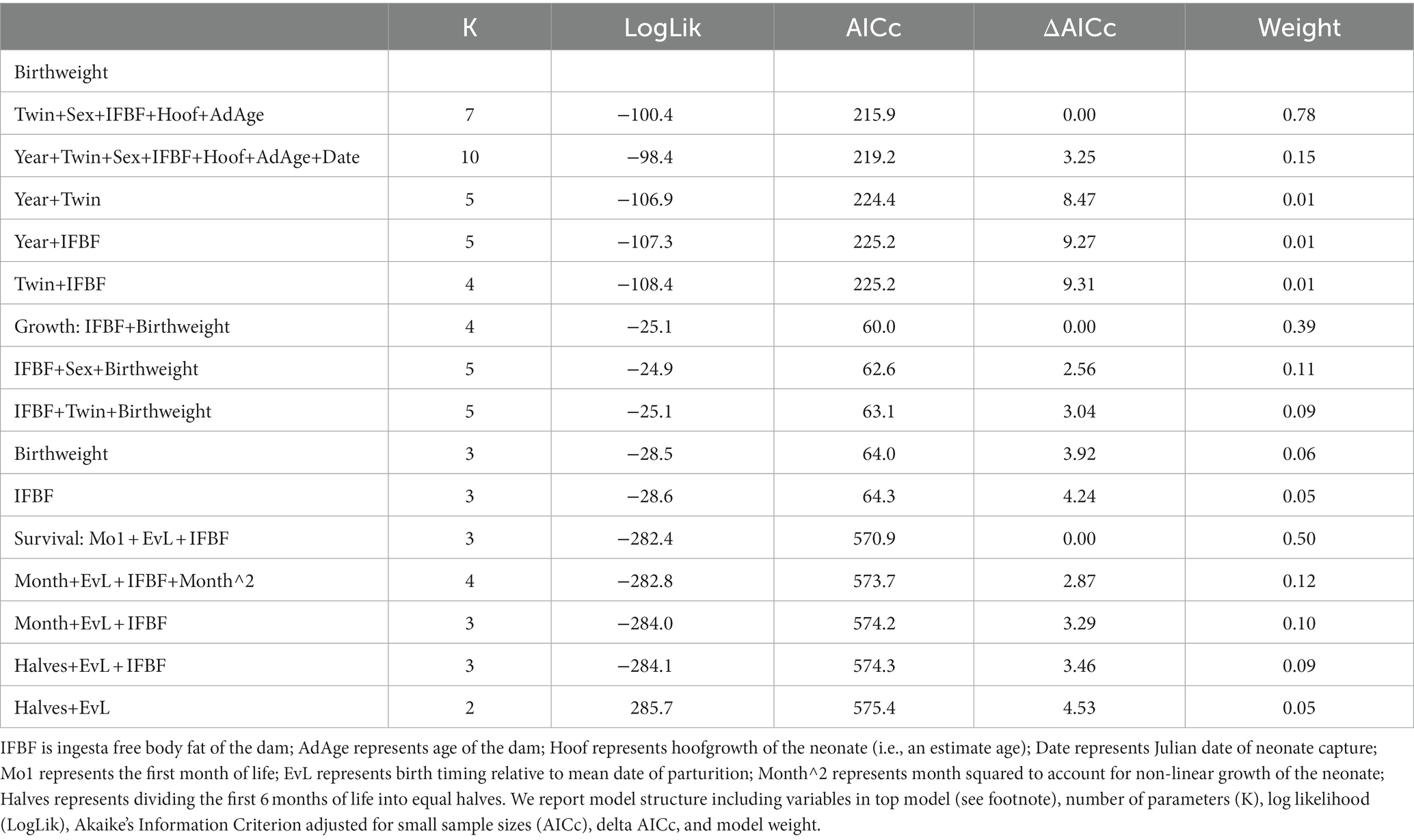
Table 1. Model selection results for response variables of birthweight, growth, and survival to 6 months of age for mule deer (Odocoileus hemionus) in northern Utah, United States, 2018–2020.
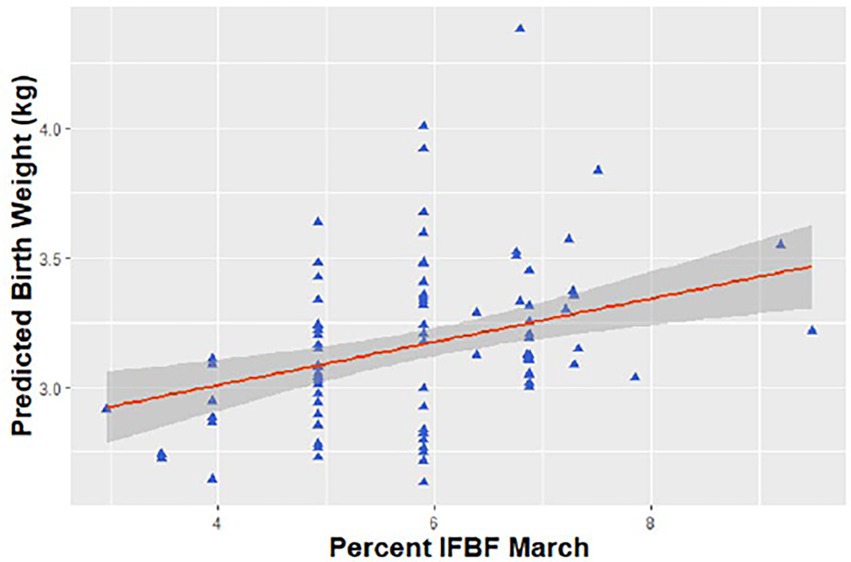
Figure 1. Predicted relationship between birth weight of neonatal mule deer (Odocoileus hemionus) and maternal condition (measured in March) in northern Utah, United States, 2018–2020.
Growth rate of neonates from birth to 6 months of age was related to nutritional condition of the dam. We had four competing models (ΔAICc<2) and averaged all models that carried >5% AICc weight. The averaged models had a cumulative weight of 65.3% (Table 1). Variables in supported models included maternal IFBF, birth weight, sex, and presence of a twin. Maternal IFBF during gestation was positively related to growth through the first 6 months of life. On average neonates from females with higher IFBF grew at a rate of 5.2 kg per month (SE = 0.2), while those from females with low IFBF grew at a rate of 4.4 kg per month (SE = 0.2; Figure 2).
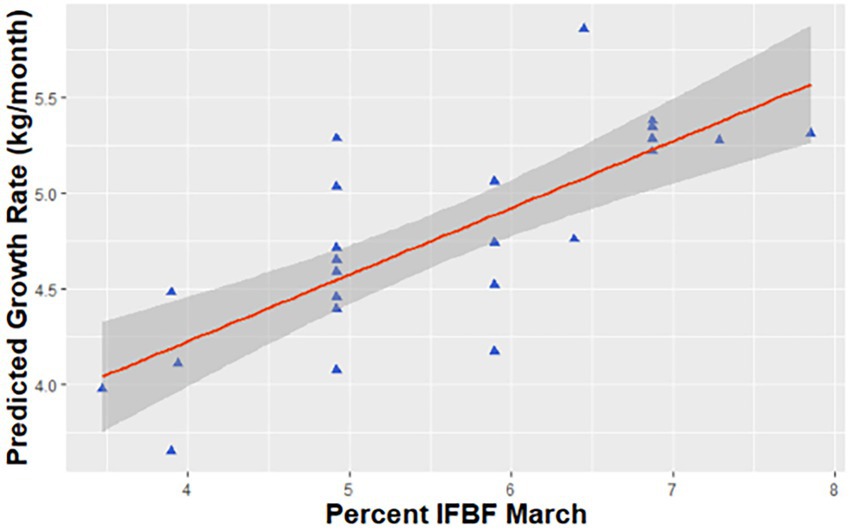
Figure 2. Predicted relationship between growth of neonatal mule deer (Odocoileus hemionus) to 6 months of age and maternal condition in March for animals from northern Utah, United States, 2018–2020.
Our top CPH model for neonate survival to 6 months accounted for 50% of the AICc weight (no competing models, ΔAICc<2.0) and included the influence of maternal IFBF, a variable time component of month one versus months 2–6, and whether the neonate was born before or after the peak of parturition (Table 1). Neonate survival to 6 months was positively influenced by maternal IFBF. Neonates born to females with higher IFBF had a higher likelihood of survival than neonates from females with lower IFBF (Figure 3). There was a lower likelihood of survival in month one compared to months two through six, especially for fawns born after peak parturition.
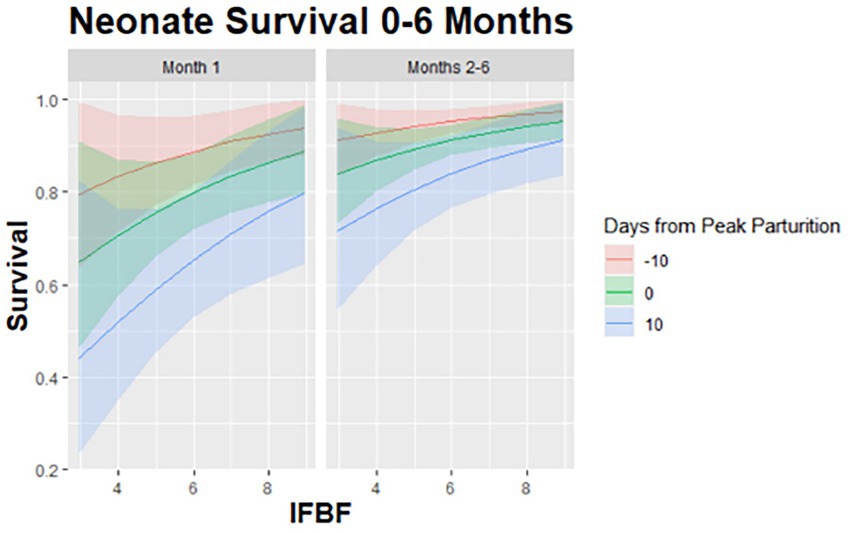
Figure 3. Predicted relationship for survival of neonatal mule deer (Odocoileus hemionus) to 6 months of age based on maternal condition and birth timing (EVL) in northern Utah, United States, 2018–2020.
We also examined the influence of maternal IFBF on body size at 6 months for 59 individuals. The top model, with 43% of the AICc weight included maternal age and birth weight (Table 2). After accounting for the effect of maternal age and birth weight, the next ranked model, with 24% of the AICc weight, included maternal IFBF. While the effect was not as strong as at birth, juveniles from females with higher IFBF weighed an average of 4.1 kg more than juveniles from females with lower IFBF (SE = 1.3; Figure 4).
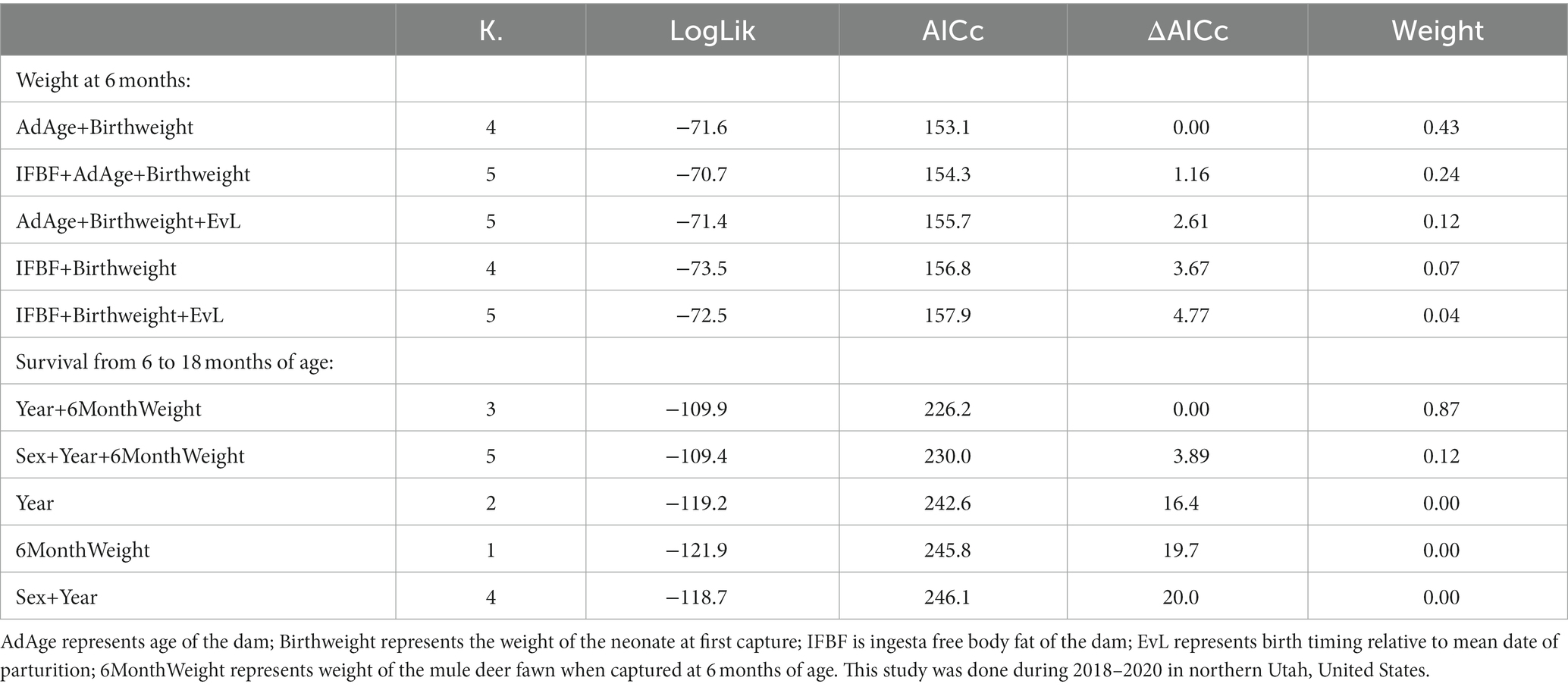
Table 2. Model selection results (Akaike’s Information Criterion) from analyzes examining the effect of covariates on weight of mule deer fawns at 6 months of age and survival of mule deer fawns from 6 months of age to 18 months of age.
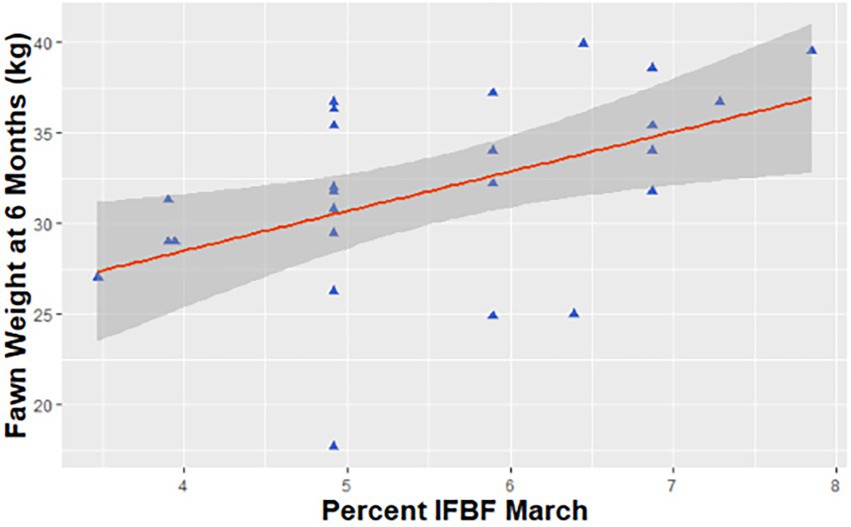
Figure 4. Predicted relationship between weight of mule deer (Odocoileus hemionus) fawns at 6 months of age and maternal condition in northern Utah, United States, 2018–2020.
Our top CPH model for survival from six to 18 months accounted for 87% AICc weight. Our most supported model of survival included the influence of weight and year (Table 2). As predicted, larger fawns had a greater likelihood of survival. Deer fawns weighing 35 kg at 6 months of age had at least a 50% probability of survival (Figure 5). Year of birth was a random effect in our models that also influenced survival likely due to the variation in summer precipitation (i.e., food availability) and winter severity among years.
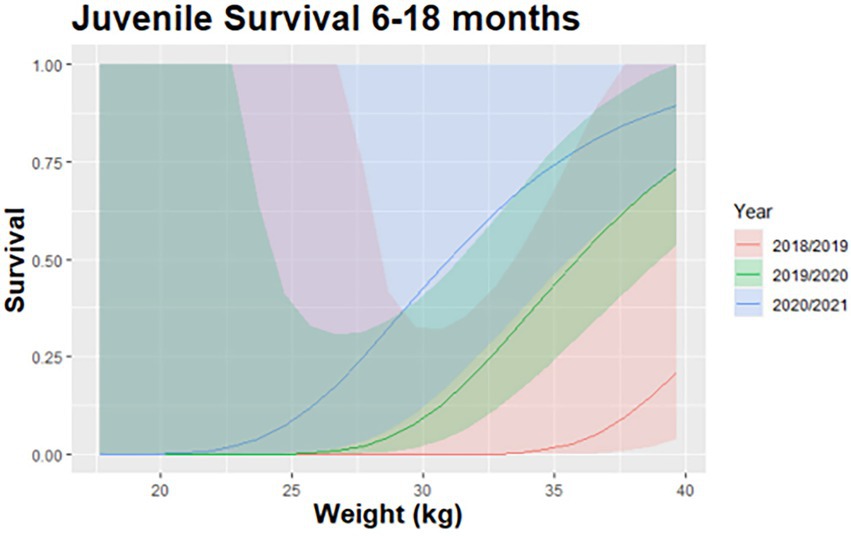
Figure 5. Predicted relationship between survival of mule deer (Odocoileus hemionus) fawns from 6 months of age to 18 months of age and juvenile weight in northern Utah, United States, 2018–2020.
4. Discussion
The study of maternal effects to determine phenotypic quality of offspring has become foundational for understanding life-history characteristics (Kirkpatrick and Lande, 1989; Bernardo, 1996). Until recently, technological constraints have limited the ability to monitor free-ranging ungulates and their offspring. Recent technological advancements, however, allow insight into the relationship between maternal nutritional condition and outcomes for offspring (Johnson et al., 2019). These advances have enabled the more precise study of mother-offspring relationships and the natural history of mule deer during early life. Nutritional condition of the dam has been linked to increased survival of neonatal mule deer to 140 days (Monteith et al., 2014). Here, for the first time, however, we connect the influence of maternal nutritional condition to indicators of health (including survival) in mule deer from birth to recruitment at 18 months of age. Consistent with other ungulates, nutritional condition influenced neonatal mule deer both before (e.g., birth weight of neonate mule deer) and after (e.g., growth rate and survival of neonate mule deer) parturition (Duquette et al., 2014; Feiner et al., 2016). Consistent with our prediction, increased nutritional condition of the dam was correlated with increased birth weight, growth rate, and survival of fawns to 6 months of age. Additionally, the effects of maternal nutritional condition lasted through early life and influenced juvenile body weight at 6 months of age and survival through recruitment at 18 months of life.
Mule deer experienced a pre-parturition direct effect of allocation of energy to offspring in utero, manifest as birth weight. Our findings indicate that nutritional condition of females (measured as IFBF) during gestation had a significant impact on birthweight of offspring. Fawns born to females in relatively good nutritional condition were approximately 40% heavier at birth, which could lead to greater potential fitness (Monteith et al., 2009). This finding is consistent with previous research (Hudson and Browman, 1959; Short, 1970; Heffelfinger et al., 2018). Moreover, birth weight is a strong predictor of growth and survival (Monteith et al., 2014).
Post-parturition direct effects were also present through the lactation period, as demonstrated by the influence of maternal nutritional condition on growth rates. There is likely a correlation between quality or quantity of milk and maternal nutritional condition during gestation. The amount and quality of milk produced by the mother is important for growth and development of young (Cook et al., 2004; Tollefson et al., 2010, 2011). Adult females in better condition likely produce more or higher quality milk, which in turn provides greater energy to nursing fawns and subsequent increased growth and survival rates. While there are many environmental factors that may also influence growth to 6 months, few investigators have been able to recapture free-ranging juveniles to monitor growth during the initial months of life.
We were able to clearly link maternal nutritional condition to survival of juvenile mule deer after parturition consistent with current understanding in this species (Monteith et al., 2014). This effect was present even after accounting for the influence of parturition timing or Julian date that the neonate was born. Fawns have a higher likelihood of survival if their mother is in good nutritional condition and if they are born at or before the mean date of parturition. Specifically, fawns from females in the best nutritional condition, born before peak parturition had a roughly 50% greater chance of survival than fawns from females in poor nutritional condition, born after peak parturition (Figure 3). There are likely two advantages associated with being born early—the animal becomes mobile before predators have developed a search image for neonates and the neonates have extra time to grow before the onset of winter. The effects of maternal nutritional condition on survival are consistent with evidence in other ungulate species that heavier females give birth earlier (Cameron et al., 1993; Keech et al., 2000).
Our results do not support the assertion that maternal effects weaken after maternal care ends (Gendreau et al., 2005). Maternal care lessens after weaning, which occurs during late summer and early fall for mule deer (Bowyer, 1991; Tollefson et al., 2011). Here, however, we found that maternal nutritional condition influenced offspring even after weaning, as fawns from mothers in better nutritional condition were heavier when recaptured in early winter at approximately 6 months of age (Figure 4). Further, the influence of maternal nutritional condition persists through the first 18 months of life because capture weight at 6 months of age strongly predicted survival to recruitment age at 18 months. Small sample sizes of recaptured individuals with known parentage prevented us from directly examining the influence of maternal nutritional condition on survival to 18 months. Nonetheless, our data demonstrate that weight at 6 months of age influenced survival to 18 months. Therefore, we infer a connection between maternal nutritional condition, mass of fawns at 6 months of age, and survival of fawns to 18 months of age.
Understanding the link between maternal effects and population dynamics is critical for the conservation and management of mule deer and other long-lived ungulates. For example, the reproductive potential of a population is not only influenced by pregnancy rate and litter size (Noyes et al., 2002; Souza et al., 2022), but also nutritional condition of the dams that influences the survival and expression of phenotypic traits in offspring. The phenotypic traits of young ungulates likely influence all aspects of life history, beyond just survival as we have demonstrated here, including susceptibility to predation (Murray, 2002) and disease (Beldomenico and Begon, 2010), and potential size and reproductive success (Keech et al., 2000; Freeman et al., 2013) as an adult. Therefore, monitoring and managing nutritional condition is fundamental to management and conservation of mule deer, and likely other ungulate populations.
Data availability statement
The raw data supporting the conclusions of this article will be made available by the authors, without undue reservation.
Author contributions
SL, BM, PF, KH, and RL conceived and designed the study. SL, BM, KH, and RL collected the field data. SL, MK, and RL conducted the statistical analysis. SL and BM wrote the manuscript with input from all authors. All authors contributed to the article and approved the submitted version.
Funding
This research was funded by Sportsmen for Fish and Wildlife, Utah Division of Wildlife Resources (Award #166154), and Brigham Young University.
Acknowledgments
We express our thanks to T. Hughes, T. Billin, J. Chandler, D. Sallee, and all other volunteers who helped with neonate captures. Additionally, we acknowledge the professional expertise of the Heliwild crew in capturing the adult mule deer for this study.
Conflict of interest
The authors declare that the research was conducted in the absence of any commercial or financial relationships that could be construed as a potential conflict of interest.
Publisher’s note
All claims expressed in this article are solely those of the authors and do not necessarily represent those of their affiliated organizations, or those of the publisher, the editors and the reviewers. Any product that may be evaluated in this article, or claim that may be made by its manufacturer, is not guaranteed or endorsed by the publisher.
References
Akaike, H. (1973). Information Theory as an Extension of the Maximum Likelihood Principle. Budapest, Hungary: Akademiai Kiado.
Anderson, D. R., and Burnham, K. P. (2002). Avoiding pitfalls when using information-theoretic methods. J. Wildl. Manag. 66, 912–918. doi: 10.2307/3803155
Bardsen, B. J., Fauchald, P., Tveraa, T., Langeland, K., Yoccoz, N. G., and Ims, R. A. (2008). Experimental evidence of a risk-sensitive reproductive allocation in a long-lived mammal. Ecology 89, 829–837. doi: 10.1890/07-0414.1
Barrett, M. W., Nolan, J., and Roy, L. D. (1982). Evaluation of a hand-held net-gun to capture large mammals. Wildl. Soc. Bull. 10, 108–114.
Beldomenico, P. M., and Begon, M. (2010). 'Vicious circles' and disease spread: elements of discussion response. Trends Ecol. Evol. 25:132. doi: 10.1016/j.tree.2009.10.006
Benton, T. G., Ranta, E., Kaitala, V., and Beckerman, A. P. (2001). Maternal effects and the stability of population dynamics in noisy environments. J. Anim. Ecol. 70, 590–599. doi: 10.1046/j.1365-2656.2001.00527.x
Bernardo, J. (1996). Maternal effects in animal ecology. Am. Zool. 36, 83–105. doi: 10.1093/icb/36.2.83
Bishop, C. J., Freddy, D. J., White, G. C., Watkins, B. E., Stephenson, T. R., and Wolfe, L. L. (2007). Using vaginal implant transmitters to aid in capture of mule deer neonates. J. Wildl. Manag. 71, 945–954. doi: 10.2193/2006-123
Bowyer, R. T. (1991). Timing of parturition and lactation in southern mule deer. J. Mammal. 72, 138–145. doi: 10.2307/1381988
Cameron, R. D., Smith, W. T., Fancy, S. G., Gerhart, K. L., and White, R. G. (1993). Calving success of female caribou in relation to body weight. Can. J. Zool. 71, 480–486. doi: 10.1139/z93-069
Cook, R. C., Cook, J. G., Stephenson, T. R., Myers, W. L., Mccorquodale, S. M., Vales, D. J., et al. (2010). Revisions of rump fat and body scoring indices for deer, elk, and moose. J. Wildl. Manag. 74, 880–896. doi: 10.2193/2009-031
Cook, J. G., Johnson, B. K., Cook, R. C., Riggs, R. A., Delcurto, T., Bryant, L. D., et al. (2004). Effects of summer-autumn nutrition and parturition date on reproduction and survival of elk. Wildl. Monogr. 155, 1–61. doi: 10.2193/0084-0173(2004)155[1:EOSNAP]2.0.CO;2
Duquette, J. F., Belant, J. L., Svoboda, N. J., Beyer, D. E. Jr., and Lederle, P. E. (2014). Effects of maternal nutrition, resource use and multi-predator risk on neonatal white-tailed deer survival. PLoS One 9:e100841. doi: 10.1371/journal.pone.0100841
Einum, S., and Fleming, I. A. (2000). Highly fecund mothers sacrifice offspring survival to maximize fitness. Nature 405, 565–567. doi: 10.1038/35014600
Feiner, Z. S., Wang, H. Y., Einhouse, D. W., Jackson, J. R., Rutherford, E. S., Schelb, C., et al. (2016). Thermal environment and maternal effects shape egg size in a freshwater fish. Ecosphere 7:e01304. doi: 10.1002/ecs2.1304
Festa-Bianchet, M., Gaillard, J.-M., and Jorgenson, J. T. (1998). Mass-and density-dependent reproductive success and reproductive costs in a capital breeder. Am. Nat. 152, 367–379. doi: 10.1086/286175
Festa-Bianchet, M., and Jorgenson, J. T. (1998). Selfish mothers: reproductive expenditure and resource availability in bighorn ewes. Behav. Ecol. 9, 144–150. doi: 10.1093/beheco/9.2.144
Fox, J., and Weisberg, S. (2002). Cox Proportional-Hazards Regression for Survival Data. An R and S-PLUS Companion to Applied Regression, 2002.
Freeman, E. D., Larsen, R. T., Clegg, K., and Mcmillan, B. R. (2013). Long-lasting effects of maternal condition in free-ranging cervids. PLoS One 8:e58373. doi: 10.1371/journal.pone.0058373
Gendreau, Y., Côté, S. D., and Festa-Bianchet, M. (2005). Maternal effects on post-weaning physical and social development in juvenile mountain goats (Oreamnos americanus). Behav. Ecol. Sociobiol. 58, 237–246. doi: 10.1007/s00265-005-0938-2
Gese, E. M., and Grothe, S. (1995). Analysis of coyote predation on deer and elk during winter in Yellowstone National Park, Wyoming. Am. Midl. Nat. 133, 36–43. doi: 10.2307/2426345
Haugen, A. O., and Speake, D. W. (1958). Determining age of young fawn white-tailed deer. J. Wildl. Manag. 22, 319–321. doi: 10.2307/3796471
Haywood, S., and Perrins, C. M. (1992). Is clutch size in birds affected by environmental conditions during growth? Proc. R. Soc. Lond. Ser. B Biol. Sci. 249, 195–197. doi: 10.1098/rspb.1992.0103
Heffelfinger, L. J., Stewart, K. M., Bush, A. P., Sedinger, J. S., Darby, N. W., and Bleich, V. C. (2018). Timing of precipitation in an arid environment: effects on population performance of a large herbivore. Ecol. Evol. 8, 3354–3366. doi: 10.1002/ece3.3718
Hewison, A. M., and Gaillard, J.-M. (1999). Successful sons or advantaged daughters? The Trivers–Willard model and sex-biased maternal investment in ungulates. Trends Ecol. Evol. 14, 229–234. doi: 10.1016/S0169-5347(99)01592-X
Hudson, P., and Browman, L. G. (1959). Embryonic and fetal development of the mule deer. J. Wildl. Manag. 23, 295–304. doi: 10.2307/3796888
Johnson, B. K., Jackson, D. H., Cook, R. C., Clark, D. A., Coe, P. K., Cook, J. G., et al. (2019). Roles of maternal condition and predation in survival of juvenile Elk in Oregon. Wild. Mon. 201, 3–60. doi: 10.1002/wmon.1039
Keech, M. A., Bowyer, R. T., Jay, M., Hoef, V., Boertje, R. D., Dale, B. W., et al. (2000). Life-history consequences of maternal condition in Alaskan moose. J. Wildl. Manag. 64, 450–462. doi: 10.2307/3803243
Kirkpatrick, M., and Lande, R. (1989). The evolution of maternal characters. Evolution 43, 485–503. doi: 10.2307/2409054
Kojola, I. (1993). Early maternal investment and growth in reindeer. Can. J. Zool. 71, 753–758. doi: 10.1139/z93-099
Krausman, P. R., Hervert, J. J., and Ordway, L. L. (1985). Capturing deer and mountain sheep with a net-gun. Wildl. Soc. Bull. 13, 71–73.
Lomas, L. A., and Bender, L. C. (2007). Survival and cause-specific mortality of neonatal mule deer fawns, north-Central New Mexico. J. Wildl. Manag. 71, 884–894. doi: 10.2193/2006-203
Marshall, D. J., and Uller, T. (2007). When is a maternal effect adaptive? Oikos 116, 1957–1963. doi: 10.1111/j.2007.0030-1299.16203.x
Monteith, K. L., Bleich, V. C., Stephenson, T. R., Pierce, B. M., Conner, M. M., Kie, J. G., et al. (2014). Life-history characteristics of mule deer: effects of nutrition in a variable environment. Wildl. Monogr. 186, 1–62. doi: 10.1002/wmon.1011
Monteith, K. L., Schmitz, L. E., Jenks, J. A., Delger, J. A., and Bowyer, R. T. (2009). Growth of male white-tailed deer: consequences of maternal effects. J. Mammal. 90, 651–660. doi: 10.1644/08-MAMM-A-191R1.1
Murray, D. L. (2002). Differential body condition and vulnerability to predation in snowshoe hares. J. Anim. Ecol. 71, 614–625. doi: 10.1046/j.1365-2656.2002.00632.x
Noyes, J. H., Johnson, B. K., Dick, B. L., and Kie, J. G. (2002). Effects of male age and female nutritional condition on elk reproduction. J. Wildl. Manag. 66, 1301–1307. doi: 10.2307/3802963
Robbins, C. T., and Robbins, B. L. (1979). Fetal and neonatal growth patterns and maternal reproductive effort in ungulates and subungulates. Am. Nat. 114, 101–116. doi: 10.1086/283456
Robinette, W. L., Baer, C. H., Pillmore, R. E., and Knittle, C. E. (1973). Effects of nutritional change on captive mule deer. J. Wildl. Manag. 37, 312–326. doi: 10.2307/3800121
Robinette, W. L., Jones, D. A., Rogers, G., and Gashwiler, J. S. (1957). Notes on tooth development and wear for Rocky Mountain mule deer. J. Wildl. Manag. 21, 134–153. doi: 10.2307/3797579
Sams, M. G., Lochmiller, R. L., Hellgren, E. C., Warde, W. D., and Varner, L. W. (1996). Morphometric predictors of neonatal age for white-tailed deer. Wildl. Soc. Bull. 24, 53–57.
Severinghaus, C. W. (1949). Tooth development and wear as criteria of age in white-tailed deer. J. Wildl. Manag. 13, 195–216. doi: 10.2307/3796089
Short, C. (1970). Morphological development and aging of mule and white-tailed deer fetuses. J. Wildl. Manag. 34, 383–388. doi: 10.2307/3799025
Sikes, R. S. (2016). Guidelines of the American Society of Mammalogists for the use of wild mammals in research and education. J. Mammal. 97, 663–688. doi: 10.1093/jmammal/gyw078
Smith, C. C., and Fretwell, S. D. (1974). The optimal balance between size and number of offspring. Am. Nat. 108, 499–506. doi: 10.1086/282929
Smith, R. B., and Lindzey, F. G. (1982). Use of ultrasound for detecting pregnancy in mule deer. J. Wildl. Manag. 46, 1089–1092. doi: 10.2307/3808248
Souza, L. L., Moura, E. G., and Lisboa, P. C. (2022). Litter size reduction as a model of overfeeding during lactation and its consequences for the development of metabolic diseases in the offspring. Nutrients 14:2045. doi: 10.3390/nu14102045
Stonehouse, K. F., Anderson, C. R., Peterson, M. E., and Collins, D. R. (2016). Approaches to Field Investigations of Cause-specific Mortality in Mule Deer (Odocoileus hemionus), Fort Collins, CO, Colorado Parks and Wildlife.
Therneau, T. (2015). A Package for Survival Analysis in S. version 2.38. Available at: https://CRAN.R-project.org/package=survival.
Tollefson, T. N., Shipley, L. A., Myers, W. L., and Dasgupta, N. (2011). Forage quality's influence on mule deer fawns. J. Wildl. Manag. 75, 919–928. doi: 10.1002/jwmg.113
Tollefson, T. N., Shipley, L. A., Myers, W. L., Keisler, D. H., and Dasgupta, N. (2010). Influence of summer and autumn nutrition on body condition and reproduction in lactating mule deer. J. Wildl. Manag. 74, 974–986. doi: 10.2193/2008-529
Wauters, L. A., De Crombrugghe, S. A., Nour, N., and Matthysen, E. (1995). Do female roe deer in good condition produce more sons than daughters. Behav. Ecol. Sociobiol. 37, 189–193. doi: 10.1007/BF00176716
White, M. (1973). Description of remains of deer fawns killed by coyotes. J. Mammal. 54, 291–293. doi: 10.2307/1378900
White, G. C., and Bartmann, R. M. (1994). Drop nets versus helicopter net guns for capturing mule deer fawns. Wildl. Soc. Bull. 22, 248–252.
Wickham, H. (2016). Programming with ggplot2. Ggplot2: Elegant Graphics for Data Analysis. Berlin: Springer Science & Business Media, 241–253.
Keywords: maternal condition, mule deer, Odocoileus hemionus, recruitment, neonate survival, birthweight
Citation: Lamb S, McMillan BR, van de Kerk M, Frandsen PB, Hersey KR and Larsen RT (2023) From conception to recruitment: Nutritional condition of the dam dictates the likelihood of success in a temperate ungulate. Front. Ecol. Evol. 11:1090116. doi: 10.3389/fevo.2023.1090116
Edited by:
Vernon Bleich, University of Nevada, Reno, United StatesReviewed by:
Darren Clark, Oregon Department of Fish and Wildlife, United StatesRhiannon Jakopak, University of Wyoming, United States
Copyright © 2023 Lamb, McMillan, van de Kerk, Frandsen, Hersey and Larsen. This is an open-access article distributed under the terms of the Creative Commons Attribution License (CC BY). The use, distribution or reproduction in other forums is permitted, provided the original author(s) and the copyright owner(s) are credited and that the original publication in this journal is cited, in accordance with accepted academic practice. No use, distribution or reproduction is permitted which does not comply with these terms.
*Correspondence: Brock R. McMillan, YnJvY2tfbWNtaWxsYW5AYnl1LmVkdQ==