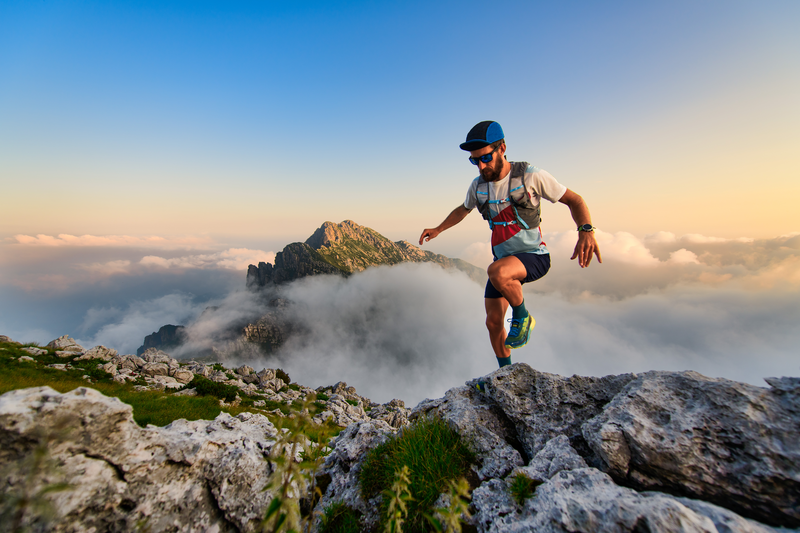
94% of researchers rate our articles as excellent or good
Learn more about the work of our research integrity team to safeguard the quality of each article we publish.
Find out more
REVIEW article
Front. Ecol. Evol. , 23 September 2022
Sec. Ecophysiology
Volume 10 - 2022 | https://doi.org/10.3389/fevo.2022.983172
This article is part of the Research Topic Ecological and Evolutionary Relevance of Phenotypic Plasticity in a Changing World View all 7 articles
Although many species shift their phenology with climate change, species vary significantly in the direction and magnitude of these responses (i.e., phenological sensitivity). Studies increasingly detect early phenology or high phenological sensitivity to climate in non-native species, which may favor non-native species over natives in warming climates. Yet relatively few studies explicitly compare phenological responses to climate between native vs. non-native species or between non-native populations in the native vs. introduced range, limiting our ability to quantify the role of phenology in invasion success. Here, we review the empirical evidence for and against differences in phenology and phenological sensitivity to climate in both native vs. non-native species and native and introduced populations of non-native species. Contrary to common assumptions, native and non-native plant species did not consistently differ in mean phenology or phenological sensitivity. However, non-native plant species were often either just as or more sensitive, but rarely less sensitive, to climate as natives. Introduced populations of non-native plant species often show earlier reproduction than native populations of the same species, but there was mixed evidence for differences in phenological sensitivity between introduced and native plant populations. We found very few studies comparing native vs. invasive animal phenology. Future work should characterize phenological sensitivity to climate in native vs. non-native plant and animal species, in native vs. introduced populations of non-native species, and across different stages of invasion, and should carefully consider how differences in phenology might promote invasion success or disadvantage native species under climate change.
The ability to colonize and persist across a broad range of environmental conditions is often considered a hallmark characteristic of non-native species (Metasanz et al., 2012). As biological invasions continue to increase worldwide (Seebens et al., 2017), many studies have investigated potential traits underlying the success of non-native species (i.e., “invasiveness”; see Glossary for bolded terms) and improve management (Levine et al., 2003; van Kleunen et al., 2010). For example, relative to native species, non-native species often demonstrate faster growth rates, greater biomass, and higher reproduction (Dlugosch and Parker, 2008; van Kleunen et al., 2010; Leishman et al., 2014; Jelbert et al., 2015), increased resource acquisition (Funk, 2013), and fewer interactions with local antagonists (sensu “enemy release hypothesis”; Elton, 1958; Maron and Vilà, 2001; Engelkes et al., 2008). Understanding the traits underlying successful introduction and spread could help predict future invasions (Richardson and Pyšek, 2006).
The timing of life-history events (i.e., phenological traits) may play an important role in invasiveness by allowing non-native species to respond appropriately to novel environmental conditions in their introduced ranges. In particular, phenology may be tied to resource access (Both et al., 2006; Wainwright et al., 2012; Gioria and Osborne, 2014; Gioria and Pyšek, 2017), performance (e.g., survival and reproduction) and population growth rates (Iler et al., 2021), and abundance (Willis et al., 2008; Cleland et al., 2012), making phenology an important trait to consider as a correlate for invasiveness. Here, we review the literature to evaluate support for four non-mutually exclusive hypotheses for how native and non-native species may differ in phenological traits in ways that might benefit non-native species (Wolkovich and Cleland, 2011; Figure 1).
Figure 1. Conceptual diagram illustrating the four hypotheses proposed by Wolkovich and Cleland (2011; shown in italics) to explain how phenology may shape invasion. Each line represents the phenology of a species across a growing season. In (A), non-native species (purple) fill a vacant niche in time, either earlier (also known as priority effects) or later than native species (green). In (B), non-native species have a longer duration of phenology than native species, filling a broader temporal niche than natives. Here, Q1 addresses whether non-native species demonstrate earlier or later phenology (vacant niche/priority effects) or shorter or longer phenological duration (niche breadth) relative to native species. In (C), native species’ (left) phenology occurs at a similar time under both ambient (blue) and climate change (red) conditions, whereas non-native species (right) can shift their phenology earlier in the season (i.e., are more phenologically sensitive to climate). Here, Q2 addresses whether non-native species demonstrate greater or less sensitivity to climate than native species.
The first three hypotheses relate to differences in mean phenology between non-native and native species. First, non-native species may invade vacant niches (Elton, 1958) in time currently unfilled by resident native species (Figure 1A), allowing them to utilize temporarily available resources like nutrients, light, space, pollinators, or food. Here, non-native species might demonstrate either early or late phenology relative to native species. Second, non-native species may benefit from priority effects (Sale, 1977), wherein they demonstrate earlier phenology than resident native species (Figure 1A; a subset of the vacant niche hypothesis), allowing them to access and exploit resources earlier than native species. Third, non-native species may occupy a broader niche by having longer phenological phases than resident native species (e.g., longer flowering or reproductive period, growth, or foraging; Figure 1B; Gerlach and Rice, 2003; Lake and Leishman, 2004; Cadotte et al., 2006; Fridley, 2012; Wolkovich et al., 2013), potentially allowing them extended access to resources.
In addition to hypotheses regarding mean phenology, non-native species may also demonstrate greater phenological sensitivity to environmental conditions (sensu “phenological plasticity hypothesis” in Wolkovich and Cleland, 2011) than native species (Baker, 1965; Figure 1C). Although Wolkovich and Cleland (2011) originally posed this hypothesis as a form of phenotypic plasticity, we use the broader term “phenological sensitivity” because, while many studies address phenological responses to environmental variation, relatively few distinguish between plastic and evolutionary responses to environmental change. Assuming that species shift their phenology appropriately in response to environmental conditions, then greater phenological sensitivity may confer a fitness or abundance advantage (Willis et al., 2008, 2010; Munguía-Rosas et al., 2011; Cleland et al., 2012; Iler et al., 2021; Zettlemoyer et al., 2021), particularly for non-native species experiencing novel environmental conditions and under the warmer and more heterogeneous environments predicted under climate change (Nicotra et al., 2010; Intergovernmental Panel on Climate Change [IPCC], 2022). Consistent with this prediction, non-native plants often demonstrate greater phenological sensitivity to warming than their native counterparts (Willis et al., 2010; Hulme, 2011; Wolkovich et al., 2013; Du et al., 2017; Giejsztowt et al., 2019; Zettlemoyer et al., 2019; Stuble et al., 2021), although the evidence for this trend is mixed, with other studies detecting similar sensitivity between groups (Bertin et al., 2017; Welshofer et al., 2018). More generally, phenotypic plasticity is a common mechanism proposed to facilitate invasion success (Richards et al., 2006). Yet previous meta-analyses comparing phenotypic plasticity between non-native and native species have proved inconclusive, with studies detecting either greater plasticity in non-natives (Davidson et al., 2011) or no differences in plasticity between natives and non-natives (Palacio-López and Gianoli, 2011). However, to our knowledge, no meta-analyses explicitly address differences in phenological sensitivity to environmental conditions between native and non-native species.
In addition to comparisons between native and non-native species in a particular region, a related literature has investigated differences between populations of non-native species occurring in their native vs. introduced range. This is because non-native species establishing in a novel range typically experience distinct environmental conditions from those historically experienced in the native range, and thus invasion success depends on the capacity of populations in the non-native range to adjust via rapid evolution, phenotypic plasticity, or a combination of both (Godoy et al., 2009). Several approaches have been used to test whether non-native species are more responsive to environmental conditions in their introduced relative to native ranges, including reciprocal transplant experiments (i.e., native and introduced populations transplanted into both the native and introduced range; Lamarque et al., 2015), common gardens that expose native and introduced populations to a range of environmental conditions (i.e., ambient vs. climate manipulation treatments; Peng et al., 2019), or common gardens in multiple locations (Williams et al., 2008). These experimental designs can contrast both the mean traits and environmental sensitivity of native vs. introduced populations of non-native species.
Although the four hypotheses outlined above were originally conceived with respect to phenological differences between native vs. non-native species, we might also expect similar differences between native vs. introduced populations of non-native species. For example, introduced populations could evolve to occupy distinct niches in time (vacant niche), earlier phenology (priority effects), or longer phenological periods (niche breadth) relative to populations in their native range. Similarly, we might expect greater phenological sensitivity to environmental conditions in introduced populations relative to native populations. Alternatively, introduced populations could be well-suited for success in the introduced range due to similar conditions in their native range, resulting in similar mean phenology and similar phenological sensitivity between native and introduced populations (Fridley and Sax, 2014). Previous work has detected greater phenotypic plasticity in leaf traits, growth, and reproductive output in non-native species in their introduced relative to native ranges (Bossdorf et al., 2005; Lavergne and Molofsky, 2007; Maron et al., 2007; Caño et al., 2008; Andonian and Hierro, 2011; Chun, 2011; Griffith et al., 2014). However, few studies have compared mean phenology or phenological sensitivity between native and introduced populations (Peng et al., 2019), despite the pervasive question of whether differences in phenology or greater phenological sensitivity contributes to non-native species’ success as invaders (Luna et al., 2017; Martinez and Fridley, 2018).
Here we survey the literature for studies that quantify phenological traits in response to changing climatic conditions in (A) native vs. non-native species within a region and (B) native vs. introduced populations of the same non-native species. We focus on climate, rather than other environmental contrasts, because climate variables are predominately implicated as drivers of phenology (Parmesan and Yohe, 2003; Thackeray et al., 2016; Cohen et al., 2018; Piao et al., 2019), non-native species often experience novel climate conditions in the introduced range (Richardson, 2011), and climate responses are highly relevant for predicting how ongoing climate change will impact species invasions (Bellard et al., 2017; Wallingford et al., 2020). Finally, we propose future directions for quantifying differences in phenological sensitivity and testing potential benefits to non-native relative to native species. Given the ongoing threat to biodiversity posed by biological invasions (Seebens et al., 2017), insights from this review will help determine whether future risks posed by invasive species are predictable from estimates of their phenological sensitivity to climate change.
We searched ISI Web of Science on 18 March, 2022, for studies that compared the phenology of (A) native vs. non-native species or (B) native vs. introduced populations of a given species in response to climate [see Supporting Information Appendix 1 for search methods; data are provided in Zettlemoyer (2022) and Appendix 2]. Specifically, we searched for empirical studies that met the following criteria: (1) measured at least one phenological trait; (2) measured phenology in ambient/historical vs. climate change conditions (including experimental and observational approaches); and (3) included at least (A) one native and one non-native species or (B) one native and one introduced population of the same species. Our requirement for a climate comparison potentially excluded studies examining differences in mean phenology in a single environment, meaning that our results for the vacant niche, priority effects, and niche breadth hypotheses are likely based on only a subset of the available research. For each study, we recorded reported results for each phenological trait (hereafter “phenophase”) in response to each climate variable (hereafter “cases”; see Appendix 1). For each case, we also collected information on study location (latitude and longitude), habitat (forest, grassland, or mixed), biome (alpine, temperate, subtropical, or tropical), and experimental design (historical records, contemporary observational field study, field manipulation, reciprocal transplant, common garden, or controlled climate conditions).
For (A), this yielded 38 studies, including data for a total of 116 cases (n = 109 cases for plants, n = 7 for animals). We found surprisingly few studies examining differences in phenology between native vs. non-native animal species. Although animal studies often measure phenology differently than plant studies (e.g., thermal requirements; Jarošik et al., 2015), we included these studies in our qualitative review (see below) when possible. However, due to the unbalanced nature of the data, the patterns we describe apply only to plants. We grouped phenophases into four categories: emergence (7 cases in plants only), growth and development (32 cases across plants and animals), reproduction (64 cases across plants and animals), and senescence/autumn phenology (13 cases in plants only) (Supplementary Table 1.1). Across both taxa, in 48 cases, phenology was examined observationally, in 22 cases phenology was assessed using historical or herbarium records, in 19 cases phenology was examined under a field manipulation, in 12 cases phenology was examined in a common garden or reciprocal transplant experiment, and in five cases phenology was examined under controlled climate conditions (e.g., greenhouse or growth chamber). 90 cases examined responses to warming [73 used temperature (°C) and 17 used growing degree days (GDD; a measure of heat accumulation; Gordon and Bootsma, 1993; Schwartz, 2003)] and 26 cases examined responses to precipitation or soil moisture. We pool results across all three climate variables in the main text, but provide results split by climate variable in Appendix 3 Supplementary Figure 3.1. Studies were mostly conducted in the United States, Europe, and China (Supplementary Figure 3.2).
For (B), our search yielded 18 studies, including data for a total of 69 cases (emergence = 6 cases; growth and development = 13 cases; reproduction = 49 cases; senescence/autumn phenology = 1 case; n = 62 cases for plants and seven cases for animals). Phenology was examined observationally in 28 cases, using historical data in seven cases, under controlled conditions in 10 cases, under a field manipulation in seven cases, and in a common garden or reciprocal transplant in 17 cases. 63 cases examined responses to warming (57 used °C and 6 used GDD) and six cases examined responses to precipitation or soil moisture.
We first performed a qualitative literature review to summarize the evidence for differences in mean phenology (hereafter Q1) and phenological sensitivity to climate (hereafter Q2) between (A) native and non-native species and (B) native and introduced populations of the same species, based on the four hypotheses developed by Wolkovich and Cleland (2011).
We first asked whether native vs. non-native species and native vs. introduced populations differ in their mean phenology. We scored whether phenology significantly differed (a = 0.05) between groups (yes/no; Q1a). If there was a significant difference, we then scored whether non-native species/introduced populations demonstrated earlier or later phenology (vacant niche/priority effects hypotheses) or shorter or longer phenological duration (niche breadth hypothesis; Q1b).
We then asked whether native vs. non-native species and native vs. introduced populations differ in their phenological sensitivity to climate. We scored whether studies detected a significant difference in phenological sensitivity to climate between groups (yes/no; Q2a). If there was a significant difference, we then scored whether non-native species/introduced populations demonstrate greater or less sensitivity to climate as native species/native populations (Q2b). Finally, we recorded which direction phenology shifts in response to climate in native species/native populations (Q2c) and non-native species/introduced populations (Q2d).
Our qualitative literature review allowed us to combine evidence across a wide range of studies that differed in their methodology and reporting. This approach provided mixed support for greater phenological sensitivity to climate in non-native species (see Results), so we also conducted a quantitative meta-analysis, using a subset of amenable studies, to more fully assess whether (A) native vs. non-native species or (B) native vs. introduced populations differ in phenological sensitivity to climate. We reassessed each of studies above, and retained 15 (for A) or 5 (for B) that met these additional criteria: (1) reported phenological responses of individual species (i.e., we excluded community-level responses and studies that only presented estimates for native vs. non-native species overall); (2) reported phenology as day of year (i.e., we excluded studies that used abundance, growth, thermal requirements, or count data as a proxy for phenology); and (3) reported the mean and associated error (e.g., standard deviation, standard error, or 95% confidence intervals) for a given phenophase in ambient vs. climate change conditions (i.e., factorial studies) or reported the slope and associated error of a phenological response to climate (i.e., continuous studies).
Many excluded studies reported means that aggregated responses across native and non-native species or did not report error. We contacted authors in these cases to request either species-specific results or raw data; for the latter, we reconstructed statistical models as similarly as possible to the published study to extract required summary statistics. We were able to compile (A) 824 cases (n = 526 natives and 298 non-natives) and (B) 58 cases (n = 29 natives and 29 introduced) for the meta-analyses. All data for these analyses are for plant species. For each case, we recorded the sample size, mean response value in the ambient and climate change condition (or slope in response to climate), and standard deviation. If data were only presented in figures, we used WebPlotDigitizer (1 Rohatgi, 2018) to extract relevant statistics.
For factorial studies, which report relative effect sizes, we calculated the effect of climate on phenological responses using Hedges’ g effect size, which standardizes the mean differences between groups after accounting for discrepancies in group sizes (Hedges, 1981; Rosenthal et al., 1994; Møller and Jennions, 2002). A negative Hedges’ g value indicates that a phenological event advanced under a climate change treatment relative to ambient conditions, and a larger absolute value of Hedges’ g indicates a larger change (in units of standard deviation). We used the “metacont” command in the “meta” package (v4.17-0; Balduzzi et al., 2019) in R v.4.0.4 (R Core Team, 2021) to estimate pairwise differences in Hedges’ g effect size between (A) native vs. non-native plant species or (B) native vs. introduced plant populations based on the moderator statistic (Q) and associated p-value. For (A), we conducted one analysis for all phenological responses to climate due to low sample sizes when climate variables were split [total: n = 476 cases across 10 studies; temperature: n = 252 cases across eight studies; GDD: n = 218 cases in one study (Mulder and Spellman, 2019); precipitation: n = 6 cases in 1 study (Wilsey et al., 2011)]. For (B), all but one study used a factorial design to examine phenological responses to warming (°C) so we limited our analysis to these studies. We then tested whether Hedges’ g varied with study characteristics by fitting linear mixed models. For (A), we included nativity (native vs. non-native), climate variable (°C, GDD, or precipitation), experiment type (field manipulation, controlled experiment, or reciprocal transplant), habitat (grassland or forest), biome (alpine, temperate, or subtropical), climate difference (average difference between ambient vs. climate conditions), and latitude and longitude as predictor variables. We included the interaction of climate variable × climate difference to account for different units among climate variables and variation among studies in the magnitude of climate treatments. We initially included the interaction of nativity × climate variable; this was not significant (χ21,476 = 0.07, p = 0.80) and dropped from the model. For (B), we included range (native vs. introduced), climate difference, experiment type (field manipulation or experimental transplant), latitude, and longitude as fixed effects. For all models, we included study label and species name (nested in study label; following Stuble et al., 2021) as random factors to control for species-specific phenological responses and variation among studies, respectively.
We tested for publication bias in our factorial datasets by comparing the Pearson’s correlation between absolute effect size and sample size for each case (“metabias” command) and examining the associated funnel plots (Supplementary Figures 3.3A,B). We detected no significant bias in either dataset (native vs. non-native: bias = 0.23 ± 0.27; native vs. introduced: bias = −6.84 ± 7.98; both p = 0.40). However, we note that the small dataset for (B) contained studies reporting larger negative standardized mean differences (Supplementary Figure 3.3B).
For continuous studies, which report absolute effect sizes (i.e., reported regression coefficients), we conducted a mixed-effect meta-regression analysis (Rao et al., 2017). For (A), we again conducted one analysis combining the three climate variables due to low sample sizes when split [total: n = 348 across five studies; temperature: n = 257 cases across five studies; GDD: n = 29 cases in one study (Maynard-Bean et al., 2020); precipitation: n = 62 cases across two studies (Maynard-Bean et al., 2020; Reeb et al., 2020)]. We included nativity (native vs. non-native), climate variable (°C, GDD, or precipitation), experiment type (observational vs. historical), latitude, and longitude as fixed effects. We excluded habitat because it was collinear with longitude and excluded biome because all continuous studies occurred in temperate sites. We again included species name and study label as random effects. We weighted the variance by the inverse of the reported standard deviation for each estimate (Lee et al., 2016). Only one study compared phenological sensitivity of native vs. introduced populations in a continuous framework (Hulme and Barrett, 2013), so we did not perform a meta-regression analysis for (B).
We used our qualitative review to address whether native plant species/populations and non-native species/introduced plant populations differ in (Q1) their mean phenology (vacant niche, priority effects, and niche breadth hypotheses) or (Q2) their phenological sensitivity to climate. Below, we briefly describe each question and then evaluate the fraction of cases that support, do not support, or yield mixed evidence for each hypothesis. Our qualitative review detected a lack of studies comparing native vs. non-native animal species’ phenology (Figure 2). Therefore, the patterns described below can be ascribed only to plant species/populations.
Figure 2. Percent of cases supporting or contradicting each of the questions outlined for all phenophases, emergence, growth and development, reproduction, and senescence (left to right), split by taxa [light blue = animals (7 cases total; 5 insects, 1 bird, and 1 tunicate); green = plants (n = 109 cases)]. Values are the number of different responses for each question out of the total number of cases that addressed that question. (Q1a) We examine whether native and non-native species differ in their mean phenology. “Yes” indicates that studies detected a significant difference in native vs. non-native species’ phenology (α = 0.05). (Q1b) Of the studies that detect a significant difference in mean phenology, we ask whether non-native species demonstrate earlier or later phenology (for phenology measured as day of year of an event) or shorter or longer phenological duration (for phenology measured as length of event or time between events) relative to native species. (Q2a) We examine whether native and non-native species differ in their phenological sensitivity to climate. “Yes” indicates that a study detected a significant difference in the response of native vs. non-native species to a climate change manipulation or a significant difference in the slopes of native vs. non-native species to a change in climate. (Q2b) Of the studies that detect a significant difference in sensitivity, we examine whether non-native species demonstrate greater or less sensitivity to climate as native species. Greater sensitivity indicates a greater shift in phenology in response to climate (i.e., greater effect size or steeper slope). Lastly, we report the direction in which phenology shifted in response to climate change scenarios (warmer and/or drier conditions) in (Q2c) native and (Q2d) non-native species. Not every study reported the direction of responses, so the denominator values vary for Q2c-d.
We found weak support for differences in mean phenology, with 40/71 (56%) and 40/61 (66%) cases detecting a significant difference between native vs. non-native plant species and native vs. introduced plant populations, respectively (Figures 2, 3: Q1a). This pattern held across phenophases, except for native vs. non-native plant species’ senescence: 9/9 cases detected a significant difference in senescence timing.
When examining the cases that detected significant differences in phenology, we found mixed evidence for priority effects. Of the cases that detected significant differences in phenology between non-native vs. native plant species, 22/40 (55%) cases detected earlier phenology in non-natives, while 17/40 (43%) detected later phenology (Figure 2: Q1b). Again, this pattern held across emergence, growth/development, and reproduction, whereas 8/9 cases detected later senescence of non-native species. In contrast, we found some evidence for earlier phenology in introduced relative to native populations of a non-native plant species (Figure 3: Q1b). Of the 40 cases that detected differences in phenology, 27 (68%) detected earlier phenology whereas 12 (30%) detected later phenology. This pattern was strongest for reproduction [22/31 (71%) cases earlier vs. 9/31 (29%) later].
Figure 3. Percent of cases supporting or contradicting each of the questions outlined in the Section “Materials and methods” for all phenophases, emergence, growth and development, reproduction, and senescence (left to right), split by taxa [light blue = animals (7 cases total; 6 insects, 1 bird); green = plants (n = 62 cases)]. Values are the number of different responses for each question out of the total number of cases that addressed that question. (Q1a) We examine whether non-native populations in their native vs. introduced range differ in their mean phenology, where “yes” indicates a significant difference (α = 0.05). (Q1b) Of the studies that detect a significant difference in mean phenology, we ask whether introduced populations demonstrate earlier or later phenology (for phenology measured as day of year of an event) or shorter or longer phenological duration (for phenology measured as length of event or time between events) relative to native populations. (Q2a) We examine whether non-native populations in their native vs. introduced range differ in their phenological sensitivity to climate. “Yes” indicates that a study detected a significant difference in the response of native vs. introduced populations to climate conditions. (Q2b) Of the studies that detect a significant difference in sensitivity, we examine whether introduced populations of non-native species demonstrate greater or less sensitivity to climate as populations in the native range. Greater sensitivity indicates a greater shift in phenology in response to climate. Lastly, we report the direction in which phenology shifted in response to climate change scenarios (warmer and/or drier conditions) in (Q2c) native and (Q2d) introduced populations. Not every study reported the direction of responses, so the denominator values vary for Q2c-d.
Few studies explicitly addressed differences in phenological duration (shorter/longer; Figures 2, 3 Q1b). In 2/3 cases comparing native and non-native plant species, duration was equivalent (Mulder and Spellman, 2019; Zettlemoyer et al., 2019). In one case, introduced species had a longer leaf lifespan than natives (Mulder and Spellman, 2019). One case detected shorter leaf lifespan in native related to introduced populations (Peng et al., 2019), as expected under the niche breadth hypothesis.
We detected little evidence that native vs. non-native plant species or native vs. introduced plant populations differ in phenological sensitivity overall, with 48/109 (44%) and 22/62 (35%) cases detecting significant differences, respectively (Figures 2, 3: Q2a). However, of the 48 studies that did detect differences in sensitivity, non-native plant species were frequently more sensitive to climate than native plant species [42/48 (88%) cases; Figure 2: Q2b]. This pattern held for growth/development, reproduction, and senescence. Similarly, of the 22 cases that detected differences in sensitivity between introduced vs. native populations of a non-native plant species, 13 (59%) found greater sensitivity in introduced populations (Figure 3: Q2b).
The majority of studies detected advancing emergence, growth/development, and reproduction in response to warmer and drier conditions (and no shifts in senescence) in both native and non-native plant species (Figure 2: Q2c-d). In contrast, native and introduced populations of non-native species demonstrated more variability in the direction of phenological shifts in response to climate (Figure 3: Q2c,d).
We used a subset of suitable studies to perform a quantitative meta-analysis to further explore support for the phenological sensitivity hypothesis. These analyses revealed no differences in phenological sensitivity for either (A) native vs. non-native plant species (Hedges’ g: Q = 1.09, p = 0.3; χ21,476 = 0.28, p = 0.60; regression coefficients: χ21,348 = 0.11, p = 0.74; Figure 4A and Supplementary Table 3.1) or (B) native vs. introduced plant populations (Q = 0.11, p = 0.74; χ21,20 = 0.54, p = 0.46; Figure 4B and Supplementary Table 3.2), although we note that the sample size for (B) was extremely small (n = 4 studies).
Figure 4. Phenological sensitivity to climate does not differ between (A) non-native vs. native plant species or (B) introduced vs. native populations of non-native plant species. Native species/populations are shown as green circles; non-native species/introduced populations are shown as purple triangles. Values are (left) mean effect sizes (Hedges’ g) or (right) regression coefficients and 95% confidence intervals estimated from mixed-effect meta-analysis models. Negative values indicate that the timing of a phenophase was earlier under climate change (warmer and/or drier) conditions relative to ambient conditions (the dashed line indicates zero, or no effect).
We detected variation in effect sizes based on study characteristics for dataset A (Supplementary Table 3.1). For factorial studies, effect sizes were larger at higher (mainly northern) latitudes (χ21,476 = 21.20, p < 0.0001; only two studies occurred in the southern hemisphere), when using GDD as a climate variable (χ21,476 = 66.09, p < 0.0001), in studies using reciprocal transplants (χ21,476 = 58.47, p < 0.0001), and in alpine biomes (χ21,476 = 18.26, p = 0.0001) (Supplementary Figure 3.4). In continuous studies, effect sizes were larger using temperature (°C) as a climate variable (χ21,348 = 62.7, p < 0.0001) and in studies using historical records (χ21,348 = 17.69, p < 0.0001) (Supplementary Figure 3.5). We did not detect significant variation in dataset B, likely due to small sample size.
Despite the common assumption that non-native species benefit from differences in phenology and an increased ability to track changing climates relative to native species, we find little evidence that phenological traits differ systematically between non-native vs. native plant species or between populations of non-native plant species in their introduced vs. native range. Instead, our literature review emphasizes the wide heterogeneity among studies and phenophases, as well as the limited number of studies currently available to answer some of these questions. For example, there were almost twice as many native species represented in our meta-analysis as non-natives, highlighting a need for more studies that explicitly characterize differences in phenological traits and sensitivity to climate in native vs. non-native species. Additionally, comparative animal studies were woefully underrepresented (n = 7 cases), highlighting a need to examine differences in phenology between native vs. non-native animals before generalizing patterns of non-native species’ phenology across taxa. Below, we discuss the limited support for each of the hypotheses proposed by Wolkovich and Cleland (2011) and outline future directions for investigating differences in phenological sensitivity between native and non-native species (and native vs. introduced populations of non-native species) and whether such differences could increase invasion success.
Contrary to the hypothesis that non-native species (and introduced populations thereof) would demonstrate differences in mean phenology by filling a vacant or broader temporal niche relative to native species/populations, phenological traits were equivalent in a substantial fraction of comparisons. There were two exceptions to this pattern. First, non-native plant species almost always demonstrated later senescence than native plant species (although sample size was small; n = 9 cases). Most of these measured leaf senescence (6/9 cases; the other three cases were for flower/fruit senescence). Extended leaf senescence could indicate a lengthening growing season, and has been identified as a trait associated with invasiveness in forest systems (Fridley, 2012; Martinez and Fridley, 2018). Although few studies in our review explicitly measured phenological duration, if non-native plant species show equivalent phenology for earlier phenophases but later senescence, they may in fact occupy broader temporal niches. Future studies should quantify phenology across multiple phenophases as well as the duration of a given phenophase to provide a more detailed view of the temporal niche space occupied by non-native species. Second, introduced plant populations were more likely to have earlier reproduction than populations in their native range. This suggests that introduced populations may benefit from reproductive priority effects in their non-native ranges, gaining earlier access to resources important for reproduction (e.g., pollinators, breeding habitat, food sources, nutrients, and light) and potentially a competitive advantage (Wolkovich and Cleland, 2011; Wainwright et al., 2012). Future studies should investigate whether earlier reproduction in introduced plant populations benefits their establishment in novel environments.
Both our qualitative review and subsequent meta-analyses indicate similar levels of phenological sensitivity to environmental conditions expected under climate change for both non-native vs. native plant species and introduced vs. native populations of non-native plant species. There are several potential reasons for this apparent similarity in phenological sensitivity. First, we focused our review on sensitivity to climate variables such as temperature or precipitation, but non-native plant species may be more responsive to other environmental conditions like light or nutrient levels instead (Fridley, 2012; Peng et al., 2019). Second, non-native plants may colonize a wide range of environments via adaptive differentiation rather than by tracking environmental variation through greater phenological sensitivity (Dlugosch and Parker, 2008). In this case, non-native plant species could spread via the persistence of various locally-adapted ecotypes (Mooney and Cleland, 2001; Lavergne and Molofsky, 2007). Third, many of the non-native plant species examined here may have been present in the invaded community for some time. If phenological sensitivity benefits earlier stages of invasion, when species’ survival depends on tolerating a novel environment in the introduced range, we may be less likely to detect it in established plant populations well-past the initial stages of invasion (Palacio-López and Gianoli, 2011). After these initial stages, any novel phenotypes resulting from environmental sensitivity might become fixed under directional selection in the novel habitat (Sexton et al., 2002), and the magnitude of environmental sensitivity may be reduced if it is costly (Pigliucci et al., 2006). Future work might focus on identifying traits associated with newly-invading species and correlate the environmental sensitivity of those traits with measures of invasion success, such as rate of spread, abundance, fitness, or population growth rates. Alternatively, studies could correlate phenological sensitivity with time since invasion: if non-native species are more likely to track environmental conditions early in invasion, then more recently introduced species should exhibit higher sensitivity. Finally, studies might consider whether phenological sensitivity differs between widespread, damaging invasive species vs. exotic species that are naturalized in the community (Schultheis et al., 2015; Zettlemoyer et al., 2019) or between archaeophytes vs. neophytes (Hulme, 2011; Essl et al., 2021). Overall, our results are consistent with a previous meta-analysis detecting no differences in phenotypic plasticity in root, shoot, and leaf traits between native and non-native species (Palacio-López and Gianoli, 2011).
Despite little evidence for consistent differences in phenological sensitivity, when such differences were detected sensitivity tended to be greater in non-native plant species and introduced plant populations. Specifically, our qualitative review demonstrated that most studies detect either greater or equivalent phenological sensitivity, and almost never detect reduced sensitivity, in non-native relative to native plant species. This finding presents limited evidence for the phenological sensitivity hypothesis, by suggesting that phenological sensitivity may facilitate, though not be required, for establishment of non-native plant species. In this case, climate change may favor some non-natives with higher phenological sensitivity, allowing them to rapidly respond to climatic shifts or increased climate variability. However, it remains unclear whether phenological sensitivity confers a fitness advantage. Although previous work has demonstrated that greater phenological sensitivity to temperature often correlates with increased performance or abundance (Willis et al., 2008, 2010; Cleland et al., 2012; Wolkovich et al., 2013; Iler et al., 2021), we lack studies quantifying the relationship between degree of phenological sensitivity and changes in fitness in both non-native and native species. Such data would help define the relative importance of phenological sensitivity in facilitating the establishment and spread of non-native species. For instance, phenological shifts in response to experimental warming in non-native Calluna vulgaris decreased reproductive success in a co-flowering native species, Dracophyllum subulatum (Giejsztowt et al., 2019). Ultimately, we need to link differences in phenological sensitivity between native and non-native species to demographic differences that might favor non-native species under warming. This comparative approach will allow us to understand which native species might be more at risk of population declines due to an inability to track environmental conditions via phenological shifts.
Despite a well-developed theoretical framework for how phenology may facilitate establishment and spread of non-native species (Wolkovich and Cleland, 2011), our review shows that the empirical evidence remains surprisingly mixed for plants and quite sparse for animals. We reiterate the plea for studies to regularly report the underlying means, standard errors, and sample sizes for individual taxa and experimental treatments needed to conduct synthetic meta-analyses (Gurevitch et al., 2018; Kambach et al., 2020). Most studies in our review compared reproductive phenology of plants in response to warming, and studies addressing emergence or senescence, responses to precipitation, animals, or tropical systems were particularly rare. Future work should prioritize including measures of phenological duration to better evaluate the niche breadth hypothesis and explicitly linking phenology to aspects of fitness, competitive ability, or invasiveness.
MZ conceived the study and led data collection, performed statistical analyses, and wrote the manuscript. All authors contributed to data collection and revisions.
The DeMarche lab is supported by NSF DEB 1753980.
We thank all the study authors who provided meta-analysis data, summary statistics or raw data, and two reviewers for valuable feedback on this manuscript.
The authors declare that the research was conducted in the absence of any commercial or financial relationships that could be construed as a potential conflict of interest.
All claims expressed in this article are solely those of the authors and do not necessarily represent those of their affiliated organizations, or those of the publisher, the editors and the reviewers. Any product that may be evaluated in this article, or claim that may be made by its manufacturer, is not guaranteed or endorsed by the publisher.
The Supplementary Material for this article can be found online at: https://www.frontiersin.org/articles/10.3389/fevo.2022.983172/full#supplementary-material
Archaeophyte: Non-native species introduced long ago (often before 1492 AD).
Broader niche/niche breadth: When a species can use a larger diversity of resources or habitats in space or time.
Exotic: Introduced species that assimilate into the native community with little effect.
Invasive: Introduced species that aggressively colonize natural communities with widespread, damaging effects.
Invasiveness: The ability of an introduced species to establish and increase their abundance or spread within the invaded community.
Neophyte: Non-native species introduced more recently (often after 1492 AD).
Phenological sensitivity: The direction and magnitude by which phenology shifts in response to changing environmental conditions (often measured as change in timing per unit of environmental change).
Phenotypic plasticity: The ability of an organism to adjust its phenotype based on the environment it experiences (note that this definition does not include genetic × environment interactions).
Priority effects: A subset of the vacant niche where a species can gain access to unused resources first.
Vacant niche: An “open space” in space or time where resources are available.
Andonian, K., and Hierro, J. L. (2011). Species interactions contribute to the success of a global plant invader. Biol. Invasions 13:2957. doi: 10.1007/s10530-011-9978-x
Baker, H. G. (1965). “Characteristics and modes of origin of weeds,” in The genetics of colonizing species, eds H. G. Baker and G. L. Stebbins (New York, NY: Academic Press), 147–169. doi: 10.1002/ps.3789
Balduzzi, S., Rücker, G., and Schwarzer, G. (2019). How to perform a meta-analysis with R: A practical tutorial. Evid. Based Ment. Health 22, 153–160. doi: 10.1136/ebmental-2019-300117
Bellard, C., Jeschke, J. M., Leroy, B., and Mace, G. M. (2017). Insights from modeling studies on how climate change affects invasive alien species geography. Ecol. Evol. 8, 5688–5700. doi: 10.1002/ece3.4098
Bertin, R. I., Searcy, K. B., Hickler, M. G., and Motzkin, G. (2017). Climate change and flowering phenology in Franklin County, Massachusetts. J. Torrey Bot. Soc. 144, 153–169. doi: 10.3159/TORREY-D-16-00019R2
Bossdorf, O., Auge, H., Lafuma, L., Rogers, W. E., Siemann, E., and Prati, D. (2005). Phenotypic and genetic differentiation between native and introduced plant populations. Oecologia 144, 1–11. doi: 10.1007/s00442-005-0070-z
Both, C., Bouwhuis, S., Lessels, C. M., and Visser, M. E. (2006). Climate change and population declines in a long-distance migratory bird. Nature 441, 81–83. doi: 10.1038/nature04539
Cadotte, M. W., Murray, B. R., and Lovett-Doust, J. (2006). Ecological patterns and biological invasions: Using regional species inventories in macroecology. Biol. Invasions 8, 809–821. doi: 10.1007/s10530-005-3839-4
Caño, L., Escarré, J., Fleck, I., Blano-Moreno, J. M., and Sans, F. X. (2008). Increased fitness and plasticity of an invasive species in its introduced range: A study using Senecio pterophorus. J. Ecol. 96, 468–476. doi: 10.1111/j.1365-2745.2008.01363.x
Chun, Y. J. (2011). Phenotypic plasticity of introduced versus native purple loosestrife: Univariate and multivariate reaction norm approaches. Biol. Invasions 13, 819–829. doi: 10.1007/s10530-010-9871-z
Cleland, E. E., Allen, J. M., Crimmins, T. M., Dunne, J. A., Pau, S., Travers, S. E., et al. (2012). Phenological tracking enables positive species responses to climate change. Ecology 93, 1765–1771. doi: 10.1890/11-1912.1
Cohen, J. M., Lajeunesse, M. J., and Rohr, J. R. (2018). A global synthesis of animal phenological responses to climate change. Nat. Clim. Change 8, 224–228. doi: 10.1038/s41558-018-0067-3
Davidson, A. M., Jennions, M., and Nicotra, A. B. (2011). Do invasive species show higher phenotypic plasticity than native species, and, if so, is it adaptive? A meta-analysis. Ecol. Lett. 14, 419–431. doi: 10.1111/j.1461-0248.2011.01596.x
Dlugosch, K. M., and Parker, I. M. (2008). Invading populations of an ornamental shrub show rapid life history evolution despite genetic bottlenecks. Ecol. Lett. 11, 701–709. doi: 10.1111/j.1461-0248.2008.01181.x
Du, Y., Chen, J., Willis, C. G., Zhou, Z., Liu, T., Dai, W., et al. (2017). Phylogenetic conservatism and trait correlates of spring phenological responses to climate change in northeast China. Ecol. Evol. 7, 6747–6757. doi: 10.1002/ece3.3207
Engelkes, T., Morriën, E., Verhoeven, K. J. F., Bezemer, T. M., Biere, A., Harvey, J. A., et al. (2008). Successful range-expanding plants experience less above-ground and below-ground enemy impact. Nature 456, 946–948. doi: 10.1038/nature07474
Essl, F., Galser, M., and Schertler, A. (2021). New and old invaders in forests in eastern Austria: The role of species attributes and invasion history. Flora 283:151922. doi: 10.1016/j.flora.2021.151922
Fridley, J. D. (2012). Extended leaf phenology and the autumn niche in deciduous forest invasions. Nature 485, 359–362. doi: 10.1038/nature11056
Fridley, J. D., and Sax, D. F. (2014). The imbalance of nature: Revisiting a Darwinian framework for invasion biology. Glob. Ecol. Biogeogr. 21, 1157–1166. doi: 10.1111/geb.12221
Funk, J. L. (2013). The physiology of invasive plants in low-resource environments. Conserv. Physiol. 1:cot026. doi: 10.1093/conphys/cot026
Gerlach, J. D., and Rice, K. J. (2003). Testing life history correlates of invasiveness using congeneric plant species. Ecol. Appl. 13, 167–179. doi: 10.1890/1051-07612003013[0167:TLHCOI]2.0.CO;2
Giejsztowt, J., Classen, A. T., and Deslippe, J. R. (2019). Climate change and invasion may synergistically affect native plant reproduction. Ecology 101:e02913. doi: 10.1002/ecy.2913
Gioria, M., and Osborne, B. A. (2014). Resource competition in plant invasions: Emerging patterns and research needs. Front. Plant Sci. 5:501. doi: 10.3389/fpls.2014.00501
Gioria, M., and Pyšek, P. (2017). Early bird catches the worm: Germination as a critical step in plant invasion. Biol. Invasions 19, 1055–1080. doi: 10.1007/s10530-016-1349-1
Godoy, I., Castro-Díez, P., Valladares, F., and Costa-Tenorio, M. (2009). Different flowering phenology of alien invasive species in Spain: Evidence for the use of an empty temporal niche? Plant Biol. 11, 803–811. doi: 10.1111/j.1438-8677.2008.00185.x
Gordon, R., and Bootsma, A. (1993). Analyses of growing degree-days for agriculture in Atlantic Canada. Clim. Res. 3, 169–176.
Griffith, A. B., Andonian, K., Weiss, C. P., and Loik, M. E. (2014). Variation in phenotypic plasticity for native and invasive populations of Bromus tectorum. Biol. Invasions 16, 2627–2638. doi: 10.1007/s10530-014-0692-3
Gurevitch, J., Koricheva, J., Nakagawa, S., and Stewart, G. (2018). Meta-analysis and the science of research synthesis. Nature 555, 175–182. doi: 10.1038/nature25753
Hedges, L. V. (1981). Distribution theory for Glass’s estimator of effect size and related estimators. J. Edu. Behav. Stat. 6, 107–128. doi: 10.3102/10769986006002107
Hulme, P. E. (2011). Contrasting impacts of climate-driven flowering phenology on changes in alien and native plant species distributions. New Phytol. 189, 272–281. doi: 10.1111/j.1469-8137.2010.03446.x
Hulme, P. E., and Barrett, S. C. H. (2013). Integrating trait- and niche-based approaches to assess contemporary evolution in alien plant species. J. Ecol. 101, 68–77. doi: 10.1111/1365-2745.12009
Iler, A. M., CaraDonna, P. L., Forrest, J. R. K., and Post, E. (2021). Demographic consequences of phenological shifts in response to climate change. Annu.Rev. Ecol. Evol. Syst. 52, 221–245. doi: 10.1145/annurev-ecolsys-011921-032939
Intergovernmental Panel on Climate Change [IPCC] (2022). “Climate change 2022: Impacts, adaptation, and vulnerability,” in Contribution of Working Group II to the Sixth Assessment Report of the Intergovernmental Panel on Climate Change, eds H.-O. Pörtner, D. C. Roberts, M. Tignor, E. S. Poloczanska, K. Mintenbeck, A. Alegría, et al. (Cambridge: Cambridge University Press).
Jarošik, V., Kenis, M., Honìk, A., Skuhrovec, J., and Pyšek, P. (2015). Invasive insects differ from non-invasive in their thermal requirements. PLoS One 10:e0131072. doi: 10.1371/journal.pone.0131072
Jelbert, K., Stott, I., McDonald, R. A., and Hodgson, D. (2015). Invasiveness of plants is predicted by size and fecundity in the native range. Ecol. Evol. 5, 1933–1943. doi: 10.1002/ece3.1432
Kambach, S., Bruelheide, H., Gerstner, K., Gurevitch, J., Beckmann, M., and Seppelt, R. (2020). Consequences of multiple imputation of missing standard deviations and sample sizes in meta-analysis. Ecol. Evol. 10, 11699–11712. doi: 10.1002/ece3.6806
Lake, J. C., and Leishman, M. R. (2004). Invasion success of exotic plants in natural ecosystems: The role of disturbance, plant attributes and freedom from herbivores. Biol. Conserv. 117, 215–226. doi: 10.1016/S0006-3207(03)00294-5
Lamarque, L. J., Lorie, C. J., Porté, A. J., and Delzon, S. (2015). Genetic differentiation and phenotypic plasticity in life-history traits between native and introduced populations of invasive maple trees. Biol. Invasions 17, 1109–1122. doi: 10.1007/s10530-014-0781-3
Lavergne, S., and Molofsky, J. (2007). Increased genetic variation and evolutionary potential drive the success of an invasive grass. Proc. Natl. Acad. Sci. U.S.A. 104, 3883–3888. doi: 10.1073/pnas.060732410
Lee, C. H., Cook, S., Lee, J. S., and Han, B. (2016). Comparison of two meta-analysis methods: Inverse-variance-weighted average and weighted sum of z-scores. Genomics Inform. 14, 173–180. doi: 10.5808/GI.2016.14.4.173
Leishman, M. R., Cooke, J., and Richardson, D. M. (2014). Evidence for shifts to faster growth strategies in the new ranges of invasive alien plants. J. Ecol. 102, 1451–1461. doi: 10.1111/1365-2745.12318
Levine, J. M., Vilà, M., D’Antonio, C. M., Dukes, J. S., Grigulis, K., and Lavorel, S. (2003). Mechanisms underlying the impacts of exotic plant invasions. Proc. R. Soc. Lond. B 270, 775–781. doi: 10.1098/rspb.2003.2327
Luna, A., Franz, D., Strubbe, D., Shwartz, A., Braun, M. P., Hernández-Brito, D., et al. (2017). Reproductive timing as a constraint on invasion success in the Ring-necked parakeet (Psittacula krameri). Biol. Invasions 19, 2247–2259. doi: 10.1007/s10530-017-1436-y
Maron, J. L., Elmendorf, S. C., and Vilà, M. (2007). Contrasting plant physiological adaptation to climate in the native and introduced range of Hypericum perforatum. Evolution 61, 1912–1924. doi: 10.1111/j.1558-5646.2007.00153.x
Maron, J. L., and Vilà, M. (2001). When do herbivores affect plant invasion? Evidence for the natural enemies and biotic resistance hypotheses. Oikos 95, 361–373. doi: 10.1034/j.1600-0706.2001.950301.x
Martinez, K. A., and Fridley, J. D. (2018). Acclimation of leaf traits in seasonal light environments: Are non-native species more plastic? J. Ecol. 106, 2019–2030. doi: 10.1111/1365-2745.12952
Maynard-Bean, E., Kaye, M., Wagner, T., and Burkhart, E. P. (2020). Citizen scientists record novel leaf phenology of invasive shrubs in eastern U.S. forests. Biol. Invasions 22, 3325–3337. doi: 10.1007/s10530-020-02326-1
Metasanz, S., Kobelski-Horgan, T., and Sultan, S. E. (2012). Phenotypic plasticity and population differentiation in an ongoing species invasion. PLoS One 7:e44955. doi: 10.1371/journal.pone.0044955
Møller, A., and Jennions, M. D. (2002). How much variance can be explained by ecologists and evolutionary biologists? Oecologia 132, 492–500. doi: 10.1007/s00442-002-0952-2
Mooney, H. A., and Cleland, E. E. (2001). The evolutionary impact of invasive species. Proc. Natl. Acad. Sci. U.S.A. 98, 5446–5451. doi: 10.1073/pnas.091093398
Mulder, C. P. H., and Spellman, K. V. (2019). Do longer growing seasons give introduced plants an advantage over native plants in Interior Alaska? Botany 97, 347–362. doi: 10.1139/cjb-2018-0209
Munguía-Rosas, M. A., Ollerton, J., Parra-Tabla, V., and De-Nova, J. A. (2011). Meta-analysis of phenotypic selection on flowering phenology suggests that early flowering plants are favoured. Ecol. Lett. 14, 511–521. doi: 10.1111/j.1461-0248.2011.01601.x
Nicotra, A. B., Atkin, O. K., Bonser, S. O., Davidson, A. M., Finnegan, E. J., Mathesius, U., et al. (2010). Plant phenotypic plasticity in a changing climate. Trends Plant Sci. 15, 684–692. doi: 10.1016/j.tplants.2010.09.008
Palacio-López, K., and Gianoli, E. (2011). Invasive plants do not display greater phenotypic plasticity than their native or non-invasive counterparts: A meta-analysis. Oikos 120, 1393–1401. doi: 10.1111/j.1600-0706.2010.19114.x
Parmesan, C., and Yohe, G. (2003). A globally coherent fingerprint of climate change impacts across natural systems. Nature 421, 37–42. doi: 10.1038/nature01286
Peng, Y., Yang, J.-X., Zhou, X.-H., Peng, P.-H., Li, J.-J., Zhang, S.-M., et al. (2019). An invasive population of Solidago canadensis is less sensitive to warming and nitrogen-addition than its native population in an invaded range. Biol. Invasions 21, 151–162. doi: 10.1007/s10530-018-1812-2
Piao, S., Liu, Q., Chen, A., Janssens, I. A., Fu, Y., Dai, J., et al. (2019). Plant phenology and global climate change: Current progresses and challenges. Glob. Chang. Biol. 25, 1922–1940. doi: 10.1111/gcb.14619
Pigliucci, M., Murren, C. J., and Schlichting, C. D. (2006). Phenotypic plasticity and evolution by genetic assimilation. J. Exp. Biol. 209, 2362–2367. doi: 10.1242/jeb.02070
R Core Team (2021). R: A language and environment for statistical computing. R Foundation for Statistical Computing, Vienna, Austria. Available online at: https://www.R-project.org/ (accessed January 1, 2021).
Rao, G., Lopez-Jimenez, F., Boyd, J., D’Amico, F., Durant, N. H., Hlatky, M. A., et al. (2017). Methodological standards for meta-analyses and qualitative systematic reviews of cardiac prevention and treatment studies: A scientific statement from the American Heart Association. Circulation 136, e172–e194. doi: 10.1161/CIR.0000000000000523
Reeb, R. A., Acevedo, I., Heberling, J. M., Isaac, B., and Kuebbing, S. E. (2020). Nonnative old-field species inhabit early season phenological niches and exhibit unique sensitivity to climate. Ecosphere 11:e03217. doi: 10.1002/ecs2.3217
Richards, C. L., Bossdorf, O., Muth, N. Z., Gurevitch, J., and Pigliucci, M. (2006). Jack of all trades, master of some? On the role of phenotypic plasticity in plant invasions. Ecol. Lett. 9, 981–993. doi: 10.1111/j.1461-0248.2006.00950.x
Richardson, D. M. (2011). Fifty years of invasion ecology: The legacy of Charles Elton. Chichester: Wiley Blackwell.
Richardson, D. M., and Pyšek, P. (2006). Plant invasions: Merging the concepts of species invasiveness and community invasibility. Prog. Phys. Geogr. 30, 409–431. doi: 10.1191/0309133306pp490pr
Rohatgi, A. (2018). WebPlotDigitizer. Available online at: https://automeris.io/WebPlotDigitizer/ (accessed March 1, 2022).
Rosenthal, R., Cooper, H., and Hedges, L. (1994). “Parametric measures of effect size,” in The handbook of research synthesis, Vol. 621, eds H. Cooper, L. V. Hedges, and J. C. Valentine (New York, NY: Russell Sage Foundation), 231–244.
Sale, P. F. (1977). Maintenance of high diversity in coral reef fish communities. Am. Nat. 111, 337–359. doi: 10.1086/283164
Schultheis, E. H., Berardi, A. E., and Lau, J. A. (2015). No release for the wicked: Enemy release is dynamic and not associated with invasiveness. Ecology 96, 2446–2457. doi: 10.1890/14-2158.1
Schwartz, M. D. (2003). Phenology: An integrative environmental science, tasks for vegetation science. New York, NY: Kluwer Academic Publishers.
Seebens, H., Blackburn, T. M., Dyer, E. E., Genovesi, P., Hulme, P. E., Jeschke, J. M., et al. (2017). No saturation in the accumulation of alien species worldwide. Nat. Commun. 8:14435. doi: 10.1038/ncomms14435
Sexton, J. P., McKay, J. K., and Sala, A. (2002). Plasticity and genetic diversity may allow saltcedar to invade cold climates in North America. Ecol. Appl. 12, 1652–1660. doi: 10.1890/1051-07612002012[1652:PAGDMA]2.0.CO;2
Stuble, K. L., Bennion, L. D., and Kuebbing, S. E. (2021). Plant phenological responses to experimental warming - a synthesis. Glob. Chang. Biol. 27, 4110–4124. doi: 10.1111/gcb.15685
Thackeray, S. J., Henrys, P. A., Hemming, D., Bell, J. R., Botham, M. C., Burthe, S., et al. (2016). Phenological sensitivity to climate across taxa and trophic levels. Nature 535, 241–245. doi: 10.1038/nature18608
van Kleunen, M., Weber, E., and Fischer, M. (2010). A meta-analysis of trait differences between invasive and non-invasive plant species. Ecol. Lett. 13, 235–245. doi: 10.1111/j.1461-0248.2009.01418.x
Wainwright, C. E., Wolkovich, E. M., and Cleland, E. E. (2012). Seasonal priority effects; implications for invasion and restoration in a semi-arid system. J. Appl. Ecol. 49, 234–241. doi: 10.1111/j.1365-2664.2011.02088.x
Wallingford, P. D., Morelli, T. L., Allen, J. M., Beaury, E. M., Blumenthal, D. M., Bradley, B. A., et al. (2020). Adjusting the lens of invasion biology to focus on the impacts of climate-driven range shifts. Nat. Clim. Change 10, 398–405. doi: 10.1038/s41558-020-0768-2
Welshofer, K. B., Zarnekske, P. L., Lany, N. K., and Read, Q. D. (2018). Short-term responses to warming vary between native vs. exotic species and with latitude in an early successional plant community. Oecologia 187, 333–342. doi: 10.1007/s00442-018-4111-9
Williams, J. L., Auge, H., and Maron, J. L. (2008). Different gardens, different results: Native and introduced populations exhibit contrasting phenotypes across common gardens. Oecologia 157, 239–248. doi: 10.1007/s00442-008-1075-1
Willis, C. G., Ruhfel, B., Primack, R. B., and Davis, C. C. (2008). Phylogenetic patterns of species loss in Thoreau’s woods are driven by climate change. Proc. Natl. Acad. Sci. U.S.A. 105, 17029–17033. doi: 10.1073/pnas.080644610
Willis, C. G., Ruhfel, B. R., Primack, R. B., Miller-Rushing, A. J., Losos, J. B., and Davis, C. C. (2010). Favorable climate change response explains non-native species’ success in Thoreau’s woods. PLoS One 5:e8878. doi: 10.1371/journal.pone.0008878
Wilsey, B. J., Daneshgar, P. P., and Polley, H. W. (2011). Biodiversity, phenology and temporal niche differences between native- and novel exotic-dominated grasslands. Perspect. Plant Ecol. Evol. Syst. 13, 265–276. doi: 10.1016/j.ppees.2011.07.002
Wolkovich, E. M., and Cleland, E. E. (2011). The phenology of plant invasions: A community ecology perspective. Front. Ecol. Environ. 9, 287–294. doi: 10.1890/100033
Wolkovich, E. M., Davies, T. J., Schaefer, H., Cleland, E. E., Cook, B. I., Travers, S. E., et al. (2013). Temperature-dependent shifts in phenology contribute to the success of exotic species with climate change. Am. J. Bot. 100, 1407–1421. doi: 10.3732/ajb.1200478
Zettlemoyer, M. A. (2022). Data from: Limited evidence for phenological differences between nonnative and native species. Figshare. Available online at: https://doi.org/10.6084/m9.figshare.20202056 (accessed June 30, 2022).
Zettlemoyer, M. A., Renaldi, K., Muzyka, M. D., and Lau, J. A. (2021). Extirpated prairie species demonstrate more variable phenological responses to warming than extant congeners. Am. J. Bot. 108, 958–970. doi: 10.1002/ajb2.1684
Keywords: climate change, introduced range, invasion, meta-analysis, native species, non-native species, phenology, phenological sensitivity
Citation: Zettlemoyer MA, Ellis SL, Hale CW, Horne EC, Thoen RD and DeMarche ML (2022) Limited evidence for phenological differences between non-native and native species. Front. Ecol. Evol. 10:983172. doi: 10.3389/fevo.2022.983172
Received: 30 June 2022; Accepted: 18 August 2022;
Published: 23 September 2022.
Edited by:
Pablo Cortes, NEOM, Saudi ArabiaReviewed by:
Jiří Skuhrovec, Crop Research Institute, CzechiaCopyright © 2022 Zettlemoyer, Ellis, Hale, Horne, Thoen and DeMarche. This is an open-access article distributed under the terms of the Creative Commons Attribution License (CC BY). The use, distribution or reproduction in other forums is permitted, provided the original author(s) and the copyright owner(s) are credited and that the original publication in this journal is cited, in accordance with accepted academic practice. No use, distribution or reproduction is permitted which does not comply with these terms.
*Correspondence: Meredith A. Zettlemoyer, bWVyZWRpdGguemV0dGxlbW95ZXIyNUB1Z2EuZWR1
Disclaimer: All claims expressed in this article are solely those of the authors and do not necessarily represent those of their affiliated organizations, or those of the publisher, the editors and the reviewers. Any product that may be evaluated in this article or claim that may be made by its manufacturer is not guaranteed or endorsed by the publisher.
Research integrity at Frontiers
Learn more about the work of our research integrity team to safeguard the quality of each article we publish.