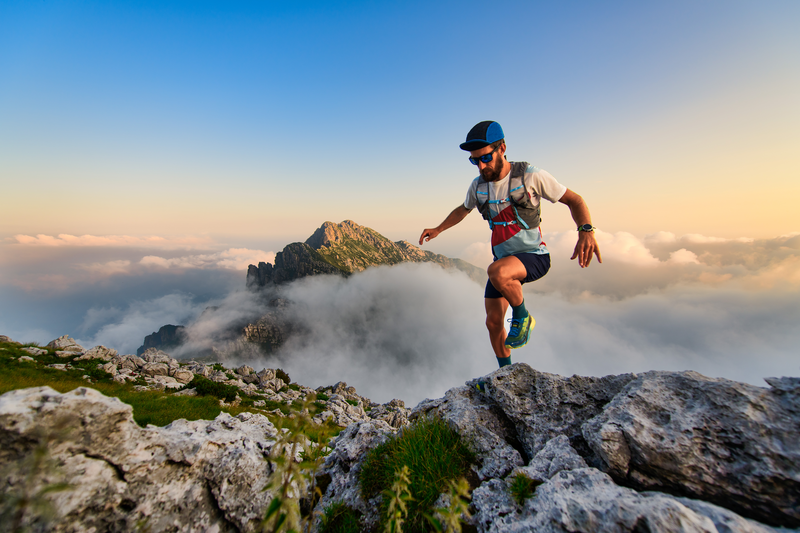
94% of researchers rate our articles as excellent or good
Learn more about the work of our research integrity team to safeguard the quality of each article we publish.
Find out more
ORIGINAL RESEARCH article
Front. Ecol. Evol. , 12 October 2022
Sec. Chemical Ecology
Volume 10 - 2022 | https://doi.org/10.3389/fevo.2022.993368
This article is part of the Research Topic Chemical Ecology of Arthropods, Plants, and Fungi: Bioactivity and Importance of Natural Products View all 5 articles
The scent glands of harvestmen (Opiliones) produce secretions of taxon-specific composition. One class of compounds, assumed to be a key group in opilionid chemosystematics and prevalent among sclerosomatid Eupnoi, was termed “sclerosomatid compounds” (SCs). Known compounds of this group comprise acyclic 4-methyl-branched ethyl-ketones and -alcohols as well as 2,4-dimethyl-branched primary alcohols and aldehydes, originally described from several species of North American Leiobuninae. We analyzed the scent gland secretions of Nelima ssp. (Sclerosomatidae, Leiobuninae) from two continents by gas chromatography-mass spectrometry, NMR, stereoselective synthesis, and chiral chromatography. We found a surprising chemical dichotomy: while North American N. paesserli produced typical acyclic SCs such as (E)-4-methyl-4-hexen-3-one, the secretions of European Nelima species exhibited a mixture of aromatic and aliphatic compounds, namely (R)-2-methyl-1-phenyl-pentan-3-one (MPP), 4-phenyl-2-butanone (PB), 2-phenylacetamide (PA), as well as (2R,4R)-2,4-dimethylhexanoic acid (DHA), all of which are new for the secretions of harvestmen. Remarkably, in N. sempronii, the secretions of juveniles (containing PB) differed from the secretions of adults (containing MPP and DHA). Though the chemistry of European Nelima species clearly diverged at first sight, all compounds detected either possess specific chemical motifs that allow an assignment to the class of SCs or appear to be biochemically/ontogenetically connected to SC-components. Thus, we here add novel compounds to the pool of SCs along with an extension of the definition of SCs to include aromatic compounds with SC-motifs. Furthermore, we describe a first case of juvenile-adult polymorphism for the exocrine secretions of harvestmen and provide a scheme of how secretions are regenerated.
Scent gland secretions of harvestmen (Arachnida: Opiliones), discharged from paired prosomal glands, serve a variety of functions, including defense against predators, antimicrobial protection and possibly also intraspecific communication (Martens, 1978; Holmberg, 1986; Machado et al., 2002; Schaider and Raspotnig, 2009). The secretions represent a phylogenetically old pool of characters, with an evolutionary history of several hundred millions of years (e.g., Garwood et al., 2014). Originating from a probably uniform chemistry of a hypothetical harvestman ancestor, the chemistry of scent glands has enormously diversified paralleling the diversification of harvestmen into different lineages and taxa (e.g., Gnaspini and Hara, 2007; Raspotnig, 2012). In extant harvestmen, scent gland secretions appear to be highly specific, and reflect the relations between taxa in a tree-like “chemophylogeny” (e.g., Raspotnig et al., 2015b,2017). In a nutshell, chemical classes in harvestmen scent gland exudates include naphthoquinones (Cyphophthalmi, and some Eupnoi and Dyspnoi), nitrogen-containing compounds (insidiatorean Laniatores), phenolics (some insidiatorean and many grassatorean Laniatores), benzoquinones (gonyleptoid Laniatores but also some Eupnoi), and various acyclic components.
The latter, i.e., acycles and their derivatives, represent the most heterogenous chemical class in the secretions of Opiliones, comprising methyl-ketones (Cyphophthalmi and some Dyspnoi), ethyl-ketones, alcohols and aldehydes (sclerosomatid Eupnoi), acids (gagrelline Sclerosomatidae and sporadically in Eupnoi), and lactones (some phalangiid Eupnoi). An overview on these compounds and their occurrence is given in Table 1.
Particularly among sclerosomatid Eupnoi, a distinct group of open-chain compounds – so-called “sclerosomatid compounds” (SCs) (sensu Raspotnig, 2012) – appears to be prevalent: SCs were originally characterized from a lineage of North American Leiobuninae (e.g., Blum and Edgar, 1971; Meinwald et al., 1971; Jones et al., 1976, 1977; Ekpa et al., 1985), but chemical investigations in Sclerosomatidae outside this lineage are completely missing. Consistently, the currently known pool of SCs is small, basically comprising a few ethyl-ketones and their corresponding alcohols, as well as unsaturated, 2,4-dimethyl-branched alcohols and aldehydes (Figure 1A). Thus, for the time being, both the actual taxonomic distribution of SCs across harvestmen and the delineation of the pool of SCs is unknown.
Figure 1. The growing pool of sclerosomatid compounds (SCs): past and present. Compounds: (1) 4-methyl-hexan-3-one; (2) 4-methyl-hexan-3-ol; (3) (E)-4-methyl-4-hexen-3-one; (4) 4-methyl-heptan-3-one; (5) 4-methyl-heptan-3-ol; (6) (E)-4-methyl-4-hepten-3-one; (7) (E)-4-methyl-4-hepten-3-ol; (8) (E)-4,6-dimethyl-6-octen-3-one; (9) (E)-4,6-dimethyl-6-nonen-3-one; (10) (E,E)-2,4-dimethyl-2,4-hexadien-1-ol; (11) (E,E)-2,4-dimethyl-2,4-hexadienal; (12) (E,E)-2,4-dimethyl-2,4-heptadien-1-ol; (13) (E)-4-methyl-4-hexen-3-ol; (14) (R)-2-methyl-1-phenyl-pentan-3-one; (15) (2R,4R)-2,4-dimethylhexanoic acid; (16) 4-phenyl-butan-2-one; (17) 2-phenyl-acetamide. (A) SCs as known from literature (Ekpa et al., 1985). Compounds 1–12 are “classical” SCs sensu stricto as already described from North American Leiobunum (nine species) and Hadrobunus (one species). All compounds are acyclic components, arranged in two chemically distinguishable subgroups. Subgroup 1 shows the “4-methyl-3-one” or “4-methyl-3-ol” motif (in red). Subgroup 2 is characterized by the “2,4-dimethyl-1-ol” and “2,4-dimethyl-1-al” motif (in blue). Compounds of subgroup 2 can be converted into compounds of subgroup 1 by a formal addition of an ethyl group to the carbonyl C atom. (B) Novel SCs, as found in the present study for European Nelima spp. and N. paessleri from USA. Compound 13 [=peak A: (E)-4-methyl-4-hexen-3-ol] is a new SC, showing the typical chemistry of SCs of subgroup 1. It has been detected for the first time in N. paessleri and represents the corresponding alcohol to (E)-4-methyl-4-hexen-3-one (the main compound of N. paessleri secretion). Compound 14 (=peak E: 2-methyl-1-phenyl-3-butanone) from the adults of European N. sempronii is an aromatic ethyl-ketone, showing the “4-methyl-3-one” motif of SCs of subgroup 1 in its side chain (in red). Compound 15 [=peak C: (2R,4R)-2,4-dimethylhexanoic acid], present in the adults of N. sempronii and N. narcisi, is considered a precursor to SCs of subgroup 2. Insertion of double bonds by dehydrogenation and reduction of the carboxyl group to an aldehyde or alcohol leads to SC compounds 10 and 11. Compound 16 (=peak D: 4-phenyl-butan-2-one) from the juveniles of N. sempronii and compound 17 (=peak F: 2-phenyl-acetamide) from the adults of N. narcisi are without SC motif, but may be biochemically connected to SCs: in N. sempronii, the replacement of 4-phenyl-butan-2-one by (2R)-2-methyl-1-phenyl-3-butanone occurs during maturation. For 4-phenyl-butan-2-one and 2-phenyl-acetamide, the classification with SCs is uncertain.
On the other hand, SCs have been considered potential key elements in harvestmen chemosystematics, presumably showing a much wider taxonomic distribution, probably also occurring outside North American Leiobuninae. Raspotnig (2012) speculated that these compounds might characterize at least the Sclerosomatidae as a whole, but similar open-chain compounds have also been detected in the secretions of Dyspnoi, Cyphophthalmi, and some Laniatores (Raspotnig et al., 2005b; Wouters et al., 2013; Shear et al., 2014). Thus, SCs may represent core structures linking several compound classes in harvestmen secretions or structures from which related compounds in other harvestmen lineages evolved (e.g., Schaider et al., 2018).
Here we address two goals: first, we aim to contribute to the delineation of the taxonomic distribution of SCs, in particular to what extent SCs characterize the Sclerosomatidae. Second, we want to expand the pool of SCs by investigating the gland chemistry of hitherto unstudied sclerosomatid taxa, as well as uncover possible biochemical connections of SCs to other classes of harvestmen gland chemistry.
We thus investigated the secretions of the hitherto chemically unstudied sclerosomatid genus Nelima (Roewer, 1910), comparing the chemistry of selected European species of Nelima (N. sempronii Szalay, 1951; N. narcisi Novak and Slana, 2003) to a North American species, N. paessleri (Roewer, 1910).
Twenty individuals of N. paessleri were collected in North America (California, Alameda County, Fire Trial, Berkeley) and sent alive to the Biological Institute of the University of Graz, Austria. One hundred and two individuals of N. sempronii were collected at different locations in Styria and Burgenland, both in Austria. In addition, seven individuals of N. narcisi were provided by a collaboration partner, Tone Novak (Maribor, Slovenia). Collection data are summarized in Table 2.
Secretion was either collected on filter paper in the moment of discharge from ozopores or by individual whole-body extraction. The latter technique is well suited to quantitatively collect the secretions of small-sized individuals, and leads to secretion discharge directly into the solvent. In all cases, methylene chloride was used. Analyses of methylene chloride extracts were performed on two GC-MS systems (GC 2000/Voyager and Trace GC/DSQI, both from Thermo, Vienna), equipped with a 30-m ZB-5 capillary column (5%-95%-dimethylpolysiloxane) and a 30-m Agilent CycloSil-B column [30% heptakis (2,3-di-O-methyl-6-O-t-butyl dimethylsilyl)-ß-cyclodextrin in DB-1701)], respectively. MS parameters were: EI at 70 eV, ion source at 200 (DSQ) and 170°C (Voyager), interface at 310 (DSQ) and 245°C (Voyager). We used the following GC parameters and temperature programs: injector at 240°C (both instruments); temperature program 1 (Trace GC-DSQ with ZB-5): 50°C (1 min), with 10°C/min to 300°C; 5 min isotherm; temperature program 2 (GC 2000-Voyager with CycloSil-B column): 70°C (1 min), with 2°C/min to 180°C, with 10°C/min to 230°C, then 5 min isotherm. All NMR spectroscopic measurements were done with a Bruker Avance II 700 MHz spectrometer equipped with a cryo-probe for biological samples and the synthetic compounds, solvent was in all cases deuterated chloroform.
Reference compounds such as racemic 2,4-dimethyl hexanoic acid, 4-phenyl-2-butanone and racemic 2-methyl-1-phenyl-pentan-3-one were purchased from Sigma, Vienna. 2-Phenylacetamide was from TCI Europe. (E)-4-methylhex-4-en-3-ol, (E)-4-methylhex-4-en-3-one, (R,S)/(R,R)- and (R,S)/(S,S)-2,4-dimethylhexanoic acid were synthesized as described below. For derivatization procedures (preparation of trimethylsilylesters), we used MSTFA [N-methyl-N-(trimethylsilyl)-trifluoro-acetamide in pyridine 2:1 containing 1% trimethylchlorosilane] from Sigma, Vienna. Retention indices (RIs) of compounds were calculated according to Van den Dool and Kratz (1963), using an alkane standard (C7–C36).
(E)-4-Methylhex-4-en-3-ol was synthesized similarly to a procedure already described (Hunt et al., 2013) via Grignard reaction with EtMgCl-solution (1M in THF). The alcohol was oxidized with activated MnO2 to the corresponding ketone, (E)-4-methylhex-4-en-3-one (Kamptmann and Brückner, 2013). (E)-2-Methyl-1-phenylpent-1-en-3-one was prepared according to Brenna et al. (2013), via an aldol condensation under basic conditions using benzaldehyde and 3-pentanone. In a following step, the reduction of this enone with baker’s yeast produced mainly the (S)-enantiomer of 2-methyl-1-phenylpentan-3-one (Kawai et al., 2001) (relation S/R was about 4/1).
The 2,4-dimethylhexanoic acids were prepared based on the method by Myers et al. (1997). (2R,4S)-2,4-Dimethylhexanoic acid resulted from stereoselective auxiliar guided alkylation of propionic anhydride with (S)-1-iodo-2-methylbutane and (+)-pseudoephedrine as an auxiliar followed by mild hydrolysis. Strong acidic conditions of the hydrolysis of the intermediate resulted in a 1:1 mixture of (2R,4S)-2,4-dimethylhexanoic acid and (2S,4S)-2,4-dimethylhexanoic acid. The mixture of (2R,4S)-2,4-dimethylhexanoic acid and (2R,4R)-2,4-dimethylhexanoic acid was prepared analogously from (±)-1-iodo-2-methylbutane and mild hydrolysis of the intermediate.
Individual whole body extracts and extracts from secretion-loaded filter paper pieces showed a consistent chromatographic pattern of two early-eluting, volatile compounds A and B (Table 3). Compound B (RImeasured = 933) was the major compound (about 90% of the secretion), exhibiting a molecular ion (M+) at m/z 112, along with fragment ions at m/z 83 and 55 (base ion). This EI fragmentation pattern corresponded to an already known SC compound from a related harvestman, (E)-4-methyl-4-hexen-3-one from Leiobunum nigropalpi (Wood, 1868) (“L. nigripalpi” in Jones et al., 1977). Compound A (RImeasured = 903; about 10% of the secretion) showed a mass spectrum similar to compound B (Table 3), but with some major fragments shifted by two mass units (e.g., molecular ion at m/z 114 and base ion at m/z 85). This pattern indicated an alcohol analogous to the ketone (E)-4-methyl-4-hexen-3-one, possibly (E)-4-methyl-4-hexen-3-ol. We further observed the loss of a methyl group (m/z 99) and the loss of water (m/z 96) from the molecular ion. The fragment ions at m/z 85 (C5H9O+) and 59 (C3H7O+; weak) evidently represented the longer (C3–C7) and shorter fragment (C1–C3) from α-cleavage at both sides of C3.
Table 3. Gas chromatographic-mass spectrometric data to the scent gland secretion components of Nelima spp.
For final identification of both compounds, reference standards for (E)-4-methyl-4-hexen-3-ol and (E)-4-methyl-4-hexen-3-one were synthesized, and fully matched the gas chromatographic and mass spectral data of extract compounds A and B, respectively. Since (E)-4-methyl-4-hexen-3-ol was a minor compound (and possibly an artifact arising from compound B), its stereochemistry (C3 is asymmetric) was not further investigated.
The secretion of juvenile individuals showed a single peak only in the gas chromatograms, compound D (RImeasured = 1,247; Figure 2). The compound appeared to be aromatic (phenyl cation at m/z 77) with benzylic substituents (m/z 91) and a molecular ion at m/z 148 (Figure 3). A library search (NIST 05) indicated that this compound was 4-phenyl-2-butanone (PB) (=benzylacetone). The secretions of older juveniles (subadults) and adults showed that compound D was gradually replaced by compound E (RImeasured = 1,358) in adults (Figure 2). Subadults still showed both components (with amounts of D already being diminished), whereas compound D got completely lost in adults. Compound E of subadults/adults appeared to be aromatic as well (fragment ion at m/z 77), exhibiting a molecular ion at m/z 176 along with fragment ions at m/z 147, 119, 91, and 57. A library search (NIST 05) did not lead to reliable hits to identify this compound. Assuming an aromatic ethyl ketone with a methyl-branch next to the carbonyl carbon atom, we preliminarily proposed the structure of 2-methyl-1-phenyl-pentan-3-one (MPP; spectrum and interpretation in Figure 4).
Figure 2. Chromatographic profiles of European Nelima sempronii show unusual aromatic compounds 4-phenyl-2-butanone and 2-methyl-1-phenyl-pentan-3-one along with juvenile-adult polymorphism. Compounds: C [(2R,4R)-2,4-dimethylhexanoic acid); D (4-phenyl-2-butanone); E (2R)-2-methyl-1-phenyl-pentan-3-one)]. (i) 4-Phenyl-2-butanone (compound D) prevails in the secretions of juveniles, while 2-methyl-1-phenyl-pentan-3-one (compound E) lacks or is present in traces only. (ii) In subadults, 4-phenyl-2-butanone diminishes, while 2-methyl-1-phenyl-pentan-3-one increases. (iii) In the adults of N. sempronii, 4-phenyl-2-butanone has been completely replaced by 2-methyl-1-phenyl-pentan-3-one. Males and females show the same pattern. Adults generally show an additional, non-aromatic compound C [=(2R,4R)-2,4-dimethylhexanoic acid)].
Figure 3. Mass spectral (EI) fragmentation of aromatic compound D (4-phenyl-2-butanone). Fragment ions from α-cleavage can be seen at m/z 43 and 133.
Figure 4. Mass spectral (EI) fragmentation of aromatic compound E [(2R)-2-methyl-1-phenyl-pentan-3-one]. Fragment ions from α-cleavage can be seen at m/z 57 and 147.
The identity of compounds D and E was finally confirmed by comparison to synthetic reference standards (for PB: RI = 1,247; for MPP: RI = 1,358). MPP contains a stereocenter at C2, and thus two enantiomers are possible. Correspondingly, the racemic MPP-reference standard showed two peaks by chiral chromatography, the later-eluting of which corresponded to compound E of our extracts. Using a synthetic 4:1 mixture of (S)-MPP:(R)-MPP as a reference, peak E was eventually identified as (R)-MPP (Figure 5).
Figure 5. Absolute configuration of 2-methyl-1-phenyl-pentan-3-one (MPP, compound E) in the extracts of Nelima sempronii. Identification of (2R)-2-methyl-1-phenyl-pentan-3-one (R-MPP) using a chiral ß-cyclodextrin-phase.
The secretions of adults also showed an early-eluting compound C (RImeasured = 1,104) which appeared to be 2,4-dimethylhexanoic acid (2,4-DHA), as indicated by the interpretation of its EI fragmentation pattern (Figure 6). 2,4-DHA is a chiral compound, possessing two stereocenters at C2 and C4, hypothetically leading to four possible stereoisomers (RR-2,4-DM; SS-2,4-DM; RS-2,4-DM; SR-2,4-DHA). An authentic racemic reference standard (i.e., containing all four stereoisomers) showed two peaks on a (non-chiral) apolar phase (at RI = 1,104 and 1,110), corresponding to two enantiomeric pairs of the compound. Compound C of our extracts corresponded to the earlier-eluting peak. Derivatization of the synthetic 2,4-DHA racemate to TMS-derivatives led to a peak-shift and produced two new, better separated peaks (RI = 1,148 and 1,156), with EI spectra characteristic of 2,4-DHA-TMS-esters (weak M+ at m/z 216; M-CH3 at m/z 201; McLafferty fragment at m/z 146; dimethylsilanol-ion at m/z 75; trimethylsilyl-ion at m/z 73). Chromatographically, the TMS-ester of compound C (RI = 1,148) again corresponded to the earlier-eluting peak. A comparison of the NMR data of compound C of our extract to data of synthetic 2,4-DHAs (Myers et al., 1997) suggested the presence of (S,S)- or/and (R,R)-2,4-DHA, but not (R,S)- nor (S,R)-2,4-DHA. In order to provide correct NMR resonance assignments for future reference and to finally uncover the absolute configuration of 2,4-DHA in our extracts, we used the following synthetic references: a mixture of (R,S)/(R,R)-2,4-DHA and a mixture of (R,S)/(S,S)-2,4-DHA, in total containing three stereoisomers of the compound (namely RS, RR, SS). All three stereoisomers were separable on the chiral phase used, with compound C corresponding to (R,R)-2,4-DHA (Figure 7).
Figure 6. Mass spectral (EI) fragmentation of compound C [=(2R,4R)-2,4-dimethylhexanoic acid]. The base ion at m/z 74 arises from McLafferty rearrangement. The molecular ion (at m/z 144) is very weak, not clearly visible in the spectrum.
Figure 7. Absolute configuration of 2,4-dimethylhexanoic acid (DHA, compound C) in the extracts of Nelima sempronii. Identification of (2R,4R)-2,4-dimethylhexanoic acid (2R,4R-DHA) using a chiral ß-cyclodextrin-phase.
The secretion of adult specimens of N. narcisi showed four different chromatographic profiles (Figure 8), namely (i) compound C only, or (ii) a mixture of C and D, or (iii) a mixture of C, D, and F, or (iv) a mixture of C and F. As in N. sempronii, compound C was identified as (R,R)-2,4-DHA by chiral chromatography. Compound F (RImeasured = 1,399) was an aromatic chemical with a molecular ion at m/z 135, and its EI mass spectrum matched that of 2-phenylacetamide (PA) (Figure 9). The identity of compound F as PA was finally confirmed by comparison to an authentic reference.
Figure 8. Different profiles of the secretion of Nelima narcisi adults, possibly indicating different states of secretion biosynthesis/regeneration of secretion after glandular depletion: hypothetically, (2R,4R)-2,4-dimethylhexanoic acid is produced first (i), followed by the production of 4-phenyl-2-butanone (ii) which is gradually converted/replaced by 2-phenylacetamide (iii,iv). Compounds: C (2,4-dimethylhexanoic acid); D (4-phenyl-2-butanone); and F (2-phenylacetamide).
Figure 9. Mass spectral (EI) fragmentation of aromatic compound F (2-phenylacetamide) from Nelima narcisi. Fragment ions from α-cleavage can be seen at m/z 44; the fragment at m/z 91 indicates benzylic cleavage.
The term “sclerosomatid compounds” (SCs) was introduced to the field of harvestmen chemosystematics to assign a distinct chemical class of open-chain secretion components that were expected to characterize the scent gland secretions of sclerosomatid Eupnoi (Raspotnig, 2012). Formally, the family Sclerosomatidae includes four subfamilies: Leiobuninae, Sclerosomatinae, Gagrellinae, and Gyantinae. We now know that gagrellines may rely on acid-rich secretions (Raspotnig et al., 2022) and gyantines are no sclerosomatids, but phalangiids (Hedin et al., 2012) and they produce benzoquinonic secretions (Raspotnig et al., 2017). Thus, SCs may occur in leiobunines and sclerosomatines or at least in a part of these: so far, SCs have been documented for North American Leiobunum and Hadrobunus species of the clade “US Leiobunines” (sensu Hedin et al., 2012) and for Nelima spp. (this study). Preliminary studies additionally indicate the occurrence of SCs in some, but not all European Leiobunum species and in some representatives of Sclerosomatinae (Raspotnig, preliminary data). Thus, SCs do not characterize all Sclerosomatidae in their traditional sense, but may prevail in the secretions of representatives of the two subfamilies, Leiobuninae and Sclerosomatinae.
Sclerosomatid compounds sensu stricto, as currently described in literature, comprise 12 compounds (Figure 1A). The compounds show characteristic motifs that allow a classification into two subgroups: we propose a “subgroup 1” of 4-methyl-branched ethyl-ketones and their corresponding alcohols (Figure 1A in red). “Subgroup 2” comprises compounds that share the motif of 2,4-dimethyl-branched di-unsaturated primary alcohols and aldehydes (Figure 1A in blue). Both subgroups appear to be biochemically connected: SC compounds of subgroup 2 are convertible into compounds of subgroup 1 by a formal addition of an ethyl residue at the carbonyl atom of the molecule. We here add five compounds from the secretions of Nelima to the hitherto existing pool of SCs (Figure 1B). Apart from typical SCs of subgroup 1 such as (E)-4-methyl-4-hexen-3-one/(E)-4-methyl-4-hexen-3-ol from the North American N. paessleri, we also add less typical aromatic compounds from European Nelima spp. that either carry a SC motif or are biochemically related to SCs, as outlined below.
Nelima is a genus of sclerosomatid harvestmen in the subfamily Leiobuninae. The genus comprises about 45 species which are mainly distributed in the Holarctic (Europe, Asia, and North America including Mexico). In his early publication on Central and South European species of Nelima, Martens (1969) already noted the morphological uniformity of Nelima species and therefrom arising difficulties in differentiating and delineating them from Leiobunum species. Indeed, Nelima is a nightmare for taxonomists: species delimitation is still based on the presence/absence of pro- and retrolateral rows of coxal denticles – which is no appropriate character to characterize Nelima, but considered to be a plesiomorphic feature among Sclerosomatidae. Recent genetic studies on the phylogeny of sclerosomatid harvestmen reflect this situation, and Nelima species pop-up at various positions in phylograms, clearly indicating the polyphyly of this genus (Hedin et al., 2012).
On the other hand, – or even because of this possible polyphyly – Nelima arises as an interesting model to study its scent gland chemistry, which might represent an independent set of taxonomically important data to characterize (the species of) this genus.
Interestingly, we found chemically dichotomic secretions: while N. paessleri from North America showed typical SCs in their secretions, the three Nelima species from Europe produced aromatic compounds along with a 2,4-dimethyl-branched carboxylic acid. North American N. paessleri produces SCs of subgroup 1, with both compounds of its secretion clearly showing the 4-methyl-3-one/-3-ol-motif. The main component in the secretion of N. paessleri is (E)-4-methyl-4-hexen-3-one, an already described SC, originally detected in L. nigropalpi (Jones et al., 1977). (E)-4-Methyl-4-hexen-3-ol has not yet been reported and adds to the pool of SCs of subgroup 1. (E)-4-Methyl-4-hexen-3-ol is a minor compound in the Nelima chromatograms, and possibly even an artifact arising from (E)-4-methyl-4-hexen-3-one in the hot GC injector. In our experiments, synthetic (E)-4-methyl-4-hexen-3-one also showed small amounts of the corresponding alcohol when analyzed under the same conditions. In the phylogenies of Hedin et al. (2012), N. paessleri shows up as a member of the clade “US Nelima”, being quite distinct from “US Leiobunines”, for which SCs were previously reported. In these terms, “US Leiobunines” (including all chemically analyzed North American Leiobunum and Hadrobunus species) share a similar chemistry with “US Nelima”, supporting the idea of a characteristic, widespread and uniform SC chemistry for North American Leiobuninae.
By contrast, European representatives of Nelima show a set of compounds distinctly different from SCs at first sight, including the aromatics 4-phenyl-2-butanone and 2-methyl-1-phenyl-3-pentanone, as well as 2,4-dimethylhexanoic acid. All these represent novel components for the scent gland chemistry of harvestmen.
A detailed look, however, identifies 2,4-dimethyl hexanoic acid as structurally closely related to SCs of subgroup 2, which comprises components such as (E,E)-2,4-dimethylhexa-2,4-dien-1-ol, (E,E)-2,4-dimethylhexa-2,4-dienal, and (E,E)-2,4-dimethylhepta-2,4-dien-1-ol (Figure 1). The SCs of subgroup 2 were found in Leiobunum calcar (Wood, 1868), L. leiopenis (Davis, 1934), and L. nigropalpi (Jones et al., 1977). 2,4-Dimethyl hexanoic acid of European Nelima species may be considered a precursor to the compounds of subgroup 2 or their product, respectively. Formally, 2,4-dimethyl hexanoic acid can be converted into (E,E)-2,4-dimethylhexa-2,4-dienal and (E,E)-2,4-dimethylhexa-2,4-dien-1-ol by specific dehydrogenases (generation of double bonds) and reductases (reduction of the carboxyl group to an aldehyde and further to a hydroxy group). Alternatively, if seen as a product of SCs of subgroup 2, oxidation of (E,E)-2,4-dimethylhexa-2,4-dienal and (E,E)-2,4-dimethylhexa-2,4-dien-1-ol would yield 2,4-dimethyl hexanoic acid, in this case even adding specificity by a stereoselective process (exclusively producing the R,R-isomer of 2,4-dimethyl hexanoic acid). This stereoselective synthesis may point to a communicative function of the compound rather than a function in allomonal defense. Interestingly, both N. sempronii and N. narcisi (which basically represent vicariant species) use the same stereoisomer. We unfortunately do not have data for Nelima doriae (Canestrini, 1872), which may occur syntopically with N. narcisi. Anyway, since convertible into each other, it may be argued that 2,4-dimethyl hexanoic acid from European Nelima species is a SC component of subgroup 2 as well.
We also consider at least two of the aromatic compounds found in European Nelima species as SCs. (i) 2-Methyl-1-phenyl-3-pentanone shows the 4-methyl ethyl-ketone-motif of SC components of subgroup 1 in its side chain, thus implying its assignment to this subgroup. (ii) For 4-phenyl-2-butanone from juvenile N. sempronii and adult N. narcisi, the situation is more complicated. 4-Phenyl-2-butanone does not show an ethyl-ketone, but a methyl-ketone-motif, which is not typical for SCs. This, however, does not necessarily indicate independent biosynthetic origins. More likely, the replacement of 4-phenyl-2-butanone by 2-methyl-1-phenyl-3-pentanone in N. sempronii in the course of maturation strongly implies a basically shared, but extended biosynthetic pathway, and thus a direct connection to SC chemistry. (iii) For the aromatic 2-phenyl-acetamide, we did not find obvious SC motifs. However, data from juveniles of N. narcisi (missing yet) might be crucial for robust conclusions.
Consequently, several – if not all – secretion compounds of European Nelima species may be considered related to the SC chemistry sensu stricto of North American leiobunines. We propose to add at least two of the newly detected components of European Nelima spp., namely 2,4-dimethylhexanoic acid and 2-methyl-1-phenyl-3-pentanone, to the already existing pool of SCs, extending and moderately modifying already known SC chemistry. The definition of SCs, so far exclusively based on open-chain compounds (sensu Raspotnig, 2012), should be extended to include aromatic compounds carrying SC motifs as well.
The replacement of 4-phenyl-2-butanone of juveniles by 2-methyl-1-phenyl-3-pentanone in the adults of N. sempronii represents the first case of juvenile-adult polymorphism for the scent gland secretions of harvestmen. Juvenile-adult polymorphism in exocrine secretions is not rare and occurs in some insect, arachnid, and millipede species (Raspotnig et al., 2005a; Takada et al., 2005; Bodner and Raspotnig, 2012; Kuwahara et al., 2015, 2019). In certain brachypyline oribatid mites of the genus Scheloribates (Berlese, 1908), the secretions of juveniles display the ancient chemotype of early-derivative oribatid groups (“astigmatid-compounds type”: Raspotnig et al., 2001), whereas adults show a novel, highly-derivative chemistry (“alkaloid-type”: Takada et al., 2005). We currently do not know whether juvenile-adult polymorphism in European Nelima spp. might indicate a similar phenomenon. Neither do we know whether this phenomenon might be more frequent in harvestmen. So far, nearly all secretions of harvestmen have been analyzed from adult individuals. For cyphophthalmids, however, it is known that the secretions of juveniles do not differ from those of adults (Raspotnig et al., 2005b), and a comparable situation appears to be true for N. paessleri (at least one juvenile individual was analyzed here).
For N. narcisi, we did not have the opportunity to check the occurrence of juvenile-adult polymorphism since all individuals analyzed were adults. The diversity of profiles found, however, might indicate a similar phenomenon, namely different states of secretion biosynthesis: though speculative for the time being, we assume that the different profiles of N. narcisi adults in Figure 8 correspond to states of secretion regeneration after glandular depletion. If so, 2,4-dimethylhexanoic acid would be produced first. 4-Phenyl-2-butanone would be produced later, and finally gradually replaced by 2-phenylacetamide. The “full” profile of adult N. narcisi might contain only two compounds, 2,4-dimethylhexanoic acid and 2-phenylacetamide, thus being very similar to the profile of N. sempronii. We plan to fully investigate the sequence of secretion regeneration and its ontogeny in N. narcisi soon.
Remarkably, 4-phenyl-2-butanone appears to be both the starting point of juvenile-adult polymorphism in N. sempronii, as well as a possible intermediate in secretion biosynthesis. It is uncertain whether 4-phenyl-2-butanone may also occur in the secretion of N. sempronii adults during secretion regeneration. For the time being, we did not find the compound in the extracts of N. sempronii adults, despite the large number of extracts analyzed.
4-Phenyl-2-butanone is a methyl-ketone that is replaced by an ethyl-ketone in N. semponii adults. This phenomenon implies a link between methyl- and ethyl-ketone biosynthesis in harvestmen, as so far only speculatively addressed by some authors (Raspotnig et al., 2014; Schaider et al., 2018). Formally, α-methylation of a methyl-ketone, hypothetically by an extension of the pathway to methyl-ketones, would lead to ethyl-ketones. Such a link between these two groups of compounds, if eventually proved, would represent a crucial step toward our understanding of a continuous and coherent network of harvestmen chemophylogeny. We know that methyl-ketones constitute a large class of harvestmen secretion products, and these are widely distributed across the secretions of all suborders of Opiliones (e.g., Raspotnig et al., 2005b,2012, 2019; Wouters et al., 2013; Shear et al., 2014; Schaider et al., 2018). Whether and how methyl-ketones might biochemically be linked to other classes of compounds of harvestmen, e.g., to (sclerosomatid) ethyl-ketones, is intriguing and a hot topic of ongoing investigation.
The original contributions presented in this study are included in the article/supplementary material, further inquiries can be directed to the corresponding author.
GR designed the study, conducted the analyses, evaluated the data, and wrote the manuscript. MB evaluated the data, edited, and finalized all figures. IE, MB, MS, and GR performed material collection and determination, as well as the preparation of extracts for GC-MS. AH and JB synthesized relevant reference compounds. OK conducted the NMR measurements. All authors read, edited, and approved the final manuscript.
This work was supported by the Austrian Science Fund (FWF), projects: P29699-B25 and P33840-B.
We are grateful to Tone and Ljuba Slana Novak (both from Maribor, Slovenia) for collecting and providing the live specimens of Nelima narcisi for our investigation.
The authors declare that the research was conducted in the absence of any commercial or financial relationships that could be construed as a potential conflict of interest.
All claims expressed in this article are solely those of the authors and do not necessarily represent those of their affiliated organizations, or those of the publisher, the editors and the reviewers. Any product that may be evaluated in this article, or claim that may be made by its manufacturer, is not guaranteed or endorsed by the publisher.
Blum, M. S., and Edgar, A. L. (1971). 4-Methyl-3-heptanone: Identification and role in opilionid exocrine secretions. Insect Biochem. 1, 181–188. doi: 10.1016/0020-1790(71)90071-0
Bodner, M., and Raspotnig, G. (2012). Millipedes that smell like bugs: (E)-alkenals in the defensive secretion of the julid diplopod Allajulus dicentrus. J. Chem. Ecol. 38, 547–556. doi: 10.1007/s10886-012-0127-5
Brenna, E., Cosi, S. L., Ferrandi, E. E., Gatti, F. G., Monti, D., Parmeggiani, F., et al. (2013). Substrate scope and synthetic applications of the enantioselective reduction of α-alkyl-ß-arylenones mediated by Old Yellow Enzymes. Org. Biomol. Chem. 11, 2988–2996. doi: 10.1039/c3ob40076j
Davis, N. W. (1934). A revision of the genus Leiobunum (Opiliones) of the United States. Am. Midl. Nat. 15, 662–705.
Ekpa, O., Wheeler, J. W., Cokendolpher, J. C., and Duffield, R. M. (1985). Ketones and alcohols in the defensive secretion of Leiobunum townsendi Weed and a review of the known exocrine secretions of Palpatores (Arachnida: Opiliones). Comp. Biochem. Physiol. 81, 555–557. doi: 10.1016/0305-0491(85)90365-7
Garwood, R. J., Sharma, P. P., Dunlop, J. A., and Giribet, G. (2014). A paleozoic stem group to mite harvestmen revealed through integration of phylogenetics and development. Curr. Biol. 24, 1017–1023. doi: 10.1016/j.cub.2014.03.039
Gnaspini, P., and Hara, M. R. (2007). “Defense mechanisms,” in Harvestmen – The Biology of Opiliones, eds R. Pinto-da-Rocha, G. Machado, and G. Giribet (Cambridge: Harvard University Press), 374–399. doi: 10.4159/9780674276833-012
Hara, M. R., Cavalheiro, A. J., Gnaspini, P., and Santos, D. (2005). A comparative analysis of the chemical nature of defensive secretions of Gonyleptidae (Arachnida: Opiliones: Laniatores). Biochem. Syst. Ecol. 33, 1210–1225. doi: 10.1016/j.bse.2005.07.012
Hedin, M., Tsurusaki, N., Macias-Ordonez, R., and Shultz, J. W. (2012). Molecular systematics of sclerosomatid harvestmen (Opiliones, Phalangioidea, Sclerosomatidae): Geography is better than taxonomy in predicting phylogeny. Mol. Phylogenet. Evol. 62, 224–236. doi: 10.1016/j.ympev.2011.09.017
Holmberg, R. G. (1986). “The scent glands of Opiliones: A review of their function,” in Proc 9th Int Congr Arachnol, Panama 1983, eds W. G. Eberhard, Y. D. Lubin, and B. C. Robinson (Washington: Smithsonian Institution Press), 131–133.
Hunt, A. D., Dion, I., Das Neves, N., Taing, S., and Beauchemin, A. M. (2013). Synthesis of azomethine imines using an intramolecular alkyne hydrohydrazination approach. J. Org. Chem. 78, 8847–8852. doi: 10.1021/jo4011409
Jones, H. J., Shear, W. A., and Giribet, G. (2009). The chemical defenses of a stylocellid (Arachnida, Opiliones, Stylocellidae) from Sulawesi with comparisons to other Cyphophthalmi. J. Arachnol. 37, 147–150. doi: 10.1636/SH08-48.1
Jones, T. H., Conner, W. E., Kluge, A. F., Eisner, T., and Meinwald, J. (1976). Defensive substances of opilionids. Experientia 32, 1234–1235. doi: 10.1007/BF01953066
Jones, T. H., Meinwald, J., Hicks, K., and Eisner, T. (1977). Characterization and synthesis of volatile compounds from the defensive secretions of some “daddy longlegs” (Arachnida: Opiliones: Leiobunum spp.). Proc. Natl. Acad. Sci. U S A. 74, 419–422. doi: 10.1073/pnas.74.2.419
Kamptmann, S. B., and Brückner, R. (2013). Stereocontrolled synthesis of a Cn-Cn+7 building block (“Eastern Moiety”) for the unnatural isomers of important polyol, polyene antibiotics based on a ring-closing metathesis and an aldol addition of a lactone enolate. Eur. J. Org. Chem. 2013, 6584–6600. doi: 10.1002/ejoc.201300183
Kawai, Y., Hayashi, M., and Tokitoh, N. (2001). Asymmetric synthesis of α-chiral ketones by the reduction of enones with baker’s yeast. Tetrahedron Asymmetry 12, 3007–3013. doi: 10.1016/S0957-4166(01)00537-7
Kuwahara, Y., Ichiki, Y., Morita, M., Tanabe, T., and Asano, Y. (2015). Chemical polymorphism in defense secretions during ontogenetic development of the millipede Niponia nodulosa. J. Chem. Ecol. 41, 15–21. doi: 10.1007/s10886-014-0536-8
Kuwahara, Y., Ichiki, Y., Morita, M., Tanabe, T., Nakata, R., Mori, N., et al. (2019). Defensive secretion of Oxidus gracilis (C.L. Koch) (Paradoxosomatidae: Polydesmida): juvenile-adult and juvenile-juvenile polymorphism, sexual maturation, and sexual dimorphism. Jpn. J. Environ. Entomol. Zool. 30, 39–49.
Machado, G., Bonato, V., and Oliveira, P. S. (2002). Alarm communication: A new function for the scent gland secretion in harvestmen (Arachnida: Opiliones). Naturwissenschaften 89, 357–360. doi: 10.1007/s00114-002-0337-8
Martens, J. (1969). Mittel- und südeuropäische Arten der Gattung Nelima (Arachnida: Opiliones: Leiobunidae). Senckenbergiana Biol. 50, 395–415.
Martens, J. (1978). Spinnentiere, Arachnida. Weberknechte, Opiliones Die Tierwelt Deutschlands. Jena: Gustav Fischer Verlag.
Meinwald, J., Kluge, A. F., Carrel, J. E., and Eisner, T. (1971). Acyclic ketones in the defensive secretion of a “daddy longlegs” (Leiobunum vittatum). Proc. Natl. Acad. Sci. U.S.A. 68, 1467–1468. doi: 10.1073/pnas.68.7.1467
Myers, A. G., Yang, B. H., Chen, H., McKinstry, L., Kopecky, D. J., and Gleason, J. L. (1997). Pseudoephedrine as a practical chiral auxiliary for the synthesis of highly enantiomerically enriched carboxylic acids, alcohols, aldehydes, and ketones. J. Am. Chem. Soc. 119, 6496–6511. doi: 10.1021/ja970402f
Novak, T., and Slana, L. (2003). Nelima narcisi n. sp., a dwarf member of the genus from the north-eastern Adriatic coast. Fragm. Entomol. 35, 1–11.
Raspotnig, G. (2012). Scent gland chemistry and chemosystematics in harvestmen. Biol. Serb. 34, 5–18.
Raspotnig, G., Anderl, F., and Clouse, R. M. (2019). The scent gland chemistry of neogoveid cyphophthalmids (Opiliones): An unusual methyljuglone from Metasiro savannahensis. Chemoecology 29, 189–197. doi: 10.1007/s00049-019-00288-y
Raspotnig, G., Anderl, F., Kunert, O., Schaider, M., Brückner, A., Schubert, M., et al. (2020). A novel class of defensive compounds in harvestmen: Hydroxy-ß-lactones from the phalangiid Egaenus convexus. J. Nat. Prod. 83, 3278–3286. doi: 10.1021/acs.jnatprod.0c00277
Raspotnig, G., Bodner, M., Blesl, J., and Viquez, C. (2022). The scent gland chemistry of Gagrellinae (Opiliones, Sclerosomatidae): Evidence for sequestration of myrmicacin in a species of Prionostemma. Chemoecology 32, 139–146. doi: 10.1007/s00049-022-00373-9
Raspotnig, G., Bodner, M., Schäffer, S., Koblmüller, S., Schönhofer, A., and Karaman, I. (2015b). Chemosystematics in the Opiliones (Arachnida): A comment on the evolutionary history of alkylphenols and benzoquinones in the scent gland secretions of Laniatores. Cladistics 31, 202–209. doi: 10.1111/cla.12079
Raspotnig, G., Fauler, G., Leis, M., and Leis, H. J. (2005b). Chemical profiles of scent gland secretions in the cyphophthalmid opilionid harvestmen, Siro duricorius and S. exilis. J. Chem. Ecol. 31, 1353–1368. doi: 10.1007/s10886-005-5291-4
Raspotnig, G., Krisper, G., and Schuster, R. (2005a). Ontogenetic changes in the chemistry and morphology of oil glands in Hermannia convexa (Acari: Oribatida). Exp. Appl. Acarol. 35, 47–58. doi: 10.1007/s10493-004-2027-z
Raspotnig, G., Schaider, M., Föttinger, P., Leutgeb, V., and Komposch, C. (2015a). Benzoquinones from scent glands of phalangiid harvestmen (Arachnida, Opiliones, Eupnoi): A lesson from Rilaena triangularis. Chemoecology 25, 63–72. doi: 10.1007/s00049-014-0177-y
Raspotnig, G., Schaider, M., Föttinger, P., and Schönhofer, A. (2017). A model for phylogenetic chemosystematics: Evolutionary history of quinones in the scent gland secretions of harvestmen. Front. Ecol. Evol. 5:139. doi: 10.3389/fevo.2017.00139
Raspotnig, G., Schaider, M., Stabentheiner, E., Leis, H. J., and Karaman, I. (2014). On the enigmatic scent glands of dyspnoan harvestmen (Arachnida, Opiliones): First evidence for the production of volatile secretions. Chemoecology 24, 43–55. doi: 10.1007/s00049-014-0146-5
Raspotnig, G., Schuster, R., Krisper, G., Fauler, G., and Leis, H. J. (2001). Chemistry of the oil gland secretion of Collohmannia gigantea (Acari: Oribatida). Exp. Appl. Acarol. 25, 933–946. doi: 10.1023/A:1020634215709
Raspotnig, G., Schwab, J., and Karaman, I. (2012). High conservatism in the composition of scent gland secretions in cyphophthalmid harvestmen: Evidence from Pettalidae. J. Chem. Ecol. 38, 437–440. doi: 10.1007/s10886-012-0108-8
Rocha, D. F. O., Hamilton, K., Goncalves, C. C. S., Machado, G., and Marsaioli, A. J. (2011). 6-Alkyl-3,4-dihydro-2H-pyrans: Chemical secretion compounds in tropical harvestmen. J. Nat. Prod. 74, 658–663. doi: 10.1021/np100719f
Rocha, D. F. O., Wouters, F. C., Zampieri, D. S., Brocksom, T. J., Machado, G., and Marsaioli, A. J. (2013). Harvestmen phenols and benzoquinones: Characterization and biosynthetic pathway. Molecules 18, 11429–11451. doi: 10.3390/molecules180911429
Roewer, C. F. (1910). Revision der Opiliones Plagiostethi (= Opiliones Palpatores). I. Teil: Familie der Phalangiidae (Subfamilien: Gagrellini, Liubunini, Leptobunini). Abh. Geb. Naturw. Hamburg 19, 1–294.
Schaider, M., Novak, T., Komposch, C., Leis, H. J., and Raspotnig, G. (2018). Methyl-ketones in the scent glands of Opiliones: A chemical trait of Cyphophthalmi retrieved in the dyspnoan Nemastoma triste. Chemoecology 28, 61–67. doi: 10.1007/s00049-018-0257-5
Schaider, M., and Raspotnig, G. (2009). Unusual organization of scent glands in Trogulus tricarinatus (Opiliones, Trogulidae): Evidence for a non-defensive role. J. Arachnol. 37, 78–83. doi: 10.1636/SH08-01.1
Shear, W. A., Tones, T. H., Guidry, H. M., Derkarabetian, S., Richart, C. H., and Minor, M. (2014). Chemical defenses in the opilionid infraorder Insidiatores: Divergence in chemical defenses between Triaenonychidae and Travunioidea and within travunioid harvestmen (Opiliones) from eastern and western North America. J. Arachnol. 42, 248–256. doi: 10.1636/B14-41.1
Szalay, L. (1951). Drei neue Weberknechte (Opiliones) aus Ungarn. Annls hist-nat Mus natn hung 1, 126–132.
Takada, W., Sakata, T., Shimano, S., Enami, Y., Mori, N., Nishida, R., et al. (2005). Scheloribatid mites as the source of pumiliotoxins in dendrobatid frogs. J. Chem. Ecol. 31, 2403–2415. doi: 10.1007/s10886-005-7109-9
Van den Dool, H., and Kratz, P. D. (1963). A generalization of the retention index system including linear temperature programmed gas-liquid partition chromatography. J. Chromatogr. A 11, 463–471. doi: 10.1016/S0021-9673(01)80947-X
Wouters, F. C., Rocha, D. F. O., Goncalves, C. C. S., Machado, G., and Marsaioli, A. J. (2013). Additional vinyl ketones and their pyranyl ketones in gonyleptid harvestmen (Arachnida: Opiliones) suggest these metabolites are widespread in this family. J. Nat. Prod. 76, 1559–1564. doi: 10.1021/np4001569
Keywords: Opiliones, Sclerosomatidae, Leiobuninae, Leiobunum, ethyl-ketones, chemosystematics
Citation: Raspotnig G, Hüfner A, Bodner M, Blesl J, Schaider M, Escalante I and Kunert O (2022) Polymorphic scent gland secretions in Nelima harvestmen: “Sclerosomatid compounds” but different chemical lineages. Front. Ecol. Evol. 10:993368. doi: 10.3389/fevo.2022.993368
Received: 13 July 2022; Accepted: 20 September 2022;
Published: 12 October 2022.
Edited by:
Srećko Ćurčić, University of Belgrade, SerbiaReviewed by:
Miriam Sannomiya, Universidade Federal de São Paulo, BrazilCopyright © 2022 Raspotnig, Hüfner, Bodner, Blesl, Schaider, Escalante and Kunert. This is an open-access article distributed under the terms of the Creative Commons Attribution License (CC BY). The use, distribution or reproduction in other forums is permitted, provided the original author(s) and the copyright owner(s) are credited and that the original publication in this journal is cited, in accordance with accepted academic practice. No use, distribution or reproduction is permitted which does not comply with these terms.
*Correspondence: Günther Raspotnig, Z3VlbnRoZXIucmFzcG90bmlnQHVuaS1ncmF6LmF0
Disclaimer: All claims expressed in this article are solely those of the authors and do not necessarily represent those of their affiliated organizations, or those of the publisher, the editors and the reviewers. Any product that may be evaluated in this article or claim that may be made by its manufacturer is not guaranteed or endorsed by the publisher.
Research integrity at Frontiers
Learn more about the work of our research integrity team to safeguard the quality of each article we publish.