- 1Université de Strasbourg, CNRS, IPHC, UMR7178, Strasbourg, France
- 2Collectivité européenne d’Alsace, CERISE, Place du Quartier Blanc, Strasbourg, Cedex, France
- 3Société Cofiroute, Centre autoroutier de l’Ackerland, Ittenheim, France
To mitigate habitat fragmentation and roadkill, roads are increasingly equipped with wildlife fences and underpasses. However, the effectiveness of such fences in preventing road access for amphibians has not been tested under controlled conditions. In 2019 and 2020, we tested the efficacy of full panel fences of differing material, height, and shape (presence/absence of an overhang), to prevent road access for adult and juvenile amphibians. We selected five species according to locomotion mode: Natterjack toads (runners), European green toads (short-distance jumpers), agile frogs (proficient jumpers), American tree frogs (proficient climbers) and smooth newts (climbers). We found that Natterjack and green toads were unable to cross a concrete fence with a height of 13 and 24 cm, respectively. Addition of a 10 cm overhang reduced the height required to prevent crossing further to 10 and 17 cm, respectively. The ability of these less agile species to cross a certain fence height depended on body length. By contrast, jumping agile frogs and climbing tree frogs were not stopped by the greatest fence height tested (40 cm). However, addition of the overhang stopped the climbing tree frogs at a concrete fence height of 35 cm. An alternative metal fence (with overhang) was tested with some species and performed similar to the concrete fence (with overhang). Finally, the greatest concrete fence height passed by climbing juveniles was 20 cm (smooth newts). Hence, to stop amphibians from road crossing, we recommend the construction of durable (concrete or galvanized metal) and well-maintained fences with a minimum height of 40 cm with a 10 cm overhang.
1. Introduction
Linear transport infrastructures, such as roads, are a threat to biodiversity and one of the most significant causes of landscape fragmentation (Maxwell et al., 2016). These structures lead to habitat loss, roadkill, and disrupt the movement of animals by creating ecological barriers (Fahrig et al., 1995; Forman and Alexander, 1998). While most taxa are impacted by roads (Forman and Alexander, 1998), some are particularly sensitive to them. According to the European Red List of Amphibians (Temple and Cox, 2009), habitat loss and degradation are the most significant threat to amphibians, affecting 76 of the 85 species found in Europe (Stuart et al., 2004; Maxwell et al., 2016). Many amphibians undertake spring and autumn migrations, which renders them particularly sensitive to roads between the various key areas used during the different phases of their life cycle (Wilbur, 1980; Miaud et al., 2000; Semlitsch, 2008; Joly, 2019; Cayuela et al., 2020). Even outside the migration periods, roads disrupt the movement of individuals between local populations and, furthermore, increase mortality through roadkill, potentially leading to the extinction of local and regional amphibian populations (Fahrig et al., 1995; Dodd et al., 2004; Petrovan and Schmidt, 2016; Testud and Miaud, 2018; Joly, 2019).
To limit roadkill and restore connectivity, mitigation measures have been implemented. These measures are numerous and include, for example, traffic and speed reduction, road closures and wildlife passages (Schmidt et al., 2008; Rytwinski et al., 2016; Testud and Miaud, 2018). The last measure is often associated with fences (e.g., wire netting, plastic mesh, or full panel concrete or metal), constructed to prevent animals from venturing onto roads and guide them instead towards passages (under/overpasses), where they can safely cross a road (Schmidt et al., 2008; Arntzen et al., 2017; Carvalho et al., 2017; Testud and Miaud, 2018). These fences are typically designed for large fauna (large mesh-size), such as ungulates (e.g., deer; Fahrig et al., 1995; Romin and Bissonette, 1996; Clevenger and Waltho, 2000; Forman et al., 2003; Dodd et al., 2004; Glista et al., 2009), but often contain additional components (fences with a small mesh-size), to stop amphibians and small mammals (Morand and Carsignol, 2019). Large fauna fences have been relatively well studied (i) because they are of obvious interest for human safety (Romin and Bissonette, 1996; Schwabe et al., 2002; Forman et al., 2003; Bouffard et al., 2012) and (ii) because their effectiveness can be easily demonstrated by monitoring the number of collisions between ungulates and vehicles.
For small fauna, different types of fences have been used but their effectiveness has rarely been studied. The few studies that have been carried out suggest a low efficacy of such fences (Dodd et al., 2004; Woltz et al., 2008; Brehme et al., 2021; Conan et al., 2022). For example, Arntzen et al. (1995) tested the effectiveness of chicken wire (40 cm height, 10 cm overhang, 1.3 cm mesh size) and plastic mesh fences (50 cm height, 10 cm overhang, 0.3 cm mesh size) to avoid amphibian road crossings (toads and newts, respectively). They found that chicken wire reduced the number of crossings by between 35 and 70% for toads, while plastic fences reduced the crossings of newts by between 34 and 48%. The findings of the above and further studies indicate that plastic mesh fences allow a relatively high number of amphibians to pass and, in some cases, do not reduce roadkill (Arntzen et al., 1995; Baxter-Gilbert et al., 2015; Ottburg and van der Grift, 2019). Plastic fence heights tested in these studies ranged from 40 cm for amphibians to 80 cm in reptiles and no overhang was used. Another type of small wildlife fence regularly found along roads is a wire netting fence (with adapted mesh size). However, this type suffers from the same defects as the plastic mesh fence; it deteriorates quickly and is easily climbed and passed by small-mammals and amphibians (Conan et al., 2022). Non-mesh (full panel) fences, constructed with different materials (e.g., PVC, concrete and metal) and a typical height of 40–60 cm (Morand and Carsignol, 2019), have also been used alongside roads. Such fences are fairly durable, overcoming the problem of fast deterioration and are, therefore, increasingly recommended (Dodd et al., 2004; Morand and Carsignol, 2019; Conan et al., 2022). However, to the best of our knowledge, only one study tested their efficacy for amphibians under controlled conditions in the laboratory (Zbierski and Schneeweiß, 2003). That study, which tested the efficacy of different shapes of full panel concrete fences (40–60 cm height without overhang) for European tree frogs (Hyla arborea), found that the most effective shapes are difficult to build and expensive to maintain (Zbierski and Schneeweiß, 2003).
1.1. Research objectives
In the current study, we investigated the efficacy of different types of full panel fences in preventing road access with five European amphibian species. We tested fences (1) made from two types of materials (concrete or galvanized metal) (2) of different height, and (3) without or with a horizontal metal overhang. To achieve more generalizable results, we selected five species with different locomotion modes (i.e., runner/jumper/climber) and included different developmental stages (adult and juvenile). We further tested fences in a dry or wet state (simulating the most common meteorological conditions) and also considered individual morphological measurements in our analysis.
We expected that: (1) A fence height exists that prohibits the crossing for all amphibian species tested (effective fence height; Heff) and this height will differ between fence types; (2) given the differences in locomotion mode of the species/developmental stages tested, Heff will differ between species/developmental stages; (3) the presence of an overhang will improve the efficacy of fences tested; (4) wetting of the fence will increase the crossing capacity of climbers; (5) the larger size and/or better body condition of some individuals will allow them to pass fences at a greater height than others.
2. Materials and methods
2.1. Model species, capture conditions and temporary captivity
Five amphibian species with different modes of locomotion in the adult stage were used in our study (Table 1). The Natterjack toad (Epidalea calamita Laurenti, 1768) moves by running on the ground; the European green toad (Bufotes viridis Laurenti, 1768) advances by small steps (low height/length ratio); the American tree frog (Dryophytes cinereus Schneider, 1799) is a good climber, due to its adhesive toepads (good adhesion on smooth surfaces, Li et al., 2021), and the agile frog (Rana dalmatina Fitzinger in Bonaparte, 1839) makes powerful jumps. The smooth/common newt (Lissotriton vulgaris Linnaeus, 1758) walks on the ground but can also climb walls by adhesion to the substrate. Given the low body mass and small size of juveniles, we assumed that juveniles of all species tested would be able to climb walls by adhesion. Hence, the behavior when crossing a vertical obstacle might differ between the developmental stages of species tested. During preliminary tests with adult male and female Natterjack toads, some females started to oviposit outside the water and without the presence of males. To limit any potential impact of our experimentation on wild populations, we consequently excluded adult females from experimentation and only included males at the adult stage. Any potential bias in our results, due to testing males only, should be limited to Natterjack toads, European green toads and tree frogs, for which size differences between sexes are small (Leskovar et al., 2006; Oromi et al., 2012). Female agile frogs are typically larger than males (~14%; Angelini et al., 1995), so that we cannot exclude a potential bias in our results for this species. However, our results suggest that this is unlikely an issue (see below).
Due to the national lockdown that occurred during the first Covid-19 outbreak in France (March–May 2020), we were unable to capture wild European tree frogs (Hyla arborea Linnaeus, 1758). Given their morphological similarity, we used American tree frogs (Dryophytes cinereus) as a substitute species. The lockdown also prevented us from completing some trials with Natterjack toads and agile frogs, so that only two fence types were tested with these species (concrete without or with a 10 cm overhang).
With the exception of American tree frogs that were purchased from a pet store (‘La ferme tropicale’, Paris, France), all individuals of the other species (both stages) were captured near their breeding sites during their terrestrial phase (see Table 1 for the number of individuals and capture dates). To minimize any potential effect on local populations, we captured individuals only at breeding sites with a sufficiently large population and which were close to the place of experimentation. Individuals were collected by hand (with gloves) or with a net during nocturnal patrols. After experimentation, individuals of all species except American tree frogs were released at the site of capture, following a maximum of 35 days in captivity. Individuals of the latter species could not be released into the wild and remained in captivity as pets.
To allow individual tracking during experimentation, a RFID PIT tag (1.4 × 9 mm; TAG LF GLT1M4X9 RO EM, Biolog-ID®, Bernay, France) was implanted into adults at the time of capture. Following implantation, the following morphological measurements were taken from adults: (1) body mass in g (BM), (2) snout-vent length in mm (SVL, from the snout to the tip of the ischium) and (3) extension length in mm (EL, the length of the straight line between the tips of hindlimbs and forelimbs, with both limbs extended, i.e., elongation size). Body mass was determined with a spring balance (0–50 g, resolution: 0.5 g, accuracy: ±0.3 g; Pesola®, Schindellegi, Switzerland), while SVL was measured using an electronic caliper (0–150 mm, accuracy: ±0.03 mm, Tesa technology®, Renens, Switzerland). The precise measure of EL was difficult with active amphibians; we used a tape measure (Dexter, Lezennes, France) and rounded measurements to the nearest 5 mm. A body condition index (BCI) was calculated for all species following Green (2001), using the individual residuals of the linear regressions between Ln(BM) and Ln(SVL).
Throughout captivity, individuals were kept in 800 × 600 × 435 mm polypropylene tanks with perforated lids (Euronorm container 12.4046.07 AXESS industries®, Strasbourg, France). Individuals were housed together according to species and developmental stage. The maximum number of individuals per tank was 20 (i.e., juveniles). The tanks were enriched with moist, sandy soil and fresh moss. Flat tiles and stones provided hiding places for individuals. Finally, during experimentation (which coincided with the breeding season), food was available ad libitum in the tanks [e.g., earthworms (Lumbricidae, ~5 cm), wax moth (Galleria mellonella, ~2 cm) and crickets (Acheta domesticus, ~1 cm)].
All procedures were carried out after obtaining the authorizations for capture and transport (2019-DREAL-EBP-0031) and a certificate for the detention of the various species in captivity (DDPP67-SPAE-FSC-2019-04). The experimental protocol was approved by the French Ethical Committee (CREMEAS) and the French Ministry of Environment: ‘Ministère de la Transition Écologique’ under agreement number (APAFIS #18546-2019011810282677.v7).
2.2. Experimental setup
2.2.1. Test arena
All tests were conducted in an arena built from PVC (60 × 80 × 70 cm, width*length*height; Figures 1, 2) that was placed inside a large semi-natural outdoor enclosure (2000 m2 large fenced in area that excluded predators). The arena consisted of three compartments: (1) the ‘departure’ compartment (~60 × 30 cm) had a bare concrete floor (i.e., unattractive), while the (2) ‘arrival’ compartment (~60 × 40 cm) was enriched with wet soil and moss, hides made of bricks and a small pool filled with freshwater. The latter was added as an attractive reward and has been used to that end in learning experiments with toads (Dall'antonia and Sinsch, 2001; Daneri et al., 2007). On the rear side of the ‘arrival’ compartment a speaker (Pulsar® 2 × 3 W speaker, Enkhuizen, Netherlands) replayed calls from Epidalea calamita and Pelophylax spp., to motivate amphibians to cross the fence. Male calls (conspecific or not) are known to attract all resident amphibian species (Gerhardt, 1995; Yeager et al., 2014; Muller and Schwarzkopf, 2017). Adjacent to the ‘arrival’ compartment was (3) a further compartment (~60 × 10 cm) that hosted two males of the tested species, which added to the acoustic stimulus provided by the speaker, while also potentially relaying visual and olfactory cues. The first two compartments were separated by the fauna fence to be tested (concrete or galvanized metal fence), while the last compartment was separated from the arrival compartment by a perforated Plexiglass plate (Figure 1). The top of the arena was covered with a regularly perforated Plexiglass lid to allow for good ventilation and its openings were covered with netting. An air ventilation system (Pump KNF N840.1.2FT.18, Freiburg im Breisgau, Germany) provided appropriate ventilation throughout the arena and also allowed for an exchange of scent throughout the system (i.e., amphibians in the departure compartment likely smelled their conspecifics in the last compartment). To test the effect that moisture might have on the crossing capacity of amphibians, half of the length of the fence tested (concrete and galvanized metal) was fitted with a water circulation system (aquarium pump; Neptus Mini P300®, Italy) that permanently wetted this part of the fence (Figure 2).
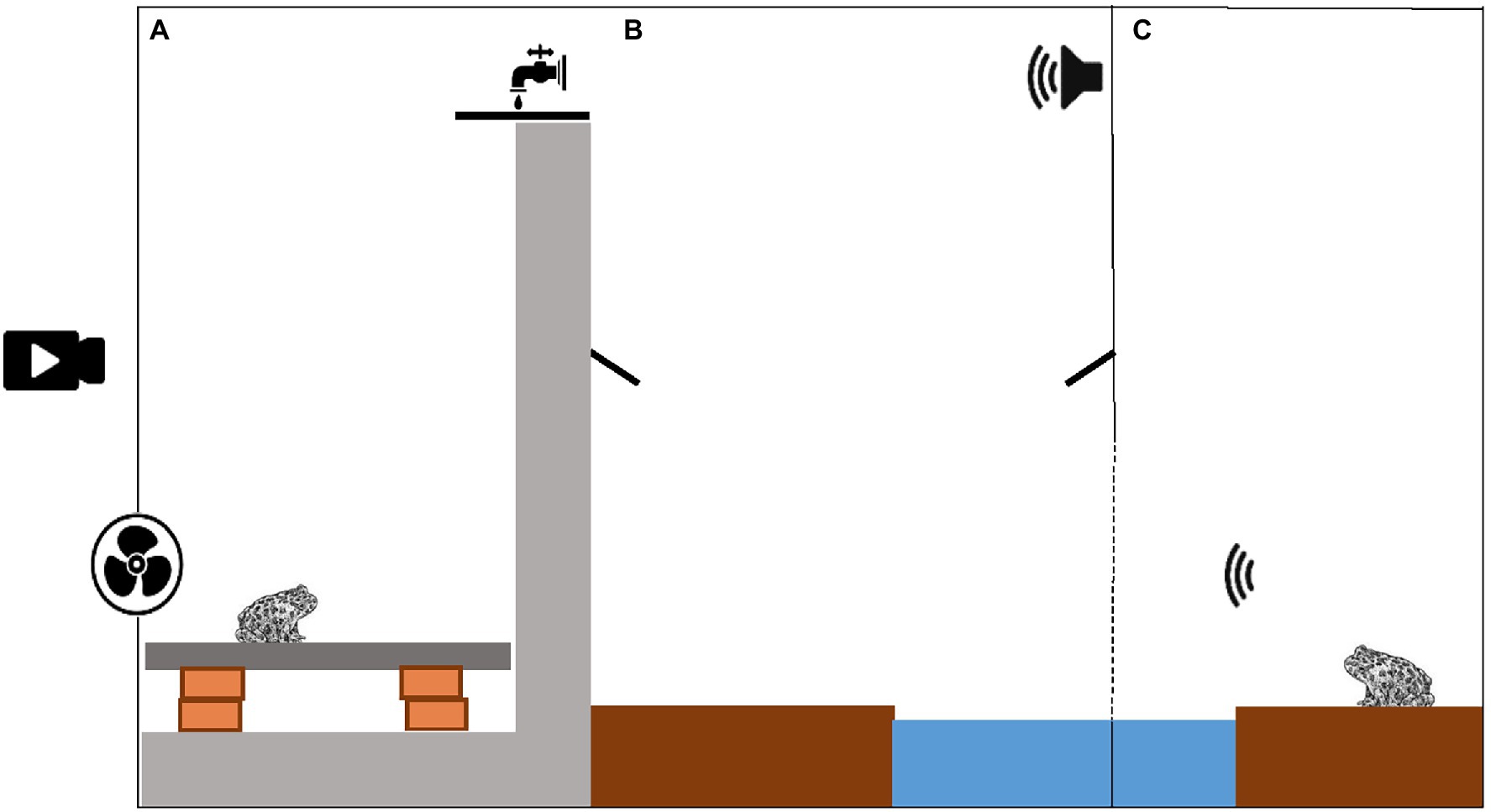
Figure 1. Illustration of the experimental arena. The aquatic part is shown in blue, the enriched soil in brown, the fauna fence is shown in grey and the overhangs are represented by solid black lines. The iconography from left to right: camera; ventilation system; species tested; water inlet to wet fence; loudspeaker; singing male. (A) ‘Departure’ compartment (not enriched) with a movable concrete base (shown in dark grey) that allowed modification of test height (see also Figure 3); (B) ‘arrival’ compartment, enriched with sandy soil and moss; (C) compartment with singing males, separated from the ‘arrival’ compartment by a perforated Plexiglass plate (covered with a net), allowing the exchange of visual and olfactory cues in addition to auditory stimulation. Overhangs in the ‘arrival’ compartment were added to prevent individuals that crossed the fence to return to the ‘departure’ compartment.
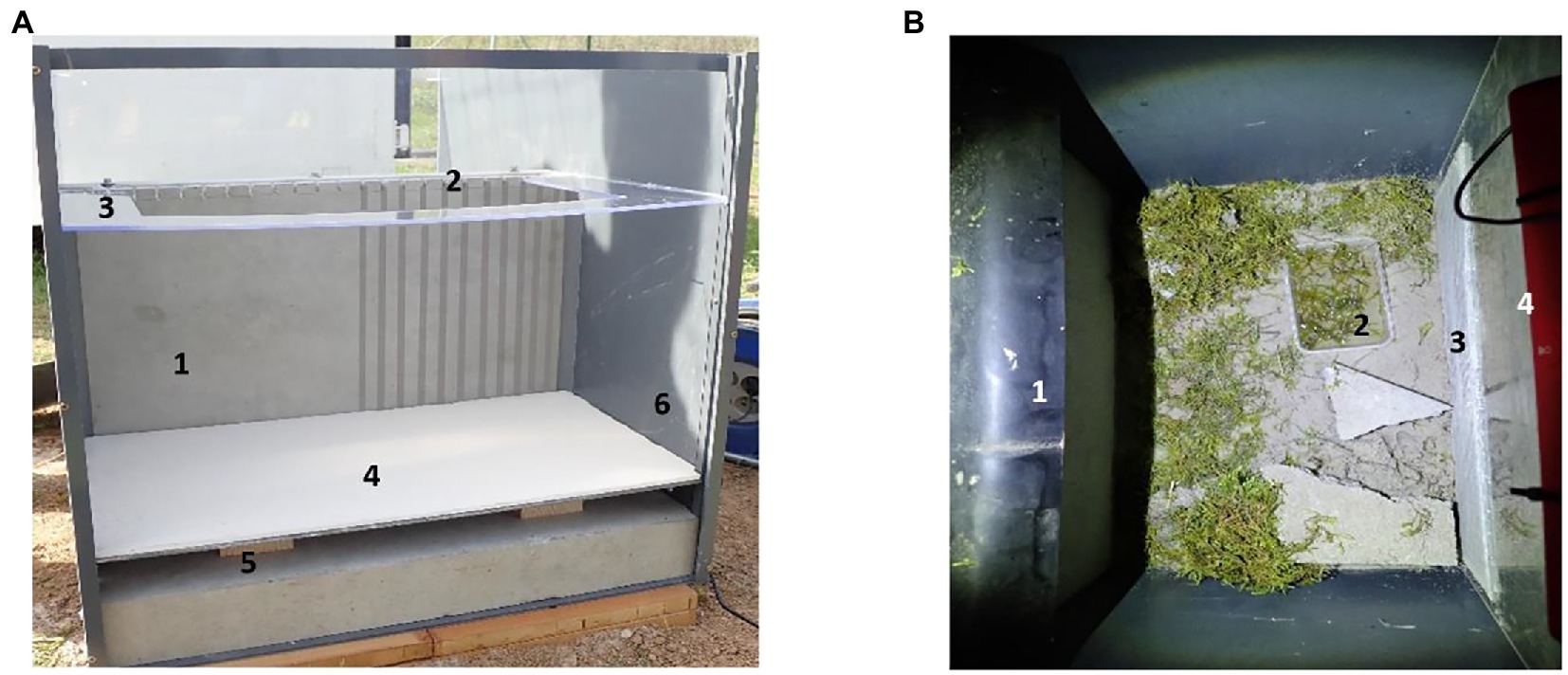
Figure 2. Experimental arena. (A) Frontal view of the ‘departure’ compartment: (1) dry part of the vertical concrete fence tested; (2) wet part of the vertical fence tested; (3) anti-escape Plexiglass plate; (4) movable concrete base (30x50 cm, width*length), allowing to adjust fence height; (5) wooden blocks for height adjustments; (6) transparent frontal Plexiglass plate allowing video recording; (B) arial view of the ‘arrival’ compartment: (1) Overhang made from a 7.5 cm wide PVC sheet, that was fixed 5 cm below the top of the fence and bent downwards (inclination angle: ~75°), to prevent animals from returning to the ‘departure’ compartment; (2) small water tank placed among other enrichments: sand, wet moss, hides made of bricks; (3) opening in Plexiglass plate (covered with a mesh) enabling ventilation throughout all compartments; (4) speaker that replayed calls of Epidalea calamita and Pelophylax spp., to stimulate amphibians to cross the fence.
2.2.2. Tested obstacles
Three full panel fence types were tested: (1) a concrete fence without overhang (Crt), (2) a concrete fence with a 10 cm horizontal overhang (Crt+) and, lastly, (3) a fence of galvanized metal (Galv) with a bent overhang that extended 5 cm horizontally and 2 cm downwards (Figure 3). The overhang dimensions for the concrete fence were chosen according to results of a previous study, in which a 10 cm overhang was sufficient to prevent the crossing of green toads and marsh frogs (Pelophylax ridibundus; Conan et al., 2022). The bent overhang for the metal fence was required to provide structural stability and the dimensions used were readily available commercially. To allow testing of different fence heights, the movable base of the departure compartment was mounted onto a varying number of wooden blocks, altering the distance to the top of the fence (Figure 3). As stated above, due to the Covid crisis lockdown, we were unable to test all fence types with all species/developmental stages.
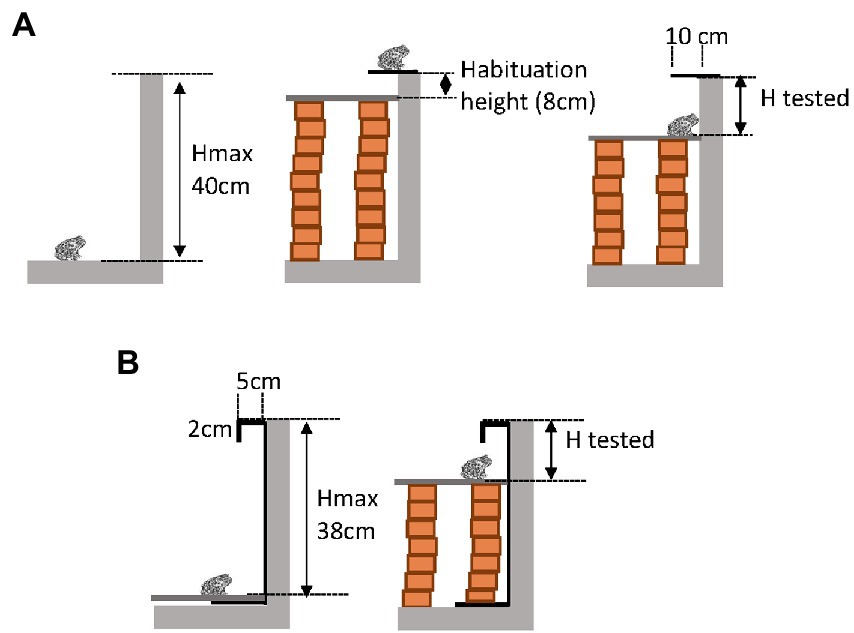
Figure 3. Setup to test the capacity of amphibians to cross different fence types and heights. We used wooden blocks to adjust the concrete base to the desired fence height to be tested. All tests with adult amphibians started with a habituation height of 8 cm, before height was increased in the following trials. (A) Concrete fence tests: Hmax (the maximal height tested) was 40 cm, without or with an overhang of 10 cm; (B) galvanized metal fence tests: Hmax was 38 cm; this fence type had a bent overhang (5 cm horizontal +2 cm downwards).
2.3. Test protocol
2.3.1. Experimental trials
The primary goal of our trials was to find the lowest effective fence height (Heff) for all fence types tested (Crt, Crt+, Galv) that prevented crossing for individuals of all species and developmental stage.
During experimental trials, individuals were tested in groups of 8, 10 or 20 individuals of the same species and developmental stage (Table 1). The difference in numbers resulted from permit limitations for adults (N = 10) and the inability to purchase more than 8 tree frogs, while we were able to include a greater number of juveniles (N = 20) per species. At the start of a trial, animals were placed into the ‘departure’ compartment at the end of a day (9:30 PM) and had until the following morning (7:30 AM) to cross the fence. During the entire trial duration, calls were replayed from the speaker (looped recording), callers were present inside the last compartment and a video camera recorded continuously (Figure 1). The following morning, after completion of a trial, individuals that had managed to cross the fence were removed from the ‘arrival’ compartment, counted and identified (RFID tags in adults only). Thereafter, all individuals that participated in a trial were transferred back to their holding tanks. To allow animals to familiarize themselves with the setup, a fence height of 8 cm (i.e., habituation height, Figure 3) was used during the first night for each group (except juveniles, see below). Following that first night, animals were tested for a maximum of three consecutive nights for a particular height. We started with the maximum height possible for the different fence types (40 cm for the concrete fence types and 38 cm for the galvanized metal fence) and iteratively decreased or re-increased height in the following trials, depending if individuals failed or succeeded to cross. As soon as at least one individual managed to cross a particular height during one night, height was increased during the following night. For example, if amphibians failed to cross the 40 cm fence for 3 consecutive nights, the following night, fence height was adjusted to half the difference between the habituation height (8 cm) and the maximal height (40 cm), hence, to 16 cm (32/2 cm). If one individual managed to cross at that height, fence height was increased to 24 cm (16 + 8 cm). Testing continued until Heff was found for a given species, stage, and type of fence.
Apart from slight modifications indicated below, testing conditions for juveniles of all four species were identical to adults. Juveniles were tested in groups of 20 individuals and with a strict limitation on the duration of experimentation. Given the fragility of amphibians during their juvenile stage, we limited experimentation to 3 consecutive nights and did not offer a habituation trial, as in adults. The first night, the height to be crossed was set to 40 cm with a 10 cm overhang (maximum difficulty possible to test with our setup), which was never reached by climbing juveniles. To estimate the greatest height reached by juveniles, we attached a measuring bar to the concrete fence, that was clearly visible in the video footage. During the following 2 nights, fence height was set to half the height reached by climbing juveniles during the previous night (as identified from video footage), to encourage successful crossing. Unfortunately, no further height increments could be tested because of the set time limit for experimentation with juveniles. This also prevented us from testing the concrete fence with overhang and the metal fence with juveniles.
Following a trial, we viewed the recorded video footage to identify how individuals crossed the fence. Our protocol was designed to strongly motivate individuals to cross the fence. Besides call replays, the presence of conspecifics (callers) in the last compartment and the enrichment inside the arrival compartment, trial durations were long (10 h) and each height was tested during a maximum of 3 consecutive nights (if amphibians failed to cross). Hence, we presume that a fence height not crossed during our experimentation will not be crossed in the wild.
2.4. Analysis and statistics
2.4.1. Test of protocol efficacy
To ensure that our protocol sufficiently motivated individuals to cross the fence and to further quantify the number of crossing attempts of individuals, we conducted a behavioral analysis on 10 h of video footage from European green toads and Natterjack toads. We chose footage from unsuccessful trials (i.e., no individuals passed the fence) and studied how the number of crossing attempts varied between individuals. Since adult amphibians were implanted with an RFID tag, we could investigate individual differences in crossing success and also determine if crossing failures were likely explained by height or differences in motivation (based on the number of observed attempts). For example, it is possible that individuals pass at a greater height but fail to do so at a lower height, simply because they were not motivated to cross.
2.4.2. Effective fence height (Heff) and the effects of morphology
Our goal was to experimentally determine Heff for all conditions and species/developmental stages. We found clear differences in Heff between species/developmental stages depending on locomotion mode and their strategy to cross the fence. However, since we could not determine Heff for all species, statistical testing was restricted to European green toads and Natterjack toads, the least agile species tested. We used an ANOVA to test for differences in Heff between the latter two species and linear regression analysis to investigate the relationship between their morphological features (EL, SVL, and BM) and Heff.
2.4.3. Effects of fence wetting
To investigate whether wetting of the fence would increase the crossing capacity of climbers (i.e., tree frogs and all juveniles), we used the recorded video footage to determine the number of crossing attempts during 12 randomly selected 15 min periods, and recorded whether crossing attempts occurred on the dry or wet side of the fence. Unfortunately, the video footage for all juveniles, except Natterjack toads, did not allow a systematic investigation (condensation fogging up the image). Hence, this analysis was conducted for juvenile Natterjack toads and adult tree frogs only. We used a generalized linear model (GLM) with a quasi-poisson distribution to test for differences in the number of crossing attempts between the wet and dry part of the fence for both species.
Analysis and graphs were conducted with R (v3.5.3, RStudio v1.2.1335 and GraphPad Prism 8.2.1). All results are shown as mean ± SEM with a statistical significance threshold of 5%.
3. Results
3.1. Test of protocol efficacy
During the 10 h of video recording we analyzed, all individuals engaged in crossing attempts. We recorded 735 and 344 unsuccessful crossing attempts of European green toads and Natterjack toads, respectively. On average, individual green toads made 8.4 ± 0.1 attempts per hour (range 4–13), while Natterjack toads engaged in 3.9 ± 0.1 attempts per hour (range 3–11). The frequency of crossing attempts remained stable throughout the night in both species. Considering all tests conducted, 50% of European green toads, failed to cross at a lower height but managed to cross at a greater height later on, while this did not occur in Natterjack toads. In addition to the video analysis conducted with the two toad species, analysis of the RFID tag data showed that, similar to the green toads, agile frogs (80%) and American tree frogs (75%) passed at a greater height after having failed to cross at a lower height.
3.2. Efficacy of fence types in preventing amphibian road access
The effective fence height (Heff) for all species and developmental stages tested with different fence types is shown in Table 2. Only the nimblest species tested (agile frogs and American tree frogs) were able to cross a full panel concrete fence (without overhang) at a height of 40 cm (the maximum height we could test). Furthermore, addition of a 10 cm overhang decreased the crossing success for all species, except for agile frogs (Crt vs. Crt+; Figure 4; Table 2). However, given the height limitations, we were unable to determine the effective fence height for the concrete fence without overhang for agile and tree frogs. In the following, results are presented separately for the nimblest species (agile and tree frogs), the toad species (green and Natterjack toads), and juveniles of all species.
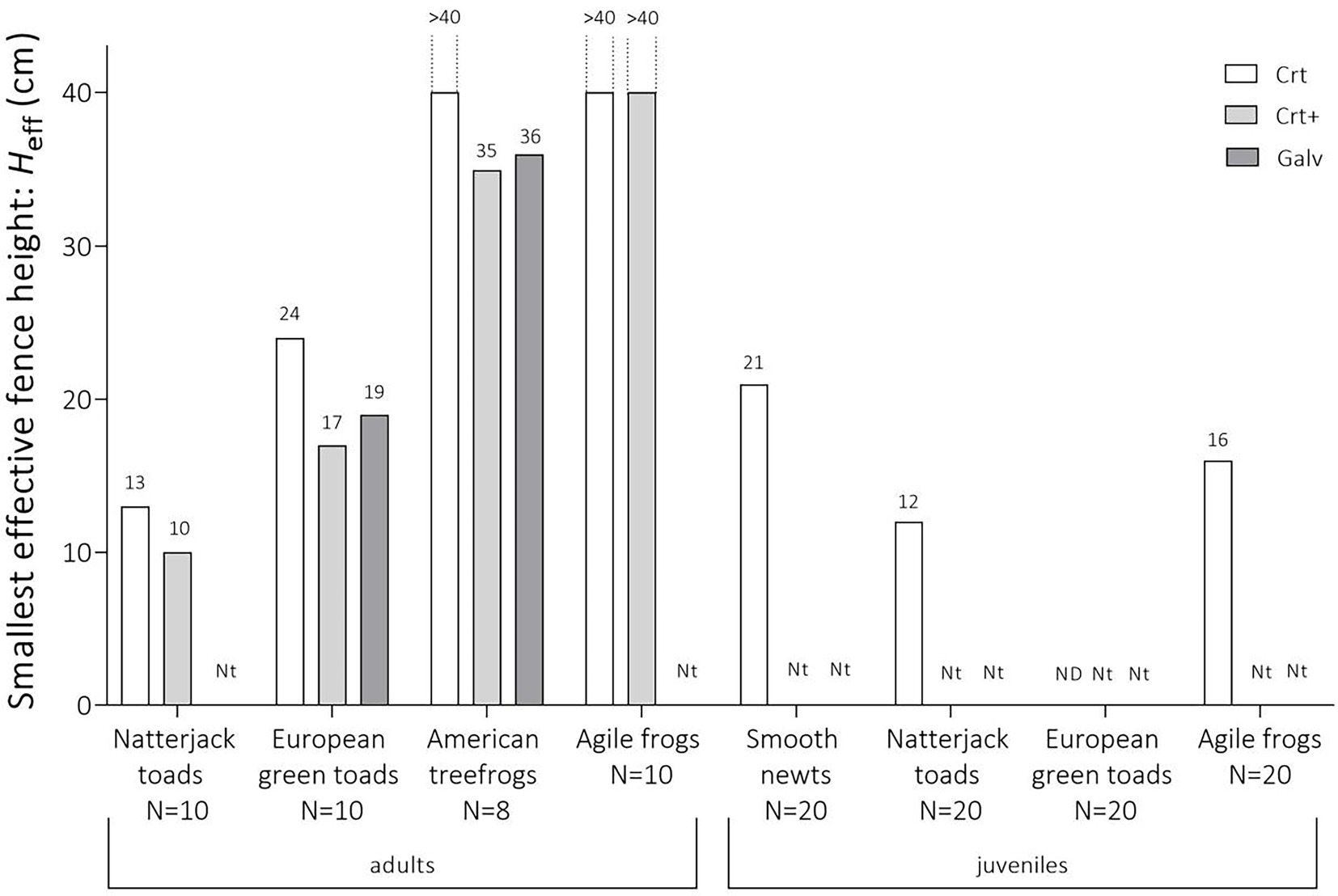
Figure 4. Smallest effective fence height (Heff) for the amphibian species/developmental stages tested that prevented them to cross over various fence types, as indicated by different bar colors [white: simple concrete fence (Crt); light gray: concrete fence with a 10 cm horizontal overhang (Crt+); dark gray: galvanized fence (Galv)] with a bent overhang. Note that not all fence types could be tested with all species/developmental stages, as indicated by ‘Nt’ (not tested). Juvenile green toads never attempted to cross the fences tested, so that we could not determine Heff in that case, as indicated by ND (not determined).
3.2.1. Adult agile frogs (proficient jumpers) and American tree frogs (proficient climbers)
With a single direct jump, agile frogs were able to reach the top of the concrete fence. However, they required a sufficient minimum distance to the fence (~30 cm), especially when jumping in a straight fashion towards it. Successful attempts at maximum fence height were mostly sideway jumps, when individuals took advantage of the 60 cm width of the compartment (see Figure 2) to increase distance to the fence, so that they could reach a sufficient height during the jump. The presence of an overhang, which reduced the distance by 10 cm, did not prevent any individuals from reaching the top of the 40 cm Crt+ fence. Fence crossing in this species often involved two steps: firstly, an individual would jump to the ‘Anti escape Plexiglas sheet’ (see #3 in Figure 2), located at a height of 40 cm, and then jump further to the top of the fence or even directly into the ‘arrival’ compartment. The greater distance possible when jumping first to the Plexiglass plate possibly contributed to the ease with which agile frogs reached the fence top. However, such a strategy is likely irrelevant in the context of wildlife fences, when such a structure will not be present. Unfortunately, the galvanized fence type could not be tested with agile frogs.
Similar to agile frogs, American tree frogs passed over the maximum fence height tested (40 cm concrete fence, without overhang) during the first night of testing (Figure 4). However, they used a different mode of locomotion; rather than jumping, they climbed the concrete fence and showed a significant preference for the wet part of the fence (t = 2.1, p = 0.04; Figure 5). In addition, tree frogs developed a different mode to cross the fence after ~8 nights. Individuals would climb the smooth PVC sidewalls or the smooth frontal Plexiglass plate and then jump onto the fence top or right into the arrival compartment. Hence, unfortunately, when testing the concrete fence with overhang (Crt+), tree frogs did not try to cross the 40 cm fence directly but used their newly developed mode instead. Nonetheless, one individual passed the overhang via the direct fence route at a height of 35 cm. Testing the galvanized fence (Galv) with the tree frogs showed that the 2 cm downward component of the overhang (see Figure 3B) was rather counterproductive. Frogs were able to cling to it and, by stretching out, they managed to reach the fence top and cross over the fence. Nevertheless, tree frogs were unable to cross the maximal metal fence height of 38 cm directly. Similar to the Crt+ tests, frogs only managed to cross at that height by climbing the side walls and then jumping over the metal fence.
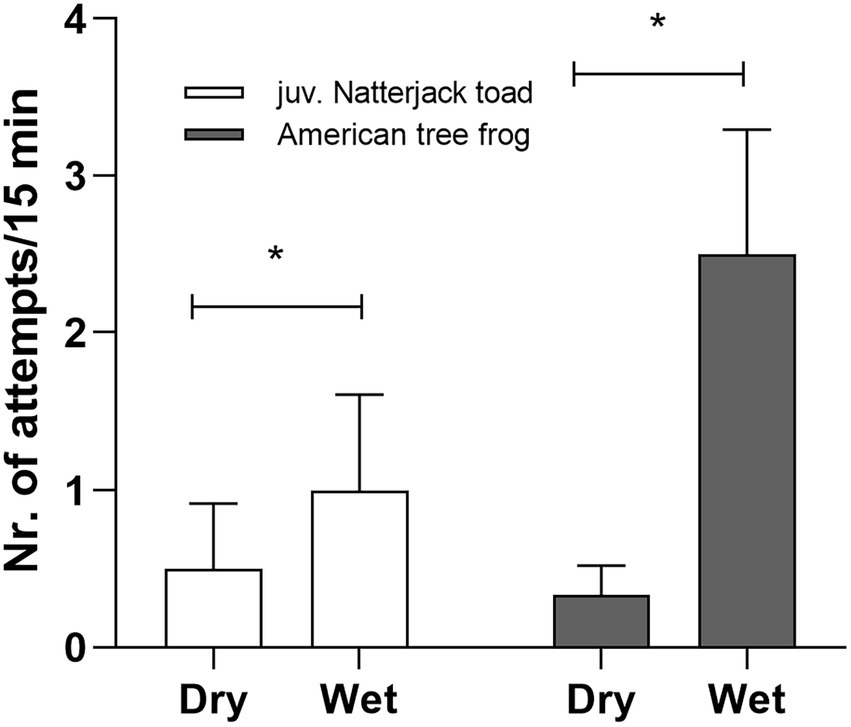
Figure 5. Number of crossing attempts per 15 min periods for two climbing species: juvenile Natterjack toads (N = 20) and adult American tree frogs (N = 8). Both species preferentially used the wetted part of the fence (t = 2,1, p < 0.05).
3.2.2. Adult Natterjack toads (runners) and European green toads (short distance jumpers)
The only way adult Natterjack toads managed to cross the tested fences was by stretching out and pulling themselves up and over the fence top. By contrast, adult green toads often jumped onto the fence and then stretched themselves towards the top to cross over. Given the jumping advantage of green toads, they managed to cross all fence types at a greater height than Natterjack toads (F = 118; d = 1; p < 0.001; Figure 4; Table 2).
3.2.3. Juvenile amphibians
The only mode by which juveniles attempted to cross the concrete fence without overhang (the only type tested with juveniles) was by climbing. Juvenile European green toads were the exception and never attempted to cross the fence. However, the juveniles of all other species were able to climb the fence by adherence of their extremities. Similar to adult tree frogs, juvenile Natterjack toads preferentially used the wet side of the fence (t = 2.1, p = 0.04; Figure 5). This was likely also the case for the juveniles of the other species but could, unfortunately, not be determined in our analysis. With the exception of the green toads, all juveniles were able to climb to a height equivalent to more than 10 times their size (SVL ~1 cm; Figure 4; Table 2). However, no juveniles were able to cross a fence height greater than 20 cm.
3.3. Effects of morphological parameters on minimum effective fence height (Heff)
Morphological features differed between species (Table 3). While green toads were the heaviest, agile frogs were the longest of all species measured. Regression analysis showed significant relationships between all morphological variables measured and Heff for Natterjack toads (Table 3; Figure 6). By contrast, this was not the case for green toads (Table 3; Figure 6). Hence, larger Natterjack toads were able to cross at a greater fence height than their smaller conspecifics (Figure 6). This is likely also true for green toads but given our small sample size, relationships failed to reach significance.
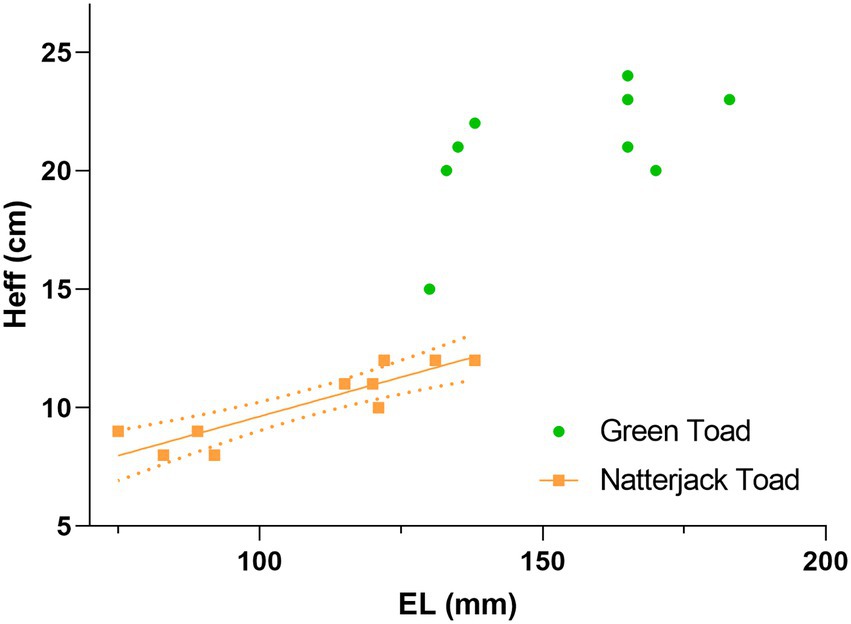
Figure 6. Relationship between elongation length (EL) and minimum effective fence height (Heff) that prevented adult Natterjack and green toads from crossing the concrete fence without overhang (N = 10 for both species). Significant relationships are indicted by the solid line, with dotted lines indicating the 95% confidence bands. There was a significant positive relationship between parameters for Natterjack toads (for EL: p < 0.001, r2 = 0.80), while this was not the case for green toads.
4. Discussion
Our experiments showed that the height of the fence types tested was sufficient to stop adult individuals of species representing the running and short-distance jumping mode (Natterjack and European green toads), as well as all juveniles from crossing. Addition of an overhang (in case of the concrete fence) further reduced height at which these species were able to cross the fence. By contrast, proficient jumping and climbing species (American tree frogs and agile frogs) were not stopped by the greatest fence height tested. However, addition of an overhang stopped the proficient climbers (tree frogs) at a height below the maximal tested height, while it had no effect on agile frogs. An alternative galvanized metal fence (with overhang), which we tested with some species, performed similar to the concrete fence with overhang. Finally, climbing juveniles were only able to pass a concrete fence at about half its maximal height and only when it was wet.
In our setup, amphibians were highly motivated to cross the barrier separating the departure and arrival compartment. When failing repeatedly to cross at a particular height, individuals nevertheless continued to try. Crossing attempts in such cases ranged between 3 and 13 per hour for the two toad species tested. Furthermore, if amphibians failed to cross at a particular height, they were given two more nights (3 consecutive nights per test height in total). A sufficient time frame for testing is important, since motivation to cross might not be identical throughout trials. For example, we observed that individuals of all adult species, except Natterjack toads, failed to cross the fence at a lower height but managed at a greater height soon after. Accordingly, results from a previous study that are based on only 6 h of testing and without replicates should be treated with caution, as they might underestimate the crossing capacity of species (Zbierski and Schneeweiß, 2003). In the wild, the motivation to cross might differ with season, breeding status, rain and further parameters. Our experimental setup consisted of a small test arena and we used a number of stimuli to ensure sufficient motivation for crossing. Hence, we believe that our setup was well suited to test the crossing capacity of amphibians.
As expected, we found that the effective fence height (Heff), i.e., the minimal height at which amphibians failed to cross, differed according to locomotion mode. However, in contrast to our most fundamental expectation, a concrete fence without overhang (Crt; maximal height tested: 40 cm) was only sufficient to stop the adults of the less nimble species, like toads (green toads and Natterjack toads) and all juveniles but failed to prevent the crossing of agile frogs and tree frogs (Figure 4). In case of the toads tested, larger individuals succeeded in crossing at a greater fence height (Figure 6). As expected, the addition of a 10 cm overhang (Crt+) increased the efficacy of the concrete fence, lowering effective fence height (Heff) for the adults of all species where an overhang was tested, albeit, not for agile frogs (Figure 4). Hence, in case of most European amphibian species, road managers are left with the choice of a greater concrete fence height without overhang or a lower fence height with overhang. However, while an increase in fence height to, for example, 50 cm, may stop agile frogs, this will unlikely stop American tree frogs, unless an overhang is also installed. Accordingly, when more nimble species (agile frogs, tree frogs) are present, we strongly recommend the addition of an overhang to a fence. Such addition stopped the American tree frog, a species able to climb trees (Gourevitch and Roger Downie, 2018), at a fence height of 36 cm. Unfortunately, we were unable to test a fence height greater than 40 cm, so Heff for agile frogs remains unknown, as they jumped across the greatest height tested, even in the presence of an overhang. This is likely of relevance for all proficient jumping amphibian species. Similar to previous recommendations (Morand and Carsignol, 2019), we therefore suggest a fence height of a least 50 cm with the addition of a horizontal overhang. The width of the overhang is crucial and anything less than 10 cm might not work well for frog species (Conan et al., 2022).
When the installation of a concrete fence is not possible (due to high costs and/or the destructive nature of its construction), road managers have the option to install other fence types. These might be less expensive to install but might also be less durable. As a first choice, road managers often consider the use of wire-netting fences to stop small animals. However, they are fragile and, most importantly, useless in preventing the crossing of green frogs, European green toad and many small mammalian species (Conan et al., 2022). A better option might be the use of galvanized metal fences of the type we tested (i.e., with a 5 cm horizontal overhang and a 2 cm downward component; Figure 3). Unfortunately, we were only able to test this fence type with green toads and tree frogs but Heff was similar to that of the concrete fence with overhang (Figure 4). However, the overhang was problematic for the tree frogs, since they could attach themselves to the downward bent and then cross over the fence, reducing the efficacy of this fence type. Unfortunately, the 2 cm downward bent of the overhang was a structural requirement for the prototype we tested. Nevertheless, increasing the horizontal dimension of this overhang (e.g., from a width of 5 to 10 cm) might improve its efficacy and should be tested.
The 40 cm concrete fence stopped all juvenile amphibians, despite their similar locomotion mode to that of tree frogs (i.e., climbing), which were able to pass such a fence. Juveniles of all tested species were unable to reach a height greater than 10 to 20 times their body size (SVL ~1 cm). Unfortunately, we were unable to test the effect of an overhang with juveniles. Such structural addition will limit the presence of water along a fence during rainy weather (Schmidt et al., 2008), reducing fence wetting. The latter is of great importance, as fence wetting will likely ease fence crossing (Figure 5).
Our results are supported by previous studies indicating that durable and opaque structures are required to stop amphibians from crossing into roads and to guide them to the nearest wildlife passage (Brehme et al., 2021; Conan et al., 2022). A concrete fence equipped with a 10 cm overhang is an effective fence to stop the majority of amphibians present in Europe but only if it is well managed. In this context, we occasionally observed American tree frogs climbing the side walls of the arena, rather than the fence itself, from which they jumped over the fence, sideways. This is of great relevance for the situation on site. If the growth of sufficiently large/high vegetation near a fence is not prevented, tree frogs and similar climbing species will be able to overcome any type of fence. Hence, maintaining the vegetation near fences sufficiently small/low is essential to ensure the effectiveness of fences, especially in the case of climbing species (Speybroeck et al., 2018).
In our study we only addressed the situation of amphibian species. However, amphibians are not the only animals concerned by roadkill. Road mortality is also of concern for numerous other small animal species, such as small mammals, reptiles and also insects (Forman and Alexander, 1998; Dodd et al., 2004; Baxter-Gilbert et al., 2015). However, few studies have addressed the efficacy of fences for these groups (Dodd et al., 2004) but instead have focused on the efficacy of the ensuing tunnels to facilitate road crossing (Dodd et al., 2004; Glista et al., 2009). Furthermore, the lack of rigorous experimental testing of fences often leads to approximate measures by road managers which are based on practice rather than scientific evidence, and this includes the situation for amphibians (Morand and Carsignol, 2019).
In conclusion, a 40 cm concrete fence with a 10 cm overhang was effective to stop some of the amphibian species tested at their adult stage and all juveniles (Bufonidae, Hylidae). However, species with a great ability to jump were not stopped by such a barrier (e.g., Rana dalmatina). Accordingly, for the latter group of species we recommend a concrete fence height of at least 50 cm (see also Morand and Carsignol, 2019). If, for some reason, a full panel concrete fence cannot be used, we advise the use of a full panel galvanized metal fence with a minimal height of 50 cm and an overhang of at least 10 cm.
Data availability statement
The raw data supporting the conclusions of this article will be made available by the authors, without undue reservation.
Ethics statement
All procedures were carried out after obtaining the authorizations for capture and transport (2019-DREAL-EBP-0031) and a certificate for the detention of the various species in captivity (DDPP67-SPAE-FSC-2019-04). The experimental protocol was approved by the French Ethical Committee (CREMEAS) and the French Ministry of Environment: ‘Ministère de la Transition Écologique’ under agreement number (APAFIS #18546-2019011810282677.v7).
Author contributions
AC, JJ, and YH conceived the study and designed the methods. AC, MLB, ND, and LG collected the data. AC analyzed the data. AC drafted the manuscript with contributions from MLB, ND, LG, JF, ME, JJ, and YH. All authors approve this version of the manuscript for publication. They agree to be accountable for the aspects of the work that they conducted and will ensure that any questions related to the accuracy or integrity of any part of their work are appropriately investigated and resolved.
Funding
This study was conducted within the framework of the CERISE project and was funded by the French Minister of Ecology (DREAL Grand-Est), the Region Grand-Est, the European collectivity of Alsace (CeA), and the Eurometropole of Strasbourg (EMS). The funders had no role in any aspect of the study and agreed to its publication.
Acknowledgments
We thank all the students who participated in this study, Sylvia Pardonnet for help with video analysis, and Pierre Uhlrich for construction of the experimental arena. Two anonymous reviewers and the editor are thanked for their valuable comments.
Conflict of interest
The authors declare that the research was conducted in the absence of any commercial or financial relationships that could be construed as a potential conflict of interest.
Publisher’s note
All claims expressed in this article are solely those of the authors and do not necessarily represent those of their affiliated organizations, or those of the publisher, the editors and the reviewers. Any product that may be evaluated in this article, or claim that may be made by its manufacturer, is not guaranteed or endorsed by the publisher.
References
Angelini, F., Cammarota, M., and Guarino, F. M. (1995). A skeletochronological analysis of three syntopic amphibian species from southern Italy. Amphibia-Reptilia 16, 297–302. doi: 10.1163/156853895X00109
Arntzen, J. W., Abrahams, C., Meilink, W. R. M., Iosif, R., and Zuiderwijk, A. (2017). Amphibian decline, pond loss and reduced population connectivity under agricultural intensification over a 38 year period. Biodivers. Conserv. 26, 1411–1430. doi: 10.1007/s10531-017-1307-y
Arntzen, J. W., Oldham, R. S., and Latham, D. M. (1995). Cost effective drift fences for toads and newts. Amphibia-Reptilia 16, 137–145. doi: 10.1163/156853895X00316
Baxter-Gilbert, J. H., Riley, J. L., Lesbarrères, D., and Litzgus, J. D. (2015). Mitigating reptile road mortality: fence failures compromise Ecopassage effectiveness. PLoS One 10:e0120537. doi: 10.1371/journal.pone.0120537
Bouffard, M., Leblanc, Y., Bédard, Y., and Martel, D. (2012). Impacts de clôtures métalliques et de passages fauniques sur la sécurité routière et le déplacement des orignaux le long de la route 175 au Québec. Le Nat. Can. 136, 8–15. doi: 10.7202/1009100ar
Brehme, C. S., Tracey, J. A., Ewing, B. A. I., Hobbs, M. T., Launer, A. E., Matsuda, T. A., et al. (2021). Responses of migratory amphibians to barrier fencing inform the spacing of road underpasses: a case study with California tiger salamanders (Ambystoma californiense) in Stanford, CA, USA. Glob. Ecol. Conserv. 31:e01857. doi: 10.1016/J.GECCO.2021.E01857
Carvalho, F., Santos, S. M., Mira, A., and Lourenço, R. (2017). “Methods to monitor and mitigate wildlife mortality in railways” in Railway ecology (Cham: Springer International Publishing), 23–42.
Cayuela, H., Valenzuela-Sánchez, A., Teulier, L., Martínez-Solano, Í., Léna, J.-P., Merilä, J., et al. (2020). Determinants and consequences of dispersal in vertebrates with complex life cycles: a review of pond-breeding amphibians. Q. Rev. Biol. 95, 1–36. doi: 10.1086/707862
Clevenger, A. P., and Waltho, N. (2000). Factors Influencing the Effectiveness of Wildlife Underpasses in Banff National Park, Alberta, Canada. Conserv. Biol. 14, 47–56. doi: 10.1046/j.1523-1739.2000.00099-085.x
Conan, A., Fleitz, J., Garnier, L., Brishoual, M. Le, Handrich, Y., and Jumeau, J. (2022). Effectiveness of wire netting fences to prevent animal access to road infrastructures: an experimental study on small mammals and amphibians. Nat. Conserv. 47, 271–281. doi:doi: 10.3897/NATURECONSERVATION.47.71472
Dall'antonia, P., and Sinsch, U. (2001). In search of water: orientation behaviour of dehydrated natterjack toads, Bufo calamita. Anim. Behav. 61, 617–629. doi: 10.1006/anbe.2000.1627
Daneri, M. F., Papini, M. R., and Muzio, R. N. (2007). Common toads (Bufo arenarum) learn to anticipate and avoid hypertonic saline solutions. J. Comp. Psychol. 121, 419–427. doi: 10.1037/0735-7036.121.4.419
Dodd, C. K., Barichivich, W. J., and Smith, L. L. (2004). Effectiveness of a barrier wall and culverts in reducing wildlife mortality on a heavily traveled highway in Florida. Biol. Conserv. 118, 619–631. doi: 10.1016/J.BIOCON.2003.10.011
Fahrig, L., Pedlar, J. H., Pope, S. E., Taylor, P. D., and Wegner, J. F. (1995). Effect of road traffic on amphibian density. Biol. Conserv. 73, 177–182. doi: 10.1016/0006-3207(94)00102-V
Forman, R. T. T., and Alexander, L. E. (1998). Roads and their major ecological effects. Annu. Rev. Ecol. Syst. 29, 207–231. doi: 10.1146/annurev.ecolsys.29.1.207
Forman, R. T. T., Sperling, D., Bissonette, J. A., Clevenger, A. P., Cutshall, C. D., Dale, V. H., et al. (2003). Road ecology: science and solutions. Washington: Island press.
Gerhardt, H. C. (1995). “Phonotaxis in female frogs and toads: Execution and design of experiments” in Methods in Comparative Psychoacoustics: BioMethods. eds. G. M. Klump, R. J. Dooling, R. R. Fay and W. C. Stebbins (Basel: Birkhäuser).
Green, A. J. (2001). Mass/length residuals: Measures of body condition or generators of spurious results? Ecology 82, 1473–1483. doi: 10.1890/0012-9658(2001)082[1473:MLRMOB]2.0.CO;2
Glista, D. J., DeVault, T. L., and DeWoody, J. A. (2009). A review of mitigation measures for reducing wildlife mortality on roadways. Landsc. Urban Plan. 91, 1–7. doi: 10.1016/j.landurbplan.2008.11.001
Gourevitch, E. H. Z., and Roger Downie, J. (2018). Evaluation of tree frog tracking methods using Phyllomedusa trinitatis (Anura: Phyllomedusidae). J. neotropical herpetology 17, 233–246. doi: 10.11606/ISSN.2316-9079.V17I2P233-246
Joly, P. (2019). Behavior in a changing landscape: using movement ecology to inform the conservation of pond-breeding amphibians. Front. Ecol. Evol. 7:155. doi: 10.3389/fevo.2019.00155
Leskovar, C., Oromi, N., Sanuy, D., and Sinsch, U. (2006). Demographic life history traits of reproductive natterjack toads (Bufo calamita) vary between northern and southern latitudes. Amphib. Reptil. 27, 365–375. doi: 10.1163/156853806778189918
Li, M., Shi, L., and Wang, X. (2021). Physical mechanisms behind the wet adhesion: From amphibian toe-pad to biomimetics. Colloids Surfaces B Biointerfaces 199:111531. doi: 10.1016/J.COLSURFB.2020.111531
Maxwell, S. L., Fuller, R. A., Brooks, T. M., and Watson, J. E. M. (2016). Biodiversity: the ravages of guns, nets and bulldozers. Nature 536, 143–145. doi: 10.1038/536143a
Miaud, C., Sanuy, D., and Avrillier, J.-N. (2000). Terrestrial movements of the natterjack toad Bufo calamita (Amphibia, Anura) in a semi-arid, agricultural landscape. Amphibia-Reptilia 21, 357–369. doi: 10.1163/156853800507426
Morand, A., and Carsignol, J. (2019). Amphibiens et dispositifs de franchissement des infrastructures de transport terrestre. CEREMA, Connaissances 58.
Muller, B. J., and Schwarzkopf, L. (2017). Success of capture of toads improved by manipulating acoustic characteristics of lures. Pest Manag. Sci. 73, 2372–2378. doi: 10.1002/ps.4629
Oromi, N., Sanuy, D., and Sinsch, U. (2012). Altitudinal variation of demographic life-history traits does not mimic latitudinal variation in natterjack toads (Bufo calamita). Zoology 115, 30–37. doi: 10.1016/j.zool.2011.08.003
Ottburg, F. G. W. A., and van der Grift, E. A. (2019). Effectiveness of road mitigation for common toads (Bufo bufo) in the Netherlands. Front. Ecol. Evol. 7:23. doi: 10.3389/fevo.2019.00023
Petrovan, S. O., and Schmidt, B. R. (2016). Volunteer conservation action data reveals large-scale and long-term negative population trends of a widespread amphibian, the common toad (Bufo bufo). PLoS One 11:e0161943. doi: 10.1371/JOURNAL.PONE.0161943
Romin, L. A., and Bissonette, J. A. (1996). Deer: vehicle collisions: status of state monitoring activities and mitigation efforts. Wildlife Society Bulletin 24, 276–283.
Rytwinski, T., Soanes, K., Jaeger, J. A. G., Fahrig, L., Findlay, C. S., Houlahan, J., et al. (2016). How effective is road mitigation at reducing road-kill? A meta-analysis. PLoS 11: e0166941. doi: 10.1371/journal.pone.0166941
Schmidt, S., Schmidt, B. R., and Zumbach, S. (2008). Amphibian road mortality and how to prevent it: a review. Urban Herpetol. 3, 157–167. doi: 10.5167/uzh-10142
Schwabe, K. A., Schuhmann, P. W., and Tonkovich, M. (2002). A dynamic exercise in reducing deer-vehicle collisions: Management through vehicle mitigation techniques and hunting. J. Agric. Resour. Econ. 27, 261–280. doi: 10.22004/ag.econ.31078
Semlitsch, R. D. (2008). Differentiating migration and dispersal processes for pond-breeding amphibians. J. Wildl. Manag. 72, 260–267. doi: 10.2193/2007-082
Stuart, S. N., Chanson, J. S., Cox, N. A., Young, B. E., Rodrigues, A. S. L., Fischman, D. L., et al. (2004). Status and trends of amphibian declines and extinctions worldwide. Science 306, 1783–1786. doi: 10.1126/science.1103538
Speybroeck, J., Beukema, W., Bok, B., Van der Voort, J., and Llian, V. (2018). Guide Delachaux des amphibiens et reptiles de France et d’Europe. Paris: Delachaux et Niestlé.
Temple, H. J., and Cox, N. (2009). European red list of amphibians. Luxembourg: Office for Official Publications of the European Communities.
Testud, G., and Miaud, C. (2018). “From effects of linear transport infrastructures on amphibians to mitigation measures” in Reptiles and Amphibians. Vol. 1. ed. D. R. Aguillón Gutiérrez (London: IntechOpen), 85–97.
Wilbur, H. M. (1980). Complex life cycles. Annu. Rev. Ecol. Syst. 11, 67–93. doi: 10.1146/annurev.es.11.110180.000435
Woltz, H. W., Gibbs, J. P., and Ducey, P. K. (2008). Road crossing structures for amphibians and reptiles: Informing design through behavioral analysis. Biol. Conserv. 141, 2745–2750. doi: 10.1016/j.biocon.2008.08.010
Yeager, A., Commito, J., Wilson, A., Bower, D., and Schwarzkopf, L. (2014). Sex, light, and sound: location and combination of multiple attractants affect probability of cane toad (Rhinella marina) capture. J. J Pest Sci (2004) 87, 323–329. doi: 10.1007/s10340-014-0555-9
Keywords: movement barriers, mitigation, road ecology, amphibians, conservation
Citation: Conan A, Le Brishoual M, Garnier L, Fleitz J, Dehaut N, Enstipp M, Jumeau J and Handrich Y (2023) Efficacy of permanent wildlife fences as barriers to amphibian movement. Front. Ecol. Evol. 11:1074072. doi: 10.3389/fevo.2023.1074072
Edited by:
Silviu O. Petrovan, University of Cambridge, United KingdomReviewed by:
Sarah E Perkins, Cardiff University, United KingdomPhilip Wheeler, The Open University (United Kingdom), United Kingdom
Copyright © 2023 Conan, Le Brishoual, Garnier, Fleitz, Dehaut, Enstipp, Jumeau and Handrich. This is an open-access article distributed under the terms of the Creative Commons Attribution License (CC BY). The use, distribution or reproduction in other forums is permitted, provided the original author(s) and the copyright owner(s) are credited and that the original publication in this journal is cited, in accordance with accepted academic practice. No use, distribution or reproduction is permitted which does not comply with these terms.
*Correspondence: Antonin Conan, ✉ YW50b25pbi5jb25hbkBpcGhjLmNucnMuZnI=