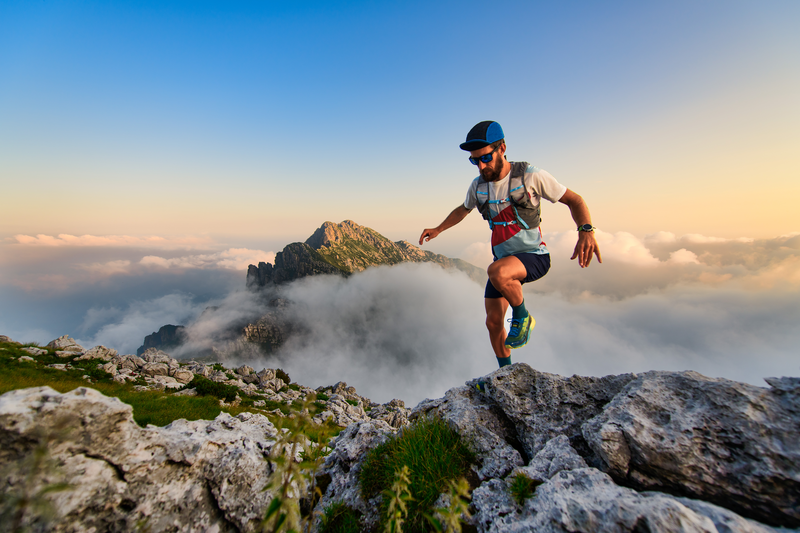
94% of researchers rate our articles as excellent or good
Learn more about the work of our research integrity team to safeguard the quality of each article we publish.
Find out more
ORIGINAL RESEARCH article
Front. Ecol. Evol. , 20 October 2022
Sec. Conservation and Restoration Ecology
Volume 10 - 2022 | https://doi.org/10.3389/fevo.2022.958655
This article is part of the Research Topic Amphibian and Reptile Road Ecology View all 12 articles
Linear transport infrastructure can alter the viability of populations and wildlife passages are used to mitigate their impacts. The assessment of their outcomes is often limited to recording the use of the tunnels by a focal species. For amphibians, the effectiveness of tunnels is poorly evaluated with little information about whether certain features encourage individuals that may be reluctant to pass through tunnels. One study showed that acoustic enrichment with anuran calls can increase the crossing of tunnels by newts. This study recorded the behavior of three European amphibian species in three tunnels, tracking them with PIT tags and detection with four RFID antennas installed on the floor of the tunnels. We tested (1) the effectiveness of the antennas in detecting the species, (2) the effect of the length of the tunnels, and (3) the effect of acoustic enrichment. Using a multi-state capture–recapture model, we evaluated the probability of an individual advancing between the tunnel sections. The effectiveness of the antennas varied according to species, higher for Urodela species than for Anuran species. Several types of paths were detected (constant and varying speeds, halt, and back-and-forth movements). The fire salamander and the great crested newt individuals exhibited a similar variety of movements in the tunnels (21 and 40 m length). Triturus cristatus made similar movements in the tunnels with and without acoustic enrichment. In water frogs, all the individuals (n = 16) made a complete crossing in the tunnel with enrichment vs. 75% (n = 71) in the tunnel without enrichment. In T. cristatus, the probability of going forward at the entrance of the tunnel was 18% higher with enrichment in one tunnel. No significant effect of acoustic enrichment was observed in two others tunnels for this species. In Pelophylax esculentus, this probability was 78% higher in the tunnel with enrichment. This multi-antenna RFID system was able to provide valuable information on the behavior of these small animals when traversing the tunnels, as well as to test the effectiveness of tunnel features. The findings indicate that acoustic enrichment to attract animals to specific locations holds promise as a new conservation tool.
Linear transportation infrastructure (LTI) such as roads and railways are one of the major anthropogenic alterations to the world’s ecosystems (Rytwinski and Fahrig, 2015; Popp and Boyle, 2016). They can have a number of negative impacts on wildlife, including habitat loss, habitat fragmentation, loss of connectivity, and direct and indirect mortality (Forman and Alexander, 1998; Schmidt and Zumbach, 2008; Rytwinski and Fahrig, 2015). While most studies on LTI have focused on roads, railways have theoretically similar effects (Bartoszek and Greenwald, 2009; Dorsey, 2011; Popp and Boyle, 2016), even with lower traffic (Rodriguez et al., 1996). Yet to date there has been a lack of studies on railway ecology (Dorsey, 2011; Popp and Boyle, 2016; Testud and Miaud, 2018), especially on reptiles and amphibians (Kornilev et al., 2006; Budzik and Budzik, 2014; Kaczmarski and Kaczmarek, 2016).
Conservation planners attempt to use knowledge of species biology and the types of impacts identified to propose mitigation measures (Testud and Miaud, 2018). Wildlife passages such as tunnels for small vertebrates (e.g., amphibians) and fences are today commonly implemented in LTI planning and construction (Jarvis et al., 2019; Schmidt et al., 2020). While these are assumed to improve the viability of impacted populations (Clevenger and Ford, 2010; Hamer et al., 2014; Jarvis et al., 2019), the evaluation is complex and costly in terms of budget, time and manpower and is often limited to recording tunnel use (e.g., Puky et al., 2007; Pagnucco et al., 2012; Matos et al., 2017; Testud and Miaud, 2018). The political agenda is often too shortsighted (Lesbarrères and Fahrig, 2012). The behavior of amphibians encountering a wildlife crossing (Matos et al., 2018) and fences (Brehme et al., 2021) has been rarely studied (Conan et al., 2022).
It is known that individuals can be reluctant to cross tunnels (Allaback and Laabs, 2003; Bain et al., 2017; Matos et al., 2017, 2018; Jarvis et al., 2019), and better knowledge on the causes of avoidance would be particularly valuable for designing new tunnels or adapting existing structures (Brehme et al., 2021). Sensory-based conservation harnesses species’ natural communication and signaling behavior to mitigate threats to wild populations (Friesen et al., 2017). Wildlife managers have begun employing acoustic attraction to lure animals to specific places by broadcasting vocalizations (Putman and Blumstein, 2019). Playbacks are frequently used to attract birds to unoccupied sites or to maintain animals in a new translocation site (Ward and Schlossberg, 2004; Molles et al., 2008) and thus are recommended for conservation and restoration (Ahlering et al., 2010; Friesen et al., 2017; Znidersic and Watson, 2022). In amphibians, using conspecific chorus calls has been shown to improve colonization (Buxton et al., 2015; James et al., 2015). A study has also shown that acoustic enrichment of tunnels with frog calls increased the speed and complete crossing rates of water frogs (Pelophylax esculentus) and the great crested newt (Triturus cristatus) (Testud et al., 2020). Individuals with PIT tags tracked by RFID antennas deployed in tunnels can give detailed information on movements inside the tunnel (Testud et al., 2019).
In this study, we investigate the movement behavior of three European amphibian species during tunnel crossings, with individuals tracked by PIT tags and RFID antennas. The complete crossing or non-crossing by a U-turn or other behaviors as well as the crossing speeds were presented in Testud et al. (2020). The experimental design allowed the description of the trajectories (direction changes, variations in speed) of the great crested newt (T. cristatus), fire salamander (Salamandra salamandra), and water frog (P. esculentus) in tunnels of varying lengths. We also broadcasted a mixed chorus of the water frog (P. esculentus) and the European tree frog (Hyla arborea) in tunnels in order to test the effects of this acoustic enrichment on the individual trajectories of the water frog (P. esculentus) and the great crested newt (T. cristatus). Multi-state models were used to evaluate the effect of acoustic enrichment on the estimated probability of the amphibians moving forward at successive positions in tunnels.
Three wildlife crossing structures built under a high-speed railway (HSR) line were studied in the Pays-de-la-Loire region in northwestern France. The three tunnels and surrounding landscape are described in Testud et al. (2020). Two tunnels are located close to the small town of Chantenay-Villedieu, at a distance of 800 m from each other (Tunnels 1 and 2). The third is located close to the small town of Beaulieu-sur-Oudon, 70 km away (Tunnel 3). The tunnels are square concrete culverts 1-m wide and 1-m high, with respective lengths of 21 m (Tunnel 1), 40 m (Tunnel 2), and 18.5 m (Tunnel 3). Tunnels 1 and 2 are “dry fauna crossing structures,” while Tunnel 3 is a hydraulic structure that had a slight trickle of water during the period of the experiments.
Specimens of three amphibian species were captured using several methods depending on the species (Table 1): fire salamanders (S. salamandra) were collected on land when migrating to aquatic sites (from February to the beginning of April 2018 and 2019) during nocturnal transects of about 100 m along the HSR fences surrounding the Tunnel 2. A total of 101 individuals were caught and used for the experiments (Table 1). Water frogs (Pelophylax kl. esculentus/lessonae) (consisting of the edible frog P. esculentus and the pool frog Pelophylax lessonae, hereafter called P. esculentus) were caught in ponds (from 35 to 460 m from Tunnel 3) at the beginning of the breeding season (mid-May 2018–2019), manually or with a dip-net at night with the help of flashlight. A total of 324 individuals were caught and used for the experiments (Table 1). Great crested newts (T. cristatus) were caught in ponds during the reproductive period (from mid-February to mid-May 2018 and 2019) with minnow traps. A total of 560 individuals were caught and used for the experiments (Table 1).
Specimens were marked with transponders (Testud et al., 2020), and stored in opaque plastic tanks (0.80 × 0.40 × 0.25 m) with moist forest litter, kept outside, close to the capture site, in a shaded place with an ambient temperature ranging from 5°C (night) to 15°C (day) before the experiments. They were released at the exact place of capture (on land or pond) the day after the experiment. Authorization to catch amphibians was provided by the regional government of the study area (Regional Environment, Housing and Planning Agency of Sarthe, Mayenne, and Ille-et-Vilaine, inter-regional derogation from the prohibition to capture and transport specimens of protected animal species, 29 May 2017).
A monitoring system was designed, composed of four antennas (Biolog-id outdoor antennas, 100 × 8.5 × 2.5 cm), connected to an electronic control unit, described in detail in Testud et al. (2019). Each antenna included an auto-tuning function and a rechargeable power battery. With the PIT tag used, the detection distance of an antenna was 3 cm. The minimum time between the detection of two successive PIT tags was 70 ms. The antennae were equidistantly spaced (between each antenna and each end of the tunnel), delimiting five sections of the same length. For example, in the 40 m tunnel, the antennas were set up every 8 m (i.e., 8, 16, 24, and 32 m) from the entrance. Wooden reflectors were attached to each end of the antennas to prevent animals from avoiding crossing the antennas (Figure 1B).
Figure 1. (A) The four antennas of the RFID device implemented in tunnels. (B) Internal view of one equipped tunnel. Wooden reflectors, set up on either end of one antenna, are visible. (C) A spined toad crossing one antenna. (D) Schematic view of the experiment. U-turn, Stay, and Complete are the behavioral categories recorded in the equipped tunnels.
Each individual was identified by a hand-recorder (reader RS1-F1, Biolog-ID©, reading distance = 4 to 10 cm). The individuals were released at the entrance every 1 min and the RFID antennas in the tunnel recorded each PIT-tagged individual with the precise time of crossing. Each individual was used only for one experiment.
These experiments were based on the concept of homing behavior, i.e., the propensity of displaced amphibians to come-back to the place of capture (Dole, 1972; Sinsch, 1987). S. salamandra were captured on land while migrating to a breeding place. The newt (T. cristatus), and the water frog (P. esculentus) were caught in ponds at dates when they were reproductively active. Newts caught in ponds can successfully return to the site of capture from displacement distances of more than 100 m (e.g., Twitty et al., 1964; Joly and Miaud, 1989). In our experiments, we performed only the first phase of the “homing” experiment design, i.e., the capture of individuals and their release at a place different from the capture place (the entrance of the tunnel). We expected that individuals will move to the capture place, located on the other side of the tunnel and then entering it.
Experiment was conducted for 22 nights from March to May 2018 and from February to May 2019. The experiments were performed after sunset (from approximately 7 p.m. to 9:30 p.m. from February to May). The experiment was stopped 4 h 30 min after the release of the last tested individual.
When an animal completely crossed the tunnel, it was not necessarily detected by each antenna. The effectiveness of the antennas was evaluated as the proportion of animals detected by each antenna among the animals that completely crossed the tunnels. This was evaluated in 2019 with 125 adult T. cristatus and 22 S. salamandra in Tunnel 1, and 34 adult T. cristatus, and 53 P. esculentus in Tunnel 3.
The four RFID antennas installed in each tunnel recorded the successive positions of the animals, and the time series of these positions allowed the one-dimensional trajectory of each animal to be reconstructed. Individual trajectories and speed variation between tunnel sections were evaluated with individuals that performed a “complete crossing” (i.e., that completely crossed the tunnel) or performed a “U-turn” (i.e., that partly crossed the tunnel and then turned around and exited from the entrance). The maximum distance crossed in the tunnel (i.e., the farthest antenna from the entrance) was measured for each individual making a U-turn.
The acoustic enrichment experiment was performed in 2019 with a soundtrack of a mixed chorus of water frog (P. esculentus) and European tree frog (H. arborea), the two main species using mating calls in the region’s amphibian community. The signals used for playback, the amplitude and the loudspeaker characteristics are provided in Testud et al. (2020). The stereo soundtrack was emitted using an audio player connected to two loudspeakers. These loudspeakers were installed outside the tunnel, 10 m from the exit and space 5 m apart. The soundtrack started when the first specimen was deposited at the tunnel entrance, and was stopped when the last released individual was collected. Great crested newt (n = 184) and water frog (n = 35) were tested in the tunnels with acoustic enrichment (Table 1).
We constructed multi-state capture–recapture models (Lebreton et al., 2009) using the program E-SURGE v2.2.3 (Choquet et al., 2009). This approach allowed us to take advantage of the Markovian structure of multi-state models to first estimate the probability of an individual going forward or backward at each antenna in a tunnel and then evaluate whether acoustic enrichment increased the probability of going forward, depending on the position in the tunnel.
The successive positions of a marked specimen in the tunnel were described as a “state.” The starting position in the tunnel for all individuals was defined as the state “Start.” The antennas were named as states 1, 2, 3, and 4 according to their antenna number (“A1,” “A2,” “A3,” and “A4”). The exit from a tunnel after a complete crossing (“CC”) was named state 5, and the exit from a tunnel through the entrance after a U-turn (“UT”) as state 6. The two matrices (of the initial state and of the probability of going forward are shown in Supplementary Figure 1). Individuals all began in state “Start,” and then the transition matrix modeled the probability of moving forward to the next antenna, or going backward to the previous one (Supplementary Figure 1). The animals that stayed 10 min at the tunnel entrance without entering, and the animals that left the tunnel entrance without entering were not taken into consideration. Individuals that stopped in the middle of the tunnel were not considered. On the other hand, the animals that have walked a small distance without passing the first antenna before making a U-turn were used in the analysis. The total number of individuals used in the analysis was 293 for T. cristatus and 167 for P. esculentus (Table 2).
Table 2. Number of individuals making a “complete crossing” or a “U-turn,” with and without acoustic enrichment, for the four datasets analyzed using multi-state models.
Using previous knowledge on antenna effectiveness, we chose to complete capture histories of individuals for which some detections were missing. The missing observations (event) were added to the detection history when the individual was detected with the previous and following antenna (an individual detected at antenna 1, then at antennas 3 and 4, and finally at the end of the tunnel would have the history: “start-1-2-3-4-5”). Individuals that exited through the entrance would have “start-6.”
Model selection used a sequential backward procedure in which all candidate models were nested within the starting model. In the first step, we identified the most parsimonious structure for describing variation in going forward (or advancing) between antennas, and then the potential effect of acoustic enrichment. Exploratory analysis showed that the probability of going forward increased gradually as individuals advanced through the tunnel. Consequently, we tested for a linear response on the logit scale to summarize the responses with more parsimony, using only two parameters (intercept and slope).” The most parsimonious model was selected using the second-order Akaike Information Criterion (QAIC; Thomson et al., 2008; Choquet et al., 2009). Models with a difference of less than two QAIC units were considered equivalent in their ability to describe the data (Burnham and Anderson, 2002). To compare the significance of the best model with regard to the question of acoustic enrichment, the likelihood ratio test was calculated between the best model and its equivalent with or without enrichment. Four datasets were analyzed, three datasets for T. cristatus (three tunnels), and one dataset for P. esculentus (Table 2).
The effectiveness of the antennas (proportion of animals detected by the antennae of the animals that completely crossed the tunnels) varied, mainly according to species (Table 3). Antenna effectiveness in Tunnel 1 and Tunnel 3 was rather similar for the two Urodela species (T. cristatus and S. salamandra), varying from 0.68 ± 0.16 to 0.97 ± 0.06. Both S. salamandra and T. cristatus marked individuals were detected by at least one antenna (except one newt) (Figure 2). For the Anuran species P. esculentus, the effectiveness varied from 0.08 ± 0.07 to 0.38 ± 0.13 (Table 3), with lower detections at the two first antennas. For P. esculentus individuals, 42% of the marked specimens were not detected by at least one antenna (Figure 2).
Table 3. Effectiveness of the antennas to detect marked individuals of the three amphibian species in Tunnel 1 (21 m in length) and Tunnel 3 (18.5 m in length).
Figure 2. Effectiveness of the antennas correspond to the proportion of animals detected by the antenna among the animals that completely crossed the tunnels. Antenna effectiveness was measured in Tunnel 1 (A) and Tunnel 3 (B). Dark gray bars represent T. cristatus, light gray bars represent S. salamandra, and white bars represent Pelophylax Kl.
Several types of movement were detected during a complete crossing: a rather “linear” trajectory at constant speed (Figures 3A,B) or non-linear trajectories (Figures 3C–I). Movements with varying speeds were also observed (the animal might accelerate or slowdown in the tunnels). These behaviors are visible in Figures 3C,D when a trajectory “stalls” as compared to an average constant speed estimated from the crossing duration. Individual trajectories can be characterized by a “halt,” when the amphibian was detected several times by the same antenna (Figures 3E,F), and by “back-and-forth” movements (Figures 3G,H). These types of trajectories could be present alone (Figure 3F) or consecutively (Figure 3I).
Figure 3. Different types of movement exhibited during a complete crossing. The dashed lines represent the entry, exit, and location of the antennas in the tunnels. The positions of the individuals in the tunnels at the time since release are represented by dots. The solid lines represent the speed of movement between the different positions. Panels (A–I) represent several patterns of movement (see section “Results”).
Similar types of movements were observed for animals that made a U-turn (Supplementary Figure 2): for example, back-and-forth trajectories (Supplementary Figure 2i). The farthest antenna from the entrance that the amphibian crossed is also available (e.g., First antenna: Supplementary Figure 2g; fourth antenna: Supplementary Figure 2b).
In S. salamandra, the trajectories were recorded in Tunnel 1 (21 m) and Tunnel 2 (40 m) (Supplementary Figure 3). In Tunnel 1, among the individuals that made a complete crossing, the trajectories of 16 salamanders were available (Supplementary Figure 3a), most had a linear trajectory, and some back-and-forth movements were observed. One individual was strongly distinguished from the others by its long crossing time and back-and-forth travel. One individual halted at antenna 1 for more than 3 h, before quickly crossing the rest of the tunnel. Among the individuals that made a U-turn (Supplementary Figure 3b): 1 individual made a rapid U-turn after crossing the first antenna at 4 min 20 s, 1 made a 3-h stop near the same antenna and 1 made a back-and-forth movement twice, getting closer to the exit before finally making a U-turn. In Tunnel 2 (40 m), among the individuals that made a complete crossing, the trajectories of nine salamanders were available (Supplementary Figure 3c), and most of the individuals moved with linear trajectories. No back-and-forth movements were observed. Among the individuals that made a U-turn, the trajectories of four salamanders were available (Supplementary Figure 3d), one individual went 8 m before turning around, two reached antenna 2 (16 m) before turning around, and one reached antenna 3 (24 m), where it stayed for about 1 h before making a U-turn.
In T. cristatus, the trajectories were recorded in Tunnel 1 and Tunnel 2 (Figure 4). Among the individuals that made a complete crossing, the trajectories of 73 newts were available (Figure 4A), with a linear trajectory with a fast or slow constant speed, a non-constant speed, a halt, or back-and-forth movements. In Tunnel 2 (40 m), among the individuals that made a complete crossing, the trajectories of nine newts were available (Figure 4E), and six had a linear trajectory, including five at a fast speed and one at a slow speed. One individual had a non-constant speed. Among the individuals that made a U-turn, the trajectories of 38 newts were available in Tunnel 1 (Figure 4B). Four individuals reached the maximum distance of 16.2 m (antenna 4), two individuals halted and exhibited back-and-forth behavior. In Tunnel 2, among the individuals that made a U-turn, the trajectories of 20 newts were available (Figure 4F), and 2 individuals reached the maximum distance of 16 m (antenna 2).
Figure 4. Trajectories of T. cristatus in Tunnels 1 and 2 without acoustic enrichment (T1) and with acoustic enrichment (T2) to complete crossing (left) and U-turn (right). The dashed lines represent the entry, exit, and location of the antennas in the tunnels. The positions of the individuals in the tunnels at the time since release are represented by dots. The solid lines represent the speed of movement between the different positions. Panel (A) represents trajectories of newts making a complete crossing in Tunnel 1 without enrichment. Panel (B) represents trajectories of newts making a U-turn in Tunnel 1 without enrichment. Panel (C) represents trajectories of newts making a complete crossing in Tunnel 1 with enrichment. Panel (D) represents trajectories of newts making a U-turn in Tunnel 1 with enrichment. Panel (E) represents trajectories of newts making a complete crossing in Tunnel 2 without enrichment. Panel (F) represents trajectories of newts making a U-turn in Tunnel 2 without enrichment. Panel (G) represents trajectories of newts making a complete crossing in Tunnel 2 with enrichment. Panel (H) represents trajectories of newts making a U-turn in Tunnel 2 with enrichment.
The maximum distance traveled in Tunnel 1 (21 m) before turning back was 16.8 m (antenna 4) for the salamander and the newts. In Tunnel 2 (40 m), this maximum distance was 24 m for the salamander (antenna 3) and 16 m for the newts (antenna 2). For this species, the probability of going forward from “Start” to antenna 2 in Tunnel 1 was ΨStart/Tunnel 1 × ΨA1/Tunnel 1 = 0.56, and was ΨStart/Tunnel 2 = 0.39 in Tunnel 2. This higher propensity was found in the next 8 m with ΨA2/Tunnel 1 × ΨA3/Tunnel 1 = 0.75, and was (ΨA1/Tunnel 2 = 0.46) in Tunnel 2.
In T. cristatus, individual trajectories were recorded in Tunnel 1 and Tunnel 2 with acoustic enrichment (Figure 4). The types of trajectory of the 32 newts performing a complete crossing in Tunnel 1 (Figure 4C, 53%) were similar to the trajectory observed without enrichment (Figure 4A). Six individuals performed a U-turn (Figure 4D, 10%), and 1 turned around after reaching 16.8 m (antenna 4). Two individuals halted at 4.20 m (antenna 1) for about an hour. In Tunnel 2, five newts made a complete crossing (Figure 4G, 10%), four individuals had a non-constant speed and one halted. Nine newts performed a U-turn (Figure 4H, 18%), and one individual turned around after reaching 32 m (antenna 4).
In P. esculentus, individual trajectories were recorded in Tunnel 3 (18.5 m), with and without acoustic enrichment conditions (Supplementary Figure 4). Without enrichment, 53 frogs performed a complete crossing (Supplementary Figure 4a, 18%), the types of movement were a linear trajectory with a fast or slow constant speed, non-constant speed, a halt, or back-and-forth movement. The same types of movements were observed in the 18 frogs that made a U-turn (Supplementary Figure 4b, 6%). One individual reached the maximum distance of 14.8 m (antenna 4). With enrichment, 16 frogs performed a complete crossing (Supplementary Figure 4c, 46%) and exhibited mainly fast linear trajectories. Only one individual did a U-turn, after reaching 3.7 m (antenna 1) (Supplementary Figure 4d).
The five best models from the selection procedure are shown in Supplementary Table 1. For T. cristatus in Tunnel 1, the best model included a linear relationship between all four antennas, as well as significant effects of acoustic enrichment on both the slope of the linear relationship and the probability of going forward from the “Start” state (Figure 5A), for this model the LRT provides a p-value of 0.0333. With enrichment, the probability of going forward from “Start” (ΨStart/With = 0.78, 95% CI: 0.70–0.84) was 18% higher than without enrichment (ΨStart/Without = 0.66, 0.56–0.75; ΔQAIC = 2.77). The probability of going forward increased as the individual advanced in the tunnel; this probability was 27% higher at antenna 4 than at antenna 1. The lower probability of advancing with enrichment observed at antenna 1 (ΨA1/Without = 0.80, 0.70–0.86; ΨA1/With = 0.69, 0.61–0.77) is most likely due to the fact that more individuals passed the first antenna. Of these individuals, several turned back after having explored more of the tunnel, thus decreasing the probability of going forward at antennas 1, 2, and 3.
Figure 5. Probability of going forward (advancing) for the great crested newt in the three tunnels (A–C), and the water frog in Tunnel 3 (D). Mean probability and confidence intervals of 95% were estimated with the best model from the selection procedure.
For T. cristatus tested in Tunnel 2, the best model from the selection procedure included a linear relationship from antenna 2 to antenna 4, with a significant effect of acoustic enrichment on its slope (Figure 5B), for this model the LRT provides a p-value of 0.02011. The probabilities of going forward at “Start” and at antenna 1 were different (ΨStart = 0.39, 0.29–0.50 to ΨA1 = 0.46, 0.31–0.62), but comparable with or without acoustic enrichment. No individuals turned back beyond antennas 2, 3, and 4 in the condition without enrichment [the slope of the linear relationship (Figure 5B) is equal to 1]. In the condition with enrichment, individuals turned back at a further distance in the tunnel. The acoustic signal to attract individuals could be limited to a distance between 24 and 32 m, as evidenced by the lack of effect of enrichment at the start and at antenna 1.
For T. cristatus tested in Tunnel 3, the best model was the one with a linear constraint between the five positions and no significant effect of acoustic enrichment (ΔQAIC = 1.15), for this model the LRT provides a p-value of 0.3451. It thus had two parameters: the intercept and the slope of the line, with a gradual increase of the probability of going forward as a function of location in the tunnel from ΨStart = 0.51, 0.43–0.60 to ΨA4 = 0.94, 0.88–0.97 (Figure 5C).
The last dataset analyzed corresponds to P. esculentus tested in Tunnel 3. The model with a linear line between the five positions with a significant effect of acoustic enrichment on the intercept was the best model from the selection procedure (ΔQAIC = 46.39), for this model the LRT provides a p-value of 3.501e−12. There was a gradual increase in the probability of going forward as a function of location in the tunnel, from ΨStart/Without = 0.55, 0.48–0.62 to 4, ΨA4/Without = 0.97, 0.94–0.99 (Figure 5D). The model supported a strong effect of acoustic enrichment with the probability of going forward at “Start” (ΨStart/With = 0.97, 0.87–0.99): 78% higher than without enrichment and to a lesser degree at the antennas.
The lack of behavioral data on small species crossing wildlife passages is partly due to methodological issues (Weber et al., 2019); evaluating the behavior of amphibians in tunnels is challenging (review in Testud et al., 2019). Marking amphibians with fluorescent pigments to record continuous tracks in tunnels has been used, but the number of monitored individuals is limited and does not provide information on data such as movement speed (Matos et al., 2018). Camera traps are also widely used for movement tracking in tunnels (reviewed in Jumeau, 2017). While their detection rate has been reported as low for amphibians (Pagnucco et al., 2012), the increase in the quality of video traps implemented makes them more relevant for such small species (Hobbs and Brehme, 2017; Pomezanski and Bennett, 2018; Jarvis et al., 2019; Brehme et al., 2021). Using PIT tags is a traditional marking technique in amphibians (e.g., Perret and Joly, 2002; Winandy and Denoël, 2011; Testud et al., 2019; Weber et al., 2019). Telemetry using RFID antennas is employed to monitor movement patterns and habitat use in small animals (Charney et al., 2009), to evaluate fish passes (Thiem et al., 2011, 2013; Ovidio et al., 2017; Benitez et al., 2018; Lothian et al., 2019) and tunnels (Boarman et al., 1998). The use of RFID antennas to track amphibian movements and habitat use in nature is increasing (Winandy and Denoël, 2011; Atkinson-Adams, 2015; Atkinson-Adams et al., 2016; Testud et al., 2019; Weber et al., 2019). To our knowledge, to date, PIT-tagged amphibians and RFID antennas have been used to evaluate equipped wildlife passages for only one toad and two carabid species (Testud et al., 2019). This design was also used to compare the performance of this type of monitoring with camera traps in tunnels crossed by a salamander species (Atkinson-Adams, 2015).
Our experiments with a system composed of four RFID antennas improve knowledge on how amphibians move inside wildlife crossings. The effectiveness of the system varied between antennas and species. The locomotion of species can greatly differ (e.g., exclusively walking locomotion in Urodela, exclusively saltatory locomotion in frogs, or both in toads). In our experiments, the lower detection rate observed with frogs (Figure 2 and Table 2) may be explained by their jumping over the antenna, the maximum detection distance of the antenna being 3 cm. The detection range could be improved using another antenna design (e.g., circular), but with the practical constraint of implementing this in the tunnels. Higher but not total detection rates were observed with the salamander and newt species (Figure 2 and Table 2). The antennas were 8.5 cm in width (Figure 1), and two marked specimens crossing the antennas simultaneously stop the recording. If one individual stays on the antenna, it will prevent the recording of the other individual crossing during this time. Thinner antennas and better positioning on the tunnel floor (i.e., not disturbing the floor) may increase the detection rate. Camera traps detected 44% of crossings of the long-toed salamander (Ambystoma macrodactylum), producing a large number of empty images (Pagnucco et al., 2011). By installing an RFID antenna at the exit of a tunnel, the detectability of the camera traps was reevaluated to a lower 15.3% (Atkinson-Adams, 2015). These kinds of comparative studies are valuable in the context of amphibian-crossing tunnels.
Tunnel effectiveness can be evaluated with the crossing rate (proportion of animals that cross the tunnels or not) and the mean speed of crossing. These parameters are used to evaluate tunnel characteristics such as length and diameter (Patrick et al., 2010; Testud et al., 2020), substrate (Lesbarrères et al., 2004; Trochet et al., 2019), light, humidity and temperature (Bain, 2014; Bain et al., 2017) and social information, including acoustic enrichment (Testud et al., 2020). However, the same duration in a tunnel may involve very different movement types, and more information about the behavior inside tunnels is needed to improve the evaluation of tunnel effectiveness (Matos et al., 2018). We observed a variety of movements with Urodela species in tunnels (Figures 3, 4): some individuals crossed the tunnels with a linear trajectory, while others made back-and-forth movements. Some individuals halted their movement in different parts of the tunnel, for different amounts of time.
The maximum distance traveled in tunnels could be an indicator of crossing effectiveness, especially in long tunnels (e.g., HSR and Highways). Individuals performing a U-turn can be also very informative for evaluating tunnel characteristics, as no U-turn by newts or salamanders was observed farther than 16 and 24 m respectively in the 40-m tunnel. In all cases, the probability of going forward increased as the amphibian progressed in the tunnel (Figure 5). The distance before making a U-turn and an increase in the probability of going forward should vary according to a threshold based on parameters linked to individual motivation (Joly, 2019), including abiotic factors (e.g., seasonality; Matos et al., 2018), biotic factors (species; Ovidio et al., 2017), personality (Chajma et al., 2020), individual history, or social conditions (including acoustic enrichment).
The length of a tunnel acts on the proportion of complete crossings (higher in S. salamandra and T. cristatus in the shorter tunnel; Testud et al., 2020), as previously shown in several amphibian species (Dodd et al., 2004; Lesbarrères et al., 2004; Patrick et al., 2010). Considering the precise movements in a tunnel (this study), the propensity to go forward in the first meters of the tunnel (8 m) was higher for the shorter tunnel. These results argue for experiments that explore the mechanisms of newts’ appreciation of tunnel length: e.g., perception of the exit (odor, brightness, temperature, ventilation, etc.) or distance traveled (Brehme et al., 2021).
Acoustic enrichment to lure animals to specific places is a new tool for active management on land and at sea (Gordon et al., 2019; Putman and Blumstein, 2019). In the terrestrial environment, this has been shown to be effective in birds and mammals (Ward and Schlossberg, 2004; Kiffner et al., 2008; Molles et al., 2008; Friesen et al., 2017). In amphibians, acoustic enrichment with conspecific or heterospecific calls can improve the colonization of new ponds (Buxton et al., 2015; James et al., 2015) and can attract newts (Diego-Rasilla and Luengo, 2004, 2007; Pupin et al., 2007; Madden and Jehle, 2017). When a soundtrack of mating calls of syntopic anurans was broadcast in a tunnel (Testud et al., 2020), frogs exhibited a large increase in complete crossings and speed, and newts showed an increase in complete crossings (but not speed) in one of the acoustically enhanced tunnels.
In this study, recording the individual trajectories improved the evaluation of the effect of acoustic enrichment. In the acoustically enriched tunnel, water frogs exhibited more linear movements, and more newts exhibited back-and-forth movements. Newts also made a U-turn at a farther distance (32 m) in the acoustically enriched tunnel. We posit that acoustic enrichment could modify the behavior of novice or hesitant individuals and shift the distance that discourages these individuals from turning back.
Acoustic enrichment had an effect on the probability of going forward for newts (in Tunnels 1 and 2, but not in Tunnel 3). In Tunnel 1, with enrichment, an increase in the probability of going forward at “Start” and then a decrease at the next antenna can be explained by the increase in the number of back-and-forth movements. In Tunnel 2, with enrichment, the effect of enrichment on the probability of going forward was only observed in the second half of the tunnel. The role of the distance to the sound source on the propensity to go forward in the tunnel remains to be confirmed.
Acoustic enrichment had an effect on the probability of going forward for frogs in Tunnel 3, where the probability reached values close to 1, and result for newts in Tunnels 1 and 2 attesting to the effectiveness of the use of amphibian calls to improve passage in tunnels. These results support the hypothesis that acoustic enrichment makes wildlife crossings more attractive (Gerhardt and Huber, 2002), and improves tunnel crossings by amphibians (Testud et al., 2020).
The use of an RFID multi-antenna system can provide information on how small animals behave when crossing tunnels (Testud et al., 2019), thus allowing tunnel characteristics to be tested to improve their use (Pomezanski and Bennett, 2018; Testud et al., 2019). However, the price of the device (reader and antenna) and the detection distance of marked individuals could be limiting (Winandy and Denoël, 2011; Testud et al., 2019; Weber et al., 2019). The simultaneous use of different methods, e.g., multiple fluorescent pigments, camera traps and RFID systems, in different contexts and with different species, would be a useful way to compare parameters such as individual detection rate (Eggert, 2002; Atkinson-Adams, 2015; Bain et al., 2017; Hobbs and Brehme, 2017; Matos et al., 2018; Testud and Miaud, 2018; Jarvis et al., 2019).
There is a high variability in wildlife passage crossing success in Amphibians (Brehm, 1989; Allaback and Laabs, 2003; Dodd et al., 2004; Lesbarrères et al., 2004; Woltz et al., 2008; Patrick et al., 2010; Malt, 2011; Hamer et al., 2014; Bain et al., 2017; Matos et al., 2018; Pomezanski and Bennett, 2018; Jarvis et al., 2019; Chajma et al., 2020). This variability can come from individual differences (this study), which has to be considered as behaviors (including movement) at the scale of the individual can have repercussions for the functioning of an entire ecosystem (e.g., Bowler and Benton, 2005; Allgeier et al., 2020).
There is also a growing need to evaluate amphibian behavior in wildlife passages (and not only their use of these crossings), and methodological advances such as RFID and other techniques that allow fine tracking are promising. Experiments that consider physiological states of individuals, age, experience of migratory routes, etc., would all increase our understanding considerably. Acoustic behavior may influence species differently. Experiments to assess the effect of acoustic enrichment with other amphibian communities would also be valuable, to evaluate the possible generalization of the method used in this study. Wildlife passages such as under-road tunnels are often equipped with barrier fencing to reduce mortality and direct amphibians toward the passages. A better knowledge of animal movements along the fencing (proportion of animals passing along them, entering the tunnels, etc.) can also help to design effective LTI mitigation for amphibians (Brehme et al., 2021).
Methodological developments (e.g., RFID, Testud et al., 2019; camera traps, Brehme et al., 2021; Conan et al., 2022) allow designing experiments to test for tunnel permeability and its link with individual behaviors, e.g., tunnel attractiveness including surroundings of the entrance and local conditions within the tunnel (substrates, open grated top to allow for natural light, temperature and moisture conditions). Knowledge about the probability of crossing a structure is also particularly relevant for friction map modeling and species distribution modeling at a regional scale in landscapes, which are often fragmented by linear transport infrastructure (Remon et al., 2018; Clauzel and Godet, 2020; Matutini et al., 2021).
The original contributions presented in this study are included in the article/Supplementary material, further inquiries can be directed to the corresponding author.
The animal study was reviewed and approved by the Structure Chargée du Bien Etre Animal, CEFE, Montpellier.
GT, CM, TL, and DL participated in the design of the experiments. GT and CC did the statistical analysis. QL, GT, and DP did the field experiments. GT and CM wrote the manuscript. All authors reviewed the manuscript.
This work was supported by the French National Research Agency (ANR), the French engineering group Egis (CIFRE grant number 2017/0212), and the French construction group Eiffage.
We would like to thank Marie-Aurélia Sabatte, Eva Garcia, Agathe Legrand, Anthony Martin, Camille Clowez, Philippe Geniez, Emelie Segrestan, Lynda Farineau, Annick Lucas, François Le Hérissé, Julien Maziere, Margot Piccinin, Clement Fauconnier, and Adrien Parais for their field participation. We are also grateful to the Janvier and to Fred and Arnaud for their hospitality. Philippe Cordier (Biolog-id©) offered us valuable RFID assistance. We also thank the two reviewers who greatly help improving the first draft of this manuscript. We also thank Philippe Geniez, Aurélien Besnard, and Pierre-André Crochet for their advice as well as the reviewers and editors for the improvements made to this manuscript. The constructive discussions with Sandra Luque, David Lesbarreres, Françoise Burel, Alain Morand, and Sylvie Vanpeene-Bruhier also contributed to the realization of this work.
The authors declare that the research was conducted in the absence of any commercial or financial relationships that could be construed as a potential conflict of interest.
All claims expressed in this article are solely those of the authors and do not necessarily represent those of their affiliated organizations, or those of the publisher, the editors and the reviewers. Any product that may be evaluated in this article, or claim that may be made by its manufacturer, is not guaranteed or endorsed by the publisher.
The Supplementary Material for this article can be found online at: https://www.frontiersin.org/articles/10.3389/fevo.2022.958655/full#supplementary-material
Ahlering, M. A., Arlt, D., Betts, M. G., Fletcher, R. J., Nocera, J. J., and Ward, M. P. (2010). Research needs and recommendations for the use of conspecific-attraction methods in the conservation of migratory songbirdsnecesidades de investigatión y recomendaciones para el uso de métodos de atracción de individuos coespecíficos en la conservatión de aves canoras migratorias. Condor 112, 252–264. doi: 10.1525/cond.2010.090239
Allaback, M. L., and Laabs, D. M. (2003). Effectiveness of road tunnels for the santa cruz long-toed salamander. Trans. West. Sect. Wildl. Soc. 38/39, 5–8.
Allgeier, J. E., Cline, T. J., Walsworth, T. E., Wathen, G., Layman, C. A., and Schindler, D. E. (2020). Individual behavior drives ecosystem function and the impacts of harvest. Sci. Adv. 6:eaax8329. doi: 10.1126/sciadv.aax8329
Atkinson-Adams, M. R. (2015). Movement and Habitat use of the Long-Toed Salamander (Ambystoma macrodactylum). Waterton Lakes National Park: Alberta.
Atkinson-Adams, M. R., Paszkowski, C., and Scrimgeour, G. (2016). Effects of substrate on PIT Tag detection with a self-made portable RFID antenna: Implications for PIT telemetry. Herpetol. Rev. 47, 198–201.
Bain, T. (2014). Evaluating the Effect of Moisture in Wildlife Crossing Tunnels on the Migration of the California Tiger Salamander, Ambystoma Californiense. Available online at: http://dspace.calstate.edu/handle/10211.3/123759 (Accessed Jul 31, 2020).
Bain, T. K., Cook, D. G., and Girman, D. J. (2017). Evaluating the effects of abiotic and biotic factors on movement through wildlife crossing tunnels during migration of the california tiger salamander, Ambystoma californiense. Herpetol. Conserv. Biol. 12, 192–201.
Bartoszek, J., and Greenwald, K. R. (2009). A Population Divided: Railroad Tracks as Barriers to Gene Flow in an Isolated Population of Marbled Salamanders (Ambystoma Opacum). Herpetol. Conserv. Biol. 4, 191–197.
Benitez, J.-P., Dierckx, A., Nzau Matondo, B., Rollin, X., and Ovidio, M. (2018). Movement behaviours of potamodromous fish within a large anthropised river after the reestablishment of the longitudinal connectivity. Fish. Res. 207, 140–149. doi: 10.1016/j.fishres.2018.06.008
Boarman, W. L., Beigel, M. L., Goodlett, G. C., and Sazaki, M. (1998). A passive integrated transponder system for tracking animal movements. Wildl. Soc. Bull. 26, 886–891.
Bowler, D. E., and Benton, T. G. (2005). Causes and consequences of animal dispersal strategies: Relating individual behaviour to spatial dynamics. Biol. Rev. 80, 205–225. doi: 10.1017/S1464793104006645
Brehm, K. (1989). “The acceptance of 0.2 metre tunnels by amphibians during their migration to the breeding site,” in Amphibians and Roads, Proceedings of the Toad Tunnel Conference, ed. T. E. S. Langton (Rendsburg: Federal Republic of Germany), 29–42.
Brehme, C. S., Tracey, J. A., Ewing, B. A. I., Hobbs, M. T., Launer, A. E., Matsuda, T. A., et al. (2021). Responses of migratory amphibians to barrier fencing inform the spacing of road underpasses: A case study with California tiger salamanders (Ambystoma californiense) in Stanford, CA, USA. Glob. Ecol. Conserv. 31:e01857. doi: 10.1016/j.gecco.2021.e01857
Budzik, K. A., and Budzik, K. M. (2014). A preliminary report of amphibian mortality patterns on railways. Acta Herpetol. 9, 103–107. doi: 10.13128/Acta_Herpetol-12914
Burnham, K. P., and Anderson, D. R. (2002). A practical information-theoretic approach. Model Sel. Multimodel Inference 2, 70–71.
Buxton, V. L., Ward, M. P., and Sperry, J. H. (2015). Use of chorus sounds for location of breeding habitat in 2 species of anuran amphibians. Behav. Ecol. 26, 1111–1118. doi: 10.1093/beheco/arv059
Conan, A., Fleitz, J., Garnier, L., Brishoual, M. L., Handrich, Y., and Jumeau, J. (2022). Effectiveness of wire netting fences to prevent animal access to road infrastructures: An experimental study on small mammals and amphibians. Nat. Conserv. 47, 271–281. doi: 10.3897/natureconservation.47.71472
Chajma, P., Kopeckı, O., and Vojar, J. (2020). Individual consistency in exploration and shyness but not activity in smooth newts (Lissotriton vulgaris): The effect of habituation? J. Zool. 311, 269–276. doi: 10.1111/jzo.12784
Charney, N. D., Letcher, B. H., Haro, A., and Warren, P. S. (2009). Terrestrial passive integrated transponder antennae for tracking small animal movements. J. Wildl. Manag. 73, 1245–1250. doi: 10.2193/2008-096
Choquet, R., Rouan, L., and Pradel, R. (2009). “Program E-Surge: A Software Application for Fitting Multievent Models,” in Modeling Demographic Processes In Marked Populations Environmental and Ecological Statistics, eds D. L. Thomson, E. G. Cooch, and M. J. Conroy (Boston, MA: Springer), 845–865. doi: 10.1007/978-0-387-78151-8_39
Clauzel, C., and Godet, C. (2020). Combining spatial modeling tools and biological data for improved multispecies assessment in restoration areas. Biol. Conserv. 250:108713. doi: 10.1016/j.biocon.2020.108713
Clevenger, A. P., and Ford, A. T. (2010). Wildlife Crossing Structures, Fencing, and Other Highway Design Considerations. Katy: National Academies.
Diego-Rasilla, F. J., and Luengo, R. M. (2004). Heterospecific call recognition and phonotaxis in the orientation behavior of the marbled newt, Triturus marmoratus. Behav. Ecol. Sociobiol. 55, 556–560.
Diego-Rasilla, F. J., and Luengo, R. M. (2007). Acoustic orientation in the palmate newt, Lissotriton helveticus. Behav. Ecol. Sociobiol. 61, 1329–1335. doi: 10.1007/s00265-007-0363-9
Dodd, K. C. J., Barichivich, W. J., and Smith, L. L. (2004). Effectiveness of a barrier wall and culverts in reducing wildlife mortality on a heavily traveled highway in Florida. Biol. Conserv. 118, 619–631.
Dole, J. W. (1972). Homing and orientation of displaced toads, Bufo americanus, to their home sites. Copeia 1972, 151–158. doi: 10.2307/1442791
Dorsey, B. P. (2011). Factors Affecting Bear and Ungulate Mortalities Along the Canadian Pacific Railroad Through Banff and Yoho National Parks. Available online at: http://scholarworks.montana.edu/xmlui/handle/1/1190 (accessed Jan 20, 2017).
Eggert, C. (2002). Use of fluorescent pigments and implantable transmitters to track a fossorial toad (Pelobates fuscus). Herpetol. J. 12, 69–74.
Forman, R. T. T., and Alexander, L. E. (1998). Roads and their major ecological effects. Annu. Rev. Ecol. Syst. 29, 207–231. doi: 10.1146/annurev.ecolsys.29.1.207
Friesen, M. R., Beggs, J. R., and Gaskett, A. C. (2017). Sensory-based conservation of seabirds: A review of management strategies and animal behaviours that facilitate success. Biol. Rev. Camb. Philos. Soc. 92, 1769–1784. doi: 10.1111/brv.12308
Gerhardt, H. C., and Huber, F. (2002). Acoustic Communication in Insects and Anurans: Common Problems and Diverse Solutions. Chicago: University of Chicago Press.
Gordon, T. A. C., Radford, A. N., Davidson, I. K., Barnes, K., McCloskey, K., Nedelec, S. L., et al. (2019). Acoustic enrichment can enhance fish community development on degraded coral reef habitat. Nat. Commun. 10:5414. doi: 10.1038/s41467-019-13186-2
Hamer, A. J., van der Ree, R., Mahony, M. J., and Langton, T. (2014). Usage rates of an under-road tunnel by three Australian frog species: Implications for road mitigation. Anim. Conserv. 17, 379–387. doi: 10.1111/acv.12105
Hobbs, M. T., and Brehme, C. S. (2017). An improved camera trap for amphibians, reptiles, small mammals, and large invertebrates. PLoS One 12:e0185026. doi: 10.1371/journal.pone.0185026
James, M. S., Stockwell, M. P., Clulow, J., Clulow, S., and Mahony, M. J. (2015). Investigating behaviour for conservation goals: Conspecific call playback can be used to alter amphibian distributions within ponds. Biol. Conserv. 192, 287–293. doi: 10.1016/j.biocon.2015.10.001
Jarvis, L. E., Hartup, M., and Petrovan, S. O. (2019). Road mitigation using tunnels and fences promotes site connectivity and population expansion for a protected amphibian. Eur. J. Wildl. Res. 65:27. doi: 10.1007/s10344-019-1263-9
Joly, P. (2019). Behavior in a changing landscape: Using movement ecology to inform the conservation of pond-breeding amphibians. Front. Ecol. Evol. 7:155. doi: 10.3389/fevo.2019.00155
Joly, P., and Miaud, C. (1989). Fidelity to the breeding site in the alpine newt Triturus alpestris. Behav. Process. 19, 47–56. doi: 10.1016/0376-6357(89)90030-2
Jumeau, J. (2017). Les possibilités de dispersion et éléments d’habitat-refuge dans un paysage d’agriculture intensive fragmenté par un réseau routier dense: Le cas de la petite faune dans la plaine du bas-rhin. Strasbourg: University of Strasbourg.
Kaczmarski, M., and Kaczmarek, J. M. (2016). Heavy traffic, low mortality–tram tracks as terrestrial habitat of newts. Acta Herpetol. 11, 227–232.
Kiffner, C., Waltert, M., Meyer, B., and Mühlenberg, M. (2008). Response of lions (Panthera leo LINNAEUS 1758) and spotted hyaenas (Crocuta crocuta ERXLEBEN 1777) to sound playbacks. Afr. J. Ecol. 46, 223–226. doi: 10.1111/j.1365-2028.2007.00813.x
Kornilev, Y. V., Price, S. J., and Dorcas, M. E. (2006). Between a rock and a hard place: Responses of eastern box turtles (Terrapene carolina) when trapped between railroad tracks. Herpetol. Rev. 37, 145–148.
Lebreton, J., Nichols, J. D., Barker, R. J., Pradel, R., and Spendelow, J. A. (2009). Chapter 3 modeling individual animal histories with multistate capture–recapture models. Adv. Ecol. Res. 41, 87–173. doi: 10.1016/S0065-2504(09)00403-6
Lesbarrères, D., and Fahrig, L. (2012). Measures to reduce population fragmentation by roads: What has worked and how do we know? Trends Ecol. Evol. 27, 374–380. doi: 10.1016/j.tree.2012.01.015
Lesbarrères, D., Lodé, T., and Merilä, J. (2004). What type of amphibian tunnel could reduce road kills? Oryx 38, 220–223. doi: 10.1017/S0030605304000389
Lothian, A. J., Gardner, C. J., Hull, T., Griffiths, D., Dickinson, E. R., and Lucas, M. C. (2019). Passage performance and behaviour of wild and stocked cyprinid fish at a sloping weir with a Low Cost Baffle fishway. Ecol. Eng. 130, 67–79. doi: 10.1016/j.ecoleng.2019.02.006
Madden, N., and Jehle, R. (2017). Acoustic orientation in the great crested newt (Triturus cristatus). Amphib. Reptil. 38, 57–65. doi: 10.1163/15685381-00003083
Malt, J. (2011). “Assessing the effectiveness of amphibian mitigation on the sea to sky highway: Population-level effects and best management practices for minimizing highway impacts,” in Proceedings of the herpetof. roads workshop – There light end tunn (St, Nanaimo, BC: Vancouver Island University).
Matos, C., Petrovan, S., Ward, A. I., and Wheeler, P. (2017). Facilitating permeability of landscapes impacted by roads for protected amphibians: Patterns of movement for the great crested newt. PeerJ 5:e2922. doi: 10.7717/peerj.2922
Matos, C., Petrovan, S. O., Wheeler, P. M., and Ward, A. I. (2018). Short-term movements and behaviour govern the use of road mitigation measures by a protected amphibian. Anim. Conserv. 22, 285–296. doi: 10.1111/acv.12467
Matutini, F., Baudry, J., Fortin, M.-J., Pain, G., and Pithon, J. (2021). Integrating landscape resistance and multi-scale predictor of habitat selection for amphibian distribution modelling at large scale. Landsc. Ecol. 36, 3557–3573. doi: 10.1007/s10980-021-01327-2
Molles, L., Calcott, A., Peters, D., Delamare, G., Hudson, J. D., Innes, J., et al. (2008). “Acoustic anchoring” and the successful translocation of North Island kokako (Callaeas cinerea wilsoni) to a New Zealand mainland management site within continuous forest. Notornis 55, 57–68.
Ovidio, M., Sonny, D., Dierckx, A., Watthez, Q., Bourguignon, S., Court, B., et al. (2017). The use of behavioural metrics to evaluate fishway efficiency. River Res. Appl. 33, 1484–1493. doi: 10.1002/rra.3217
Pagnucco, K. S., Paszkowski, C. A., and Scrimgeour, G. J. (2011). Using cameras to monitor tunnel use by long-toed salamanders (Ambystoma macrodactylum): An informative, cost-efficient technique. Herpetol. Conserv. Biol. 6, 277–286.
Pagnucco, K. S., Paszkowski, C. A., and Scrimgeour, G. J. (2012). Characterizing movement patterns and spatio-temporal use of under-road tunnels by long-toed salamanders in Waterton Lakes National Park. Canada. Copeia 2012, 331–340. doi: 10.1643/CE-10-128
Patrick, D. A., Schalk, C. M., Gibbs, J. P., and Woltz, H. W. (2010). Effective culvert placement and design to facilitate passage of amphibians across roads. J. Herpetol. 44, 618–626.
Perret, N., and Joly, P. (2002). Impacts of tattooing and pit-tagging on survival and fecundity in the alpine newt (Triturus alpestris). Herpetologica 58, 131–138.
Pomezanski, D., and Bennett, L. (2018). Developing recommendations for monitoring wildlife underpass usage using trail cameras. Environ. Monit. Assess. 190:413. doi: 10.1007/s10661-018-6794-0
Popp, J. N., and Boyle, S. P. (2016). Railway ecology: Underrepresented in science? Basic Appl. Ecol. 19, 84–93. doi: 10.1016/j.baae.2016.11.006
Puky, M., Farkas, J., and Ronkay, M. T. (2007). Use of existing mitigation measures by amphibians, reptiles, and small to medium-size mammals in Hungary: Crossing structures can function as multiple species-oriented measures. Road Ecol. Cent. Available online at: http://escholarship.org/uc/item/991742nk (accessed January 9, 2017).
Pupin, F., Sacchi, R., Puky, M., Farkas, J., and Ronkay, M. T. (2007). “Use of existing mitigation measures by amphibians, reptiles, and small to medium-size mammals in Hungary: Crossing structures can function as multiple species-oriented measures,” in Proceedings: 2007 International Conference on Ecology & Transportation. Center for Transportation and the Environment, (Raleigh: North Carolina State University).
Putman, B. J., and Blumstein, D. T. (2019). What is the effectiveness of using conspecific or heterospecific acoustic playbacks for the attraction of animals for wildlife management? A systematic review protocol. Environ. Evid. 8:6. doi: 10.1186/s13750-019-0149-3
Remon, J., Chevallier, E., Prunier, J. G., Baguette, M., and Moulherat, S. (2018). Estimating the permeability of linear infrastructures using recapture data. Landsc. Ecol. 33, 1697–1710. doi: 10.1007/s10980-018-0694-0
Rodriguez, A., Crema, G., and Delibes, M. (1996). Use of non-wildlife passages across a high speed railway by terrestrial vertebrates. J. Appl. Ecol. 33, 1527–1540. doi: 10.2307/2404791
Rytwinski, T., and Fahrig, L. (2015). “The impacts of roads and traffic on terrestrial animal populations,” in Handbook of Road Ecology, eds R. van der Ree and D. C. G. Smith (Hoboken: John Wiley and Sons), 237–246.
Schmidt, B. R., Brenneisen, S., and Zumbach, S. (2020). Evidence-based amphibian conservation: A case study on toad tunnels. Herpetologica 76, 228–239. doi: 10.1655/0018-0831-76.2.228
Schmidt, B. R., and Zumbach, S. (2008). “Amphibian Road Mortality and How to Prevent It: A Review,” in Urban Herpetology, eds J. C. Mitchell, R. E. Jung Brown, and B. Bartolomew (St. Louis: University of Zurich), 157–167. doi: 10.1111/cobi.12063
Sinsch, U. (1987). Orientation behaviour of toads (Bufo bufo) displaced from the breeding site. J. Comp. Physiol. A 161, 715–727. doi: 10.1007/BF00605013
Testud, G., Fauconnier, C., Labarraque, D., Lengagne, T., Le Petitcorps, Q., Picard, D., et al. (2020). Acoustic enrichment in wildlife passages under railways improves their use by amphibians. Glob. Ecol. Conserv. 24:e01252. doi: 10.1016/j.gecco.2020.e01252
Testud, G., and Miaud, C. (2018). “From effects of linear transport infrastructures on amphibians to mitigation measures,” in Reptiles and amphibians, Vol. 1, (London: IntechOpen), 85–97. doi: 10.5772/intechopen.74857
Testud, G., Vergnes, A., Cordier, P., Labarraque, D., and Miaud, C. (2019). Automatic detection of small PIT-tagged animals using wildlife crossings. Anim. Biotelemetry 7:21. doi: 10.1186/s40317-019-0183-5
Thiem, J. D., Binder, T. R., Dawson, J. W., Dumont, P., Hatin, D., Katopodis, C., et al. (2011). Behaviour and passage success of upriver-migrating lake sturgeon Acipenser fulvescens in a vertical slot fishway on the Richelieu River, Quebec, Canada. Endanger. Species Res. 15, 1–11. doi: 10.3354/esr00360
Thiem, J. D., Binder, T. R., Dumont, P., Hatin, D., Hatry, C., Katopodis, C., et al. (2013). Multispecies fish passage behaviour in a vertical slot fishway on the Richelieu River, Quebec, Canada. River Res. Appl. 29, 582–592. doi: 10.1002/rra.2553
Thomson, D. L., Cooch, E. G., and Conroy, M. J. (2008). Modeling Demographic Processes in Marked Populations. Berlin: Springer Science & Business Media.
Trochet, A., Le Chevalier, H., Calvez, O., Ribéron, A., Bertrand, R., and Blanchet, S. (2019). Influence of substrate types and morphological traits on movement behavior in a toad and newt species. PeerJ 6:e6053. doi: 10.7717/peerj.6053
Twitty, V., Grant, D., and Anderson, O. (1964). Long distance homing in the newt Taricha rivularis. Proc. Natl. Acad. Sci. U.S.A. 51:51. doi: 10.1073/pnas.51.1.51
Ward, M. P., and Schlossberg, S. (2004). Conspecific attraction and the conservation of territorial songbirds. Conserv. Biol. 18, 519–525. doi: 10.1111/j.1523-1739.2004.00494.x
Weber, L., Šmejkal, M., Bartoò, D., and Rulík, M. (2019). Testing the applicability of tagging the Great crested newt (Triturus cristatus) using passive integrated transponders. PLoS One 14:e0219069. doi: 10.1371/journal.pone.0219069
Winandy, L., and Denoël, M. (2011). The use of visual and automatized behavioral markers to assess methodologies: A study case on PIT-tagging in the Alpine newt. Behav. Res. Methods 43, 568–576. doi: 10.3758/s13428-011-0058-z
Woltz, H. W., Gibbs, J. P., and Ducey, P. K. (2008). Road crossing structures for amphibians and reptiles: Informing design through behavioral analysis. Biol. Conserv. 141, 2745–2750. doi: 10.1016/j.biocon.2008.08.010
Keywords: linear transport infrastructure, amphibian, wildlife passage, acoustic enrichment, multi-state capture–recapture model
Citation: Testud G, Canonne C, Le Petitcorps Q, Picard D, Lengagne T, Labarraque D and Miaud C (2022) Improving trajectories of amphibians in wildlife passages. Front. Ecol. Evol. 10:958655. doi: 10.3389/fevo.2022.958655
Received: 31 May 2022; Accepted: 26 September 2022;
Published: 20 October 2022.
Edited by:
Cheryl S. Brehme, USGS, Western Ecological Research Center, United StatesReviewed by:
Benedikt R. Schmidt, Swiss Amphibian and Reptile Conservation Programme, SwitzerlandCopyright © 2022 Testud, Canonne, Le Petitcorps, Picard, Lengagne, Labarraque and Miaud. This is an open-access article distributed under the terms of the Creative Commons Attribution License (CC BY). The use, distribution or reproduction in other forums is permitted, provided the original author(s) and the copyright owner(s) are credited and that the original publication in this journal is cited, in accordance with accepted academic practice. No use, distribution or reproduction is permitted which does not comply with these terms.
*Correspondence: Claude Miaud, Y2xhdWRlLm1pYXVkQGNlZmUuY25ycy5mcg==
Disclaimer: All claims expressed in this article are solely those of the authors and do not necessarily represent those of their affiliated organizations, or those of the publisher, the editors and the reviewers. Any product that may be evaluated in this article or claim that may be made by its manufacturer is not guaranteed or endorsed by the publisher.
Research integrity at Frontiers
Learn more about the work of our research integrity team to safeguard the quality of each article we publish.