- 1School of Life Sciences Research Complex, University of Dundee, Dundee, United Kingdom
- 2Institut de Biologia Evolutiva (CSIC-Universitat Pompeu Fabra), Barcelona, Spain
The Naked mole-rat (NMR) is becoming a prominent model organism due to its peculiar traits, such as eusociality, extreme longevity, cancer resistance, and reduced pain sensitivity. It belongs to the African mole-rats (AMR), a family of subterranean rodents that includes solitary, cooperative breeding and eusocial species. We identified and quantified the domestication syndrome (DS) across AMR, a set of morphological and behavioural traits significantly more common and pronounced amongst domesticated animals than in their wild counterparts. Surprisingly, the NMR shows apparent DS traits when compared to the solitary AMR. Animals can self-domesticate when a reduction of the fear response is naturally selected, such as in islands with no predators, or to improve the group’s harmony in cooperative breeding species. The DS may be caused by alterations in the physiology of the neural crest cells (NCC), a transient population of cells that generate a full range of tissues during development. The NCC contribute to organs responsible for transmitting the fear response and various other tissues, including craniofacial bones. Therefore, mutations affecting the NCC can manifest as behavioural and morphological alterations in many structures across the body, as seen in neurocristopathies. We observed that all social AMRs are chisel-tooth diggers, an adaption to hard soils that requires the flattening of the skull. We hypothesise that chisel-tooth digging could impose a selective pressure on the NCC that triggered the DS’s appearance, possibly facilitating the evolution of sociality. Finally, we discuss how DS traits are neutral or beneficial for the subterranean niche, strategies to test this hypothesis and report well-studied mutations in the NMR that are associated with the NCC physiology or with the control of the fear response. In conclusion, we argue that many of the NMR’s unconventional traits are compatible with the DS and provide a hypothesis about its origins. Our model proposes a novel avenue to enhance the understanding of the extraordinary biology of the NMR.
Introduction
The reduction of the fight-or-flight response is a hallmark of domestication (Belyaev, 1969). From the Neolithic period, many animals adapted to the anthropogenic environment by reducing reactivity to humans and perhaps increasing their social tolerance (Tchernov and Horwitz, 1991). Surprisingly, there is a set of physical traits that often appear in domestic mammals but not in their wild equivalents, known as the domestication syndrome (DS). The DS includes depigmentation, a shorter snout, decreased brain size, floppy ears, reduced or missing teeth and hairlessness, amongst other traits (Darwin, 1868; Sánchez-Villagra et al., 2016). These traits rarely occur in the wild, and usually, only a subset of them is present in each individual or variety of a domestic species. DS most probably was not directly selected by humans (Darwin, 1868); but instead, it appeared as a side effect of selecting for tameness (Belyaev, 1969). Experiments with wild foxes, rats and minks show that DS traits can appear as a side effect of artificially selecting for tameness (Trut, 1999; Trut et al., 2009; Singh et al., 2017; Wang et al., 2018). However, they can also appear in wild animals when selective pressures favour a reduced fight-or-flight response (self-domestication), as it occurs in islands with no predators (Ramis and Bover, 2001; Cooper et al., 2014; Sánchez-Villagra et al., 2016). Finally, these traits can be observed in primates, including humans (Theofanopoulou et al., 2017; Sánchez-Villagra and van Schaik, 2019; Zanella et al., 2019), bonobos (Hare et al., 2012), and marmoset monkeys (Ghazanfar et al., 2020), where it has been suggested that the reduced aggression associated with the DS could have been selected to enhance cooperative breeding.
There have been many attempts to explain why selecting for tameness result in the distinct morphological traits encompassed by the DS, including changes in thyroid function (Crockford, 2004; Karlsson et al., 2016; Fitak et al., 2020), in the metabolism of the adrenaline (Keeler et al., 1970; Nätt et al., 2007; Cagan and Blass, 2016), or the retention of juvenile traits in the adult animal (Shea, 1989; Geiger et al., 2017). Recently, a new, more comprehensive theory proposed that the DS syndrome is derived from mutations affecting the development of the hypothalamic–pituitary–adrenal (HPA) axis and the sympathetic nervous system (Wilkins et al., 2014). The HPA axis converts the perception of dangerous stimuli into a hormonal response by transmitting information to the adrenal medulla, which results in the release of glucocorticoids and adrenaline, leading to a long-term elevated reactivity (Sapolsky, 2005). Similarly, the sympathetic nervous system mediates the short-term fight-or-flight response and directly stimulates the adrenal glands. Mutations producing a hypofunctional HPA axis could result in the reduced fear response characteristic of domestic animals. Remarkably, the sympathetic nervous system, the adrenal glands, skin melanocytes, bone and cartilage in the skull and teeth, amongst other tissues, are derived from the neural crest cells (NCC). This way, mild neurocristopathies (disorders derived from defects in the NCC) could explain tameness and the DS simultaneously (Wilkins et al., 2014).
The family of African mole-rats (AMR, family Bathyergidae) is a well-recognised model to study the evolution of cooperative breeding (Faulkes and Bennett, 2016). This family of subterranean rodents contains solitary, social cooperative breeders and two eusocial species: The naked mole-rat (NMR) and the Damaraland mole-rat. Eusociality is an extreme form of cooperative breeding where species show sexual suppression, reproductive division of labour, overlapping generations and cooperative care of the young (Burda et al., 2000). In the case of eusocial AMRs, most members renounce to reproduce and specialise in digging, defence, or pup-caring, whilst a single queen continuously produces offspring (Faulkes and Bennett, 2001). The NMR presents the most extreme and unusual traits of its family: it is eusocial, forms extensive colonies (Jarvis, 1981), and exhibits resistance to hypoxia, delayed senescence, cancer resistance, poor control of body temperature and reduced sensitivity to pain (reviewed in Braude et al., 2021). Its scientific name Heterocephalus glaber (“atypical bald head”), also refers to its rare morphological traits: it is hairless (except for sensory hairs), has wrinkled skin, and a flat skull with a reduced number of molars when compared with the other AMR (Jarvis and Sherman, 2002). Strikingly, many of these morphological traits can be accounted as part of the DS. Is the NMR a domestic animal?
Here we propose that the social behaviour of AMR is the product of self-domestication. We document that DS traits are more prominent in social AMR species, especially in the NMR. We focus on the NMR because it presents the most extreme morphological and behavioural phenotype, and it is the best-studied member of the family. We propose that many of the unusual NMR traits could be the result of a mild-neurocristopathy that recapitulated the DS. We discuss the possible interactions between the DS, the subterranean niche, and the digging mode. Finally, we suggest that the appearance of the DS facilitated the evolution of eusociality in AMRs and argue that this integrative theory will aid NMR research on many fronts.
Domestication syndrome in the naked mole-rat
The AMR family comprises six genera and more than 15 species. All family members are subterranean rodents and present a complete set of morphological, physiological, and behavioural adaptations to the underground environment. However, genera differ in some essential traits regarding their head, digging behaviours, and sociality. Surprisingly, many differences between the social AMR, especially the NMR, and the solitary species can be accounted for as part of the DS. We compiled a list of the DS traits across the AMR family.
Skull
The skulls of domestic animals present many characteristic traits. Many domestic animals (e.g., dog, fox, pig, sheep, goat, cat, mouse, cow, and human) present a reduced snout in comparison to their wild counterparts (reviewed in Sánchez-Villagra et al., 2016; Sánchez-Villagra and van Schaik, 2019). Amongst subterranean rodents, the relative length of the snout is largely determined by their digging behaviours: forelimb diggers use their claws to scratch soft, sandy soil and possess more prominent faces, whilst chisel-tooth diggers use their incisors to exploit harder soil and have a relatively wider cranium (Lacey and Patton, 2000). Chisel-tooth diggers show enlarged zygomatic arcs (cheek bones) and shorter rostra, associated with larger masseter and temporalis muscles, presumably to enhance the bite force (Lacey and Patton, 2000; Mcintosh and Cox, 2016).
We gathered the width and length of the skull of the AMR, other African Hystricognath (the closest relatives to the AMR: Old World porcupines, the dassie rat and the cane rat) and examples of genetically unrelated convergent mole-rats (from genus Spalax, Spalacopus, and Tachyoryctes). We calculated the ratio between the length and the width of the skull for the chisel-tooth diggers in our data set (Figure 1, see Materials and methods in the Supplementary Material). In comparison, exclusive forelimb diggers (other African Hystricognath and Bathyergus mole-rats) tend to have a more prominent snout (Supplementary Figure 1). At the same time, Tachyoryctes members, which dig with both their limbs and teeth, present closer cranial proportions to exclusive chisel-tooth diggers (most AMR, Spalax and Spalacopus). We show that the NMR has a disproportionally shorter snout even for a chisel-tooth digger, probably due to a statistically significant reduction of the rostral length (Mcintosh and Cox, 2016). The relative shortening of the NMR’s snout compared to other chisel-tooth diggers is a typical DS trait.
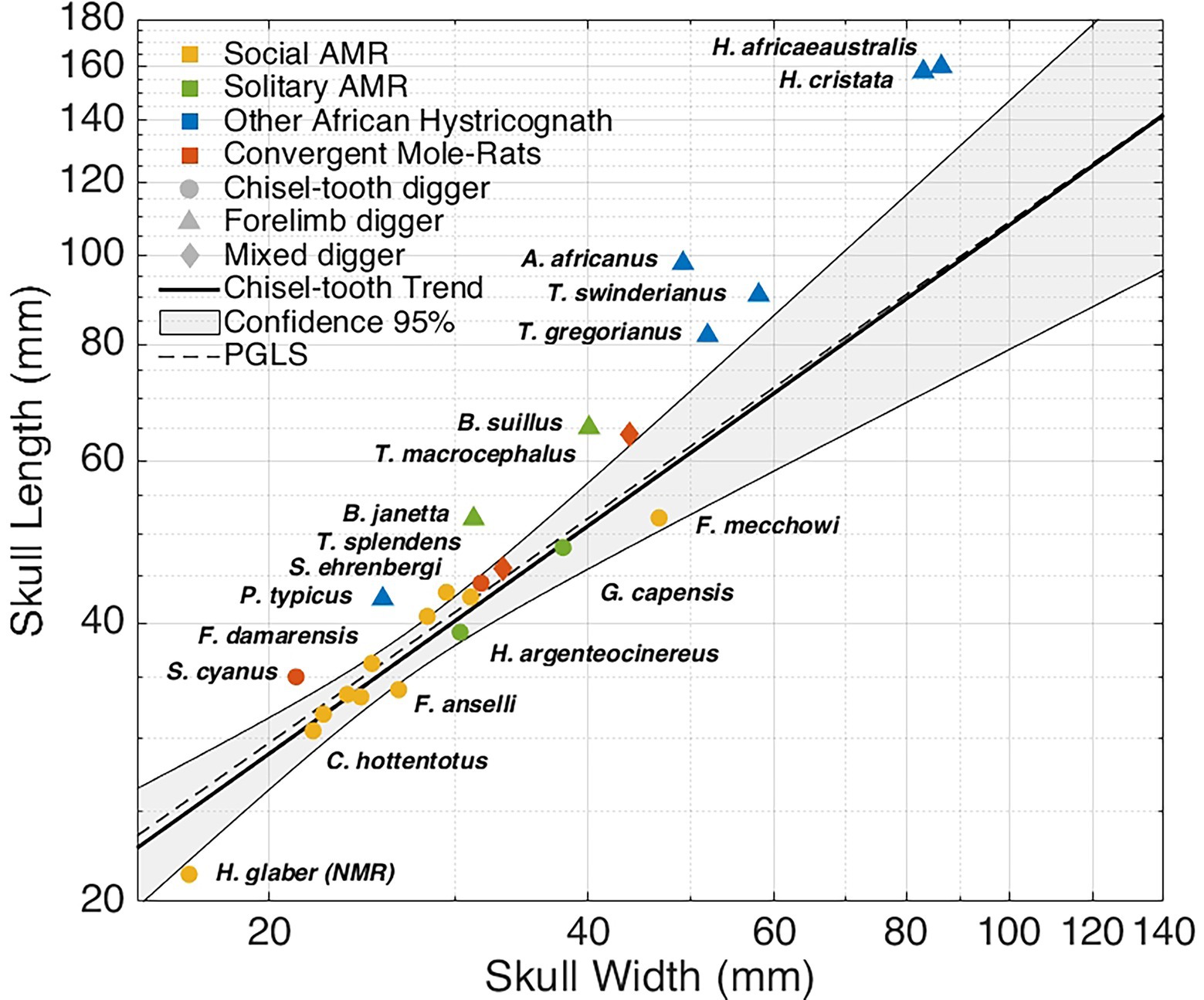
Figure 1. Relation between the length and width of the skull in African mole rats, their closes relatives, and convergent subterranean rodents. Chisel-tooth digging subterranean rodents present a smaller skull length/width ratio than forelimb diggers. The naked mole-rat (Heterocephalus glaber, NMR) has a significantly shorter snout even when compared to other chisel-tooth digging African mole-rats. Each data point is coloured according to its social or phylogenetic attributes as indicated in the figure. The shape represents digging mode. The linear regression line for chisel-tooth diggers with 95% confidence intervals is shown, together with the regression line based on phylogenetic generalized least squares (PGLS). All data was retrieved from Kingdon (2014). See Materials and methods in the SM for more details. PGLS was calculated using the evolutionary tree in Supplementary Figure 2.
All AMR species share a similar diet of geophytes, tubers and rhyzomes (Kingdon, 2014). Unfortunately, there is no data available about the relative hardness of the plants (Dominy et al., 2008) consumed by AMR in comparison with the hardness of the soil. However, it seems unlikely that any tuber or rhyzome offer more resistance than the soil. In addition, whilst all AMR species eat similar plants (Kingdon, 2014), they inhabit areas with different soil properties (Faulkes and Bennett, 2013). Solitary forelimb diggers (Bathyergus) are restricted to more humid environments where digging is easier, whilst chisel-tooth diggers-especially the social species-tend to inhabit regions with harder soils (Faulkes and Bennett, 2013). Therefore, it seems that the type of soil correlates better with the head morphology, digging behaviour and sociality (see The subterranean niche and self-domestication below), than the type of diet, which suggests that the properties of the soil are the main determinant of the skull proportions amongst AMR.
Dentition
The skull of domestic animals often manifests dental abnormalities such as crowding of the cheek teeth or a reduction of teeth size or number (dogs, pigs, mouse, human; reviewed in Kleisner and Stella, 2009; Sánchez-Villagra et al., 2016; Sánchez-Villagra and van Schaik, 2019; Lord et al., 2020). Most AMR species share the same dental formula (I 1/1, C 0/0, P 1/1, M 3/3; 20 teeth; Table 1), except for Heliphobius (Gomes Rodrigues and Šumbera, 2015), that shed their premolar teeth and substitutes them with new molars giving a higher, variable number from 20 to 28 teeth; and the NMR (Gomes Rodrigues and Šumbera, 2015) which only presents 3 molars (occasionally only 2) in each ramus for a total of 14–16 teeth (Kingdon, 2014). Therefore, the NMR also presents a reduced, variable number of teeth, when compared with other AMRs.
Ear
Another common trait of the DS is reduced or malformed ears (e.g., dogs, horses, pigs, rabbits, cattle, guinea pig; reviewed in Sánchez-Villagra et al., 2016; Lord et al., 2020). NCC contribute to the formation of all the components of the ear, and hearing defects are common in Human neurocristopathies (Ritter and Martin, 2019). Ears of AMR lack pinna whilst their closest relatives, the other African Hystricognath, all have well developed external auricles (Schlitter et al., 2014). The quantitative comparison of the NMR ear components with other AMRs (B. suillus, C. hottentotus, F. micklemi, G. capensis, and H. argenteocinereus) suggest that the NMR has a degenerated ear (Mason et al., 2016). They have a very narrow, semi occluded external canal, a smaller than expected and fused malleoincus, a poorly ossified part of the malleus, weakly developed long process of the incus, lack of structural stiffness of the stapes, reduced number of cochlear turns, and a relatively small auditory bulla (Mason et al., 2016). Furthermore, the ear morphology in the NMR shows a considerable intraspecific variation which could suggest that the ear is subjected to a relaxed selective pressure (Mason et al., 2016). Finally, some studies indicate that the NMR has relatively poor hearing, whilst other studies suggest that the auditory system is just adapted to the subterranean niche (reviewed in Braude et al., 2021).
Brain
Domestic animals (e.g., dog, cat, pig, alpaca, rabbit, mouse, horse) usually have smaller brains than their wild counterparts (reviewed in Sánchez-Villagra et al., 2016). Specifically, they tend to show an exacerbated reduction of the forebrain (mink, horse, pig, fox; Trut et al., 1991; Kruska, 2005; Wilkins et al., 2014). The brain size of the AMR has been quantitatively analysed under the hypothesis that social animals evolve larger brains in Kverková et al. (2018). However, on the contrary, they found that social AMR species have relatively smaller brains than solitary ones, with a statistically significant reduction in forebrain neurons. In particular, the NMR has a significantly smaller brain mass and fewer neurons in comparison with the other AMR (Supplementary Figure 1 in Kverková et al., 2018). This apparent contradiction could be explained by the self-domestication hypothesis, which instead predicts the smaller brain size of NMR.
Body and heart
A body size reduction occurred in early domestic breeds (pig, dog, cow, goat, and sheep) as a natural adaptation to the human environment (Tchernov and Horwitz, 1991). It could also be associated with self-domestication in islands (reviewed in Sánchez-Villagra et al., 2016), probably because of small or absent predation and low interspecies competition. The body size of AMR varies exponentially amongst species. Social AMR species tend to be smaller than the solitary ones (Table 1), with the NMR being the smallest (∼30 g) and B. suillus the heaviest (∼1 kg). In addition to its arguable dwarfism, the body of the NMR differs because it possesses a long tail (50% head-body length), whilst the other AMR present very short tails [8%−24% head-body length, depending on the genus (Table 1; Schlitter et al., 2014)]. Similarly, changes in the number of caudal vertebrae occurred in a few domesticated species (dog, cat, sheep; Sánchez-Villagra et al., 2016). Moreover, many domestic animals show a shortening of the limbs (Sánchez-Villagra et al., 2016) and, likewise, the legs of the AMRs are generally described as “short and slender” (Schlitter et al., 2014). Recent analysis show that the forelimbs of NMR are less specialised and paedomorphic than the rest of AMR (Montoya-Sanhueza et al., 2022). Finally, a reduced heart size is one of the most common traits amongst domesticated species (e.g., mouse, rat, guinea pig, pig, dog; Sánchez-Villagra et al., 2016). Currently there are not quantitative comparisons of AMR heart sizes; however, allometric analysis suggests that the NMR has a disproportionally small heart in comparison with another rodent, the mouse (Grimes et al., 2013).
Skin and fur
Probably, complete or partial depigmentation is, along with increased tameness, the most frequent trait in the DS, appearing in at least some varieties of each known domestic species (Sánchez-Villagra et al., 2016). In particular, a white forelock is a common characteristic of domestic animals and a noticeable feature of the Waardenburg syndrome, a human neurocristopathy (Wilkins et al., 2014). Furthermore, the white forelock has been recently associated with the self-domestication phenotype in marmoset monkeys (Ghazanfar et al., 2020). Interestingly, most AMR species occasionally present a white forehead patch of variable size (Figure 2). A recent report found no correlation between the size of the white forehead patch and the behaviour amongst F. anselli individuals (Begall et al., 2022). However, to our knowledge, the frequency and size of the white forehead patch have not been quantified amongst different species. The currently available interspecies data, albeit incomplete and not completely quantitative (Kingdon, 2014), suggest that the white forehead patch tends to be more prominent and frequent amongst social species and reduced and only occasionally present in the solitary mole-rats (Table 1). The NMR appearance departs from the other AMRs because of its complete depigmentation, hairlessness, and skin folds. However, all these attributes are also characteristic of some domestic variates (dog, cow, pig, goat, rabbit; Sánchez-Villagra et al., 2016) and can be understood as a more extreme domestication phenotype.
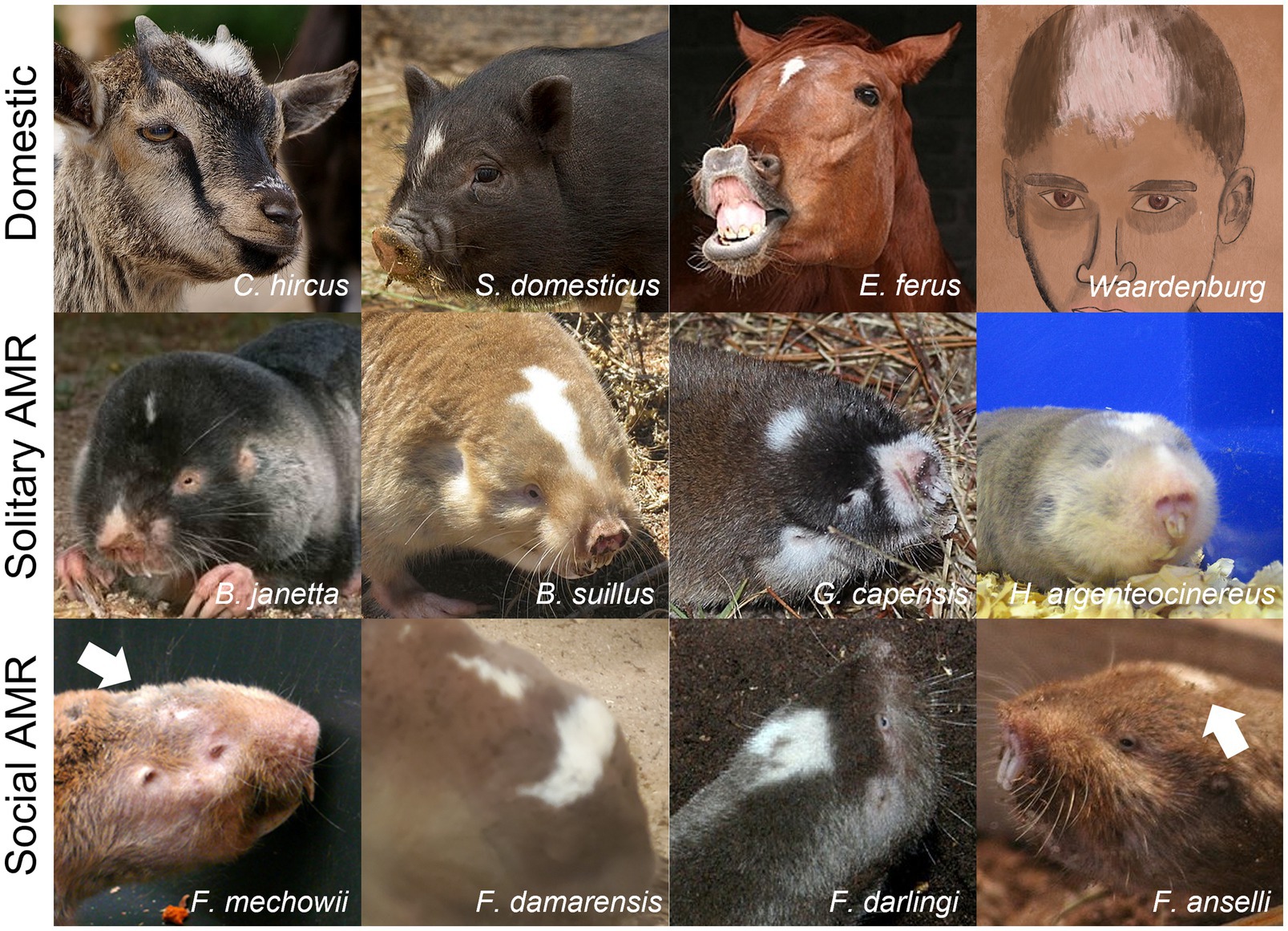
Figure 2. White forehead patch in domestic species and African mole rats. Domestic species and some neurocristopathies are associated with large white patches on the forehead. Most African mole-rats present a white forehead patch which size and frequency could be associated with their social behaviour (Table 1). All images are available under Creative Common License. Authorship credit of the images in Supplementary Table 1.
Reproductive biology
A combination of body size reduction, earlier sexual maturation, increased litter size, non-seasonal oestrus cycles, and a decrease in sexual dimorphism generally allow domestic animals to have higher reproductive rates than their wild counterparts (Tchernov and Horwitz, 1991; Sánchez-Villagra et al., 2016). Non-seasonal reproduction, higher annual reproductive output, and absent or reduced sexual dimorphism seem to be more frequent amongst social AMR (Cryptomys, Fukomys, Heterocephalus), whilst the solitary, forelimb diggers (Bathyergus) present marked sexual dimorphism (Kingdon, 2014).
The lack of behavioural and morphological sexual dimorphism is exceptionally noticeable in the non-reproductive members of a NMR colony (MacManes and Lacey, 2012): The males lack scrotum, the females have an imperforated vagina; and, unlike any other mammal, the phallus (clitoris or penis), the anogenital distance, and the perineal muscles are sexually monomorphic (Jarvis, 1981; Peroulakis et al., 2002; Seney et al., 2009). They also lack sexual dimorphism in femoral bone structure and quality (Pinto et al., 2010) and forebrain regions (Holmes et al., 2008), which has been associated with the retention of a pre-pubertal phenotype in the worker castes (Holmes et al., 2007; Swift-Gallant et al., 2015; Peragine et al., 2017).
Whilst domestic animals seem to present an earlier sexual maturity, most of the individuals in a NMR colony never reproduce, and they remain all their life sexually suppressed in a pre-pubertal state until the breeding members need to be replaced (Faulkes et al., 1990, 1991). This phenomenon can be seen as an extreme version of neoteny. Indeed, neoteny has been classically used to explain many of the characteristic reproductive and behavioural changes that occur during domestication (Sánchez-Villagra et al., 2016). Many of the characteristic traits of the NMR can be considered neotenic (Skulachev et al., 2017; Buffenstein et al., 2020) such as the lack of hair, absence of scrotum or the decline of bone mineralisation with age (Buffenstein, 2008), which could be simultaneously related to the extraordinary social organisation and longevity of this species.
Hormones and social behaviour
Glucocorticoids mediate the long-term response to environmental and social stressful situations. A reduction of the concentration of corticosteroid hormones characterises both domestic animals (Belyaev, 1969; Trut, 1999; Wilkins et al., 2014; Kikusui et al., 2019) and subordinate individuals in cooperative breeding species (Creel, 2001; Sapolsky, 2005). In domesticated animals, the reduction of glucocorticoids could lower aggressive behaviours and increase social tolerance, which could also be beneficial to maintain harmony within the group in cooperative breeding species. In this line, both eusocial species F. damarensis (Ganem and Bennett, 2004) and the NMR show little aggression amongst individuals of the same colony (O’riain and Jarvis, 1997). However, whilst F. damarensis manifest lower aggression against unfamiliar conspecifics, the NMR shows xenophobic behaviour (O’riain and Jarvis, 1997; Barker et al., 2021; Toor et al., 2022).
In NMR colonies, the queen displays most aggressive behaviours (Clarke and Faulkes, 2001); however, cortisol levels, the main glucocorticoid in the non-murine rodents (Buffenstein and Pinto, 2009), do not correlate with social or reproductive status either in the NMR (Clarke and Faulkes, 2001; Edwards et al., 2020) or F. damarensis (Medger et al., 2018) which suggest that social AMR do not suffer “stress of dominance” or “stress of subordination” (Edwards et al., 2020).
Nonetheless, cortisol levels rise after the death of the queen producing a violent period when individuals fight for the succession (Clarke and Faulkes, 1997; Medger et al., 2019), or in an individual isolated from the rest of the colony (Faykoo-Martinez et al., 2018; Edwards et al., 2020), indicating that they suffer from social stress as observed in marmoset monkeys (Smith and French, 1997) and tamarins (Ziegler et al., 1995), two cooperative breeding primates. Finally, in AMR species, there are no significant differences in the basal blood concentration of cortisol amongst non-pregnant females in one solitary species (G. capensis) and three social species (F. darlingi, F. hottentotus pretoriae, F. damarensis). However, only the solitary species showed an elevation of cortisol levels after repeated encounters with unfamiliar conspecific of the same sex (Ganem and Bennett, 2004). Altogether, these observations suggests that cooperative breeding species suppressed social stress to acquire social tolerance towards members of the same species.
Oxytocin is also a mediator of the HPA axis that has been associated with increased social cohesion and maternal behaviour in domesticated animals (Kikusui et al., 2019). In the same line, the NMR expresses more oxytocin receptor levels in the nucleus accumbens, a forebrain centre controlling monogamic, maternal and allomaternal behaviour in comparison with the solitary G. capensis (Kalamatianos et al., 2010). Moreover, non-breeding individuals of a NMR colony present more oxytocin-producing neurons than breeding individuals, suggesting that oxytocin contributes to maintaining the prosocial behaviour of the worker caste (Mooney and Holmes, 2013).
Other traits linked to an altered NCC functionality
The NMR shows a series of peculiar biological traits that are not generally included in the DS, but that could be explained through defects in the physiology of the NCC.
Peripheral nervous system
Most of the structures of the peripheral nervous system (cranial and spinal nerves and ganglia; and enteric nervous system) are derived from the NCC (Prendergast and Raible, 2014). The NMR also manifest alterations in the cutaneous innervation. It presents a significantly reduced ratio of unmyelinated nociceptive C-fibres and A-fibres in the saphenous and sural nerves (1.5:1), whilst in other AMRs (H. argenteocinereus, F. mechowii, F. damarensis, F. darlingi, C. hottentotus) and most mammals C-fibres generally are 3–4 times more abundant (Smith et al., 2012). Innervation defects are typical of certain neurocristopathies (Indo, 2009) and could be manifestation of mutations affecting the biology of NCC (see Trka below).
Thymus
NCC participate in the development of the stroma of the thymus, an organ necessary for the maturation of the T-cells (Zachariah and Cyster, 2010); and consequently, the T-cell population is affected in several neurocristopathies (Chinen et al., 2003; Vega-Lopez et al., 2018). The NMR has a reduced thymus/body weight ratio and presents a poor delineation between cortex and medulla in comparison to mice (Lin and Buffenstein, 2021). Moreover, the immune system of the NMR differs from mice and humans in having predominantly myeloid cells and a notable deficit in cytotoxic T-cells (Shebzukhov et al., 2019), which could be related to a defective thymus development due to alterations in the NCC.
Thyroid
The NCC also contribute to creating the stroma of the thyroids (Maeda et al., 2016) and altered thyroid function is a common trait amongst neurocristopathies (Vega-Lopez et al., 2018), and it has been previously associated with domestication (Crockford, 2004). Interestingly, the NMR exhibits unique low levels of thyroid hormone (Buffenstein et al., 2001; Buffenstein and Pinto, 2009).
Gall bladder
Gall bladder derives from the NCC (Mawe et al., 1997). Although most vertebrates possess this gland, it is absent in the NMR (Hill et al., 1957).
Gene candidates
Here we discuss some well-studied mutations in the NMR that are associated with the NCC physiology or with the control of the fear response.
Trka
Trka (tropomyosin receptor kinase A) binds to NGF (nerve growth factor), promoting survival and differentiation of the sensory neurons during embryonic development, and modulating the sensitivity to pain later in life (Lewin et al., 2014). Trka is implicated in cell migration in normal and pathological conditions (Wislet et al., 2018); and it is necessary for the formation of the dorsal root and sympathetic ganglia by the trunk NCC in mouse (Smeyne et al., 1994).
In humans, mutations in the Trka gene produce congenital insensitivity to pain (CIP) with anhidrosis (CIPA), a neurocristopathy that results in a total loss of the C-fibres, lack of nociception, inability to sweat (hypotrophic sweat glands), lack of hair (bald patches in the scalp), poor thermoregulation, and oral and craniofacial manifestation including missing teeth and nasal malformations (Indo, 2009; Gao et al., 2013). Furthermore, CIPA patients seem to be unable to learn new fears and probably fail to exhibit a fight-or-flight response (Indo, 2009).
Knock out mice for Trka present a similar phenotype: Abnormal peripheral small nerves fibres, deficient nociception, small body size, early death, and severe cell loss in trigeminal, dorsal root, and sympathetic ganglia. However, they do not manifest anhidrosis, and over time they develop a mottled fur with scabs (Smeyne et al., 1994; Indo, 2009). Trka null mice also show an acute decrease of the basal forebrain cholinergic neurons (BFCNs). Notably, BFCNs have been implicated in the control of conditioned fear behaviours (Jiang et al., 2016), and mice lacking Trka signalling in the forebrain show defective fear conditioning (Sanchez-Ortiz et al., 2012). Furthermore, Trka null mice show a defective thymus formation with no clear delimitations between the cortex and the stroma and a reduced number of thymocites (Garcıa-Suárez et al., 2000).
The NCC hypothesis states that domesticated animals suffer a mild neurocristopathy produced by mutations that only partially reduce the activity of genes implicated in the NCC biology (Wilkins et al., 2014). The NMR possess between one and three amino acid substitutions in the kinase domain of Trka that turn the receptor hypofunctional which are absent or rare in other animals or amongst other AMR (Omerbašić et al., 2016). Moreover, the expression level of Trka in the NMR is significantly lower than that of mouse (Lewin, 2020). It is tempting to see many of the traits of the NMR as partly produced by a mild version of CIPA, caused by the reduced expression of a hypofunctional version of Trka. In this line, PRDM12 (PRDI-BF1 and RIZ homology domain containing protein 12), a transcription factor downstream of Trka also associated with CIP in humans (Desiderio et al., 2019), shows lower expression and unique amino acid variants in the NMR (Lewin, 2020). Altogether, mutations in the Trka pathway could explain the reduction in the C-fibres and the reduction in pain sensitivity in the NMR (Omerbašić et al., 2016); but also, the lack of fur and sweat glands, the poor thermoregulation, the reduced and defective thymus, missing teeth, shorter muzzle and the smaller forebrain. Indeed, Trka could provide a genetic connection between the neural crest and the reduction of the forebrain suggested by the neural crest domestication hypothesis (Wilkins et al., 2014). Finally, Trka mutations also seem to cause a reduction in the fear response in mice and humans and could potentially explain the high social tolerance of the NMR.
HIF1α
The NMR presents critical molecular adaptations to survive in hypoxic/hypercapnic environments produced by large numbers of individuals living in borrows (Park et al., 2021). For example, the NMR carries a hyperactive version of HIF1α (Hypoxia Inducible factor 1 α), a transcription factor that regulates the expression of dozens of genes in response to low-oxygen conditions. In normal-oxygen conditions, HIF1α is targeted for degradation by VHL (Von Hippel–Lindau tumour suppressor); however, low-oxygen levels prevent the HIF1α-VHL interaction. The NMR possesses a unique mutation in HIF1α (T395I, Figure 3) that prevents its interaction with VHL, as well as a mutation in VHL (V166I) which probably further reduces the ubiquitination of HIF1α (Kim et al., 2011). Moreover, the NMR expresses higher levels of HIF1α than mice in several tissues (Xiao et al., 2017). Lastly, HIF1α probably promotes a glycolytic metabolism (Varum et al., 2011; Prigione et al., 2014), a less efficient pathway to obtain ATP than oxidative phosphorylation that works in the absence of oxygen. Glycolysis produces lactic acid as a by-product, which blocks glycolysis by inhibiting PFK1 (phosphofructokinase 1). The NMR presents a unique adaptation to bypass the glycolysis block during hypoxia by using fructose (instead of glucose) as a substrate, enabling the glycolysis even in high lactic-acid conditions (Park et al., 2017).
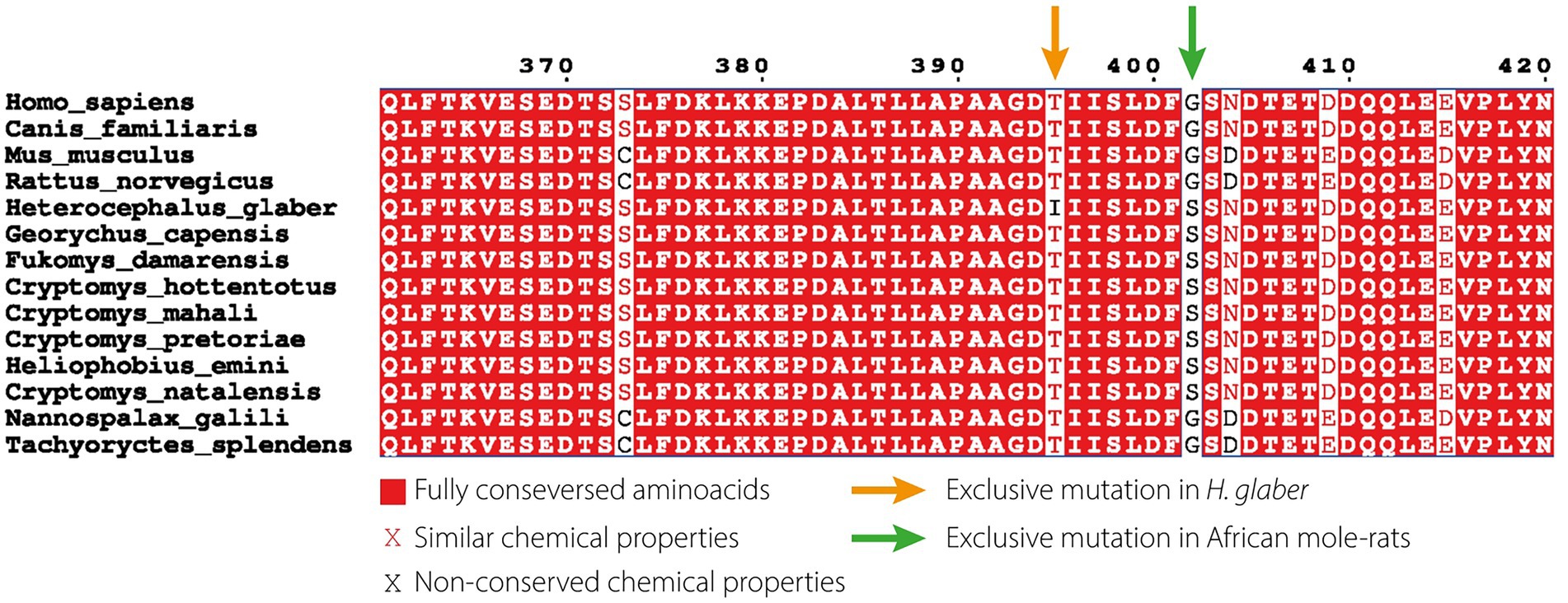
Figure 3. Conservation of HIF1α amongst African mole-rat and other subterranean rodents. The naked mole-rat (H. glaber) presents a unique mutation (T395I) that presumably prevents being targeted for degradation. Alignment generated with ENDscript2 (Robert and Gouet, 2014) using data from Eigenbrod et al. (2019).
Recently, it has been suggested that metabolic regulation is essential for the migration and differentiation of the NCC (Bhattacharya et al., 2021). Indeed, genetic disorders caused by mutations affecting the glucose metabolism produce severe congenital disabilities with severe craniofacial deformations similar to neurocristopathies (Chappell et al., 2009; Smith et al., 2014). The NCC switch to glycolytic metabolism during delamination and migration and return to oxidative phosphorylation during differentiation (Bhattacharya et al., 2021). In fact, HIF1α upregulates many NCC-specific genes and increased, and reduced HIF1α activity results in defects in the NCC migration (Barriga et al., 2013). Furthermore, alterations in VHL are associated with the von Hippel–Lindau syndrome, a neurocristopathy that can cause a variety of neoplasms, including the abnormal tissue growth of the adrenal gland (Vega-Lopez et al., 2018). Intriguingly, more than the 20% of the NMRs in a zoo study presented adrenal hyperplasia (Delaney et al., 2013).
ASIC4
The NMR also presents adaptations to protect the brain against acidification. Acidotoxicity occurs when the accumulation of lactic acid during hypoxia acidifies the medium to pathological levels, triggering the Ca2+ ASICs (acid-sensing ion channels; Xiong et al., 2004). The NMR shows a significantly reduced expression of ASIC4 in the brain compared to mouse, which protects its brain during acidosis (Husson and Smith, 2018). Furthermore, a recent report has identified ASIC4 as a marker of the early differentiation of the NCC (Faure et al., 2020), and ASICs expression has been implicated in the processing of the fear response (Wemmie et al., 2004; Vralsted et al., 2011; Taugher et al., 2017). In particular, ASIC4 in mice seems to modulate the freezing behaviour in the presence of predator odour, but not the shock-evoked fear learning (Lin et al., 2015). This way, mutations affecting the expression of ASICs could reduce the fight-or-flight response in the NMR giving rise to a more docile phenotype.
The subterranean niche and self-domestication
Although AMR and NMR present many of the expected attributes in underground rodents [fusiform body, short limbs, reduced external protuberances (Lacey and Patton, 2000)], many other NMR’s traits cannot be simply explained by adaptation to subterranean niche (reduction of the forebrain, hairlessness, lack of sexual dimorphism, reduction of pain sensitivity, immature thymus). Instead, these traits could be associated with a mild-neurocristopathy.
Traditionally, the DS has been explained as a pleiotropic side effect of selecting for tameness (Belyaev, 1969; Wilkins et al., 2014). However, it is conceivable that the DS is a side effect of selecting for other traits associated with the NCC biology different from the reduction of the adrenal glands and the peripheral nervous system as proposed by Wilkins et al. (2014). We propose that the selection of chisel-tooth digging could result in the appearance of the DS. Chisel-tooth digging is an adaptation to hard soils that requires the widening of the skull to enhance the attachment of larger muscles (Lacey and Patton, 2000; Mcintosh and Cox, 2016). Therefore, hard soils could impose an evolutionary pressure on the cranial NCC to favour the widening of the rostrum that, in turn, caused the appearance of the morphological and behavioural side effects associated with the DS. Furthermore, hard compacted soils can be better exploited in cooperation with other individuals to reduce the cost of burrowing extensive tunnels and improving the chances of finding dispersed food (Faulkes and Bennett, 2013). Consequently, the increased social tolerance associated with the DS could be directly beneficial for subterranean rodents. Curiously, amongst AMR, the only forelimb digger genus (Bathyergus) is solitary and territorially aggressive, whilst all social species (Fukomys, Cryptomys, Heterocephalus) are chisel-tooth diggers (Kingdon, 2014) which suggest that evolution of sociality is easier in chisel-tooth digging species.
Another factor that might have an important impact in the physiology of the NCC is the hypoxic subterranean environment. Adaptations to low oxygen conditions that affect the HIF1α gene-regulatory network and that promote a glycolytic metabolism could have an impact in the NCC migration (see HIF1α). Nevertheless, it is probable that hypoxic environments only get relevant to changing the NCC physiology as subterranean species become more social with larger colony sizes, as seen in the NMR, where hundreds of individuals can inhabit the same colony.
Finally, some of the characteristic traits of the DS could be either beneficial or neutral for a subterranean animal: the dwarfism, reduction of the ear, shortening of the limbs and flattening of the rostrum could be beneficial for moving through tunnels and digging, whilst alterations in colouration are probably less critical to animals living underground. Therefore, once a DS trait is selected (e.g., the flattening of the rostrum for chisel-tooth digging) the other associated traits will be maintained during evolution because they are beneficial or neutral for the subterranean environment.
In summary, we argue that the genes associated with the physiology of the NCC in the NMR have been under evolutionary pressure (Figure 4). We propose that hard soils associated with chisel-tooth digging, in conjunction with the tolerance of subterranean niche for DS traits, fostered the evolution of the DS in AMR. AMR ancestors evolved more solitary and aggressive behaviours -a wild-like phenotype- as they radiated towards more humid areas and encountered more resources and softer soils (Faulkes and Bennett, 2013). Indeed, whilst the solitary AMR (Bathyergus, Georychus, and Heliophobius) are restricted to more humid environments where digging is easier, all social AMR are chisel-tooth diggers (Fukomys and Cryptomys) that mostly inhabit coarse sandy soils and the eusocial AMR (NMR and Damaraland mole-rat) tend to live in areas with hard, compacted soils (Faulkes and Bennett, 2013).
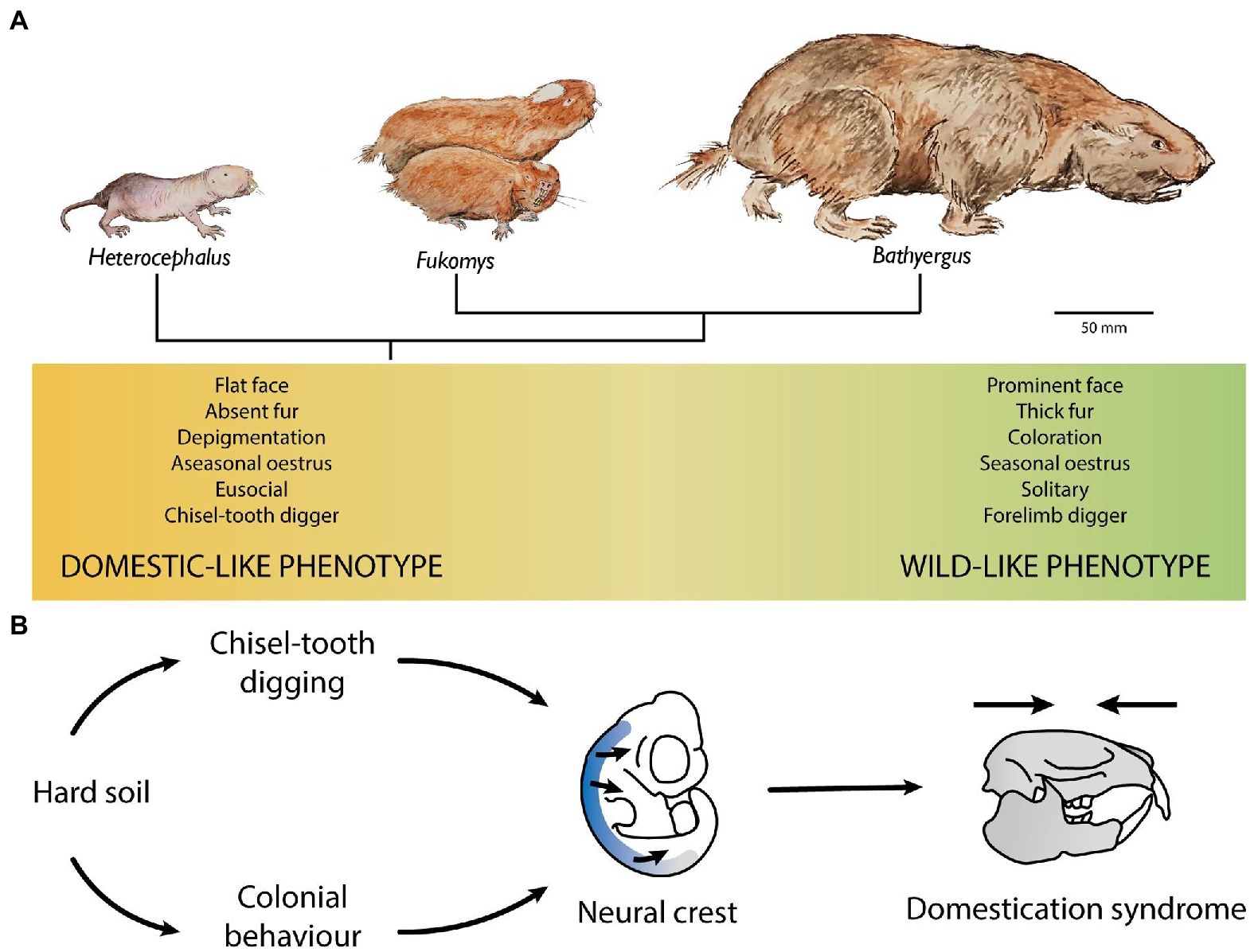
Figure 4. Domestication syndrome in subterranean rodents. (A) Evolution of the African mole-rats from a domestic to a wild morph. (B) The subterranean niche could promote self-domestication. Chisel-tooth digging facilitates breaking hard ground, and a colonial, cooperative lifestyle reduces the amount of work carried out of each individual. Therefore, coloniality and chisel-tooth digging could be adaptations to hard soils. Coloniality needs a high degree of social tolerance, and chisel-tooth digging needs flat faces for large muscle attachments, which could serve as selective pressures foe the neural crest producing the appearance of the domestication syndrome in subterranean rodents. Furthermore, large number of individuals underground generate a hypoxic environment which could favour the appearance of defects in the neural crest development.
Still, the preferred digging mode in the great majority of rodents is scratching the soil with their forelimbs (Vassallo et al., 2021). Indeed, forelimb digging is the primary digging mode in Geomys and Ctenomys, whilst many Spalax species are head-lift diggers (Lacey and Patton, 2000). Altogether, this suggest that that most subterranean rodents will not present domestication syndrome traits. Yet other, genetically unrelated, subterranean chisel-tooth digging species could also present signs of self-domestication. For example, Spalax ehrenbergi is a superspecies of chisel-tooth digging subterranean rodents that inhabit the eastern Mediterranean from Israel to Egypt. Similarly, to AMRs, they present morphological adaptations to living underground such as short limbs, wide crania (Figure 1), with varying coat colouration with occasional white forehead patches (Wolff and Sherman, 2008). Strikingly, although they are described as solitary, chromosomally distinct species present different ratios of aggressive and “pacifist” individuals. The Egyptian Spalax variant, which includes only “pacifist” animals, inhabits the aridest environment (Beiles et al., 1992) and can be distinguished by its shorter mandible (Corti et al., 1996). Furthermore, they present convergent genetic adaptations to the hypoxic environment (Fang et al., 2014; Zheng et al., 2022). Therefore, the Egyptian Spalax could also present DS traits.
Testing the NMR self-domestication hypothesis
The self-domestication hypothesis for NMR presented in this paper can be tested in various ways. First, new behavioural studies are necessary to evaluate the fear response in different AMR species, for example through the evaluation of the freezing behaviour in classic fear-conditioning experiments where a mild electric footshock is applied after an acoustic stimulus. However, the voltage threshold must be carefully calibrated for each species because they potentially present very different pain sensitivities (Lewin, 2020). Alternatively, the freezing behaviour could be measured in the presence of the odour of a predator relevant for AMR species, for example, using snake or small carnivore faeces (Kingdon, 2014). We expect AMRs with a more domesticated phenotype to present an altered fear-conditioned behaviour compared to solitary, forelimb diggers. Furthermore, it is difficult to compare the aggressiveness and fear response of eusocial and solitary species, because cooperative breeders can be affected by their ranking a reproductive status (Toor et al., 2022), variables that should be controlled. Gathering data on the size and activity of the adrenal glands and stress hormones during the fear response across different species could also be informative to test this hypothesis.
To test the implication of NCC in the evolution of AMRs we propose inter-species GFP-labelled NCC grafting experiments (Wilkins et al., 2014). We expect NCC from the NMR to show a deficient migration and/or differentiation in comparison with Bathyergus mole-rats. In addition, we expect that phylogenetic analysis of genes undergoing positive and relaxed selection (Mitterboeck et al., 2017) amongst AMR will highlight genes known for their involvement in NCC development or in neurocristopathies.
Finally, we propose the generation of an extensive database of subterranean and fossorial rodents containing their digging mode and other relevant morphological and behavioural traits. Using this database in combination with statistical analysis corrected by phylogeny to avoid evolutionary relationships affecting the independence of the individual samples (Freckleton et al., 2002), it would be possible to test if the digging mode in subterranean rodents is associated with DS traits and with an increased social behaviour.
Discussion
We showed that the NMR presents a phenotype compatible with the DS when compared with solitary AMRs. We suggest that the DS could be beneficial for the evolution of life underground; and that the digging mode, determined by the properties of the soil, could create an evolutionary pressure on the NCC that generated the DS amongst AMRs. Furthermore, we highlighted some known mutations compatible with the evolution of a domestic phenotype and proposed experiments to test our hypothesis.
Taken all these together, we propose that the high degree of social tolerance necessary for the evolution of cooperative breeding and eusociality in AMRs might have occurred through self-domestication. Hard soils could impose an evolutionary pressure towards chisel-tooth digging, which requires a flatter face to increase the muscle attachments. If the muzzle reduction occurs through the selection of less active NCC, this could generate the pleiotropic effects seen in domestic animals and in neurocristopathies and might increase the social tolerance as a side effect. Moreover, the gathering of large number of individuals in subterranean tunnels generates a hypoxic environment which could lead to molecular adaptations that affect to the NCC development, further enhancing a self-domesticated phenotype.
An increased social tolerance associated with self-domestication could be beneficial for subterranean rodents because a colonial, cooperative lifestyle reduces the digging work carried out for any individual and increases the chances to find disperse food (Faulkes and Bennett, 2013). Therefore, combination of scarce resources in increasingly arid environments (Faulkes and Bennett, 2013, 2016), together with the constraints imposed by hard soils and the underground environment, could create selective pressures that directly and indirectly lead to the self-domestication of the AMR. Intriguingly, in this way, the properties of the soil could act simultaneously as a distal (Faulkes and Bennett, 2013) and proximal factors for the evolution of sociality. In other words, the evolution of sociality in subterranean rodents could be initiated as a side effect of the underground environment and properties of the soil.
Previously, neoteny has been used as a unifying biological explanation of the many distinctive NMR’s morphological and physiological traits (Skulachev et al., 2017; Buffenstein et al., 2020). Nevertheless, it is unclear what evolutionary pressures guided the evolution of a neotenic phenotype or how neotenic traits relate to each other. Instead, we argue that the self-domestication hypothesis is a more comprehensive framework for the evolution of the NMR. In fact, neoteny is often associated with a domestic phenotype, and neotenic and DS traits can be confounded (Sánchez-Villagra et al., 2016). Our hypothesis offers a clear rationale to explain many NMR’s attributes, how they relate to each other and pinpoints the evolutionary pressures that drove them.
The DS can be controversial due to the lack of a consistent definition of the traits amongst different publications (Lord et al., 2020). Under the mild neurocristopathy theory of domestication, the DS is seen as a collection of pleiotropic effects of variable penetrance produced by mutations affecting the NCC, as can be readily seen in human neurocristopathies (Wilkins et al., 2014; Vega-Lopez et al., 2018). Furthermore, in viable animals, the effects of the mutations affecting the NCC can be further confounded by compensatory mutations in some of the altered organs (Pavlicev and Wagner, 2012). For example, all the components of the ear in the NMR show signs of degeneration and intraspecific variation (Mason et al., 2016), however the NMR accumulated positively selected mutations affecting the cochlear hair bundles (Pyott et al., 2020). A plausible interpretation is that the general ear degeneration produced by a mild-neurocrystopathy was later compensated by mutations that readapted the NMR hearing to the subterranean environment. In addition, some other traits associated with DS are neutral in the subterranean niche, and as such, they will not be compensated. This relaxed selective pressure in the underground environment could produce the exacerbation of certain traits, explaining perhaps the highly variable white forehead patch or the defects in the pain perception in the AMR family. Thus, the AMR could show how the combination of positive compensatory selection and relaxed selection can produce variations within the DS.
The study of the NCC could guide future research in the NMR as an animal model and provide new clues to understand its extraordinary biological traits. The recognition of self-domestication and its pleiotropic effects could lead to a deeper understanding of the interactions of social behaviour and the physical environment during evolution.
Data availability statement
The original contributions presented in the study are included in the article/Supplementary material, further inquiries can be directed to the corresponding author.
Author contributions
GSN envisioned the project and wrote the first version of the manuscript. GSN and KK acquired, analysed the data, and revised and corrected the manuscript. KK carried out the phylogenetic analysis. All authors contributed to the article and approved the submitted version.
Acknowledgments
GSN acknowledges support from an EASTBIO BBSRC PhD student training grant (1785593). KK acknowledges support through JSPS Overseas Research Fellowship (H28-1002) and la Caixa postdoctoral junior Leader fellowship (LCF/BQ/PI20/11760009). Both authors thank Kees Weijer for his comments and support, Juan Pedro Fernández Doctor for the illustrations of the African mole-rats and Silvia Estrada Arráez for the representation of the Waardenburg syndrome.
Conflict of interest
The authors declare that the research was conducted in the absence of any commercial or financial relationships that could be construed as a potential conflict of interest.
Publisher’s note
All claims expressed in this article are solely those of the authors and do not necessarily represent those of their affiliated organizations, or those of the publisher, the editors and the reviewers. Any product that may be evaluated in this article, or claim that may be made by its manufacturer, is not guaranteed or endorsed by the publisher.
Supplementary material
The Supplementary material for this article can be found online at: https://www.frontiersin.org/articless/10.3389/fevo.2022.987177/full#supplementary-material
References
Barker, A. J., Veviurko, G., Bennett, N. C., Hart, D. W., Mograby, L., and Lewin, G. R. (2021). Cultural transmission of vocal dialect in the naked mole-rat. Science 371, 503–507. doi: 10.1126/science.abc6588
Barriga, E. H., Maxwell, P. H., Reyes, A. E., and Mayor, R. (2013). The hypoxia factor hif-1α controls neural crest chemotaxis and epithelial to mesenchymal transition. J. Cell Biol. 201, 759–776. doi: 10.1083/jcb.201212100
Begall, S., Bottermann, L., and Caspar, K. R. (2022). Self-domestication underground? Testing for social and morphological correlates of animal personality in cooperatively-breeding Ansell’s mole-rats (Fukomys anselli). Front. Ecol. Evol. 10: 364. doi: 10.3389/fevo.2022.862082
Beiles, A., Heth, G., Nevo, E., and Simson, S. (1992). Adaptive pacifistic behaviour in subterranean mole rats in the Sahara desert, contrasting to and originating from polymorphic aggression in Israeli species. Behaviour 123, 70–76. doi: 10.1163/156853992X00110
Bhattacharya, D., Khan, B., and Simoes-Costa, M. (2021). Neural crest metabolism: at the crossroads of development and disease. Dev. Biol. 475, 245–255. doi: 10.1016/j.ydbio.2021.01.018
Braude, S., Holtze, S., Begall, S., Brenmoehl, J., Burda, H., Dammann, P., et al. (2021). Surprisingly long survival of premature conclusions about naked mole-rat biology. Biol. Rev. 96, 376–393. doi: 10.1111/brv.12660
Buffenstein, R. (2008). Negligible senescence in the longest living rodent, the naked mole-rat: insights from a successfully aging species. J. Comp. Physiol. B 178, 439–445. doi: 10.1007/s00360-007-0237-5
Buffenstein, R., Lewis, K. N., Gibney, P. A., Narayan, V., Grimes, K. M., Smith, M., et al. (2020). Probing Pedomorphy and prolonged lifespan in naked mole-rats and dwarf mice. Physiology (Bethesda) 35, 96–111. doi: 10.1152/physiol.00032.2019
Buffenstein, R., and Pinto, M. (2009). Endocrine function in naturally long-living small mammals. Mol. Cell. Endocrinol. 299, 101–111. doi: 10.1016/j.mce.2008.04.021
Buffenstein, R., Woodley, R., Thomadakis, C., Daly, T. J., and Gray, D. A. (2001). Cold-induced changes in thyroid function in a poikilothermic mammal, the naked mole-rat. Am. J. Physiol. Regul. Integr. Comp. Physiol. 280, R149–R155. doi: 10.1152/ajpregu.2001.280.1.R149
Burda, H., Honeycutt, R. L., Begall, S., Locker-Grütjen, O., and Scharff, A. (2000). Are naked and common mole-rats eusocial and if so, why? Behav. Ecol. Sociobiol. 47, 293–303. doi: 10.1007/s002650050669
Cagan, A., and Blass, T. (2016). Identification of genomic variants putatively targeted by selection during dog domestication. BMC Evol. Biol. 16:10. doi: 10.1186/s12862-015-0579-7
Chappell, J. H., Wang, X. D., and Loeken, M. R. (2009). Diabetes and apoptosis: neural crest cells and neural tube. Apoptosis 14, 1472–1483. doi: 10.1007/s10495-009-0338-6
Chinen, J., Rosenblatt, H. M., Smith, E., Shearer, W. T., and Noroski, L. M. (2003). Long-term assessment of t-cell populations in digeorge syndrome. J. Allergy Clin. Immunol. 111, 573–579. doi: 10.1067/mai.2003.165
Clarke, F. M., and Faulkes, C. G. (1997). Dominance and queen succession in captive colonies of the eusocial naked mole-rat, Heterocephalus glaber. Proc. R. Soc. B Biol. Sci. 264, 993–1000. doi: 10.1098/rspb.1997.0137
Clarke, F. M., and Faulkes, C. G. (2001). Intracolony aggression in the eusocial naked mole-rat, Heterocephalus glaber. Anim. Behav. 61, 311–324. doi: 10.1006/anbe.2000.1573
Cooper, W. E., Pyron, R. A., and Garland, T. (2014). Island tameness: living on islands reduces flight initiation distance. Proc. R. Soc. B Biol. Sci. 281, 1–7. doi: 10.1098/rspb.2013.3019
Corti, M., Fadda, C., Simson, S., and Nevo, E. (1996). “Size and shape variation in the mandible of the fossorial rodent Spalax ehrenbergi: a Procrustes analysis of three dimensions” in Advances in Morphometrics. eds. L. F. Marcus, M. Corti, A. Loy, G. Naylor, and D. E. Slice (New York: Plenum), 303–320.
Creel, S. (2001). Social dominance and stress hormones. Trends Ecol. Evol. 16, 491–497. doi: 10.1016/S0169-5347(01)02227-3
Crockford, S. J., (2004). Animal Domestication and Vertebrate Speciation: A Paradigm for the Origin of Species. Ph.D. Dissertation. University of Victoria, British Columbia.
Delaney, M., Nagy, L., Kinsel, M., and Treuting, P. (2013). Spontaneous histologic lesions of the adult naked mole rat (Heterocephalus glaber) a retrospective survey of lesions in a zoo population. Vet. Pathol. 50, 607–621. doi: 10.1177/0300985812471543
Desiderio, S., Vermeiren, S., Van Campenhout, C., Kricha, S., Malki, E., Richts, S., et al. (2019). Prdm12 directs nociceptive sensory neuron development by regulating the expression of the NGF receptor TrkA. Cell Rep. 26, 3522–3536. doi: 10.1016/j.celrep.2019.02.097
Dominy, N. J., Vogel, E. R., Yeakel, J. D., Constantino, P., and Lucas, P. W. (2008). Mechanical properties of plant underground storage organs and implications for dietary models of early hominins. Evol. Biol. 35, 159–175. doi: 10.1007/s11692-008-9026-7
Edwards, P. D., Mooney, S. J., Bosson, C. O., Toor, I., Palme, R., Holmes, M. M., et al. (2020). The stress of being alone: removal from the colony, but not social subordination, increases fecal cortisol metabolite levels in eusocial naked mole-rats. Horm. Behav. 121:104720. doi: 10.1016/j.yhbeh.2020.104720
Eigenbrod, O., Debus, K. Y., Reznick, J., Bennett, N. C., Sánchez-Carranza, O., Omerbašić, D., et al. (2019). Rapid molecular evolution of pain insensitivity in multiple African rodents. Science 364, 852–859. doi: 10.1126/science.aau0236
Fang, X., Nevo, E., Han, L., Levanon, E. Y., Zhao, J., Avivi, A., et al. (2014). Genome-wide adaptive complexes to underground stresses in blind mole rats spalax. Nat. Commun. 5, 1–11. doi: 10.1038/ncomms4966
Faulkes, C. G., Abbott, D. H., and Jarvis, J. U. (1990). Social suppression of ovarian cyclicity in captive and wild colonies of naked mole-rats, Heterocephalus glaber. J. Reprod. Fertil. 88, 559–568. doi: 10.1530/jrf.0.0880559
Faulkes, C. G., Abbott, D. H., and Jarvis, J. U. (1991). Social suppression of reproduction in male naked molerats, Heterocephalus glaber. J. Reprod. Fertil. 91, 593–604. doi: 10.1530/jrf.0.0910593
Faulkes, C. G., and Bennett, N. C. (2001). Family values: group dynamics and social control of reproduction in African mole-rats. Trends Ecol. Evol. 16, 184–190. doi: 10.1016/S0169-5347(01)02116-4
Faulkes, C. G., and Bennett, N. C. (2013). Plasticity and constraints on social evolution in African mole-rats: ultimate and proximate factors. Philos. Trans. R. Soc. B Biol. Sci. 368, 1–10. doi: 10.1098/rstb.2012.0347
Faulkes, C. G., and Bennett, N. C. (2016). “Damaraland and naked mole-rats: convergence of social evolution” in Cooperative Breeding in Vertebrates: Studies of Ecology, Evolution, and Behavior eds. K. D. Walter and Dickinson (Cambridge, UK: Cambridge University Press), 338–352.
Faure, L., Wang, Y., Kastriti, M. E., Fontanet, P., Cheung, K. K., Petitpré, C., et al. (2020). Single cell RNA sequencing identifies early diversity of sensory neurons forming via bi-potential intermediates. Nat. Commun. 11, 1–15. doi: 10.1038/s41467-020-17929-4
Faykoo-Martinez, M., Monks, D. A., Zovkic, I. B., and Holmes, M. M. (2018). Sex-and brain region-specific patterns of gene expression associated with socially-mediated puberty in a eusocial mammal. PLoS One 13:e0193417. doi: 10.1371/journal.pone.0193417
Fitak, R. R., Mohandesan, E., Corander, J., Yadamsuren, A., Chuluunbat, B., Abdelhadi, O., et al. (2020). Genomic signatures of domestication in Old World camels. Commun. Biol. 3:316. doi: 10.1038/s42003-020-1039-5
Freckleton, R. P., Harvey, P. H., and Pagel, M. (2002). Phylogenetic analysis and comparative data: a test and review of evidence. Am. Nat. 160, 712–726. doi: 10.1086/343873
Ganem, G., and Bennett, N. C. (2004). Tolerance to unfamiliar conspecifics varies with social organization in female African mole-rats. Physiol. Behav. 82, 555–562. doi: 10.1016/j.physbeh.2004.05.002
Gao, L., Guo, H., Ye, N., Bai, Y., Liu, X., Yu, P., et al. (2013). Oral and craniofacial manifestations and two novel missense mutations of the NTRK1 gene identified in the patient with congenital insensitivity to pain with anhidrosis. PLoS One 8:e66863. doi: 10.1371/journal.pone.0066863
Garcıa-Suárez, O., Germanà, A., Hannestad, J., Ciriaco, E., Laurà, R., Naves, J., et al. (2000). TrkA is necessary for the normal development of the murine thymus. J. Neuroimmunol. 108, 11–21. doi: 10.1016/S0165-5728(00)00251-4
Geiger, M., Evin, A., Sánchez-Villagra, M. R., Gascho, D., Mainini, C., and Zollikofer, C. P. E. (2017). Neomorphosis and heterochrony of skull shape in dog domestication. Sci. Rep. 7:13443. doi: 10.1038/s41598-017-12582-2
Ghazanfar, A. A., Kelly, L. M., Takahashi, D. Y., Winters, S., Terrett, R., and Higham, J. P. (2020). Domestication phenotype linked to vocal behavior in marmoset monkeys. Current Biol.: CB 30, 5026–5032.e3. doi: 10.1016/j.cub.2020.09.049
Gomes Rodrigues, H., and Šumbera, R. (2015). Dental peculiarities in the silvery mole-rat: an original model for studying the evolutionary and biological origins of continuous dental generation in mammals. PeerJ 3:e1233. doi: 10.7717/peerj.1233
Grimes, K. M., Voorhees, A., Chiao, Y. A., Han, H.-C., Lindsey, M. L., and Buffenstein, R. (2013). Cardiac function of the naked mole-rat: ecophysiological responses to working underground. Am. J. Phys. Heart Circ. Phys. 306, H730–H737. doi: 10.1152/ajpheart.00831.2013
Hare, B., Wobber, V., and Wrangham, R. (2012). The self-domestication hypothesis: evolution of bonobo psychology is due to selection against aggression. Anim. Behav. 83, 573–585. doi: 10.1016/j.anbehav.2011.12.007
Hill, W. C. O., Porter, A., Bloom, R. T., Seago, J., and Southwick, M. D. (1957). Field and laboratory studies on the naked mole rat, Heterocephalus glaber. Proc. Zool. Soc. London 128, 455–514. doi: 10.1111/j.1096-3642.1957.tb00272.x
Holmes, M. M., Goldman, B. D., and Forger, N. G. (2008). Social status and sex independently influence androgen receptor expression in the eusocial naked mole-rat brain. Horm. Behav. 54, 278–285. doi: 10.1016/j.yhbeh.2008.03.010
Holmes, M. M., Rosen, G. J., Jordan, C. L., Vries, G. J. D., Goldman, B. D., and Forger, N. G. (2007). Social control of brain morphology in a eusocial mammal. Proc. Natl. Acad. Sci. U. S. A. 104, 10548–10552. doi: 10.1186/s13041-018-0369-4
Husson, Z., and Smith, E. S. J. (2018). Naked mole-rat cortical neurons are resistant to acid-induced cell death. Mol. Brain 11, 1–10. doi: 10.1073/pnas.0610344104
Indo, Y. (2009). Nerve growth factor, interoception, and sympathetic neuron: lesson from congenital insensitivity to pain with anhidrosis. Auton. Neurosci. 147, 3–8. doi: 10.1016/j.autneu.2009.01.008
Jarvis, J. U. (1981). Eusociality in a mammal: cooperative breeding in naked mole-rat colonies. Science 212, 571–573. doi: 10.1126/science.7209555
Jarvis, J. U. M., and Sherman, P. W. (2002). Heterocephalus glaber. Mamm. Species 2002, 1–9. doi: 10.1644/1545-1410(2002)706<0001:HG>2.0.CO;2
Jiang, L., Kundu, S., Lederman, J. D., López-Hernández, G. Y., Ballinger, E. C., Wang, S., et al. (2016). Cholinergic signaling controls conditioned fear behaviors and enhances plasticity of cortical-amygdala circuits. Neuron 90, 1057–1070. doi: 10.1016/j.neuron.2016.04.028
Kalamatianos, T., Faulkes, C. G., Oosthuizen, M. K., Poorun, R., Bennett, N. C., and Coen, C. W. (2010). Telencephalic binding sites for oxytocin and social organization: a comparative study of eusocial naked molerats and solitary cape mole-rats. J. Comp. Neurol. 518, 1792–1813. doi: 10.1002/cne.22302
Karlsson, A. C., Fallahshahroudi, A., Johnsen, H., Hagenblad, J., Wright, D., Andersson, L., et al. (2016). A domestication related mutation in the thyroid stimulating hormone receptor gene (TSHR) modulates photoperiodic response and reproduction in chickens. Gen. Comp. Endocrinol. 228, 69–78. doi: 10.1016/j.ygcen.2016.02.010
Keeler, C., Mellinger, T., Fromm, E., and Wade, L. (1970). Melanin, adrenalin and the legacy of fear. J. Hered. 61, 81–88. doi: 10.1093/oxfordjournals.jhered.a108047
Kikusui, T., Nagasawa, M., Nomoto, K., Kuse-Arata, S., and Mogi, K. (2019). Endocrine regulations in human–dog coexistence through domestication. Trends Endocrinol. Metab. 30, 793–806. doi: 10.1016/j.tem.2019.09.002
Kim, E. B., Fang, X., Fushan, A. A., Huang, Z., Lobanov, A. V., Han, L., et al. (2011). Genome sequencing reveals insights into physiology and longevity of the naked mole rat. Nature 479, 223–227. doi: 10.1038/nature10533
Kingdon, J. (2014). Mammals of Africa: Volume III: Rodents, Hares and Rabbits. Bloomsbury Publishing, London, England (2013).
Kleisner, K., and Stella, M. (2009). Monsters we met, monsters we made: on the parallel emergence of phenotypic similarity under domestication. Sign Syst. Stud. 37, 454–476. doi: 10.12697/SSS.2009.37.3-4.04
Kruska, D. C. (2005). On the evolutionary significance of encephalization in some eutherian mammals: effects of adaptive radiation, domestication, and feralization. Brain Behav. Evol. 65, 73–108. doi: 10.1159/000082979
Kverková, K., Bělíková, T., Olkowicz, S., Pavelková, Z., O’Riain, M. J., Šumbera, R., et al. (2018). Sociality does not drive the evolution of large brains in eusocial African mole-rats. Sci. Rep. 8, 1–14. doi: 10.1038/s41598-018-26062-8
Lacey, E. A., and Patton, J. L. (2000). Life Underground: The Biology of Subterranean Rodents Chicago, USA: University of Chicago Press.
Lewin, G. R. (2020). William D. Willis, Jr, MD, PhD Memorial Lecture: the evolutionary history of nerve growth factor and nociception. Pain 161:S36. doi: 10.1097/j.pain.0000000000001889
Lewin, G. R., Lechner, S. G., and Smith, E. S. J. (2014). Nerve growth factor and nociception: from experimental embryology to new analgesic therapy. Handb. Exp. Pharmacol. 220, 251–282. doi: 10.1007/978-3-642-45106-5_10
Lin, T., and Buffenstein, R. (2021). The unusual immune system of the naked mole-rat. Adv. Exp. Med. Biol. 1319, 315–327. doi: 10.1007/978-3-030-65943-1_12
Lin, S.-H., Chien, Y.-C., Chiang, W.-W., Liu, Y.-Z., Lien, C.-C., and Chen, C.-C. (2015). Genetic mapping of asic 4 and contrasting phenotype to asic 1a in modulating innate fear and anxiety. Eur. J. Neurosci. 41, 1553–1568. doi: 10.1111/ejn.12905
Lord, K. A., Larson, G., Coppinger, R. P., and Karlsson, E. K. (2020). The history of farm foxes undermines the animal domestication syndrome. Trends Ecol. Evol. 35, 125–136. doi: 10.1016/j.tree.2019.10.011
MacManes, M. D., and Lacey, E. A. (2012). The social brain: transcriptome assembly and characterization of the hippocampus from a social subterranean rodent, the colonial Tuco-Tuco (Ctenomys sociabilis). PLoS One 7:e45524. doi: 10.1371/journal.pone.0045524
Maeda, K., Asai, R., Maruyama, K., Kurihara, Y., Nakanishi, T., Kurihara, H., et al. (2016). Postotic and preotic cranial neural crest cells differently contribute to thyroid development. Dev. Biol. 409, 72–83. doi: 10.1016/j.ydbio.2015.10.026
Mason, M. J., Cornwall, H. L., and Smith, E. S. J. (2016). Ear structures of the naked mole-rat, Heterocephalus glaber, and its relatives (rodentia: Bathyergidae). PLoS One 11:e0167079. doi: 10.1371/journal.pone.0167079
Mawe, G. M., Talmage, E. K., Cornbrooks, E. B., Gokin, A. P., Zhang, L., and Jennings, L. J. (1997). Innervation of the gallbladder: structure, neurochemical coding, and physiological properties of Guinea pig gallbladder ganglia. Microsc. Res. Tech. 39, 1–13. doi: 10.1002/(SICI)1097-0029(19971001)39:1<1::AID-JEMT1>3.0.CO;2-R
Mcintosh, A. F., and Cox, P. G. (2016). Functional implications of craniomandibular morphology in African mole-rats (rodentia: Bathyergidae). Biol. J. Linn. Soc. 117, 447–462. doi: 10.1111/bij.12691
Medger, K., Bennett, N. C., Ganswindt, S. B., Ganswindt, A., and Hart, D. W. (2019). Changes in prolactin, cortisol and testosterone concentrations during queen succession in a colony of naked mole-rats (Heterocephalus glaber): a case study. Sci. Nat. 106, 1–7. doi: 10.1007/s00114-019-1621-1
Medger, K., Bennett, N. C., Lutermann, H., and Ganswindt, A. (2018). Non-invasive assessment of glucocorticoid and androgen metabolite levels in cooperatively breeding damaraland mole-rats (Fukomys damarensis). Gen. Comp. Endocrinol. 266, 202–210. doi: 10.1016/j.ygcen.2018.05.018
Mitterboeck, T. F., Liu, S., Adamowicz, S. J., Fu, J., Zhang, R., Song, W., et al. (2017). Positive and relaxed selection associated with flight evolution and loss in insect transcriptomes. GigaScience 6, 1–14. doi: 10.1093/gigascience/gix073
Montoya-Sanhueza, G., Šaffa, G., Šumbera, R., Chinsamy, A., Jarvis, J. U., and Bennett, N. C. (2022). Fossorial adaptations in African mole-rats (Bathyergidae) and the unique appendicular phenotype of naked mole-rats. Communications Biology, 5, 1–13.
Mooney, S. J., and Holmes, M. M. (2013). Social condition and oxytocin neuron number in the hypothalamus of naked mole-rats (Heterocephalus glaber). Neuroscience 230, 56–61. doi: 10.1016/j.neuroscience.2012.11.014
Nätt, D., Kerje, S., Andersson, L., and Jensen, P. (2007). Plumage color and feather pecking—behavioral differences associated with pmel17 genotypes in chicken (Gallus gallus). Behav. Genet. 37, 399–407. doi: 10.1007/s10519-006-9125-0
O’riain, M., and Jarvis, J. (1997). Colony member recognition and xenophobia in the naked mole-rat. Anim. Behav. 53, 487–498. doi: 10.1006/anbe.1996.0299
Omerbašić, D., Smith, E. S. J., Moroni, M., Homfeld, J., Eigenbrod, O., Bennett, N. C., et al. (2016). Hypofunctional TrkA accounts for the absence of pain sensitization in the African naked mole-rat. Cell Rep. 17, 748–758. doi: 10.1016/j.celrep.2016.09.035
Park, T. J., Reznick, J., Peterson, B. L., Blass, G., Omerbašić, D., Bennett, N. C., et al. (2017). Fructose-driven glycolysis supports anoxia resistance in the naked mole-rat. Science 356, 307–311. doi: 10.1126/science.aab3896
Park, T. J., Smith, E., Reznick, J., Bennett, N. C., Applegate, D. T., Larson, J., et al. (2021). African naked mole-rats demonstrate extreme tolerance to hypoxia and Hypercapnia. Adv. Exp. Med. Biol. 1319, 255–269. doi: 10.1007/978-3-030-65943-1_9
Pavlicev, M., and Wagner, G. P. (2012). A model of developmental evolution: selection, pleiotropy and compensation. Trends Ecol. Evol. 27, 316–322. doi: 10.1016/j.tree.2012.01.016
Peragine, D. E., Pokarowski, M., Mendoza-Viveros, L., Swift-Gallant, A., Cheng, H. Y. M., Bentley, G. E., et al. (2017). Rfamide-related peptide-3 (rfrp-3) suppresses sexual maturation in a eusocial mammal. Proc. Natl. Acad. Sci. U. S. A. 114, 1207–1212. doi: 10.1073/pnas.1616913114
Peroulakis, M. E., Goldman, B., and Forger, N. G. (2002). Perineal muscles and motoneurons are sexually monomorphic in the naked mole-rat (Heterocephalus glaber). J. Neurobiol. 51, 33–42. doi: 10.1002/neu.10039
Pinto, M., Jepsen, K. J., Terranova, C. J., and Buffenstein, R. (2010). Lack of sexual dimorphism in femora of the eusocial and hypogonadic naked mole-rat: a novel animal model for the study of delayed puberty on the skeletal system. Bone 46, 112–120. doi: 10.1016/j.bone.2009.08.060
Prendergast, A., and Raible, D. W. (2014). Neural Crest Cells and Peripheral Nervous System Development. Boston, USA: Elsevier Inc.
Prigione, A., Rohwer, N., Hoffmann, S., Mlody, B., Drews, K., Bukowiecki, R., et al. (2014). Hif1α modulates cell fate reprogramming through early glycolytic shift and upregulation of pdk1–3 and pkm2. Stem Cells 32, 364–376. doi: 10.1002/stem.1552
Pyott, S. J., van Tuinen, M., Screven, L. A., Schrode, K. M., Bai, J.-P., Barone, C. M., et al. (2020). Functional, morphological, and evolutionary characterization of hearing in subterranean, eusocial African mole-rats. Curr. Biol. 30, 4329–4341. doi: 10.1016/j.cub.2020.08.035
Ramis, D., and Bover, P. (2001). A review of the evidence for domestication of Myotragus balearicus bate 1909 (artiodactyle, caprinae) in the Balearic Islands. J. Archaeol. Sci. 28, 265–282. doi: 10.1006/jasc.2000.0548
Ritter, K. E., and Martin, D. M. (2019). Neural crest contributions to the ear: implications for congenital hearing disorders. Hear. Res. 376, 22–32. doi: 10.1016/j.heares.2018.11.005
Robert, X., and Gouet, P. (2014). Deciphering key features in protein structures with the new ENDscript server. Nucleic Acids Res. 42, W320–W324. doi: 10.1093/nar/gku316
Sanchez-Ortiz, E., Yui, D., Song, D., Li, Y., Rubenstein, J. L., Reichardt, L. F., et al. (2012). Trka gene ablation in basal forebrain results in dysfunction of the cholinergic circuitry. J. Neurosci. 32, 4065–4079. doi: 10.1523/JNEUROSCI.6314-11.2012
Sánchez-Villagra, M. R., Geiger, M., and Schneider, R. A. (2016). The taming of the neural crest: a developmental perspective on the origins of morphological covariation in domesticated mammals. R. Soc. Open Sci. 3:160107. doi: 10.1098/rsos.160107
Sánchez-Villagra, M. R., and van Schaik, C. P. (2019). Evaluating the self-domestication hypothesis of human evolution. Evol. Anthropol. 28, 133–143. doi: 10.1002/evan.21777
Sapolsky, R. M. (2005). The influence of social hierarchy on primate health. Science 308, 648–652. doi: 10.1126/science.1106477
Schlitter, D. A., Ferguson, A. W., and McDonough, M. M. (2014). Mammals of Africa (vol. i-vi.). J. Mammal. 95, 1299–1303. doi: 10.1644/14-MAMM-R-122
Seney, M. L., Kelly, D. A., Goldman, B. D., Šumbera, R., and Forger, N. G. (2009). Social structure predicts genital morphology in African mole-rats. PLoS One 4:7477. doi: 10.1371/journal.pone.0007477
Shea, B. T. (1989). Heterochrony in human evolution: the case for neoteny reconsidered. Am. J. Phys. Anthropol. 32, 69–101. doi: 10.1002/ajpa.1330320505
Shebzukhov, Y., Holtze, S., Hirseland, H., Schäfer, H., Radbruch, A., Hildebrandt, T., et al. (2019). Identification of cross-reactive antibodies for the detection of lymphocytes, myeloid cells and haematopoietic precursors in the naked mole rat. Eur. J. Immunol. 49, 2103–2110. doi: 10.1002/eji.201948124
Singh, N., Albert, F. W., Plyusnina, I., Trut, L., P bo, S., and Harvati, K. (2017). Facial shape differences between rats selected for tame and aggressive behaviors. PLoS One 12:e0175043. doi: 10.1371/journal.pone.0175043
Skulachev, V. P., Holtze, S., Vyssokikh, M. Y., Bakeeva, L. E., Skulachev, M. V., Markov, A. V., et al. (2017). Neoteny, prolongation of youth: from naked mole rats to “naked apes” (humans). Physiol. Rev. 97, 699–720. doi: 10.1152/physrev.00040.2015
Smeyne, R. J., Klein, R., Schnapp, A., Long, L. K., Bryant, S., Lewin, A., et al. (1994). Severe sensory and sympathetic neuropathies in mice carrying a disrupted Trk/NGF receptor gene. Nature 368, 246–249. doi: 10.1038/368246a0
Smith, T. E., and French, J. A. (1997). Social and reproductive conditions modulate urinary cortisol excretion in black tufted-ear marmosets (Callithrix kuhli). Am. J. Primatol. 42, 253–267. doi: 10.1002/(SICI)1098-2345(1997)42:4<253::AID-AJP1>3.0.CO;2-W
Smith, S. M., Garic, A., Flentke, G. R., and Berres, M. E. (2014). Neural crest development in fetal alcohol syndrome. Birth Defects Res. C. Embryo Today 102, 210–220. doi: 10.1002/bdrc.21078
Smith, E. S. J., Purfürst, B., Grigoryan, T., Park, T. J., Bennett, N. C., and Lewin, G. R. (2012). Specific paucity of unmyelinated c-fibers in cutaneous peripheral nerves of the African naked-mole rat: comparative analysis using six species of bathyergidae. J. Comp. Neurol. 520, 2785–2803. doi: 10.1002/cne.23133
Swift-Gallant, A., Mo, K., Peragine, D. E., Monks, D. A., and Holmes, M. M. (2015). Removal of reproductive suppression reveals latent sex differences in brain steroid hormone receptors in naked mole-rats, Heterocephalus glaber. Biol. Sex Differ. 6, 1–9. doi: 10.1186/s13293-015-0050-x
Taugher, R. J., Lu, Y., Fan, R., Ghobbeh, A., Kreple, C. J., Faraci, F. M., et al. (2017). Asic1a in neurons is critical for fear-related behaviors. Genes Brain Behav. 16, 745–755. doi: 10.1111/gbb.12398
Tchernov, E., and Horwitz, L. K. (1991). Body size diminution under domestication: unconscious selection in primeval domesticates. J. Anthropol. Archaeol. 10, 54–75. doi: 10.1016/0278-4165(91)90021-O
Theofanopoulou, C., Gastaldon, S., O’Rourke, T., Samuels, B. D., Messner, A., Martins, P. T., et al. (2017). Self-domestication in homo sapiens: insights from comparative genomics. PLoS One 12, 5–7. doi: 10.1371/journal.pone.0185306
Toor, I., Maynard, R., Peng, X., Beery, A. K., and Holmes, M. M. (2022). Naked mole-rat social phenotypes vary in investigative and aggressive behavior in a laboratory partner preference paradigm. Front. Ecol. Evol. :379. doi: 10.3389/fevo.2022.860885
Trut, L. N. (1999). Early Canid domestication: the farm-fox experiment: foxes bred for tamability in a 40-year experiment exhibit remarkable transformations that suggest an interplay between behavioral genetics and development. Am. Sci. 87, 160–169. doi: 10.1511/1999.2.160
Trut, L. N., Dzerzhinski, I. F. I., and Nikol’ski, I. V. S. (1991). Intracranial allometry and craniologic changes during domestication of silver foxes. Genetika 27, 1605–1611. doi: 10.1002/bies.200800070
Trut, L., Oskina, I., and Kharlamova, A. (2009). Animal evolution during domestication: the domesticated fox as a model domestication in evolutionary terms – from Darwin to the present day. National Inst. Health 31, 349–360.
Varum, S., Rodrigues, A. S., Moura, M. B., Momcilovic, O., Easley, C. A. IV, Ramalho-Santos, J., et al. (2011). Energy metabolism in human pluripotent stem cells and their differentiated counterparts. PLoS One 6:e20914. doi: 10.1371/journal.pone.0020914
Vassallo, A. I., Becerra, F., Echeverría, A. I., Buezas, G. N., Díaz, A. O., Longo, M., et al. (2021). “Biomechanics and strategies of digging” in Tuco-Tucos eds T. R. O. Freitas, G. L. Gonçalves, and R. Maestri (Springer), 141–165.
Vega-Lopez, G. A., Cerrizuela, S., Tribulo, C., and Aybar, M. J. (2018). Neurocristopathies: new insights 150 years after the neural crest discovery. Dev. Biol. 444, S110–S143. doi: 10.1016/j.ydbio.2018.05.013
Vralsted, V. C., Price, M. P., Du, J., Schnizler, M., Wunsch, A. M., Ziemann, A. E., et al. (2011). Expressing acid-sensing ion channel 3 in the brain alters acid-evoked currents and impairs fear conditioning. Genes Brain Behav. 10, 444–450. doi: 10.1111/j.1601-183X.2011.00683.x
Wang, X., Pipes, L., Trut, L. N., Herbeck, Y., Vladimirova, A. V., Gulevich, R. G., et al. (2018). Genomic responses to selection for tame/aggressive behaviors in the silver fox (Vulpes vulpes). Proc. Natl. Acad. Sci. U. S. A. 115, 10398–10403. doi: 10.1073/pnas.1800889115
Wemmie, J. A., Coryell, M. W., Askwith, C. C., Lamani, E., Leonard, A. S., Sigmund, C. D., et al. (2004). Overexpression of acid-sensing ion channel 1a in transgenic mice increases acquired fear-related behavior. Proc. Natl. Acad. Sci. U. S. A. 101, 3621–3626. doi: 10.1073/pnas.0308753101
Wilkins, A. S., Wrangham, R. W., and Fitch, W. T. (2014). The "domestication syndrome" in mammals: a unified explanation based on neural crest cell behavior and genetics. Genetics 197, 795–808. doi: 10.1534/genetics.114.165423
Wislet, S., Vandervelden, G., and Rogister, B. (2018). From neural crest development to cancer and vice versa: how p75ntr and (pro) neurotrophins could act on cell migration and invasion? Front. Mol. Neurosci. 11:244. doi: 10.3389/fnmol.2018.00244
Wolff, J. O., and Sherman, P. W. (2008). Rodent Societies: An Ecological and Evolutionary Perspective, Chicago, USA University of Chicago Press.
Xiao, B., Wang, S., Yang, G., Sun, X., Zhao, S., Lin, L., et al. (2017). Hif-1α contributes to hypoxia adaptation of the naked mole rat. Oncotarget 8:109941. doi: 10.18632/oncotarget.22767
Xiong, Z.-G., Zhu, X.-M., Chu, X.-P., Minami, M., Hey, J., Wei, W.-L., et al. (2004). Neuroprotection in ischemia: blocking calcium-permeable acid-sensing ion channels. Cells 118, 687–698. doi: 10.1016/j.cell.2004.08.026
Zachariah, M. A., and Cyster, J. G. (2010). Neural crest–derived pericytes promote egress of mature thymocytes at the corticomedullary junction. Science 328, 1129–1135. doi: 10.1126/science.1188222
Zanella, M., Vitriolo, A., Andirko, A., Martins, P. T., Sturm, S., O’Rourke, T., et al. (2019). Dosage analysis of the 7q11.23 Williams region identifies baz1b as a major human gene patterning the modern human face and underlying self-domestication. Sci. Adv. 5:eaaw7908. doi: 10.1126/sciadv.aaw7908
Zheng, Z., Hua, R., Xu, G., Yang, H., and Shi, P. (2022). Gene losses may contribute to subterranean adaptations in naked mole-rat and blind mole-rat. BMC Biol. 20, 1–17. doi: 10.1186/s12915-022-01243-0
Keywords: naked mole-rat, African mole-rat, eusociality, domestication, neural crest, digging behaviour, chisel-tooth digging
Citation: Serrano Nájera G and Kin K (2022) Unusual occurrence of domestication syndrome amongst African mole-rats: Is the naked mole-rat a domestic animal? Front. Ecol. Evol. 10:987177. doi: 10.3389/fevo.2022.987177
Edited by:
Edivaldo Herculano Correa De Oliveira, Evandro Chagas Institute, BrazilReviewed by:
Ricardo Lehtonen Rodrigues Souza, Federal University of Paraná, BrazilStan Braude, Washington University in St. Louis, United States
Copyright © 2022 Serrano Nájera and Kin. This is an open-access article distributed under the terms of the Creative Commons Attribution License (CC BY). The use, distribution or reproduction in other forums is permitted, provided the original author(s) and the copyright owner(s) are credited and that the original publication in this journal is cited, in accordance with accepted academic practice. No use, distribution or reproduction is permitted which does not comply with these terms.
*Correspondence: Guillermo Serrano Nájera, Z3VpbGxlcm1vLnNlcnJhbm8tbmFqZXJhQGdlbi5jYW0uYWMudWs=
†ORCID: Guillermo Serrano Nájera https://orcid.org/0000-0001-5841-8408
Koryu Kin https://orcid.org/0000-0003-2409-7276