- 1ICAR-Indian Institute of Soil and Water Conservation, RC, Vasad, Gujarat, India
- 2ICAR-Indian Institute of Soil and Water Conservation, Dehradun, Uttarakhand, India
- 3ICAR-Central Soil Salinity Research Institute, Karnal, Haryana, India
- 4ICAR-Central Coastal Agricultural Research Institute, Old Goa, India
- 5ICAR-Central Arid Zone Research Institute, Jodhpur, Rajasthan, India
- 6ICAR-Central Soil Salinity Research Institute, RRS, Bharuch, Gujarat, India
- 7ICAR-National Institute of Abiotic Stress Management, Pune, India
- 8Veer Chandra Singh Garhwali Uttarakhand University of Horticulture and Forestry, College of Forestry, Tehri, Uttarakhand, India
- 9ICAR-Indian Institute of Soil and Water Conservation, RC, Datia, Madhya Pradesh, India
- 10ICAR-Indian Institute of Soil and Water Conservation, RC, Udhagamandalam, Tamil Nadu, India
- 11Assam University, Silchar, Assam, India
- 12Agriculture University, Jodhpur, India
- 13Rajmata Vijyaraje Scindia Krishi Vishva Vidhyalaya, Gwalior, Madhya Pradesh, India
- 14Sri Karan Narendra Agriculture University, Jaipur, Rajasthan, India
Land degradation is one of the most important factors responsible for the alarming situation of food security, human health, and socioeconomic development in the country. Currently, 120.7 M ha of land in the country is affected by land degradation, out of which 85.7 M ha of land is affected by soil erosion caused by water and wind. Moreover, physical, chemical, and biological degradation are the major forms of land degradation in the country. Deforestation or tree cover loss (2.07 M ha) from 2001 to 2021, intensive rainfall (>7.5 mm ha−1), uncontrolled grazing (5.65 M ha), indiscriminate use of fertilizers (32 MT year−1), and shifting cultivation (7.6 M ha) are other major factors that further aggravate the process of land degradation. In order to alleviate the problem of land degradation, numerous agroforestry technologies have been developed after years of research in different agroclimatic zones of the country. The major agroforestry systems observed in the country are agri-horticulture, silvipasture, and agri-silviculture. This review indicates the potential of agroforestry in enhancing carbon sequestration (1.80 Mg C ha−1 year−1 in the Western Himalayan region to 3.50 Mg C ha−1 year−1 in the island regions) and reduced soil loss and runoff by 94% and 78%, respectively, in Northeast India. This can be concluded that the adoption of the agroforestry system is imperative for the rehabilitation of degraded lands and also found to have enough potential to address the issues of food, environmental, and livelihood security. This review’s findings will benefit researchers, land managers, and decision-makers in understanding the role of agroforestry in combating land degradation to enhance ecosystem service in India and planning suitable policies for eradicating the problem effectively.
Introduction
Land resources are the basis for human livelihood and societal development (Xie et al., 2020). Since the 20th century, land degradation has escalated and aggravated due to ecological degradation, increased food demand of a burgeoning population, rapid urbanization, industrialization, and indiscriminate use of land resources (Hammad and Tumeizi, 2012). Approximately 60% of the world’s land area is regarded as degraded, which is considered one of the most important challenges to bringing them to sustainable use (Pimentel, 2006). It has been reported that accelerated land degradation is a major threat to soil, and ~24 billion tonnes (BT) of fertile soil is lost annually only through water erosion, and 75 BT of soil is eroded annually from arable lands globally, which resulted in the estimated financial loss of US $400 billion per year (GSP. Global Soil Partnership, 2017). In India, ~120.7 M ha of land suffers from various forms of degradation (NAAS, 2010). The primary process of land degradation is soil erosion (due to water and wind erosion), contributing ~71% of (85.7 M ha) degraded land in the country (Table 1). Water-induced soil erosion alone contributes ~60.7% (73.3 M ha), while wind erosion contributes 10.3% (12.4 M ha). The other processes include the problem of chemical (salinity, alkalinity, and acidity) and physical degradation (water logging and mining) also contribute significantly to the degradation of land resources. Deforestation or tree cover loss (2.07 M ha) from 2001 to 2021 (Global Forest Watch, 2022), intensive rainfall (>7.5 mm ha-1), uncontrolled grazing in 5.65 M ha (Down To Earth, 2019), indiscriminate use (32 MT year-1) of fertilizers (Fertilizer Association of India, 2021), and shifting cultivation in 7.6 M ha (Bhat et al., 2022) are other major factors that further aggravate the process of land degradation. According to SAC (2021), at present, 97.8 M ha (29.7%) of the area is degraded in the country. The states of Rajasthan, Maharashtra, Gujarat, Karnataka, Ladakh Union Territory, Jharkhand, Odisha, Madhya Pradesh, and Telangana have the highest area under such lands (23.7% of TGA), contributing about two-thirds of the total degraded area of the country.
Degraded lands are poor in organic matter and soil nutrients, which consequently do not support any kind of vegetation. In the absence of any management or conservation measures, these become irreversible and unproductive resulting in serious ecological and socioeconomic consequences. Rehabilitation of degraded lands is, therefore, one of the most viable options for improving land productivity. These degraded lands can be ameliorated by practicing agronomical, mechanical, and biological measures (Chaturvedi et al., 2018; Jinger and Kakade, 2019; Kumar et al., 2020). Biological measures which involve the growing of trees, shrubs, and crops/herbs are simple, cost-effective, and can help relieve the pressure on traditional cultivated lands and forests. Among different biological measures, agroforestry is considered ideal in terms of enhancing productivity and providing ecological and economic security. Furthermore, the agroforestry system also provides numerous ecosystem services (provisioning, regulating, supporting, and cultural), which directly or indirectly help in land restoration (Paramesh et al., 2019; Jinger et al., 2022b). India in COP 27 emphasized joining the LiFE movement—Lifestyle for Environment, a pro-people and pro-planet effort that seeks to shift the world from mindless and wasteful consumption to mindful and deliberate utilization of natural resources. Furthermore, India aimed to continue its battle against all global environmental challenges in the appeal to defend humanity’s planetary home. India is dedicated to both domestic action and multilateral collaboration on climate change. However, global warming also issues a warning that success depends on equity and global cooperation, where the privileged must take the lead. To achieve a pollution-free environment, the adoption of climate-resilient practices is imperative. Agroforestry is one such potential eco-friendly system having huge scope to restore degraded land, fix atmospheric carbon, and also to enhance soil quality by reducing soil erosion.
With this background, we hypothesized that agroforestry is beneficial in the rehabilitation of degraded land and enhances the ecosystem services to improve the livelihood security of farm families for achieving sustainability. Considering the importance of the agroforestry system in carbon sequestration, biomass production, food production, industrial value, economics, and employment generation to the farm family, an attempt has been made to collect the published research outputs and perform a detailed review of the same. The objective of the study was to know the importance of agroforestry in improving carbon sequestration, biomass production, food production, economics, and soil fertility enhancement. The review is extended to collect information on degraded lands in India and its causes. Furthermore, this chapter deals with agroforestry solutions for different kinds of degraded lands in India.
Literature search and data collection
We systematically searched for scientific literature using the following search terms in Google Scholar: “Agroforestry system AND Carbon sequestration AND Erosion control Food Production AND Land rehabilitation,” of which the first 102 results were selected. Studies were selected if they included the agroforestry system and any of the keywords searched. We collected further records from the reference lists of review articles and research articles meeting the initial eligibility criteria. Targeted searches of governmental and independent agricultural research organizations were also performed in countries where medium to large-scale, commercially oriented agroforestry systems are known to occur.
• The study scope was extended to all the agroforestry systems such as agro-pastoral systems, agro-silvo-pastoral systems, agri-horti, silvipasture, and silvi-horti;
• Studies involving different land holding sizes were also considered;
• Both on-farm (farmer’s field) and on-station (research station) trails were considered; and
• The study was original research, dataset, or dissertation, i.e., not a review, book chapter, or conference proceeding also considered.
Land degradation mechanism
Land degradation refers to the temporary or permanent decline in the biological productivity of land due to anthropogenic activities (United Nations Environment Programme, 1993; Xie et al., 2020). Land degradation also includes wind erosion and water erosion, resulting in the loss of soil material and long-term loss of natural vegetation (Warren, 2002). As a result, disasters such as floods and droughts are exacerbated, which seriously threatens the survival and development of humankind (Guo et al., 2018). Land degradation is the systematic decline in the quality of the land resulting from a mismatch between land use and land quality. It is the consequence of different natural processes, but it is accelerated by human activities. The result in declining functions (Chalise et al., 2019). The land undergoing degradation normally passes through three phases, viz. (1) Natural degradation is generally slow because a steady state develops between soil formation and soil degradation. Natural degradation represents inherent land quality, (2) induced degradation results from inappropriate land-use management. Soils decline in quality, but productivity can be maintained by applying artificial nutrients and by appropriate soil management. Induced degradation happens more quickly than natural degradation, and (3) desertification occurs when the degree of degradation is such that the resilience of the land is impaired. In unmanaged systems, desertification is indicated by changes in the quality and quantity of biomass and biota (Singh D. V. et al., 2017; Singh C. et al., 2017).
Types of land degradation
Mechanisms that initiate land degradation include physical, chemical, and biological processes. Important among physical processes is a decline in soil structure, leading to crusting, compaction, erosion, desertification, anaerobism, environmental pollution, and the unsustainable use of natural resources. Significant chemical processes include acidification, leaching, salinization, decrease in cation retention capacity, and fertility depletion. Biological processes include a reduction in total and biomass carbon and a decline in land biodiversity. The latter comprises important concerns related to the eutrophication of surface water, contamination of groundwater, and emissions of trace gases (CO2, CH4, N2O, and NOx) from terrestrial/aquatic ecosystems to the atmosphere. Soil structure is an important property that affects all three degradative processes (Lal and Steward, 1990) (Figure 1).
Factor affecting land degradation
The sustainability of natural resources is under serious threat due to indiscriminate cutting of trees, conversion of forest land into agriculture, shifting cultivation, exploitation of fragile and marginal lands, faulty management practices, and reduction in the frequency of fallowing and excessive use of chemicals (Sharda and Juyal, 2016). The problem is further compounded by over-exploitation of sweet ground water aquifers, ingress of sea water in the coastal ecosystem, floods, and droughts in different parts of the country (Table 2).
Agroforestry
Woody perennials (trees, shrubs, palms, bamboo, etc.) are intentionally used on the same land management units as annual crops and/or animals in some kind of spatial arrangement or temporal sequence, and these systems and technologies are together referred to as agroforestry. The various components of agroforestry systems must interact both ecologically and economically (Lundgren and Raintree, 1982). Agroforestry has a great deal of promise to supply the demand for fuel, fodder, timber, medicine, and other non-woody forest products while boosting green cover, easing the strain on forests, and reducing greenhouse gas emissions. Agroforestry provides a practical way to prevent hazards brought on by weather anomalies, manage soil erosion, and guarantee long-term sustainable output. Central Agroforestry Research Institute, Jhansi, recently reported a 26.3 m ha area under agroforestry in India (Arunachalam et al., 2021).
Agroforestry practices in India
Indian traditional agroforestry systems range from seemingly straightforward forms of shifting cultivation to intricate home gardens, from systems involving sparse stands of trees on farmlands to high-density complex multi-story homesteads of humid lowlands, and from systems in which trees primarily serve as a “service” to those in which they are the primary source of commercial good. The majority of them are anecdotal, but some of them have received enough research in recent years (GOI, 2001; Pathak et al., 2006). The foundation of the All India Coordinated Research Project on Agroforestry (AICRPAF) by the Indian Council of Agricultural Research (ICAR) at 20 centers in 1983 and the subsequent construction of the National Research Centre for Agroforestry at Jhansi in 1988 marked the beginning of systematic agroforestry research in the nation. There are currently 37 agroforestry centers operating throughout the nation. Agroforestry research was also started by the Indian Council of Forestry Research and Education (ICFRE) in various regions of the nation. Agroforestry research was also started by numerous business corporations limited companies, including ITC (Indian Tobacco Company Limited), WIMCO (Western India Match Company), West Coast Paper Mills Ltd., Hindustan Paper Mills Ltd., and others, with a focus on the production of improved planting material of the fast-growing species to meet their demand for raw materials (Dhyani et al., 2015). A diagnosis and design exercise for current agroforestry methods in India has been carried out through ICAR centers. The survey revealed agri-silviculture as the most prominent agroforestry system in seven agroclimatic regions, followed by agri-horticulture in six agroclimatic regions, and agri-hortisilviculture and silvi-pastoral systems in two agroclimatic regions.
Types of agroforestry system: Based on structure, agroforestry can be grouped into agri-silviculture, agri-horticulture, agri-silvi-horticulture, silvi-pasture, horti-pasture, and agri-silvi-pasture system. The agro-climatic zone-wise agroforestry system has been mentioned in Table 3. Some of the most prevalent agroforestry systems in India have been discussed below.
Agri-silviculture system: In this system, crops (cereals, pulses, oilseeds, vegetables, and aromatic plants) are grown with tree crops concurrently on the same piece of land. It is the most prominent agroforestry system in India. Tree components give fodder, fuel, or timber, including green-leaf manure. It is ideal for class IV soils of drylands with an annual rainfall of ~750 mm. Hedgerow intercropping (alley cropping) is a classic example of the agri-silviculture system. Wind breaks and shelterbelts also come under the agri-silviculture system. Cultivation of wheat + poplar is a prominent agroforestry system in the Indo-Gangetic plains of India (Chavan et al., 2022).
Agri-horticulture system: In this system tree component is a fruit tree. It is also called as food cum fruit system. In this system, short-duration arable crops are grown in the interspaces of fruit trees. Some of the fruit trees that can be considered are guava, pomegranate, custard apple, sapota, and mango. Pulses are the important arable crops for this system. This is the second most widely practiced agroforestry system in India. For example, the cultivation of cowpea + sapota is one kind of agri-horticulture system (Jinger et al., 2022a).
Agri-silvi-horticulture system: It is the integration of agri-silviculture and the horti-silviculture system. Integration of horticultural plant species provides regular income to the farmers in addition to the production from crops during the early stages of tree establishment, whereas silviculture species provide income in the later stage sustaining long-term productivity (Kaushik et al., 2014). The system has been advocated to arrest degradation and increase the fertility status of soil and enhance yields. Productivity in the agri-silvi-horticultural system is comparatively higher than the productivity of sole agriculture (Kaushik et al., 2017).
Silvi-pasture system: The production of woody plants combined with grasses is referred to silvipasture system. This system is primarily meant for augmenting the scarce fodder supply to livestock, or it may be practiced for timber, fuel wood, and improving the soil. In marginal lands (class IV onwards), this system enhances sustainability through resource conservation and its efficient use, improvement in soil health, and by linking agriculture with cattle. Cultivation of Leucaena leucocephala and Gliricidia sepium-based silvi-pasture system is most prevalent in India (Chauhan et al., 2014).
Horti-pasture system: In this system, fruit trees are grown with fodder grasses concurrently on the same piece of land. Guava, aonla, custard apple, ber suits well in the horti-pasture system with grasses such as Cenchrus ciliaris, Cenchrus setigerus, Panicum antidotale, Dicanthium annulatum, Chloris gayana, and legumes such as Stylosanthes hamata, Stylosanthes scarab, and Macroptilum artopurpuream. Cultivation of aonla + Cenchrus ciliaris is widely practiced horti-pasture systems in arid and semiarid regions of India (Kumar et al., 2015).
Agri-silvi-pasture system: In this system, crops and grasses are intercropped with woody components on the same piece of land. This system is practiced for food production and soil conservation and provides fodder and fuel. This system is recommended for highland humid tropics. It may be a tree-livestock crop mix around a homestead, wood-hedgerow for browsing, green-leaf manure, and soil conservation (Rao et al., 2018).
Agroforestry nexus land rehabilitation
Agroforestry can be considered a versatile panacea for multiple problems being faced by the agriculture sector. Agroforestry has huge potential to mitigate the impacts of climate change by sequestering atmospheric carbon in their biomass and soil. The average carbon sequestered by agroforestry practices has been estimated to be 9, 21, 50, and 63 Mg C ha−1 in semiarid, sub-humid, humid, and temperate regions (Murthy et al., 2013). Moreover, a dense canopy spread in an agroforestry system reduces the intensity of rainfall by reducing the kinetic energy of raindrops. Thereafter, water moves very slowly from the tree-crop (agroforestry) structure to the soil. Hence, runoff velocity is reduced, and the soil gets more opportunity time to absorb the water. Thus, ultimately soil erosion is reduced (Figure 2). Furthermore, the agroforestry system has tremendous potential to produce a huge quantity of leaf litter which also acts as mulch and also forms humus after decomposition and improves soil’s physical, chemical, and biological properties (Jama et al., 2006). Soil improvement in agroforestry systems is linked to biological nitrogen fixation, recycling of nutrients from deeper layers to the surface soil, building up soil organic matter (SOM) from aboveground and below-ground parts of plants, increasing soil microbial activity, improving soil enzyme activity, and enhancing the activity of arbuscular mycorrhizal fungi (Dollinger and Jose, 2018). The different direct and indirect benefits received from agroforestry has mentioned in Figure 3. Moreover, the carbon sequestration potential and food production potential of different agroforestry systems in agro-climatic zones of India have been mentioned in Table 4.
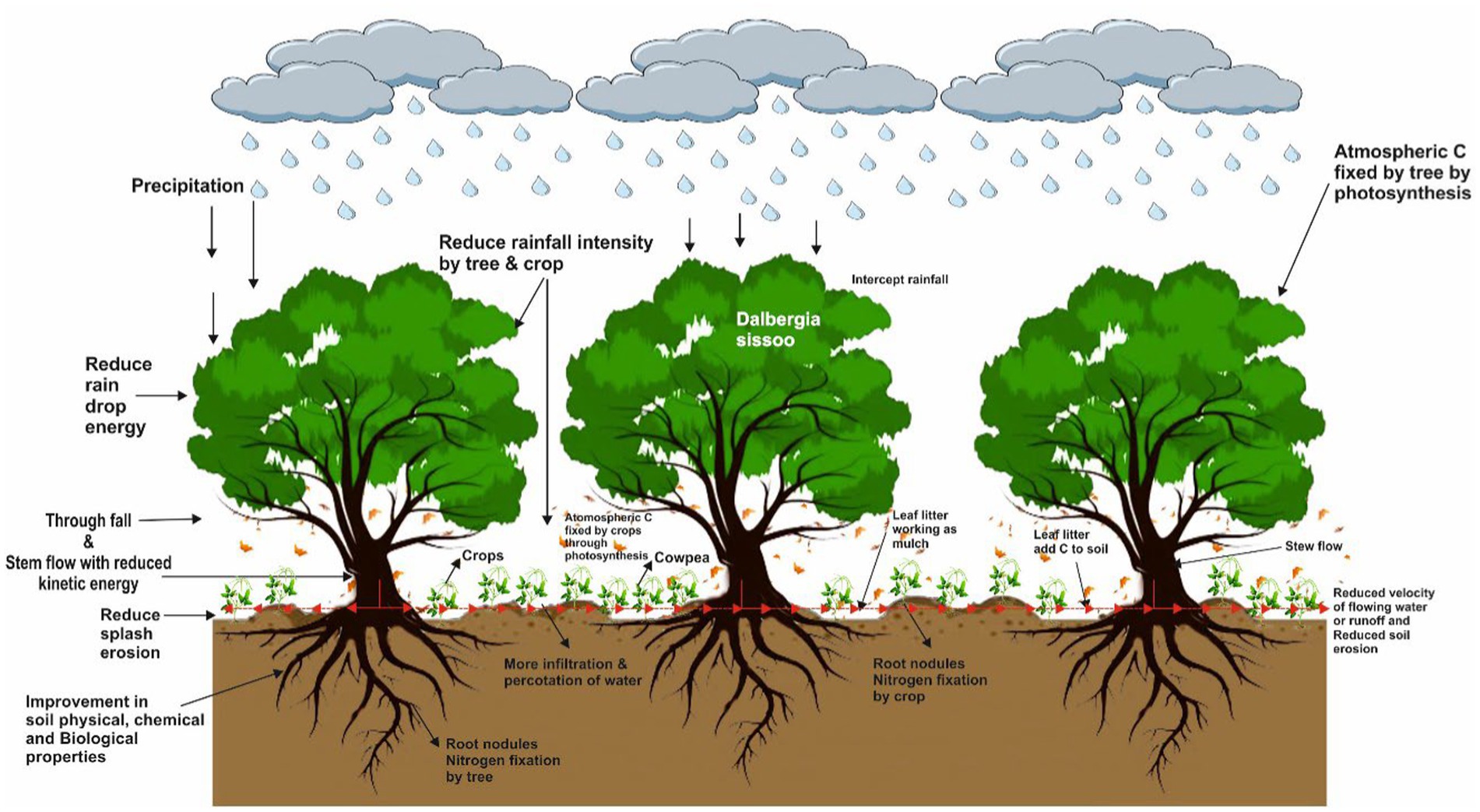
Figure 2. Agroforestry system and its impact on carbon sequestration, soil erosion, and soil fertility.
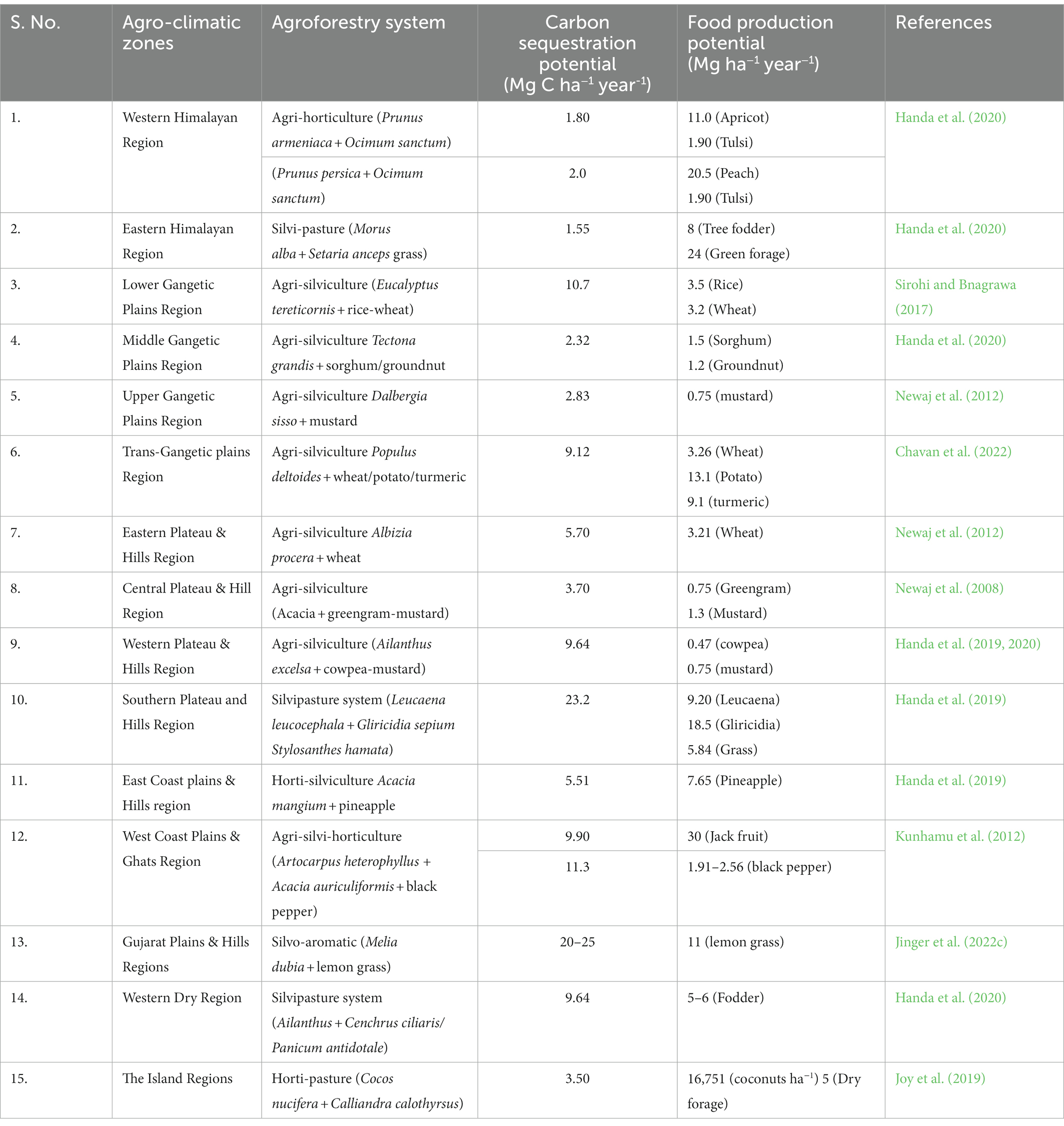
Table 4. Carbon sequestration and food production potential different most prevalent agroforestry systems in agro-climatic zones of India.
Agroforestry for rehabilitation of degraded lands
By 2050, India wants to have 53 M ha under agroforestry, which will be accomplished by reclaiming fallows, cultivable fallows, pastures, groves, and problematic soils (Dhyani and Handa, 2013). In India, agroforestry practices are an important component in the various river rejuvenation programs like Namami Gange, Green India Mission, National Highway Mission, Pradhan Mantri Krishi Sinchayee Yojana (PMKSY), and Mahatma Gandhi National Rural Employment Guarantee Act (MNREGA). Several research Institutes like the Indian Council of Agricultural Research (ICAR), Forest Research Institute (FRI), Council of Scientific and Industrial Research (CSIR), State Agricultural Universities (SAUs), and Krishi Vigyan Kendra (KVKs) have developed cost-effective agroforestry technologies for the rehabilitation of degraded lands. ICAR-CAFRI and AICRPAF published a summary on Agroforestry technologies for different agroclimatic zones of Country, whereas it consists of 84 grassroots levels farmers adopted technologies developed by 26 AICRPAF centers from five different agroclimatic regions of the country (Chaturvedi et al., 2016). FRI has also come up with various river rejuvenation technologies for the rehabilitation of basin areas. The different agroforestry technologies for lands affected by soil erosion (water and wind), physical (mining and industrial waste and waterlogged lands), chemical (saline, sodic, and acidic soils), and biologically degraded lands due to depletion of SOM, reduction in soil fauna, and emission of green house gases (GHGs) are discussed below:
Water eroded lands
One of the main reasons for the degradation of the land is water-induced soil erosion. A moderate (>10 Mg ha−1 year−1) to extremely severe (>80 Mg ha−1 year−1) intensity of water erosion affects ~68.4% (83 M ha) of the total degraded land (120.7 M ha) in India. The main danger to soil quality posed by runoff water is water erosion. Loss of organic carbon, nitrogen imbalance, compaction, a decrease in soil biodiversity, and pesticide and heavy metal contamination are the results. The lands affected by water erosion can be categorized into three categories: (a) degraded sloping lands, (b) gully and ravine lands, and (c) shifting cultivation lands.
The importance of trees in controlling soil erosion is widely accepted. In agroforestry, tree canopy checks soil erosion mainly by intercepting rainfall, thereby reducing the impact of raindrops and decreasing their erosive capacity (Kaushal et al., 2017). Litter helps in producing water-stable aggregates, thereby reducing the surface runoff volume. Roots and stems restrict sediments from moving down the slope and help water infiltrate. Shallow landslides are prevented by deep tree roots that stabilize slopes. Vegetation affects water and sediment fluxes over the long run by boosting soil water infiltration and soil aggregate stability and cohesiveness (Zuazo and Pleguezuelo, 2008). The most widely utilized agroforestry techniques for erosion management include windbreaks and shelterbelts, hedgerow (alley) cropping, multilayer tree gardens, home gardens, plantation crop combinations, and multilayer tree gardens (Young, 1997). According to McDonald et al. (2002), agroforestry reduced soil erosion by 21 times and surface runoff by seven times compared to control soils. The retention, infiltration, and storage of rainfall-induced overland flow are improved by adding tree leaf litter, its subsequent decomposition in the soil, and the activities of trees’ roots (Dass et al., 2011). The different agroforestry models/technologies developed for lands affected by soil erosion are as below.
Degraded sloping lands
In addition, mudslides, landslides, and other gravity erosion processes are more likely to occur in steeper terrain. Longer, steeper slopes are more prone to erosion during heavy rains than shorter, less steep slopes because they lack sufficient plant cover. Various agroforestry models have been developed and evaluated for water-eroded lands in the northwestern and northeastern Himalayan regions (Kaushal et al., 2021a). The best filter strips to stop erosion and boost agricultural output in marginal lands are vegetative barriers made of hedgerows of trees such as Leucaena and Gliricidia and grasses. In various land-use regimes, hedgerows are very helpful in preventing the loss of nutrients and organic carbon during the erosion processes. Hedgerows’ blockage caused soil and nutrients to accumulate close to the biological barrier system. According to Lenka et al. (2012), grass filter strips and hedgerows (Indigofera) have a great capacity to conserve soil organic carbon (SOC) (43%), as well as accessible nitrogen (56%), phosphorus (54%), and potassium (48%) in the soil. According to Hombegowda et al. (2020), under Gliricidia and Leucaena-based hedgerows, respectively, the configuration of the land slope reduced by 0.41 and 0.27 degrees year−1, showing the deposition of eroded soils on the lower slope side and the consequent decrease in the land slope.
On degraded slopes, silvipasture systems have proven effective (Chaturvedi et al., 2014). In the Shiwalik foothills, Eucalyptus tereticornis and Eulaliopsis binata (@ 2,500 trees ha−1) were grown in paired rows with understorey grass planted at 50-cm × 50-cm spacing. This method prevented soil loss and produced an annual return of approximately Rs. 4,000 (50 USD) ha−1 year−1 from commercial grass alone, in addition to returns from the Eucalyptus, which made the crop more profitable (Sharda and Venkateswarlu, 2007). When combined with Chrysopogon fulvus (gorda) and Eulaliopsis binate (bhabar) in the Doon Valley, the plant’s Albizia lebbek (siris), Grewia optiva (bhimal), Bauhinia purpurea (kachnar), and Leucaena leucocephala (subabul) were found to be promising. The average yearly production of savanna pasture systems is 8–10 Mg ha−1, consisting of 4.5–5.0 Mg ha−1 of grass-based fodder, 1.5–2.0 Mg ha−1 of leaf fodder from tree loppings, and 2.0–2.5 Mg ha−1 of fuelwood from the lopped branches (Raizada and Singh, 2010).
On degraded sloping lands, the plantation of trees, along with trenching, is useful for improving the survival and growth of plantations. The trenches effectively stop soil erosion and improve the soil moisture regime by breaking the slope and lowering the surface runoff velocity. According to Kaushal et al. (2021a), semicircular ditches and Dendrocalamus. hamiltonii species can be a successful land restoration approach on degraded sloping soils in the Himalayan foothills. Comparing the semicircular trenches to the control treatment, the soil moisture was 16% greater (without trenches). Runoff and soil loss were drastically reduced with bamboo + trenching treatment. After the 5th year of the plantation, no runoff or soil loss was seen, demonstrating the effectiveness of bamboo and in situ water-conserving techniques in preventing soil erosion. In the Eastern Ghats, the Gliricidia + Trench and Leucaena + Trench planting systems, respectively, boosted the efficiency of SOC and accessible NPK by 44%, 63%, 56%, and 33%, 46%, 44%, 42% (Hombegowda et al., 2020). According to Satapathy (2005), mixed land-use schemes that included bench terraces and contour trenches effectively retained 90%–100% of the yearly rainfall and mimicked the impacts of a natural forest in the northeastern region. Base flow accounted for 70%–90% of the water yields in watersheds with continuous stream flow characteristics. The watershed that had been subjected to jhum (shifting) cultivation produced the greatest peak runoff, whereas the watershed that had been left to its natural vegetation produced the least peak runoff.
In the Himalayan area, agri-horticulture is the most significant system in terms of productivity, financial rewards to farmers, and preferences on sloping slopes. As an economically feasible strategy for rehabilitating marginal lands in the Shiwalik region, intercropping of guar, cowpea, or pearl millet with peach, turmeric with papaya, Chrysopogon fulvus or Pennisetum purpureum (Napier grass) with aonla or ber, has been discovered. With pigeon peas, Aonla produced the largest production of 86-kg fruits tree−1; however, the output was decreased by 17% and 23% with Chrysopogon and Napier grass, respectively (Sharda and Venkateswarlu, 2007). Runoff was 8.0%, 13.1%, and 18.6% for aonla + Chrysopogon fulvus, aonla + hybrid Napier, and aonla + perennial pigeon pea, respectively, over control during a period of 10 years (pure aonla). Chrysopogon, Napier, and pigeon pea were the most successful intercrops at reducing soil loss, with reductions of 81, 56, and 25% over sole aonla. By contrast to runoff, less sediment was lost during the post-bearing phases (Yadav et al., 2005). The cowpea-toria sequence was shown to be very profitable, with a gross revenue of Rs. 16,850 ha−1 (210 USD), and successful in preserving soil and water, according to an analysis of mango-based agri-horticulture systems (Rathore et al., 2012).
Gully and ravine lands
In dry and semi-arid locations, erosion by water is a serious issue that results in gullies and ravines. The use of gullies by land capacity classes, soil and water conservation measures, and permanent plant cover through afforestation or agroforestry systems are all necessary for the revival of ravine lands (Chaturvedi et al., 2014). The newly planted trees reduce soil and nutrient loss from these lands while providing risk protection against the uncertainties of agricultural production in the tough circumstances of ravine regions (Soni et al., 2018). In the ravine region, the silvi-pastoral system has been proven to be quite successful. Several significant grass species are useful for enhancing the fodder availability in the ravine regions, including Pennisetum purpureum, Brachiaria mutica, Cenchrus ciliaris, Cenchrus setigerus, Panicum antidotale, and Panicum maximum (Chaturvedi et al., 2011). Plantations of Acacia nilotica and Acacia tortilis with Cenchrus ciliaris produced 28.7 Mg ha−1 and 27 Mg ha−1 of fuel wood, respectively, at a spacing of 3 m × 3 m on the top, slopes, and bottom of ravines. Under Acacia nilotica and Acacia tortilis, the mean annual pasturage yield varied from 1.52 Mg ha−1 year−1 to 2.06 Mg ha−1 year−1, respectively (Kurothe et al., 2018).
For ravine areas, fruit plants that can endure moisture stress are appropriate. In humps and gully beds, fruit trees, including lemon, mango, ber, and aonla are planted alongside agricultural products. Agroforestry and soil water conservation practices, according to Kumar et al. (2019), boosted the carbon storage and sequestration capacity in semi-arid, climate change-vulnerable ravine landscapes while also increasing agro-ecosystem resilience to harsh weather. According to Kumar et al. (2020), a sapota-based agri-horticulture system with bench terraces and trenches reduced runoff by 16%–34% and soil loss by 15%–25% when compared to sapota on the slope. In a similar vein, Jinger et al. (2022a) found that using an agroforestry system with cowpea, castor, and sapota reduced soil loss and runoff overall by 37.7% and 19.1%, respectively, when compared to using only one crop. The agroforestry system, which boosted system production by 162% and 81.9%, respectively, above the sole crop and sole tree plantation, yielded the highest system productivity.
Dendrocalamus strictus, a bamboo species, holds great promise for preserving soil and maximizing the use of gullies and ravine areas for agricultural purposes (Rao et al., 2012). Bamboo roots effectively increase infiltration, decrease runoff, and safeguard soil from additional gully bed expansion (Singh et al., 2015; Kaushal et al., 2020, 2021b). A silvopasture system based on Anjan (Cenchrus ciliaris) and bamboo (D. strictus) grass has been created to increase the productivity of ravines. More than 80% of rainwater can be absorbed by this system, which also reduces soil and nutrient losses by 90% and 70%, respectively. These interventions gave an average annual net return varying from USD 814 to Rs. 1,130 ha−1 and a cost–benefit ratio varying from 1.96 to 2.09. Melia dubia + dragon fruit and Melia dubia + lemon grass cultivation along with soil moisture conservation practices has resulted in better fruit yield of dragon fruit and biomass yield of Melia dubia and lemon grass compared to control besides conservation of soil and water in Mahi ravines of Central Gujarat (Jinger et al., 2020, 2021; Kakade et al., 2020).
Shifting cultivation lands
Approximately 7.60 M ha of shifting agriculture, or jhum cultivation, is performed in India’s eastern and northeastern areas (Bhat et al., 2022). Because of the diminished jhum cycle brought on by strain from a growing human population, significant soil erosion, low productivity, and loss of soil fertility, shifting farming has become unsustainable (Markose and Jayappa, 2016). Agroforestry systems with many stories and improved fallows are essential for rehabilitating regions damaged by shifting farming. Fast-growing, nitrogen-fixing trees are cultivated during the fallow period in an upgraded fallows system. Improved fallows improve soil qualities such as organic matter, greater aggregates stabilizing soil, higher infiltration rate, and carbon sequestration in addition to increasing crop productivity (Chirwa et al., 2004). According to reports, soil in planted fallows contains larger pores and more macropores due to better aggregation and channels formed by dead and decomposing roots (Nyamadzawo et al., 2008). With the aid of the region’s natural resources, suitable alternative land-use systems for agriculture, horticulture, forestry, and agroforestry have been developed with almost equivalent hydrological behavior under the natural system. For the general development of these places, an integrated farming system strategy comprising fruit and forest trees, arable crops, livestock, fisheries, and poultry with sufficient conservation measures for natural resources has been determined to be suitable. In addition to preserving and safeguarding the hill soils, agri-horticultural systems that combine the production of ginger with fruit trees like mandarin and guava be successful. Pineapple is commonly connected with multi-use trees that are arranged in paired rows on a hillside. According to Saha et al. (2012), agri-horti-silvi-pastoral farming systems and bench terrace farming may successfully control runoff and soil losses. In mixed land-use systems with soil water conservation features like bench terraces and contour trenches kept because these systems resemble natural forests, 90%–100% of the yearly rainfall has reportedly been found to be retained. According to some reports, contour hedgerow technology (bio-terracing) is more cost-effective than bench terraces built using the cut-and-fill method across slopes. Saha et al. (2005) found that multi-storied agroforestry systems (3.06) and silvi-horti-pastoral (3.07) had low erosion ratio values, indicating that these systems were best suited for conserving soil and water in the hilly habitat. When compared to traditional farmers’ practices of growing finger millet, a mixed plantation of Moringa oleifera, Gliricidia sepium, Zingiber officinale, and Cajanus cajan in East India reduced runoff and soil loss by 8.26% and 3.45 Mg ha−1, respectively, while increasing SOC, P, and K by 74%, 64%, and 66%, respectively. Drumstick pod output increased 24%–27% as a result of the multipurpose Gliricidia hedgerow approach (Jakhar et al., 2017). Table 5 lists the agroforestry systems for various degraded soils. To check runoff and soil losses, alley cropping (hedgerow intercropping) has also been recommended as an alternative to shifting cultivation. The hedge serves as a barrier for checking the movement of soil and water along the slope and contributes to soil conservation (Sharda and Mandal, 2018). Continuously soil deposition near hedges leads to the formation of biological terrace-like structures in the long term. Leucaena leucocephala and Cassia siamea are the most recommended species for alley-cropping systems in the northeastern region of India (Kaushal et al., 2021a). Improved animal-based and horticulture-based integrated farming system (IFS) models were found to reduce soil erosion (34%–48%) and loss of SOM (26%–51%), N (33%–45%), P (19%–54%), and K (27%–51%) compared to the traditional shifting cultivation system in Nagaland, India (Chatterjee et al., 2021).
Wind eroded lands
After water erosion and vegetation degradation, wind erosion is the third most contributing factor for land degradation in India, covering 18.19 M ha, i.e., ~6% of the total geographical area. The causes, impact, and control of wind erosion are summarized in Figure 2. In dry and semi-arid areas, such as the states of Rajasthan, Haryana, Gujarat, and Punjab, wind erosion can range from mild to severe. In addition, it is common in coastal locations with sandy soils predominating and in the chilly desert regions of Leh (Jammu & Kashmir), which are both in the far northwestern part of India (Singh D. V. et al., 2017; Singh C. et al., 2017). In drylands, erosion by wind is one of the principal processes associated with land degradation covering 33%–37% of the continental areas of the planet (Sivakumar et al., 1998) and is of major concern for policymakers and land managers (Duniway et al., 2019).
Agroforestry for wind-eroded lands
The most widely used agroforestry technologies to restore wind-eroded lands are stabilizing dunes, windbreaks, shelterbelts, alley-cropping, and silvopastoral systems (Figure 2). According to CAZRI (2015), covering the space beneath trees, providing a surface cover of grasses, and protecting them from biotic intervention are the most crucial steps in stabilizing sand dunes. On the windward side of the dune, small windbreaks are built in strips or chessboard patterns of 5 m each. Locally accessible brushwood species such as Leptadenia pyrotechnica (Khimp), Aerua tomentosa, Ziziphus nummularia (Pala), Crotalaria burhia (Sania), and Calligonum polygonides (Phog) are constructed upside down for the purpose of producing micro-wind barriers (Singh D. V. et al., 2017; Singh C. et al., 2017). Mulching is done in April and May to slow down the speed of the wind and stop sand from moving. To build the plants in the trench and create a dry hedge, sand is removed to a depth of 25 cm along the mulching line. Before the start of the rain, trees, bushes, and grasses are planted (Luna, 2006). After the rains have started, 1 × 1 m of grass is sown. During the monsoon, seeds of grasses and leguminous plants are sown on the side that receives micro-wind breaks and is mixed with clay and sodium arsenate. Many different types of grasses are employed, including Lasiurus sindicus, Panicum turgidum, P. antidotale, Saccharum munja, Cenchrus ciliaris, C. setigerus, Dichanthium annulatum, and Sachharum bengalense. The vegetation used to stabilize sand dunes is extremely drought resistant and has deep roots that may draw rainwater from shallower soil layers. The most effective combination of trees for stabilizing sand dunes has been determined to be Acacia tortilis, Acacia jacquimontii, Acacia leucophloea, Acacia senegal, Azadirachta indica, Balanites roxburghii, Prosopis cineraria, P. juliflora, and Holoptelia integrifolia (Singh D. V. et al., 2017; Singh C. et al., 2017). Sand dune stabilization technology helped in fixing up 0.4 M ha of sand dunes with the help of the Rajasthan state forest department (Harsh and Tewari, 1993).
Raising shelterbelts around the agricultural fields also minimizes wind hazards and increases farm productivity through the moderation of micro-climate (Prasad et al., 2009). The technology involves raising strips of vegetation composed of trees and local shrub wood material against the prevailing wind direction. Shelterbelt plantation helps fix the movement of sand from dunes and provides multiple products to farmers. It was reported that almost 84% of farmers have received the benefits of better groundwater availability and improved soil texture for the production of crops by raising shelterbelts (The Energy and Resources Institute, 2016). In the IGNP (Indira Gandhi Nahar Pariyojna) area of Jaisalmer (W. Rajasthan), the assessment of efficiency of single- and double-row shelterbelts of Dalbergia sissoo revealed that the double-row shelterbelt of 15–20 years of age having 8–10 m height hold great promise for moderating micro-climate and providing effective shelter to crops against wind-borne hazards in arid areas. Shelterbelts have improved soil characteristics and modified air temperature (Prasad et al., 2009). Another study reported that the shelterbelts increased net returns from agricultural production by 430.8% in the net returns due to shelterbelt plantation, in which shelterbelt technology has contributed ~399% (Gajja et al., 2008).
Silvi-pastoral systems are other important agroforestry systems in wind-eroded areas. In addition, providing nutritional fodder to livestock protects them from hot and warm winds, improving overall animal health and productivity (Atangana et al., 2014). There are several examples where different agroforestry systems have contributed to minimizing erosion and enhancing farm productivity, soil fertility, and ameliorated micro-climate (Table 6). With all these efforts sand dune area has reduced by 12% between 1980 and till date. The portion of western Rajasthan that is impacted by wind erosion has shrunk by 3%. The region has seen a rise in the net sown area, a decrease in culturable wastelands, and an increase in total crop area. This impact has come from using different agroforestry techniques for combating wind erosion in the area (Moharana et al., 2018).
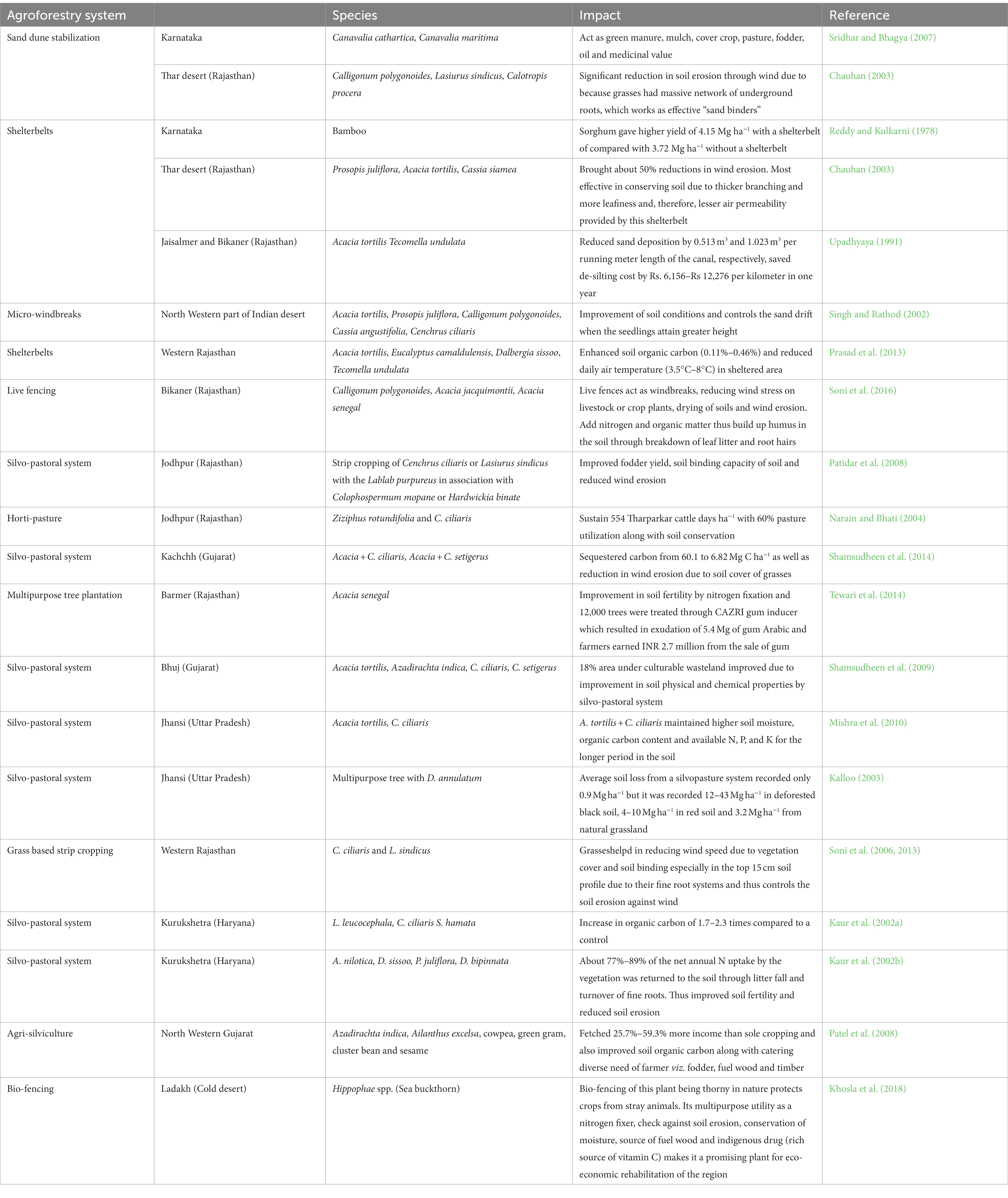
Table 6. Different agroforestry systems for wind-eroded areas and their impact on controlling erosion.
Physical land degradation
It is a degradation of the soil’s structure, hampering its role and ecosystem services. It is mostly occurred due to indiscriminate anthropogenic activities, uncontrolled or faulty agriculture practices, climate change (flood), and industrial development. It may become a threat to meeting the visions of the SDGs. Mining (mined land), industrial wastewater, and waterlogged lands are the major form of physical land degradation affecting 1.07-M ha of land in India. Different agroforestry systems have been developed for rehabilitating physical land degradation.
Mined area
The mining sector, which plays an essential role in national economic growth, involves more than 20,000 known mineral deposits and provides the bulk of employment and job creation of ~5,60,000 individuals daily in India. India’s mining sector is large, with 9,200 mines spread across 11 states producing 84 different minerals, comprising four fuel, 11 metallic, 49 non-metallic industrial, and 20 minor minerals (Ministry of Mines, 2018), according to Das et al. (2018), ~3,100 mines operating in India. It is estimated that ~12,000 stone crusher units are operating in India (Patil, 2001). Although it contributes 10%–12% of GDP to India’s entire industrial sector, it is also harmful to the environment. Unscientific mineral mining is a severe environmental issue, resulting in forest loss, widespread soil erosion, and pollution of air, water, and land (Pal and Mandal, 2017). Mining produces vast amounts of tailings and trash containing heavy metals, posing a serious hazard to water sources, agricultural soils, and food. Increased heavy metal concentrations in soils can induce phytotoxicity, a direct threat to human health, as well as indirect impacts such as pollution of water bodies (Pulford et al., 2002). Unplanned mining activities, as well as a lack of care for land reclamation or mining’s environmental effects, have resulted in the formation of vast swaths of industrial wastelands (Ghosh, 1991).
Agroforestry for mined area
Trees can penetrate the stony layer with their roots, causing fissures and allowing surface water to percolate, or holding any quantity of dirt by their roots to protect against erosion and percolation. Ghosh (1991) reported successful species survival after 1 year of reclamation of wastelands of Jharia coalfield, India, by mixed plantation of Azadirachta indica, Ricinus communis, Phoenix dactylifera, Psidium guajava, Butea frandosa, Leucaena leucocephala, and Artocarpus integrifolia. Species selected for rehabilitation should be N-fixing, fast-growing, well adopted to arid-zone climatic circumstances (i.e., extreme heat and sunlight), and have drought-tolerant root architectural adoption with significant socioeconomic utility. It also helps increase soil microbial activity leading to nutrient mineralization, increased below-ground biodiversity, increased nutrient cycling, and soil matrix stabilization (Datar et al., 2011). Due to the long rotation age of trees to obtain an economic return, combining trees with crops or grasses is a good land-use option for land-use choice for the restoration of mined areas. Seedlings of Ceiba pentandra have shown 100% survival when transplanted to ex-tin mining land. Although Acacia mangium has thrived in the ex-tin mining land, Paraserianthes falcataria does not appear to be adopted to these conditions. Other species such as Casuarina equisetiolia, Terminalia catappa, and Acacia auriculiformis, also perform well. Datar et al. (2011) reviewed the rehabilitation of post-mined in India and found that four species Pongamia sp., Dalbergia sp., Albizia sp., and Azadirachta sp. species have the highest potential for recovering agro-ecosystem function within the context of Indian post-mined landscapes.
Dadhwal et al. (1991) reported that 20 Mg ha−1 of mulch was the optimal dose for Eulaliopsis binata, resulting in superior results. Grevillea pteridifolia and Eucalyptus camaldulensis showed excellent survival and growth in 5-year-old plantations in the bauxite mine of Amarkantak. Planting nitrogen-rich leguminous species such as Leucaena leucocephala and Peuraria hirsuta in mine debris offered fodder, organic manure, mulch, and other benefits. Under geotextiles, Thysonoleana maximum, Saccharum munja, Pennisetum purpureum, Eulaliopsis binata, Ipomoea carnea, and Vitex negundo performed well (Juyal et al., 2007). Approximately 80% survival and good establishment were obtained when two-thirds of the pit was filled with a mixture of red soil, FYM, and sand after leveling for Eucalyptus citridora, Dalbergia sissoo, Albizia lebbeck, Casuarina equisetifolia, Acacia auriculaeformis, Leuceana leucocephala, and A. nilotica and in Neyveli (Narayana, 1987). Norem et al. (1982) found that Acchariss arothroides and Nicotiana glauca were shown prolific growth on the north aspect, while only one shrub, Dodonea viscosa, survived on the east aspect. This study suggested the chemical makeup of mine waste material, slope exposure, and species compatibility to the dry climate all have a role in the restoration of mined areas. Successful techniques adopted for the planting of trees in mined areas have been summarized in Table 7.
Industrial wastewater
Industries such as plastics, electronics, electrical, mineral-based dyes, fabrics, chemicals, and other materials, produce industrial wastewater of 50 million liter daily containing a high concentration of heavy metals, chemicals, and dyes (Singh, 2018). With an installed sewage treatment capacity of 21.9 billion liters per day, India produces ~0.5 billion liters of industrial wastewater and 61.7 billion liters of sewage per day (Roy, 2020). Increasingly, industrial effluents and municipal wastes are making their way into freshwater bodies, posing major health and environmental risks (Ranjan, 2021). Many industrial facilities release untreated effluents into water streams due to capacity constraints and poor pricing processes. Oil has been illegally spilled into the Hindon river by industries operating along its banks in the past (Rajput, 2019).
Agroforestry for industrial wastewater
Agroforestry-based wastewater reclamation contributes to the solution to agriculture’s growing water scarcity (Ranjan, 2021). Large amounts of industrial wastewater are used as irrigation water. They are regarded as a reliable source of vital nutrients, primarily N, P, K, and organic matter, which are beneficial to soil fertility, plant growth, and production (Libutti et al., 2018). Rasheed et al. (2020) reported an increase of 30% in growth parameters and biomass production, 34% in net CO2 assimilation rate, and 42% in water use efficiency under wastewater treatment compared to control in Conocarpus lancifolius seedlings. Plant species that will be employed for phytoextraction must be able to tolerate heavy metals (HMs). In some plants, HMs are accumulated in the roots, while in others, HMs are transferred to the leaves (Kafil et al., 2019). In response to wastewater irrigation, Zn, Pb, and Cd accumulation increased dramatically in roots, followed by leaves and shoots in C. lancifolius. The high translocation of Zn and Cd from the root to the aerial portions of C. lancifolius suggests that it has significant phytoextraction capability. Conocarpus lancifolius may thus be employed to rehabilitate soils polluted with Zn, Pb, and Cd, because of its improved biomass production, water usage efficiency, metals accumulation, tolerance, and translocation factor (Rasheed et al., 2020). The largest biomass distribution to stem in A. indica, twigs in P. cineraria, and roots in other species demonstrated the adoption mechanisms by different species when comparing the average contributions of various components in total dry biomass (Singh et al., 2021). The species include Acacia nilotica, Azadirachta indica, Cupressus spp., Casuarina spp., Eucalyptus spp., Khaya senegalensis, Morus spp., Swietenia mahogany, and Tamarix spp. were evaluated successfully under afforestation using untreated and secondary treated wastewater (Singh et al., 2021). Both Acacia ampliceps and Azadirachta indica demonstrated high biomass increment, high Pb concentrations, and an excellent antioxidative defence mechanism, indicating that they may be utilized for planting in industrial water irrigated soils in Pakistan (Hussain et al., 2021). Wastewater can be an alternative source of water in dryland afforestation if proper species are selected. Several studies highlighted the importance of treated wastewater in urban greening and reducing land degradation along with the usage of freshwater in arid areas. Eucalyptus camaldulensis, S. persica, S. oleoides, and T. undulata responded better in terms of survival, adaptation, and growth to wastewater for enhanced productivity in Indian deserts condition (Singh et al., 2021). It was found that plant growth-promoting rhizobacteria can effectively accelerate the phytoremediation process through a variety of mechanisms, including methylation, altering soil pH, encouraging redox processes, and secreting siderophores, bio-surfactants, and a variety of organic acids (Khan et al., 2009). Plant selection, as well as physicochemical soil factors and the research of plant–microbe interactions, could aid in the development of cost-effective remediation solutions.
Waterlogged area
Heavy or prolonged rainfall, over-irrigation, flooding, or high water table leads to waterlogging. In recent years, more intense and unpredictable rainfalls associated with climate change have raised waterlogging incidents worldwide and become one of hazardous abiotic stress (IPCC, 2014). Waterlogging affects various chemical, biological, and physical properties of soil, which in turn affects the ability of soils to support vegetation growth (Kozlowski, 1997). Highly adapted species survive waterlogging periods without any injuries up to a specific period. By contrast, sensitive or less tolerant species can suffer damage within a short period under the oxygen deprivation stage (Kreuzwieser and Rennenberg, 2014). Season, height and duration of flooding, water movement (moving or stagnant), environmental conditions, and various plant-specific characteristics determine the damage’s extent (Vreugdenhil et al., 2006). Within the same species, adult trees were more tolerant than younger ones. As an aerobic organism, trees depend on a constant oxygen supply to all living cells of the body and disturbance in metabolism. This leads to disruption in normal functioning at the cellular level based on their tolerance toward the depletion of soil oxygen and damage becomes visible later. Some of the negative impacts of the waterlogging stress in fruit crops include a decrease in net photosynthetic rate, stomatal conductance and root hydraulic conductivity, and hormonal changes (increase in ABA and ethylene), which ultimately result in reduced leaf size, leaf abscission, restriction of vegetative and root growth, etc. (Schaffer et al., 2006). The activity of several metabolic pathways (mineral, carbohydrate, organic acid, protein, lipid metabolism, and hormone relations) reduces under anaerobic circumstances (Kennedy et al., 1991). Waterlogging hampers root growth, root formation, branching, and mycorrhizae formation and causes root decay (Kozlowski and Pallardy, 1997). Ultimately it causes a reduction in flowering, yield, and in severe cases, death of plants through wilting, root necrosis, root rot, etc.
Agroforestry for waterlogged area
Studies have revealed that fast-growing and short-rotation tree-based systems have bio-drainage potential to prevent waterlogging in areas irrigated by canals. The bio-drainage techniques are eco-friendly and economically attractive. Fast-growing tree species such as Eucalyptus spp. Terminalia arjuna, Casuarina glauca, Syzygium cuminii, and Pongamia pinnata are also suitable species for bio-drainage (Uthappa et al., 2015). The block planting of Eucalyptus tereticornis at the IGNP site in Rajasthan and Dhob-Bhali in Haryana is very successful in lowering the water table. According to reports, the tree plantations built along the canal drained 14 m of water in just 6 years (Kapoor, 2001). In addition, Ram et al. (2011) observed that the 5-year-old E. tereticornis had an average transpiration rate of 30.9 L day−1 tree−1, which was 268 mm per year by 240 trees ha−1 in comparison to the mean annual rainfall of 212 mm. According to Behera et al. (2015), multifunctional woodlots, agri-silviculture, agi-horticulture, and silvi-pasture are popular strategies for addressing saline and waterlogged situations. Rice, wheat, berseem, mustard, cowpea, pigeon pea, sorghum, turmeric, and annual oat crops were effectively produced beneath Salix, Eucalyptus, Acacia, Albizia, Terminalia, Prosopis, and Populus tree species in the instance of an agri-silviculture system (Sarvade et al., 2017). Table 8 provides a selection of plants that are appropriate for bio-drainage therapy in salt-affected, waterlogged regions of India.
Chemical degradation
Alteration in soil pH, deficiency or toxicity of nutrients, salinization, alkalinization, and acidification are the processes that lead to the chemical degradation of lands. Both natural and anthropogenic factors contribute to the development of the chemical degradation of land. Saline, sodic and acidic soil are the major form of chemical degradation covering 17.4 M ha of land in India. Agroforestry can play an instrumental role in the rehabilitation of these lands.
Saline and sodic soils
Salt-affected soils adversely affect the physiological processes and productivity of plant species across the globe (Singh et al., 2009). These soils modify the soil properties and reduce plant water availability, resulting in the alteration of the structure and function attributes of the exposed plants (Gentili et al., 2018). Salt-affected soils mostly contain Na+, K+, Ca2+, and Mg2+ cations, CO32−, and HCO3−1 (alkali), and Cl− and SO42− (saline) as dominant anions (Zhang et al., 2006). The presence of salts in soils causes alteration of the physiological and biochemical traits of the exposed plant, resulting in low agriculture productivity and deterioration of precious land resources (Parida et al., 2016). Therefore, the reduction in agricultural productivity as a consequence of salinization promotes the interest in growing salt-tolerant tree plantations to increase the sustainability, productivity, and profitability of the salinity-afflicted landscapes (Kumar et al., 2020). Tree species can be characterized as sensitive, moderately tolerant, highly tolerant, or extremely highly tolerant to salt, depending on their level of tolerance (Tomar et al., 2003; Dagar, 2014). Moreover, different salt stress could have a contracting effect on the biomass production and yield of tree species (Banyal et al., 2017). Therefore, agroforestry seems to be the only viable option for obtaining greater ecological and economic benefits for such soils.
Agroforestry for saline and sodic soils
Most of the research findings have indicated that tree plantation improves the soil’s physicochemical properties-decrease, the soil pH and EC, and improves the nutrient cycling, organic carbon, and cation exchange capacity of the salt-affected soils (Garg and Jain, 1992; Singh, 1998). In northwest India, salt-tolerant crops such as pearl millet and mustard can be intercropped with Eucalyptus tereticornis and Melia composita in saline soils (Banyal et al., 2017). The various fruit species, such as Bael (Aegel marmelos), Aonla (Emblica officinalis), and Karonda (Carrisa carandas), along with the salt-tolerant annual crops, were observed to be suitable and economically viable under moderate saline irrigation water conditions (Dagar et al., 2008, 2016). The salt-tolerant multipurpose tree species—such as P. juliflora, A. nilotica, Casuarina equisetifolia, Tamarixarjuna, T. articulata, and Pongamia pinnata have grown in association with various grass species such as, Leptochloa fusca, Chloris gayana, Brachiaria mutica, and Sporobolus spp. in the form of the silvopastoral system have been found highly effective for reclaiming high alkali soil as well as for the fodder production (Dagar, 2014). The block plantation of various tree species, such as A. nilotica, Albizia procera, L. leucocephala, Azadirachta indica, and Eucalyptus hybrid, was observed to be the best practice for reclaiming the alkali soils. Furthermore, there is tremendous scope for bio-fuels (Energy plantations) in saline conditions under the prevailing scenario of climate change. In areas having abundant saline water, the saline aquaforestry practice consisting of various salt-tolerant fishes reared in the saline water and trees planted on the pond bund has also indicated immense potential in the saline areas (Banyal et al., 2018). In low-lying areas prone to water logging due to the high water table is a major issue, and the biodrainage technique can be designed, which consists of planting waterlogging tolerant species to transpire excess water into the atmosphere and to lower the high water table. Dagar et al. (2016) evaluated the impact of three planting spacings viz. 1 × 1, 1 × 2, and 1 × 3 m of Eucalyptus tereticornis in waterlogged saline soils. Due to the high transpiration rate of Eucalyptus, the water table was lowered by 43.0 cm in 1 × 1 m, 38.5 cm in 1 × 2 m, and 31.5 cm in 1 × 3 m spacing during the 4th year of the plantation than in adjacent fields without plantation. Therefore, the above evidence indicates that agroforestry and tree plantation is the only ecologically and economically viable option to improve the productivity potential of salt-affected soils. The various agroforestry systems developed for saline and sodic soils of India are summarized in Table 9.
Acidic soils
Acidic soil covers ~800 M ha globally (Behera et al., 2011), accounting for half of the world’s arable land (Tarin et al., 2021); especially in the humid tropics, soil acidity affects nearly one-third of India’s farmed land (Kumar et al., 2012). Acidity affects ~48 M ha of India’s 142 M ha of fertile land (Mandal, 1997). Soil acidification is caused by natural pedological processes such as carbonic acid influx from the atmosphere and internal acidity generation resulting from organic matter and nutrient cycling (Wong et al., 2004). Soil acidity can also be caused by heavy rainfall combined with nutrient leaching, cation mining from high-yielding crops, acidic soil parent material, and the use of acidifying fertilizers, or a combination of these causes (Xu et al., 2019). In high-rainfall regions, nitrate leaching appears to be the primary source of acidification, followed by the removal of harvested materials (Olego et al., 2021). Acidification in arable soils is primarily caused by crop nutrient extraction and losses of basic cations owing to leaching with downward water flow (Xu et al., 2019), particularly of Ca (Litvinovich et al., 2021).
Agroforestry for acidic soil
Trees play a great role in the agroforestry system. Tree-based systems reduce nitrate leaching more efficiently than tree-less systems, and deep-rooted trees absorb leached base cation from deep layers and are pumped back to the top layer via litterfall through nutrient pumping. In general, tree-based systems keep the SOM content higher than tree-less systems (Muchane et al., 2020). The key soil acidity management component in tropical habitats is the agroforestry method of management (Fageria and Nascente, 2014). Muchane et al. (2020) reviewed the acidity alleviation potential of agroforestry systems and concluded that overall, agroforestry practices elevated soil pH compared to monoculture, with minor differences depending on soil type. The incorporation of plant materials into acid soils or litterfall has resulted in higher soil pH, decreased Al saturation, and enhanced plant growth conditions in several laboratory trials. These plant materials also provide base cations such as Ca, Mg, and K (Wong et al., 2004). Gliricidia sepium had the greatest effect on improving soil pH and lowering monomeric Al content at the 10th week of incubation by its high base cation content. Application of 15 Mg ha−1 Gliricidia sepium biomass suppresses monomeric Al to the same amount as 90 Mg ha−1 Melastoma biomass or 15 Mg ha−1 Peronema biomass, according to a prior pot experiment (Hairiah et al., 1996). Alkalinity resulting from the deposition of leached base cations in a deep soil and mineral weathering might be exploited by deep-rooted perennial plant species in the agroforestry system (Wong et al., 2004). Senna siamea has been proven to recycle calcium from subsoils and improve pH in the top soil considerably (Vanlauwe et al., 2005). Trees that produce high-calcium litter are frequently linked to soils with higher exchangeable Ca, percent base saturation, and pH and are also associated with greater abundance and activity of soil organisms (Muchane et al., 2020). In charcoal-making areas of Acacia decurrens-based agroforestry landscape in Ethiopia, soil pH improved by one unit, and SOC increased by 10%, which was comparable to applying lime 4–5 t−1 (Amare et al., 2022). Incorporation of pruning’s of immature tree branches of Calliandra calothyrsus, Cassia siamea, Flemingia congesta, Grevillea robusta, Gliricidia sepium, Leucaena diversifolia, and Leucaena leucocephala resulted in a rise in soil pH and a reduction in exchangeable Al concentration due to high base cation content of the pruning 0.94–2.25 mol kg−1 (Wong et al., 2000). The liming impact of adding plant material or perennial components of agroforestry depends on is proportional to the total base cation charge (Wong et al., 2004). Trees with higher amounts of P or base cations such as Gmelina arborea rich in Ca and Mg alleviated soil P and pH (Wong et al., 2004).
Biological degradation
Degradation of soil biological quality is often associated with all forms of land degradation and does not occur exclusively as biological degradation of soil. Land degradation of any form negatively affects microbial diversity and impacts the sustainability of the ecosystem (Araujo et al., 2014). Biological degradation involves the loss of SOM or soil organic C, which is a prime component for the survival and proliferation of soil microbes. This subsequent loss of SOM/SOC triggers various negative impacts on the soil’s biological properties, which in turn harms the capacity of soil to perform several ecosystem functions and services. Loss of SOC often triggers microbial biodiversity loss (Singh, 2015), and it is expected that the greater the soil microbial diversity greater the chance for the ecosystem to establish functional equilibrium (Seneviratne, 2012). Soil microorganisms are the key to regulating the C cycle, as they are directly involved in the mineralization and immobilization of C from organic matter. The microbial diversity in the soil is thus important to regulate the fate of SOC and maintain soil health and productivity. It is estimated that enhancing soil biodiversity could result in 2.3 BT of additional crop production per year in the United States, with a monetary value of US$ 1.4 trillion (IUCN, 2019). The relationship between soil biodiversity and soil erosion losses is multifaceted and non-unidirectional (Orgiazzi and Panagos, 2018). Soil diversity may impact the problem of soil erosion in many ways. When considering macrofauna such as earthworms which are more abundant in less disturbed soils with high organic matter content, their abundance will harm soil erodibility. Certain studies have shown that the presence of earthworms can bring about a 50% decline in soil erosion rates (Blouin et al., 2013), which is mainly attributed to the increased soil porosity, higher infiltration rates, etc. On the contrary, mammals inhabiting the soil such as moles and rats can lead to the weakening of soil structure by burrowing activities which enhances soil erosion (Shuster et al., 2002). According to global research by Guerra et al. (2020), soil erosion affects not only soil conditions’ sensitivity but also its biodiversity, with 6.4% of these susceptible locations for soil macrofauna and 7.6% for soil fungus also occurring in areas with high soil biodiversity. Wu et al. (2021) studied the impact of N-enrichment and vegetation loss on soil microbial diversity. Both the degradation process had a detrimental effect on microbial diversity. However, the common soil fungi were less affected by N-enrichment compared to other microbial groups, which can give a clue about the resilience of the microbial groups toward degradation.
Fungi, particularly, have a major role in soil conservation through their physical and biochemical association with plants. Symbiotic association of plant roots with fungal hyphae, particularly arbuscular mycorrhizal fungi (AMF), can have a positive impact on soil structure and hence helps in arresting soil erosion. Plant root architecture and the soil moisture regime in the rhizosphere are greatly influenced by the association of AMF with plant roots. AMF imparts efficient water utilization, stronger wetting–drying cycles, stronger aggregates (Roy et al., 2021), and a reduction in soil loss (Mardhiah et al., 2016). The AMF inoculation helps in river bank slope stabilization and acts as a measure of soil conservation (Kimura and Scotti, 2016) due to the presence of Glomalin, a stable protein in AMF (Treseder and Allen, 2000). Glomalin has revolutionized the importance of AMF in soil conservation and is likely to play a crucial role while discussing soil biodiversity and land degradation.
Agroforestry for biologically degraded lands
Global food security and environmental sustainability depend on maintaining and improving soil fertility. Intercropping and mixed arable-livestock systems are two examples of ecologically appropriate agroforestry systems that can improve the sustainability of agricultural output. A helpful alternative to chemical fertilizers for increasing soil fertility is agroforestry. SOM restoration is more successful with alternative land-use systems including agro-horticulture, agro-pastoral, and agro-silvipasture. Choudhary and Rijhwani (2020) reported that the higher microbial diversity rates in agroforestry systems and the agro-silvopastoral system as compared to monoculture reported in Rajasthan as integrating trees provide favorable conditions for soil microflora to flourish. Yadav et al. (2011) reported that tree-based traditional agroforestry systems involving Acacia leucophloea, Dalbergia Sissoo, and Prosopis cineraria improved the microbial biomass of carbon by 40%–72%, microbial biomass of N by 38%–82% and microbial biomass of P by 38%–85% compared to open fallow in a semi-arid region of Rajasthan, India. Ghosh et al. (2019) observed that an anonla-based horti-pasture system improved the biological health of degraded lands in the Bundelkhand region of Uttar Pradesh, India. They reported that an anonla-based horti-pasture system along with staggered contour trenches and continuous contour trenches improved the SOC, bacterial and fungal density, soil enzyme activity, and treated soil quality index by 51% and 31%, 20% and 95%, 42% and 89%, and 154% and 184%, respectively, in surface soil over control. With the right species, soil fertility may also be restored in mobile farming zones. Sesbania rostrata and S. cannabina produce 307 and 209 kg N ha−1 of biological N2 fixation, respectively, when stem cuttings are planted, and irrigation is used to restore fertility (Pandey, 2007). The species mix should be planned for nutrient release that helps crops, even if trees are not completely harvested. Alnus nepalensis, Albizzia lebbek, Boehmeria rugulosa, Dalbergia sissoo, Ficus glomerata, and F. roxburghii were the six MPTS with the highest rates of nutrient release when established on an abandoned land at 1,200 m altitude in Central Himalaya. Thus, even though the only source of nutrients for crops in mixed agroforestry is leaf litter, Kharif crops (crops grown during the rainy season) contain high levels of nutrients. A variety of multifunctional trees contribute to steady nutrient cycling and a wide range of goods (Kerkhoff, 2006). Changes to aspects like the delivery of regulated ecosystem services are seldom taken into consideration in field and crop management, in part because there is a lack of thorough quantification of how trees alter biophysical field features. This is especially true for temperate climate arable systems. As a result, the effect of rows of trees of different sizes on the dominant soil properties in an agroforestry system for arable land was evaluated. In the area of trees in field margins, significantly increased SOC and soil nutrient concentrations of N, P, K, Mg, and Na were reported. This is likely due to the entry of tree litter and nutrient-enriched fall water (for K and Na). The potential of middle-aged to mature tree rows to boost SOC stocks and nutrient availability for the agricultural crop in an agroforestry system is highlighted by these results (Pardon et al., 2017). Large plant residue deposits on the soil caused by agroforestry systems result in high SOM content, greater soil biodiversity, and improved soil conservation. The cacao agroforestry systems used for cacao cultivation in the southern Bahia area of Brazil have positive impacts on the faunal populations that live in the soil and litter, and such systems of cacao cultivation may be thought of as a strategy for soil fauna conservation. A higher quantity and variety of soil fauna were produced as a result of the formation of a litter layer (da Silva Moco et al., 2009).
A notion known as “planetary health” refers to how human actions alter the structure and function of the environment with repercussions for human health. Agroforestry, which involves managing trees alongside crops and animals, affects biodiversity, hydrology, and biogeochemistry. But aside from the added nutritional value of eating more fruit, agroforestry’s effects on human health are rarely discussed. The research implies that agroforestry is likely to address a variety of urgent health conditions, notwithstanding some elevated risks of infectious diseases (Rosenstock et al., 2019). Agroforestry contributes to a reduction in soil-borne diseases. Such research was carried out in Brazil’s Atlantic rainforest. Fusarium wilt, often known as Panama wilt, is one of the most detrimental banana diseases and is brought on by the soil-borne fungus Fusarium oxysporum f. sp. cubense (Foc). Foc is pervasive in practically all banana-growing regions and is resistant to chemical or biological management. The development of disease-suppressiveness in soils may be able to control fusarium wilt, but little is understood about how soils might become more disease-suppressive and how crop management can affect this. Banana variety (cv.) In Pedra Dourada, Brazil, where Foc race 1 is found in the soil, maca, a cultivar that is extremely vulnerable to Foc race 1, was produced on a farm run as an agroforestry system. The findings of this study show that a good plant arrangement, in which cv. Maca is grown in mixed stands with other banana types, which might aid in the promotion of Fusarium wilt suppression in soils with positive abiotic qualities (Deltour et al., 2017). Contrary to monocultures, the integration of trees into agroforestry systems can boost the sustainability of the system. The altered structure and functions of the soil microbial community as a result of the system complexity increase are expected to have an impact on soil-N cycling. In both soil types, the ratio of soil fungus to bacteria was higher in the tree row than in the crop or grass rows of monoculture farmland and open grassland, presumably because more tree wastes were added and there was no tillage in the Phaeozem (cropland) soil. It has been demonstrated that the number of soil bacteria, fungi, and soil-N-cycling genes, particularly those involved in ammonium oxidation, can change in temperate regions when monoculture cropland and open grassland are converted to agroforestry systems (Beule et al., 2019).
According to Roy et al. (2009), the farm stage is a significant contribution to the effect categories of global warming, eutrophication, and toxicity. Agricultural production is often a hotspot in the life cycle of food goods (Salomone, 2003; Humbert et al., 2009; Pleanjai and Gheewala, 2009; Cappelletti et al., 2010). Hence there is a need for a paradigm shift from a monoculture to an agro forestry system to promote sustainable agricultural practices. With contributions of 3.67E ± 01 kg CO2, 4.31E-02 kg SO2, and 2.25E-05 kg PO4-eq, respectively, to global warming, acidification, and eutrophication, cocoa–coconut agroforestry had the least influence on these global impact categories. In addition, cocoa–coconut agroforestry had the highest SOC and SOM, fostering the development and activity of helpful soil microbes (Pseudomonas sp. and Trichoderma sp.). In addition, the cocoa–coconut agroforestry total land equivalent ratio got the greatest value, 1.36, indicating a higher production advantage. As a result, promoting cocoa–coconut agroforestry might be a smart move to ensure the environmental sustainability of cocoa farming (Utomo et al., 2015). In forest ecosystems, soil microorganisms, particularly bacteria, are common and essential to processes like the biogeochemical cycle and nitrogen transformation for plant development. The main factors influencing these bacterial community patterns were found to be soil pH, accessible P, and dissolved organic nitrogen. Establishing rubber-based agroforestry systems is a viable management strategy for reducing the negative impacts of rubber monoculture on bacterial biodiversity, including severe acidification and nutrient depletion. This is especially true for systems in mature stands (Liu et al., 2019).
National Agroforestry Policy 2014
The Indian government has formulated National Agroforestry Policy, 2014, to converge and establish synergy between various elements of agroforestry scattered in various existing missions, programs, and schemes of the Government. The policy aim is to promote agroforestry programs and encourage farmers to practice agroforestry to meet the ever-increasing demand for timber, and non-timber forest produces, increase forest/tree cover, conserve the environment, and mitigate climate change. The policy was targeted to address the various constraints and challenges, such as the provision of quality plating material, relaxation of legal provisions, financing and marketing of agroforestry produce, and improving agroforestry research and extension in the country. The major goal of the policy is to improve productivity, increase employment, provide livelihood opportunities, and generate income for smallholder farmers through agroforestry interventions. The proper implementation of this policy could fulfill the target of land degradation neutrality through planting trees in the degraded lands. Subsequently, the national agroforestry mission was established to fulfill the objective envisaged in the national agroforestry policy (Chavan et al., 2015).
Future strategies and action plans for agroforestry
Agroforestry has demonstrated immense potential in reclaiming the degraded lands of India. The government of India has also initiated various programs and formulated several policies for supporting agroforestry in the country. Therefore, the following strategies and action plans should be considered for large-scale rehabilitation of degraded lands through agroforestry in the country:
• Development of need-based agroforestry technologies through multi-locational trials in various agro-ecological zones to upscale the best agroforestry practices and models in the different regions of the country;
• Cloning and mass multiplication of superior germplasm of tree species for developing the quality planting material suitable for the degraded soils;
• Development of Hi-tech nurseries for the production of quality planting materials for potential species in different agro-climatic zones of the country;
• Creation of the center for the excellence of each species in different regions by involving the nursery growers and state governments;
• Developing cultivation and management practices of high-value tree species for degraded soil conditions;
• Identifying and developing climate-resilient agroforestry systems for climate change mitigation and adaptation in degraded lands;
• Enhancing the abiotic stress tolerance of tree spp. through selection, improvement, and breeding approaches;
• Develop appropriate metrics and indicators to measure the intangible advantages and environmental externalities of ecosystem payment services provided by agroforestry;
• Simplifying the regulations about harvesting and selling of tree produce for exploring the full potential of agroforestry;
• Development of agroforestry-based business models by involving local farmers, research institutions, and private industries;
• Adopting mechanization in agroforestry right from plating to harvesting to reduce dependency on manual labor;
• Post-harvest and value addition in trees for getting the maximum benefits;
• Provision of easy and subsidized access to quality plating material to the farmers;
• Removal of legal hurdles hindering the planting, harvesting, and transporting of tree produce;
• Initiation of insurance and credit and subsidy scheme and low-interest financing mechanisms to farmers for adopting agroforestry practices;
• Comprehensive awareness-raising and capacity-building initiatives to promote agroforestry at all levels, including those of farmers, extension agents, village-level organizations, and cooperative societies for tree producers; and
• Creation of institutional mechanisms for coordinating and bringing together the ministries’ and schemes’ efforts to pursue agroforestry thematically.
In addition, Parthiban et al. (2021) have suggested several research, technological and policy interventions, such as an organized supply chain, buyback agreement with MSP, analysis, and sensitization of price, creation of supportive price mechanism, insurance and credit facilities, tax exemption, processing technology facilities, promotion of mechanization, efficient post-harvest management, value addition, development of high-yielding varieties, and clonal planting material, reducing the rotation, adoption of precision silviculture and profitable tree crop model, that could be helpful in dissemination and promotion of agroforestry models in India. Moreover, considering the success and constraints of past and present agroforestry practices, there is an urgent need to focus on developing high-value tree species-based agroforestry practices with a focus on productivity, profitability, and sustainability. The tree species, such as Santalum album, Tectona grandis, Pterocarpus santalinus, Aquilaria, Swietenia Mahagoni, and Melia dubia are considered highly valuable and preferred by the farmers. These species could be easily integrated with different agroforestry practices, such as agri-silviculture, silvi-pastoral, agri-horti-silviculture, and block plantations and can greatly increase farm income, restore degraded lands, and provide resilience against climate change.
Constraints and challenges for agroforestry in degraded lands
In India, significant progress has been made in agroforestry research and development. The different agroforestry models and practices have been identified and developed for rehabilitating degraded lands, resulting in substantial improvement in productivity and profitability from such lands. Despite the huge potential of agroforestry in terms of reclaiming problematic soils, enhancing land productivity, generating additional income, and mitigating climate change in the degraded lands. Several constraints and challenges at the regional and national levels are hindering the promotion and large-scale adoption of agroforestry practices. The constraints, such as lack of quality planting material, poor scientific management inputs, several legal barriers on cultivation, harvesting, transportation and selling, non-payment for ecosystems and environment services, and long gestation period of trees are the main bottlenecks hampering the large-scale cultivation of trees in agroforestry. Furthermore, the lack of suitable silviculture and cultivation techniques, poor extension strategy and training facility, non-implementation of policies, non-availability of organized markets, a monopoly in price fixation, low price, and non-availability of tree loan and insurance schemes are further hindering the cultivation of trees (Cooper and Denning, 2000). In addition, Parthiban et al. (2021) highlighted several challenges, such as an unorganized supply chain, weak buy-back system, insufficient market intelligence, policy issues on taxes, lack of alternate processing technology, poor mechanization, under-utilization of residues, inferior genetic resources of trees, low productive species, and lack of profitable trees-crop model, which were responsible for the poor dissemination of agroforestry in India. Moreover, most of the agroforestry practices developed in India were primarily focused on obtaining the environmental and reclamation benefits and secondary getting the economic benefits, which were one of the main reasons for the low adoption of agroforestry by the farmers. These issues get further aggravated while practicing agroforestry in degraded lands. Therefore, there is an urgent need to develop strategies and devise action plans for a greater success of agroforestry in India and particularly in degraded lands.
Conclusion
Restoration of degraded land is an important challenge in the Indian subcontinent. Agroforestry has demonstrated great potential in the sustainable rehabilitation of degraded lands. The various kinds of agroforestry practices, such as agri-silviculture, silvi-pastroral, and agri-horticulture have shown strong potential to provide ecological and economic benefits from these lands. Many potential species for agroforestry exist in India; the only requirement is harnessing their potential and bringing them into agroforestry-based business models. Moreover, the widespread cultivation and adoption of high-value trees by farmers can lead to a tremendous impact in terms of an increase in farm income. More studies are required to develop agroforestry practices that can be widely accepted by farmers and stakeholders. Understanding fundamental processes and implementing technology well would be crucial for successfully managing and repairing degraded lands through agroforestry. Moreover, effective agroforestry-based technological implementation is urgently needed to obtain the desired result to fulfill the “Bonn Challenge” goal of rehabilitating 26 M ha of degraded land by 2030. Therefore, to meet the UN’s land degradation neutrality target, it is necessary to develop appropriate agroforestry practices, policies, and action plans for the greater promotion and adoption of agroforestry in India.
Author contributions
DJ, RKa, RKu, VP, AV, MS, SC, VK, SD, AU, TR, VS, MM, DK, PK, DD, GS, AS, AN, NJ, EJ, and SK together designed the scope of the manuscript, wrote individual chapters, and prepared the figures. All authors contributed to the article and approved the submitted version.
Conflict of interest
The authors declare that the research was conducted in the absence of any commercial or financial relationships that could be construed as a potential conflict of interest.
Publisher’s note
All claims expressed in this article are solely those of the authors and do not necessarily represent those of their affiliated organizations, or those of the publisher, the editors and the reviewers. Any product that may be evaluated in this article, or claim that may be made by its manufacturer, is not guaranteed or endorsed by the publisher.
References
Adhikary, P. P., Hombegowda, H. C., Barman, D., Jakhar, P., and Madhu, M. (2017). Soil erosion control and carbon sequestration in shifting cultivated degraded highlands of eastern India: performance of two contour hedgerow systems. Agrofor. Syst. 91, 757–771. doi: 10.1007/s10457-016-9958-3
Ahirwal, J., Kumar, A., and Maiti, S. K. (2020). Effect of fast-growing trees on soil properties and carbon storage in an afforested coal mine land (India). Fortschr. Mineral. 10:840. doi: 10.3390/min10100840
Ahirwal, J., Maiti, S. K., and Reddy, M. S. (2017b). Development of carbon, nitrogen and phosphate stocks of reclaimed coal mine soil within 8 years after forestation with Prosopis juliflora (Sw.) dc. Catena 156, 42–50. doi: 10.1016/j.catena.2017.03.019
Ahirwal, J., Maiti, S. K., and Singh, A. K. (2017a). Changes in ecosystem carbon pool and soil CO2 flux following post-mine reclamation in dry tropical environment, India. Sci. Total Environ. 583, 153–162. doi: 10.1016/j.scitotenv.2017.01.043
Akram, S., Kashkouli, H. A., and Pazira, E. (2008). Sensitive variables controlling salinity and water-table in a biodrainage system. Irrig. Drain. Syst. 22, 271–285. doi: 10.1007/s10795-008-9056-4
Amare, T., Amede, T., Abewa, A., Woubet, A., Agegnehu, G., Gumma, M., et al. (2022). Remediation of acid soils and soil property amelioration via Acacia decurrens-based agroforestry system. Agrofor. Syst. 96, 329–342. doi: 10.1007/s10457-021-00721-8
Araujo, A. S. F., Borges, C. D., Tsai, S. M., Cesarz, S., and Eisenhauer, N. (2014). Soil bacterial diversity in degraded and restored lands of Northeast Brazil. Antonie Van Leeuwenhoek 106, 891–899. doi: 10.1007/s10482-014-0258-5
Arunachalam, A., Ramanan, S. S., and Handa, A. K. (2021). Administering agroforestry at the district level. Curr. Sci. 121, 473–474.
Atangana, A., Khasa, D., Chang, S., and Degrande, A. (2014). Agroforestry for soil conservation. Netherlands: Springer.
Banyal, R., Rajkumar Kumar, M., Yadav, R. K., and Dagar, J. C. (2017). “Agroforestry for rehabilitation and sustenance of saline ecologies” in Agroforestry. eds. J. C. Dagar and V. P. Tewari (Singapore: Springer), 413–454.
Banyal, R., Yadav, R. K., Sheoran, P., Bhardwaj, A. K., Rajkumar, P. K., and Tolia, R. (2018). Managing saline soils of indo-Gangetic plains with eucalyptus and Melia based agroforestry systems. Indian J. Ecol. 45, 841–849.
Barman, D., Sangar, C., Mandal, P., Bhattacharjee, R., and Nandita, R. (2013). Land degradation: its control, management and environmental benefits of management in reference to agriculture and aquaculture. Environ. Ecol. 31, 1095–1103.
Barradas, V. L., Nicolas, E., Torrecillas, A., and Alarcon, J. J. (2005). Transpiration and canopy conductance in young apricot (Prunus armenica L.) trees subjected to different PAR levels and water stress. Agric. Water Manag. 77, 323–333. doi: 10.1016/j.agwat.2004.09.035
Behera, L., Nayak, M. R., Patel, D., Mehta, A., Sinha, S. K., and Gunaga, R. (2015). Agroforestry practices for physiological amelioration of salt affected soils. J. Plant Stress Physiol. 1, 13–18. doi: 10.5455/jpsp.2015-06-06
Behera, S. K., Singh, M. V., Singh, K. N., and Todwal, S. (2011). Distribution variability of total and extractable zinc in cultivated acid soils of India and their relationship with some selected soil properties. Geoderma 162, 242–250. doi: 10.1016/j.geoderma.2011.01.016
Beule, L., Corre, M. D., Schmidt, M., Gobel, L., Veldkamp, E., and Karlovsky, P. (2019). Conversion of monoculture cropland and open grassland to agroforestry alters the abundance of soil bacteria, fungi and soil-N-cycling genes. PLoS One 14, e0218779–e0218719. doi: 10.1371/journal.pone.0218779
Bhantana, P., and Lazarovitch, N. (2010). Evapotranspiration, crop coefficient and growth of two young pomegranate (Punica granatum L.) varieties under salt stress. Agric. Water Manag. 97, 715–722. doi: 10.1016/j.agwat.2009.12.016
Bhat, Y., Nandy, S., Padalia, H., Nath, A. J., and Pebam, R. (2022). Role of geospatial technology in shifting cultivation studies in India: a systematic review. J. Indian Soc. Remote Sens. 50, 2359–2379. doi: 10.1007/s12524-022-01607-7
Bhattacharyya, R., Ghosh, B. N., Mishra, P. K., Mandal, B., Rao, C. S., Sarkar, D., et al. (2015). Soil degradation in India: challenges and potential solutions. Sustainability 7, 3528–3570. doi: 10.3390/su7043528
Blouin, M., Hodson, M. E., Delgado, E. A., Baker, G., Brussaard, L., Butt, K. R., et al. (2013). A review of earthworm impact on soil function and ecosystem services. Eur. J. Soil Sci. 64, 161–182. doi: 10.1111/ejss.12025
Cappelletti, G. M., Nicoletti, G. M., and Russo, C. (2010). Life cycle assessment (LCA) of Spanish-style green table olives. Ital. J. Food Sci. 22, 3–14.
Chalise, D., Kumar, L., and Kristiansen, P. (2019). Land degradation by soil erosion in Nepal: a review. Soil Syst. 3:12. doi: 10.3390/soilsystems3010012
Chandini, K. R., Kumar, R., and Prakash, O. (2019). The impact of chemical fertilizers on our environment and ecosystem. Res. Trend. Environ. Sci. 35, 69–86.
Chatterjee, D., Kuotsu, R., Ray, S. K., Patra, M. K., Thirugnanavel, A., Kumar, R., et al. (2021). Preventing soil degradation in shifting cultivation using integrated farming system models. Arch. Agron. Soil Sci. 68, 1841–1857. doi: 10.1080/03650340.2021.1937139
Chaturvedi, O. P., Dagar, J. C., Handa, A. K., Kaushal, R., and Pandey, V. C. (2018). “Agroforestry potential for higher productivity from degraded ravine watersheds” in Ravine lands: Greening for livelihood and environmental security. eds. J. C. Dagar and A. K. Singh (Singapore: Springer Nature), 335–360.
Chaturvedi, S., Kaushal, R., Tewari, S., and Dhyani, V. C. (2011). Agroforestry: an integrated land management option for fragile ecosystem. J. Funct. Environ. Bot. 1, 45–53. doi: 10.5958/j.2231-1742.1.1.004
Chaturvedi, O. P., Kaushal, R., Tomar, J. M. S., Prandiyal, A. K., and Panwar, P. (2014). “Agroforestry for wasteland rehabilitation: mined, ravine, and degraded watershed areas,” in Agroforestry systems in India: Livelihood security & ecosystem services, advances in agroforestry. eds. J. C. Dagar, A. K. Singh, and A. Arunachalam (India: Springer), 233–271.
Chaturvedi, O. P., Sikka, A. K., Handa, A. K., and Bajpai, C. K. (2016). Agroforestry technologies for different agro-climatic zones of the country. All India coordinated research project on agroforestry, ICAR-Central Agroforestry Research institute, Jhansi, Uttar Pradesh, India.
Chauhan, S. S. (2003). Desertification control and management of land degradation in the Thar Desert of India. Environmentalist 23, 219–227. doi: 10.1023/B:ENVR.0000017366.67642.79
Chauhan, S. K., Singh, A., Sikka, S. S., Tiwana, U. S., Sharma, R., and Saralch, H. S. (2014). Yield and quality assessment of annual and perennial fodder intercrops in Leucaena alley farming system. Range Mgmt. Agrofor. 35, 230–235.
Chavan, S. B., Dhillon, R. S., Sirohi, C., Keerthika, A., Kumari, S., Bharadwaj, K. K., et al. (2022). Enhancing farm income through boundary plantation of poplar (Populus deltoids): an economic analysis. Sustainability 14:8663. doi: 10.3390/su14148663
Chavan, S. B., Keerthika, A., Dhyani, S. K., Handa, A. K., Newaj, R., and Rajarajan, K. (2015). National agroforestry policy in India: a low hanging fruit. Curr. Sci. 108, 1826–1834.
Chirwa, T. S., Mafongoya, P. L., Mbewe, D. N. M., and Chishala, B. H. (2004). Changes in soil properties and their effects on maize productivity following Sesbania sesban and Cajanus cajan improved fallow systems in eastern Zambia. Biol. Fert. Soils 40, 20–27. doi: 10.1007/s00374-004-0740-8
Choudhary, A., and Rijhwani, S. (2020). Microbial diversity in selected agroforestry systems of Central Rajasthan. Int. J. Life Sci. Pharma. Res. 10, 65–73. doi: 10.22376/ijpbs/lpr.2020.10.5.L65-73
Cooper, P. J. M., and Denning, G. L. (2000). Scaling up the impact of agroforestry research. World Agroforestry Centre (ICRAF), Nairobi. 43.
Dadhwal, K. S., Singh, B., and Narain, P. (1991). Effect of placing and mixing of a normal soil on the performance of two grasses in limestone minespoil. J. Indian Soc. Soil Sci. 39, 751–756.
Dagar, J. C. (2014). “Greening salty and waterlogged lands through agroforestry systems for livelihood security and better environment” in Agroforestry Systems in India: Livelihood Security & Ecosystem Services-Advances in agroforestry. eds. J. C. Dagar, A. K. Singh, and A. Arunachalam (New Delhi: Springer), 273–332.
Dagar, J. C., Singh, G., and Singh, N. T. (2001). Evaluation of forest and fruit trees used for rehabilitation of semiarid alkali-sodic soils in India. Arid Land Res. Manag. 15, 115–133. doi: 10.1080/15324980151062742
Dagar, J. C., Tomar, O. S., Minhas, P. S., Singh, G., and Ram, J. (2008). Dryland biosaline agriculture-Hisar experience. Karnal, India: Central Soil Salinity Research Institute.
Dagar, J. C., Yadav, R. K., Tomar, O. S., Minhas, P. S., and Gajender, L. K. (2016). Fruit-based agroforestry systems for saline water-irrigated semi-arid hyperthermic camborthids soils of north-West India. Agrofor. Syst. 90, 1123–1132. doi: 10.1007/s10457-015-9889-4
Das, D. K., Chaturvedi, O. P., Jha, R. K., and Kumar, R. (2011). Yield, soil health and economics of aonla (Emblica officinalis Gaertn.)-based Agri-horticultural systems in eastern India. Curr. Sci. 101, 786–790.
Das, P. K., Das, B. P., and Dash, P. (2018). Role of plant species as hyper-accumulators in the decontamination of hexavalent chromium contaminated soil. Indian J. Environ. Health 38, 1016–1102.
Das, R., and Maiti, S. K. (2016). Estimation of carbon sequestration in reclaimed coalmine degraded land dominated by Albizia lebbeck, Dalbergia sissoo and Bambusa arundinacea plantation: a case study from Jharia coalfields, India. Int. J. Coal Sci. Technol. 3, 246–266. doi: 10.1007/s40789-016-0131-4
Dass, A., Sudhishri, S., Lenka, N. K., and Patnaik, U. S. (2011). Run–off capture through vegetative barriers and planting methodologies to reduce erosion and improve soil moisture, fertility and crop productivity in southern Orissa, India. Nutr. Cycl. Agroecosyst. 89, 45–57. doi: 10.1007/s10705-010-9375-3
Datar, A., Patrick, A., and David, M. (2011). “Post-mined land rehabilitation in India: cataloguing plant species used in land revegetation,” in Australian Mine Rehabilitation Workshop. eds. N. Vikukis and O. Nichols (Australia: JK Tech), 197–208.
Deltour, P., França, S. C., Pereirab, O. L., Cardosoc, I., Neve, S. D., Debodee, J., et al. (2017). Disease suppressiveness to Fusarium wilt of banana in an agroforestry system: influence of soil characteristics and plant community. Agric. Ecosyst. Environ. 239, 173–181. doi: 10.1016/j.agee.2017.01.018
Dhyani, S. K., Dadhwal, K. S., and Katiyar, V. S. (1988). Biological measures for rehabilitation of abandoned minespoil areas. Indian Farming 38, 15–18.
Dhyani, S. K., and Handa, A. K. (2013). India needs agroforestry policy urgently: issues and challenges. Indian J. Agrofor. 15, 1–9.
Dhyani, S. K., Kareemulla, K., and Handa, A. K. (2009). Agroforestry potential and scope for development across agro-climatic zones in India. Indian J. Forestry 32, 181–190. doi: 10.54207/bsmps1000-2009-RV2OE8
Dhyani, S. K., Newaj, R., Alam, B., and Dev, I. (2015). Agroforestry: present status and way forward. New Delhi, India: Biotech Books.
Dhyani, S. K., Samra, J. S., Ajit Handa, A. K., and Uma, (2007). Forestry to support increased agricultural production: focus on employment generation and rural development. Agric. Econ. Res. Rev. 20, 179–202.
Dollinger, J., and Jose, S. (2018). Agroforestry for soil health. Agrofor. Syst. 92, 213–219. doi: 10.1007/s10457-018-0223-9
Down To Earth (2019). Available at: https://www.downtoearth.org.in/news/agriculture/india-lost-31-of-grasslands-in-a-decade-66643 (Accessed January 11, 2023).
Duniway, M. C., Pfennigwerth, A. A., Fick, S. E., Nauman, T. W., Belnap, J., and Barger, N. N. (2019). Wind erosion and dust from US drylands: a review of causes, consequences, and solutions in a changing world. Ecosphere 10:e02650. doi: 10.1002/ecs2.2650
Fageria, N. K., and Nascente, A. S. (2014). Management of soil acidity of south American soils for sustainable crop production. Adv. Agron. 128, 221–275. doi: 10.1016/B978-0-12-802139-2.00006-8
Farid, H. U., Ahmad, I., Anjum, M. N., Khan, Z. M., Iqbal, M. M., Shakoor, A., et al. (2019). Assessing seasonal and long-term changes in groundwater quality due to over-abstraction using geostatistical techniques. Environ. Earth Sci. 78:386. doi: 10.1007/s12665-019-8373-2
Fertilizer Association of India (2021). Available at: https://www.faidelhi.org/general/con-npk.pdf (Accessed January 11, 2023).
Gajja, B. L., Prasad, R., Mertia, R. S., Chand, K., and Samra, J. S. (2008). Impact of shelterbelts on net returns from agricultural production in arid Western Rajasthan. Agric. Econ. Res. Rev. 21, 118–122.
Garg, V. K., and Jain, R. K. (1992). Influence of fuelwood trees on sodic soils. Can. J. For. Res. 22, 729–735. doi: 10.1139/x92-098
Gentili, R., Ambrosini, R., Montagnani, C., Caronni, S., and Citterio, S. (2018). Effect of soil pH on the growth, reproductive investment and pollen allergenicity of Ambrosia artemisiifolia L. Front. Plant Sci. 9:1335. doi: 10.3389/fpls.2018.01335
Ghosh, R. (1991). Reclaiming wastelands of Jharia coalfield, eastern India. Int. J. Min. Reclam. Environ. 5, 185–190. doi: 10.1080/09208119108944306
Ghosh, A., Kumar, A., Manna, M. C., Singh, A. K., Sharma, P., Sarkar, A., et al. (2019). Long-term in situ moisture conservation in horti-pasture system improves biological health of degraded land. J. Environ. Manag. 248:109339. doi: 10.1016/j.jenvman.2019.109339
Global Forest Watch (2022). Available at: https://www.globalforestwatch.org/ (Accessed January 11, 2023).
Global Soil Partnership. (2017). Endorses guidelines on sustainable soil management. Available at: http://www.fao.org/global-soil-partnership/resources/highlights/detail/en/c/416516/ (Accessed January 8, 2023).
GOI (2001). Report of the task force on greening India for livelihood security and sustainable development, planning commission. New Delhi: Government of India.
Guerra, C. A., Rosa, I., Valentini, E., Wolf, F., Filipponi, F., Karger, D. N., et al. (2020). Global vulnerability of soil ecosystems to erosion. Landsc. Ecol. 35, 823–842. doi: 10.1007/s10980-020-00984-z
Guo, B., Yang, G., Zhang, F., Han, F., and Liu, C. (2018). Dynamic monitoring of soil erosion in the upper Minjiang catchment using an improved soil loss equation based on remote sensing and geographic information system. Land Degrad. Dev. 29, 521–533. doi: 10.1002/ldr.2882
Hairiah, K., Adawiyah, R., and Widyaningsih, J. (1996). Amelioration of aluminium toxicity with organic matter: selection of organic matter based on its total cation concentration. Agri 19, 158–164.
Hammad, A., and Tumeizi, A. (2012). Land degradation: socioeconomic and environmental causes and consequences in the eastern Mediterranean. Land Degrad. Dev. 23, 216–226. doi: 10.1002/ldr.1069
Handa, A. K., Chavan, S. B., Kumar, V., Vishnu, R., Ramanan, S. S., Tewari, R. K., et al. (2020). Agroforestry for income enhancement, climate resilience and ecosystem services. Indian Council of Agricultural Research, New Delhi.
Handa, A. K., Dev, I., Rizvi, R. H., Kumar, N., Ram, A., Kumar, D., et al. (2019). Successful agroforestry models for different agro-ecological regions in India. Jointly published by the Central Agroforestry Research Institute (Jhansi, India: CAFRI) and the South Asia Regional Programme, World agroforestry (New Delhi: ICRAF).
Harsh, L. N., and Tewari, J. C. (1993). “Sand dune stabilization, shelterbelts and silvi-pastoral plantations in dry zones” in Desertification and its control in the Thar, Sahara and Sahel regions. eds. A. K. Sen and A. Kar (Jodhpur: Scientific Publishers), 269–279.
Hobbs, P., Sayre, K., and Gupta, R. (2008). The role of conservation agriculture in sustainable agriculture. Philos. Trans. R. Soc. Lond. Ser. B Biol. Sci. 363, 543–555. doi: 10.1098/rstb.2007.2169
Hombegowda, H. C., Adhikary, P. P., Jakhar, P., Madhu, M., and Barman, D. (2020). Hedge row intercropping impact on run-off, soil erosion, carbon sequestration and millet yield. Nutr. Cycl. Agroecosys. 116, 103–116. doi: 10.1007/s10705-019-10031-2
Hossain, M. A. (2011). An overview on shifting cultivation with reference to Bangladesh. Sci. Res. Essays 6, 6509–6514. doi: 10.5897/SRE11.1282
Humbert, S., Loerincik, Y., Rossi, V., Margni, M., and Jolliet, O. (2009). Life cycle assessment of spray dried soluble coffee and comparison with alternatives (drip filter and capsule espresso). J. Clean. Prod. 17, 1351–1358. doi: 10.1016/j.jclepro.2009.04.011
Hussain, Z., Rasheed, F., Tanvir, M. A., Zafar, Z., Rafay, M., Mohsin, M., et al. (2021). Increased antioxidative enzyme activity mediates the phyto-accumulation potential of Pb in four agroforestry tree species: a case study under municipal and industrial wastewater irrigation. Int. J. Phytoremediation 23, 704–714. doi: 10.1080/15226514.2020.1849016
IPCC (2014). Climate change 2014 synthesis report, summary for policymakers. Geneva: Intergovernmental Panel on Climate Change.
IUCN (2019) Issues briefs–conserving healthy soils. Gland: International Union for Conservation of Nature.
Jakhar, P., Dass, A., Adhikary, P. P., Sudhishri, S., Naik, B. S., Hombegowda, H. C., et al. (2017). Multitier agroforestry system for integrated resource conservation on uplands of Eastern Ghats region in India. Agrofor. Syst. 91, 697–712. doi: 10.1007/s10457-016-9976-1
Jama, B., Kwesiga, F, and Niang, A. (2006). Agroforestry innovations for soil fertility management in sub-Saharan Africa: prospects and challenges ahead. World Agroforestry Centre, Kenya.
Jha, M. N. (1987). “Environmental management of mining operations in Singrauli coal fieds—NCC” in Proceeding national workshop on environmental management of mining operations in India. ed. B. B. Dhar (Varanasi: BHU), 47–53.
Jinger, D., and Kakade, V. D. (2019). Land degradation and its management through soil and water conservation measure on arable land. Kerala Karshakan 7, 12–20.
Jinger, D., Kakade, V. D., Kaushal, R., Dinesh, D., Bhatnagar, P. R., and Singhal, V. (2021). Rehabilitation of degraded lands through different planting techniques in Melia dubia Cav. Food Sci. Rep. 2, 7–9.
Jinger, D., Kakade, V. D., Singhal, V., and Dinesh, D. (2020). Lemon grass: a lucrative crop for degraded lands of Central Gujarat. Agric. Environ. 1, 26–29.
Jinger, D., Kaushal, R., Dinesh, D., Singh, G., and Singh, A. K. (2022c). “Sivlo-aromatic system for enhancing ecosystem services in Mahi ravines of Central Gujarat,” in Proceedings of National Conference on landscape Management for Preventing Flood and Reservoir Sedimentation; 2022 September 22–24; Ranchi, Jharkhand, India. 39.
Jinger, D., Khatri, P., Kumari, K., Kumar, D., and Dinesh, D. (2022b). Agroforestry-based ecosystem services for livelihood resilience. Food Sci. Rep. 3, 50–55.
Jinger, D., Kumar, R., Kakade, V., Dinesh, D., Singh, G., Pande, V. C., et al. (2022a). Agroforestry system for controlling soil erosion and enhancing system productivity in ravine lands of Western India under climate change scenarios. Environ. Monit. Assess. 194:267. doi: 10.1007/s10661-022-09910-z
Joy, J., Raj, A. K., Kunhamu, T. K., Jamaludheen, V., and Jayasree, K. (2019). Fodder production and carbon stock of calliandra under coconut plantation. Range Mgmt. Agrofor. 40, 109–117.
Juyal, G. P., Katiyar, V. S., Dadhwal, K. S., and Joshie, P. (1998). “Reclamation of mine spoils in outer Himalayas” in Soil and water conservation: Challenges and opportunities: 8th ISCO conference. eds. L. S. Bhushan, I. P. Abrol, M. S. Rama, and M. Rao (Dehradun: Indian Association Soil and Water Conservation, Dehradun), 1384–1392.
Juyal, G. P., Katiyar, V.S., Dhadwal, K. S., Joshie, P., and Arya, R. K. (2007). Mined area rehabilitation in Himalayas Shastradhara experience. (Dehradun: Central Soil and water Conservation Research and Training Institute), 104.
Kafil, M., Boroomand Nasab, S., Moazed, H., and Bhatnagar, A. (2019). Phytoremediation potential of vetiver grass irrigated with wastewater for treatment of metal contaminated soil. Int. J. Phytoremediation 21, 92–100. doi: 10.1080/15226514.2018.1474443
Kakade, V. D., Jinger, D., Dayal, V., Chavan, S., Nangare, D. D., Wakchaure, G. C., et al. (2020). Dragon fruit: wholesome and remunerative fruit crop for India. Food Sci. Rep. 1, 44–48.
Kallarackal, J. (2010). Water use by Eucalyptus grandis plantations in comparison with grasslands located in the downhill areas of Mannavan shola in the Western Ghats of Kerala. Kelara: Forestry Research Institute.
Kalloo, G. (2003). Forage research-new dimensions. 42nd foundation Day lecture. Indian Grassland and Fodder Research Institute, Jhansi, UP, India.
Kapoor, A. S. (2001). Biodrainage – a biological option for controlling waterlogging and salinity. New Delhi: Tata McGraw – Hill Publishing Company Limited.
Kaur, B., Gupta, S. R., and Singh, G. (2002a). Carbon storage and nitrogen cycling in silvo-pastoral systems on a sodic soil in North Western India. Agrofor. Syst. 54, 21–29. doi: 10.1023/A:1014269221934
Kaur, B., Gupta, S. R., and Singh, G. (2002b). Bio-amelioration of a sodic soil by silvo-pastoral systems in North Western India. Agrofor. Syst. 54, 13–20. doi: 10.1023/A:1014221306004
Kaushal, R., Kumar, A., Alam, N. M., Mandal, D., Jayaparkash, J., Tomar, J. M. S., et al. (2017). Effect of different canopy management practices on rainfall partitioning in Morus alba. Ecol. Eng. 102, 374–380. doi: 10.1016/j.ecoleng.2017.02.029
Kaushal, R., Kumar, A., Alam, N. M., Singh, I., Mandal, D., Tomar, J. M. S., et al. (2021b). Assessment of eco-hydrological parameters for important sympodial bamboo species in Himalayan foothills. Environ. Monit. Assess. 193:468. doi: 10.1007/s10661-021-09231-7
Kaushal, R., Mandal, D., Panwar, P., Kumar, R., Kumar, P., Tomar, J. M. S., et al. (2021a). “Soil and water conservation benefits of agroforestry” in Forest resources resilience and conflicts. eds. P. K. Shit, P. P. Adhikary, V. P. Sati, H. R. Pourghasemi, and G. S. Bhunia (Netherland: Elsevier)
Kaushal, R., Singh, I., Thapliyal, S. D., Gupta, A. K., Mandal, D., Tomar, J. M. S., et al. (2020). Rooting behaviour and soil properties in different bamboo species of Western Himalayan foothills, India. Sci. Rep. 10:4966. doi: 10.1038/s41598-020-61418-z
Kaushik, N., Kumari, S., Singh, S., and Kaushik, J. C. (2014). Productivity and economics of different Agri–silvi–horti systems under drip irrigation. Indian J. Agric. Sci. 84, 1166–1171.
Kaushik, N., Tikkoo, A., Yadav, P. K., Deswal, R. P. S., and Singh, S. (2017). Agri-silvihorti systems for semiarid regions of north-West India. Agric. Res. 6, 150–158. doi: 10.1007/s40003-017-0247-9
Kennedy, R. A., Fox, T. C., Everard, J. D., and Rumpho, M. E. (1991). “Biochemical adaptations to anoxia: potential role of mitochondrial metabolism to flood tolerance in Echinochloa phyllopogon (barnyard grass)” in Plant life under oxygen deprivation: ecology, physiology and biochemistry. eds. M. B. Jackson, D. D. Davies, and H. Lambers (The Hague: SPB Academic Publishing), 217–227.
Kerkhoff, E. E. (2006). “Shifting cultivation in the eastern Himalayas: Farmers innovations as lessons for policy.” in Proceedings of Sustainable Sloping Lands and Watershed Management: Linking Research to Strengthen Upland Policies and Practices; 2006 December 12-15; Luang Prabang, Laos PDR. 36.
Khamzina, A., Lamers, J. P. A., Martius, C., Worbes, M., and Vlek, P. L. G. (2006). Potential of nine multipurpose tree species to reduce saline ground water tables in the lower Amu Darya River region of Uzbekistan. Agrofor. Syst. 66, 129–141. doi: 10.1007/s10457-005-4677-1
Khan, S. A., and Shukla, I. N. (2003). Suitable MPTs for sodic soils of central plain zone of Uttar Pradesh. Indian J. Agrofor. 5, 119–121.
Khan, M. S., Zaidi, A., Wani, P. A., and Oves, M. (2009). Role of plant growth promoting rhizobacteria in the remediation of metal contaminated soils. Environ. Chem. Lett. 7, 1–19. doi: 10.1007/s10311-008-0155-0
Khanzada, A. N., Morris, J. D., Ansari, R., Slavich, P. G., and Collopy, J. J. (1998). Groundwater uptake and sustainability of acacia and Prosopis plantations in southern Pakistan. Agric. Water Manag. 36, 121–139. doi: 10.1016/S0378-3774(98)00030-4
Khosla, P. K., Kumar, K., Dutt, N., Kathuria, K., Mehta, P., and Farhana, M. (2018). ITKs for land use and water management in cold deserts of Ladakh valley in Jammu and Kashmir. J. Pharmacogn. Phytochem. 7, 521–524.
Kimura, A. C., and Scotti, M. R. (2016). Soil aggregation and arbuscular mycorrhizal fungi as indicators of slope rehabilitation in the São Francisco River basin (Brazil). Soil Water Res. 11, 114–123. doi: 10.17221/23/2015-SWR
Kozlowski, T. T. (1997). Responses of woody plants to flooding and salinity. Tree Physiol. 17:490. doi: 10.1093/treephys/17.7.490
Kozlowski, T. T., and Pallardy, S. G. (1997). Growth control in woody plants. San Diego: Academic Press.
Kreuzwieser, J., and Rennenberg, H. (2014). Molecular and physiological responses of trees to waterlogging stress. Plant Cell Environ. 37, 2245–2259. doi: 10.1111/pce.12310
Kumar, R., Bhardwaj, A. K., Rao, B. K., Vishavkarma, A. K., Bhatnagar, P. R., Patra, S., et al. (2020). Development of degraded ravine lands of Western India via Sapota (Achras zapota) plantation with terracing vs. trenching-on-slope based conservation measures. Land Degrad. Dev. 32, 101–111. doi: 10.1002/ldr.3691
Kumar, R., Bhatnagar, P. R., Kakade, V., and Dobhal, S. (2019). Tree plantation and soil water conservation enhances climate resilience and carbon sequestration of agro ecosystem in semi-arid degraded ravine lands. Agric. For. Meteorol. 282-283:107857. doi: 10.1016/j.agrformet.2019.107857
Kumar, M., Hazarika, S., Choudhury, B. U., Ramesh, T., Verma, B. C., and Bordoloi, L. J. (2012). Liming and integrated nutrient management for enhancing maize productivity on acidic soils of Northeast India. Indian J. Hill Far. 25, 35–37.
Kumar, S., Singh, R., and Shukla, A. K. (2015). “Sustaining productivity in aonla based horti-pasture system through in-situ soil moisture conservation in semi-arid region of India,” in Proceedings of The XXIII International Grassland Congress; November 24; New Delhi, India.
Kunhamu, T. K., Kumar, B. M., and Jamaludheen, V. (2012). Utility of multipurpose trees as black pepper (Piper nigrum L.) standards in the humid tropics of Kerala. Indian J. Agrofor. 14, 17–22.
Kurothe, R. S., Singh, H. B., Tiwari, S. P., Pande, V. C., Bagdi, G. L., Sena, D. R., et al. (2018). 60 years of research on soil and water conservation in Gujarat. Anand: ICAR-Indian Institute of Soil and Water Conservation, Research Centre, Vasad.
Lal, R., and Steward, B. A. (1990). Need for action: research and development priorities. Adv. Soil Sci. 17, 331–336. doi: 10.1007/978-1-4612-3322-0_9
Lenka, N. K., Dass, A., Sudhishri, S., and Patnaik, U. S. (2012). Soil carbon sequestration and erosion control potential of hedge rows and grass filter strips in sloping agricultural lands of eastern India. Agric. Ecosyst. Environ. 158, 31–40. doi: 10.1016/j.agee.2012.05.017
Libutti, A., Gatta, G., Gagliardi, A., Vergine, P., Pollice, A., Beneduce, L., et al. (2018). Agro-industrial wastewater reuse for irrigation of a vegetable crop succession under Mediterranean conditions. Agric. Water Manag. 196, 1–14. doi: 10.1016/j.agwat.2017.10.015
Litvinovich, A., Pavlova, O., Lavrishchev, A., Bure, V., and Saljnikov, E. (2021). Dynamics of soil pH after utilization of by-products of industrial rock processing as a calcareous material in acid soils. Commun. Soil Sci. Plant Anal. 52, 93–101. doi: 10.1080/00103624.2020.1849267
Liu, C., Jina, Y., Hu, Y., Tanga, J., Xiong, Q., Xu, M., et al. (2019). Drivers of soil bacter.Ial community structure and diversity in tropical agroforestry systems. Agric. Ecosyst. Environ. 278, 24–34. doi: 10.1016/j.agee.2019.03.015
Lundgren, B. O., and Raintree, J. B. (1982). “Sustained agroforestry” in Agricultural research and development-potential and challenges in Asia. ed. B. Nestel (The Hague: ISNAR), 37–49.
Mandal, S. C. (1997). “Introduction and historical overview,” in Acid Soils of India. eds. I. C. Mahapatra, S. C. Mandal, C. Misra, G. N. Mitra, and N. Panda (New Delhi, India: ICAR), 3–24.
Mardhiah, U., Caruso, T., Gurnell, A., and Rillig, M. C. (2016). Arbuscular mycorrhizal fungal hyphae reduce soil erosion by surface water flow in a greenhouse experiment. Appl. Soil Ecol. 99, 137–140. doi: 10.1016/j.apsoil.2015.11.027
Markose, V. J., and Jayappa, K. S. (2016). Soil loss estimation and prioritization of sub-watersheds of Kali River basin, Karnataka, India, using RUSLE and GIS. Environ. Monit. Assess. 188:225. doi: 10.1007/s10661-016-5218-2
Mazumdar, S., and Kulkarni, K. (2016). Remediation of mined out areas and abandoned mines: a case study on Sesa’s approach. Available at: https://mines.gov.in/writereaddata/UploadFile/remediationofmined4.pdf (Accessed August 2022).
McDonald, M. A., Healey, J. R., and Stevens, P. A. (2002). The effects of secondary forest clearance and subsequent land use on erosion losses and soil properties in the Blue Mountains of Jamaica. Agric. Ecosyst. Environ. 92, 1–9. doi: 10.1016/S0167-8809(01)00286-9
Ministry of Mines (2018). Annual reports, Ministry of Mines, Government of India. Available at: http://mines.gov.in/writereaddata/UploadFile/Mines_AR_2017-18_English.pdf (Accessed February 2018).
Mishra, A. K., Tiwari, H. S., and Bhatt, R. K. (2010). Growth, biomass production and photosynthesis of Cenchrus ciliaris L. under Acacia tortilis (Forssk.) Hayne based silvo-pastoral systems in semiarid tropics. J. Environ. Biol. 31, 987–993.
Moharana, P. C., Pandey, C. B., and Kumar, M. (2018). Managing sand dunes of Thar Desert for sand control measures and agriculture. Indian Farming. 68, 16–20.
Muchane, M. N., Sileshi, G. W., Gripenberg, S., Jonsson, M., Pumarino, L., and Barrios, E. (2020). Agroforestry boosts soil health in the humid and sub-humid tropics: a meta-analysis. Agric. Ecosyst. Environ. 295:106899. doi: 10.1016/j.agee.2020.106899
Mughal, A. H., and Makaya, A. S. (2000). “Suitable agroforestry models for the degraded and wastelands of Srinagar district of Kashnir” in Environment biodiversity and conservation. ed. M. A. Khan (New Delhi: APH Publication Corporation), 493–500.
Mukhopadhyay, S., and Masto, R. E. (2022). Comparative evaluation of Cassia siamea and Albizia lebbeck for their potential to recover carbon and nutrient stocks in a chronosequence post-mining site. Catena 208:105726. doi: 10.1016/j.catena.2021.105726
Murthy, I. K., Gupta, M., Tomar, S., Munsi, M., Tiwari, R., Hegde, G. T., et al. (2013). Carbon sequestration potential of agroforestry systems in India. J. Earth Sci. Clim. Chang. 4:131. doi: 10.4172/2157-7617.1000131
Mythili, G., and Goedecke, J. (2016). “Economics of land degradation in India. Economics of land degradation and improvement” in A global assessment for sustainable development. eds. E. Nkonya, A. Mirzabaev, and J. V. Braun (Berlin, Germany: Springer), 431–471.
NAAS (2010). Degraded and wastelands of India–status of spatial distribution. New Delhi: National Academy of Agricultural Sciences.
Narain, P., and Bhati, T. K. (2004). “Alternative farming system: issues and opportunities in arid ecosystem” in Alternative farming Systems. eds. A. K. Singh, B. Gangwar, and S. K. Sharma (Modipuram: Farming System Research And Development Association), 57–64.
Narayana, M. P. (1987). Neyveli open cast mine – a review of environmental management of mining operation in India. (Varanasi: Banaras Hindu University), 54–62.
National Academy of Agricultural Sciences. (2012). Management of Crop Residues in the context of conservation agriculture; policy paper no. 58. National Academy of Agricultural Sciences: New Delhi, India, 12. Available at: http://naas.org.in/Policy%20Papers/policy%2058.pdf (Accessed January 10, 2023).
Newaj, R., Dar, S. A., Yadav, R. S., Ajit Shanker, A. K., and Dhyani, S. K. (2008). Management effect on growth, biomass, carbon, and nitrogen accumulation in Albizia procera at 4-years age in agroforestry system. J. Trop. For. 23, 73–80.
Newaj, R., Dhyani, S. K., Alam, B., Prasad, R., Rizvi, R. H., Ajit Handa, A. K., et al. (2012). Role of agroforestry for mitigating climate change-some research initiative, National Research Center for Agroforestry, Jhansi, India.
Nicu, I. C. (2018). Is overgrazing really influencing soil erosion? Water 10:1077. doi: 10.3390/w10081077
Norem, M. A., Day, A. D., and Ludeke, K. L. (1982). An evaluation of shrub and tree species used for revegetating copper mine wastes in the South-Western United States. J. Arid Environ. 5, 299–304. doi: 10.1016/S0140-1963(18)31611-2
Nyamadzawo, G., Nyamugafata, P., Chikowo, R., and Giller, K. (2008). Residual effects of fallows on selected soil hydraulic properties in a kaolinitic soil subjected to conventional tillage (CT) and no tillage (NT). Agrofor. Syst. 72, 161–168. doi: 10.1007/s10457-007-9057-6
Olego, M. A., Quiroga, M. J., Mendana-Cuervo, C., Cara-Jimenez, J., Lopez, R., and Garzon-Jimeno, E. (2021). Long-term effects of calcium-based liming materials on soil fertility sustainability and rye production as soil quality indicators on a typic palexerult. PRO 9:1181. doi: 10.3390/pr9071181
Orgiazzi, A., and Panagos, P. (2018). Soil biodiversity and soil erosion: it is time to get married: adding an earthworm factor to soil erosion modelling. Glob. Ecol. Biogeogr. 27, 1155–1167. doi: 10.1111/geb.12782
Pagare, R. L., Pawar, K., and Nirgude, V. (2014). Evapotranspiration and transpiration rate of some tropical tree species. Int. J. Sci. Res. Biol. Sci. 1, 1–2.
Pal, S., and Mandal, I. (2017). Impacts of stone mining and crushing on stream characters and vegetation health of Dwarka river basin of Jharkhand and West Bengal, eastern India. J. Environ. Geogr. 10, 11–21. doi: 10.1515/jengeo-2017-0002
Paramesh, V., Arunachalam, V., and Nath, A. J. (2019). Enhancing ecosystem services and energy use efficiency under organic and conventional nutrient management system to a sustainable arecanut based cropping system. Energy 187:115902. doi: 10.1016/j.energy.2019.115902
Pardon, P., Reubensa, B., Reheul, D., Mertens, J., Frenne De, P., Coussement, T., et al. (2017). Trees increase soil organic carbon and nutrient availability in temperate agroforestry systems. Agric. Ecosyst. Environ. 247, 98–111. doi: 10.1016/j.agee.2017.06.018
Parida, A. K., Veerabathini, S. K., Kumari, A., and Agarwal, P. K. (2016). Physiological, anatomical and metabolic implications of salt tolerance in the halophyte Salvadora persica under hydroponic culture condition. Front. Plant Sci. 7:351. doi: 10.3389/fpls.2016.00351
Parihar, A. K. S., and Saxena, A. K. (2016). “Dalbergia sissoo based silvi-pastoral system on sodic land” in Agroforestry technologies for different agro-climatic zones of the country. eds. O. P. Chaturvedi, A. K. Sikka, A. K. Handa, and C. K. Bajpai (Jhansi: ICAR-CAFRI), 46–47.
Parthiban, K. T., Fernandaz, C. C., Sudhagar, R. J., Sekar, I., Kanna, S. U., Rajendran, P., et al. (2021). Industrial agroforestry—A sustainable value chain innovation through a consortium approach. Sustainability 13:7126. doi: 10.3390/su13137126
Patel, J. M., Jamini, S. N., and Patel, S. B. (2008). “Evaluation of neem and ardu based Agri-silvi system” in Diversification of arid farming systems. eds. P. Narain, M. P. Singh, A. Kar, S. Kathju, and P. Kumar (Jodhpur, India: Arid Zone Research Association of India and Scientific Publisher), 114–118.
Pathak, P. S., Slanki, K. R., Rai, P., Handa, A. K., and Pateria, H. M. (2006). “Agroforestry” in Hanbook of agriculture. ed. R. Singh (New Delhi: ICAR), 1298–1352.
Patidar, M., Rajora, M. P., and Singh, R. (2008). Forage production potential of different silvipastoral systems under arid conditions of Rajasthan. Indian J. Agron. 53, 234–238.
Patil, M. A. (2001). Environmental management scenario in stone crusher industry sector and cleaner production possibilities. TERI Inform. Monit. Environ. Sci. 6, 83–92.
Pimentel, D. (2006). Soil erosion: A food and environmental threat. Environ. Dev. Sustain. 8, 119–137. doi: 10.1007/s10668-005-1262-8
Pleanjai, S., and Gheewala, S. H. (2009). Full chain energy analysis of biodiesel production from palm oil in Thailand. Appl. Energy 86, S209–S214. doi: 10.1016/j.apenergy.2009.05.013
Prakash, O., Dubey, R. K., Dubey, S. K., and Deshwal, J. S. (2011). Evaluation of Ber (Zizyphus mauritiana Lamk.) based Agri-horti systems under various tree root management practices on reclaimed Yamuna ravines. Indian J. Soil Conserv. 39, 59–62.
Prasad, R. (1989). Removal of forest covers through mining and technology for retrieval. J. Trop. For. 5, 109–116.
Prasad, R., and Chadhal, S. K. (1987). Afforestation of dolomite over burden in Madhya Pradesh. J. Trop. For. 3, 124–131.
Prasad, R., Mertia, R. S., Gajja, B. L., Singh, D., and Chandra, P. (2013). Morphological characteristics of tree shelterbelts and their impact on wind regimes, air temperature and soil quality. Indian J. Agrofor. 15, 74–81.
Prasad, R., Mertia, R. S., Kumawat, R. N., and Samra, J. S. (2009). Shelter efficiency of Dalbergia sissoo shelterbelt in IGNP command area in arid Western Rajasthan, India. Indian J. Agrofor. 11, 41–43.
Prasad, R., and Shukla, P. K. (1985). Reclamation and re-vegetation of coal mine overburden in Madhya Pradesh. J. Trop. For. 1, 74–84.
Prasad, R., Yadav, R. P., and Panwar, P. (2012). “Aonla based agroforestry systems for soil and water conservation in Shivaliks,” in Agroforestry System for Resource Conservation & Livelihood Security in Lower Himalayas. eds. P. Panwar, A. K. Tiwari, and K. S. Dhadwal (New Delhi, India: New India Publishing Agency), 171–178.
Prasath, C. N. H., Balasubramanian, A., Radhakrishnan, S., Sudarshan, A., and Jaisankar, I. (2014). Eco-physilogical behaviour of 2 year grown afforested tree in Tamil Nadu, South India. J. Andaman Sci. Assoc. 19, 181–184.
Pulford, I. D., Riddell-Black, D., and Stewart, C. (2002). Heavy metal uptake by willow clones from sewage sludge-treated soil: the potential for phytoremediation. Int. J. Phytoremediation 4, 59–72. doi: 10.1080/15226510208500073
Raizada, A., and Singh, C. (2010). Silvipastoral systems for wasteland utilization in foothills of the western Himalayas. Technology Brouchere. CSWCRTI, Dehardun, India.
Rajput, V. (2019). Oil found in Hindon river, samples taken for study, Vol. 197. Uttar Pradesh: Hindustan Times-Noida News.
Ram, J., Dagar, J. C., Lal, K., Singh, G., Toky, O. P., Tanwar, V. S., et al. (2011). Bio-drainage to combat waterlogging, increase farm productivity and sequester carbon in canal command areas of Northwest India. Curr. Sci. 100, 1673–1680.
Ramajayam, D., Mishra, P. K., Nalatwadmath, S. K., Mondal, B., and Adhikari, R. N., aand Murthy, B. K. N. (2007). Evaluation of different grass species for growth performance and soil conservation in vertisols of Karnataka. Indian J. Soil Conserv. 35, 54–57.
Ranjan, R. (2021). A potential PES mechanism for agroforestry-led industrial wastewater remediation using short-rotation trees. Water Econ. Policy 7:2150006. doi: 10.1142/S2382624X21500065
Rao, B. K., Kurothe, R. S., Singh, A. K., Parandiyal, A. K., Pande, V. C., and Kumar, G. (2012). Bamboo plantation based technological interventions for reclamation and productive utilization of ravine lands. Technology Brouchere. CSWCRTI, Research Centre, Vasad, Anand, India.
Rao, G. R., Prabhakar, M., Venkatesh, G., Srinivas, I., and Reddy, K. S. (2018). Agroforestry opportunities for enhancing resilience to climate change in rainfed areas. ICAR – Central Research Institute for Dryland Agriculture, Hyderabad, India. 224
Rasheed, F., Zafar, Z., Waseem, Z. A., Rafay, M., Abdullah, M., Salam, M. M. A., et al. (2020). Phytoaccumulation of Zn, Pb, and cd in Conocarpus lancifolius irrigated with wastewater: does physiological response influence heavy metal uptake? Int. J. Phytoremediation 22, 287–294. doi: 10.1080/15226514.2019.1658711
Rathore, A. C., Saroj, P. L., Sharma, N. K., Shrimali, S. S., Jayaprakash, J., Chaturvedi, O. P., et al. (2012). Mango based Agri-horticultural system for degraded lands of north western Himalaya. Technology Brouchere. CSWCRTI, Dehardun, India.
Reddy, M. G., and Kulkarni, G. N. (1978). Effect of shelterbelt on water use and yield of CSH-1 sorghum. Ann. Arid Zone 17, 343–347.
Rosenstock, T. S., Dawson, I. K., Aynekulu, E., Chomba, S., Degrande, A., Fornace, K., et al. (2019). A planetary health perspective on agroforestry in sub-Saharan Africa. One Earth 1, 330–344. doi: 10.1016/j.oneear.2019.10.017
Roy, S. (2020). Wastewater generation and treatment-present status in India. Available at: https://indianinfrastructure.com/2020/02/01/wastewater-generation-and-treatment-present-status-in-india (Accessed August 2022).
Roy, T., Mandal, U., Mandal, D., and Yadav, D. (2021). Role of arbuscular mycorrhizal fungi in soil and water conservation: a potentially unexplored domain. Curr. Sci. 120:1573. doi: 10.18520/cs/v120/i10/1573-1577
Roy, P., Nei, D., Orikasa, T., Xu, Q., Okadome, H., Nakamura, N., et al. (2009). A review of life cycle assessment (LCA) on some food products. J. Food Eng. 90, 1–10. doi: 10.1016/j.jfoodeng.2008.06.016
SAC (2021). Desertification and land degradation atlas of India (assessment and analysis of changes over 15 years based on remote sensing). Ahmedabad, India: Space Applications Centre.
Saha, S. R., Chaudhary, R. S., and Somasundaram, J. (2012). Soil health management under hill agro ecosystem of north East India. Appl. Environ. Soil Sci. 1:9. doi: 10.1155/2012/696174
Saha, R., Mishra, V. K., and Tomar, J. M. S. (2005). Effect of agroforestry systems on erodibility and hydraulic properties of Alfisols in eastern Himalayan region. Indian J. Soil Conserv. 33, 251–253.
Sahu, H. B., and Dash, S. (2011). “Land degradation due to Mining in India and its mitigation measures,” in Proceedings of the second international conference on environmental science and technology; February 26–28; Singapore.
Salomone, R. (2003). Life cycle assessment applied to coffee production: investigating environmental impacts to aid decision making for improvements at company level. J. Food Agric. Environ. 1, 295–300.
Sarvade, S., Gautam, D. S., Kathal, D., and Tiwari, P. (2017). Waterlogged wasteland treatment through agro-forestry: a review. J. Appl. Nat. Sci. 9, 44–50. doi: 10.31018/jans.v9i1.1147
Satapathy, K. K. (2005). “Runoff production on hill slopes under different land use systems” in Agroforestry in north East India: Opportunities and challenges. eds. B. P. Bhatt and K. M. Bujarbaruah (Umiam, Meghalaya: ICAR Research Complex for NEH Region), 451–459.
Schaffer, B., Davis, F. S., and Crane, J. H. (2006). Responses of subtropical and tropical fruit trees to flooding in calcareous soil. Hortic. Sci. 41, 549–555. doi: 10.21273/HORTSCI.41.3.549
Seneviratne, G. (2012). Are we wrong in conventional approach of biocontrol? Curr. Sci. 103, 1387–1388.
Shamsudheen, M., Devi, D., Meena, S. L., and Ram, B. (2009). “Improvement of soil properties under silvipastoral systems in the Kachchh region of arid Gujarat” in Abstract retrieved from 4th world congress on conservation agriculture (New Delhi: National Academy of Agricultural Sciences)
Shamsudheen, M., Devi, D., Meena, S. L., and Ram, B. (2014). Carbon sequestration in agroforestry and pasture systems in arid northwestern India. Curr. Sci. 107, 1290–1293.
Sharda, V. N., and Juyal, G. P. (2016). “Conservation technologies for sustaining natural resources,” in Handbook of Agriculture. ed. R. Singh (New Delhi: Indian Council of Agricultural Research (ICAR)), 323–370.
Sharda, V. N., and Mandal, D. (2018). Prioritization and field validation of erosion risk areas for combating land degradation in north western Himalayas. Catena 164, 71–78. doi: 10.1016/j.catena.2017.12.037
Sharda, V. N., and Venkateswarlu, B. (2007). “Crop diversification and alternate land use systems in watershed management.” in Proceedings of the Comprehensive Assessment of Watershed Programs in India ; 2007 July 23–27; (Andhra Pradesh, India: ICRISAT), 332.
Shuster, W. D., McDonald, L. P., McCartney, D. A., Parmelee, R., Studer, N., and Stinner, B. (2002). Nitrogen source and earthworm abundance affected runoff volume and nutrient loss in a tilled-corn agroecosystem. Biol. Fertil. Soils 35, 320–327. doi: 10.1007/s00374-002-0474-4
da Silva Moco, M. K., da Gama-Rodrigues, E. F., da Gama-Rodrigues, A. C., Machado, R. C. R., and Baligar, V. C. (2009). Soil and litter fauna of cacao agroforestry systems in Bahia, Brazil. Agrofor. Syst. 76, 127–138. doi: 10.1007/s10457-008-9178-6
Singh, B. (1998). Contribution of fine roots in reclamation of semiarid sodic soils. Arid Soil Res. Rehabil. 12, 207–222. doi: 10.1080/15324989809381510
Singh, J. S. (2015). Microbes: the chief ecological engineers in reinstating equilibrium in degraded ecosystems. Agri. Ecosys. Environ. 203, 80–82. doi: 10.1016/j.agee.2015.01.026
Singh, A. P. (2018). “Status report on CETPs, STPs and industrial pollution in Jojari River (Jodhpur to Balotra),” in Submitted in reference to Hon’ble National Green Tribunal (NGT), New Delhi, India.
Singh, D. V., Ghosh, B. N., Mandal, D., Ranjan, R., Avasthe, R. K., Roy, T., et al. (2017). Technical resource book on soil management for controlling land degradation, ICAR-Indian Institute of Soil and Water Conservation, Dehradun, India.
Singh, A. K., Kala, S., Dubey, S. K., Pande, V. C., Rao, B. K., Sharma, K. K., et al. (2015). Technology for rehabilitation of Yamuna ravines – cost-effective practices to conserve natural resources through bamboo plantation. Curr. Sci. 108, 1527–1533.
Singh, G., and Lal, K. (2014). Bio-drainage for management of and soil salinity. Available at: https://www.icid.org/report_bmw_n_ss.pdf (Accessed August 2022).
Singh, C., Mehta, H., Tomar, J. M. S., Rathore, A. C., Kaushal, R., Jayprakash, J., et al. (2017). Conservation forestry and horticulture. Dehradun, India: ICAR-Indian Institute of Soil and Water Conservation.
Singh, G., Nagora, P. R., Haksar, P., and Chauhan, A. R. (2021). Utilizing treated wastewater in tree plantation in Indian desert: part I–species suitability, plant growth and biomass production. Int. J. Phytoremediation 24, 1–11. doi: 10.1080/15226514.2021.1993784
Singh, G., and Rathod, T. R. (2002). Plant growth, biomass production and soil water dynamics in a shifting dune of Indian desert. For. Ecol. Manag. 171, 309–320. doi: 10.1016/S0378-1127(01)00791-5
Singh, S. V., Singh, S., Kumar, P., and Tikkoo, A. (2009). Response of crop rotations to sodic waters and amendments in loamy-sand soil of southern Haryana. Ann. Arid Zone 47, 111–116.
Sirohi, C., and Bnagrawa, K. S. (2017). Effect of different spacings of poplar-based agroforestry system on soil chemical properties and nutrient status in Haryana, India. Curr. Sci. 113, 1403–1407. doi: 10.18520/cs/v113/i07/1403-1407
Sivakumar, M. V. K., Zobisch, M. A., Koala, S., and Maukonen, T. (1998). “Wind erosion in Africa and West Asia: Problems and control strategies.” in Proceedings of the ICARDA/ICRISAT/UNEPN/WMO Expert Group Meeting; 1997 April 22-25; Cairo, Egypt. (Aleppo, Syria: ICARDA), 198.
Soni, M. L., Beniwal, R. K., Talwar, H. S., Yadava, N. D., Singh, J. P., and Kumar, S. (2006). Root distribution pattern of two predominant grasses viz., Lasiurus sindicus and Cenchrus ciliaris of arid ecosystem of western Rajasthan in relation to their soil binding capacity. Indian J. Agric. Sci. 76, 716–720.
Soni, M. L., Subbulakshmi, V., Renjith, P. S., Dagar, J. C., and Yadava, N. D. (2018). “Reclamation of ravine lands for higher production” in Ravine lands: greening for livelihood and environmental security. eds. J. C. Dagar and A. K. Singh (Singapore: Springer Nature), 279–307.
Soni, M. L., Subbulakshmi, V., Yadava, N. D., Tewari, J. C., and Dagar, J. C. (2016). “Silvopastoral agroforestry systems: lifeline for dry regions,” in Agroforestry Research Developments. eds. J. C. Dagar and J. C. Tewari (New York: Nova Science Publishers), 245–305.
Soni, P., Vasistha, H. B., and Kumar, O. (1990). “Restoration technology for mined lands”, in Proceeding of the national seminar on technologies for afforestation of wastelands, (FRI, Dehradun), 6–8 Nov.
Soni, M. L., Yadava, N. D., Beniwal, R. K., Singh, J. P., Kumar, S., and Birbal, (2013). Grass based strip cropping systems for controlling soil erosion and enhancing system productivity under drought situations of hot arid western Rajasthan. Int. J. Agric. Stat. Sci. 9, 685–692.
Sridhar, K. R., and Bhagya, B. (2007). Coastal sand dune vegetation: a potential source of food, fodder and pharmaceuticals. Livest. Res. Rural. Dev. 19, 1–2.
Tarin, M. W. K., Fan, L., Xie, D., Tayyab, M., Rong, J., Chen, L., et al. (2021). Response of soil fungal diversity and community composition to varying levels of bamboo biochar in red soils. Microorganisms 9:1385. doi: 10.3390/microorganisms9071385
Tewari, J. C., Ram, M., Roy, M. M., and Dagar, J. C. (2014). Livelihood improvements and climate change adaptations through agroforestry in hot arid environments. Adv. Agrofor. 10, 155–183. doi: 10.1007/978-81-322-1662-9_6
The Energy and Resources Institute (2016). Economics of desertification, land degradation and drought in India Vol II: Six micro-economic case studies of degradation. Ministry of Environment, Forest and Climate Change, New Delhi. Available at: https://www.teriin.org/ (Accesses January 12, 2023).
Tomar, O. S., Minhas, P. S., Sharma, V. K., Singh, Y. P., and Gupta, R. K. (2003). Performance of 31 tree species and soil conditions in a plantation established with saline irrigation. For. Ecol. Manag. 177, 333–346. doi: 10.1016/S0378-1127(02)00437-1
Treseder, K. K., and Allen, M. F. (2000). Mycorrhizal fungi have a potential role in soil carbon storage under elevated CO2 and nitrogen deposition. New Phytol. 147, 189–200. doi: 10.1046/j.1469-8137.2000.00690.x
United Nations Environment Programme (1993). Good news in the fight against desertification. Desertification control bulletin no. 22. Available at: https://wedocs.unep.org/20.500.11822/29112 (Accessed January 15, 2023).
Upadhyaya, A. K. (1991). Shelterbelt plantations effectively check sand deposition in Indira Gandhi canal. Indian Forester 117, 511–514.
Uthappa, A. R., Bana, O. P. S., Kumar, M., and Kanwal, M. (2015). Soil physico-bio-chemical properties as influenced by varying tree densities in poplar (Populus deltoids Bartr. Ex marsh.) based agroforestry system. Indian J. Agrofor. 17, 81–90.
Utomo, B., Prawoto, A. A., Bonnet, S., Bangviwat, A., and Gheewala, S. H. (2015). Environmental performance of cocoa production from monoculture and agroforestry systems in Indonesia. J. Clean. Prod. 134, 583–591. doi: 10.1016/j.jclepro.2015.08.102
Vanlauwe, B., Aihou, K., Tossah, B. K., Diels, J., Sanginga, N., and Merckx, R. (2005). Senna siamea trees 650 recycle Ca from a Ca-rich subsoil and increase the topsoil pH in agroforestry systems in the west 651 African derived savannah zone. Plant Soil 269, 285–296. doi: 10.1007/s11104-004-0599-3
Venkatraman, K., and Ashwath, N. (2016). Transpiration in 15 tree species grown on a phytocapped landfill site. Hydrol. Curr. Res. 7:236. doi: 10.4172/2157-7587.1000236
Vreugdenhil, S. J., Kramer, K., and Pelsma, T. (2006). Effects of flooding duration, −frequency and -depth on the presence of saplings of six woody species in north-West Europe. For. Ecol. Manag. 236, 47–55. doi: 10.1016/j.foreco.2006.08.329
Wairiu, M. (2017). Land degradation and sustainable land management practices in Pacific Island countries. Reg. Environ. Chang. 17, 1053–1064. doi: 10.1007/s10113-016-1041-0
Warren, A. (2002). Land degradation is contextual. Land Degrad. Dev. 13, 449–459. doi: 10.1002/ldr.532
Wong, M. T. F., Gibbs, P., Nortcliff, S., and Swift, R. S. (2000). Measurement of the acid neutralizing capacity of agroforestry tree prunings added to tropical soils. J. Agric. Sci. 134, 269–276. doi: 10.1017/S0021859699007388
Wong, M. T. F., Hairiah, K., and Alegre, J. (2004). “Managing soil acidity and aluminium toxicity in tree-based agroecosystems” in Below-ground interactions in tropical agroecosystems: concepts and models with multiple plant components. eds. M. Noordwijk, C. G. Van, and C. K. Ong (UK: CABI Publishing), 143–156.
Wu, Y., Chen, D., Saleem, M., Wang, B., Hu, S., Delgado-Baquerizo, M., et al. (2021). Rare soil microbial taxa regulate the negative effects of land degradation drivers on soil organic matter decomposition. J. Appl. Ecol. 58, 1658–1669. doi: 10.1111/1365-2664.13935
Xie, H., Zhang, Y., Wu, Z., and Lv, T. (2020). A bibliometric analysis on land degradation: current status, development, and future directions. Land 9:28. doi: 10.3390/land9010028
Xu, H., Demetriades, A., Reimann, C., Jiménez, J. J., Filser, J., and Zhang, C. (2019). Identification of the co-existence of low total organic carbon contents and low pH values in agricultural soil in north-Central Europe using hot spot analysis based on GEMAS project data. Sci. Total Environ. 678, 94–104. doi: 10.1016/j.scitotenv.2019.04.382
Yadav, R. P., Prasad, R., Aggarwal, R. K., Agnihotri, Y., and Samra, J. S. (2005). Aonla (Embilica officinalis) based silvi-pastoral systems for soil and water conservation in degraded lands of Shivalik foothills. Indian J. Soil Cons. 33, 207–212.
Yadav, R. S., Yadav, B. L., Chhipa, B. R., Dhyani, S. K., and Ram, M. (2011). Soil biological properties under different tree based traditional agroforestry systems in a semi-arid region of Rajasthan. India. Agrofor. Syst. 81, 195–202. doi: 10.1007/s10457-010-9277-z
Zhang, X., Takano, T., and Liu, S. (2006). Identification of a mitochondrial ATP synthase small subunit gene (RMtATP6) expressed in response to salts and osmotic stresses in rice (Oryza sativa L.). J. Exp. Bot. 57, 193–200. doi: 10.1093/jxb/erj025
Keywords: acidic soils, carbon sequestration, mined area, ravine lands, soil erosion
Citation: Jinger D, Kaushal R, Kumar R, Paramesh V, Verma A, Shukla M, Chavan SB, Kakade V, Dobhal S, Uthappa AR, Roy T, Singhal V, Madegowda M, Kumar D, Khatri P, Dinesh D, Singh G, Singh AK, Nath AJ, Joshi N, Joshi E and Kumawat S (2023) Degraded land rehabilitation through agroforestry in India: Achievements, current understanding, and future prospectives. Front. Ecol. Evol. 11:1088796. doi: 10.3389/fevo.2023.1088796
Edited by:
Sudhakar Srivastava, Banaras Hindu University, IndiaReviewed by:
Meraj Alam Ansari, ICAR-Indian Institute of Farming System Research, Modipuram, IndiaSubhash Babu, Indian Agricultural Research Institute (ICAR), India
Copyright © 2023 Jinger, Kaushal, Kumar, Paramesh, Verma, Shukla, Chavan, Kakade, Dobhal, Uthappa, Roy, Singhal, Madegowda, Kumar, Khatri, Dinesh, Singh, Singh, Nath, Joshi, Joshi and Kumawat. This is an open-access article distributed under the terms of the Creative Commons Attribution License (CC BY). The use, distribution or reproduction in other forums is permitted, provided the original author(s) and the copyright owner(s) are credited and that the original publication in this journal is cited, in accordance with accepted academic practice. No use, distribution or reproduction is permitted which does not comply with these terms.
*Correspondence: Dinesh Jinger, ✉ dineshjinger28@gmail.com; Venkatesh Paramesh, ✉ parameshagron@gmail.com