- 1Department of Biological Sciences, Ohio University, Athens, OH, United States
- 2Center for Environmental Research, University of Bucharest, Bucharest, Romania
Migratory amphibians require movements to complete their biphasic life cycle, often across altered landscapes fragmented by roadways, which can have severe consequences on their populations. To manage this threat, transportation agencies have begun to implement exclusion fencing to separate natural areas from the roadway to prevent wildlife-vehicle collisions. Although fences are an effective conservation tool, the tendency of animals to access the road by circumventing the fence ends, known as the fence-end effect, threatens to jeopardize management efforts to reduce road associated mortality. One strategy to lessen the impacts of the fence-end effect is to construct fence-end treatments to block amphibian movement and guide the animals to safe crossing locations. By using experimental fence arenas, we examined how nine amphibian species responded to two alternative fence-end structures: horizontal v-shape and perpendicular fence-end treatments. Using a generalized linear model framework, we found both fence-end treatments to be an effective strategy to reduce the impacts of the fence-end effect, with our predictor variable, fence-end treatment, explaining most of the variation in amphibian response. Structure effectiveness also started to improve by 20% with each 7°C increase in temperature, however, this was not significant. Despite these promising findings, we also found for each additional 312 s an amphibians spent attempting to navigate around the experimental fence resulted in a 25% decline in structure effectiveness, suggesting longer fences are not an adequate protection measure to combat the fence-end effect for amphibians. In addition, Anaxyrus americanus was not found to differ in their response, performing equally well to both experimental fence-end treatments. In contrast, Rana spp., Pseudacris crucifer, and Notopthalmus viridescens showed a greater response to the horizontal v-shape fence-end treatment compared to the perpendicular fence-end treatment. Variation in response for Ambystoma spp. could not be detected due to a small sample size; however, no individuals responded positively to the perpendicular fence-end treatment. Guidelines for amphibian fences should continue to incorporate fence-end treatments into the design and implementation to mitigate for the fence-end effect, and preferably angle the fence-ends inward in the horizontal v-shape pattern with the fence ends diagonal to the road for migratory amphibians.
1. Introduction
Roads that fragment habitats and are constructed within 2 km of a wetland are a continuous source of additive mortality that results in the loss of hundreds of thousands of pond-breeding amphibians each year (Fahrig et al., 1995; Ashley and Robinson, 1996; Forman and Alexander, 1998; Forman et al., 2003; Glista et al., 2008; Gryz and Krauze, 2008; Fahrig and Rytwinski, 2009; Andrews et al., 2015). Unlike species that adapt their behavior to cope with road threats (Andrews and Gibbons, 2005), amphibians do not demonstrate road avoidance behaviors and will readily attempt to cross a road that bisects their migratory pathway (Beebee, 2013). Additionally, amphibians are generally small-bodied animals with slow movement patterns that commonly rely on cryptic coloration and immobility to respond to external threats, which prolongs the time spent crossing a road and increases the likelihood of being struck (Hels and Buchwald, 2001; Mazerolle et al., 2005; Andrews et al., 2006; Rytwinski and Fahrig, 2012). For these reasons, migratory amphibians frequently experience higher rates of road mortality compared to other vertebrates (Fahrig and Rytwinski, 2009). Therefore, preventing migratory amphibians from accessing the roadway during seasonal migration events is critical for the long-term sustainability of their populations (Jaeger and Fahrig, 2004; Rytwinski and Fahrig, 2012).
When the primary management objective is to reduce occurrences of road mortality caused by wildlife-vehicle collisions, installing an artificial barrier system to partition natural areas from traffic, called wildlife exclusion or barrier fences (hereafter, fences), is encouraged (Forman et al., 2003; van der Ree et al., 2015). Previous studies assessing changes in roadkill frequencies and their distribution after implementation of a fence found significant declines (86−98%) in the number of amphibian carcasses detected within the fence boundaries (Rytwinski et al., 2016). In addition, fences have also been shown to increase wildlife movement by directing animals to safe crossing locations, typically artificial structures built either above (bridges) or below (tunnels) the road called eco-passages (Forman et al., 2003; Andrews et al., 2015; van der Ree et al., 2015). Thus, if maintained, fences can be a long-lasting conservation tool for mitigating the detrimental effects of roads, particularly if paired with eco-passages (Dodd et al., 2004; Aresco, 2005). Although many studies have demonstrated fences improve use of eco-passages and effectively reduce the frequency of road mortality detected within the boundary walls, an increased concentration of road mortality occurring at the fence ends suggest management efforts are compromised (Dodd et al., 2004; Aresco, 2005; Rytwinski et al., 2016; Markle et al., 2017), an unintended consequence known as the “fence-end effect” (Huijser et al., 2016; Plante et al., 2018; Spanowicz et al., 2020).
To combat the fence-end effect, management guidelines propose installing a barrier system to block animals from circumventing the fence ends, called fence-end treatments (Huijser et al., 2016). For smaller, less mobile animals such as amphibians, two different types of fence-end treatments have been deployed: (1) bend the fence ends inward perpendicular to the road at a 90° angle or (2) bend the fence ends further inward, creating a 45° angle, in a horizontal v-shape (Huijser et al., 2015; Gunson et al., 2016). Animal movements can then either be guided back to the landscape from which they emerged or redirected back to eco-passages built under the road for amphibians (Dodd et al., 2004; Aresco, 2005; Huijser et al., 2016). Assessments to date suggest structure design for herpetofauna should be guided by the habitat requirements an animal needs to survive and reproduce (e.g., whether the animal can be guided back to the landscape from which they emerged or if they need to be redirected back to eco-passages to cross the road; Dodd et al., 2004; Aresco, 2005; Huijser et al., 2016). For migratory amphibians, if the terrestrial and wetland habitats are separated by a road, fence-end treatments should encourage them to move in the opposite direction of the structure toward an eco-passage. Thus, given the substantial financial commitment to implement road mitigation structures (Forman, 2000), more research consideration is warranted to determine if migratory amphibians respond to the current fence-end treatment recommendations to maximize the conservation outcome to preserve their populations.
The purpose of this study is to improve fence design practices by evaluating fence-end treatment effectiveness for nine commonly occurring migratory amphibians, for which high rates of road mortality endangers long-term persistence of these populations (Fahrig and Rytwinski, 2009; Hopkins et al., 2019). Specifically, our study objectives are (1) to determine if fence-end treatments mitigate for the fence-end effect, and (2) to compare the effectiveness of the perpendicular and horizontal v-shape fence-end treatments for migratory amphibians. To accomplish our objectives, we created experimental arenas with an attached fence-end treatment. As a result, we were able to observe if movement of migrating breeding adults were affected by the presence of an experimental fence-end treatment when they encountered a replica fence structure. If fence-end treatments effectively mitigated for the fence-end effect, we hypothesized more amphibians would turn away from the fence-end treatment and move around the fence end that remained unobstructed (i.e., toward the hypothetical eco-passage entrance). As a result, we expected less amphibians would circumvent the fence-end treatment side, which would suggest amphibian response to a fence-end treatment is deliberate. Thus, fence-end treatments would effectively lessen the intensity of the fence-end effect by hindering movement onto the road and guiding amphibian movements back to safer locations. In addition, previous studies have shown species-specific responses to different structural attributes of crossing structures (Woltz et al., 2008; Hopkins et al., 2019). Because amphibians are a diverse clade displaying distinct morphological and behavioral differences (Wells, 2007; Pfingsten et al., 2013) with varying degrees of vagility (Hels and Buchwald, 2001), we predicted amphibian species would display a species-specific response, showing a greater preference for one of the fence-end treatments.
2. Materials and methods
2.1. Experimental fence arenas
We constructed four experimental arenas to test amphibian response to either the perpendicular fence-end treatment or the horizontal v-shape fence-end treatment. The perpendicular fence-end treatment was created by bending the fence ends inward to create a 90° angle between the treatment and the fence (Figure 1) compared to the horizontal v-shape fence-end treatment that was bent further inward to create a sharper 45° angle between the treatment and the fence (Figure 2). These arenas were then placed within 3 m of an established breeding wetland along State Route 78 in Athens County, Ohio (39.449297 and −82.198720). We then positioned the arenas so that the fence-end treatment faced toward the terrestrial habitat to intercept reproductive adults as they migrated to the wetlands. Under this setup, we were able to observe how breeding adults navigated around a mitigation fence under natural conditions. Fence arenas were constructed from a 1 m tall silt fencing material held up with wood posts (Willson and Gibbons, 2010). The fence segments were 2 m long with a 0.5 m experimental fence-end treatment attached to either the left or right end. We then lined the top of each fence with multiple 142 g weights to create an overhanging lip. The fence arenas were set flush with an untreated plywood board (58/81 cm × 1.2 m × 2.4 m) to provide a level substrate with no obstructions to movement. Arenas were weathered onsite to create a naturally moist substrate for 1 month prior to the start of the trials.
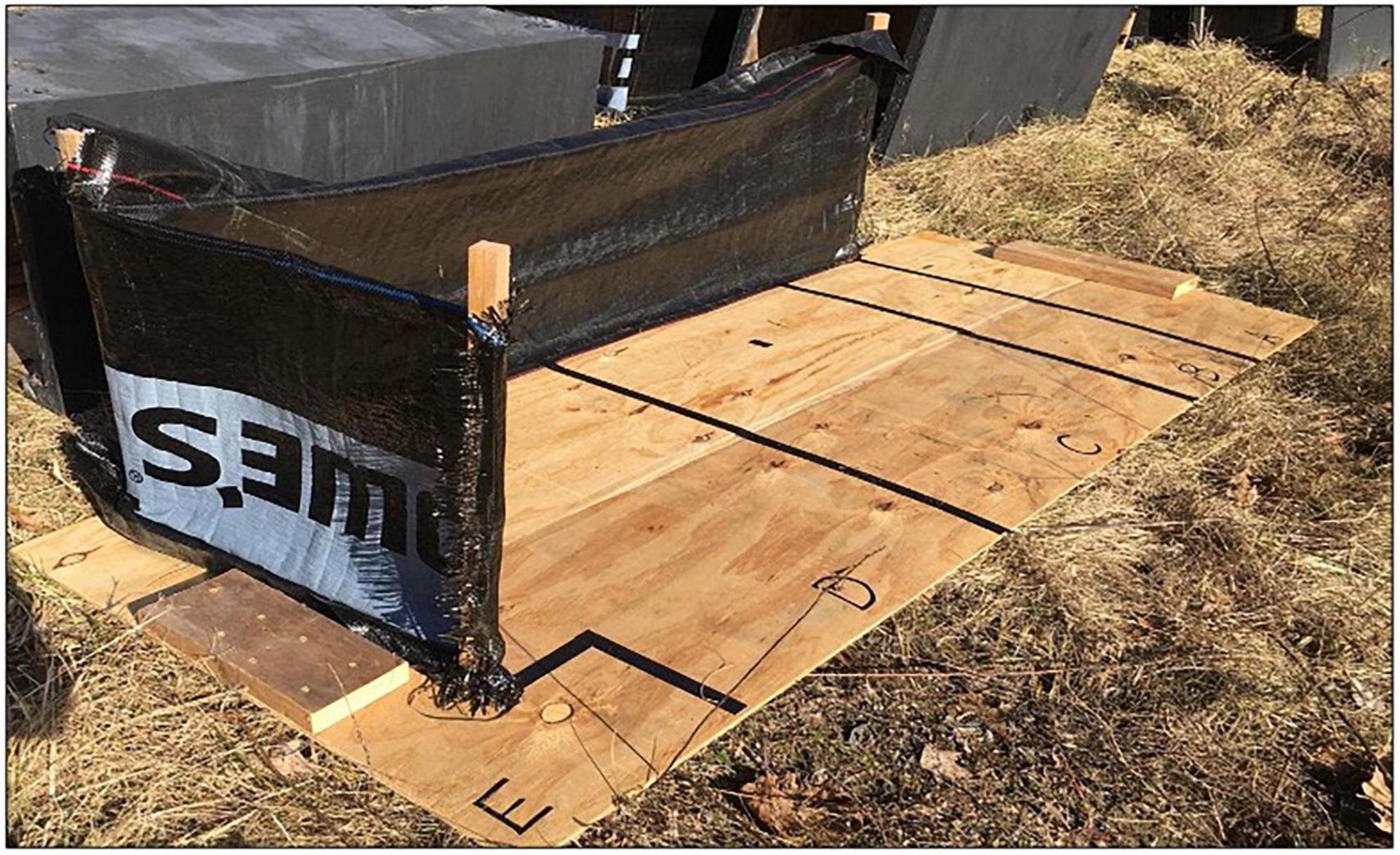
Figure 1. Experimental fence arenas used to evaluate the perpendicular fence-end treatment effectiveness in Athens County, Ohio, 2020. The arenas measured 58/81 cm × 1.2 m × 2.4 m with a 1 m experimental perpendicular fence-end treatment with a 90° angle attached to one side (showing attached to the right side). Amphibians were placed on a designated spot (black dot) in the middle of the experimental setup and positioned 15 cm from the fence.
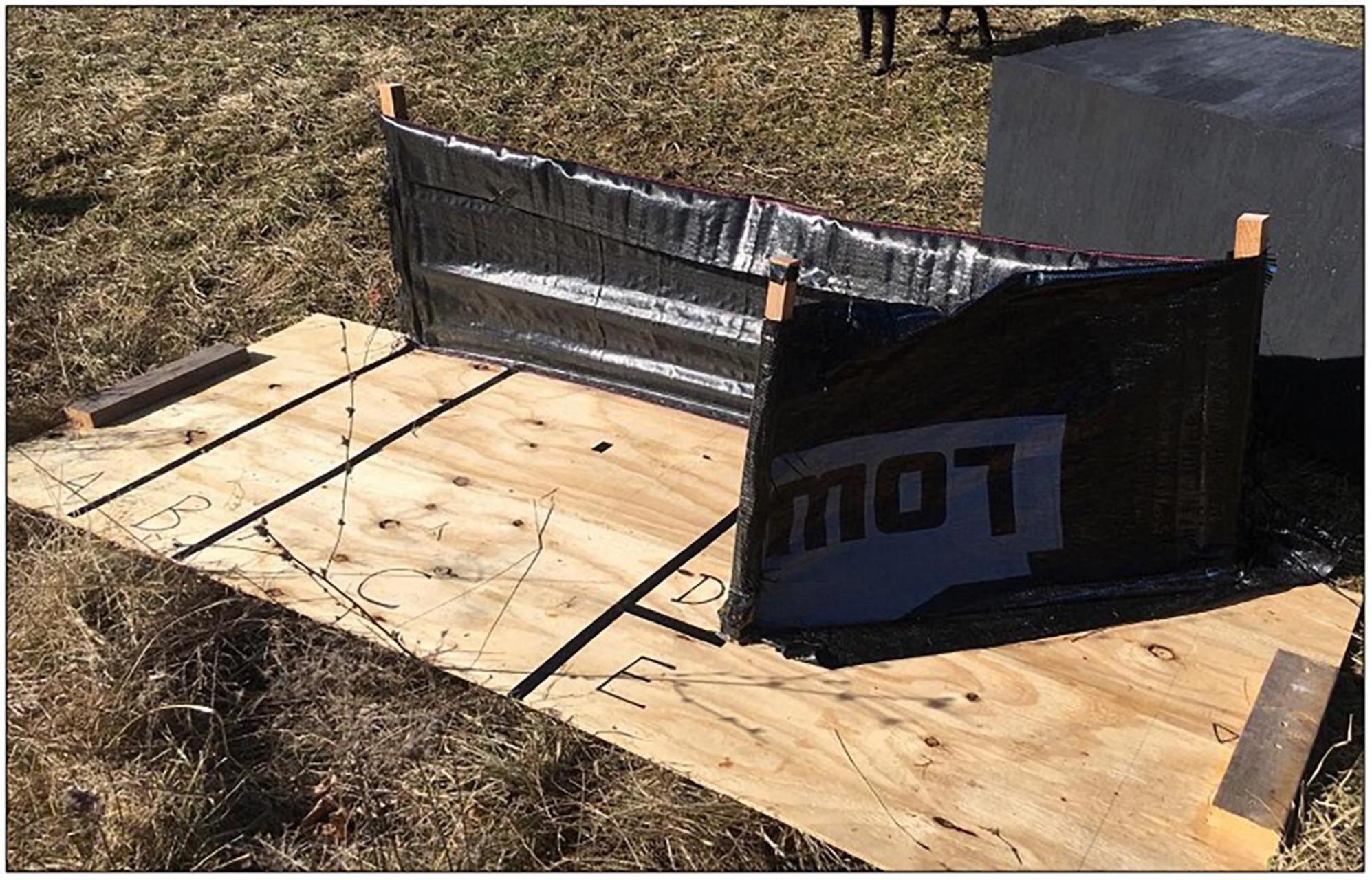
Figure 2. Experimental fence arenas used to evaluate the horizontal v-shape fence-end treatment effectiveness in Athens County, Ohio, 2020. The arenas measured 58/81 cm × 1.2 m × 2.4 m with a 1 m experimental horizontal v-shape fence-end treatment with a 45° angle attached to one side (showing attached to the left side). Amphibians were placed on a designated spot (black dot) in the middle of the experimental setup and positioned 15 cm from the fence.
2.2. Behavioral trials
Amphibians were collected from pitfall traps located at the end of an existing mitigation fence and hand collected from the road within 0.4 km from the experimental site (the total length of the migration corridor based on a multi-year road mortality survey; see Hopkins et al., 2019). The trials lasted seven and half weeks in the spring (4 February 2020−31 March 2020) and two and half weeks in the fall (11 October 2020−29 October 2020) for a total of 29 test nights. We processed all amphibians and recorded species, weight (g), snout-vent length (mm), and sex (Dodd, 2010). We tested nine amphibian species that commonly occur in the area: American Toad (Anaxyrus americanus), Spring Peeper (Pseudacris crucifer), Green Frog (Rana clamitans), Leopard Frog (R. pipiens), Pickerel Frog (R. palustris), Wood Frog (R. sylvatica), Red-spotted Newt (Notopthalmus viridescens), Jefferson Salamander (Ambystoma jeffersonianum), and the Spotted Salamander (A. maculatum). Anurans and Ambystoma spp. were all reproductive adults, whereas N. viridescens complete maturation after returning to natal ponds so were recorded as non-reproductive subadults (Petranka, 1998). Experimental animals were held individually in a 19 L plastic bucket prior to testing (Woltz et al., 2008). We were unable to avoid direct contact with animals prior to the trial because some amphibian species can climb the bucket wall. Therefore, to maintain consistency across all species, we placed all animals by hand on a designated spot in the middle of the experimental setup positioned 15 cm from the fence (Woltz et al., 2008). Prior to sunset, we randomized the order of the arenas to control for potential orientation cues influenced by calling males in the adjacent ponds or olfactory scent trails of other migratory individuals (Sinsch, 1991). All trials were conducted on rainy nights, starting at least 30 min after sunset, and typically concluded before midnight, except for a single explosive migration event that concluded at 3:00 a.m. After each trial, individuals were released into the adjacent wetland within 3 h of capture.
Test animals were observed as they traversed the experimental fence and the trial concluded when the test animal walked around one of the fence ends (treated vs. non-treated side). We recorded whether the animal responded to the treated side with the attached experimental fence-end treatment as success or fail. A “successful response” was recorded when an animal moved around the non-treated fence end either directly or after the animal approached the fence-end treatment but returned toward the non-treated side. A “fail response” was recorded when an animal escaped the fence arena by circumventing the experimental fence-end treatment. Anurans were given 20 min to make a fence-end choice (movement around one of the fence ends) and we extended the length of trial to 25 min for caudate to accommodate for their slower movements. If an animal remained on the board after the given time (i.e., trapped at the fence) or walked off the back of the board (i.e., abandoned the fence), a “no choice” was recorded and these animals were removed from analysis (Woltz et al., 2008). Amphibians were randomly assigned to one of the arenas, and only tested once and independent to other test individuals. Weather variables were taken from a weather station (KOHBUCHT1; 39.46°N, 82.189°W) located within 0.5 m from the field site.
2.3. Data analysis
Variation in our response variable, fence-end choice, was examined using a generalized linear model with a binomial distribution of errors in R (R Core Team, 2016). Fence-end choice was coded as 1 (success) or 0 (fail). Continuous variables were checked for correlation via Pearson’s correlation coefficient, r, to test the strength of association between −1 and 1. If sets of continuous variables were highly correlated (>|0.7|) we removed one variable of the set from analysis. Wind speed and wind gust were the only variables found to be highly correlated (r = 0.99). We then removed wind gust from analysis. Our final set of continuous variables were then standardized. We then used Akaike’s Information Criterion (AICc), corrected for small sample sizes, in a model selection framework to identify important factors influencing response to an experimental fence-end treatment for amphibians. Models incorporated combinations of the following parameters: (1) environmental variables, (2) experimental variables, and (3) individual variables (Table 1). Environmental variables included: temperature (°C) at the time of the experiment and precipitation rate (in/hr) because these variables have been positively correlated with amphibian activity (Gibbons and Bennett, 1974; Sexton et al., 1990). We also included how long after sunset the trial started, recorded as time since sunset, (the difference in seconds between the time the trial started from the time of last light; Hels and Buchwald, 2001) and date of the trial (day of the year coded as Julian day; Mazerolle, 2001). Experimental variables included treatment type (horizontal v-shape or perpendicular) and length of trial (the total time in seconds it took to move around one of the fence ends). Individual variables included sex, genus, and fence experience (coded as experienced for test animals collected from pitfall traps at the mitigation fence or virgin if the test animals were collected from the road). We then used a model selection procedure to determine the best supported models with the lowest AICc score using the package MuMin in R. When a top model was identified, we calculated the odds ratio expressing relative contribution of various predictor variables for explaining the likelihood of fence-end choice (R Core Team, 2016; Barton, 2020; Pinheiro et al., 2020).
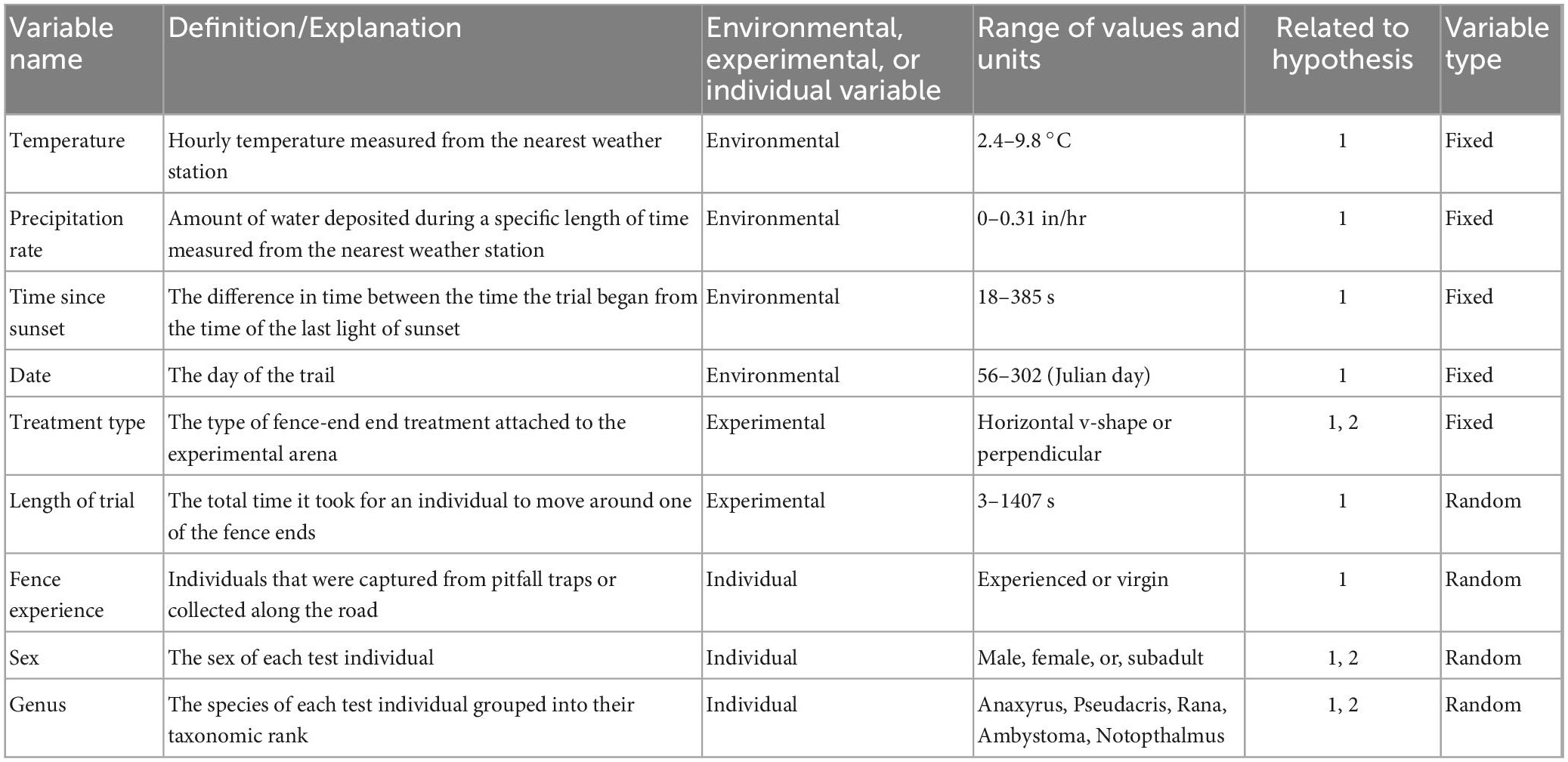
Table 1. Variables considered in the analysis for evaluating amphibian response to fence-end treatments in the spring and fall 2020.
Lastly, we used a goodness of fit G-test with a Yates correction to evaluate species-specific response to the perpendicular and horizontal v-shape experimental fence-end treatments. We observed amphibians were 50% likely to move either toward or away from the fence-end treatment upon their initial interactions with the fence. Therefore, we assumed a 50:50 probability of randomly circumventing either the treated side with the experimental fence-end treatment or the untreated side that remained unobstructed if the fence-end treatment had no effect on individual response. We grouped species into genera because of the small sample size of the Ambystoma spp. and Rana spp. Statistical significance was assessed at α = 0.05 via a two-tailed test.
3. Results
We collected 302 individuals during the spring migration and 34 individuals (all N. viridescens) during the fall migration: A. americanus, n = 149; P. crucifer, n = 57; Rana spp., n = 39; Ambystoma spp., n = 7; N. viridescens, n = 84 (Table 2). Most amphibians made a fence-end choice: Ambystoma spp. made a fence-end choice most frequently (100%), followed by the A. americanus (73%), P. crucifer (65%), N. viridescens (61%), and Rana spp. (56%). Of those that made a fence-end choice, between 72 and 75% successfully responded to the fence-end treatment for all genera except Ambystoma spp. where only 42% responded positively (Table 2). Of the individuals that did not make a fence-end choice, P. crucifer and A. americanus were the least likely to abandon the fence arena (9 and 20 %, respectively), whereas we observed 57 % of Rana spp. and 61% of N. viridescens demonstrate arena abandonment (Table 2). Rana spp. were the most likely to remain on the board for the entire length of the trial (41%) compared to P. crucifer (26%), N. viridescens (18%), and A. americanus (7%). N. viridescens were on average ( ± σ) the slowest to complete the fence arenas (400.1 ± 347.0 s), while Ambystoma spp. were on average ( ± σ) the fastest (233.1 ± 158.1 s), followed by P. crucifer (353.7 332.2 s), A. americanus (326.2 ± 275.0 s), and Rana spp. (323.2 ± 369.4 s).
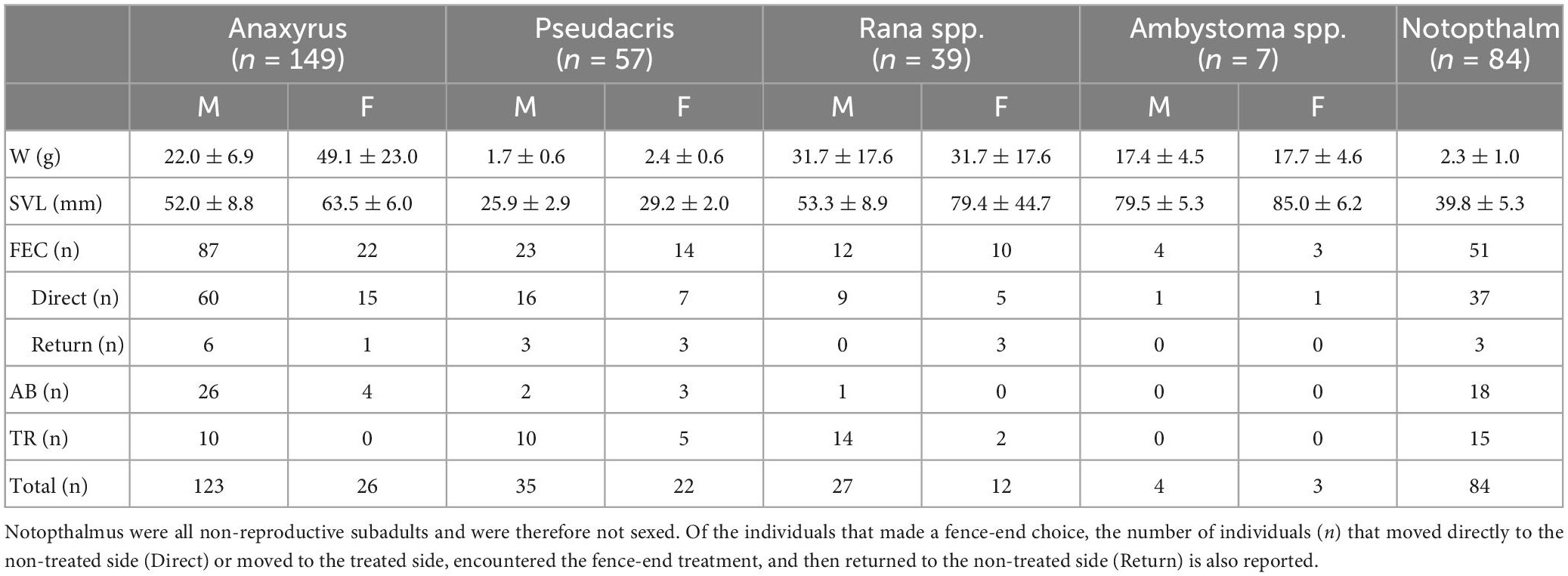
Table 2. Summary data for the experimental fence arenas including mean and standard deviation ± σ) for weight (W), snout-vent length (SVL), and the number of individuals (n) that made a FEC, fence-end choice; AB, abandoned the fence arena, or TR, remained trapped on the board after 20 min for anurans or 25 min for caudates for males (M) and females (F).
Fence-end treatment and length of trial were the top factors explaining amphibian response to a fence-end treatment in our dataset (Table 3). Both the horizontal v-shape and perpendicular fence-end treatments were positively associated with success and were efficient at directing amphibians toward the non-treated side of the fence (horizontal v-shape, odds = 4.87, 95% CI [3.01, 8.34], p < 0.0001; perpendicular, odds = 2.32, 95% CI [1.56, 3.52], p < 0.0001). Conversely, length of trial was negatively correlated with success; however, this was not significant (odds ratio = 0.75, 95% CI [0.56, 1.02], p = 0.06). Temperature at the time of the trial was also found to be a potentially important factor explaining treatment response, but the model that included this variable was slightly greater than 2.0 AIC units from the top model (Table 3). However, temperature also showed a positive trend associated with success, though the relationship was also not significant (odds ratio = 1.20, 95% CI [0.88, 1.63], p = 0.24). Our data suggests that for each additional standard deviation in time spent navigating (312 s) the fence arena, there was a 25% decline in the likelihood to respond successfully to a fence-end treatment. However, in contrast, for each additional standard deviation increase in temperature (7°C), there was a corresponding 20% increase in the likelihood of successfully responding to the experimental fence-end treatment.
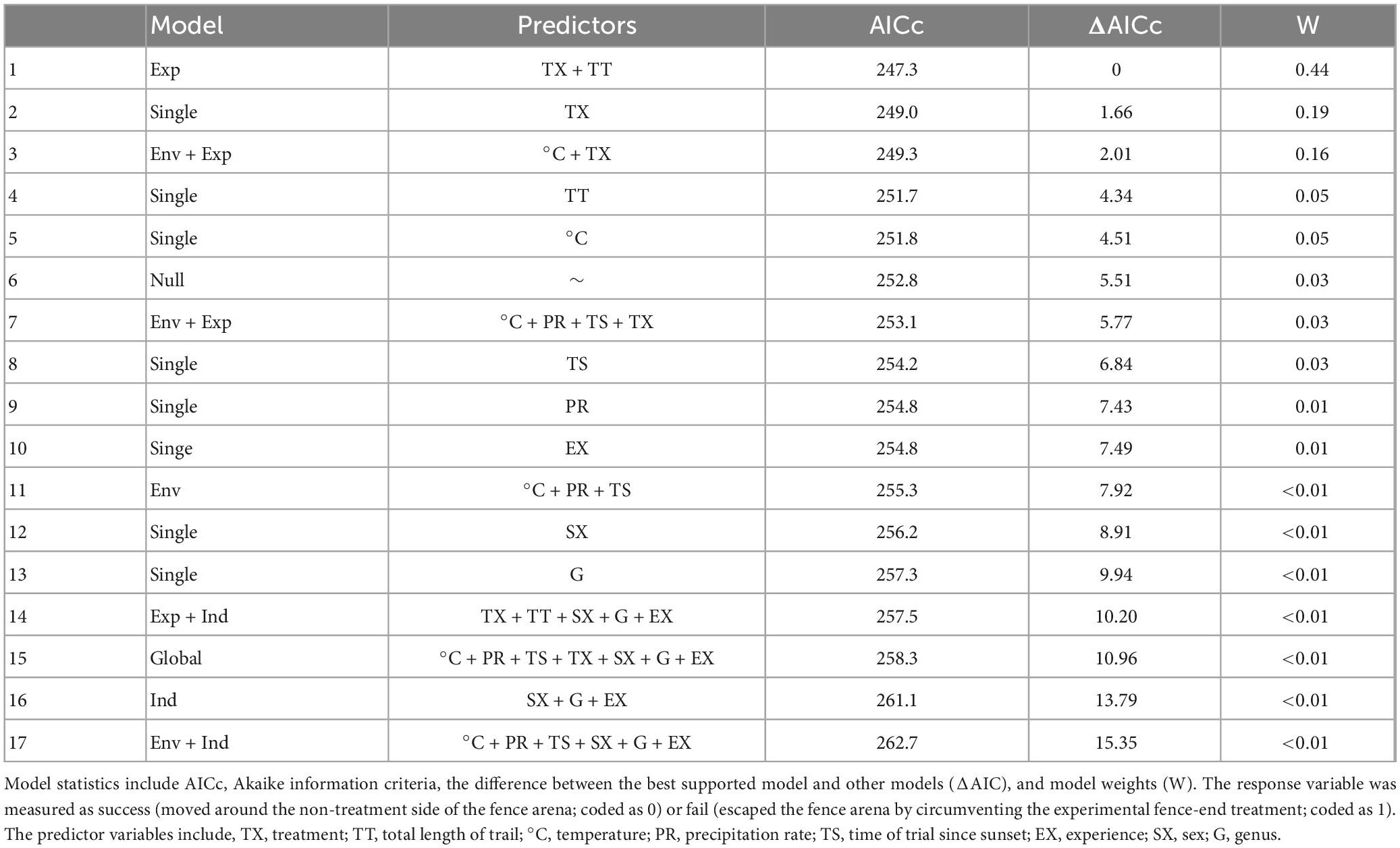
Table 3. Model selection for the fence arena experiment for all amphibians along state route 78 in Athens County, Ohio near the city of Nelsonville.
In testing for species-specific response to one of the fence-end treatments (Figure 3), A. americanus was the only species to demonstrate a successful response to both treatment types (horizontal v-shape, G = 10.12, p < 0.05; perpendicular, G = 17.29, p < 0.05), whereas Rana spp. and P. crucifer showed only a successful response for the horizontal v-shape fence-end treatment (Rana spp.: G = 5.42, p < 0.05; P. crucifer: G = 15.84, p < 0.001), but not the perpendicular fence-end treatment (Rana spp.: G = 0.76, p = 0.38; P. crucifer: G = 0.50, p = 0.48). Similarly, N. viridescens also showed a successful response for the horizontal v-shape treatment (G = 17.32, p < 0.001) but not the perpendicular fence-end treatment (G = 2.41, p = 0.12). Due to small sample size, no significant difference in a successful response was detected for Ambystoma spp. for either treatment type (horizontal v-shape: G = 1.46, p = 0.23; perpendicular: G = 2.53, p = 0.11).
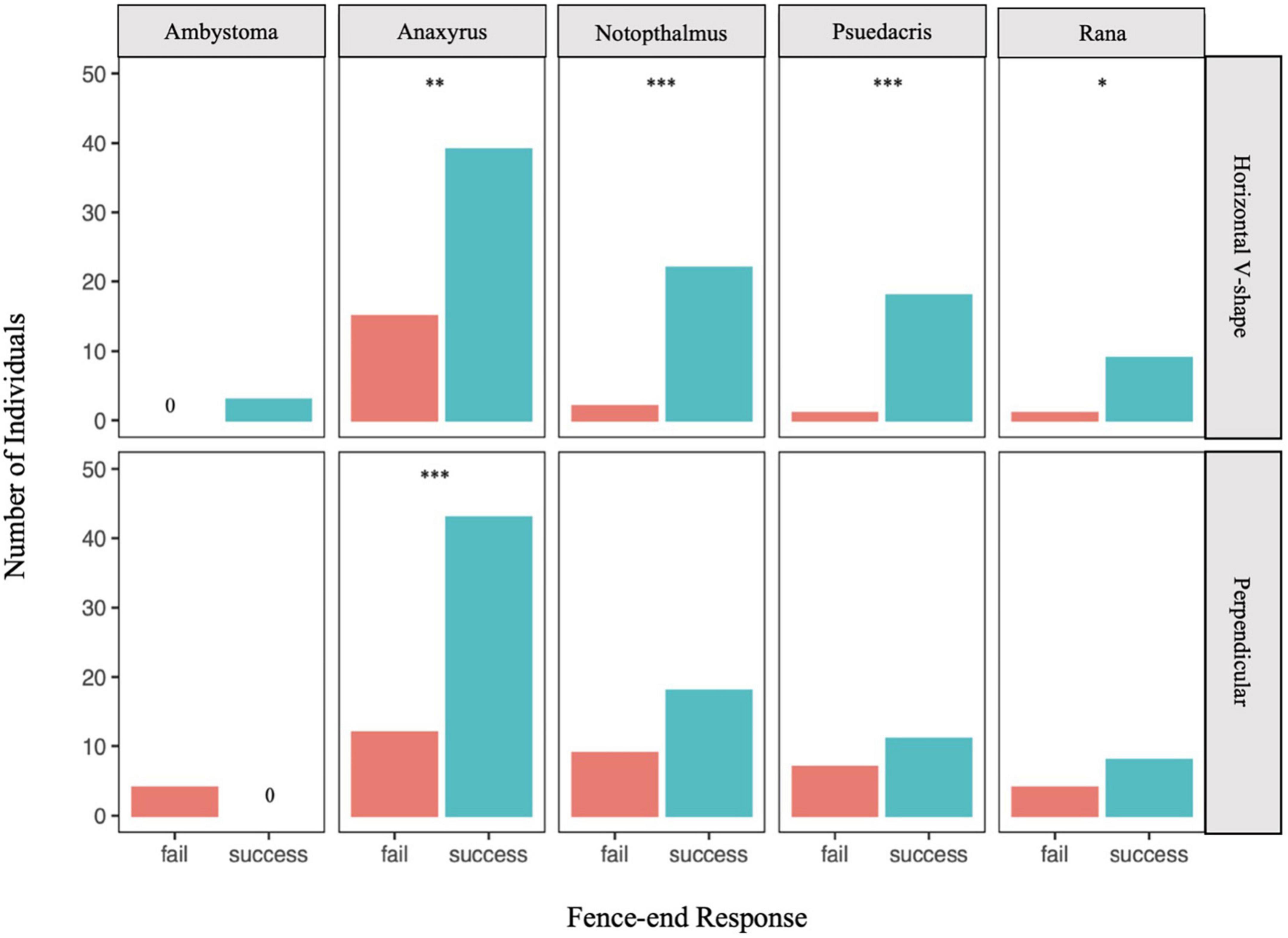
Figure 3. Number of individual amphibians that successfully responded to the fence-end treatment by moving around the side of the fence that remained unobstructed (success) or failed to respond by moving around the experimental fence-end treatment (fail). Asterisks denote significance level of the G-test with a Yates correction: *p < 0.05, **p = 0.001, ***p < 0.001.
4. Discussion
Our analysis indicates fence-end treatments are an effective conservation tool to mitigate for the fence-end effect for migratory amphibians; treatment ranked in our top three models, carrying a cumulative AICc weight of 0.79. Knowledge of fence-end treatment effectiveness is limited, as few studies have evaluated before and after measures of roadkill frequencies after installing a mitigation fence with fence-end treatments (see review by Rytwinski et al., 2016), however, those that have assessed effectiveness of mitigation fences with fence-end treatments reported similar benefits for herpetofauna. For example, Aresco (2005) found mitigation fences with perpendicular fence-end treatments protected an estimated 98% of aquatic turtles and 73% of amphibians, excluding hylids, from accessing the highway. However, these structures extended 50–150 m and followed the edge of the lake; thereby, created a “pseudo-pen” that primarily functioned to guide turtles and other herpetofauna back to the aquatic habitat from which they emerged. In another study conducted by Huijser et al. (2016), the authors found similar results for ungulates: longer fences greater than 5 km resulted in at least 80% reduction in wildlife-vehicle collisions at the fence ends. Elongated fence-end treatments like these structures can be problematic when the road bisects migration corridors and movement across the road is necessary for accessing resources and reproduction. Thus, failure to direct movement to safe crossing locations can be counterproductive to conservation efforts.
Our results also indicate the probability of trespassing a fence-end treatment increases the longer an amphibian spends attempting to navigate to an opening in the fence structure. Therefore, transportation agencies should be cautious of designing and implementing extended fences as a measure to combat the fence-end effect beyond the distance amphibians will approach a fence-end treatment and return to the eco-passage. Although it is beyond the scope of this study, further investigation of various fence lengths to determine the maximum effective distance between an eco-passage and a fence-end treatment, where an amphibian is still willing to return to the eco-passage, would be valuable and could influence important management recommendations for future road projects. In our study, when placed within 1 m of a fence-end treatment we found 16% of amphibians circumvented the experimental fence-end treatment and 33% either abandoned the arena or became trapped at the fence. Therefore, when the distance an amphibian must traverse along a fence wall before it encounters a fence-end treatment increases, failure to respond to a fence-end treatment should also be expected to increase. Thus, amphibians may show a higher propensity to circumvent the fence-end treatment rather than retrace their movements back toward the eco-passage. Further consideration is also required when considering extending fence length as a conservation measure to combat the fence-end effect because it may subsequently escalate the barrier effect of fences, which occurs when amphibians forgo breeding opportunities by abandoning migration efforts and returning to terrestrial habitats when they are unable to locate an opening to cross through (Jaeger and Fahrig, 2004; Matos et al., 2019). One solution for longer terminal fences is to angle the terminal fence segment toward the terrestrial or wetland habitat with the fence ends diagonal to the eco-passage to create a gradient that funnels animals in the direction of the eco-passage, opposite from the fence ends (Allaback and Laabs, 2002; Pagnucco et al., 2012). Thus, fence extensions to dilute the fence-end effect could be permissible under this scenario. However, this possibility remains untested, and in many cases, fences are constructed parallel to the road as landscape features such as ditches, hillsides, and private property often restrict fence design options. Ottburg and van der Grift (2019) suggested “tailor-made measures” that include increasing the number of eco-passages based on mean movement distance of different species and then installing fences beyond the location of the migration corridor. This is certainly a viable option, particularly if eco-passages are constructed at the edge of a well-defined migration corridor. We expect that mitigation structures constructed under this scenario to potentially eliminate most of the issues associated with the fence-end effect. Until further research is conducted to determine optimal fence length between a tunnel and fence-end treatment for amphibians, we suggest following suggestions of Broekmeyer and Steingröver (2001). The authors recommend tunnels should be built at distances up to 0.25 × the dispersal distance of the target species. However, for terminal fences, we would need to adjust for the target species return to the eco-passage, thus we suggest that fence length not exceed 0.125 × dispersal distance or mean movement distance (if known) of the target species. For existing mitigating structures that require retrofitting or new mitigation structures that require longer fences to span the entire length of a migration corridor, we also encourage installing multiple short horizontal v-shape barriers bent inward toward the eco-passage throughout the entire span of the terminal fence. By segmenting a terminal fence in this way, the v-shaped barriers can reduce the time and distance an amphibian would take to walk in the wrong direction until it eventually encounters a fence-end treatment. This would balance the need for longer fences by creating shorter segments within it, thus making the fences more cost-efficient. Furthermore, this fence design also accounts for multiple species with different movement capacities (Hels and Buchwald, 2001), thus expanding conservation efforts to protect the local community of amphibians rather than a single target species.
Furthermore, our data indicates that the probability of success may increase with warmer temperatures. Despite our attempt to isolate behavioral responses to alternative fence-end treatments under natural migratory conditions, COVID-19 restrictions placed on logistics, personnel, and work schedule, prohibited testing with prolonged breeders such as N. viridescens, P. crucifer, and Rana spp. when they are most active (Pfingsten et al., 2013). Although we were unable to capture the full migratory season for some genera, our data indicates the probability of success improves with warmer temperatures. Thus, we expect late season breeders (April to May) to display more willingness to respond to the fence-end treatment compared to their early breeding counterparts based on the positive trend observed between February and March. For this reason, future road mortality studies should consider seasonal effects when reporting roadkill frequencies when the fence-end effect is detected. Additionally, we were unable to test fence-end treatments for non-breeding adults or dispersing juveniles. Prior fence experience ranked low in our model selection, suggesting amphibian response to a fence-end treatment is not influenced by previous experiences. Thus, adults returning to overwintering sites may respond differently to alternative treatments. Furthermore, variation in treatment response between breeding and non-breeding individuals (e.g., subadults, juveniles) has not been well studied in road ecology literature but could influence the design and implementation of mitigation fences (e.g., whether the fence is built on the aquatic or terrestrial side of the road).
In testing for alternative behavioral responses to two different fence-end treatments, we found only A. americanus showed a similar response to both the horizontal v-shape and perpendicular treatment. In contrast, N. viridescens, P. crucifer, and Rana spp. only showed a successful response to the horizontal v-shape treatment, but not the perpendicular treatment. Additionally, due to the small sample size, no significant difference was found for Ambystoma spp. However, all test individuals failed to respond to the perpendicular fence-end treatment; in contrast all test individuals successfully responded to the horizontal v-shape fence-end treatment, suggesting the horizontal v-shape fence-end treatment may be a better option for these species as well. Overall, our study indicates the horizontal v-shape fence-end treatment is a more effective measure to mitigate for the fence-end effect compared to the perpendicular fence-end treatment for a variety of migratory amphibian species. Our results corroborate a study by Dodd et al. (2004) on mitigation structures consisting of perpendicular fence-end treatments. The authors found road mortality increased at the fence ends by 109 and 300%, respectively, suggesting perpendicular fence-end treatments are not optimal for amphibians, reptiles, or mammals. In contrast, Markle and Stapleton (2022) found that road mortality frequencies did not increase at the end-fence after installing j-shaped fence-end treatments (e.g., a horizontal v-shape fence-end treatment with a rounded corner), suggesting the treatment effectively prevented turtles from escaping the mitigation system and returned animals back to the habitat they emerged from. It is unclear what structure attributes contribute to the higher rate of success observed for the horizontal v-shape fence-end treatment for amphibians; however, we observed amphibians were more likely to turn around after becoming trapped in the corner created by the sharper angle, which may significantly decrease the fence-end effect but could also act as a prey-trap (Baxter-Gilbert et al., 2015). With few exceptions (Ford and Clevenger, 2010), little attention has been paid to examining predation events along fences; however, several studies evaluated the prey-trap hypothesis in eco-passage have largely found this risk to be minimal (Little et al., 2002; Ford and Clevenger, 2010). Furthermore, it is unclear if the angle of the fence-end treatment is the important factor influencing amphibian response compared to other potential structure attributes, such as the length of the fence-end treatment or if a rounded corner like the j-shape would perform better than the horizontal v-shape. In addition, our study suggests for some amphibian species (N. viridescens, P. crucifer, and Rana spp.) the angle of the fence-end treatment may be a sufficient measure of success given effectiveness improved when the fence ends were bent further inward at a sharper 45° angle. However, it remains unclear if a 45° angle is the optimal design for these species or if, for example, this angle represents a minimum or maximum limit above or below which the treatment becomes less effective. Thus, given the conservation crisis of protecting amphibian populations against local extirpation resulting from additive road mortality, asserting optimal design features is critical and requires further research attention.
Despite our attempts to isolate migratory amphibian response to alternative fence-end treatments, additional insights, and evidence are needed to optimize structure design for amphibians. Nevertheless, our study illustrates an experimental approach to begin resolving fence attributes for migratory amphibians, providing a general guideline for fence-end treatments. More specifically, the fence-end effect can be mitigated for by installing fence-end treatments and that these structures should be bent inward to guide amphibians away from the road and to eco-passages, preferably in the horizontal v-shape for migratory amphibians that require movement through associated crossing structures.
Data availability statement
The original contributions presented in this study are included in the article/Supplementary material, further inquiries can be directed to the corresponding author.
Ethics statement
This animal study was reviewed and approved by the Ohio University Animal Care and Use Committee.
Author contributions
KH planned and provided the logistical support that shaped the research. KH and KO conducted the fieldwork and data collection. KH and VP carried out the statistics analysis and were involved in writing the manuscript and providing critical feedback. All authors contributed to the article and approved the submitted version.
Funding
This work was supported by the Ohio Department of Transportation (ODOT), the Federal Highway Administration (Agreement #30218 SJN #135504), funded the fieldwork and equipment and Ohio University, Graduate College funded the materials used to make the experimental arenas.
Acknowledgments
We thank the Ohio Department of Transportation (ODOT), the Federal Highway Administration, and Ohio University for funding our research. We are grateful for the support of Drs. Molly Morris, Shawn Kuchta, and Willem Rosenberg for helpful advice and support. We thank to Dr. Shawn Kuchta for also assisting with the construction and maintenance of the experimental arenas. We are particularly grateful to our field volunteers, Jane Armstrong, Brennan Brindley, David Cole, and Alexa Tanner for extensive data collection. Special thanks to Julia Joos, Meredith Fitschen-Brown, and Mattea Lewis for assisting with data collection during a particularly explosive migration night. We also sincerely appreciate all the time and effort of the reviewers who provided valuable comments and suggestions on our manuscript.
Conflict of interest
The authors declare that the research was conducted in the absence of any commercial or financial relationships that could be construed as a potential conflict of interest.
Publisher’s note
All claims expressed in this article are solely those of the authors and do not necessarily represent those of their affiliated organizations, or those of the publisher, the editors and the reviewers. Any product that may be evaluated in this article, or claim that may be made by its manufacturer, is not guaranteed or endorsed by the publisher.
Supplementary material
The Supplementary Material for this article can be found online at: https://www.frontiersin.org/articles/10.3389/fevo.2023.1008462/full#supplementary-material
References
Allaback, M. L., and Laabs, D. M. (2002). Effectiveness of road tunnels for the Santa Cruz long-toed salamander. Trans. Western Section Wildlife Soc. 38, 5–8.
Andrews, K. M., and Gibbons, J. W. (2005). How do highways influence snake movement? behavioral responses to roads and vehicles. Copeia 2005, 772–782. doi: 10.1643/0045-8511(2005)005[0772:HDHISM]2.0.CO;2
Andrews, K. M., Gibbons, J. W., and Jochimsen, D. M. (2006). Literature synthesis of the effects of roads and vehicles on amphibians and reptiles federal highway administration (FHWA), U.S. department of transportation, report no. FHWA-HEP-08-005.
Andrews, K. M., Nanjappa, P., and Riley, S. P. (eds) (2015). Roads and ecological infrastructure: concepts and applications for small animals. Maryland: JHU Press. doi: 10.1353/book.39710
Aresco, M. J. (2005). Mitigation measures to reduce highway mortality of turtles and other herpetofauna at a north Florida lake. J. Wildl. Manag. 69, 549–560. doi: 10.2193/0022-541X(2005)069[0549:MMTRHM]2.0.CO;2
Ashley, P. E., and Robinson, J. T. (1996). Road mortality on the long point causeway, lake erie, ontario. Can. Field Nat. 110, 403–412.
Baxter-Gilbert, J. H., Riley, J. L., Lesbarrères, D., and Litzgus, J. D. (2015). Mitigating reptile road mortality: fence failures compromise ecopassage effectiveness. PLoS One 10:e0120537. doi: 10.1371/journal.pone.0120537
Beebee, T. J. (2013). Effects of road mortality and mitigation measures on amphibian populations. Conserv. Biol. 27, 657–668. doi: 10.1111/cobi.12063
Broekmeyer, M. E. A., and Steingröver, E. G. (2001). Handboek robuuste verbindingen: [ecologische randvoorwaarden]. Alterra 2001, 25–24.
Dodd, C. K. (ed.) (2010). Amphibian ecology and conservation: a handbook of techniques. Oxford: Oxford University Press.
Dodd, C. K., Barichivich, W. J., and Smith, L. L. (2004). Effectiveness of a barrier wall and culverts in reducing wildlife mortality on a heavily traveled highway in Florida. Biol. Conserv. 118, 619–631. doi: 10.1016/j.biocon.2003.10.011
Fahrig, L., and Rytwinski, T. (2009). Effects of roads on animal abundance: an empirical review and synthesis. Ecol. Soc. 14:20. doi: 10.5751/ES-02815-140121
Fahrig, L., Pedlar, J. H., Pope, S. E., Taylor, P. D., and Wegner, J. F. (1995). Effects of road traffic on amphibian density. Biol. Conserv. 73, 177–182. doi: 10.1016/0006-3207(94)00102-V
Ford, A. T., and Clevenger, A. P. (2010). Validity of the prey-trap hypothesis for carnivore-ungulate interactions at wildlife-crossing structures. Con. Bio. 24, 1679–1685. doi: 10.1111/j.1523-1739.2010.01564.x
Forman, R. T. (2000). Estimate of the area affected ecologically by the road system in the United States. Conserv. Biol. 14, 31–35. doi: 10.1046/j.1523-1739.2000.99299.x
Forman, R. T., and Alexander, L. E. (1998). Roads and their major ecological effects. Annu. Rev. Ecol. Evol. Syst. 29, 207–231. doi: 10.1146/annurev.ecolsys.29.1.207
Forman, R. T., Sperling, D., Bissonette, J. A., Clevenger, A. P., Cutshall, C. D., Dale, V. H., et al. (2003). Road ecology: science and solutions. Washington D.C: Island Press.
Gibbons, J. W., and Bennett, D. H. (1974). Determination of anuran terrestrial activity patterns by a drift fence method. Copeia 1974, 236–243. doi: 10.2307/1443029
Glista, D. J., Devault, T. L., and Dewoody, J. A. (2008). Vertebrate road mortality predominately impacts amphibians. Herpetol. Conserv. Biol. 3, 77–87.
Gryz, J., and Krauze, D. (2008). Mortality of vertebrates on a road crossing the Biebrza Valley (NE Poland). Eur. J. Wildl. Res. 54, 709–714. doi: 10.1007/s10344-008-0200-0
Gunson, W. K., Seburn, D., Kintsch, J., and Crowley, J. (2016). Best management practices for mitigating the effects of roads on amphibian and reptile species at risk in ontario. Ontario Ministry Nat. Res. For. 2016:122.
Hels, T., and Buchwald, E. (2001). The effect of road kills on amphibian populations. Biol. Conserv. 99, 331–340. doi: 10.1016/S0006-3207(00)00215-9
Hopkins, C. B., Harman, K. E., and Kuchta, S. R. (2019). Improving amphibian roadway mitigation to decrease mortality and increase connectivity by experimenting with ecopassage design (No. FHWA/OH-2019-27). Columbus: Ohio Department of Transportation, Office of Statewide Planning and Research.
Huijser, M. P., Fairbank, E. R., Camel-Means, W., Graham, J., Watson, V., Basting, P., et al. (2016). Effectiveness of short sections of wildlife fencing and crossing structures along highways in reducing wildlife–vehicle collisions and providing safe crossing opportunities for large mammals. Biol. Conserv. 197, 61–68. doi: 10.1016/j.biocon.2016.02.002
Huijser, M. P., Kociolek, A. V., Allen, T. D. H., and McGowen, P. (2015). Construction of guidelines for wildlife fencing and associated escape and lateral access control measures. national cooperative highway research program, transportation research board. Bozeman: Western Transportation Institute.
Jaeger, J. A., and Fahrig, L. (2004). Effects of road fencing on population persistence. Con. Bio. 18, 1651–1657. doi: 10.1111/j.1523-1739.2004.00304.x
Little, S. J., Harcourt, R. G., and Clevenger, A. P. (2002). Do wildlife passages act as prey-traps? Biol. Conserv. 107, 135–145. doi: 10.1016/S0006-3207(02)00059-9
Markle, C. E., Gillingwater, S. D., Levick, R., and Chow-Fraser, P. (2017). The true cost of partial fencing: evaluating strategies to reduce reptile road mortality. Wildl. Soc. Bull. 41, 342–350. doi: 10.1002/wsb.767
Markle, T., and Stapleton, S. (2022). Reduce vehicle-animal collisions with installation of small animal exclusion fences (No. MN 2022-19). Saint Paul, MN: Minnesota Department of Transportation.
Matos, C., Petrovan, S. O., Wheeler, P. M., and Ward, A. I. (2019). Short-term movements and behaviour govern the use of road mitigation measures by a protected amphibian. Anim. Conserv. 22, 285–296. doi: 10.1111/acv.12467
Mazerolle, M. J. (2001). Amphibian activity, movement patterns, and body size in fragmented peat bogs. J. Herpetol. 2001, 13–20. doi: 10.2307/1566017
Mazerolle, M. J., Huot, M., and Gravel, M. (2005). Behavior of amphibians on the road in response to car traffic. Herpetologica 61, 380–388. doi: 10.1655/04-79.1
Ottburg, F. G., and van der Grift, E. A. (2019). Effectiveness of road mitigation for common toads (Bufo bufo) in the Netherlands. Front. Ecol. Evol. 7:23. doi: 10.3389/fevo.2019.00023
Pagnucco, K. S., Paszkowski, C. A., and Scrimgeour, G. J. (2012). Characterizing movement patterns and spatio-temporal use of under-road tunnels by long-toed salamanders in waterton lakes national park. Canada. Copeia 2012, 331–340. doi: 10.1643/CE-10-128
Petranka, J. W. (1998). Salamanders of the United States and Canada. Washington, D.C: Smithsonian Institution Press.
Pfingsten, R. A., Davis, J. G., Matson, T. O., Lipps, G. J., Wynn, D. E., and Armitage, B. J. (2013). Amphibians of Ohio. Cleveland: Case Western Reserve University.
Pinheiro, J., Bates, D., DebRoy, S., Sarkar, D., and Team, R. C. (2020). Linear and nonlinear mixed effects models R package version 3.
Plante, J., Bélanger-Smith, K., Spanowicz, A. G., Clevenger, A. P., and Jaeger, J. A. (2018). Road mortality locations of small and medium-sized mammals along a partly-fenced highway in Quebec, Canada, 2012–2015. Data Brief 21, 1209–1215. doi: 10.1016/j.dib.2018.10.048
Rytwinski, T., and Fahrig, L. (2012). Do species life history traits explain population responses to roads? A meta-analysis. Biol. Conserv. 147, 87–98. doi: 10.1016/j.biocon.2011.11.023
Rytwinski, T., Soanes, K., Jaeger, J. A., Fahrig, L., Findlay, C. S., Houlahan, J., et al. (2016). How effective is road mitigation structures at reducing road-kill? A meta-analysis. PLoS One 11:e0166941. doi: 10.1371/journal.pone.0166941
Sexton, O. J., Phillips, C., and Bramble, J. E. (1990). The effects of temperature and precipitation on the breeding migration of the spotted salamander (ambystoma maculatum). Copeia 1990, 781–787. doi: 10.2307/1446443
Spanowicz, A. G., Teixeira, F. Z., and Jaeger, J. A. (2020). An adaptive plan for prioritizing road sections for fencing to reduce animal mortality. Conserv. Biol. 34, 1210–1220. doi: 10.1111/cobi.13502
van der Ree, R., Smith, D. J., and Grilo, C. (2015). Handbook of road ecology. New Jersey: John Wiley & Sons, Inc. doi: 10.1002/9781118568170
Wells, K. D. (2007). The ecology and behavior of amphibians. Chicago, IL: The University of Chicago Press. doi: 10.7208/chicago/9780226893334.001.0001
Willson, J. D., and Gibbons, J. W. (2010). Drift fences, coverboards, and other traps in Amphibian ecology and conservation: a handbook of techniques. Oxford: Oxford University Press. 229–245.
Keywords: road mortality, fence-end effect, amphibian tunnel, road mitigation, road ecology
Citation: Harman KE, Omlor K and Popescu V (2023) Evaluating fence-end treatments for migratory amphibians. Front. Ecol. Evol. 11:1008462. doi: 10.3389/fevo.2023.1008462
Received: 31 July 2022; Accepted: 22 March 2023;
Published: 17 April 2023.
Edited by:
Bilal Habib, Wildlife Institute of India, IndiaReviewed by:
Akanksha Saxena, Wildlife Institute of India, IndiaJochen Jaeger, Concordia University, Canada
Copyright © 2023 Harman, Omlor and Popescu. This is an open-access article distributed under the terms of the Creative Commons Attribution License (CC BY). The use, distribution or reproduction in other forums is permitted, provided the original author(s) and the copyright owner(s) are credited and that the original publication in this journal is cited, in accordance with accepted academic practice. No use, distribution or reproduction is permitted which does not comply with these terms.
*Correspondence: Kristine E. Harman, keharman19@gmail.com