- 1Guizhou Provincial Key Laboratory for Information Systems of Mountainous Areas and Protection of Ecological Environment, Guizhou Normal University, Guiyang, China
- 2School of Architecture and Engineering, Shenzhen Polytechnic, Shenzhen, China
Forests habituated by Rhododendron delavayi often lack understory vegetation, which could possibly be a consequence of allelopathy. It is a phenomenon by virtue of which certain plant species produce allelochemicals that affect the growth and behavior of surrounding plants. To elucidate the allelopathic potential and allelochemicals present in the different layers of a R. delavayi forest, extracts obtained from three layers of the forest were used for seed germination bioassays and gas chromatography-mass spectrometry (GC–MS) analysis. Aqueous extracts of the litter and humus layers significantly inhibited the seed germination of R. delavayi, Festuca arundinacea, and Lolium perenne, with the litter layer causing the strongest inhibitory effect. A total of 26 allelochemicals were identified in the litter, humus, and soil layers by GC–MS analysis. The primary allelochemicals in the soil and humus layer were organic acids, while the main allelochemicals in the litter layer were phenolic acids. The redundancy analysis revealed the significance of total nitrogen (TN) and relative water content (RWC) in explaining the distribution of the allelochemicals. The results indicated that the litter layer exerted the maximum allelopathic effect due to presence of maximum amount of allelochemical especially the phenolic acids.
Introduction
Allelopathy refers to the phenomenontextbf by which metabolic secretions released by plants or microorganisms cause beneficial or detrimental effects on organisms in the local environment (Rice, 1992; Wei et al., 2020; Fernandez et al., 2021; Worsley et al., 2021). Dominant species tend to have strong allelopathic effects as their partially decomposed litter releases phytotoxins in their surrounding, thereby affecting community regeneration (Walbott et al., 2018; Huo et al., 2019; Huang et al., 2020). Allelochemicals also accumulate in the soil through leachation and root exudation (Medina-Villar et al., 2017; Gfeller et al., 2018; Luo et al., 2020). However, only a few studies have compared the allelopathic effects of phytochemicals present in the litter, humus, and soil layers that support plant communities, especially in natural forests.
The Rhododendron is the largest genus in Ericaceae family with approximately 1,000 species. These plants are mainly distributed in Asia and more than 650 species are found in China (MacKay and Gardiner, 2017). Certain Rhododendron species exert allelopathic effects on other plant species, and through this mechanism, they can form dominant communities. For instance, Rhododendron ponticum forests, which are extensively naturalized in the British Isles, produce chemicals that threaten other native communities (Manzoor et al., 2018; Jones et al., 2019). Similarly, Rhododendron maximum, an evergreen shrub invading Appalachian forests, also possesses strong allelopathic properties (Osburn et al., 2018; Raulerson et al., 2020).
Rhododendron delavayi Franch is a subtropical evergreen shrub native to East and Southeast Asia (Zhang et al., 2022). Its forests can be found in association with Rhododendron agastum and Rhododendron irroratum, covering some regions of the Yungui Plateau (Ma et al., 2016; Zhang et al., 2022). Except for a few grasses, no other vegetation survive beneath R. delavayi forests, potentially because of the allelochemicals released by its litter (Li et al., 2019). The allelopathic interactions that occur in the layers of forest floor can negatively affect the growth of understory plants, and most forests are characterized by thick litter and humus layers (Kato-Noguchi et al., 2017; Muturi et al., 2017). Previous studies have mainly focused on the invasiveness of Rhododendron, but only a few studies have focused on the allelopathic dynamics in Rhododendron forests.
In this study, we examined the allelopathic effects of phytochemicals released by R. delavayi in the soil ecosystem of Baili Rhododendron Nature Reserve, Guizhou Province, China. Our main objectives were as follows: (1) to assess the allelopathic effect of the litter, humus, and top soil layer of R. delavayi forest on its own germination the germination of two herbaceous species (Festuca arundinacea and Lolium perenne) that cohabitate the forest community; (2) to identify the allelochemicals in the litter, humus, and soil layers of R. delavayi forest that may be responsible for the observed allelopathic effects.
Materials and methods
Study site
The samples of soil layers were collected from Baili Rhododendron Nature Reserve, a typical Rhododendron forest park located in NW Guizhou Province, China (105°50′16′′−106°04′57′′E, 27°10′07′′−27°17′55′′N; altitude 1060–2121 m). The area is characterized by a mean annual temperature of 11.8°C and relative air humidity of 84% as recorded during the period of 2011–2021. The soil of the area is limestone type and the dominant native species is R. delavayi with trees over 100 years in age. The understory of these forests was characterized by a thick deciduous and humus layer (>10 cm). Though a few herb species, such as F. arundinacea and L. perenne, are present in the understory, but there existed an issue of poor natural regeneration and a lack of tree seedlings, as shown in Figure 1.
Three-layer sample collection
The forest soil is divided into three layers, namely, litter layer (senesced leaves and twigs deposited on forest floor), humus layer (decomposed part below litter layer; no longer recognizable as leaves or twigs), and top soil layer (soil below litter and humus layer). Samples of these three layers were collected from three sampling sites, i.e., Pudi (PD), Renhe (RH), and Gamu (GM), in the Baili Rhododendron Nature Reserve in December 2015 (Table 2). The samples were collected by five-point sampling method (Yun et al., 2016), stored in a cold box and immediately transported to the laboratory. The samples were air-dried in the shade, ground with an electric grinder, and passed through a 2-mm sieve.
The sampled layers were subjected to soil analysis in triplicates (one replicate per sampling site). To determine pH of the samples, they were mixed with deionized water in a ratio of 1–2.5 (w/v) and measured electrometrically with a glass electrode (model PHS-3C, LEICI, Shanghai, China). The relative water content (RWC) of each sample was determined as per the method suggested by Kampowski et al. (2018). Organic carbon (OC) was analyzed by dichromate oxidation and titration with ferrous sulfate (Nelson and Sommers, 1996). The total nitrogen (TN) of the samples was measured by the Kjeldahl method (Bremner and Mulvaney, 1982). Total phosphorous (TP) and total potassium (TK) were measured in the laboratory using standard procedures. The Mo-Sb colorimetric method was used to determine total phosphorus content, and the flame photometry method was used to determine TK content (Vogt et al., 2015).
Laboratory experiments
Seeds of the three test species, R. delavayi, F. arundinacea, and L. perenne were collected in the Baili Rhododendron Nature Reserve. The seeds were surface sterilized with 15% sodium hypochlorite for 20 min and then rinsed with distilled water. Seed germination experiments were conducted in germinating boxes where the seeds were placed on double-layered filter papers. Extracts of the sampled layers were prepared in triplicates (one replicate per sampling site) by mixing 10 g of powder with 30 ml of distilled water as suggested by Alrababah et al. (2009). These extracts were used to moisten the filter papers only once during the experiment. The germinating boxes were placed in a plant growth chamber (model BIC-400, BOXUN, Shanghai, China) at 25°C, 70% relative humidity and a 16/8 photoperiod of 1500 lux light. The seed germination of each plant species was determined under the following treatments: distilled water as the control, water extracts of the litter layer, water extracts of the humus layer, and water extracts of the soil layer. The germinated seeds were counted on daily basis up to 30 days. The experiments were replicated three times (100 seeds each time) and arranged in a completely randomized design. The percent inhibition of seed germination was calculated from the following equation:
Gas chromatography-mass spectrometry analysis of the extracts
Gas chromatography-mass spectrometry (GC–MS) analysis in the sampled layers was performed in triplicates (one replicate per sampling site). Initially, the samples (200 mg) were placed in a 10-ml centrifuge tube and then added with 80 μL adipic acid (235.2 μg/mL) and 5 mL methyl alcohol-water-chloroform solution (V:V = 5:3:2) (Zhang et al., 2013). The samples then underwent ultrasound-assisted extraction for 30 min. The resulting mixed solution was centrifuged for 10 min at 6,000 rpm and the supernatant was subsequently transferred into a 5-mL centrifuge tube to be evaporated under a nitrogen stream at room temperature. Oximation and silylation procedures were applied for derivatization. All derivatives were transferred to a 100 μL polypropylene insert with polymer feet in a 2 mL septum vial and analyzed with GC–MS analysis. Adipic acid was used as the internal standard (purity >99.0%; Sigma–Aldrich, Saint Louis, MO, USA). Before GC–MS analysis, 1 μL of adipic acid solution was added to the samples.
The allelochemicals in the sample were investigated using a GC–MS system operating in EI mode. An HP-5 MS capillary column (60 m × 0.25 mm ID, film thickness of 0.25 mm) was directly coupled to the MS. The carrier gas used was helium with a flow rate of 1.0 ml/min. The oven temperature was programmed to operate at 60°C for 4 min, then increased from 60 to 280°C at a rate of 5°C per min, and thereafter, was held at 280°C for 5 min. The injector port and the detector each had a temperature of 280°C and the split ratio of 10:1. The parameters of the MS system were as follows: ion source of 70 eV EI at a temperature of 230°C, transfer line temperature of 280°C, a mass range of 36 to 600 amu for the collection, and solvent delay of 15 min. The identification of the allelochemicals was based on mass spectra (NIST08 and Wiley08) and the total ion chromatogram of the samples are shown in Supplementary Figure 1. The quantity of allelochemicals were calculated using the internal standard method (Li et al., 2019).
Data collection and statistical analysis
One-way analysis of variance (ANOVA) and least significant difference (LSD) post-hoc test by SPSS 19.0 software (IBM Corp., Armonk, NY, USA) was conducted to determine the significance of the differences among the sampled layers. CANOCO software (version 4.5, Microcomputer Power, Inc., Ithaca, NY, USA) was used to analyze the distribution of allelochemicals considering the three sampling sites (PD, RH, GM) as response variable indices, and RWC, pH, SOC, TN, TP, TK as the explanatory variables in a redundancy analysis (RDA). The “ggplot2” package of R (version 3.1.2, R Development Core Team, 2014) was used to draw the cluster heatmap to figure out interrelationship between different classes of allelochemicals and sampled layers.
Results
Soil properties of the Rhododendron delavayi forest
The properties and chemical composition of the R. delavayi forest layers are shown in Table 1. The litter layer was found to contain more nutrients than the humus and soil layer, such as OC, TN, total phosphate, and TK. The content of TN, total phosphate, TK, and pH in litter and humus layers was significantly higher than those in soil layer (p < 0.01). The content of OC varied significantly (p < 0.01) among the three layers in an order of litter layer >humus layer >soil layer (Table 1).
Allelopathic effect on the seed germination of different plants
The extracts significantly inhibited the seed germination of the three tested plant species. The litter extract had the highest inhibitory effect on the germination of the seeds, and there was a significant difference in the germination rate of test plants subjected to the extracts of different layers (p < 0.01), as shown in Figure 2.
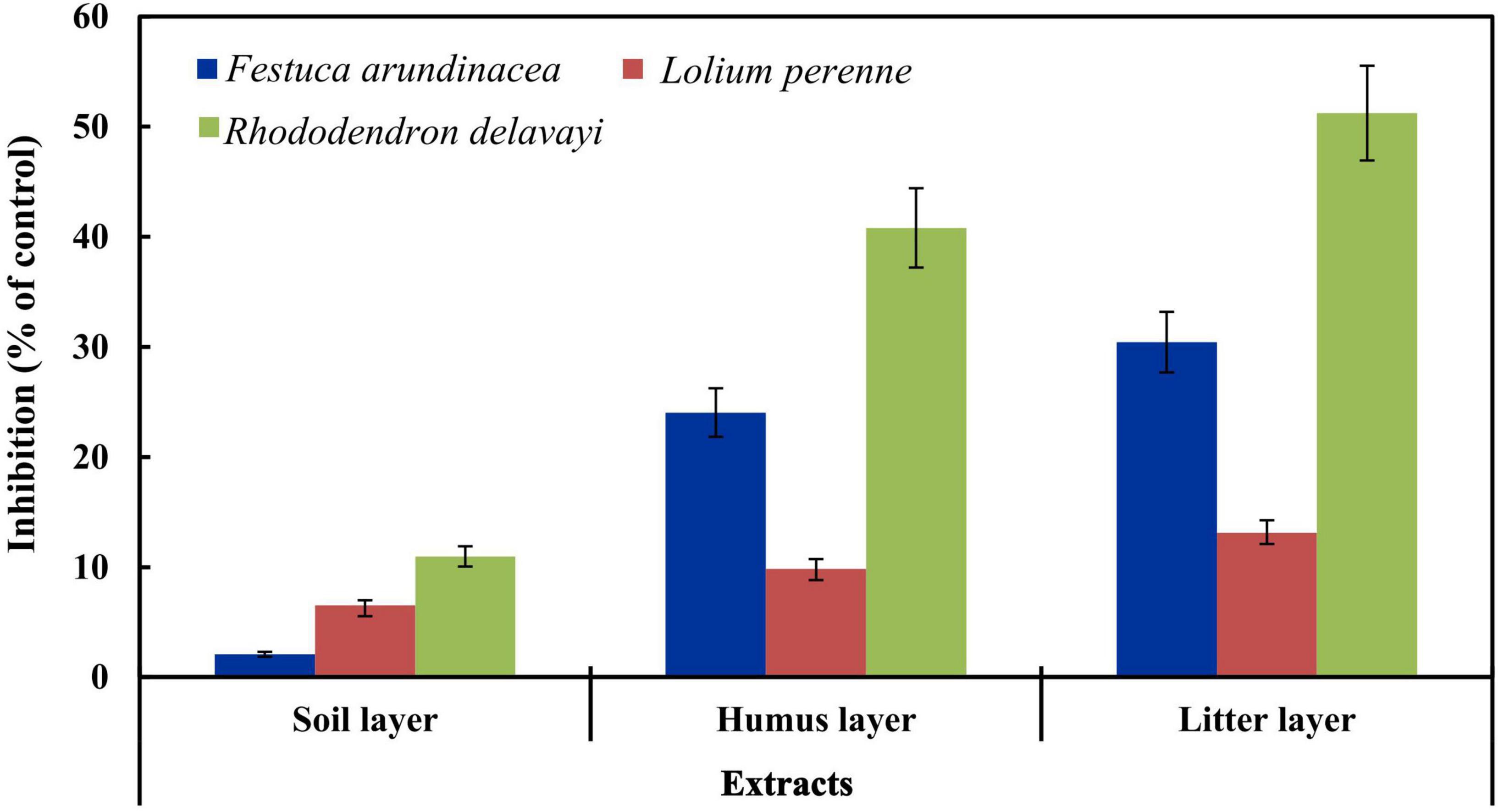
Figure 2. Inhibitory effects of extracts on seed germination. Vertical bars represent the standard deviations (n = 3).
Extracts from all the three layers reduced the germination of test species compared to the control. One-way ANOVA showed that there was a significant difference between the treatments (p < 0.01) in case of F. arundinacea. Contrastingly L. perenne, there were no significant differences in the inhibitory effect of the humus and the soil layer extract, as shown in Figure 2.
The extracts had the strongest inhibitory effect on the seed germination of R. delavayi, compared with the other two species of grass. The extracts reduced the germination rate of R. delavayi, and there was a significant difference between the treatments (p < 0.01). The order of percent inhibition of the extracts on the germination of R. delavayi was litter layer >humus layer >soil layer.
Allelochemicals in the three layers of the Rhododendron forest
Based on a predefined set of metabolites known to be allelochemicals (Reigosa and Gonzalez, 2006; Shen et al., 2022), a total of 26 compounds, including fatty acids, organic acids, phenolic acids, amino acids, and alcohols, were found to be present in the sampled layers (Table 2). There were four allelochemicals with content greater than 30 ng/g found in the soil layer, namely, palmitic acid, lactic acid, stearic acid, and glycolic acid, whose average concentrations were 93.41, 81.17, 39.94, and 31.01 ng/g, respectively. The main allelochemicals in the humus layer included glycolic acid, palmitic acid, glycerol, and inositol at relative concentrations of 130.94, 96.55, 41.88, and 41.64 ng/g, respectively. The main allelochemicals in the litter layer included palmitic acid, glycerol, stearic acid, inositol, glycolic acid, and protocatechuic acid, at the relative concentrations of 227.28, 113.79, 59.19, 50.29, 40.82, and 34.89 ng/g, respectively (Table 2).
The total amounts of allelochemicals in the litter, humus, and soil layers were 688.81, 479.13, and 328.93 ng/g, respectively, and there was a significant difference among three layers (p < 0.01). The concentration of fatty acids and alcohols gradually decreased with decomposition and there was a significant difference among the three layers (p < 0.01). The concentration of organic acids in the humus and soil layer were significantly higher than that of the litter layer (p < 0.01). The concentration of amino acids was the highest in the soil layer, and there was a significant difference among all three layers (p < 0.01). The concentration of phenolic acids in the litter layer (265.16 ng/g) was significantly higher than that of the humus and soil layers (p < 0.01). Phenolic acids were the most abundant allelochemicals found in the samples obtained from the investigated Rhododendron forest.
Environmental factors and allelochemicals of the Rhododendron forest
The redundancy analysis (RDA) showed that 97.1% of the variance was explained by the first and second axis. TN and RWC were the main environmental factors affecting allelochemicals, explaining 74.2 and 20.0% of the chemicals, respectively. TN had the highest negative correlation with the first axis (r = −0.9677), as well as strong negative correlations with 3-hydroxybenzoic acid, lactic acid, and malic acid. It had a strong positive correlation with oleic acid and docosanoic acid. The RWC had the highest positive correlation with the second axis (r = 0.5154) and positive correlations with myristic acid, eicosanoic acid, pentadecanoic acid, behenyl alcohol, phenylacetic acid, 4-hydroxybenzoic acid, and α-linolenic acid, as shown in Figure 3.
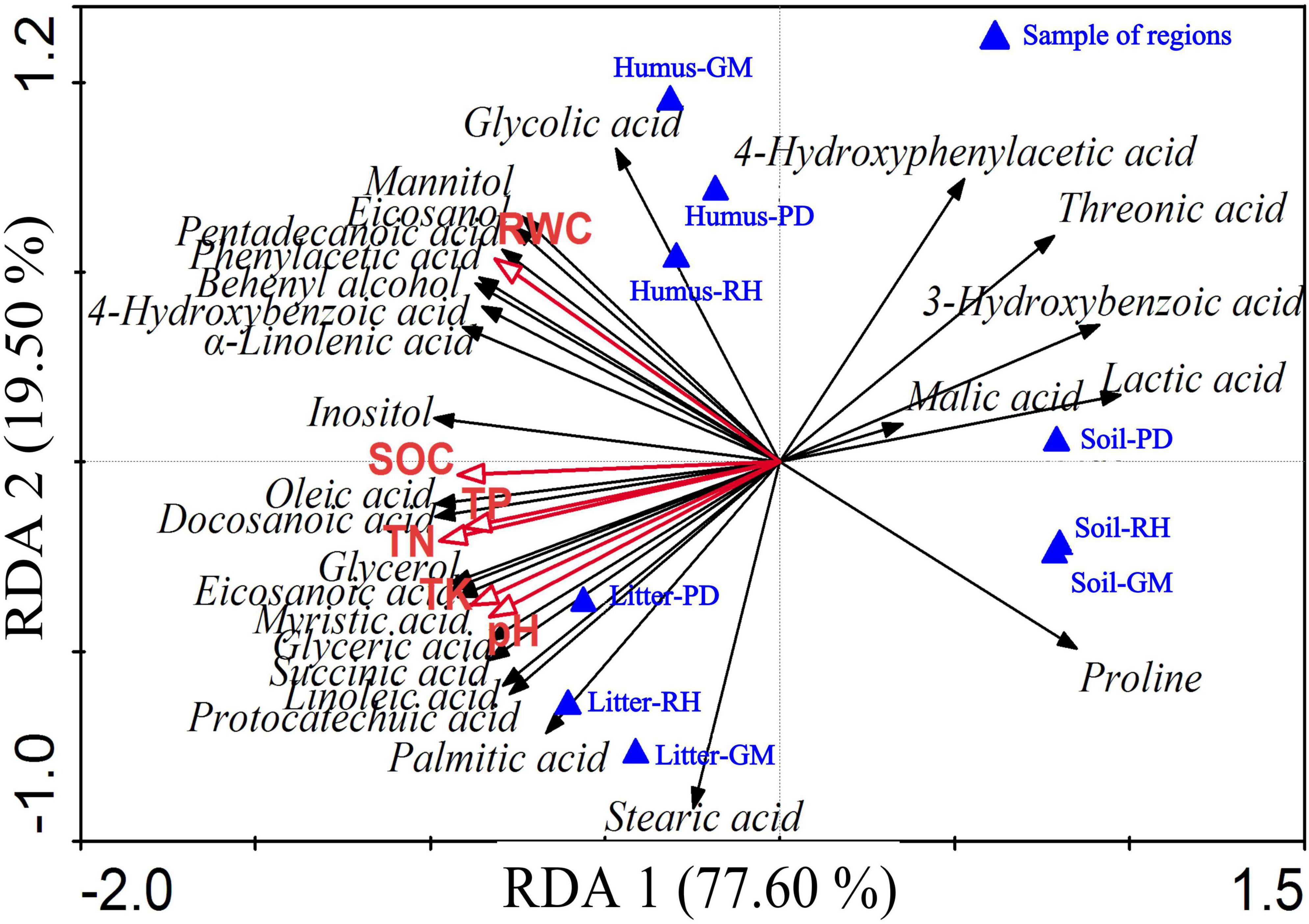
Figure 3. Redundancy analysis between chemicals and soil layers. RWC, SOC, TN, TP, and TK denote relative water content, soil organic carbon, total nitrogen, total phosphorous, and total potassium, respectively; Renhe (RH), Pudi (PD), and Gamu (GM) denote the three regions.
The clustering tree diagram shows that the allelochemicals could be divided into two categories. Phenolic acids, fatty acids, and alcohols were clustered into one category, while organic acids and amino acids were clustered into another category. The vertical clustering tree diagram shows the humus and soil layers clustered into one category, and the litter layer alone as a category, as shown in Figure 4. Among the detected allelochemicals, phenolic acids and fatty acids showed the strongest positive correlation with the litter layer samples obtained from three regions (RH, PD, and GM). Conversely, organic acids exhibited the strongest positive correlation with the humus layer samples obtained from three regions, while the amino acids exhibited the strongest positive correlation with the soil layer samples. However, the alcohols and fatty acids were negatively correlated with the soil layer samples obtained from three regions (Figure 4).
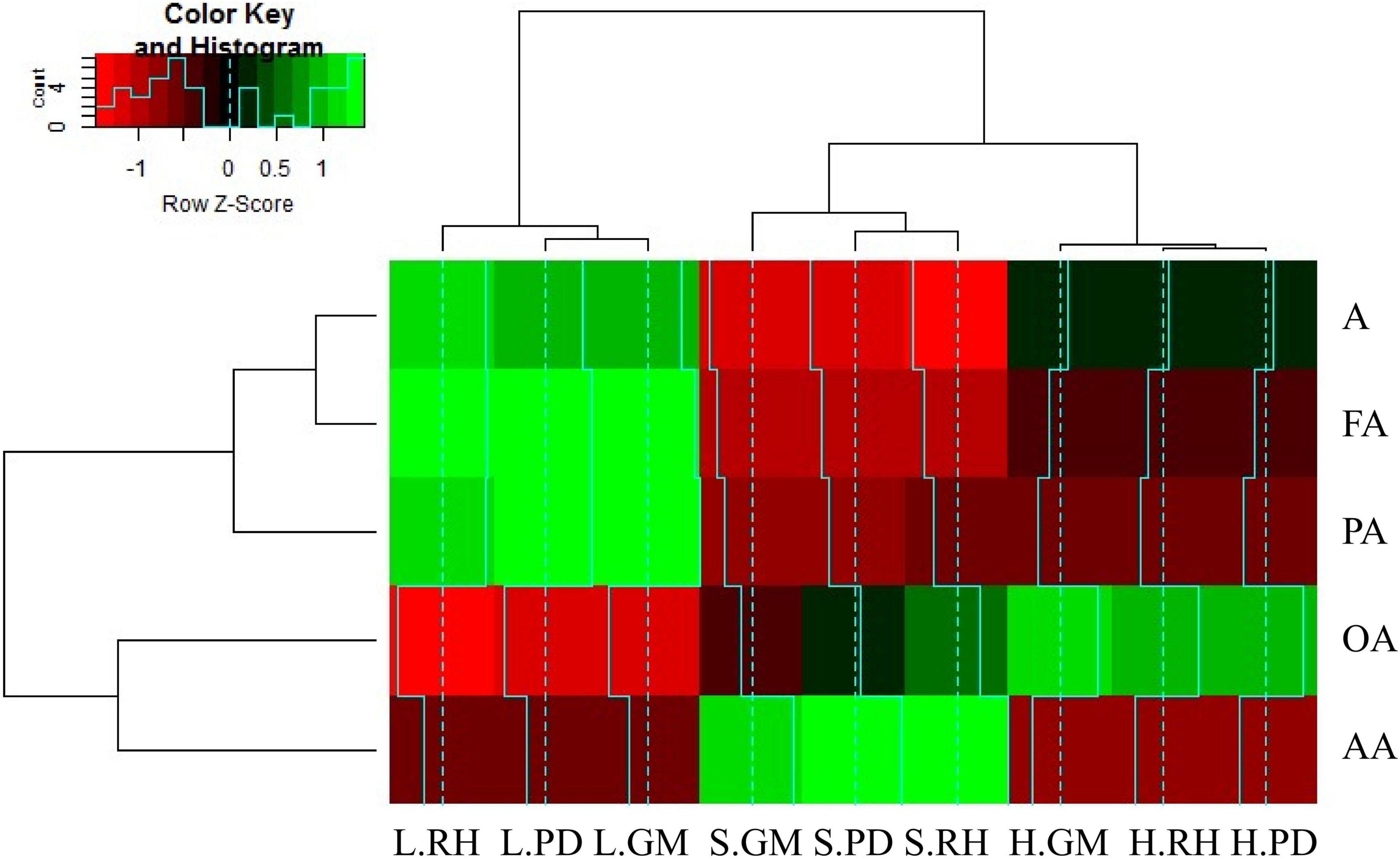
Figure 4. Hierarchical cluster analysis heatmap between chemical components and soil layers. A, HFA, PA, OA, and AA denote alcohols, higher fatty acids, phenolic acids, organic acids, and amino acids, respectively; L, H, and S indicate litter layer, humus layer, and soil layer, respectively; Renhe (RH), Pudi (PD), and Gamu (GM) denote the three regions.
Discussion
Seed germination is the most crucial stage in the life cycle of a plant species and it is often inhibited or delayed under the effect of allelochemicals (Fernandez et al., 2013). In previous studies, phytotoxic effects were found to reduce the seed germination rate (Chotsaeng et al., 2017; Luo et al., 2017). This study showed that the inhibition of seed germination in R. delavayi was stronger than that of the two herbaceous species (F. arundinacea and L. perenne). These results are consistent with the poor regeneration observed under the Rhododendron forest. The difficulty in regeneration of the Rhododendron forest can be attributed to the chemical legacy effects (Maclean et al., 2018). Seed germination inhibition has also been observed in bioassays using Lactuca sativa L. grown in Rhododendron litter leachates (Chou et al., 2010).
We found the three soil layers to be quite acidic in nature, and such a low pH value may have its own effect on the seed germination. Seed germination of some species are negatively affected by low pH values, such as Cenchrus biflorus (Masood and Muhammad, 2018) and Ambrosia artemisiifolia (Gentili et al., 2018); In contrast, good germination occurs at low pH value, was reported in Origanum compactum (Laghmouchi et al., 2017). Some studies have shown that pH has no effect on the Cerastium glomeratum seed germination (Park et al., 2020). Current results have shown that plant tolerance to pH is species-dependent, and the pH range suitable for seed germination is quite wide (Ortiz et al., 2019). Therefore, we prefer that allelochemicals inhibit seed germination of the three test species.
Forest litter is an important component of forest ecosystems, and it affects the natural regeneration of forests through both physical and chemical factors (Liu et al., 2018; Jin et al., 2019; Veen et al., 2019; Luo et al., 2020). Plants produce numerous secondary metabolites, including unique compounds that are only produced by specific plant species (Jones et al., 2019). After being liberated into the soil via decomposition of plant litter, some compounds with allelopathic potential affect the germination and growth of understory plant species (Jin et al., 2019). Similar findings were obtained in response to the litter produced by Cunninghamia lanceolata in greenhouse experiments (Liu et al., 2017). In this study, extracts obtained from the litter layer of Rhododendron forest demonstrated the strongest inhibitory effect on seed germination, which may be related to their high content of allelochemicals. Previous studies have suggested that the partial removal of forest litter could reduce its inhibitory effect (Osburn et al., 2018; Huo et al., 2019). The litter of Rhododendron forests is rich in allelochemicals, so the removal of litter material can possibly promote natural forest regeneration. A thicker litter layer negatively affects natural regeneration and seedling recruitment (Uddin and Robinson, 2017). While this is known of the litter layer, further in-depth research on the humus and soil layers is still needed.
The effectiveness of allelochemicals is controlled by soil factors (Abd-ElGawad et al., 2020). Generally, the secretion of allelochemicals is regulated by pH levels and nitrogen levels (Sun et al., 2022). In this study, RWC, SOC, TP, TN, TK, and pH were found to determine allelopathic effects. In addition, 4-hydroxyphenylacetic acid, threonic acid, 3-hydroxybenzoic acid, lactic acid, malic acid, and proline were found to be negatively correlated with these physical and chemical indicators. This study revealed the existence of chemicals with great phytotoxic potential in litter samples but not in soil samples. This result may be related to the higher relative concentrations of carbon and nitrogen that reside in the litter compared to other layers (Zheng et al., 2018).
Allelochemicals from exudates play an important role in regulating soil nutrient cycling (Keiluweit et al., 2015). TN is the main driver of soil microbial activity and allelopathy is often manifested through microbial activity (Qu et al., 2021). Similarly, fate of allelochemicals are regulated by water availability in the soil (Novoa et al., 2012). In accordance with the previous studies, our results show the significance of both TN and RWC in explaining the distribution of allelochemicals in RDA (Novoa et al., 2012; Qu et al., 2021).
Phenolic acids are the most potent allelochemicals that are mainly released by leaching and affect both understory plants and resident soil organisms, such as bacteria, fungi, and arthropods (Gavinet et al., 2019; Li et al., 2020). In addition, phenolic acids can form polyphenol organic complexes that are difficult to degrade; these complexes reduce nitrogen mineralization, which in turn reduces the availability of inorganic nitrogen in the soils (Horton et al., 2009; Wurzburger and Hendrick, 2009). Phenolic acid was found to be highly concentrated in the Rhododendron forest samples, especially those of the litter layer. The results of this study showed that phenolic acids were mainly present in the litter but did not produce enrichment effects in the soil. Therefore, the soil layer, as characterized by a low phenolic acid content, serves as an effective substrate for seed germination and seedling growth, and represents a key layer for overcoming barriers to forest regeneration.
Conclusion
Allelopathy plays an important role in the inhibition of natural regeneration of Rhododendron forests. In the present study, the litter layer sampled from the understory of R. delavayi forest was found to exert the maximum negative effect on the seed germination rate. Under natural conditions, the thicker litter layer could reduce the chances of seed contact with the soil layer. The descending content of allelochemicals in the different layers followed the order of litter layer >humus layer >soil layer, and the main allelochemicals across the three layers were phenolic acids. The RDA results revealed the significance of TN and RWC in explaining the distribution of the allelochemicals. The cluster heatmap demonstrated that the characteristics of the allelochemicals were similar in the humus and soil layer, and markedly different from those in the litter layer. This research provides insights into the allelopathic differences among three soil layers of Rhododendron forest and the major allelochemicals involved in the process. The study identifies the role of litter layer in inhibition of seedling recruitment and suggest litter removal under forest management programs to promote Rhododendron forest regeneration.
Data availability statement
The original contributions presented in this study are included in the article/Supplementary material, further inquiries can be directed to the corresponding authors.
Author contributions
CL conceived the structure of the manuscript. WQ and AW collected materials and data. WQ wrote the manuscript. CL and LX revised and approved the manuscript. All authors contributed to the article and approved the submitted version.
Funding
This work was supported by the National Natural Science Foundation of China (31960312) and the Guizhou Provincial Characteristic Key Laboratory (QJHKY [2021]002).
Conflict of interest
The authors declare that the research was conducted in the absence of any commercial or financial relationships that could be construed as a potential conflict of interest.
Publisher’s note
All claims expressed in this article are solely those of the authors and do not necessarily represent those of their affiliated organizations, or those of the publisher, the editors and the reviewers. Any product that may be evaluated in this article, or claim that may be made by its manufacturer, is not guaranteed or endorsed by the publisher.
Supplementary material
The Supplementary Material for this article can be found online at: https://www.frontiersin.org/articles/10.3389/fevo.2022.963116/full#supplementary-material
References
Abd-ElGawad, A. M., El-Amier, Y. A., Assaeed, A. M., and Al-Rowaily, S. L. (2020). Interspecific variations in the habitats of Reichardia tingitana (L.) Roth leading to changes in its bioactive constituents and allelopathic activity. Saudi J. Biol. Sci. 27, 489–499. doi: 10.1016/j.sjbs.2019.11.015
Alrababah, M. A., Tadros, M. J., Samarah, N. H., and Ghosheh, H. (2009). Allelopathic effects of Pinus halepensis and Quercus coccifera on the germination of mediterranean crop seeds. New For. 38, 261–272. doi: 10.1007/s11056-009-9145-8
Bremner, J. M., and Mulvaney, C. S. (1982). “Nitrogen-total,” in Methods of Soil Analysis, Part 2, Chemical and Microbial Properties. 9. Agronomy Society of America, Agronomy Monograph, eds A. L. Page, R. H. Miller, and D. R. Keeney (Wisconsin: Madison), 595–624. doi: 10.2134/agronmonogr9.2.2ed.c31
Chotsaeng, N., Laosinwattana, C., and Charoenying, P. (2017). Herbicidal activities of some allelochemicals and their synergistic behaviors toward Amaranthus tricolor L. Molecules 22:1841. doi: 10.3390/molecules22111841
Chou, S. C., Huang, C. H., Hsu, T. W., Wu, C. C., and Chou, C. H. (2010). Allelopathic potential of Rhododendron formosanum Hemsl in Taiwan. Allelopath. J. 25, 73–91. doi: 10.1021/ja308350f
Fernandez, C., Santonja, M., Gros, R., Monnier, Y., Chomel, M., Baldy, V., et al. (2013). Allelochemicals of Pinus halepensis as drivers of biodiversity in Mediterranean open mosaic habitats during the colonization stage of secondary succession. J. Chem. Ecol. 39, 298–311. doi: 10.1007/s10886-013-0239-6
Fernandez, M., Malagoli, P., Gallet, C., Fernandez, C., Vernay, A., Améglio, T., et al. (2021). Investigating the role of root exudates in the interaction between oak seedlings and purple moor grass in temperate forest. For. Ecol. Manag. 491:119175. doi: 10.1016/j.foreco.2021.119175
Gavinet, J., Santonja, M., Baldy, V., Hashoum, H., Peano, S., Tchong, T., et al. (2019). Phenolics of the understory shrub Cotinus coggygria influence mediterranean oak forests diversity and dynamics. For. Ecol. Manag. 441, 262–270. doi: 10.1016/j.foreco.2019.03.049
Gentili, R., Ambrosini, R., Montagnani, C., Caronni, S., and Citterio, S. (2018). Effect of soil pH on the growth, reproductive investment and pollen allergenicity of Ambrosia artemisiifolia L. Front. Plant Sci. 9:1335. doi: 10.3389/fpls.2018.01335
Gfeller, A., Glauser, G., Etter, C., Signarbieux, C., and Wirth, J. (2018). Fagopyrum esculentum alters its root exudation after Amaranthus retroflexus recognition and suppresses weed growth. Front. Plant Sci. 9:50. doi: 10.3389/fpls.2018.00050
Horton, J. L., Clinton, B. D., Walker, J. F., Beier, C. M., and Nilsen, E. T. (2009). Variation in soil and forest floor characteristics along gradients of ericaceous, evergreen shrub cover in the southern appalachians. Castanea 74, 340–352. doi: 10.2179/08-016R3.1
Huang, W., Reddy, G. V. P., Shi, P., Huang, J., Hu, H., and Hu, T. (2020). Allelopathic effects of Cinnamomum septentrionale leaf litter on Eucalyptus grandis saplings. Glob. Ecol. Conserv. 21:e00872. doi: 10.1016/j.gecco.2019.e00872
Huo, X., Wang, D., Bing, D., Li, Y., Kang, H., Yang, H., et al. (2019). Appropriate removal of forest litter is beneficial to Pinus tabuliformis Carr. regeneration in a pine and oak mixed forest in the Qinling mountains. China. Forests 10:735. doi: 10.3390/f10090735
Jin, M., Wang, Z., He, Z., Jiang, L., Liu, J., Lan, Y., et al. (2019). Allelopathic effect of Castanopsis kawakamii forest litter on seed germination of small Philippine Acacia (Acacia confusa). Appl. Ecol. Environ. Res. 17, 15103–15116. doi: 10.15666/aeer/1706_1510315116
Jones, G. L., Tomlinson, M., Owen, R., Scullion, J., Winters, A., Jenkins, T., et al. (2019). Shrub establishment favoured and grass dominance reduced in acid heath grassland systems cleared of invasive Rhododendron ponticum. Sci. Rep. 9:2239. doi: 10.1038/s41598-019-38573-z
Kampowski, T., Demandt, S., Poppinga, S., and Speck, T. (2018). Kinematical, structural and mechanical adaptations to desiccation in poikilohydric Ramonda myconi (Gesneriaceae). Front. Plant Sci. 9:1701. doi: 10.3389/fpls.2018.01701
Kato-Noguchi, H., Kimura, F., Ohno, O., and Suenaga, K. (2017). Involvement of allelopathy in inhibition of understory growth in red pine forests. J. Plant Physiol. 218, 66–73. doi: 10.1016/j.jplph.2017.07.006
Keiluweit, M., Bougoure, J., Nico, P., Pett-Ridge, J., Weber, P. K., and Kleber, M. (2015). Mineral protection of soil carbon counteracted by root exudates. Nat. Clim. Change 5, 588–595. doi: 10.1038/nclimate2580
Laghmouchi, Y., Belmehdi, O., Bouyahya, A., Skali Senhaji, N., and Abrini, J. (2017). Effect of temperature, salt stress and pH on seed germination of medicinal plant Origanum compactum. Biocatal. Agric. Biotechnol. 10, 156–160. bcab.2017.03.002. doi: 10.1016/j
Li, C., Quan, W., Qian, C., and Wu, Y. (2019). Distribution of chemical compounds in different soil layers of rhododendron forest. Allelopathy J. 48, 191–202. doi: 10.26651/allelo.j/2019-48-2-1254
Li, Y., Jian, X., Li, Y., Zeng, X., Xu, L., Khan, M. U., et al. (2020). OsPAL2-1 Mediates allelopathic interactions between rice and specific microorganisms in the rhizosphere ecosystem. Front. Microbiol. 11:1411. doi: 10.3389/fmicb.2020.01411
Liu, B., Daryanto, S., Wang, L., Li, Y., Liu, Q., Zhao, C., et al. (2017). Excessive accumulation of Chinese fir litter inhibits its own seedling emergence and early growth—A greenhouse perspective. Forests 8:341. doi: 10.3390/f8090341
Liu, B., Liu, Q., Daryanto, S., Ma, X., Guo, S., Wang, L., et al. (2018). Seedling emergence and early growth of Chinese fir under different light regimes and seed positions: Implications for natural regeneration. Can. J. For. Res. 48, 1034–1041. doi: 10.1139/cjfr-2017-0412
Luo, Y., Du, Z., Yan, Z., Zhao, X., Li, Y., Jiang, H., et al. (2020). Artemisia halodendron litters have strong negative allelopathic effects on earlier successional plants in a semi-arid sandy dune region in China. Front. Plant Sci. 11:961. doi: 10.3389/fpls.2020.00961
Luo, Y., Zhao, X., Li, Y., and Wang, T. (2017). Effects of foliage litter of a pioneer shrub (Artemisia halodendron) on germination from the soil seedbank in a semi-arid sandy grassland in China. J. Plant Res. 130, 1013–1021. doi: 10.1007/s10265-017-0954-0
Ma, Y., Xie, W., Sun, W., and Marczewski, T. (2016). Strong reproductive isolation despite occasional hybridization between a widely distributed and a narrow endemic Rhododendron species. Sci. Rep. 6:19146. doi: 10.1038/srep19146
MacKay, M. B., and Gardiner, S. E. (2017). Geographic analysis of Red List Rhododendron (Ericaceae) taxa by country of origin identifies priorities for ex situ conservation. Blumea 62, 103–120. doi: 10.3767/blumea.2017.62.02.05
Maclean, J. E., Mitchell, R. J., Burslem, D. F. R. P., Genney, D., Hall, J., and Pakeman, R. J. (2018). Seed limitation, not soil legacy effects, prevents native understorey from establishing in oak woodlands in scotland after removal of Rhododendron ponticum. Restor. Ecol. 26, 865–872. doi: 10.1111/rec.12664
Manzoor, S. A., Griffiths, G., Iizuka, K., and Lukac, M. (2018). Land cover and climate change may limit invasiveness of Rhododendron ponticum in Wales. Front. Plant Sci. 9:664. doi: 10.3389/fpls.2018.00664
Masood, P. A., and Muhammad, N. (2018). Germination ecology of Cenchrus biflorus roxb.: Effects of environmental factors on seed germination. Rangel. Ecol. Manag. 71, 424–432. doi: 10.1016/j.rama.2018.04.002
Medina-Villar, S., Alonso, Á., Castro-Díez, P., and Pérez-Corona, M. E. (2017). Allelopathic potentials of exotic invasive and native trees over coexisting understory species: The soil as modulator. Plant Ecol. 218, 579–594. doi: 10.1007/s11258-017-0713-2
Muturi, G. M., Poorter, L., Bala, P., and Mohren, G. M. J. (2017). Unleached Plitter inhibits germination but leached stimulates seedling growth of dry woodland species. J. Arid Environ. 138, 44–50. doi: 10.1016/j.jaridenv.2016.12.003
Nelson, D. W., and Sommers, L. E. (1996). “Total carbon, organic carbon, and organic matter,” in Methods of Soil Analysis. Part 3-Chemical Methods, eds D. L. Sparks, A. L. Page, P. A. Helmke, R. H. Loeppert, P. N. Soltanpour, M. A. Tabatabai, et al. (Madison, WI: Segoe Rd), 961–1010. doi: 10.2136/sssabookser5.3.c34
Novoa, A., González, L., Moravcová, L., and Pysek, P. (2012). Effects of soil characteristics, allelopathy and frugivory on establishment of the invasive plant carpobrotus edulis and a co-occuring native. PLoS One 7:e53166. doi: 10.1371/journal.pone.0053166
Ortiz, T. A., Urbano, M. R., and Takahashi, L. S. A. (2019). Effects of water deficit and ph on seed germination and seedling development in Cereus jamacaru. Semin. Cienc. Agrar. 40, 1379–1392. doi: 10.5433/1679-0359.2019v40n4p1379
Osburn, E. D., Elliottt, K. J., Knoepp, J. D., Miniat, C. F., and Barrett, J. E. (2018). Soil microbial response to rhododendron understory removal in southern appalachian forests: Effects on extracellular enzymes. Soil Biol. Biochem. 127, 50–59. doi: 10.1016/j.soilbio.2018.09.008
Park, E. J., Muhammad, K. A., Kim, L. R., and Lee, I. J. (2020). Influence of elevated pH on germination, growth attributes and aba contents of sticky mouse-ear chickweed (Cerastium glomeratum Thuill.). J. Agr. Life Sci. 54, 1–7. doi: 10.14397/jals.2020.54.3.1
Qu, T., Du, X., Peng, Y., Guo, W., and Losapio, G. (2021). Invasive species allelopathy decreases plant growth and soil microbial activity. PLoS One 16:e0246685. doi: 10.1371/journal.pone.0246685
R Development Core Team (2014). R: A language and environment for statistical computing. Vienna: R Foundation for Statistical Computing.
Raulerson, S., Jackson, C. R., Melear, N. D., Younger, S. E., and Elliott, K. J. (2020). Do southern appalachian mountain summer stream temperatures respond to removal of understory rhododendron thickets? Hydrol. Process. 34, 3045–3060. doi: 10.1002/hyp.13788
Reigosa, M. J., and Gonzalez, L. (2006). “Forest ecosystems and allelopathy,” in Allelopathy, eds M. Reigosa, N. Pedrol, and L. González (Dordrecht: Springer), doi: 10.1007/1-4020-4280-9_20
Rice, E. L. (1992). “Allelopathic effects on nitrogen cycling,” in Allelopathy, eds S. J. H. Rizvi and V. Rizvi (Dordrecht: Springer). doi: 10.1007/978-94-011-2376-1_4
Shen, S., Ma, G., Xu, G., Li, D., Jin, G., Yang, S., et al. (2022). Allelochemicals Identified From Sweet Potato (Ipomoea batatas) and Their Allelopathic Effects on Invasive Alien Plants. Front. Plant Sci. 13:823947. doi: 10.3389/fpls.2022.823947
Sun, J., He, Y., Wu, N., Javed, Q., Xiang, Y., and Du, D. (2022). Nitrogen deposition elevated the allelopathic effects of three compositae invasive species on indigenous Lactuca sativa. Pol. J. Environ. Stud. 31, 3809–3818. doi: 10.15244/pjoes/146940
Uddin, M. N., and Robinson, R. W. (2017). Allelopathy and resource competition: The effects of Phragmites australis invasion in plant communities. Bot. Stud. 58:29. doi: 10.1186/s40529-017-0183-9
Veen, G. F., Fry, E. L., Ten Hooven, F. C., Kardol, P., Morriën, E., and De Long, J. R. (2019). The role of plant litter in driving plant-soil feedbacks. Front. Environ. Sci. 7:168. doi: 10.3389/fenvs.2019.00168
Vogt, D., Tilley, J., and Edmonds, R. (2015). Soil and plant analysis for forest ecosystem characterization. Berlin: Higher Education Press and Walter de Gruyter GmbH, doi: 10.1515/9783110290479
Walbott, M., Gallet, C., and Corcket, E. (2018). Beech (Fagus sylvatica) germination and seedling growth under climaticand allelopathic constraints. C. R. Biol. 341, 444–453. doi: 10.1016/j.crvi.2018.09.003
Wei, C., Zhou, S., Shi, K., Zhang, C., and Shao, H. (2020). Chemical profile and phytotoxic action of Onopordum acanthium essential oil. Sci. Rep. 10:13568. doi: 10.1038/s41598-020-70463-7
Worsley, S. F., Macey, M. C., Prudence, S., Wilkinson, B., Murrell, J. C., and Hutchings, M. I. (2021). Investigating the role of root exudates in recruiting streptomyces bacteria to the Arabidopsis thaliana microbiome. Front. Mol. Biosci. 8:686110. doi: 10.3389/fmolb.2021.686110
Wurzburger, N., and Hendrick, R. L. (2009). Plant litter chemistry and mycorrhizal roots promote a nitrogen feedback in a temperate forest. J. Ecol. 97, 528–536. doi: 10.1111/j.1365-2745.2009.01487.x
Yun, Y., Wang, H., Man, B., Xiang, X., Zhou, J., Qiu, X., et al. (2016). The relationship between pH and bacterial communities in a single Karst ecosystem and its implication for soil acidification. Front. Microbiol. 7:1955. doi: 10.3389/fmicb.2016.01955
Zhang, L., Song, J., Peng, L., Xie, W., Li, S., and Wang, J. (2022). Comprehensive biochemical, physiological, and transcriptomic analyses provide insights into floral bud dormancy in Rhododendron delavayi Franch. Front. Genet. 13:856922. doi: 10.3389/fgene.2022.856922
Zhang, L., Wang, X., Guo, J., Xia, Q., Zhao, G., Zhou, H., et al. (2013). Metabolic profiling of Chinese tobacco leaf of different geographical origins by GC-MS. J. Agric. Food Chem. 61, 2597–2605. doi: 10.1021/jf400428t
Keywords: litter, GC–MS, allelochemical, allelopathic effect, phenolic acid
Citation: Quan W, Wang A, Li C and Xie L (2022) Allelopathic potential and allelochemical composition in different soil layers of Rhododendron delavayi forest, southwest China. Front. Ecol. Evol. 10:963116. doi: 10.3389/fevo.2022.963116
Received: 27 June 2022; Accepted: 04 October 2022;
Published: 18 October 2022.
Edited by:
Jiasheng Wu, Zhejiang Agriculture and Forestry University, ChinaReviewed by:
Amarpreet Kaur, Panjab University, IndiaMarta Bożym, Opole University of Technology, Poland
Daizy Rani Batish, Panjab University, India
Copyright © 2022 Quan, Wang, Li and Xie. This is an open-access article distributed under the terms of the Creative Commons Attribution License (CC BY). The use, distribution or reproduction in other forums is permitted, provided the original author(s) and the copyright owner(s) are credited and that the original publication in this journal is cited, in accordance with accepted academic practice. No use, distribution or reproduction is permitted which does not comply with these terms.
*Correspondence: Chaochan Li, Y2hhb2NoYW5sQGd6bnUuZWR1LmNu; Lijuan Xie, eGxqQHN6cHQuZWR1LmNu