- 1College of Biology and Environmental Science, Jishou University, Jishou, Hunan Province, China
- 2Guangdong Key Laboratory of Animal Conservation and Resource Utilization, Guangdong Public Library of Wild Animal Conservation and Utilization, Institute of Zoology, Guangdong Academy of Sciences, Guangzhou, Guangdong Province, China
Introduction: Avian head lice comprise a diverse group of distantly related genera of lice that exhibit a strongly convergent morphology. Due to their lack of free-living stages, their strong morphological adaptations to living on the host’s head, and the limited opportunities for transfer between hosts during mating or nesting, the lateral transmission of head lice between non-conspecific hosts may be presumed to be restricted. Despite this, many species of head lice are ostensibly host generalists. We here examine lice of the head louse genus Philopteroides Mey, 2004, from bulbuls (Passeriformes: Pycnonotidae).
Methods: We use two different methods, ParaFit and Jane, to get insights on the co-evolutionary history of Philopteroides species and their bulbul hosts. Jane was run with a variation of event costs.
Results: Our phylogenetic analysis indicate that several morphologically cryptic species can be found in this group, most of which appear to be host specific. However, co-phylogenetic analyses indicate that host-switching has been common in the history of these lice, and co-speciation events have been rarer than expected. Moreover, lowest-cost co-evolutionary reconstructions under a variety of event costs are indistinguishable from random. An expanded dataset with more Philopterus-complex lice was found to be evenly balanced between host-switching and co-speciation events.
Discussion: The transfer of avian head lice between host species is poorly understood, but evidently fairly common. Several potential routes are discussed, but direct evidence is missing. Potentially, the presence of multiple bulbul species at fruiting trees may be an important factor in this transfer. However, such transfer routes also do not explain why Philopteroides lice on bulbuls appear to be distinct from those of other hosts. Moreover, as many of the species recovered in our analysis are morphologically indistinguishable, cryptic speciation appears to be common in this group.
Introduction
Ischnoceran lice (Phthiraptera) of the so-called “head louse ecomorph” have a wide distribution across most groups of birds (Price et al., 2003). Head lice do not form a monophyletic group; instead, they are often more closely related to lice of other ecomorphs (Johnson et al., 2012). Presumably, similar selection pressures operate on lice living on the host’s head regardless of host identity, resulting in similar gross morphology in distantly related louse groups. For instance, the main host defense against head lice is foot scratching (Clayton and Cotgreave, 1994), which flushes lice from the head to the body feathers (Goodman et al., 2020). Convergence in morphology among different head louse groups is likely at least in part to withstand foot scratching.
The typical head louse ecomorph morphology includes (see, e.g., Gustafsson et al., 2022a,b): a proportionately large, triangular head with extensive dorsal preantennal sutures (Figure 1); large, rounded abdomens that are not dorsoventrally flattened, with medianly interrupted tergopleurites and reduced or absent sternites; proportionately short legs. Presumably, the large head and extensive sutures aid in attachment to head feathers, whereas the large abdomen may be an effect of the correlation between body size and fecundity of lice (Villa et al., 2018) made possible by the limited efficiency of foot scratching. The short legs may have evolved in response to the smaller feathers on the head; head lice are generally not slower than other ischnoceran lice when climbing on head feathers of live birds (DRG, personal observations).
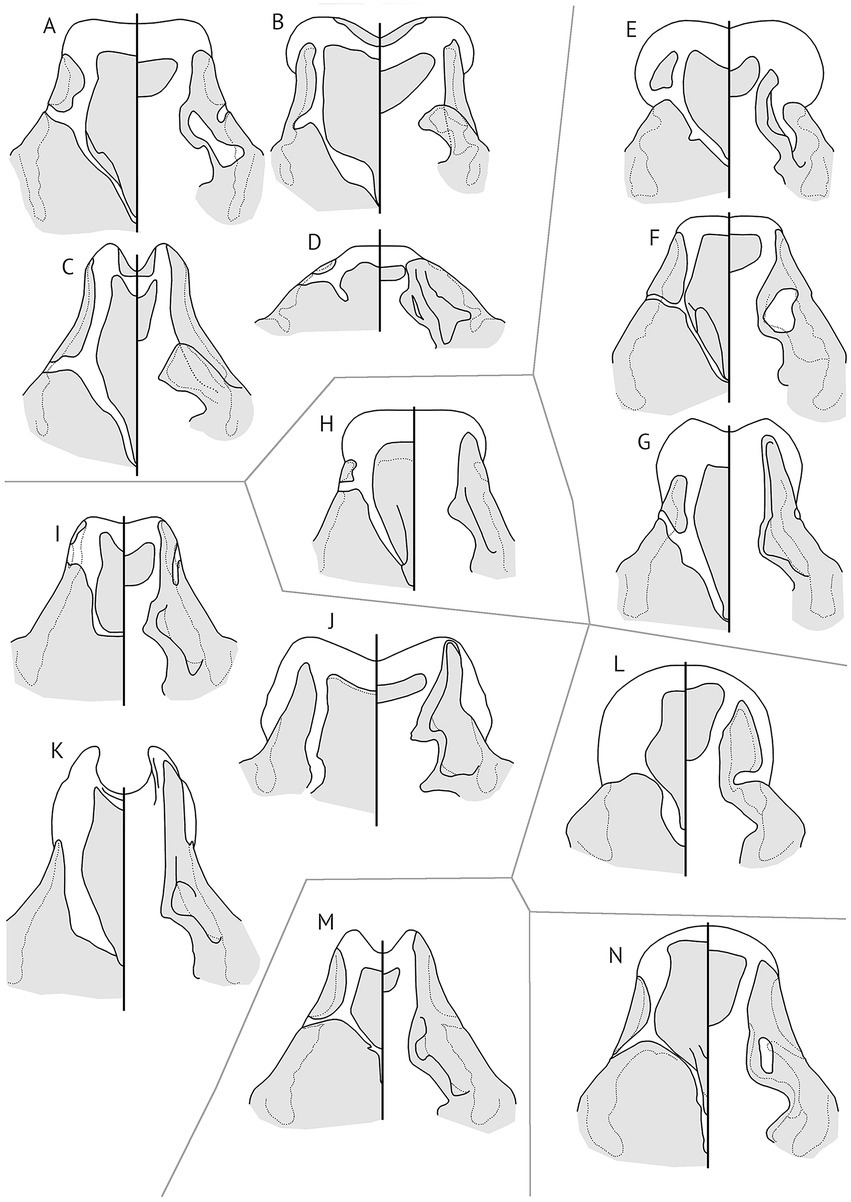
Figure 1. Comparison of the anterior ends of heads of lice of the head louse ecomorph from different radiations, showing the strong convergence on similar head structures in lice occupying the same niche on different groups of birds. For simplicity, setae, pulvinus, and some ornamental structures have been omitted. Gray lines separate lice from different complexes. Gray areas on each head represent sclerotized parts, whereas white areas represent hyaline regions. Philopterus-complex: (A) Philopterus garruli ex Garrulus glandarius; (B) Vinceopterus erythrocephali ex Harpactes erythrocephalus; (C) Philopteroides haerixos ex Ixos mcclellandii; (D) Corcorides biocellata ex Struthidea cinerea. Expanded Philopterus-complex: (E) Alcedoecus constrictus ex Halcyon pileata; (F) Strigiphilus cursor ex Asio flammeus; (G) Craspedorrhynchus platystomus ex Buteo japonicus. Mulcticola-complex: (H) Galliphilopterus sp. ex Arborophila gingica. Brueelia-complex: (I) Sturnidoecus bannoo ex Acridotheres cristatellus; (J) Meropoecus balisong ex Merops americanus; (K) Schizosairhynchus philippinus ex Basilornis miranda. Anaticola-complex: (L) Anatoecus dentatus ex Aix galericulata. Penenirmus-complex: (M) Picophilopterus blythipici ex Blythipicus pyrrhotis. Quadraceps-complex: (N) Saemundssonia lari ex Saundersilarus saundersi.
The morphological characters of head lice may be disadvantageous elsewhere on the host. Birds have developed a variety of active and passive defenses against parasites (Clayton et al., 2010; Bush and Clayton, 2018), but the main method of removing lice is preening, i.e., the manipulation of feathers with the beak (Clayton et al., 1999, 2005; Vezzoli et al., 2015). On most bird groups, lice occurring on parts of the body where the bird can preen are generally more slender than head lice, presumably to allow them to hide between feather barbs. This has resulted in a correlation between the size of lice and the size of their hosts, a phenomenon known as Harrison’s rule (Johnson et al., 2005); however, this correlation is not seen in all ecomorphs of lice (Johnson et al., 2005; Harnos et al., 2017). Experimental data have shown that transfers of lice between birds of different size may severely impact the fitness of lice (Bush and Clayton, 2006). Alternatively, lice occurring on the body of their hosts may be quick enough to escape preening (Johnson et al., 2005). Large-headed and large-bodied head lice may not be able to employ either of these escape behaviors successfully and would easily be preened off by the bird. Notably, detailed studies on the positioning of eggs and adult lice on their hosts suggest that head lice spend their whole life on the head (Baum, 1968; Mey, 1982; Cicchino, 2007).
If head lice are confined to the head of their host, this would indicate that their opportunities for transmission between hosts are limited. As lice have no free-living stages, the most common modes of transmission between hosts require either the hosts to come into physical contact or the presence of hippoboscid flies on which the lice can hitchhike (Keirans, 1975; Harbison et al., 2009; Bartlow et al., 2016; Lee et al., 2022). Relatively few records of head lice being phoretic on hippoboscids are known (Lee et al., 2022), therefore most transmission probably occurs through physical contact between conspecific hosts, e.g., during mating or nesting (Clayton and Tompkins, 1994; Lee and Clayton, 1995; Hillgarth, 1996; Brooke, 2010). Due to their limited dispersal capabilities, head lice present a unique opportunity to study Fahrenholz’s rule (Eichler, 1942, 1948).
Fahrenholz’s rule is the assertion that, given a lack of free-living stages and limited opportunities to spread between non-conspecific hosts, permanent parasites should be strictly host specific and co-speciate with their hosts. Over time, this should produce host and parasite phylogenies that are mirror images of each other. This proposed rule, often summarized as “parasite phylogeny mirrors host phylogeny” (e.g., Kim, 1988; Mey, 1999), has a long and complicated history (Von Kéler, 1959; Klassen, 1992; Gustafsson and Najer, 2022), and has received mixed support in co-phylogenetic analyses across a range of louse species (e.g., Sweet and Johnson, 2016, 2018; Sweet et al., 2018;Catanach et al., 2019; Johnson et al., 2021). Co-phylogenetic analyses between lice and their hosts most often show that strict co-speciation is eroded by host-switching of lice, including between distantly related hosts. However, most co-phylogenetic analyses of lice and their hosts to date have examined either wing lice or “generalists,” groups of lice that are known to be phoretic on hippoboscids (Lee et al., 2022). Co-phylogenetic analyses of head lice are rare, but in both the genera Penenirmus and Alcedoecus host-switching, including between distantly related hosts, appears to have played a major part in the evolution of host associations (Catanach et al., 2019; Johnson et al., 2021). Other phylogenetic analyses of head lice indicate that at least some species are host generalists (e.g., Grossi et al., 2014; Yamagishi et al., 2014; Bush et al., 2016; Najer et al., 2021; Kolencik et al., 2022).
Here, we examine head lice in the genus Philopteroides (Mey, 2004), parasitizing bulbuls (Pycnonotidae), a bird family comprising 151 species of medium-sized frugivores distributed across Africa and tropical and subtropical Asia. Similarly, Philopteroides is widely distributed throughout the Old World tropics, occurring on a wide range of host families (Valim and Palma, 2013; Gustafsson et al., 2022b: table 8). Recent genetic data indicate that the genus may not be monophyletic (Kolencik et al., 2022), with species parasitizing bulbuls forming a morphologically homogeneous and closely related group separate from the species occurring on members of other host families (Mey, 2004; Valim and Palma, 2013; Najer et al., 2016, 2021; Gustafsson et al., 2022a). The morphological homogeneity of these lice may suggest that host-switches occur regularly between different bulbul species.
Several factors of louse and host biology are expected to influence the co-phylogenetic history of Philopteroides and bulbuls. First, no species of Philopteroides are known to be phoretic on hippoboscids (Lee et al., 2022), suggesting that lateral transmission between host species may be limited. Moreover, Philopteroides are generally common on bulbuls in Southeast Asia (Najer et al., 2012a; Chu et al., 2019), suggesting that host-switching Philopteroides will only rarely encounter “empty niches” on bulbul hosts. Together, this would tend to increase the level of host specificity and degree of co-evolution between Philopteroides and their bulbul hosts. Only rarely is more than one head louse species known from the same host species (e.g., some thrushes; Price et al., 2003), and it is unknown whether the cases of multiple head louse species on the same host species are influenced by geography. The number of head lice found on any species of bird is usually small (<15), suggesting that the head feather habitat is limited, and may not support more than one louse species competing for the same resource. If empty niches are relatively rare, and co-occurrence of more than one head louse species on the same host is uncommon, lateral transmission between hosts should fail more often than not, and the phylogeny of Philopteroides should be similar to that of their hosts.
However, as bulbuls often form mixed flocks at, e.g., fruiting trees, this may increase the opportunities for lateral transfer between hosts. If such contact is sufficiently frequent, this may increase the chances for successful host switches, thus eroding the co-phylogenetic structure of the system. The rate of success of host switching may also increase due to the similarity in size of most bulbuls (e.g., Arlott, 2017), which would limit the influence of Harrison’s rule.
Materials and methods
Birds were caught and fumigated for lice in several localities across South China during 2012–2021 (Figure 2) using standard mist nets (net size: 2 m × 6 m; 2 m × 12 m) following the methods outlined by Gustafsson et al. (2019a). Hosts were identified using MacKinnon and Phillipps (2000) or Arlott (2017); host taxonomy has been updated to conform with Clements et al. (2021). Specimens of Philopteroides were obtained from seven of the 20 species of bulbuls occurring in China, representing 5 of the 7 genera of bulbuls in this country. Additional sequence data derived from Najer et al. (2021) was obtained from GenBank (Table 1), representing another seven host species from three genera not obtained from China. Lice were stored in a −80°C freezer at the Institute of Zoology, Guangdong Academy of Sciences (IZGAS), Guangdong, China, and identified tentatively to genus level through a dissection microscope. Specimens corresponding to the morphology of Philopteroides using the key of Gustafsson et al. (2019b) were selected for DNA extraction; identity of slide-mounted specimens were later confirmed using the same key.
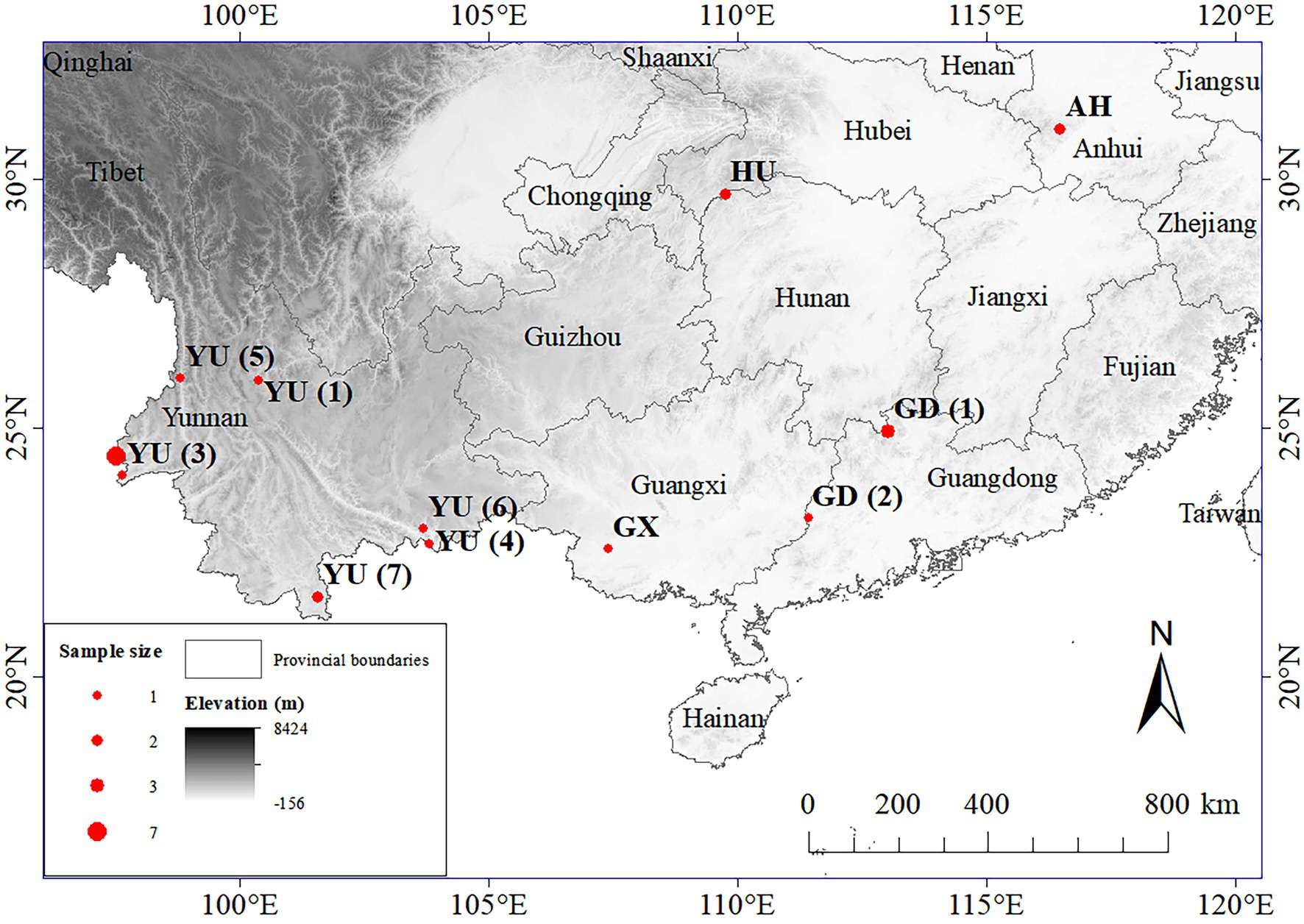
Figure 2. Collections localities in South China for new samples collected during this study. Size of circles correspond to number of specimens collected at that locality. Locality abbreviations used: AH = Yuexi, Anhui Province; GD (1) = Nanling National Nature Reserve, Guangdong Province; GD (2) = Tongle Provincial Nature Reserve, Guangdong Province; GX = Changzuo, Guangxi Province; HU = Badagongshan National Nature Reserve, Hunan Province; YU (1) = Jizu Mountain of Dali, Yunnan Province; YU (2) = Hongbeng River, Yingjiang, Yunnan Province; YU (3) = Ruili, Yunnan Province; YU (4) = Hekou, Yunnan Province; YU (5) = Gaoligong Mountains National Nature Reserve, Yunnan Province; YU (6) = Daweishan Nature Reserve, Yunnan Province; YU (7) = primary forests near Banna, Yunnan Province.
At least 1 louse specimen (typically 1 male and 1 female, when available) identified as Philopteroides from each host species was selected for DNA extraction. Selected lice (Table 1) were cut halfway through the pterothorax and extracted for DNA using the DNeasy Blood and Tissue Kit (Qiagen, Shanghai, China) following the manufacturer’s instructions except that extractions were left in 55°C water baths for 24 h, and only 50 μℓ were used for each elution. Exoskeletons were retrieved from the extraction fluid and slide mounted in Canada balsam as vouchers, following Palma (1978). Vouchers are deposited in the collection at IZGAS.
For all specimens, we attempted to amplify three mitochondrial markers (COI, 12S, 16S) and three nuclear markers (EF-1α, hyp, TMEDE6), following the protocols used by Gustafsson and Olsson (2012), Sweet et al. (2014), and Tian et al. (2022). PCRs were performed using Cytiva PureTaq Ready-To-Go beads (GE Healthcare, Vienna, Austria), following the manufacturer’s instructions. Only COI, TMEDE6 and hyp amplified consistently, and analysis proceeded based only on these genes. Samples showing satisfactory bands were sent for sequencing using the same primers as for PCR to Tianyi Huiyuan Gene Technology, Co. Ltd. (Guangzhou, China). Sequences were assembled in Seqman Pro 7.1.0 (DNAStar Inc., Madison, Wisconsin) and checked manually to rule out mismatches between forward and reverse sequencing results for each gene and each individual.
Two separate datasets were constructed. A smaller dataset (“Bulbul-only” dataset) was restricted to only Philopteroides specimens parasitizing bulbuls derived from our own samples and Najer et al. (2021). To examine whether patterns obtained for this group were consistent across a larger diversity of head lice, a second dataset (“Expanded” dataset) was constructed using a range of different Philopterus-complex species derived from Najer et al. (2021). The Expanded dataset was rooted by including specimens of an undescribed species of Sturnidoecus Eichler, 1944, from Geokichla citrina (Passeriformes: Turdidae), whereas the Bulbul-only dataset was rooted on specimens from the Expanded dataset.
Sequences were aligned separately in MEGA 11 using ClustalW and MUSCLE (Edgar, 2004; Larkin et al., 2007; Kumar et al., 2018). Substitution models for each gene were evaluated in MEGA 11; the best model for COI was TN93 + G + I, for hyp was HKY + I, and for TMEDE6 was TN93 + G. The 3 aligned and partitioned genes were imported into and concatenated by BEAST v1.10.4 (Suchard et al., 2018), with the default strict clock prior and a Yule speciation process prior, using random starting trees, with the options of linked trees, separated clock models for each gene, 4 Gamma Categories under the strict clock, and constant size of coalescence. Markov chain Monte Carlo (MCMC) tests were run for 1 × 108 generations and sampled every 1,000 generations. We used Tree Annotator v1.10.4 (Suchard et al., 2018) for tree integration and discarded the first 10,000,000 trees as “burnin.” The output tree from Tree Annotator was imported to FigTree v1.4.31 for figure illustration and edited in GIMP (GIMP Development Team, 2019). Louse species limits were tested using online GMYC2 (Fujisawa and Barrowclough, 2013) and bPTP3 (Zhang et al., 2013) analyses.
For co-phylogenetic analyses, Bayesian phylogenetic trees of the hosts were constructed online from www.birdtree.org, using the “Ericson All Species: A set of 10,000 trees with 9,993 OTUs each” as the tree source to construct 10,000 trees. TreeAnnotator V1.10.4 was used to discard the first 10% (1,000 trees), and host trees were checked in FigTree v1.4.3. A TXT matrix of the associations of the parasites and hosts were constructed for use in R,4 through the (phytools) cophylo function (Revell, 2011).
Cophylogenetic analysis was performed using the ParaFit method in ape (Legendre et al., 2002) in R using the consensus trees of the respective post-burnin tree distributions for the lice and hosts outlined above. Patristic distance matrices were constructed from the host and parasite trees for each dataset separately using the cophenetic (stats) function in ape. ParaFit was then run for 99,999 generations, using the “lingoes” correction, to test for the contributions of individual host–parasite links with ParaFitLink1 and ParaFitLink2 tests.
We also ran Jane v. 4 (Conow et al., 2010) for each of the two datasets separately, to find optimal solutions of evolutionary events. Default costs were used for different evolutionary events (cospeciation 0; Duplication 1; Duplication and Host-switching 2; Loss 1; Failure to Diverge 1). Event analysis was performed after setting the genetic algorithm (GA) parameters to 100 generations and a population size of 500 in the Stats Mode, and the same genetic algorithm was set in the Solve Mode to test the statistical significance of the best score for our data. This was compared to a set of randomized tip associations run 999 times to test for the statistical significance of our optimal score. For the Bulbul-only dataset, the Jane analyses were repeated with a variety of costs for Host Switching and Cospeciation; for each of these events, we varied the costs between 0 and 5, in increments of 1.
Results
Sampling
A total of 205 bulbuls were examined for lice, but each separate field trip normally resulted in only 1–3 bulbuls being caught; for this reason, prevalence data of Philopteroides, which is usually low (overall prevalence for all bulbul species examined 14.15%), cannot be calculated in a meaningful way.
Specimen identity
All Philopteroides specimens from bulbuls were keyed to species using the key of Gustafsson et al. (2022a), except specimens derived from Najer et al. (2021). The identity of Philopteroides kayanobori (Uchida, 1948) could not be confirmed, as the original description of this species (Uchida, 1948) includes characters that are not found in any species of Philopteroides (see Gustafsson et al., 2022a). As specimens from Hemixos flavala, Hemixos castanonotus and the two species of Alophoixus are morphologically very similar, they could not be separated using this key, indicating that these may represent cryptic species. The specimens from Hypsipetes leucocephalus were identified as a morphologically distinct species.
Phylogenetic reconstruction
Each of our datasets resulted in a single tree (Figures 3, 4). In the expanded dataset (Figure 3), five main clades were recovered inside the Philopterus-complex. Philopteroides was divided into two major clades, one comprising only specimens from bulbuls (PP = 1.00), corresponding to the Philopteroides group of Najer et al. (2021), and one comprising specimens from all other hosts (PP = 0.95), corresponding to the “Philopterus complex group 3” of Najer et al. (2021). However, neither of these two clades received high support, nor was the relationship between these two clades and Tyranniphilopterus clarified by our analysis.
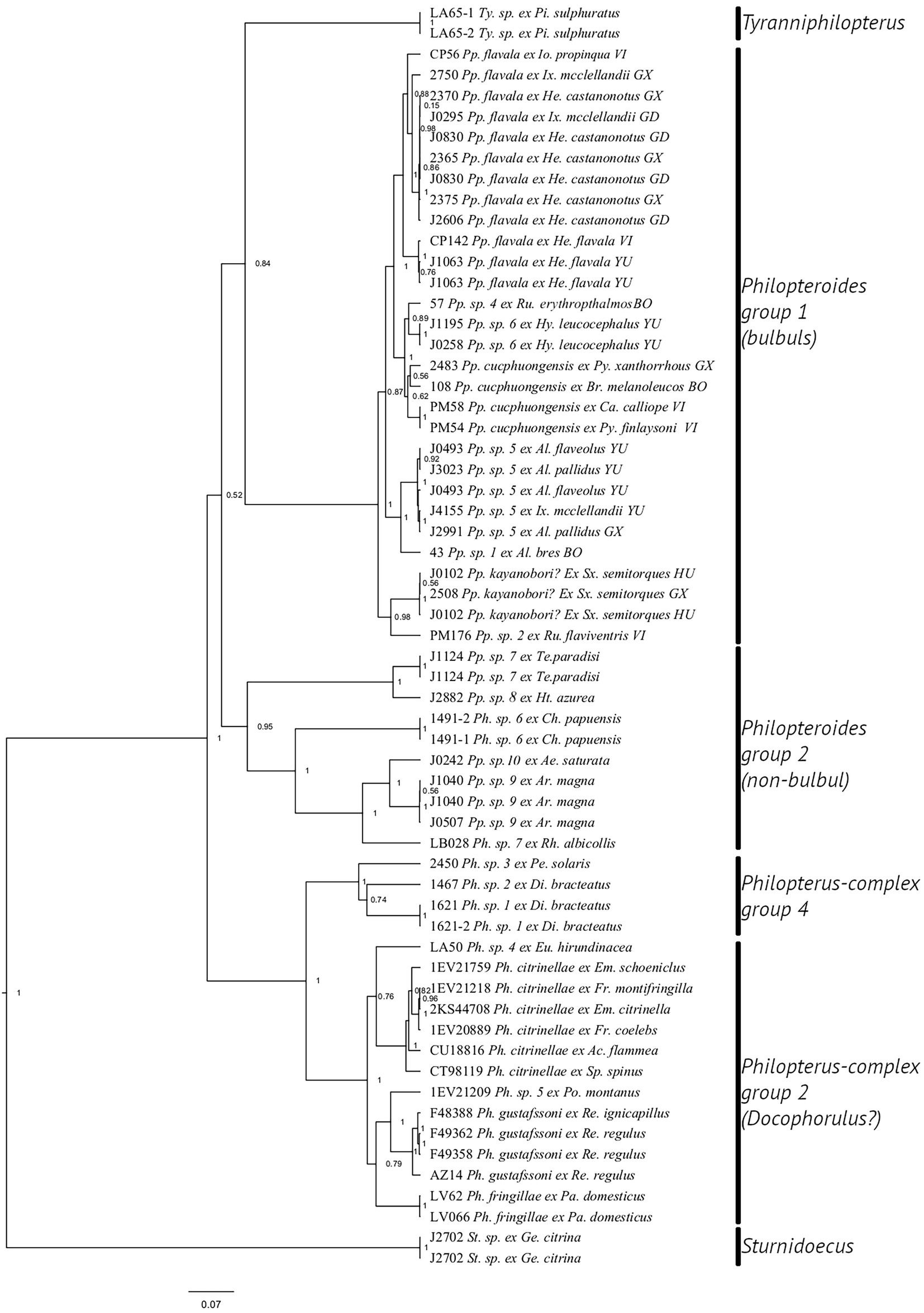
Figure 3. Phylogeny of the Philopterus-complex based on the mitochondrial COI and nuclear TMEDE6 and hyp sequences and generated in BEAST v1.10.4. Abbreviations of louse genera: Ph., Philopterus; Pp., Philopteroides; St., Sturnidoecus; Ty., Tyranniphilopterus. Abbreviations of host genera: Ac., Acanthis; Al., Alophoixus; Ca., Calliope; Ch., Chaetorhynchus; Di., Dicrurus; Em., Emberiza; Eu., Euphonia; Fr., Fringilla; Ge., Geokichla. He., Hemixos; Ht., Hypothymis; Hy., Hypsipetes; Io., Iole; Ix., Ixos; Pa., Passer; Pe., Pericrocotus; Pi., Pitangus; Po., Poecile; Ps., Psarisomus; Py., Pycnonotus; Re., Regulus; Rh., Rhipidura; Sp., Spinus; Sx., Spizixos. Abbreviations for localities are given only for bulbuls: BO, Borneo, Indonesia; GD, Guangdong, China; GX, Guangxi, China; GZ, Guizhou, China; HU, Hunan, China; VI, Vietnam; YU, Yunnan, China. Numbers before louse names correspond to voucher numbers in Table 1.
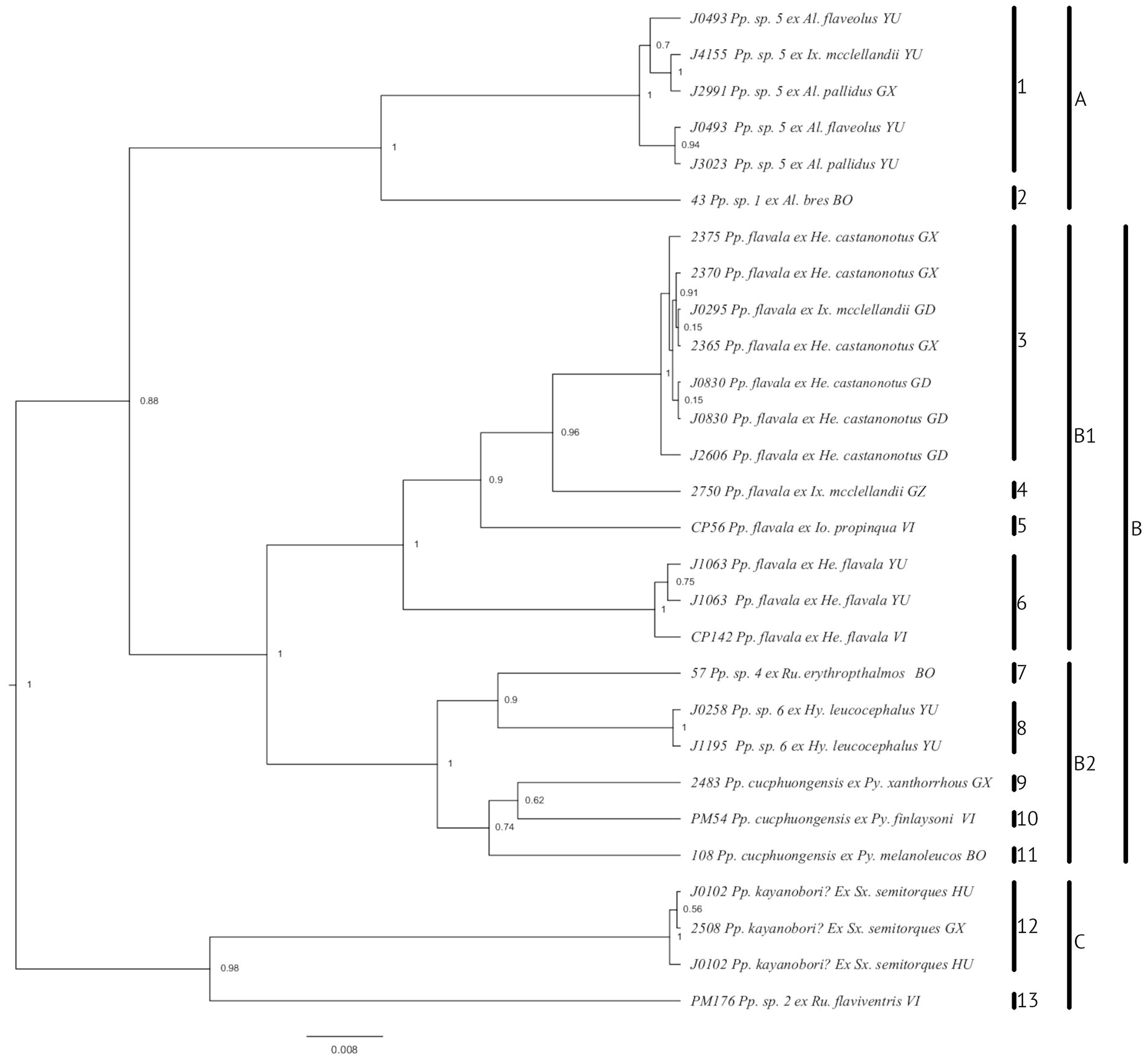
Figure 4. Phylogeny of Philopteroides (Mey, 2004), parasitic on bulbuls (Pycnonotidae) based on the mitochondrial COI and nuclear TMEDE6 and hyp sequences and generated in BEAST v1.10.4. Vertical bars to the right correspond to clades discussed in the text. Species-level identifications follow the key of Gustafsson et al. (2022a); note that Philopterus kayanobori cannot be adequately identified, as original description show characters that are not found in any Philopteroides, and specimens from Sx. semitorques are identified tentatively. Abbreviations of genus names: Al., Alophoixus; He., Hemixos; Hy., Hypsipetes; Io., Iole; Ix., Ixos; Pp, Philopteroides; Py., Pycnonotus; Sx., Spizixos. Abbreviations for localities: BO, Borneo, Indonesia; GD, Guangdong, China; GX, Guangxi, China; GZ, Guizhou, China; HU, Hunan, China; VI, Vietnam; YU, Yunnan, China. Numbers before louse names correspond to voucher numbers in Table 1.
A total of 13 species-level clades were identified in the Bulbul-only dataset (numbered 1–13 in Figure 4) by both the GMYC and the bPTP algorithm, with high posterior probabilities in the latter analysis for all non-monotypic clades except species 1 and 6 in Figure 4. Eight of these species-level clades were monotypic, and of the remaining 5 clades, 3 comprised only specimens derived from the same host species; all non-monotypic species-level clades received good support (PP = 1.00). With the exception of lice from Ixos mcclellandii which were divided into three clades, all lice from the same host grouped together in the same clade. In one case (clade A in Figure 4), specimens from two different species of Alophoixus were mixed together with a single specimen from Ix. mcclellandii. These 13 species were divided into three main groups, all with good support (clades A–C in Figure 4; PP = 0.98–1.00).
Cophylogenetic analyses
Our ParaFit analyses showed strong congruence between the host and louse trees for both datasets (ParaFitGlobal 179828.70, value of p-value 0.000001 for Expanded dataset; ParaFitGlobal 170.82, p-value 0.00548 for the Bulbul-only dataset). Independence of the host and parasite trees can thus be rejected for both datasets.
In contrast, the Jane analyses yielded different results for the two datasets (Table 2). Each dataset resulted in >15,000 solutions. In the Expanded dataset, these could be compressed to two isomorphic solutions of equal cost. The observed cost (44) in the Expanded dataset was well below that of random tip associations (60–70; p < 0.01). Each of the two compressed solutions had equal numbers of inferred Cospeciations and Host Switches (18), with smaller numbers of Duplications (2) and Losses (6).
The Bulbul-only dataset compressed into a single set of isomorphic solutions, in which the cost of the optimal solution (21) fell within the range of random associations (18–25) and was thus not significant (p > 0.05). This solution had equal numbers of Cospeciations and Host Switches (7), but also many Losses (6). Changing statistical or algorithmic parameters or event costs (Host Switching 0–5, Cospeciation 0–5, increments of 1) did not significantly change the overall result, and the observed cost was always within the range of random associations (data not shown). Only when Cospeciation costs were set to 0 and Host Switching costs set to 4 was the observed cost (32) lower than the cost distribution of random tip associations (33–47), but this result was not significant; increasing the cost of Host Switching to 5 placed the observed cost within the range of random costs again. The co-speciation events inferred by our Jane analysis were scattered rather evenly throughout the phylogeny (Figure 5).
As one host species (Ixos mcclellandii) was parasitized by three different lineages of lice, two of which were shared with lice from other hosts, we reran the Jane analysis with standard settings, removing the samples J0295 and J4155, to test whether these two potential contaminations or stragglers (specimens incidentally associated with a host at the time of collection, but not representative of an established population) disrupted co-phylogenetic patterns. This analysis resulted in 17,134 solutions, which could be compressed into six isomorphic solutions (B1–B6 in Table 2). These varied in their inferred number of co-evolutionary events, but in most solutions the numbers of Host Switches (8–9) were inferred to be greater than the number of Cospeciations (4–5). In all these solutions, the observed cost fell within the range of costs for random associations, and were thus not significant.
Discussion
The transfer of head lice between hosts is poorly understood. Their position on the head is far away from the main points of contact between different birds during mating and nesting, and their gross morphology suggest that they would be easily preened off when leaving the head. These disadvantages for transferring between hosts are presumably even greater when hosts of different species are concerned, as direct contact between the heads of two different birds may be limited. As such, head lice should be prime candidates for adherence to Fahrenholz’s rule: head lice would be expected to be host specific and to have co-speciated with their hosts.
The ParaFit analysis of the Expanded dataset, including a variety of Philopterus-complex genera, indicated that host and louse trees were overall congruent. The Jane reconciliation of this dataset also indicated that the two trees are consistent with each other (Table 3). The number of inferred Cospeciation events in this dataset was the same as the number of inferred Duplications and Host-switches (Table 2). In contrast, while the ParaFit analysis of the Bulbul-only dataset showed that the trees were significantly congruent, the Jane Analysis did not result in significant results for any combination of costs. Under standard cost settings, the Jane analysis of the Bulbul-only dataset resulted in a solution that included as many Cospeciation as Host Switching events, and almost as many Losses (Table 2). Removing potential stragglers of louse species 1 and 3 from Ixos mcclellandii did not alter the results (Table 2).
In general, congruence between the two organism groups is thus high regardless of dataset, and in both datasets host-switching is inferred to have been as common as cospeciation. Collectively, the Philopterus-complex and its close relatives (e.g., Alcedoecus, Craspedorrhynchus, Cuculoecus, Strigiphilus) parasitize a range of distantly related host groups (Supplementary Table 1), suggesting that host-switching has been prevalent throughout their evolutionary history (De Moya et al., 2019). This expanded Philopterus-complex notably comprises only head lice, whereas most other head louse groups (e.g., Saemundssonia, Anatoecus, Echinophilopterus) belong to radiations that include a variety of louse ecomorphs (Johnson et al., 2012). More data from different genera within the Philopterus-complex and its close relatives are needed to establish whether deeper nodes in this group are dominated by cospeciation or host-switching.
The Jane analysis could not separate the observed host-louse associations from random, but even accepting the inferred events at face value, there is no strong signal of louse-host cospeciation among the Philopteroides on bulbuls. The bulbul-Philopteroides dataset results are thus in line with previous data from head louse studies (Table 3). Several species of head lice occur on multiple closely related host species (Weckstein, 2004; Grossi et al., 2014; Yamagishi et al., 2014), and formal co-phylogenetic analyses typically result in at best partial congruence between host and louse trees (e.g., Johnson et al., 2021). Moreover, head lice are as common on first-year cuckoos, a known brood-parasite in which parents never come into contact with their chicks in the nest, as on older cuckoos (Brooke and Nakamura, 1998), indicating that transfer of lice between older and younger birds may happen outside the mating season.
Transfer of head lice
In our two datasets, there are either as many inferred host-switches as cospeciation events (Expanded dataset; Figure 6), or the host-louse association patterns are not significantly different from random (Bulbul-only dataset; Figure 5), indicating no strong signal of co-speciation. One interpretation of this data is that successful host-switches have been common within the Philopteroides-bulbul group, as perhaps indicated by the presence of three different louse species on Ixos mcclellandii (species 1, 3 and 4; Figure 4) and the apparent lack of species limits between lice on Alophoixus flaveolus and A. pallidus (species 1; Figure 4). As all bulbuls belong to the same family, and many of our bulbul hosts are congeneric, this may indicate that successful host-switches are more likely between closely related hosts, and that cophylogenetic analyses within a head louse genus will typically result in mixtures of host-switching and cospeciation. Over time, host-switches between distantly related hosts would be expected to be less and less frequent, perhaps even impossible, resulting in more congruence between trees that take longer time spans into account. However, even in the larger dataset, there are cases of distantly related hosts being parasitized by closely related lice, for instance the Philopteroides from Arachnothera magna and Aethopyga saturata (Passeriformes: Nectariniidae) being closely related to lice from hosts in the Corvides radiation (Philopteroides group 2; Figure 3). Host-switching thus appears to be an ongoing process within head louse evolution, both between distantly and closely related hosts.
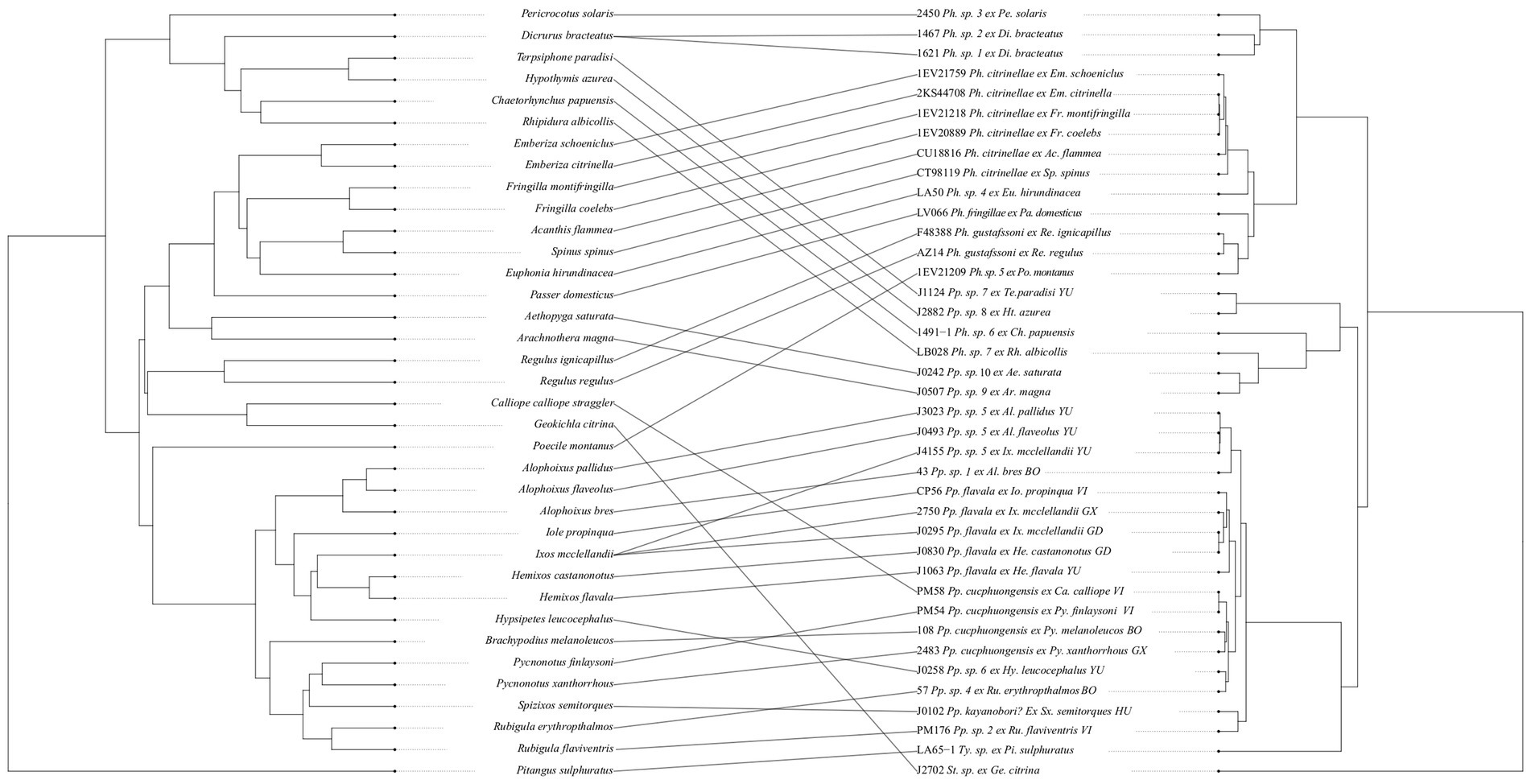
Figure 5. Tanglegram showing the relationships between bulbul hosts (left) and Philopteroides lice (right). Host phylogeny was obtained from www.birdtree.org and louse phylogeny generated in BEAST v1.10.4 from mitochondrial COI and nuclear TMEDE6 and hyp sequences. Both louse and host trees are cladograms of the trees resulting from the BEAST analysis or the online consensus tree, respectively. Black circles denote co-speciation events inferred by the Jane v.4 analysis, with numbers on each tree corresponding to the same event. Note that the solution containing these inferred co-speciation events was found not to be different from random in our analyses, and they are included here for completeness. Abbreviation of louse genus: Pp., Philopteroides. Abbreviations of host genera: Al., Alophoixus; Ca., Calliope; He., Hemixos; Hy., Hypsipetes; Io., Iole; Ix., Ixos; Py., Pycnonotus; Sx., Spizixos. Abbreviations for localities: BO, Borneo, Indonesia; GD, Guangdong, China; GX, Guangxi, China; GZ, Guizhou, China; HU, Hunan, China; VI, Vietnam; YU, Yunnan, China. Numbers before louse names correspond to voucher numbers in Table 1.
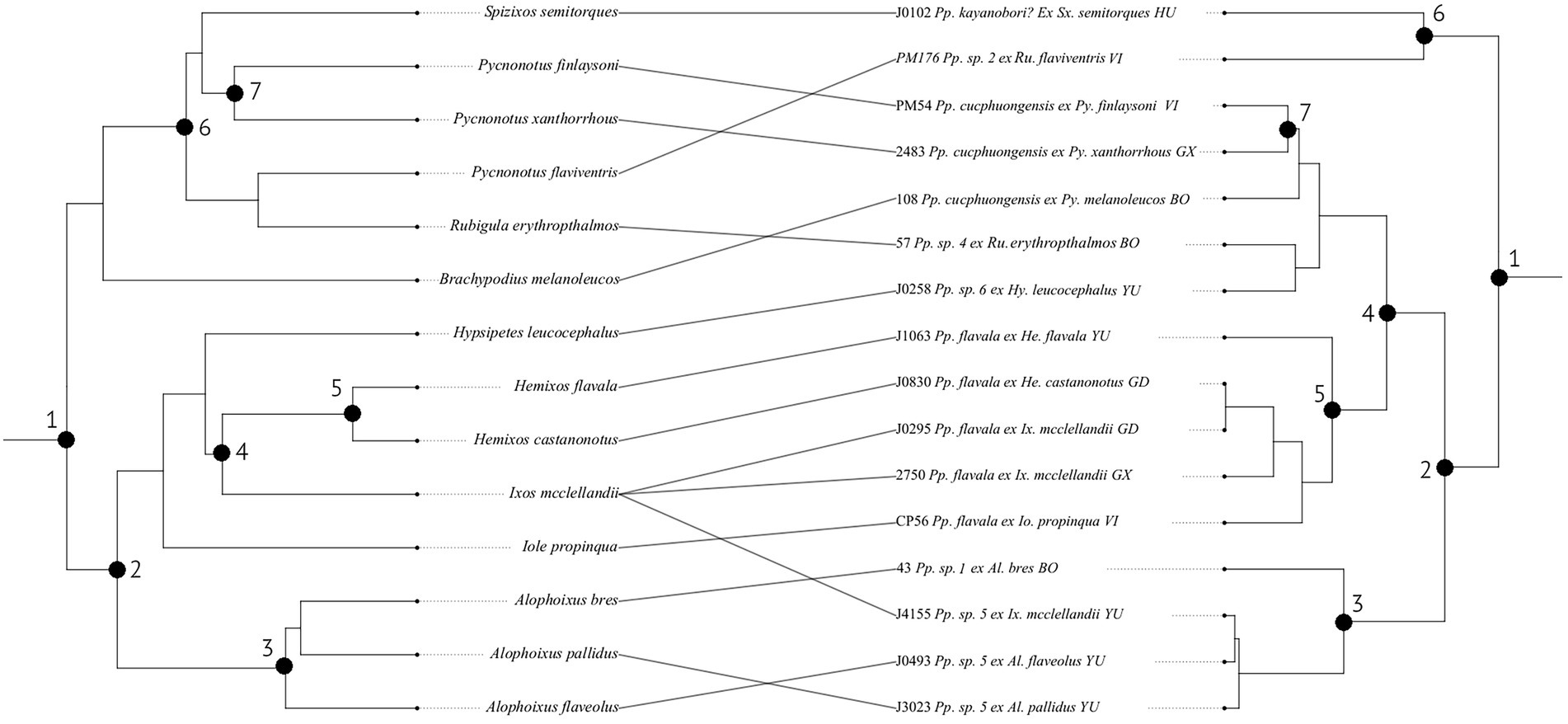
Figure 6. Tanglegram showing the relationships between the hosts (left) and lice (right) used in this study. Host phylogeny was obtained from www.birdtree.org and louse phylogeny generated in BEAST v1.10.4 from mitochondrial COI and nuclear TMEDE6 and hyp sequences. Both louse and host trees are cladograms of the trees resulting from the BEAST analysis or the online consensus tree, respectively. Inferred co-speciation events in Jane v. 4 not shown. Abbreviations of louse genera: Ph., Philopterus; Pp., Philopteroides; St., Sturnidoecus; Ty., Tyranniphilopterus. Abbreviations of host genera: Ac., Acanthis; Al., Alophoixus; Ca., Calliope; Ch., Chaetorhynchus; Di., Dicrurus; Em., Emberiza; Eu., Euphonia; Fr., Fringilla; Ge., Geokichla. He., Hemixos; Ht., Hypothymis; Hy., Hypsipetes; Io., Iole; Ix., Ixos; Pa., Passer; Pe., Pericrocotus; Pi., Pitangus; Po., Poecile; Ps., Psarisomus; Py., Pycnonotus; Re., Regulus; Rh., Rhipidura; Sp., Spinus; Sx., Spizixos. Abbreviations for localities are given only for bulbuls: BO, Borneo, Indonesia; GD, Guangdong, China; GX, Guangxi, China; GZ, Guizhou, China; HU, Hunan, China; VI, Vietnam; YU, Yunnan, China. Numbers before louse names correspond to voucher numbers in Table 1.
This raises the question: how do head lice on different host groups overcome the apparent limitations to spread from one host to another, especially when the hosts are of different species? Not only do lice lack free-living stages, but head lice are uniquely disadvantaged by having a gross morphology that is adapted to the head of the bird, and are limited in their habitat to areas of the birds that do not easily get into contact with birds of other species. Presumably, transfer of head lice between host species depends primarily on two main groups of routes: (1) routes that involve direct physical contact between the head of one host species and any feathered part of the body of another, and: (2) routes that do not involve physical contact between two hosts.
The first group of transmission routes involve a diverse array of host interactions. Interspecific allopreening has been observed for many different bird species (e.g., Selander et al., 1961; Harrison, 1965; Verbeek et al., 1981; Mo, 2016) and could conceivably allow head lice to transfer between species. In some species pairs, interspecific allopreening may be relatively common (e.g., caracaras and New World vultures; Ng and Jasperson, 1984; Lopes Palmeira, 2008; Souto et al., 2009), although in this case the two host groups do not share any head louse genera (Price et al., 2003), and the New World vultures have nude heads and thus cannot support head louse populations. Interspecific aggression or defense that includes pecking, biting or other attacks with the head may be a possible route. This may include cases of nest site defense (e.g., Cordero and Senar, 1990), defense against nest predation (e.g., Ehrlich and McLaughlin, 1988), or aggression at feeding sites (e.g., Minock, 1972). Host kleptoparasitism may also be a possible route (Hopkins, 1942). Finally, interspecific feeding may be a possible route to transmission of head lice (Muszalski Shy, 1982; Jiang et al., 2016; Harmackova, 2021).
The second group of possible transmission routes may be more limited, as head lice or their eggs need to remain on feathers left behind by birds. Transmission through sequentially shared nests has repeatedly been suggested as a possible transmission route (Harrison, 1915; Eveleigh and Threlfall, 1976; Clayton, 1990; Johnson et al., 2002; Weckstein, 2004). However, as the lifespan of lice and eggs are short (Price et al., 2003), this relies on short time frames between one bird moving out and the next moving in. Nest usurpation, in which one bird moves into an active nest and evicts the previous birds, is relatively common (Lindell, 1996), and may be more important than sequential nest use. Notably, both sequential nest use and nest usurpation has been observed in a range of different birds, often including interspecific interactions, and may result in mixed broods (e.g., Pulliainen and Saari, 1991; Samplonius and Both, 2013; Kubelka et al., 2014; Chedad et al., 2022). Theft of nest material may be another route of transmission falling into this category (Jones et al., 2007; Thompson et al., 2017), especially in cases where nest theft results in defense behavior (Tortosa and Redondo, 1992), but this would likely be limited to cases where feathers are stolen.
Presumably, different mechanisms are more important to different groups of head lice. Moreover, the importance of phoresy on hippoboscid flies for transmission of head lice requires further study. Of the 135 records of phoretic lice that were identified to at least genus level listed by Lee et al. (2022), 25 records (18.5%) refer to head lice. However, 18 of those 25 records (72%) refer to a single genus of lice, Sturnidoecus, and another 6 records (24%) refer to lice in the genus Philopterus s. lat. The only remaining record belongs to the genus Strigiphilus on an owl. No records of phoretic lice in speciose head louse genera such as Saemundssonia, Ibidoecus, Penenirmus, or Anatoecus have been published, although hippoboscid flies are known from the hosts of at least some of these head louse genera (Maa, 1969). Possibly, phoretic head lice have been overlooked.
In the specific case of Philopteroides from bulbuls, no records of phoretic lice are known, and host behavioral data of bulbuls that could be used to ascertain whether the other mechanisms discussed above are valid transmission routes are limited. Bulbuls are frequent members of mixed-species flocks (e.g., McClure, 1967; Partridge and Ashcroft, 1976; Hino, 2002; Kotagama and Goodale, 2004; Zou et al., 2011; Srinivasan et al., 2012; Sridhar and Shanker, 2014; Chandran and Vishnudas, 2018), and intraspecific kleptoparasitism has been observed in some bulbuls (Partridge and Ashcroft, 1976). Significantly, heterospecific bulbul assemblages may feed at the same fig trees, and interspecific aggression at these feeding sites have been reported repeatedly (e.g., Sanitjan and Chen, 2009; Wydhayagarn et al., 2009; Sreekar et al., 2010; Kamtaeja et al., 2012; Shermila and Wikramsinghe, 2013; Balakrishnan, 2014). In at least one case, mid-air fights between feeding bulbuls have been reported, in which birds grasped each other and fell to the ground (Sreekar et al., 2010). Bulbuls have also been reported to fight with or be attacked by other birds (e.g., Clunie, 1976; Blanvillain et al., 2003), and at least one case of nest material theft from a bulbul nest has been published (Mahesh et al., 2010). However, the potential of these interactions as transmission routes of Philopteroides lice between bulbul hosts is unknown and would need to be tested by targeted collection from bulbuls in mixed flocks.
Nevertheless, such transmission evidently occurs. Many species of Philopteroides from bulbuls included in our dataset occur on more than one host species, and lice from Ixos mcclellandii were divided into more than one species (Figure 4). The morphospecies Philopteroides cucphuongensis occurs on three different bulbul species from Borneo (Brachypodius melanoleucos), Vietnam (Pycnonotus finlaysoni), and South China (Pycnonotus xanthorrhous). This diversity of hosts for many species of bulbul Philopteroides was previously suggested by, e.g., Gustafsson et al. (2022a) on a morphological basis, although that study underestimated the diversity of Philopteroides lice on bulbuls somewhat. Our genetic data suggests that several undescribed species of Philopteroides may occur on bulbuls, including a species largely confined to hosts in the genus Alophoixus, specimens of which were considered conspecific with Philopteroides flavala by Gustafsson et al. (2022a). More detailed examinations of the morphology of these specimens are needed before they can be described formally.
Relationships within Philopteroides
Our analysis confirmed the results of Najer et al. (2021), which indicated that the Philopteroides parasitizing bulbuls form a closely related group of species. Kolencik et al. (2022) also recovered a clade of African Philopteroides from bulbuls. However, differences in gene selection between our study and that of Kolencik et al. (2022) prevented us from testing whether these two clades are closely related. Most African bulbuls form a separate radiation from the Asian species, but the Asian bulbul species have colonized Africa several times (Shakya and Sheldon, 2017).
Notably, both Najer et al. (2021) and Kolencik et al. (2022) found a second group of Philopteroides. Najer et al. (2021) called this group “Philopterus complex group 3,” whereas Kolencik et al. (2022) called this group “mitsusui species-group.” We also recovered a second group of Philopteroides in our analysis. This group includes specimens from monarch flycatchers, sunbirds, and some other hosts (Figure 3): “Philopteroides group 2 (non-bulbul).” This group includes some of the samples published by Najer et al. (2021), and some of the samples in the “mitsutsui species-group” clade of Kolencik et al. (2022) are from monarch flycatchers, which may be closely related to those included in our analysis. The relationship between the bulbul-group Philopteroides group and the second group of Philopteroides is not resolved in either of these three studies.
Morphologically, specimens from the two Philopteroides groups we have examined are not generically separable. Mey (2004) included Philopteroides in two different morphotypes in his outline of the structure of the preantennal area (Mey, 2004; Figure 4), but the differences between morphotypes D1 and D2 appear to be gradual and may be species-level differences only. Moreover, neither of these groups in our data represent the “beckeri” species group as described by Valim and Palma (2013), contra Kolencik et al. (2022). The morphology of the dorsal anterior plate may be different between the “bulbul” and “monarch flycatcher” groups (cf. Najer et al., 2012b; Gustafsson et al., 2022a), but few species of either group are known. As noted by Gustafsson et al. (2022b) for Philopterus, somatic and setal characters may not be very useful for taxon delimitation in Philopteroides, and more detailed studies of the male genitalia may be necessary. For the present, no taxonomic recommendations about the status of these two groups, and the beckeri-species group, can be made.
Both groups of Philopteroides in our dataset include undescribed species. Preliminary examinations of the specimens from Alophoixus spp. (species 1; Figure 4) indicate that this species appears to be morphologically indistinguishable from Philopteroides flavala. Similarly, the specimens from Hemixos flavala (species 6; Figure 4) and Hemixos castanonotus (species 3; Figure 4) appear morphologically similar and were treated as conspecific by Gustafsson et al. (2022a). In both cases, specimens from non-type hosts of Pp. flavala may be cryptic species impossible to separate morphologically, but detailed examinations of the male genitalia need to be performed before this can be ascertained. The deep branches separating the species identified as Philopteroides cucphuongensis (species 9–11; Figure 4) may also indicate that this is a complex of cryptic species; we have not seen these specimens, and cannot ascertain whether differences in, e.g., male genitalia can be used to separate these populations into different species. Finally, a new species from Hypsipetes leucocephalus (species 8; Figure 4) may be close to Philopteroides holosternus (not included in analyses). These species will be described in a separate manuscript.
Three different species of lice were recovered from Ixos mcclellandii (species 1, 3, 4; Figure 4). One of these species is otherwise known from He. castanonotus, one from Alophoixus spp., whereas the specimen from Najer et al. (2021) is a singleton. Neither of the specimens we examined correspond to Philopterus haerixos described from this host by Gustafsson et al. (2022a), but the specimen derived from Najer et al. (2021) was not seen, and may represent this species. No other species of bulbuls were caught on the days our samples were collected, indicating that these specimens are not contaminations. Potentially, these records indicate recent host-switches to I. mcclellandii from other host species. Removing these two specimens under the assumption that they represent fortuitous collections of stragglers did not significantly affect the cophylogenetic analysis (Table 2). This suggests that the overall lack of significant co-speciation between Philopteroides and its bulbul hosts may be genuine, and not affected by the presence of stragglers in the dataset.
Asian bulbuls are divided into two major clades (Oliveros and Moyle, 2010; Shakya and Sheldon, 2017; Jha et al., 2021). Our dataset includes louse samples from both of these radiations: the host genera Alophoixus, Hemixos, Hypsipetes, Iole and Ixos from Clade 2 (sensu Jha et al., 2021), and Brachypodius, Pycnonotus, Rubigula and Spizixos from Clade 1 (sensu Jha et al., 2021). However, the lice do not form two clades that correspond to those of the hosts (Figure 4). The deepest supported division of bulbul Philopteroides in our dataset is between three different clades (Figure 4), one of which is divided into two major subclades. Philopteroides Clades A and B1 include only specimens from hosts in bulbul Clade 2, Philopteroides Clade C includes only specimens from hosts in bulbul Clade 1, and Philopteroides Clade B2 includes a mixture of specimens from bulbul Clades 1 and 2 hosts. The basal split within our bulbul Philopteroides phylogeny was inferred by Jane to be a co-speciation event (event 1 in Figure 5), suggesting that the early history of these bulbul lice may have been characterized by cospeciation, but that such patterns have later been eroded due to host switching.
More samples from a larger variety of bulbuls are needed before a thorough analysis of host association patterns can be performed. However, even in this limited dataset there are some interesting patterns. For instance, the relationships among lice from Alophoixus spp. closely mirror that of their hosts, with lice from the Bornean population of Al. bres well separated from those from mainland hosts. The lice from Al. flaveolus and Al. pallidus are closely related, which may be connected to the fact that their hosts comprise a ring species with gene flow between Al. flaveolus and Al. pallidus (Fuchs et al., 2015).
Conclusion
In conclusion, our data from Philopteroides head lice of Chinese bulbuls indicate that a mixture of host-switching and co-speciation with their hosts has probably shaped host association patterns in this group. Conceivably, the sociality of bulbuls, which may include heterospecific birds in the same feeding flocks, may contribute toward the lack of evidence of cospeciation in this group. However, more direct observations of lice from bulbuls would be desirable before the influence of mixed species feeding flocks can be established. The presence of three different species of Philopteroides on three different Ixos mcclellandii sampled at different localities may suggest that host switching is frequent in these lice, even if the mechanisms are currently unknown.
Data availability statement
The datasets presented in this study can be found in online repositories. The names of the repository/repositories and accession number(s) can be found at: https://www.ncbi.nlm.nih.gov/genbank/, OP476469-OP476492; OP555755–OP555801.
Ethics statement
The studies involving animals were reviewed and approved by the Animal Care and Welfare Committee, Institute of Zoology, Guangdong Academy of Sciences [then: Guangdong Institute of Applied Biological Resources], and the lice studied for this paper were collected in strict accordance with the Regulation for the Administration of Laboratory Animals (Decree No. 2, State Science and Technology Commission of the People’s Republic of China). A collection permit was previously obtained from the Panel on Laboratory Animal Care of the Institute of Zoology, Guangdong Academy of Sciences (then Guangdong Institute of Applied Biological Resources). In addition, specific collecting permits were obtained from forestry bureaus in the respective provinces (no number on permits; copies available on request).
Author contributions
MR performed fieldwork, extracted DNA, and performed analyses. DG performed fieldwork, planned and wrote the manuscript. CT performed analyses. AG wrote the manuscript. ZL helped with fieldwork. FZ helped editing the manuscript. All authors contributed to the article and approved the submitted version.
Funding
This research was funded by the Introduction of Full-Time High-Level Talent Fund of the Institute of Zoology, Guangdong Academy of Sciences (grant GIABR-GJRC201701), the National Natural Science Foundation of China (grant 31961123003), the Foreign Young Talent Plan (QN20200130012), the Pearl River Talent Recruitment Program of Guangdong Province (grant 2019QN01N968), and Science and Technology Program of Guangzhou (grant 202103000065). These agencies had no influence over the design and execution of this study, but provided resources for fieldwork, labwork, and the salaries of MR, DG, CT, and AG.
Acknowledgments
Two reviewers provided comments on an earlier draft of this manuscript, for which we are grateful.
Conflict of interest
The authors declare that the research was conducted in the absence of any commercial or financial relationships that could be construed as a potential conflict of interest.
Publisher’s note
All claims expressed in this article are solely those of the authors and do not necessarily represent those of their affiliated organizations, or those of the publisher, the editors and the reviewers. Any product that may be evaluated in this article, or claim that may be made by its manufacturer, is not guaranteed or endorsed by the publisher.
Supplementary material
The Supplementary material for this article can be found online at: https://www.frontiersin.org/articles/10.3389/fevo.2023.1053820/full#supplementary-material
Footnotes
1. ^http://tree.bio.ed.ac.uk/software/figtree/
2. ^https://species.h-its.org/gmyc/
References
Arlott, N. (2017). Collins field guide to birds of South-East Asia. Harper Collins, London, United Kingdom. 432.
Balakrishnan, P. (2014). Foraging behaviour of the near threatened grey-headed bulbul Pycnonotus priocephalus in relation to seasons and breeding stages. J. Bombay Nat. Hist. Soc. 111, 10–18. doi: 10.17087/bnhs/2014/v111i1/56520
Bartlow, A. W., Villa, S. M., Thompson, M. W., and Bush, S. E. (2016). Walk or ride? Phoretic behaviour of amblyceran and ischnoceran lice. Int. J. Parasitol. 46, 221–227. doi: 10.1016/j.ijpara.2016.01.003
Baum, H. (1968). Biologie und Ökologie der Amselfederläuse. Angew. Parasitol. 9, 129–175. + foldouts
Blanvillain, C., Salducci, J. M., Tutururai, G., and Maeura, M. (2003). Impact of introduced birds on the recovery of the Tahiti flycatcher (Pomarea nigra), a critically endangered forest bird of Tahiti. Biol. Conserv. 109, 197–205. doi: 10.1016/S0006-3207(02)00147-7
Brooke, M. D. L. (2010). Vertical transmission of feather lice between adult blackbirds Turdus merula and their nestlings: a lousy perspective. J. Parasitol. 96, 1076–1080. doi: 10.1645/GE-2513.1
Brooke, M. D. L., and Nakamura, H. (1998). The acquisition of host-specific feather lice by common cuckoos (Cuculus canorus). J. Zool. (Lond.) 244, 167–173. doi: 10.1111/j.1469-7998.1998.tb00022.x
Bush, S. E., and Clayton, D. H. (2006). The role of body size in host specificity: reciprocal transfer experiments with feather lice. Evolution 60, 2158–2167. doi: 10.1111/j.0014-3820.2006.tb01853.x
Bush, S. E., and Clayton, D. H. (2018). Anti-parasite behaviour of birds. Philos. Trans. R. Soc. Ser. B 373:20170196. doi: 10.1098/rstb.2017.0196
Bush, S. E., Weckstein, J. D., Gustafsson, D. R., Allen, J., DiBlasi, E., Shreve, S. M., et al. (2016). Unlocking the black box of feather louse diversity: a molecular phylogeny of the hyper-diverse genus Brueelia. Mol. Phylogenet. Evol. 94, 737–751. doi: 10.1016/j.ympev.2015.09.015
Catanach, T. A., Johnson, K. P., Marks, B. D., Moyle, R. G., Valim, M. P., and Weckstein, J. D. (2019). Two lineages of kingfisher lice exhibit differing degrees of cospeciation with their hosts. Parasitology 146, 1083–1095. doi: 10.1017/S0031182019000453
Chandran, K., and Vishnudas, C. K. (2018). A comparative study of mixed-species bird flocks in shaded coffee plantation and natural forest in Wayanad, Kerala. Indian Birds 14, 97–102.
Chedad, A., Bouzid, A., Bendjoudi, D., Horo, A., Guezoul, O., and Samraoui, B. (2022). Interspecific nest reuse between two species of waders in northern Sahara and an overview of records of mixed clutches in Algerian waterbirds. Afr. J. Ecol. 60, 838–842. doi: 10.1111/aje.12958
Chu, X., Dik, B., Gustafsson, D. R., Che, X., Zhang, Q., and Zou, F. (2019). The influence of host body size and food guild on prevalence and mean intensity of chewing lice (Phthiraptera) on birds in southern China. J. Parasitol. 105, 334–344. doi: 10.1645/17-137
Cicchino, A. C. (2007). Tyranniphilopterus polioptilus new species (Phthiraptera: Philopteridae) parasitic on the masked gnateater, Polioptila dumicola (Passeriformes, Polioptilidae) in Argentina. Zootaxa 1547, 43–50. doi: 10.11646/zootaxa.1547.1.4
Clayton, D. H. (1990). Host specificity of Strigiphilus owl lice (Ischnocera: Philopteridae), with the description of new species and host associations. J. Med. Entomol. 27, 257–265. doi: 10.1093/jmedent/27.3.257
Clayton, D. H., and Cotgreave, P. (1994). Relationship of bill morphology to grooming behaviour in birds. Anim. Behav. 47, 195–201. doi: 10.1006/anbe.1994.1022
Clayton, D. H., Koop, J. A. H., Harbison, C. W., Moyer, B. R., and Bush, S. E. (2010). How birds combat ectoparasites. Open Ornith. J. 3, 41–71. doi: 10.2174/1874453201003010041
Clayton, D. H., Lee, P. L. M., Tompkins, D. M., and Brodie, E. D. III (1999). Reciprocal natural selection om host-parasite phenotypes. Am. Nat. 154, 261–270. doi: 10.1086/303237
Clayton, D. H., Moyer, B. R., Bush, S. E., Jones, T. G., Gardiner, D. W., Rhodes, B. B., et al. (2005). Adaptive significance of avian beak morphology for ectoparasite control. Proc. R. Soc. B 272, 811–817. doi: 10.1098/rspb.2004.3036
Clayton, D. H., and Tompkins, D. M. (1994). Ectoparasite virulence is linked to mode of transmission. Proc. R. Soc. Lond. B 256, 211–217. doi: 10.1098/rspb.1994.0072
Clements, J. F., Schulenberg, T. S., Iliff, M. J., Roberson, D., Fredericks, T. A., Sullivan, B. L., et al. (2021). The eBird/Clements checklist of birds of the world: v2021. Available at: http://www.birds.cornell.edu/clementschecklist/download/ (Accessed March 3, 2022).
Conow, C., Fielder, D., Ovadia, Y., and Libeskind-Hadas, R. (2010). Jane: a new tool for the cophylogeny reconstruction problem. Algorithms Mol. Biol. 5:16. doi: 10.1186/1748-7188-5-16
Cordero, P. J., and Senar, J. C. (1990). Interspecific nest defence in European sparrows: different strategies to deal with a different species of opponent? Ornis Scand. 21, 71–73. doi: 10.2307/3676381
De Moya, R. S., Allen, J. M., Sweet, A. D., Walden, K. K. O., Palma, R. L., Smith, V. S., et al. (2019). Extensive host-switching of avian feather lice following the cretaceous-Paleogene mass extinction event. Commun. Biol. 2:445 [6 pp.]. doi: 10.1038/s42003-019-0689-7
Edgar, R. C. (2004). MUSCLE: multiple sequence alignment with high accuracy and high throughput. Nucleic Acids Res. 32, 1792–1797. doi: 10.1093/nar/gkh340
Ehrlich, P. R., and McLaughlin, J. F. (1988). Scrub jay predation on starlings and swallows: attach and interspecific defense. Condor 90, 503–505. doi: 10.2307/1368584
Eichler, W. (1942). Die Entfaltungsregel und andere Gesetzmäßigkeiten in den parasite-genetischen Beziehungen der mallophagen und anderer ständiger Parasiten zu ihren Wirten. Zool. Anz. 137, 77–83.
Eichler, W. (1948). Some rules in ectoparasitism. Ann. Mag. Nat. Hist. 1, 588–598. doi: 10.1080/00222934808653932
Eichler, W. (1963). “Fünfter band: Arthropoda. III. Abteilung: Insecta. 7. Buch. (b) Phthiraptera. 1. Mallophaga” in Klassen und Ordnungen des Tierreichs. ed. H. G. Bronns, vol. vii (Leipzig, Germany: Akademische Verlagsgesellschaft Geest & Portig K.–G.), 291.
Eveleigh, E. S., and Threlfall, W. (1976). Population dynamics of lice (Mallophaga) on auks (Alcidae) from Newfoundland. Can. J. Zool. 54, 1694–1711. doi: 10.1139/z76-197
Fuchs, J., Ericson, P. G. P., Bonillo, C., Couloux, A., and Pasquet, E. (2015). The complex phylogeography of the indo-Malayan Alophoixus bulbuls with the description of a putative new ring species complex. Mol. Ecol. 24, 5460–5474. doi: 10.1111/mec.13337
Fujisawa, T., and Barrowclough, T. G. (2013). Delimiting species using single-locus data and the generalized mixed yule coalescent approach: a revised method and evaluation on simulated data sets. Syst. Biol. 62, 707–724. doi: 10.1093/sysbio/syt033
GIMP Development Team (2019). GIMP. Available at: https://www.gimp.org (Accessed July 26, 2021).
Goodman, G. B., Klingensmith, M. C., Bush, S. E., and Clayton, D. H. (2020). The role of scratching in the control of ectoparasites on birds. Auk 137, 1–9. doi: 10.1093/auk/ukaa010
Grossi, A. A., Sharanowski, B. J., and Galloway, T. D. (2014). Anatoecus species (Phthiraptera: Philopteridae) from Anseriformes in North America and taxonomic status of Anatoecus dentatus and Anatoecus icterodes. Can. Entomol. 146, 598–608. doi: 10.4039/tce.2014.12
Gustafsson, D. R., Adam, C., and Zou, F. (2022c). One new genus and three new species of the Penenirmus-complex (Phthiraptera: Ischnocera) from China, with resurrection of Picophilopterus Ansari, 1947. Zootaxa 5087, 401–426. doi: 10.11646/zootaxa.5087.3.1
Gustafsson, D. R., Lei, L., Chu, X., Zou, F., and Bush, S. E. (2019b). New genus and two new species of chewing lice from southeast Asian trogons (Aves: Trogoniformes), with a revised key to the Philopterus-complex. Acta Parasitol. 64, 86–102. doi: 10.2478/s11686-018-00011-x
Gustafsson, D. R., Lei, L., Luo, K., Chu, X., Zhao, X., Zhang, Q., et al. (2019a). Chewing lice from high-altitude and migrating birds in Yunnan, China, with descriptions of two new species of Guimaraesiella. Med. Vet. Entomol. 33, 407–419. doi: 10.1111/mve.12378
Gustafsson, D. R., and Najer, T. (2022). Fahrenholz’s rule is not a valid methodology for determining species limits in chewing lice (Phthiraptera). Bionomina 29, 1–73. doi: 10.11646/bionomina.29.1.1
Gustafsson, D. R., Najer, T., Zou, F., and Bush, S. E. (2022a). The ischnoceran chewing lice (Phthiraptera: Ischnocera) of bulbuls (Aves: Passeriformes: Pycnonotidae), with descriptions of 18 new species. Eur. J. Taxon. 800, 1–88. doi: 10.5852/ejt.2022.800.1683
Gustafsson, D. R., Najer, T., Zou, F., and Bush, S. E. (2022b). New species of Philopterus Nitzsch, 1818 (Ischnocera: Philopteridae), with notes on Cypseloecus Conci, 1941. Eur. J. Taxon. 790, 1–52. doi: 10.5852/ejt.2022.790.1641
Gustafsson, D. R., and Olsson, U. (2012). Flyway homogenization or differentiation? Insights from the phylogeny of the sandpiper (Charadriiformes: Scolopacidae: Calidrinae) wing louse genus Lunaceps (Phthiraptera: Ischnocera). Int. J. Parasitol. 42, 93–102. doi: 10.1016/j.ijpara.2011.11.003
Harbison, C. W., Jacobsen, M. V., and Clayton, D. H. (2009). A hitchhiker’s guide to parasite transmission: the phoretic behaviour of feather lice. Int. J. Parasitol. 39, 569–575. doi: 10.1016/j.ijpara.2008.09.014
Harmackova, L. (2021). Interspecific feeding in birds: a short review. Acta Ornithologica 56, 1–14. doi: 10.3161/00016454AO2021.56.1.001
Harnos, A., Lang, Z., Petrás, D., Bush, S. E., Szabó, K., and Rózsa, L. (2017). Size matters for lice on birds: coevolutionary allometry of host and parasite body size. Evolution 71, 421–431. doi: 10.1111/evo.13147
Harrison, L. (1915). Mallophaga from apteryx, and their significance; with a note on the genus Rallicola. Parasitology 8, 88–100. doi: 10.1017/S0031182000010428
Harrison, C. J. O. (1965). Allopreening as agonistic behaviour. Behaviour 24, 161–208. doi: 10.1163/156853965X00011
Hillgarth, N. (1996). Ectoparasite transfer during mating in ring-necked pheasants Phasianus colchicus. J. Avian Biol. 27, 260–262. doi: 10.2307/3677232
Hino, T. (2002). Breeding bird community and mixed-species flocking in a deciduous broad-leaved forest in western Madagascar. Ornithol. Sci. 1, 111–116. doi: 10.2326/osj.1.111
Hopkins, G. H. E. (1942). The Mallophaga and an aid to the classification of birds. Ibis 84, 94–106. doi: 10.1111/j.1474-919X.1942.tb03423.x
Jha, A., Seneviratne, S., Prayag, H. S., and Vasudevan, K. (2021). Phylogeny identifies multiple colonization events and Miocene aridification as drivers of south Asian bulbul (Passeriformes: Pycnonotidae) diversification. Org. Divers. Evol. 21, 783–794. doi: 10.1007/s13127-021-00506-y
Jiang, A., Jiang, D., Goodale, E., Zhou, F., and Wen, Y. (2016). Olive-backed sunbird Cinnyris jugularis assisting crested bunting Melophus lathami at the nest: substantiated evidence for interspecific feeing, Guangxi, south-West China. Forktail 32, 93–96.
Johnson, K. P., Bush, S. E., and Clayton, D. H. (2005). Correlated evolution of host and parasite body size: test of Harrison’s rule using birds and lice. Evolution 59, 1744–1753. doi: 10.1554/05-035.1
Johnson, K. P., Shreve, S. M., and Smith, V. S. (2012). Repeated adaptive divergence of microhabitat specialization in avian feather lice. BMC Biol. 10:52 [11 pp.]. doi: 10.1186/1741-7007-10-52
Johnson, K. P., Weckstein, J. D., Virrueta Herrera, S., and Doña, J. (2021). The interplay between host biogeography and phylogeny in structuring diversification of the feather louse genus Penenirmus. Mol. Phylogenet. Evol. 165:107297. doi: 10.1016/j.ympev.2021.107297
Jones, K. C., Roth, K. L., Islam, K., Hamel, P. B., and Smith, C. G. III (2007). Incidence of nest material kleptoparasitism involving cerulean warblers. Wilson J. Ornithol. 119, 271–275. doi: 10.1676/05-145.1
Johnson, K. P., Adams, R. J., and Clayton, D. H. (2002). The phylogeny of the louse genus Brueelia does not reflect host phylogeny. Biol. J. Linn. Soc. 77, 233–247.
Kamtaeja, S., Sitasuwan, N., Chomdej, S., Jatisatienr, A., and Mennill, D. J. (2012). Species-distinctiveness in the vocal behaviour of six sympatric bulbuls (genus Pycnonotus) in south-East Asia. Emu – Austral Ornith. 112, 199–208. doi: 10.1071/MU11044
Keirans, J. E. (1975). A review of the phoretic relationship between Mallophaga (Phthiraptera: Insecta) and Hippoboscidae (Diptera: Insecta). J. Med. Entomol. 12, 71–76. doi: 10.1093/jmedent/12.1.71
Kim, K. C. (1988). “Evolutionary parallelism in Anoplura and eutherian mammals,” in Biosystematics of Haematophagous insects. Systematics association special. ed. Service, M.W, vol. 37 (United Kingdom: Oxford (Clarendon Press)), 376.
Klassen, G. J. (1992). Coevolution: a history of the macroevolutionary approach to studying host-parasite associations. J. Parasitol. 78, 573–587. doi: 10.2307/3283532
Kolencik, S., Johnson, K. P., Grant, A. R., Valim, M. P., Kuabara, K. M. D., Weckstein, J. D., et al. (2022). Molecular phylogenetics of the avian feather louse Philopterus-complex (Phthiraptera: Philopteridae). Mol. Phylogenet. Evol. 174:107556:7. doi: 10.1016/j.ympev.2022.107556
Kotagama, S. W., and Goodale, E. (2004). The composition and spatial organization of mixed-species flocks in a Sri Lankan rainforest. Forktail 20, 63–70.
Kubelka, V., Sladecek, M., and Salek, M. (2014). Inter-specific nest scrape reuse in waders: little ringed plovers taking over the nest scrapes of northern lapwings. Bird Study 61, 282–286. doi: 10.1080/00063657.2014.899965
Kumar, S., Stecher, G., Li, M., Knyaz, C., and Tamura, K. (2018). MEGA X: molecular evolutionary genetics analysis across computing platforms. Mol. Biol. Evol. 35, 1547–1549. doi: 10.1093/molbev/msy096
Larkin, M. A., Blackshields, G., Brown, N. P., Chenna, R., McGettigan, P. A., McWilliam, H., et al. (2007). Clustal W and Clustal X version 2.0. Bioinformatics 23, 2947–2948. doi: 10.1093/bioinformatics/btm404
Lee, P. L. M., and Clayton, D. H. (1995). Population biology of swift (Apus apus) ectoparasites in relation to host reproductive success. Ecol. Entomol. 20, 43–50. doi: 10.1111/j.1365-2311.1995.tb00427.x
Lee, L., Tan, D. J. X., Obona, J., Gustafsson, D. R., Ang, Y., and Meier, R. (2022). Hitchhiking into the future on a fly: toward a better understanding of phoresy and avian louse evolution (Phthiraptera) by screening bird carcasses for phoretic lice on hippoboscid flies (Diptera). Syst. Entomol. 47, 420–429. doi: 10.1111/syen.12539
Legendre, P., Desdevises, Y., and Bazin, E. (2002). A statistical test for host – parasite coevolution. Syst. Biol. 51, 217–234. doi: 10.1080/10635150252899734
Lindell, C. (1996). Patterns of nest usurpation: when should species converge on nest niches? Condor 98, 464–473. doi: 10.2307/1369560
Lopes Palmeira, F. B. (2008). Allopreening behavior between black vulture (Coragyps atratus) and southern caracara (Caracara plancus) in the Brazilian Pantanal. Revista Brasileira de Ornitologia 16, 172–174.
Maa, T. C. (1969). A revised checklist and concise host index of Hippoboscidae (Diptera). Pacific Insects Monograph 20, 261–299.
Mackinnon, J., and Phillipps, K. (2000). A field guide to the birds of China. Oxford University Press, Oxford, UK, 571.
Mahesh, S. S., Shyamal, L., and Thomas, V. (2010). Nest material kleptoparasitism by the oriental white-eye Zosterops palpebrosus. Indian Birds 6, 22–23.
McClure, H. E. (1967). The composition of mixed species flocks in lowland and sub-montane forests of Malaya. Wilson Bulletin 79, 131–154.
Mey, E. (1982). Mallophagen-Befall bei mongolischen Vögeln. Mitteilungen aus dem Zoologischen Museum in Berlin, Supplementheft 1982 58, 55–75.
Mey, E. (1999). “Phylogenetic relationships of the Megapodiidae as indicated by their ischnoceran, in particular goniodid, chewing lice (Insecta: Phthiraptera).” in Proceedings of the third international Megapode symposium. eds. R. Dekker, D. Jones, and J. Benshemesh (Netherlands: Leiden (Zoologische Verhandlungen)), 174.
Mey, E. (2004). Zur Taxonomie, Verbreitung unf parasitophyletischer Evidenz des Philopterus-Komplexes (Insecta, Phthiraptera, Ischnocera). Ornithol. Anz. 43, 149–203.
Minock, M. E. (1972). Interspecific aggression between black-capped and mountain chickadees at winter feeding stations. Condor 74, 454–461. doi: 10.2307/1365898
Mo, M. (2016). An apparent case of interspecific allopreening by a royal spoonbill Platalea regia. Aust. Zool. 38, 214–216. doi: 10.7882/AZ.2016.020
Muszalski Shy, M. (1982). Interspecific feeding among birds: a review. J. Field Ornithol. 53, 370–393.
Najer, T., Gustafsson, D. R., and Sychra, O. (2016). Two new species of Philopteroides (Phthiraptera: Ischnocera: Philopteridae) of the beckeri species-group, from new Guinean painted berrypeckers (Aves: Passeriformes: Paramythiidae). Zootaxa 4139, 527–541. doi: 10.11646/zootaxa.4139.4.5
Najer, T., Papousek, I., Sychra, O., Sweet, A. D., and Johnson, K. P. (2021). Combining nuclear and mitochondrial loci provides phylogenetic information in the Philopterus complex of lice (Psocodea: Ischnocera: Philopteridae). J. Med. Entomol. 58, 252–260. doi: 10.1093/jme/tjaa166
Najer, T., Sychra, O., Hung, N. M., Capek, M., Podzemny, P., and Literak, I. (2012a). New species and new records of chewing lice (Phthiraptera: Amblycera and Ischnocera) from bulbuls (Passeriformes: Pycnonotidae) in Vietnam. Zootaxa 3357, 37–48. doi: 10.11646/zootaxa.3357.1.3
Najer, T., Sychra, O., Literák, I., Procházka, P., Capek, M., and Koubek, P. (2012b). Chewing lice (Phthiraptera) from wild birds in Senegal, with descriptions of three species of the genera Brueelia and Philopteroides. Acta Parasitol. 57, 90–98. doi: 10.2478/s11686-012-0005-x
Ng, D., and Jasperson, B. D. (1984). Interspecific allopreening between crested caracara and black vulture. Condor 86, 214–215. doi: 10.2307/1367047
Oliveros, C. H., and Moyle, R. G. (2010). Origin and diversification of Philippine bulbuls. Mol. Phylogenet. Evol. 54, 822–832. doi: 10.1016/j.ympev.2009.12.001
Palma, R. L. (1978). Slide-mounting of lice: a detailed description of the Canada balsam technique. N. Z. Entomol. 6, 432–436. doi: 10.1080/00779962.1978.9722313
Partridge, L., and Ashcroft, R. (1976). Mixed-species flocks of birds in hill forest in Ceylon. Condor 78, 449–453. doi: 10.2307/1367093
Price, R. D., Hellenthal, R. A., Palma, R. L., Johnson, K. P., and Clayton, D. H. (2003). The chewing lice: world checklist and biological overview, Vol. 24 Champaign, Illinois, United States: Illinois Natural History Survey Special Publication, 501.
Pulliainen, E., and Saari, L. (1991). Breeding biology of the wood sandpiper Tringa glareola in eastern Finnish Lapland. Ornis Fennica 68, 127–128.
Revell, L. J. (2011). Phytools: an R package for phylogenetic comparative biology (and other things). Methods Ecol. Evol. 3, 217–223. doi: 10.1111/j.2041-210X.2011.00169.x
Samplonius, J. M., and Both, C. (2013). A case of three species mixed broods after two interspecific next takeovers. Ardea 101, 105–107. doi: 10.5253/078.102.0113
Sanitjan, S., and Chen, J. (2009). Habitat and fig characteristics influence the bird assemblage and network properties of fig trees from Xishuangbanna, south-West China. J. Trop. Ecol. 25, 161–170. doi: 10.1017/S0266467409005847
Selander, R. K., and La Rue, C. J. Jr. (1961). Interspecific preening invitation display of parasitic cowbirds. Auk 78, 473–504. doi: 10.2307/4082183
Shakya, S. B., and Sheldon, F. H. (2017). The phylogeny of the world’s bulbuls (Pycnonotidae) inferred using a supermatrix approach. Ibis 159, 498–509. doi: 10.1111/ibi.12464
Shermila, W. G. D. D. M., and Wikramsinghe, S. (2013). Composition of mix species foraging flocks of birds in Riverstan of montane region, Sri Lanka. J. Trop. For. Environ. 3, 55–63. doi: 10.31357/jtfe.v3i1.1123
Souto, H. N., Franchin, A. G., and Júnior, O. M. (2009). New records of allopreening between black vultures Coragyps atratus (Ciconiiformes: Cathartidae) and crested caracara (Caracara plancus) (Falconiformes: Falconidae). Sociobiology 53, 125–129.
Sreekar, R., Le, N. T. P., and Harrison, R. D. (2010). Vertebrate assemblage at a fruiting fig (Ficus caulocarpa) in Maliau basin, Malaysia. Trop. Conserv. Sci. 3, 218–227. doi: 10.1177/194008291000300208
Sridhar, H., and Shanker, K. (2014). Using intra-flock association patterns to understand why birds participate in mixed-species foraging flocks in terrestrial habitats. Behav. Ecol. Sociobiol. 68, 185–196. doi: 10.1007/s00265-013-1633-3
Srinivasan, U., Raza, R. H., and Quader, S. (2012). Patterns of species participation across multiple mixed-species flock types in a tropical forest in northeastern India. J. Nat. Hist. 46, 2749–2762. doi: 10.1080/00222933.2012.717644
Suchard, M. A., Lemey, P., Baele, G., Ayres, D. L., Drummond, A. J., and Rambaut, A. (2018). Bayesian phylogenetic and phylodynamic data integration using BEAST 1.10. Virus. Evolution 4:VEY016. doi: 10.1093/ve/vey016
Sweet, A. D., Allen, J. M., and Johnson, K. P. (2014). Novel primers from informative nuclear loci for louse molecular phylogenetics (Insecta: Phthiraptera). J. Med. Entomol. 51, 1122–1126. doi: 10.1603/ME13218
Sweet, A. D., Bush, S. E., Gustafsson, D. R., Allen, J. M., DiBlasi, E., Skeen, H. R., et al. (2018). Host and parasite morphology influence congruence between host and parasite phylogenies. Int. J. Parasitol. 48, 641–648. doi: 10.1016/j.ijpara.2018.01.007
Sweet, A. D., and Johnson, K. P. (2016). Cophylogenetic analysis of New World ground-doves (Aves: Columbidae) and their parasitic wing lice (Insecta: Phthiraptera: Columbicola). Mol. Phylogenet. Evol. 103, 122–132. doi: 10.1016/j.ympev.2016.07.018
Sweet, A. D., and Johnson, K. P. (2018). The role of parasite dispersal in shaping a host-parasite system at multiple evolutionary scales. Mol. Ecol. 27, 5104–5119. doi: 10.1111/mec.14937
Thompson, L. J., Davies, J. P., Bildstein, K. L., and Downs, C. T. (2017). Removal (and attempted removal) of material from a hooded vulture Necrosyrtes monachus nest by a starling and a hooded vulture. Ostrich 88, 183–187. doi: 10.2989/00306525.2017.1316786
Tian, C., Yu, X., Wang, Z., Zou, F., and Gustafsson, D. R. (2022). Phylogenetic relationships of Guimaraesiella and Priceiella (Phthiraptera: Ischnocera) from babblers and non-babblers (Passeriformes). J. Parasitol. 108, 107–121. doi: 10.1645/21-91
Tortosa, F. S., and Redondo, T. (1992). Frequent copulations despite low sperm competition in white storks (Ciconia ciconia). Behaviour 121, 288–314. doi: 10.1163/156853992X00408
Uchida, S. (1948). Studies on the biting-lice (Mallophaga) of Japan and adjacent territories (suborder Ischnocera Pt. I). Jpn. Med. J. 1, 303–326. doi: 10.7883/yoken1948.1.303
Valim, M. P., and Palma, R. L. (2013). Three new species of the genus Philopteroides Mey, 2004 (Phthiraptera, Ischnocera, Philopteridae) from New Zealand. ZooKeys 297, 71–89. doi: 10.3897/zookeys.297.5118
Verbeek, N. A. M., Butler, R. W., and Richardson, H. (1981). Interspecific allopreening solicitation in female Brewer’s blackbird. Condor 83, 179–180. doi: 10.2307/1367425
Vezzoli, G., Mullens, B. A., and Mench, J. A. (2015). Relationships between beak condition, preening behavior and ectoparasite infestation levels in laying hens. Poult. Sci. 94, 1997–2007. doi: 10.3382/ps/pev171
Villa, S. M., Evans, M. D., Subhani, Y. K., Altuna, J. C., Bush, S. E., and Clayton, D. H. (2018). Body size and fecundity are correlated in feather lice (Phthiraptera: Ischnocera) implications for Harrison’s rule. Ecol. Entomol. 43, 394–396. doi: 10.1111/een.12511
Von Kéler, S. (1959). Über die Wirtstreue und die Verbreitung der Mallophagen. Z. Parasitenkd. 19, 375–410. doi: 10.1007/BF00260225
Weckstein, J. D. (2004). Biogeography explains cophylogenetic patterns in toucan chewing lice. Syst. Biol. 53, 154–164. doi: 10.1080/10635150490265085
Wydhayagarn, C., Elliot, S., and Wangpakapattanawong, P. (2009). Bird communities and seedling recruitment in restoring seasonally dry forest using the framework species method in northern Thailand. New For. 38, 81–97. doi: 10.1007/s11056-009-9133-z
Yamagishi, A., Yao, I., Johnson, K. P., and Yoshizawa, K. (2014). Comparisons of host specificity in feather louse genera (Insecta: Phthiraptera: Philopteridae) parasitizing gulls (Aves: Laridae: Larus). Zool. Sci. 31, 383–389. doi: 10.2108/zs130263
Zhang, J., Kapli, P., Pavlidis, P., and Stamatakis, A. (2013). A general species delimitation method with applications in phylogenetic placements. Bioinformatics 29, 2869–2876. doi: 10.1093/bioinformatics/btt499
Keywords: coevolution, host-switching, Phthiraptera, Ischnocera, Philopterus-complex, bulbuls
Citation: Ren M, Gustafsson DR, Tian C, Grossi AA, Liu Z and Zou F (2023) Coevolutionary analysis of the Philopteroides Mey, 2004 (Phthiraptera: Ischnocera) parasitizing bulbuls (Passeriformes: Pycnonotidae). Front. Ecol. Evol. 11:1053820. doi: 10.3389/fevo.2023.1053820
Edited by:
Jenny C. Dunn, University of Lincoln, United KingdomReviewed by:
Roi Dor, Open University of Israel, IsraelKaren Denise McCoy, Centre National de la Recherche Scientifique (CNRS), France
Andrew Sweet, Arkansas State University, United States
Copyright © 2023 Ren, Gustafsson, Tian, Grossi, Liu and Zou. This is an open-access article distributed under the terms of the Creative Commons Attribution License (CC BY). The use, distribution or reproduction in other forums is permitted, provided the original author(s) and the copyright owner(s) are credited and that the original publication in this journal is cited, in accordance with accepted academic practice. No use, distribution or reproduction is permitted which does not comply with these terms.
*Correspondence: Zhixiao Liu, enhsaXUxOTY1QDE2My5jb20=; Fasheng Zou, em91ZnNAZ2l6LmdkLmNu
†These authors have contributed equally to this work and share first authorship
‡ORCID: Mengjiao Ren https://orcid.org/0000-0002-0108-6104
Daniel R. Gustafsson https://orcid.org/0000-0001-8868-8310
Chunpo Tian https://orcid.org/0000-0001-8681-4475
Alexandra A. Grossi https://orcid.org/0000-0002-1397-4221
Zhixiao Liu https://orcid.org/0000-0002-1151-1864
Fasheng Zou https://orcid.org/0000-0002-8913-5651