- 1Clinic for Zoo Animals, Exotic Pets and Wildlife, Vetsuisse Faculty, University of Zurich, Zurich, Switzerland
- 2Applied and Analytical Paleontology, Institute of Geosciences, Johannes Gutenberg University, Mainz, Germany
- 3Department of Natural Environmental Studies, Graduate School of Frontier Sciences, The University of Tokyo, Chiba, Japan
- 4Center of Natural History, University of Hamburg, Hamburg, Germany
- 5Nash Family Department of Neuroscience, Friedman Brain Institute, Center for Anatomy and Functional Morphology, Icahn School of Medicine at Mount Sinai, New York, NY, United States
- 6Department of Zoology and Entomology, University of the Free State, Bloemfontein, South Africa
- 7Department of Cariology, Endodontology and Periodontology, University of Leipzig, Leipzig, Germany
Dental microwear texture (DMT) analysis is used to differentiate abrasive dental wear patterns in many species fed different diets. Because DMT parameters all describe the same surface, they are expected to correlate with each other distinctively. Here, we explore the data range of, and correlations between, DMT parameters to increase the understanding of how this group of proxies records wear within and across species. The analysis was based on subsets of previously published DMT analyses in guinea pigs, sheep, and rabbits fed either a natural whole plant diet (lucerne, grass, bamboo) or pelleted diets with or without added quartz abrasives (guinea pigs and rabbits: up to 45 days, sheep: 17 months). The normalized DMT parameter range (P4: 0.69 ± 0.25; M2: 0.83 ± 0.16) and correlation coefficients (P4: 0.50 ± 0.31; M2: 0.63 ± 0.31) increased along the tooth row in guinea pigs, suggesting that strong correlations may be partially explained by data range. A comparison between sheep and guinea pigs revealed a higher DMT data range in sheep (0.93 ± 0.16; guinea pigs: 0.47 ± 0.29), but this did not translate into more substantial correlation coefficients (sheep: 0.35 ± 0.28; guinea pigs: 0.55 ± 0.32). Adding rabbits to an interspecies comparison of low abrasive dental wear (pelleted lucerne diet), the softer enamel of the hypselodont species showed a smaller data range for DMT parameters (guinea pigs 0.49 ± 0.32, rabbit 0.19 ± 0.18, sheep 0.78 ± 0.22) but again slightly higher correlations coefficients compared to the hypsodont teeth (guinea pigs 0.55 ± 0.31, rabbits 0.56 ± 0.30, sheep 0.42 ± 0.27). The findings suggest that the softer enamel of fast-replaced ever-growing hypselodont cheek teeth shows a greater inherent wear trace consistency, whereas the harder enamel of permanent and non-replaced enamel of hypsodont ruminant teeth records less coherent wear patterns. Because consistent diets were used across taxa, this effect cannot be ascribed to the random overwriting of individual wear traces on the more durable hypsodont teeth. This matches literature reports on reduced DMT pattern consistency on harder materials; possibly, individual wear events become more random in nature on harder material. Given the species-specific differences in enamel characteristics, the findings suggest a certain species-specificity of DMT patterns.
Introduction
Standardized experiments have recently been used to explore the mechanics of abrasive dental wear and the various proxies to record it (Schulz et al., 2013; Merceron et al., 2016; Winkler et al., 2019; Ackermans et al., 2020; Louail et al., 2021; Martin et al., 2022). The principal aim of those studies is to supply information for the understanding of evolutionary adaptations and to increase the precision of paleodietary reconstructions. In vitro experiments on a single tooth or enamel sample use a standardized approach to model dental wear dependent on diet. Many diet samples can be run in a short period, or one diet can be “chewed on” by speeding up the chewing apparatus to simulate more extended periods (Hua et al., 2015; Karme et al., 2016; Rodriguez-Rojas et al., 2020; Fischer et al., 2022). This standardization, however, oversimplifies a process that is dependent on the physical properties of the dental tissues, tooth geometry [i.e., arrangement of enamel, dentine, and cementum (Winkler and Kaiser, 2015)], tooth position along the tooth row and the corresponding jaw (Taylor et al., 2016; Ramdarshan et al., 2017), physical properties, and concentration of the abrasive elements in the diet and biomechanical properties of the diet matrix (Kaiser et al., 2016). Therefore, in vivo experiments better represent the complex nature of abrasive tooth wear but are time and labor intensive and must be ethically justified.
Whether the assay is in vitro or in vivo, the amount of tissue lost to wear is inherently difficult to quantify. By contrast, changes to the remaining tissue are comparatively easier to record and are commonly assessed using macroscopic or microscopic parameters serving as proxies for dental wear. Dental microwear texture (DMT) analysis characterizes tooth wear on a micrometer scale. It has been widely employed in the study of dental wear in wild animals (Winkler et al., 2016; Yamada et al., 2018; Schulz-Kornas et al., 2019; Robinet et al., 2020) or fossil species (Donohue et al., 2013; Desantis, 2016) as well as in experimental settings (Schulz et al., 2013; Merceron et al., 2016; Teaford et al., 2017; Winkler et al., 2019, 2020a; Ackermans et al., 2020; Louail et al., 2021) and has sometime shown a high intra- and interspecies variability (Robinet et al., 2022). For DMT analysis (DMTA), a selected enamel surface is scanned and described using up to 46 areal surface texture parameters. Some of the parameters follow international standards, e.g., the ISO 25178 and the ISO 12871. In contrast, other parameters home in on specific geometric elements such as particular lines, points, or specific scale-dependent features (such as motif, furrow, isotropy parameters, and SSFA parameters). Following the ISO 25178 and MountainsMap® Software reference guide, the areal surface texture parameter can be grouped by their main characterizing features (area, complexity, density, direction, height, peak sharpness, plateau size, slope and volume; e.g., Winkler et al. (2021) for further description) to ease the understanding and facilitate the presentation of results. While all 46 DMT parameters describe at least parts of the same surface and should therefore correlate with each other (Ackermans et al., 2021), the relationship between DMT parameters within and between functional groups needs to be analyzed, to increase the understanding how abrasive tooth wear is dependent on species, tooth position, and abrasive elements, among many other factors (Scott et al., 2006). Preliminary explorations of the relationship between the DMT parameters in goats and sheep (Schulz-Kornas et al., 2020; Ackermans et al., 2021) and in rabbits (Martin et al., 2020) have led us to hypothesize that the putatively softer enamel of hypselodont teeth—as seen in Fischer et al. (2022) for rodent incisors and in Shakila et al. (2015) with a preliminary test in cheek teeth in rabbits—should make the detection of interrelationships between different wear proxies easier; a hypothesis also supported by the observation that on soft material like cartilage, more significant correlations between DMTA parameters were evident (Tian et al., 2012; Wang et al., 2013) than on a hard material like steel (Korzynski et al., 2018).
We used the skulls of three groups of guinea pigs (Cavia porcellus) from feeding experiments focusing on the effect of natural diets (lucerne, grass and bamboo) and different external abrasives added to a pelleted diet on DMT analysis for this study. The DMT data was reported previously (Winkler et al., 2019, 2020b) for the effect of plant internal silica, the impact of different shapes, sizes and quantities of external abrasives, and a difference between tooth positions along the tooth row. Because several identical pelleted diets had been used for a feeding experiment in sheep (Ovis aries) (Ackermans et al., 2021) and rabbits (Oryctolagus cuniculus) (Martin et al., 2020), a direct comparison of the interrelationships among DMT measures within each species was feasible. Using specific data subsets, we hypothesize more DMT parameters correlations, or, in other words, a more consistent tooth wear pattern, if (a) the tooth position causes more intensive masticatory forces and if (b) the substrate (enamel) is more malleable (“softer”) (i.e., rodent vs. ruminant). Because one of the many reasons for the emergence of correlations lies in the range of the data submitted to correlation analysis, we do not only consider correlation coefficients but also the (normalized) range of the DMT measurements.
Materials and methods
The dental microwear texture (DMT) analysis datasets were accumulated from feeding experiments approved by the Cantonal Veterinary Office of Zurich (guinea pigs: license no. ZH135/2016; sheep ZH10/2016; rabbits: ZH80/2012). The guinea pigs were fed either a natural whole plant diet (lucerne, grass or bamboo) or a pelleted formulation based on lucerne meal with or without the addition of different external abrasive agents (i.e., quartz in different amounts and grain sizes; see Table 1 and Supplementary Table 1 for diet composition, feeding time and body mass). In guinea pigs, each diet had been fed for a period of three to 6 weeks, and for rabbits for 2 weeks; due to the fast turnover of the chewing surface of these species’ hypselodont cheek teeth (Schulz et al., 2013), this time is sufficient to ensure only traces of the respective diet are recorded. The identical pelleted diets were used in sheep for a feeding time of 17 months with the addition of lucerne hay at a rate of 200 g as fed per animal and day. Additional data were available from six rabbits that had also received a pelleted lucerne diet for 2 weeks. Detailed experimental conditions, including husbandry, feed management and descriptions, are available in the corresponding original publications [guinea pigs: Winkler et al. (2019), Winkler et al. (2020b); sheep: Ackermans et al. (2020); rabbits: Müller et al. (2014)].
The above publications offer a detailed description of the dental microwear texture analysis following the published routine for DMT data in MountainsMap v.9.0.9878 (Schulz et al., 2010, 2013) with adjustment for guinea pigs (Winkler et al., 2019, 2020a; 2021). In short, the surface texture of a predefined molar enamel area (guinea pigs 60 μm2, sheep 120 μm2, rabbits 40 μm2) is scanned using a high-resolution confocal disk-scanning measurement system (μsurf custom, NanoFocus AG, Oberhausen, Germany) at the LIB Hamburg (former Center of Natural History (CeNak) of the University of Hamburg). From the mean of 4 non-overlapping scans, forty-six surface texture parameters (Supplementary Table 2) are quantified using the ISO 25178 (roughness), motif, furrow, isotropy, ISO 12871 (flatness), and Scale- sensitive fractal analysis (SSFA). Only scans from maxillary cheek teeth were included in the respective datasets, and subsets are based on criteria for the respective analysis.
Analysis
One reason for more significant non-parametric correlations in a dataset might simply be a more extensive range of parameter values. To account for this, we normalized all DMT measurements in a dataset in which comparisons were made (all guinea pig tooth positions within a diet category; guinea pigs and sheep within the common diet category) to a scale from 0–1 and expressed the range within each subset (a tooth position; a species) as the difference between the normalized maximum and minimum for each parameter. Subsequently, each subset was characterized by the average (± standard deviation SD) normalized range of all parameters.
Because many parameter measures were not normally distributed, all correlations were assessed using non-parametric correlation matrices (Supplementary Figure 1 for an example plot); to further visualize these results, we show histograms representing the distribution of the Spearman’s correlation coefficients (from −1 to 1), and a boxplot of all absolute Spearman’s correlation coefficients. Descriptive and test statistics were conducted in R Studio (Version 1.3.1093) using the packages tidyverse (Wickham et al., 2019), corrplot (Wei and Simko, 2021) and ggplot (Wickham, 2016).
Datasets
The example plots that outline our approach were built using all scans available for the guinea pigs (dataset A, Table 1). To account for DMT differences between tooth positions (Winkler et al., 2021), only guinea pigs for which scans of each tooth position (P4 to M3) were available were included in the actual analysis, and DMT parameter correlations and ranges were compared between P4 and M2 (dataset B, Table 1). Two groups of guinea pigs (6 animals per group) had received low-abrasive lucerne diets but in a different formulation (dried whole plant diet or dried and pelleted) which allowed for a direct comparison of parameter ranges and correlations (dataset C, Table 1). Some guinea pigs and sheep received the same pelleted diet formulations with the addition of external quartz abrasives [control (quartz free), 4% fine silt, 4% coarse silt, 4% fine sand, 8% fine silt], facilitating an inter-species comparison (dataset D, Table 1). Moreover, the rabbit DMT data was added from a pilot on DMT correlations (Martin et al., 2020) to expand the interspecies comparison of animals fed only a lucerne pellet diet (dataset E, Table 1).
Results
In guinea pigs (dataset A), DMT parameters generally correlated well with each other. For area, height, plateau size, slope, peak sharpness, and volume, most parameters correlated positively (or, in fewer cases, negatively) with each other. The direction, density, and complexity parameters show fewer significant correlations with other measures, evident in the “lighter areas” of the triangular diagram (Supplementary Figure 1 and Supplementary Table 3). The mean absolute Spearman’s correlation coefficient was 0.53 ± 0.31.
Tooth positions (dataset B)
The normalized DMT data range based on all teeth was lower for P4 (0.69 ± 0.25) than for M2 (0.83 ± 0.16; Figure 1A), indicating more variance in DMT measures on the M2. For all teeth, the mean absolute Spearman’s correlation coefficient was 0.56 ± 0.31 (Figure 1B). Here again, the mean for P4 (0.50 ± 0.31) was lower than for the M2 (0.63 ± 0.31; Figure 1B), which is also visible in the distribution of the Spearman’s correlation coefficients (Figures 1D–F), indicating more significant correlations in the tooth position with the larger measurement range.
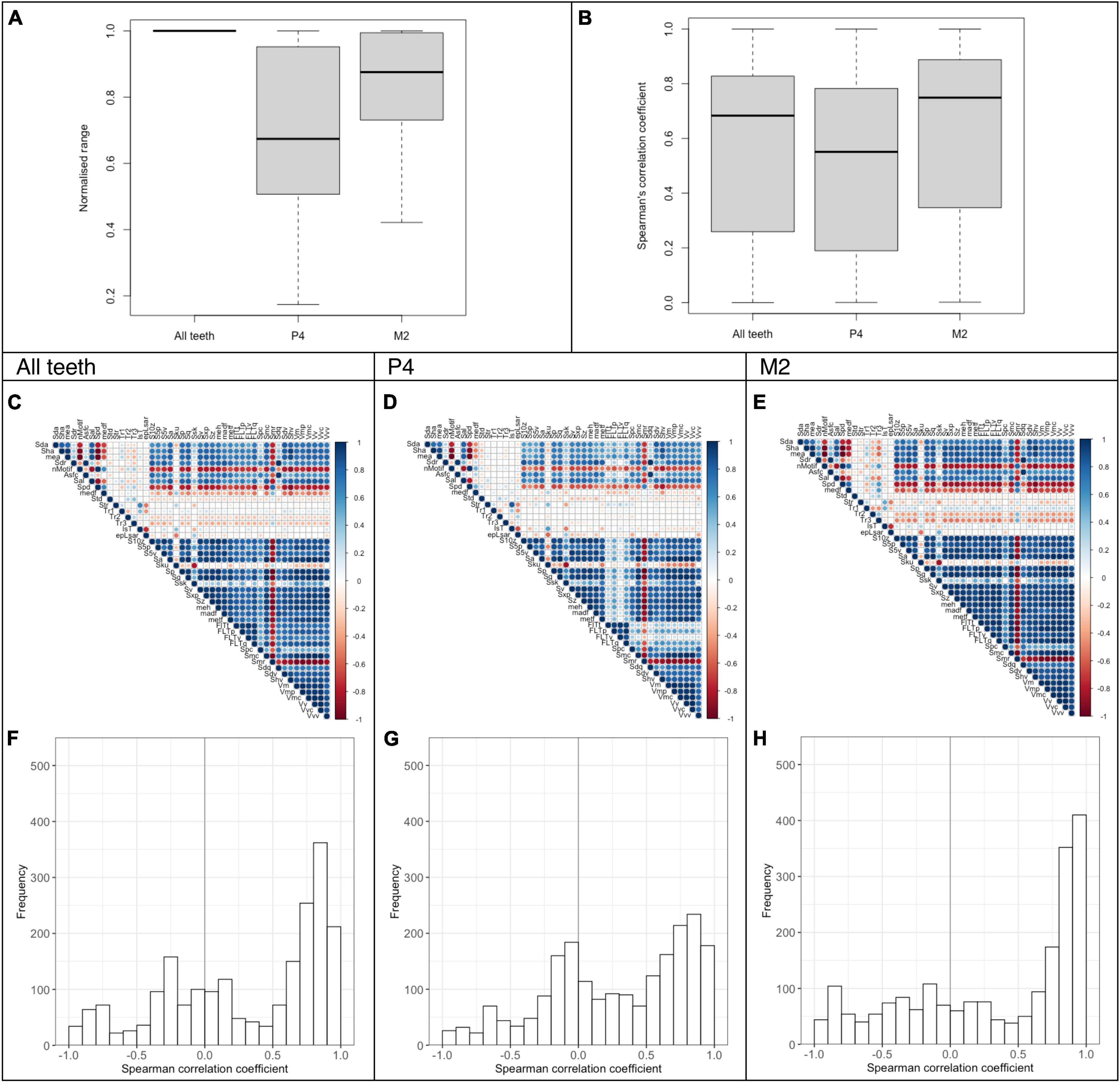
Figure 1. Analysis of DMT parameters for guinea pigs fed different natural (lucerne, grass, bamboo) and pelleted diets with additional external abrasives (dataset B). The data is presented as a summary for all teeth (P4-M3, 228 scans) and separately for P4 (57 scans) and M2 (57 scans). The normalized data range and the Spearman’s correlations coefficients are given as boxplots (A,B). The Spearman’s correlation of the DMT parameters is also shown as a correlation matrix (C–E) and a histogram (F–H).
Diet consistency (dataset C)
Comparing two diet groups with six guinea pigs each, fed either natural whole plant lucerne hay or pelleted lucerne, the normalized DMT data range was smaller for the animals receiving the natural diet (0.44 ± 0.32) than for those receiving the pelleted diets (0.91 ± 0.13; Figure 2A). The mean absolute Spearman’s correlation coefficient was 0.38 ± 0.27 for the natural diets compared to 0.55 ± 0.31 for the pelleted diets (Figure 2B), again indicating more significant correlations in the diet consistency with the larger measurement range.
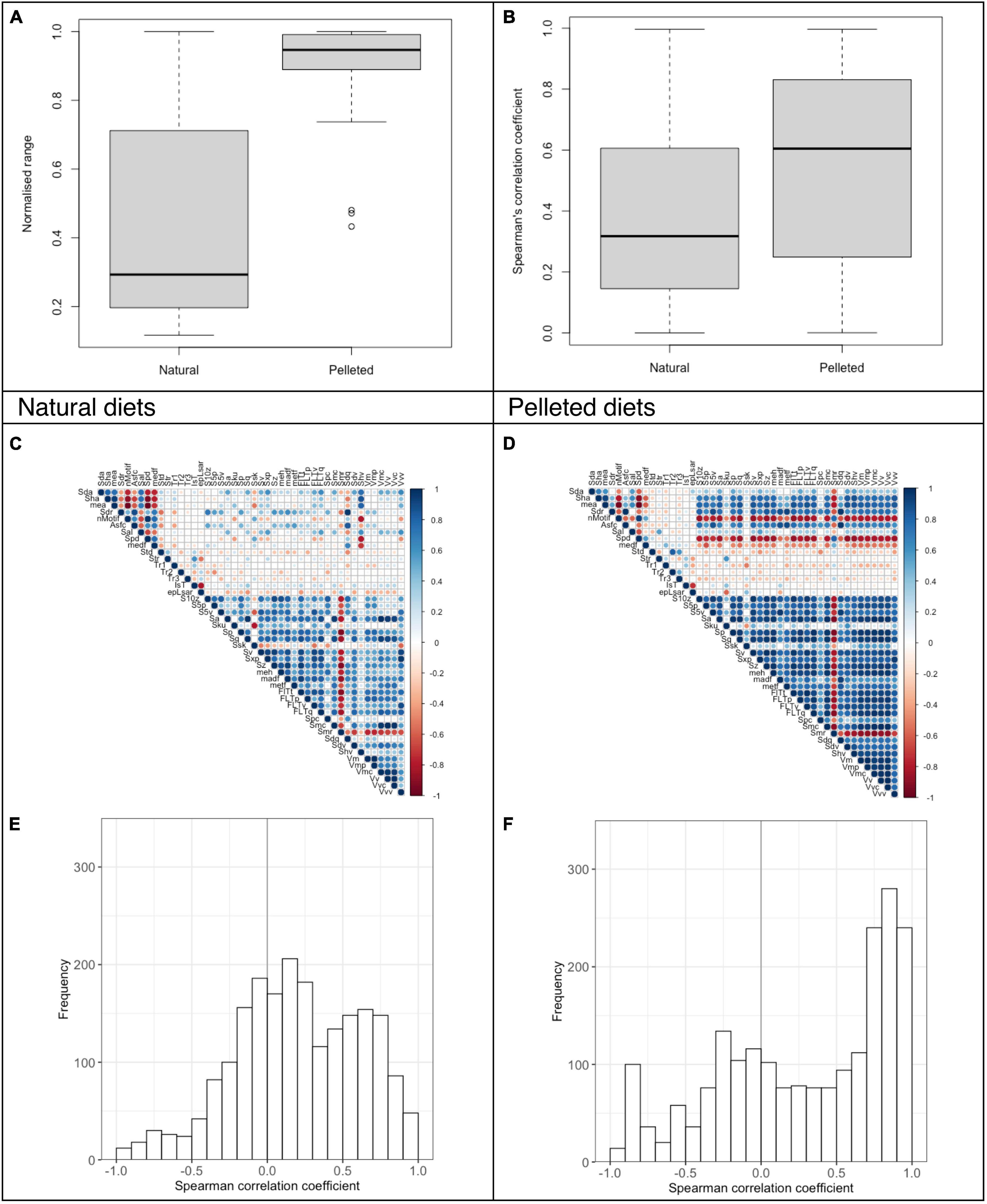
Figure 2. Analysis of DMT parameters for guinea pigs fed a lucerne diet either as whole plant hay or as a pelleted diet for P4 to M3 (dataset C). The data is presented as a summary for all teeth (24 scans for natural diet, 22 scans for pelleted diet. For two animals on the pelleted diet M3 could not be measured). The normalized data range and the Spearman’s correlation coefficients are given as boxplots (A,B). The Spearman’s correlation of the DMT parameters is also shown as a correlation matrix (C,D) and a histogram (E,F).
Species comparison of external abrasive diets (dataset D)
When comparing two data subsets of animals fed identical pelleted diets with added abrasives (i.e., quartz) across all tooth positions, the measurement range for DMT in sheep was higher (0.93 ± 0.16) than in guinea pigs (0.47 ± 0.29; Figure 3A). But this did not translate into a more robust correlation pattern, with a mean absolute Spearman’s correlation coefficient of 0.35 ± 0.28 for sheep compared to 0.55 ± 0.32 for the guinea pigs (Figures 3B–F).
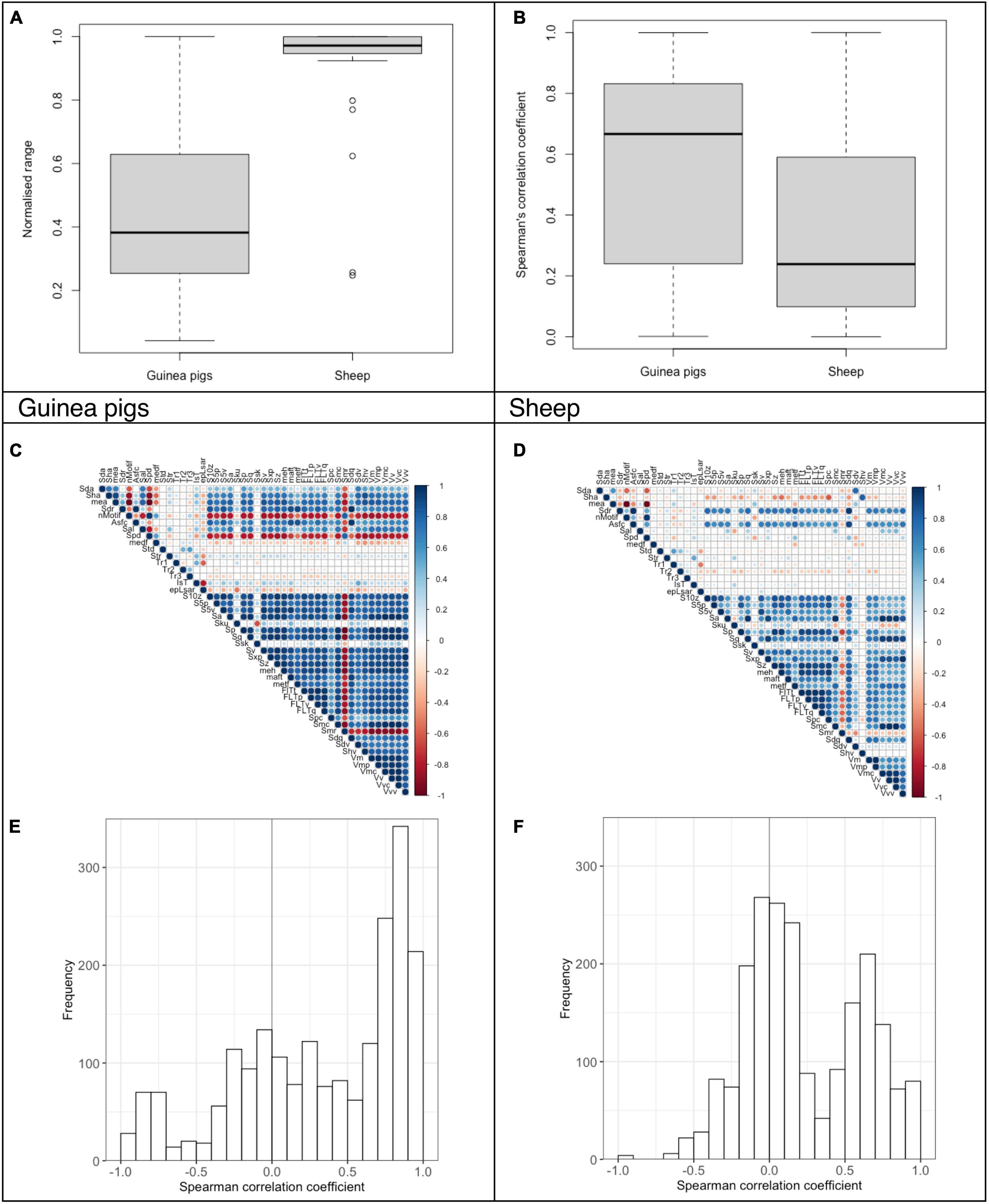
Figure 3. Analysis of DMT parameters for guinea pigs (96 scans) and sheep (78 scans) fed a lucerne based pelleted diet with or without external quartz abrasives (diet groups: control, 4% fine silt, 4% coarse silt, 4% fine sand, 8% fine silt; dataset D). The normalized data range and the Spearman’s correlation coefficients are given as boxplots (A,B). The Spearman’s correlation of the DMT parameters is also shown as a correlation matrix (C,D) and a histogram (E,F).
Species comparison lucerne diets (dataset E)
The normalized data range for rabbits fed a lucerne based pelleted diet was small compared to the guinea pigs and the sheep that had received the same diet (rabbit 0.19 ± 0.18, guinea pigs 0.49 ± 0.32, sheep 0.78 ± 0.22, Figure 4A). However, the correlation pattern was still more robust in the rabbits and guinea pigs (rabbits 0.56 ± 0.30, guinea pigs 0.55 ± 0.31) than in the sheep (sheep 0.42 ± 0.27, Figures 4B–H).
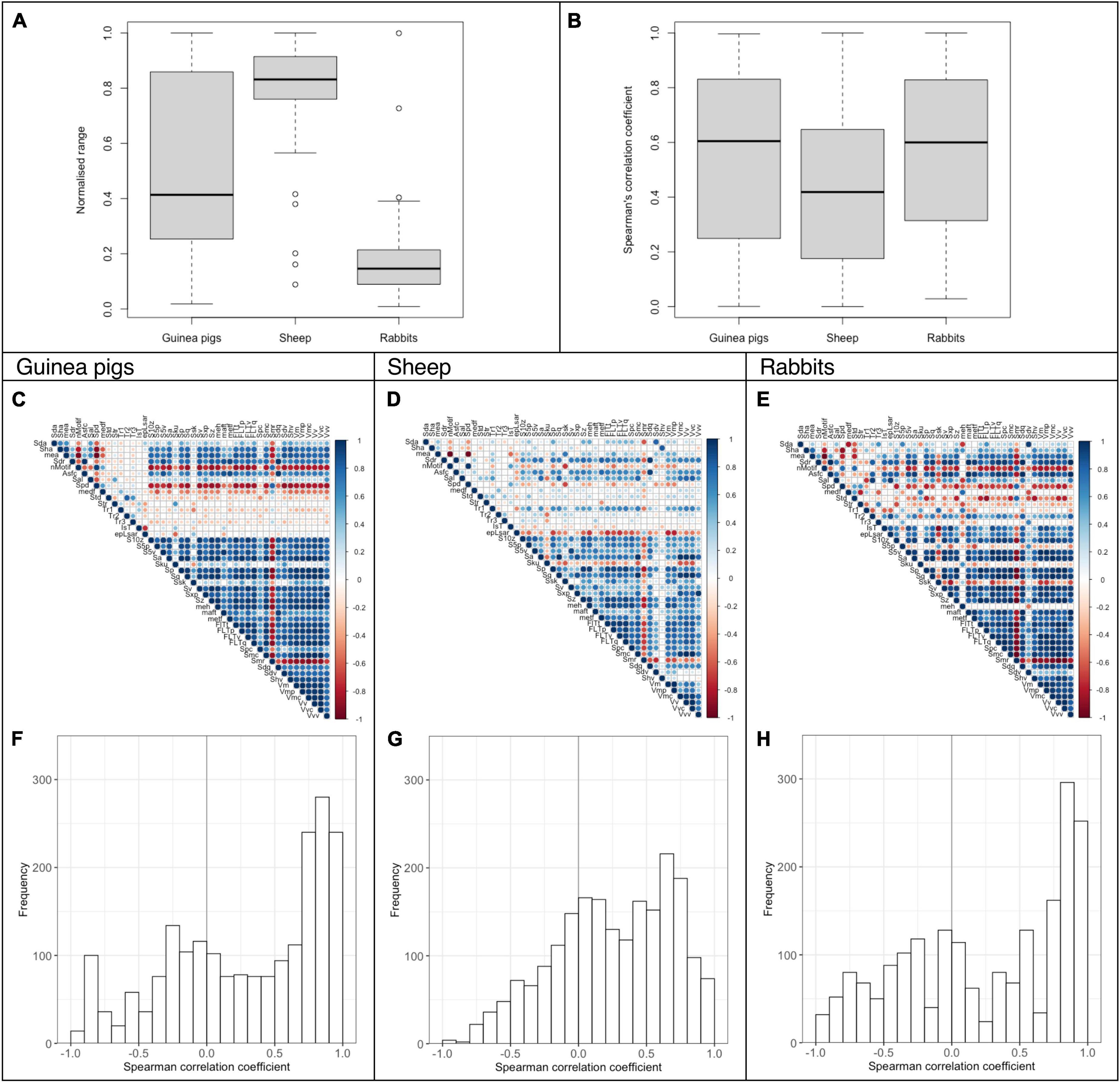
Figure 4. Analysis of DMT parameters for guinea pigs (22 scans), sheep (19 scans) and rabbits (6 scans) fed a lucerne based pelleted diet (without any abrasive added; dataset E). The normalized data range and the Spearman’s correlation coefficients are given as boxplots (A,B). The Spearman’s correlation of the DMT parameters is also shown as a correlation matrix (C–E) and a histogram (F–H).
Discussion
The present study confirms the well-known fact that DMT parameters correlate among each other (Martin et al., 2020; Ackermans et al., 2021). Due to the nature of DMT parameters, which are applied on the same surface and are partially calculated from, or complementary to, each other, this finding in itself is not surprising (International Organization For Standardization, 2012). Therefore, correlations between DMT parameters are rarely reported and re-examined. Due to these interdependencies and the implied redundancy of information when reporting all parameters, some studies only report “key” parameters. These are selected based on various criteria, e.g., by robust but complex statistical approaches (Calandra et al., 2012), often justified and defined as reporting the parameters which have the most significant and stable relations to the anticipated functionalities (Tian et al., 2012). Other studies considered most representative and independent parameters (Martisius et al., 2018), or applied factor analysis to reduce the large number of parameters to put them in a common score (Stuhlträger et al., 2019) or did a review on how commonly they have previously been reported in the DMT literature (Winkler et al., 2020b). Louail et al. (2021) combined 25 DMT parameters into a principal component as an approach to summarize DMT for a comparison between two chewing phase surfaces. Another way to summarize DMT results, “key” parameters could be defined as those that render other parameters superfluous due to consistent correlations—ideally, regardless of tooth position, diet, or species. However, the present study suggest that all these factors influence how well DMT parameters correlate with each other, or, in other words, how inherently consistent the DMT description of a surface is.
Methodological constraints
Constraints of our study were mainly the size differences between the DMT scan windows, and that the sheep received additional lucerne hay (200 g per animal per day) to fulfill their physiological diet requirements which might have had a polishing effect on otherwise more consistent DMT patterns (Ackermans et al., 2020). At present, we cannot rule out that the observed differences between the small mammals and the sheep were due to the larger scan windows investigated in the latter. If there was no random component that makes DMT wear patterns inherently inconsistent, then the size of the window should play no role. It should also play no role if a random component made a scale-independent, constant contribution to DMT patterns because a larger window would, in that scenario, just mean a larger number of both inherently consistent and inherently inconsistent wear elements. On the contrary, if we assume that a larger scan window increases the chance of detecting a more extensive range of DMT signals (Ramdarshan et al., 2017), we might expect more significant correlations among the DMT parameters due to these larger scan windows, not less. Future studies in guinea pigs and rabbits could further investigate the effect of different scan windows on DMT patterns.
The assumption that larger measurement ranges are more likely to yield significant parameter correlations seemed to hold within the guinea pig results. In the tooth position comparison (Figure 1) and the diet comparison (Figure 2), the group with the larger mean measurement range also had the higher mean correlation coefficient. This makes the inter-specific comparisons even more remarkable, where the species with the largest measurement range, the sheep, had less significant correlations between parameters (Figure 3). By carefully selecting teeth of animal groups that differed mainly in only one specific factor, such as tooth position (in the same set of animals), diet type across similar-sized groups of animals, or species across individuals that received similar diets, our analysis provides evidence that these factors do influence the inherent consistency of the wear pattern.
Dental microwear texture data range across the diet groups
Based on macroscopic measurements, it is well known that tooth wear affects individual tooth positions differently (Clauss et al., 2007; Laws, 2008; Müller et al., 2014, 2015; Martin et al., 2020, 2022), either due to the way the abrasives elements are distributed during chewing, the occlusal force along the jaw, the morphology of the occlusal surface, or the ontogenetic difference in the time the teeth are subjected to wear due to their eruption sequence. The same has been explored for microscopic wear along the tooth row: no gradient could be shown in ruminating species such as sheep (Ramdarshan et al., 2017; Ackermans et al., 2020) and blue wildebeest (Schulz et al., 2010), most likely due to the bidirectional chewing process while ruminating. By contrast, a microwear gradient could be identified using DMTA in several crocodilians and varanid species (Bestwick et al., 2021) as well as in Grevyi zebras [Equus grevyi, (Schulz et al., 2010), rabbits (Martin et al., 2020) and guinea pigs (Winkler et al., 2021)]. The biomechanical properties of all the diets used in Winkler et al. (2021) and this study were undoubtedly very different: some animals received natural whole plant diets containing different amounts of phytoliths (up to 3.25% of acid detergent insoluble ash, a proxy for silica, in dry matter of bamboo); other lucerne based pellets with different size quartz abrasives (up to 8% fine silt for 8sS). After feeding on a pelleted diet, several DMT parameters in guinea pigs showed an increasing abrasive signal along the maxillary tooth row. Here, we show that the DMT data range across the diet groups is also higher on M2 compared to P4 and the concomitant correlation pattern is more robust with a higher mean correlation. The abrasive gradient seems to be represented not only in a few selected parameters and visual overview as seen in Winkler et al. (2021) but also in the overall DMT signal summarized as a mean correlation coefficient. Correlation patterns could be more efficient to recognize tooth wear gradients and mechanical changes in the chewing process than looking at each DMT parameter individually. The explanation for the more pronounced patterns in M2 compared to P4 may lie in the higher chewing forces that apply closer to the mandibular joint (Watson et al., 2014; Taylor et al., 2016). The M2 has a broader measurement range (Figure 1), implying that the higher chewing forces do not only lead to more distinct wear features but also a higher number of very mild wear traces, possibly caused by diet components that would not leave any trace at lower chewing forces.
We did not have enough data points to compare the gradient of the correlation pattern for individual diet groups. Still, we found a marked difference in correlation patterns between the natural and the pelleted diets when comparing the DMT across all teeth. Although consisting of the same material (dried lucerne), the pelleted diets most likely have a harder consistency than the whole dried plants. Additionally, whole plant processing most likely depends more on gnawing particle size reduction by the incisors prior to grinding by the cheek teeth, giving the material that reaches the cheek teeth a certain homogeneity that likely does not lead to particularly distinct wear traces. By contrast, pellets are crushed on the cheek teeth (Weijs and Dantuma, 1981), resulting in a large variety of wear traces, with larger DMT measurement ranges and more DMT correlations.
Dental microwear texture parameter correlations between and within different species
Comparing the correlation of DMT measured in sheep after a 17-month feeding experiment with the results in guinea pigs fed the identical pelleted diets for 28–45 days, we show a clear difference between these species. DMT data in sheep was distributed over a more extensive data range, but DMT parameters correlate stronger and more consistently in the guinea pigs. The original sheep study reported solid correlations within the functional groups for height and volume parameters (Ackermans et al., 2021). Yet these correlations seem small compared to the intra- and intergroup correlations seen in guinea pigs (see Supplementary Tables 3, 4). This difference cannot be attributed to the feeding time difference, as microwear is thought to be an effect of a few tooth diet interactions (Teaford and Oyen, 1989) with a turnover rate of around 2 weeks in rats (Rattus norvegicus), for example (Winkler et al., 2020a). To further elucidate interspecies differences and avoid bias by looking only at highly abrasive diets, we compared sheep, guinea pigs, and rabbits fed a low-abrasive diet consisting of pelleted lucerne only. The normalized data range was again greater in sheep than in guinea pigs, while the small sample size can explain the narrow range in rabbits. Nevertheless, the correlations were again better in the hypselodont species.
In ruminant teeth, the chewing surface is not replaced anywhere near as expeditiously as in animals with hypselodont cheek teeth. Under natural feeding conditions in free-ranging animals, we expect the ruminant chewing surface to still display wear traces of diet items ingested a long time ago (Solounias et al., 1994; Damuth and Janis, 2014), which have not been overwritten entirely yet. However, in the present study, this effect was excluded due to the prolonged time the sheep received a consistent diet. Hence, another factor than the slow replacement of the chewing surface must be evoked to explain our findings. We hypothesize that the main driver for the more stable DMT correlations in guinea pigs is the supposedly softer enamel of species with hypselodont dentition (Shakila et al., 2015; Fischer et al., 2022). For the harder ruminant enamel, we hypothesize that wear trace formation is subject to a greater degree of randomness at the microscopic level due to the many factors involved in forming DMT wear traces. These factors include the volume of diet material between the teeth during a chewing stroke, the exact position of the abrasive components in the diet matrix, or the forces during a particular chewing stroke (Kaiser et al., 2016). The less malleable the trace-bearing surface, the more inconsistency in the recorded wear patterns of a specific diet is the apparent consequence. Again, this finding warns against considering tooth wear a taxon-free signal (Fischer et al., 2022), and suggests that understanding the formation of a single wear trace will remain a particular challenge (Van Casteren et al., 2020).
Conclusion
In guinea pigs, we demonstrate a stable correlation pattern of DMT parameters dependent on biomechanical properties of the diet and tooth position. Interspecies comparisons show that regardless of abrasive source, DMT in hypselodont species shows a narrower data range and more robust correlation patterns. We propose that the correlation pattern is stronger when the abrasive wear increases on a particular tooth position or because of a higher abrasive diet, and when the tooth wear is measured in softer enamel. Based on the correlations pattern shown here, key DMT parameters can be identified in DMT datasets but require at least a diet consistency and diet abrasiveness, and a species or taxon-specific approach taking into consideration different chewing mechanisms and dental anatomy.
Data availability statement
The original contributions presented in this study are included in the article/Supplementary material, further inquiries can be directed to the corresponding author.
Ethics statement
This animal study was reviewed and approved by Cantonal Veterinary Office of Zurich, Switzerland.
Author contributions
DW, TT, TK, DC, ES-K, J-MH, and MC designed the study. LM, DW, NA, JM, and MC performed the animal experiment. TK and ES-K provided analytical tools. LM and MC analyzed the data. LM and MC wrote the first draft of the manuscript, which received input from all co-authors. All authors contributed to the article and approved the submitted version.
Funding
The work of LM was funded in part by the Candoc Forschungskredit of the University of Zurich (FK-16-052) and a SNF grant (Schweizerischer Nationalfonds zur Förderung der Wissenschaftlichen Forschung, 31003A_163300/1) to J-MH, which also funded the sheep experiment. The guinea pig feeding experiment was funded by the European Research Council (ERC) under the European Union’s Horizon 2020 Research and Innovation Program (ERC CoG grant agreement No. 681450) to TT. DW was granted a Postdoctoral fellowship from the Japan Society for the Promotion of Science (KAKENHI Grant No. 20F20325).
Acknowledgments
We thank Sandra Heldstab, Kathrin Zbinden (both University of Zurich), and Annelies De Cuyper (Ghent University) for their support in animal husbandry and Lisa Krause (University of Hamburg) for her initial DMTA work in the rabbits.
Conflict of interest
The authors declare that the research was conducted in the absence of any commercial or financial relationships that could be construed as a potential conflict of interest.
Publisher’s note
All claims expressed in this article are solely those of the authors and do not necessarily represent those of their affiliated organizations, or those of the publisher, the editors and the reviewers. Any product that may be evaluated in this article, or claim that may be made by its manufacturer, is not guaranteed or endorsed by the publisher.
Supplementary material
The Supplementary Material for this article can be found online at: https://www.frontiersin.org/articles/10.3389/fevo.2022.958576/full#supplementary-material
References
Ackermans, N. L., Winkler, D. E., Martin, L. F., Kaiser, T. M., Clauss, M., and Hatt, J.-M. (2020). Dust and grit matter: Abrasives of different size lead to opposing dental microwear textures in experimentally fed sheep (Ovis aries). J. Exp. Biol. 223:jeb220442. doi: 10.1242/jeb.220442
Ackermans, N. L., Winkler, D. E., Schulz-Kornas, E., Kaiser, T. M., Martin, L. F., Hatt, J. M., et al. (2021). Dental wear proxy correlation in a long-term feeding experiment on sheep (Ovis aries). J. R. Soc. Interface 18:20210139. doi: 10.1098/rsif.2021.0139
Bestwick, J., Unwin, D. M., Henderson, D. M., and Purnell, M. A. (2021). Dental microwear texture analysis along reptile tooth rows: Complex variation with non-dietary variables. R. Soc. Open Sci. 8:201754. doi: 10.1098/rsos.201754
Calandra, I., Schulz, E., Pinnow, M., Krohn, S., and Kaiser, T. M. (2012). Teasing apart the contributions of hard dietary items on 3D dental microtextures in primates. J. Hum. Evol. 63, 85–98. doi: 10.1016/j.jhevol.2012.05.001
Clauss, M., Franz-Odendaal, T. A., Brasch, J., Castell, J. C., and Kaiser, T. (2007). Tooth wear in captive giraffes (Giraffa camelopardalis): Mesowear analysis classifies free-ranging specimens as browsers but captive ones as grazers. J. Zoo Wildl. Med. 38, 433–445. doi: 10.1638/06-032.1
Damuth, J., and Janis, C. M. (2014). A comparison of observed molar wear rates in extant herbivorous mammals. Ann. Zool. Fennici 51, 188–200. doi: 10.5735/086.051.0219
Desantis, L. R. G. (2016). Dental microwear textures: Reconstructing diets of fossil mammals. Surf. Topogr. 4:23002. doi: 10.1088/2051-672X/4/2/023002
Donohue, S. L., Desantis, L. R. G., Schubert, B. W., and Ungar, P. S. (2013). Was the giant short-faced bear a hyper-scavenger? A new approach to the dietary study of ursids using dental microwear textures. PLoS One 8:e77531. doi: 10.1371/journal.pone.0077531
Fischer, V. L., Winkler, D., Głogowski, R., Attin, T., Hatt, J.-M., Clauss, M., et al. (2022). Species-specific enamel differences in hardness and abrasion resistance between the permanent incisors of cattle (Bos primigenius taurus) and the ever-growing incisors of nutria (Myocastor coypus). PLoS One 17:e0265237. doi: 10.1371/journal.pone.0265237
Hua, L. C., Brandt, E. T., Meullenet, J. F., Zhou, Z. R., and Ungar, P. S. (2015). Technical note: An in vitro study of dental microwear formation using the Bite Master Ii chewing machine. Am. J. Phys. Anthropol. 158, 769–775. doi: 10.1002/ajpa.22823
International Organization For Standardization (2012). ISO 25178-2, geometrical product specifications (Gps)–surface texture: Areal–Part 2: Terms, definitions and surface texture parameters. Geneva: ISO.
Kaiser, T. M., Clauss, M., and Schulz-Kornas, E. (2016). A set of hypotheses on tribology of mammalian herbivore teeth. Surf. Topogr. 4:014003. doi: 10.1088/2051-672X/4/1/014003
Karme, A., Rannikko, J., Kallonen, A., Clauss, M., and Fortelius, M. (2016). Mechanical modelling of tooth wear. J. R. Soc. Interface 13:20160399. doi: 10.1098/rsif.2016.0399
Korzynski, M., Dudek, K., Palczak, A., Kruczek, B., and Kocurek, P. (2018). Experimental models and correlations between surface parameters after slide diamond burnishing. Meas. Sci. Rev. 18, 123–129. doi: 10.1515/msr-2018-0018
Laws, R. (2008). Dentition and ageing of the hippo. Afr. J. Ecol. 6, 19–52. doi: 10.1111/j.1365-2028.1968.tb00899.x
Louail, M., Ferchaud, S., Souron, A., Walker, A. E. C., and Merceron, G. (2021). Dental microwear textures differ in pigs with overall similar diets but fed with different seeds. Palaeogeogr. Palaeoclimatol. Palaeoecol. 572:110415. doi: 10.1016/j.palaeo.2021.110415
Martin, L. F., Ackermans, N. L., Tollefson, T. N., Kircher, P. R., Richter, H., Hummel, J., et al. (2022). Tooth wear, growth and height in rabbits (Oryctolagus cuniculus) fed pelleted or extruded diets with or without added abrasives. J. Anim. Physiol. Anim. Nutr. 106, 630–641. doi: 10.1111/jpn.13565
Martin, L. F., Krause, L., Ulbricht, A., Winkler, D. E., Codron, D., Kaiser, T. M., et al. (2020). Dental wear at macro- and microscopic scale in rabbits fed diets of different abrasiveness: A pilot investigation. Palaeogeogr. Palaeoclimatol. Palaeoecol. 556:109886. doi: 10.1016/j.palaeo.2020.109886
Martisius, N. L., Sidéra, I., Grote, M. N., Steele, T. E., Mcpherron, S. P., and Schulz-Kornas, E. (2018). Time wears on: Assessing how bone wears using 3D surface texture analysis. PLoS One 13:e0206078. doi: 10.1371/journal.pone.0206078
Merceron, G., Ramdarshan, A., Blondel, C., Boisserie, J.-R., Brunetiere, N., Francisco, A., et al. (2016). Untangling the environmental from the dietary: Dust does not matter. Proc. R. Soc. B Biol. Sci. 283:20161032. doi: 10.1098/rspb.2016.1032
Müller, J., Clauss, M., Codron, D., Schulz, E., Hummel, J., Fortelius, M., et al. (2014). Growth and wear of incisor and cheek teeth in domestic rabbits (Oryctolagus cuniculus) fed diets of different abrasiveness. J. Exp. Zool. 321, 283–298. doi: 10.1002/jez.1864
Müller, J., Clauss, M., Codron, D., Schulz, E., Hummel, J., Kircher, P., et al. (2015). Tooth length and incisal wear and growth in guinea pigs (Cavia porcellus) fed diets of different abrasiveness. J. Anim. Physiol. Anim. Nutr. 99, 591–604. doi: 10.1111/jpn.12226
Ramdarshan, A., Blondel, C., Gautier, D., Surault, J., and Merceron, G. (2017). Overcoming sampling issues in dental tribology: Insights from an experimentation on sheep. Palaeontol. Electron. 20, 1–19. doi: 10.26879/762
Robinet, C., Merceron, G., Candela, A. M., and Marivaux, L. (2020). Dental microwear texture analysis and diet in caviomorphs (Rodentia) from the Serra do Mar Atlantic forest (Brazil). J. Mammal. 101, 386–402. doi: 10.1093/jmammal/gyz194
Robinet, C., Merceron, G., Catzeflis, F., Candela, A. M., and Marivaux, L. (2022). About inter- and intra-specific variability of dental microwear texture in rodents: Study of two sympatric Proechimys (Echimyidae) species from the Cacao locality, French Guiana. Palaeogeogr. Palaeoclimatol. Palaeoecol. 591:110880. doi: 10.1016/j.palaeo.2022.110880
Rodriguez-Rojas, F., Borrero-Lopez, O., Constantino, P. J., Henry, A. G., and Lawn, B. R. (2020). Phytoliths can cause tooth wear. J. R. Soc. Interface 17:20200613. doi: 10.1098/rsif.2020.0613
Schulz, E., Calandra, I., and Kaiser, T. (2010). Applying tribology to teeth of hoofed mammals. Scanning 32, 162–182. doi: 10.1002/sca.20181
Schulz, E., Piotrowski, V., Clauss, M., Mau, M., Merceron, G., and Kaiser, T. M. (2013). Dietary abrasiveness is associated with variability of microwear and dental surface texture in rabbits. PLoS One 8:e56167. doi: 10.1371/journal.pone.0056167
Schulz-Kornas, E., Stuhltrager, J., Clauss, M., Wittig, R. M., and Kupczik, K. (2019). Dust affects chewing efficiency and tooth wear in forest dwelling Western chimpanzees (Pan troglodytes verus). Am. J. Phys. Anthropol. 169, 66–77. doi: 10.1002/ajpa.23808
Schulz-Kornas, E., Winkler, D. E., Clauss, M., Carlsson, J., Ackermans, N. L., Martin, L. F., et al. (2020). Everything matters: Molar microwear texture in goats (Capra aegagrus hircus) fed diets of different abrasiveness. Palaeogeogr. Palaeoclimatol. Palaeoecol. 552:109783. doi: 10.1016/j.palaeo.2020.109783
Scott, R. S., Ungar, P. S., Bergstrom, T. S., Brown, C. A., Childs, B. E., Teaford, M. F., et al. (2006). Dental microwear texture analysis: Technical considerations. J. Hum. Evol. 51, 339–349. doi: 10.1016/j.jhevol.2006.04.006
Shakila, N., Ali, A., and Zaidi, S. (2015). Micro hardness of dental tissues influenced by administration of aspirin during pregnancy. Int. J. Morphol. 33, 586–593. doi: 10.4067/S0717-95022015000200028
Solounias, N., Fortelius, M., and Freeman, P. (1994). Wear rates in ruminants. Ann. Zool. Fennici 31, 219–227.
Stuhlträger, J., Schulz-Kornas, E., Wittig, R. M., and Kupczik, K. (2019). Ontogenetic dietary shifts and microscopic tooth wear in western chimpanzees. Front. Ecol. Evol. 7:298. doi: 10.3389/fevo.2019.00298
Taylor, L. A., Müller, D. W. H., Schwitzer, C., Kaiser, T. M., Castell, J. C., Clauss, M., et al. (2016). Comparative analyses of tooth wear in free-ranging and captive wild equids. Equine Vet. J. 48, 240–245. doi: 10.1111/evj.12408
Teaford, M. F., and Oyen, O. J. (1989). In vivo and in vitro turnover in dental microwear. Am. J. Phys. Anthropol. 80, 447–460. doi: 10.1002/ajpa.1330800405
Teaford, M. F., Ungar, P. S., Taylor, A. B., Ross, C. F., and Vinyard, C. J. (2017). In vivo rates of dental microwear formation in laboratory primates fed different food items. Biosurf. Biotribol. 3, 166–173. doi: 10.1016/j.bsbt.2017.11.005
Tian, Y., Wang, J., Peng, Z., and Jiang, X. (2012). A new approach to numerical characterisation of wear particle surfaces in three-dimensions for wear study. Wear 282, 59–68. doi: 10.1016/j.wear.2012.02.002
Van Casteren, A., Strait, D. S., Swain, M. V., Michael, S., Thai, L. A., Philip, S. M., et al. (2020). Hard plant tissues do not contribute meaningfully to dental microwear: Evolutionary implications. Sci. Rep. 10:582. doi: 10.1038/s41598-019-57403-w
Wang, M., Peng, Z., Wang, J., and Jiang, X. (2013). Wear characterisation of articular cartilage surfaces at a nano-scale using atomic force microscopy. Tribol. Int. 63, 235–242. doi: 10.1016/j.triboint.2012.11.001
Watson, P. J., Gröning, F., Curtis, N., Fitton, L. C., Herrel, A., Mccormack, S. W., et al. (2014). Masticatory biomechanics in the rabbit: A multi-body dynamics analysis. J. R. Soc. Interface 11:20140564. doi: 10.1098/rsif.2014.0564
Wei, T., and Simko, V. (2021). R package ‘corrplot’: Visualization of a correlation matrix. *cy,pub.
Weijs, W. A., and Dantuma, R. (1981). Functional anatomy of the masticatory apparatus in the rabbit (Oryctolagus cuniculus). Neth. J. Zool. 31, 99–147. doi: 10.1163/002829680X00212
Wickham, H. (2016). ggplot2: Elegant graphics for data analysis. New York, NY: Springer-Verlag. doi: 10.1007/978-3-319-24277-4
Wickham, H., Averick, M., Bryan, J., Chang, W., Mcgowan, L., François, R., et al. (2019). Welcome to the tidyverse. J. Open Source Softw. 4:1686. doi: 10.21105/joss.01686
Winkler, D. E., Andrianasolo, T. H., Andriamandimbiarisoa, L., Ganzhorn, J. U., Rakotondranary, S. J., Kaiser, T. M., et al. (2016). Tooth wear patterns in black rats (Rattus rattus) of Madagascar differ more in relation to human impact than to differences in natural habitats. Ecol. Evol. 6, 2205–2215. doi: 10.1002/ece3.2048
Winkler, D. E., Clauss, M., Rölle, M., Schulz-Kornas, E., Codron, D., Kaiser, T. M., et al. (2021). Dental microwear texture gradients in guinea pigs reveal that material properties of the diet affect chewing behaviour. J. Exp. Biol. 224:jeb242446. doi: 10.1242/jeb.242446
Winkler, D. E., and Kaiser, T. (2015). Uneven distribution of enamel in the tooth crown of a Plains Zebra (Equus quagga). PeerJ. 3:e1002. doi: 10.7717/peerj.1002
Winkler, D. E., Schulz-Kornas, E., Kaiser, T. M., Codron, D., Leichliter, J., Hummel, J., et al. (2020a). The turnover of dental microwear texture: Testing the” last supper” effect in small mammals in a controlled feeding experiment. Palaeogeogr. Palaeoclimatol. Palaeoecol. 557:109930. doi: 10.1016/j.palaeo.2020.109930
Winkler, D. E., Schulz-Kornas, E., Kaiser, T. M., De Cuyper, A., Clauss, M., and Tütken, T. (2019). Forage silica and water content control dental surface texture in guinea pigs and provide implications for dietary reconstruction. Proc. Natl. Acad. Sci. U.S.A. 116, 1325–1330. doi: 10.1073/pnas.1814081116
Winkler, D. E., Tütken, T., Schulz-Kornas, E., Kaiser, T. M., Müller, J., Leichliter, J., et al. (2020b). Shape, size, and quantity of ingested external abrasives influence dental microwear texture formation in guinea pigs. Proc. Natl. Acad. Sci. U.S.A. 117:22264. doi: 10.1073/pnas.2008149117
Keywords: dental microwear texture analysis, rodent, ruminant, phytolith, quartz abrasive, feeding experiment
Citation: Martin LF, Winkler DE, Ackermans NL, Müller J, Tütken T, Kaiser T, Codron D, Schulz-Kornas E, Hatt J-M and Clauss M (2022) Dental microwear texture analysis correlations in guinea pigs (Cavia porcellus) and sheep (Ovis aries) suggest that dental microwear texture signal consistency is species-specific. Front. Ecol. Evol. 10:958576. doi: 10.3389/fevo.2022.958576
Received: 31 May 2022; Accepted: 29 July 2022;
Published: 25 August 2022.
Edited by:
Ferran Estebaranz-Sánchez, Milá y Fontanals Institution for Research in Humanities (CSIC), SpainReviewed by:
Miriam Belmaker, University of Tulsa, United StatesCéline Robinet, Universidad Nacional de La Plata, Argentina
Copyright © 2022 Martin, Winkler, Ackermans, Müller, Tütken, Kaiser, Codron, Schulz-Kornas, Hatt and Clauss. This is an open-access article distributed under the terms of the Creative Commons Attribution License (CC BY). The use, distribution or reproduction in other forums is permitted, provided the original author(s) and the copyright owner(s) are credited and that the original publication in this journal is cited, in accordance with accepted academic practice. No use, distribution or reproduction is permitted which does not comply with these terms.
*Correspondence: Louise Françoise Martin, bGZtYXJ0aW5AdmV0Y2xpbmljcy51emguY2g=
†ORCID: Louise Françoise Martin, orcid.org/0000-0003-4326-0788; Daniela Eileen Winkler, orcid.org/0000-0001-7501-2506; Nicole Lauren Ackermans, orcid.org/0000-0001-8336-1888; Thomas Kaiser, orcid.org/0000-0002-8154-1751; Daryl Codron, orcid.org/0000-0001-5223-9513; Ellen Schulz-Kornas, orcid.org/0000-0003-1657-8256; Jean-Michel Hatt, orcid.org/0000-0002-7043-7430; Marcus Clauss, orcid.org/0000-0003-3841-6207