- 1Cátedra de Química de Biomoléculas, Facultad de Agronomía, Universidad de Buenos Aires (UBA), Ciudad Autónoma de Buenos Aires, Argentina
- 2Instituto de Investigaciones en Biociencias Agrícolas y Ambientales, Consejo Nacional de Investigaciones Científicas y Tecnológicas (CONICET), UBA, Ciudad Autónoma de Buenos Aires, Argentina
- 3Instituto de Investigaciones Fisiológicas y Ecológicas Vinculadas a la Agricultura, CONICET, UBA, Ciudad Autónoma de Buenos Aires, Argentina
- 4Department of Biochemistry, Max Planck Institute for Chemical Ecology, Jena, Germany
- 5Cátedra de Bioquímica, Facultad de Agronomía, UBA, Ciudad Autónoma de Buenos Aires, Argentina
- 6Centro de Investigaciones en Hidratos de Carbono, Departamento de Química Orgánica, Facultad de Ciencias Exactas y Naturales, CONICET, UBA, Ciudad Autónoma de Buenos Aires, Argentina
Introduction: Insect oviposition can enhance plant defenses and decrease plant quality in response to future feeding damage by hatched larvae. Induced resistance triggered by egg deposition and its negative effect on insect herbivore performance is known for several annual plants but has been much less studied in woody perennials, such as species of the Salicaceae. Here we studied the response of the willow Salix babylonica to oviposition by the specialist willow sawfly Nematus oligospilus and its impact on insect performance.
Methods: We measured the effect of oviposition on larval feeding and pupa formation and evaluated its influence on plant phytohormones and volatile emission profile.
Results: We showed that oviposition reduced neonate larval growth and increased the proportion of prepupae that delayed their transition to pupae, thus extending the length of the sawfly cocoon phase. Oviposited willows increased jasmonic acid levels and changed their volatile profile through enhanced concentrations of the terpenoids, (E/E)-α-farnesene, (Z)- and (E)-β-ocimene. Volatile profiles were characteristic for each type of insect damage (oviposition vs. feeding), but no priming effect was found.
Discussion: We demonstrated that willows could perceive sawfly oviposition per se as a primary factor activating defense signaling via the jasmonic acid pathway. This induced response ultimately determined changes in pupation dynamics that may affect the whole insect population cycle.
1. Introduction
Plants are well known to increase defenses in response to the feeding of insect herbivores, and also in response to oviposition. Plant responses to insect oviposition include strategies focused on eliminating eggs, and the juveniles that hatch from those eggs. Direct mechanisms include defenses that remove or kill insect eggs, such as production of ovicides, necrosis, neoplasm formation and egg crushing or extrusion (Doss et al., 2000; Desurmont and Weston, 2011; Fatouros et al., 2012; Yang et al., 2013). Indirect mechanisms are based on egg or larval parasitoids attracted to the host plant through emission of oviposition-induced plant volatiles (OIPVs; Meiners and Hilker, 2000; Bruce et al., 2010). Last, in plant defense, priming is a physiological process by which a plant prepares to quicker or more aggressively respond to future biotic or abiotic stress (Frost et al., 2008). For several plant species it was also demonstrated that plants use eggs or even OIPV as a cue to prime defenses against the hatched larvae (Frost et al., 2008; Beyaert et al., 2012; Hilker and Fatouros, 2016; Pashalidou et al., 2020) or enhance the opposite effect by suppressing plant defenses (Bruessow et al., 2010). Oviposition-primed plants undergo an alteration of their quality as a food source and negatively influence the performance of emerging neonates. Evidence of these direct mechanisms against larvae has been found mostly for annual plants (various Brassicaceae, Geiselhardt et al., 2013; Pashalidou, 2015; Solanaceae, Kim et al., 2012; Bandoly et al., 2015; and Fabaceae, Rondoni et al., 2018) and rarely for woody perennials (elm: Austel et al., 2016; Schott et al., 2021 and pine: Beyaert et al., 2012).
Changes in plant volatile organic compounds (VOCs) emission are one of the most common responses of plants following insect oviposition, even before feeding larvae initiate damage. In several plant species, OIPVs changes consist of enhanced terpenoid and reduced green leaf volatile emission, leading to attraction of parasitoids that kill the eggs or their later juvenile stages (Hilker and Fatouros, 2015). There is also specificity in volatile release when plants are damaged by different developmental stages of the same herbivore species (McCormick et al., 2012). For example, OIPVs emission presents different volatile blends from those induced by larval feeding (Hilker and Meiners, 2011; Pashalidou et al., 2015b). Although previous studies have investigated herbivore-induced volatiles in Salicaceae (Yoneya and Takabayashi, 2013; McCormick et al., 2019; Valladares et al., 2020), little is known about the specificity of volatile emission according to herbivore stage of development. In addition, there is no information about defense responses of Salicaceae to oviposition.
Plant defensive responses against herbivores are often regulated by phytohormones such as jasmonic (JA), salicylic (SA), and abscisic acids (ABA; Erb and Reymond, 2019). JA and SA can play a role in mediating plant responses to hatching larvae after oviposition (Meiners and Hilker, 2000; Mumm et al., 2003; Little et al., 2007; Bruessow et al., 2010). The activation of defensive phytohormone signaling pathways in egg-induced anti-herbivore defense has been demonstrated in some Brassicaceae (A. thaliana and B. nigra, e.g., Bonnet et al., 2017; Valsamakis et al., 2020) Solanaceae (N. attenuata, and S. lycopersicum, e.g., Kim et al., 2012; Bandoly et al., 2015), and two woody plants (pine, Bittner et al., 2019; and elm, Büchel et al., 2012; Schott et al., 2021). In Populus spp. (Salicaceae) insect feeding increased levels of ABA, JA, and its bioactive derivative JA-isoleucine locally in damaged leaves, which induced VOC emission, protease inhibitor, gene expression, and condensed tannin levels (Boeckler et al., 2013; McCormick et al., 2019; Eberl et al., 2021). To our knowledge, however, no study has reported the effects of oviposition on the phytohormonal responses of the Salicaceae.
The willow sawfly Nematus oligospilus Förster (Hymenoptera: Tenthredinidae) feeds exclusively on leaves of the genus Salix L. (Salicaceae) worldwide (Dapoto and Giganti, 1994; Urban and Eardley, 1995; Charles and Allan, 2000). This sawfly reproduces by parthenogenesis in the Southern Hemisphere, including in Argentina, where it is an exotic species (Urban and Eardley, 1995). Upon emergence from mid-spring to end of summer, females assess oviposition sites on leaves and lay individual eggs without feeding (Dapoto and Giganti, 1994). Sawfly egg-laying, unlike that of most lepidopterans, causes damage to leaf tissue (Bertea et al., 2020). Through a highly stereotyped behavior, the saw-shaped ovipositor of the sawfly is distended to lift the leaf cuticle and to saw a flat pouch where the female lays a single egg inside the leaf and covers it with an oviduct secretion (Braccini et al., 2013; Fernández et al., 2019). Eggs hatch after 5 days and each neonate establishes a feeding hole, which always occurs at a distance from the eggshell. This neonate behavior was observed both in the lab, under field conditions, and across different willow species (personal observation), which raised the idea that newborns are avoiding defenses induced around the egg. Since the oviposition process of N. oligospilus makes an injury, we hypothesize that oviposition damage is perceived by Salix spp. leaves and induces chemical defenses. In N. oligospilus, the mature larval stage occurring inside the cocoon has two phases: prepupa (i.e., last instar larva which does not feed) and pupa (when the insect acquires adult morphology, including visible legs and antennae, Ovruski and Smith, 1993; Battisti, 1994). The following spring, prepupa will transform to pupa, and then to adult (Haack and Mattson, 1993; Smith, 1993; Charles and Allan, 2000). The prepupa is a particular sawfly stage in which it can remain dormant in soil during winter, but its duration is sensitive to rearing conditions (Alderete, 2005).
In order to study Salix spp. responses to sawfly egg laying and its consequences for later larval feeding and pupa formation, three issues were addressed. The effects of N. oligospilus oviposition were investigated on (i) insect performance, (ii) phytohormone accumulation in willow leaves, and (iii) OIPVs emission from foliage. We hypothesized that willow plants respond to oviposition through phytohormonal induction affecting larval performance and releasing OIPVs. Since oviposition is a highly reliable cue predicting herbivory, we also expected that previous oviposition prepares the plant to respond to subsequent larval feeding. Thus, egg presence could act as a priming stimulus which enhances feeding-induced VOCs emission. We found that previous oviposition decreased the larval performance of N. oligospilus, mainly by increasing the proportion of prepupae that delayed their transition to pupae. Oviposition per se induced the accumulation of JA and OIPVs emission. The VOCs blend of leaves damaged by larval feeding differed between leaves with prior oviposition and those without eggs. Thus, no clear evidence was found for sawfly oviposition priming volatile emission on subsequent herbivory.
2. Materials and methods
2.1. Plants and insects
Willow cuttings from the National Program of Genetic Improvement for Salicaceae (willow genetic improvement effort of the Instituto Nacional de Tecnología Agropecuaria, INTA, Argentina) were used. To perform the bioassays, the commercial willow genotype Salix babylonica var. Sacramenta (‘Soveny Americano’) was chosen. In order to get cuttings for all laboratory experiments, cuttings (30 cm long) from S. babylonica were planted in consecutive rows during late winter of 2016 and 2017, thus forming a nursery grown in the experimental station of the Faculty of Agronomy of the University of Buenos Aires (Buenos Aires, Argentina). In October 2017 and 2018, cuttings of S. babylonica were planted individually in pots and kept outdoors under natural conditions. After 4 months of growing, potted plants were subjected to bioassays. All tested potted plants were approximately 1.5 m tall and had approximately 100–150 leaves. Both nursery willows and potted plants were protected from insect damage (i.e., leaf-cutting ants) by placing ant baits in their surroundings. Potted plants were moved from outdoors to a controlled environment chamber (25°C, L16:D8, light intensity 350 μmol m−1 s−1) 24 h prior to treatments and kept under those conditions until completion of the experiments. Leaves were checked for insect damage before being placed in the chambers.
Adult N. oligospilus females from a laboratory population were used in all bioassays. Every spring, the population was started from larvae and pupae collected in a field with a history of sawfly infestation in the lower Delta of the Paraná River (34° 10′ 23.08″ S, 58° 45′ 57.67″ W). Larvae were reared until pupation on fresh cut twigs of Salix alba L. × S. alba in transparent plastic boxes (46 × 30 × 32 cm) in a controlled environment chamber at 25°C and L16:D8 h. photoperiod. Under these conditions, egg development takes 5 days. Pupae were removed from the boxes and held in separate plastic jars until adult females emerged. During the growing season, the laboratory population was renewed several times with field-collected material to minimize selection for laboratory-adapted insects. Larvae remained in the same climate chamber throughout their entire larval development until pupation.
2.2. Experimental setup and plant samplings
2.2.1. Experimental setup I
This setup was designed to study the effects of prior conspecific oviposition on N. oligospilus performance and VOC emission upon subsequent larval feeding.
For both larval performance and volatile emission studies, the same plant groups were used, subjected to the same experimental scheme with two sequential treatments: oviposition and feeding damage (Figure 1). These experiments were conducted with matched pairs of willows starting on different, consecutive weeks in the summer season (i.e., each week was considered a blocking factor accounting for treatment variability between each plant pair). Within each blocking factor we used a repeated-measures design with the following experimental groups: NO = no oviposition-no feeding by N. oligospilus larvae, O = oviposition by N. oligospilus-no larval feeding, O + F = prior oviposition followed by larval feeding and NO + F = no oviposition but feeding by N. oligospilus larvae.
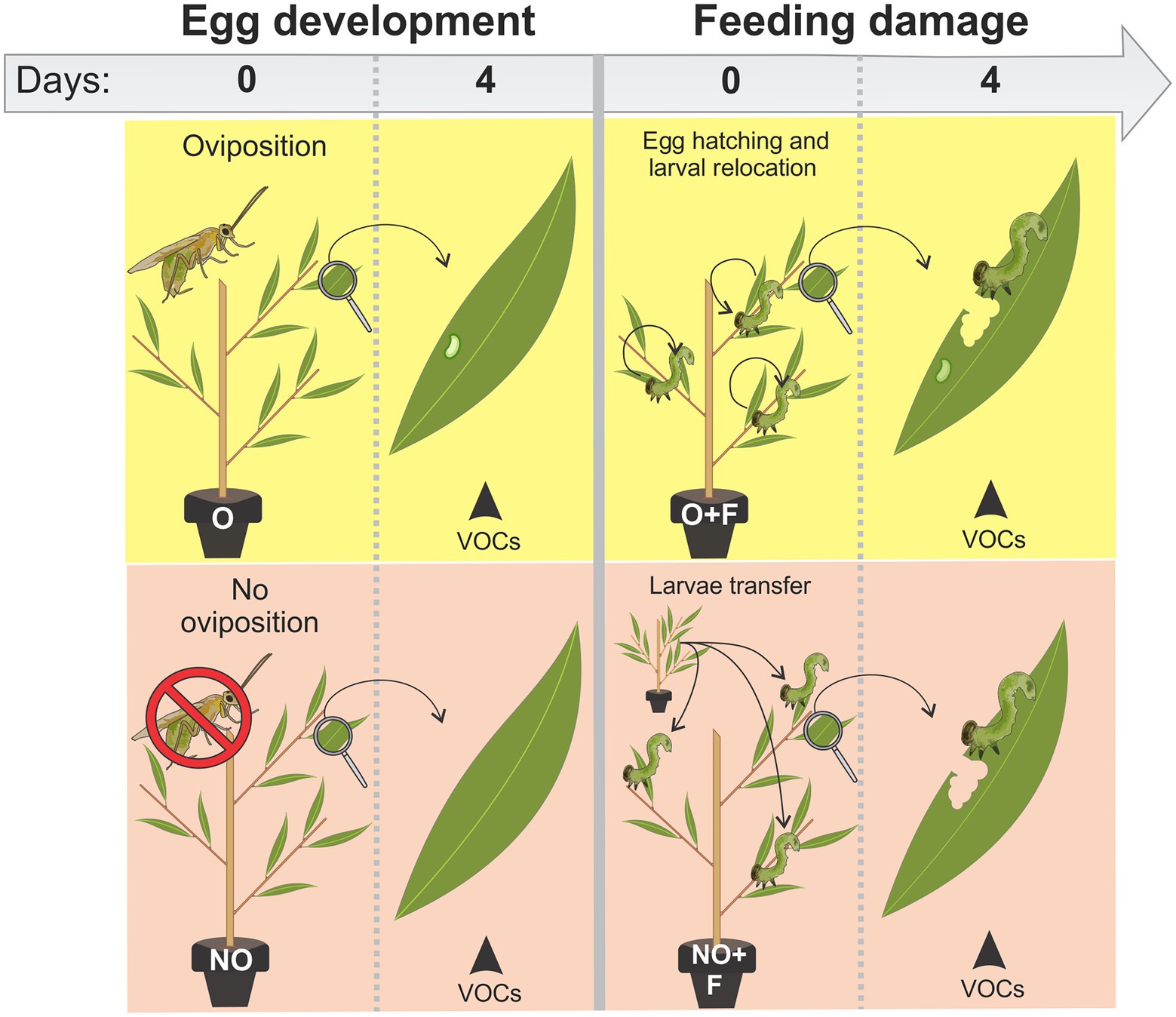
Figure 1. Experimental setup I scheme to evaluate insect performance and plant volatile emission. Plants were subjected to two sequential treatments: oviposition (4 days of egg development) and feeding damage (4 days of larval feeding). A first group of willows were subjected to prior oviposition (O). A second group was left without eggs (no oviposition treatment, NO). O and NO plants were subjected to feeding damage by neonates from their hatching day on (Day 0 of feeding damage). O + F plants were damaged with neonate larvae hatched from their own egg depositions. NO + F plants carried neonates transferred from oviposited non-experimental willows. VOCs arrows indicate the time when volatiles emission was measured.
To obtain oviposited plants (O, Figure 1), each intact potted willow enclosed in a voile bag was exposed to 15–20 females that were allowed to lay eggs for 1–5 h (until 80% of the leaves were laden with at least one egg). Each oviposited plant had a total of 200–250 eggs (ca. 3.6 ± 0.2 eggs per leaf). This procedure allowed us to obtain a comparable egg load among plant replicates and also ensured synchronously hatching to follow larval performance.
To obtain feeding damaged plants (O + F and NO + F plants, Figure 1) newborn larvae were allowed to feed for 4 days. A total of 100 ca. neonates were placed on egg-free leaves of previously non-oviposited plants, one per leaf (NO + F plants, Figure 1). These larvae had hatched from eggs laid on non-experimental S. babylonica willows and transferred to NO + F as soon as they hatched (i.e., even before their first bite). Simultaneously, another ca. 100 neonates newly hatched from eggs laid on O plants, were taken out and immediately placed next to their hatching site onto the leaf that previously had carried eggs (O + F, Figure 1). This procedure ensured that all tested larvae, experienced the same manipulation using a soft brush and all plants were exposed to the same number of feeding larvae. The remaining neonates which hatched from O + F plants were discarded. In this case, we used fewer larvae than the number of eggs allowed in the previous measurement to avoid complete defoliation.
Volatile collection was performed on each experimental group: after 4 days of egg development (O and NO, Figure 1) and after 4 days of larval feeding (O + F and NO + F, Figure 1). In those plants which had been exposed to feeding damage (O + F and NO + F, Figure 1), larvae were removed an hour before volatile collection. In the meanwhile, larvae were transferred to transparent plastic boxes containing fresh cut twigs (10 cm length) of undamaged S. babylonica and kept there during volatile sampling (6 h) in another climate chamber. After finishing collection, larvae were returned to their original O + F and NO + F willow sapling to continue the performance study.
2.2.2. Experimental setup II
A second experimental setup was designed to study the effect of N. oligospilus oviposition on phytohormonal accumulation in S. babylonica leaves. Individual leaves were selected from potted willows, enclosed in a voile bag, offered to 3 N. oligospilus females for egg-laying (oviposited leaves) and harvested 4 days after oviposition with 20–60 eggs laid per leaf. Since this sawfly causes a tiny leaf wounding during oviposition, a higher egg load per leaf was used to increase the probability of phytohormonal response. Simultaneously, potted willows were left without oviposition (egg-free control). In all cases mature leaves at an equivalent position were harvested at the same time (i.e., two per control and two per oviposited plant). These experiments were conducted with matched pairs of willows starting on different, consecutive days in the summer season (i.e., each day was considered a blocking factor).
Due to the fact that eggs are laid inside the leaf epidermis (Braccini et al., 2013), they cannot be removed without tearing the leaf. Thus, oviposited leaves samples were processed together with the eggs inside. To test the possibility that eggs contain a level of phytohormone that could interfere with the leaf analysis, three egg samples were obtained directly from the abdomen of newly emerged females to quantify their chemical content. Sawflies were dissected under a stereoscopic microscope (Olympus SZ61, Tokio, Japan) to extract the ovaries and isolate the oocytes. Each egg sample consisted of 400–1,300 eggs approximately from 10 to 30 females. Leaves and female oocytes were flash frozen in liquid nitrogen and stored at −80°C until phytohormone analysis.
2.3. Insect performance
A group of 10–12 neonate larvae per willow sapling were randomly chosen from O + F and NO + F plants and the following variables were determined: 1-day larval growth, larval and prepupal development time, larval mass, pupal mass, proportion of late prepupal stage and larval survival (Figure 2).
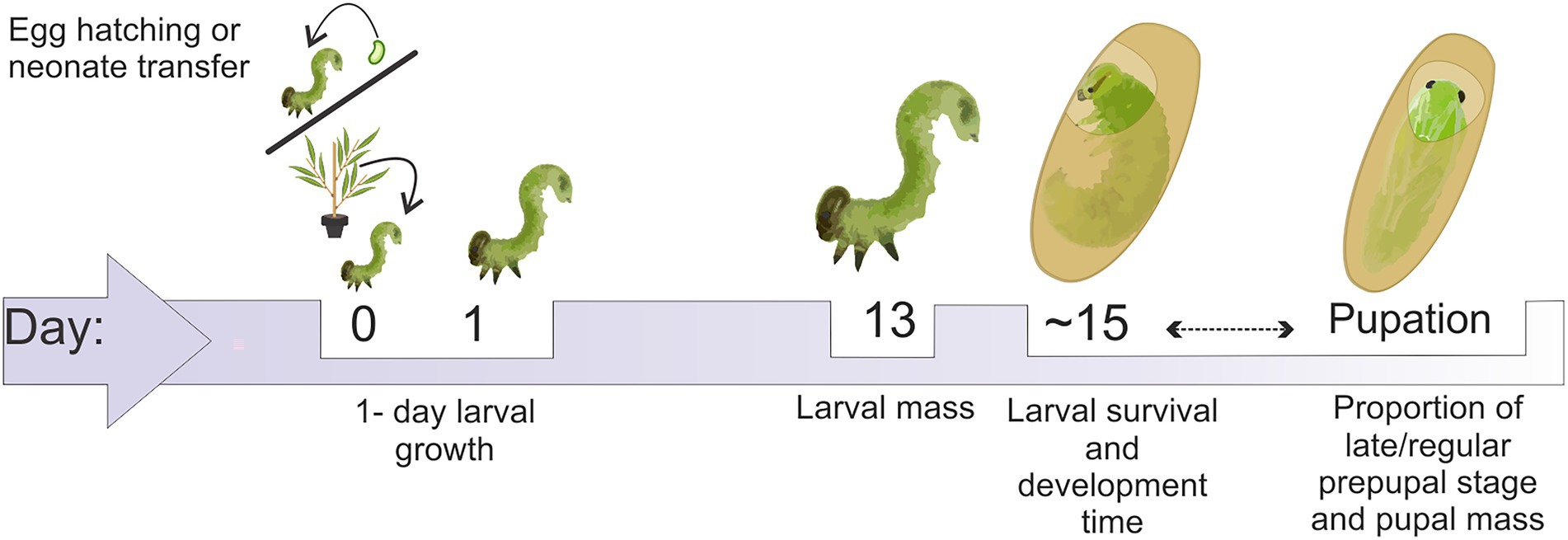
Figure 2. Variables of N. oligospilus performance determined on oviposited and non-oviposited willows. Numbers indicate the larval development day in which the measurement was registered. Day 0 refers to egg hatching or neonate transfer from non-experimental plants. Prepupal development time (double headed arrow), is the time period between cocoon spinning (ca. day 15) and day of pupa formation (Pupation).
To estimate 1-day larval growth, the neonate larval size was measured one and 24 h after transfer to NO + F plants (Figure 2). An indirect size measurement was chosen because neonate larvae are small and delicate so that their manipulation for weighing causes their death (Valladares et al., 2020). The same larvae as newly emerged and as 1 day-old were photographed with a digital camera (Canon EOS 700 D Rebel T5i) adjusted to a stereoscopic microscope (Olympus SZ61, Tokio, Japan). In the visual field a millimeter scale was placed to calibrate the measurement. Larval area was determined by dorsal view of the body which included the head, thorax and abdomen, excluding the legs. This was quantified by the image processing software, ImageJ version 1.45 s (National Institute of Health, Bethesda, Maryland). Finally, 1-day larval growth was calculated as the difference between larval area, one and 24 h after transfer.
After 13 days of feeding on prior oviposited (O + F) and non-oviposited plants (NO + F), larvae were transferred to transparent plastic boxes (33 × 23 × 14 cm) containing fresh cut twigs (10 cm length) of undamaged S. babylonica, placed in small jars with tap water. The twigs were replaced by fresh ones every other day. Larvae continued their development inside the boxes, until the cycle was completed.
Larval development time was estimated as the number of days from egg hatching to onset of cocoon spinning (Figure 2). Larval mass was measured at the 13th day of development (near cocoon stage) by weighing on a scale (Figure 2). Larval survival was estimated as the proportion of larvae that complete pupation (i.e., number of transferred or hatched neonates/number of cocoons, Figure 2).
Prepupal development time was measured as the number of days prepupae took to pupate (the time lapse between the spinning of the cocoon by the last instar larvae and the acquiring of adult morphology inside the cocoon, Figure 2). The cocoon phase normally lasts 7–10 days (including prepupal and pupal stages, after rearing on S. babylonica at 25°C, Alderete, 2005), but there is a percentage of prepupae that took longer during our assay (more than 10 days). Due to this fact, all prepupae were classified into regular (equal or less than 10 days in cocoon phase) and late prepupae stage (more than 10 days in that stage). Proportion of late prepupal stage was estimated as the number of late prepupae/total prepupae. To quantify pupal mass, individual pupae were first taken out of their cocoon and weighed. Pupal developmental time was not measured since pupae are unable to survive outside the cocoon after being weighed.
2.4. Phytohormone analysis
Leaf tissue and female oocytes from experimental setup II were lyophilized and ground to fine powder by agitation on a paint shaker (Skandex SO-10 M, Fluid Management Europe, Netherlands). A 10 mg portion of each sample was extracted with 1 ml of pre-cooled methanol containing the following internal standards [D6-abscisic acid (Santa Cruz Biotechnology, Dallas, TX, United States; 40 ng ml−1, D4-salicylic acid (Santa Cruz Biotechnology; 40 ng ml−1), D6-jasmonic acid (HPC Standards GmbH, Cunnersdorf, Germany; 40 ng ml−1)]. Samples were shaken for 30 s with a paint shaker. Then, they were centrifuged at 2000 g for 5 min, and 400 μl of the supernatant were transferred into a new tube. The rest of the supernatant was carefully removed from the solid phase using a pipette. Subsequently, 1 ml of fresh methanol (without labeled standards) was added to the solid phase before repeating the extraction procedure (shaker + centrifuge). Again, 400 μl of the supernatant was collected and combined with the supernatant of the first extraction. The extracts were stored at −20°C until measurement. Phytohormones were analyzed by using a high-performance liquid chromatography (Agilent 1,100, Agilent Technologies, Waldbronn, Germany) coupled to a mass spectrometer (API 5000 LC/MS/MS System, AB Sciex, Framingham, MA, United States). The analytes were separated on a C18 column (XDB-C18, 50 × 4.6 mm × 1.8 μm, Agilent, Santa Clara, CA, United States) using a formic acid (0.05% in water)/acetonitrile gradient (flow: 1.1 ml min−1) and detected via multiple reactions monitoring (MRM) in negative ionization mode (ion spray at −4,500 eV at 700°C) as described in Vadassery et al. (2012). Data were processed using Analyst 1.5.2 (Applied Biosystems, Foster City, CA, United States), and hormones were quantified according to the peak area of their corresponding standard.
2.5. Volatile collection and chemical analysis
Plants from experimental setup I, were illuminated from above with blue and red LED lamps and a high-pressure metal halide lamp (light intensity 350 μmol m−1 s−1). Headspace samples were taken by enclosing the whole plant (approximately 1 m tall) into a polyethylene (PET) bag (Toppits oven bags, Minden, Germany). Charcoal-filtered air was pushed into the PET bag with an aquarium air pump and then pulled out by a suction pump (Tuff, model Standard, Bedford, United Kingdom) at a constant rate of 0.9 l per min. Air leaving the bag through an outlet passed through a volatile collection trap made of 30 mg HayeSep Q adsorbant (Grace, Deerfield, IL, United States). After a sampling period of 6 h (between 10:00 and 16:00 h), the volatile collection traps were wrapped in Teflon tape and foil and stored in the freezer until elution. Empty PET bags were used as blanks to exclude contaminants.
Volatile compounds were eluted from the filters with 150 μl of dichloromethane containing 5 ng μl−1 of dodecane as internal standard. Volatile samples were analyzed by coupled gas chromatography–mass spectrometry (Agilent 7890 instrument coupled to Agilent 5977 selective mass detector). A DB5-MS capillary column was used (0.25-mm i.d., film thickness 0.25 μm). Samples (1 μl) were injected at 240°C in splitless mode. Helium was used as carrier gas at 0.7 ml per min (inlet pressure: 20.48 kPa). The column temperature was held at 35°C for 1 min, and then increased at a rate of 10°C per min until it reached 230°C. Finally, the temperature was held at 230°C for 15 min. Compounds were identified by computer matching with our own mass spectra libraries and published data (Adams, 2007); comparison of their Kovats retention index on a DB5-MS column and by comparison of retention times with authentic standards: α-pinene, limonene, (E)- and (Z)-β-ocimene, (E)-β-caryophyllene, α-humulene, (E,E)-α-farnesene, and the alkane series C8 to C25 (Sigma, Aldrich). Data were collected with ChemStation software (Hewlett-Packard), and the detected volatiles were quantified on the basis of their peak area from total ion chromatogram in comparison with the area of the internal standard. Compounds were expressed as the mass equivalent of the internal standard (ng μl−1). Previously, blank values were subtracted from those of each compound in the samples.
2.6. Statistical analysis
The effect of oviposition on phytohormone concentrations in willow leaves was performed with a linear mixed model (LMM) with normal distribution. In case of heteroscedasticity, the variance structure was modeled with varIdent function in nlme package (Pinheiro and Bates, 2022). Oviposition factor (Oviposition/Control) was placed as fixed effect, and day as random effect (blocking factor). To evaluate if prior oviposition affected the performance of N. oligospilus, a LMM was performed on four of the parameters of the larvae: 1-day larval growth, larval development time, larval and pupal mass. In the analysis of the proportion of late prepupal stage and larval survival, a generalized linear mixed effect model (GLMM) with binomial distribution and logit as link function was performed. The models were built using glmmTMB function, in glmmTMB package (Brooks et al., 2017). For all performance parameters, Oviposition treatment (O/NO) was taken as fixed part of the model and week (blocking factor) and plant (to avoid pseudoreplication) as nested random effects. The histogram and density plot of distribution of prepupal developmental time was built using ggplot2 package (Wickham, 2016) and the calculation of the average development days of regular and long prepupae was made with plyr package (Wickham, 2011). All above mentioned data in LMMs were graphically screened for normal distribution (Q-Q-plots) and for homoscedasticity (Residual scatterplots) and tested for residual normality (Shapiro–Wilk).
To determine differences between individual compounds and total volatiles, a GLMM with repeated measures design was performed. The model consisted of a gamma distribution with log link function. Goodness of fit for gamma was previously tested with goft package (González-Estrada and Villaseñor, 2018). Oviposition (OP) and feeding damage (FD) treatments, and their interactions were placed as fixed effects; week and plant as nested random effects. Multiple comparisons among treatments were performed with Tukey’s HSD post hoc test using lsmeans package (Lenth, 2016). To identify a pattern in volatile blends related to plant treatments (OP and FD), relative concentrations of all volatile components were subjected to a multivariate correspondence analysis for all treatments with FactoMineR (Lê et al., 2008) and factoextra packages (Kassambara and Mundt, 2016). Those main components that explain the variability in a significant way were selected for analysis. Volatile compounds that were not detectable, were assigned a value of zero in the corresponding sample. All aforementioned analyses were performed in the statistical software R 1.3.1093 (Rstudio Team, 2020).
3. Results
3.1. Effects of prior oviposition on insect performance
Prior oviposition affected N. oligospilus performance at two life stages: neonate and prepupae. One day-old larvae that had started feeding on plants with prior oviposition (O + F) grew less than those that fed on plants without oviposition (NO + F; F = 13.7, p = 0.003, Figure 3A). The average mass of mature larvae (i.e., 13 days) from the O + F treatment was marginally smaller than that from the NO + F treatment, but did not differ significantly (F = 3.05, p = 0.08, Figure 3B). There were also non-significant differences in pupal mass (F = 1.2, p = 0.28, Figure 3C) and larval development time (F = 0.004, p = 0.95, Figure 3D). Prepupal development time showed a bimodal frequency distribution that was strongly influenced by oviposition history (Figure 4). The major mode consisted of prepupae with regular development times (average of 4.16 ± 1.28 days, Figure 4), while the minor mode consisted of late prepupae with an average development time eight-fold longer than that of the regular prepupae (32.27 ± 12.36 days) Noteworthy, the proportion of late prepupae was significantly higher if larvae started their development on O + F instead of NO + F plants (χ2 = 7.30, p = 0.007, Figure 3E). Previous oviposition on willows did not affect larval survival (χ2 = 0.62, p = 0.45, Figure 3F).
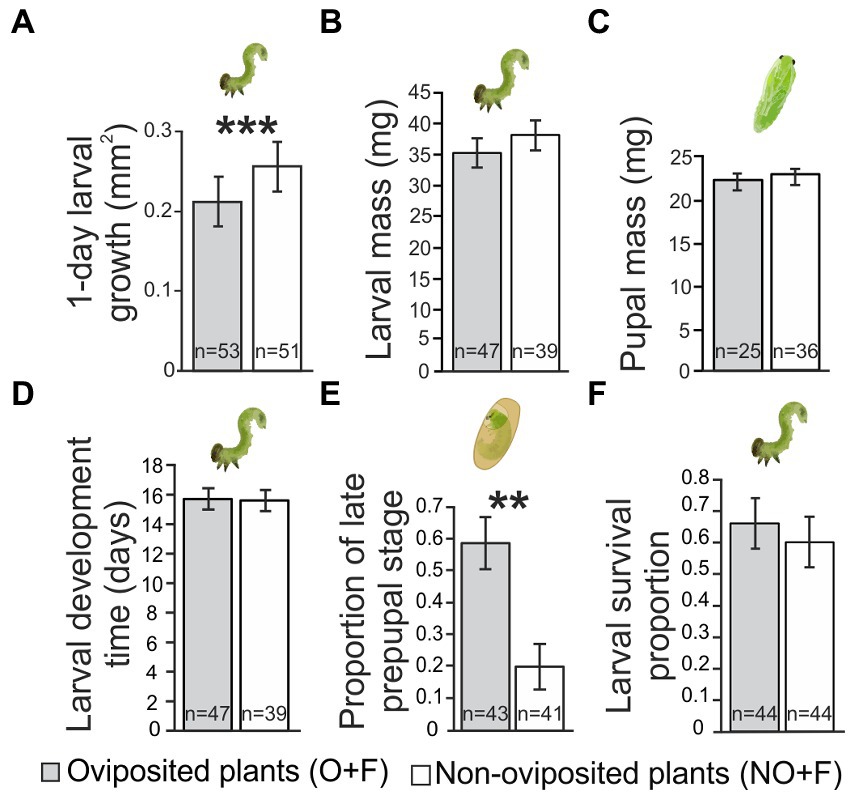
Figure 3. Performance of N. oligospilus on S. babylonica that had been subjected to prior oviposition (gray bars) or no oviposition (white bars; A–F). Means ± estimated standard errors are shown for all values. Statistical analysis for mean values was performed by LMMs, and proportions by Wald χ2 test. Asterisks (**/***) indicate significant differences between larvae on O + F and NO + F plants at p < 0.01/0.001. Total number of larvae per treatment is shown inside each bar. Biological replicates (plants) per treatment: N = 5.
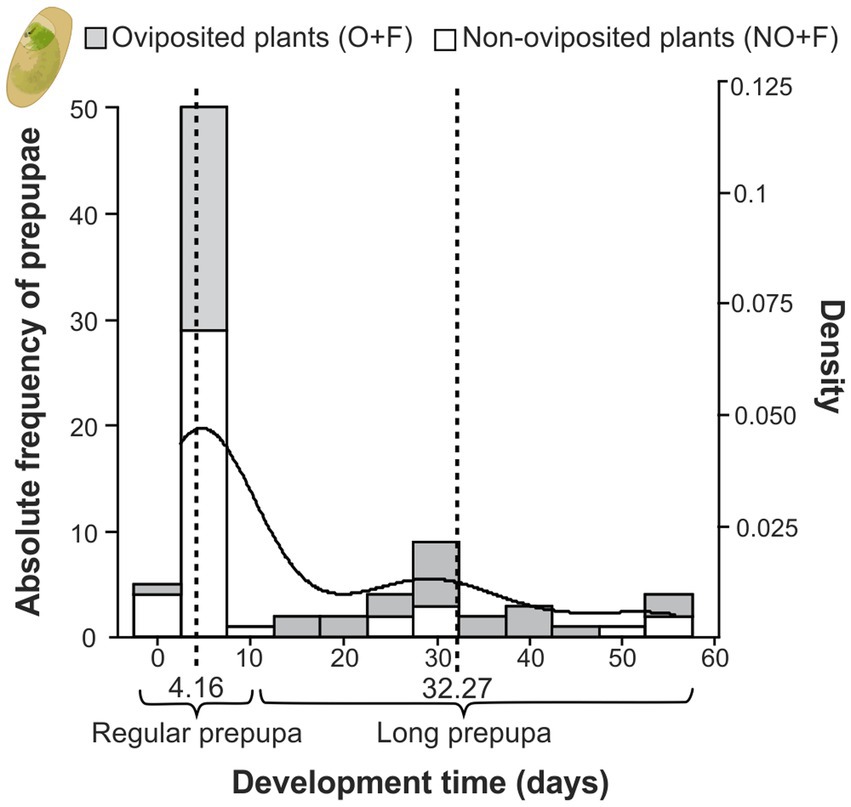
Figure 4. Histogram and density plot of distribution of prepupal developmental time. Absolute frequency of prepupae that developed from willows with prior oviposition (gray bars) or without oviposition (white bars) is shown in each bin of development time. Mean development time for regular and long prepupae is indicated with vertical dotted lines. The estimated probability density function (solid line) shows the bimodal frequency distribution of the data.
3.2. Effect of oviposition on willow phytohormones
Among the phytohormones analyzed, only the JA levels were increased in response to oviposition after 4 days from egg-laying (F = 19.11, p = 0.01, Figure 5). Average SA and ABA concentrations did not significantly differ between oviposited and non-oviposited leaves (F = 0.48, p = 0.5 and F = 1.07, p = 0.36, respectively). To rule out that eggs contain phytohormone levels that could interfere with the study, female oocytes were analyzed. Egg samples also contained SA, ABA and JA, but their mean concentrations were 6, 8 and 100 times lower than leaf control samples, respectively, (means and standard error levels: 77.5 ± 2.6, 3.5 ± 0.5, 0.7 ± 0.3 ng g−1 dry weight). Considering that each egg sample was prepared from approximately 2000 eggs, the contribution of eggs to leaf phytohormonal quantification must have been negligible.
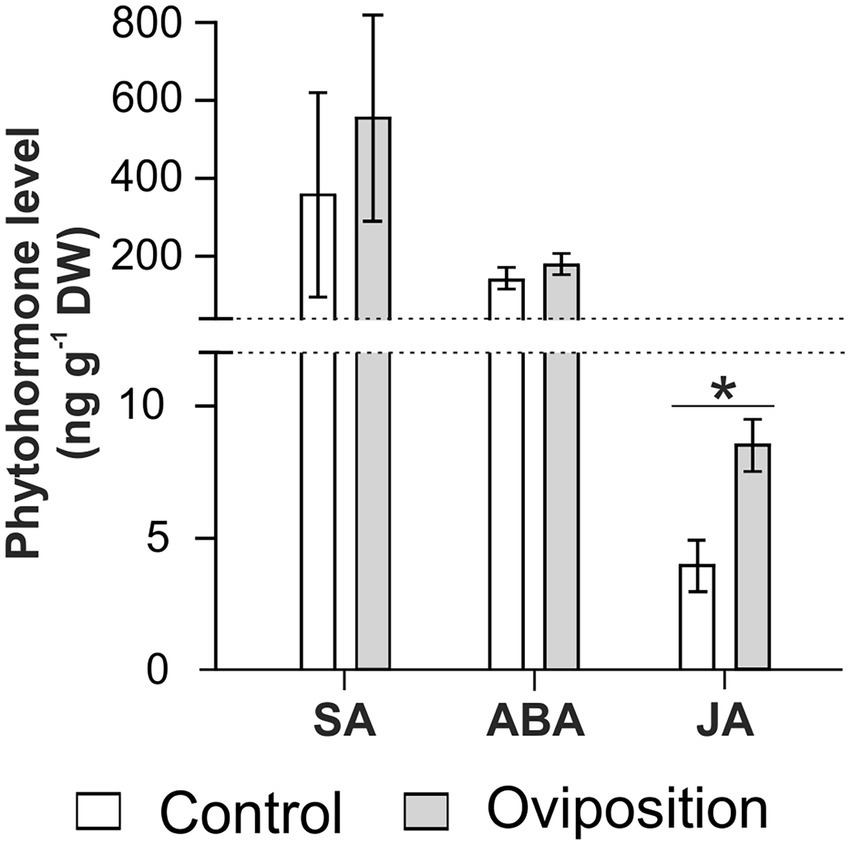
Figure 5. Phytohormone levels (means ± estimated SEM) in control and oviposited Salix babylonica leaves 4 days after Nematus oligospilus egg-laying. Concentrations of salicylic acid (SA), abscisic acid (ABA) and jasmonic acid (JA) in egg-free (control: white bars) and oviposited leaves (oviposition: gray bars). Statistical analysis for mean values was performed by LMMs between treatments. Asterisk (*) indicates significant difference between oviposited and control leaves for JA, (p < 0.05). Non- significant differences were found for the rest of the compounds. Biological replicates (plants) per treatment: N = 4.
3.3. Effect of oviposition and feeding damage on volatile emission
A total of 15 volatile compounds were identified from the willow plants studied (Table 1), all of which are of terpenoid origin, both mono- and sesqui-terpenes. After feeding damage, the same compounds were detected in both previously oviposited willows (O + F) and those without oviposition (NO + F).
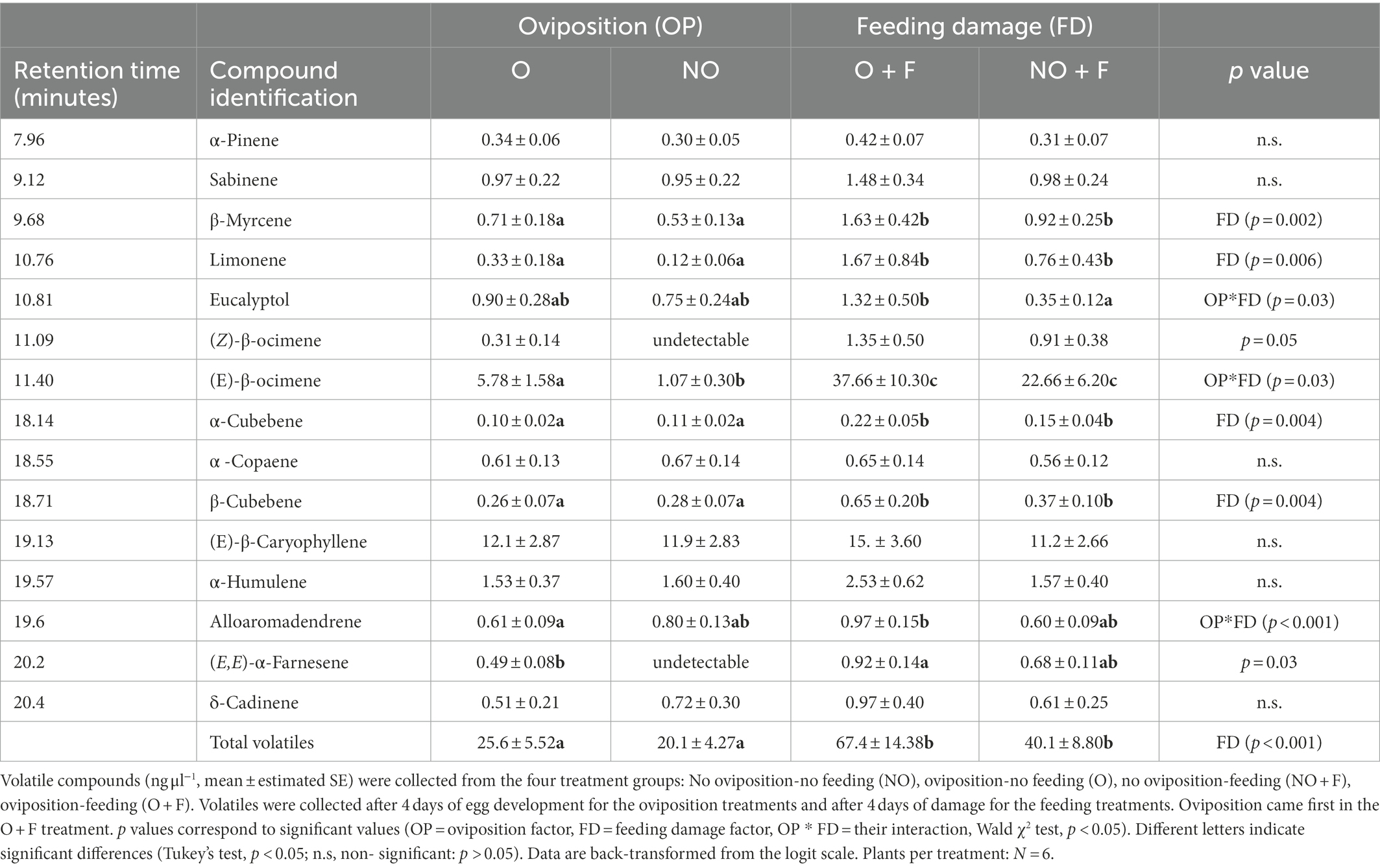
Table 1. The effect of N. oligospilus feeding and oviposition on volatile emission from S. babylonica.
Oviposition per se significantly increased the amount of (E)-β-ocimene compared to non-oviposited willows (Tukey’s test, p = 0.002, O, Table 1). Additionally, (Z)-β-ocimene and (E,E)-α-farnesene, were undetectable from non-oviposited plants, but only emitted as a result of oviposition (respectively NO and O, Table 1). After feeding damage, (E)-β-ocimene levels increased significantly regardless of previous oviposition history (O + F and NO + F, Table 1). Four other compounds (β-myrcene, limonene and α- and β-cubebene, Table 1), also significantly increased after feeding damage (FD, p < 0.01, Table 1). Even though the interaction was highly significant for eucalyptol and alloaromadendrene, the Tukey test showed no differences between the means of each treatment for these compounds (OP*FD, p < 0.01, Table 1). The rest of the volatile compounds were not changed by treatment (n.s., Table 1). Total volatile emission significantly increased in response to feeding damage, but there was no effect of previous oviposition history (FD, p < 0.001, Table 1). Thus, oviposition had no priming effect on volatile emission.
Relative concentrations of volatiles were subjected to a correspondence analysis, a multivariate technique to visualize differences among treatments. Results showed that the main pattern of volatile component variability was influenced by both plant treatments, oviposition and feeding damage, with the first axis explaining 81.8% of total inertia (Figure 6). Axis 1 contrasts feeding damage (O + F and NO + F, Figure 6) with oviposition (O and NO, Figure 6). The plot shows a similar volatile emission profile for both feeding damage treatments regardless of previous oviposition (O + F and NO + F, Figure 6). However, without feeding, oviposited and non-oviposited plants exhibited different profiles in relation to each other and also in comparison with both feeding damage treatments (O and NO, Figure 6).
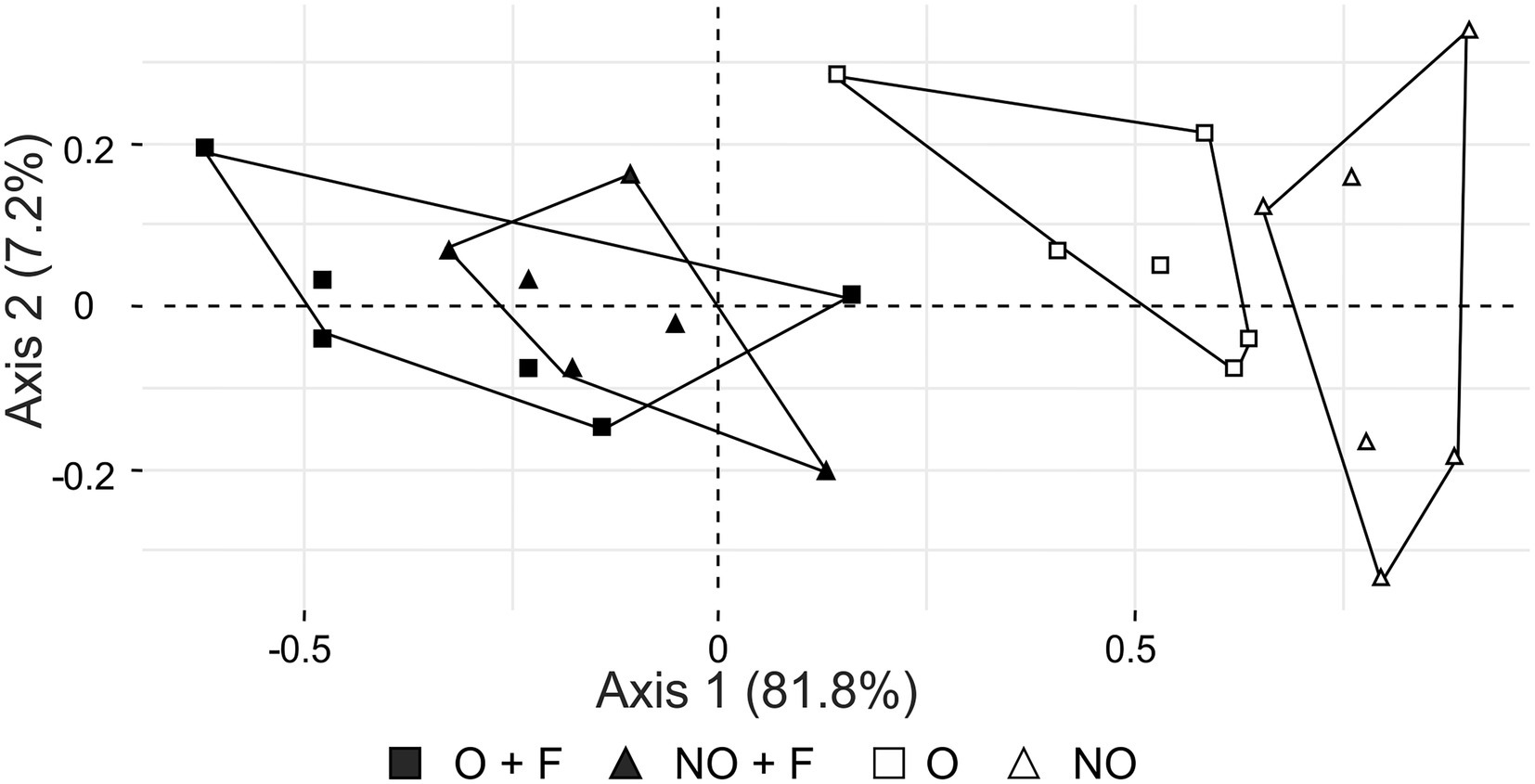
Figure 6. Correspondence analysis showing the effects of N. oligospilus oviposition and feeding on the volatile emission profiles of willows. Ellipses represents a plot of the convex hull of the samples of each treatment: (△NO) no oviposition-no feeding, (□O) oviposition-no feeding, (▲NO + F) no oviposition-feeding and (■O + F) oviposition-feeding. Oviposition always preceded feeding. Percentage of explained inertia is expressed in parentheses.
4. Discussion
We showed that oviposition of a specialist hymenopteran herbivore, Nematus oligospilus, on Salix babylonica foliage changed foliage quality for future herbivore feeding, impairing larval growth and development (Figures 3, 4). These changes may be triggered by various defensive signals. Oviposition increased JA levels (Figure 5) and altered foliage volatile emission profile. Yet feeding damage caused even more dramatic changes in volatile emission than oviposition (Table 1 and Figure 6).
The performance of insect herbivores can be negatively affected when they feed on plants with prior egg deposition. Yet most previous studies on this phenomenon have been carried out with herbaceous rather than woody plant species (Austel et al., 2016; Hilker and Fatouros, 2016; Lortzing et al., 2020). Here, sawfly larvae that fed on willows where they hatched from eggs exhibited poorer performance compared to larvae that fed on plants without prior oviposition. This was observed at the beginning of larval development with decreased neonate growth and marginally lower larval biomass (Figure 3). The prepupa was the most affected stage as evidenced by the long delay in transition to the pupa (Figures 3, 4). In earlier work, feeding on leaves with previous egg deposition generally decreased insect performance at the larval stage causing higher larval mortality, reduction of larval weight and an extension of larva-to-pupa development time (Austel et al., 2016; Bandoly et al., 2016; Hilker and Fatouros, 2016; Lortzing et al., 2019; Pashalidou, 2015; Pashalidou et al., 2015a; Voirol et al., 2020). Notably, here we found that during the cocoon phase, a high proportion of the prepupae showed an 8-fold increase of their development time, lengthening life cycle by up to two-fold. Interestingly, similar results were found for N. oligospilus larvae reared on senescent S. babylonica leaves instead of mature ones, where mortality of first instar larvae also increased and the length of the prepupa development time doubled (Alderete, 2005). Considering that senescent leaves are of lower nutritional quality due to decreases in nitrogen and carbohydrate levels as metabolites are mobilized to other organs (Kudo, 2003), this may impact pupal physiology. Prolonging the pupal stage may be seen in this case as an insect strategy to overcome unfavorable changes in willow foliage quality, and the same arguments may apply if prior oviposition decreases foliage quality.
An extended cocoon stage may significantly increase exposure to predation from natural enemies. For forest insects such as the willow sawfly, the immobile pupal stage is especially vulnerable to predation (Olofsson, 1987; Haack and Mattson, 1993; Lindstedt et al., 2019). Diprionid sawflies can escape from pupal predators by spinning cocoons directly on the foliage, as opposed to the soil, to avoid contact with mammalian predators, although this may increase exposure to insectivorous birds (Hanski and Parviainen, 1985). The willow sawfly can also spin cocoons on willow leaves or bury them in the soil. The variation in the life cycle length that we report in this study can also be considered a risk spreading strategy (Olofsson et al., 2009) in which insects switch between phenotypes (different length life cycles) to avoid the consequences of feeding on egg-laden plants.
Prior oviposition may have decreased foliage quality in this study through the induction of JA, the major regulator of herbivore-induced defenses in plants. We propose that willows perceive sawfly oviposition as a warning cue against herbivory and so increase JA levels. JA induction was also observed in A. thaliana after egg deposition of the specialist butterfly P. brassicae, which triggered a strong priming of defense against the hatching larvae (Valsamakis et al., 2020). Since both SA and JA seem to have important roles in plant defense regulation against hatching larvae in other species (Reymond, 2013; Hilker and Fatouros, 2016), we should not discard the possible involvement of the high basal SA levels found here in response to oviposition. In addition, the induction of VOCs as indirect defenses in response to herbivore feeding damage has also been reported to be regulated by JA in Salicaceae species (Irmisch et al., 2013; Mrazova and Sam, 2018; McCormick et al., 2019). This study showed a concomitant induction of JA and OIPVs emission suggesting that OIPVs act in indirect defense or as further signals for defense activation in other parts of the plant or in other plants. We did not elucidate if this JA increase is activated by egg-associated molecular patterns present in sawfly oviposition secretions (Braccini et al., 2013) or by the leaf wounding that willow sawfly females cause when inserting their saw ovipositor into leaf tissue, as previous reported for other systems (Hilker et al., 2005; Bruessow et al., 2010; Voirol et al., 2020; Hundacker et al., 2021). Salicaceae not only induce VOCs after feeding damage, but also increase direct defenses, such as trypsin proteinase inhibitors and several phenolic derivatives (Boeckler et al., 2011; Fabisch et al., 2019; Eberl et al., 2021). Although a type of phenolic glycosides (salicinoids) and the condensed tannins are the major classes of specialized phenolic metabolites constitutively present in willow and poplar species (Barbehenn and Peter Constabel, 2011; Boeckler et al., 2011), induction of these compounds by herbivory is highly variable (Ruuhola et al., 2001; Stevens and Lindroth, 2005; Fields and Orians, 2006; Julkunen-tiitto et al., 1995). Here we did not find changes in the accumulation of salicinoids, i.e., salicin, salicortin and HCH-salicortin, in response to oviposition (data not shown).
In our study we showed that VOC composition was very sensitive to the type of damage received by willows (oviposition or feeding; Table 1 and Figure 6). Oviposition increased emission particularly of three terpenoids, (E,E)-α-farnesene, (Z)-, and (E)-β-ocimene, the latter being one of the major compounds of the total blend in oviposited plants. (E)-β-ocimene increased from 5% of the total blend released from O plants to 22% released from O + F plants. This acyclic monoterpene is one of the major volatile compounds reported for poplars and willows after feeding damage (Yoneya and Takabayashi, 2013; McCormick et al., 2019). Moreover, α-(E,E)-farnesene and (E)-β-ocimene have been found to be involved in the attraction of parasitoids and herbivore predators in oviposition and feeding damaged plants (Hilker and Meiners, 2011; McCormick et al., 2012; Farré-Armengol et al., 2017). Herbivore predators not only detect individual major and minor compounds present in the volatiles emitted by damaged plants, but also perceived changes in their relative amounts withing the whole blend (McCormick et al., 2012). The egg parasitoid of the pine sawfly (Diprion pini) Closterocerus ruforum was more attracted to a volatile blend that includes (E)-β-farnesene than to this compound alone (Beyaert et al., 2010). In some tritrophic systems, feeding damage after egg deposition is necessary for attraction of herbivore enemies (Conti and Colazza, 2012). Even parasitoid host preference varies with insect stage and the duration of larval herbivory, consistent with changes in the composition of the volatile blends (Pashalidou et al., 2015b). Oviposition and larval feeding by N. oligospilus emitted different blends of VOCs in S. babylonica, which may be exploited differently by natural enemies (like pupal and larval predators; Alderete, 2005; Alderete et al., 2010; Luft Albarracin et al., 2013), as previously reported on other plant-insect systems (Yoneya et al., 2009; Hilker and Meiners, 2011). Conversely, VOC emission can also be a deterrent cue for gravid females to avoid plants infested by conspecific eggs (Fatouros et al., 2012). N. oligospilus females decreased oviposition preference to plants of the South American willow (S. humboldtiana) damaged by conspecific feeding larvae with increased emission of germacrene D (Valladares et al., 2020). Previous studies have also shown than OIPVs can be perceived by neighboring plants and primed them for incoming herbivore attack, increasing their resistance. Brassica nigra plants exposed to OIPVs from neighboring conspecific plants primed their defenses leading to a reduction in Pieris brassicae larval performance and an increase in seed and flower production (Pashalidou et al., 2020). Another priming response was found in poplars, where egg-free Populus plants that neighbor egg-laden ones increased VOC emission and prevented egg laying by the moth Micromelalopha sieversi (Guo et al., 2019). In both studies, the effects of plant exposure to individual OIPVs were tested, obtaining an induced defensive response. It would be interesting to test whether the three terpenoids induced by N. oligospilus oviposition might act as a priming cue for future herbivory or as a conspecific repellent for egg-laying.
Oviposition of insect herbivores is well-known to reduce the future performance of feeding herbivores on annual herbaceous plants, especially model plants like Arabidopsis thaliana. Our results help extend this trend to non-model, woody plants and their herbivores. The hymenopteran herbivore studied here, the willow sawfly, suffered a many-fold increase in prepupal development time while feeding on foliage that had prior oviposition, which could alter insect population cycles in dramatic ways. These effects may have been triggered by oviposition-induced changes in plant hormone levels and volatile emission. The changes in volatile emission upon oviposition and herbivore feeding are distinct and could lead to selective attraction of herbivore enemies specific to the egg and larval stages, respectively.
Data availability statement
The raw data supporting the conclusions of this article will be made available by the authors, without undue reservation.
Author contributions
CD conducted the biological experiments, statistically analysed the data, and wrote the first draft of the manuscript. PF and JZ designed the research. JF contributed to the statistical analysis. PF and MR performed leaf sample processing and phytohormonal analysis. PF, JZ, and JG reviewed, commented, and polished the manuscript. All authors contributed to the article and approved the submitted version.
Funding
This work was funded by PNFOR 1104072 from Instituto Nacional de Tecnología Agropecuaria, PIA 14010 from Unidad para el Cambio Rural, Ministerio de Agricultura, Ganadería y Pesca, Argentina and a DAAD research grant (2017) granted to PF. Scholarship support for CD was provided by the Consejo Nacional de Investigaciones Científicas y Técnicas, Argentina (CONICET).
Acknowledgments
We thank to Ing Teresa Cerrillo for providing S. babylonica cuttings and also to both reviewers for constructive comments on the paper.
Conflict of interest
The authors declare that the research was conducted in the absence of any commercial or financial relationships that could be construed as a potential conflict of interest.
Publisher’s note
All claims expressed in this article are solely those of the authors and do not necessarily represent those of their affiliated organizations, or those of the publisher, the editors and the reviewers. Any product that may be evaluated in this article, or claim that may be made by its manufacturer, is not guaranteed or endorsed by the publisher.
References
Adams, R. P. (2007). Identification of essential oil components by gas chromatograpy/mass spectrometry. Carol Stream: Allured Publishing Corporation.
Alderete, C. (2005). Estudios poblacionales de Nematus oligospilus Foerster (Hymenoptera: Tenthredinidae), en Tucumán, plaga de sauces (Salicáceas) en Argentina. Tesis Dr. Fac. Ciencias Nat. e IML, UNT.
Alderete, M., Liljesthrom, G., and Fidalgo, P. (2010). Bio-ecología y perspectivas para el manejo de la avispa sierra del sauce. Ser. técnica Manejo Integr. Plagas For. INTA ediciones 10, 12 p.
Austel, N., Eilers, E. J., Meiners, T., and Hilker, M. (2016). Elm leaves “warned” by insect egg deposition reduce survival of hatching larvae by a shift in their quantitative leaf metabolite pattern. Plant Cell Environ. 39, 366–376. doi: 10.1111/pce.12619
Bandoly, M., Grichnik, R., Hilker, M., and Steppuhn, A. (2016). Priming of anti-herbivore defence in Nicotiana attenuata by insect oviposition: herbivore-specific effects. Plant Cell Environ. 39, 848–859. doi: 10.1111/pce.12677
Bandoly, M., Hilker, M., and Steppuhn, A. (2015). Oviposition by Spodoptera exigua on Nicotiana attenuata primes induced plant defence against larval herbivory. Plant J. 83, 661–672. doi: 10.1111/tpj.12918
Barbehenn, R. V., and Peter Constabel, C. (2011). Tannins in plant-herbivore interactions. Phytochemistry 72, 1551–1565. doi: 10.1016/j.phytochem.2011.01.040
Battisti, A. (1994). Voltinism and diapause in the spruce web-spinning sawfly Cephalcia arvensis. Entomol. Exp. Appl. 70, 105–113. doi: 10.1111/j.1570-7458.1994.tb00738.x
Bertea, C. M., Casacci, L. P., Bonelli, S., Zampollo, A., and Barbero, F. (2020). Chemical, physiological and molecular responses of host plants to lepidopteran egg-laying. Front. Plant Sci. 10, 1–7. doi: 10.3389/fpls.2019.01768
Beyaert, I., Köpke, D., Stiller, J., Hammerbacher, A., Yoneya, K., Schmidt, A., et al. (2012). Can insect egg deposition “warn” a plant of future feeding damage by herbivorous larvae? Proc. R. Soc. B Biol. Sci. 279, 101–108. doi: 10.1098/rspb.2011.0468
Beyaert, I., Wäschke, N., Scholz, A., Varama, M., Reinecke, A., and Hilker, M. (2010). Relevance of resource-indicating key volatiles and habitat odour for insect orientation. Anim. Behav. 79, 1077–1086. doi: 10.1016/j.anbehav.2010.02.001
Bittner, N., Hundacker, J., Achotegui-Castells, A., Anderbrant, O., and Hilker, M. (2019). Defense of scots pine against sawfly eggs (Diprion pini) is primed by exposure to sawfly sex pheromones. Proc. Natl. Acad. Sci. U. S. A. 116, 24668–24675. doi: 10.1073/pnas.1910991116
Boeckler, G. A., Gershenzon, J., and Unsicker, S. B. (2011). Phenolic glycosides of the Salicaceae and their role as anti-herbivore defenses. Phytochemistry 72, 1497–1509. doi: 10.1016/j.phytochem.2011.01.038
Boeckler, G. A., Gershenzon, J., and Unsicker, S. B. (2013). Gypsy moth caterpillar feeding has only a marginal impact on phenolic compounds in old-growth black poplar. J. Chem. Ecol. 39, 1301–1312. doi: 10.1007/s10886-013-0350-8
Bonnet, C., Lassueur, S., Ponzio, C., Gols, R., Dicke, M., and Reymond, P. (2017). Combined biotic stresses trigger similar transcriptomic responses but contrasting resistance against a chewing herbivore in Brassica nigra. BMC Plant Biol. 17, 127–114. doi: 10.1186/s12870-017-1074-7
Braccini, C. L., Vega, A. S., Chludil, H. D., Leicach, S. R., and Fernandez, P. C. (2013). Host selection, oviposition behaviour and leaf traits in a specialist willow sawfly on species of Salix (Salicaceae). Ecol. Entomol. 38, 617–626. doi: 10.1111/een.12053
Brooks, M. E., Kristensen, K., van Benthem, K. J., Magnusson, A., Berg, C. W., Nielsen, A., et al. (2017). glmmTMB balances speed and flexibility among packages for zero-inflated generalized linear mixed modeling. R J. 9, 378–400. doi: 10.32614/rj-2017-066
Bruce, T. J. A., Midega, C. A. O., Birkett, M. A., Pickett, J. A., and Khan, Z. R. (2010). Is quality more important than quantity? Insect behavioural responses to changes in a volatile blend after stemborer oviposition on an African grass. Biol. Lett. 6, 314–317. doi: 10.1098/rsbl.2009.0953
Bruessow, F., Gouhier-Darimont, C., Buchala, A., Metraux, J. P., and Reymond, P. (2010). Insect eggs suppress plant defence against chewing herbivores. Plant J. 62, 876–885. doi: 10.1111/j.1365-313X.2010.04200.x
Büchel, K., McDowell, E., Nelson, W., Descour, A., Gershenzon, J., Hilker, M., et al. (2012). An elm EST database for identifying leaf beetle egg-induced defense genes. BMC Genomics 13, 1–18. doi: 10.1186/1471-2164-13-242
Charles, J. G., and Allan, D. J. (2000). Development of the willow sawfly, nematus oligospilus, at different temperatures, and an estimation of voltinism throughout New Zealand. New Zeal. J. Zool. 27, 197–200. doi: 10.1080/03014223.2000.9518226
Conti, E., and Colazza, S. (2012). Chemical ecology of egg parasitoids associated with true bugs. Psyche 2012, 1–11. doi: 10.1155/2012/651015
Dapoto, G., and Giganti, H. (1994). Bioecología de Nematus desantisi Smith (Hymenoptera: Tenthredinidae: Nematinae) en las provincias de Río Negro y Neuquén (Argentina). Bosque 15, 27–32. doi: 10.4206/bosque.1994.v15n1-03
Desurmont, G. A., and Weston, P. A. (2011). Aggregative oviposition of a phytophagous beetle overcomes egg-crushing plant defences. Ecol. Entomol. 36, 335–343. doi: 10.1111/j.1365-2311.2011.01277.x
Doss, R. P., Oliver, J. E., Proebsting, W. M., Potter, S. W., Kuy, S., Clement, S. L., et al. (2000). Bruchins: insect-derived plant regulators that stimulate neoplasm formation. Proc. Natl. Acad. Sci. U. S. A. 97, 6218–6223. doi: 10.1073/pnas.110054697
Eberl, F., Fabisch, T., Luck, K., Köllner, T. G., Vogel, H., Gershenzon, J., et al. (2021). Poplar protease inhibitor expression differs in an herbivore specific manner. 1–11.
Erb, M., and Reymond, P. (2019). Molecular interactions between plants and insect herbivores. Annu. Rev. Plant Biol. 70, 527–557. doi: 10.1146/annurev-arplant-050718-095910
Fabisch, T., Gershenzon, J., and Unsicker, S. B. (2019). Specificity of herbivore defense responses in a woody plant, black poplar (Populus nigra). J. Chem. Ecol. 45, 162–177. doi: 10.1007/s10886-019-01050-y
Farré-Armengol, G., Filella, I., Llusià, J., and Peñuelas, J. (2017). β-Ocimene, a key floral and foliar volatile involved in multiple interactions between plants and other organisms. Molecules 22:1148. doi: 10.3390/molecules22071148
Fatouros, N. E., Lucas-Barbosa, D., Weldegergis, B. T., Pashalidou, F. G., van Loon, J. J. A., Dicke, M., et al. (2012). Plant volatiles induced by herbivore egg deposition affect insects of different trophic levels. PLoS One 7:e43607. doi: 10.1371/journal.pone.0043607
Fernández, P. C., Braccini, C. L., Dávila, C., Barrozo, R. B., Aráoz, M. V. C., Cerrillo, T., et al. (2019). The use of leaf surface contact cues during oviposition explains field preferences in the willow sawfly Nematus oligospilus. Sci. Rep. 9, 1–13. doi: 10.1038/s41598-019-41318-7
Fields, M. J., and Orians, C. M. (2006). Specificity of phenolic glycoside induction in willow seedlings (Salix sericea) in response to herbivory. J. Chem. Ecol. 32, 2647–2656. doi: 10.1007/s10886-006-9188-7
Frost, C. J., Mescher, M. C., Carlson, J. E., and De Moraes, C. M. (2008). Plant defense priming against herbivores: getting ready for a different battle. Plant Physiol. 146, 818–824. doi: 10.1104/pp.107.113027
Geiselhardt, S., Yoneya, K., Blenn, B., Drechsler, N., Gershenzon, J., Kunze, R., et al. (2013). Egg laying of cabbage white butterfly (Pieris brassicae) on Arabidopsis thaliana affects subsequent performance of the larvae. PLoS One 8:e59661. doi: 10.1371/journal.pone.0059661
González-Estrada, E., and Villaseñor, J. A. (2018). An R package for testing goodness of fit: goft. J. Stat. Comput. Simul. 88, 726–751. doi: 10.1080/00949655.2017.1404604
Guo, L., Liu, F., Zhang, S., Kong, X., and Zhang, Z. (2019). Egg deposition of Micromelalopha sieversi (Staudinger) on clones of Populus from section Aigeiros induces resistance in neighboring plants. Forest 10:110. doi: 10.3390/f10020110
Haack, R. A., and Mattson, W. J. (1993). “Life history patterns of north American tree-feeding sawflies” in Sawfly Adaptations to Woody Plants. eds. M. R. Wagner and K. F. Raffa (San Diego, CA: Academic Press), 503–545.
Hanski, I., and Parviainen, P. (1985). Cocoon predation by small mammals, and pine sawfly population dynamics. Oikos 45:125. doi: 10.2307/3565230
Hilker, M., and Fatouros, N. E. (2015). Plant responses to insect egg deposition. Annu. Rev. Entomol. 60, 493–515. doi: 10.1146/annurev-ento-010814-020620
Hilker, M., and Fatouros, N. E. (2016). Resisting the onset of herbivore attack: plants perceive and respond to insect eggs. Curr. Opin. Plant Biol. 32, 9–16. doi: 10.1016/j.pbi.2016.05.003
Hilker, M., and Meiners, T. (2011). Plants and insect eggs: how do they affect each other? Phytochemistry 72, 1612–1623. doi: 10.1016/j.phytochem.2011.02.018
Hilker, M., Stein, C., Schröder, R., Varama, M., and Mumm, R. (2005). Insect egg deposition induces defence responses in Pinus sylvestris: characterisation of the elicitor. J. Exp. Biol. 208, 1849–1854. doi: 10.1242/jeb.01578
Hundacker, J., Bittner, N., Weise, C., Bröhan, G., Varama, M., and Hilker, M. (2021). Pine defense against eggs of an herbivorous sawfly is elicited by an annexin-like protein present in egg-associated secretion. Plant Cell Environ. 45, 1033–1048. doi: 10.1111/pce.14211
Irmisch, S., McCormick, A. C., Andreas Boeckler, G., Schmidt, A., Reichelt, M., Schneider, B., et al. (2013). Two herbivore-induced cytochrome P450 enzymes CYP79D6 and CYP79D7 catalyze the formation of volatile aldoximes involved in poplar defense. Plant Cell 25, 4737–4754. doi: 10.1105/tpc.113.118265
Julkunen-tiitto, A. R., Bryant, J. P., Kuropat, P., and Roininen, H. (1995). Slight tissue wounding fails to induce consistent chemical defense in three willow (Salix spp.) clones. Oecologia 101, 467–471. doi: 10.1007/BF00329425
Kassambara, A., and Mundt, F. (2016). Factoextra: extract and visualize the results of multivariate data analyses. R package version. 1, 337–354.
Kim, J., Tooker, J. F., Luthe, D. S., De Moraes, C. M., and Felton, G. W. (2012). Insect eggs can enhance wound response in plants: a study system of tomato Solanum lycopersicum L. and Helicoverpa zea Boddie. PLoS One 7, 1–8. doi: 10.1371/journal.pone.0037420
Kudo, G. (2003). Variations in leaf traits and susceptibility to insect herbivory within a Salix miyabeana population under field conditions. Plant Ecol. 169, 61–69. doi: 10.1023/A:1026209017627
Lê, S., Josse, J., and Husson, F. (2008). FactoMineR: an R package for multivariate analysis. J. Stat. Softw. 25, 1–18. doi: 10.18637/jss.v025.i01
Lenth, R. V. (2016). Least-squares means: the R package lsmeans. J. Stat. Softw. 69, 1–33. doi: 10.18637/jss.v069.i01
Lindstedt, C., Murphy, L., and Mappes, J. (2019). Antipredator strategies of pupae: how to avoid predation in an immobile life stage? Philos. Trans. R. Soc. B Biol. Sci. 374:20190069. doi: 10.1098/rstb.2019.0069
Little, D., Gouhier-Darimont, C., Bruessow, F., and Reymond, P. (2007). Oviposition by pierid butterflies triggers defense responses in Arabidopsis. Plant Physiol. 143, 784–800. doi: 10.1104/pp.106.090837
Lortzing, T., Kunze, R., Steppuhn, A., Hilker, M., and Lortzing, V. (2020). Arabidopsis, tobacco, nightshade and elm take insect eggs as herbivore alarm and show similar transcriptomic alarm responses. Sci. Rep. 10, 16281–16216. doi: 10.1038/s41598-020-72955-y
Lortzing, V., Oberländer, J., Lortzing, T., Tohge, T., Steppuhn, A., Kunze, R., et al. (2019). Insect egg deposition renders plant defence against hatching larvae more effective in a salicylic acid-dependent manner. Plant Cell Environ. 42, 1019–1032. doi: 10.1111/pce.13447
Luft Albarracin, E., Hill, J. G., and Alderete, M. (2013). Aportes biológicos de Gelis tenellus (Hymenoptera: Ichneumonidae), parasitoide de Nematus oligospilus (Hymenoptera:Tenthredinidae) en Argentina. Acta zoológica lilloana 57 - Supl. V Reun. Argentina Parasitoloidologos, 90–92.
McCormick, A. C., Irmisch, S., Boeckler, G. A., Gershenzon, J., Köllner, T. G., and Unsicker, S. B. (2019). Herbivore-induced volatile emission from old-growth black poplar trees under field conditions. Sci. Rep. 9, 7714–7710. doi: 10.1038/s41598-019-43931-y
McCormick, A. C., Unsicker, S. B., and Gershenzon, J. (2012). The specificity of herbivore-induced plant volatiles in attracting herbivore enemies. Trends Plant Sci. 17, 303–310. doi: 10.1016/j.tplants.2012.03.012
Meiners, T., and Hilker, M. (2000). Induction of plant synomones by oviposition of a phytophagous insect. J. Chem. Ecol. 26, 221–232. doi: 10.1023/A:1005453830961
Mrazova, A., and Sam, K. (2018). Application of methyl jasmonate to grey willow (Salix cinerea) attracts insectivorous birds in nature. Arthropod Plant Interact. 12, 1–8. doi: 10.1007/s11829-017-9558-9
Mumm, R., Schrank, K., Wegener, R., Schulz, S., and Hilker, M. (2003). Chemical analysis of volatiles emitted by Pinus sylvestris after induction by insect oviposition. J. Chem. Ecol. 29, 1235–1252. doi: 10.1023/A:1023841909199
Olofsson, E. (1987). Mortality factors in a population of Neodiprion sertifer (Hymenoptera: Diprionidae). Oikos 48:297. doi: 10.2307/3565517
Olofsson, H., Ripa, J., and Jonzén, N. (2009). Bet-hedging as an evolutionary game: the trade-off between egg size and number. Proc. R. Soc. B Biol. Sci. 276, 2963–2969. doi: 10.1098/rspb.2009.0500
Ovruski, S., and Smith, D. (1993). Descriptions of immature stages of Nematus desantisi (Hymenoptera, Tenthredinidae), a Pest of Salicaceae in Argentina and Chile. Entomol. News 104, 153–160.
Pashalidou, F. G. (2015). Plant-mediated effects of butterfly egg deposition on subsequent caterpillar and pupal development, across different species of wild Brassicaceae. Eco. Entomol. 40, 444–450. doi: 10.1111/een.12208
Pashalidou, F. G., Eyman, L., Sims, J., Buckley, J., Fatouros, N. E., De Moraes, C. M., et al. (2020). Plant volatiles induced by herbivore eggs prime defences and mediate shifts in the reproductive strategy of receiving plants. Ecol. Lett. 23, 1097–1106. doi: 10.1111/ele.13509
Pashalidou, F. G., Frago, E., Griese, E., Poelman, E. H., van Loon, J. J. A., Dicke, M., et al. (2015a). Early herbivore alert matters: plant-mediated effects of egg deposition on higher trophic levels benefit plant fitness. Ecol. Lett. 18, 927–936. doi: 10.1111/ele.12470
Pashalidou, F. G., Gols, R., Berkhout, B. W., Weldegergis, B. T., van Loon, J. J. A., Dicke, M., et al. (2015b). To be in time: egg deposition enhances plant-mediated detection of young caterpillars by parasitoids. Oecologia 177, 477–486. doi: 10.1007/s00442-014-3098-0
Pinheiro, J., and Bates, D. (2022). R Core Team. nlme: Linear and nonlinear mixed effects models. R package version 3.1-161. Available at: https://CRAN.R-project.org/package=nlme
Reymond, P. (2013). Perception, signaling and molecular basis of oviposition-mediated plant responses. Planta 238, 247–258. doi: 10.1007/s00425-013-1908-y
Rondoni, G., Bertoldi, V., Malek, R., Djelouah, K., Moretti, C., Buonaurio, R., et al. (2018). Vicia faba plants respond to oviposition by invasive Halyomorpha halys activating direct defences against offspring. J. Pest. Sci. 91, 671–679. doi: 10.1007/s10340-018-0955-3
RStudio Team (2020). RStudio: Integrated development for R. RStudio, PBC, Boston, MA. Available at: http://www.rstudio.com/
Ruuhola, T. M., Sipura, M., Nousiainen, O., and Tahvanainen, J. (2001). Systemic induction of salicylates in Salix myrsinifolia (Salisb.). Ann. Bot. 88, 483–497. doi: 10.1006/anbo.2001.1491
Schott, J., Fuchs, B., Böttcher, C., and Hilker, M. (2021). Responses to larval herbivory in the phenylpropanoid pathway of Ulmus minor are boosted by prior insect egg deposition. Planta 255:16. doi: 10.1007/s00425-021-03803-0
Smith, D. R. (1993). “Systematics, life history, and distribution of sawflies” in Sawfly life history adaptations to Woody plants. ed. K. K. R. M. R. Wagner (San Diego, CA: Academic Press, Inc), 3–32.
Stevens, M. T., and Lindroth, R. L. (2005). Induced resistance in the indeterminate growth of aspen (Populus tremuloides). Oecologia 145, 297–305. doi: 10.1007/s00442-005-0128-y
Urban, A. J., and Eardley, C. D. (1995). A recently introduced sawfly, Nematus oligospilus Forster (Hymenoptera: Tenthredinidae), that defoliates willows in southern Africa. Afr. Entomol. 3, 23–27.
Vadassery, J., Reichelt, M., Hause, B., Gershenzon, J., Boland, W., and Mithöfer, A. (2012). CML42-mediated calcium signaling coordinates responses to Spodoptera herbivory and abiotic stresses in Arabidopsis. Plant Physiol. 159, 1159–1175. doi: 10.1104/pp.112.198150
Valladares, G. A., Coll-Aráoz, M. V., Alderete, M., Vera, M. T., and Fernández, P. C. (2020). Previous herbivory alerts conspecific gravid sawflies to avoid unsuitable host plants. Bull. Entomol. Res. 110, 438–448. doi: 10.1017/S0007485319000786
Valsamakis, G., Bittner, N., Fatouros, N. E., Kunze, R., Hilker, M., and Lortzing, V. (2020). Priming by timing: Arabidopsis thaliana adjusts its priming response to Lepidoptera eggs to the time of larval hatching. Front. Plant Sci. 11:619589. doi: 10.3389/fpls.2020.619589
Voirol, P., Hilker, M., Lortzing, V., Johnston, P. R., Fatouros, N. E., and Kunze, R. (2020). Plant responses to insect eggs are not induced by egg-associated microbes, but by a secretion attached to the eggs. Plant Cell Environ. 43, 1815–1826. doi: 10.1111/pce.13746
Wickham, H. (2011). The split-apply-combine strategy for data analysis. J. Stat. Softw. 40, 1–29. doi: 10.18637/jss.v040.i01
Yang, J. O., Nakayama, N., Toda, K., Tebayashi, S., and Kim, C. S. (2013). Elicitor(s) in Sogatella furcifera (Horváth) causing the Japanese rice plant (Oryza sativa L.) to induce the ovicidal substance, benzyl benzoate. Biosci. Biotechnol. Biochem. 77, 1258–1261. doi: 10.1271/bbb.130055
Yoneya, K., Kugimiya, S., and Takabayashi, J. (2009). Can herbivore-induced plant volatiles inform predatory insect about the most suitable stage of its prey? Physiol. Entomol. 34, 379–386. doi: 10.1111/j.1365-3032.2009.00701.x
Keywords: Tenthredinidae, insect-plant interaction, volatiles, egg deposition, prepupal development, herbivory, jasmonic acid
Citation: Dávila C, Fiorenza JE, Gershenzon J, Reichelt M, Zavala JA and Fernández PC (2023) Sawfly egg deposition extends the insect life cycle and alters hormone and volatile emission profiles. Front. Ecol. Evol. 11:1084063. doi: 10.3389/fevo.2023.1084063
Edited by:
Stefano Colazza, University of Palermo, ItalyReviewed by:
Lukasz Lech Stelinski, University of Florida, United StatesFoteini G. Pashalidou, Institut National de la Recherche Agronomique (INRA), France
Copyright © 2023 Dávila, Fiorenza, Gershenzon, Reichelt, Zavala and Fernández. This is an open-access article distributed under the terms of the Creative Commons Attribution License (CC BY). The use, distribution or reproduction in other forums is permitted, provided the original author(s) and the copyright owner(s) are credited and that the original publication in this journal is cited, in accordance with accepted academic practice. No use, distribution or reproduction is permitted which does not comply with these terms.
*Correspondence: Camila Dávila, ✉ Y2RhdmlsYUBhZ3JvLnViYS5hcg==; Patricia C. Fernández, ✉ cGNmZXJuYW5AYWdyby51YmEuYXI=