- 1Department of Oceanography, Dalhousie University, Halifax, NS, Canada
- 2Global Institute of Sustainability and Innovation, Center for Biodiversity Outcomes, Arizona State University, Tempe, AZ, United States
- 3Smithsonian Tropical Research Institute, Balboa, Panama
- 4Radiogenic Isotope Facility, School of Earth Sciences, The University of Queensland, Brisbane, QLD, Australia
- 5Center for Marine Biodiversity and Conservation, Scripps Institution of Oceanography, University of California, San Diego, San Diego, CA, United States
Humans have utilized the Mesoamerican Reef (MAR) for millennia but the effects of prehistorical and historical fishing on this ecosystem remain understudied. To assess the long-term coupling of reef ecosystem and human dynamics in this region, we tracked trends in the structure and functioning of lagoonal reefs within the Belizean portion of the MAR using fish teeth fossils and sediment accumulation rates within reef sediment cores. We then paired this with a timeline of demographic and cultural changes in this region’s human populations. The ∼1,300-year timeline encompassed in the core record shows that declines in the relative abundance and accumulation rate of teeth from parrotfish, a key reef herbivore, occurred at all three reef sites and began between ∼1500 and 1800 AD depending on site and metric of abundance. A causality analysis showed that parrotfish relative abundance had a positive causal effect on reef accretion rates, a proxy of coral growth, reconfirming the important role of these fish in reef ecosystem functioning. The timing of initial declines in parrotfish teeth occurred during a time of relatively low human population density in Belize. However, declines were synchronous with cultural and demographic upheaval resulting from European colonization of the New World. The more recent declines at these sites (∼1800 AD) occurred in tandem with increased subsistence fishing on reefs by multiple immigrant groups, a pattern that was likely necessitated by the establishment of an import economy controlled by a small group of land-owning European elites. These long-term trends from the paleoecological record reveal that current parrotfish abundances in central Belize are well below their pre-European contact peaks and that increased fishing pressure on parrotfish post-contact has likely caused a decline in reef accretion rates. The origins of reef degradation in the Belizean portion of the MAR began hundreds of years before the onset of modern declines resulting from the combined effects of local human disturbances and climate change.
Introduction
The threats facing coral reefs are varied and include: climate change, land-based pollution, overfishing, invasive species, and resulting impacts such as coral disease and bleaching (Bellwood et al., 2004; Jackson et al., 2014; Gress et al., 2019). The Mesoamerican Reef (MAR) is the world’s second largest coral reef tract, stretching over 1,000 miles across the coastlines of Mexico, Belize, Guatemala, and Honduras (Gress et al., 2019). Belizean reefs constitute a major portion of the MAR, an important tourist destination and source of food and livelihood for adjacent coastal communities (McField et al., 2020). Although Belize contains an extensive network of coral reef marine protected areas and has banned the commercial take of herbivorous parrotfish since 2009, coral cover within lagoonal areas of the Belizean MAR is low and macroalgal cover remains high (McField et al., 2020). These reefs are also heavily influenced by sediment-laden freshwater runoff during the rainy season and are characterized by low water clarity year-round (Burke and Sugg, 2006; Soto et al., 2009; Jackson et al., 2014, personal obs.). The low coral cover of these reefs today has been primarily attributed to coral bleaching from anthropogenic climate change, but the long-term impacts of fishing and land-based pollution have been relatively unassessed due to a lack of historical data on reef fish abundance and reef water quality.
Since the onset of systematic monitoring in the 1970s, researchers have documented the same series of disturbances on Belizean reefs that have occurred throughout the Caribbean (Jackson et al., 2014; Gress et al., 2019). First, the long-term overfishing of reef herbivores, including parrotfish, allowed for the replacement of some reef-building corals with macroalgae (Jackson et al., 2001; Hughes et al., 2007), a trend that was exacerbated by the mass dieoff of the keystone herbivore urchin Diadema antillarum from an unknown pathogen in the 1980s (Lessios, 1988; Hughes, 1994; Jackson et al., 2014). Coral abundance was further reduced by outbreaks of White Band Disease (WBD) that became widespread in the 1980s and resulted in mass mortality of the architecturally important reef-building corals Acropora cervicornis and Acropora palmata (Aronson and Precht, 1997; Rodriguez-Martinez et al., 2014). Paleoecological and historical data show that Acropora dominated Belize’s central lagoonal reefs for at least the last 3,000 years, implicating human disturbance as the driver of recent changes (Aronson and Precht, 1997; Aronson et al., 1998, 2002a). Coral bleaching and disease outbreaks linked to anthropogenic climate change and land-based pollution have become widespread since the 1990s and 2000s, further decreasing coral cover (Aronson et al., 2002b).
Reef herbivores, including fish and urchins, play a key role in reef ecosystem health, resilience after disturbance, and maintenance of high coral cover (Hughes, 1994; Aronson and Precht, 2000; Mumby, 2006; Hughes et al., 2007; Adam et al., 2011, 2015; Steneck et al., 2014; Bozec et al., 2016). By consuming algal competitors, parrotfish (family Labridae) promote coral recruitment and reef resilience, complexity, and accretion (Hawkins and Roberts, 2004; Mumby, 2006; Adam et al., 2011, 2015; Steneck et al., 2014; Wild et al., 2014; Cramer et al., 2017). As large predatory reef fish became depleted from overfishing, parrotfish have become the next target and thus are routinely overfished (McManus et al., 2000; Comeros-Raynal et al., 2012; Edwards et al., 2014; Adam et al., 2015; Bozec et al., 2016). As a result, heavily fished reefs are dominated by small fish including parrotfish, damselfish, and juveniles of other species incapable of limiting macroalgal dominance (Hawkins and Roberts, 2004; Hughes et al., 2007; Edwards et al., 2014; Adam et al., 2015). Following the dieoff of the Diadema antillarum urchin in the early 1980s, parrotfish herbivory became more important for the maintenance of coral dominance on Caribbean reefs (Hay, 1984; Hay and Taylor, 1985; Carpenter, 1986; Sandin and McNamara, 2012; Adam et al., 2015).
Although these dramatic changes have been well-documented on Belizean reefs since the 1970s, humans have been interacting with and altering Caribbean reefs for millennia. Evidence from paleoecological and zooarcheological records offer insight into reef history before scientific monitoring and have provided evidence of prehistoric and historic overfishing. The analysis of fish bones from middens across several Caribbean islands suggests prehistoric human exploitation, beginning almost 2,000 years ago and continuing until European contact, decreased the size of reef fishes (Wing and Wing, 2001). Prehistoric and historic human fishing before 1800 heavily reduced Caribbean populations of large vertebrates, including turtles, manatee, and the now extinct Caribbean monk seal (Jackson, 1997). In Caribbean Panama, archaeological investigations showed prehistoric switching from conch and clams to less favorable molluscs and decreases in the trophic level of reef fishes consumed, suggesting overexploitation of preferred resources (Wake et al., 2013). Significant declines have also been shown in size at maturity of conch in the Caribbean, suggesting that the effects of prehistoric, subsistence harvesting began at least 1,500 years ago and intensified over the last 700 years; modern sizes continue to be smaller than pre-human exploitation conch (O’Dea et al., 2014). Changes in fossil coral and molluscan assemblages in this same region suggest that reef environmental degradation began by the 19th century, likely due to land-based runoff from agriculture (Cramer et al., 2012).
Reef sediment cores contain an abundance of continuously accumulating fossil material from diverse taxa, making them ideal for reconstructing quantitative, high-resolution trends in long-term reef ecosystem change (Lybolt et al., 2011; Roff et al., 2013; Clark et al., 2017; Cramer et al., 2017). The analysis of reef sediment cores collected from Caribbean Panama uncovered several long-term trends that illuminate some of the mechanisms of recent reef declines: (1) unprecedented dieoffs of Acropora corals occurred in tandem with the onset of industrialized agriculture, (2) parrotfish have a positive effect on reef accretion rates, (3) a tthe lack of recovery of the Diadema urchin may be linked with overfishing of predatory reef fishes, and (4)changes in reef sponge communities may be linked with the loss of sea turtles from historical overfishing (Aronson and Precht, 1997; Aronson et al., 2002a; Cramer et al., 2012, 2017, 2018; Łukowiak et al., 2018). Importantly, reef fish teeth fossils are readily preserved in reef matrix cores, making it possible to track past reef fish abundances and infer the impacts of historical fishing on reef ecosystems (Cramer et al., 2017).
Long-term trends in parrotfish on Belizean reefs and the link between parrotfish overexploitation and reef ecosystem change are unknown due to a lack of historical data on reef fish abundance and human use of reef resources. To assess the long-term influence of fishing on the structure and functioning of Belizean reefs, we tracked changes in reef fish abundance, reef growth rates, and human demographic and cultural dynamics from the prehistoric period to the present in southern Belize. We focused on Belize due to the availability of archeological and historical data on human dynamics and the significant economic, ecological, and cultural importance of the MAR.
We obtained a millennial-scale record of reef change from the analysis of fossil fish teeth and sediment accumulation rates within reef sediment cores collected from three reef sites within the southern lagoon of the Belizean MAR. This time series encompasses the period prior to and since intensive reliance on coral reefs for food and livelihoods in this region (Adams, 1985), allowing us to assess whether demographic and cultural shifts have contributed to reef ecosystem change. We focused specifically on parrotfish due to their role as keystone herbivores on reefs (Jackson et al., 2014), the robust, abundant, and identifiable nature of their (oral) teeth (Cramer et al., 2017), and the positive effect on reef ecosystem health (via reef accretion rates) they were found to have in another part of the Caribbean (Cramer et al., 2017). To pinpoint the precise timing of reef changes, we developed a high-precision chronology via uranium-thorium (U–Th) dating of coral skeletal fragments along the length of each of our cores. We combined records of ecological change from the reef sediment cores with historical and archeological information to assess the long-term coupling of human and reef ecosystem dynamics. We reconstructed human population trends when available, as this metric has been shown to be correlated with fishing pressure elsewhere in the Caribbean (Hardt, 2009). Our work provides a historical baseline for reef fish populations in this region as our quantitative data begins more than a millennia before underwater scientific monitoring efforts. This analysis suggests that European colonization resulted in cultural changes in this region which contributed to decreases in the abundance of parrotfish on neighboring reefs. Declines in this key herbivore group may have weakened Belize’s coral reef ecosystems and contributed to the degradation currently observed two centuries later.
History of human dynamics and use of reef resources
Human use of reef resources in Belize has likely been occurring for at least four millennia, the minimum estimate for the period of continuous human habitation (Jones, 1994; Lohse et al., 2006). Belize has been part of the heartland of the Maya empire throughout its four major periods: (a) the Preclassic (2000 BC–25 AD), (b) the Classic (25–900 AD) characterized by the height of population density and social complexity, (c) the Postclassic (900–1550 AD) characterized by overexploitation of terrestrial resources, societal disruption, and population declines, and (d) post-Spanish arrival from 1528 to present characterized by accelerated societal disruption, population collapse, and increased exploitation of coastal resources (Figure 2). Although most major Maya archeological sites are located inland and show heavy reliance on terrestrial resources, including large-scale domesticated plant production (White et al., 1993; Kunen, 2001; Chase and Chase, 2016), coastal sites are likely underrepresented due to submergence following sea level rise (McKillop, 2009, 2017).
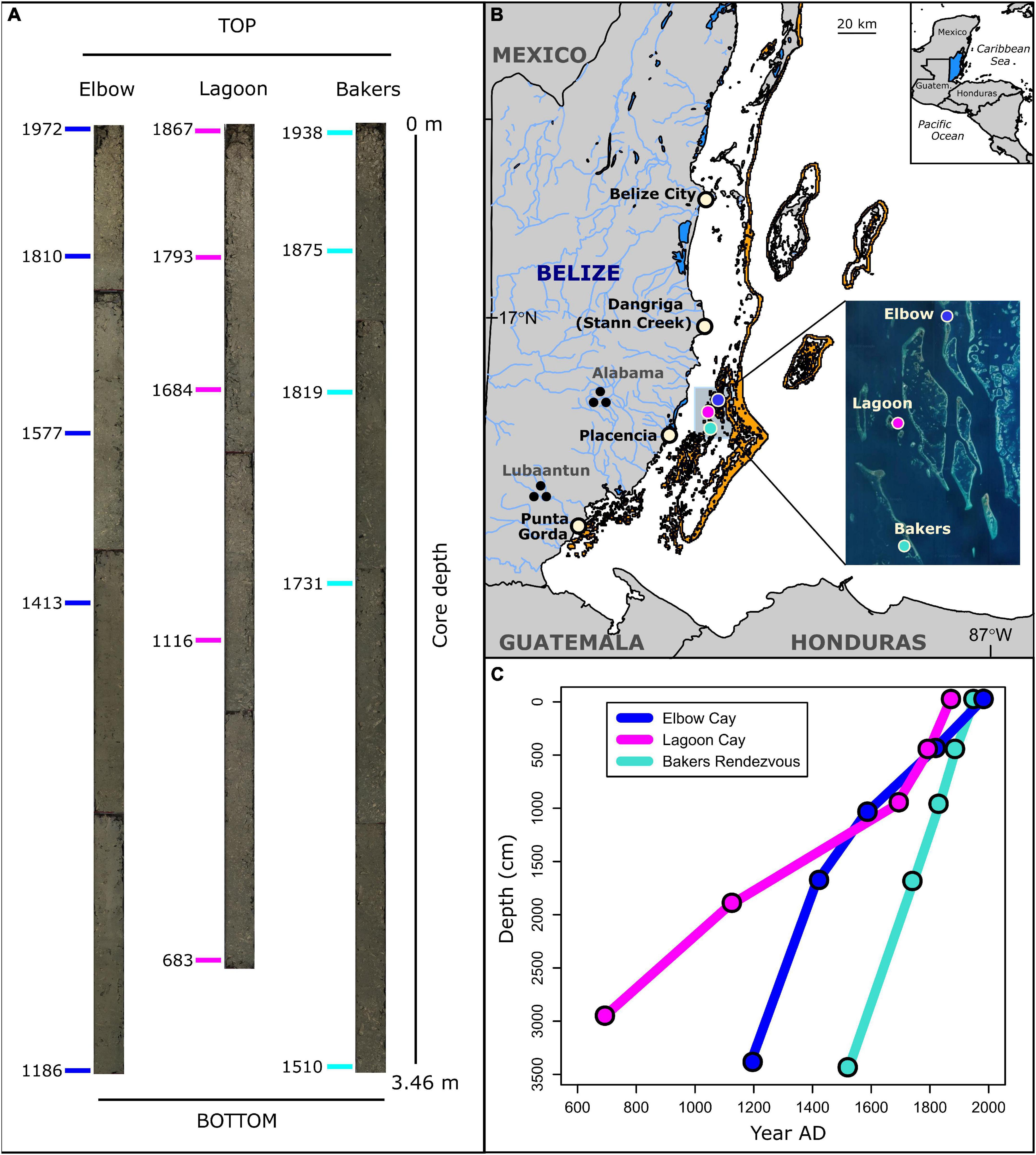
Figure 1. (A) Reef matrix cores and U–Th dates (n = 15) obtained (in year AD) along length of cores. Bakers = Bakers Rendezvous, Elbow = Elbow Cay, Lagoon = Lagoon Cay. (B) Map of coring locations (colored circles), shallow reef habitat (orange areas), prehistoric and modern human population centers, and rivers in Belize. Inset: Google Earth. (C) Age-depth plot showing reef accretion trends.
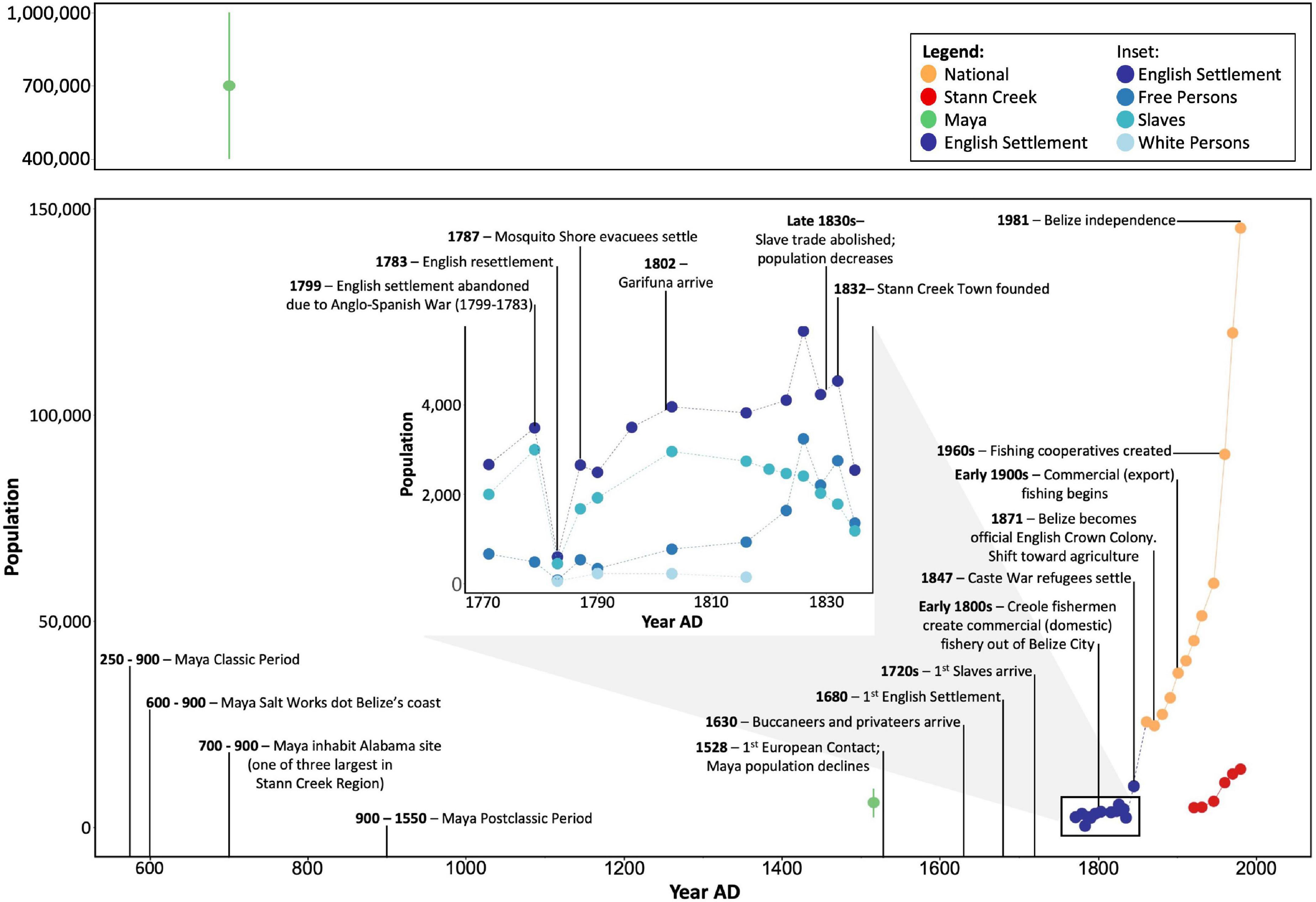
Figure 2. Human population and demographic trends and timeline of major events in Belize with relevance to human utilization of coral reef resources and reef ecosystem functioning and over the past ∼1,300 years. Points are estimates of population subsets within Belize; these numbers are not necessarily directly comparable across groups due to differing survey methods. Error bars represent lower and upper estimates, points represent means or single available estimates. Sources and methods of computing human population estimates are provided in Supplementary Table 1.
Coastal sites, including salt works and the nearby inland city, Alabama, show that Classic and Postclassic Period Maya inhabited and utilized resources from the coastal zone of southern Belize that is hydrologically connected to our reef coring sites (Figure 1B; Sills, 2016; McKillop and Aoyama, 2018; Peuramaki-Brown et al., 2020). During this time, Maya exploited estuarine and marine resources including manatee, conch, jacks, grouper, snook, and parrotfish and transported them via river networks to engage in trade with inland cities (Wing, 1975; McKillop, 2017; Peuramaki-Brown et al., 2020). Zooarchaeological data from coastal sites suggest a preference for shellfish, manatee, and terrestrial food sources over reef fish (McKillop, 1984; Williams et al., 2009; Simmons and Graham, 2016).
The arrival of Europeans in 1528 AD resulted in massive demographic and cultural changes and an increase in the exploitation of coastal marine resources across the New World (McClenachan and Kittinger, 2013). The introduction of novel human pathogens and the killing and enslavement of native peoples resulted in an estimated 50–90% loss of the Maya population (Bolland, 1973; Graham et al., 1989; Pendergast, 1993). By the 17th century, coastal Belize contained growing communities of European buccaneers, privateers, and pirates who utilized the cays and lagoons as bases from which to loot Spanish ships transporting goods on their established trade routes (Dampier, 1697; Camille, 1996a; Hanna, 2015). Though this coastal-dwelling group likely never numbered more than a hundred, they heavily exploited coral reef resources, focusing primarily on turtle and manatee and to a lesser extent on reef fish (including parrotfish; Dampier, 1697; Adams, 1985; Bentley et al., 2016).
The 18th century establishment and expansion of an English settlement to exploit Belize’s coastal hardwoods likely further increased reef resource utilization; Spanish territorial claims to the region resulted in a prohibition on English settlers (“Baymen”) pursuing large-scale agriculture or plantations, creating a reliance on local seafood as well as imports from Jamaica and other English holdings (Bolland, 1973). Baymen preference for turtles and manatee is well documented (Craig, 1966; Adams, 1985; Bentley et al., 2016). Belize’s population increased in the early 18th century as intensified logging led to an influx of slaves from other English colonies and Africa to support inland exploration beyond the denuded coastline (Joseph, 1974). This trend continued until the onset of the Anglo-Spanish War (1799–1783), during which Baymen and their slaves temporarily fled Belize. After the Spanish-English peace treaty in 1783, many Baymen returned with their slaves and laid claim to land well beyond the legal borders of the English settlement (Bolland, 1973). The tenuous legal status of these settlements on Spanish-claimed land continued to prevent conspicuous agricultural activities (Bolland, 1973), necessitating reliance on local seafood.
Throughout the 18th century, Belize’s small, wealthy population of English settlers controlled a lucrative import trade while slaves and the poor relied on subsistence fishing on coral reefs and/or illegal subsistence farming for survival (Bolland, 1973; Johnson, 2003). As a result, in 1787 when over 2,000 evacuees from the Mosquito Shore of Honduras fled to Belize, they faced a dearth of unclaimed land and food shortages (Bolland, 1973). Although the Spanish eventually permitted the cultivation of subsistence gardens in 1789 to support the growing population, many new settlers chose to focus on subsistence fishing (Bolland, 1973). By the 1861 census, many fishermen inhabited Belize’s cays (Craig, 1966; Camille, 1996b) and by the early 19th century, “Creole” (people of mixed African and European ancestry) fishermen in Belize City had expanded their activities to create a commercial fishery for domestic consumption (Craig, 1966).
Reliance on coral reef resources likely accelerated in the 1800s with the arrival of the Garifuna (descendants of escaped African slaves and indigenous people from St. Vincent Island) who formed the first recognized coastal settlement in southern Belize (Bolland, 1986; Moberg, 1992; Woods et al., 1997). Numbering no more than 300 in the early part of the 19th century (Camille, 1996b), the Garifuna are traditionally subsistence farmers and fishermen (Craig, 1966; Adams, 1985). Though initially pressured to work in the mahogany industry, the Garifuna spent off-seasons fishing and turtling (Camille, 1996b; Woods et al., 1997; Taylor, 2013); by 1823, Garifuna agriculture and fishing even supplied food to the main settlement in Belize City (Camille, 1996b; Woods et al., 1997). By the 1861 census, over 2,000 Garifuna had settled in Belize, with 80% living in coastal settlements in southern Belize (Camille, 1996b) and relying almost entirely on marine sources for meat, specifically fish and manatee (Conzemius, 1928; Craig, 1966). Garifuna have traditionally had Belize’s highest per-capita consumption of fish (Adams, 1985).
Population increases and economic changes during the 19th and 20th centuries further affected coastal resource utilization in Belize. In the mid-19th century, refugees fleeing the Caste War in the Yucatan began settling in northern Belize, doubling the English settlement’s population and introducing farming, cattle ranching, and large-scale agriculture to the import-dominated economy (Camille, 1996b; Woods et al., 1997). This new mestizo population engaged in fishing in the north and off Belize’s cays (Craig, 1966; Adams, 1985; Sutherland, 1996). Belize’s immigrant population further increased in the 1880s–1890s with the immigration of Mopan and Q’eqchi Maya from Guatemala and Mexico into Southern Belize (Woods et al., 1997). In the early 1900s, commercial fishing expanded in Belize, with local fishing cooperatives formed by the 1960s (Adams, 1985; Jackson et al., 2014). Despite population influxes, coastal development remained low; 98% of Belize’s coastal mangroves remained intact until the 1990s (Murray et al., 2003; Cherrington et al., 2010). By the 1990s, “fishing down the food web”(Pauly et al., 1998) was apparent on Belizean reefs, with declines in parrotfish abundance lagging the depletion of higher trophic level fishes such as groupers and snappers (Mumby et al., 2012). At this time, Belize shifted its economy from agriculture and fishing and toward ecotourism, resulting in increased coastal development and demand for local seafood (Sutherland, 1996; Gibson et al., 1998; Key, 2002).
Materials and methods
Collection of reef sediment cores
We collected sediment cores from 5 to 8 m water depth at three reef sites within the central lagoon of the Belize Barrier Reef in 2014: Bakers Rendezvous, Lagoon Cay, and Elbow Cay (Figure 1). These sites were selected because they were previously cored by Aronson et al. (2002a) and shown to have sufficiently high sedimentation rates, allowing for a relatively high-resolution record of reef ecosystem change. Cores were collected from the outer slope of diamond-shaped reefs, termed “rhomboid shoals,” that characterize this portion of the Belizean shelf lagoon. These shoals have an atoll-like configuration that reach sea level and contain a sediment-filled basin up to 30 m in depth (Macintyre and Aronson, 1997; Aronson et al., 2002a). Before the late 1980s, the outer flanks of the rhomboid shoals between 2 and 15 m depth were covered by living populations of the architecturally complex, competitive staghorn coral, Acropora cervicornis, and by the low-relief weedy coral, Agaricia tenuifolia, below 15 m (Shinn et al., 1979; Aronson and Precht, 1997). During the 1980s, WBD nearly eliminated the Acropora cervicornis populations on the rhomboid shoals. At the time of coring in 2013, the reef slope zones were composed of A. cervicornis rubble interspersed with A. tenuifolia rubble and thickets of living A. tenuifolia.
Sediment core collection and processing was conducted as described in Cramer et al. (2017). Using a diver-operated push core technique, an aluminum core tube was driven into rubble areas immediately adjacent to living reef at 5–8 m water depth (Cramer et al., 2017). Cores were sliced lengthwise and samples were obtained from 5 cm samples sectioned from one half of the core. The other core half was archived within the Scripps Institution of Oceanography Geological Collections. To provide a higher-resolution record of recent change and encompass millennial timescales, each sample from the top 1 m of each core was analyzed and every third sample from the rest of the core was sampled (Figure 1; Cramer et al., 2017).
Reconstructing long-term change in reef fish communities and reef accretion rates
Utilizing an established protocol for the isolation and quantification of fish teeth fossils (Sibert et al., 2017), sediment samples were washed, sieved, and separated into five size fractions (63–104 μm, 104–250 μm, 250–500 μm, 500 μm–2 mm, >2 mm) to aid in tooth counting. Samples smaller than 250 μm were acidified in 5–10% acetic acid to remove carbonates. The 63–104 μm size fraction was dyed using an Alizarin Red S solution described in Sibert et al. (2017) which turns fish teeth pink and makes them more easily distinguished from the siliceous sponge spicules and non-carbonate grains that constitute the acid-insoluble portion of these samples (Sibert et al., 2017). Samples were then inspected using a dissecting microscope to locate, taxonomically identify, and count fish teeth. Parrotfish teeth were identified via a reference collection for Caribbean reef fish teeth that was compiled from samples housed within the Smithsonian Institution’s National Museum of Natural History (Cramer et al., 2017).
A high-resolution chronology of reef ecosystem change was obtained by U–Th dating coral fragments within the cores following the methods outlined in Cramer et al. (2017). U–Th dating was conducted in the Radiogenic Isotope Facility at University of Queensland, following chemical treatment procedures and MC-ICP-MS analytical protocols described in Clark et al. (2012, 2017). For each core, a highly constrained chronology was produced where four 230Th ages were obtained at approximately 0.5 m intervals in the top 2 m portion of the core and one age from the bottom of the core, yielding five ages per core (Figure 1). From these cores, accretion rates (mm sediment accumulated per year) were estimated using linear interpolation between each pair of ages.
Reconstructing long-term change in human dynamics
Human population abundance was reconstructed using multiple sources. The “Census History Timeline” on the Statistical Institute of Belize’s official website provided raw numbers for partial censuses conducted decadally between 1790 and 1911 (Statistical Institute of Belize, 2022a). Population numbers given in primary sources, including letters between the Belize settlement’s ruling authorities and those in England, provided in Bolland (1973), were used to represent the population and demographics of the settlement before the official census in 1790, and to supplement years in which there was data given but no official census (Figure 2 and Supplementary Table 1). Population estimates and demographic information and percentages similarly based on primary sources and given by Bolland (1973) and Bolland (1994) were also used to calculate the population and demographics of the settlement during this time (Figure 2 and Supplementary Tables 1, 2). A population estimate for 1845 was also taken from Woods et al. (1997). However, these estimates do not include Maya or Garifuna people, and therefore significantly underestimate the total population. Population data from 1921 to 2000 at the district and national level were obtained from comprehensive decadal censuses carried out by the Belizean government and available from the Statistical Institute of Belize (2022b). Due to incomplete response rates, these are likely underestimates as well. To reconstruct the timeline of Belize’s history, demographic changes, and resource utilization, we synthesized peer-reviewed articles and books on the prehistory and history of Belize. Due to inconsistent sampling methodologies and geographic and demographic scopes of population estimates through time, these values are not directly comparable across time periods. We therefore interpret these as rough estimates and are not able to conduct quantitative analyses relating population trends to parrotfish abundance metrics or reef accretion rates.
Assessing change in reef ecosystems and human dynamics
Trends in parrotfish teeth abundance metrics, reef accretion rates, and human population were visually assessed by plotting raw values for each sample or time period. Trends in parrotfish teeth and reef accretion rates were qualitatively assessed using non-parametric locally weighted regression (‘loess’)-smoothed trendlines (Cleveland and Devlin, 1988). The loess smoothing parameter was set to 0.5 for detection of high magnitude changes across the full time series as well as shorter-term trends.
To assess the presence and direction of causal relationships between parrotfish relative abundance and reef accretion rates, we used convergent cross mapping (CCM), a technique that compares the ability of time-lagged components of one process to estimate the dynamics of another. CCM tests for significant causal relationships by recognizing that the observed values of a forcing process should be significantly better explained by observed values of a response process than expected by chance, and the accuracy of that explanation should improve with increasing time series length (Clark et al., 2015; Ye et al., 2015). We used a variant of CCM, multispatial CCM, which is appropriate for spatially replicated time series that individually contain short series (∼≤30) of sequential ecological observations of systems that share similar dynamics, such as samples from our three individual cores. We followed the CCM algorithm outlined in Cramer et al. (2017) to confirm that predictive skill (Pearson’s correlation coefficient) increased with greater number of historical observations (here, number of samples from a single core). This algorithm employed a bootstrapping with replacement procedure with 500 iterations to (a) leverage spatial information to reshuffle the order of spatial replicates and calculate the correlation coefficient and (b) test whether predictions indicated a significant causal relationship by determining whether the calculated correlation coefficient was significantly greater than zero and whether it increased significantly with sample size. A causal relationship was deemed significant if its corresponding P-value was <0.1, a threshold commonly used to increase the power of detecting trends with smaller sample sizes. To reduce the potential volatility in the relative abundance of parrotfish teeth due to low total tooth numbers, we conducted a separate CCM as above after excluding samples with less than 30 teeth. All statistical analyses were conducted using the R software package version 3.5.1; causality analyses were conducted using the ‘Multispatial CCM’ package (Clark et al., 2015).
Results
Reef matrix composition and U–Th chronology
The reef matrix cores ranged from 5.5 to 5.3 m in length when extracted and 3.5–3.0 m in length after settling and compaction and were primarily composed of a dense matrix of coral skeleton and mollusk shell fragments (>2 mm) within a sandy-muddy matrix of carbonate grains (Figure 1A). The total number of fish teeth within each sample ranged from 4 to 136 (mean = 46), the number of parrotfish teeth ranged from 0 to 70 (mean = 17), and the proportion of teeth from parrotfish ranged from 0 to 0.65 (mean = 0.36). On average, post-coring settlement reduced core lengths by 2 m, or to 64% of their original length. A total of 15 230Th ages obtained from all three cores reveal that these cores span the period 683 ± 10 AD to 1972 ± 2.4 AD. Corrected 230Th age errors (2σ) range between 2 and 12 years (see Supplementary Table 3). Within each core, all five 230Th ages are in chronological order, confirming minimal vertical mixing of larger grain reef sediments. Because we cored dead rubble zones adjacent to living reef, 230Th ages from the top of three cores are not modern day. The core from Lagoon Cay has the lowest reef accretion rates (4.6 mm/year before core compaction and from 1.6 to 6.4 mm/year after compaction) and represents the prehistoric to early industrial period (683–1867 AD), the core from Elbow Cay has intermediate accretion rates (7.0 mm/year before compaction and from 2.6 to 7.6 mm/year after compaction) and spans the prehistoric to modern period (1186–1972 AD), and the core from Bakers Rendezvous has the highest accretion rates (12.4 mm/year before compaction and from 7.4 to 9.3 mm/year after compaction) and begins just before European contact and spans through the early industrial period (1510–1938 AD; Figure 1C). Because U–Th dates were obtained from cores post-compaction, it is not possible to determine pre-compaction accretion rates from individual samples along the core length or to determine pre-compaction temporal trends in accretion rates.
Trends in reef accretion and parrotfish abundance
Reef accretion rates and proxies of parrotfish abundance show varying long-term trends across the three reef sites. Bakers Rendezvous has the highest reef accretion rate that peaks in the early 19th century then declines until the core top in 1938 AD (Figure 3A). Elbow Cay has an intermediate accretion rate that declines circa 1400 AD until the core top in 1972 AD. Lagoon Cay has the lowest accretion rate that begins to increase circa 1660 AD through the core top in 1867 AD. Across cores, parrotfish teeth abundance accumulation rates (AAR) generally correspond to relative reef accretion rates, with Bakers Rendezvous having the highest and Lagoon Cay the lowest values throughout the majority of their records (Figure 1B). Increases and decreases in parrotfish teeth AAR generally track trends in reef accretion, at least in part due to the similar way in which these metrics are calculated (they both contain number of years encompassed within a sample in their denominator). One exception is at Lagoon Cay, where, despite increases in reef accretion circa 1700 AD, parrotfish teeth AAR declines approximately one century later (Figure 3B).
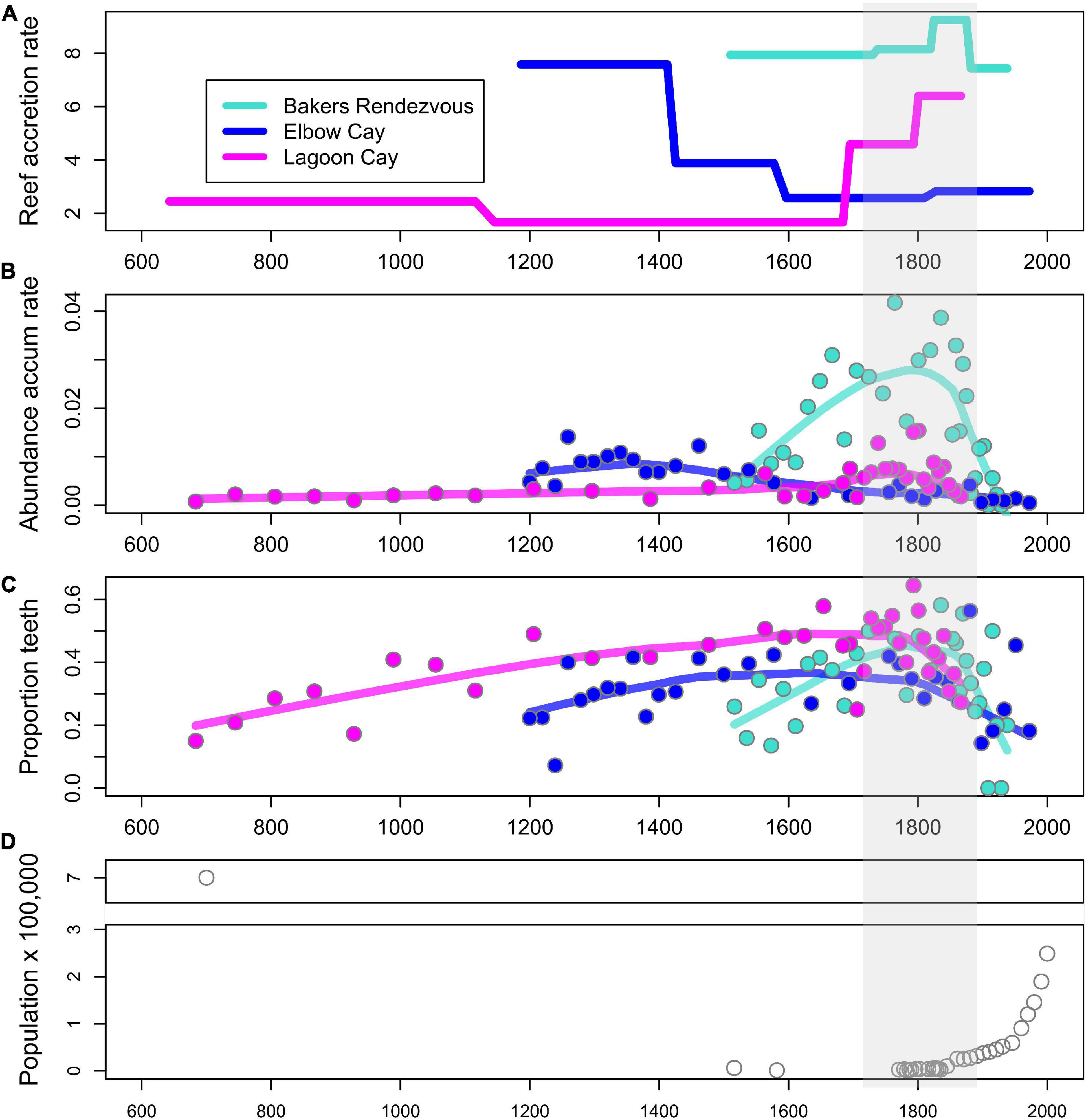
Figure 3. Reef accretion rate, two metrics of parrotfish abundance, and human population trends over the past ∼1,300 years. (A) Reef accretion rate (mm of core thickness per year). (B) Abundance accumulation rate of parrotfish teeth (number of teeth/dry weight of sediment × number of years encompassed in a sample). (C) Relative abundance of parrotfish (proportion of all fish teeth that are from parrotfish). (D) Human population trends. Shaded region indicates period of initial declines in proportion of teeth that are parrotfish (C).
In contrast to parrotfish teeth accumulation rates, the proportion of teeth fossils belonging to parrotfish is relatively consistent across sites, indicating that parrotfish comprise a similar proportion of the total reef fish community at all three reefs despite their varying accretion rates (Figure 3C). Trends in this proxy of parrotfish relative abundance are also consistent across sites, with each site showing a peak in this metric within the middle portion of each core (between approximately 1700–1800 AD) and subsequent declines (Figure 3C).
Link between reef accretion and parrotfish abundance
The convergent cross mapping causality analysis revealed a unidirectional causal relationship between one of our proxies of parrotfish abundance and reef accretion rates. The relative abundance of parrotfish teeth had a significant positive causal effect on reef accretion rates, but the reverse was not true—reef accretion rates did not causally affect the relative abundance of parrotfish (Figure 4). This result was consistent when samples with less than 30 total fish teeth were excluded (Supplementary Figure 1). Therefore, declines in parrotfish are at least one of the causes of the observed declines in reef accretion rates that occurred at two of the reef coring sites, Bakers Rendezvous and Elbow Cay (Figure 3A).
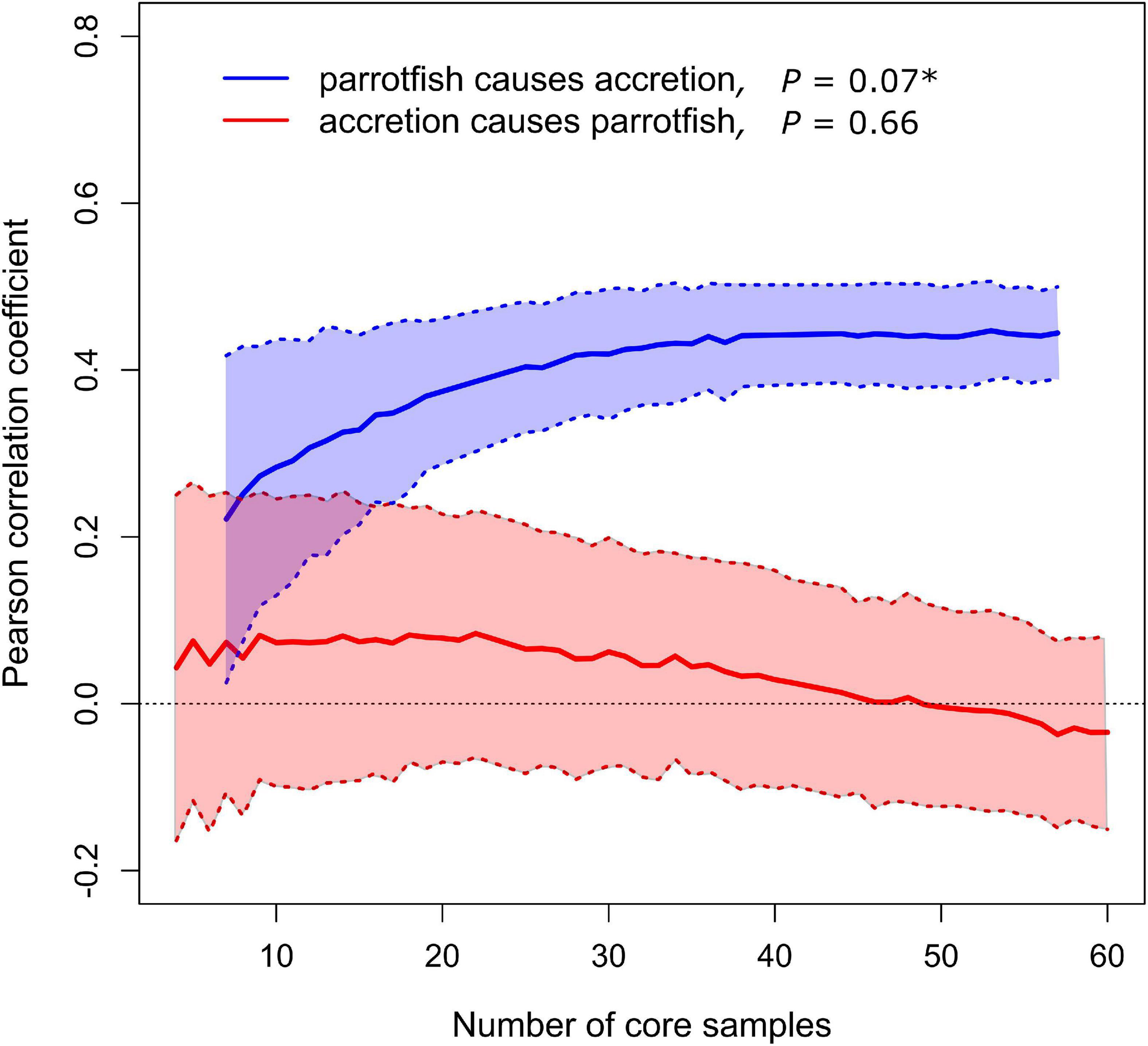
Figure 4. Causal relationships between parrotfish relative abundance and reef accretion rate. Lines and shaded regions show mean ± standard deviation from 500 bootstrapped iterations. Significant causal forcing (*) determined from bootstrap test with 500 iterations and indicated when the Pearson’s correlation coefficient is significantly greater than zero for larger sample sizes (number of core samples, including all spatial replicates in the composite time series), and when correlation coefficient increases significantly with increasing number of core samples.
Link between reef and human dynamics
Trends in parrotfish abundance proxies at our reef sites were not clearly related to our semi-quantitative proxy of human alteration of reef ecosystems: national trends in human population. While declines in parrotfish tooth accumulation rates were initiated in the 1700s–1800s depending on site, human population was in decline during this period following European contact, and the population was consistently low from 1800 to 1950 (Figure 3D). By the onset of exponential population growth in Belize in the 1950s, both proxies of parrotfish abundance were already much reduced compared to their peak abundances (Figures 3B–D).
Discussion
The past millennium of human history in Belize is characterized by massive changes in human dynamics that may have affected parrotfish abundances. The reef matrix core record begins during the height of Maya civilization (Classic Period) in 683 AD and spans several major transitions before it ends in 1972 AD: (1) European colonization in the 16th century and subsequent Maya depopulation and influx of European settlers and other groups, (2) accelerated and often unsustainable terrestrial and marine resource extraction in the 17th–20th centuries, and (3) exponential population growth, coastal development, commercialization of marine resources, and anthropogenic climate change beginning in the 20th century. The two proxies of parrotfish abundance from tooth fossils show varying trends, suggesting a combination of human and environmental factors may be responsible. While parrotfish tooth abundance accumulation rates closely track reef accretion rates which vary across sites (Figure 3B), the relative abundance of parrotfish teeth peaks near the turn of the 19th century at all three sites (Figure 3C) and coincides with a period of increased utilization of reef resources. Below we investigate the most likely environmental and anthropogenic drivers of millennial-scale change in parrotfish abundance.
Possible environmental drivers of parrotfish abundance
Overfishing is currently the primary threat to parrotfish populations worldwide (Comeros-Raynal et al., 2012; Jackson et al., 2014) and likely played a role in the historical declines observed in this study. However, reef environmental change may also have contributed to parrotfish trends, which we assess here.
Paleoclimatic fluctuations may have affected parrotfish populations in Belize via storm and/or temperature-driven disturbances. Three major climate anomalies occurred in the Caribbean during the period encompassed by the core record: (1) the Medieval Warm Period (roughly spanning themid-10th to mid-15th centuries) characterized by warmer sea surface temperatures and higher tropical cyclone (hurricane) activity (Gischler et al., 2008; Mann et al., 2009), (2) the Little Ice Age (roughly spanning the mid-15th to late 19th century) characterized by cooler sea surface temperatures and drier conditions with lower hurricane activity (Mann et al., 1998; Watanabe et al., 2001; Gischler et al., 2008; Denommee et al., 2014), and (3) anthropogenic warming and increased hurricane activity beginning in the 19th century (Gischler et al., 2008; Abram et al., 2016). Higher hurricane activity could negatively impact parrotfish abundance via destruction of coral habitat or positively increase parrotfish abundance via increased macroalgal growth following coral mortality from storm damage. However, these effects would likely be transitory (Kaufman, 1983; Mumby et al., 2021) and parrotfishes are typically insensitive or even positively affected by climate-induced habitat degradation, including from storms and bleaching (Adam et al., 2011; Emslie and Pratchett, 2018; Taylor et al., 2019). Sea surface temperature extremes in either direction could negatively impact parrotfish abundance if they exceeded physiological tolerance limits, but no empirical data have yet been collected on physiological responses of parrotfishes to temperature change (Hoey et al., 2018).
Neither proxy of parrotfish abundance appears to correspond to the above paleoclimatic changes in an easily discernible manner. During the period of more intense hurricane activity and sea surface temperatures in Belize (throughout most of the period spanning the 7th–14th centuries), both metrics of parrotfish abundance were increasing (except for abundance accumulation rate in the Elbow Cay site; Figures 3B,C). These metrics continued to increase, peaked, and then declined during the Little Ice Age, a period of low hurricane activity and sea surface temperature (Figures 3B,C). These declines in parrotfish abundance proxies were initiated a century before anthropogenic warming and increased hurricane activity in the late 19th century (Denommee et al., 2014; Donnelly et al., 2015). In addition, our core records lack any age reversals, indicating minimal vertical mixing from heavy wave or storm action (Figure 1A), and no storm layers are visible in the cores (Figure 1B).
Land-based sedimentation from anthropogenic land alteration could also have affected parrotfish abundances via degradation of coral habitat and changes in algal abundance (Fabricius, 2005; Jackson et al., 2014; Mumby et al., 2021). Although widespread deforestation in Belize occurred during the Maya Late Classic Period (550–900 AD), parrotfish abundance proxies were stable or increasing during this time (Figures 3B,C; Jacob, 1995; Beach et al., 2006). Forests in this region increased from this time until European colonization in the 16th century (Mueller et al., 2010), and high levels of soil erosion did not begin again until the 20th century (Beach et al., 2006, 2018). Following European contact, coastal harvesting of logwood and mahogany dominated Belize’s economy through the 1800s (Joseph, 1974; Camille, 1996a; Peedle, 1999). However, legal extraction was concentrated in Northern Belize and was selective in nature (Bolland, 1973; Joseph, 1974), making historical deforestation an unlikely factor in parrotfish declines in the more southern Stann Creek area where our cores were collected. Most of the sediments and nutrients delivered by watersheds along the Mesoamerican Reef originate in Honduras, which has undergone widespread land-use changes over the past century due to agriculture (Harborne et al., 2001; Burke and Sugg, 2006; Leonard, 2011). However, declines in parrotfish teeth predate industrial agricultural activities in Honduras by 1–2 centuries, making this an unlikely driver. Coastal mangrove deforestation in Belize is also unlikely to be responsible for parrotfish declines initiated in the 18th and 19th centuries, as Belize maintained an estimated 98% of its original mangrove cover until the 1990s and the Stann Creek region did not experience significant mangrove loss until the 1980s (Murray et al., 2003; Cherrington et al., 2010).
Possible link between fishing and parrotfish abundance
Although dramatic human population fluctuations occurred during the period encompassed in the core record, these trends are asynchronous with those observed in the parrotfish abundance records from our cores, indicating that cultural and demographic changes may have had a more significant influence on levels of reef fish exploitation than absolute population numbers. The timing and direction of parrotfish abundance trends from the 7th to 16th centuries and zooarcheological data on Maya food preferences suggest that observed declines in parrotfish abundance were not related to Maya use of reef resources. First, both the accumulation rate and relative abundance of parrotfish teeth were increasing or at high levels within the two reef cores that encompass the Maya Classic and Postclassic periods (Figures 3B,C). Second, although Maya have utilized marine resources throughout their habitation of Belize, data from middens reveal that coastal Maya focused on manatee and shellfish more than reef fish and that parrotfish were not a preferred food fish (McKillop, 1984; Simmons and Graham, 2016). The inland Classic Period site of Lubaantun is notable for an unusually high usage of reef fish but this preference is specifically for jacks, not parrotfish (Wing, 1975). In modern Belize, Maya continue to show a low preference for marine fish (Adams, 1985). Although Maya could be expected to shift their exploitation toward lower trophic level species like parrotfish if their preferred food choices became scarce, at the time of European arrival, manatee, turtle, and shellfish were still relatively abundant (Dampier, 1697; McKillop, 1985; Bentley et al., 2016). Last, the timing of initial parrotfish declines in the 1700s–1800s occurred over a century after the decimation of Maya populations from disease, war, and subjugation following European colonization. This contrasts with insular areas of the Caribbean which show declines in parrotfish population and size resulting from Pre-Columbian indigenous fishing (Wing and Wing, 2001), possibly reflecting the greater reliance of island populations on reef fish.
In contrast, the declines in parrotfish abundance proxies that were initiated in the 18th and 19th centuries may have been caused by the shift from lower-effort Maya reef fishing to higher-intensity reef fishing by European colonists and subsequent influxes of immigrant groups brought to Belize voluntarily and by force. English buccaneers inhabited and fished from cays in the Stann Creek District where our reef coring sites are located (Joseph, 1974). In addition, the inequitable economic structure of the English settlement allowed the moneyed elite to afford imported goods from England and its colonial holdings while requiring the remainder of the settlement’s population to rely exclusively on the local production of food including subsistence fishing. Population increases within southern Belize in the 19th century could also have contributed to parrotfish declines observed during this period. The settlement, founding, and growth of Stann Creek Town (now Dangriga), the closest population center to the lagoonal reefs from which our cores were collected (Figure 1B), occurred in the early 1800s by Garifuna settlers. Although the Garifuna population in Belize numbered less than 300 in the early 1800s, it grew to over 2,000 by 1861 AD (Camille, 1996a). Despite their modest population size, the Garifuna relied strongly on marine resources, including reef fish, for nutrition and livelihood, engaging in small-scale commercial fishing to supply food to the settlement at Belize City (Conzemius, 1928). The founding of the Creole fishing village Placencia in the late 1800s may also have played a role in observed continued declines in parrotfish abundance (Bolland, 1988; Key, 2002). Small human populations with low effort and artisanal fishing gear have been shown to cause significant declines in fish populations globally (Pinnegar and Engelhard, 2008), particularly in the Caribbean (Coblentz, 1997; Hawkins and Roberts, 2004; Hardt, 2009). In post-industrial era Belize, parrotfish became a preferred food fish after larger fishes, such as groupers and snappers, became depleted over the past 50 years (Koslow et al., 1994; Mumby et al., 2012). However, parrotfish declines may have occurred prior to declines in higher trophic level species due to their use as bait in traps (Paz and Truly, 2007; Box and Canty, 2011). Last, historical parrotfish declines could have also been due to indirect fishing effects-declines in top predators such as larger groupers and snappers from overfishing has increased parrotfish predation by meso-predators and resulted in parrotfish declines on modern reefs in Belize (Mumby et al., 2012).
Although declines in parrotfish abundance beginning in the 18th century appear to coincide with the intensification of reef resource use, earlier trends in parrotfish abundance do not have any clear relationship with contemporary human dynamics. Parrotfish teeth accumulation rate is tightly linked to trends in reef accretion at all three reef sites (Figures 3A,B). This could be a result of more quickly growing reefs supporting more favorable habitat for parrotfish (which could explain the exceptionally high tooth abundance accumulation rates at the fast-growing reef at Bakers Rendezvous), the mathematical relationship between tooth accumulation rates and accretion rates (both have in their denominators the number of years represented in a sample, preventing a formal statistical analysis such as CCM), or both. Although the causality analysis found that reef accretion did not causally drive the relative abundance of parrotfish teeth (Figure 4), reef growth rate could affect the absolute number of parrotfish without affecting the relative abundance if it resulted in similar increases or decreases in multiple fish taxa. Similarly, changes in the abundance of teeth from other reef fish taxa in response to changes in reef environment and predation pressure could affect the relative abundance of parrotfish teeth. Last, the increases in parrotfish relative abundance observed in the bottom half of the cores across all three reef sites could be related to the early establishment of coral reef habitat as sea level changes altered the hydrology of the Mesoamerican Reef lagoon, similar to trends observed within reef sediment cores collected in Panama (Cramer et al., 2017). These factors were not explored in this study.
Nuances with interpreting the reef matrix core record
Factors associated with the preservation, accumulation, and dating of reef sediments and fish teeth fossils could have affected our results. Ages and accretion rates could be affected by uncertainties in age model estimation, possibly caused by linear interpolations between samples with dated coral pieces and/or uncertainties in the precise core depth of individual samples from sediment compaction during or after the coring process (on average, post-coring settlement reduced core lengths by 2 m, or to 64% of their original length). Linear interpolations also resulted in constant accretion rates for samples occurring between successive U/Th dates, which could conceivably have hindered our ability to detect causal effects between accretion rate and the relative abundance of parrotfish teeth. However, this did not prevent the detection of a causal effect of parrotfish relative abundance on accretion rates.
Despite similar geomorphologies and water depths and the close proximity (5–10 km) of our three coring sites, the magnitude and temporal trajectory of reef accretion rates varied notably across sites (Figures 1C,3A). For instance, the Bakers Rendezvous core shows relatively rapid and consistent accretion rates, the Elbow Cay core shows initial rapid accretion followed by gradually decreasing accretion rates beginning ∼1400 AD, and the Lagoon Cay core shows relatively low accretion rates that increased dramatically beginning ∼1660 AD (Figure 3A). These contrasting accretion histories could be related to their specific local coral growth and sediment deposition environment, including the developmental history of the adjacent reef flat that determines sediment supply to the reef slope habitat from which cores were collected. However, initial geological studies of these three sites have described similar mechanisms of formation and coral community composition for these rhomboid shoals (Macintyre and Aronson, 1997; Aronson et al., 2002a), precluding a conclusive explanation for the observed differences in reef accretion.
Although reef accretion rates were used as a proxy of coral growth, non-coral carbonate producing organisms also contributed to sediment production. However, in a similar set of reef matrix cores our team collected from Caribbean Panama, 83% of the weight of the undigested sediments >500 μm is made up of coral (Cramer et al., 2017). Accretion rates could also have been affected by the degree of bioerosion or physical erosion which could create finer sediments that might be more easily removed from the reef matrix by current or wave action. Accretion rates were in fact positively related to the proportional weight of the smallest grain size fraction (less than 104 μm), indicating that erosional forces (including bioerosion caused by parrotfish feeding) influence reef accretion rates (Supplementary Figure 2). Similarly, declines in reef growth and architectural complexity could result in less baffling of sediments between the coral fragments comprising the reef framework, possibly affecting the total abundance of smaller subfossils such as fish teeth. However, these changes would not be expected to significantly affect the taxonomic composition of fish teeth, as fish taxa contain teeth of similar size. Smaller grains <2 mm (including fish teeth) could have been winnowed through cores via bioturbation or the coring process, moving them deeper along the core length than larger coeval particles (corals), leading to mismatch in ages between corals and smaller fossils. However, the significant causal relationship between reef accretion rates (computed from ages of coral fragments >2 mm in size) and parrotfish teeth relative abundance indicates minimal vertical reworking or winnowing occurred. Finally, the fossil tooth record likely overemphasizes the contribution of parrotfish to the total living reef fish community due to the dentition of many parrotfish species including a multi-element tooth battery and scraping and excavating feeding behaviors causing high tooth turnover rates. However, these factors would not be expected to vary significantly over time.
Conservation implications
Our results confirm the positive effect of parrotfish on coral reef ecosystem functioning via enhancing overall reef accretion, demonstrating this trend in lagoonal reefs across two regions of the Caribbean (Cramer et al., 2017). They also indicate that long-term losses of parrotfish likely resulting from historical and modern overfishing have resulted in declines in the resilience of lagoonal reefs in Belize via two mechanisms. First, the decline in reef accretion rates has reduced the capacity of these reefs to keep up with sea level rise from anthropogenic climate change. Second, the loss of parrotfish herbivory increased the importance of herbivory by the urchin Diadema antillarum for maintaining coral dominance, setting the stage for macroalgal dominance following the disease outbreak that killed Diadema en mass in the 1980s (Hughes et al., 1987; Aronson and Precht, 2000; Jackson et al., 2001). Last, overfishing of parrotfish has been linked to a decreased ability of reefs to recover after hurricane damage; coral cover on Belize’s Central Barrier reefs decreased by 49% following three hurricanes while protected reefs elsewhere in the Caribbean showed no declines in average coral cover (Jackson et al., 2014). As hurricane events are likely to become more frequent and intense with warming oceans, coral reefs will face greater stress and risk of destruction (Knutson et al., 2020; IPCC, 2022).
The loss of reef resilience due to long-term declines in parrotfish has significant consequences for Belize’s human populations. Declines in reef ecosystem functioning, including growth, could detrimentally impact Belize’s subsistence and commercial fishing activities which provide local food security and support livelihoods (contributing $13–14 million USD annually to the economy as one of Belize’s largest export industries; Cooper et al., 2008). Declining reef health may also impact reef tourism which provides $135–176 million USD to Belize’s economy annually (Cooper et al., 2008). Reduced rates of reef accretion from parrotfish loss will also reduce Belizean reefs’ capacity to provide coastline protection from storm erosion and wave damage, a service which helps the nation avoid $120–180 million USD in damages annually (Cooper et al., 2008) and is of increasing importance as sea level rise accelerates due to anthropogenic climate change (Guannel et al., 2016).
Our results show that although recent management interventions have helped to improve the status of parrotfish on Belize’s reefs, these ecosystems remain significantly altered by human activities. Though the commercial take of parrotfish has been banned within Belize since 2009 and parrotfish abundance has increased during this time, coral cover within the lagoonal areas of the Belizean Barrier reef is low, macroalgal cover remains high, and the Diadema urchin has failed to recover nearly 40 years since its die-off (Jackson et al., 2014; McField et al., 2020). As elevated macroalgal abundance requires correspondingly high populations of herbivores to return reef ecosystems to coral dominance (Burkepile and Hay, 2010; Steneck et al., 2014), the continued protection of parrotfish is crucial. In the face of increasing climate impacts, a return of parrotfish abundances to their pre-1970s numbers is just the beginning; our data suggests that this benchmark is far from its historical peak and much progress toward recovery remains. Our long-term record also suggests that baselines set on reefs in the 1970s and 1980s were underrepresenting the role of parrotfish in Belizean reef ecosystems as a result of centuries of overfishing.
Data availability statement
The datasets presented in this study can be found in online repositories. The names of the repository/repositories and accession number(s) can be found below: https://github.com/klcramer/Belize-parrotfish-study/tree/main.
Author contributions
WM, KC, RN, and AO contributed to the study conception and design. KC, AO, and RN collected reef sediment cores in the field. WM, KC, JZ, NL, and RN completed sample processing and analysis in the laboratory. WM and KC conducted statistical analyses and prepared the original manuscript draft. All authors reviewed the results and contributed to subsequent drafts of the manuscript.
Funding
This work was funded by the Smithsonian Institution Marine Global Earth Observatory (MarineGEO), National Geographic Society/Waitt Foundation, Frontiers of Innovation Scholars Program at University of California San Diego support to KC, Kaplan Foundation support to RN, and Sistema Nacional de Investigadores (SENACYT) support to AO.
Acknowledgments
We thank the staff at Smithsonian Institution’s Caribbean Coral Reef Ecosystems Program, especially Zachary Foltz, Scott Jones, Valerie Paul, and Emmett Duffy, for supporting and enabling this work. We also thank Ignacio Vilchis, Chris Angioletti, Brigida de Gracia, and Marcos Alvarez for their invaluable help in the field, Brian Oller for his help imaging and processing core sediments, and Kim McComas, Helen Jannke, and Elizabeth Sibert for providing technical expertise and assistance in the laboratory. This is contribution 111 from the Smithsonian’s MarineGEO and Tennenbaum Marine Observatories Network.
Conflict of interest
The authors declare that the research was conducted in the absence of any commercial or financial relationships that could be construed as a potential conflict of interest.
Publisher’s note
All claims expressed in this article are solely those of the authors and do not necessarily represent those of their affiliated organizations, or those of the publisher, the editors and the reviewers. Any product that may be evaluated in this article, or claim that may be made by its manufacturer, is not guaranteed or endorsed by the publisher.
Supplementary material
The Supplementary Material for this article can be found online at: https://www.frontiersin.org/articles/10.3389/fevo.2022.972172/full#supplementary-material
References
Abram, N. J., McGregor, H. V., Tierney, J. E., Evans, M. N., McKay, N. P., and Kaufman, D. S. (2016). Early onset of industrial-era warming across the oceans and continents. Nature 536, 411–418. doi: 10.1038/nature19082
Adam, T. C., Burkepile, D. E., Ruttenberg, B. I., and Paddack, M. J. (2015). Herbivory and the resilience of Caribbean coral reefs: knowledge gaps and implications for management. Mar. Ecol. Prog. Series 520, 1–20. doi: 10.3354/meps11170
Adam, T. C., Schmitt, R. J., Holbrook, S. J., Brooks, A. J., Edmunds, P. J., Carpenter, R. C., et al. (2011). Herbivory, connectivity, and ecosystem resilience: response of a coral reef to a large-scale perturbation. PLoS One 6:e23717. doi: 10.1371/journal.pone.0023717
Adams, J. E. (1985). Fish markets of Belize: problems of fish consumption. J. Cultural Geography 5, 43–60. doi: 10.1080/08873638509478548
Aronson, R. B., and Precht, W. F. (1997). Stasis, biological disturbance, and community structure of a Holocene coral reef. Paleobiology 23, 326–346. doi: 10.1017/S0094837300019710
Aronson, R. B., and Precht, W. F. (2000). Herbivory and algal dynamics on the coral reef at Discovery Bay. Jamaica. Limnol. Oceanography 45, 251–255. doi: 10.4319/lo.2000.45.1.0251
Aronson, R. B., Precht, W. F., and Macintyre, I. G. (1998). Extrinsic control of species replacement on a Holocene reef in Belize: the role of coral disease. Coral Reefs 17, 223–230. doi: 10.1007/s003380050122
Aronson, R. B., Macintyre, I. G., Precht, W. F., Murdoch, T. J. T., and Wapnick, C. M. (2002a). The expanding scale of species turnover events on coral reefs in Belize. Ecol. Monographs 72, 233–249. doi: 10.1890/0012-9615(2002)072[0233:TESOST]2.0.CO;2
Aronson, R. B., Precht, W. F., Toscano, M. A., and Koltes, K. H. (2002b). The 1998 bleaching event and its aftermath on a coral reef in Belize. Mar. Biol. 141, 435–447. doi: 10.1007/s00227-002-0842-5
Beach, T., Dunning, N., Luzzadder-Beach, S., Cook, D. E., and Lohse, J. (2006). Impacts of the ancient Maya on soils and soil erosion in the central Maya Lowlands. Catena 65, 166–178. doi: 10.1016/j.catena.2005.11.007
Beach, T., Ulmer, A., Cook, D., Brennan, M. L., Luzzadder-Beach, S., Doyle, C., et al. (2018). Geoarchaeology and tropical forest soil catenas of northwestern Belize. Quaternary Int. 463, 198–217. doi: 10.1016/j.quaint.2017.02.031
Bellwood, D. R., Hughes, T. P., Folke, C., and Nystrom, M. (2004). Confronting the coral reef crisis. Nature 429, 827–833. doi: 10.1038/nature02691
Bentley, J. H., Garber, J., Stanchly, N., Searle, L., and Springs, L. (2016). Turtlers, pirates and trade: a history of sea turtle exploitation on St. George’s Caye. Res. Rep. Belizean Archaeol. 13, 369–377.
Bolland, O. N. (1973). The social structure and social relations of the Settlement in the Bay of Honduras (Belize) in the 18th century. J. Caribbean History 6, 1–42.
Bolland, O. N. (1977). The Formation of a Colonial Society: Belize, from Conquest to Crown Colony. Baltimore, MD: Johns Hopkins University Press.
Bolland, O. N. (1988). Colonialism and Resistance in Belize: Essays in Historical Sociology. Belize: Cubola Productions.
Bolland, O. N. (1994). Colonization and slavery in central America. Slavery Abolition 15, 11–25. doi: 10.1002/ajpa.23887
Bonaldo, R. M., Hoey, A. S., and Bellwood, D. R. (2014). “The ecosystem roles of parrotfishes on tropical coral reefs,” in Oceanography and Marine Biology: an Annual Review, eds R. N. Hughes, D. J. Hughes, and I. P. Smith (Boca Raton, FL: CRC Press-Taylor & Francis Group), 81–132. doi: 10.1201/b17143-3
Bozec, Y. M., O’Farrell, S., Bruggemann, J. H., Luckhurst, B. E., and Mumby, P. J. (2016). ’Tradeoffs between fisheries harvest and the resilience of coral reefs. Proc. Natl. Acad. Sci. U S A. 113, 4536–4541. doi: 10.1073/pnas.1601529113
Burke, L., and Sugg, Z. (2006). Hydrologic Modeling of Watersheds Discharging Adjacent to the Mesoamerican Reef. Washington, DC: World Resources Institute.
Burkepile, D. E., and Hay, M. E. (2010). Impact of herbivore Identity on algal succession and coral growth on a Caribbean reef. PLoS One 5:e8963. doi: 10.1371/journal.pone.0008963
Camille, M. A. (1996a). Historical geography of the Belizean logwood trade. Yearbook. Conf. Latin Am. Geographers 22, 77–85.
Camille, M. A. (1996b). “Population and ethnicity of Belize, 1861,” in Proceedings from the Second Interdisciplinary Conference, ed. M. D. Phillips (Lanham, MD: University Press of America).
Carpenter, R. C. (1986). Partitioning herbivory and its effects on coral reef algal communities. Ecol. Monographs 56, 345–363. doi: 10.2307/1942551
Chase, A. F., and Chase, D. Z. (2016). The ancient maya city: anthropogenic landscapes. settlement archaeology, and Caracol, Belize. Res. Rep. Belizean Archaeol. 13, 3–14.
Cherrington, E. A., Hernandez, B. E., Trejos, N. A., Smith, O. A., Anderson, E. R., Flores, A. I., et al. (2010). Identification of threatened and resilient mangroves in the belize barrier reef system. Panama City: Water Center for the Humid Tropics of Latin America and the Caribbean.
Clark, A. T., Ye, H., Isbell, F., Deyle, E. R., Cowles, J., Tilman, G. D., et al. (2015). Spatial convergent cross mapping to detect causal relationships from short time series. Ecology 96, 1174–1181. doi: 10.1890/14-1479.1
Clark, T. R., Roff, G., Zhao, J. X., Feng, Y. X., Done, T. J., McCook, L. J., et al. (2017). U-Th dating reveals regional-scale decline of branching Acropora corals on the Great Barrier Reef over the past century. Proc. Natl. Acad. Sci. U S A. 114, 10350–10355. doi: 10.1073/pnas.1705351114
Clark, T. R., Zhao, J.-X., Feng, Y.-X., Done, T. J., Jupiter, S., Janice, L., et al. (2012). Spatial variability of initial 230Th/232Th in modern Porites from the inshore region of the Great Barrier Reef. Geochim. Cosmochimica Acta 78, 99–118. doi: 10.1016/j.gca.2011.11.032
Cleveland, W. S., and Devlin, S. J. (1988). Locally weighted regression: an approach to regression analysis by local fitting. J. Am. Stat. Assoc. 83, 596–610. doi: 10.1080/01621459.1988.10478639
Coblentz, B. E. (1997). Subsistence consumption of coral reef fish suggests non-sustainable extraction. Conserv. Biol. 11, 559–561. doi: 10.1046/j.1523-1739.1997.95504.x
Comeros-Raynal, M. T., Choat, J. H., Polidoro, B. A., Clements, K. D., Abesamis, R., Craig, M. T., et al. (2012). The likelihood of extinction of iconic and dominant herbivores and detritivores of Coral Reefs: the parrotfishes and surgeonfishes. PLoS One 7:e39825. doi: 10.1371/journal.pone.0039825
Conzemius, E. (1928). Ethnographical notes on the Black Carib (Garif). Am. Anthropol. 30, 183–205. doi: 10.1525/aa.1928.30.2.02a00010
Cooper, E., Burke, L., and Bood, N. (2008). Coastal Capital: Economic Contribution of Coral Reefs and Mangroves to Belize. Washington DC: World Resources Institute.
Box, S., and Canty, S. W. (2011). “Long and short term economic drivers of overexploitation in Honduran coral reef fisheries due to their dependence on export markets,” in Proceedings of the 63rd gulf and caribbean fisheries institute, San Juan.
Craig, A. K. (1966). The Geography of Fishing in British Honduras and Adjacent Coastal Areas. Baton Rouge, LA: Louisiana State University, LSU Historical. Ph.D.
Cramer, K. L., Jackson, J. B. C., Angioletti, C. V., Leonard-Pingel, J., and Guilderson, T. P. (2012). Anthropogenic mortality on coral reefs in Caribbean Panama predates coral disease and bleaching. Ecol. Lett. 15, 561–567. doi: 10.1111/j.1461-0248.2012.01768.x
Cramer, K. L., O’Dea, A., Carpenter, C., and Norris, R. D. (2018). A 3000 year record of Caribbean reef urchin communities reveals causes and consequences of long-term decline in Diadema antillarum. Ecography 41, 164–173.
Cramer, K. L., O’Dea, A., Clark, T. R., Zhao, J. X., and Norris, R. D. (2017). Prehistorical and historical declines in Caribbean coral reef accretion rates driven by loss of parrotfish. Nat. Commun. 8:8. doi: 10.1038/ncomms14160
Dampier, W. (1697). A New Voyage Round the World. London: James Knapton. Obtained from Project Gutenberg of Australia.
Denevan, W. M. (1992). The Native Population of the Americas in 1492, 2 Edn. Madison: University of Wisconsin press.
Denommee, K. C., Bentley, S. J., and Droxler, A. W. (2014). ’Climatic controls on hurricane patterns: a 1200-y near-annual record from Lighthouse Reef, Belize’. Sci. Rep. 4:3876. doi: 10.1038/srep03876
Donnelly, J. P., Hawkes, A. D., Lane, P., MacDonald, D., Shuman, B. N., Toomey, M. R., et al. (2015). Climate forcing of unprecedented intense-hurricane activity in the last 2000 years. Earth’s Future 3, 49–65. doi: 10.1002/2014EF000274
Edwards, C. B., Friedlander, A. M., Green, A. G., Hardt, M. J., Sala, E., Sweatman, H. P., et al. (2014). Global assessment of the status of coral reef herbivorous fishes: evidence for fishing effects. Proc. R. Soc. B-Biol. Sci. 281:10. doi: 10.1098/rspb.2013.1835
Emslie, M. J., and Pratchett, M. S. (2018). “Differential vulnerabilities of parrotfishes to habitat degradation,” in Biology of Parrotfishes, eds Hoey Andrew S. Bonaldo and M. Roberta (Boca Raton, FL: Taylor & Francis). doi: 10.1201/9781315118079-15
Fabricius, K. E. (2005). Effects of terrestrial runoff on the ecology of corals and coral reefs: review and synthesis. Mar. Pollution Bull. 50, 125–146. doi: 10.1016/j.marpolbul.2004.11.028
Gibson, J., McField, M., and Wells, S. (1998). Coral reef management in Belize: an approach through Integrated Coastal Zone Management. Ocean Coastal Manag. 39, 229–244. doi: 10.1016/S0964-5691(98)00007-6
Gischler, E., Shinn, E. A., Oschmann, W., Fiebig, J., and Buster, N. A. (2008). ’A 1500-Year holocene caribbean climate archive from the blue hole, lighthouse reef, belize’. J. Coastal Res. 24, 1495–1505. doi: 10.2112/07-0891.1
Gobalet, K. W. (1989). Morphology of the parrotfish pharyngeal jaw apparatus. Am. Zool. 29, 319–331. doi: 10.1093/icb/29.1.319
Graham, E., Pendergast, D. M., and Jones, G. D. (1989). ’On the fringes of conquest – Maya-Spanish contact in colonial Belize. Science 246, 1254–1259. doi: 10.1126/science.246.4935.1254
Gress, E., Voss, J. D., Eckert, R. J., Rowlands, G., and Andradi-Brown, D. A. (2019). “The Mesoamerican Reef,” in Mesophotic Coral Ecosystems. Coral Reefs of the World, eds Y. Loya, K. A. Puglise, and T. C. L. Bridge (Cham: Springer), 71–84. doi: 10.1007/978-3-319-92735-0_5
Guannel, G., Arkema, K., Ruggiero, P., and Verutes, G. (2016). The power of three: coral reefs, seagrasses, and mangroves protect coastal regions and increase their resilience. PLoS One 11:e0158094. doi: 10.1371/journal.pone.0158094
Hanna, M. G. (2015). Pirate Nests and the Rise of the British Empire, 1570-1740. Chapel Hill, NC: University of North Carolina Press.
Harborne, A. R., Afzal, D. C., and Andrews, M. J. (2001). Honduras: Caribbean coast. Mar. Pollution Bull. 42, 1221–1235. doi: 10.1016/S0025-326X(01)00239-9
Hardt, M. J. (2009). Lessons from the past: the collapse of Jamaican coral reefs. Fish Fish. 10, 143–158. doi: 10.1111/j.1467-2979.2008.00308.x
Hawkins, J. P., and Roberts, C. M. (2004). Effects of artisanal fishing on Caribbean coral reefs. Conservation Biol. 18, 215–226. doi: 10.1111/j.1523-1739.2004.00328.x
Hay, M. E. (1984). Patterns of fish and urchin grazing on Caribbean coral reefs – are previous results typical. Ecology 65, 446–454. doi: 10.2307/1941407
Hay, M. E., and Taylor, P. R. (1985). Competition between herbivorous fishes and urchins on Caribbean reefs. Oecologia 65, 591–598. doi: 10.1007/BF00379678
Hoey, A. S., Taylor, B. M., Hoey, J., and Fox, R. J. (2018). “Parrotfishes, are we still scraping the surface? Emerging topics and future research directions,” in Biology of Parrotfishes, eds A. S. Hoey and R. M. Bonaldo (Boca Raton, FL: CRC Press). doi: 10.1201/9781315118079
Hughes, T. P. (1994). Catastrophes, phases-shifts, and large-scale degradation of a Caribbean coral reef. Science 265, 1547–1551. doi: 10.1126/science.265.5178.1547
Hughes, T. P., Reed, D. C., and Boyle, M. J. (1987). Herbivory on coral reefs – community structure following mass mortalities of sea urchins. J. Exp. Mar. Biol. Ecol. 113, 39–59.
Hughes, T. P., Rodrigues, M. J., Bellwood, D. R., Ceccarelli, D., Hoegh-Guldberg, O., McCook, L., et al. (2007). Phase shifts, herbivory, and the resilience of coral reefs to climate change. Curr. Biol. 17, 360–365. doi: 10.1016/0022-0981(87)90081-5
IPCC (2022). “Climate change 2022: impacts, adaptation, and vulnerability,” in Contribution of Working Group II to the Sixth Assessment Report of the Intergovernmental Panel on Climate Change, eds H.-O. Pörtner, D. C. Roberts, M. Tignor, E. S. Poloczanska, K. Mintenbeck, A. Alegría, et al. (Cambridge: Cambridge University Press). doi: 10.1016/j.cub.2006.12.049
Jackson, J. B. C., Donovan, M. K., Cramer, K. L., and Lam, V. (2014). Status and Trends of Caribbean Coral Reefs. Gland: IUCN.
Jackson, J. B. C., Kirby, M. X., Berger, W. H., Bjorndal, K. A., Botsford, L. W., Bourque, B. J., et al. (2001). Historical overfishing and the recent collapse of coastal ecosystems. Science 293, 629–638. doi: 10.1126/science.1059199
Jacob, J. S. (1995). Ancient Maya wetland agricultural fields in Cobweb Swamp, Belize: construction, chronology, and function’. J. Field Archaeol. 22:175. doi: 10.2307/530320
Johnson, M. A. (2003). The making of race and place in nineteenth-century British Honduras. Environ. History 8, 598–617. doi: 10.2307/3985885
Jones, J. G. (1994). Pollen evidence for early settlement and agriculture in northern Belize’. Palynology 18, 205–211. doi: 10.1080/01916122.1994.9989445
Joseph, G. M. (1974). British loggers and Spanish governors: the logwood trade and its settlements in the Yucatan Peninsula: Part I’. Caribbean Stud. 14, 7–37.
Kaufman, L. S. (1983). Effects of Hurricane Allen on reef fish assemblages near Discovery Bay, Jamaica. Coral Reefs 2, 43–47. doi: 10.1007/BF00304731
Key, C. J. (2002). The political economy of the transition from fishing to tourism, in Placencia, Belize. Int. Rev. Modern Sociol. 30, 1–18.
Knutson, T., Camargo, S. J., Chan, J. C. L., Emanuel, Kerry, Ho, C.-H., et al. (2020). Tropical cyclones and climate change assessment: Part II: projected response to anthropogenic warming. Bull. Am. Meteorol. Soc. 101, E303–E322. doi: 10.1175/BAMS-D-18-0194.1
Koch, A., Brierley, C., Maslin, M. M., and Lewis, S. L. (2019). Earth system impacts of the European arrival and Great Dying in the Americas after 1492. Quaternary Sci. Rev. 207, 13–36. doi: 10.1016/j.quascirev.2018.12.004
Koslow, J. A., Aiken, K., Auil, S., and Clementson, A. (1994). Catch and effort analysis of the reef fisheries of Jamaica and Belize. Fish. Bull. 92, 737–747.
Kunen, J. L. (2001). Ancient Maya agricultural installations and the development of intensive agriculture in NW Belize’. J. Field Archaeol. 28, 325–346. doi: 10.1179/jfa.2001.28.3-4.325
Leonard, T. M. (2011). “The history of honduras,” in The greenwood histories of the modern nations (Santa Barbara, CA: Greenwood).
Lessios, H. A. (1988). Mass mortality of Diadema antillarum in the Caribbean – what have we learned? Ann. Rev. Ecol. Systematics 19, 371–393. doi: 10.1146/annurev.es.19.110188.002103
Lohse, J. C., Awe, J., Griffith, C., Rosenswig, R. M., and Valdez, F. (2006). Preceramic occupations in Belize: updating the Paleoindian and Archaic record. Latin Am. Antiquity 17, 209–226. doi: 10.2307/25063047
Łukowiak, M., Cramer, K. L., Madzia, D., Hynes, M. G., Norris, R. D., and O’Dea, A. (2018). Historical change in a Caribbean reef sponge community and long-term loss of sponge predators. Mar. Ecol. Prog. Series 601, 127–137. doi: 10.3354/meps12694
Lybolt, M., Neil, D., Zhao, J. X., Feng, Y. X., Yu, K. F., and Pandolfi, J. (2011). Instability in a marginal coral reef: the shift from natural variability to a human-dominated seascape. Front. Ecol. Environ. 9:154–160. doi: 10.1890/090176
Macintyre, I. G., and Aronson, R. B. (1997). “’Field guidebook to the reefs of Belize’,” in Proceedings of the 8th International Coral Reef Symposium, eds H. Lessios and I. G. Macintyre (Panama: Smithsonian Tropical Research Institute, Balboa, Republic of Panama).
Mann, M. E., Bradley, R. S., and Hughes, M. K. (1998). Global-scale temperature patterns and climate forcing over the past six centuries. Nature 392, 77 9–787.
Mann, M. E., Woodruff, J. D., Donnelly, J. P., and Zhang, Z. (2009). Atlantic hurricanes and climate over the past 1,500 years. Nature 460, 880–883.
McClenachan, L., and Kittinger, J. N. (2013). Multicentury trends and the sustainability of coral reef fisheries in Hawai”i and Florida. Fish Fisheries 14, 239–255.
McField, M., Kramer, P., Giroì Petersen, A., Soto, M. l., Drysdale, I., Craig, N., et al. (2020). 2020 Mesoamerican reef report card. Healthy reefs initiative. Available online at: https://www.healthyreefs.org/cms/wpcontent/uploads/2020/02/2020_Report_Card_MAR.pdf
McKillop, H. (1984). Prehistoric maya reliance on marine resources: analysis of a midden from moho cay, Belize. J. Field Archaeol. 11, 25–35.
McKillop, H. (2009). The geopolitics of coastal Maya economy in southern Belize: relations between the coastal and inland Maya. Res. Rep. Belizean Archaeol. 6, 55–61.
McKillop, H. (2017). “Early maya navigation and maritime connections in mesoamerica,” in The Sea in History - The Medieval World. NED - New edition ed, eds M. Balard and C. Buchet (Woodbridge: Boydell and Brewer).
McKillop, H., and Aoyama, K. (2018). Salt and marine products in the Classic Maya economy from use-wear study of stone tools. Proc. Natl. Acad. Sci. U S A. 115, 10948–10952. doi: 10.1073/pnas.1803639115
McKillop, H. I. (1985). Prehistoric exploitation of the manatee in the Maya and circum-Caribbean areas’. World Archaeol. 16, 337–353.
McManus, J. W., Menez, L. A. B., Kesner-Reyes, K. N., Vergara, S. G., and Ablan, M. C. (2000). Coral reef fishing and coral-algal phase shifts: implications for global reef status. ICES J. Mar. Sci. 57, 572–578.
Moberg, M. (1992). Continuity under colonial rule: the Alcalde System and the Garifuna in Belize, 1858-1969. Ethnohistory 39, 1–19.
Mueller, A. D., Islebe, G. A., Anselmetti, F. S., Ariztegui, D., Brenner, M., Hodell, D. A., et al. (2010). Recovery of the forest ecosystem in the tropical lowlands of northern Guatemala after disintegration of Classic Maya polities. Geology 38, 523–526.
Mumby, P. J. (2006). The impact of exploiting grazers (scaridae) on the dynamics of Caribbean coral reefs. Ecol. Appl. 16, 747–769. doi: 10.1890/1051-0761(2006)016[0747:tioegs]2.0.co;2
Mumby, P. J., Steneck, R. S., Edwards, A. J., Ferrari, R., Coleman, R., Harborne, A. R., et al. (2012). Fishing down a Caribbean food web relaxes trophic cascades. Mar. Ecol. Prog. Series 445, 13–24.
Mumby, P. J., Steneck, R. S., Roff, G., and Paul, V. J. (2021). Marine reserves, fisheries ban, and 20 years of positive change in a coral reef ecosystem. Conservation Biol. 35, 1473–1483. doi: 10.1111/cobi.13738
Murray, M. R., Zisman, S. A., Furley, P. A., Munro, D. M., Gibson, J., Ratter, J., et al. (2003). The mangroves of Belize Part 1. distribution, composition and classification. Forest Ecol. Manag. 174, 265–279.
O’Dea, A., Shaffer, M. L., Doughty, D. R., Wake, T. A., and Rodriguez, F. A. (2014). Evidence of size-selective evolution in the fighting conch from prehistoric subsistence harvesting. Proc. R. Soc. B 281:20140159. doi: 10.1098/rspb.2014.0159
Paz, G. E., and Truly, E. (2007). The Nassau Grouper Spawning Aggregation at Caye Glory, Belize: a brief history. Belize City: The Nature Conservancy.
Pauly, D., Christensen, V., Dalsgaard, J., Froese, R., and Torres, F. (1998). Fishing down marine food webs. Science 279, 860–863.
Peedle, I. (1999). History and Politics: from Enclave to Nationhood, Belize in Focus; A guide to the People, Politics and Culture. London: Latin American Bureau.
Pendergast, D. M. (1993). Worlds in collision: the Maya/Spanish encounter in sixteenth and seventeenth century Belize. Proc. Br. Acad. 81, 105–143.
Peuramaki-Brown, M. M., Morton, S. G., and Jordan, J. M. (2020). Maya archaeology of the Stann Creek District, Belize: early explorations to recent research. Res. Rep. Belizean Archaeol. 17, 221–235.
Pinnegar, J. K., and Engelhard, G. H. (2008). The ‘shifting baseline’ phenomenon: a global perspective. Rev. Fish Biol. Fish. 18, 1–16. doi: 10.1007/s11948-009-9147-0
Rodriguez-Martinez, R. E., Banaszak, A. T., McField, M. D., Beltran-Torres, A. U., and Alvarez-Filip, L. (2014). Assessment of Acropora palmata in the Mesoamerican Reef System. PLoS One 9:e96140. doi: 10.1371/journal.pone.0096140
Roff, G., Clark, T. R., Reymond, C. E., Zhao, J. X., Feng, Y. X., McCook, L. J., et al. (2013). Palaeoecological evidence of a historical collapse of corals at Pelorus Island, inshore Great Barrier Reef, following European settlement. Proc. R. Soc. B-Biol. Sci. 280:10. doi: 10.1098/rspb.2012.2100
Sandin, S. A., and McNamara, D. E. (2012). Spatial dynamics of benthic competition on coral reefs. Oecologia 168, 1079–1090.
Shinn, E. A., Halley, R. B., Hudson, J. H., Lidz, B., and Robbin, D. M. (1979). Three-dimensional aspects of Belize patch reefs (abstract). Am. Assoc. Petroleum Geol. Bull. 63:528.
Sibert, E. C., Cramer, K. L., Hastings, P. A., and Norris, R. D. (2017). Methods for isolation and quantification of microfossil fish teeth and elasmobranch dermal denticles (ichthyoliths) from marine sediments. Palaeontol. Electron. 20:14.
Sills, E. C. (2016). Re-evaluating the ancient Maya salt works at Placencia Lagoon. Belize, Mexicon 38, 69–74.
Simmons, S. E., and Graham, E. (2016). “Maya coastal adaptations in classic and postclassic times on ambergris caye, Belize,” in Trading Spaces: The Archaeology of Interaction, Migration and Exchange. Proceedings of the 46th Annual Chacmool Archaeology Conference, eds M. Patton and J. Manion (Calgary CA: Chacmool Archaeology Association, University of Calgary), 167–180.
Soto, I., Andréfouët, S., Hu, C., Muller-Karger, F. E., Wall, C. C., Sheng, J., et al. (2009). Physical connectivity in the Mesoamerican Barrier Reef System inferred from 9 years of ocean color observations. Coral Reefs 28, 415–425.
Statistical Institute of Belize (2022a). Census History Timeline. Belize: Statistical Institute of Belize.
Statistical Institute of Belize (2022b).. Census Population by District, 1921-2010. Belize: Statistical Institute of Belize.
Steneck, R. S., Arnold, S. N., and Mumby, P. J. (2014). Experiment mimics fishing on parrotfish: insights on coral reef recovery and alternative attractors. Mar. Ecol. Prog. Series 506, 115–127.
Sutherland, A. (1996). Tourism and the human mosaic In Belize. Urban Anthropol. Stud. Cultural Systems World Econ. Dev. 25, 259–281.
Taylor, B. M., Benkwitt, C. E., Choat, H., Clements, K. D., Graham, N. A. J., and Meekan, M. G. (2019). Synchronous biological feedbacks in parrotfishes associated with pantropical coral bleaching. Global Change Biol. 26, 1285–1294. doi: 10.1111/gcb.14909
Taylor, P. (2013). “’Belize’,” in The Encyclopedia of Caribbean Religions, eds P. Taylor, F. I. Case, and S. Meighoo (Champaign, IL: University of Illinois Press).
Wake, T. A., Doughty, D. R., and Kay, M. (2013). Archaeological investigations provide late Holocene baseline ecological data for Bocas del Toro, Panama. Bull. Mar. Sci. 89, 1015–1035.
Watanabe, T., Winter, A., and Oba, T. (2001). Seasonal changes in sea surface temperature and salinity during the Little Ice Age in the Caribbean Sea deduced from Mg/Ca and 18O/16O ratios in corals. Mar. Geol. 173, 21–35.
White, C. D., Healy, P. F., and Schwarcz, H. P. (1993). Intensive agriculture, social status, and Maya diet at Pacbitun, Belize. J. Anthropol. Res. 49, 34 7–375.
Wild, C., Jantzen, C., and Kremb, S. G. (2014). Turf algae-mediated coral damage in coastal reefs of Belize, Central America. Peerj 2:14. doi: 10.7717/peerj.571
Williams, J. S., White, C. D., and Longstaffe, F. J. (2009). Maya marine subsistence: isotopic evidence from Marco Gonzalez and San Pedro, Belize. Latin Am. Antiquity 20, 37–56.
Wing, E. S. (1975). “Animal remains from lubaantun,” in Lubaantun: A Classic Maya Realm, ed. N. Hammond (Cambridge, MA: Peabody Museum of Archaeology and Ethnology, Harvard University), 379–383.
Woods, L. A., Perry, J. M., and Steagall, J. W. (1997). The composition and distribution of ethnic groups in Belize: immigration and emigration patterns, 1980-1991. Latin Am. Res. Rev. 32, 63–88.
Keywords: Mesoamerican Reef, fishing, historical ecology, conservation paleobiology, social-ecological system (SES), shifting baselines, European colonization
Citation: Muraoka WT, Cramer KL, O’Dea A, Zhao J, Leonard ND and Norris RD (2022) Historical declines in parrotfish on Belizean coral reefs linked to shifts in reef exploitation following European colonization. Front. Ecol. Evol. 10:972172. doi: 10.3389/fevo.2022.972172
Received: 17 June 2022; Accepted: 05 September 2022;
Published: 04 October 2022.
Edited by:
Gregory Dietl, Cornell University, United StatesReviewed by:
Rachel E. B. Reid, Virginia Tech, United StatesMarcelo Michel Rivadeneira, Universidad Católica del Norte, Chile
Copyright © 2022 Muraoka, Cramer, O’Dea, Zhao, Leonard and Norris. This is an open-access article distributed under the terms of the Creative Commons Attribution License (CC BY). The use, distribution or reproduction in other forums is permitted, provided the original author(s) and the copyright owner(s) are credited and that the original publication in this journal is cited, in accordance with accepted academic practice. No use, distribution or reproduction is permitted which does not comply with these terms.
*Correspondence: Katie L. Cramer, a2F0aWUuY3JhbWVyQGFzdS5lZHU=
†These authors have contributed equally to this work