- 1Institute of Limnology, University of Konstanz, Konstanz, Germany
- 2Swiss Ornithological Institute, Sempach, Switzerland
- 3Faubourg de l'Hôpital 58, Neuchâtel, Switzerland
- 4Staatliches Museum für Naturkunde Stuttgart (SMNS), Stuttgart, Germany
Introduction: Tropical rainforest species interact with each other and their environment over a wide range of spatiotemporal scales. However, our understanding of resource partitioning and the mechanisms of avian species coexistence is largely restricted to subjective visual observations or acoustic monitoring. Therefore, the relative magnitudes of interspecific and intraspecific differences in resource use have remained difficult to quantify, particularly regarding different diets and habitat use. The eastern rainforest belt of Madagascar is inhabited by several species of insectivorous tetrakas belonging to an endemic bird family of Madagascar (Bernieridae). These species occupy similar habitats in the forest understory and are morphologically similar but because of likely differences (e.g., in foraging behaviors) we expect their foraging niches to be segregated allowing coexistence.
Methods: We examined the niche differentiation of four of these species: the Grey-crowned Tetraka (Xanthomixis cinereiceps), Long-billed Tetraka (Bernieria madagascariensis), Spectacled Tetraka (Xanthomixis zosterops), and White-throated Oxylabes (Oxylabes madagascariensis) in the Maromizaha rainforest in eastern Madagascar combining morphometry with stable carbon, nitrogen, and sulfur isotope ratios (δ13C, δ15N, and δ34S) from feathers.
Results: We show considerable variation in isotopic niche positions, niche breadth and interspecific niche overlap. In two species, the Long-billed Tetraka and Spectacled Tetraka, we found an indication of sex-specific niche space, with males exhibiting a larger isotopic niche-area relative to females. Morphological traits of five species (including the Wedge-tailed Tetraka, Hartertula flavoviridis) coupled with stable isotope data provided explanations of patterns of niche overlap and isotopic position.
Discussion: The observed isotopic niche differences may be explained by differences in resource acquisition strategies that might be associated with specific morphological traits and spatial distribution. This may play an important role in niche differentiation among coexisting and phylogenetically closely related species.
Introduction
Despite the coexistence of phylogenetically closely related and morphologically similar species, niche theory suggests that a complete niche overlap may evolutionarily be unlikely (e.g., Hutchinson, 1957; Hardin, 1960). Coexisting species evolved varying forms of resource utilization, such as niche partitioning or niche differentiation (Levins, 1968; MacArthur, 1972), which have been widely used to explain coexistence patterns in community assemblages (Schoener, 1974; Giller, 1984; Ross, 1986). Coexistence may occur due to segregation of specific resources (also known as resource partitioning) or through spatiotemporal variation in resource use (Pianka, 1974; Schoener, 1974; Ross, 1986). In tropical forest bird assemblages, partitioning of dietary resources is one of the fundamental mechanisms of niche separation (e.g., Frith, 1984; Symes and Woodborne, 2010; Mansor and Ramli, 2017; Mansor et al., 2022). In line with the competitive exclusion principle (Hardin, 1960) if any species with identical niches or ecological roles compete, one will drive the other to extinction. By implication, multiple species cannot occupy the same exact niche in one habitat and coexist in a stable manner. Consequently, when these species differentiate their niches, they tend to reduce competition, and promote coexistence. This niche differentiation can be achieved through different mechanisms, which includes consuming various dietary items or partitioning of the environment, such as using different vertical strata of the tropical rainforest (Thiel et al., 2021).
The family of tetrakas (Bernieridae) consists of mostly greenish to yellowish songbirds, all of which are endemic to Madagascar. Some resemble typical warblers (Sylviidae), others are bulbul-like (Pycnotidae), and some did not appear to fit in any systematic group (Cibois et al., 1999, 2001). Their systematic relationship was resolved by the genetic studies of Cibois et al. (2010), which referred to them as a separate family, the Bernieridae. Under current taxonomy, they represent an adaptive radiation of 13 species in eight genera (Reddy et al., 2022; Safford et al., 2022). Some show extensive interspecific resemblance but also intraspecific cryptic diversity (Bickford et al., 2007; Block, 2012; Safford and Hawkins, 2013; Block et al., 2015; Reddy et al., 2022).
The adaptive radiation of tetrakas has been at a relatively continuous diversification rate (Block, 2012) since its estimated dispersal to Madagascar between 9–17 MY (Cibois et al., 2001) or 19.2–25.2 MY ago (Beresford et al., 2005). These range estimates are approximately the same as that for the better known adaptive radiation of a Malagasy bird family, the vangas (Vangidae) (Jønsson et al., 2012; Reddy et al., 2012). Contrary to the vangas, the adaptive radiation of the tetrakas does not appear to have resulted in such a large range of morphological variations and feeding behaviors. Present knowledge of the tetrakas describes all of them as almost exclusively insectivorous (Goodman and Parrillo, 1997; Raherilalao and Goodman, 2011; Block, 2012; Hawkins et al., 2015; Faliarivola et al., 2020). Using their slender bills, their feeding behaviors are relatively homogenous, mostly gleaning insects, probably also using flutter-chase or even flush-pursue strategies (Remsen and Robinson, 1990) when participating in mixed-species foraging flocks (Eguchi et al., 1993; Sridhar et al., 2009). Eleven species are understory or even ground-dwelling in relatively pristine or even exclusively in primary forests, while the Rand’s Warbler (Randia pseudozosterops) and Cryptic Warbler (Cryptosylvicola randrianasoloi) can be found in the canopy (Goodman et al., 1996; Raherilalao and Goodman, 2011; Hawkins et al., 2015).
In the eastern tropical rainforests of Madagascar, up to eight species of tetraka can be found living sympatrically. Since they share dense habitats or the high canopy, they can be very difficult to observe. Previously, some information relating to the ecological niche and microhabitats of these species has been described (Raherilalao and Goodman, 2011; Safford and Hawkins, 2013). However, the mechanisms behind the successful sympatry of these phylogenetically closely related species have yet to be explained in detail. A species’ ecological space, which is often linked to diet, substrate use and foraging behavior can be indirectly approximated by the morphological space filled by each species (Jønsson et al., 2012) and may be visible in their isotopic signatures (Procházka et al., 2010; Yohannes and Woog, 2020).
Stable isotopes have been employed effectively as a tool to determine the origin of dietary nutrient sources for various taxonomic groups (Kelly, 2000). The stable isotopes in feathers can reveal the dietary sources of birds by providing information on the habitat use and nutrient intake during feather growth, which occurs during molt. These isotopes can indicate whether the birds consumed plant matter, such as fruits or seeds, or invertebrate-based diets, such as insects or spiders or both. The stable isotope ratios, particularly nitrogen (δ15N), can provide insight into the trophic level of the prey and thus the bird, as (δ15N) values tend to increase at higher trophic levels (Inger and Bearhop, 2008). Stable nitrogen isotopes undergo an increase ranging from 2 to 4‰ in heavy isotope enrichment with each trophic level and can therefore serve as a tool to determine dietary shifts and trophic positions (Inger and Bearhop, 2008). Stable carbon isotopes can be used to distinguish between plants that use either C3, C4 or CAM modes of photosynthesis, since the C4 and CAM pathways lead to lower carbon fractionation than C3 photosynthesis (Karasov and Martínez del Rio, 2007). Carbon isotopes can thus be used to reconstruct habitat use regarding C3, C4 and CAM plants and therefore, the diet preferences of the study species (Hobson, 1999). Changes in δ13C due to consumer tissue-diet fractionation range from 1 to 2‰. Furthermore, there is a discernible difference in the stable isotope ratios of plants growing in different vertical strata within closed canopy forests, as seen in carbon (δ13C) values. This phenomenon is commonly referred to as the canopy effect (van der Merwe and Medina, 1991), whereby vertical gradients in sunlight penetration, humidity, water source and photosynthetic processes regulate the stable isotope values. Consistently, this produces 13C-depleted plant values from ground to canopy; the most positive values are usually at the upper vertical portion. Canopy effects on avian studies (understorey or ground-dwelling birds) have been measured as height from the ground, rather than estimating or measuring the vertical distance from the canopy (e.g., Rajaonarivelo et al., 2020, 2021; Lowry et al., 2021).
Compared with carbon and nitrogen isotopes, stable sulfur isotopes have been used less frequently, and their potential as tracers in biochemical and physiological studies are only beginning to be realized. Mechanisms and accompanying minor or null isotope fractionations involved during the uptake and assimilation of sulfur compounds by animals can be utilized as bioindicators of the foraging niche (Richards et al., 2003; Arneson and MacAvoy, 2005; Florin et al., 2011), to distinguish the origin of dietary sources (e.g., Lott et al., 2003) and to track movement and habitat use (e.g., Date et al., 2022). Sulfur plays an essential role in the synthesis of key metabolic intermediates. For example, the metabolism of sulfur-containing amino acids, methionine and cysteine are essential to protein synthesis in birds (Griffith, 1987). For optimal growth and survival of individuals, the diet must provide these two amino acids and sulfur-containing vitamins, such as thiamine and biotin (Brosnan and Brosnan, 2006). Variations in δ34S values on a small geographic scale are described as local soil sulfate variability. It can significantly influence the plants that grow in these soils and is expressed in the animal tissues (e.g., insects, birds) that feed on them. The fractionation of sulfur isotopes in increasing food chains is comparatively low (McCutchan et al., 2003). Consequently, a wide range of feather δ34S values indicate variable input of sulfur-containing amino acids from a range of consumed dietary sources or recycled body proteins during molt.
In tetrakas, only qualitative descriptions of their feeding habits and diets have been published so far, but information on the stable isotopes in their feathers or prey have not yet been assessed. As a first step, to explore their niche differentiation that may be related to their diet or to the strata they use in the forest, we used a multi-elemental approach analyzing feather isotopes combined with morphometric measurements. We aim to link multi-isotope results toward canopy stratigraphy and avian feeding niches in a rainforest ecosystem and to construct isotopic food web relations among the species dwelling in the same ecosystem. To uncover this diversification and niche segregation, we use a combination of morphometry and multi-element stable isotope analyses of body feathers (δ13C, δ15N, and δ34S) that can be linked to a variety of microhabitats and prey. For keratinous tissues that are metabolically inert following synthesis (e.g., feather), their isotopic values are “fixed” specifically representing the time over which the feather was grown (Hobson, 1999). We hypothesize that niche partitioning and dietary resource segregation may explain the sympatry of insectivorous tetrakas in the Maromizaha rainforest in eastern Madagascar. Specifically, we compare five species of Tetraka: the Grey-crowned Tetraka (Xanthomixis cinereiceps), Long-billed Tetraka (Bernieria madagascariensis), Spectacled Tetraka (Xanthomixis zosterops), White-throated Oxylabes (Oxylabes madagascariensis), and Wedge-tailed Tetraka (Hartertula flavoviridis) and explore niche partitioning patterns and the mechanism of coexistence between them. In two sexually dimorphic species (Long-billed Tetraka and Spectacled Tetraka), we investigate the effect of sex on isotopic niches. We examine whether morphological trait-based niche differentiation was evident. The degree of isotopic niche overlap was expected to reflect the extent of interspecific feeding competition between these co-occurring species.
Methods
Study site
The Maromizaha rainforest (18°56′49′S, 48°27′33′E, Figure 1) is an officially protected area located approximately 150 km east of the capital Antananarivo in the commune of Andasibe, district of Moramanga, Madagascar. It is an area of approximately 1,881 ha, and is characterized by mountainous terrain ranging from 751 to 1,250 m in altitude with many small streams and is part of the Ankeniheny-Zahamena forest corridor. At the edges, along the central valley and along the “route nationale,” approximately 600 ha have been deforested to obtain firewood and to create agricultural areas. Bird capture sites were located in five different habitats between 1,005 and 1,110 m altitude: a pristine mountain ridge, a mostly undisturbed river site, a mountain saddle, a plantation of vegetables and fruits surrounded by forest and a degraded open savannah near a quarry (Woog et al., 2006; Yohannes and Woog, 2020).
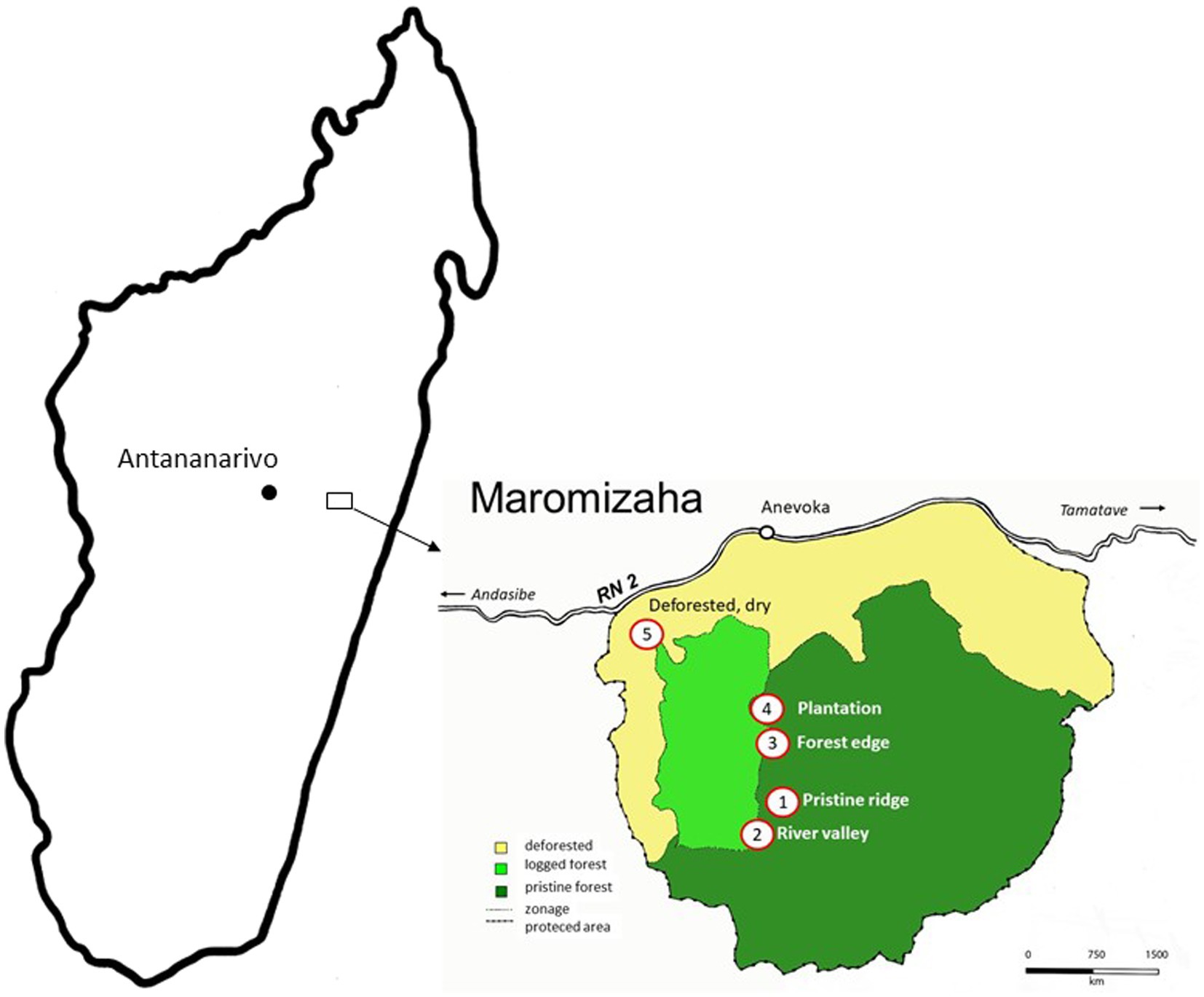
Figure 1. Map of Madagascar and the approximate location of the Maromizaha rainforest (indicated by a black square symbol).
Study species
Tetrakas exhibit a range of morphological traits, including variation in bill shapes, wing, tail, and tarsus lengths (Safford and Hawkins, 2013). Wings are mostly short and rounded, the tail of medium length and somewhat graduated. While all study species can be found in undisturbed forests, some may also forage in secondary forest habitats (Benjara et al., 2021). Long-billed Tetrakas are found in both wet and dry habitats throughout Madagascar, except for the central mountain range and the Southwest. Tetrakas feed on invertebrates, typically captured from the substrate, and may form either single or mixed species flocks, often containing previous offspring (Raherilalao and Goodman, 2011). They are considered as resident species (Raherilalao and Goodman, 2011; Safford and Hawkins, 2013). Expressed as the percentage of individuals recaptured between years of all ringed birds per species, tetrakas showed high site fidelity (recapture percentage at the original ringing site between years (2003–2016): Grey-crowned Tetraka 9.7%, Long-billed Tetraka 25.5%, Spectacled Tetraka 15.4%, Wedge-tailed Tetraka 30% and White-throated Oxylabes 8.3%; Woog et al., 2018). Safford and Hawkins (2013) provide a description of the known habitat uses and feeding behavior, as summarized in Table 1.
Bird capture, morphometry and sampling
Between 2003 and 2018, birds were caught in mist nets during field seasons lasting about a month between September and January. This period represents the southern spring and coincides with the breeding season for many species. For the identification and ageing of the birds, Morris and Hawkins (1998) and Sinclair and Langrand (2013) were used. Birds were caught and ringed with aluminum rings from SAFRING to enable individual identification in the future. All measurements were taken by FW following Eck et al. (2011) and included wing (maximum chord), 3rd (outermost) primary, Kipp measure (outermost secondary to tip of wing), tip of outermost primary to longest primary, minimum tarsus (metatarsal bone), tail, bill to skull, bill length, height, and width from distal edge of nostril, weight, breeding and molting status. Up to five flank feathers from underneath the wing and a tail feather were collected for stable isotope analyses. For molecular sexing, a blood sample was taken from the brachial vein and placed in a DNA buffer (Wink, 2006) before the birds were released back into the wild.
There is no published information about the molting periods of this bird family. During our study, the onset of molt appeared to start after the young fledged. Body molt for all tetraka species started in November and lasted into January, after which no data was collected and was synchronous for males and females. Wing molt started in November and December, except for the Long-billed Tetraka, where no wing molt was observed in this period. Currently, there is no information available regarding post-juvenile molt in tetrakas, which may occur in January or February, coinciding with the cyclone season. Because of high site fidelity during breeding and molt and a presumed minor variation of habitat use throughout the year, a reduced temporal effect on feather isotope values, as well as a limited variation between sexes was assumed. To test for these effects, flank and tail feathers (which could be molted at different times of the year) were tested for significant differences in the stable isotope ratio values. Following the absence of differences (Paired t-test, p > 0.05), the focus was put on flank feather samples, as they provided the larger sample size. The current approach has limitations as more data are needed on molt in these birds, and repeated feather sampling throughout the annual cycle.
Capture height
Regular mist-nets were used to capture the birds. By design, they had five pockets measuring 50 cm each, reaching from the ground to 2.5 m in height. High nets were built using two superposed nets and reached from the ground to 5 m in height. Ten height classes were marked out between 1 and 5 m height above the ground, representing the pocket in which the birds were caught. The pocket (referred here as capture height) was noted for each individual at capture. A Chi-square test of independence was performed between the Long-billed Tetraka and the four other species. All captures above the height of class 4 were pooled, and the repartition of captures of Long-billed Tetraka was tested against the other species. The canopy height was variable and reached higher than the mist-nets in most cases, but capturing birds at greater heights was technically not feasible. Therefore, all capture height data reported in this study refer to birds caught within the mist-nets, at heights between 0 and 5 m. This approach is commonly used on research in habitat usage by ground-dwelling or understory birds (e.g., Rajaonarivelo et al., 2020, 2021; Lowry et al., 2021), but its drawback is the lack of information it provides regarding birds inhabiting the strata above the nets.
Feather stable isotope measurements
In the laboratory, the flank feather samples were prepared by washing each individual sample in a 3:1 solution of chloroform and methanol for 24 h, followed by a thorough rinse with distilled water and drying to ensure the purity of the samples. Samples of ca. 1 mg were pre-weighed in tin cups and combusted using a vario MICRO cube elemental analyzer (Elementar, Analysensysteme GmbH, Germany). Simultaneous resultant CO2, N2 and SO2 gases were introduced into a Micromass Isoprime isotope ratio mass spectrometer (Isoprime Ltd., United Kingdom) via a continuous flow-through inlet system. Sample 13C/12C, 15N/14N, and 34S/32S ratios are expressed in the delta (δ13C, δ15N, and δ34S) notation in parts per thousand (‰) relative to the following standards: the Vienna Pee Dee Belemnite (VPDB) for C, atmospheric N2 for nitrogen, and sulphanilamide-calibrated and traceable to NBS-127 (barium sulfate) for S. Stable isotope ratios were obtained using the equation:
δX(‰) = 1,000 × (Rsample/Rstandard − 1), where X is 13C, 15N or 34S and R is 13C/12C, 15N/14N or 34S/32S. Internal laboratory standards indicate that our measurement errors (SD) were ± 0.15‰, 0.03‰ and 0.05‰ for δ15N, δ13C, and δ34S, respectively.
Molecular and morphological based sexing
Blood samples (n = 118) were collected in the field. DNA was extracted using the DNeasy Blood and Tissue Kit (QIAGEN, Hilden, Germany), following the manufacturer’s protocol (Çakmak et al., 2017). PCRs using the primer pair CHD1F/CHD1R (Lee et al., 2010) were conducted with the kit HotStarTaq Plus DNA Polymerase (QIAGEN, Hilden, Germany), following the standard protocol. The resulting PCR products were separated by electrophoresis on a 2% agarose gel containing 1 μl GelRed® Nucleic Acid Gel Stain (BIOTIUM, Fremont, United States) for 40 min at 100 V in a standard Tris-borate-EDTA buffer. The primer pair yielded only one band for both sexes, but after separation by electrophoresis, a characteristic difference in fragment size was evident. For the birds for which blood samples could not be collected, sexing was conducted using plumage characteristics and presence/absence of a brood patch in females or the shape of the cloacal protuberance for males (Redfern et al., 2001). Samples of individuals which were sexed both in the field and using the molecular approach (n = 42) showed a concordance of more than 90%. Molecular sexing of tetrakas was not as straightforward as expected and is subject to further research.
Morphometric data analyses
All data analyses were performed in R V. 4.2.1 and RStudio V. 2022.7.1.554 (R Core Team, 2022; RStudio Team, 2022). Some morphological variables within one anatomical feature (i.e., wing or beak) were strongly correlated. As a result, the following measurements for morphometric analyses were selected: (1) wing length: maximum chord, (2) tarsus: metatarsal bone, (3) bill width: bill width at distal edge of nostril, and (4) bill length: bill to skull. Preliminary and descriptive analyses were performed on the data using different packages in R (Wickham, 2016; Dowle and Srinivasan, 2021; Wickham et al., 2022), before checking for multivariate normality using an Energy test (Rizzo and Szekely, 2022), as well as Mardia’s test (Fletcher, 2022). Homoscedasticity was tested with Levene’s test (Fox and Weisberg, 2019) and the homogeneity of variance–covariance matrices was tested using Box’s M test (da Silva, 2021). ANOVAs were performed for each variable, and the species were sorted into statistically significant groups using Tukey’s Honestly Significant Differences (HSD) post-hoc tests (Bryan, 2017; Wickham et al., 2019; de Mendiburu, 2021; Iannone et al., 2022). In each case, the normal distribution of the residuals was verified by a Shapiro–Wilk test. The significance of the disparity between males and females was tested for each variable and for each species using Welch’s t-tests.
Quadratic discriminant analysis (QDA) and Linear discriminant analysis (LDA) were used for classification and graphical representation. The log-transformed values of the four morphological variables, which were also scaled for the LDA (Legendre and Legendre, 2012), were used as the response variable and because of sample size restrictions, only the species were taken as the supervised grouping variable (Sievert, 2020). Both analyses (LDA and QDA) were applied to investigate the morphological classification and categorization of individuals into their respective groups using a leave-one-out cross-validation (also known as jack-knifed validation) method (Venables and Ripley, 2002). To infer the difference between the sexes, the sexes were subsequently displayed in the graphical representation. For each species, the results of the QDA were sorted by sex and the species assignment accuracy was explored.
Stable isotope data analyses
This part of the analysis was performed for only four species since stable isotope values were not available for Wedge-tailed Tetrakas. Analysis of variance (ANOVA) and Student’s t-tests were employed to test for differences in stable isotope values between sites for each species and for each element separately (ANOVA and T-test, p > 0.05). Since there were no statistically significant differences, samples from all sites were pooled. Species-specific trophic niche width was calculated using metrics that include the total convex hull area (TA) occupied by all individuals of each species in the δ15N/δ13C and δ34S/δ13C biplots (Layman et al., 2007). As described by Jackson et al. (2011), standard ellipse areas (SEA) with a correction for small sample sizes (SEAc) were used. This technique relies on a Bayesian method that accounts for data uncertainty and permits isotopic community metrics comparison between groups implemented through stable isotope Bayesian ellipses (Jackson et al., 2011) using 40% overlap analysis. To estimate the extent of overlap between the isotopic niches of a given species pair, triple isotopic nestedness based on convex hulls was calculated using scaled isotope data following the scale function described by Cucherousset and Villéger (2015). Convex hulls for the species and sexes were computed, and pairs of isotopes were graphically depicted (Wickham, 2016; Habel et al., 2022).
Isotopic overlap metrics [isotopic similarity (ISim) and nestedness (Ines)] were calculated following Villéger et al. (2011 2013) and Cucherousset and Villéger (2015) using the isotopic richness (convex hull volume) of two species (pairwise species comparisons) and the volume of isotopic space they shared (i.e., intersection volume). Isotopic niche has typically been characterized through carbon and nitrogen ratios, limiting the modeling approaches to two dimensions (Layman et al., 2007). Yet, other stable isotopes (e.g., δ34S) can provide additional power to resolve questions associated with variations in resource use and simultaneously enhance the dimensions to three elements (3D). An analogous methodology was used, which incorporated variation across three dimensions, comparing all three stable isotopes at the same time to estimate the significant features of a species isotopic space. ISim is the ratio between the volume of the intersection and the volume of the union of the two groups of organisms in the stable isotope space. ISim ranges from 0 in cases where organisms fill totally different parts to 1 when the species fill the same portion of the isotopic space. It is multi-dimensional and unitless but could nonetheless be influenced by the differences in size of the convex hull area of the species considered. Ines was also applied as a complementary index. It represents the ratio between the volume of the intersection and the minimal volume filled by the species.
Morphometry combined with stable isotope data
After checking for normal distribution for each group and variable, a Pearson correlation coefficient table was calculated for the log-transformed morphometric variables and for the stable isotope values. A principal component analysis (PCA) was performed on the scaled data as an unsupervised dimension reduction method. Then, it was possible to check which combinations of variables were most relevant to resolving the ecological space of the species and sexes. The variation along the first two principal components (PC1 and PC2) was presented as a biplot, showing the best possible spread of the data points along these axes (Borcard et al., 2011), and the species and sexes were also displayed graphically.
Results
Between 2003 and 2018, a total of 205 tetrakas were caught and measured at five different sites (mountain ridge: n = 13, river valley: n = 99, mountain saddle: n = 67, plantation: n = 25, dry savannah: n = 1). Tetrakas were abundant in almost all habitats, except for the highly degraded dry savannah. All species were most abundant in the river valley and were captured predominantly in the lower pockets of the nets: 0.01–0.5 m (n = 29); 0.51–1.0 m (n = 63); 1.01–1.5 m (n = 57); 1.51–2.0 m (n = 29); 2.01–2.5 m (n = 14); 2.51–3.0 m (n = 4); 3.01–3.5 m (n = 0); 3.51–4.0 m (n = 2); 4.01–4.5 m (n = 5); and 4.51–5.0 m (n = 2) (Figure 2). White-throated Oxylabes and Wedge-tailed Tetrakas were consistently captured at the lower pockets, most often between 0.5 and 1 m (68% of total captures). In contrast, Long-billed Tetrakas were captured at higher net heights (capture heights 5 to 10, above 2 m) more often than the other species (Figure 2). Chi-Square test of independence: χ2 = 12.57, df = 4, p = 0.014, for a total of 205 captures; Grey-crowned Tetraka: n = 32, Long-billed Tetraka: n = 65, Spectacled Tetraka: n = 71, Wedge-tailed Tetraka: n = 12, White-throated Oxylabes: n = 25).
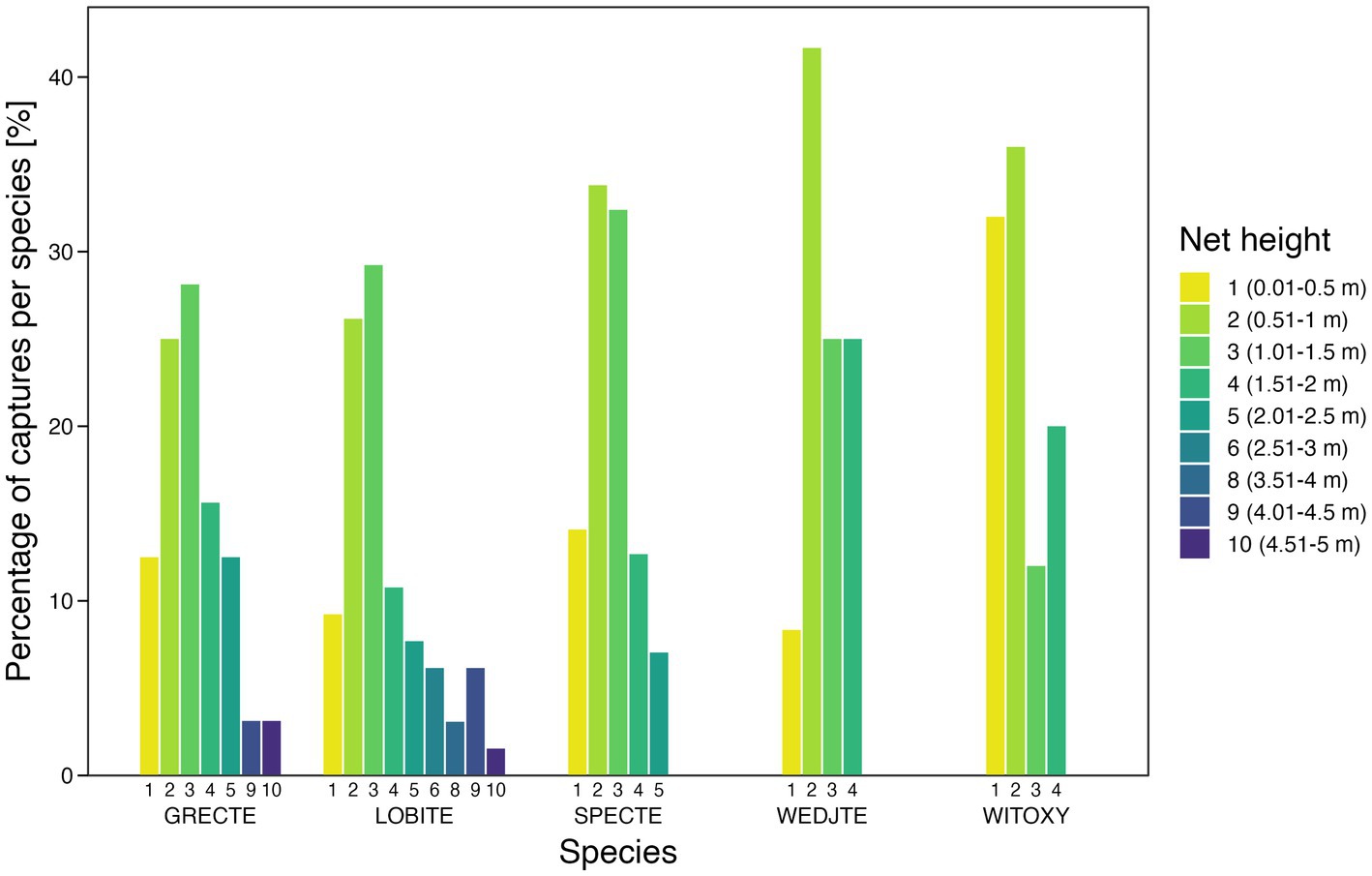
Figure 2. Percentage of captured individuals per species at each capture height. Number of captures at each height class (1–10 for each species respectively): GRECTE (4, 8, 9, 5, 4, 0, 0, 0, 1, 1), LOBITE (6, 17, 19, 7, 5, 4, 0, 2, 4, 1), SPECTE (10, 24, 23, 9, 5, 0, 0, 0, 0, 0), WEDJTE (1, 5, 3, 3, 0, 0, 0, 0, 0, 0), WITOXY (8, 9, 3, 5, 0, 0, 0, 0, 0, 0). For species abbreviations, see Table 1.
Morphometrics
The five species of tetraka differed morphologically from one another and sexual dimorphism of varying degrees was apparent (Figures 3A–D; Table 2; Supplementary Table S1). Grey-crowned and Spectacled Tetrakas were morphologically similar regarding their tarsi and wing lengths, but regarding bill length, the Grey-crowned Tetraka was closer to the Wedge-tailed Tetraka, whereas the Spectacled Tetraka was more similar to the White-throated Oxylabes. Very strong sexual dimorphism was apparent in Long-billed Tetrakas, where males had much longer tarsi, wings and bills than females. To a lesser extent, this was also the case for White-throated Oxylabes. Spectacled Tetraka females also had shorter wings and bills than males, while for the Grey-crowned Tetraka this was only the case for wing length. Contrastingly, bill width was very similar for both sexes in all species and was therefore not a good predictor of sexual dimorphism. Wedge-tailed Tetrakas exhibited smaller values for all measurements. Therefore, the Wedge-tailed Tetraka was excluded from the subsequent analyses that aimed at differentiating species of very similar morphology.
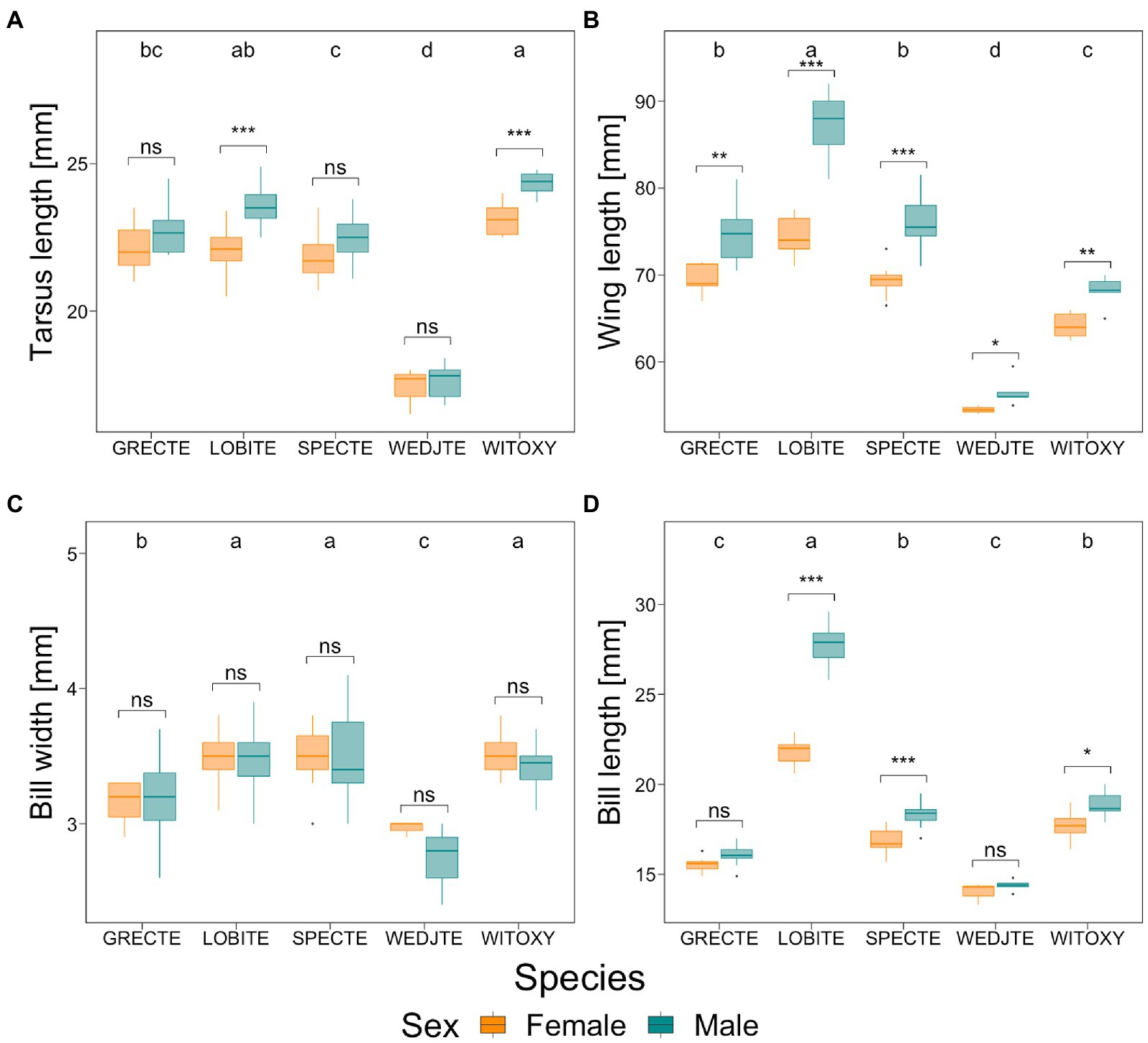
Figure 3. Tarsus length (A), wing length (B), bill width (C) and bill length (bill to skull) (D) of males (green) and females (orange) in five species of tetrakas. Brackets indicate differences between males and females calculated using Welch’s T-tests (Table 2). Significance levels: <0.001 = ***; <0.01 = **; <0.05 = *; ≥0.05 = ns. Sample sizes: GRECTE: females (f) = 7, males (m) = 10; LOBITE: f = 21, m = 23; SPECTE: f = 11, m = 19; WEDJTE: f = 3, m = 5; WITOXY: f = 9, m = 6. The classification into groups through one-way ANOVAs and Tukey’s HSD tests are indicated as a–d (Supplementary Table S1). For species abbreviations, see Table 1.
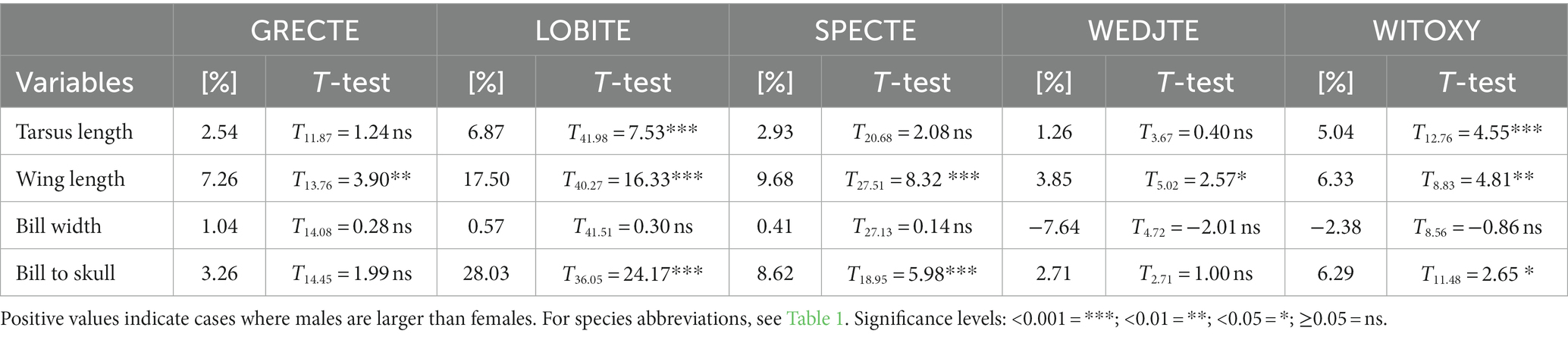
Table 2. Sexual dimorphism (%) in four morphological variables and results of pairwise Welsh’s T-tests of these measures between males and females.
The two supervised classification analyses of the four morphometrically closest species (LDA and QDA) showed an overlap between Grey-crowned and Spectacled Tetrakas (as well as female Long-billed Tetrakas to a lesser degree; Figure 4; Table 3; Supplementary Tables S2, S3). White-throated Oxylabes remained separated, implying the morphological distinctiveness of the species. A strong diffused clustering of the male and female Long-billed Tetrakas was apparent, even without taking sex into account in the analysis (Figure 4), illustrating the sexual dimorphism. In all species except for White-throated Oxylabes, females had a lower species assignment accuracy, but this was particularly evident for Grey-crowned and Spectacled Tetrakas (Table 3). Nonetheless, our models using only four morphometric measurements were very robust toward classifying each species, accordingly, indicating a strong segregation in the morphological space (Supplementary Table S3). The QDA showed a higher prediction accuracy than the LDA (96.24%, versus 93.98%).
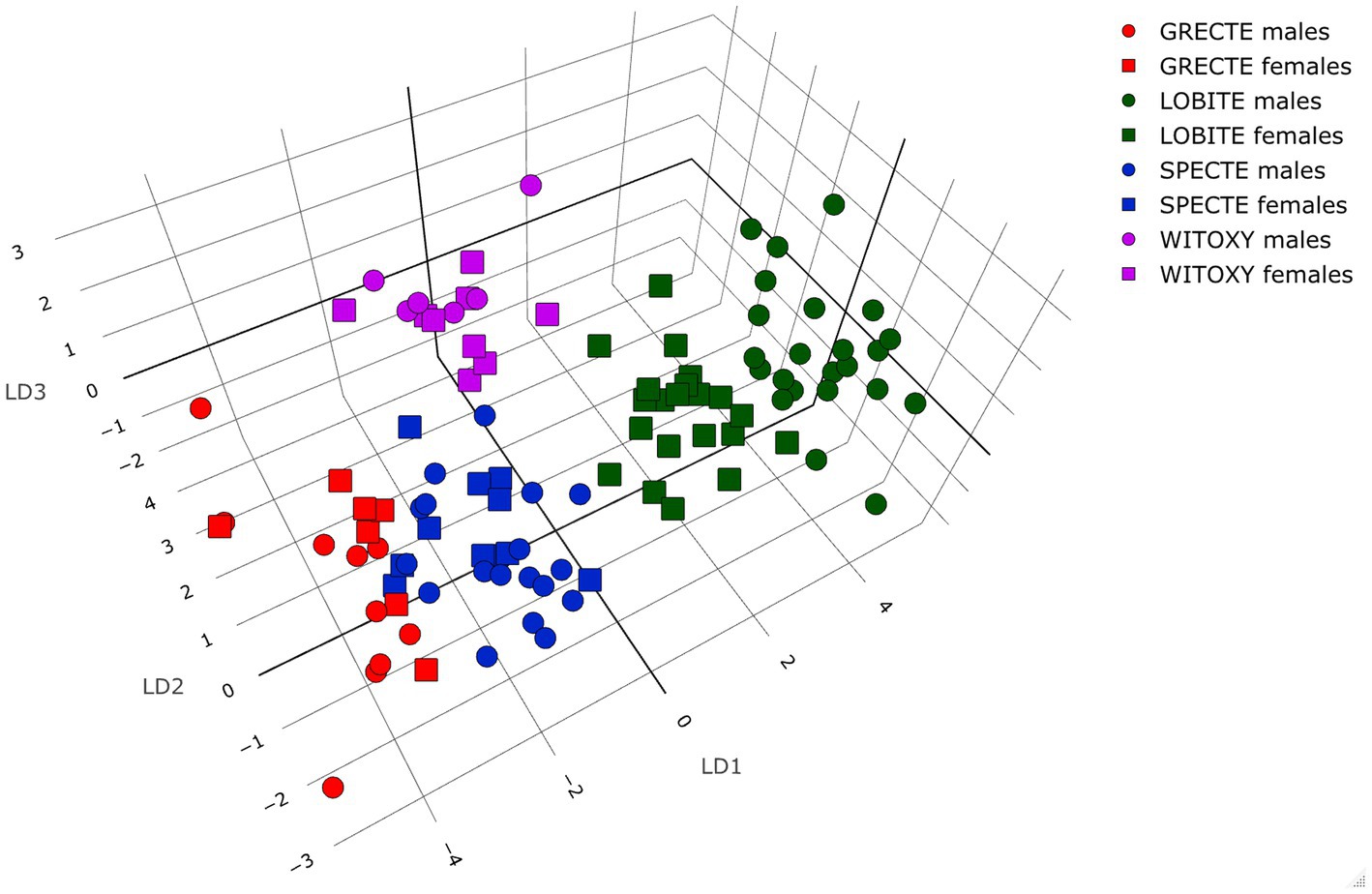
Figure 4. Linear discriminant analysis (LDA) showing clustering by length of tarsus and wing, bill width and bill length of the four morphologically closest species. Assignment accuracy per analysis (%) is shown in Supplementary Table S3. For species abbreviations, see Table 1.
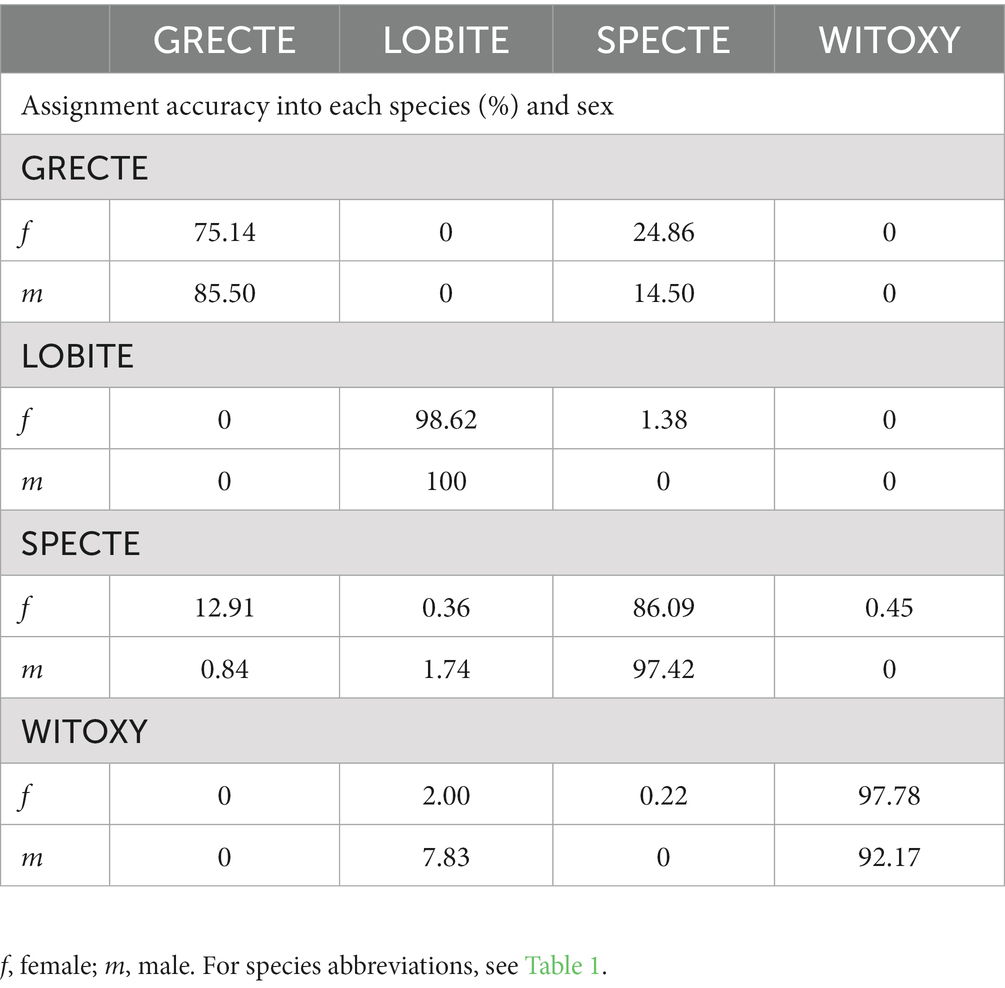
Table 3. Individual assignment accuracy (%) of the Quadratic Discriminant Analysis of (n = 205) into each supervised grouping variable (namely the species in this analysis), which was sorted by sex and species.
Stable isotopes
The stable isotope ratios in flank feathers from the four species of tetraka showed a wide variation [ranges (‰): δ13C: −26.30 to −23.26; δ15N: 5.11 to 10.29; δ34S: 16.18 to 18.79] even between males and females of the same species (Figure 5). Overall, Long-billed Tetrakas had higher values of δ13C than all the other species. On the other hand, ground-dwelling species like the White-throated Oxylabes and the Spectacled Tetraka showed much lower δ13C values and higher δ15N values, whereas δ15N values in Long-billed Tetraka feathers were lower on average. Male and female Spectacled Tetrakas showed a considerable overlap in their δ15N isotopic values.
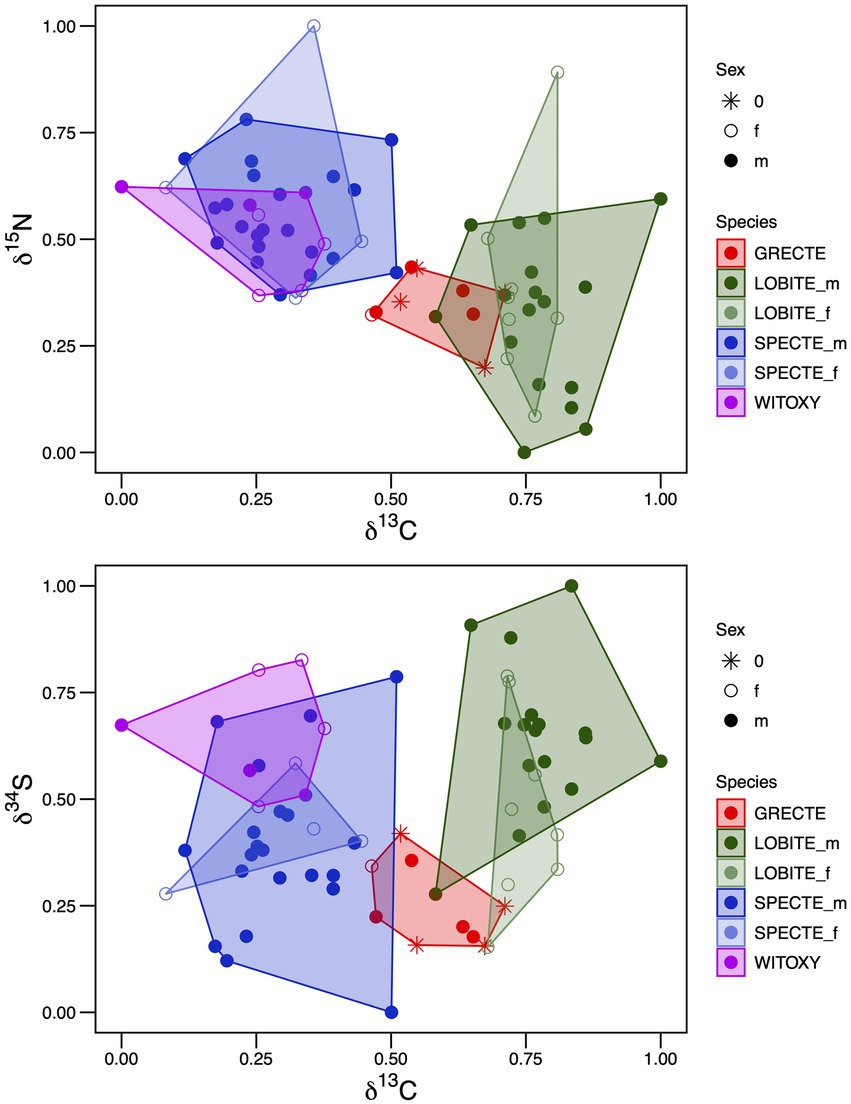
Figure 5. Scaled flank feather stable isotope results (δ13C, δ15N, and δ34S) showing isotopic niche of four Tetraka species. f = female, m = male, 0 = sex unidentified. For species abbreviations, see Table 1.
A comparison of the δ34S data between the four species showed a different trend. On average, White-throated Oxylabes exhibited higher values compared to other ground dwelling species, among these, Grey-crowned Tetrakas had the lowest values. In both species, the males exhibited a much larger δ34S niche than the females. Altogether, Grey-crowned Tetrakas had the smallest spread and the least overlap with the other four tetraka species. Pairwise comparison of species flank feather stable isotope similarity and nestedness using three elements (δ13C, δ15N, and δ34S) showed low isotopic similarity and nestedness (Table 4). Nonetheless, Spectacled Tetrakas and White-throated Oxylabes had a 62% overlap, while Grey-crowned and Spectacled Tetrakas shared 19% of the three-element isotopic niche area. Long-billed and Spectacled Tetrakas had the highest values of total isotopic area and SEAc (Supplementary Table S4).
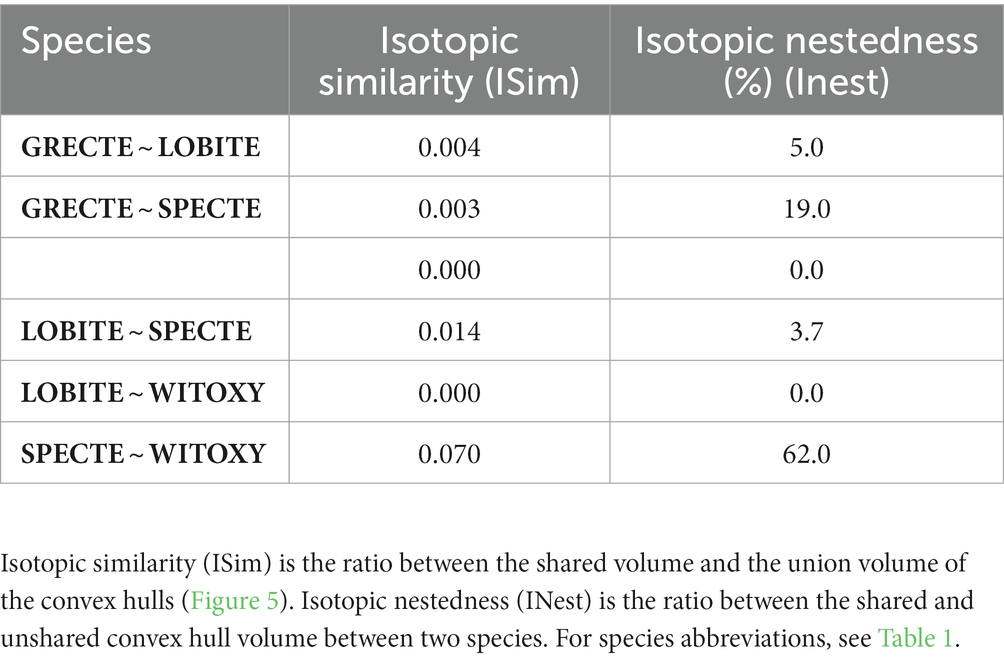
Table 4. Pairwise comparison of species flank feather isotopic overlap metrics (based on convex hull volume) in three dimensions (δ13C, δ15N, and δ34S).
Morphometry combined with stable isotope data
Wing and bill length were strongly (positively) correlated with higher δ13C values, while being negatively correlated with δ15N values to a lesser extent (Supplementary Table S5).
δ34S values seemed to be very similarly positively correlated with both bill dimensions. This can also be seen in the loading and eigenvectors of the PCA (Figure 6 and Supplementary Table S6), in which δ15N stands out by itself. δ13C and wing length, and δ34S and bill width are very closely linked, respectively, and of similar relative importance in the first two principal axes, while bill length is linked with both in both axes. Having the smallest eigenvectors in the first two principal axes, tarsus length does not seem to be a useful variable for segregation of the groups at first. But in PC3, which represents about 13% of the variance, its eigenvector is by far the highest, indicating its relative importance in the multidimensional scale. Supplementary Figures S1A,B shows variation of isotope values by species and sex groups.
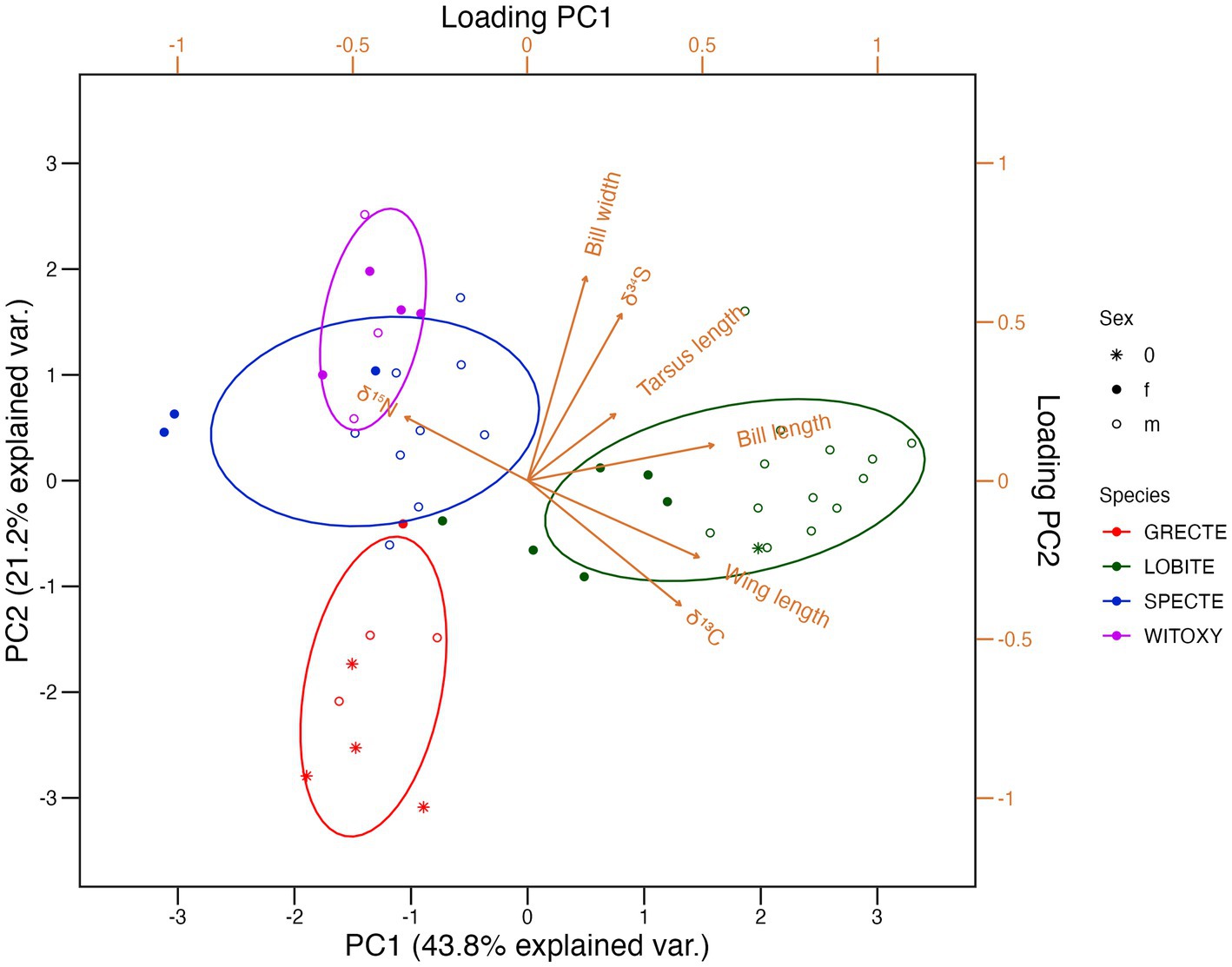
Figure 6. Principal component analysis biplot of the morphological and stable isotope data. Species and sexes were not included in the analysis and only displayed for graphical analysis purposes. Correlation values are given in Supplementary Table S5. The proportion of variation explained by each PCA axis, and the relative importance of each variable are given in Supplementary Table S6. f = female, m = male, 0 = sex unidentified. For species abbreviations, see Table 1.
Discussion
The five tetraka species studied herein, all members of a bird family endemic to Madagascar and representing an adaptive radiation, showed considerable differences in morphological characters, exhibited varying degrees of sexual dimorphism, and had low isotopic similarity and limited isotopic niche overlap. The observed trait-based niche differentiation in tetrakas leads to the assumption that at Maromizaha, each of the five species (and individuals within the species) might consume a subset of the resources locally available, a phenomenon which could be described as species diet specialization in relation to morphology. Such differentiation might play a pivotal role in the diversification, adaptation and speciation in tropical birds. It is possible that these species have overlapping diets acquired from different strata of the forest canopy. This could explain why there is dietary variation among these tropical birds, with differences in the utilization of vertical forest strata playing a critical role in establishing unique niches (Frith, 1984).
Long-billed Tetrakas were the most widely distributed, surprisingly even using a dry quarry outside the forest, whereas all other taxa were restricted to native forest with little degradation. Within the forest, most tetraka species were caught in the lower strata below 2 m and very rarely above 2.5 m, with only Long-billed Tetrakas regularly using higher forest strata. Long-billed Tetrakas seem to have a larger ecological niche, as they have been observed to venture beyond the forest and utilize higher strata, which is different from the behavior of other tetraka species investigated in this study. Additionally, the morphological differentiation of this species is more pronounced than anticipated, particularly with regards to their tarsus, wings, and bill length. Each of these measures has been attributed to specialization regarding avian feeding ecology and behavior. For instance, differences in bill morphology point toward how species or sexes within a species specialize in the prey they feed on (Leisler and Winkler, 1985, 1991), shorter wings may facilitate moving through dense undergrowth (Winkler and Leisler, 1985; Forstmeier et al., 2001), whilst more robust tarsi enable birds to have a stronger grip on vegetation and substrate such as branches (Winkler, 1988; Leisler et al., 1989) and may provide insight into the types of perching substrates used by the birds. Males were often larger than females and this was most striking in the Long-billed Tetraka, where males had 7% longer tarsi, 18% longer wings and impressively 28% longer bills than females. The other tetraka species also showed sexual size dimorphism in multiple morphological measures, including the wing, tarsus, and beak, which point toward possible resource partitioning between sexes.
Regarding stable isotopes, there were only slight differences in δ15N, as expected by a comparison between insectivorous taxa, however, species occurring closer to the ground had higher δ15N values, indicating that their prey may be higher up in the food chain. Spiders, for example, should have higher δ15N values as they prey on arthropods and are abundant in leaf litter (Nyffeler, 1999). However, elevated δ15N values may indicate not only trophic level but also the patterns of nitrogen cycling in the ecosystem. Different habitats can have varying levels of nitrogen availability and cycling rates, which can affect the δ15N values of organisms living in those habitats (Craine et al., 2009).
Comparison of the δ34S data between the four species shows an overall similar trend, with higher values of δ34S for the ground-dwelling White-throated Oxylabes, but interestingly also for the Long-billed Tetrakas, which uses higher strata more often than the other species. This may indicate differences in protein sources and sulfur-containing amino acids in diet such as cysteine and methionine (Brosnan and Brosnan, 2006), the two principal sulfur-containing building blocks of proteins. However, additional research is necessary to confirm this hypothesis, for example, through the study of protein and amino acid specific stable isotopes found in insect prey.
The canopy effect describes vertical variation in δ13C within plants throughout the forest canopy (van der Merwe and Medina, 1991). Long-billed Tetrakas had the highest δ13C values, which may be due to their use of higher strata in the canopy. Significant variation in δ13C in response to height in combination with light availability and tree species was expected, as leaves in lower strata of the forest tend to exhibit lower δ13C values compared to those in higher strata. While this vertical isotopic variation was not tested using plant leaves and insect consumers, the effect was expected to be directly reflected in the δ13C of avian insect diet in the forest. Based on the triple elemental dissimilarity in the four tetraka species, we argue that some prey taxa were commonly consumed by one species and not others. Alternatively, the tetraka species might be preying on different taxa at the same trophic level. The correlations between wing and bill length and δ13C and δ15N values provides evidence that individual tetrakas may segregate into different habitat structures based on a combination of their physical characteristics and food preferences. Potential ecological factors that may contribute to this habitat segregation may include differences in microhabitat preference.
Thus, marginal nestedness in isotopic niche space indicates that the species use unique resources, enhancing their ability to avoid competition and the means for niche partitioning. We are aware that assumed dietary segregation was not assessed concurrently with resource abundance, which is likely to influence the degree of partitioning (Holmes et al., 1986). More data is needed on this.
The observation of incomplete niche overlap and distinct isotopic signatures can be interpreted as evidence for the absence of strong competitive interactions between the organisms in question. Yet, the enhanced dissimilarities in isotope values could parsimoniously be explained by species foraging non-opportunistically as specialists, targeting specific prey items. Nevertheless, since there is a lack of detailed information on the diet and insect species involved, this assumption remains uncertain. The presence of differences and dissimilarities in these sympatric species appears to be essential to species coexistence, at least during the molting season. Insofar the presence of resource partitioning is not expected to lead to exclusion in a resource-rich system. Yet, if prey is scarce and resources are depleted, particularly during the dry season, it can skew the resource utilization pattern if molting coincides with this period. Overall, the isotopic distributions are consistent with well-known stable isotope distribution patterns along vertical strata in forest ecosystems (Van der Merwe and Medina, 1991), supporting the prediction that similar endemic species partitioned their resources and habitat use in a complex manner.
Tetrakas varied in their morphology and isotopic niches, which could be described as ecological specializations, presumably necessary for coexistence. Some species showed a certain level of overlap in resource use, with modest resource specialization. The nature of this resource segregation, coupled with the data on morphological traits, provided evidence that birds occupy different niches to avoid dietary competition. In groups with high resource overlap with complementary distinct specializations, it is often understood that coexistence is explained as species being imperfect generalists with “own” resources available to individuals or species (Sánchez-de León et al., 2014). In fact, this might be linked to morphological traits. Elsewhere, this pattern is referred to as Liem’s Paradox, where species show foraging specializations that permit resource access to unique prey items but still exhibit high niche overlap (Liem, 1980; Bandl et al., 2015; Golcher-Benavides and Wagner, 2019). This might well be the case for the Spectacled Tetraka and its potential competitor, the White-throated Oxylabes, since they had an overlap of about 62% in the three dietary elements.
While the capture height data in this study is only qualitative and cannot be statistically linked to the isotope data, it can provide additional insight into the strata that the birds utilized, along with observations from previously published studies on foraging heights. Evidently, tetrakas enhance the likelihood of coexistence by dividing foraging spaces at a microhabitat level and not necessarily resources partitioning per se (e.g., Schoener, 1974). These results complement the concept of species utilizing resources that other competitors could not, presumably due to morphological or behavioral constraints (e.g., Robinson and Wilson, 1998; Sánchez-de León et al., 2014). It could well be expected that this may lead to a coexistence mechanism in the tetraka species, generating sex-based morphometric and isotopic niche differentiation within the larger niche space (Wilson, 2010).
In Madagascar, when not breeding, insectivorous birds of different sizes, taxonomic groups and dietary preferences are known to form mixed-species flocks (Rand, 1936), which are characterized by two or more individuals moving together to forage, profiting (1) from the disturbance created by the other members of the flock, i.e., by flushing up insects and (2) from increased vigilance with increasing flock size to detect predators. Most tetraka species are known to take part in such flocks (Safford and Hawkins, 2013). This highly specialized cooperative behavior, coupled with the dissimilarities in their respective morphometric and isotopic spaces, could be an indication of their shared sympatric coevolutionary history. We assume that the co-adaptation of these species is based on niche differentiation, rather than outcompeting one another. This theory needs to be corroborated by detailed foraging studies and more precise data on the diet and habitat use of the tetrakas, including more species.
Morphological differences between the sexes were also reflected in their isotopic niches. The female isotopic niche was only part of the male niche in at least two species, the Long-billed and the Spectacled Tetraka. Males of at least two species (Long-billed and Spectacled Tetraka) appear to have extended niches, interestingly, away from the isotopic niches of the other taxa, which suggests that this leads to a reduction in competition for food but more data is needed. Long-billed and Spectacled Tetrakas had a wide range of δ34S values implying a diverse sulfur containing dietary origins. Male Long-billed Tetrakas had heavier bills and it is very likely they prey on larger insects relative to females, presumably contributing to trophic niche extension in males. Thus, it could be argued that species dietary selection plays an important role in micro-habitat partitioning (vertical spatial distribution) and toward avoiding competition and therefore modulating the patterns of spatial use and coexistence between the sexes. Moreover, these data complement the assumption that niche overlaps and intraspecific competition might be minimized by increased specialization enforced through sexual dimorphism (e.g., Pyke et al., 1977). These and additional assumptions described here are solely based on morphology and isotope values obtained during the feather growing season. To validate this, repeated and long-term empirical data as well as evidence for the differences in prey items taken by individuals of the same species are essential (Roughgarden, 1972 Bolnick et al., 2002 Bolnik et al., 2007). Furthermore, stable isotope composition of the different species of insect prey needs to be studied in the different forest strata across different seasons and habitats. Morphometric data are crucial for the description of a species’ niche. Detailed assessment of diet and foraging behaviors are required to infer the sources of differences in morphometry. Identifying which ecological and evolutionary factors are the most important drivers of species specialization is pivotal in understanding how these ecological variations are promoted and sustained.
Conclusion
We report considerable variation in isotopic niche positions, niche breadth and interspecific niche overlap in tetraka species that exhibit a close phylogenetic relationship. Morphological traits coupled with potential dietary groups and micro-habitat trends provided explanations of patterns of isotopic niche areas and positions. We conclude that dietary segregation may be based on specialized foraging strata and species “spatial-resource” specialization. The measurable differences found among these related species could be considered stratified resource use along the vertical canopy gradient, indicating habitat-based structural niches in tetrakas that might assist in reducing interspecific competition. These results refine knowledge of the species and their ecology. Even minor differences in the degree of species specialization among related taxa may have important implications for trophic structure. While disentangling these causes and differences is beyond the scope of this study, these results offer insights into the evolution of differential resource utilization through comparison of both phenotype and ecological aspects of endemic species in a tropical rainforest community. Finally, our results emphasize the efficacy of these proposed coexistence mechanisms through spatial segregation and therefore variation in nutrient acquisition. Future studies should examine the role of coexistence as a flexible strategy in complex, multi-species communities with phylogenetically closely related species. Studies that do not focus on a species’ sex groups may miss important ecological relationships, such as differences in resource use between sex groups. More detailed mechanisms can be elucidated by conducting further studies with larger sample sizes per species and sex group in multiple tetraka species across a wider geographic scale. Additionally, information on the isotopic signatures of plants and invertebrates in their habitats would be useful in interpreting the findings further.
Data availability statement
The raw data supporting the conclusions of this article will be made available by the authors, without undue reservation.
Author contributions
J-LB, EY, and FW conceived and designed this study and prepared and edited the manuscript. FW collected the samples in the field and took all the measurements. EY performed the stable isotope analyses at the Stable Isotope Lab of the University of Konstanz. J-LB performed the laboratory work for molecular sexing at the Natural History Museum Stuttgart. J-LB and EY performed the morphological and stable isotope data analyses. All authors have read and agreed to the published version of the manuscript.
Funding
This project was supported by funding by the “Ausschuss für Forschungsfragen” from the University of Konstanz.
Acknowledgments
We are indebted to the Malagasy authorities for granting all the relevant research and export permits (available upon request) and to the Groupe d’Étude et de Recherche sur les Primates de Madagascar (GERP), namely Jonah Ratsimbazafy and Rose Marie Randrianarison, for letting us work at Maromizaha. Hajanirina Rakotomanana, Daniel Rakotondravony, and Zafimahery Rakotomalala at the Department of Animal Biology, University of Antananarivo supported us throughout the field study. We thank Jean-Robert Lekamisi, Lova Tahiry Rasolondraibe, Onja Randriamalala, Nicola Lillich, Pia Reufsteck and all the other assistants for their help in the field. We thank Wolfgang Kornberger and Claudia Greis for the assistance in the stable isotope lab. We are especially grateful to Jonah Ulmer for language editing and for commenting on earlier drafts of the manuscript. We thank Karl-Otto Rothhaupt for all the support. Finally, we thank the three reviewers for their valuable comments and suggestions that helped to improve the manuscript. All field research, collection of bird samples (specimens released after sampling) and their export was approved by the Madagascan Ministère de l’Environnement, des Eaux et des Forêts (Direction des Eaux et Forêts, DEF) now la Direction de la Préservation de la Biodiversité, under the following permits: 10 September 2003 (No. 0182 et 0184/MINENVEF/SG/DGEF/DPB/SCBLF); 19 October 2004 (No. 234/MINENVEF/SG/DGEF/DPB/SCBLF/RECH); 4 November 2005 (No. 262 et 261/MINENVEF/SG/DGEF/DPB/SCBLF/RECH); 21 November 2006 (No. 275 et 276/MINENVEF/SG/DGEF/DPB/SCBLF/RECH); 4 December 2007 (No. 0296/07/MEEFT/SG/DGEF/ DPSAP/SSE); 19 November 2010 (No. 335/10/MEF/SG/ DGF/DCB.SAP/SCB; renewal of No. 296/07); 8 November 2012 (No. 284/12/MEF/SG/DGF/DCB.SAP/SCB); 7 October 2014 (No. 265/14/MEEF/SG/DGF/DCB.SAP/ScB); 11 October 2016 (No. 204/16/MEEF/SG/DGF/DCB.SAP/ScB.Re); 13 November 2018 (No. 279/18/MEEF/SG/DGF/DCB.SAP/ScB.Re).
Conflict of interest
The authors declare that the research was conducted in the absence of any commercial or financial relationships that could be construed as a potential conflict of interest.
Publisher’s note
All claims expressed in this article are solely those of the authors and do not necessarily represent those of their affiliated organizations, or those of the publisher, the editors and the reviewers. Any product that may be evaluated in this article, or claim that may be made by its manufacturer, is not guaranteed or endorsed by the publisher.
Supplementary material
The Supplementary material for this article can be found online at: https://www.frontiersin.org/articles/10.3389/fevo.2023.1082226/full#supplementary-material
References
Arneson, L. S., and MacAvoy, S. E. (2005). Carbon, nitrogen, and sulfur diet-tissue discrimination in mouse tissues. Can. J. Res Section D Zool. Sci. 83, 989–995. doi: 10.1139/z05-083
Bandl, S. J., Williams, D. R., and Bellwood, D. R. (2015). Exploring the nature of ecological specialization in a coral reef fish community: morphology, diet and foraging microhabitat use. Proc. R. Soc. B 282:1147. doi: 10.1098/rspb.2015.1147
Benjara, A., de Roland, L. A. R., Rakotondratsima, M., and Thorstrom, R. (2021). Effects of tropical rainforest fragmentation on bird species: a case study from the Bemanevika protected area, northwestern Madagascar. Ostrich 92, 257–269. doi: 10.2989/00306525.2021.2005704
Benson, C. W., Colebrook-Robjent, J. F. R., and Williams, A. (1976). Contribution à l'ornithologie de Madagascar. Oiseau Rev. Franç. Ornithol. 46, 103–386.
Beresford, P., Barker, F. K., Ryan, P. G., and Crowe, T. M. (2005). African endemics span the tree of songbirds (Passeri): molecular systematics of several evolutionary "enigmas". Proc. Royal Soc. B. 272, 849–858. doi: 10.1098/rspb.2004.2997
Bickford, D., Lohman, D. J., Sodhi, S. N., Ng, K. L. P., Meier, R., Winker, K., et al. (2007). Cryptic species as a window on diversity and conservation. Trends Ecol. Evol. 22, 148–155. doi: 10.1016/j.tree.2006.11.004
Block, N. L. (2012). Cryptic diversity and phylogeography in the Bernieridae, an endemic Malagasy passerine radiation. The University of Chicago. Dissertations Publishing.
Block, N. L., Goodman, S. M., Hackett, S. J., Bates, J. M., and Raherilalao, M. J. (2015). Potential merger of ancient lineages in a passerine bird discovered based on evidence from host-specific ectoparasites. Ecol. Evol. 5, 3743–3755. doi: 10.1002/ece3.1639
Bolnick, D. I., Yang, L. H., Fordyce, J. A., Davis, J. M., and Svanbäck, R. (2002). Measuring individual-level resource specialization. Ecology. 83, 2936–2941.
Bolnik, D. I., Svanback, R., Araujo, M., and Persson, L. (2007). Comparative support for the niche variation hypothesis that more generalized populations also are more heterogeneous. Proc. Nat. Acad. Sci. 104, 10075–10079.
Borcard, D., Gillet, F., and Legendre, P. (2011). Numerical ecology with R. New York: Springer-Verlag.
Brosnan, T. G., and Brosnan, E. M. (2006). The Sulphur-containing amino acids: an overview. J. Nutr. 136, S1636–S1640. doi: 10.1093/jn/136.6.1636S
Bryan, J. (2017). gapminder: Data from Gapminder. R package version 0.3.0. Available at https://CRAN.R-project.org/package=gapminder
Çakmak, E., Akın Pekşen, Ç., and Bilgin, C. C. (2017). Comparison of three different primer sets for sexing birds. J. Vet. Diagn. Investig. 29, 59–63. doi: 10.1177/1040638716675197
Cibois, A., David, N., Gregory, S. M. S., and Pasquet, E. (2010). Bernieridae (Aves: Passeriformes): a family-group name for the Malagasy sylvioid radiation. Zootaxa 2554:65. doi: 10.11646/zootaxa.2554.1.6
Cibois, A., Pasquet, E., and Schulenberg, T. S. (1999). Molecular systematics of the Malagasy babblers (Passeriformes: Timaliidae) and warblers (Passeriformes: Sylviidae), based on cytochrome b and 16S rRNA sequences. Mol. Phyl. Evol. 13, 581–595. doi: 10.1006/mpev.1999.0684
Cibois, A., Slikas, B., Schulenberg, T. S., and Pasquet, E. (2001). An endemic radiation of Malagasy songbirds is revealed by mitochondrial DNA sequence data. Evolution 55, 1198–1206. doi: 10.1111/j.0014-3820.2001.tb00639.x
Craine, J. M., Elmore, A. J., Aidar, M. P. M., Bustamante, M., Dawson, T. E., Hobbie, E. A., et al. (2009). Global patterns of foliar nitrogen isotopes and their relationships with climate, mycorrhizal fungi, foliar nutrient concentrations, and nitrogen availability. New Phytol. 183, 980–992. doi: 10.1111/j.1469-8137.2009.02917.x
Cucherousset, J., and Villéger, S. (2015). Quantifying the multiple facets of isotopic diversity: new metrics for stable isotope ecology. Ecol. Indic. 56, 152–160. doi: 10.1016/j.ecolind.2015.03.032
da Silva, A. R. (2021). Biotools: Tools for biometry and applied statistics in agricultural science. R package version, vol. 4, 2. Available at https://cran.r-project.org/package=biotools
Date, Y., Managave, S., Jathar, G., Khot, R., and Hobson, K. A. (2022). Stable Sulphur isotope (δ34S) ratios in bird feathers from India indicate strong segregation between the Himalaya and Gangetic plain, and the rest of India. Isot. Environ. Health Stud. 58, 327–339. doi: 10.1080/10256016.2022.2113995
de Mendiburu, F. (2021). Agricolae: statistical procedures for agricultural research. R package version, vol. 1, 3–5. Available at http://CRAN.R-project.org/package=agricolae
Dowle, M., and Srinivasan, A. (2021). Data.Table: Extension of ‘data.Frame’. R package version 1.14.2. Available at https://CRAN.R-project.org/package=data.table
Eck, S., Fiebig, J., Fiedler, W., Heynen, I., Nicolai, B., Töpfer, T., et al. (2011). Measuring Birds-Vögel vermessen. Radolfzell: Deutsche Ornithologen-Gesellschaft.
Eguchi, K., Yamagishi, S., and Randorianasolo, V. (1993). The composition and foraging behaviour of mixed-species flocks of forest-living birds in Madagascar. Ibis 135, 91–96. doi: 10.1111/j.1474-919X.1993.tb02814.x
Evans, M. I., Duckworth, J. W., Hawkins, A. F. A., Safford, R. J., Sheldon, B. C., and Wilkinson, R. J. (1992). Key bird species of Marojejy strict nature reserve. Bird Conserv. Int. 2, 201–220. doi: 10.1017/S0959270900002446
Faliarivola, M. L., Raherilalao, M. J., Andrianarimisa, A., and Goodman, S. M. (2020). The diet of Malagasy dry forest understory birds based on faecal samples. Ostrich 91, 35–44. doi: 10.2989/00306525.2019.1661309
Fletcher, T.D. (2022). QuantPsyc: Quantitative psychology tools. R package version. Available at: https://CRAN.R-project.org/package=QuantPsyc
Florin, S. T., Felicetti, L. A., and Robbins, C. T. (2011). The biological basis for understanding and predicting dietary-induced variation in nitrogen and sulphur isotope ratio discrimination. Funct. Ecol. 25, 519–526. doi: 10.1111/j.1365-2435.2010.01799.x
Forstmeier, W., Bourski, O. V., and Leisler, B. (2001). Habitat choice in Phylloscopus warblers: the role of morphology, phylogeny and competition. Oecologia 128, 566–576. doi: 10.1007/s004420100678
Fox, J., and Weisberg, S. (2019). An {R} companion to applied regression, 3rd. Thousand Oaks CA: Sage
Frith, D. W. (1984). Foraging ecology of birds in an upland tropical rainforest in North Queensland. Aust. Wildlife Res. 11, 325–347. doi: 10.1071/WR9840325
Golcher-Benavides, J., and Wagner, C. E. (2019). Playing out Liem's paradox: opportunistic Piscivory across Lake Tanganyikan cichlids. Am. Nat. 194, 260–267. doi: 10.1086/704169
Goodman, S. M., Langrand, O., and Whitney, B. M. (1996). A new genus and species of passerine from the eastern rainforest of Madagascar. Ibis 138, 153–159. doi: 10.1111/j.1474-919X.1996.tb04322.x
Goodman, S. M., and Parrillo, P. (1997). A study of the diets of malagasy birds based on stomach contents. Ostrich 68, 104–123. doi: 10.1080/00306525.1997.9639723
Goodman, S., Tello, J., and Langrand, O. (2000). Patterns of morphological and molecular variation in Acrocephalus newtoni on Madagascar. Ostrich 71, 367–370. doi: 10.1080/00306525.2000.9639832
Griffith, O. W. (1987). Mammalian sulfur amino acid metabolism: an overview. Methods Enzymol. 143, 366–376. doi: 10.1016/0076-6879(87)43065-6
Habel, K., Grasman, R., Gramacy, R., Mozharovskyi, P., and Sterratt, D. (2022). Geometry: Mesh generation and surface tessellation. Available at https://CRAN.R-project.org/package=geometry
Hardin, G. (1960). The competitive exclusion principle. Science 131, 1292–1297. doi: 10.1126/science.131.3409.1292
Hawkins, F., Safford, R., and Skerrett, A. (2015). Birds of Madagascar and the Indian Ocean Islands. London, UK: Christopher Helm.
Hawkins, A. F. A., Thiollay, J.-M., and Goodman, S. M. (1998). The birds of the reserve Spéciale d'Anjanaharibe-Sud Madagascar. Fieldiana. Zoology 90, 93–127.
Hino, T. (1998). Mutualistic and commensal organization of avian mixed-species foraging flocks in a forest of western Madagascar. J. Avian Biol. 29, 17–24. doi: 10.2307/3677336
Hobson, K. (1999). Tracing origins and migration of wildlife using stable isotopes: a review. Oecologia 120, 314–326. doi: 10.1007/s004420050865
Holmes, R. T., Sherry, T. W., and Sturges, F. W. (1986). Bird community dynamics in a temperate deciduous forest: long-term trends at Hubbard brook. Ecol. Monogr. 56, 201–220. doi: 10.2307/2937074
Hutchinson, G. E. (1957). Concluding remarks. Cold spring harbor symposia on quant. Biol. 22, 415–427. doi: 10.1101/SQB.1957.022.01.039
Iannone, R., Cheng, J., Schloerke, B., and Hughe, E. (2022). Gt: Easily create presentation-ready display tables. R package version 0.7.0 Available at https://CRAN.R-project.org/package=gt
Inger, R., and Bearhop, S. (2008). Application of stable isotope analyses to avian ecology. Ibis 150, 447–461. doi: 10.1111/j.1474-919X.2008.00839.x
Jackson, A. L., Inger, R., Parnell, A. C., and Bearhop, S. (2011). Comparing isotopic niche widths among and within communities: SIBER−stable stable isotope Bayesian ellipses in R. J. Anim. Ecol. 80, 595–602. doi: 10.1111/j.1365-2656.2011.01806.x
Jønsson, K. A., Fabre, P.-H., Fritz, A. S., Etienne, R. S., Ricklefs, R. E., Jørgensen, T. B., et al. (2012). Ecological and evolutionary determinants for the adaptive radiation of the Madagascan vangas. Proc. Nat. Acad. Sci. 109, 6620–6625. doi: 10.1073/pnas.1115835109
Karasov, W. H., and Martínez del Rio, C. (2007). Physiological ecology. How animals process energy, nutrients, and toxins. Princeton and Oxford: Princeton University Press. 741
Kelly, J. F. (2000). Stable isotopes of carbon and nitrogen in the study of avian and mammalian trophic ecology. Can. J. Zool. 78, 1–27. doi: 10.1139/z99-165
Layman, C. A., Arrington, D. A., Montana, C. G., and Post, D. M. (2007). Can stable isotope ratios provide for community-wide measures of trophic structure? Ecology 88, 42–48. doi: 10.1890/0012-9658(2007)88[42:CSIRPF]2.0.CO;2
Lee, J. C. I., Tsai, L. C., Hwa, P. Y., Chan, C. L., Huang, A., Chin, S. C., et al. (2010). A novel strategy for avian species and gender identification using the CHD gene. Mol. Cell. Probes 24, 27–31. doi: 10.1016/j.mcp.2009.08.003
Leisler, B., Ley, H. W., and Winkler, H. (1989). Habitat, behaviour and morphology of Acrocephalus warblers: an integrated analysis. Ornis Scand. 20, 181–186. doi: 10.2307/3676911
Leisler, B., and Winkler, H. (1985). Ecomorphology. Curr. Ornithol. 2, 155–186. doi: 10.1007/978-1-4613-2385-3_5
Leisler, B., and Winkler, H. (1991). Ergebnisse und Konzepte ökomorphologischer Untersuchungen an Vögeln. J. Ornithol. 132, 373–425. doi: 10.1007/BF01640381
Levins, R. (1968). Evolution in changing environments. Princeton University Press. Princeton, New Jersey
Liem, K. F. (1980). Adaptive significance of intra-and interspecific differences in the feeding repertoires of cichlid fishes. Integr. Comp. Biol. 20, 295–314. doi: 10.1093/icb/20.1.295
Lott, C. A., Meehan, T. D., and Heath, J. A. (2003). Estimating the latitudinal origins of migratory birds using hydrogen and sulfur stable isotopes in feathers: influence of marine prey base. Oecologia 134, 505–510. doi: 10.1007/s00442-002-1153-8
Lowry, B. E., Wittig, R. M., Pittermann, J., and Oelze, V. M. (2021). Stratigraphy of stable isotope ratios and leaf structure within an African rainforest canopy with implications for primate isotope ecology. Sci. Rep. 11:14222. doi: 10.1038/s41598-021-93589-8
MacArthur, R. H. (1972). “Coexistence of species” in Challenging biological problems. ed. A. J. Behnke (Oxford: Oxford Univ. Press), 253–259.
Mansor, M. S., and Ramli, R. (2017). Foraging niche segregation in Malaysian babblers (family: Timaliidae). PLoS One 12:e0172836. doi: 10.1371/journal.pone.0172836
Mansor, M. S., Rozali, F. Z., Davies, S., Nor, S. M., and Ramli, R. (2022). High-throughput sequencing reveals dietary segregation in Malaysian babblers. Curr. Zool. 68, 381–389. doi: 10.1093/cz/zoab074
McCutchan, J. H. Jr., Lewis, W. M. Jr., Kendall, C., and McGrath, C. C. (2003). Variation in trophic shift for stable isotopic ratios of carbon, nitrogen, and sulfur. Oikos 102, 378–390. doi: 10.1034/j.1600-0706.2003.12098.x
Morris, P., and Hawkins, F. (1998). Birds of Madagascar: A photographic guide. Robertsbridge, East Sussex: Pica Press.
Pianka, E. R. (1974). Niche overlap and diffuse competition. Proc. Natl. Acad. Sci. U. S. A. 71, 2141–2145. doi: 10.1073/pnas.71.5.2141
Procházka, P., Reif, J., Hořák, D., Klvaňa, P., Lee, R. W., and Yohannes, E. (2010). Using stable isotopes to trace resource acquisition and trophic position in four Afrotropical birds with different diets. Ostrich 81, 273–275. doi: 10.2989/00306525.2010.519889
Pyke, G. H., Pulliam, H. R., and Charnov, E. L. (1977). Optimal foraging: a selective review of theory and tests. Q. Rev. Biol. 52, 137–154. doi: 10.1086/409852
R Core Team (2022). R: A language and environment for statistical computing. R Foundation for Statistical Computing, Vienna, Austria. Available at: https://www.R-project.org/
Raherilalao, M. J., and Goodman, S. M. (2011). Histoire naturelle des familles et sous-familles endémiques d'oiseaux de Madagascar. Antananarivo: Association Vahatra.
Rajaonarivelo, J. A., Andrianarimisa, A., Raherilalao, M. J., and Goodman, S. (2020). Vertical distribution and daily patterns of birds in the dry deciduous forests of central western Madagascar. Tropical Zool. 33, 36–52. doi: 10.4081/tz.2020.66
Rajaonarivelo, J. A., Raherilalao, M. J., Andrianarimisa, A., and Goodman, S. M. (2021). Seasonal variation in the vertical distribution of birds in the dry deciduous forest of central western Madagascar. Wilson J. Ornithol. 133, 258–265. doi: 10.1676/20-00088
Rand, A. L. (1936). The distribution and habits of Madagascar birds. Bull. Am. Mus. Nat. Hist. 72, 143–499.
Reddy, S., Driskell, A., Rabosky, D. L., Hackett, S. J., and Schulenberg, T. S. (2012). Diversification and the adaptive radiation of the vangas of Madagascar. Proc. R. Soc. B Biol. Sciences 279, 2062–2071. doi: 10.1098/rspb.2011.2380
Reddy, S., Schulenberg, T. S., and Block, N. (2022). “Passeriformes: Bernieriedae, Tetrakas” in The new natural history of Madagascar. ed. S. Goodman (Princeton and Oxford: Princeton University Press)
Redfern, C. P. F., Clark, J. A., Wilson, A., Gough, S., and Robertson, D. (2001). Ringers' manual. Thetford: British Trust for Ornithology.
Remsen, J. V., and Robinson, S. K. A. (1990). Classification scheme for foraging behavior of birds in terrestrial habitats. Stud. Avian Biol. 13, 144–160.
Richards, M. P., Fuller, B. T., Sponheimer, M., Robinson, T., and Ayliffe, L. (2003). Sulphur isotopes in paleodietary studies: a review and results from a controlled feeding experiment. Int. J. Osteoarchaeol. 13, 37–45. doi: 10.1002/oa.654
Rizzo, M., and Szekely, G. (2022). Energy: E-statistics: Multivariate inference via the energy of data. R package version. Available at: https://CRAN.R-project.org/package=energy
Robinson, B. W., and Wilson, D. S. (1998). Optimal foraging, specialization and a solution to Liem's paradox. Am. Nat. 151, 223–235. doi: 10.1086/286113
Ross, S. T. (1986). Resource partitioning in fish assemblages: a review of field studies. Copeia 352–388. doi: 10.2307/1444996
Safford, R. J., Goodman, S. M., Raherilalao, M. J., and Hawkins, A. F. A. (2022). “Introduction to the 676 birds” in The new natural history of Madagascar. ed. S. Goodman, pp. 677, 1553–1602.
Safford, R., and Hawkins, F. (2013). The birds of Africa: Volume VIII: The Malagasy region: Madagascar, Seychelles, Comoros, Mascarenes. London, UK: Christopher Helm
Sánchez-de León, Y., Lugo-Pérez, J., Wise, D. H., Jastrow, J. D., and González-Meler, M. A. (2014). Aggregate formation and carbon sequestration by earthworms in soil from a temperate forest exposed to elevated atmospheric CO2: a microcosm experiment. Soil Biol. Biochem. 68, 223–230. doi: 10.1016/j.soilbio.2013.09.023
Schoener, T. W. (1974). Resource partitioning in ecological communities. Science 185, 27–39. doi: 10.1126/science.185.4145.27
Sievert, C. (2020). Interactive web-based data visualization with R, plotly, and shiny Chapman and Hall/CRC Florida.
Sinclair, I., and Langrand, O. (2013). Birds of the Indian Ocean Islands: Madagascar, Mauritius, Reunion, Rodrigues, Seychelles and the Comoros. Cape Town: Struik Publishers. 184
Sridhar, H., Beauchamp, G., and Shanker, K. (2009). Why do birds participate in mixed- species foraging flocks? A large-scale synthesis. Anim. Behav. 78, 337–347. doi: 10.1016/j.anbehav.2009.05.008
Symes, C. T., and Woodborne, S. M. (2010). Trophic level delineation and resource partitioning in a south African afromontane forest bird community using carbon and nitrogen stable isotopes. Afr. J. Ecol. 48, 984–993. doi: 10.1111/j.1365-2028.2009.01201.x
Thiel, S., Tschapka, M., Heymann, E. W., and Heer, K. (2021). Vertical stratification of seed- dispersing vertebrate communities and their interactions with plants in tropical forests. Biol. Rev. 96, 454–469. doi: 10.1111/brv.12664
Thompson, P. M., and Evans, M. I. (1991). The birds of Ambatovaky. Madagascar. London: Madagascar Environmental Research Group.
Van der Merwe, N. J., and Medina, E. (1991). The canopy effect, carbon isotope ratios and food webs in Amazonia. J. Archaeol. Sci. 18, 249–259. doi: 10.1016/0305-4403(91)90064-V
Venables, W. N., and Ripley, B. D. (2002). Modern applied statistics with S. 4th. New York: Springer
Villéger, S., Novack‐Gottshall, P. M., and Mouillot, D. (2011). The multidimensionality of the niche reveals functional diversity changes in benthic marine biotas across geological time. Ecology Letters. 14, 561–568.
Villéger, S., Grenouillet, G., and Brosse, S. (2013). Decomposing functional β‐diversity reveals that low functional β‐diversity is driven by low functional turnover in European fish assemblages. Global Ecology and Biogeography. 22, 671–681.
Wickham, H., Averick, M., Bryan, J., Chang, W., McGowan, L. D., François, R., et al. (2019). Welcome to the tidyverse. J. Open Source Softw. 4:1686. doi: 10.21105/joss.01686
Wickham, H., François, R., Henry, L., and Müller, K. (2022). Dplyr: A grammar of data manipulation. R package version 1.0.10. Available at https://cran.r-project.org/package=dplyr
Wilson, R. P. (2010). Resource partitioning and niche hypervolume overlap in free-living Pygoscelid penguins. Funct. Ecol. 24, 646–657. doi: 10.1111/j.1365-2435.2009.01654.x
Wink, M. (2006). Use of DNA markers to study bird migration. J. Ornithol. 147, 234–244. doi: 10.1007/s10336-006-0065-5
Winkler, H. (1988). An examination of concepts and methods in ecomorphology. ACTA XIX Proc. Int. Ornithol. Congr. 19, 2246–2253.
Winkler, H., and Leisler, B. (1985). “Morphological aspects of habitat selection in birds” in Habitat selection in birds. ed. M. L. Cody (London: Academic Press), 415–434.
Woog, F., Ramanitra, N., and Rasamison, S. (2006). Effects of deforestation on eastern Malagasy bird communities. In Proceedings of the German-Malagasy research cooperation in life and earth sciences, Eds. C. Schweitzer, S. Brandt, O. Ramilijaona, H. Rakotomalala, M. Razanahoera, and D. Ackermann, et al. Concept Verlag, Berlin, 203–214.
Woog, F., Ramanitra, N., Rasamison, A. S., and Tahiry, R. L. (2018). Longevity in some Malagasy rainforest passerines. Ostrich 89, 281–286. doi: 10.2989/00306525.2018.1502693
Keywords: Bernieridae, morphometry, ecological niche, stable isotopes, stable carbon (δ13C), stable nitrogen (δ15N) isotope, stable sulfur isotope, Madagascar
Citation: Yohannes E, Berthoud J-L and Woog F (2023) Trait based niche differentiation in tetrakas (Bernieridae) endemic to Madagascar: A multi-isotope approach. Front. Ecol. Evol. 11:1082226. doi: 10.3389/fevo.2023.1082226
Edited by:
Keith Alan Hobson, Western University, CanadaReviewed by:
Kevin John Kardynal, Environment and Climate Change Canada (ECCC), CanadaSteven Goodman, Field Museum of Natural History, United States
†PRESENT ADDRESS:
Elizabeth Yohannes, Swiss Ornithological Institute, Migration Uni, Sempach, SwitzerlandCopyright © 2023 Yohannes, Berthoud and Woog. This is an open-access article distributed under the terms of the Creative Commons Attribution License (CC BY). The use, distribution or reproduction in other forums is permitted, provided the original author(s) and the copyright owner(s) are credited and that the original publication in this journal is cited, in accordance with accepted academic practice. No use, distribution or reproduction is permitted which does not comply with these terms.
*Correspondence: Elizabeth Yohannes, ZWxpemFiZXRoLnlvaGFubmVzQHZvZ2Vsd2FydGUuY2g=
‡These authors share first authorship