- 1Institut Català de Paleoecologia Humana i Evolució Social (IPHES-CERCA), Tarragona, Spain
- 2Departament d’Història i Història de l’Art, Universitat Rovira i Virgili (URV), Tarragona, Spain
- 3Departament de Biologia Evolutiva, Ecologia i Ciències Ambientals, Secció de Zoologia i Antropologia Biològica, Universitat de Barcelona, Barcelona, Spain
- 4Institut d’Arqueologia de la Universitat de Barcelona, Barcelona, Spain
- 5Faculté des Sciences, Département de Géologie, Université Mohamed Premier, Oujda, Morocco
- 6Institució Catalana de Recerca i Estudis Avançats (ICREA), Barcelona, Spain
- 7Department of Prehistory, Autonomous University of Barcelona (UAB), Bellaterra, Spain
- 8Archaeology of Social Dynamics (ASD), Institución Milá y Fontanals (IMF), Spanish National Research Council (CSIC), Barcelona, Spain
- 9Instituto de Evolución en África (IDEA, Madrid), Madrid, Spain
- 10Consejo Superior de Investigaciones Científicas (CSIC), Museo Nacional de Ciencias Naturales, Departamento de Paleobiología, Madrid, Spain
- 11Institut National des Sciences de l’Archéologie et du Patrimoine (INSAP), Rabat, Morocco
- 12UMR 7194 - Histoire Naturelle de l’Homme Préhistorique (CNRS/MNHN/UPVD), Paris, France
The genus Macaca belongs to Cercopithecidae (Old World monkeys), Cercopithecinae, Papionini. The presence of Macaca in North Africa is well known from the Late Miocene to the Late Pleistocene. However, the diet of fossil Macaca has been poorly described in the literature. In this study, we investigated the feeding habits of Macaca cf. sylvanus (n = 4) from the Plio-Pleistocene site Guefaït-4.2 in eastern Morocco through multiproxy analysis combining analyses of stable carbon and oxygen isotopes from tooth enamel, buccal microtexture, and low-magnification occlusal dental microwear. For both microwear analyses, we compared the macaques with a new reference collection of extant members of Cercopithecoidea. Our occlusal microwear results show for the fossil macaque a pattern similar to the extant Cercocebus atys and Lophocebus albigena, African forest-dwelling species that are characterized by a durophagous diet based mainly on hard fruit and seed intake. Buccal microtexture results also suggest the consumption of some grasses and the exploitation of more open habitats, similar to that observed in Theropithecus gelada. The δ13C of M. cf. sylvanus indicates a C3 based-diet without the presence of C4 plants typical of the savanna grassland in eastern Africa during this period. The high δ18O values of M. cf. sylvanus, compared with the contemporary ungulates recovered from Guefaït-4.2, could be associated with the consumption of a different resource by the primate such as leaves or fresh fruits from the upper part of trees. The complementarity of these methods allows for a dietary reconstruction covering a large part of the individual’s life.
Introduction
Modern Cercopithecoidea primates inhabit a wide range of habitats, including tropical forests, high-altitude woodlands, and savanna grasslands (Jablonski et al., 2000). This adaptability across a range of environments began with a radiation within and beyond Africa, probably due to the increasingly open and fragmented environments of the Late Miocene and the Plio-Pleistocene (Elton, 2007; Hughes et al., 2008; Roos et al., 2019). For example, the closing of the Tethys Ocean and subsequent aridification of northern Africa (Zhang et al., 2014), and fluctuations in Milankovitch cycles (DeMenocal, 1995), formed intermittent biogeographical barriers. It is hypothesized that these ecological barriers diversified Cercopithecoidea, specifically the Papionini tribe, into different genera. Theropithecus adapted to wet lowlands, Papio, Cercocebus, Lophocebus, and Mandrillus filled niches within more forested environments, while Macaca became more-generalized and settled a wide variety of habitats within higher latitudes (Delson, 1980). Paleontological and molecular evidence indicates that the macaque lineage originated during the Miocene in Africa and diverged from other African Papionini around 7–5.5 Ma (Delson, 1980; Tosi et al., 2005; Roos et al., 2019). According to the fossil record, macaques dispersed throughout Eurasia during the Late Miocene, inhabiting primarily the Mediterranean region 6–5 Ma (Alba et al., 2014; Piñero et al., 2017) and then in the Late Pliocene, eastern Asia (~4 Ma; Szalay and Delson, 1979; Elton, 2007; Meloro and Elton, 2012). In this radiation, macaques experienced a rapid diversification into several species (Elton and O’Regan, 2014; Roos et al., 2019). The genus Macaca consists of approximately 23 extant species and occupies more diverse ecological environments and geographical ranges (from 10° south to over 40° north latitude) than any other genus of nonhuman primates. In fact, the expansion of this genus in Africa and Eurasia is one of the most successful among primates (Jablonski, 2002; Jablonski and Frost, 2010; Fleagle, 2013; Roos et al., 2019), and its ecological distribution and adaptative capacity reflect great dietary diversity and interspecific behavioral responses to seasonality (Kato et al., 2014).
The oldest macaque fossils in Africa have been found in the Miocene paleontological sites of Menacer (Algeria, Macaca sp.; Arambourg, 1959) and Wadi Natrum (Egypt, Macaca lybica; Stromer, 1913, 1920). Other Macaca fossil remains have been recorded in the Pliocene Tunisian sites of Garaet Ichkeul (Szalay and Delson, 1979; Delson, 1993; Alemseged and Geraads, 1998) and Aïn Brimba (Arambourg and Coque, 1959; Arambourg, 1979; Szalay and Delson, 1979). Contemporaneous to the remains of Macaca cf. sylvanus from the Guefaït-4.2 (GFT-4.2) site in eastern Morocco analyzed in this work, fossil remains of Macaca sp. have been found in the Plio-Pleistocene site of Ahl al Oughlam in western Morocco (Geraads, 2006). Macaca sylvanus has also been described from the Late Pleistocene sites of Chrafate and Ez Zarka in Morocco (Ouahbi et al., 2001; Fooden, 2007), and in the Middle and Late Pleistocene sites of Traras, Monts des Nedroma, Afalou bou Rhummel, and Tamar Hat in Algeria (Pomel, 1892; Joleaud, 1926; Delson, 1974; Szalay and Delson, 1979; Geraads, 1987; Fooden, 2007).
At present, there is only one species of the genus Macaca in Africa, M. sylvanus (Barbary macaques) from Algeria (Chiffa, Tigounatine, Icetcifère, Akfadou, and Kherrata) and Morocco (High Atlas, Middle Atlas, and Rif; Fooden, 2007). Field observations have defined feeding habits as varied and to include an important seasonal component, with diet consisting of more than 100 species of plants, fungi, lichens, mosses, and animal prey (Deag, 1983; Ménard, 1985; Ménard and Vallet, 1986). Studies that have taken into account the annual composition of the diet indicate that seeds, leaves, and herbs are the main foods of Barbary macaques in Akfadou and Tigounatine (Algeria; Ménard, 1985; Ménard and Vallet, 1986, 1996). However, in the Rif mountains of northern Morocco, more than 50% of their diet is based on seeds (Mehlman, 1988), and some studies of the populations that inhabit the Middle Atlas suggest that fruits could constitute a significant part of their diet (Deag, 1983; Drucker, 1984; Ménard and Mohamed, 1999), demonstrating high intra-species local variation at the population level. Nevertheless, the diet of fossil M. cf. sylvanus is virtually unknown, with the exception of a study on dental microwear of Macaca sp. from the Pliocene of northwestern China, which suggests a hard-object feeder or extractive forager, without grass consumption (Williams and Holmes, 2011).
Previous work on the feeding habits of African Plio-Pleistocene Cercopithecidae, through analysis of stable isotopes and dental microwear, have mainly focused on Eastern Africa (Teaford, 1993; Ungar and Teaford, 1996; Cerling et al., 2011, 2013a,b; Sponheimer et al., 2013; Levin et al., 2015; Shapiro et al., 2016; Robinson et al., 2017; Ungar et al., 2017; Martin et al., 2018; Souron, 2018; Manthi et al., 2020; Merceron et al., 2021) and Southern Africa (Lee-Thorp et al., 1989, 2010; Daegling and Grine, 1999; Codron et al., 2005; El-Zaatari et al., 2005; Scott et al., 2005; Fourie et al., 2008; Williams and Patterson, 2010; Williams and Holmes, 2011; Sponheimer et al., 2013; Grine et al., 2020). In these studies, the most represented genera are Theropithecus, Parapapio, and Papio, among which there is a consensus on the predominance of a graminivore diet with a high component of C4-based resources for Theropithecus (Lee-Thorp et al., 1989; Cerling et al., 2013a; Levin et al., 2015; Souron, 2018) and more diverse food intake with a proportion of C3 plants in the diet of Parapapio and Papio (Lee-Thorp et al., 1989; Codron et al., 2005; Fourie et al., 2008; Williams and Patterson, 2010; Levin et al., 2015). In Northern Africa, analysis of the feeding behavior of Theropithecus oswaldi leakeyi (Jolly, 1972; Delson, 1993) from Tighennif (ca. 1 Ma) and Theropithecus atlanticus (Alemseged and Geraads, 1998) from Ahl al Oughlam (ca. 2.5 Ma) suggest a different pattern in carbon values, with a clear domination of C3 plants, although other authors suggest bulk-feeding graminivory (Fannin et al., 2021).
Many cercopithecoid fossils have been recovered from the same sites where Hominin fossils have been found (Delson, 1980). The genus Homo experienced a similar out-of-Africa dispersion during the Pleistocene and inhabited the same ecosystems as members of Papionini. Therefore, both lineages had to confront the same climatic constrictions and habitat partitioning. Understanding the ecology of the fossil Cercopithecoidea primates is of interest for interpreting the paleoecology and niche partitioning of both groups. Consequently, we aimed to analyze the feeding strategies of M. cf. sylvanus from GFT-4.2 on different timescales using, for the first time, a multi-proxy approach. On the one hand, the isotopic signal (δ18O and δ13C) of tooth enamel bioapatite provides dietary information during the first years of the animal’s life following the tooth mineralization times proposed by Sirianni and Swindler (1985) for the extant Macaca nemestrina. On the other hand, dental microwear analysis, combining buccal (microtexture analysis) and occlusal (low magnification) surfaces, provides dietary information over the long term (weeks to months; Romero et al., 2012; Hernando et al., 2021) and short term (last days; Semprebon et al., 2004), respectively, but always toward the end of the animal’s life. Occlusal microwear studies in primates have suggested that mechanical properties of food items are the primary cause of different microwear pattern among primates. For instance, primates that incorporate hard objects, such as seeds and nuts, have more pitted surfaces than folivores primates that exhibit heavily scratched areas (Ungar, 1996; Scott et al., 2006). Occlusal microwear has a short-term turnover therefore, occlusal microwear patterns provide information on the nature of food intake during the last days prior to death (Teaford and Oyen, 1989). On the other hand, buccal surfaces should only interact with the food items consumed while they are being processed in the oral cavity (Ungar and Teaford, 1996; Romero et al., 2012), and experimental studies have shown that the buccal pattern is more stable through time, at least in the absence of significant dietary shift (Romero et al., 2012).
We hypothesize that combining occlusal and buccal microwear analyses will strengthen the dietary discrimination among these species. Based on the dietary information of extant M. sylvanus from Morocco and Algeria, we expect, for the GFT-4.2 specimens, a buccal and occlusal pattern similar to folivore-frugivore primates with the incorporation of seeds during the dry season. In the case of stable carbon and oxygen isotopes, we expect values similar to those of the Theropithecus from Ahl al Oughlam (Morocco). That is, a predominance of C3 plants in the diet and high oxygen values in relation to ungulates. To compare the dental microwear of M. cf. sylvanus from GFT-4.2, we present a new reference collection of wild modern cercopithecines including the same individuals for both microwear methods. The integration of the data obtained from these three high-resolution techniques has allowed us to obtain a more precise and robust picture of the feeding habits of M. cf. sylvanus from GFT-4.2.
Site: GUEFAÏT-4.2
An interdisciplinary Spanish–Moroccan project started in 2006 as an international cooperation between the Université Mohamed Premier Oujda (UMP, Eastern Morocco) and the Catalan Institute for Human Palaeoecology and Social Evolution from Tarragona (IPHES-CERCA, Catalonia, Spain). The aim of this cooperation is to establish the geochronological and archeo-paleontological context of the Aïn Beni Mathar/Guefaït fluvio-lacustrine basin, in the northern part of the High Plateaus (Jerada Province, Eastern Morocco; Aouraghe et al., 2016; Chacón et al., 2016; Sala-Ramos et al., 2022). One of the systematic surveys in the region revealed the fossiliferous level of Guefaït-4. This horizon yielded a rich and diverse faunal assemblage of vertebrates, which was systematically excavated at the GFT-4.2 site (reptiles, amphibians, and both small and large mammals), including the macaque fossil remains analyzed in this work (Alba et al., 2021). The fossil remains were concentrated in a palustrine level constituted of clay and marls, at the base of Unit 2, within the Dhar Iroumyane stratigraphic section (Aouraghe et al., 2019b). The fieldwork carried out between 2017 and 2019 recovered more than 3,000 fossil remains in an excavated area of 28 m2. Biostratigraphic correlations with other sites in Northern Africa, such as the lower levels of the Ain Boucherit site in Algeria (Sahnouni et al., 2018) and Ahl al Oughlam in Morocco (Geraads, 2006), suggest an age close to the Plio-Pleistocene boundary (2.58 Ma). The absence of the genus Equus (despite the abundance of Hipparion equid remains; Aouraghe et al., 2019a,b) indicates an age older than ~2.4 Ma (Sahnouni et al., 2018). This chronological limit is supported by the study of the murid assemblage (Agustí et al., 2017; Piñero et al., 2019), the carnivore assemblage (Madurell-Malapeira et al., 2021), and preliminary paleomagnetism studies (Parés et al., 2020).
Materials
A total of six teeth of M. cf. sylvanus were recovered from GFT-4.2 during the fieldwork in 2018 and 2019 (Alba et al., 2021). The materials are housed in the Faculty of Science, Mohammed I University in Oujda, Morocco. We discarded two of them for the final analysis because they correspond to dental germ fragments (GFT4.2018-1-P13-191, right M2 or M3, and GFT4.2019-1-R15-45, right M2).
The studied teeth (n = 4) correspond to a left upper central incisor (I1, GFT4.2018-1-Q14-70), a right lower first molar (M1, the lingual crown fragment of GFT4.2018-1-Q14-13), a right second lower molar (M2, GFT4.2019-1-S15-65), and a left lower third molar (M3, GFT4.2019-1-R13-63). All four available fossil teeth (M1, M2, M3, and I1) were sampled for stable isotope analysis, three (M1—GFT140, M2—GFT138, and M3—GFT137) were sampled for buccal dental microtexture analysis, and two (M1—GFT140 and M2—GFT138) were samples for occlusal dental microwear analysis. M3 was discarded for the occlusal dental microwear analysis due to the absence of wear facets on this surface. Metrical comparisons suggests that the incisor would belong to a different individual than the lower molars. However, it cannot be ruled out that the lower molars were from the same individual (Alba et al., 2021).
The extant Cercopithecoidea comparative sample for the buccal microtexture and occlusal microwear analyses correspond to a total of 41 well-preserved left second lower molars (M2; Supplementary Table 1). We excluded 10 samples from occlusal microwear analysis and 12 samples from buccal microtexture due to bad preservation or other taphonomic defects on the analyzed surface. The selected species have well-defined habitat and dietary preferences and include five extant species (Theropithecus gelada, Mandrillus sphinx, Lophocebus albigena, Cercocebus atys, and Macaca mulatta). Whenever possible, we chose specimens of the same taxa from close localities to minimize the variability of their dietary regime. We selected the extant species based on the criteria of phylogenetic affinity (Perelman et al., 2011) and the dental morphology that characterizes all cercopithecines, namely bilophodont molars (Kay and Hylander, 1978; Monson and Hlusko, 2014). This comparative sample served to establish a broad spectrum of ecological and dietary diversity.
1. Theropithecus gelada specimens from Ethiopia have a plant-based dietary regime (Dunbar and Bose, 1991), with more than 80% consisting of blades (Mau et al., 2009). However, those T. gelada living on the Guassa Plateau also incorporate large quantities of forbs into their diet (Fashing et al., 2014), and are often selective of tender forbs that are rare on the plateau (Souron, 2018).
2. Mandrillus sphinx is a frugivore primate that usually lives in gallery forest. Fruits, supplemented with various plant parts like leaves and flowers, constitute the Mandrillus diet. However, when fruit is scarce due to seasonality, M. sphinx fallback on mechanically challenging items like seeds and hard-shell fruits from the forest floor (Hoshino, 1985; Lahm, 1986; Tutin et al., 1997; Powell et al., 2017).
3. Cercocebus atys forages on the forest’s floor and shrub layers, relying on hard-shelled nuts like Sacoglottis gabonensis year-round. Yet, due to seasonal fluctuations, the total contribution of S. gabonensis can range anywhere from 19% to 80% of the monthly diet (Daegling et al., 2011; McGraw et al., 2014).
4. Lophocebus albigena is classified as a hard object feeder (Lambert et al., 2004; McGraw et al., 2011) that consumes a significant portion of hard seeds and nuts (Lambert et al., 2004). In actuality, due to seasonal fluctuations, L. albigena is a generalist that consumes a high (40%) percentage of soft ripe fruit (Lambert et al., 2004) in addition to seeds, flowers and young leaves (Poulsen et al., 2001). During seasons with low fruit availability, L. albigena will eat bark that, although hard, is rich in protein and soluble sugars (Rogers et al., 1994; Olupot, 1998). This fall-back feeding strategy has also been described for Barbary macaques (Macaca sylvanus; Ménard and Mohamed, 1999).
5. Macaca mulatta has the largest geographical distribution with its ranges spanning from Afghanistan to India, Pakistan, China, Thailand and Vietnam, among others (Sengupta et al., 2014). Its wide distribution reflects highly opportunistic feeding strategy, even though fruits may constitute as much as 70% of their diet in some regions (Fooden, 2007), yet less than 9% in other ecosystems, such as Pakistan (Goldstein and Richard, 1989). They are semi-terrestrial primates that can also feed up with species that are in different strata of the forest (Albert et al., 2013).
We have not included extant M. sylvanus specimens because they have a narrow distribution, and some populations are fed by humans like those of Gibraltar (Spain). Therefore, the baseline samples included in the present study consist of wild specimens with well-defined diet and habitat. To contextualize the oxygen and carbon values of M. cf. sylvanus, we have also included contemporaneous herbivore samples from GFT-4.2 corresponding to the same paleontological level 1. These samples include the remains of Tragelaphini (n = 4), Gazella sp. (n = 4), and Hipparion sp. (n = 4). The list of specimens sampled and analyzed and the raw data for stable isotope analysis are provided in Supplementary Table 2.
Methods
Stable isotope analysis
Tooth surfaces were cleaned with a tungsten abrasive drill bit to remove any adhering external material. Enamel powder for bulk isotope analysis was then obtained using a diamond-tipped drill, passed along the full length of the buccal surface to ensure a representative measurement for the entire period of enamel formation.
Powdered enamel samples (2–6 mg) were chemically treated at the Biomolecular Laboratory of IPHES. Chemical treatment of samples was based on protocols originally proposed by Koch et al. (1997) and later modified by Tornero et al. (2013). The samples were treated for 4 h in 0.1 M acetic acid [CH3COOH] (0.1 mL solution/0.1 mg of sample), neutralized with distilled water, and freeze-dried.
The δ18O and δ13C values of tooth enamel bioapatite were measured using an automated carbonate preparation device (KIEL-III) coupled to a gas-ratio mass spectrometer (Finnigan MAT 252) at the Environmental Isotope Laboratory, University of Arizona, United States. Samples were reacted with dehydrated phosphoric acid under a vacuum at 70°C. The accuracy and precision of the measurements were checked and calibrated using the calcium carbonate international standards (NBS-19 and NBS-18). Replicate measurements of the standards during analysis had errors of ±0.1‰ for δ18O and ±0.08‰ for δ13C (1 sigma). Carbon isotope composition is reported in δ notation, where δ13C = [(Rsample/Rstandard) – 1], R = 13C/12C, and δ is expressed as per mil notation (‰). Carbon and oxygen values are reported relative to PDB (Vienna Pee Dee Belemnite).
In terrestrial ecosystems there is a marked isotopic distinction between the two photosynthetic pathways in plants, C3 (Calvin cycle) and C4 (Hatch-Slack cycle), which differ mainly in their discrimination against 13C during the fixation of CO2 (Farquhar et al., 1989). Modern plants that use the C3 photosynthetic pathway (including most trees, woody shrubs, bushes, herbs and temperate grasses) have an average isotopic composition of −28.5‰ (global range −20 to −37‰), with −23‰ as the maximum value recommended for typical C3 plants and a cutoff of −31.5‰ for closed-canopy forests (Kohn, 2010). Due to the fact that we discarded the presence of that environment in GFT-4.2. For this study, we use the average δ13C value of −27‰ for modern C3 plants, excluding analyses from the understory of closed-canopy forests below −31.5‰ (Kohn, 2010). Modern plants that use the C4 photosynthetic pathway (primarily tropical grasses and sedges) have an average isotopic composition of −12‰ (global range −10 to −14‰; Cerling and Harris, 1999; Kohn and Cerling, 2002). Crassulacean Acid Metabolism (CAM) plants can have δ13C values corresponding to both C3 and C4 plants. However, such plants do not seem to be consumed by primates (Cerling et al., 2013a). The δ13C values of atmospheric CO2 have fluctuated over time and this impacts δ13C values in plants (Fiedli et al., 1986; Keeling et al., 2017). We have corrected by ~ +1.5 the δ13C values of modern plants, due to the difference between the pre-Industrial δ13CCO2 value of ~ −6.5‰ established for the Plio-Pleistocene (Tipple et al., 2010) and the δ13CCO2 value of ~ −8‰ corresponding to the time at which the plants were collected (Kohn, 2010). In this paper, we have used the enamel-diet enrichment factor of +11.8 ± 0.3‰ calculated for chimpanzees (Pan troglodytes) at Ngogo in Kibale National Park in Uganda for macaques (Malone et al., 2021) and the enamel-diet enrichment factor of +14.1 ± 0.5‰ for ungulates (Cerling and Harris, 1999; Passey et al., 2005) to interpret tooth enamel δ13C values and estimate potential consumption of C3- or C4-plant. These isotopic enrichment factor values for C3 and C4 plants are assumed to provide tooth enamel values of < −18.2‰ for closed canopy diets, < −13.7‰ for pure C3 diets, < −9.7‰ for C3-dominated diets, from −9.7 to −0.7‰ for C3–C4 mixed feeders, >−0.7‰ for C4-dominated diets and > +1.3‰ for pure C4 diets in primates and tooth enamel values of < −15.9‰ for closed canopy diets, < −11.4‰ for pure C3 diets, < −7.4‰ for C3-dominated diets, from −7.4 to +1.6‰ for C3–C4 mixed feeders, > +1.6‰ for C4-dominated diets and > +3.6‰ for pure C4 diets in ungulates.
The δ18O values in enamel bioapatite is directly related to the drinking water (i.e., springs, rivers, lakes, water holes), plant/food water (i.e., leaves, grass, fruit) and metabolic water by reaction with carbohydrates. Consequently, the precipitation, altitude, humidity/aridity and local temperatures will have an effect on the δ18O of locally available water and food (Longinelli, 1984; Luz and Kolodny, 1985; Bryant and Froelich, 1995; Pederzani and Britton, 2019). Oxygen values can also vary within the same habitat, especially in tropical forest environments, depending on where the species forages. Species feeding in the upper part of the canopy tend to have higher δ18O values as opposed to species feeding in the understory which tend to have lower δ18O values (Krigbaum et al., 2013; Crowley, 2014; Fannin and McGraw, 2020).
The approximate initial and final mineralization estimated for the extant Macaca nemestrina (Sirianni and Swindler, 1985), in relation to the teeth analyzed in fossil M. cf. sylvanus, is ca. birth to 1 year for M1, I1 ca. 1 to 2 years for I1, ca. 1 to 2.5 years for M2 and ca. 3 to 4 years for M3. Related to dental development, the only permanent tooth to begin development prenatally in the non-human primates is the first molar, which may have one to three cusps mineralized at birth and a complete development of the crown for about 1 year (Sirianni and Swindler, 1985). Relatively recent studies in Macaca mulatta suggest that the longest weaning periods are ca. 10 months and the shortest weaning period, between 2 and 5 months (Reitsema et al., 2015). Considering the data on nursing and mineralization timing in extant Macaca, we assume that the I1, M2 and M3 enamel apatite should reflect a post-weaning signal and the M1 enamel apatite could therefore reflect a pre-weaning signal for the M. cf. sylvanus from GFT-4.2. This allows us to reconstruct the diet from birth to approximately 4 years of age.
The bivariate graphs were made with the Past 4.02 software (Hammer et al., 2001).
Occlusal microwear analysis (low magnification)
Enamel microwear features were observed via standard light microscopy using a Zeiss Stemi 2000C stereomicroscope at 35× magnification on high-resolution epoxy casts of teeth, following the cleaning, molding, casting, and examination protocol developed by Solounias and Semprebon (2002) and Semprebon et al. (2004). We used the wear facets of the mesiobuccal cusp of M2 (normally the paracone of M2). Before molding, the occlusal surface of each specimen was cleaned using acetone and then 96% ethanol. The surface was molded using high-resolution silicone (Heraeus Kulzer, PROVIL novo Vinylpolysiloxane, Light C.D. 2 regular set), and transparent casts were created using clear epoxy resin (C.T.S. Spain, EPO 150 + K151). A standard 0.16 mm2 ocular reticle was employed to quantify the number of small pits (round scars—relatively shallow, refract light easily and consequently appear bright and shiny) and large pits (round scars—deeper, wider, and consequently less refractive), coarse scratches (elongated scars with parallel sides—narrow, and barely etched into the enamel surfaces) and fine scratches (elongated scars with parallel sides—wider and more obviously etched into the enamel surface), gouges (features with irregular edges and much larger and deeper than large pits), puncture pits (craterlike features with regular margins), and cross scratches (oriented perpendicularly to the majority of scratches; Supplementary Figure 1). The scratch width score (SWS) was obtained by giving a score of “0” to teeth with predominantly fine scratches per tooth surface, “1” to those with a mixture of fine and coarse types of textures, and “2” to those with predominantly coarse scratches. We took microphotographs using a Blackfly S digital camera and the Kivy Mic Capture Z software. We used the Helicon Focus 7 software to merge images from various focal planes and to produce a single image with a greater depth of field. We added scale bars using ImageJ. We compared the quantitative results with the reference dataset of the extant Cercopithecidae species. A single observer (IRP) analyzed all specimens to avoid inter-observer errors. We used the Addinsoft™ XLSTAT-3.02 statistical packages to generate the linear discriminant analysis (LDA) and the correspondence analysis (CA).
Buccal microtexture analysis
The original specimens (Supplementary Table 1) were cleaned with acetone and alcohol-soaked cotton swabs, and vinyl impressions were made using President’s Jet Regular Body (Coltène-Whaledent). Positive casts were made with Ferropur (Feroca®) polyurethane (Martínez et al., 2022). Buccal areas were obtained using a Sensofar Plu Neox laser scanning confocal microscope, with a 20× (0.45NA) objective, a spatial sampling of 0.83 μm, an optical resolution of 0.31 μm, a vertical resolution of 20 nm, and a z-step interval of 1 μm (Ibáñez et al., 2020; Martínez et al., 2022). Buccal surfaces were placed perpendicular to the objective in a horizontal position and an image of the field of view of the microscope (600 μm × 500 μm) was obtained (see 3D photosimulations in Supplementary Figure 3). From this image, four subsamples of 138 μm × 102 μm (a standard area in DMTA studies) were cropped using the Mountain 7® software from Digital Surf.1 We have tested the S-filter prior to the obtention of the 38 parameters, following (Winkler et al., 2022). The workflow included leveling the surface to remove plane inclination, eliminating the curvature applying a 2nd degree polynomial, removing the anomalous points by applying upper and lower 0.5% (a total of 1% of threshold values), following the threshold operator included in the same software and applying a Spatial filter (Gaussian 3 × 3). The Gaussian filter was applied because is widely used for technical surfaces and was applied after the trimming because it is sensitive to outliers (Schulz et al., 2013). Surface roughness was later quantified according to 38 ISO 25178/ISO 12781 parameters (Martínez et al., 2022; Supplementary Table 5).
To standardize the measured area on the buccal surface, we calculated the median of the parameters derived from the several (one to four) measurements of a single buccal surface (Scott et al., 2006; Calandra et al., 2012; Martínez et al., 2022), and used the median per specimen for analysis. We used log-transformed texture data for analyses because some texture parameters have non-normal distributions (Shapiro–Wilk, p < 0.05). To ensure we did not exclude any parameter (e.g., Ssk has negative values), we log transformed the data as log [1 + parameter] (Supplementary Table 6). We identified the outliers for both original and log-transformed variables according to their Studentized residual values (absolute value > 3) and removed them from the final data set (Schulz et al., 2013; Martínez et al., 2022). To test the null hypothesis that microwear textures do not differ between teeth from different species, we applied analysis of variance (ANOVA) with pair-wise testing (Tukey’s honest significant difference [HSD]) to each texture parameter for each species. Where homogeneity of variance test (Levene’s test) revealed evidence of unequal variances, we used Welch’s ANOVA. We selected the parameters that showed significant differences between species and applied a linear discriminant analysis (LDA). Descriptive statistics, tests and graphs were performed using Addinsoft™ XLSTAT-3.02 statistical packages.
Results
Stable isotope analysis
The δ13C values of all samples measured in GFT-4.2 range from −12.5 to −8.1‰ (n = 16; Supplementary Table 2). Macaca cf. sylvanus carbon values range from −12.5 to −11.8‰ (n = 4), with a mean of −12.3 ± 0.3‰. The values for the herbivore samples from GFT-4.2 are: Hipparion sp. (n = 4; range = −10.4 to −9.2‰; mean = −9.5 ± 1‰), Tragelaphini (n = 4; range = −11.3 to −8.1‰; mean = −10 ± 1.5‰), and Gazella sp. (n = 4; range = −12.2 to −9.4‰; mean = −10.9 ± 1.1‰). The δ13C values of the M. cf. sylvanus and ungulates from GFT-4.2 range from −12.5 to −8.1‰, these values correspond to a C3-dominated diets for macaques and ungulates.
The δ18O values range from −3.5 to 1.8‰ (n = 16; Supplementary Table 2). Macaca cf. sylvanus oxygen values range from 0.6 to 1.8‰, with a mean of 1 ± 0.5‰. The values for the herbivore samples from GFT-4.2 are: Hipparion sp. (n = 4; range = −3.6 to −0.3‰; mean = −1.8 ± 0.7‰), Tragelaphini (n = 4; range = −1.4 to 0.3‰; mean = −0.5 ± 0.4‰), and Gazella sp. (n = 4; range = −1.7 to 1.8‰; mean = −0.4 ± 0.8‰).
The mineralization of M1 in non-human primates starting from birth of the individual may reflect the nursing signal and thus affecting carbon and oxygen isotope values. However, our results do not indicate a 13C depletion or 18O enrichment in relation to the other M. cf. sylvanus teeth analyzed with a post-weaning isotopic signal (Supplementary Table 2).
Occlusal microwear analysis (low magnification)
The M. cf. sylvanus dental microwear pattern is characterized by a high number of pits (NP = 28.5) and a medium number of scratches (NS = 16). The extant cercopithecids show a similar pattern, namely a higher number of pits than scratches (Table 1). Lophocebus albigena has the highest number of pits (NP = 40.6; raw data in Supplementary Table 3). The exception is T. gelada with a microwear pattern dominated by scratches. Considering the SWS, all species show a high proportion of coarse scratches, and gouges are present in all species except T. gelada. L. albigena (G = 4.3), M. sphinx (G = 3.8), and the fossil macaque (G = 4) have a high abundance of gouges. L. albigena (PP = 5.4), M. mulatta (PP = 4.3), and the fossil macaque (PP = 4.5) have the highest number of puncture pits; this trait is absent in T. gelada and M. sphinx. All extant and fossil primates analyzed have a few hyper-coarse scratches and lack cross scratches (Table 1).
We performed correspondence analysis to compare four microwear variables (pits, scratches, puncture pits, and gouges) of the extant species and macaque from GFT-4.2 (Figure 1). We plot the results for discriminant functions 1 and 2 (DF1 and DF2, respectively) because they explain a higher percentage of the variance than the other axes (Supplementary Table 4). Three groups are well defined in the graph. The first group is located on the left and comprises T. gelada. The second group, located in the middle, includes M. sphinx. The third group, on the right, includes L. albigena, C. atys, and M. mulatta. T. gelada falls far from the other species because it has the highest number of scratches (NS = 25.9), the smallest number of pits (NP = 10.1), and the absence of puncture pits and gouges. M. sphinx has intermediate microwear values relative to the other two groups. This species has more pits than T. gelada, but also more scratches than the components of the third group. M. sphinx does not have puncture pits. The last group (L. albigena, C. atys, and M. mulatta) is characterized by a high number of pits (NP > 30) and a small number of scratches (NS < 12). The two specimens of the fossil macaque are located near the third group.
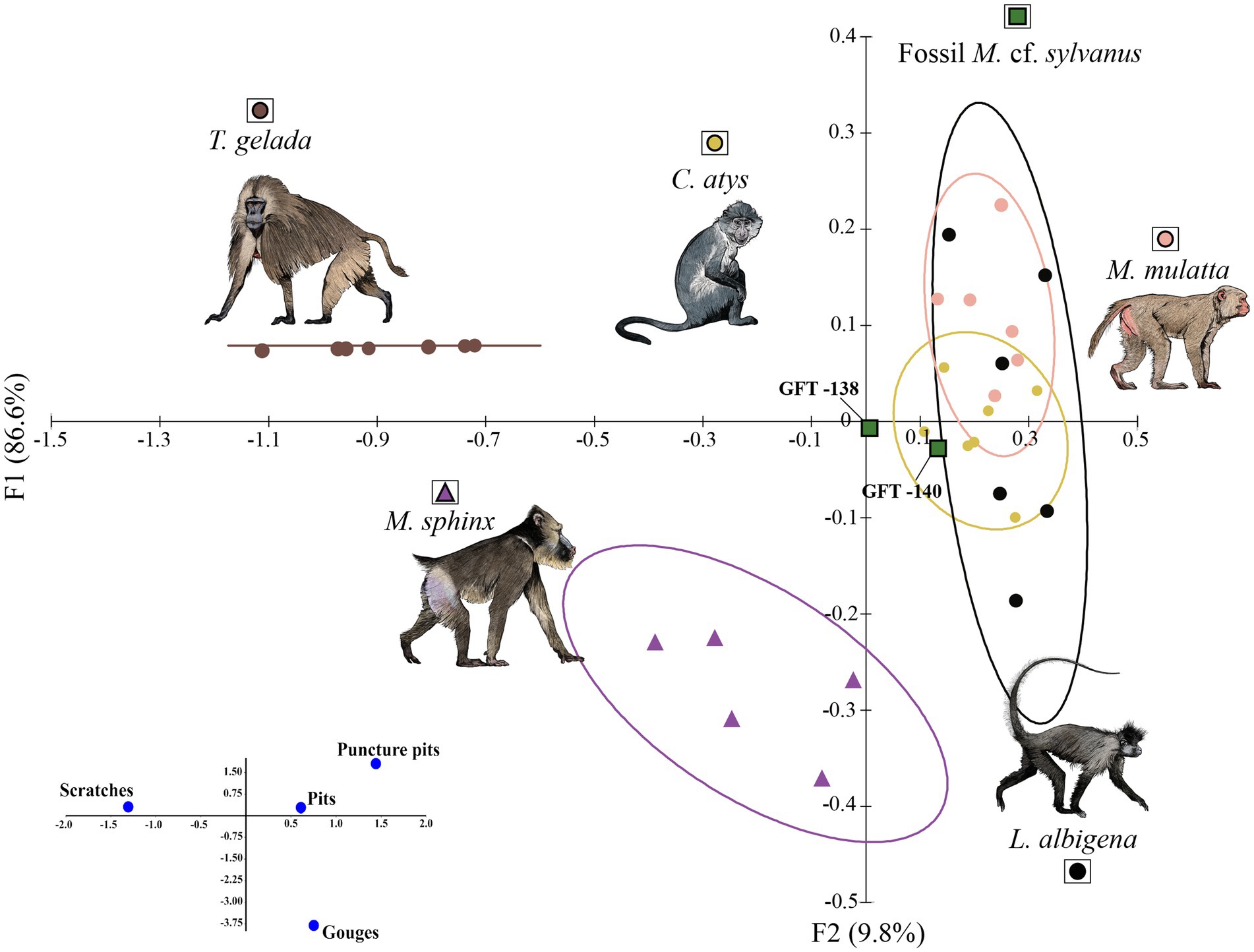
Figure 1. Correspondence analysis based on four occlusal microwear variables (number of puncture pits, number of gouges, number of scratches, and number of pits). Ellipses show 68% confidence limits for means (one standard deviation). Eigenvalues, percentages of variance and cumulative percentages of each axis in Supplementary Table 4 (illustrations by Diego Rodríguez-Robredo).
We performed LDA including the four microwear variables (pits, scratches, puncture pits, and gouges) to predict group membership of the fossil M. cf. sylvanus specimens from GFT-4.2. The LDA showed significant between-species differences (Wilks’ λ = 0.05; F = 20.826; p < 0.0001) and correctly classified 90.48% of the species. The two first discriminant functions explained 96.68% of the total variance. DF1 (85.88%) was positively correlated with scratches and negatively correlated with the presence of puncture pits and pits. DF2 (10.80%) was negatively correlated with the presence of gouges. GFT140 was classified as C. atys (99% of predicted classification probability) and GFT138 was classified as L. albigena (83% of predicted probability).
Buccal microtexture analysis
A total of 15 buccal enamel microtexture parameters out of the 38 (39.47%) showed significant differences (ANOVA and Tukey’s HSD post hoc procedure p < 0.05 and Welch correction, see Supplementary Table 7) among the Cercopithecoidea species. The main significant differences are between the folivore-frugivore M. mulatta and the graminivorous T. gelada and the hard fruit eater C. atys and the folivore-frugivore M. sphinx. T. gelada mainly differentiate from M. mulatta in volume parameters, functional and flatness (Vv, Vmc, Vvc, Sxp, Smc, FLTp, FLTv, and FLTq p < 0.05) while the hard fruit eater differentiates from M. sphinx in spatial, feature and flatness parameters (Sal, Shv, FLTp, FLTt, FLTv, and FLTq; Supplementary Figure 4). No significant differences are found between hard fruit eaters taxa, the arboreal L. albigena and terrestrial C. atys and, only one difference was found between the hard fruit eaters and the graminivorous T. gelada (the feature parameter Spc between L. albigena and T. gelada p < 0.05).
The LDA includes the 15 parameters to classify the extinct GFT-4.2 samples. DF1 and DF2 account for the 82.51% of the total original variance (Wilks’ λ = 0.014; F = 1.371; p-value = 0.143). DF1 (57.15%) is positively correlated with flatness and volume parameters and negatively with Shv (mean hill volume) DF2 (25.36%) was positively correlated with all the parameters except for Spc (Figure 2; see Supplementary Table 8 for the r values and significance). Overall, 86.21% of the teeth were correctly classified in their taxonomic category. Mixed feeders that incorporate more seeds and leaves compared to the other of species, such as M. sphinx and M. mulatta, have the highest loadings for DF2. Their buccal surfaces show the greatest texture relief, with sharp features and different slopes and heights compared to hard fruit eaters and T. gelada. M. sphinx and M. mulatta incorporate more seeds and leaves in their diets compared to the rest of species and their buccal surfaces show coarse features (high Sal) that is significantly flatter that T. gelada and C. atys. Moreover, T. gelada has the lowest loading for DF1, differing from L. albigena, because these features are rounded (lower Spc).
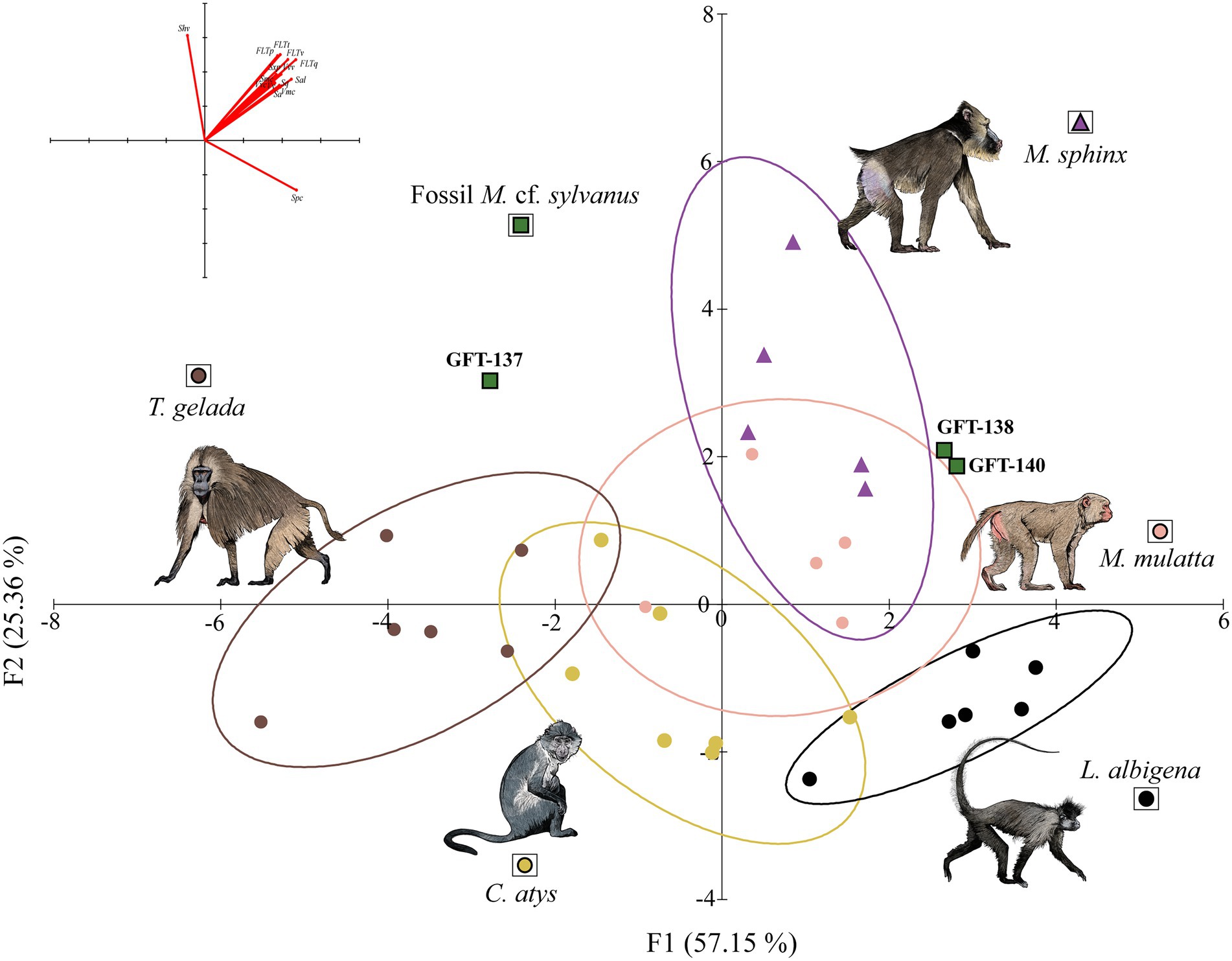
Figure 2. Plot of the two first discriminant functions (DF1-2) of buccal-dental microtextural parameters. Ellipses show 68% confidence limits for means (one standard deviation). On the top left are the loadings of the ISO texture parameters onto DFs. Raw data in Supplementary Table 8 (illustrations by Diego Rodríguez-Robredo).
The extant LDA model have been used to predict group membership of the fossil specimens from GFT-4.2. GFT-137 is classified as T. gelada (74.9% of probability as T. gelada and 25.1% as M. sphinx), GFT-138 (Figure 3) as M. mulatta (97.5% of probability) and GFT140 as M. sphinx (69.8% of probability as M. sphinx and 26.7% as L. albigena). The mean heigh, volume and flatness parameters of the three specimens from GFT-4.2 differ significantly (p < 0.05) from both C. atys and T. gelada, and were similar to folivores and seed eaters. Two GFT-4.2 specimens, therefore, resemble to primates that exploit seasonally leaves, fruits and seeds in major proportion, on the ground of mixed forest. GFT137, however, is classified as T. gelada a graminivorous primate that exploit also forbs in open ecosystems, due to similarities on buccal microtexture feature parameters.
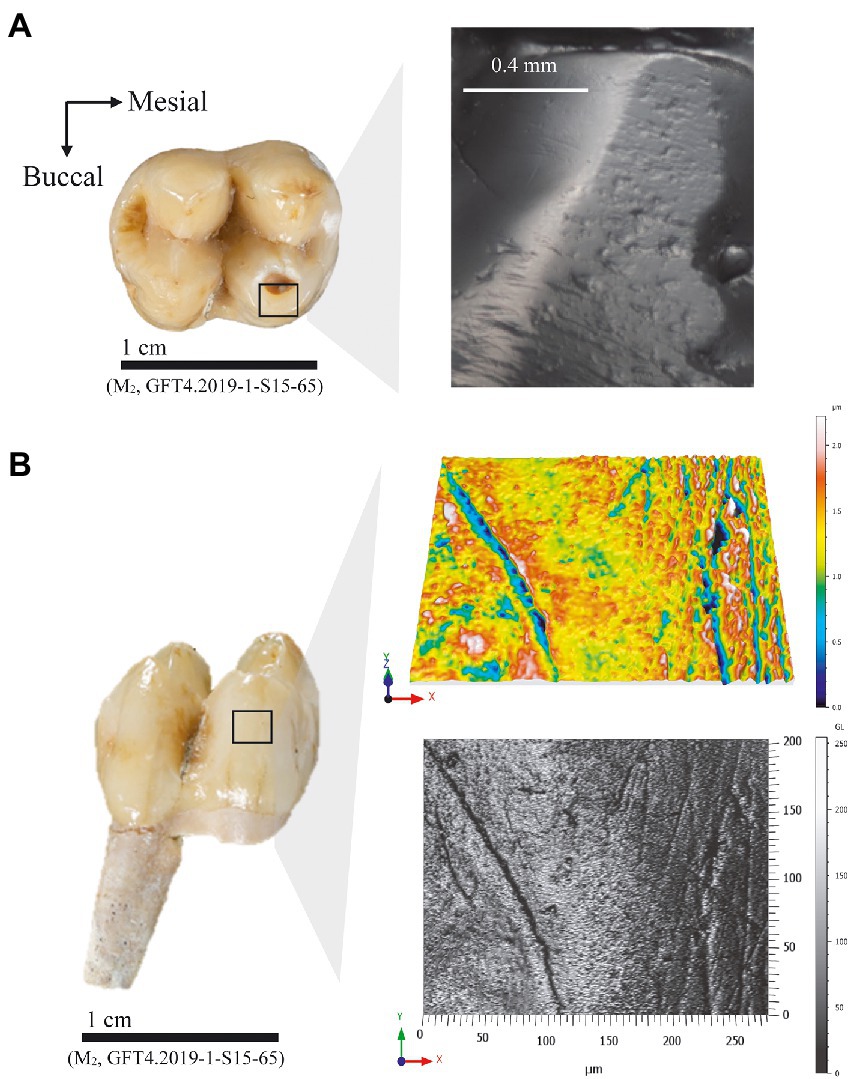
Figure 3. Image showing the two surfaces analyzed from the same tooth (M2, GFT4.2019-1-S15-65) of Macaca cf. sylvanus specimen from GFT-4.2 site. (A) Microphotographs at 35X magnifications of wear facet in the mesiobuccal cusp; (B) 3D views and photosimulations from buccal microtexture.
Discussion
Our multiproxy analysis suggests a different short-term (occlusal) and long-term (buccal) feeding habits of M. cf. sylvanus from GFT-4.2, but with an overall dominance of abrasive, tough and brittle food items in an environment dominated by C3 plants. The most commonly consumed foods would be fruit, seeds and leaves but also some grasses (Table 2). This dietary variety reflects the ability and flexibility of fossil macaques to consume different foods. As well as the richness of resources, and probably microhabitats, present in the GFT-4.2 area during the Plio-Pleistocene.
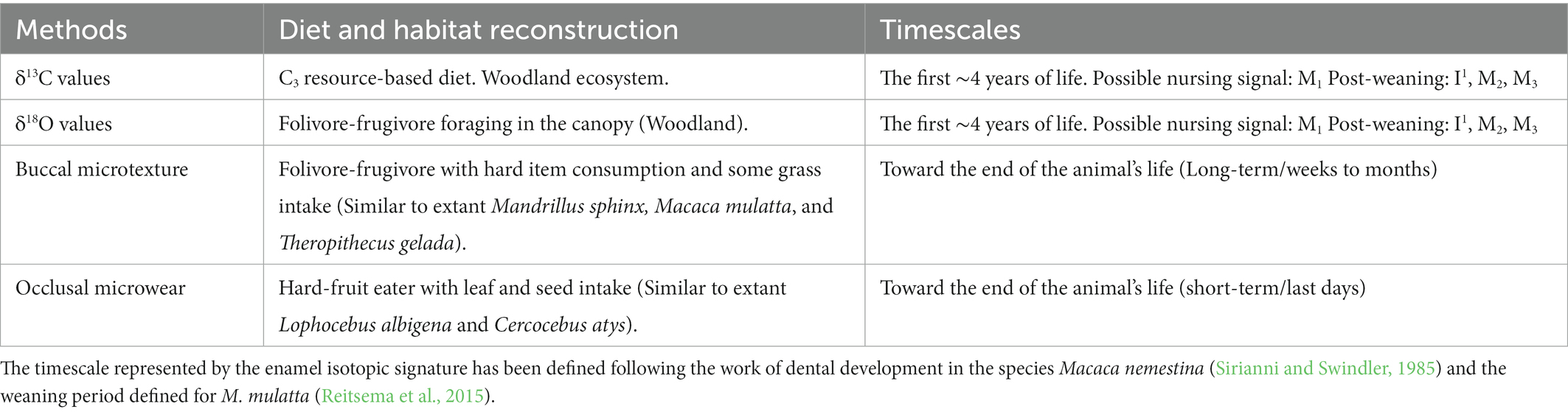
Table 2. Summary of the results provided by the three methods used to reconstruct the diet and habitat of Macaca cf. sylvanus from GFT-4.2.
Based on short-term resolution, the occlusal microwear analysis classifies the M. cf. sylvanus specimens (GFT138 and GFT140) as L. albigena and C. atys, which have a diet rich in hard fruit and seeds (Poulsen et al., 2001; Shah, 2003; Lambert et al., 2004; Daegling et al., 2011; McGraw et al., 2011, 2012, 2014). The extant L. albigena and C. atys species and the fossil M. cf. sylvanus have puncture pits and gouges, which is a distinctive microwear feature of hard-object feeders (Godfrey et al., 2004; Semprebon et al., 2004; Williams and Geissler, 2014). Both Cercocebus and Lophocebus have thicker enamel compared to other primate groups, which allows both genera to rely on hard foods throughout the year (McGraw et al., 2012). Macaca cf. sylvanus occlusal surfaces differs significantly from the extant T. gelada, which is characterized by an almost exclusively graminivorous diet with seasonal incorporation of forbs (Fashing et al., 2014; Jarvey et al., 2018). The occlusal microwear pattern of T. gelada is dominated by a high number of scratches and the absence of puncture pits and gouges. This surface is characteristic of animals with grass-dominated feeding habits (Godfrey et al., 2004; Semprebon et al., 2004). Even if it was not the dominant food item in their diet, we cannot exclude that M. cf. sylvanus consumed some grasses due to the large number of scratches found in specimen GFT138.
Occlusal microwear and buccal microtexture analysis group fossil M. cf. sylvanus with different extant species, an outcome that is explained by differences in the temporal resolution and turnover of the analyzed tooth surfaces. Buccal microtexture analysis provides medium long-term, compared to occlusal microwear, from weeks to months (Romero et al., 2012) temporal resolution classifying two of the three M. cf. sylvanus specimens (GFT 138, and GFT 140) from GFT-4.2 as M. sphinx and M. mulatta. These species primarily consume fruits, leaves, and seeds in closed and riparian forests during the wet season, and seeds foraged from the ground year-round. The sample included in the present analysis comes from the population that inhabit Pakistan where M. mulatta rely primarily on leaves and fruits depending on their availability (Goldstein and Richard, 1989; Sengupta and Radhakrishna, 2016). Both taxa, therefore, consume abrasive items from the ground in mixed forest. Mandrillus sphinx and M. mulatta diet also includes herbs such as piths and blades and occasionally woody tissue during seasons of heightened fruit scarcity (Sengupta and Radhakrishna, 2016; Hongo et al., 2017). Based on observations of M. sphinx from Gabon, more seeds are consumed during the dry season than in the peak fruit season. Moreover, finely crushed seeds in fecal samples indicate that M. sphinx uses the occlusal surface to fracture seeds (Hongo et al., 2017), and this mechanical action correlates with gouges on these surfaces (Figure 3; Supplementary Figure 2). M. sphinx consumes large nuts, like S. gabonensis, during seasons of heightened fruit scarcity (Hongo et al., 2017). Hard items are consumed habitually by L. albigena and C. atys year-round (McGraw et al., 2012). The microtexture buccal pattern of M. sphinx, characterized by higher relief, with peaks and dales and coarse features compared with the rest of analyzed species, may correlate with the presence of more abrasive fractured seeds or phytoliths from the leaves that are processed in the oral cavity while being fractured along occlusal surfaces. The similarity in the microtexture pattern of the two GFT-4.2 specimens with M. sphinx and M. mulatta suggests that M. cf. sylvanus regularly consumed abrasives food items. The LDA of the buccal microtexture plot two specimens (GFT138 and GFT140) close to each other and to the hard fruit eaters (Figures 1,2). All these species rely on hard-shelled fruits and seeds with seasonal fluctuations. Specially, M. mulatta diet resembles M. sphinx (Sengupta and Radhakrishna, 2016) with more soft fruit ingestion in the former. Both M. mulatta and M. sphinx incorporate hard and brittle dietary resources but, this is dependent on fruit availability during the year (Sengupta and Radhakrishna, 2016). When fruit is scarce, M. mulatta relies heavily on seeds. However, the buccal microtexture pattern of the GFT137 is also similar to T. gelada (Figure 2). Theropithecus gelada is a graminivorous primate that inhabit the open highlands of Ethiopia and consume grasses and forbs (Jarvey et al., 2018; Souron, 2018).
Three out of four extant species in which the microwear classifies the macaque of GFT-4.2 are species whose diet is related to the presence of trees. In this sense, oxygen values could also reinforce this link. The four M. cf. sylvanus specimens (GFT137, GFT138, GFT139, and GFT140) from GFT-4.2 show high δ18O values compared with the contemporary ungulates recovered from the same site. The same pattern occurs with other fossil cercopithecines (T. atlanticus and T. oswaldi) from North Africa (Figure 4). Extant M. sylvanus are highly adaptable and have an eclectic diet which adds to the variables that can affect their oxygen isotope values. A significant amount of their water ingestion comes from leaf, fruit and vegetable consumption, especially during the rainy season. Meanwhile, during the dry season, they drink more water from open water sources (Fooden, 2007). In the case of M. cf. sylvanus from GFT-4.2, the differences in oxygen values in relation to contemporary ungulates could be associated with differential access to and consumption of certain food resources by the primates, such as fresh fruits and leaves from trees. Sun-exposed leaves, especially in arid conditions, are particularly sensitive to evaporative fractionation that could lead to 18O enrichment (Gonfiantini et al., 1965; Barbour, 2007; Crowley et al., 2015; Carter and Bradbury, 2016; Fannin and McGraw, 2020). Other authors argue that this enrichment by evapotranspiration also occurs in other parts of the plant like fruits (Yakir, 1997; McCarroll and Loader, 2004). Paleoenvironmental data of GFT-4.2 based on the study of paleoherpetological and small mammal records, suggest dry conditions in an open woodland habitat with a permanent water body nearby (Agustí et al., 2017; Piñero et al., 2019; Alba et al., 2021).
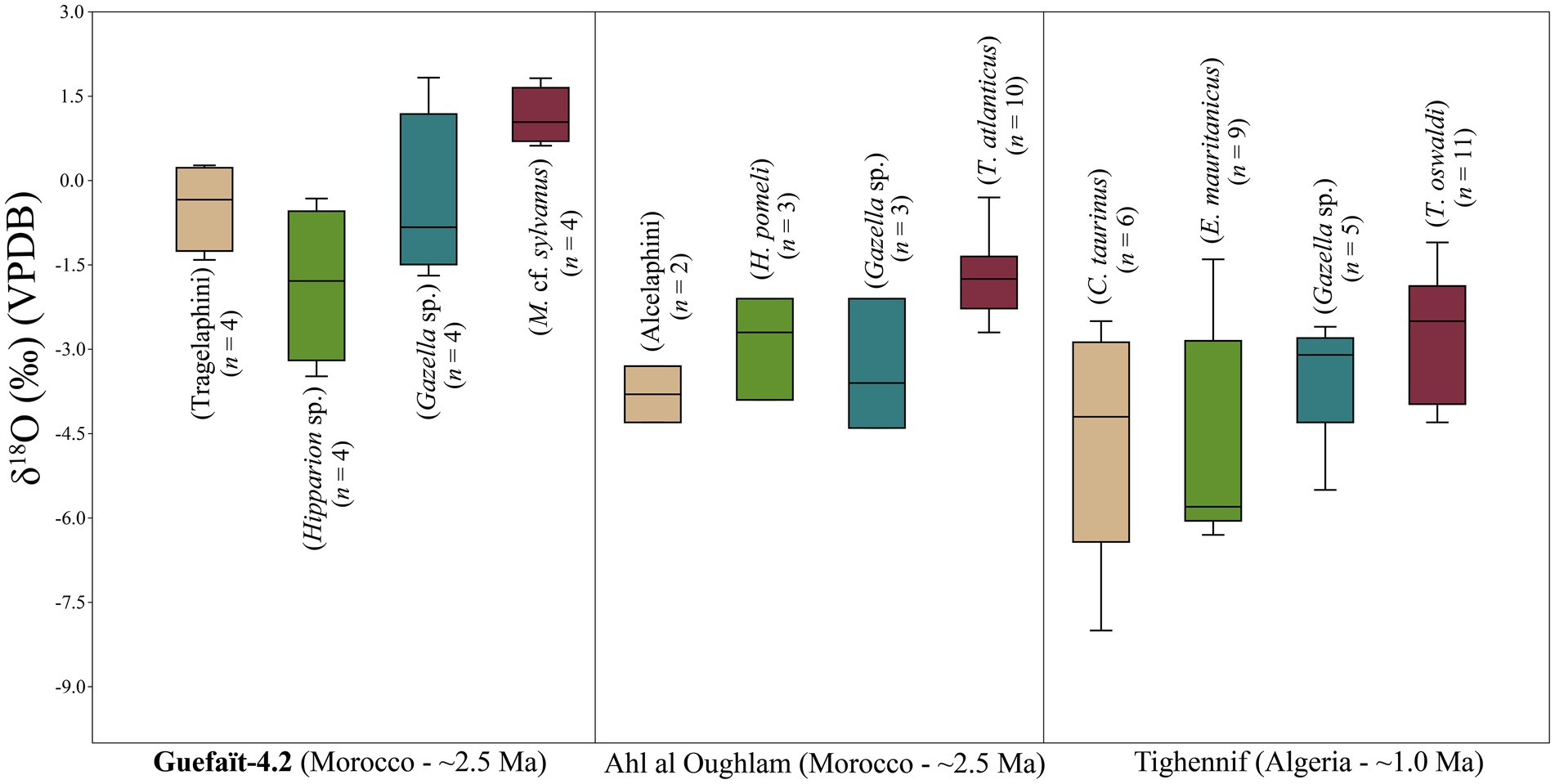
Figure 4. Box plots for δ18O values of Macaca cf. sylvanus, Hipparion sp., Gazella sp. and Tragelaphini from GFT-4.2 in comparison with extinct Theropithecus and ungulates from the Plio-Pleistocene sites of Ahl al Oughlam and Tighennif in North Africa published in Fannin et al. (2021). The boxes show the median and the lower (25%) and upper (75%) quartiles.
Considering that aridity affects all GFT-4.2 taxa, we suggest that the oxygen enrichment of M. cf. sylvanus may be due to the ingestion of some different foods. An example in extant primates is the one exposed by Moritz et al. (2012) that measured the δ18Ohair from two baboon species (Papio anubis and Papio hamadryas) which inhabit the arid hybrid zone of Awash National Park (Ethiopia). The diets of the two species in this area are similar and both obtain their meteoric water from the same source (Awash River). However, the oxygen values of P. hamadryas are relatively enriched due to the ingestion of a greater proportion of 18O-enriched leaf water in the arid thornbush. Krigbaum et al. (2013) show how δ18Oap values of different cercopithecids covary depending on foraging position in the closed tropical canopy forest (Taï forest, Côte d’Ivoire). Higher humidity on the forest floor is associated with low δ18Oap values, with enrichment of 18O observed along a vertical gradient. The C. atys exhibits low δ18Oap values relative to guenons and colobines, which reflects the C. atys terrestrial foraging habits. Although we do not have a tropical canopy forest in GFT-4.2, these differences in δ18O values between feeding on the ground and in the upper parts of the tree could explain the 18O enrichment of macaques compared to ungulates.
The 18O enrichment points to a diet based on tree foraging in a forest ecosystem. These findings are consistent with the microtexture and low magnification signals and support the carbon isotope results of M. cf. sylvanus from GFT-4.2. The δ13C values indicate consumption of C3 plants (i.e., trees, shrubs, fruit/seeds, and C3-grass adapted to a mild growth season). M. cf. sylvanus, Hipparion sp., Gazella sp. and Tragelaphini from GFT-4.2 have been compared with the fossil T. atlanticus from Ahl al Oughlam, Morocco (ca. 2.5 Ma) and T. oswaldi from Tighenif, Algeria (ca. 1 Ma) and other ungulates from the same sites (Fannin et al., 2021; Figure 5; Supplementary Table 2). The M. cf. sylvanus (n = 4) mean is −12.3 ± 0.3‰, the T. atlanticus (n = 10) mean is −12.4 ± 0.8‰, and the T. oswaldi (n = 11) mean is −11.3 ± 1.9‰ (Figure 5). These three fossil species show similar median carbon values and no consumption of C4 plants. Except for one individual of T. oswaldi from Tighenif with a δ13C value of −5.9‰ that includes some C4 plant consumption.
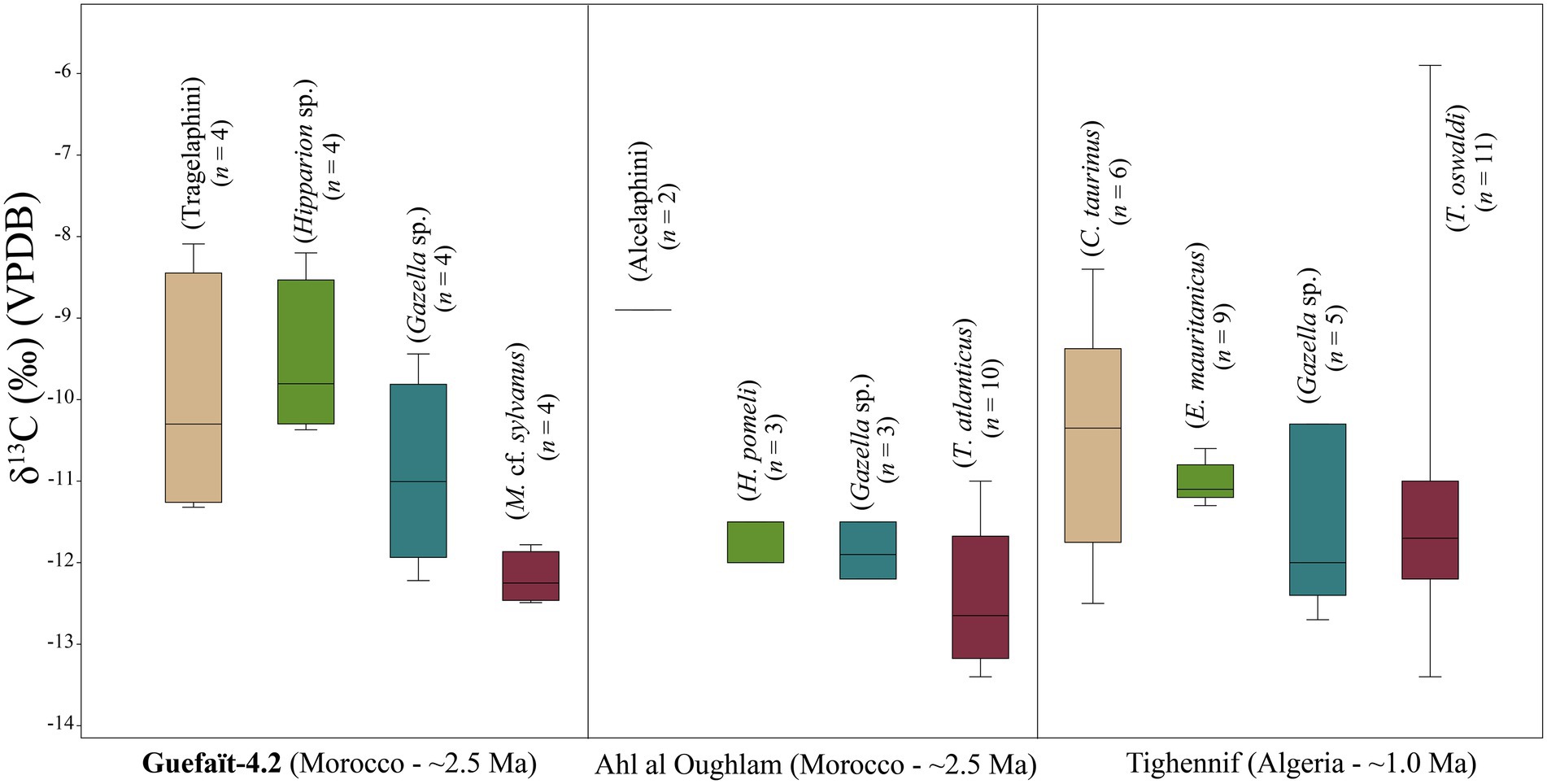
Figure 5. Box plots for δ13C values of Macaca cf. sylvanus, Hipparion sp., Gazella sp. and Tragelaphini from GFT-4.2 in comparison with extinct Theropithecus and ungulates from the Plio-Pleistocene sites of Ahl al Oughlam and Tighennif in North Africa published in Fannin et al. (2021). The boxes show the median and the lower (25%) and upper (75%) quartiles.
Our data differ from the mean δ13C values of 1.9 ± 1.8‰ (n = 49) from Plio-Pleistocene fossils of Theropithecus from Eastern Africa, 3.5 to 1.5 Ma. In Southern Africa, Theropithecus living 2.8 and 1.6 Ma have mean value of −2.4 ± 1.4‰ (n = 13). In both areas, these species indicate a strongly C4-based diet composed mostly of grasses (Cerling et al., 2011; Souron, 2018), especially after the 2 Ma (Figure 6). The carbon values of M. cf. sylvanus from GFT-4.2 are similar to the δ13C values of C3 consumers Papio (2.1 to 1.6 Ma; −9.8 ± 2.3‰; n = 26), Parapapio (2.8 to 1.7 Ma; −8.4 ± 2‰; n = 38), and Cercopithecoides (2.8 to 1.7 Ma; −9.8 ± 3.6‰; n = 12) from the Plio-Pleistocene of Southern Africa (Figure 6; Lee-Thorp et al., 1989; Codron et al., 2005). The largest proportion of Southern African cercopithecids fall into the C3–C4 mixed feeders’ area, suggesting a consumption of some C4 plants (Figure 6). Cercopithecids with carbon values resembling those of GFT-4.2 macaques are the Cercopithecoides haasgati (2.1 Ma; −12.3 ± 1.4‰; n = 2) from Haasgat HGD, Papio robinsoni (1.85 Ma; −11.2 ± 0.9‰; n = 5) and Parapapio jonesi (1.85 Ma; −11.2 ± 2.3‰; n = 2) from Swartkrans (Figure 6). The δ13C values of C. haasgati could indicate a preference toward food sources associated with closed woodland environments (Adams et al., 2013). This interpretation is consistent with the carbon values of all of them, including the fossil macaque. Feeding habits of P. robinsoni and P. jonesi from Swartkrans have been reconstructed as a frugivore-folivore on the basis of cusp form (Benefit, 2000). We also propose this using the occlusal and buccal microwear for M. cf. sylvanus from GFT-4.2, although the exploitation of grasses and forbs in a more open habitats cannot rule out due to the buccal microtexture pattern of GFT137 and the high number of scratches on the occlusal surface of GFT138. Carbon isotopic signal of the Macaca from GFT-4.2 would indicate that these consumed grasses would be C3. Consumption of C3 grasses has also been identified in the diet of T. oswaldi from the Plio-Pleistocene site of Ahl al Oughlam (Morocco), as well as in extant T. gelada at Guassa Plateau (Ethiopia; Fannin et al., 2021).
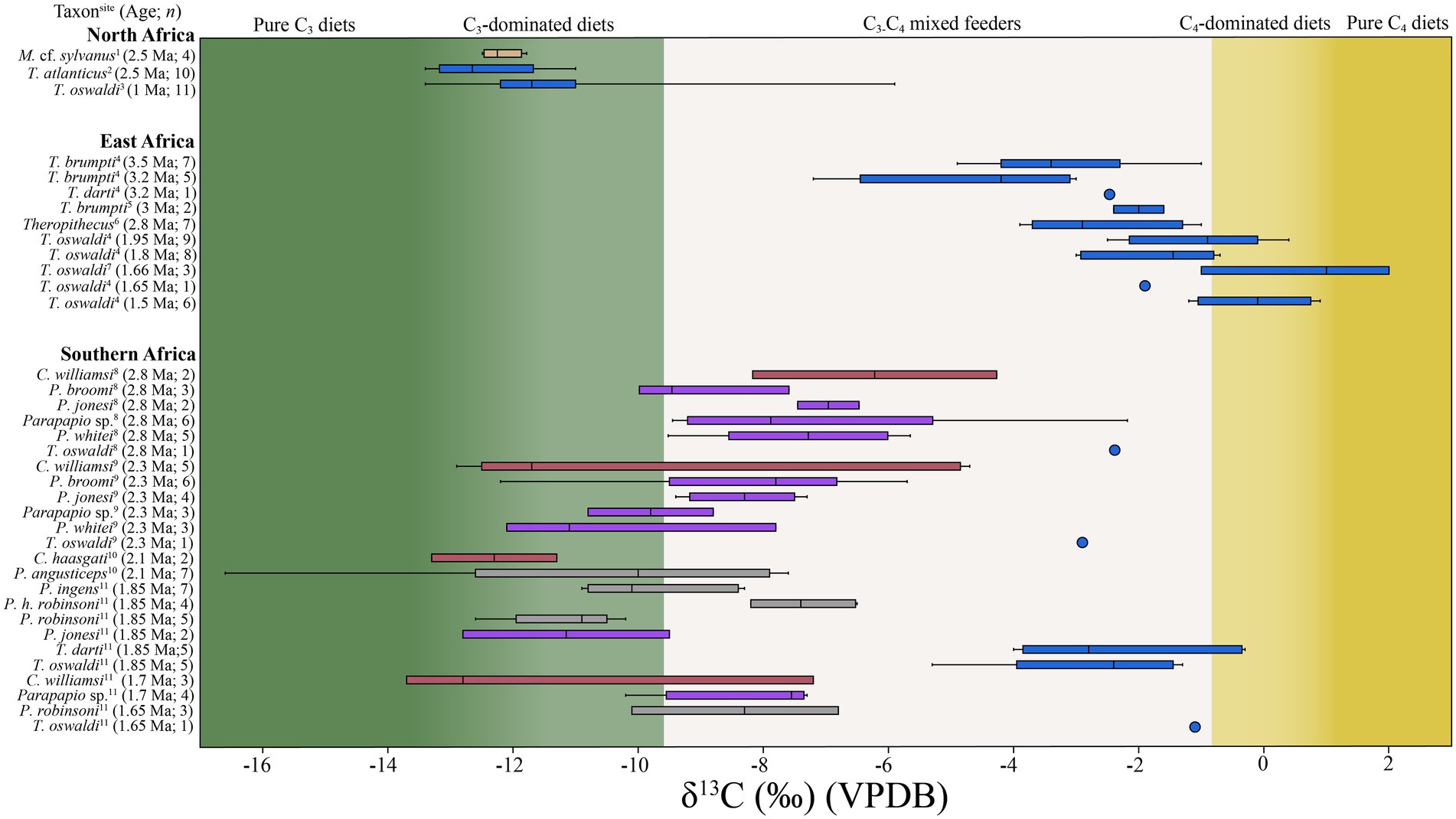
Figure 6. Box plots comparing the δ13C values of Plio-Pleistocene fossil cercopithecids (3.5 to 1 Ma) from North Africa (data from this study and Fannin et al., 2021). Sites: 1 = Guefaït 4.2; 2 = Ahl al Oughlam; 3 = Tighennif, East Africa [data from Levin et al. (2015), Robinson et al. (2017), and Uno et al. (2018)]. Sites: 4 = Koobi Fora, East Turkana; 5 = Nachuki, West Turkana; 6 = Ledi-Geraru, Gurumaha; 7 = Bed II, Olduvai and Southern Africa [data from Levin et al. (2015)]. Sites: 8 = Makapansgat, 3 and 4; 9 = Sterkfontein; 10 = Haasgat HGD; 11 = Swartkrans. The boxes show the median and the lower (25%) and upper (75%) quartiles. The colors of the box plots represent the different genus (beige for Macaca, blue for Theropithecus, red for Cercopithecoides, purple for Parapapio, and gray for Papio).
The microwear analysis shows that the diet of the fossil macaque is close, among others, to that of extant M. sphinx, L. albigena, and C. atys, species that live in dense tropical forests. Isotopic studies on extant M. sphinx and C. atys have shown carbon values strongly affected by canopy effect. According to Oelze et al. (2020), the δ13C values of M. sphinx hair sections from Gabon are within the range (−25.1‰ ± 0.3‰) of those obtained from the hair of chimpanzees and gorillas in Loango National Park. Like these species, mandrills live in dense forest habitats. Another study by Krigbaum et al. (2013) on bone apatite from seven sympatric cercopithecine species, including C. atys, in the Taï forest (Côte d’Ivoire) obtained carbon values range between −19.9‰ and −17.9‰ for all species. In contrast, the carbon values of M. cf. sylvanus from GFT-4.2 (−12.3 ± 0.3‰) do not correspond to those of a species foraging in a closed canopy forest. Considering the enamel-diet enrichment factor for primates (+11.8 ± 0.3‰; Malone et al., 2021) and the cutoff of −30‰ (δ13CCO2 corrected by ~ +1.5) for closed-canopy forests (Kohn, 2010), the δ13C values of tooth enamel for a diet in a closed-canopy forest should be lower than ~ −18‰ for fossil Macaca. The carbon values of the taxa analyzed are compatible with an open woodland. This is in line with the paleoherpetological and small mammal work in which taxa characteristic of open woodland have been defined (Agustí et al., 2017; Piñero et al., 2019).
The use of these three methods has allowed us to produce a paleodietary reconstruction at different times in the life of the individuals, although we cannot discard that the analyzed molars belong to the same specimen. The isotopic signal, corresponding to approximately the first 4 years of life, is in agreement with the data of the two dental microwear proxies in the diet-tree relationship for M. cf. sylvanus, and the absence of C4 typical of open grassland. Buccal and occlusal microwear turnover is a dynamic process; however, the buccal signal is more stable in the long term and may indicate the diet consumed year-round (Romero et al., 2012). Whereas the low magnification occlusal microwear results may indicate the consumption of hard fruit and seeds similar to mangabeys the last days or weeks prior to death (Semprebon et al., 2004), perhaps representing the diet of a single season. The buccal microtexture analysis suggest that the abrasiveness of their diet was similar to primates (M. sphinx and M. mulatta) that consumes hard, tough, and brittle food items year round with the incorporation of leaves, and the consumption of grasses as T. gelada does. The complementarity of these proxies helps to differentiate between seasonal, short-term durophagous species from those that exploit tough and brittle items actively in a long term, broadening the dietary range of consumed resources throughout the year.
Conclusion
Our research represents the first report related to the feeding ecology of fossil Macaca during the Plio-Pleistocene in Africa. Our results highlight a tight correlation between the fossil M. cf. sylvanus specimens from GFT-4.2 and extant primates with a predominant abrasive, tough and brittle food items. Evidence from the multiproxy analysis suggests that the macaques foraged for food in a wooded habitat, but also foraging in more open environments. The versatility of macaques to occupy wide habitat ranges makes them a useful candidate for analogies with hominins and for understanding how they adapted to different environments. Evidence of early hominins in North Africa is dated close to the Plio-Pleistocene boundary (ca. 2.4 Ma) at the Ain Boucherit site in Algeria. The ecological context of this first human population is a key question to understand the dispersions of humans and other mammals in these territories. Considering the proximity of GFT to Ain Boucherit, understanding the feeding ecology of M. cf. sylvanus from GFT may provide clues about some of the ecological resources that these early humans may have had. This initial investigation is only one step toward understanding the feeding habits of M. cf. sylvanus. More data are needed from additional individuals to be able to confidently interpret foraging ecology of this species.
Data availability statement
The original contributions presented in the study are included in the article/Supplementary material, further inquiries can be directed to the corresponding authors. The surface data are available from the corresponding author upon reasonable request.
Author contributions
IR-P, LM, FR, CT, and FE-S conceived and designed this research. IR-P and LM performed the laboratory analyses and collected and analyzed the data. JI provided access to the laser scanning confocal microscope. AA, AR-H, JM, HA, AO, HH, IR-P, HM, MS, RS-R and MC participated in the fieldwork and provided materials and background for the research. MC, RS-R, and HA are in charge of the project administration and the funding acquisition. AR-H supervised the excavation. AR-H, HA, and JM identified the faunal remains. All authors contributed to the article and approved the submitted version.
Funding
This work has been funded by Palarq Foundation, Spanish Ministry of Culture and Sport (Ref: 42-T002018N0000042853 and 170-T002019N0000038589), Direction of Cultural Heritage (Ministry of Culture and Communication, Morocco), Faculty of Sciences (Mohamed 1r University of Oujda, Morocco), INSAP (Institut National des Sciences de l’Archéologie et du Patrimoine), Spanish Ministry of Science, Innovation and Universities (Ref: CGL2016-80975-P, CGL2016-80000-P, PGC2018-095489-B-I00, and PID2021-122355NB-C33), Research Groups Support of the Generalitat de Catalunya (2017 SGR 836, 2017 SGR 1040, 2017 SGR 102, and 2017 SGR 859) and PDC2021-121613-I00 and PID2020-112963GB-I00 by ERDF A way of making Europe, by the European Union. RS-R, MC, AR-H, and CT research was funded by CERCA Programme Generalitat de Catalunya. IR-P is beneficiary of predoctoral fellowship (2020-FI-B-00731) funded by AGAUR and the Fons Social Europeu (FSE). AA and is beneficiary of a fellowship from the Erasmus Mundus Program to do the Master in Quaternary and Prehistory at the Universitat Rovira i Virgili (Tarragona, Spain). CT was supported by the Spanish Ministry of Science and Innovation through the “Ramón y Cajal” program (RYC2020-029404-I). The Institut Català de Paleoecologia Humana i Evolució Social (IPHES-CERCA) has received financial support from the Spanish Ministry of Science and Innovation through the “María de Maeztu” program for Units of Excellence (CEX2019-000945-M), including the postdoctoral fellowships of AR-H.
Acknowledgments
We thank the Direction of Cultural Heritage (Ministry of Culture and Communication, Morocco), the Jerada Government and Local Authorities of Aïn Béni Mathar and Guefaït for local permits to develop geological, archeological and paleontological fieldwork in the region. Lastly, we want to thank the local population for their support, collaboration and help in the discovery of some of the fossils and certain archeo-paleontological sites and above all for their hospitality and welcome. We also acknowledge the two reviewers, the editor Brooke E. Crowley and Robert Patalano for the constructive comments raised, which considerably helped to improve this research and Diego Rodríguez-Robredo for the illustrations of extant primates. We are grateful to all the curators and technical personnel of the different institutions where the extant specimens were molded.
Conflict of interest
The authors declare that the research was conducted in the absence of any commercial or financial relationships that could be construed as a potential conflict of interest.
Publisher’s note
All claims expressed in this article are solely those of the authors and do not necessarily represent those of their affiliated organizations, or those of the publisher, the editors and the reviewers. Any product that may be evaluated in this article, or claim that may be made by its manufacturer, is not guaranteed or endorsed by the publisher.
Supplementary material
The Supplementary material for this article can be found online at: https://www.frontiersin.org/articles/10.3389/fevo.2023.1011208/full#supplementary-material
Footnotes
References
Adams, J. W., Kegley, A. D. T., and Krigbaum, J. (2013). New faunal stable carbon isotope data from the Haasgat HGD assemblage, South Africa, including the first reported values for Papio angusticeps and Cercopithecoides haasgati. J. Hum. Evol. 64, 693–698. doi: 10.1016/j.jhevol.2013.02.009
Agustí, J., Piñero, P., Blain, H.-A., Aouraghe, H., Haddoumi, H., El Hammouti, K., et al. (2017). “The early Pleistocene small vertebrates from Guefaït 4 (Jerada, Morocco)” in III Jornadas de Prehistoria Africana. Book of abstracts. eds. M. G. Chacón, I. Caceres, V. Altés, M. Soto, and R. Sala (Tarragona), 49.
Alba, D. M., Delson, E., Carnevale, G., Colombero, S., Delfino, M., Giuntelli, P., et al. (2014). First joint record of Mesopithecus and cf. Macaca in the Miocene of Europe. J. Hum. Evol. 67, 1–18. doi: 10.1016/j.jhevol.2013.11.001
Alba, D. M., Rodríguez-hidalgo, A., Aouraghe, H., Van Der Made, J., Oujaa, A., Haddoumi, H., et al. (2021). New macaque fossil remains from Morocco. J. Hum. Evol. 153, 102951–102958. doi: 10.1016/j.jhevol.2021.102951
Albert, A., Hambuckers, A., Culot, L., Savini, T., and Huynen, M. C. (2013). Frugivory and seed dispersal by northern pigtailed macaques (Macaca leonina), in Thailand. Int. J. Primatol. 34, 170–193. doi: 10.1007/s10764-012-9649-5
Alemseged, Z., and Geraads, D. (1998). Theropithecus atlanticus (Thomas, 1884) (primates: Cercopithecidae) from the late Pliocene of Ahl al Oughlam, Casablanca, Morocco. J. Hum. Evol. 34, 609–621. doi: 10.1006/jhev.1998.9999
Aouraghe, H., Haddoumi, H., Hajji, N.-E., Sala, R., Rodríguez-Hidalgo, A., Van der Made, J., et al. (2019a). “Découverte d’un nouveau site paléontologique plio-quatenaire dans le Maroc oriental (Guefaït 4, province de Jerada),” in Recueil des Résumés, 23éme Colloque des Bassins Sédimentaires. Fès, 21–23 novembre., 34–35.
Aouraghe, H., Haddoumi, H., Rodríguez-Hidalgo, A., Van der Made, J., Piñero, P., Agustí, J., et al. (2019b). “Nouvelles données sur le site du Pliocène Final/Pléistocène Inférieur de Guefaït 4: Mission 2019,” In 10éme Rencontre des Quaternaristes Marocains, Kénitra. Recueil des Résumés, 47–48.
Aouraghe, H., Sala, R., and Chacón, M. (2016). “Bilan de dix ans de recherches archéologiques dans la region de Jerada (Maroc Oriental),” in 10 Ans de Recherches & de Cooperations Scientifiques Maroco-Espagnole dans la Province de Jerada (Maroc Oriental). Séminaire International sur le Patrimoine Archéologique de la Province de Jerada. Recueil du Seminaire, Jerada, 8–9.
Arambourg, C. (1959). Vertebres continentaux du Miocene Superieur de l’Afrique du Nord. Publ. du Serv. la Cart. Geol. l’Algerie Paleontologie Mem. 4, 5–159.
Arambourg, C. (1979). Vertébrés villafranchiens d’Afrique du Nord (Artiodactyles, Carnivores, Primates, Reptiles, Oiseaux). Paris: Fondation Singer-Polignac.
Arambourg, C., and Coque, R. (1959). Le gisement villafranchien de l’Aïn Brimba (Sud-Tunisien) et sa faune. Bull. la Société Géologique Fr. 8, 607–614.
Barbour, M. M. (2007). Stable oxygen isotope composition of plant tissue: a review. Funct. Plant Biol. 34, 83–94. doi: 10.1071/FP06228
Benefit, B. R. (2000). “Old World monkey origins and diversification: an evolutionary study of diet and dentition” in Old World monkeys. eds. P. F. Whitehead and C. J. Jolly (Cambridge: Cambridge University Press), 133–179.
Bryant, J. D., and Froelich, P. N. (1995). A model of oxygen isotope fractionation in body water of large mammals. Geochim. Cosmochim. Acta 59, 4523–4537. doi: 10.1016/0016-7037(95)00250-4
Calandra, I., Schulz, E., Pinnow, M., Krohn, S., and Kaiser, T. M. (2012). Teasing apart the contributions of hard dietary items on 3D dental microtextures in primates. J. Hum. Evol. 63, 85–98. doi: 10.1016/j.jhevol.2012.05.001
Carter, M. L., and Bradbury, M. W. (2016). Oxygen isotope ratios in primate bone carbonate reflect amount of leaves and vertical stratification in the diet. Am. J. Primatol. 78, 1086–1097. doi: 10.1002/ajp.22432
Cerling, T. E., Chritz, K. L., Jablonski, N. G., Leakey, M. G., and Manthi, F. K. (2013a). Diet of Theropithecus from 4 to 1 ma in Kenya. Proc. Natl. Acad. Sci. U. S. A. 110, 10507–10512. doi: 10.1073/pnas.1222571110
Cerling, T. E., and Harris, J. M. (1999). Carbon isotope fractionation between diet and bioapatite in ungulate mammals and implications for ecological and paleoecological studies. Oecologia 120, 347–363. doi: 10.1007/s004420050868
Cerling, T. E., Manthi, F. K., Mbua, E. N., Leakey, L. N., Leakey, M. G., Leakey, R. E., et al. (2013b). Stable isotope-based diet reconstructions of Turkana Basin hominins. Proc. Natl. Acad. Sci. U. S. A. 110, 10501–10506. doi: 10.1073/pnas.1222568110
Cerling, T. E., Mbua, E., Kirera, F. M., Manthi, F. K., Grine, F. E., Leakey, M. G., et al. (2011). Diet of Paranthropus boisei in the early Pleistocene of East Africa. Proc. Natl. Acad. Sci. U. S. A. 108, 9337–9341. doi: 10.1073/pnas.1104627108
Chacón, M., Aouraghe, H., Agustí, J., Alvárez, C., Arnold, L., Benito-Calvo, A., et al. (2016). “Ten years of archaeological research in the Aïn Béni-Mathar/Guefaït region (eastern Morocco): results and perspectives,” in 58th Annual Meeting in Budapest. March 29th e April 2nd 2016. Hugo Obermaier-Gesellschaft für Erforschung des Eiszeitalers und der Steinzeit e.V., Erlangen., 21–22.
Codron, D., Luyt, J., Lee-Thorp, J. A., Sponheimer, M., De Ruiter, D., and Codron, J. (2005). Utilization of savanna-based resources by Plio-Pleistocene baboons. S. Afr. J. Sci. 101, 245–248. doi: 10.5167/uzh-25352
Crowley, B. E. (2014). Oxygen isotope values in bone carbonate and collagen are consistently offset for New World monkeys. Biol. Lett. 10:20140759. doi: 10.1098/rsbl.2014.0759
Crowley, B. E., Melin, A. D., Yeakel, J. D., and Dominy, N. J. (2015). Do oxygen isotope values in collagen reflect the ecology and physiology of neotropical mammals? Front. Ecol. Evol. 3, 1–12. doi: 10.3389/fevo.2015.00127
Daegling, D. J., and Grine, F. E. (1999). Terrestrial foraging and dental microwear in Papio ursinus. Primates 40, 559–572. doi: 10.1007/BF02574831
Daegling, D. J., McGraw, W. S., Ungar, P. S., Pampush, J. D., Vick, A. E., and Bitty, E. A. (2011). Hard-object feeding in sooty mangabeys (Cercocebus atys) and interpretation of early hominin feeding ecology. PLoS One 6:e23095. doi: 10.1371/journal.pone.0023095
Deag, J. (1983). Feeding habits of Macaca sylvanus (primates: Cercopithecinae) in a commercial Moroccan cedar forest. J. Zool. 201, 570–575. doi: 10.1111/j.1469-7998.1983.tb05080.x
Delson, E. (1974). Preliminary review of Cercopithecid distribution in the Circum Mediterranean region. Mem. du Bur. Rech. Geol. Minieres 78, 131–135.
Delson, E. (1980). “Fossil macaques, phyletic relationships and a scenario of deployment” in The macaques. studies in ecology, behavior and evolution. ed. D. E. Lindburg (New York: Van Nostrand), 10–30.
Delson, E. (1993). “Theropithecus fossils from Africa and India and the taxonomy of the genus” in Theropithecus: The rise and fall of a primate genus. ed. N. G. Jablonski (Cambridge: Cambridge University Press), 157–189.
DeMenocal, P. B. (1995). Plio-Pleistocene African climate. Science 270, 53–59. doi: 10.1126/science.270.5233.53
Drucker, G. (1984). “The feeding ecology of the barbary macaque and cedar forest conservation in the Moroccan Moyen atlas” in The barbary macaque: A case study in conservation. ed. J. E. Fa (New York: Springer), 135–164.
Dunbar, R. I. M., and Bose, U. (1991). Adaptation to grass-eating in gelada baboons. Primates 32, 1–7. doi: 10.1007/BF02381596
Elton, S. (2007). Environmental correlates of the cercopithecoid radiations. Folia Primatol. 78, 344–364. doi: 10.1159/000105149
Elton, S., and O’Regan, H. J. (2014). Macaques at the margins: the biogeography and extinction of Macaca sylvanus in Europe. Quat. Sci. Rev. 96, 117–130. doi: 10.1016/j.quascirev.2014.04.025
El-Zaatari, S., Grine, F. E., Teaford, M. F., and Smith, H. F. (2005). Molar microwear and dietary reconstructions of fossil Cercopithecoidea from the Plio-Pleistocene deposits of South Africa. J. Hum. Evol. 49, 180–205. doi: 10.1016/j.jhevol.2005.03.005
Fannin, L. D., and McGraw, W. S. (2020). Does oxygen stable isotope composition in primates vary as a function of vertical stratification or folivorous behaviour? Folia Primatol. 91, 219–227. doi: 10.1159/000502417
Fannin, L. D., Yeakel, J. D., Venkataraman, V. V., Seyoum, C., Geraads, D., Fashing, P. J., et al. (2021). Carbon and strontium isotope ratios shed new light on the paleobiology and collapse of Theropithecus, a primate experiment in graminivory. Palaeogeogr. Palaeoclimatol. Palaeoecol. 572:110393. doi: 10.1016/j.palaeo.2021.110393
Farquhar, G. D., Ehleringer, R., and Hubick, K. T. (1989). Carbon isotope discrimination and photosynthesis. Annu. Rev. Plant Physiol. Plant Mol. Bioi 40, 505–537.
Fashing, P. J., Nguyen, N., Venkataraman, V. V., and Kerby, J. T. (2014). Gelada feeding ecology in an intact ecosystem at Guassa, Ethiopia: variability over time and implications for theropith and hominin dietary evolution. Am. J. Phys. Anthropol. 155, 1–16. doi: 10.1002/ajpa.22559
Fiedli, H., Lötscher, H., Oeschger, H., Siegenthaler, U., and Stauffer, B. (1986). Ice core record of the 13C/12C ratio of atmospheric CO2 in the past two centuries. Nature 324, 237–238. doi: 10.1038/324237a0
Fooden, J. (2007). Systematic review of the barbary macaque, Macaca sylvanus (Linnaeus, 1758). FIELDIANA Zool. 26, 1–60. doi: 10.5962/bhl.title.14256
Fourie, N. H., Lee-Thorp, J. A., and Ackermann, R. R. (2008). Biogeochemical and craniometric investigation of dietary ecology, niche separation, and taxonomy of plio-pleistocene cercopithecoids from the Makapansgat limeworks. Am. J. Phys. Anthropol. 135, 121–135. doi: 10.1002/ajpa.20713
Geraads, D. (1987). Dating the northern African cercopithecid fossil record. Hum. Evol. 2, 19–27. doi: 10.1007/BF02436528
Geraads, D. (2006). The late Pliocene locality of Ahl al Oughlam, Morocco: vertebrate fauna and interpretation. Trans. R. Soc. South Africa 61, 97–101. doi: 10.1080/00359190609519958
Godfrey, L. R., Semprebon, G. M., Jungers, W. L., Sutherland, M. R., Simons, E. L., and Solouniase, N. (2004). Dental use wear in extinct lemurs: evidence of diet and niche differentiation. J. Hum. Evol. 47, 145–169. doi: 10.1016/j.jhevol.2004.06.003
Goldstein, S. J., and Richard, A. F. (1989). Ecology of rhesus macaques (Macaca mulatta) in Northwest Pakistan. Int. J. Primatol. 10, 531–567. doi: 10.1007/BF02739364
Gonfiantini, R., Gratziu, S., and Tongiorgi, E. (1965). “Oxygen isotopic composition of water in leaves,” in Isotopes and Radiation in Soil Plant Nutrition Studies (Vienna: International atomic energy agency), 405–410.
Grine, F. E., Lee-Thorp, J., Blumenthal, S., Sponheimer, M., Teaford, M. F., Ungar, P. S., et al. (2020). “Stable carbon isotope and molar microwear variability of south African australopiths in relation to paleohabitats and taxonomy” in Dental Wear in evolutionary and biocultural contexts (New York: Elsevier Inc.), 187–223.
Hammer, Ø., Harper, D. A., and Ryan, P. D. (2001). Past: paleontological statistics software package for education and data analysis. Palaeontol. Electron. 76, 543–551. doi: 10.1016/j.bcp.2008.05.025
Hernando, R., Gamarra, B., Mccall, A., and Cheronet, O. (2021). Integrating buccal and occlusal dental microwear with isotope analyses for a complete paleodietary reconstruction of Holocene populations from Hungary. Sci. Rep. 11:7034. doi: 10.1038/s41598-021-86369-x
Hongo, S., Nakashima, Y., Akomo-Okoue, E. F., and Mindonga-Nguelet, F. L. (2017). Seasonal change in diet and habitat use in wild mandrills (Mandrillus sphinx). Int. J. Primatol. 39, 27–48. doi: 10.1007/s10764-017-0007-5
Hoshino, J. (1985). Feeding ecology of mandrills (Mandrillus sphinx) in campo animal reserve, Cameroon. Primates 26, 248–273. doi: 10.1007/BF02382401
Hughes, J. K., Elton, S., and O’Regan, H. J. (2008). Theropithecus and “out of Africa” dispersal in the Plio-Pleistocene. J. Hum. Evol. 54, 43–77. doi: 10.1016/j.jhevol.2007.06.004
Ibáñez, J. J., Jiménez-Manchón, S., Blaise, É., Nieto-Espinet, A., and Valenzuela-Lamas, S. (2020). Discriminating management strategies in modern and archaeological domestic caprines using low-magnification and confocal dental microwear analyses. Quat. Int. 557, 23–38. doi: 10.1016/j.quaint.2020.03.006
Jablonski, N. G. (2002). “Fossil Old World monkeys: the late Neogene radiation” in The primate fossil record. ed. W. Hartwig (Cambridge: Cambridge University Press), 225–300.
Jablonski, N., and Frost, S. (2010). “Cercopithecoidea” in Cenozoic mammals of Africa. eds. L. Werdelin and W. J. Sanders (Berkeley: University of California Press), 393–428.
Jablonski, N. G., Whitfort, M. J., Roberts-Smith, N., and Qinqi, X. (2000). The influence of life history and diet on the distribution of catarrhine primates during the Pleistocene in eastern Asia. J. Hum. Evol. 39, 131–157. doi: 10.1006/jhev.2000.0405
Jarvey, J. C., Low, B. S., Pappano, D. J., Bergman, T. J., and Beehner, J. C. (2018). Graminivory and fallback foods: annual diet profile of geladas (Theropithecus gelada) living in the Simien Mountains National Park, Ethiopia. Int. J. Primatol. 39, 105–126. doi: 10.1007/s10764-018-0018-x
Joleaud, L. (1926). “Les vestiges des anciennes associations biologiques de la Berbérie” in Compte Rendu Publié par le Secrétaire Général du Congrès. eds. A. Cattaui and G. Douin (Cairo: Congrès International de Géographie), 128–134.
Jolly, C. J. (1972). The classification and natural history of Theropithecus (Simopithecus) (Andrews, 1916), baboons of the African Plio-Pleistocene. Bull. Br. Museum Geol. 22, 1–124. doi: 10.5962/p.313829
Kato, A., Tang, N., Borries, C., Papakyrikos, A. M., Hinde, K., Miller, E., et al. (2014). Intra- and interspecific variation in macaque molar enamel thickness. Am. J. Phys. Anthropol. 155, 447–459. doi: 10.1002/ajpa.22593
Kay, R. F., and Hylander, W. L. (1978). “The dental structure of mammalian folivores with special reference to Primates and Phalangeroidea (Marsupialia),” in The Ecology of arboreal folivores. ed. G. Montgomery (Washington: Smithsonian Institution Press), 173–191.
Keeling, R. F., Graven, H. D., Welp, L. R., Resplandy, L., Bi, J., Piper, S. C., et al. (2017). Atmospheric evidence for a global secular increase in carbon isotopic discrimination of land photosynthesis. Proc. Natl. Acad. Sci. U. S. A. 114, 10361–10366. doi: 10.1073/pnas.1619240114
Koch, P. L., Tuross, N., and Fogel, M. L. (1997). The effects of sample treatment and diagenesis on the isotopic integrity of carbonate in biogenic hydroxylapatite. J. Archaeol. Sci. 24, 417–429. doi: 10.1006/jasc.1996.0126
Kohn, M. J. (2010). Carbon isotope compositions of terrestrial C3 plants as indicators of (paleo)ecology and (paleo)climate. Proc. Natl. Acad. Sci. U. S. A. 107, 19691–19695. doi: 10.1073/pnas.1004933107
Kohn, M. J., and Cerling, T. E. (2002). Stable isotope compositions of biological apatite. Phosphates geochemical, Geobiol. Mater. Importance 48, 455–488. doi: 10.2138/rmg.2002.48.12
Krigbaum, J., Berger, M. H., Daegling, D. J., and Scott McGraw, W. (2013). Stable isotope canopy effects for sympatric monkeys at Taï Forest, Côte d’Ivoire. Biol. Lett. 9, 20130466–20130410. doi: 10.1098/rsbl.2013.0466
Lahm, S. A. (1986). Diet and habitat preference of Mandrillus sphinx in Gabon: implications of foraging strategy. Am. J. Primatol. 11, 9–26. doi: 10.1002/ajp.1350110103
Lambert, J. E., Chapman, C. A., Wrangham, R. W., and Conklin-Brittain, N. L. (2004). Hardness of cercopithecine foods: implications for the critical function of enamel thickness in exploiting fallback foods. Am. J. Phys. Anthropol. 125, 363–368. doi: 10.1002/ajpa.10403
Lee-Thorp, J. A., Sponheimer, M., Passey, B. H., De Ruiter, D. J., and Cerling, T. E. (2010). Stable isotopes in fossil hominin tooth enamel suggest a fundamental dietary shift in the Pliocene. Philos. Trans. R. Soc. B Biol. Sci. 365, 3389–3396. doi: 10.1098/rstb.2010.0059
Lee-Thorp, J. A., van der Merwe, N. J., and Brain, C. K. (1989). Isotopic evidence for dietary differences between two extinct baboon species from Swartkrans. J. Hum. Evol. 18, 183–189. doi: 10.1016/0047-2484(89)90048-1
Levin, N. E., Haile-Selassieb, Y., Frostc, S. R., and Saylord, B. Z. (2015). Dietary change among hominins and cercopithecids in Ethiopia during the early Pliocene. Proc. Natl. Acad. Sci. U. S. A. 112, 12304–12309. doi: 10.1073/pnas.1424982112
Longinelli, A. (1984). Oxygen isotopes in mammal bone phosphate: a new tool for paleohydrological and paleoclimatological research? Geochim. Cosmochim. Acta 48, 385–390. doi: 10.1016/0016-7037(84)90259-X
Luz, B., and Kolodny, Y. (1985). Oxygen isotope variations in phosphate of biogenic apatites, IV. Mammal teeth and bones. Earth Planet. Sci. Lett. 75, 29–36. doi: 10.1016/0012-821X(85)90047-0
Madurell-Malapeira, J., Rodríguez-Hidalgo, A., Aouraghe, H., Haddoumi, H., Lucenti, S. B., Oujaa, A., et al. (2021). First small-sized Dinofelis: evidence from the Plio-Pleistocene of North Africa. Quat. Sci. Rev. 265:107028. doi: 10.1016/j.quascirev.2021.107028
Malone, M. A., MacLatchy, L. M., Mitani, J. C., Kityo, R., and Kingston, J. D. (2021). A chimpanzee enamel-diet δ13C enrichment factor and a refined enamel sampling strategy: implications for dietary reconstructions. J. Hum. Evol. 159:103062. doi: 10.1016/j.jhevol.2021.103062
Manthi, F. K., Cerling, T. E., Chritz, K. L., and Blumenthal, S. A. (2020). Diets of mammalian fossil fauna from Kanapoi, northwestern Kenya. J. Hum. Evol. 140:102338. doi: 10.1016/j.jhevol.2017.05.005
Martin, F., Plastiras, C. A., Merceron, G., Souron, A., and Boisserie, J. R. (2018). Dietary niches of terrestrial cercopithecines from the Plio-Pleistocene Shungura formation, Ethiopia: evidence from dental microwear texture analysis. Sci. Rep. 8, 14052–14013. doi: 10.1038/s41598-018-32092-z
Martínez, L. M., Estebaranz-Sánchez, F., Romero, A., Ibáñez, J. J., Hidalgo-Trujillo, L., Avià, Y., et al. (2022). Effectiveness of buccal dental-microwear texture in African Cercopithecoidea dietary discrimination. Am. J. Biol. Anthropol. 179, 678–686. doi: 10.1002/ajpa.24635
Mau, M., Südekum, K. H., Johann, A., Sliwa, A., and Kaiser, T. M. (2009). Saliva of the graminivorous Theropithecus gelada lacks proline-rich proteins and tannin-binding capacity. Am. J. Primatol. 71, 663–669. doi: 10.1002/ajp.20701
McCarroll, D., and Loader, N. J. (2004). Stable isotopes in tree rings. Quat. Sci. Rev. 23, 771–801. doi: 10.1016/j.quascirev.2003.06.017
McGraw, W. S., Pampush, J. D., and Daegling, D. J. (2012). Brief communication: enamel thickness and durophagy in mangabeys revisited. Am. J. Phys. Anthropol. 147, 326–333. doi: 10.1002/ajpa.21634
McGraw, W. S., Vick, A. E., and Daegling, D. J. (2011). Sex and age differences in the diet and ingestive behaviors of sooty mangabeys (Cercocebus atys) in the tai forest, Ivory Coast. Am. J. Phys. Anthropol. 144, 140–153. doi: 10.1002/ajpa.21402
McGraw, W. S., Vick, A. E., and Daegling, D. J. (2014). Dietary variation and food hardness in sooty mangabeys (Cercocebus atys): implications for fallback foods and dental adaptation. Am. J. Phys. Anthropol. 154, 413–423. doi: 10.1002/ajpa.22525
Mehlman, P. T. (1988). Food resources of the wild barbary macaque (Macaca sylvanus) in high-altitude fir forest, Ghomaran Rif. Morocco. J. Zool. 214, 469–490. doi: 10.1111/j.1469-7998.1988.tb03754.x
Meloro, C., and Elton, S. (2012). The evolutionary history and palaeo-ecology of primate predation: Macaca sylvanus from Plio-Pleistocene Europe as a case study. Folia Primatol. 83, 216–235. doi: 10.1159/000343494
Ménard, N. (1985). Le régime alimentaire de Macaca sylvanus dans différents habitats d’Algérie. I: Régime en chênaie décidue. Rev. d’écologie 40, 451–466. doi: 10.3406/revec.1985.5296
Ménard, N., and Mohamed, Q. (1999). Bark stripping and water availability: a comparative studt between Moroccan and Algerian barbary macaques (Macaca sylvanus). Rev. Estomatol. 54, 123–132. doi: 10.3406/revec.1999.2282
Ménard, N., and Vallet, D. (1986). Le régime alimentaire de Macaca sylvanus dans différents habitats d’Algérie: II.—Régime en forêt sempervir-ente et sur les sommets rocheux. Rev. d’écologie 41, 173–192. doi: 10.3406/revec.1986.5381
Ménard, N., and Vallet, D. (1996). “Demography and ecology of barbary macaques (Macaca sylvanus) in two different habitats” in Evolution and ecology of macaque societies. eds. J. E. Fa and D. G. Lindburg (Cambridge: Cambridge University Press), 106–131.
Merceron, G., Kallend, A., Francisco, A., Louail, M., Martin, F., Plastiras, C.-A., et al. (2021). Further away with dental microwear analysis: food resource partitioning among Plio-Pleistocene monkeys from the Shungura formation, Ethiopia. Palaeogeogr. Palaeoclimatol. Palaeoecol. 572:110414. doi: 10.1016/j.palaeo.2021.110414
Monson, T. A., and Hlusko, L. J. (2014). Identification of a derived dental trait in the Papionini relative to other old world monkeys. Am. J. Phys. Anthropol. 155, 422–429. doi: 10.1002/ajpa.22586
Moritz, G. L., Fourie, N., Yeakel, J. D., Phillips-Conroy, J. E., Jolly, C. J., Koch, P. L., et al. (2012). Baboons, water, and the ecology of oxygen stable isotopes in an arid hybrid zone. Physiol. Biochem. Zool. 85, 421–430. doi: 10.1086/667533
Oelze, V. M., Percher, A. M., Nsi Akoué, G., El Ksabi, N., Willaume, E., and Charpentier, M. J. E. (2020). Seasonality and interindividual variation in mandrill feeding ecology revealed by stable isotope analyses of hair and blood. Am. J. Primatol. 82, e23206–e23211. doi: 10.1002/ajp.23206
Olupot, W. (1998). Long-term variation in mangabey (Cercocebus albigena johnstoni Lydekker) feeding in Kibale National Park, Uganda. Afr. J. Ecol. 8, 70–72. doi: 10.1016/S1468-1641(10)60457-4
Ouahbi, Y., Aberkan, M., and Serre, F. (2001). Climatic effect on late Pleistocene mammals from the northern Rif Mountains, northern Morocco. Paleontol. Zhurnal 35, 641–646.
Parés, J., Álvarez, C., Pla-Pueyo, S., Benito-Calvo, A., Duval, M., Aouraghe, H., et al. (2020). “Magnetostratigraphy of the sedimentary fill of the Aïn Beni Mathar-Guefaït Basin (high plateau, E Morocco),” in AGU fall meeting (San Francisco).
Passey, B. H., Cerling, T. E., Schuster, G. T., Robinson, T. F., Roeder, B. L., and Krueger, S. K. (2005). Inverse methods for estimating primary input signals from time-averaged isotope profiles. Geochim. Cosmochim. Acta 69, 4101–4116. doi: 10.1016/j.gca.2004.12.002
Pederzani, S., and Britton, K. (2019). Oxygen isotopes in bioarchaeology: principles and applications, challenges and opportunities. Earth Sci. Rev. 188, 77–107. doi: 10.1515/9783110441154-001
Perelman, P., Johnson, W. E., Roos, C., Seuánez, H. N., Horvath, J. E., Moreira, M. A. M., et al. (2011). A molecular phylogeny of living primates. PLoS Genet. 7, e1001342–e1001317. doi: 10.1371/journal.pgen.1001342
Piñero, P., Agustí, J., Haddoumi, H., El Hammouti, K., Chacón, M. G., and Sala-Ramos, R. (2019). Golunda aouraghei sp. nov., the last representative of the genus Golunda in Africa. J. Vertebr. Paleontol. 39, 1–6. doi: 10.1080/02724634.2020.1742726
Piñero, P., Agustí, J., Oms, O., Fierro, I., Montoya, P., Mansino, S., et al. (2017). Early Pliocene continental vertebrate fauna at Puerto de la Cadena (SE Spain) and its bearing on the marine-continental correlation of the late Neogene of eastern Betics. Palaeogeogr. Palaeoclimatol. Palaeoecol. 479, 102–114. doi: 10.1016/j.palaeo.2017.04.020
Pomel, A. (1892). Sur un macaque fossile des phosphorites quaternaires de l’Algérie, Macacus trarensis. Comptes Rendus l’Académie des Sci. la vie 115, 157–160.
Poulsen, J. R., Clark, C. J., and Smith, T. B. (2001). Seasonal variation in the feeding ecology of the grey-cheeked mangabey (Lophocebus albigena) in Cameroon. Am. J. Primatol. 54, 91–105. doi: 10.1002/ajp.1015
Powell, L. E., Isler, K., and Barton, R. A. (2017). Re-evaluating the link between brain size and behavioural ecology in primates. Proc. R. Soc. B Biol. Sci. 284, 20171765–20171768. doi: 10.1098/rspb.2017.1765
Reitsema, L. J., Partrick, K. A., and Muir, A. B. (2015). Inter-individual variation in weaning among rhesus macaques (Macaca mulatta): serum stable isotope indicators of suckling duration and lactation. Am. J. Primatol. 78, 1113–1134. doi: 10.1002/ajp.22456
Robinson, J. R., Rowan, J., Campisano, C. J., Wynn, J. G., and Reed, K. E. (2017). Late Pliocene environmental change during the transition from Australopithecus to homo. Nat. Ecol. Evol. 1, 1–7. doi: 10.1038/s41559-017-0159
Rogers, E., Tutin, C., Parnell, R., Voysey, B., and Fernandez, M. (1994). “Seasonal feeding on bark by gorillas: an unexpected keystone food?,” in Abstracts from the XIVTH Congress of the International Primatological Society (Strasbourg), 154.
Romero, A., Galbany, J., De Juan, J., and Pérez-Pérez, A. (2012). Brief communication: short- and long-term in vivo human buccal-dental microwear turnover. Am. J. Phys. Anthropol. 148, 467–472. doi: 10.1002/ajpa.22054
Roos, C., Kothe, M., Alba, D. M., Delson, E., and Zinner, D. (2019). The radiation of macaques out of Africa: evidence from mitogenome divergence times and the fossil record. J. Hum. Evol. 133, 114–132. doi: 10.1016/j.jhevol.2019.05.017
Sahnouni, M., Parés, J. M., Duval, M., Cáceres, I., Harichane, Z., van der Made, J., et al. (2018). 1.9-million- and 2.4-million-year-old artifacts and stone tool–cutmarked bones from Ain Boucherit, Algeria. Science 362, 1297–1301. doi: 10.1126/science.aau0008
Sala-Ramos, R., Aouraghe, H., Haddoumi, H., Morales, J., Rodríguez-Hidalgo, A., Tornero, C., et al. (2022). Article Pleistocene and Holocene peopling of Jerada province, eastern Morocco: introducing a research project Le peuplement humain pendant le Pléistocène et l’ Holocène dans la province de Jerada, Maroc oriental: introduction d’ un projet de recherche. Bull. d’Archéologie Marocaine 27, 27–40.
Schulz, E., Calandra, I., and Kaiser, T. M. (2013). Feeding ecology and chewing mechanics in hoofed mammals: 3D tribology of enamel wear. Wear 300, 169–179. doi: 10.1016/j.wear.2013.01.115
Scott, R. S., Ungar, P. S., Bergstrom, T. S., Brown, C. A., Childs, B. E., Teaford, M. F., et al. (2006). Dental microwear texture analysis: technical considerations. J. Hum. Evol. 51, 339–349. doi: 10.1016/j.jhevol.2006.04.006
Scott, R. S., Ungar, P. S., Bergstrom, T. S., Brown, C. A., Grine, F. E., Teaford, M. F., et al. (2005). Dental microwear texture analysis shows within-species diet variability in fossil hominins. Nature 436, 693–695. doi: 10.1038/nature03822
Semprebon, G. M., Godfrey, L. R., Solounias, N., Sutherland, M. R., and Jungers, W. L. (2004). Can low-magnification stereomicroscopy reveal diet? J. Hum. Evol. 47, 115–144. doi: 10.1016/j.jhevol.2004.06.004
Sengupta, A., Mcconkey, K. R., and Radhakrishna, S. (2014). Seed dispersal by rhesus macaques Macaca mulatta in northern India. Am. J. Primatol. 76, 1175–1184. doi: 10.1002/ajp.22302
Sengupta, A., and Radhakrishna, S. (2016). Influence of fruit availability on fruit consumption in a generalist primate, the rhesus macaque Macaca mulatta. Int. J. Primatol. 37, 703–717. doi: 10.1007/s10764-016-9933-x
Shah, N. (2003). Foraging strategies in two sympatric mangabey species (Cercocebus agilis and Lophocebus albigena). PhD Dissertation, Stony Brook University.
Shapiro, A. E., Venkataraman, V. V., Nguyen, N., and Fashing, P. J. (2016). Dietary ecology of fossil Theropithecus: inferences from dental microwear textures of extant geladas from ecologically diverse sites. J. Hum. Evol. 99, 1–9. doi: 10.1016/j.jhevol.2016.05.010
Sirianni, J. E., and Swindler, D. R. (1985). Growth and development of the pigtailed macaque. Boca Raton: CRC Press.
Solounias, N., and Semprebon, G. M. (2002). Advances in the reconstruction of ungulate ecomorphology with application to early fossil equids. Am. Museum Novit. 3366, 1–49. doi: 10.1206/0003-0082(2002)366<0001:AITROU>2.0.CO;2
Souron, A. (2018). Morphology, diet, and stable carbon isotopes: on the diet of Theropithecus and some limits of uniformitarianism in paleoecology. Am. J. Phys. Anthropol. 166, 261–267. doi: 10.1002/ajpa.23414
Sponheimer, M., Alemseged, Z., Cerling, T. E., Grine, F. E., Kimbel, W. H., Leakey, M. G., et al. (2013). Isotopic evidence of early hominin diets. Proc. Natl. Acad. Sci. U. S. A. 110, 10513–10518. doi: 10.1073/pnas.1222579110
Stromer, E. (1913). Mitteilungen über Wirbeltierreste aus dem Mittelpliocän des Natrontales (Ägypten). Zeitschrift der Dtsch. Geol. Gesellschaft Band 65, 350–372.
Stromer, E. (1920). Mitteilungen über Wirbeltierreste aus dem Mittelpliocän des Natrontales (Ägypten). 5. Nachtrag zu 1. Affen. Sitz. Math.-Phys. Kl. Bay. Akad. Wiss. München: Verlag der Bayerischen Akademie der Wissenschaften, 345–370.
Teaford, M. F. (1993). “Dental microwear and diet in extant and extinct Theropithecus: preliminary analyses” in Theropithecus: The rise and fall of a primate genus. ed. N. G. Jablonski (Cambridge: Cambridge University Press), 331–350.
Teaford, M. F., and Oyen, O. J. (1989). Differences in the rate of molar Wear between monkeys raised on different diets. J. Dent. Res. 68, 1513–1518. doi: 10.1177/00220345890680110901
Tipple, B. J., Meyers, S. R., and Pagani, M. (2010). Carbon isotope ratio of Cenozoic CO2: a comparative evaluation of available geochemical proxies. Paleoceanography 25, 1–11. doi: 10.1029/2009pa001851
Tornero, C., Bǎlǎşescu, A., Ughetto-Monfrin, J., Voinea, V., and Balasse, M. (2013). Seasonality and season of birth in early Eneolithic sheep from Cheia (Romania): methodological advances and implications for animal economy. J. Archaeol. Sci. 40, 4039–4055. doi: 10.1016/j.jas.2013.05.013
Tosi, A. J., Detwiler, K. M., and Disotell, T. R. (2005). X-chromosomal window into the evolutionary history of the guenons (primates: Cercopithecini). Mol. Phylogenet. Evol. 36, 58–66. doi: 10.1016/j.ympev.2005.01.009
Tutin, C. E. G., Ham, R. M., White, L. J. T., and Harrison, M. J. S. (1997). The primate community of the lope reserve, Gabon: diets, responses to fruit scarcity, and effects on biomass. Am. J. Primatol. 42, 1–24. doi: 10.1002/(SICI)1098-2345(1997)42:1<1::AID-AJP1>3.0.CO;2-0
Ungar, P. S. (1996). Dental microwear of European Miocene catarrhines: evidence for diets and tooth use. J. Hum. Evol. 31, 335–366. doi: 10.1006/jhev.1996.0065
Ungar, P. S., Abella, E. F., Burgman, J. H. E., Lazagabaster, I. A., Scott, J. R., Delezene, L. K., et al. (2017). Dental microwear and Pliocene paleocommunity ecology of bovids, primates, rodents, and suids at Kanapoi. J. Hum. Evol. 140, 102315–102310. doi: 10.1016/j.jhevol.2017.03.005
Ungar, P. S., and Teaford, M. F. (1996). Preliminary examination of non-occlusal dental microwear in anthropoids: implications for the study of fossil primates. Am. J. Phys. Anthropol. 100, 101–113. doi: 10.1002/(SICI)1096-8644(199605)100:1<101::AID-AJPA10>3.0.CO;2-4
Uno, K. T., Rivals, F., Bibi, F., Pante, M., Njau, J., and de la Torre, I. (2018). Large mammal diets and paleoecology across the Oldowan–Acheulean transition at Olduvai Gorge, Tanzania from stable isotope and tooth wear analyses. J. Hum. Evol. 120, 76–91. doi: 10.1016/j.jhevol.2018.01.002
Williams, F. L. E., and Geissler, E. (2014). Reconstructing the diet and paleoecology of Plio-Pleistocene Cercopithecoides williamsi from Sterkfontein, South Africa. PALAIOS 29, 483–494. doi: 10.2110/palo.2014.046
Williams, F. L. E., and Holmes, N. A. (2011). Evidence of terrestrial diets in Pliocene Eurasian papionins (Mammalia: primates) inferred from low-magnification stereomicroscopy of molar enamel use-wear scars. PALAIOS 26, 720–729. doi: 10.2110/palo.2010.p10-139r
Williams, F. L., and Patterson, J. W. (2010). Reconstructing the paleoecology of Taung, South Africa from low magnification of dental microwear features in fossil primates. PALAIOS 25, 439–448. doi: 10.2110/palo.2009.p09-116r
Winkler, D. E., Clauss, M., Kubo, M. O., Schulz-Kornas, E., Kaiser, T. M., Tschudin, A., et al. (2022). Microwear textures associated with experimental near-natural diets suggest that seeds and hard insect body parts cause high enamel surface complexity in small mammals. Front. Ecol. Evol. 10, 1–16. doi: 10.3389/fevo.2022.957427
Yakir, D. (1997). “Oxygen-18 of leaf water: a crossroad for plant associated isotopic signals” in Stable isotopes: Integration of biological, ecological, and geochemical processes. ed. H. Griffiths (London: BIOS, Oxford), 147–168.
Keywords: habitat, paleodiet, Africa, buccal microtexture, occlusal microwear, stable isotopes
Citation: Ramírez-Pedraza I, Martínez LM, Aouraghe H, Rivals F, Tornero C, Haddoumi H, Estebaranz-Sánchez F, Rodríguez-Hidalgo A, van der Made J, Oujaa A, Ibáñez JJ, Mhamdi H, Souhir M, Aissa AM, Chacón MG and Sala-Ramos R (2023) Multiproxy approach to reconstruct fossil primate feeding behavior: Case study for macaque from the Plio-Pleistocene site Guefaït-4.2 (eastern Morocco). Front. Ecol. Evol. 11:1011208. doi: 10.3389/fevo.2023.1011208
Edited by:
Brooke Crowley, University of Cincinnati, United StatesReviewed by:
Gina Marie Semprebon, Bay Path University, United StatesMugino O. Kubo, The University of Tokyo, Japan
Copyright © 2023 Ramírez-Pedraza, Martínez, Aouraghe, Rivals, Tornero, Haddoumi, Estebaranz-Sánchez, Rodríguez-Hidalgo, van der Made, Oujaa, Ibáñez, Mhamdi, Souhir, Aissa, Chacón and Sala-Ramos. This is an open-access article distributed under the terms of the Creative Commons Attribution License (CC BY). The use, distribution or reproduction in other forums is permitted, provided the original author(s) and the copyright owner(s) are credited and that the original publication in this journal is cited, in accordance with accepted academic practice. No use, distribution or reproduction is permitted which does not comply with these terms.
*Correspondence: Iván Ramírez-Pedraza, ✉ ramirezpedrazaivan@gmail.com; Laura M. Martínez, ✉ lmartinez@ub.edu