- 1Department of Natural Environmental Studies, Graduate School of Frontier Sciences, The University of Tokyo, Kashiwa, Japan
- 2Department of Biological Sciences, Clemson University, Clemson, SC, United States
- 3Nagoya University Museum, Nagoya, Japan
- 4Department of Comparative Biomedical Sciences, The Royal Veterinary College, North Mymms, Hertfordshire, United Kingdom
- 5The University Museum, The University of Tokyo, Tokyo, Japan
- 6Marine Macroevolution Unit, Okinawa Institute of Science and Technology Graduate University, Onna-son, Japan
Dental wear analyses are classically applied to mammals because they have evolved heterodont dentitions for sophisticated mastication. Recently, several studies have shown a correlation between pre-assigned and analytically inferred diet preferences in extant reptiles through dental microwear texture analysis (DMTA), a method using quantitative assessment of microscopic wear marks to reconstruct the diet material properties. The first tentative applications of DMTA to extinct reptiles have followed. However, for large and small mammals, microwear analyses have undergone a long time of ground-truthing through direct feeding observations, stomach content analyses, and feeding experiments. Such data are currently lacking for reptiles, but are necessary to further extend DMTA, especially to Archosauria, as the application to dinosaurs could be of great interest to the scientific community. We herein present data from a pilot feeding experiment with five juvenile American alligators (Alligator mississippiensis). Each individual received a diet of assumed different hardness for ~4 months: crocodylian pellets (control), sardines, quails, rats, or crawfish. All individuals initially received the same pellet diet, and we found them to show similar dental microwear texture patterns before they were switched to their designated experimental diet. From the first feeding bout on, dental microwear textures differed across the diets. The crawfish-feeder showed consistently higher surface complexity, followed by the rat-feeder. Quail- and fish-feeding resulted in similar wear signatures, with low complexity. Fast tooth replacement and selective tooth use likely affected microwear formation, but we were able to detect a general hard (crawfish and rat) versus soft (quail and fish) DMTA signature. Such patterns can support the identification of hard-object feeding in the fossil record.
Introduction
The study of dental microwear texture (DMT) using standardized textural parameters for quantification of diet-related wear patterns has gained considerable interest in the last decade, particularly for application to non-mammalian species. With pioneering studies on fish (Purnell et al., 2012; Purnell and Darras, 2015), lepidosaurs and archosaurs (Bestwick et al., 2019; Winkler et al., 2019a), pterosaurs (Bestwick et al., 2020), phytosaurs (Bestwick et al., 2021a), and sauropods (Sakaki et al., 2022), it has been shown that diet-related DMT features are formed (and preserved) in species without heterodont, occluding teeth, and without sophisticated mastication. Many of these non-mammalian species exhibit frequent tooth replacement, but tooth-to-food contact still seems to be sufficient to result in significant DMT differences between diet preference groups, information that can be used for dietary discrimination in extant and extinct taxa. However, reptiles were also found to display non-diet-induced DMT patterns that may be related to tooth position, bite force, and behavioral differences in tooth use (Bestwick et al., 2021b), thus complicating the assessment of diet-related DMTs.
Because diet proxies based on tooth-wear patterns can enable inferences of niche partitioning among sympatric species (Fiorillo, 1998; Mallon and Anderson, 2014), they can enhance reconstructions of paleoecosystems. Several studies have linked microscopic (2D microwear) and macroscopic tooth wear to paleodiet in extinct archosauriforms (Schubert and Ungar, 2005; Williams et al., 2009; Varriale, 2016; Virag and Osi, 2017) and squamates (Holwerda et al., 2013; Gere et al., 2021). Therefore, comparisons of DMT observed in extant reptiles could also be helpful for the reconstruction of the paleoecology of taxa such as dinosaurs, with extant toothed archosaurs (crocodylians) likely being the best candidates to study as a model for theropod dental wear. Dental microwear texture analysis (DMTA) has undergone a long time of ground-truthing for large (Merceron et al., 2016; Ackermans et al., 2020; Schulz-Kornas et al., 2020) and small mammals (Schulz et al., 2013; Winkler et al., 2019b, 2020a,b, 2021) through feeding experiments. However, so far, no direct observations regarding diet and tooth wear exist for extant archosaurs that could validate our interpretation of diet-related wear marks. Instead, DMTA for reptiles still relies on dietary preference data compiled from the literature (from observations and stomach content analysis).
To observe a direct effect of ingested diet on dental microwear texture, we performed controlled feeding experiments with five juvenile American alligators (Alligator mississippiensis). Extant crocodylians are opportunistic carnivores, with most species having a generalistic diet of animal prey (Pooley, 1989). Still, dietary differences exist across crocodylian taxa. For example, the Indian gharial, the most slender-snouted of extant crocodylians, is primarily piscivorous (Thorbjarnarson, 1990; Stevenson and Whitaker, 2010; Grigg and Kirshner, 2015), whereas broader snouted crocodylians include harder prey in their diet, such as crustaceans (Taylor, 1979; Platt et al., 2006, 2013) and turtles (Taylor, 1986; Barr, 1997). Moreover, most crocodylians undergo distinct ontogenetic dietary changes. Juveniles often feed on invertebrates and small vertebrates (fish and amphibians), but switch to larger vertebrates (mammals and fish) as adults (Cott, 1961; Taylor, 1979; Delany and Abercrombie, 1986; Hutton, 1987; Wolfe et al., 1987; Webb and Manolis, 1989; Platt et al., 2006, 2013; Wallace and Leslie, 2008). If a specialized diet would result in distinct DMTA signatures, transitions in diet and the main components of diet might be detectable in crocodylians, such that microwear data could help to resolve ontogenetic dietary changes in fossil archosaurs.
For our comparisons, we selected four diet items assumed to be of different hardness, representing hypothetical, specialized feeding types, and compared DMTA patterns from shed teeth of alligators on each of these diets to those from a control alligator kept on the same pelleted diet received before our experiment. Pellets were provided as a control food item because we expected that their consumption would not leave distinct dental wear, as they are not seized or processed with the teeth, but instead only swallowed. Our four experimental foods included sardines (representing a piscivorous diet), quails (representing a “soft” vertebrate diet), rats (representing a “hard” vertebrate diet), and crawfish (representing a “hard” invertebrate diet). Consistent comparative data of mechanical and material properties of our selected diet items are difficult to compile, but several studies support our intuitive assessment of these diets being different in their “hardness” or differently mechanically challenging to process: Young's modulus and bending strength of bones have been found to be lower in teleost fish when compared to rats and other mammals (Erickson and Catanese, 2002; Horton and Summers, 2009). Birds have overall thinner, pneumatized bones (Swartz et al., 1992; Cubo and Casinos, 2000) than mammals, while crawfish, as crustaceans, possess a resistant exoskeleton (Raabe et al., 2005) that needs to be fractured during prey processing.
While the exact interrelations between mechanical properties and DMT are unclear (Winkler et al., 2022), previous studies have shown that extant archosaurs with a presumed piscivorous diet had the lowest enamel surface roughness (Bestwick et al., 2019), whereas extant archosaurs and lepidosaurs assumed to feed on “hard” invertebrates (mollusks and crustaceans) exhibited higher surface roughness and surface complexity (Bestwick et al., 2019; Winkler et al., 2019a). Therefore, sardines and crawfish represent the two extreme endpoints of our experimental diet spectrum, with sardine-feeding expected to result in overall lower wear (low roughness and complexity), and crawfish expected to have the strongest effect on dental enamel wear (large roughness and complexity). Quails and rats were chosen as intermediate hardness samples in our dietary continuum because bird skeletons are generally lightweight and delicate, in contrast to the skeletons of terrestrial mammals; therefore, it could be assumed that quails represent an overall softer diet than rats. There is some uncertainty in these assessments. For example, in birds and mammals of similar body mass, the skeleton contributes equally to total body mass (Prange et al., 1979); however, long bones of birds are often pneumatized, with significantly thinner walls than marrow-filled bones found in mammals (Cubo and Casinos, 2000). The surprisingly large skeletal mass of birds results from higher bone density compared to terrestrial rodents (Dumont, 2010), which gives bird skeletons a higher strength-to-weight and stiffness-to-weight ratio. Thus, our use of both quails and rats as samples should help to determine whether these prey items actually pose different mechanical challenges to alligators, or if these two tetrapod vertebrate diets might result in similar DMT patterns.
Materials and methods
Five juvenile American alligators (Alligator mississippiensis) were housed individually at Clemson University, South Carolina (USA), where they are part of a larger number of alligators kept for locomotion studies. Alligator husbandry and further experimental procedures were approved by the Clemson University IACUC (protocol 2019-037). The sex of the animals was unknown. In July 2020, the average initial body mass of the animals was 5,076.46 g (SD ± 1,368.98 g), while the average snout-vent length was 612.00 mm (SD ± 53.40 mm) (Table 1). For two individuals, weight and length were determined again in December 2021, resulting in an average weight of 6,484.30 g (SD ± 1,252.00 g) and snout-vent length of 632.00 mm (SD ± 56.57 mm). Further details about the individuals and their husbandry can be found in a study by (Iijima et al., 2021).
Before the start of the feeding experiments, all alligators were fed a diet of commercial pellets for crocodylians (Mazuri crocodylian diet, small) two times a week. The feeding experiment took place in two phases, from February/March until May/June 2021 (~4 months per diet) and from September to November/December 2021 (~2 months per diet). At the beginning of the feeding experiment, four out of five juvenile alligators were switched to their designated experimental diet, either receiving whole sardines (wild-caught, frozen sardines from Portugal, ordered through wholey.com; alligator individual identifier #2), whole quails (frozen extra-large Coturnix, ordered through rodentpro.com; #4), whole crawfish (live red swamp crawfish Procambarus clarkia, ordered through lacrawfish.com, and frozen red swamp crawfish ordered through acadiacrawfish.com; #3), or whole rats (frozen medium feeder rats, ordered through rodentpro.com; #1). One individual was kept on the pelleted diet as a control during phase 1 (#6). The experimental feeds were first thawed, and then presented as whole items two times a week. During phase 1, quails and rats were similar in body weight and size, while sardines were longer and more lightweight (Supplementary Table S1). Crawfish were much smaller than the vertebrate diets, and hence ~7 crawfish were given for each feeding bout (Supplementary Table S1). Phase 2 was a repetition of sardine- and crawfish-feeding, with alligators that had received different foodstuffs during the first period and were switched back to pellets from June to late September 2021. The individual receiving quails during phase 1 (#4) received crawfish and the one kept on the pellets (#6) received sardines. During phase 2, sardines were smaller and more lightweight than during phase 1, thus ~1.8 sardines were fed for each feeding bout. Details of the feeding schedule and observations of feeding behavior are given in Supplementary Table S1. After each feeding bout, shed teeth were collected from each individual tank and the source individual and date of the collection were recorded. Feeding events were captured on video, from which the number of bites and behavioral notes were derived.
Dental microwear texture sampling strategy
Collected teeth were shipped to the University of Tokyo with export permission from the United States Fish and Wildlife Service (CITES permit # 21US05232E/9). The original enamel surfaces of the shed alligator teeth were scanned using a confocal laser microscope (VK-9700, Keyence, Osaka, Japan) with a violet laser (408 nm), equipped with a long working distance 100x lens (N.A. = 0.95) (resolution in x, y = 0.138 μm, step size in z = 0.001 μm). Scans were obtained from the buccal side (which was identified through the curvature of the tooth) as close to the apex as possible, but always within the third of the tooth crown closest to the apex for several reasons. The enamel is thickest at the tooth tip (Kvam, 1959) and gets thinner along the tooth crown toward the root. Therefore, the apex is likely to preserve the enamel wear without exposing the dentine quickly. Additionally, the apex is most likely to get in contact with food items, either for seizing and holding them, or for processing the prey. The apex is therefore the focus area of a conical tooth (without a developed occlusal surface or visible wear facets) to show diet-related wear.
Teeth were cleaned with acetone-soaked cotton swaps. If teeth still showed attached dirt particles afterward, they were additionally subjected to cleaning in an ultrasound bath. First, teeth were individually placed in a 2% NaClO solution for 3 min, and then transferred to a milli-Q water bath for 3 min.
Up to four scans (141 × 106 μm) were taken for each tooth, and if several teeth were retrieved after the same feeding bout, these were treated as belonging to the same date. All scans were trimmed in MountainsMap v. 9.0.9878 to 100 × 100 μm to exclude peripheral damage. For each tooth, median parameter values were calculated from the obtained scans. Then, for each collection date, mean parameter values were calculated from all obtained teeth recovered on that date.
Teeth could not be assigned to a specific tooth position or jaw. However, because posterior teeth are broader and shorter, with blunter tips compared to anterior teeth, overall tooth shape could be used to designate teeth as having come from either posterior (molariform) or central/anterior (caniniform) positions (Berkovitz and Shellis, 2017). Similar to Bestwick et al. (2019), we avoided including molariform teeth, as they are used for crushing and might be used differently on food items of different hardness, and because they were more scarce among the collected teeth. Instead, we concentrated on sharp, conical teeth in our sample.
The teeth of alligators often show pronounced enamel wrinkles (Sander, 1999). Through dental wear, the wrinkles are worn away and enamel surfaces appear smoother (pers. observation, Supplementary Figures S1, S2). We found that the pellet-feeding individual as well as sardine- and quail-feeding individuals showed the most pronounced enamel wrinkles. These are problematic for assessing diet-related DMTs, as the commonly applied surface roughness parameters from ISO 25871 would record higher surface roughness from the natural enamel topography of relatively unworn teeth. In DMTA, filtering protocols are usually applied to account for the gross shape and waviness of the enamel surface. The commonly applied filtering procedure in MountainsMap v. 9.0.9878 for the employed microscope (compare Kubo and Fujita, 2021; Winkler and Kubo, 2022) did not result in the satisfactory removal of the enamel wrinkles (Supplementary Figures S1, S2). Thus, we tested stronger filtering algorithms until satisfied with the result (Supplementary Figures S1, S2), and settled on a robust Gaussian filter (with a cut-off value of 0.8 μm, using the resulting S-F surface) followed by a Gaussian filter (with a cut-off-value of 20 μm, using the resulting S-L surface). As this strong filtering procedure not only removed enamel wrinkles, but also reduced the height and depth of diet-induced wear features, we found that most of the commonly applied DMTA parameters from ISO 25178, motif, and furrow analysis did not detect differences between diet treatments. However, parameters reflecting the complexity of the surface such as the scale-sensitive fractal analysis (SSFA) parameter area-scale fractal complexity (Asfc) and ISO 25178 developed interfacial area ratio (Sdr) were less influenced by the strong filter routine, and still showed significant differences between diets. Complexity is known to indicate hard-object feeding in primates (Scott et al., 2005, 2012; Ungar et al., 2008), carnivores (DeSantis et al., 2012), and reptiles (Winkler et al., 2019a), and hence we only concentrate on complexity parameters in this study. We note that Asfc is calculated over the whole scale of a surface (Scott et al., 2006). Our approach uses filtered, scale-limited surfaces, because using the whole scale of the surface would result in misinterpretation of enamel wrinkles as wear-induced topography (hills and valleys). Thus, we are diverging from the common practice of SSFA-based DMTA, which makes our results less comparable to other studies. The range of Asfc, and the absolute values obtained, will be smaller in the current study as compared to previous studies. Still, we consider this approach justified due to the nature of the studied surfaces, i.e., the natural (unworn) enamel topography, and because the observed differences in Asfc were also confirmed by the ISO 25178 parameter Sdr, which is applicable to describe surface geometry of the scale-limited surface.
Statistics
All statistical analyses were conducted in JMP v.16.0. We pooled data for each experimental diet starting with teeth collected after the first feeding bout. Thereby, teeth experiencing only one feeding event on an experimental diet and teeth experiencing several feeding events were grouped. A Shapiro–Wilk test indicated that mean food item weight (p = 0.217), mean bite count (p = 0.652), mean Asfc (p = 0.950), and mean Sdr (p = 0.931) per diet were normally distributed, hence we analyzed their relationship using linear correlations (Pearson coefficient). We used a heteroscedastic pairwise comparison test (Wilcoxon test) to compare complexity patterns between all dietary pairs. Data were also visualized per tooth on the collection day over the course of the feeding experiment. However, because on several days only one tooth was recovered, we did not statistically compare differences between individual dates within or between diets.
Results
Feeding observations
The pelleted diet was consumed slower and more reluctantly by individual #6. The four individuals on experimental diets displayed high levels of anticipation prior to and during feeding time, standing on their hindlegs, jumping, and frantically searching for the prey item once it was dropped into the water (see Supplementary Videos). They often missed the prey during the first seconds due to this turmoil and had to be pointed toward the food with a long stick.
During the first experimental phase, the mean bite count on the four experimental feeds was lowest on the rat diet, followed by quails, and highest on crawfish and sardines (Figure 1, Supplementary Figure S3, Supplementary Table S1). During the second phase, the bite count on crawfish was even higher than during the first phase, while the bite count on sardines was lower, even though most of the time two instead of one sardine were fed (Supplementary Figure S3, Supplementary Table S1). Individual #4 received different diets during each experimental phase and displayed different mean bite counts, with many fewer bites on quails (23.96) than on crawfish (42.71) (Supplementary Figure S3). There was no significant correlation between the weight of the diet items and bite count (p = 0.691, Figure 1). Bite count was not recorded for the pellet-feeding individual, as the feeding motion did not include visible biting or processing of pellets.
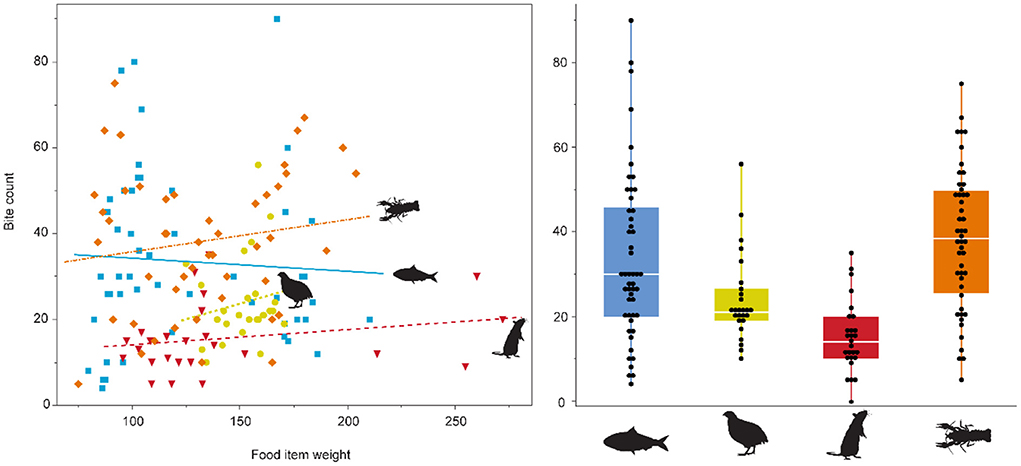
Figure 1. Bite count vs. food item weight (g), and per diet. No relationship between diet type and bite count, or food item weight and bite count was found. Overall, the alligator feeding on rats displayed the least bites per feeding bout, while the crawfish feeder showed the most bites. The pelleted diet is not displayed, as the alligator did not exhibit “chewing” behavior on pellets. Sardine = blue, quail = yellow, rat = red, crawfish = orange.
Dental microwear texture analysis
Feeding on the pelleted diet resulted in overall smoother dental enamel surface textures (Figure 2). Visually, surfaces of teeth from pellet-feeders seem to show no or little wear marks. Sardine-feeding and quail-feeding resulted in similar surface wear as pellet-feeding, with only small visible wear marks. Rat- and crawfish-feeding, on the contrary, resulted in visible, deep wear marks (scratches).
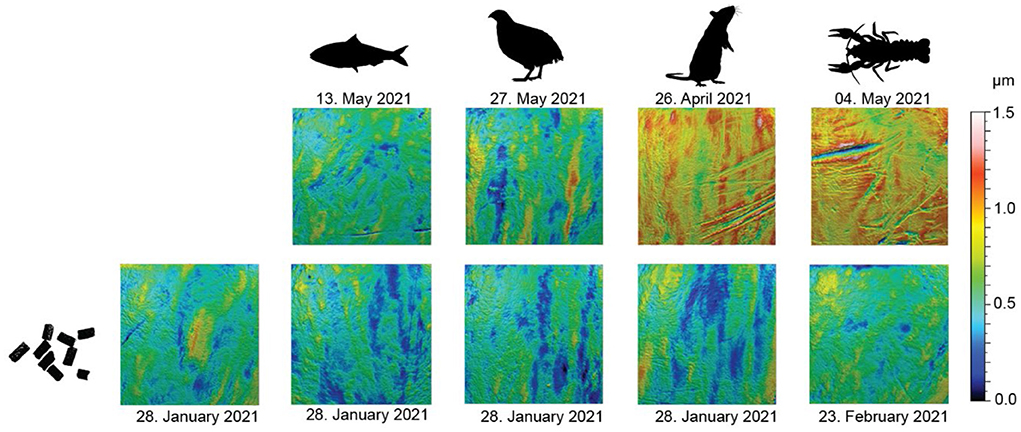
Figure 2. Scale-limited, filtered, 3D photosimulations of enamel surface textures for each diet. All surfaces are to the same scale. Scan size: 100 × 100 μm. The upper row shows a diet-related surface from late April/mid-May (~3 months into the first phase of the feeding experiment). The lower row shows a surface from a tooth of the same individual as in the corresponding upper row but collected immediately before the start of the feeding experiment (while still feeding on pellets).
The pelleted diet resulted in similar complexity (Asfc and Sdr) for all individuals (Figure 3, Supplementary Table S2). Before being placed on their designated experimental diet, at least one shed tooth was collected from each individual. The DMT of these falls within the range of Asfc and Sdr values observed over the duration of the first experimental phase for the control individual which remained on the pelleted diet (Figure 3). Only one tooth collected for individual #6, before being switched to sardine-feeding, shows significantly lower Asfc and Sdr values than teeth from the same individual when compared to the pooled data for itself over the course of phase 1, and individuals #2 (dedicated sardine-feeder) and #3 (dedicated crawfish-feeder).
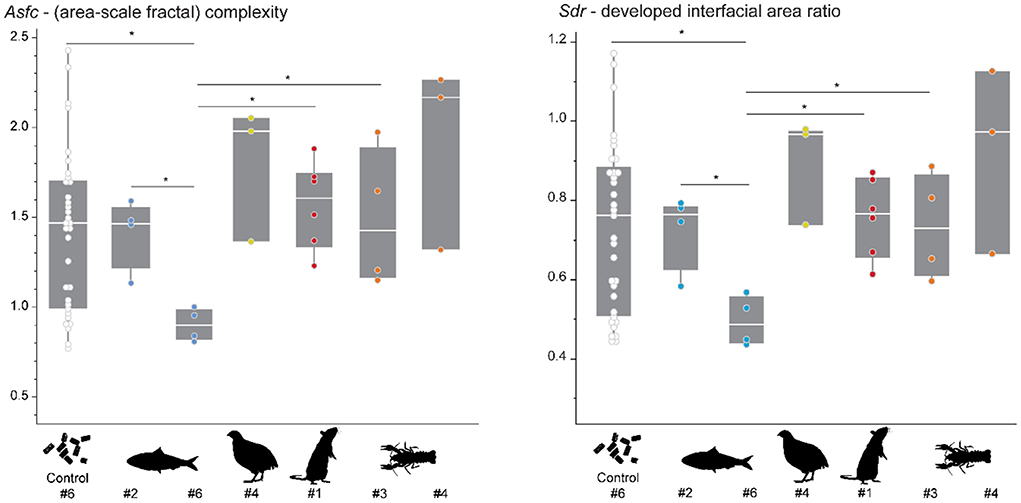
Figure 3. Asfc and Sdr recorded in different individuals while feeding on the pelleted diet. The control animal (#6) received the pelleted diet over the course of the feeding experiment. For the other individuals, 1–2 teeth were collected immediately before starting the feeding experiment. Each scan is treated as an individual datapoint in this plot. Significance level: * = 0.05. Colored points indicate the diet which was assigned to each individual during the feeding experiment: sardine = blue, quail = yellow, rat = red, crawfish = orange.
Pooling of all data over the course of the experiment highlights that already after the first feeding bout, several experimental diets resulted in a change of DMT. Each experimental diet except for quails displays significantly higher Asfc and Sdr values than the control individual (Figure 4). Within experimental diets, complexity increases in the following order: quail ≤ sardine < rat ≤ crawfish (Supplementary Table S2). The crawfish feeder showed significantly larger Asfc and Sdr values than the sardine and quail feeders, and non-significantly larger Asfc and Sdr values than the rat feeder. Between sardine and quail, or rat and crawfish, no significant differences in Asfc and/or Sdr values were found. It is evident that Asfc and Sdr reflect the same qualities of the surface. Hence, over the course of the experiment, we only displayed a change in Asfc (Figure 5). Mean complexity values were not significantly correlated to mean bite counts in any of the diets (Asfc: p = 0.425, Sdr: p = 0.353, Supplementary Figure S3).
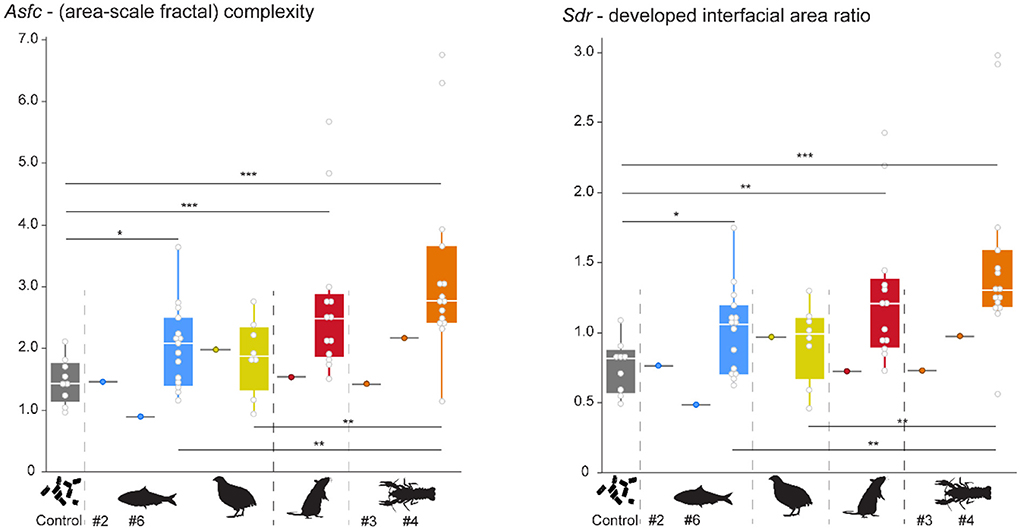
Figure 4. Pooled Asfc and Sdr observed for all diets over the whole experimental duration. Significance level: * = 0.05, ** = 0.01, *** = 0.001. Sardine = blue, quail = yellow, rat = red, crawfish = orange. Gray boxplots with colored points indicate that teeth were collected before the start of the feeding experiments, while the alligator was still feeding on pellets. Pooled data were created by using the median from all scans per tooth, and then calculating a mean per collection date. Each collection date represents one point.
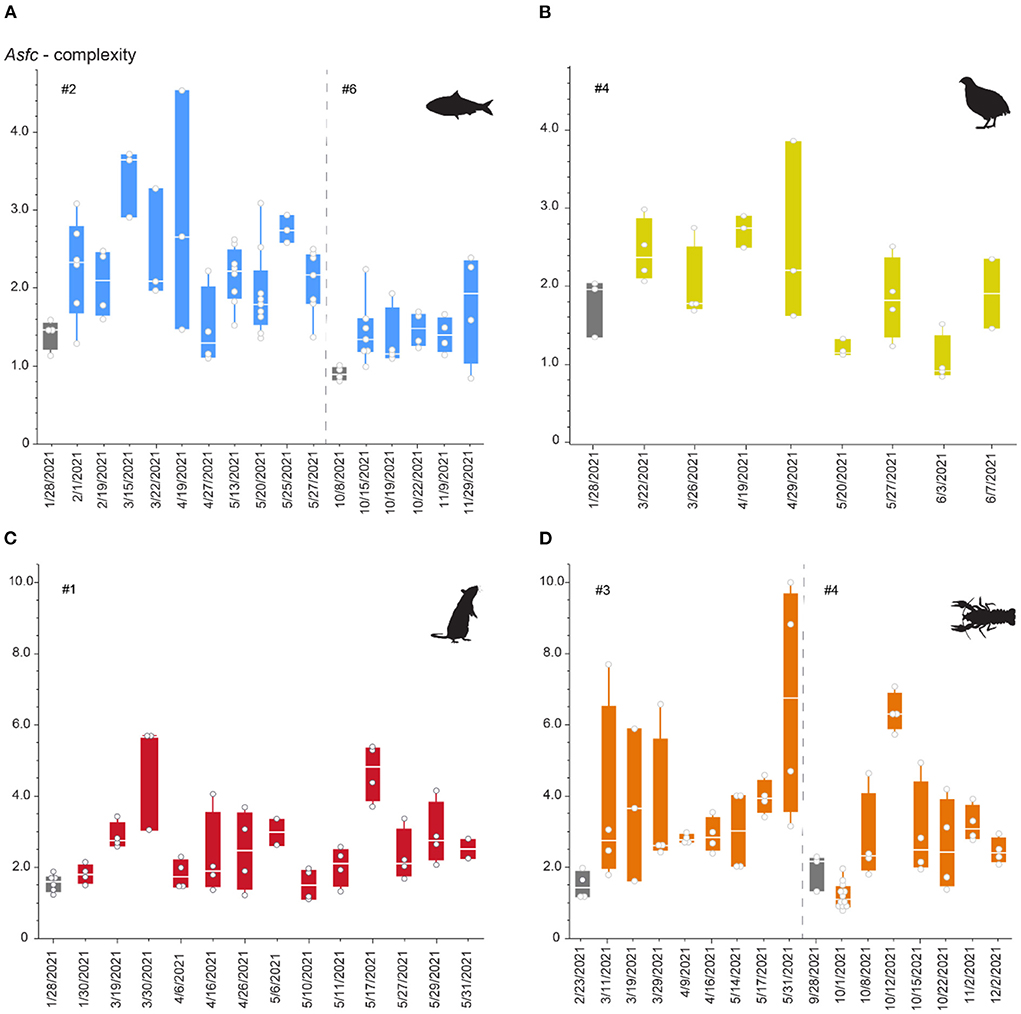
Figure 5. Asfc over the duration of the first and (for sardine and crawfish) second phase of the feeding experiment. Phases 1 and 2 are separated by a dashed line. Each datapoint represents one scan and can be derived from several teeth collected on the same date. (A) Sardine-feeder (alligators #2 and #6), (B) quail feeder (alligator #4), (C) rat-feeder (alligator #1), (D) crawfish-feeder (alligators #3 and #4). Gray boxplots mark teeth collected before switching to the experimental diet, when the alligator has only consumed pellets for at least 3 months. Note that different scales are used for pairs of sardine-/quail- and rat-/crawfish-feeding.
All diets resulted in large variability in surface complexity over the first experimental phase (Figure 5). There was no distinct trend for increase or decrease of complexity values, but an undulating pattern was observed. During the second phase, variability seems to be lower for both the sardine- and crawfish-feeding individuals. Already for the first recovered teeth at the beginning of the feeding experiment, complexity increased on all diets, except for the crawfish-feeding individual during the second phase. For several dates during the feeding experiment, single teeth showed complexity values as low as before (when still feeding on a pelleted diet). Overall, rat- and crawfish-feeding resulted in complexity values that were two times as high as recorded for sardine- or quail-feeding.
Discussion
Though they do not truly chew, oral processing in young alligators includes rapid orthal biting movements of the lower jaw (Busbey, 1989). During initial prey acquisition, positioning, and these crushing bites, we observed that teeth contacted several times with the prey (Supplementary Videos). However, these contacts are of varying frequency and intensity, depending on the tooth position. We found that on diets of similar size and weight (quails and rats), on average 16–24 bites were used before the prey was swallowed (Supplementary Figure S1, Supplementary Table S1). From seizing to swallowing, alligators used a highly variable number of bites for sardines. Alligators used more bites when being presented with one large sardine than when being presented with two smaller sardines. The total number of bites, however, does not seem to affect the observed complexity of dental microwear textures (Supplementary Figure S3). Complexity values were higher for the individuals feeding on rats than for the individuals feeding on quails (Figure 4), but quails were on average consumed with more bites, and overall lower complexity values were observed when only one large sardine was consumed with more bites than two small sardines (Supplementary Table S2). Crawfish-feeding resulted in the largest complexity values observed, and alligators also took the largest number of bites to process crawfish. However, for each feeding bout, ~7 crawfish were fed on average. Therefore, the large number of bites here is the total number of bites used to consume all crawfish.
Dietary differences in dental microwear texture analysis
The pelleted control diet resulted in variable, but overall lowest complexity values and differed significantly from all experimental vertebrate and invertebrate diets except for quails (Figure 4). This is in accordance with our expectations, as the pelleted diet was not seized or processed with the teeth, but instead swallowed whole. The low complexity observed for sardine-feeders and the high complexity observed for crawfish- feeders were also in accordance with our expectations that they would be on opposite ends of the DMT spectrum observed. Crawfish can be considered a “hard” invertebrate diet, because of their highly mineralized exoskeleton (Aiken and Waddy, 1992; Raabe et al., 2005). In mammals (including hominins, modern humans, and Neanderthals) and reptiles, high complexity has been consistently associated with hard-object feeding, whether bones, nuts, seeds, or mollusks (Scott et al., 2005, 2012; Ungar et al., 2008; DeSantis et al., 2012; Schmidt et al., 2016, 2019; Williams et al., 2019, 2021; Winkler et al., 2019a, 2022). Alligators feeding on crustaceans fit within this pattern and emphasize the universal interrelation between complex DMT and hard objects in the diet.
The difference between surface complexity observed for teeth used on rat and quail prey is striking, with quail-feeding resulting in similar complexity values as sardine-feeding, whereas rat-feeding resulted in similarly high values as crawfish-feeding (though crawfish-feeding resulted in the highest complexity values observed). We can only speculate on the source of these differences, and of course with a sample size of one animal per diet, individual variability might also play a role in our observed patterns. However, as hypothesized, bird skeletons may exhibit different levels of resistance to breaking by alligator teeth than rat skeletons. The overall thinner but denser bird bone is optimized to withstand torsional stresses during flight (Swartz et al., 1992), while making the skeletal construction lightweight (Dumont, 2010). Bird bones, especially the skull and humeri, are pneumatized (Cubo and Casinos, 2000). Rat bones, however, have thicker walls and are marrow-filled, which makes them more resistant to bending under the localized impact (Currey and Alexander, 1985). Moreover, the skull is much heavier in relation to the rest of the body than in birds, and also bears teeth (Dumont, 2010). Teeth are composed of the hardest biological material, and it is plausible that during feeding, the alligator's teeth contact not only with the rat postcranial skeleton, but also with the skull several times. The observed distinct wear marks (Figure 2) and high surface complexity (Figure 4) might result from these contacts with the rat skull, their teeth, and the overall more bending-resistant bones.
Individual variability and limitations of the study
During capture, crocodylians use only the teeth of one side of their jaws (Cleuren and DeVree, 2000; Erickson et al., 2012), before repositioning the prey with inertial bites (Cleuren and DeVree, 2000). Bestwick et al. (2021b) found that the middle–distal and distal teeth of Alligator mississippiensis and Caiman crocodilus, for example, exhibit the roughest microwear textures (indicating higher abrasion due to more tooth-to-food contacts). This may stem from alligators using preferred teeth when acquiring, manipulating, and crushing prey (Busbey, 1989). As our experimental design did not allow to control for tooth position, some teeth may show less use due to such differences. Moreover, we do not know if the shed teeth were in use for the same duration, or if some individual tooth positions were exchanged more frequently than others. Therefore, it is not surprising that our data show high intra-individual variability over the course of the experiment.
The surface structure of enamel in alligators, with distinct wrinkles that form small ridges, posed a challenge for evaluating DMTA as compared to other species with smooth enamel (Supplementary Figures S1, S2). This problem can likely occur in other crocodylian taxa, theropods, or possibly in other archosaurs with wrinkled or fluted enamel. Particularly, if teeth have newly erupted, or the diet is soft and less abrasive, the wrinkles do not wear down and present a topography that will result in high surface roughness, height, and volume. However, these large roughness, height, and volume values are not related to diet-induced wear marks, and therefore not comparable to the wear observed in mammals or non-mammalian species with smooth enamel. This problem can be overcome by choosing parameters that are less affected by the wrinkled enamel surface, and by using a strong filtering routine that eliminates the original enamel surface topography. However, such strong filters will also reduce or erase diet-induced wear features. Therefore, it is difficult to compare low abrasive diets to highly abrasive diets in very young individuals with frequent tooth replacement. In older specimens, when tooth replacement is slower, and thus teeth experience wear over a longer period, this problem seems not to occur. Bestwick et al. (2019) did not report such problems when analyzing DMTA in extant crocodylians. Due to this limitation, we can only report on two complexity parameters that seem to effectively reflect diet hardness in young alligators, as well as older individuals.
Our results confirm the expectations and encourage the idea that hard-object feeding can be detected in archosaurs, even in juveniles with frequent tooth replacement. It must be noted, however, that our sample size of one (two for crawfish and sardine) is too low to draw definitive conclusions. Individual variability and behavioral differences might influence feeding behavior, and thus dental wear. Still, the repetition of feeding two out of four experimental diets supports that the observed patterns are stable and repeatable, and in the pooled sample, we were able to analyze 8–20 teeth per diet. If these teeth would stem from different individuals, it would be an acceptable sample size. Hence, we may at least consider the DMT signature is representative, for each individual.
Captive alligator diets and behavior
We observed that alligators feeding on the four experimental diets displayed a great level of “excitement” during feeding time. They were actively searching for their food, standing on their hind legs, and jumping toward the food. Hence, including dietary items similar to their natural diets (small vertebrates and crustaceans) may be used as an enrichment for captive crocodylians and promote their natural feeding behavior.
Outlook and conclusions
This experiment highlighted both challenges and opportunities when analyzing archosaur DMT. Unworn dental enamel surfaces are wrinkled, which biases the assessment of topography and requires strong surface filtering. Tooth position-specific usage of teeth during prey processing, for example posterior teeth being utilized for crushing, is known in alligators and might result in tooth position-specific wear patterns. Isolated teeth are difficult to assign to a certain tooth position (unless they are blunt, button-like posterior teeth). It would therefore be of great interest to explore tooth position-specific DMT in greater detail in archosaurs, along the jaw, and especially comparing caniniform and molariform teeth. Obviously, a continuation of similar feeding experiments with a larger number of individuals would be desirable, but may be impractical. Therefore, even though these results need to be treated with great caution, our feeding experiments provide unique data on how different food types affect DMT in alligators and support the universal interrelation of hard-object feeding and high surface complexity. Such patterns may also support the identification of hard-object feeding in the fossil record, and thus shed light on the paleodiet of extinct faunivorous taxa, including dinosaurs.
Data availability statement
The original contributions presented in the study are included in the article/Supplementary material, and the original scan dataset (unfiltered surface scans) used in this study is published in the online repository Zenodo under the https://www.doi.org/10.5281/zenodo.6597169.
Ethics statement
The animal study was reviewed and alligator husbandry and further experimental procedures were approved by the Clemson University IACUC (protocol 2019-037).
Author contributions
DEW, TK, and MOK: conceptualization. DEW, MI, RWB, and MOK: methodology. DEW: formal analysis, writing of the original draft, and visualization. MI, RWB, MOK, and TK: resources. DEW and TK: funding acquisition. All authors: reviewing and editing. All authors contributed to the article and approved the submitted version.
Funding
The research was funded by the Japan Society for the Promotion of Science (JSPS) under a grant-in-aid for DEW, No. 20F20325 and for MI, No. 19J00701.
Acknowledgments
We thank R. Elsey (Louisiana Department of Wildlife and Fisheries, Rockefeller Wildlife Refuge) for providing alligators, M. Hart (South Carolina Department of Natural Resources), R. Flynt (Mississippi Department of Wildlife, Parks and Fisheries), C. Threadgill and T. Ancelet (Alabama Department of Conservation and Natural Resources), and J. Hawkins (Georgia Department of Natural Resources) for granting permission to transport and house alligators, Kent Vliet (University of Florida) for his helpful suggestions regarding alligator husbandry and feeding, and D. Munteanu, K. Diamond, C. Kinsey, A. Palecek, and D. Adams (Clemson University) for assisting with animal care, and Kodai Usami (The University of Tokyo) for support in the cleaning of alligator teeth. We further thank two reviewers for their helpful comments and suggestions.
Conflict of interest
The authors declare that the research was conducted in the absence of any commercial or financial relationships that could be construed as a potential conflict of interest.
Publisher's note
All claims expressed in this article are solely those of the authors and do not necessarily represent those of their affiliated organizations, or those of the publisher, the editors and the reviewers. Any product that may be evaluated in this article, or claim that may be made by its manufacturer, is not guaranteed or endorsed by the publisher.
Supplementary material
The Supplementary Material for this article can be found online at: https://www.frontiersin.org/articles/10.3389/fevo.2022.957725/full#supplementary-material
References
Ackermans, N. L., Winkler, D. E., Martin, L. F., Kaiser, T. M., Clauss, M., and Hatt, J. M. (2020). Dust and grit matter: abrasives of different size lead to opposing dental microwear textures in experimentally fed sheep (Ovis aries). J. Exp. Biol. 223, jeb220442. doi: 10.1242/jeb.220442
Barr, B. R. (1997). Food habits of the American alligator, Alligator mississippiensis, in the Southern Everglades Ph.D. Thesis. University of Miami, Coral Gables, FL, United States.
Berkovitz, B. K., and Shellis, R. P. (eds.). (2017). “Reptiles 3: crocodylia,” in The Teeth of Non-Mammalian Vertebrates (Amsterdam: Elsevier; Academic Press), 225–226.
Bestwick, J., Jones, A. S., Purnell, M. A., and Butler, R. J. (2021a). Dietary constraints of phytosaurian reptiles revealed by dental microwear textural analysis. Palaeontology 64, 119–136. doi: 10.1111/pala.12515
Bestwick, J., Unwin, D. M., Butler, R. J., and Purnell, M. A. (2020). Dietary diversity and evolution of the earliest flying vertebrates revealed by dental microwear texture analysis. Nat. Commun. 11, 1–9. doi: 10.1038/s41467-020-19022-2
Bestwick, J., Unwin, D. M., Henderson, D. M., and Purnell, M. A. (2021b). Dental microwear texture analysis along reptile tooth rows: complex variation with non-dietary variables. R. Soc. Open Sci. 8, 201754. doi: 10.1098/rsos.201754
Bestwick, J., Unwin, D. M., and Purnell, M. A. (2019). Dietary differences in archosaur and lepidosaur reptiles revealed by dental microwear textural analysis. Sci. Rep. 9, 1–11. doi: 10.1038/s41598-019-48154-9
Busbey, A. B. (1989). Form and function of the feeding apparatus of Alligator mississippiensis. J. Morphol. 202, 99–12. doi: 10.1002/jmor.1052020108
Cleuren, J., and DeVree, F. (2000). “Feeding in crocodilians,” in Feeding: Form, Function, and Evolution in Tetrapod Vertebrates, ed K. Schwenk (San Diego, CA, Academic Press), 337–358.
Cott, H. B. (1961). Scientific results of an inquiry into the ecology and economic status of the Nile Crocodile (Crocodilus niloticus) in Uganda and northern Rhodesia. Trans. Zool. Soc. Lond. 29, 211–357. doi: 10.1111/j.1096-3642.1961.tb00220.x
Cubo, J., and Casinos, A. (2000). Incidence and mechanical significance of pneumatization in the long bones of birds. Zool. J. Linn. Soc. 130, 499–510. doi: 10.1111/j.1096-3642.2000.tb02198.x
Currey, J. D., and Alexander, R. M. (1985). The thickness of the walls of tubular bones. J. Zool. 206, 453–468. doi: 10.1111/j.1469-7998.1985.tb03551.x
Delany, M. F., and Abercrombie, C. L. (1986). American alligator food habits in northcentral Florida. J. Wildl. Manag. 50, 348–353. doi: 10.2307/3801926
DeSantis, L. R., Schubert, B. W., Scott, J. R., and Ungar, P. S. (2012). Implications of diet for the extinction of saber-toothed cats and American lions. PLoS ONE 7, e52453. doi: 10.1371/journal.pone.0052453
Dumont, E. R. (2010). Bone density and the lightweight skeletons of birds. Proc. R. Soc. B Biol. Sci. 277, 2193–2198. doi: 10.1098/rspb.2010.0117
Erickson, G. M., Catanese, J. III., and Keaveny, T. M. (2002). Evolution of the biomechanical material properties of the femur. Anat. Rec. 268, 115–124. doi: 10.1002/ar.10145
Erickson, G. M., Gignac, P. M., Steppan, S. J., Lappin, A. K., Vliet, K. A., Brueggen, J. D., et al. (2012). Insights into the ecology and evolutionary success of crocodilians revealed through bite-force and tooth-pressure experimentation. PLoS ONE 7, e31781. doi: 10.1371/journal.pone.0031781
Fiorillo, A. R. (1998). Dental microwear patterns of the sauropod dinosaurs. Camarasaurus and Diplodocus: Evidence for resource partitioning in the late Jurassic of North America. Hist. Biol. 13, 1–16. doi: 10.1080/08912969809386568
Gere, K., Bodor, E. R., Makádi, L., and Osi, A. (2021). Complex food preference analysis of the Late Cretaceous (Santonian) lizards from Iharkút (Bakony Mountains, Hungary). Hist. Biol. 33, 3686–3702. doi: 10.1080/08912963.2021.1887862
Grigg, G. C., and Kirshner, D. (2015). Biology and Evolution of Crocodylians. Clayton South, VIC, Australia: CSIRO Publishing, 672.
Holwerda, F. M., Beatty, B. L., and Schulp, A. S. (2013). Dental macro-and microwear in Carinodens belgicus, a small mosasaur from the type Maastrichtian. Neth. J. Geosci. 92, 267–274. doi: 10.1017/S0016774600000202
Horton, J. M., and Summers, A. P. (2009). The material properties of acellular bone in a teleost fish. J. Exp. Biol. 212, 1413–1420. doi: 10.1242/jeb.020636
Hutton, J. M. (1987). Growth and feeding ecology of the Nile crocodile Crocodylus niloticus at Ngezi, Zimbabwe. J. Anim. Ecol. 56, 25–38. doi: 10.2307/4797
Iijima, M., Munteanu, V. D., Elsey, R. M., and Blob, R. W. (2021). Ontogenetic changes in limb posture, kinematics, forces and joint moments in American alligators (Alligator mississippiensis). J. Exp. Biol. 224, jeb242990. doi: 10.1242/jeb.242990
Kubo, M. O., and Fujita, M. (2021). Diets of Pleistocene insular dwarf deer revealed by dental microwear texture analysis. Palaeogeogr. Palaeoclimatol. Palaeoecol. 562, 110098. doi: 10.1016/j.palaeo.2020.110098
Kvam, T. (1959). The Teeth of Alligator mississippiensis Daud.: V. Morphol. Enamel. Acta Odontol. Scand. 17, 45–59. doi: 10.3109/00016355909011232
Mallon, J. C., and Anderson, J. S. (2014). The functional and palaeoecological implications of tooth morphology and wear for the megaherbivorous dinosaurs from the Dinosaur Park Formation (Upper Campanian) of Alberta, Canada. PLoS ONE 9, e98605. doi: 10.1371/journal.pone.0098605
Merceron, G., Ramdarshan, A., Blondel, C., Boisserie, J.-R., Brunetiere, N., Francisco, A., et al. (2016). Untangling the environmental from the dietary: dust does not matter. Proc. R. Soc. B 283, 20161032. doi: 10.1098/rspb.2016.1032
Platt, S. G., Rainwater, T. R., Finger, A. G., Thorbjarnarson, J. B., Anderson, T. A., and McMurry, S. T. (2006). Food habits, ontogenetic dietary partitioning and observations of foraging behaviour of Morelet's crocodile (Crocodylus moreletii) in northern Belize. Herpetol. J. 16, 281–290.
Platt, S. G., Thorbjarnarson, J. B., Rainwater, T. R., and Martin, D. R. (2013). Diet of the American crocodile (Crocodylus acutus) in marine environments of coastal Belize. J. Herpetol. 47, 1–10. doi: 10.1670/12-077
Pooley, A. C. (1989). “Food and feeding habits,” in Crocodiles and Alligators, eds Ross, C. A., New York: Facts on File. 76–91.
Prange, H. D., Anderson, J. F., and Rahn, H. (1979). Scaling of skeletal mass to body mass in birds and mammals. Am. Nat. 113, 103–122. doi: 10.1086/283367
Purnell, M., Seehausen, O., and Galis, F. (2012). Quantitative three-dimensional microtextural analyses of tooth wear as a tool for dietary discrimination in fishes. J. R. Soc. Interface 9, 2225–2233. doi: 10.1098/rsif.2012.0140
Purnell, M. A., and Darras, L. P. (2015). 3D tooth microwear texture analysis in fishes as a test of dietary hypotheses of durophagy. Surf. Topogr.: Metrol. Prop. 4, 014006. doi: 10.1088/2051-672X/4/1/014006
Raabe, D., Sachs, C., and Romano, P. J. A. M. (2005). The crustacean exoskeleton as an example of a structurally and mechanically graded biological nanocomposite material. Acta Mater. 53, 4281–4292. doi: 10.1016/j.actamat.2005.05.027
Sakaki, H., Winkler, D. E., Kubo, T., Hirayama, R., Uno, H., Miyata, S., et al. (2022). Non-occlusal dental microwear texture analysis of a titanosauriform sauropod dinosaur from the Upper Cretaceous (Turonian) Tamagawa Formation, northeastern Japan. Cretac. Res. 136, 105218. doi: 10.1016/j.cretres.2022.105218
Sander, P. M. (1999). The Microstructure of Reptilian Tooth Enamel: Terminology, Function, and Phylogeny. Mnchner Geowissenschaftliche Abhandlungen Reihe A: Geologie und Paläontologie Band 38. Friedrich Pfeil Verlag.
Schmidt, C. W., Beach, J. J., McKinley, J. I., and Eng, J. T. (2016). Distinguishing dietary indicators of pastoralists and agriculturalists via dental microwear texture analysis. Surf. Topogr.: Metrol. Prop. 4, 014008. doi: 10.1088/2051-672X/4/1/014008
Schmidt, C. W., Remy, A., Van Sessen, R., Willman, J., Krueger, K., Scott, R., et al. (2019). Dental microwear texture analysis of Homo sapiens sapiens: foragers, farmers, and pastoralists. Am. J. Phys. Anthropol. 169, 207–226. doi: 10.1002/ajpa.23815
Schubert, B. W., and Ungar, P. S. (2005). Wear facets and enamel spalling in tyrannosaurid dinosaurs. Acta Palaeontol. Pol. 50, 93–99. Available online at: http://app.pan.pl/acta50/app50-093.pdf
Schulz, E., Piotrowski, V., Clauss, M., Mau, M., Merceron, G., and Kaiser, T. M. (2013). Dietary abrasiveness is associated with variability of microwear and dental surface texture in rabbits. PLoS ONE 8, e56167. doi: 10.1371/journal.pone.0056167
Schulz-Kornas, E., Winkler, D. E., Clauss, M., Carlsson, J., Ackermans, N. L., Martin, L. F., et al. (2020). Everything matters: molar microwear texture in goats (Capra aegagrus hircus) fed diets of different abrasiveness. Palaeogeogr. Palaeoclimatol. Palaeoecol. 552, 109783. doi: 10.1016/j.palaeo.2020.109783
Scott, R., Ungar, P., Bergstrom, T., et al. (2005). Dental microwear texture analysis shows within-species diet variability in fossil hominins. Nature 436, 693–695. doi: 10.1038/nature03822
Scott, R. S., Teaford, M. F., and Ungar, P. S. (2012). Dental microwear texture and anthropoid diets. Am. J. Phys. Anthropol. 147, 551–579. doi: 10.1002/ajpa.22007
Scott, R. S., Ungar, P. S., Bergstrom, T. S., Brown, C. A., Childs, B. E., Teaford, M. F., et al. (2006). Dental microwear texture analysis: technical considerations. J. Hum. Evol. 51, 339–349. doi: 10.1016/j.jhevol.2006.04.006
Stevenson, C., and Whitaker, R. (2010). “Indian gharial gavialis gangeticus,” in Crocodiles Status Survey and Conservation Action Plan. Third Edn, eds S. C. Manolis and C. Stevenson (Darwin, NT: Crocodile Specialist Group), 139–143.
Swartz, S. M., Bennett, M. B., and Carrier, D. R. (1992). Wing bone stresses in free flying bats and the evolution of skeletal design for flight. Nature 359, 726–729. doi: 10.1038/359726a0
Taylor, D. (1986). Fall foods of adult alligators from Cypress Lake habitat, Louisiana. Proc. Annu. Conf. Southeast Fish and Wildl. Agencies 40, 338–341.
Taylor, J. A. (1979). The foods and feeding habits of subadult Crocodylus porosus Schneider in northern Australia. Wildl. Res. 6, 347–359. doi: 10.1071/WR9790347
Thorbjarnarson, B. (1990). Notes on the feeding behavior of the gharial (Gavialis gangeticus) under semi-natural conditions. J. Herpetol. 24, 99–100. doi: 10.2307/1564301
Ungar, P. S., Grine, F. E., and Teaford, M. F. (2008). Dental microwear and diet of the Plio-Pleistocene hominin Paranthropus boisei. PLoS ONE 3, e2044. doi: 10.1371/annotation/195120f0-18ee-4730-9bd6-0d6effd68fcf
Varriale, F. J. (2016). Dental microwear reveals mammal-like chewing in the neoceratopsian dinosaur Leptoceratops gracilis. PeerJ 4, e2132. doi: 10.7717/peerj.2132
Virag, A., and Osi, A. (2017). Morphometry, microstructure, and wear pattern of neornithischian dinosaur teeth from the upper cretaceous iharkut locality (Hungary). Anat. Rec. Adv. Integr. Anat. Evol. Biol. 300, 1439–1463. doi: 10.1002/ar.23592
Wallace, K. M., and Leslie, A. J. (2008). Diet of the Nile crocodile (Crocodylus niloticus) in the Okavango Delta, Botswana. J. Herpetol. 42, 361–368. doi: 10.1670/07-1071.1
Williams, F. L., Schmidt, C. W., Droke, J., Willman, J. C., Semal, P., Becam, G., et al. (2019). Dietary reconstruction of Spy I using dental microwear texture analysis. C. R. Palevol 18, 1083–1094. doi: 10.1016/j.crpv.2019.06.004
Williams, F. L., Schmidt, C. W., Droke, J. L., Willman, J. C., Neruda, P., Becam, G., et al. (2021). Reconstructing the diet of Kulna 1 from the Moravian karst (Czech Republic). J. Paleolit. Archaeol. 4, 21. doi: 10.1007/s41982-021-00099-0
Williams, V. S., Barrett, P. M., and Purnell, M. A. (2009). Quantitative analysis of dental microwear in hadrosaurid dinosaurs, and the implications for hypotheses of jaw mechanics and feeding. Proc. Nat. Acad. Sci. 106, 11194–11199. doi: 10.1073/pnas.0812631106
Winkler, D. E., Clauss, M., Kubo, M. O., Schulz-Kornas, E., Kaiser, T. M., Tschudin, A., et al. (2022). Microwear textures associated with experimental near-natural diets suggest that seeds and hard insect body-parts cause high enamel surface complexity in small mammals. Front. Ecol. Evol. 10, 957427. doi: 10.3389/fevo.2022.957427
Winkler, D. E., Clauss, M., Rölle, M., Schulz-Kornas, E., Codron, D., Kaiser, T. M., et al. (2021). Dental microwear texture gradients in guinea pigs reveal that material properties of the diet affect chewing behaviour. J. Exp. Biol. 224, jeb242446. doi: 10.1242/jeb.242446
Winkler, D. E., and Kubo, M. O. (2022). Inter-microscope comparability of dental microwear texture data obtained from obtained from different optical profilometers. bioRxiv [Preprint]. doi: 10.1101/2022.03.08.483539
Winkler, D. E., Schulz-Kornas, E., Kaiser, T. M., Codron, D., Leichliter, J., Hummel, J., et al. (2020b). The turnover of dental microwear texture: testing the “last supper” effect in small mammals in a controlled feeding experiment. Palaeogeogr. Palaeoclimatol. Palaeoecol. 557, 109930. doi: 10.1016/j.palaeo.2020.109930
Winkler, D. E., Schulz-Kornas, E., Kaiser, T. M., De Cuyper, A., Clauss, M., and Tütken, T. (2019b). Forage silica and water content control dental surface texture in guinea pigs and provide implications for dietary reconstruction. Proc. Nat. Acad. Sci. 116, 1325–1330. doi: 10.1073/pnas.1814081116
Winkler, D. E., Schulz-Kornas, E., Kaiser, T. M., and Tütken, T. (2019a). Dental microwear texture reflects dietary tendencies in extant Lepidosauria despite their limited use of oral food processing. Proc. R. Soc. B 286, 20190544. doi: 10.1098/rspb.2019.0544
Winkler, D. E., Tütken, T., Schulz-Kornas, E., Kaiser, T. M., Müller, J., Leichliter, J., et al. (2020a). Shape, size, and quantity of ingested external abrasives influence dental microwear texture formation in guinea pigs. Proc. Nat. Acad. Sci. 117, 22264–22273. doi: 10.1073/pnas.2008149117
Keywords: microwear, DMTA, diet reconstruction, crocodylia, dental wear, hard-object feeding
Citation: Winkler DE, Iijima M, Blob RW, Kubo T and Kubo MO (2022) Controlled feeding experiments with juvenile alligators reveal microscopic dental wear texture patterns associated with hard-object feeding. Front. Ecol. Evol. 10:957725. doi: 10.3389/fevo.2022.957725
Received: 31 May 2022; Accepted: 20 September 2022;
Published: 27 October 2022.
Edited by:
Larisa R. G. DeSantis, Vanderbilt University, United StatesReviewed by:
Frank Williams, Georgia State University, United StatesBrian Lee Beatty, New York Institute of Technology, United States
Copyright © 2022 Winkler, Iijima, Blob, Kubo and Kubo. This is an open-access article distributed under the terms of the Creative Commons Attribution License (CC BY). The use, distribution or reproduction in other forums is permitted, provided the original author(s) and the copyright owner(s) are credited and that the original publication in this journal is cited, in accordance with accepted academic practice. No use, distribution or reproduction is permitted which does not comply with these terms.
*Correspondence: Daniela E. Winkler, daniela.eileen.winkler@k.u-tokyo.ac.jp
†ORCID: Daniela E. Winkler orcid.org/0000-0001-7501-2506
Masaya Iijima orcid.org/0000-0003-2701-0391
Richard W. Blob orcid.org/0000-0001-5026-343X
Tai Kubo orcid.org/0000-0003-3207-0399
Mugino O. Kubo orcid.org/0000-0002-7748-7377