- Department of Biology, University of Prince Edward Island, Charlottetown, PE, Canada
Light pollution is becoming prevalent among other coastal stressors, particularly along intertidal habitats, arguably the most exposed to anthropogenic light sources. As the number of light pollution studies on sandy beaches, rocky shores and other intertidal habitats raises, commonalities, research gaps and venues can be identified. Hence, the influence of light pollution on the behavior and ecology of a variety of intertidal macro-invertebrates and vertebrates are outlined by examining 54 published studies. To date, a large majority of the reported effects of light pollution are negative, as expected from the analysis of many species with circadian rhythms or nocturnal habits, although the severity of those effects ranges widely. Experimental approaches are well represented throughout but methodological limitations in measurement units and standardization continue to limit the proposal of general conclusions across species and habitats. In addition, studies targeting community variables and the explicit influence of skyglow are heavily underrepresented. Likewise, studies addressing the interaction between light pollution and other natural and anthropogenic stressors are critically needed and represent a key venue of research. The nature of those interactions (synergistic, additive, antagonistic) will likely dictate the impact and management of light pollution in the decades ahead.
Introduction
Natural ecosystems are facing an unprecedented increase in the number of human-related stressors (Halpern et al., 2008; Bellard et al., 2012). So, it is critical to identify what aspects of the species’ behavior and ecology are being altered and what changes can be expected in the short- and long-term (Griffen et al., 2016). An examination of the published evidence also helps to identify research gaps and venues, particularly in emerging fields of study (Dicks et al., 2014). Arguably, one of such fields is the influence of artificial light pollution at night (hereafter light pollution). Compared to other equally pervasive stressors, light pollution has only recently gathered considerable attention (Davies and Smyth, 2017) despite its fast growth worldwide (Sánchez de Miguel et al., 2021). Historically, most research on light pollution has focused on terrestrial settings and species (Vaz et al., 2020), while studies on aquatic systems (Baz et al., 2022) and on marine systems have lagged behind (Longcore and Rich, 2004). Among the latter, intertidal settings are of interest because they are already exposed to a wide range of other stressors (Gunderson et al., 2016), and are arguably the most directly exposed to the main sources of light pollution.
Our review focuses on the influence of light pollution upon the behavior and ecology of marine intertidal species. We define intertidal settings broadly to include soft- (sandy beaches, sandy and muddy flats, saltmarshes) and hard-bottoms (rocky shores, tide pools and man-made structures), and to encompass a diverse range of invertebrates and vertebrates, and the nature of their use of the intertidal (permanent or seasonal). Even though light pollution effects may emerge from exposure to discrete light sources or the more diffuse and widespread skyglow (Kyba et al., 2015; Cox et al., 2022), most studies with intertidal species have focused on the former type of pollution. This is reflected in the results of this review. Nonetheless, our goals are threefold: (i) to establish commonalities on species’ responses to light pollution, (ii) to identify gaps emerging from those studies, and (iii) to propose key venues of research in relation to other co-occurring stressors. The geographic scope of our review is outlined in Table 1 and Figure 1 and includes 54 published articles (as of July 1st, 2022). These were searched using keywords such as “light pollution” “intertidal” and other relevant descriptors from databases (e.g., Academic Search Complete, BioOne), peer-reviewed and reputed sources, and cross-referencing.
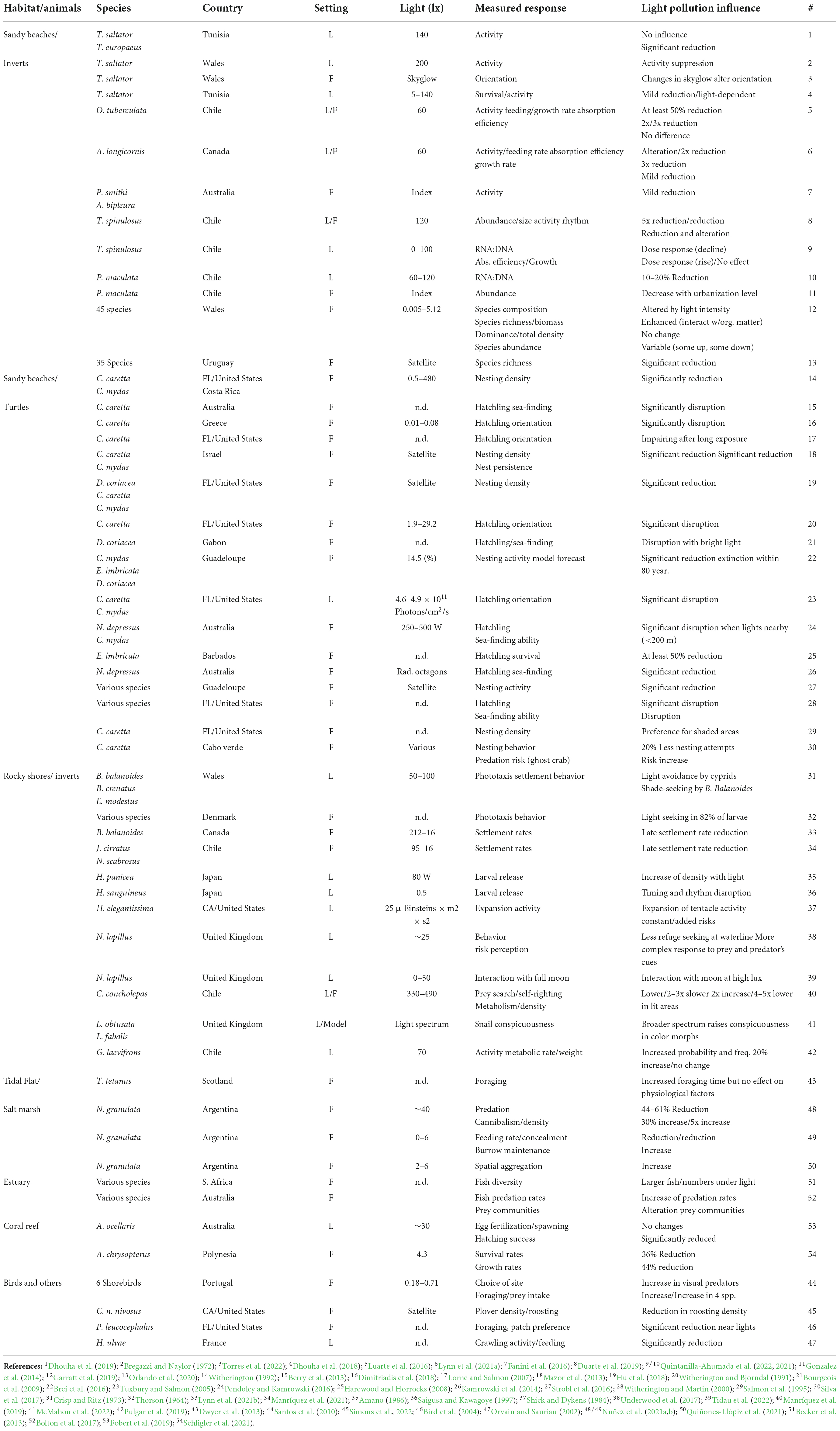
Table 1. Habitat and type of animals studied, target species, location (country), study setting (L, laboratory; F, field), light pollution intensity reported (in lux unless otherwise specified; n.d.: unclear), measured response, light pollution influence and reference (#).
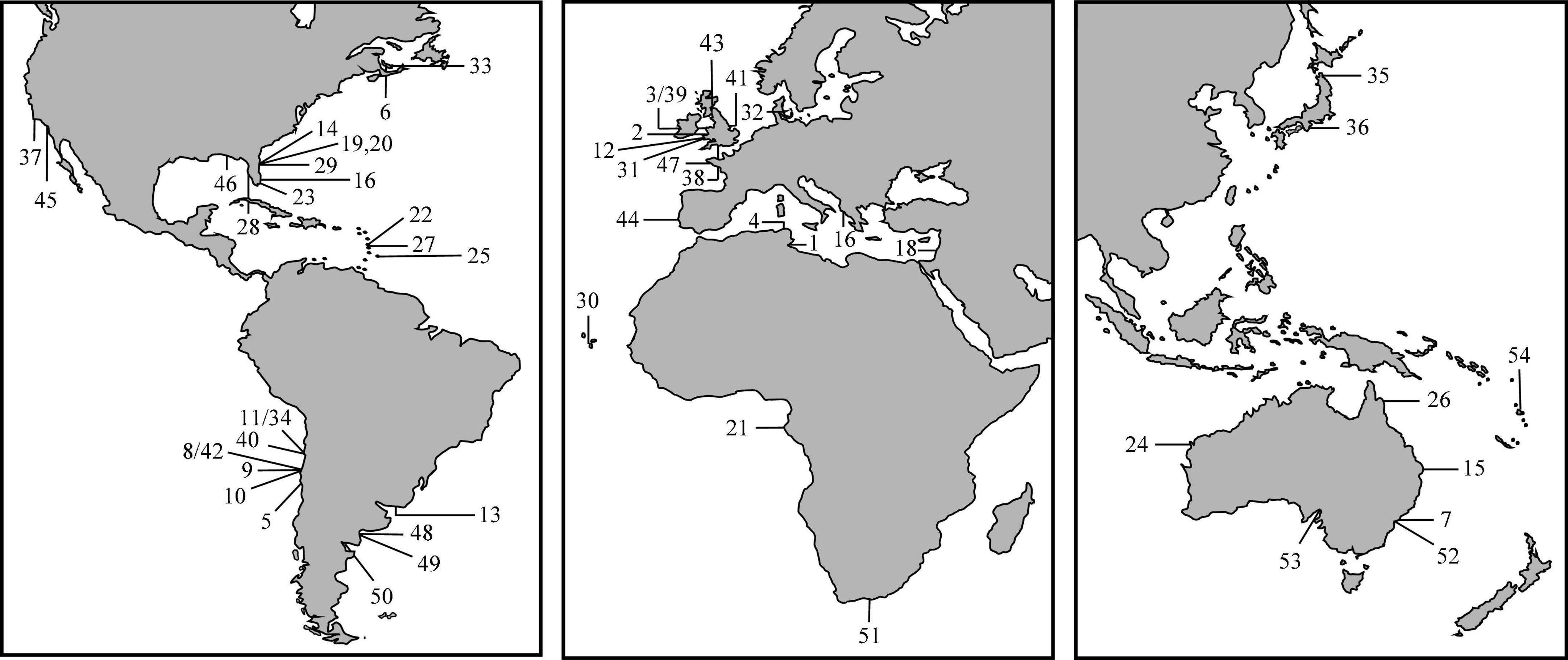
Figure 1. Outline maps of the main geographic regions where the study of light pollution on (macro) intertidal organisms has taken place this far. The maps are for illustrative purposes only, so locations are all approximate and not necessarily at scale. Numbers refer to the 54 studies listed and detailed in Table 1.
Sandy beaches and resident invertebrates
A wide range of light pollution (0.005–200 lx) has been directly or indirectly measured in sandy beaches by thirteen articles nearly equally split in laboratory and field studies. Often referred to as semi-terrestrial species, talitrid amphipods were among the first model-species used in the study of circadian rhythms (see Scapini et al., 2005) and light pollution. Among them, Talitrus saltator was the first to be used to assess the influence of skyglow changes on the species’ orientation across the intertidal (Torres et al., 2022). T. saltator on beaches in Wales and Tunisia, Orchestoidea tuberculata in Chile, Americorchestia longicornis in Canada and Platorchestia smithi in Australia have all been used to measure responses to more direct sources of light pollution. Locomotor activity, a variable intuitively expected to be sensitive to light pollution, has been measured across most studies but has offered inconsistent results. While the activity of three of these species was drastically reduced in the presence of high (200 lx; Bregazzi and Naylor, 1972) or mid-level light pollution (60 lx; Luarte et al., 2016; Lynn et al., 2021a), it remained only slightly altered in other cases (Fanini et al., 2016; Dhouha et al., 2019). The same can be said about burrowing isopods such as Tylos europaeus in Tunisia, T. spinulosus in Chile and A. bipleura in Australia. While activity declined in the former two species, it remained unaltered in the latter one (Fanini et al., 2016). As suggested by Dhouha et al. (2018), these responses seem to be dictated by the strength of the stressor (light intensity), although there are also methodological differences, which in the case of Fanini et al. (2016) entailed the application of a recreation index (McLachlan et al., 2013) as a proxy of light pollution.
Various other behavioral and life history traits have been measured in beach amphipods, isopods and coleopteran insects (Phalerisida maculata), and their outcome is more consistent with respect to light pollution stress. Abundance declined in response to light pollution in T. spinulosus (Duarte et al., 2019), and in response to an urbanization index used as a proxy of light pollution (Legendre and Legendre, 1998) in P. maculata (Gonzalez et al., 2014). Similarly, light pollution was detrimental to feeding rates in O. tuberculata (Luarte et al., 2016) and A. longicornis (Lynn et al., 2021a) and closely related variables such as food absorption efficiency in A. longicornis (Lynn et al., 2021a), RNA:DNA ratios in P. maculata and in T. spinulosus (Quintanilla-Ahumada et al., 2021, 2022) as well as growth rates in O. tuberculata (Luarte et al., 2016). Although the trends are consistent across species, there have been exceptions: Not all the differences between controls and light pollution treatments have been significant. Only two studies have addressed the effects of light pollution on sandy beach communities located in Wales (Garratt et al., 2019) and Uruguay (Orlando et al., 2020). While species diversity increased with light pollution in some sites (Garratt et al., 2019) it declined in others (Orlando et al., 2020). Garratt et al. (2019) also documented a handful of other responses to light pollution stress: an increase in overall biomass, a change in species composition but a lack of effects on overall abundance and dominance.
Sandy beaches and seasonal vertebrates
Seasonal vertebrates such as loggerhead (Caretta caretta), leatherback (Dermochelys coriacea), green (Chelonia mydas), hawksbill (Eretmochelys imbricata), and flatback turtles (Natator depressus) are among the species that have gathered the most public awareness about light pollution impacts (Mazor et al., 2013; Pendoley and Kamrowski, 2016). Seventeen studies to date, most of them field-oriented, have addressed the influence of a wide range of light pollution (0.01–480 lx) on the nesting and hatching of turtles. Studies on the behavior of female turtles attempting to nest have measured light pollution with different methodologies, from direct in situ measurements (Witherington, 1992; Mazor et al., 2013) to satellite imagery (Strobl et al., 2016). These studies have shown rather consistent responses to light pollution: leatherback, green and hawksbill turtle nests have declined in lit areas (Strobl et al., 2016; Silva et al., 2017; Hu et al., 2018). While some species avoided illuminated beaches (Brei et al., 2016), others such as leatherback turtles nesting in Israel used these sites less persistently (Mazor et al., 2013). Furthermore, some females took longer to choose and complete their nests in lit than in dark beaches (Silva et al., 2017). Numerical models have also predicted that permanent light exposure may cause local-scale extinction in some species within 80 years (Brei et al., 2016). Light color (Silva et al., 2017) or source (mercury vs. low pressure sodium; Witherington, 1992) have been also suggested to influence the effects of light pollution on nesting. These should be carefully considered when attempting the management of this source of stress (Gaston et al., 2012).
The second main impact of light pollution is upon turtle hatchling success (Witherington and Martin, 2000; Lorne and Salmon, 2007). Although light pollution remains detrimental, the precise effects are less consistent than those described above. Light pollution disrupts orientation and/or local survival of small hatchlings emerging in sandy beaches of Australia, Greece and Florida, United States (e.g., Witherington and Bjorndal, 1991; Salmon et al., 1995; Lorne and Salmon, 2007). For example, in loggerhead hatchlings exposed to this stressor in Greece, nearly 50% were led astray from a direct path to the water (Dimitriadis et al., 2018). Such effects are mitigated by the distance to light sources or the presence of physical shields (Pendoley and Kamrowski, 2016) or by the influence of full moons (Tuxbury and Salmon, 2005; Berry et al., 2013; Kamrowski et al., 2014). Similar results were found for leatherback hatchlings in Gabon (Bourgeois et al., 2009), which in the presence of light pollution, could not use natural cues such as backshore silhouettes to orient themselves. Regarding another stressor, predation, Silva et al. (2017) found that ghost crab’s activity and predation on loggerhead turtle nests was exacerbated under light pollution in beaches of Cabo Verde (NW Africa). In contrast, Harewood and Horrocks (2008) found that predation on hatchlings crawling over the beach was unaltered, but these authors also suggested that disoriented hatchlings incurred on a harmful waste of energy, that later imposed limitations to their ability to swim.
Rocky shores and related hard-bottom species
These studies (12) have used a wide range of light pollution intensities (0.5–490 lux), mainly on experimental studies conducted in laboratory settings. These studies were preceded by seminal articles focusing on the influence of natural light levels (e.g., moon cycles) on the establishment of barnacles (e.g., Thorson, 1964; Crisp and Ritz, 1973). They were followed by recent studies addressing the differential influence of light pollution on the settlement of acorn barnacles (Semibalanus balanoides) in Atlantic Canada (Lynn et al., 2021b) and Jellhius cirratus and Notochthamalus scabrosus in northern Chile (Manríquez et al., 2021). In both studies, early settling stages (cyprids) were only mildly affected by light pollution, whereas late settling stages (spats) suffered a significant reduction in density with respect to (naturally dark) controls. The literature offers two other examples of light pollution’s detrimental effects at even earlier life stages: Light pollution stress delayed the release of larvae in the sponge Halichondria panicea (Amano, 1986) and a prominent crab species (Hemigrapsus sanguineus) (Saigusa and Kawagoye, 1997) both in Japan.
Established stages (juveniles-adults) have reacted rather consistently to light pollution. In an artificially built jetty in California, light pollution changed the diel activity of sea anemones (Anthopleura elegantissima; Shick and Dykens, 1984). This prompted these anemones to keep expanding their tentacles into the night hours, which at low and high tide conditions exposed them to higher desiccation and predation risk, respectively. Avoidance of light pollution by slow moving marine snails has been reported in the UK (dogwhelks; Nucella lapillus; Underwood et al., 2017) and Chile (large predatory “locos,” Concholepas concholepas; Manríquez et al., 2019). In dogwhelks, light exposure caused a reduction in their response to predator’s olfactory cues, and has also been shown to interact with natural moonlight cycles on the foraging rates of this species on acorn barnacles (Tidau et al., 2022). Light pollution also slowed down the ability of locos to up-right their bodies from an upside-down position, a key antipredator ability in this species (Manríquez et al., 2019). Another anti-predator strategy (camouflage) has been assessed on Littorina obtusata and L. fabalis in the UK (McMahon et al., 2022). Testing the light spectra perceived by three predators of these snails, these authors concluded that light pollution makes some littorinid’s color morphs more conspicuous, removing the benefits originally provided by camouflage. The influence of light pollution on a direct indicator of stress, metabolic rates, has been also measured in the locos referred above and small tide-pool rockfish (Girella geofrans; Pulgar et al., 2019). In both species, light pollution increased metabolic rates, adding energy costs and demands in both species. In the case of the snails, this was accompanied by reduced feeding rates while avoiding lit areas (Manríquez et al., 2019), whereas in the rockfish light pollution altered circadian and circatidal rhythms (Pulgar et al., 2019).
Estuarine and vegetated bottoms used by shorebirds and other species
Tidal flats and vegetated bottoms are used as stopovers by many shorebirds (Murray and Fuller, 2015) which become exposed to the same light pollution sources outlined above. Still, most light-related studies continue to focus on urban skylights altering the orientation of migratory birds (Cabrera-Cruz et al., 2018), on the mortality of seabirds at or near colonies (Oro et al., 2005) or on seabird collisions with light-emitting structures (buildings, platforms or wharves; Jones and Francis, 2003). Reports on this stressor’s effects directly linked to the intertidal zone are more limited (ten, primarily field-oriented are listed here), and for shorebirds they stem from the study of foraging at daylight vs. night hours (e.g., Rojas et al., 1999). For example, a study of the common redshank (Tringa tetanus) in estuarine flats in Scotland showed that birds exposed to light pollution turned to sight-based foraging and fed well into the night hours (Dwyer et al., 2013). Similarly, among six shorebirds in Portugal, visual foragers used light pollution to their advantage and ended up feeding longer and heavier in lit areas (Santos et al., 2010). Tactile foragers faced a disadvantage and where feasible they switched to visual foraging. Simons et al. (2022) also found that Western snowy plovers (Charadrius nivosus) in California were less likely to roost in areas exposed to light pollution due to higher predation risk.
Bird et al. (2004) reported light pollution effects on nocturnal beach mice on tidal flats of Florida, United States: These mice foraged less and reduced food patch preference in areas closer to light sources. Similarly, Orvain and Sauriau (2002) studying mud snails (Hydrobia ulvae) on French mud flats found a reduction in snails’ crawling activity (and growth) in response to light. The impact of light included reduced survival rates, as this stressor entailed enhanced predation rates on the snails by their visual predators. The influence of light pollution on a more mobile invertebrate, the burrowing crab Neohelica granulata has been reported in saltmarsh habitats from Argentina (Nuñez et al., 2021a,b). Using tethering and cage experiments, these authors showed that juvenile crab survival decreased by 30–60% when exposed to light pollution, while the abundance of adult crabs increased up to five times (Nuñez et al., 2021a). Light exposure also reduced crab feeding rates and increased burrow maintenance (Nuñez et al., 2021a). The effects of light pollution on the activity and spatial distribution (aggregation) of this key bioturbator species were also found to be site (context) dependent (Nuñez et al., 2021b; Quiñones-Llópiz et al., 2021).
Additional studies have addressed the influence of light pollution on fish living in borderline habitats, defined here as shallow subtidals closely connected to the intertidal. Since these are not tidal habitats, the studies below illustrate impacts but are not meant to be a comprehensive list (see Bassi et al., 2022 for studies on light pollution and fish). Becker et al. (2013) found that shallow water fish diversity in a South African estuary changed in the presence of light pollution: Large predatory and small shoaling fish aggregated near light sources at night. Given that they were primarily visual predators, these authors suggested that this would enhance feeding rates on nearby prey. Likewise, Bolton et al. (2017) studied fish predation and behavior under a wharf in Australia and found that light pollution reduced fish abundance but increased their predation rates on sessile invertebrates. Also in Australia, but in coral reefs habitats, Fobert et al. (2019) found that the reproductive success (egg hatching) of a clownfish (Amphiprion ocellaris) was reduced under light pollution. Another study from coral reefs in the French Polynesia examined the long-term effects of light pollution on juvenile anemonefish (Amphiprion chrysopterus; Schligler et al., 2021) and found that this stressor reduced fish survival and growth. While light pollution has positive effects on some species, these benefits often extend across trophic levels to their own predators, a balance (or unbalance) that warrants further study.
Commonalities, gaps, venues, and co-occurring stressors
To date, a large majority of the reported effects of light pollution is negative. To some degree, this is related to the targets of most of these studies, which have included species with marked circadian rhythms (e.g., talitrid amphipods), nocturnal habits (e.g., foraging shorebirds), or tradeoffs involving visual predators (e.g., rocky shore snails). The severity of these effects ranges widely, from mild responses to the complete suppression or disruption of animal activity. From the literature reviewed, a first important gap has been identified already: The limited number of studies focusing explicitly on community-level effects, which to date include only Garratt et al. (2019) and Orlando et al. (2020). A second gap is the virtual absence of studies explicitly assessing the response of intertidal organisms to the influence of skyglow, or the brightening of the night sky resulting from the reflection and large-scale scattering of light emissions (see Kyba et al., 2015). Skyglow is often mentioned, but with one exception (Torres et al., 2022), most studies continue to focus on discrete light emissions (either direct sources or their reflection). A third outstanding gap is the virtual absence of studies addressing the combined effects of light pollution and other stressors (see Miller et al., 2017 for examples in terrestrial insects). Exceptions include a few studies referred above: Orvain and Sauriau (2002) and Garratt et al. (2019) found that the effects of light pollution on community biomass and mud snails, respectively, were modulated by the availability of organic matter in the habitat. Similarly, the influence of light pollution on dogwhelk foraging (Tidau et al., 2022) and sea turtle hatchlings (a few studies) interacted with natural moonlight cycles. These studies mark the beginning of the examination of potential synergistical effects (see Halfwerk and Slabbekoorn, 2015) between light pollution and other natural or anthropogenic stressors and should likely escalate in complexity as new research becomes available.
Research on light pollution has not been free of limitations. Experimental approaches are well represented, but a standardization of methodologies and more consistent light intensities are needed to allow more meaningful comparisons among experiments. The use of lux units has allowed studies across species and habitats in intertidal and terrestrial habitats (e.g., Grunst et al., 2022). However, species’ spectral detection ranges change among distinct types of organisms (Dominoni et al., 2020) and lux measurements do not necessarily capture those differences. Alternative units and methodologies further dissecting light characteristics and species perception ranges need to be consistently applied to describe this stressor’s impacts (Gaston et al., 2012). Likewise, more dose-response analyses are needed to better understand the reaction of species to current and predicted light-stress scenarios (see Quintanilla-Ahumada et al., 2022). At the landscape scale, the use of remote sensing tools is growing in popularity and applicability (Hu et al., 2018). However, as in other habitats (e.g., Raap et al., 2017) and disciplines, a combination of rigorous exploratory and experimental approaches is likely to provide the best prospects for understanding how species respond to light pollution. With regards to the interplay between light pollution and other natural and anthropogenic stressors, it remains to be seen whether additive, non-additive or antagonistic effects predominate in these interactions. Their nature will likely dictate the impact and management of light pollution in the decades ahead.
Author contributions
KDL and PQ conceived and conducted the review of existing literature and drafted and edited the full manuscript. Both authors contributed to the article and approved the submitted version.
Funding
This work was supported by the Natural Sciences and Engineering Research Council, Canada (NSERC) through Discovery grants (PQ) and CGS-D scholarships (KDL).
Acknowledgments
We thank the handling editor and two reviewers for their comments and feedback on an earlier version of the manuscript.
Conflict of interest
The authors declare that the research was conducted in the absence of any commercial or financial relationships that could be construed as a potential conflict of interest.
Publisher’s note
All claims expressed in this article are solely those of the authors and do not necessarily represent those of their affiliated organizations, or those of the publisher, the editors and the reviewers. Any product that may be evaluated in this article, or claim that may be made by its manufacturer, is not guaranteed or endorsed by the publisher.
References
Amano, S. (1986). Larval release in response to a light signal by the intertidal sponge Halichondria panicea. Biol. Bull. 171, 371–378. doi: 10.2307/1541679
Bassi, A., Love, O. P., Cooke, S. J., Warriner, T. R., Harris, C. M., and Madliger, C. L. (2022). Effects of artificial light at night on fishes: A synthesis with future research priorities. Fish Fish. 23, 631–647. doi: 10.1111/faf.12638
Baz, E.-Z., Hussein, A. A. A., Vreeker, E. M. T., Soliman, M. F. M., Tadros, M. M., El-Shenawy, N. S., et al. (2022). Consequences of artificial light at night on behavior, reproduction, and development of Lymnaea stagnalis. Environ. Pollut. 307:119507. doi: 10.1016/j.envpol.2022.119507
Becker, A., Whitfield, A. K., Cowley, P. D., Jarnegren, J., and Naesje, T. F. (2013). Potential effects of artificial light associated with anthropogenic infrastructure on the abundance and foraging behaviour of estuary-associated fishes. J. Appl. Ecol. 50, 43–50. doi: 10.1111/1365-2664.12024
Bellard, C., Bertelsmeier, C., Leadley, P., Thuiller, W., and Courchamp, F. (2012). Impacts of climate change on the future of biodiversity. Ecol. Lett. 15, 365–377. doi: 10.1111/j.1461-0248.2011.01736.x
Berry, M., Booth, D. T., and Limpus, C. J. (2013). Artificial lighting and disrupted sea-finding behaviour in hatchling loggerhead turtles (Caretta caretta) on the Woongarra coast, southeast Queensland, Australia. Aust. J. Zool. 61, 137–145. doi: 10.1071/ZO13028
Bird, B. L., Branch, L. C., and Miller, D. L. (2004). Effects of coastal lighting on foraging behavior of beach mice. Conserv. Biol. 18, 1435–1439. doi: 10.1111/j.1523-1739.2004.00349.x
Bolton, D., Mayer-Pinto, M., Clark, G. F., Dafforn, K. A., Brassil, W. A., Becker, A., et al. (2017). Coastal urban lighting has ecological consequences for multiple trophic levels under the sea. Sci. Total Environ. 576, 1–9. doi: 10.1016/j.scitotenv.2016.10.037
Bourgeois, S., Gilot-Fromont, E., Viallefont, A., Boussamba, F., and Deem, S. L. (2009). Influence of artificial lights, logs, and erosion on leatherback sea turtle hatchling orientation at Pongara National Park, Gabon. Biol. Conserv. 142, 85–93. doi: 10.1016/j.biocon.2008.09.028
Bregazzi, P. K., and Naylor, E. (1972). The locomotor activity rhythm of Talitrus saltator (Montagu) (Crustacea, Amphipoda). J. Exp. Biol. 57, 375–391. doi: 10.1242/jeb.57.2.375
Brei, M., Perez-Barahona, A., and Strobl, E. (2016). Environmental pollution and biodiversity: Light pollution and sea turtles in the Caribbean. J. Environ. Econ. Manage. 77, 95–116.
Cabrera-Cruz, S., Smolinsky, J. A., and Buler, J. J. (2018). Light pollution is greatest within migration passage areas for nocturnally-migrating birds around the world. Sci. Rep. 8:3261. doi: 10.1038/s41598-018-21577-6
Cox, D. T. C., Sánchez de Miguel, A., Bennie, J., Dzurjak, S. A., and Gaston, K. J. (2022). Majority of artificially lit earth surface associated with the non-urban population. Sci. Total Environ. 841:156782. doi: 10.1016/j.scitotenv.2022.156782
Crisp, D. J., and Ritz, D. A. (1973). Responses of cirripede larvae to light. I. Experiments with white light. Mar. Biol. 23, 327–335. doi: 10.1007/BF00389340
Davies, T. W., and Smyth, T. (2017). Why artificial light at night should be a focus for global change research in the 21st century. Glob. Change Biol. 24, 872–882. doi: 10.1111/gcb.13927
Dhouha, B. A., Raja, J., Elfed, M., and Karima, N. A. (2018). Effect of light intensity on the locomotor activity rhythm of Talitrus saltator (Montagu 1808) from Korba beach. Biol. Rhythm Res. 49, 735–745. doi: 10.1080/09291016.2017.1413764
Dhouha, B. A., Raja, J., Elfed, M., and Karima, N. A. (2019). Locomotor activity rhythm of two sympatric species Talitrus saltator (Amphipoda) and Tylos europaeus (Oniscidea) from Tunisian beaches under continuous light. Biol. Rhythm Res. 50, 180–191. doi: 10.1080/09291016.2018.1433471
Dicks, L. V., Walsh, J. C., and Sutherland, W. J. (2014). Organizing evidence for environmental management decisions: A ‘4S’ hierarchy. Trends Ecol. Evol. 29, 607–613. doi: 10.1016/j.tree.2014.09.004
Dimitriadis, C., Fournari-Konstantinidou, I., Sourbes, L., Koutsoubas, D., and Mazaris, A. D. (2018). Reduction of sea turtle population recruitment caused by nightlight: Evidence from the Mediterranean region. Ocean Coast. Manage. 153, 108–115. doi: 10.1016/j.ocecoaman.2017.12.013
Dominoni, D. M., Halfwerk, W., Baird, E., Buxton, R. T., Fernández-Juricic, E., Fristrup, K. M., et al. (2020). Why conservation biology can benefit from sensory ecology. Nat. Ecol. Evol. 4, 502–511. doi: 10.1038/s41559-020-1135-4
Duarte, C., Quintanilla-Ahumada, D., Anguita, C., Manriquez, P. H., Widdicombe, S., Pulgar, J., et al. (2019). Artificial light pollution at night (ALAN) disrupts the distribution and circadian rhythm of a sandy beach isopod. Environ. Pollut. 248, 565–573. doi: 10.1016/j.envpol.2019.02.037
Dwyer, R. G., Bearhop, S., Campbell, H. A., and Bryant, D. M. (2013). Shedding light on light: Benefits of anthropogenic illumination to a nocturnally foraging shorebird. J. Anim. Ecol. 82, 478–485. doi: 10.1111/1365-2656.12012
Fanini, L., Hughes, L. E., Springthorpe, R., Tosetto, L., and Lowry, J. K. (2016). Surface activity patterns of macrofauna on pocket, tidal beaches: Insights into the role of wrack and artificial lighting. Reg. Stud. Mar. Sci. 7, 63–71. doi: 10.1016/j.rsma.2016.05.007
Fobert, E. K., Burke Da Silva, K., and Swearer, S. E. (2019). Artificial light at night causes reproductive failure in clownfish. Biol. Lett. 15:20190272. doi: 10.1098/rsbl.2019.0272
Garratt, M. J., Jenkins, S. R., and Davies, T. W. (2019). Mapping the consequences of artificial light at night for intertidal ecosystems. Sci. Total Environ. 691, 760–768. doi: 10.1016/j.scitotenv.2019.07.156
Gaston, K. J., Davies, T. W., Bennie, J., and Hopkins, J. (2012). Reducing the ecological consequences of night-time light pollution: Options and developments. J. Appl. Ecol. 49, 1256–1266. doi: 10.1111/j.1365-2664.2012.02212.x
Gonzalez, S. A., Yanez-Navia, K., and Munoz, M. (2014). Effect of coastal urbanization on sandy beach coleoptera Phaleria maculata (Kulzer, 1959) in northern Chile. Mar. Pollut. Bull. 83, 265–274. doi: 10.1016/j.marpolbul.2014.03.042
Griffen, B. D., Belgrad, B. A., Cannizzo, Z. J., Knotts, E. R., and Hancock, E. R. (2016). Rethinking our approach to multiple stressor studies in marine environments. Mar. Ecol. Prog. Ser. 543, 273–281. doi: 10.3354/meps11595
Grunst, M. L., Grunst, A. S., Pinxten, R., Eens, G., and Eens, M. (2022). An experimental approach to investigating effects of artificial light at night on free-ranging animals: Implementation, results, and directions for future research. J. Vis. Exp. e63381. ∗∗volQ. doi: 10.3791/63381
Gunderson, A. R., Armstrong, E. J., and Stillman, J. H. (2016). Multiple stressors in a changing world: The need for an improved perspective on physiological responses to the dynamic marine environment. Annu. Rev. Mar. Sci. 8, 357–357. doi: 10.1146/annurev-marine-122414-033953
Halfwerk, H., and Slabbekoorn, H. (2015). Pollution going multimodal: The complex impact of human-altered sensory environment on animal perception and performance. Biol. Lett. 11:20141051. doi: 10.1098/rsbl.2014.1051
Halpern, B. S., Walbridge, S., Selkoe, K. A., Kappel, C. V., Micheli, F., D’Agrosa, C., et al. (2008). A global map of human impact on marine ecosystems. Science 319, 948–952. doi: 10.1126/science.1149345
Harewood, A., and Horrocks, J. (2008). Impacts of coastal development on hawksbill hatchling survival and swimming success during the initial offshore migration. Biol. Conserv. 141, 394–401. doi: 10.1016/j.biocon.2007.10.017
Hu, Z., Hu, H., and Huang, Y. (2018). Association between nighttime artificial light pollution and sea turtle nest density along Florida coast: A geospatial study using VIIRS remote sensing data. Environ. Pollut. 239, 30–42. doi: 10.1016/j.envpol.2018.04.021
Jones, J., and Francis, C. M. (2003). The effects of light characteristics on avian mortality at lighthouses. J. Avian Biol. 34, 328–333. doi: 10.1111/j.0908-8857.2003.03183.x
Kamrowski, R. L., Limpus, C., Pendoley, K., and Hamann, M. (2014). Influence of industrial light pollution on the sea-finding behaviour of flatback turtle hatchlings. Wildl. Res. 41, 421–434. doi: 10.1071/WR14155
Kyba, C. M., Tong, K. P., Bennie, J., Birriel, I., Birriel, J. J., Cool, A., et al. (2015). Worldwide variations in artificial skyglow. Sci. Rep. 5:8409. doi: 10.1038/srep08409
Legendre, P., and Legendre, L. (1998). Variation partitioning of species data matrices: Estimation and comparison of fractions. Ecology 87, 2614–2625. doi: 10.1890/0012-9658(2006)87[2614:VPOSDM]2.0.CO;2
Longcore, T., and Rich, C. (2004). Ecological light pollution. Front. Ecol. Environ. 2, 191–198. doi: 10.1890/1540-9295(2004)002[0191:ELP]2.0.CO;2
Lorne, J. K., and Salmon, M. (2007). Effects of exposure to artificial lighting on orientation of hatchling sea turtles on the beach and in the ocean. Endang. Species Res. 3, 23–30. doi: 10.3354/esr003023
Luarte, T., Bonta, C. C., Silva-Rodriguez, E. A., Quijón, P. A., Miranda, C., Farias, A. A., et al. (2016). Light pollution reduces activity, food consumption, and growth rates in a sandy beach invertebrate. Environ. Pollut. 218, 1147–1153. doi: 10.1016/j.envpol.2016.08.068
Lynn, K. D., Quintanilla-Ahumada, D., Anguita, C., Widdicombe, S., Pulgar, J., Manriquez, P. H., et al. (2021a). Artificial light at night alters the activity and feeding behaviour of sandy beach amphipods and pose a threat to their ecological role in Atlantic Canada. Sci. Total Environ. 780:146568. doi: 10.1016/j.scitotenv.2021.146568
Lynn, K. D., Tummon Flynn, P., Manríquez, K., Manríquez, P. H., Pulgar, J., Duarte, C., et al. (2021b). Artificial light at night alters the settlement of acorn barnacles on a man-made habitat in Atlantic Canada. Mar. Pollut. Bull. 163:111928. doi: 10.1016/j.marpolbul.2020.111928
Manríquez, K., Quijón, P. A., Manríquez, P. H., Miranda, C., Pulgar, J., Quintanilla-Ahumada, D., et al. (2021). Artificial light at night (ALAN) negatively effects the settlement success of two prominent intertidal barnacles in the southeast Pacific. Mar. Pollut. Bull. 168:112416. doi: 10.1016/j.marpolbul.2021.112416
Manríquez, P. H., Jara, M. E., Díaz, M. I., Quijón, P. A., Widdicombe, S., Pulgar, J., et al. (2019). Artificial light pollution influences behavioural and physiological traits in a keystone predator species Concholepas concholepas. Sci. Total Environ. 661, 543–552. doi: 10.1016/j.scitotenv.2019.01.157
Mazor, T., Levin, N., Possingham, H. P., Levy, Y., Rocchini, D., Richardson, A. K., et al. (2013). Can satellite-based night lights be used for conservation? The case of nesting sea-turtles in the Mediterranean. Biol. Conserv. 159, 63–72. doi: 10.1016/j.biocon.2012.11.004
McLachlan, A., Defeo, O., Jaramillo, E., and Short, A. D. (2013). Sandy beaches conservation and recreation: Guidelines for optimizing management strategies for multipurpose use. Ocean Coast. Manage. 71, 256–268. doi: 10.1016/j.ocecoaman.2012.10.005
McMahon, O., Smyth, T., and Davies, T. W. (2022). Broad spectrum artificial light at night increases the conspicuousness of camouflaged prey. J. Appl. Ecol. 59, 1324–1333. doi: 10.1111/1365-2664.14146
Miller, C. R., Barton, B. T., Zhu, L., Radeloff, V. C., Oliver, K. M., Harmon, J. P., et al. (2017). Combined effects of night warming and light pollution on predator–prey interactions. Proc. R. Soc. B 284:20171195. doi: 10.1098/rspb.2017.1195
Murray, N. J., and Fuller, R. A. (2015). Protecting stopover habitat for migratory shorebirds in East Asia. J. Ornithol. 156, S217–S225. doi: 10.1007/s10336-015-1225-2
Nuñez, J. D., Bas, C., Peréz García, M., Ocampo, E. H., Ribeiro, P. D., and Luppi, T. A. (2021a). Artificial light at night increases the predatory pressure in a salt marsh keystone species. Mar. Environ. Res. 167:105285. doi: 10.1016/j.marenvres.2021.105285
Nuñez, J. D., Sbragaglia, V., Ribeiro, P. D., Spivak, E., and Luppi, T. A. (2021b). The magnitude of behavioral responses to artificial light at night depends on the ecological context in a coastal marine ecosystem engineer. Mar. Environ. Res. 165:105238. doi: 10.1016/j.marenvres.2020.105238
Orlando, L., Ortega, L., and Defeo, O. (2020). Urbanization effects on sandy beach macrofauna along an estuarine gradient. Ecol. Indic. 111:106036. doi: 10.1016/j.ecolind.2019.106036
Oro, D., de León, A., Minguez, E., and Furness, R. W. (2005). Estimating predation on breeding European storm-petrels (Hydrobates pelagicus) by yellow-legged gulls (Larus michahellis). J. Zool. 265, 421–429. doi: 10.1017/S0952836905006515
Orvain, O., and Sauriau, P. G. (2002). Environmental and behavioural factors affecting activity in the intertidal gastropod Hydrobia ulvae. J. Exp. Mar. Biol. Ecol. 272, 191–216. doi: 10.1016/S0022-0981(02)00130-2
Pendoley, K., and Kamrowski, R. L. (2016). Sea-finding in marine turtle hatchlings: What is an appropriate exclusion zone to limit impacts of industrial light at night? J. Nat. Conserv. 30, 1–11. doi: 10.1016/j.jnc.2015.12.005
Pulgar, J., Zeballos, D., Vargas, J., Aldana, M., Manriquez, P., Manriquez, K., et al. (2019). Endogenous cycles, activity patterns, and energy expenditure of an intertidal fish is modified by artificial light at night (ALAN). Environ. Pollut. 244, 361–366. doi: 10.1016/j.envpol.2018.10.063
Quiñones-Llópiz, J. D., Ribeiro, P. D., Luppi, T. A., Chiaradia, N. M., and Nuñez, J. D. (2021). Artificial light at night (ALAN) mediates transient spatial aggregation of an ecosystem engineer, the crab Neohelice granulata (Dana, 1851) (Decapoda: Brachyura: Varunidae), under different ecological contexts. J. Crustac. Biol. 41:ruab060. doi: 10.1093/jcbiol/ruab060
Quintanilla-Ahumada, D., Quijón, P. A., Manríquez, P. H., Pulgar, J., García-Huidobro, M. R., Miranda, C., et al. (2022). Artificial light at night (ALAN) causes variable dose-responses in a sandy beach isopod. Environ. Sci. Pollut. Res. Int. 29, 35977–35985. doi: 10.1007/s11356-021-17344-2
Quintanilla-Ahumada, D., Quijón, P. A., Pulgar, J., Manriquez, P. H., Roberto Garcia-Huidobro, M., and Duarte, C. (2021). Exposure to artificial light at night (ALAN) alters RNA:DNA in a sandy beach coleopteran insect. Mar. Pollut. Bull. 165:112132. doi: 10.1016/j.marpolbul.2021.112132
Raap, T., Pinxten, R., and Eens, M. (2017). Rigorous field experiments are essential to understand the genuine severity of light pollution and to identify possible solutions. Glob. Change Biol. 23, 5024–5026. doi: 10.1111/gcb.13843
Rojas, L. M., McNeil, R., Cabana, T., and Lachapelle, P. (1999). Diurnal and nocturnal visual capabilities in shorebirds as a function of their feeding strategies. Brain Behav. Evol. 53, 29–43. doi: 10.1159/000006580
Saigusa, M., and Kawagoye, O. (1997). Circatidal rhythm of an intertidal crab Hemigrapsus sanguineus: Synchrony with unequal tide height and involvement of a light-response mechanism. Mar. Biol. 129, 87–96. doi: 10.1007/s002270050149
Salmon, M., Tolbert, M. G., Paynter, D. P., Goff, M., and Reiners, R. (1995). Behavior of loggerhead sea turtles on an urban beach. II. Hatchling orientation. J. Herpetol. 29, 568–576. doi: 10.2307/1564740
Sánchez de Miguel, A., Bennie, J., Rosenfeld, E., Dzurjak, S., and Gaston, K. J. (2021). First estimation of global trends in nocturnal power emissions reveals acceleration of light pollution. Remote Sens. 13:3311. doi: 10.3390/rs13163311
Santos, C. D., Miranda, A. C., Granadiero, J. P., Lourenco, P. M., Saraiva, S., and Palmeirim, J. M. (2010). Effects of artificial illumination on the nocturnal foraging of waders. Acta Oecol. 36, 166–172. doi: 10.1016/j.actao.2009.11.008
Scapini, F., Rossano, C., Marchetti, G. M., and Morgan, E. (2005). The role of biological clock in sun compass orientation of free-running individual of Talitrus saltator. Anim. Behav. 69, 835–843. doi: 10.1016/j.anbehav.2004.08.008
Schligler, J., Cortese, D., Beldade, R., Swearer, S. E., and Mills, S. C. (2021). Long-term exposure to artificial light at night in the wild decreases survival and growth of a coral reef fish. Proc. R. Soc. B 288:20210454. doi: 10.1098/rspb.2021.0454
Shick, J. M., and Dykens, J. A. (1984). Photobiology of the symbiotic sea anemone Anthopleura elegantissima: Photosynthesis, respiration, and behaviour under intertidal conditions. Biol. Bull. 166, 608–619. doi: 10.2307/1541166
Silva, E., Marco, A., da Graça, J., Pérez, H., Abella, E., Patino-Martinez, J., et al. (2017). Light pollution affects nesting behavior of loggerhead turtles and predation risk of nests and hatchlings. J. Photochem. Photobiol. B Biol. 173, 240–249. doi: 10.1016/j.jphotobiol.2017.06.006
Simons, A. L., Martin, K. L. M., and Longcore, T. (2022). Determining the effects of artificial light at night on the distributions of western snowy plovers (Charadrius nivosus nivosus) and California grunion (Leuresthes tenuis) in southern California. J. Coast. Res. 38, 302–309. doi: 10.2112/JCOASTRES-D-21-00107.1
Strobl, E., Brei, M., and Perez-Barahona, A. (2016). Environmental pollution and biodiversity: Light pollution and sea turtles in the Caribbean. J. Environ. Econ. Manage. 77, 95–116. doi: 10.1016/j.jeem.2016.02.003
Thorson, G. (1964). Light as an ecological factor in the dispersal and settlement of larvae of marine bottom invertebrates. Ophelia 1, 167–208. doi: 10.1080/00785326.1964.10416277
Tidau, S., Whittle, J., Jenkins, S. R., and Davies, T. W. (2022). Artificial light at night reverses monthly foraging pattern under simulated moonlight. Biol. Lett. 18:20220110. doi: 10.1098/rsbl.2022.0110
Torres, D., Tidau, S., Jenkins, S., and Davies, T. (2022). Artificial skyglow disrupts celestial migration at night. Curr. Biol. 30, R677–R697. doi: 10.1016/j.cub.2020.05.002
Tuxbury, S. M., and Salmon, M. (2005). Competitive interactions between artificial lighting and natural cues during seafinding by hatchling marine turtles. Biol. Conserv. 121, 311–316. doi: 10.1016/j.biocon.2004.04.022
Underwood, C. N., Davies, T. W., and Queirós, A. M. (2017). Artificial light at night alters trophic interactions of intertidal invertebrates. J. Anim. Ecol. 86, 781–789. doi: 10.1111/1365-2656.12670
Vaz, S., Manes, S., Gama-Maia, D., Silveira, L., Mattos, G., Paiva, P. C., et al. (2020). Light pollution is the fastest growing potential threat to firefly conservation in the Atlantic Forest hotspot. Insect Conserv. Divers. 14, 211–224. doi: 10.1111/icad.12481
Witherington, B. E. (1992). Behavioural response of nesting sea turtles to artificial lighting. Herpetologica 48, 31–39.
Witherington, B. E., and Bjorndal, K. A. (1991). Influences of artificial lighting on the seaward orientation of hatchling loggerhead turtles Caretta caretta. Biol. Conserv. 55, 139–149. doi: 10.1016/0006-3207(91)90053-C
Witherington, B. E., and Martin, R. E. (2000). Understanding, assessing, and resolving light pollution problems on sea turtle nesting beaches. 2nd ed. rev. Florida Marine Research Institute Technical Report TR-2 73. St. Petersburg, FL: Florida Fish and Wildlife Conservation Commission, Marine Research Institute.
Keywords: light pollution, sandy beaches, rocky shores, tidal flats, species responses
Citation: Lynn KD and Quijón PA (2022) Casting a light on the shoreline: The influence of light pollution on intertidal settings. Front. Ecol. Evol. 10:980776. doi: 10.3389/fevo.2022.980776
Received: 28 June 2022; Accepted: 12 August 2022;
Published: 31 August 2022.
Edited by:
Marcel Eens, University of Antwerp, BelgiumReviewed by:
Karen Manríquez, Andres Bello University, ChileMaría Bagur, CONICET Centro Austral de Investigaciones Científicas (CADIC), Argentina
Copyright © 2022 Lynn and Quijón. This is an open-access article distributed under the terms of the Creative Commons Attribution License (CC BY). The use, distribution or reproduction in other forums is permitted, provided the original author(s) and the copyright owner(s) are credited and that the original publication in this journal is cited, in accordance with accepted academic practice. No use, distribution or reproduction is permitted which does not comply with these terms.
*Correspondence: Pedro A. Quijón, cHF1aWpvbkB1cGVpLmNh