Corrigendum: Delineating origins of cheetah cubs in the illegal wildlife trade: improvements based on the use of hair δ18O measurements
- 1NHRC Stable Isotope Laboratory, Environment and Climate Change Canada, Saskatoon, SK, Canada
- 2Cheetah Conservation Fund, Otjiwarongo, Namibia
- 3Department of Biology, University of Western Ontario, London, ON, Canada
All African felids are listed as vulnerable or endangered according to the IUCN (International Union for Conservation of Nature) Red List of Threatened Species. Cheetahs (Acinonyx jubatus) in particular have declined rapidly as a result of human impacts so that development of effective strategies and tools for conservation of this highly vulnerable species, as well as African felids in general, are essential for their survival in the wild. Here we use the oxygen stable isotopic compositions of cheetah hair to determine origins of cheetah cubs destined for the illegal exotic pet trade by associating individual cubs with predicted δ18O isoscape locations. We found that cheetah cubs most likely originated in East Africa, close to the corridors responsible for this aspect of the illegal wildlife trade to the Middle East. Further refinement of these assignments using a two isotope analysis (δ18O and δ13C values) indicate that these cubs were likely sourced in Southern Ethiopia or possibly as far as Tanzania. We also demonstrate that δ18O values in tissues can provide provenance information in cases where results of δ2H analyses may be obscured by the effects of metabolic routing of nutrients during nursing, starvation, or dehydration. This study demonstrates the utility of stable isotopic tools for conservation and forensic uses for endangered mammalian species.
1. Introduction
The cheetah (Acinonyx jubatus) is one of the most endangered felids in the world. Once wide ranging, they are now only extant in parts of Africa, except for a very small, critically endangered, population of the sub-species Acinonyx jubatus venaticus (Asiatic cheetah) in Iran (Farhadinia, 2004). Asiatic cheetahs were hunted to extinction in India, the last three likely to have been killed in 1948 (Velho et al., 2012). The subsequent half century has seen the remaining cheetah distributions contract sharply to only about 10% of their historical range and their numbers similarly decline to only about 7,000 individuals, with over half of the worlds remaining cheetahs resident in southwest Africa (Durant et al., 2017). Other populations are fragmented and small, with numbers of 200 or fewer. The threats to cheetahs are many and, like other wild felids, are primarily the result of anthropogenic pressures. Sub-Saharan Africa has one of the largest growing human populations (Cilliers, 2017) and this has resulted in decimation of wild felid numbers as a consequence of habitat destruction, agricultural expansion, prey reduction, livestock related conflicts, and direct hunting for food, trophies, or curatives (Ripple et al., 2014; Di Minin et al., 2016; Wolf and Ripple, 2017). Cheetahs are in direct competition for prey with other, larger, carnivores, such as lions (Panthera leo), leopards (Panthera pardus) and hyenas (Hyaenidae) and thus naturally occur in low numbers and are widely dispersed, a situation that makes conservation by inclusion in national parks difficult. Moreover, their low densities, declining numbers, and solitary nature has resulted in species genetic fragility (O'Brien et al., 1985). These combination of factors make the cheetah, perhaps of all the wild felids, especially vulnerable to existing and growing anthropogenic impacts (Cardillo et al., 2004).
An additional and emerging concern for conservation of cheetahs as well as other charismatic species is the illegal trade of wildlife parts and, more recently, as exotic pets. In some cases, the number of animals affected by the illegal pet market exceeds those from the smuggling of animal parts (Pires, 2012; Daut et al., 2015). Animals entering the exotic pet trade are distributed through illegal criminal organizations and are usually poached from wild populations, although some captive breeding facilities exist (Pires and Moreto, 2011; Dalberg, 2012; Ayling, 2013).
Cheetahs (once called “hunting leopards”) were historically kept and used for hunting in India because they are relatively less ferocious, smaller bodied, and easier to domesticate than other big cats (O'Brien et al., 1986; Bothma and Walker, 1999). Although no longer used for hunting, they are still captured by the modern illegal wildlife trade in Africa whereby cubs are taken alive, usually after killing the mother, and subsequently sold into middle Eastern markets to be kept as “status” pets by the very wealthy (Warchol, 2004; Tricorache et al., 2018; Marker, 2019). Cub mortality during the smuggling process is high with most cubs dying before reaching market (Warchol et al., 2003). Kept as domestic pets, the outlook is similarly grim because cheetahs do poorly in captivity, surviving only a few years without large spatial freedom and specialized diets that mimic those of wild cheetahs (Marker-Kraus, 1997; Tordiffe et al., 2016). Cheetahs are difficult to breed in captivity (O'Brien et al., 1985) so that cubs for the pet trade must be continuously supplied from wild populations, already fragmented and vulnerable. As a result, along with increasing habitat loss, this particular aspect of illegal wildlife trade is now one of the largest current threats to cheetah conservation and survival.
Poached cheetah cubs are funneled through the horn of Africa, across the Gulf of Aden, and into the Arabian Peninsula on their way to illegal markets for exotic pets. The semi-autonomous region of Somaliland, in conjunction with other wildlife agencies, manage to intercept some of these shipments and confiscate a few of the over 300 cheetah cubs that are estimated to move along trafficking routes and into the pet trade every year (Tricorache et al., 2018; Marker, 2019). However, while rescued in Somaliland, the origins of these cubs remain unknown. This is of great concern to conservation and law enforcement agencies, because it is important to know where these cubs are captured so that they may focus their conservation efforts. To address this, we measured the H, O, N, and C stable isotopic compositions of hair from rescued cheetah cubs to try to determine their origins.
The stable isotopic compositions of animal tissues hold great potential for conservation because they may be linked to provenance (Meier-Augenstein et al., 2013; Chesson et al., 2018) or determination of migration patterns of wildlife (Hobson and Wassenaar, 2008). The H and O stable isotopic compositions of hair are related to those of body water, and thus ingested environmental waters (Hobson et al., 1999). Because the H and O stable isotopic compositions of water varies regionally, so do those of animal tissues. Similarly, the carbon isotopic compositions of tissues are related to those of regional vegetation which varies with the relative distribution of C3 and C4 plants and is transferred upwards through the food web. The resulting isotopic landscapes, or “isoscapes,” can be used to link organisms with their environment (West et al., 2009; Hobson and Wassenaar, 2018; Vander Zanden et al., 2018). Because of their water conserving nature and obligate carnivore physiology, isotopic linking of felids to environmental water and thus location was not considered feasible (Pietsch et al., 2011). However, a recent study by Koehler and Hobson (2019) has determined that a relationship between environmental water and felid hair does indeed hold for domestic and wild cats and therefore may be used for provenance estimation. A remaining issue pertains to the use of cub hair because young mammals may be influenced by maternal nutrient inputs through nursing and such maternal effects have not been previously considered in isotopic assignments. Our objective, then, was to use isotopic techniques to establish the provenance of cheetah cubs confiscated in Somaliland and to explore means to resolve the maternal nutrient transfer issue. Ultimately though, it is our hope that this proof of concept can be further expanded to other terrestrial mammals, particularly those that are currently threatened by illegal activities.
2. Methods
Hair samples (ca. 20 mg) were collected from 2015 to 2021 during veterinary health checks from lawfully confiscated cheetah cubs (n = 96) after rescue in Somaliland. The ages of cubs ranged between 3 and 7 months, as determined by veterinary assessments after rescue. All hair samples were shipped to the NHRC Stable Isotope Laboratory under CITES export permits 0061077 and 0063888 and imported with Canadian CITES import permit 21CA01252/CWHQ. This study was approved by the University of Saskatchewan Research Services and Ethics Office, RSEO Reference number 003Exempt2020.
2.1. Stable isotope analysis
Stable isotopic compositions of all hair samples were performed at the NHRC Stable Isotope Laboratory of Environment and Climate Change Canada in Saskatoon, SK, Canada. Prior to analyses, hair samples were cleaned of adherent debris and any surface oils were removed by rinsing in 2:1 chloroform:methanol. Hair was homogenized to powder with a ball grinder (Retsch model MM-301, Haan, Germany). The root end of the hairs were discarded as these will reflect the most recent growth and may include hair grown in captivity prior to rescue. For hydrogen and oxygen, our approach involved the measurement of both δ2H and δ18O values with the same analytical run (i.e., both H2 and CO gases were analyzed from the same pyrolysis) (Hobson and Koehler, 2015). Samples and standards were weighed to 350 ± 20 μg in silver capsules and analyzed using a Delta V Plus IRMS system (Thermo Finnigan, Bremen, Germany) equipped with a Costech Zero-Blank autosampler. The helium carrier gas rate was set to 120 mL/min. We used a HTC 1.5 m 0.25 inch 5 Å molecular sieve (80–100 mesh) GC column. The glassy carbon reactor was operated at a temperature of 1,400°C, and the GC column temperature was set to 90 °C. After separation, the gases were introduced into a Delta V plus isotope-ratio mass spectrometer via a ConFlo IV interface (Thermo Finnigan, Bremen, Germany). The eluted N2 was flushed to waste by withdrawing the CF capillary from the ConFlo interface. We used Environment Canada keratin reference standards CBS (Caribou hoof) and KHS (Kudu horn) to calibrate sample δ2H (–197 and –54.1 per mil, respectively) and δ18O values (+2.50 and +21.46 per mil, respectively Qi et al., 2011). This normalization with calibrated keratins also corrects for any hydrogen isotope measurement artifact caused by production of HCN (Gehre et al., 2015) in the glassy carbon reactor as described by Soto et al. (2017). Based on replicate (n = 10) within-run measurements of keratin standards and from historical analyses of an in-house QA/QC reference (SPK keratin), sample measurement error was estimated at ±2 per mil for δ2H and ± 0.4 per mil for δ18O . All H results are reported for nonexchangeable H and for both H and O in the standard delta notation, normalized on the Vienna Standard Mean Ocean Water—Standard Light Antarctic Precipitation (VSMOW-SLAP) scale.
For carbon and nitrogen isotope analyses, we weighed 1 mg of ground hair into precombusted tin capsules. Encapsulated hair was combusted at 1,030°C in a Carlo Erba NA1500 elemental analyser. The resulting N2 and CO2 were separated chromatographcally and introduced to an Elementar Isoprime isotope ratio mass spectrometer (Langenselbold, Germany–www.elementar.de). We used two calibrated in–house reference materials to normalize the results to VPDB and AIR: BWBIII keratin (δ13C = –20.18, δ15N = 14.31 per mil, respectively) and PRCgel (δ13C = –13.64, δ15N = 5.07 per mil, respectively). Precisions as determined from both reference and sample duplicate analyses and from within-run analyses of QA/QC bovine gelatin (BVgel) were ± 0.1 per mil for both δ13C and δ15N . For both HO and CN stable isotope analyses, most samples were run in duplicate.
2.2. Assignment to origin
We used geographical assignment algorithms as described in Van Wilgenburg and Hobson (2011). Briefly, we used rastered δ18O hair-specific isoscapes as a basis for predicting origins of cheetah cubs produced from the rescaling factors measured for known–origin cats across North America (Figure 1A) (Koehler and Hobson, 2019). These hair δ18O isoscapes were then imported into custom R scripts using the rgdal, sp and raster packages and used to plot 2-D spatial data in map form, and perform spatial statistics on the raster surfaces. We used current cheetah range shapefiles from the IUCN, but did not include the ranges of two critically endangered (i.e., < 30 individuals) cheetah subspecies, the Asiatic cheetah (A. j. venaticus) and the North African cheetah (A. j. hecki). We then applied a normalized probability density method (Hobson et al., 2009) to estimate the probability that individual cells in the calibrated felid isoscape represented a potential origin for each cub at the 67% (i.e., 2:1 odds ratio) confidence level. Digital file manipulation and assignment to origin analyses were conducted using multiple packages including “raster” v.3.6-3 (Hijmans and Van Etten, 2015) and “maptools” v. 1.1-4 (Bivand and Lewin-Koh, 2015) in the R statistical computing environment v.4.1.2 (R Core Team, 2021).
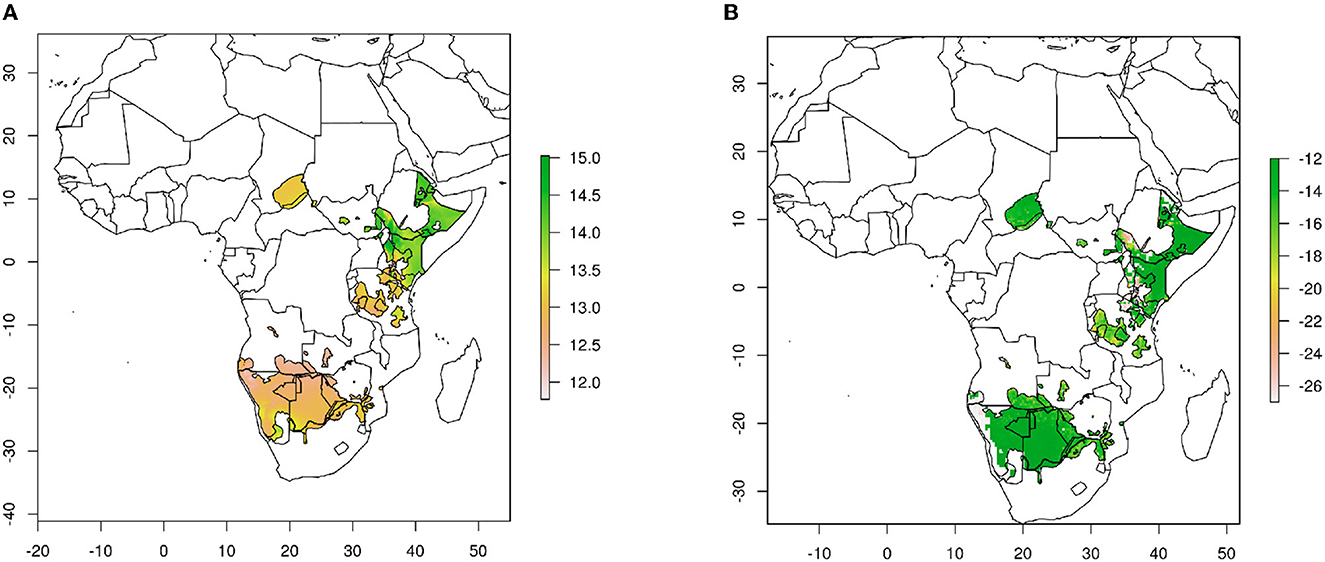
Figure 1. Cheetah hair isoscapes used in this study. (A) δ18O values. (B) δ13C values. Base maps produced from data of Terzer et al. (2013) and Terzer-Wassmuth et al. (2021) for δ18O and Still and Powell (2010) for δ13C.
For carbon isotopes, we used δ13C isoscapes for Africa produced from the theoretical spatial distribution of plants obtained from C3/C4 plant abundance remote sensing maps (Still and Powell, 2010; Hobson et al., 2012). We did not use a rescaling factor because previous studies have indicated that cheetah fur has similar δ13C values to those of food (Voigt et al., 2014), whereas an enrichment of 1 to 4 per mil is measured for other felids (Parng et al., 2014; Montanari and Amato, 2015) (Figure 1B).
3. Results and discussion
The δ2H, δ18O, δ13C, and δ15N values of hair from 96 cheetah cubs are shown in Table 1. Values of δ15N of these cubs ranged from +8.6 to +16.9 per mil, and are significantly higher (mean = 13.4, σ = 1.7 per mil) than those reported values for African lions or leopards (Codron et al., 2007). Adult cheetahs from Namibia have similar δ15N values (+12.3 per mil) to those of other African felids (Voigt et al., 2014), largely because most felid species tend to prey on ungulates or other small bodied herbivores (Eaton, 1970; Mills, 1984). The most likely reason for the observed high δ15N values in these cheetah cubs is the well known trophic effect from nursing young to offspring, although other effects such as malnutrition, cannot be ruled out. Adult mammals isotopically integrate their environment through coupling of H, O, C, and N isotopes into tissues by direct ingestion of food and water (Hobson and Wassenaar, 2018). For nursing young, however, this relationship is complicated because young mammals obtain a significant amount of environmental water and nutrients indirectly through maternal milk thereby placing young at a higher trophic position (i.e., higher δ15N values) than their mothers. The high lipid content of milk also tends to drive cub tissue δ13C values lower than those formed after weaning.
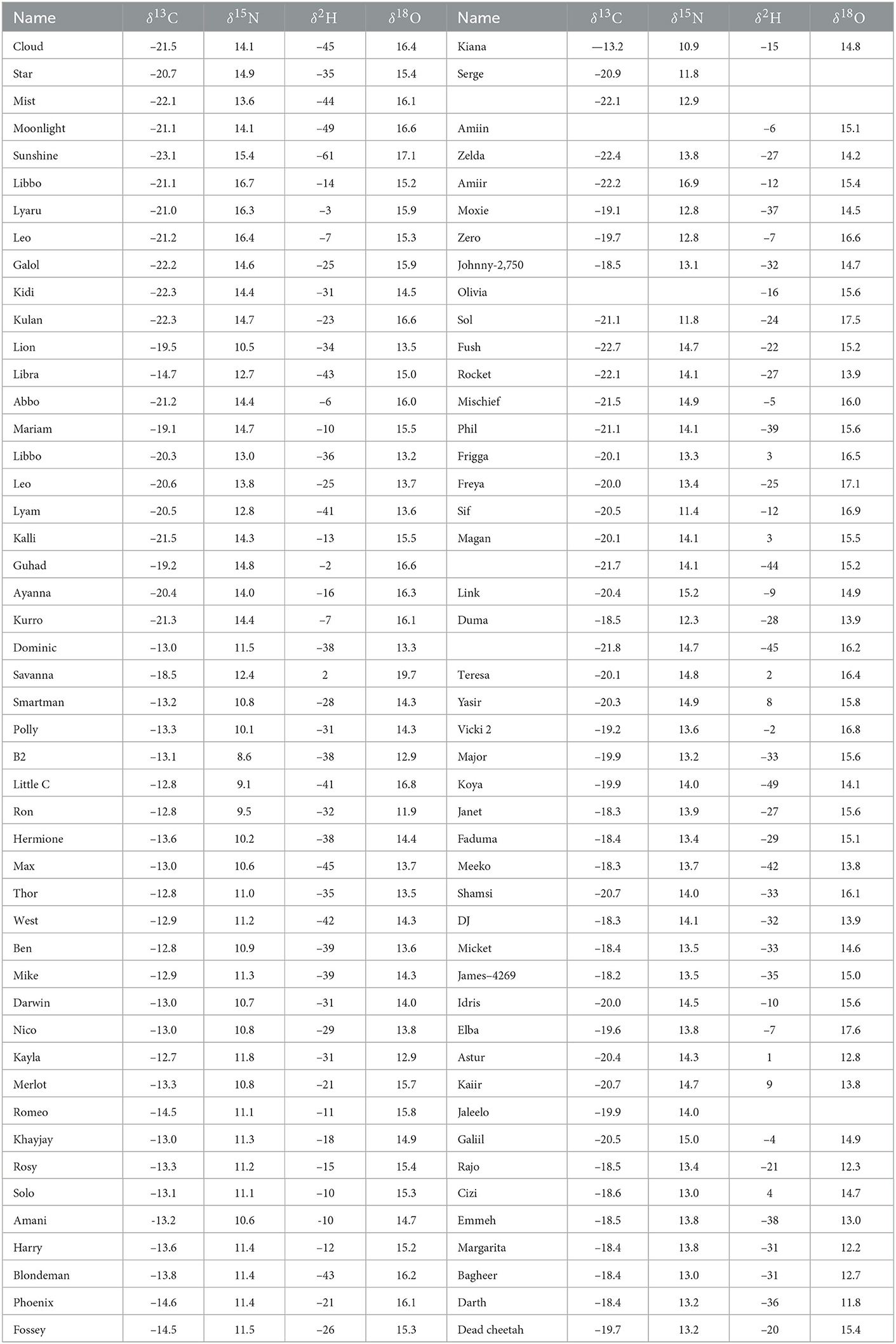
Table 1. Nitrogen, carbon, hydrogen, and oxygen stable isotopic compositions of hair from cheetah cubs confiscated from the illegal pet trade, Somaliland.
With the exception of humans, there is very little information in the literature on the relative discrimination of stable isotopes between tissues of mothers and nursing young. A few studies have compared the stable isotopic compositions of herbivores, generally cows (Bos taurus), with diet (Kornexl et al., 1997; Camin et al., 2008), or location (Chesson et al., 2010), mostly for detection of food adulteration. Jenkins et al. (2001) determined that isotopic differences between maternal and offspring plasma were variable and species dependent. Lipids are generally 13C depleted relative to other animal tissues (Tieszen et al., 1983) and also to the rest of the components in milk (Melzer and Schmidt, 1987; Wilson et al., 1988), so that we would expect tissues of nursing offspring to have lower δ13C values than those of the mother. The low δ13C values of many of these cheetah cubs (Table 1) compared with Namibian cheetahs (δ13C = –14.8 per mil Voigt et al., 2014) are consistent with this, however the δ13C values of the maternal diet are unknown so it is impossible to make comparisons.
Polar bear (Ursus maritimus) cubs have higher δ15N values and lower δ13C values than do adults from the same subpopulations (Polischuk et al., 2001; Koehler et al., 2019). Hair δ13C and δ2H values of polar bear cubs are typically lower than those of adults (Koehler et al., 2019), most likely because of the high lipid content of maternal milk with correspondingly low δ13C and δ2H values. The amount of the differences in these isotopic compositions is likely dependent on the relative ages of cubs, and thus amount of nursing, and of the lipid content of the milk. The fat content of cheetah milk (64 g/kg) is lower than milk from polar bears (350 g/kg Derocher et al., 1993) and slightly lower than lions or domestic cats (Felis catus) (De Waal et al., 2004; Osthoff et al., 2006). Therefore compared to polar bears, we would certainly expect a smaller or perhaps no difference in δ2H and δ13C values between adult cheetahs and cheetah cubs. Ultimately, though, there is no way to determine this other than using controlled diet studies.
Values of hair δ2H for these Cheetah cubs were more variable and positive than we anticipated, ranging from –61 to +9 per mil. Because the δ2H values of lipids are much lower than that of other macromolecules (Sessions et al., 1999; Sachse et al., 2012), we expected the δ2H values of nursing cub hair to be lower than those of adult cats relative to their oxygen isotopic compositions, however this was clearly not the case (Figure 2). One possible explanation for this observation is that the relative enrichment in 2H in hair in cubs is the result of a trophic effect between mother and cub. Because these cubs were generally young (3–7 months), it is likely that a portion of the hair was grown before birth and prior to nursing and introduction of the lipid-rich milk diet.
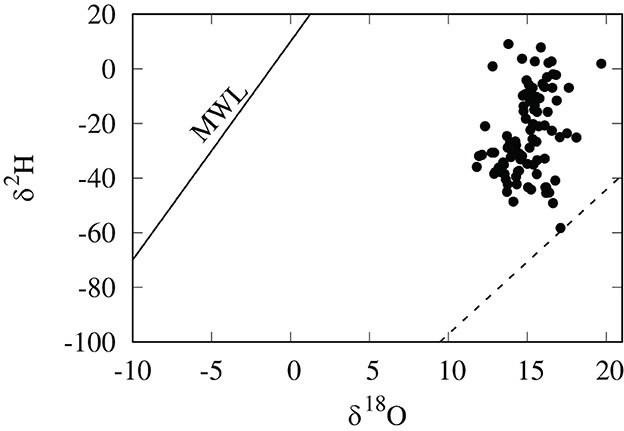
Figure 2. δ2H and δ18O values in per mil (SMOW) of cheetah cub hair. The dashed line is the relationship for domestic and wild cats in North America (Koehler and Hobson, 2019). Also shown is the global meteoric water line (MWL) of Craig (1961).
For placental mammals, in utero nutrient and gas exchange occurs across the fetal villi without exchange of blood. In humans, fetal amino acid concentrations are generally higher than maternal levels (Cetin et al., 1992), reflecting an active transport mechanism across the placental membranes. It seems unlikely that active transport of large molecules such as amino acids will result in significant isotopic fractionation, and thus we would expect the familiar trophic effect in utero that is observed in nursing young, and is consistent with the δ15N values of cubs in this survey. This has been observed in humans, where δ15N and δ13C values of newborn infant hair were higher than those of the mother (De Luca et al., 2012). For hydrogen isotopes, a trophic enrichment of 30 to 50 per mil is predicted from mechanistic models (Magozzi et al., 2019) and observed in a few studies (Birchall et al., 2005; Topalov et al., 2013). If this is the case for cheetahs, the variability in hydrogen isotope enrichment in cheetah cubs may be related to their variable ages where the δ2H values of older cubs would be more negative, reflecting more a contribution of nursing in the hair. Unfortunately, we do not have exact ages for the cubs and, historically, it is has been difficult to separate a hydrogen isotopic trophic effect from other metabolic and dietary processes with any certainty (reviewed by Vander Zanden et al., 2016).
Another complication with the cheetah cubs we examined was that they are usually extremely malnourished and dehydrated when rescued. Starvation in humans generally increases the δ13C and δ15N of hair by recycling of proteins within the body pool (Hatch et al., 2006; Mekota et al., 2006), a phenomenon also noted in birds (Hobson et al., 1993; Cherel et al., 2005). Nutritional and water stress has been suggested as the mechanism for large increases in the δ15N values in bone collagen in African herbivores (Ambrose and DeNiro, 1986). For hydrogen and oxygen isotopes, however, there appears to be very little in the literature on the effects of starvation on the isotopic compositions of animal protein. For dehydration, it seems intuitive that evaporation concurrent with respiration and diffusive water loss would result in higher δ2H and δ18O values of the body water pool with corresponding increases of those of hair. Indeed, McKechnie et al. (2004) observed that feathers of water-stressed Rock Doves (Columba livia) were enriched in 2H, similar to previous observations in humans (Schoeller et al., 1986). However, the picture is not as clear for oxygen isotopes for which there are very few measurements. Storm-Suke et al. (2012) observed similar hydrogen isotope results in Japanese quail (Coturnix japonica) as was observed for Rock Doves by McKechnie et al. (2004), but did not see any differences for oxygen isotopes. Ultimately, though, whether it can be attributed to maternal trophic effects, malnutrition, or dehydration, the relatively high δ2H values of these cubs remain enigmatic.
For geographical assignments, tissue δ2H values in consumers are generally preferred over δ18O values because of their greater range and smaller relative error (Hobson and Koehler, 2015). But, because of all the aforementioned uncertainties involved with the use of δ2H values of hair from cheetah cubs, we decided to avoid these complexities altogether and instead use hair δ18O values for geographical placement. The δ18O values of adult and cub hair from polar bears from the same locations did not show any significant difference, although there were large differences in δ2H , δ15N, and δ13C values (Koehler et al., 2019). This is most likely because lipids in maternal milk contain little oxygen and the δ18O values of milk protein is similar to those of the maternal diet. Because oxygen is more weakly routed from diet to consumer tissues, it is likely that the isotopic compositions of oxygen in these tissues will be less confounded by environmental and dietary considerations than hydrogen (Bowen et al., 2009; Soto et al., 2011; Wolf et al., 2015), and thus will more closely reflect the isotopic compositions of environmental water. It is currently unknown whether malnutrition or dehydration can cause changes to the δ18O values of mammal hair.
3.1. Geographical origins of cheetah cubs
Cheetah females are polyesterous and are thus non-seasonal breeders (Crosier et al., 2018, 2022). As a result, cubs are captured in the illegal trade throughout the year, with a slight bias to dry season (Jul-Aug), perhaps as a result of easier transport along illegal trade routes. Assuming the cubs are, on average, 6 months old, this indicates they were likely born during a wet season in East Africa. Here, with the exception of northern Ethiopia, the majority of precipitation falls during two wet seasons (Levin et al., 2009). In this case, the mean annual oxygen isoscape is appropriate because the bulk of the precipitation occurs in the wet seasons and the isoscape is representative of this. Considering the wide range of ages of the cubs and that cubs can be taken at any time during the year, it is impossible to associate a particular season with most of the cubs with any accuracy.
For geographic placement, we cannot assume a single batch of cubs captured at a single time. In reality, cubs were captured in small batches (1 to a few) from 2015 to 2021. We also have to consider the isotopic integration that occurs through the foodweb which will tend to average out wet season-dry season differences in stable isotopic compositions of hair. While prey species are more closely coupled to the underlying precipitation isoscape, they will also tend to average out yearly precipitation to some extent based on growth characteristics and seasonal isotope patterns in plants. Considering all of this, we treated all 96 cubs as a single batch of time-integrated samples and assigned them to origin using the mean amount averaged oxygen isoscape. This approach provides an estimation of the total geographic range of cub origins, an important consideration for law enforcement and conservation.
Because oxygen isotopic compositions are rarely used, it is important to take into account the error envelope involved in geographical placement of cheetah cubs. If we examine the standard error of δ18O values reported for the RCWIP dataset and translate those to the cheetah isoscape as seen on Figure 1A, oxygen isotopic compositions of cheetah fur are only expected to vary by about 0.4 per mil at any single location in east Africa, similar to our measurement error for δ18O values. Our measured range of δ18O values in cheetah fur exceeds 5 per mil (Table 1) so that we are confident that geographic placement is outside the analytical error. In addition, the assignment algorithm takes this error into account so that the oxygen isotopic composition of any cub that matches the isoscape does so at the 2:1 odds ratio or the 67% (1σ) confidence interval.
The Geographical placement of cheetah cubs based on δ18O values of hair are shown in Figure 3. This analysis places these cubs in northwestern Kenya, northern Ethiopia, or southern Ethiopia close to the border with Somalia. These areas, particularly along the Somalian border are close to the expected routes for the illegal trade in live cubs (Marker, 2019).
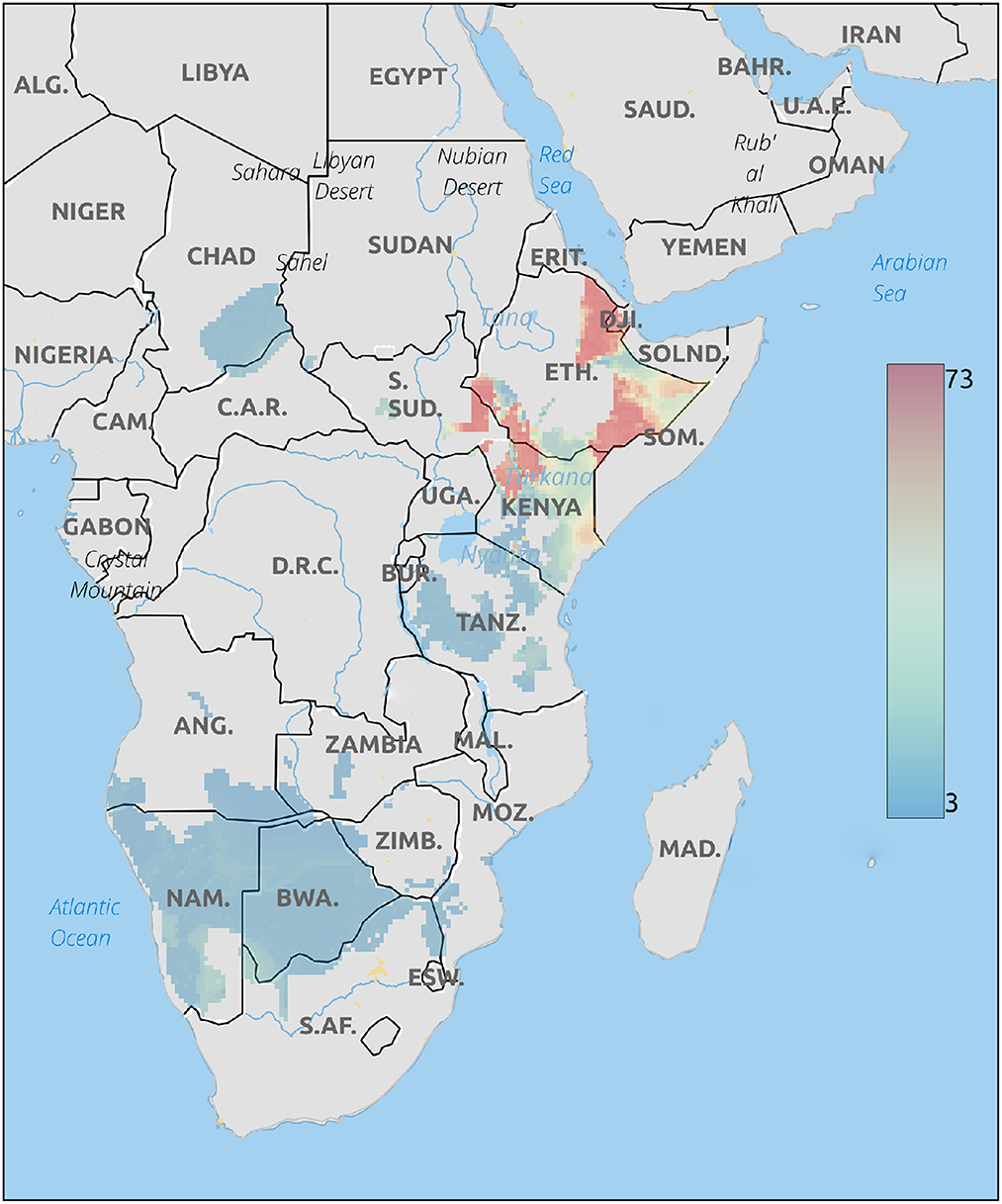
Figure 3. Probable origins of cheetah cubs rescued from the illegal pet trade based on δ18O isoscapes. Legend is the number of individuals assigned to a pixel based on the odds ratio criterion used.
Although regionally accurate, assignments of cheetah cubs to geographic origin are fairly broad based only on δ18O values. To further refine these assignments we examined the utility of a multivariate placement using both δ18O and δ13C values. Values of δ13C of cheetah cubs range from –22.7 to –12.7 per mil and show a clear bimodal distribution (Figure 4 and Table 1). While relatively high δ13C values of cubs were expected from the east African savannahs where the C4 grasses dominate in the warm, arid lowlands (Tieszen et al., 1979b), many cubs had unexpectedly low δ13C values. At first, we attributed these low δ13C values of these cubs to consumption of a high lipid diet during nursing, but on further analysis this seems unlikely. Polar bear cubs have δ13C hair values that are only 1–3 per mil lower than those of adults (Koehler et al., 2019) concurrent with very high concentrations of lipids in maternal milk. Cheetahs have lower amounts of lipids in maternal milk, so it seems unlikely that there would be asimilar large negative offset in δ13C values between cheetahs adults and nursing young. If ingestion of lipid rich milk were responsible for the low δ13C values observed, we would also expect a similar decrease in δ2H values, but we see the opposite. Furthermore, fur from free ranging Namibian cheetahs have δ13C values about –14.8 per mil (Voigt et al., 2014), very close to the –12 to –13 per mil predicted from the African carbon isoscape (Figure 1B), which reflects the abundance of C4 plants and therefore C4-eating prey in tropical grasslands. Feeding studies of adult cheetahs also indicate there only minor differences in δ13C values between cheetahs and food sources (Voigt et al., 2014). These observations suggest that, overall, the isotopic discrimination of carbon isotopes is close to 0 per mil between cheetahs and their environment. Therefore, if the consumption of a lipid rich milk is responsible for the low δ13C values measured in these cubs, we would expect that hair from cheetah cubs should have δ13C values around –15 per mil.
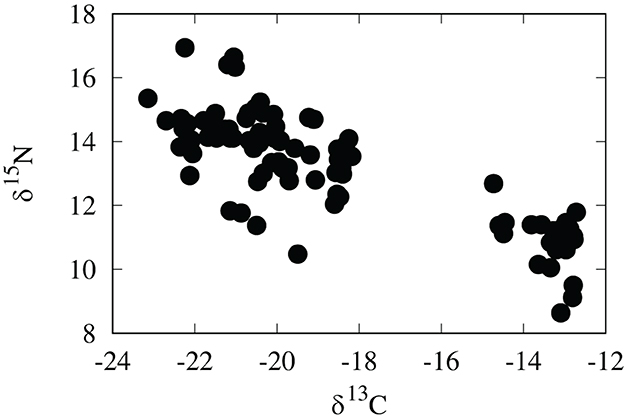
Figure 4. Carbon and nitrogen stable isotopic compositions of cheetah cub fur in per mil PDB and AIR, respectively.
An important consideration is that accurate transfer of carbon isoscapes based on terrestrial foodwebs have never been ground-tested for mammals. Stable carbon isoscapes are based on the predicted spatial proportions of C4 and C3 plants obtained from remote sensing and, as a result, there is a uncertainty differentiating between grasses and non-tree herbaceous layers in the present carbon isoscapes (Still and Powell, 2010). In theory, carnivores should integrate the total carbon isotopic compositions of these relative proportions and therefore should closely reflect their 13C environment. Herbivores tend to be selective and can be classified as grazers (C4-eating) or browsers (C3-eating). Therefore, provided that browsers and grazers are in proportion to the relative proportions of C3 to C4 plants and that predators are non-selective, the carbon isotopic compositions of predator tissues should be similar to those of the carbon isotope biome in which they inhabit.
In practice, however, it is undoubtedly more complicated. The δ13C values of tissues in carnivores will be a function not just of the underlying carbon isoscape, but will also be compounded by trophic discrimination factors, hunting behavior, and foraging behavior of prey. For higher level taxa, such as felids or canids, we would also expect a variation of hunting behaviors depending on availability and/or individual preferences. Indeed, we know that cheetahs can develop strategies to overcome particular prey animals (Mills, 1984; Marker et al., 2003) and this may account for the clear exploitation of either grazers or browsers as prey in a mixed tree/grass savannah (Figure 4). Higher δ15N values in cub hair with low δ13C values seem to confirm this as mixed feeding gazelles have relatively high δ15N values compared to grazers (Ambrose and DeNiro, 1986).
Impalas (Aepyceros melampus), Grants gazelles (Nanger granti) and Thompsons gazelles (Eudorcas thomsonii) comprise a large part of the diet of East African cheetahs (Eaton, 1970), all of which are mixed feeders wherein their diet consists of both C3 and C4 plants and varies temporally depending on species and the season (Spinage et al., 1980). Grants gazelles, which tend to browse more than they graze, occur in Ethiopia and South Sudan, whereas Thomsons gazelles and impalas, which can graze or browse, are more common in Kenya and Tanzania (Arctander et al., 1996). Consequently the δ13C values of the stomach contents of these prey species can vary from average values of –21.9 per mil for Grants gazelles to –17 per mil for Thompsons gazelles, with Impalas averaging about –19 per mil (Tieszen et al., 1979a). Therefore, it is likely that the bimodal δ13C and δ15N values of these cubs simply reflect prey preference of the mother and thus are only loosely coupled to the underlying carbon isoscape.
An alternate, but less likely, explanation is that the C3 group of cubs are younger because they were captured closer to the trafficking routes in the Horn of Africa in the mixed savannah proximal to the Ethiopian highlands. In this case, the low δ13C values will be augmented by lipids in milk and the elevated δ15N values may be partially the result of maternal trophic effects.
The dual-isotope model places the origins of these cubs primarily in south western Ethiopia, as do the assignments based only on δ18O values (Figure 5). However, the abundance of low δ13C values in cheetah cub hair suggests many cubs originated in the savannah highlands where most of the forbs, shrubs, and trees are C3 types or, possibly, as far south as the great rift region of Tanzania. Considering all of the complexity involved in transfer of the carbon isotopes into predator tissues, however, this analysis cannot be taken as diagnostic and is of limited usefulness. While it is unlikely that these cubs could survive transport over the >1,000 km between Tanzania and Somalia, it is certainly possible that many could have been captured in southwestern Ethiopia where, presumably, Grants gazelles would make up a larger part of the adult diet.
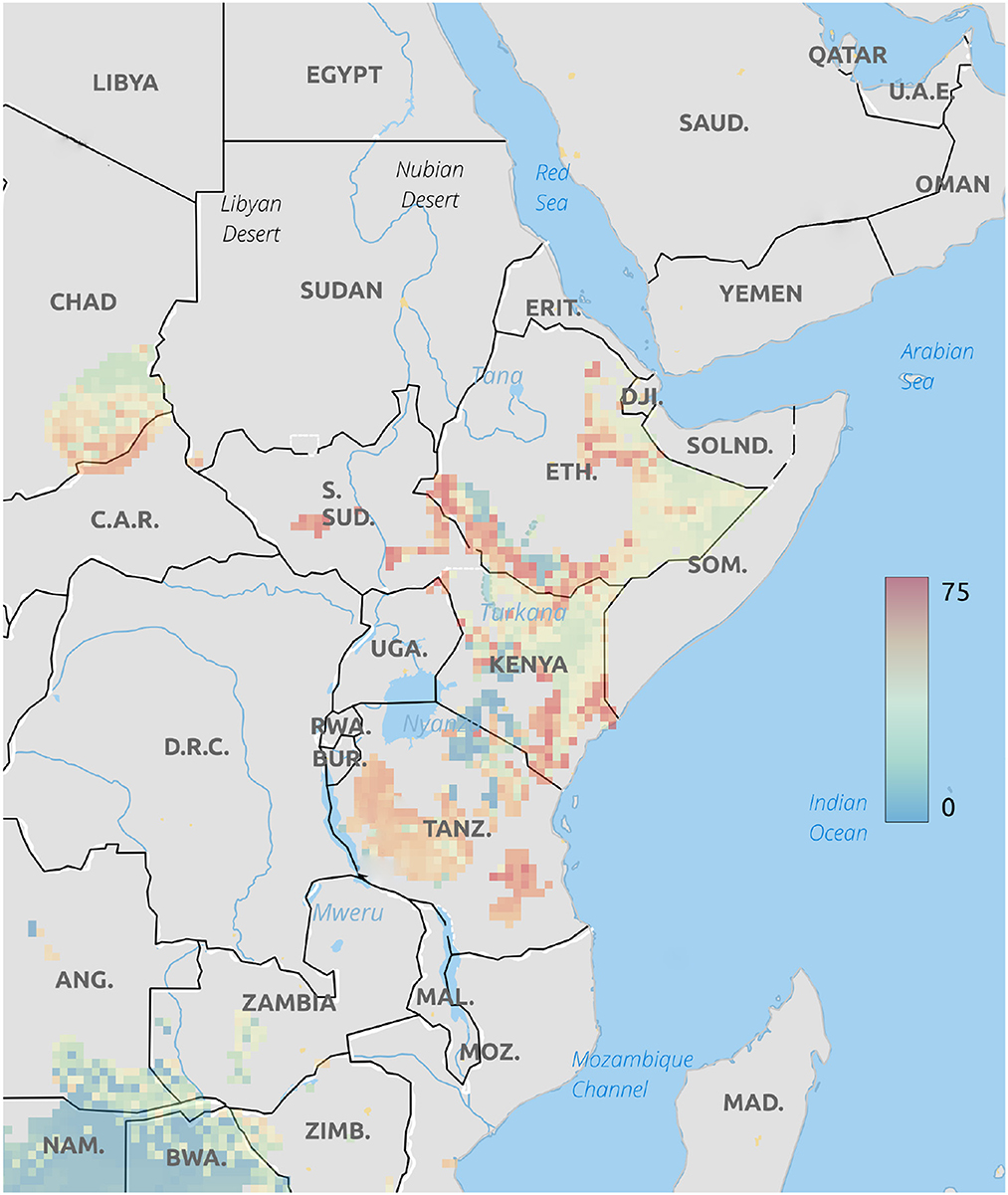
Figure 5. Multivariate isotope assignment of cheetah cubs to location based on a δ18O and δ13C values. Legend is the number of individuals assigned to a pixel based on the odds ratio criterion used.
4. Conclusions
Based on δ18O values only, it is evident that cheetah cubs destined for the illegal pet trade are sourced from East Africa, primarily northern Ethiopia, western Kenya, and along the Somali border with Kenya. Further refining these placements by using a two isotope multivariate assignment suggests that it is possible that these cubs could have been sourced as far south as Tanzania. The two isotope analysis is, at best, uncertain because of complexities relating the underlying carbon isoscape to the terrestrial foodweb. It seems obvious that geographic placement of cheetah cubs places them proximal to the illegal trade routes. However, this appoach also demonstrates the general usefulness of stable isotopic placement for forensic analyses in terrestrial mammals, particularly the carnivores. In addition, it is our strong recommendation that oxygen isoscapes be considered for geographic placement in cases where the normal transfer of nutrients into tissues are complicated by the effects of nursing or nutritional stress.
Moving forward, it is our hope that these isotopic techniques can be developed into a robust analytical protocol that can be used by law enforcement and conservation agencies to address the many aspects of the illegal wildlife trade, not just for cheetahs, but for other vulnerable or endangered species. To this end, the addition of other intrinsic markers, such as genetic or chemical information, will help to refine geographical placement or identification of individuals for forensic applications. In the short term, automatic sampling and archiving of all confiscated illegally captured cheetahs is needed. Ultimately though, successful development and use of stable isotopic techniques will require a concerted effort from scientists, law enforcement, conservation groups, and policy makers. Like cheetahs, many of the worlds iconic species are currently under threat and these novel avenues for wildlife conservation may contribute to their very survival.
Data availability statement
The original contributions presented in the study are included in the article/supplementary material, further inquiries can be directed to the corresponding author.
Ethics statement
This animal study was reviewed and approved by the University of Saskatchewan, 003Exempt2020.
Author contributions
GK and KH contributed to conception and design of the study. AS-K and LM orchestrated sampling and logistics. GK collected the data, performed the statistical analysis, and wrote the first draft of the manuscript. GK and KH contributed equally to manuscript revision and all authors approved the submitted version.
Funding
This study was funded by Environment and Climate Change Canada.
Acknowledgments
The contributions of Andrei Mihalca, Carolyn Farquhar, and Patricia Tricorache were essential for sample collection, CITES permits, and logistics. The help and guidance of the Cheetah Conservation Fund was invaluable and we look forward to working with them in the future. We thank Chantel Gryba and Jessica Fehr for sample preparation and for assistance in stable isotope analyzes.
Conflict of interest
The authors declare that the research was conducted in the absence of any commercial or financial relationships that could be construed as a potential conflict of interest.
Publisher's note
All claims expressed in this article are solely those of the authors and do not necessarily represent those of their affiliated organizations, or those of the publisher, the editors and the reviewers. Any product that may be evaluated in this article, or claim that may be made by its manufacturer, is not guaranteed or endorsed by the publisher.
References
Ambrose, S. H., and DeNiro, M. J. (1986). The isotopic ecology of east african mammals. Oecologia 69, 395–406. doi: 10.1007/BF00377062
Arctander, P., Kat, P. W., Aman, R. A., and Siegismund, H. R. (1996). Extreme genetic differences among populations of gazella granti, grant's gazelle, in kenya. Heredity 76, 465–475. doi: 10.1038/hdy.1996.69
Ayling, J. (2013). What sustains wildlife crime? rhino horn trading and the resilience of criminal networks. J. Int. Wildlife Law Policy 16, 57–80. doi: 10.1080/13880292.2013.764776
Birchall, J., O'Connell, T. C., Heaton, T. H. E., and Hedges, R. E. M. (2005). Hydrogen isotope ratios in animal body protein reflect trophic level. J. Anim. Ecol. 74, 877–881. doi: 10.1111/j.1365-2656.2005.00979.x
Bivand, R., and Lewin-Koh, N. (2015). maptools: Tools for reading and handling spatial objects. R package version 0.8–39.
Bothma, J. d. P, and Walker, C. (1999). “The cheetah,” in Larger Carnivores of the African Savannas (Springer), 92–115.
Bowen, G. J., Ehleringer, J. R., Chesson, L. A., Thompson, A. H., Podlesak, D. W., and Cerling, T. E. (2009). Dietary and physiological controls on the hydrogen and oxygen isotope ratios of hair from mid-20th century indigenous populations. Am. J. Phys. Anthropol. 139, 494–504. doi: 10.1002/ajpa.21008
Camin, F., Perini, M., Colombari, G., Bontempo, L., and Versini, G. (2008). Influence of dietary composition on the carbon, nitrogen, oxygen and hydrogen stable isotope ratios of milk. Rapid Commun. Mass Spectrometry 22, 1690–1696. doi: 10.1002/rcm.3506
Cardillo, M., Purvis, A., Sechrest, W., Gittleman, J. L., Bielby, J., and Mace, G. M. (2004). Human population density and extinction risk in the world's carnivores. PLoS Biol. 2, e197. doi: 10.1371/journal.pbio.0020197
Cetin, I., Marconi, A., Corbetta, C., Lanfranchi, A., Baggiani, A., Battaglia, F., et al. (1992). Fetal amino acids in normal pregnancies and in pregnancies complicated by intrauterine growth retardation. Early Hum. Dev. 29, 183–186. doi: 10.1016/0378-3782(92)90136-5
Cherel, Y., Hobson, K. A., Bailleul, F., and Groscolas, R. (2005). Nutrition, physiology, and stable isotopes: new information from fasting and molting penguins. Ecology 86, 2881–2888. doi: 10.1890/05-0562
Chesson, L. A., Barnette, J. E., Bowen, G. J., Brooks, J. R., Casale, J. F., Cerling, T. E., et al. (2018). Applying the principles of isotope analysis in plant and animal ecology to forensic science in the americas. Oecologia 187, 1077–1094. doi: 10.1007/s00442-018-4188-1
Chesson, L. A., Valenzuela, L. O., O'Grady, S. P., Cerling, T. E., and Ehleringer, J. R. (2010). Hydrogen and oxygen stable isotope ratios of milk in the united states. J. Agric. Food Chem. 58, 2358–2363. doi: 10.1021/jf904151c
Cilliers, J. (2017). “Fertility, growth and the future of aid in sub-Saharan Africa,” in Growth and the Future of Aid in Sub-Saharan Africa (New York: Springer).
Codron, D., Codron, J., Lee-Thorp, J. A., Sponheimer, M., Ruiter, D., and Brink, J. S. (2007). Stable isotope characterization of mammalian predator-prey relationships in a South African savanna. Eur. J. Wildl Res. 3, 161–170. doi: 10.1007/s10344-006-0075-x
Craig, H. (1961). Isotopic variations in meteoric waters. Science 133, 1702–1703. doi: 10.1126/science.133.3465.1702
Crosier, A., Byron, M., and Comizzoli, P. (2022). Connecting the spots: understanding cheetah reproduction to improve assisted breeding and population management. Theriogenology 185, 70–77. doi: 10.1016/j.theriogenology.2022.03.025
Crosier, A. E., Wachter, B., Schulman, M., Luders, I., Koester, D., Wielebnowski, N., et al. (2018). “Reproductive physiology of the cheetah and assisted reproductive techniques,” in Cheetahs: Biology and Conservation; ed A. Valutkevich (London: Elsevier Inc.), 385–402.
Daut, E. F., Brightsmith, D. J., and Peterson, M. J. (2015). Role of non-governmental organizations in combating illegal wildlife-pet trade in peru. J. Nat. Conservat. 24, 72–82. doi: 10.1016/j.jnc.2014.10.005
De Luca, A., Boisseau, N., Tea, I., Louvet, I., Robins, R. J., Forhan, A., et al. (2012). δ15N and δ13C in hair from newborn infants and their mothers: a cohort study. Pediatr. Res. 71, 598–604. doi: 10.1038/pr.2012.3
De Waal, H., Osthoff, G., Hugo, A., Myburgh, J., and Botes, P. (2004). The composition of African lion (Panthera leo) milk collected a few days postpartum. Mammalian Biol. 69, 375–383. doi: 10.1078/1616-5047-00159
Derocher, A. E., Andriashek, D., and Arnould, J. P. (1993). Aspects of milk composition and lactation in polar bears. Can. J. Zool. 71, 561–567. doi: 10.1139/z93-077
Di Minin, E., Slotow, R., Hunter, L. T., Pouzols, F. M., Toivonen, T., Verburg, P. H., et al. (2016). Global priorities for national carnivore conservation under land use change. Sci. Rep. 6, 23814. doi: 10.1038/srep23814
Durant, S. M., Mitchell, N., Groom, R., Pettorelli, N., Ipavec, A., Jacobson, A. P., et al. (2017). The global decline of cheetah Acinonyx jubatus and what it means for conservation. Proc. Natl. Acad. Sci. U.S.A. 114, 528–533. doi: 10.1073/pnas.1611122114
Eaton, R. L. (1970). Hunting behavior of the cheetah. J. Wildlife Manag. 34, 56–67. doi: 10.2307/3799492
Gehre, M., Renpenning, J., Gilevska, T., Qi, H., Coplen, T. B., Meijer, H. A., et al. (2015). On-line hydrogen-isotope measurements of organic samples using elemental chromium: an extension for high temperature elemental-analyzer techniques. Anal. Chem. 87, 5198–5205. doi: 10.1021/acs.analchem.5b00085
Hatch, K. A., Crawford, M. A., Kunz, A. W., Thomsen, S. R., Eggett, D. L., Nelson, S. T., et al. (2006). An objective means of diagnosing anorexia nervosa and bulimia nervosa using 15N/14N and 13C/12C ratios in hair. Rapid Commun. Mass Spectrom. 20, 3367–3373. doi: 10.1002/rcm.2740
Hijmans, R. J., and Van Etten, J. (2015). Raster: geographic data analysis and modeling. R package version 2.3–40.
Hobson, K., Van Wilgenburg, S., Wassenaar, L., Powell, R., Still, C., and Craine, J. (2012). A multi-isotope (δ13C, δ15N, δ2H) feather isoscape to assign afrotropical migrant birds to origins. Ecosphere 3, 1–20. doi: 10.1890/ES12-00018.1
Hobson, K. A., Alisauskas, R. T., and Clark, R. G. (1993). Stable-nitrogen isotope enrichment in avian tissues due to fasting and nutritional stress: Implications for isotopic analyses of diet. Condor 95, 388–394. doi: 10.2307/1369361
Hobson, K. A., Atwell, L., and Wassenaar, L. I. (1999). Influence of drinking water and diet on the stable-hydrogen isotope ratios of animal tissues. Proc. Natl. Acad. Sci. U.S.A. 96, 8003–8006. doi: 10.1073/pnas.96.14.8003
Hobson, K. A., and Koehler, G. (2015). On the use of stable oxygen isotope (δ18O) measurements for tracking avian movements in North America. Ecol. Evol. 5, 799–806. doi: 10.1002/ece3.1383
Hobson, K. A., and Wassenaar, L. I. (2008). Tracking Animal Migration With stable Isotopes. Academic Press. doi: 10.1016/S1936-7961(07)00006-1
Hobson, K. A., and Wassenaar, L. I. (2018). Tracking Animal Migration With Stable Isotopes. Academic Press.
Hobson, K. A., Wunder, M. B., Van Wilgenburg, S. L., Clark, R. G., and Wassenaar, L. I. (2009). A method for investigating population declines of migratory birds using stable isotopes: origins of harvested lesser scaup in North America. PLoS ONE 4, e7915. doi: 10.1371/journal.pone.0007915
Jenkins, S. G., Partridge, S. T., Stephenson, T. R., Farley, S. D., and Robbins, C. T. (2001). Nitrogen and carbon isotope fractionation between mothers, neonates, and nursing offspring. Oecologia 129, 336–341. doi: 10.1007/s004420100755
Koehler, G., and Hobson, K. A. (2019). Tracking cats revisited: placing terrestrial mammalian carnivores on δ2H and δ18O isoscapes. PLoS ONE 14, e0221876. doi: 10.1371/journal.pone.0221876
Koehler, G., Kardynal, K. J., and Hobson, K. A. (2019). Geographical assignment of polar bears using multi-element isoscapes. Sci. Rep. 9, 9390. doi: 10.1038/s41598-019-45874-w
Kornexl, B. E., Werner, T., Roßmann, A., and Schmidt, H.-L. (1997). Measurement of stable isotope abundances in milk and milk ingredients–a possible tool for origin assignment and quality control. Zeitschrift für Lebensmitteluntersuchung und-Forschung A 205, 19–24. doi: 10.1007/s002170050117
Levin, N. E., Zipser, E. J., and Cerling, T. E. (2009). Isotopic composition of waters from ethiopia and kenya: Insights into moisture sources for eastern africa. J. Geophys. Res. Atmospheres 114, D23. doi: 10.1029/2009JD012166
Magozzi, S., Vander Zanden, H., Wunder, M., and Bowen, G. (2019). Mechanistic model predicts tissue-environment relationships and trophic shifts in animal hydrogen and oxygen isotope ratios. Oecologia 191, 777. doi: 10.1007/s00442-019-04532-8
Marker, L. (2019). Loving a species to death. Biodiversity 20, 50–55. doi: 10.1080/14888386.2019.1591300
Marker, L. L., Muntifering, J., Dickman, A., Mills, M., and Macdonald, D. (2003). Quantifying prey preferences of free-ranging namibian cheetahs. South Afr. J. Wildlife Res. 33, 43–53.
Marker-Kraus, L. (1997). History of the Cheetah: Acinonyx jubatus in zoos 1829-1994. Int. Zoo Yearbook 35, 27–43. doi: 10.1111/j.1748-1090.1997.tb01186.x
McKechnie, A. E., Wolf, B. O., and Del Rio, C. M. (2004). Deuterium stable isotope ratios as tracers of water resource use: an experimental test with rock doves. Oecologia 140, 191–200. doi: 10.1007/s00442-004-1564-9
Meier-Augenstein, W., Hobson, K. A., and Wassenaar, L. I. (2013). Critique: measuring hydrogen stable isotope abundance of proteins to infer origins of wildlife, food and people. Bioanalysis 5, 751–767. doi: 10.4155/bio.13.36
Mekota, A.-M., Grupe, G., Ufer, S., and Cuntz, U. (2006). Serial analysis of stable nitrogen and carbon isotopes in hair: monitoring starvation and recovery phases of patients suffering from anorexia nervosa. Rapid Commun. Mass Spectrom. 20, 1604–1610. doi: 10.1002/rcm.2477
Melzer, E., and Schmidt, H. (1987). Carbon isotope effects on the pyruvate dehydrogenase reaction and their importance for relative carbon-13 depletion in lipids. J. Biol. Chem. 262, 8159–8164. doi: 10.1016/S0021-9258(18)47543-6
Mills, M. L. (1984). Prey selection and feeding habits of the large carnivores in the southern Kalahari. Koedoe 27, 281–294. doi: 10.4102/koedoe.v27i2.586
Montanari, S., and Amato, G. (2015). Discrimination factors of carbon and nitrogen stable isotopes from diet to hair and scat in captive tigers (Panthera tigris) and snow leopards (uncia uncia). Rapid Commun. Mass Spectrom. 29, 1062–1068. doi: 10.1002/rcm.7194
O'Brien, S. J., Roelke, M. E., Marker, L., Newman, A., Winkler, C., Meltzer, D., et al. (1985). Genetic basis for species vulnerability in the cheetah. Science 227, 1428–1434. doi: 10.1126/science.2983425
O'Brien, S. J., Wildt, D. E., and Bush, M. (1986). The cheetah in genetic peril. Sci. Am. 254, 84–95. doi: 10.1038/scientificamerican0586-84
Osthoff, G., Hugo, A., and De Wit, M. (2006). The composition of cheetah (Acinonyx jubatus) milk. Comparat. Biochem. Physiol. B Biochem. Mol. Biol. 145, 265–269. doi: 10.1016/j.cbpb.2006.05.016
Parng, E., Crumpacker, A., and Kurle, C. M. (2014). Variation in the stable carbon and nitrogen isotope discrimination factors from diet to fur in four felid species held on different diets. J. Mammal. 95, 151–159. doi: 10.1644/13-MAMM-A-014.1
Pietsch, S. J., Hobson, K. A., Wassenaar, L. I., and Tütken„, T. (2011). Tracking cats: problems with placing feline carnivores on δ18O, δD isoscapes. PLoS ONE 6, e24601. doi: 10.1371/journal.pone.0024601
Pires, S. F. (2012). The illegal parrot trade: a literature review. Global Crime 13, 176–190. doi: 10.1080/17440572.2012.700180
Pires, S. F., and Moreto, W. D. (2011). Preventing wildlife crimes: solutions that can overcome the ‘tragedy of the commons'. Eur. J. Crim. Policy Res. 17, 101–123. doi: 10.1007/s10610-011-9141-3
Polischuk, S. C., Hobson, K. A., and Ramsay, M. A. (2001). Use of stable-carbon and-nitrogen isotopes to assess weaning and fasting in female polar bears and their cubs. Can. J. Zool. 79, 499–511. doi: 10.1139/z01-007
Qi, H., Coplen, T. B., and Wassenaar, L. I. (2011). Improved online δ18O measurements of nitrogen-and sulfur-bearing organic materials and a proposed analytical protocol. Rapid Commun. Mass Spectrom. 25, 2049–2058. doi: 10.1002/rcm.5088
R Core Team (2021). R: A Language and Environment for Statistical Computing. Vienna: R Foundation for Statistical Computing.
Ripple, W. J., Estes, J. A., Beschta, R. L., Wilmers, C. C., Ritchie, E. G., Hebblewhite, M., et al. (2014). Status and ecological effects of the world's largest carnivores. Science 343, 1241484. doi: 10.1126/science.1241484
Sachse, D., Billault, I., Bowen, G. J., Chikaraishi, Y., Dawson, T. E., Feakins, S. J., et al. (2012). Molecular paleohydrology: interpreting the hydrogen-isotopic composition of lipid biomarkers from photosynthesizing organisms. Annu. Rev. Earth Planet. Sci. 40, 221–249. doi: 10.1146/annurev-earth-042711-105535
Schoeller, D. A., Leitch, C. A., and Brown, C. (1986). Doubly labeled water method: in vivo oxygen and hydrogen isotope fractionation. Am. J. Physiol. Regulat. Integr. Compar. Physiol. 251, R1137-R1143. doi: 10.1152/ajpregu.1986.251.6.R1137
Sessions, A. L., Burgoyne, T. W., Schimmelmann, A., and Hayes, J. M. (1999). Fractionation of hydrogen isotopes in lipid biosynthesis. Org. Geochem. 30, 1193–1200. doi: 10.1016/S0146-6380(99)00094-7
Soto, D. X., Koehler, G., Wassenaar, L. I., and Hobson, K. A. (2017). Re-evaluation of the hydrogen stable isotopic composition of keratin calibration standards for wildlife and forensic science applications. Rapid Commun. Mass Spectrom. 31, 1193–1203. doi: 10.1002/rcm.7893
Soto, D. X., Wassenaar, L. I., Hobson, K. A., and Catalan, J. (2011). Effects of size and diet on stable hydrogen isotope values (δD) in fish: implications for tracing origins of individuals and their food sources. Can. J. Fish. Aquat. Sci. 68, 2011–2019. doi: 10.1139/f2011-112
Spinage, C., Ryan, C., and Shedd, M. (1980). Food selection by the Grant's gazelle. Afr. J. Ecol. 18, 19–25. doi: 10.1111/j.1365-2028.1980.tb00267.x
Still, C. J., and Powell, R. L. (2010). “Continental-scale distributions of vegetation stable carbon isotope ratios,” in Isoscapes, eds J. West, G. Bowen, T. Dawson, and K. Tu (Dordrecht: Springer). doi: 10.1007/978-90-481-3354-3_9
Storm-Suke, A., Wassenaar, L. I., Nol, E., and Norris, D. R. (2012). The influence of metabolic rate on the contribution of stable-hydrogen and oxygen isotopes in drinking water to quail blood plasma and feathers. Funct. Ecol. 26, 1111–1119. doi: 10.1111/j.1365-2435.2012.02014.x
Terzer, S., Wassenaar, L., Araguás-Araguás, L., and Aggarwal, P. (2013). Global isoscapes for δ18O and δ2H in precipitation: improved prediction using regionalized climatic regression models. Hydrol. Earth Syst. Sci. 17, 4713. doi: 10.5194/hess-17-4713-2013
Terzer-Wassmuth, S., Wassenaar, L. I., Welker, J. M., and Araguás-Araguás, L. J. (2021). Improved high-resolution global and regionalized isoscapes of δ18o, δ2h and d-excess in precipitation. Hydrol. Process. 35, e14254. doi: 10.1002/hyp.14254
Tieszen, L. L., Boutton, T. W., Tesdahl, K. G., and Slade, N. A. (1983). Fractionation and turnover of stable carbon isotopes in animal tissues: implications for δ13C analysis of diet. Oecologia 57, 32–37. doi: 10.1007/BF00379558
Tieszen, L. L., Hein, D., Qvortrup, S., Troughton, J., and Imbamba, S. (1979a). Use of δ13C values to determine vegetation selectivity in East African herbivores. Oecologia 37, 351. doi: 10.1007/BF00347911
Tieszen, L. L., Senyimba, M. M., Imbamba, S. K., and Troughton, J. H. (1979b). The Distribution of C3 and C4 grasses and carbon isotope discrimination along an altitudinal and moisture gradient in Kenya. Oecologia 37, 337–350. doi: 10.1007/BF00347910
Topalov, K., Schimmelmann, A., Polly, P. D., Sauer, P. E., and Lowry, M. (2013). Environmental, trophic, and ecological factors influencing bone collagen δ2H. Geochim. Cosmochim. Acta 111, 88–104. doi: 10.1016/j.gca.2012.11.017
Tordiffe, A. S., Wachter, B., Heinrich, S. K., Reyers, F., and Mienie, L. J. (2016). Comparative serum fatty acid profiles of captive and free-ranging cheetahs (Acinonyx jubatus) in Namibia. PLoS ONE 11, e0167608. doi: 10.1371/journal.pone.0167608
Tricorache, P., Nowell, K., Wirth, G., Mitchell, N., Boast, L. K., and Marker, L. (2018). “Pets and pelts: Understanding and combating poaching and trafficking in cheetahs,” in Biodiversity of the World-Cheetahs: Biology and Conservation, 1st Edn (San Diego, CA: Elsevier), 191–205.
Van Wilgenburg, S. L., and Hobson, K. A. (2011). Combining stable-isotope (δD) and band recovery data to improve probabilistic assignment of migratory birds to origin. Ecol. Appl. 21, 1340–1351. doi: 10.1890/09-2047.1
Vander Zanden, H. B., Nelson, D. M., Wunder, M. B., Conkling, T. J., and Katzner, T. (2018). Application of isoscapes to determine geographic origin of terrestrial wildlife for conservation and management. Biol. Conserv. 228, 268–280. doi: 10.1016/j.biocon.2018.10.019
Vander Zanden, H. B., Soto, D. X., Bowen, G. J., and Hobson, K. A. (2016). Expanding the isotopic toolbox: applications of hydrogen and oxygen stable isotope ratios to food web studies. Front. Ecol. Evol. 4, 20. doi: 10.3389/fevo.2016.00020
Velho, N., Karanth, K. K., and Laurance, W. F. (2012). Hunting: a serious and understudied threat in India, a globally significant conservation region. Biol. Conserv. 148, 210–215. doi: 10.1016/j.biocon.2012.01.022
Voigt, C. C., Thalwitzer, S., Melzheimer, J., Blanc, A.-S., Jago, M., and Wachter, B. (2014). The conflict between cheetahs and humans on Namibian farmland elucidated by stable isotope diet analysis. PLoS ONE 9, e101917. doi: 10.1371/journal.pone.0101917
Warchol, G. L. (2004). The transnational illegal wildlife trade. Crim. Justice Stud. 17, 57–73. doi: 10.1080/08884310420001679334
Warchol, G. L., Zupan, L. L., and Clack, W. (2003). Transnational criminality: an analysis of the illegal wildlife market in Southern Africa. Int. Crim. Justice Rev. 13, 1–27. doi: 10.1177/105756770301300101
West, J. B., Bowen, G. J., Dawson, T. E., and Tu, K. P. (2009). Isoscapes: Understanding Movement, Pattern, and Process on Earth Through Isotope Mapping. Dordrecht: Springer. doi: 10.1007/978-90-481-3354-3
Wilson, G., Mackenzie, D., Brookes, I., and Lyon, G. (1988). Importance of body tissues as sources of nutrients for milk synthesis in the cow, using 13C as a marker. Br. J. Nutr. 60, 605–617. doi: 10.1079/BJN19880131
Wolf, C., and Ripple, W. J. (2017). Range contractions of the world's large carnivores. R. Soc. Open Sci. 4, 170052. doi: 10.1098/rsos.170052
Keywords: hydrogen, oxygen, stable isotope, conservation, cheetah, maternal nutrients, metabolic routing
Citation: Koehler G, Schmidt-Küntzel A, Marker L and Hobson KA (2023) Delineating origins of cheetah cubs in the illegal wildlife trade: Improvements based on the use of hair δ18O measurements. Front. Ecol. Evol. 11:1058985. doi: 10.3389/fevo.2023.1058985
Received: 30 September 2022; Accepted: 24 January 2023;
Published: 10 February 2023.
Edited by:
Gábor Árpád Czirják, Leibniz Institute for Zoo and Wildlife Research (LG), GermanyReviewed by:
Stefania Milano, Leibniz Institute for Zoo and Wildlife Research (LG), GermanyStephan Woodborne, TAMS Laboratory, South Africa
Copyright © 2023 Koehler, Schmidt-Küntzel, Marker and Hobson. This is an open-access article distributed under the terms of the Creative Commons Attribution License (CC BY). The use, distribution or reproduction in other forums is permitted, provided the original author(s) and the copyright owner(s) are credited and that the original publication in this journal is cited, in accordance with accepted academic practice. No use, distribution or reproduction is permitted which does not comply with these terms.
*Correspondence: Geoff Koehler, geoff.koehler@usask.ca