- 1Key Laboratory of Vegetation Ecology of the Ministry of Education, Institute of Grassland Science, Jilin Songnen Grassland Ecosystem National Observation and Research Station, Northeast Normal University, Changchun, China
- 2College of Geography and Ocean Sciences, Yanbian University, Yanji, China
- 3Shandong Key Laboratory of Eco-Environmental Science for Yellow River Delta, Binzhou University, Binzhou, China
- 4Key Laboratory of Wetland Ecology and Environment, Heilongjiang Xingkai Lake Wetland Ecosystem National Observation and Research Station, Northeast Institute of Geography and Agroecology, Chinese Academy of Sciences, Changchun, China
Plant nutrient stoichiometry indicates the balance of plant internal nutrients and its nutrient-use strategies in response to environmental changes. However, the responses of nutrient stoichiometry in different wetland plant organs under agricultural intervention are poorly understood. Here, we compared the nitrogen (N), phosphorus (P), and the ratio of N:P in the plant organs (leaves, stems, roots, and root hair) of a typical helophyte plant (Glyceria spiculosa) in reference, drained, nutrient-rich, and cultivated wetlands (CW) located downstream of the Tumen River in Northeast China. Compared with that in reference wetlands (RW), the results indicate that the average N content in plant leaves, stems, roots, and root hair in nutrient-rich wetlands (NW) was significantly higher by 76, 61, 56, and 39%, respectively (p < 0.05), whereas the N content of roots and root hair in drained wetlands (DW) was significantly higher by 17 and 32%, respectively (p < 0.05). It was found that plant root P increased only in nutrient-rich and DW (p < 0.05). Interestingly, the agricultural interventions significantly affected soil N and P availability, resulting in positive effects on plant leaves, stems, roots, and root hair. Nutrient stoichiometry analysis showed the highest increase in plant leaf N:P ratio in NW, followed by that in drained and CW, but its ratio in root and root hair showed no significant changes under different agricultural interventions, which suggests that G. spiculosa allocates nutrients differently in different organs under agricultural interventions. These results imply that plant nutrient stoichiometry should incorporate various plant organs for an in-depth understanding of plant strategies against environmental changes.
Introduction
The concept of ecological stoichiometry refers to the balance between multiple chemical elements in ecological interactions (Sterner and Elser, 2003). Nitrogen (N) and phosphorus (P) are the most important nutrients for proper plant growth and functioning (Li et al., 2018). Plant stoichiometry of N and P in plant organs indicates the limitation of plant nutrients as well as the plant homeostasis under the influence of environmental changes (Alvarez-Clare and Mack, 2015; Zhang et al., 2021), and this concept has been widely used to identify plant strategies that control population stability, competition, and succession (Yu et al., 2015; Guiz et al., 2016).
Wetlands are land areas that are inundated or saturated by water frequently, resulting in low nutrient availability (Bedford et al., 1999). To overcome the limitation of insufficient nutrients, wetland plants have evolved strategies for survival, including forming aerenchyma, and altering nutrient and biomass allocation (Aerts and Chapin, 1999; Moor et al., 2017). During the past decades, anthropogenic activities of wetland cultivation have significantly caused over 50% of degradation or loss of wetlands (Zedler and Kercher, 2005), consequently increasing wetland fragmentation and altering the hydrological conditions as well as soil nutrient conditions due to drainage and fertilization (Zedler and Kercher, 2005; Zheng et al., 2017; Qin et al., 2021). Further, these variations in hydrology and soil nutrients have been found to affect plant growth, reproduction, and community structures in wetlands (Li et al., 2018; Bai et al., 2021).
The response of nutrient levels and nutrient stoichiometry have been widely studied under the conditions of water level and nutrient addition in wetlands (Güsewell, 2004; Mao et al., 2016; Li et al., 2018; Zhang et al., 2021). However, these studies tend to focus on the plant leaf or stem and neglect the belowground plant organs as plant growth and reproduction depend upon the roots to absorb and fix nutrients (Kramer-Walter et al., 2016). Given the tendency of plants to allocate nutrients among different organs to maximize soil nutrient availability (Yu et al., 2015; Di Palo and Fornara, 2017; Bai et al., 2021), the study of plant nutrient stoichiometries above- and below-ground could lead to a better understanding of wetland plant responses to agricultural interventions.
Glyceria spiculosa (Schmidt) Roshev. (Gramineae) is an emergent macrophyte commonly found in freshwater marshes and is widely distributed in China, East Russia, and North Korea (Bai et al., 2021). Related studies have shown that both N and P fertilizers can contribute to the dominance of G. spiculosa (Mao et al., 2014, 2016). The responses of biomass allocation and root clonal structures of G. spiculosa under nutrient input and flooding show that it has high plasticity under environmental changes (Luo et al., 2010; Bai et al., 2021). Nevertheless, little is known about the nutrient stoichiometries of roots, stems, and leaf organs of the species, and this limits the understanding of the responses of G. spiculosa and its community succession in different agricultural interventions.
To address this knowledge gap, we conducted a field investigation in different wetlands located downstream of the Tumen River, where G. spiculosa dominates the wetland community. In this region, agricultural practices have caused a drastic reduction in water levels and increased nutrient levels within the wetlands (Zheng et al., 2017). Here, we investigated the stoichiometry of N and P in G. spiculosa organs (from roots to stems to leaves) among reference (natural), drained, nutrient-enriched, and drained wetlands (DW), as well as the boundary of agriculture [paddy fields converted from wetlands, hereafter referred to as cultivated wetlands (CW)]. We hypothesized that agricultural intervention would weakly alter nutrient concentrations in different organs of plants, but nutrient stoichiometry would remain relatively constant.
Materials and methods
Study site
The study site is located downstream of the Tumen River Basin (129°52′00″E–131°18′30″E, 42°25′20″N–43°30′18″N), which is the boundary area of China, Russia, and North Korea. This area is characterized by the temperate-zone monsoon climate with mean annual precipitation of around 400–650 mm and average temperature of 2°C–6°C (Zheng et al., 2017). In this area, the wetlands are widely distributed and they include marshes, riverine habitats, and lakeshore habitats and the dominant plants include G. spiculosa, Carex appendiculata, and Phragmites australis. Many wetlands have been converted into paddy fields due to the growing population and food demand. By the application of fertilizers and building of a drainage ditch near natural wetlands, agricultural development has not only resulted in the loss of wetlands but also altered their hydrology and nutrient conditions (Zheng et al., 2017).
Field sampling and measurement
We conducted field investigations near Jintang village, where natural wetlands have been converted into disturbed wetlands and paddy fields. Four agricultural impacted wetlands were selected (Figure 1): (1) a nutrient-rich wetland (NW), which is adjacent to a paddy field, (2) a DW, which was drained for agriculture in 2010 due to wetland protection policy, and tillage is forbidden but the drainage ditch is still present; there is no obvious surface runoff throughout the year in this wetland; (3) a CW, which is an agricultural land (paddy field) and the species G. spiculosa is distributed at the boundary of the field; and (4) a reference wetland (RW), which is a natural wetland.
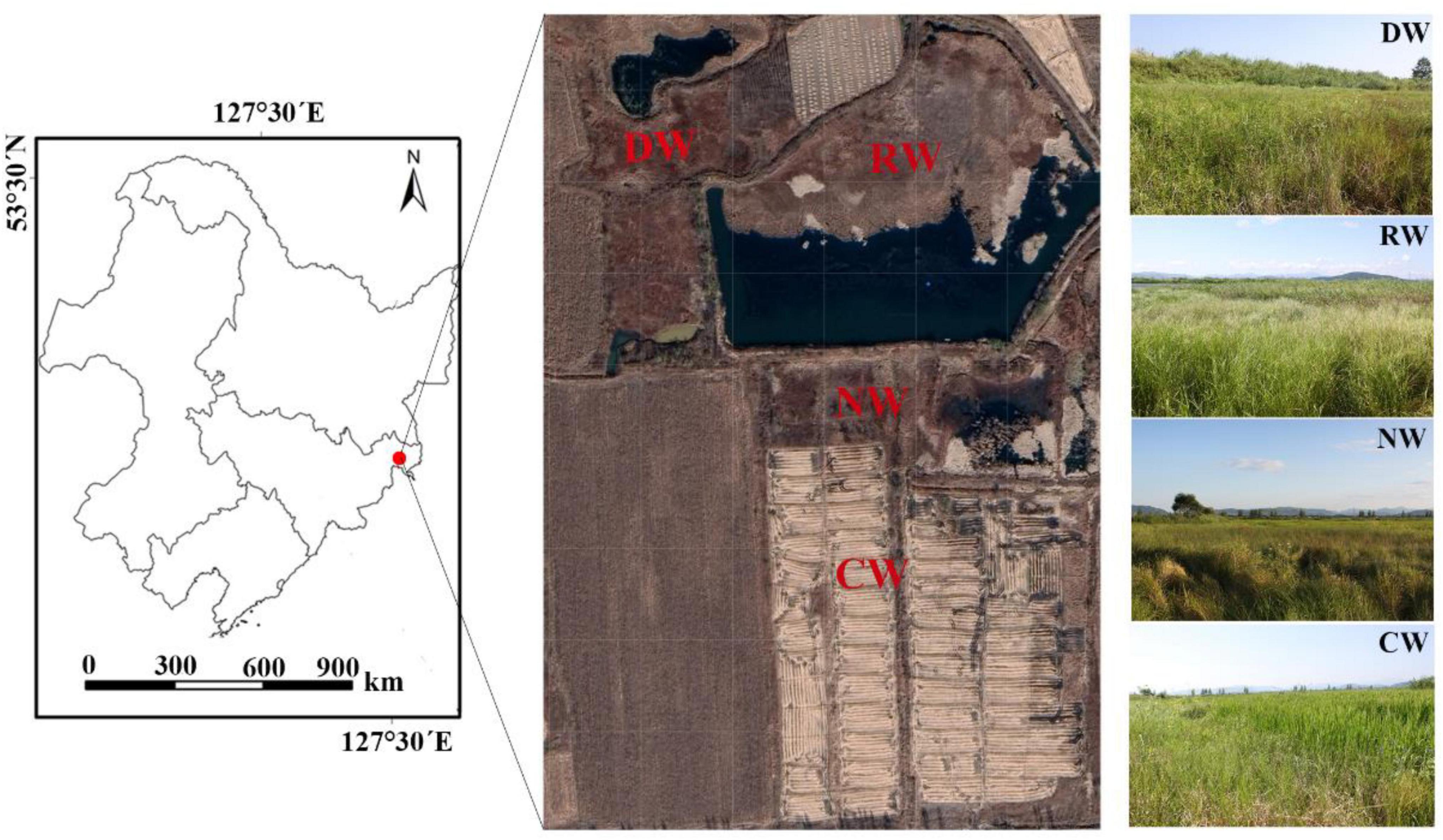
Figure 1. Map of the wetland study sites downstream of the Tumen River. RW, reference wetlands; DW, drained wetlands; NW, nutrient-rich wetlands; CW, cultivated wetlands.
Sampling was conducted in early August 2016. In reference, nutrient-rich, and DW, samples were collected along two transects at equidistant intervals. The length of the transects depended on the diameters of the sites, and three sampling plots were set on each transect, with six replicates for each type of wetland. Soil samples and plants were collected from 1 m × 1 m plots. The soil samples were combined into a composite sample; for the plant samples, five replicates were randomly selected and the plants were divided into leaves, stems, roots, and root hair, and the same plant organ was combined as a composite plant sample within each 1 m × 1 m plot. Since G. spiculosa grows only along the boundary of paddy fields, we randomly collected six soil and plant samples along the paddy fields as described above. Both soil and plant samples were frozen in ice and transported for analysis to the laboratory of Yanbian University.
Following air-drying and grinding of soil samples, soil samples (0.4 g) were digested with catalysts (1.8 g, potassium sulfate: copper sulfate pentahydrate: selenium = 100:10:1) and 4 ml sulfuric acid (400°C, 2–3 h) and the digests were diluted with distilled water (100 ml) and measured using a continuous flow analyzer (SAN + +, Skalar, Breda, Netherlands) (Wang et al., 2019). The soil N and P were extracted by 2 M potassium chloride and 0.5 M sodium bicarbonate (pH = 8), respectively. The soil N and P concentrations in all extractions were determined using a continuous flow analyzer (SAN + +, Skalar, Breda, Netherlands). The plant organs were oven-dried at 65°C for 48 h and subsequently ground to measure the N and P concentrations. Subsamples of organ samples (0.1 g) were digested with hydrogen peroxide and sulfuric acid. The extracted solutions were used for the measurement of plant N and P concentrations using a continuous flow analyzer (SAN + +, Skalar, The Netherlands).
Statistical analysis
One-way analysis of variance (ANOVA) was used to test the effects of the agricultural intervention on the N and P content of the soil and plant, coupled with Duncan’s test for significance (p < 0.05). Similarly, we compared the percentages of N and P in each organ under different agricultural interventions. A linear regression model was carried out to determine the effects of soil N and P on plant organ N and P content values. All statistical analyses were performed using R 4.1.2 (R Core Team, 2019).
Results
Soil properties between wetland types
The average soil total nitrogen (TN) content in the RW was 4.05 mg/g, which was similar to the value in DW, but was significantly lower than the value of 6.56 mg/g observed in NW (p < 0.05, Figure 2A). The soil total phosphorus content (TP) was similar between reference and DW, which were both significantly lower than the average content values of 0.96 mg/g and 0.97 mg/g in nutrient-rich and cultivated wetlands, respectively (p < 0.05, Figure 2B). The average soil available nitrogen content was 28.07 mg/kg, with the maximum value of 41.32 mg/kg recorded in NW and a value of 30.07 mg/kg recorded in DW (Figure 2C). Similarly, the soil available P in RW was 13.64 mg/kg, which was significantly lower than the values in drained and NW (p < 0.05, Figure 2D).
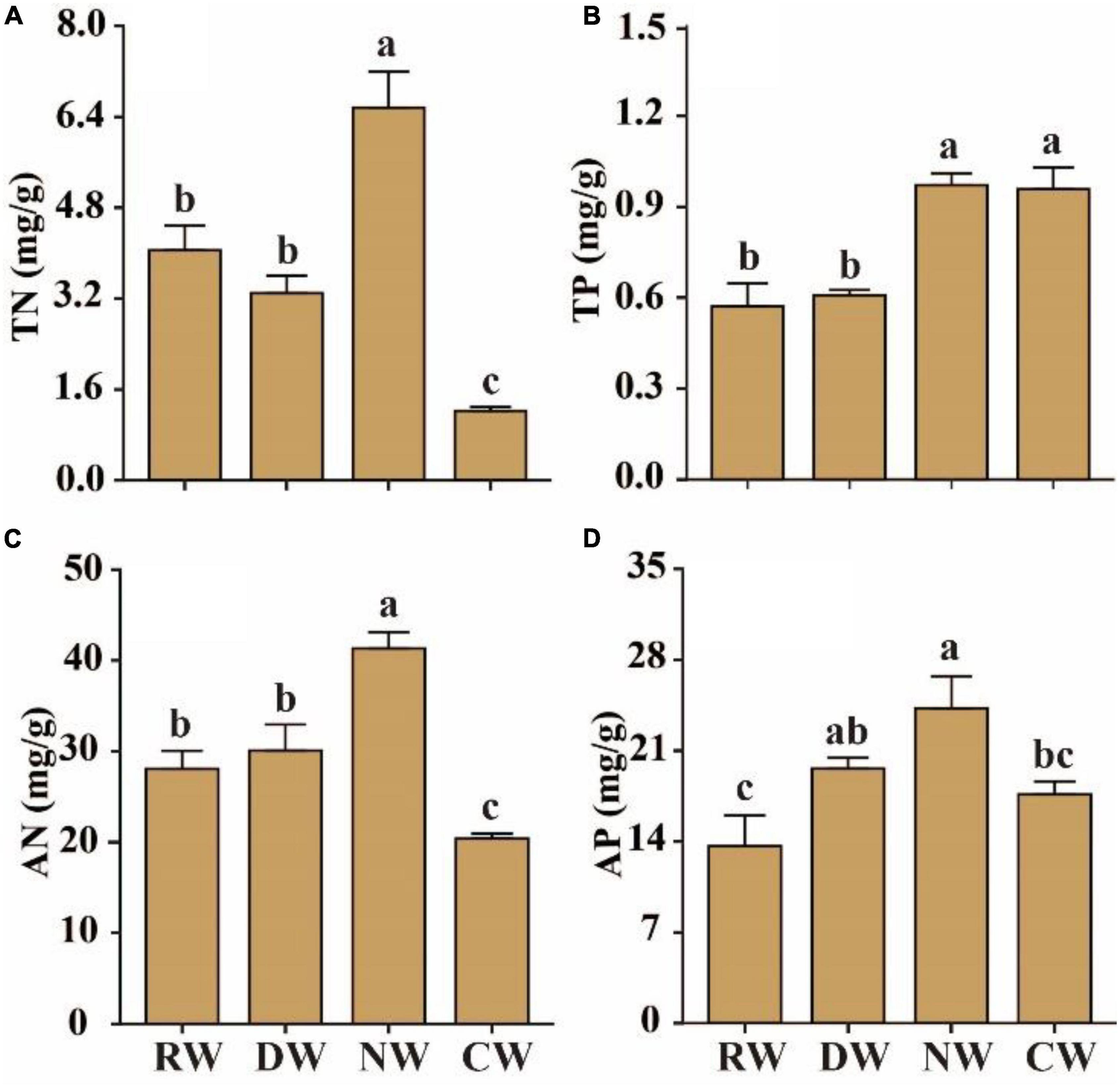
Figure 2. Effects of agricultural intervention on soil properties. (A) Soil total nitrogen (TN); (B) soil total phosphorus (TP); (C) soil available nitrogen (AN); (D) soil available phosphorus (AP). RW, reference wetlands; DW, drained wetlands; NW, nutrient-rich wetlands; CW, cultivated wetlands. Error bars represent ± SE. Different letters indicate significant differences (p < 0.05).
Nitrogen and phosphorus levels in plant organs
In RW, N values in plant leaves, stems, roots, and root hair averaged 12.19, 6.65, 5.41, and 4.87 mg/kg, respectively, and were higher by 76, 61, 56, and 39% in NW, respectively (p < 0.05, Figures 3A–D). While the average N content values of root and root hair in DW were 9.07 and 7.12 mg/kg, these values were also significantly higher than those in RW (p < 0.05, Figures 3A–D). Plant leaves, stems, roots, and root hair in RW had average P content values of 1.50, 1.05, 1.29, and 1.63 mg/kg, respectively. The P content of plant roots in cultivated wetlands and plant stems in DW differed significantly only from the values in RW by 58 and 33%, respectively (p < 0.05, Figures 3E–H).
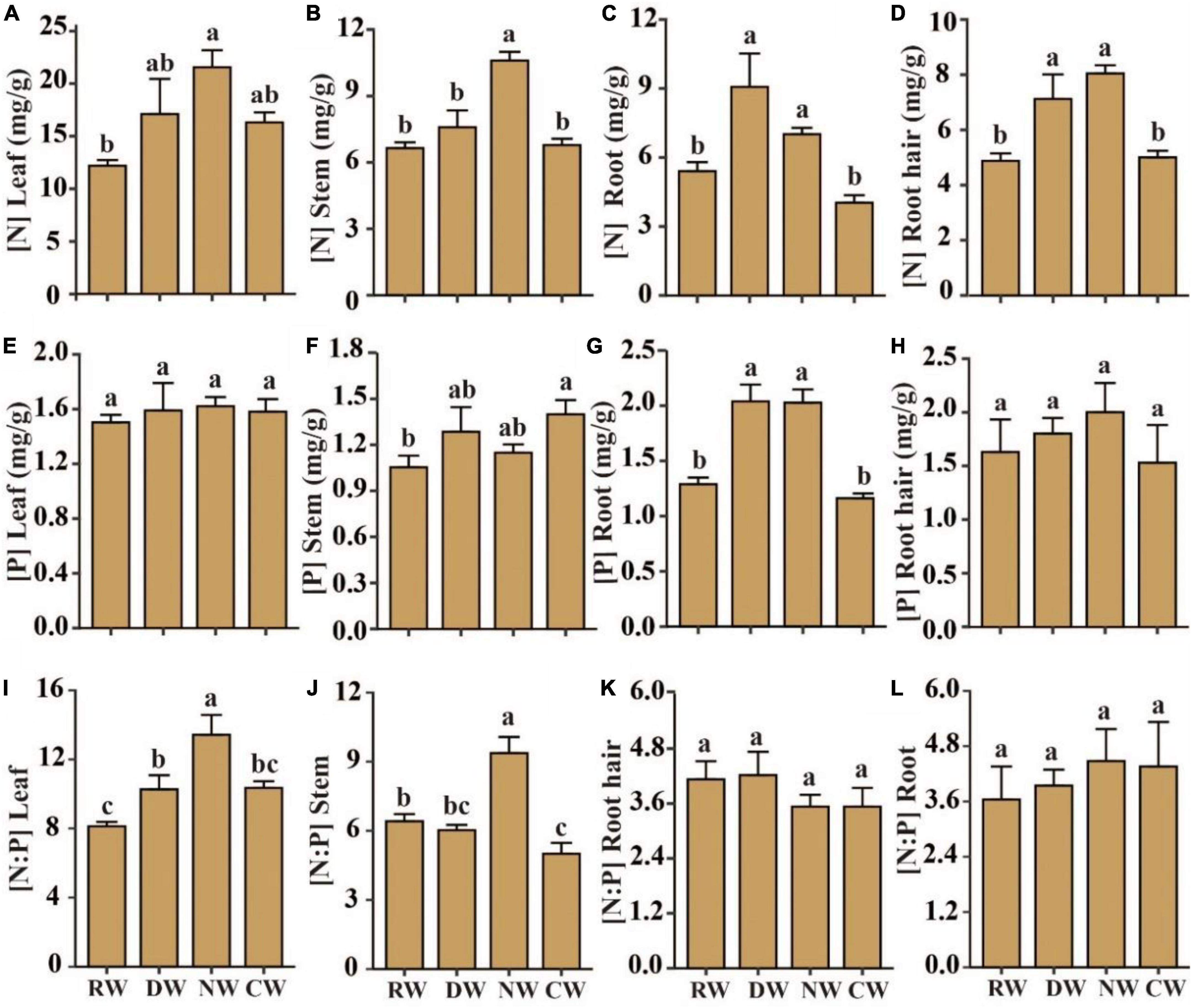
Figure 3. Effects of agricultural intervention on N and P stoichiometry of plant tissues. (A–D) Plant tissue N content; (E–H) plant tissue P content; (I–L) plant tissue N and P stoichiometry. RW, reference wetlands; DW, drained wetlands; NW, nutrient-rich wetlands; CW, cultivated wetlands. Error bars represent ± SE. Different letters indicate significant differences between sites (p < 0.05).
Nitrogen and phosphorus stoichiometry in plant organs
The average leaf N:P ratio in RW was 8.12, while the maximum value of 13.42 was observed in NW, followed by similar ratios of 10.34 and 10.27 in cultivated and DW, respectively (Figure 3I). In the DW, the average stem N:P ratio was 6.02, similar to the average ratio of 6.41 in the RW, while that in cultivated wetlands was significantly lower, but was higher by 46% in the NW (p < 0.05, Figure 3J). The root and root hair N:P ratio remained constant under different intervention conditions (Figures 3K,L).
The relationship between soil nutrients and plant organ nitrogen or phosphorus content
Soil available N and P positively influenced N content values in plant root hair, roots, stems, and leaves, with the maximum effects observed on leaf N, which showed the highest correlation with leaf N (p < 0.05, Figures 4A–H). Soil available N and P were found to positively influence root P content and had no influence on the other organs (p < 0.05, Figures 4I–P).
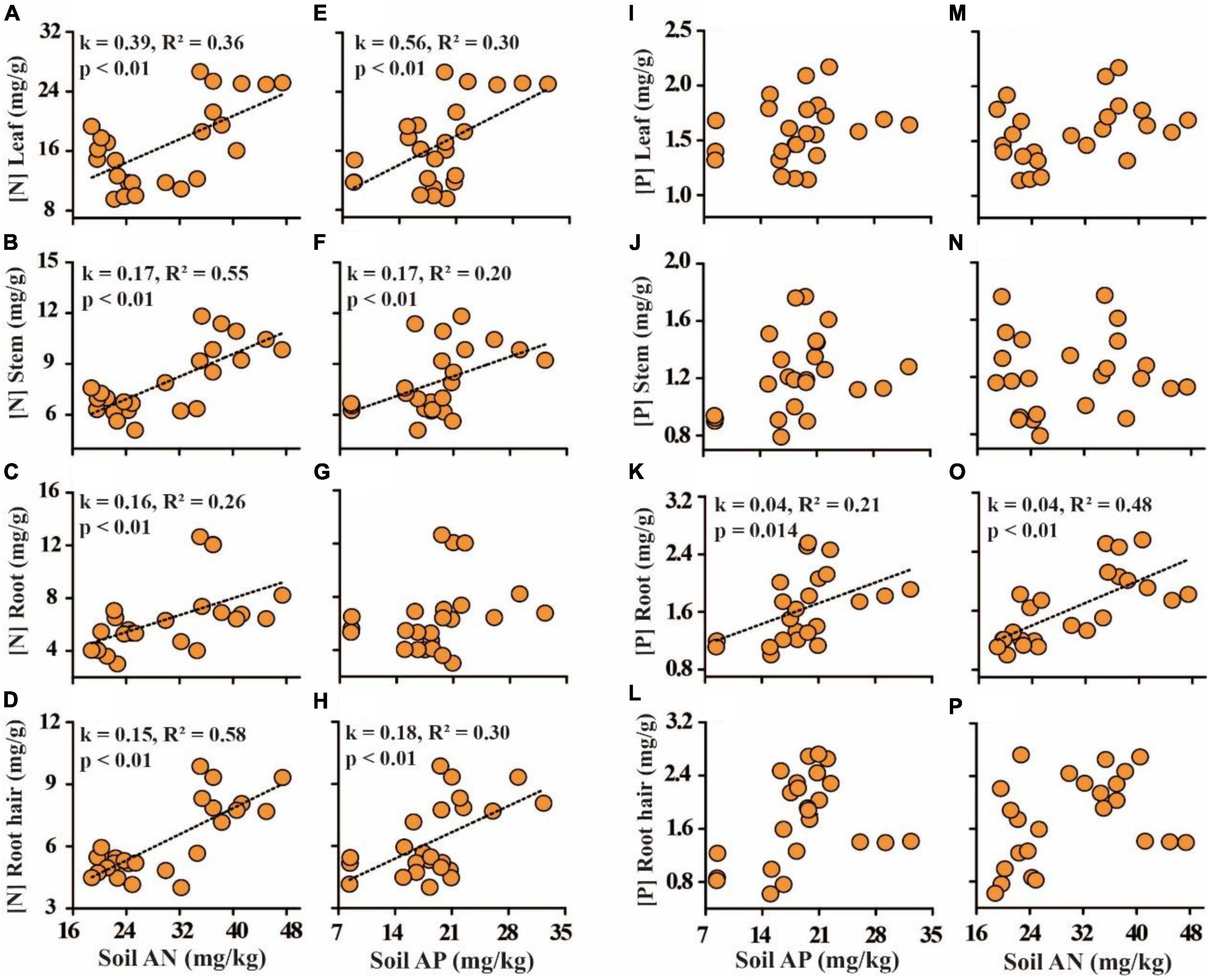
Figure 4. The relationship between soil nutrient availability on the N and P of plant tissues. (A–D) Soil available nitrogen (AN) and plant tissue N; (E–H) soil available phosphorus (AP) and plant tissue P; (I–L) soil AP and plant tissue P; (M–P) soil AN and plant tissue P.
Discussion
While the nutrient content of natural wetlands is determined by groundwater and rainfall, chemical fertilizers used during agricultural development possibly influenced the nutrient composition (Craft and Richardson, 1993). The nutrient loading not only enhances nutrient availability but also facilitates the accumulation of nutrients (Li et al., 2019). The findings of these studies are consistent with our results that TN, TP, and available N and P in the wetlands which exchange flow frequently with paddy (NW) were significantly higher than that in natural wetlands (Figures 2A,B). Although the content values of TN and TP in DW were similar to those in natural wetlands, available P significantly increased (Figure 2D). This is mainly because drainage increases soil oxygen availability, potentially accelerating soil nutrient cycling and influencing nutrient availability (Laiho et al., 1999). In cultivated wetlands, TN decreased significantly, while TP increased significantly (Figures 2A–D). Previously, it has been shown that changes in soil physical factors, water conditions, and nutrient levels contribute to N mineralization (Laiho et al., 1999; Raiesi, 2006; Wang et al., 2017). On the other hand, P cycling differs from N cycling since it does not involve atmospheric recharge, therefore soil P stocks are determined by P input and export with the flow (Nesme et al., 2018). Furthermore, soil minerals including Fe and Al oxidases have a strong adsorption capacity for orthophosphate ions (Negassa and Leinweber, 2009), which could possibly increase P retention in cultivated wetlands.
Plants often trade off nutrient allocation and environmental changes for growth and reproduction (Zhang et al., 2021). Our results showed that the N content values in plant leaves, stems, roots, and root hair in NW were significantly higher than those in the RW (Figures 3A–D). Interestingly, the N content values in leaves, roots, and root hair were similar to those in nutrient-rich and DW. This may be explained by the complementarity of environmental elements (nutrients vs. hydrology). Lower water levels reduce plant above- and below-ground biomass and inhibit plant height, leaf area, and root length (Bai et al., 2021). Declining biomass production and accumulation increase the relative content values of organ nutrients based on a fundamental trade-off in plant functioning between the rapid production of biomass and efficient conservation of nutrients (Garnier et al., 2001). In DW, microbial biomass and activity increased the coupling of nutrient mineralization. This is consistent with the findings of Brown et al. (2017) that draining significantly increased nitrification and P mineralization. In the cultivated wetlands, the leaf N content was similar to that in the RW, but the N content values in the stem, root, and root hair were significantly lower (Figures 3A–D). N and P availability in the study wetlands were lower than those in the RW (Figure 2), however, long-term agricultural fertilizer application possibly compensates for the plant’s nutrient requirements. Furthermore, long-term cultivation also reduces soil organic matter, thereby affecting plant nutrient uptake and utilization (Seiter and Horwath, 2004). Soil N and P availability showed strong positive effects on plant organ N, especially on plant leaf N in this study (Figures 4A–H). N is essential for photosynthetic C fixation and biomass accumulation in plants (Staswick, 1994), and adding N increases plant nitrate concentration (Güsewell, 2004; Esmeijer-Liu et al., 2009; Mao et al., 2014). The addition of P to plants also improves their capacity to absorb dissolved organic nitrogen in cold and nutrient-limited conditions (Feller et al., 2003; Mao et al., 2016).
Phosphorus is the second most essential element after nitrogen for the synthesis of nucleic acids and is a component of the adenosine phosphates, which play a key role in the transfer of energy (Elser et al., 1996). Despite different agricultural interventions, P levels in leaves, stems, and root hair remained similar (Figures 3E–H). The amount of root P in nutrient-rich and DW was significantly higher than that in agricultural and RW. As roots are the primary organs responsible for nutrient storage, they may have a strong capacity to retain phosphorus. In addition, our results showed a positive relationship between N and P availability and root P concentration in plants (Figures 4I–P). Plants have evolved a P economy when nutrient availability is limited, and they conserve nutrients through the resorption of nutrients from senescing to new leaves or storage in the roots (Rejmánková and Snyder, 2008). Plant P resorption efficiency can reach approximately 52% (Aerts, 1996; Huang et al., 2021). On the other hand, plants can acquire P by secreting hydrolytic enzymes such as phosphates, which could promote organic P mineralization (Rejmánková and Snyder, 2008). Plants can also reduce phosphate secretion when soil P availability increases (Olander and Vitousek, 2000).
N:P ratios reflect plant nutrient demand, which is a critical indicator to determine population size, stability, and organization (Koerselman and Meuleman, 1996). We found that the responses of the N:P ratios in plant organs varied significantly from roots to leaves (Figures 3I–L), which did not support the hypothesis that agricultural disturbance may have weak effects on the N:P ratios in plant organs. Both the root and root hair showed little variation in the N:P ratio under agricultural intervention, however, the N:P ratios of leaves and stems were higher in NW than that in the other sites (Figures 3I–L). Roots are important sources of energy storage, so they typically maintain a constant variation ratio in their functionality (Rejmánková and Snyder, 2008). Despite the fact that the aboveground biomass is often reduced in DW, the N:P ratio in the leaf is maintained to meet its photosynthesis requirements for growth and reproduction (Staswick, 1994). A mass N:P ratio of less than 14 or greater than 16 indicates N or P limitation, respectively, and a value between 14 and 16 indicates N and P co-limitation (Koerselman and Meuleman, 1996). In this study, the N:P ratio was less than 14 in all wetlands, indicating that N had a determinant effect on G. spiculosa, and high nutrient levels also increased its requirement for nutrients. Interestingly, cultivation and drainage had similar effects on plant leaf N:P ratios, which were higher than those of the RW (Figures 3I–L). These findings indicate that plants exhibit high plasticity and subsequent self-regulation strategies under agricultural disturbances (Weiner, 2004).
Overall, the results of this study show that agricultural interventions significantly altered the N levels and nutrient stoichiometry of G. spiculosa, particularly in the leaves. Soil N and P availability co-influenced plant N levels, and G. spiculosa showed relatively high plasticity in mediating its nutrient stoichiometry in the plant organs under different agricultural interventions. In addition, roots are the most important organs for nutrient acquisition, storage, and transformation. Although their nutrient levels fluctuate with soil nutrient levels, they maintain a constant N to P ratio throughout, highlighting the plasticity of the roots as well as the importance of nutrient stoichiometry from root to leaf.
Data availability statement
The raw data supporting the conclusions of this article will be made available by the authors, without undue reservation.
Author contributions
TW, QL, and ZW contributed to the conception and design of the study. TW and QL performed the statistical analysis and wrote the first draft of the manuscript. ZD, CG, and XW contributed to manuscript revision. All authors contributed to the article and read and approved the submitted version.
Acknowledgments
We acknowledge the funders of this study; the National Natural Science Foundation of China (41830643 and 42101114) and the China Postdoctoral Science Foundation (2021M703199). We thank two reviewers, Abel B. Ahungu, and Wang Guodong for their valuable suggestions.
Conflict of interest
The authors declare that the research was conducted in the absence of any commercial or financial relationships that could be construed as a potential conflict of interest.
Publisher’s note
All claims expressed in this article are solely those of the authors and do not necessarily represent those of their affiliated organizations, or those of the publisher, the editors and the reviewers. Any product that may be evaluated in this article, or claim that may be made by its manufacturer, is not guaranteed or endorsed by the publisher.
References
Aerts, R. (1996). Nutrient resorption from senescing leaves of perennials: Are there general patterns? J. Ecol. 84, 597–608. doi: 10.2307/2261481
Aerts, R., and Chapin, F. S. (1999). The mineral nutrition of wild plants revisited: A re-evaluation of processes and patterns. Adv. Ecol. Res. 30, 1–67. doi: 10.1016/S0065-2504(08)60016-1
Alvarez-Clare, S., and Mack, M. C. (2015). Do foliar, litter, and root nitrogen and phosphorus concentrations reflect nutrient limitation in a lowland tropical wet forest? PLoS One 10:e0123796. doi: 10.1371/journal.pone.0123796
Bai, J. S., Tang, H. R., Chen, F. Y., and Lou, Y. J. (2021). Functional traits response to flooding depth and nitrogen supply in the helophyte Glyceria spiculosa (Gramineae). Aquat. Bot. 175:103449.
Bedford, B. L., Walbridge, M. R., and Aldous, A. (1999). Patterns in nutrient availability and plant diversity of temperate North American wetlands. Ecology 80, 2151–2169. doi: 10.2307/176900
Brown, R. L., Hangs, R., Schoenau, J., and Bedard-Haughn, A. (2017). Soil nitrogen and phosphorus dynamics and uptake by wheat grown in drained prairie soils under three moisture scenarios. Soil Sci. Soc. Am. J. 81, 1496–1504. doi: 10.2136/sssaj2017.01.0036
Craft, C. B., and Richardson, C. J. (1993). Peat accretion and N, P, and organic C accumulation in nutrient-enriched and unenriched everglades peatlands. Ecol. Appl. 3, 446–458. doi: 10.2307/1941914
Di Palo, F., and Fornara, D. A. (2017). Plant and soil nutrient stoichiometry along primary ecological successions: Is there any link? PLoS One 12:e0182569. doi: 10.1371/journal.pone.0182569
Elser, J. J., Dobberfuhl, D. R., MacKay, N. A., and Schampel, J. H. (1996). Organism size, life history, and N:P stoichiometry. Bioscience 46, 674–684. doi: 10.2307/1312897
Esmeijer-Liu, A. J., Aerts, R., Kurschner, W. M., Bobbink, R., Lotter, A. F., and Verhoeven, J. T. A. (2009). Nitrogen enrichment lowers Betula pendula green and yellow leaf stoichiometry irrespective of effects of elevated carbon dioxide. Plant Soil 316, 311–322. doi: 10.1007/s11104-008-9783-1
Feller, I. C., McKee, K. L., Whigham, D. F., and O’Neill, J. P. (2003). Nitrogen vs. phosphorus limitation across an ecotonal gradient in a mangrove forest. Biogeochemistry 62, 145–175. doi: 10.1023/A:1021166010892
Garnier, E., Laurent, G., Bellmann, A., Debain, S., Berthelier, P., Ducout, B., et al. (2001). Consistency of species ranking based on functional leaf traits. New Phytol. 152, 69–83. doi: 10.1046/j.0028-646x.2001.00239.x
Guiz, J., Hillebrand, H., Borer, E. T., Abbas, M., Ebeling, A., Weigelt, A., et al. (2016). Long-term effects of plant diversity and composition on plant stoichiometry. Oikos 125, 613–621. doi: 10.1111/oik.02504
Güsewell, S. (2004). N:P ratios in terrestrial plants: Variation and functional significance. New Phytol. 164, 243–266. doi: 10.1111/j.1469-8137.2004.01192.x
Huang, Y., Lou, C., Luo, L., and Wang, X. C. (2021). Insight into nitrogen and phosphorus coupling effects on mixotrophic Chlorella vulgaris growth under stably controlled nutrient conditions. Sci. Total Environ. 752:141747. doi: 10.1016/j.scitotenv.2020.141747
Koerselman, W., and Meuleman, A. F. M. (1996). The vegetation N:P ratio: A new tool to detect the nature of nutrient limitation. J. Appl. Ecol. 33, 1441–1450. doi: 10.2307/2404783
Kramer-Walter, K. R., Bellingham, P. J., Millar, T. R., Smissen, R. D., Richardson, S. J., Laughlin, D. C., et al. (2016). Root traits are multidimensional: Specific root length is independent from root tissue density and the plant economic spectrum. J. Ecol. 104, 1299–1310. doi: 10.1111/1365-2745.12562
Laiho, R., Sallantaus, T., and Laine, J. (1999). The effect of forestry drainage on vertical distributions of major plant nutrients in peat soils. Plant Soil 207, 169–181. doi: 10.1023/A:1026470212735
Li, F., Hu, J. Y., Xie, Y. H., Yang, G. S., Hu, C., Chen, X. S., et al. (2018). Foliar stoichiometry of carbon, nitrogen, and phosphorus in wetland sedge Carex brevicuspis along a small-scale elevation gradient. Ecol. Indic. 92, 322–329. doi: 10.1016/j.ecolind.2017.04.059
Li, T., Bu, Z. J., Liu, W. Y., Zhang, M. Y., Peng, C. H., Zhu, Q. A., et al. (2019). Weakening of the ‘enzymatic latch’ mechanism following long-term fertilization in a minerotrophic peatland. Soil Biol. Biochem. 136:107528.
Luo, W. B., Xie, Y. H., Chen, X. S., Li, F., and Qin, X. Y. (2010). Competition and facilitation in three marsh plants in response to a water-level gradient. Wetlands 30, 525–530. doi: 10.1007/s13157-010-0064-4
Mao, R., Chen, H. M., Zhang, X. H., Shi, F. X., and Song, C. C. (2016). Effects of P addition on plant C:N:P stoichiometry in an N-limited temperate wetland of Northeast China. Sci. Total Environ. 559, 1–6. doi: 10.1016/j.scitotenv.2016.03.158
Mao, R., Zhang, X. H., and Song, C. C. (2014). Effects of nitrogen addition on plant functional traits in freshwater wetland of Sanjiang Plain, Northeast China. Chin. Geogr. Sci. 24, 674–681. doi: 10.1007/s11769-014-0691-4
Moor, H., Rydin, H., Hylander, K., Nilsson, M. B., Lindborg, R., and Norberg, J. (2017). Towards a trait-based ecology of wetland vegetation. J. Ecol. 105, 1623–1635. doi: 10.1111/1365-2745.12734
Negassa, W., and Leinweber, P. (2009). How does the Hedley sequential phosphorus fractionation reflect impacts of land use and management on soil phosphorus: A review. J. Plant Nutr. Soil Sci. 172, 305–325. doi: 10.1002/jpln.200800223
Nesme, T., Metson, G. S., and Bennett, E. M. (2018). Global phosphorus flows through agricultural trade. Glob. Environ. Chang. 50, 133–141. doi: 10.1016/j.gloenvcha.2018.04.004
Olander, L. P., and Vitousek, P. M. (2000). Regulation of soil phosphatase and chitinase activity by N and P availability. Biogeochemistry 49, 175–191. doi: 10.1023/a:1006316117817
Qin, L., Freeman, C., Jia, X. Y., Zhang, Z. S., Liu, B., Zhang, S. Q., et al. (2021). Microbial enzyme activity and stoichiometry signal the effects of agricultural intervention on nutrient cycling in peatlands. Ecol. Indic. 122:107242.
R Core Team (2019). R: A language and environment for statistical computing. Vienna: R Foundation for Statistical Computing.
Raiesi, F. (2006). Carbon and N mineralization as affected by soil cultivation and crop residue in a calcareous wetland ecosystem in Central Iran. Agric. Ecosyst. Environ. 112, 13–20. doi: 10.1016/j.agee.2005.07.002
Rejmánková, E., and Snyder, J. M. (2008). Emergent macrophytes in phosphorus limited marshes: Do phosphorus usage strategies change after nutrient addition? Plant Soil 313, 141–153. doi: 10.1007/s11104-008-9687-0
Seiter, S., and Horwath, W. R. (2004). “Strategies for managing soil organic matter to supply plant nutrients,”in Soil organic matter in sustainable agriculture, eds F. Magdoff and R. Weil (Boca Raton, FL: CRC Press), 269–293. doi: 10.1201/9780203496374.ch9
Staswick, P. E. (1994). Storage proteins of vegetative plant tissues. Annu. Rev. Plant Physiol. Plant Mol. Biol. 45, 303–322. doi: 10.1146/annurev.pp.45.060194.001511
Sterner, R. W., and Elser, J. J. (2003). Ecological stoichiometry: The biology of elements from molecules to the biosphere. Princeton, NJ: Princeton University Press. doi: 10.1515/9781400885695
Wang, G., Otte, M. L., Jiang, M., Wang, M., Yuan, Y., and Xue, Z. (2019). Does the element composition of soils of restored wetlands resemble natural wetlands? Geoderma 351, 174–179. doi: 10.1016/j.geoderma.2019.05.032
Wang, Z. C., Liu, S. S., Huang, C., Liu, Y. Y., and Bu, Z. J. (2017). Impact of land use change on profile distributions of organic carbon fractions in peat and mineral soils in Northeast China. Catena 152, 1–8. doi: 10.1016/j.catena.2016.12.022
Weiner, J. (2004). Allocation, plasticity and allometry in plants. Perspect. Plant Ecol. 6, 207–215. doi: 10.1078/1433-8319-00083
Yu, Q., Wilcox, K., La Pierre, K., Knapp, A. K., Han, X., and Smith, M. D. (2015). Stoichiometric homeostasis predicts plant species dominance, temporal stability, and responses to global change. Ecology 96, 2328–2335. doi: 10.1890/14-1897.1
Zedler, J. B., and Kercher, S. (2005). Wetland resources: Status, trends, ecosystem services, and restorability. Annu. Rev. Environ. Resour. 30, 39–74. doi: 10.1146/annurev.energy.30.050504.144248
Zhang, D. J., Qi, Q., Tong, S. Z., Wang, J., Zhang, M. Y., Zhu, G. L., et al. (2021). Effect of hydrological fluctuation on nutrient stoichiometry and trade-offs of Carex schmidtii. Ecol. Indic. 120:106924.
Keywords: wetland, stoichiometry, plant organs, nutrient, drainage, agricultural intervention
Citation: Wei T, Dongjie Z, Guanglan C, Wanling X, Weihong Z and Lei Q (2022) Effect of agricultural intervention on nutrient stoichiometry from root to leaf in the helophyte species Glyceria spiculosa. Front. Ecol. Evol. 10:964198. doi: 10.3389/fevo.2022.964198
Received: 08 June 2022; Accepted: 18 July 2022;
Published: 03 August 2022.
Edited by:
He Yixin, Key Laboratory of Mountain Ecological Rehabilitation and Biological Resource Utilization, Chengdu Institute of Biology (CAS), ChinaReviewed by:
Meng Li, Chinese Academy of Forestry, ChinaHuan Meng, Shenyang Agricultural University, China
Copyright © 2022 Wei, Dongjie, Guanglan, Wanling, Weihong and Lei. This is an open-access article distributed under the terms of the Creative Commons Attribution License (CC BY). The use, distribution or reproduction in other forums is permitted, provided the original author(s) and the copyright owner(s) are credited and that the original publication in this journal is cited, in accordance with accepted academic practice. No use, distribution or reproduction is permitted which does not comply with these terms.
*Correspondence: Zhu Weihong, whzhu@ybu.edu.cn; Qin Lei, qinlei@iga.ac.cn