- 1Department of Urban Planning, Faculty of Planning and Environmental Sciences, University of Tabriz, Tabriz, Iran
- 2Department of Landscape Architecture, Faculty of Agriculture, University of Tabriz, Tabriz, Iran
Population growth is a significant challenge in developing countries because it leads to the destruction of green infrastructure and urban agriculture lands around cities, resulting in dissatisfaction with the environment. This study aims to revitalize abandoned agricultural lands within city limits due to urban planning, which can be considered a kind of green infrastructure. In Tabriz, Iran, urban agriculture and urban texture coexist, and the effect of native plant-crop combinations on micrometeorological conditions and thermal comfort on agricultural land was examined. The ENVI-MET model simulated the research area, and the output parameters were validated by comparing their values to field data. The model identified seven proposed scenarios that demonstrated higher performance in improving outdoor thermal comfort. The results showed that edible functions of deciduous plants increased with canopy height and diameter, which improved thermal comfort in high-temperature conditions. Adopting this form of planting would rejuvenate abandoned agricultural fields. Consequently, outdoor thermal comfort may be improved [1.42 predicted mean value (PMV) reduction in the land without plants and a 1.37 PMV reduction in the agriculture land simulation], leading to the rehabilitation of urban agricultural lands that have lost their function in developing cities. These research findings can be utilized to help address the impacts of population growth on green infrastructure and urban agriculture lands.
1. Introduction
The rapid expansion of urbanization over the past few decades has resulted in a range of negative consequences for urban environments, including increased temperatures and changing climates. Urban areas contribute to local climate change through the release of large amounts of heat energy, rising greenhouse gas emissions, and changes in land use. These issues have caused significant harm to the environment (Rad et al., 2017; Herath et al., 2021). To improve thermal comfort and create sustainable urban solutions, it is essential to plan initiatives that can benefit urban areas and make them more pleasant for residents (Raymond et al., 2017; Crank et al., 2018). One common method for mitigating urban heat island effects is using trees and plants in urban areas. Vegetation has multiple mechanisms that can significantly contribute to improving thermal comfort, such as reducing the amount of solar radiation that reaches urban surfaces below the canopy layer, evaporation, and transpiration [US EPA (US Environmental Protection Agency), 2008]. Although building materials like asphalt have a higher albedo value than vegetation, plants absorb less heat due to their lower specific heat capacity. Additionally, vegetation with leaves and branches shades urban surfaces to reduce the amount of solar radiation that reaches them below the canopy layer, according to Targhi and Van Dessel (2015). They enhance solar absorption and prevent long-wave radiation transmission by replacing hard urban surfaces with natural components. They help reduce the temperature of the atmosphere by trapping short-wave radiation in the near-Earth atmosphere (Wu et al., 2017). Trees are more effective in reducing the climate and ambient temperature than other plant species (El-Bardisy et al., 2016; Lee and Mayer, 2016). Developing the concept of urban agriculture is one strategy to protect and establish urban ecosystems. As a result, it is a sustainable and efficient system for supporting the city’s ecological and economic goals by restoring the agricultural landscape’s identity and sense of place (Sartison and Artmann, 2020). Prevent urban suburbs from being destroyed due to land-use changes and devastation. It can also revitalize and restore landscapes, resulting in the stability of the urban environment.
In many developed nations, urban agriculture is often viewed as a hobby and a space for community members to unwind and escape the stresses of city life. Its territory is primarily composed of sparsely populated, unused lands in densely populated urban areas. Urban agriculture is a multifaceted strategy that goes beyond a single definition. According to the FAO definition, inclusive agricultural activities contribute to food security and income creation (Nzimande, 2013). Agriculture’s territory can include both suburbs and inner cities (Burgin, 2018); its ability to incorporate the urban economy and ecology distinguishes it from rural agriculture (Peng et al., 2015). As a type of green infrastructure, urban agriculture can significantly contribute to the sustainability of the urban environment and help mitigate the impacts of urban heat islands (Ackerman et al., 2014; Hosseinpour et al., 2022).
Selecting appropriate trees to enhance micrometeorological conditions is crucial to obtaining the most effective planting design, as leaf morphology and tree species can impact green spaces and local climate change. Trees provide more shade than grass and have higher cooling advantages (Li et al., 2016), thus improving thermal comfort.
The primary purpose of urban agriculture is to respect the potential of healthy life, create relationships with nature in the city, and pursue three goals: enhancing the ecological structure of the land, restoring social effectiveness, and growing the city’s economy (Partalidou and Anthopoulou, 2017). The goal of this study is related to abandoned agricultural lands being urbanized. Some agricultural lands are urbanized after being abandoned and later incorporated into urban planning (e.g., house construction; Tzoulas et al., 2007; Grădinaru et al., 2015; Fayet et al., 2022). Evaluating how current and future abandoned farmlands can contribute to social and environmental policy goals requires assessing how these trajectories develop. Multi-functional landscapes, including economic diversification through recreational activities, have often been described as valuable options for revitalizing urban agricultural lands. Altieri (1989) described sustainable agriculture as a system that maintains production over time without depleting resources through the use of low-input technologies that diversify production. Modifying crop cultivation patterns, such as crop combination with trees, and increasing food supply and ecological function in urban agricultural areas are steps toward this goal. Therefore, seven scenarios with varied planting patterns compatible with the region were simulated in ENVI-Met software to examine microclimatic and thermal comfort indicators. For each scenario, the PMV index, Ta (c), and PET (c) were calculated. The outcomes of this research will help mitigate the effects of heat islands in urban areas, improve residents’ thermal comfort, and guide landscape designers and urban planners in managing urbanization growth challenges by rehabilitating urban agricultural lands.
2. Material and method
2.1. Study area and methods headings
2.1.1. Thermal comfort index
Thermal comfort refers to people’s feelings of contentment in a specific area when they are both intellectually and physically comfortable (De Dear, 1998). The temperature equilibrium between the body and the environment relates to thermal comfort. Because the human body in this condition does not feel cold or hot (Watson, 1983), the PMV index, developed by Fanger (1970), is one of the most significant indices in assessing physiological temperatures, and it is used to forecast the population perception of a group subjected to the same microclimatic circumstances. This index has a seven-point range from −3 to +3, with 0 representing a neutral temperature experience and four positive numbers suggesting physiological stress during the hot season (Matzarakis and Mayer, 2000; Wimalarathne and Perera, 2019), as well as A higher positive sign value indicates increased heat stress, while a higher negative sign value indicates increased cold stress (UNI. Ergonomia degli ambienti termici, 2006). This concept’s foundation is based on the principle that the human body attempts to achieve thermal balance with its surroundings, which means that heat is acquired or lost in the process. This strategy is based on two indicators: environmental circumstances and personal characteristics. Air temperature (Ta), mean radiation temperature (Tmrt), specific humidity (RH), air velocity (V), and atmospheric pressure all contribute to environmental quality. Human characteristics include a person’s metabolism rate, clothing level, type of physical activity, weight, and age (UNI. Ergonomia degli ambienti termici, 2006).
Physiologically equivalent temperature (PET) allows an individual to evaluate the effect of a wide range of outdoor thermal comfort on personal experience (Lin et al., 2010). The thermal comfort index is calculated in enclosed spaces without physical activity and using conventional clothing at temperatures ranging from 18 to 23 degrees Celsius (Matzarakis et al., 1999). This indicator’s validation examinations in various climatic conditions in open urban areas revealed the most substantial relationship with the satisfaction of thermal comfort (Fabbri, 2013).
2.2. Study site
The site is in Hokmabad (an urban agriculture region), Tabriz is a city in the northwest of Iran, and the capital of East Azerbaijan Province; it is the fourth largest city in Iran and is situated at an altitude of 1,350 m at the junction of the Quri River and Aji River (Breuste and Rahimi, 2015; Rahimi, 2016). According to the Iranian Census Center, this county has a land area of 2,270 km2. Summer average temperatures range from 14 to 30°C, and winter temperatures range from 5 to −4°C (Ministry Governorate of East Azerbaijan Province, 2008; see Figure 1).
For the simulation, June 30, 2020, was chosen as one of the warmest days of the year. The East-Tabriz Meteorological Station provides meteorological data such as minimum and maximum temperatures (Ta), relative humidity, wind speed at 10 meters, and wind direction. The information entered into the ENVI-MET software is shown in Table 1. The 3-hourly measured T, relative humidity, and water vapor pressure values at the weather station “East of Tabriz” on the simulation day are shown in Figure 2.
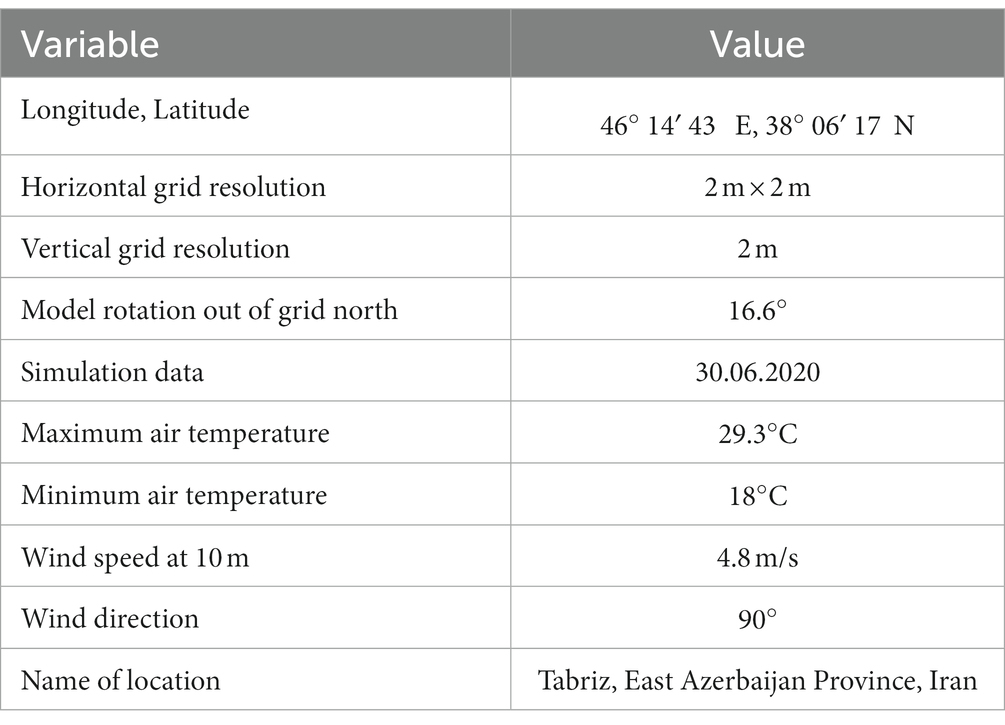
Table 1. Input data applied in simulation, speed at the meteorological station “East Tabriz” on June 30th, 2020, in the period 10:00–18:00 LST.
2.3. Simulation model
ENVI-Met, a tridimensional model that studies the interactions of the surface, vegetation, and atmosphere and provides simulations for the microscale dimension, is one of the most well-known tools for urban climate modeling (Gusson and Duarte, 2016). This tool allows for the research and monitoring of the effects of urban design and architecture on outdoor microclimates (Simon et al., 2020). Envi-Met is well-known for its ability to predict variations in solar radiation by creating buildings and materials in the context of a specific place (Middel et al., 2014). This program also calculates the impacts of vegetation, such as the potential temperature of leaves, considering photosynthetic rates, soil moisture content, and local evaporation rates (Bruse, 2004). One of its significant advantages is that it reproduces the major atmospheric processes that affect microclimate, such as wind, turbulence, radiation fluxes, air temperature, and relative humidity, using fundamental thermodynamic and fluid mechanics rules (McRae et al., 2020). From a microclimate perspective, the simulations consider daily cycles in complex urban structures, including buildings and plants of various forms and sizes (Celis and Frederico, 2018). ENVI-Met has been used in several studies to predict near-ground air temperatures and to help understand the impact of urban form on microclimate (Tsoka et al., 2018; Aslam and Rana, 2022).
As a result, the simulation ignored the effect of these parameters, which means that altering these variables could change the thermal comfort conditions. Four groups of plant species that serve a helpful purpose in urban agricultural regions were chosen for this study’s simulations. Utilize a variety of plants, such as trees, shrubs, and groundcovers. They were simulated, including plant groups: 1-green space (simulation of the urban agricultural area with crops); 2-shading trees (coniferous and deciduous); 3-shrubs. The Leonardo V4.4 sub-model was used to extract two-dimensional maps and weather data generated by the ENVI-Met V4.4.6 software. The PMV index was extracted using the Biomet V1.5.exe sub-model. In addition to the availability of a variety of recommended bio-metrological indicators, this study focuses on the physiologically equivalent temperature (PET) as a useful thermal indicator for analyzing the components of the chosen outdoor environment (Höppe, 2002). Rayman 3.1 software was used to present and calculate this data (Matzarakis et al., 1999, 2007).
2.4. Scenario design
With an emphasis on using native species because of their physiological and morphological features that improve climate conditions and cause urban sustainability, which is an ideal way to restore the urban agricultural lands that are being destroyed, the selected plants and design scenarios in this study were generally chosen with consideration of climate and regional conditions. These scenarios were divided into three plant groupings. This research examined six different types of species in three other groups, including green cover (A) and shading trees, including coniferous and deciduous trees (deciduous trees with edible functions, such as Quercus alba (B1) from the Fagaceae and Malus domestica (B2) from the Rosaceae), evergreen trees (Cupressus arizonica (C1) from the Cupressaceae and Platycladus orientalis (C2) from the Cupressaceae), and shrubs (E). All of the evergreen trees in the simulation were conifers. These trees were designed for seven different scenarios. The first six scenarios (A, B1, B2, C1, C2, and E) analyzed the species and environmental circumstances. In contrast, the eighth scenario was the optimal function of a species–crop combination that caused thermal comfort to improve (Table 2). Furthermore, these planting patterns were designed densely with a gap between the tree canopies to allow for wind flow. Using the LEONARDO application to compare the micrometeorological indices of the different scenarios with a base scenario that featured no trees and a green cover scenario (a simulation of agricultural land with crops), The simulation output files were imported into the BIOMET application, which is a tool within the Envi-Met software, to determine the thermal comfort index (PMV) and other parameters (Figure 3). These patterns and planting combinations were picked from existing patterns in urban agriculture and natural plants in the region; the effects of each of these patterns and species have never been investigated previously.
3. Results
The comparison was made among seven scenarios in 8 h, from 10:00 to 18:00. The results demonstrated that in all scenarios, there was a reduction in the mean T and PMV index, which improved thermal comfort conditions as compared to the base scenario (without plant and crop). Furthermore, it resulted in a decrease in physiological equivalent temperature (PET) in the surrounding area. Output parameters demonstrate that successful species promoting thermal comfort have a broad leaf area and a high height. They also have a broad canopy to help absorb the sun’s rays and circulate airflow, so deciduous trees have a better function than evergreen trees. This result was obtained on the hottest day of the year. Furthermore, each group contains two types of species. Finally, select the best species from each group and create a combination scenario, considering the result: The combination of these includes A, B1, and C1.
3.1. Air temperature (Ta)
The Ta simulation results show that all scenarios, including scenario A, which simulates agricultural land, performed better than the base state. In the B1 scenario, a deciduous tree from the edible group’s trees has the highest Ta reduction (1.02°C) from the base (without plant) and (0.0266°C) from the A scenario. Furthermore, the best function for shading trees is related to C1 mitigation of 0.8375°C from the base and 0.837°C from the A. The bushes are associated with the weakness function. It only reduced 0.02225°C from the base and 0.02275°C from the A. Finally, deciduous trees had a better process than evergreen trees. Deciduous trees’ average was reduced by 0.78°C and evergreen trees’ mitigation by 0.481°C from the A scenario, which is related to their characteristics in this climate condition (Figures 4, 5).
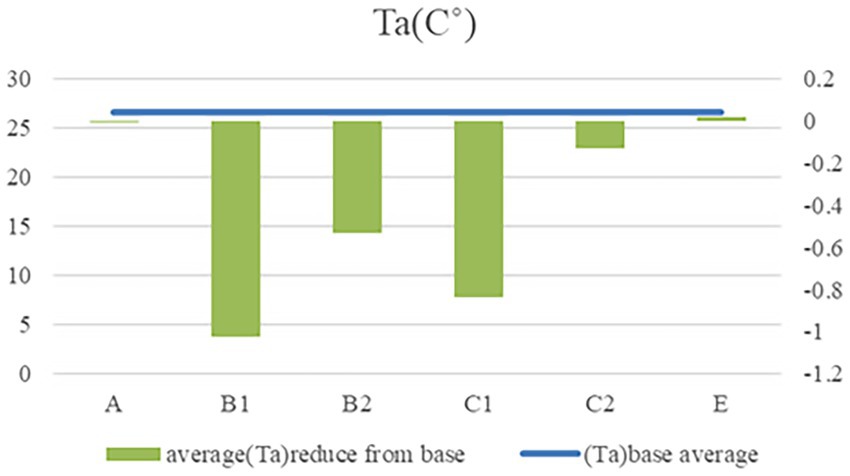
Figure 4. Plants’ effect reduces the temperature of the base (without plants), 1.8 m, 8 h (10:00–18:00) LST.
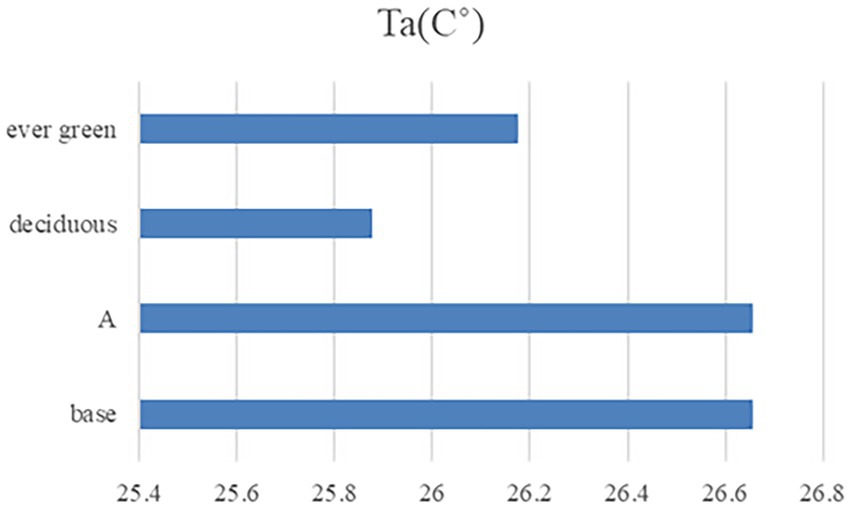
Figure 5. Comparison between deciduous and evergreen with the base [agriculture land simulation (vegetation) and without plants], 1.8 m, 8 h (10:00–18:00).
3.2. Thermal comfort (PMV, PET)
The simulation results show that people feel more comfortable in all situations when compared to a basic state [without plants and in scenario A (simulation agriculture land)]. In the B1 scenario, a deciduous tree from the edible group’s trees has the highest PMV index reduction (1.63) from the base (without plant) and (1.57) from the A scenario. In addition to that, among the shade trees, group C1 has a mitigation of 1.43 from the base and 1.37 from A. Shrubs are associated with the operation of weakness. It only reduced 0.063 from the base and 0.0044 from the A. The shrubs are associated with the function of weakness. It only reduced 0.063 from the base and 0.0044 from the A. The PMV of a group of participants is calculated using Fanger’s formulas for a given air temperature, mean radiant temperature, relative humidity, air velocity, metabolic rate, and clothing insulation. The comfort zone is computed by combining the six elements for which the PMV falls within the suggested values. The PMV is a seven-point scale that ranges from −3 (cold) to +3 (hot) (Zambrano et al., 2006; Rezaei Rad et al., 2021). Finally, deciduous trees function more effectively than evergreen trees. Deciduous trees mean an average reduction of (1.22), and evergreen trees mean mitigation of (2.104) from the A scenario. It is related to their characteristics in this climate condition (Figure 6). The general results of the PET (°C) were similar to those of the PMV (Abdi et al., 2020), as deciduous (B1) and evergreen (C1) trees performed better. Deciduous plants’ performance was better than evergreen trees in reducing the PET (°C). Among the shade trees, deciduous ones had better performance than evergreen ones. The mean average evergreen tree mitigation temperature (°C) was 2.98°C at the base and 2.55°C in the A scenario. Against this reduction, deciduous plants effectively functioned, 56.41% from the base and 55.53% from the A scenario. Moreover, shrubs performed poorly in mitigating PET (°C; Figure 7).
3.2.1. Combination scenario
The acquired data revealed the significance of planting crops in urban agriculture to rehabilitate (see Figure 8). Furthermore, it has been discovered that the simultaneous use of trees and grasses is more effective in reducing air temperatures and improving outdoor thermal comfort (Lobaccaro and Acero, 2015). Considering this theory and the region’s necessity, preparing the combination theory with the best-performing species (C1, B1, and A) made this scenario effectively function on mitigation Ta, PMV index, PPD (percent), PET, and also improved thermal comfort in the environment [The T (°C) was mitigated by 0.8106 (°C) from the base and 0.8101 (°C) from the A scenario (Figure 9)]. The PMV index was reduced by (1.42) from the base and (1.37) from the A scenario (Figure 10). It also affected PET (°C) in this plant’s design reduction, 5.2 (°C) from the base and 4.77 from the A scenario (Figure 11) 0.3.4 Field measurement and validation of the results of ENVI-MET.
Lee et al. (2014), Taleghani et al. (2014), Salata et al. (2016), Forouzandeh (2018), and López-Cabeza et al. (2022) validated the ENVI-Met model in research determined by comparing simulated values to field measurements. In addition, Taleghani et al. (2014), Morakinyo et al. (2017), Zhao and Fong (2017), Zhang L. et al. (2018), Zhang W. et al. (2018) validated the ENVI-Met model in research determined by comparing simulated values to field measurements. In addition, Morakinyo et al. (2017), Zhao and Fong (2017), Zhang L. et al. (2018), Zhang W. et al. (2018) measured and simulated relative humidity for validation purposes in their research. This study investigates the importance of urban agriculture land rehabilitation in managing urbanization challenges and mitigating heat island effects.
This location was chosen for simulation, and Ta and relative humidity were measured in the field. To examine the micrometeorological consequences of vegetation, simulations of circumstances with different configurations than the confirmed environment were used on June 30, 2020, from 10:00 a.m. to 18:00 p.m. at the receptor point (23, 23). El-Bardisy et al. (2016) and Sodoudi et al. (2018) used earlier research to assess the cooling potential of each of the planting patterns that were not present in this environment but were created as scenarios. To examine the micrometeorological consequences of vegetation, simulations of circumstances with different configurations than the confirmed environment were employed.
Eventually, for statistical validation of the ENVI-met model’s results, four quantitative measurements were computed: the coefficient of determination (r2), Willmott’s index of agreement (d), the values between 0 and 1, the mean absolute error (MAE), whose value must be between 0 and 1, and the root mean squared error (RMSE) that show the divergence between observed and simulated measures (Golden, 2004; Faragallah and Ragheb, 2022; Table 3).
D: Index of agreement [−].
MAE: Mean absolute error [−].
MBE: Mean bias error [−].
ND: Number of analyzed data [−].
Ō: Mean of the observed variable.
Oi; Observed variables for each instant j.
Pi: Model-predicted variables for each instant j.
4. Discussion
The micrometeorological simulation findings of this study suggest that planting trees in urban agriculture reduces Ta and improves outdoor thermal comfort conditions during the day, which influences micrometeorological conditions and thermal comfort in Tabriz, Iran, as a result of the juxtaposition of urban agriculture and urban texture. There has been limited research into the function of agricultural lands, and understanding how existing and future abandoned farmlands can contribute to social and environmental policy goals, as well as whether passive or active interventions are required, requires assessing how this trajectory unfolds (Heisler, 1989; Vailshery et al., 2013; Coutts et al., 2016; Sun et al., 2017; Zhang L. et al., 2018; Zhang W. et al., 2018; Nasrollahi et al., 2020).
complement the conclusions given here. According to the findings of this study, urban green space enhances thermal comfort. Jin et al. (2017) and Lee and Mayer (2018) assessed the impacts of urban green space on daytime outdoor heat stress in various locations. According to Munroe et al. (2013), Gallemore et al. (2018), and Fayet et al. (2022) agriculturally abandoned lands have the potential to result in a variety of outcomes, including re-cultivation and natural succession. The study’s conclusions showed that different planting strategies for trees, along with the use of native species, impact the reduction of the Ta and PMV indexes, improvement of the predicted percentage of dissatisfaction, and physiological equivalent temperature, with the various planting strategies having a positive impact.
4.1. Effect of a combination of plants, a decrease in on-air temperature (Ta), and thermal comfort enhancement on abandoned urban agricultural land
When the urbanization process increases, individuals re-adapt urban space to optimize their profitability, and certain economically more viable land uses may infiltrate surrounding rural regions. As a result, farmland near cities is frequently fragmented and less economical for farming (Grădinaru et al., 2015; Fayet et al., 2022). Individuals living in urban areas rely on natural ecosystems not only outside the city borders but also within the city limits, such as urban agriculture lands (Bolund and Hunhammar, 1999), which are positively associated with human well-being and comfort, particularly human physical and mental health, as proved by various epidemiological studies (Akbari, 2002; Tzoulas et al., 2007). Rehabilitating urban agricultural land has a lot of benefits, like providing opportunities to respond to biodiversity and other environmental policy goals.
These studies demonstrate the relevance of planting patterns in improving environmental thermal comfort and rehabilitation of urban agricultural land with prevalent crops. As a result, tree species for this study were chosen based on their nativeness to this region and divided into four groups focusing on different roles in this habitat: Shading trees include two types of species that require deciduous trees to operate well during the six hottest months of the year and coniferous trees to perform successfully during the six coldest months. In addition, wind direction and the shadowing of trees in various planting patterns cause alterations in the Ta and PMV indexes, as well as increased thermal comfort with the PET. The density and patterns of the plants were the same in all scenarios. Furthermore, the same cover tree planting pattern is a significant component in reducing the PET index; therefore, a combination pattern with the best-functioning plants is preferable to a one-species design. This study did not look at the number of rows, but the findings are in line with earlier studies on the importance of planting design and how it affects the environment’s thermal comfort and shading patterns. Shade trees are recommended in the summer, spring, and autumn but are useless in the winter, according to Hwang et al., 2011. As a result, they advocated for deciduous trees. Skelhorn et al. (2014) discovered that planting 5% of mature deciduous trees can reduce temperatures by 1°C. In addition, Cheung and Jim (2018) demonstrated that controlling solar radiation is the most significant component in enhancing thermal comfort during hot seasons. Where trees are planted, as shown by Yahia et al. (2018), the physiologically equivalent temperature (PET) is reduced by up to 14°C in specific places and by an average of 4°C across the overall area. The fundamental reality of lowering the temperature and increasing thermal comfort related to the tree canopy and tree arrangement leads to sensible heat reduction and temperature variation (Zhang L. et al., 2018; Zhang W. et al., 2018; Balany et al., 2020). According to the research, the use of urban green structures, particularly trees, helps to mitigate UHI by reducing air temperature, the amount of which varies depending on the specific environment. In this study, deciduous trees had the most significant influence on Ta reduction, while evergreen trees had the least. However, in this study, when compared to the base scenario, deciduous trees reduced air temperature by 0.78°C, the PMV index by 1.28, and PET by 4.70°C, while evergreen trees reduced air temperature by 0.48°C, the PMV index by 0.77, and PET by 2.90°C. The pattern of deciduous trees combined with evergreens and crops reduced the T, and this type of planting performed well in lowering the PMV and PET indexes, making people feel more at ease. Furthermore, the relative humidity in the environment was affected differently by evergreen and deciduous trees. The outcomes thus showed that evergreen and deciduous trees serve different purposes in various planting patterns with varying wind directions, with cooling performance varying in various tree rows. Although this study was intended to assess this effect during the summer, it should be noted that deciduous trees have a different impact on thermal comfort during the winter.
5. Conclusion
Urbanization is one of the most significant challenges facing developing countries, and its mismanagement can lead to many problems. Tabriz is one of Iran’s fastest-growing cities, and urbanization has caused the blending of agricultural and urban lands, resulting in the destruction of agricultural lands.
This research focuses on rehabilitating urban agricultural areas in Tabriz, Iran. It examines the impact of the number of tree species on climate characteristics and the arrangement of trees and crops on thermal comfort in a section of farms in Hokmabad, Tabriz’s urban agricultural land. The micro-meteorological model ENVI-met V4 was used to conduct numerical simulations for eight different scenarios, and the results showed that farmland trees had several benefits when used as deciduous plants that produce fruit, including mitigating Ta, PMV, and PET. Furthermore, deciduous tree species have a greater potential for reducing air temperatures than evergreen tree species, and changes in leaf and crown morphology, particularly crown volume, may result in species differences in altering the urban microclimate. The goal of this study is to demonstrate how agricultural lands that were previously utilized for farming have been rejuvenated and how incorporating trees (evergreen or deciduous) provides them with an ecological role. Consequently, the abandoned area and surrounding ecosystem will be revitalized. These findings can serve as a model for landscape architects and urban planners to improve their landscape layouts and designs, create a more sustainable city, rehabilitate urban agriculture areas, and manage regional micro-meteorology.
6. Limitation
The primary limitation of this research was the unavailability of the original version of the ENVI-Met software, requiring us to use the free version. As a result, it was not possible to evaluate and assess large portions of the city, and the simulation was limited to an average scale of 50 × 50 × 40.
Data availability statement
The original contributions presented in the study are included in the article/supplementary material, further inquiries can be directed to the corresponding author.
Author contributions
ZN is a Master’s student and AR her supervisor; both worked on all aspects of this paper. Both authors contributed to the article and approved the submitted version.
Conflict of interest
The authors declare that the research was conducted in the absence of any commercial or financial relationships that could be construed as a potential conflict of interest.
Publisher’s note
All claims expressed in this article are solely those of the authors and do not necessarily represent those of their affiliated organizations, or those of the publisher, the editors and the reviewers. Any product that may be evaluated in this article, or claim that may be made by its manufacturer, is not guaranteed or endorsed by the publisher.
References
Abdi, B., Hami, A., and Zarehaghi, D. (2020). Impact of small-scale tree planting patterns on outdoor cooling and thermal comfort. Sustain. Cities Soc. 56:102085. doi: 10.1016/j.scs.2020.102085
Ackerman, K., Conard, M., Culligan, P., Plunz, R., Sutto, M. P., and Whittinghill, L. (2014). Sustainable food systems for future cities: The potential of urban agriculture. Econ. Soc. Rev. 45, 189–206.
Akbari, H. (2002). Shade trees reduce building energy use and CO2 emissions from power plants. Environ. Pollut. 116, S119–S126. doi: 10.1016/S0269-7491(01)00264-0
Altieri, M. A. (1989). Agroecology: a new research and development paradigm for world agriculture. Agric. Ecosyst. Environ. 27, 37–46. doi: 10.1016/0167-8809(89)90070-4
Aslam, A., and Rana, I. A. (2022). The use of local climate zones in the urban environment: A systematic review of data sources, methods, and themes. Urban Clim. 42:101120. doi: 10.1016/j.uclim.2022.101120
Balany, F., Ng, A. W., Muttil, N., Muthukumaran, S., and Wong, M. S. (2020). Green infrastructure as an urban heat island mitigation strategy—a review. Water 12:3577. doi: 10.3390/w12123577
Bolund, P., and Hunhammar, S. (1999). Ecosystem services in urban areas. Ecol. Econ. 29:e301. doi: 10.1016/S0921-8009(99)00013-0
Breuste, J., and Rahimi, A. (2015). Many public urban parks, but who profits from them? The example of Tabriz. Iran. Ecol. Process. 4, 1–15. doi: 10.1186/s13717-014-0027-4
Bruse, M. (2004). ENVI-met 3.0: updated model overview. University of Bochum. Retrieved from: www. envi-met. com.
Burgin, S. (2018). Back to the future? Urban backyards and food self-sufficiency. Land Use Policy 78, 29–35. doi: 10.1016/j.landusepol.2018.06.012
Celis, A. M. C., and Frederico, C. (2018). Protocolo de elaboração de arquivo climático de cidades brasileiras para o software ENVI-met 4.0. Paranoá. 22, 32–50.
Cheung, P. K., and Jim, C. Y. (2018). Comparing the cooling effects of a tree and a concrete shelter using PET and UTCI. Build. Environ. 130, 49–61. doi: 10.1016/j.buildenv.2017.12.013
Coutts, A. M., White, E. C., Tapper, N. J., Beringer, J., and Livesley, S. J. (2016). Temperature and human thermal comfort effects of street trees across three contrasting street canyon environments. Theor. Appl. Climatol. 124, 55–68. doi: 10.1007/s00704-015-1409-y
Crank, P. J., Sailor, D. J., Ban-Weiss, G., and Taleghani, M. (2018). Evaluating the ENVI-met microscale model for suitability in analysis of targeted urban heat mitigation strategies. Urban Clim. 26, 188–197. doi: 10.1016/j.uclim.2018.09.002
De Dear, R. J. (1998). A global database of thermal comfort field experiments. ASHRAE Trans. 104:1141.
El-Bardisy, W. M., Fahmy, M., and El-Gohary, G. F. (2016). Climatic sensitive landscape design: towards a better microclimate through plantation in public schools, Cairo. Egypt. Proc. Soc. Behav. Sci. 216, 206–216. doi: 10.1016/j.sbspro.2015.12.029
Fabbri, K. (2013). Thermal comfort evaluation in kindergarten: PMV and PPD measurement through datalogger and questionnaire. Build. Environ. 68, 202–214. doi: 10.1016/j.buildenv.2013.07.002
Fanger, P. O. (1970). Thermal comfort. Analysis and applications in environmental engineering. New York: Sage
Faragallah, R. N., and Ragheb, R. A. (2022). Evaluation of thermal comfort and urban heat island through cool paving materials using ENVI-Met. Ain Shams Eng. J. 13:101609. doi: 10.1016/j.asej.2021.10.004
Fayet, C. M., Reilly, K. H., Van Ham, C., and Verburg, P. H. (2022). What is the future of abandoned agricultural lands? A systematic review of alternative trajectories in Europe. Land Use Policy 112:105833. doi: 10.1016/j.landusepol.2021.105833
Forouzandeh, A. (2018). Numerical modeling validation for the microclimate thermal condition of semi-closed courtyard spaces between buildings. Sustain. Cities Soc. 36, 327–345. doi: 10.1016/j.scs.2017.07.025
Gallemore, C., Munroe, D., and van Berkel, D. (2018). Rural-to-urban migration and the geography of absentee non-industrial private forest ownership: A case from southeast Ohio. Appl. Geogr. 96, 141–152. doi: 10.1016/j.apgeog.2018.05.010
Golden, J. S. (2004). The built environment induced urban heat island effect in rapidly urbanizing arid regions–a sustainable urban engineering complexity. Environ. Sci. 1, 321–349.
Grădinaru, S. R., Iojă, C. I., Onose, D. A., Gavrilidis, A. A., Pătru-Stupariu, I., Kienast, F., et al. (2015). Land abandonment as a precursor of built-up development at the sprawling periphery of former socialist cities. Ecol. Indic. 57, 305–313. doi: 10.1016/j.ecolind.2015.05.009
Gusson, C. S., and Duarte, D. H. (2016). Effects of built density and urban morphology on urban microclimate-calibration of the model ENVI-met V4 for the subtropical Sao Paulo, Brazil. Proc. Eng. 169, 2–10. doi: 10.1016/j.proeng.2016.10.001
Heisler, G. M. (1989). Tree plantings that save energy. Proceedings of 4th Urban Forestry Conference.
Herath, P., Thatcher, M., Jin, H., and Bai, X. (2021). Effectiveness of urban surface characteristics as mitigation strategies for the excessive summer heat in cities. Sustain. Cities Soc. 72:103072. doi: 10.1016/j.scs.2021.103072
Höppe, P. (2002). Different aspects of assessing indoor and outdoor thermal comfort. Energ. Buildings 34, 661–665. doi: 10.1016/S0378-7788(02)00017-8
Hosseinpour, N., Kazemi, F., and Mahdizadeh, H. (2022). A cost-benefit analysis of applying urban agriculture in sustainable park design. Land Use Policy 112:105834. doi: 10.1016/j.landusepol.2021.105834
Hwang, R. L., Lin, T. P., and Matzarakis, A. (2011). Seasonal effects of urban street shading on long-term outdoor thermal comfort. Build. Environ. 46, 863–870. doi: 10.1016/j.buildenv.2010.10.017
Jin, H., Siqi, L., and Jian, K. (2017). The thermal comfort of urban pedestrian street in the severe cold area of Northeast china. Energy Proc. 134, 741–748. doi: 10.1016/j.egypro.2017.09.571
Lee, H., and Mayer, H. (2016). Validation of the mean radiant temperature simulated by the Rayman software in urban environments. Int. J. Biometeorol. 60, 1775–1785. doi: 10.1007/s00484-016-1166-3
Lee, H., and Mayer, H. (2018). Thermal comfort of pedestrians in an urban street canyon is affected by increasing albedo of building walls. Int. J. Biometeorol. 62, 1199–1209. doi: 10.1007/s00484-018-1523-5
Lee, H., Mayer, H., and Schindler, D. (2014). Importance of 3-D radiant flux densities for outdoor human thermal comfort on clear-sky summer days in Freiburg Southwest Germany. Meteorologische Zeitschrift 23, 315–330. doi: 10.1127/0941-2948/2014/0536
Li, H., Lee, W. L., and Jia, J. (2016). Applying a novel extra-low temperature dedicated outdoor air system in office buildings for energy efficiency and thermal comfort. Energy Convers. Manag. 121, 162–173. doi: 10.1016/j.enconman.2016.05.036
Lin, T. P., Matzarakis, A., and Hwang, R. L. (2010). Shading effect on long-term outdoor thermal comfort. Build. Environ. 45, 213–221. doi: 10.1016/j.buildenv.2009.06.002
Lobaccaro, G., and Acero, J. A. (2015). Comparative analysis of green actions to improve outdoor thermal comfort inside typical urban street canyons. Urban Clim. 14, 251–267. doi: 10.1016/j.uclim.2015.10.002
López-Cabeza, V. P., Diz-Mellado, E., Rivera-Gómez, C., Galán-Marín, C., and Samuelson, H. W. (2022). Thermal comfort modelling and empirical validation of predicted air temperature in hot-summer Mediterranean courtyards. J. Build. Perform. Simul. 15, 39–61. doi: 10.1080/19401493.2021.2001571
Matzarakis, A., and Mayer, H. (2000). Atmospheric conditions and human thermal comfort in urban areas. In 11th Seminar on Environmental Protection Environment and Health.
Matzarakis, A., Mayer, H., and Iziomon, M. G. (1999). Applications of a universal thermal index: physiological equivalent temperature. Int. J. Biometeorol. 43, 76–84. doi: 10.1007/s004840050119
Matzarakis, A., Rutz, F., and Mayer, H. (2007). Modeling radiation fluxes in simple and complex environments—application of the Rayman model. Int. J. Biometeorol. 51, 323–334. doi: 10.1007/s00484-006-0061-8
McRae, I., Freedman, F., Rivera, A., Li, X., Dou, J., Cruz, I., et al. (2020). Integration of the WUDAPT, WRF, and ENVI-met models to simulate extreme daytime temperature mitigation strategies in San Jose California. Buil. Environ. 184:107180
Middel, A., Häb, K., Brazel, A. J., Martin, C. A., and Guhathakurta, S. (2014). Impact of urban form and design on mid-afternoon microclimate in Phoenix Local Climate Zones. Landsc. Urban Plan. 122, 16–28. doi: 10.1016/j.landurbplan.2013.11.004
Ministry Governorate of East Azerbaijan Province. (2008). Land use planning of the Tabriz County, National project. Azerbaijan: Ministry Governorate of East Azerbaijan Province.
Morakinyo, T. E., Kong, L., Lau, K. K. L., Yuan, C., and Ng, E. (2017). A study on the impact of shadow-cast and tree species on in-canyon and neighborhood's thermal comfort. Build. Environ. 115, 1–17. doi: 10.1016/j.buildenv.2017.01.005
Munroe, D. K., van Berkel, D. B., Verburg, P. H., and Olson, J. L. (2013). Alternative trajectories of land abandonment: causes, consequences and research challenges. Curr. Opin. Environ. Sustain. 5, 471–476. doi: 10.1016/j.cosust.2013.06.010
Nasrollahi, N., Ghosouri, A., Khodakarami, J., and Taleghani, M. (2020). Heat-mitigation strategies to improve pedestrian thermal comfort in urban environments: a review. Sustainability 12:10000. doi: 10.3390/su122310000
Nzimande, P. (2013). Does urban agriculture create job opportunities? ResearchGate 12:10000. doi: 10.13140/RG.2.2.33210.18882.
Partalidou, M., and Anthopoulou, T. (2017). Urban allotment gardens during precarious times: from motives to lived experiences. Sociol. Rural. 57, 211–228. doi: 10.1111/soru.12117
Peng, J., Liu, Z., Liu, Y., Hu, X., and Wang, A. (2015). Multifunctionality assessment of urban agriculture in Beijing City, China. Sci. Total Environ. 537, 343–351. doi: 10.1016/j.scitotenv.2015.07.136
Rad, H. R., Rafieian, M., and Sozer, H. (2017). Evaluating the effects of increasing of building height on land surface temperature. Int. J. Urban Manage Energy Sustain 1, 11–16.
Rahimi, A. (2016). A methodological approach to urban land-use change modeling using infill development pattern—a case study in Tabriz. Iran. Ecol. Process. 5:1. doi: 10.1186/s13717-016-0044-6
Raymond, C. M., Frantzeskaki, N., Kabisch, N., Berry, P., Breil, M., Nita, M. R., et al. (2017). A framework for assessing and implementing the co-benefits of nature-based solutions in urban areas. Environ. Sci. Pol. 77, 15–24. doi: 10.1016/j.envsci.2017.07.008
Rezaei Rad, H., Khodaee, Z., Ghiai, M. M., Tabe Arjmand, J., and El Haj Assad, M. (2021). The quantitative assessment of the effects of the morphology of urban complexes on the thermal comfort using the PMV/PPD model (a case study of Gheytariyeh neighborhood in Tehran). Int. J. Low Carb. Technol. 16, 672–682. doi: 10.1093/ijlct/ctaa100
Salata, F., Golasi, I., de Lieto Vollaro, R., and de Lieto Vollaro, A. (2016). Urban microclimate and outdoor thermal comfort. A proper procedure to fit ENVI-met simulation outputs to experimental data. Sustain. Cities Soc. 26, 318–343. doi: 10.1016/j.scs.2016.07.005
Sartison, K., and Artmann, M. (2020). Edible cities–An innovative nature-based solution for urban sustainability transformation? An explorative study of urban food production in German cities. Urban For. Urban Green. 49:126604. doi: 10.1016/j.ufug.2020.126604
Simon, H., Sinsel, T., and Bruse, M. (2020). Introduction of fractal-based tree digitalization and accurate in-canopy radiation transfer modelling to the microclimate model ENVI-met. Forests 11:869. doi: 10.3390/f11080869
Skelhorn, C., Lindley, S., and Levermore, G. (2014). The impact of vegetation types on air and surface temperatures in a temperate city: A fine scale assessment in Manchester, UK. Landsc. Urban Plan. 121, 129–140. doi: 10.1016/j.landurbplan.2013.09.012
Sodoudi, S., Zhang, H., Chi, X., Müller, F., and Li, H. (2018). The influence of spatial configuration of green areas on microclimate and thermal comfort. Urban For. Urban Green. 34, 85–96. doi: 10.1016/j.ufug.2018.06.002
Sun, L., Li, H., Dong, L., Fang, K., Ren, J., Geng, Y., et al. (2017). Eco-benefits assessment on urban industrial symbiosis based on material flows analysis and emergy evaluation approach: a case of Liuzhou city, China. Resour. Conserv. Recycl. 119, 78–88. doi: 10.1016/j.resconrec.2016.06.007
Taleghani, M., Tenpierik, M., van den Dobbelsteen, A., and Sailor, D. J. (2014). Heat in courtyards: A validated and calibrated parametric study of heat mitigation strategies for urban courtyards in the Netherlands. Sol. Energy 103, 108–124. doi: 10.1016/j.solener.2014.01.033
Targhi, M. Z., and Van Dessel, S. (2015). The potential contribution of urban developments to outdoor thermal comfort conditions: the influence of urban geometry and form in Worcester, Massachusetts, USA. Proc. Eng. 118, 1153–1161. doi: 10.1016/j.proeng.2015.08.457
Tsoka, S., Tsikaloudaki, A., and Theodosiou, T. (2018). Analyzing the ENVI-met microclimate model’s performance and assessing cool materials and urban vegetation applications–A review. Sustain. Cities Soc. 43, 55–76. doi: 10.1016/j.scs.2018.08.009
Tzoulas, K., Korpela, K., Venn, S., Ylipelkonen, V., Kazmierczak, A., Niemela, J., et al. (2007). Promoting ecosystem and human health in urban areas using green infrastructure: a literature review. Landsc. Urban Plan. 81:167e78. doi: 10.1016/j.landurbplan.2007.02.001
UNI. Ergonomia degli ambienti termici. Determinazione analitica e interpretazione del benessere termico mediante il calcolo degli indici PMV e PPD e dei criteri di benessere termico locale. Norma UNI EN ISO 7730 (2006). Milano: Ente Italiano di unificazione.
US EPA (US Environmental Protection Agency) (2008), Reducing Urban Heat Islands: Compendium of Strategies. California: Heat Island Reduction Program US EPA.
Vailshery, L. L., Madhumitha, J., and Harini, N. (2013). Effect of street trees on microclimate and air pollution in a tropical city. Urban Forest. Urban Green. 12, 408–415. doi: 10.1016/j.ufug.2013.03.002
Watson, D. (1983). Climatic design: Energy-efficient building principles and practices New York: McGraw-Hill.
Wimalarathne, H. D. A., and Perera, P. C. D. (2019). Potentials of water hyacinth as livestock feed in Sri Lanka. Ind. J. Weed Sci. 51, 101–105. doi: 10.5958/0974-8164.2019.00024.8
Wu, H., Wang, T., Riemer, N., Chen, P., Li, M., and Li, S. (2017). Urban heat island impacted by fine particles in Nanjing China. Sci. Rep. 7:11422.
Yahia, M. W., Johansson, E., Thorsson, S., Lindberg, F., and Rasmussen, M. I. (2018). Effect of urban design on microclimate and thermal comfort outdoors in warm-humid Dar es Salaam Tanzania. Int. J. Biometeorol. 62, 373–385. doi: 10.1007/s00484-017-1380-7
Zambrano, L., Malafaia, C., and Bastos, L. E. (2006) Thermal comfort evaluation in outdoor space of tropical humid climate. In 23rd Conference on Passive and Low Energy Architecture. Geneva, Switzerland.
Zhang, W., Hu, W., and Wen, Y. (2018). Thermal comfort modeling for smart buildings: a fine-grained deep learning approach. IEEE Internet Things J. 6, 2540–2549.
Zhang, L., Zhan, Q., and Lan, Y. (2018). Effects of the tree distribution and species on outdoor environment conditions in hot summer and cold winter zone: a case study in Wuhan residential quarters. Build. Environ. 130, 27–39. doi: 10.1016/j.buildenv.2017.12.014
Keywords: thermal comfort, EVVI-MET, abandoned land, urban agriculture, planting scenarios
Citation: Rahimi A and Nobar Z (2023) The impact of planting scenarios on agricultural productivity and thermal comfort in urban agriculture land (case study: Tabriz, Iran). Front. Ecol. Evol. 11:1048092. doi: 10.3389/fevo.2023.1048092
Edited by:
Jorge Doña, University of Illinois at Urbana–Champaign, United StatesReviewed by:
Salman Shooshtarian, RMIT University, AustraliaIoan Cristian Ioja, University of Bucharest, Romania
Copyright © 2023 Rahimi and Nobar. This is an open-access article distributed under the terms of the Creative Commons Attribution License (CC BY). The use, distribution or reproduction in other forums is permitted, provided the original author(s) and the copyright owner(s) are credited and that the original publication in this journal is cited, in accordance with accepted academic practice. No use, distribution or reproduction is permitted which does not comply with these terms.
*Correspondence: Akbar Rahimi, YWtiYXIucmFoaW1pQHRhYnJpenUuYWMuaXI=; YWtiYXIucmFoaW1pQGdtYWlsLmNvbQ==