- 1Department of Geography, University of Oviedo, Oviedo, Spain
- 2Regional Agrifood Research and Development Service (SERIDA), Government of the Principality of Asturias, Villaviciosa, Spain
Agrosilvopastoral systems are multifunctional, complex, and knowledge-intensive systems with the potential to deliver multiple ecosystem services. However, their future is hindered by socio-ecological factors which influence the dynamics of the associated landscapes by modifying how the natural resources are used over time and across the territory. An integrated analysis of the most influential factors and the associated dynamics urges due to the strategic potential of these systems to provide locally adapted ecosystem services to face both local and global challenges. We investigated the changes in the demography, productive activities and the strategies of land use, and how they influenced the landscape dynamics, in the Redes Biosphere Reserve. Datasets with demographic, socioeconomic, and landscape parameters from 1956, 1985, and 2016 were created. Landscape metrics were calculated for the whole Reserve and for six areas covering the bioclimatic variability. Historical data, interviews with the local population, and repeated images were used to reconstruct the land uses and the landscape maps in each period. The number of inhabitants declined 60% from 1956 to 2016, and only 4.7% are less than 15 years old nowadays. The human group’s capacity to maintain sustainable and productive activities is highly questionable. During the same period, the number of farms decreased by 80% and the herds evolved from multispecific (63% cattle and 37% small ruminants) to monospecific ones (around 88% cattle). The complex land management strategies simplified: subsistence agriculture almost disappeared and the diversity of livestock and agroforestry strategies also decreased. The landscape metrics revealed the most drastic changes in the lowlands associated with the construction of two water reservoirs and the disappearance of croplands and open chestnut forests. At middle altitudes, around 43% of the hay meadows disappeared and the remaining grasslands are only grazed, whereas grazed grasslands declined by 77% at the highest altitudes. Two drivers repeated across the three bioclimatic scenarios: expansion of dense woodlands (average 220%) and shrublands (295%). All these changes led to the reduction of the landscape diversity and the simplification of the mosaic, with possible environmental implications. Integrated approaches including environmental and socioeconomic measures are needed to preserve the landscapes and associated services.
Introduction
Agroforestry is a very ancient traditional practice that is still widely implemented across Europe. Agroforestry systems are multifunctional and usually more complex and knowledge-intensive than conventional agriculture, especially the agrosilvopastoral ones, as they involve a combination of animals, trees, and crops, together with the human population as an integral part of them (Nair et al., 2021). These systems, often found in mountain areas, are recognized for their biodiversity and cultural importance and they are classified as high nature and cultural value (HNCV) agroforestry (Moreno et al., 2018) and/or high nature value (HNV) farming systems (O’Rourke et al., 2016). They frequently occur in socio-cultural and/or environmentally sensitive territories like the Biosphere Reserves (BRs), emblematic areas aimed at confronting a major challenge of integrating nature conservation with sustainable social, cultural, and economic development through locally adapted solutions for global challenges.
The agrosilvopastoral systems result from the interaction of the socio-economic contexts (products, financial resources, demography, etc.), the biological components (flora and fauna), and the cultural ones (including how the natural resources are used). They are also dynamic in the way their components are arranged in space and time and how the functional relationships between them evolve (Chará et al., 2019).
The diverse composition of land uses and resources of these systems could potentially favor the diversification of products and services and lower the impacts of stand-alone activities on the environment (Moreno et al., 2018). However, they often run afoul of ongoing intensification/abandonment and simplification in agricultural production that reduces their economic and ecological resilience (Plieninger and Huntsinger, 2018).
A fundamental transformation of the existing production systems urges to confront major challenges like climate change, food security, biodiversity conservation, rural development, etc., and it requires a revision of the locally adapted strategies and the social and environmental contexts linked to them. Therefore, testing and evaluating the potential of the EU agrosilvopastoral systems to face those challenges is timely, especially within sensitive areas like the BRs.
The multi-functionality of these systems is often limited by the knowledge gaps about their historical demographic evolution, the assessment of the main factors influencing the land-use and land cover changes, and the direct and indirect implications of those changes on the dynamics of the landscape patterns (Huber et al., 2020). Previous studies monitoring land use and land cover change tended to focus either on conservation or productive perspectives and they missed a comprehensive and integrative perspective on the complex interactions between the characteristics of the human communities, their activities and strategies of land management, and the ecological processes, including diverse aspects, from urban settlement and agricultural intensification to land abandonment and reforestation or desertification (Gallant et al., 2004).
We characterized the current agrosilvopastoral systems in the Redes Biosphere Reserve and investigated their evolution, taking into consideration the changes in the demography, the productive activities, and the strategies of land management. For each factor, we identified the main drivers of the changes and how they influence the landscape dynamics. The study took into account the variability along time (by considering meaningful historical periods) and across the space, by analyzing the Redes BR as a whole as well as within specific bioclimatic areas. Such a study will contribute to improving the understanding of the interlinkages between socioeconomic developments and the multifunctionality of mountain landscapes hosting agrosilvopastoral systems.
Materials and methods
Study area
The case study was conducted in Redes Biosphere Reserve (Figure 1), a marginal territory that is classified as a Natural Park too and holds representative examples of the EU’s traditional agrarian landscapes and their environmental and socioeconomic dynamics. The traditional agrarian systems in the Atlantic mountains were characterized by their autarchic and multifunctional nature, as well as their extreme dependence on natural resources, which were used on a communal basis by the local communities (Ortega Valcárcel, 2004; Fernández García, 2005).
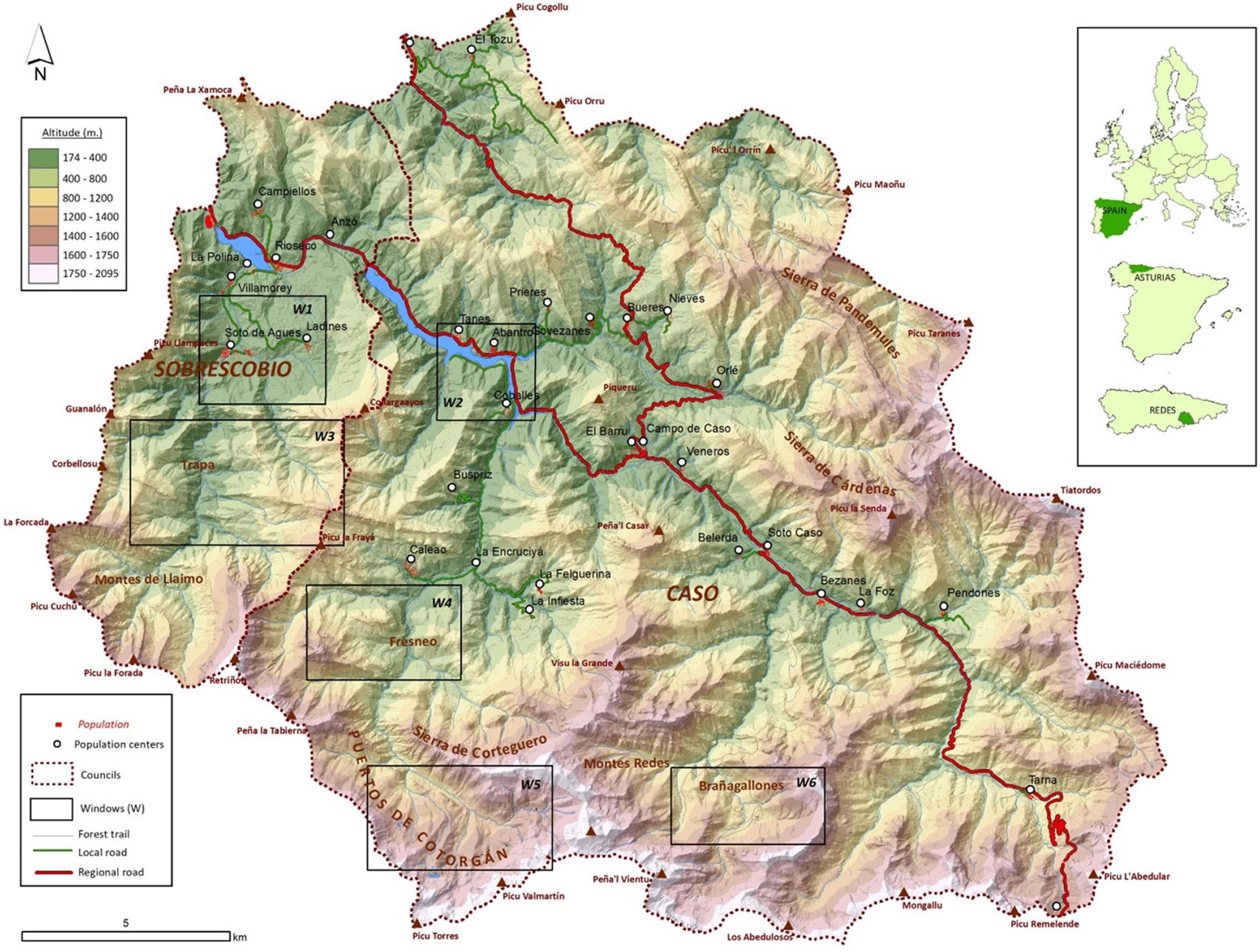
Figure 1. Location of Redes Biosphere Reserve in Asturias, northern Spain. The map of Redes includes the landscape windows (W1–W6) where detailed analyses of the landscape were performed.
The area of Redes BR has received little attention from the scientific community although it can be a Living Lab to explore the sustainability of locally adapted strategies to address both local and major challenges. Redes BR covers 376 km2 in two municipalities (Caso and Sobrescobio) of the province of Asturias in northwest Spain (Vázquez and Díaz, 2006).
The altitudes range between 350 and 2104 m a.s.l. (75% of the surface occurs above 800 m a.s.l) and steep slopes dominate; only 12% of the territory has less than 20° (Table 1). The climate is Atlantic, the average annual temperature is 10.3°C, the total annual precipitation is 1520.5 mm, there are at least 130 days of rainfall and a frost-free period of 5 months per year (averaged data for the period 1970–2000 from Bezanes meteorological station located at 650 m a.s.l.).

Table 1. Summary of the basic ecological characteristics of Redes Biosphere Reserve and the six landscape windows.
The study area extends along three bioclimatic belts: coline (350–900 m a.s.l), montane (900–1700 m a.s.l), and subalpine (1700–2100 m a.s.l) (Table 1). A total of 14 vegetation series are present within two phytogeographic provinces, the Cantabro-Atlantic (restricted to low altitudes) and the Orocantabric (in the rest of Redes).
Numerous protected flora and fauna species inhabit the mature forests (38.8% of the total surface), shrublands (40%), grasslands (12.1%), and pastures (3.2%) (Vázquez and Díaz, 2006).
The human presence in Redes goes back to the Late Bronze Age (de Blas Cortina, 1983). Nowadays (data from SADEI for the year 2021) there are 2,271 inhabitants distributed in 33 population entities with less than 350 inhabitants each. The current low population density (less than 6.5 inhabitants/km2) is common in other European mountain regions and explains its inclusion in the Southern Sparsely Populated Areas Network (Carbone, 2018).
Several bioclimatic belts are present in Redes (Table 1) and they influence the location of the natural resources, land uses, and productive activities.
To track the changes in the socioeconomy and the landscape along time, we selected 3 distinct periods which cover three models of land management:
- Up to 1960: traditional or pre-industrial period due to the late contemporary industrialization of Spain (Lasanta, 2002).
- 1960–1986: Rural transition, an intermediate period between the pre-industrial, agrarian-based rural societies and the modern urban societies arising from the contemporary capitalist industrialization (Ortega Valcárcel, 2004).
- 1986–2016: Current model emerged once Spain entered the EU in 1986 (Corbera Millán et al., 1999).
The analysis for each period was based on the information gathered from multiple data sources that we describe in the next subsections of this document.
Analysis of the changes in population characteristics
To understand the evolution of the agrosilvopastoral systems and the associated landscape mosaics, it is necessary to explore the demographic and socioeconomic evolution of the activities that supported them. The studies of the evolution of the population and its demographic structure are based on the analysis of the data available from 1900 to 2016 from the official Population Censuses and Population Entity Gazetteers for the whole Redes as well for the different entities. The analysis of the changes in population censuses relied on the calculations of the relative changes for the whole data series.
The current demographic structure (sex and age) was evaluated through the following indicators (Lasanta et al., 2014):
Femininity Index (FI) = Female Population/Male Population) × 100. It compares the percentage of women and men. The 100 value indicates an equal number of both sexes.
Ageing Index (AI) = (Population > 65 years/Population < 15 years) × 100. Populations over 65 years old compared with those less than 15 years old. Values above 50% reveal the population is aging.
Dependency Index (DI) = [(Population < 16 years + Population > 64 years)/Population 16 to 64 years] × 100. The ratio between the non-working age population and the working age population (15–64 years old in Spain). Data above 50% reveals that dependency is present.
Replacement Index (RI) = (Population aged 15–19 years/Population aged 60–64 years) × 100. It relates the younger active population to the older one, and it can be used to track if the current jobs of the older population can be replaced once they retire. The possibility to fill those jobs with the new generations will increase as the index shows values above 100.
Analysis of the changes in productive activities and management strategies
The parameters which have been selected to analyze the changes in livestock production were the evolution of the number of farms and the evolution of the composition of the livestock herds (number of heads of each species). The information was collected from local, regional and national historical databases (Supplementary Table 1): Catastro del Marques de Ensenada (1756), Relaciones Ganaderas Municipales (1950), Campañas de Saneamiento Ganadero (1986) and regional official databases from the Sociedad Asturiana de Estudios Económicos e Industriales (SADEI). For the livestock species, data collection focused on cattle, horses, goats, and sheep, the species regularly included in documents and/or databases.
To study the evolution of the agriculture two indicators were selected: the cultivated area and the types of crops in each period (depending on data availability). The information was obtained from historical sources and official agricultural statistics (Supplementary Table 1): Catastro Marqués de Ensenada (1861), Resúmenes de riqueza rústica y pecuaria (1962), Catastro Rústica (1982), Censo Agrario and Cuadernos de superficies ocupadas por los cultivos agrícolas (1984). From 1990 onward, two regional data sources were used: Superficies y producciones agrícolas and Encuestas anuales de superficies y rendimientos de cultivos.
Regarding forestry activities, the data availability for the pre-industrial period is limited and inconsistent for the three study periods. The only official sources with some continuity are the Planes de Aprovechamiento de los Montes de Utilidad Pública available from the Biblioteca Histórica Virtual (Supplementary Table 1). They include information for the communal lands (which cover 82% of Redes) since 1900. From 2000 onward, the Estadísticas de las Cortas de Maderas (Supplementary Table 1) provided by SADEI completed the data sources for timber and dead firewood harvest.
The information about agroecological practices (mowing, silage production, fertilization, irrigation.) and management strategies (e.g., livestock movements) was linked to different periods and locations in Redes. The collection of traditional ecological knowledge (Berkes, 1993) was essential to accomplish this goal. The data sources were: (a) informal interviews (80). (b) study of 46 local ordinances which are normative codes, elaborated and approved by the local communities.
The surveys focused on the local elderly population with personal experience during the three periods and gathered the following information in each case: age, gender, education (primary, secondary, university), place of residence, profession (main activity and secondary ones), type of activities (agriculture, livestock, forestry, industry, hunting, others), the Spatio-temporal context for each activity (where and when they were carried out), characteristics of the natural resources linked to each activity (plant and animal species/communities), agroecological strategies for the use of the natural resources (techniques and resources used for each activity in each area and period).
The ordinances provided additional valuable information as they specified the agrosilvopastoral uses and the productions throughout the annual cycle and for specific areas in Redes. These regulations persisted until the second half of the 20th century in the mountain areas of northern Spain (Fernández García, 2005).
The study of the most recent activities and land uses focused on the evolution of the surface of the flooded surfaces linked to water storage and the evolution of the number of hotel beds as an indicator of the evolution of rural tourism. The information was obtained from the databases of the regional Government of the Principality of Asturias and the rural development group (Grupo de Desarrollo Rural Comarca del Alto Nalón, Supplementary Table 1).
Analysis of the changes in the landscape
The study of the changes in the landscape was carried out at two spatial scales:
(1) The whole Redes Biosphere Reserve through the study of the Mapa de cultivos y aprovechamientos (MCA). These maps of crops and land uses are available at a scale of 1:50000 and in digital format for the years 1975 (based on photo interpretation of aerial images from 1956) and 2010. The MCA is considered a valuable source to study the evolution of the Spanish rural landscapes, despite certain limitations associated with the scale or the absolute predominance of the land uses (Fernández García, 2010). The photo-interpretation analyses and the transitions from the paper cartography to the digital format (for the 1st edition, 1975) were reviewed to generate an updated and improved data set after correcting the detected discrepancies between the two data sources. The original eight classes of the MCA were reclassified (see Supplementary Table 2) into the new eight classes complementary to the studies at the next spatial scale.
(2) Detailed studies in landscape windows. The results for the whole study area with the MCA were complemented with detailed analyses in three areas of Redes with different bioclimatic conditions and distribution of the land covers and land uses. Two subareas (landscape windows) representative of the diverse character of the landscape (Burel and Baudry, 1999; Lasanta et al., 2015; Table 1) and the socioeconomic and environmental scenarios were selected in each area. The selection of each window took into account that they included representative environmental and sociocultural characteristics present in each bioclimatic belt to allow a further detailed study of the drivers and trends within each area and for the whole reserve.
To analyze the changes in the landscape in each window, aerial images from three different periods were used: the ortho aerial images of the American Flight in 1956 (1:33000) for the traditional period, images of the Interministerial flight (1:18000) in 1986 for the rural transition and digital orthophotography (1:5000) from 2016 for the modern period. A pre-treatment of the historical aerial photographs was performed to compare them with the modern ones from the same area (Colwell, 1966; Gerard et al., 2010). The images from 1956 and 1986 were scanned at 600 dpi, orthorectified using Agisoft Photo Scan and ArcGIS10.1, and projected into a common UTM (Universal Transverse Mercator) grid. The orthorectification of the series was co-registered across years with an RMSE smaller than 2 m. The photo interpretation was based on the images from the three periods, and it was carried out by digitizing the patches with the module ArcMap 10.1 to a scale of 1:1000, attending to the new classes defined for the MCA (Supplementary Table 2). The woodland class was subdivided into two subclasses according to its internal structural characteristics: dense woodland (when the distribution of the vegetation within the patches was homogeneous) and sparse woodland (when trees are more scattered and associated with grasslands and/or shrublands). The photo interpretation was supported by additional fieldwork, interviews with the local community, and repeated terrestrial photographs (Carré and Metailié, 2008) for each period.
The databases associated with the land cover maps were used with the program V-LATE (Lang and Tiede, 2003) to calculate the set of relevant landscape metrics for each area (whole Redes or each landscape window) and period (1956 and 2010 for the MCA and 1956, 1986, and 2016 for the windows). The metrics allow quantifying complex spatial processes monitoring the landscape changes (Midha and Mathur, 2010), and comparing different landscapes. The following metrics were used: (a) Landscape-level: Number of Classes (NC), Number of Patches (NP), Class Area (CA), and Mean Patch Size (MPS). (b) Class level: Number of Patches (NP) and Mean Patch Size (MPS). The rate of change was calculated as: [(current value/previous value)-1]*100. One rate of change (1956–2010) was calculated for the MCA and two for the landscape windows: P1 (comparing 1956 and 1986) and P2 (comparing 1986 and 2016). The analysis of the changes of these metrics for those different periods can help to detect dynamic processes, such as landscape fragmentation (resulting from the emergence of new patches and/or the division of the classes into increasingly smaller and less connected patches), or landscape homogenization (resulting from the disappearance of certain classes and the appearance and/or growth of the patches of other ones).
Data collection about population and productive activities was gathered for the same periods to explore the implications for the landscape dynamics.
Results
Changes in demography
To understand the evolution of the agrosilvopastoral systems and the associated landscape mosaics, it is necessary to explore the demographic and socioeconomic evolution of the activities that supported them. Throughout the last century, the population census in Redes BR decreased by 70% (Figure 2A); it peaked in 1920 (7789 inhabitants) and dropped progressively to the minimum in 2016 (2425 inhabitants). However, during the same period, the census of the whole province (Asturias) peaked in 1981 and the population decreased by only 8% as a result of the migration to the central area to work as miners and industry activities.
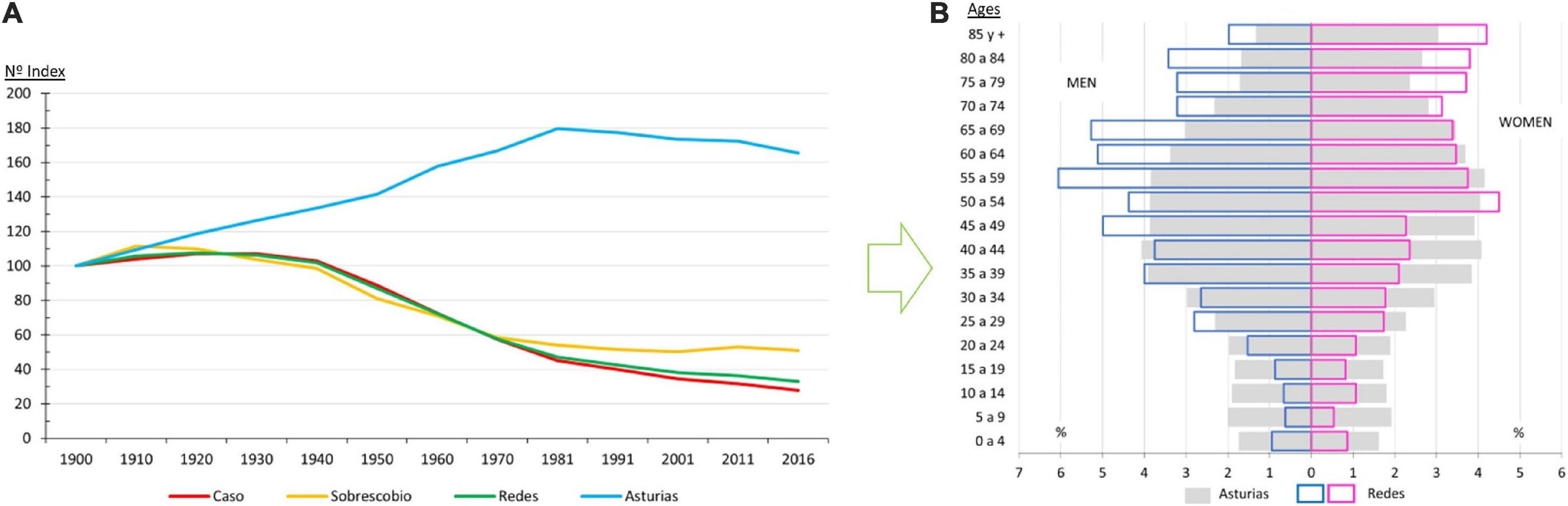
Figure 2. Evolution of the population between 1900 and 2016 in Redes Biosphere Reserve (A) and population pyramid for men and women for the year 2016. Sources: (A) Nomenclátores y Censos de Población (1900–2016). (B) Padrón municipal de habitantes (2016). See Supplementary Table 1 for additional information.
Three distinct demographic periods were identified in Redes. From 1920 to 1960, the population declined by 30% as a result of the emigration to America and the previous Civil War. Between 1960 and 1981, the rural exodus to regional, national and European urban-industrial areas was associated with the reduction of 25% of the population in Redes. Between 1981 and 2016, the demographic curve is flatter (Figure 2A), although the population declined another 14% as a result of negative natural growth, i.e., the number of deaths was higher than the number of births.
Simultaneously, the demographic pressure relaxed notably (–70% inhabitants/km2) between 1920 and 2016: from 20.7 inhabitants/km2 to 14, 9, and finally 6.4 inhabitants/km2 in 1920, 1960, 1981, and 2016, respectively. According to the European Commission Guidelines on Regional State Aid for 2014-2020 (2013/C 209/01), sparsely populated areas are defined as NUTS 2 regions with fewer than 8 inhabitants per km2 and NUTS 3 regions with fewer than 12.5 inhabitants per km2. According to these values, Redes can be considered a sparsely populated area or a demographic desert (less than 10 inhabitants/km2) nowadays.
The current inverted population pyramid (Figure 2B) shows the demographic situation in Redes: there are fewer young inhabitants (in the base) than aged adults (dominate toward the top). This pyramid is typical of marginal territories with a deep aging population and it is partially explained by the selective migration of young people and women, so older resident groups became dominant. The demographic indicators are coherent with these trends and offer additional information: the feminity index (124.5%) specifies the degree of sex imbalance due to the absence of women, whereas the high aging index (752%) and the high dependency index (67%) show the relevance of the elderly group and the implications of their dominance in terms of their increased dependency. Finally, the low replacement rate in the economic activities (19.8% for the replacement index) highlights the limitations of the population to carry on both current and future production activities.
Changes in livestock production
The multifunctional traditional agrosilvopastoral systems in Redes included diverse productive activities. The interviews with the local population, the fieldwork, the study of the maps, and the historical documents allowed the reconstruction of the activities in different areas and their evolution.
Livestock production has been the main economic activity since historical times, followed by agriculture and forestry as complementary activities (López Fernández et al., 2006). However, animal production has gone through profound changes, both in the number and characteristics of the households and in the census and characteristics of the livestock species (Table 2).
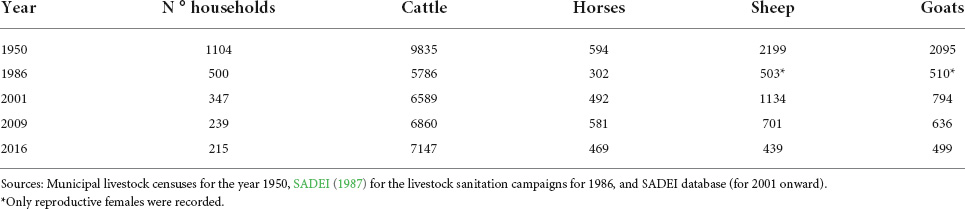
Table 2. Evolution of the number of households and number of heads of the main livestock species in Redes Biosphere Reserve.
Between 1950 and 2016, around 80% of the farms disappeared (Table 2), especially between 1950-1986 (54% of the farms shut down), while another 26% were lost between 1986 and 2016. The density of livestock farms (n°farms/km2) evolved from 2.9/km2 in 1950 to 0.6 farms/km2 in 2016. Meanwhile, the number of heads per farm also changed. In the case of cattle (the most common species), the farms grew from 8.9 heads/farm to 33.2 heads/farm, i.e., they multiplied by 3.6 their size. Meanwhile, the local workforce declined as revealed by the demographic analysis.
The disappearance of farms concurred with a decline in the total number of livestock heads (–41%, Table 2), especially of small ruminants (sheep –80% and goats –76%) compared to horses and cattle (–21 and –27% respectively).
The changes in the number of livestock species run in parallel with the simplification of the herds (lower variety of livestock species on the same farm) and a productive specialization (Table 2). The livestock censuses for 1950 (illustrative of the pre-industrial scenario) show that the mixed herds were composed of cattle (66.8%) and small ruminants (14.9% sheep and 14.2% goats), while horses were minority labor animals (4%). The interviews revealed that the herds were multifunctional (meat and milk production as well as animal traction). The native breeds dominated (Asturiana de la Mountaña cow, Asturcón horse, Xalda sheep, and Bermeya goat) and they fed on varied natural resources through complex and precise (in time and space) transhumant livestock movements.
After the rural transition, the data for 1986 shows drastic changes in the number and characteristics of the cattle. The attempts at dairy specialization led to the dominance of cattle (81% of all the livestock heads in Redes BR), while small ruminants decreased by 15% (–7.1% sheep and –7.2% goats) and horses hardly changed (4.3%). The interviews revealed the simplification of the herds was accompanied by the arrival of foreign breeds (Brown Alpine and Friesian in cattle), the relocation of the animals to private properties (private meadows), a parallel decline of livestock grazing in the communal areas, and an additional simplification of the livestock movements within the different areas of Redes.
The livestock statistics for 2016 mirror the situation once Spain entered the EU. Cattle are still dominant (83.6%) and focused on meat production from breeds with lower rusticity than the native ones (e.g., Asturiana de Los Valles double rump). Small ruminants are a minority (goats 5.8% and sheep 5.1%) while horses increased subtly (5.5%). The interviews clarified the progressive loss of grazing areas (abandonment of marginal lands) and the drastic reduction or even disappearance of numerous land management practices (i.e., controlled grazing, hay cut, maintenance of hedgerows, maintenance of livestock infrastructures, etc.).
Changes in agriculture
Agriculture was crucial for local self-sufficiency during the pre-industrial period when the provision of services linked to local food and animal feed production was a priority in rather isolated and marginal locations like Redes. According to the Catastro del Marqués de la Ensenada (1756) and the Diccionario geográfico-estadístico-histórico de España y sus posesiones de ultramar (1802), three crops dominated (spelt, corn and potatoes) and were associated with complementary crops like beans, chestnuts, hazelnuts, walnuts, pears, apples, cherries and legumes. The study of the local ordinances and the interviews revealed that croplands were divided into three groups: private properties with horticulture and fruit orchards, communal cultivation areas in open fields, and finally fruit orchards (apple orchards and chestnut groves). The total cultivated area covered 310 ha at the end of the pre-industrial period according to several local data sources, such as the Resúmenes de riqueza rústica y pecuaria (1962). Later on, the Cuadernos de superficies ocupadas por los cultivos agrícolas (1984) indicated that the total cultivated area decreased 60% to end up covering 123 ha during the Rural Transition. Spelt disappeared, corn became marginal (only 8 ha) whereas potatoes dominated (115 ha, 93% of the cultivated area). The loss of crops and croplands (Supplementary Figures 1–3) was partially driven by: (1) population censuses decline and (2) the improvement of the road network so locals established new socio-cultural and economic relationships outside Redes. Croplands were then replaced by grasslands in the vicinity of the villages to support an emergent dairy production. Meanwhile, chestnut groves ended their role as food providers and evolved into wooded forests.
Changes in forestry
Forestry was a complementary activity during the pre-industrial period. Around 40% of the total surface was covered by communal forests, especially in the villages/towns located at higher altitudes (above 800 m a.s.l.), where steep slopes, harsh climatic conditions (e.g., more extreme temperatures), poorer soils, etc. hindered other activities like agriculture. According to the Catastro del Marqués de la Ensenada (1756), 50 families worked on making wooden clogs. The interviews with the last artisans revealed that 150 of them were still operating during the 1950s, and they produced around 16000 pairs of clogs per month (192,000 pairs annually). The interviews also revealed that around 50 pairs could be obtained from each tree, so about 3850 trees (mainly beech) were cut to sustain such production. According to the ordinances, specific tress was cut and the healthier and most adequate ones were preserved to ensure future timber provision. This production was also important for the construction of buildings, enclosures, manufacturing tools, agricultural implements, etc. Finally, dead woods played an important role in house heating.
Woodlands were also part of the local agri-food system. The ordinances revealed that villagers had the right to plant fruit trees every year in specific locations within communal spaces, holding the ownership of the trees and fruits, while the land remained as communal property. This mixed public-private ownership of nut crops was common and frequently related to a specific landscape structure, the chestnut groves, where scattered large trees coexisted with a matrix of herbaceous vegetation which allowed a mixed agrosilvopastoral use (nut production and livestock grazing).
The shrublands were also involved in the local productive systems. They were grazed, and the gorse and fern, in particular, were mowed and stored for livestock breeding and subsequent generation of manure which was used as fertilizer in croplands and grasslands.
The ownership of the communal forests evolved drastically during the Rural Transition and had deep consequences on the strategies of land use and the associated dynamics of the landscapes. The Catálogo de Montes de Utilidad Pública (which began in 1901) identified 35 Public Utility Mountains (Montes de Utilidad Pública) which covered 30,953 hectares (82% of Redes) and subsequently (1) the municipalities legally appropriated the forests (2) their forest management was transferred from the local communities to the public administration (initially the Forest District). The review of the new Forest Harvesting Plans revealed that timber production was then drastically limited (it almost disappeared) and mostly dead firewood extraction was authorized. The interviews and the review of the local archives revealed a limited acceptance of the new regulations by the locals as conflicts emerged, especially for those whose productive activity was directly linked to the timer production (e.g., the artisans who elaborated the clogs).
During the next period (between 1986 and 2016), the declaration of Redes Natural Park (1996) and Redes Biosphere Reserve (2003) on the same territory reinforced the conservation objectives over the productive ones for the forests in Redes, as revealed by the latest integrated management tool (Instrumento de Gestión Integrada), which indicates that “the habitats with forest communities are the most suitable ones for active conservation through specific action plans (restoration or recovery).” The latest forestry inventory for Asturias (MAAMA, 2012) indicated that the wooded areas extend over 8,886 ha (50% of the total surface) and they are mostly covered by beech forests (31.2%), mixed forests (7.61%), and chestnut groves (7.47%). Regarding timber production, the same inventory quantifies 2962171.04 m3 of wood with bark in Redes, whereas the species-specific logging statistics (Cortas de madera según especie) for the year 2012 estimate the timber harvest to be 251 m3, therefore 0.001% of the available stock. The review of the official documents and the interviews revealed that the traditional activities in the wooded areas (e.g., maintenance of firebreaks, thinning, etc.) became either rare or absent.
New productive activities in Redes
During the Transition period, two water reservoirs and a hydroelectric power plant were built to supply water and energy to the central area of Asturias (where most densely populated areas occur). The valley bottom with the best agro-ecological conditions was flooded: 200 hectares of flat land once occupied by croplands, meadows, a furniture factory, and an agri-food-hospitality complex.
Further efforts to diversify and reactivate the rural economy included increasing support for local tourism through the European aid programs PRODER and LEADER (2001–2016) managed by a Local Action Group (LAG Alto Nalón). The statistics of the tourist activity showed a progressive increase in the number of available beds since 1986, and an acceleration after the creation of the Natural Park in 1996 and the LAG in 2001: from 18 beds in 1986 to 724 in 2016 (Figure 3). A deeper analysis of the typology of the tourist accommodations, based on the reports elaborated by the LAG Alto Nalón, revealed that rural apartments and village houses together account for 84% of the accommodations and 63% of the beds in 2016. These typologies are frequently owned by outsiders and do not require their presence on a daily basis. By contrast, only 1.75% of the tourist accommodations can be classified as agrotourism (a complementary activity for the local farms/agri-food industries).
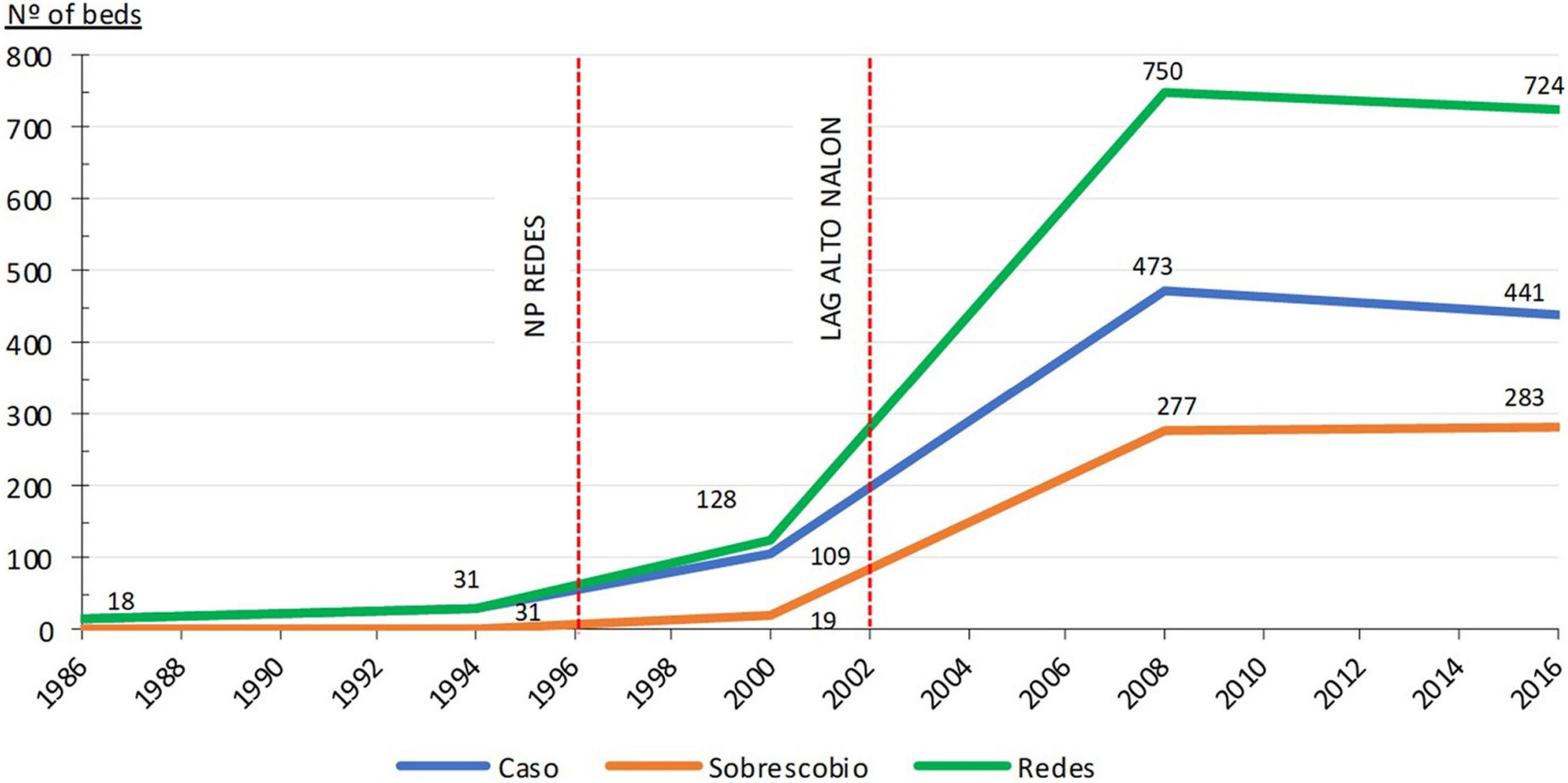
Figure 3. Evolution of the number of hotel beds in Redes (1986–2016). Sources: Reseña de los Municipios Asturianos 1986 y 1996 (SADEI), datasets from LAG Alto Nalón. See Supplementary Table 1 for additional information.
Changes in the landscape
Global analysis through the Mapa de cultivos y aprovechamientos
The maps of crops and uses (MCA) for 1956 and 2010 revealed that landscape fragmentation progressed in Redes between those periods (Supplementary Figure 1 and Supplementary Table 3) as abundant, new, and smaller-sized patches emerged (NP + 105.3%; MPA –51.3%). A new class appeared (the water reservoir) although the classes leading the changes were shrublands and woodlands (Supplementary Table 4) as their total surface rose (CA + 98.3% and 16.9% respectively) through the appearance of numerous new and smaller patches (NP + 173.4%, MPA –27.5% for shrublands and NP + 102.2%, MPA –42.2% for woodlands). The surface covered by grasslands and pastures decreased (CA –22.9% and –95.2%, respectively) through different patterns: grasslands fragmented into numerous smaller patches (NP + 190.0%, MPA –73.5%) whereas pastures persisted in a reduced number of smaller patches (NP –73.1%, MPA –82.2%). The croplands became relict (CA –94.2%) and survived in smaller and scarcer patches (MPA –54%, NP –87.5%). The MCA maps help to visualize the trends in the whole landscape, but, a deeper analysis across the three bioclimatic levels will clarify the observed patterns and allow detect level-specific dynamics.
Analysis of the landscape windows
The first two windows (Supplementary Figures 2, 3) are located on south-facing slopes up to 600 m a.m.l (Table 1) and exemplify traditional mosaics with a mixture of land uses and land covers, including constructions (houses, stables, and auxiliary buildings, etc.), croplands, grasslands for forage production and/or livestock grazing and forests (e.g., dominated by chestnut trees) in the areas with better quality soils. Above them, pasturelands occur in the poorer soils. Around Soto de Agues (Window 1, Supplementary Figure 2) two landscape dynamics were identified (Supplementary Table 3): fragmentation during the first period 1956–1986 (P1) and natural vegetation regeneration during the second one from 1986 to 2016 (P2). The fragmentation resulted from the emergence of smaller-sized patches (NP + 31.2%, MPS –23.7%). Croplands disappeared and pastures reduced their number and size (NP –21.43% and MPS –23.2%). Fragmentation was favored by the rise of abundant new undersized patches of dense and sparse woodlands (NP + 700%, MPS –71.6% and NP + 142.8%, and MPS –79%, respectively). The higher total coverage of dense woodlands and shrublands (CA + 127.1 and 172.7% respectively) compared to grasslands (CA + 36.4%) revealed that forest expansion was already occurring in P1 (Supplementary Figure 2). During P2 pastures disappeared and grassland decline persisted (Supplementary Table 4), whereas dense woodlands continued expanding through new and smaller patches (NP + 37.5% and MPS –6.6%) which ended up covering bigger total surfaces (CA + 28.4%). The sparse woodlands also expanded by P2 (CA + 25.6%), especially through the growth of existing patches (MPS + 16.46), and the emergence of new ones (NP + 7.84%).
The landscape in the area of Tanes (Window 2) became less fragmented (Supplementary Table 3 and Supplementary Figure 3): the total number of patches decreased in both periods (NP –29.3% in P1 and –23.3% in P2) whereas MPS increased (+41.5% in P1 and +30.4% in P2). NP remained rather stable (rates of change below 10%) but further analyses at the class level clarified local trends behind this apparent stability. The grasslands and pastures dominated the traditional landscape in 1956 (together covering 56.6% of the total surface) followed by the sparse woodlands (24.6%). During P1 drastic changes occurred; a water reservoir was built (covering 11.7% of the total surface; Supplementary Figure 3) and the agrarian classes declined: croplands disappeared and grasslands were confined to scarcer patches (NP –27.7% in P1 and –31.9% in P2). Pastures were marginalized too, first, into an increased number of smaller patches (NP + 11.1% and MPS 69.6%) and later to a smaller number of even smaller patches (NP –85.0% and MPS –96.8%). The decline of the early successional stages ran parallel to the forest regeneration through the expansion of shrublands and woodlands (Supplementary Table 4 and Supplementary Figure 3). Shrublands surface consistently increased (CA + 355.4% in P1 and +52.8% in P2) through the growth of existing patches in P1 (NP –10%, MPS + 405.9%) and with new smaller ones in P2 (NP + 144.4% and MPS –37.5%). New dense woodlands also emerged (NP + 55.6% in P1 and + 35.7% in P2) and the class globally covered bigger surfaces (CA + 196.6% in P1 and 15.6% in P2). Sparse woodlands seemed to decline in P1 (NP –61.6%, CA –27.1%) to further expand through new patches (NP + 82.1%) which covered bigger areas (CA + 37.6%) during P2.
At medium altitude (around 900 m.a.sl.), the dominant traditional land uses were linked to forage production for the local livestock herds on the less steep slopes whereas pastures and woodlands are located in stepper territories (Supplementary Figures 4, 5). In 1956 the most characteristic land covers in Fresnedo (Window 3, Supplementary Figure 4) were grasslands (which covered 28.1% of the whole area) and woodlands (27.1% the dense and 24.9% the sparse ones). Landscape fragmentation decreased (Supplementary Table 3) as less numerous (NP –25.4% in P1 and –15.8% in P2) and bigger patches (MPS + 33.4% in P1 and +18.7% in P2) appeared. P1 concentrated the most drastic changes related to the decline of the grassier areas and the expansion of woodlands (Supplementary Figure 4 and Supplementary Table 4). Pastures transitioned from 20 patches in 1956 to a single and smaller one in 2016 whereas grasslands progressively concentrated in a reduced number of smaller patches (NP –24.5%, MPS –10.6% in P1, and NP –17.4%, and MPS –2.7%). Natural reforestation was led by the expansion of shrublands and dense woodlands. The shrubland mean patch size hardly changed (Supplementary Table 4) but numerous new patches rose during P1 (NP + 140%) so the whole surface covered by this class increased (CA + 138.9%). This trend persisted during P2 (NP and CA increased by 16.7 and 16.1%, respectively). Both types of woodlands reduced the number of patches during P1 (NP –43.7% and NP –40.5% for dense and sparse respectively), and the remaining ones followed two trends: they expanded in the dense woodlands (CA + 47.6 in P1 and + 42.7% in P2) and reduced in the sparse ones (CA –10.1% in P1 and –48.6% in P2).
The next window in the areas of Trapa (Window 4) is located in areas traditionally called “invernales,” transitional territories between the lowlands and the highlands which are composed of a mosaic of private grasslands, woodlands, and common lands (Supplementary Figure 5). They provided forage for livestock during the harsh wintering periods. In 1956 pastures dominated (31.3% of the whole window) followed by dense woodlands (22.9%) and grasslands (18.4%) 1956. During P1 the landscape compacted as patch size reduced whereas its mean size increased (NP –35.5%; MPS + 54.9%; Supplementary Table 3). The loss of NP (Supplementary Table 4) is related to the drastic decline of the grassy areas. Grasslands reduced both their number (NP –44.9%) and coverage (CA –21.4%) during P1. During P2 their marginalization progressed into smaller patches (MPS –28.1%) over a reduced total surface (CA –26.7%). The existing pastures reduced their size (MPS –42%) during P1, but later on (during P2) numerous patches disappeared (NP –46.8%) and only smaller-sized ones persisted (MPS –47 to 9%). Natural reforestation is led by the consistent expansion of shrublands and dense woodlands in both periods. New and bigger patches of shrublands were detected in both periods (NP + 100%, MPS + 43.3% in P1 and NP + 84.6% in P2) whereas dense woodland expansion (CA + 26.2% in P1 and 24.6% in P2) relied on the emergence of new patches (NP + 6.4% in P1 and + 30.3% in P2). The sparse woodlands shifted from initial compaction (NP –15.4% and MPS + 30.6%) toward further fragmentation (NP + 109.1%, MPS –56.9%) and partial conversion into dense woodlands which left more isolated and smaller patches of this class during P2 (Supplementary Figure 5). This window shows a subtle reduction of the rocky areas (around 6%) as a result of the natural revegetation once sheep and goat grazing ended there.
The next two windows include characteristic upland areas aimed at livestock grazing and groups of buildings (majadas or brañas) traditionally used by farmers during spring and summer. Pastures, woodlands, and shrublands are present in both windows, but in different proportions (Supplementary Figures 6, 7).
In Cotorgan (Window 5) pastures and shrublands dominated the traditional landscape (41.7 and 21.1% of the window, respectively). The landscape shifted from an initial fragmentation toward a subsequent fusion/compaction (Supplementary Table 3). During P1 numerous smaller patches proliferated (NP + 13.4% and MPS –11.8%) whereas scarcer but bigger ones (NP –41.7% and MPS + 71.62%) were detected during P2. This shift was linked to drastic changes in certain classes (Supplementary Table 4). The pastures vanished from the landscape progressively. They initially fragmented into multiple and smaller patches (NP + 56.8% and MPS –76.1%) to end up covering less than 6% of the total area during P2, once half of the patches disappeared (NP –57.9%) and only smaller-sized ones (MPS –21.56%) persisted. The initial fragmentation was associated with the proliferation of patches of shrublands within the pastures as a result of natural succession (Supplementary Figure 5). The progression of the natural succession is also observed in the wooded areas through the consistent appearance of new patches of dense woodlands (in areas previously occupied by sparse ones, NP + 100% in both periods) which progressively cover bigger surfaces (CA + 203.3% in P1 and 138.8% in P2) and the growth of already existing shrublands (MPS + 183.0% in P1 and 134.7% in P2).
The last window (Window 6, Supplementary Figure 7) in the Brañagallones area was dominated by woodlands (they covered 64.7% of the whole area), followed by pastures (17.9%) whereas shrublands were marginal (5.2%). During P1 the landscape became less fragmented (NP –21.0%, MPS + 26.7%; Supplementary Table 3), whereas the internal reorganization of certain classes during P2 led to increased dominance of certain classes like woodlands and shrublands (Supplementary Table 4 and Supplementary Figure 7). The progressive decline of pastures (CA –28.2% in P1 and –55.5% in P2) and sparse woodlands (CA –19.9% in P1 and –27.2% in P2) concurred with the growth of dense woodlands (CA + 12.1%) within a reduced number of bigger sized patches during P1 (NP –66.7% and MPS + 236.4%). These woodlands continued expanding (CA + 34.7%) during P2 as new smaller parches emerged (NP + 100% and MPS –32.7).
Discussion
The combination of quantitative data (local demographics, productive activities, and landscape metrics) with repeated photographs and interviews with the local communities in Redes allowed a detailed reconstruction of the traditional use of the natural resources and the identification of changes in the land uses and land covers invisible and/or incomprehensible through other techniques of data recording (González Díaz et al., 2018).
This integral methodology evidenced non-linear trends in the rates of change between periods as well as characteristic dynamics for the whole Redes and/or for the different landscape scales led by specific classes and within specific periods.
A variety of driving forces interacted dynamically to boost specific sequences and trajectories of change in the land uses and land covers attending to the environmental, social, political, and cultural context in each moment.
Changes in demography
In Redes, several demographic trends cooperate to hinder the future of the agrosilvopastoral systems as farming largely depends on the numbers, age, and sex pattern of the agricultural workers and managers (Coopmans et al., 2021). First, we detected a double negative demographic imbalance (Mantale, 2010): negative migratory changes together with negative natural balances (deaths exceed deaths). Second, after the rural exodus (carried out mostly by young people and women), few and mostly aged inhabitants remained, and the generational replacement is minimal. Meanwhile, other areas at the regional level (especially the urban ones) follow opposite trends (e.g., population increases as a result of emerging industrial activities). The contrasting trends at local and regional levels were common in Spain during the second half of the 20th century and they were linked to the exodus in rural areas and the population concentration in the urban-industrial areas (Fernández García, 2005).
These trends also occur in other European rural locations (Rey Poveda et al., 2009; López Fernández, 2016), and Redes BR can be considered an example of the ‘the young farmer problem’ (Zagata and Sutherland, 2015) and of the generational renewal crisis in the EU (Burton and Fischer, 2015). No study has attended to the situation of crucial groups for the survival of the farming systems like young generations or women in Redes, although the new CAP strategies support their inclusion in the conversations.
The negative demographic trends detected in Redes have additional negative consequences for the maintenance of multifunctional, sustainable, and productive agrosilvopastoral activities. First, the depopulation and abandonment of farming stop/reduce the transmission of the Local Ecological Knowledge (Berkes, 1993), which is essential for a locally adjusted use of the natural resources, but also for the future design of nature-based strategies. Second, these trends have also been associated with a reduced capacity of the territories to provide ecosystem services, from a reduced provision of local food, the simplification of landscape mosaics (Otero et al., 2015), biodiversity losses (Preiss et al., 1997) or increased fire risk (Chergui et al., 2018). Furthermore, emerging evidence suggests that nature-based solutions could provide diverse ecosystem services and low-cost solutions to varied climate change-related impacts, and offer key advantages, especially to more vulnerable sectors of society, over engineered-only ones (Seddon et al., 2020).
Changes in the productive activities
The traditional agrosilvopastoral systems in mountain areas were diversified at different levels: at the local economic level through varied and frequently interconnected and synchronized land uses and productive strategies, as well as within each strategy (which could deliver several products). The productive activities followed specific calendars in different areas of Redes and all together contributed to the configuration and composition of the landscape mosaics through their impact on the arrangement and characteristics of the different land covers.
The productive specialization/intensification during the Rural Transition involved techno-economic and social changes which could not arrest the socioeconomic marginality of the mountain agro-silvopastoral systems. It also coincided with the disappearance of numerous agro-ecological strategies necessary to provide specific ecosystem services. These changes in the productive activities also launched transformations in the landscape mosaics as a result of specific trends, from biotic ones linked to natural succession (across all the altitudinal levels) to deagrarianisation (which concentrated in the lower lands).
Subsequent attempts opted for diversification and productive reactivation based on beef cattle production and rural tourism; neither did they counteract the demographic trends (e.g., aging, minimum generational renewal) and population censuses decline, nor did they help to halt the loss of landscape heterogeneity based on diversified and multifunctional production systems.
The changes in the characteristics of the households influenced the evolution of the animal production systems. The farms tried to adapt to the new market demands when labor availability was already limited (population censuses had already dropped) and they focused on cattle rearing, a species with less labor demand than small ruminants (Rosa García et al., 2012). The numerous households with small multispecies herds were followed by fewer and bigger farms focused on dairy production during the Transition period, and on beef cattle later on (Table 2). These trends are partially explained by the human demographic evolution; the migrations and subsequent population aging favored the persistence of less labor-demanding livestock species (cattle and horses), which also received greater governmental support (e.g., EU payments).
The specialization of the farms increased their dependence on external inputs (concentrates, fertilizers, etc.) to cope with the nutritional needs of the large herbivores, especially the dairy farms with foreigners and less rustic breeds which thrive to survive on the local resources. Recent studies in Asturias confirmed the higher ecological footprint of the less self-sufficient dairy farms (Jiménez-Calderón et al., 2020).
These social and techno-economic changes within the farms influenced the surrounding landscape in Redes as observed in other marginal mountain areas in Northern Spain where productive activities have changed too (Morán-Ordóñez et al., 2013; García-Llamas et al., 2019; Díaz et al., 2021). Other works linked the changes in vegetation covers to the shifts in livestock species/breeds with different diet selection and grazing behavior (Rosa García et al., 2013). Simultaneously to the loss of the labor force, the new production models managed greater numbers of heads per farm which required longer daily periods focused on animal production. The reorganization of the daily and seasonal activities reduced the time available for other agroecological activities which also played an important role in the conservation of specific landscape classes (e.g., croplands), or specific elements within each class (e.g., the hedgerows surrounding the plots).
The changes in the configuration of the landscape mosaic did not follow a continuous pattern, the rate of change varied between periods that had specific socio-ecological characteristics.
Changes in the croplands
Agriculture concentrated in the best soils on the valley bottom and flatter lands (e.g., Soto de Agues and Tanes) where it played a crucial role in the local food safety (Vázquez and Díaz, 2006). Their replacement by meadows as a result of the specialization in animal production and the reduction of the available workforce also occurred in other northern Spanish mountain areas (Ortega Valcarcel, 1989). Their disappearance led to reorganizations of other land covers over the same territory (especially shrublands), as well as the loss of multiple cultivated and locally adapted plant species (from cereals to vegetables and fruit trees) and the agroecological uses to produce them. The losses of agrobiodiversity ran parallel with decreases in landscape heterogeneity during P2. However, around 50% of the EU wildlife depends on agricultural habitats (Stoate et al., 2009); and multiple studies confirmed the crucial role of agricultural biodiversity in climate change resilience in agrarian landscapes (Mijatović et al., 2013).
The restoration of agriculture in Redes BR requires relocating the grazed areas back to specific areas in the common lands (according to the topography, accessibility, and vegetation cover) to free up space for agriculture in the lowlands. The restoration of cultivated lands or other relevant classes (e.g., pastures) to increase the heterogeneity, resilience, and autonomy of the local mountain communities implies rehabilitating the functional and Spatio-temporal complementarity of the different activities according to the current socio-economic and environmental contexts. To achieve this goal, a fusion of the TEK with other sources of knowledge (e.g., scientific) is needed. The restoration and reorganization of the land use have got additional implications resulting from its implications in the reconfiguration of the land covers. For example, the croplands around the houses or the pastures in specific areas could play a relevant role as productive firebreaks which also increase the global resilience of the area (Moreira et al., 2009).
Changes in the meadows
The meadows disappeared from the higher altitudes and concentrated in more accessible areas with less steep slopes and closer to the villages where they also occupied numerous former croplands as observed in Soto de Agues and Tanes (Supplementary Figures 2, 3). They initially expanded to provide fodder to the dairy systems and later on declined when the surviving farms switched to beef cattle. In Tanes, they first declined when the water reservoir was built and later as a result of the continuous depopulation and land abandonment. The traditional hay cut has almost disappeared and the grasslands are currently grazed and/or ensiled. However, semi-natural hay meadows are among the most biodiversity-rich terrestrial ecosystems (Dahlström et al., 2013). The decline of grasslands is widespread across Europe where they account for 53% of the EU endangered habitats threatened by shifts into intensive crop cultivation or loss of traditional management (Stoate et al., 2009). Within grasslands, meadows and certain open wooded grasslands are one of the most threatened ones (European Union, 2016). However, long-term managed grasslands can harbor higher diversity than more recent ones resulting from modern agriculture (Straubinger et al., 2021). By contrast, the current Integrated Management Instrument (IMI) which includes both the Redes Natural Park and Biosphere Reserve (see Supplementary Table 1) indicates that grassland habitats (Natura 2000 habitat codes 6210 and 6510) may constitute a secondary objective for the conservation of the Special Protection Zone and their conservation will depend intimately on the maintenance of traditional livestock farming practices: extensive grazing and the use of hay cut meadows. For the alpine and subalpine grasslands (code 6170) the conservation objective of the IMI is to identify and preserve the best-preserved areas of these habitats, at least in their present locations. No previous study has assessed the situation of the grasslands in Redes, neither their location nor their characteristics and their future are uncertain as the activities for their conservation are clearly disappearing as evidenced in this study. Most of the meadows have evolved into grazed grasslands and later on to shrublands and woodlands (e.g., landscape windows 3 and 4). Their abandonment involved the cessation of agroecological activities and associated elements, such as hedgerows, irrigation ditches, and associated auxiliary constructions.
Changes in the pastures
The pastures in the common lands ended up disappearing or becoming relict in most of the areas except the highest altitudes during the whole study, although their decline accelerated during P2. The conservation of these habitats has also attracted little attention although previous studies detected that they can hold even higher plant biodiversity than meadows (Pornaro et al., 2021). Their negative trends are linked to the disappearance of the multispecies herds, especially the small ruminants, the support to less rustic cows and horses, as well as the simplification of the livestock movements, which excluded rotational grazing and the use of less accessible areas as the workforce also dropped. Along with the vegetation, the cessation of activity also affected the maintenance of the auxiliary infrastructures (huts, corrals, watering places, etc.), trails, etc. All these changes reduce the functionality of this class which has blurred from the landscape as natural succession progressed and shrublands expanded from the slopes to the valley bottoms.
Changes in the shrublands
The patches of shrubby vegetation expanded across the whole Redes due to: the expansion and fusion of existing patches (e.g., in Tanes, Cotorgan, and Brañagallones during P1), as well as through the emergence of new patches in the grassy areas with better soils (e.g., in Soto, Fresnedo y Trapa during P1 too). The emergence of trees is favored by the reduction of livestock grazing (especially small ruminants) and the cessation of certain techniques like small prescribed fires or mowing for animal bedding.
Nowadays the use of shrublands is limited mostly to extensive grazing by cattle and horses which graze them preferably when the availability in their preferred grassier areas is reduced (Ferreira et al., 2013). However, they could deliver several services, from apiculture to obtaining extracts or compounds of veterinary or human interest. Prescribed clearing and targeted livestock grazing with small ruminants in certain areas could provide additional benefits by controlling the accumulation of flammable shrubby vegetation, a chronic problem across Northern Spain (Lasanta et al., 2017).
Changes in the woodlands
The woodlands have been multifunctional in Redes too; they provided forestry goods, such as fuel wood, poles, or timber, together with fruits (e.g., chestnuts), in areas where local livestock herds may graze the understory as well.
The sparse woodlands traditionally coexisted with dense woodlands and they were mostly allocated in the common lands. Their management attended to specific strategies established by the local communities until the beginning of the 20th century (Ortega Valcarcel, 1989; Fernández García, 2005). The new regulations reorganized the land uses in a more imprecise way than ordinances, and without taking the opinion of the locals into account. As a result, the local communities, who felt disconnected from the new regulations, abandoned the management of the common lands. Meanwhile, the new strategies prioritized the conservation of the common woodlands over most of the previous productive activities, while depopulation and land abandonment were also progressing.
The cessation of varied agro-ecological activities favored the expansion of dense woodlands and the evolution of sparse ones into dense ones through natural succession, as observed in other mountain areas (Lasanta et al., 2006). Forest regeneration is related to biodiversity, landscape dynamics, rural development, and ecosystem health (plant, animal, and human) in several ways, both positive and negative. The spontaneous increases in the connectivity of denser woodlands can help the recovery of endangered species which depend on these habitats or provide corridors to reconnect isolated plant and animal communities. However, the growth of woodlands can also increase the risks associated with the expansion of animal and vegetal diseases and pests. Previous studies in forest pathology linked forest growth to the expansion of tree diseases (Holdenrieder et al., 2004), including the chestnut chancre which is currently affecting the chestnut forests in Redes (González-Varela and González-Fernández, 2006) or an increased incidence of tick-borne diseases which accounts for the majority of vectorborne disease cases in temperate areas of Europe (Diuk-Wasser et al., 2021).
Therefore, forest and land-use management must incorporate the functional role of tree and animal and plant threats in the long term at a restricted scale and also in the short term for larger regions taking into account the current climate change and biological globalization.
The expansion of dense woodlands increases fire risk too, even more, when they are close to flammable shrublands (very common in northern Spain) like heathlands and heath-gorse shrublands, and the productive firebreaks like croplands, grasslands, and pastures, have been reduced drastically. Additionally, an increased number of studies warn that the incidence of mega-fires will probably become more common in the future owing to climate change (Fulé, 2008).
However, proactive management measures aimed at restoring the agriculture in the landscapes, together with others within the forest like thinning or prescribed burning can also relax the pressure from certain pests and pathogens, and reduce fire risk (Aquilué et al., 2020). Long-term measures which increase diversity at different scales (e.g., genetic diversity, tree species diversity, structural diversity, diversity at the landscape scale) could also help to increase the resistance and resilience of forests and their ecosystem services to these threats (Seidl et al., 2018).
Regarding the new productive activities, they provide diverse ecosystem services, but the beneficiaries are mainly located outside Redes and the local community has little role in their management.
Emergent water reservoirs
The water reservoir and the hydroelectric power plant were built during the Transition period to provide essential provisioning services (water and electricity) to the growing population in the urban-industrial areas of the province, not to attend to local needs. These constructions influenced other productive activities which depended on natural resources whose availability drastically decreased (e.g., the most productive lands for agriculture and animal. Therefore, the impacts were local whereas the benefits occurred at longer distances so Redes can be considered a good example of a land-use change that may reduce the livelihood risks for some stakeholders (especially those deciding on land-use changes) but increase risks for others (Fedele et al., 2018), locally in this case.
The constructions had implications in the landscape dynamics too as they increased landscape richness, but they also accelerated cascading effects which cooperated with the landscape homogenization as revealed by the changes in the metrics measured from the MCA maps and the first two landscape windows (Soto and Tanes). The drastic changes in the valley bottom pushed the reorganization of the adjacent areas to maintain the productive activities, resulting in a concentration of agrarian activities in smaller areas during P1 whereas croplands and herbaceous areas on the slopes were replaced by shrublands and woodlands during P2 (as observed in Tanes, window 2).
The impact of the landscape changes on the local hydrology and flow regulation is still poorly understood (Guswa et al., 2017). However, drastic changes in areas close to the water reservoirs could be indifferent, beneficial, or even negative for the water quality (e.g., through eutrofización or toxicity) or local biodiversity (Rust et al., 2019). In northern Spain, additional risks emerge for the water resources as flammable vegetation accumulates around the water reservoirs (Pereira et al., 2021). This is the case of Redes, where such woody vegetation has expanded too, so further studies should explore adaptive strategies to increase the resilience of the whole area and the water resources in particular.
New activities: Rural tourism
The emergent activities like rural tourism have had limited success in revitalizing the local economy and two factors may contribute to this situation: business initiatives are capitalized by non-residents and they are disconnected from other activities. Rural tourism thus neither cooperates with local farming nor is an element of rural culture and environment valorization (Streifeneder, 2016). However, agrotourism, a minority in Redes, and considered by some authors as “authentic agritourism” (Flanigan et al., 2014), could provide a more immersive experience with the local context and a more relevant role in the local socioeconomic (Streifeneder, 2016). Rural tourism could also contribute to connecting the primary sector and the local gastronomy based on sustainable, singular, and Km 0 products associated with the different components of the landscape.
Regarding the link between tourism and landscape dynamics, it slowed the deterioration of the built heritage by rehabilitating and improving the farmhouses (as observed in Windows 1 and 2). However, the revalorization and speculation of the private properties limited the access of locals to them (including young people), while the natururbanisation progresses, including economic activities related to the natural park and daily tourist visits (Prados, 2009).
The study of the complex and dynamic agrosilvopastoral systems evidenced that an interdisciplinary team of experts (sociologists, economists, lawyers, geographers, biologists, etc.) is required to confront future challenges through a holistic perspective.
Conclusion
The integrated analysis of the multiple dimensions (sociocultural, economic, and environmental) associated with these systems along time, and their relationships with the dynamics of the landscape mosaics, is essential to identify the challenges and design locally adapted techniques to specific areas and periods. Depopulation is linked to population aging in Redes and it cooperates to hinder the preservation of the activities and the traditional ecological knowledge (TEK). The collection of TEK urges to better adapt the productive activities to the local context and as a reservoir of information to face future challenges.
The restoration of diversified and interconnected productions (plant and animal) in specific areas and periods could reinforce the resilience and self-sufficiency (including food safety) of the mountain territories, and it would benefit from a combination of the TEK with advanced technologies. Alternative pasture-based animal production systems with mixed local livestock species may be a more resilient alternative to the current beef cattle-specialized models, and they could also deliver a wider variety of ecosystem services, including the conservation/restoration of the landscape mosaics. The new models require a reorganization and restoration of the pasturelands, especially the abandoned communal ones with a mosaic of woodlands, shrublands, and grasslands. The relocation of the animal production based on increased use of the common pasturelands would free the better soils in the valley bottom for small-scale agriculture with local species/varieties. The active conservation of the woodlands ran parallel to the decline of productive activities by local communities that are more disconnected from those areas. The lack of a specific forestry program and the spontaneous expansion, interconnection, and densification of the woodlands reduce the global resilience of Redes by increasing diverse risks. However, these woodlands could boost agroecological activities resulting from the production of high-quality timber, biomass, nuts, mushrooms, etc. Emergent forestry certifications linked to carbon sequestration may also bring additional income to the locals. Strategic livestock grazing in these areas may contribute to controlling the accumulation of flammable material while decreasing the grazing pressure over others. The construction of the water reservoirs and the electricity power station influenced the landscape dynamics and provided crucial services and benefits for distant and more dynamic areas, whereas possible local environmental or socioeconomic impacts were detected. Rural tourism neither contributed to increasing the local incomes nor to the productive diversification of the farms nor to the valorization of the role of agrosilvopastoral systems in the conservation of the landscape. New models of agrotourism are necessary to build an efficient circular economy and preserve the natural and cultural heritage.
Parallel to the regressive evolution of the local socio-economy, and of the agrosilvopastoral systems linked to it, the landscape mosaic as a whole lost heterogeneity, complexity, and diversity. The dominance of forests and scrublands, the drastic decline of grasslands and meadows, and the disappearance of croplands are major drivers of the loss. The resulting landscape is less resilient and capable of providing varied ecosystem services to local communities and society as a whole. Neither the declaration of the Natural Park nor the Biosphere Reserve has been able to arrest the detected trends.
The recovery and/or reorganization of the multiple activities which may revitalize the agrosilvopastoral systems require new approaches that go beyond the current urban logic and adapt to the environmental and socioeconomic local context. The integrated territorial planning and management of the natural resources (in the different areas and periods) should take advantage of the active participation of local communities to create new governance scenarios. The recovery of young people (and especially women) is urgent to stop depopulation, ensure the transmission of the TEK and develop new and improved activities which sustain the diverse landscapes and the associated mosaics. The resultant agrosilvopastoral systems can be HVN farming systems aligned with the goals of the European Green Deal, including specific strategies like the Farm to Fork, the Forest, and Biodiversity strategies, or the Circular Economy Action Plan, among others, but also with more global goals, such as those derived from the 2030 Agenda and the SDGs.
Data availability statement
The original contributions presented in this study are included in the article/Supplementary material, further inquiries can be directed to the corresponding author.
Author contributions
JG and RR-G conceived the study. JG and BG collected the data. BG calculated the landscape metrics and created the maps and figures. RR-G and JG analyzed the landscape dynamics. JG and RR-G wrote the manuscript with substantial input from BG. All authors contributed to the article and approved the submitted version.
Funding
This research was partially funded by the Government of the Principality of Asturias (project GRUPIN IDI/2021/000081) and co-financed by the European Union through the ERDF (European Regional Development Fund).
Acknowledgments
We express our gratitude to the local community of Redes who provided valuable information during the interviews.
Conflict of interest
The authors declare that the research was conducted in the absence of any commercial or financial relationships that could be construed as a potential conflict of interest.
Publisher’s note
All claims expressed in this article are solely those of the authors and do not necessarily represent those of their affiliated organizations, or those of the publisher, the editors and the reviewers. Any product that may be evaluated in this article, or claim that may be made by its manufacturer, is not guaranteed or endorsed by the publisher.
Supplementary material
The Supplementary Material for this article can be found online at: https://www.frontiersin.org/articles/10.3389/fevo.2022.949093/full#supplementary-material
References
Aquilué, N., Fortin, M. J., Messier, C., and Brotons, L. (2020). The potential of agricultural conversion to shape forest fire regimes in mediterranean landscapes. Ecosystems 23, 34–51. doi: 10.1007/s10021-019-00385-7
Berkes, F. (1993). “Traditional ecological knowledge. concept and cases,” in Traditional Ecological Knowledge. Concept and Cases, ed. J. T. Inglis (Ottawa: Canadian Museum of Nature), 1–8. doi: 10.4324/9781315114644-1
Burel, F., and Baudry, J. (1999). Écologie du paysageconcepts, méthodes et applications. Paris: Technique et Documentation.
Burton, R. J. F., and Fischer, H. (2015). The succession crisis in european agriculture. Sociol. Rural 55, 155–166. doi: 10.1111/soru.12080
Carbone, G. (2018). Expert analysis on geographical specifities Mountains, Islands and Sparsely Populated Areas. Available online at: https://ec.europa.eu/regional_policy/sources/docgener/studies/pdf/expert_analysis_geographical_specificities_en.pdf (accessed May 15, 2022).
Carré, J., and Metailié, J. P. (2008). De los paisajes de ayer a los paisajes de mañana. Metodología de un observatorio fotográfico para el análisis de las dinámicas paisajísticas: El valle de Vicdessos, Pirineos de Ariége (Francia). Bogotá: Universidad de los Andes.
Chará, J., Reyes, E., Peri, P., Otte, J., Arce, E., and Schneider, F. (2019). Silvopastoral Systems and their Contribution to Improved Resource Use and Sustainable Development Goals: Evidence from Latin America. Rome: FAO.
Chergui, B., Fahd, S., Santos, X., and Pausas, J. G. (2018). Socioeconomic factors drive fire-regime variability in the mediterranean basin. Ecosystems 21, 619–628. doi: 10.1007/s10021-017-0172-6
Colwell, R. N. (1966). Aerial photography of the earth’s surface; its procurement and use. Appl. Optics 5, 883–892. doi: 10.1364/AO.5.000883
Coopmans, I., Dessein, J., Accatino, F., Antonioli, F., Bertolozzi-Caredio, D., Gavrilescu, C., et al. (2021). Understanding farm generational renewal and its influencing factors in Europe. J. Rural Stud. 86, 398–409. doi: 10.1016/j.jrurstud.2021.06.023
Corbera Millán, M., Calcedo Ordoñez, V., Mauleón, J., Fernández Gutierrez, J., Fernández García, F., Ruiz Urresterazu, E., et al. (1999). Cambios En Los Espacios Rurales Cantaìbricos Tras La Integracioìn De Espanϸa En La Ue. Santander: Universidad de Cantabria.
Dahlström, A., Iuga, A. M., and Lennartsson, T. (2013). Managing biodiversity rich hay meadows in the EU: a comparison of swedish and romanian grasslands. Environ. Conserv. 40, 194–205. doi: 10.1017/S0376892912000458
de Blas Cortina, M. A. (1983). La prehistoria reciente en Asturias, Estudios de Arqueología Asturiana. Oviedo: Fundación Pública de Cuevas y Yacimientos Prehistóricos de Asturias.
Díaz, J. A. G., Díaz, B. G., and Rosa García, R. (2021). Revisión de los objetivos de los espacios protegidos: el parque natural y reserva de la biosfera de Redes. Madrid: Espacio Tiempo y Forma. Serie VI: Geografía 67–88. doi: 10.5944/etfvi.14.2021.28843
Diuk-Wasser, M. A., Vanacker, M. C., and Fernandez, M. P. (2021). Impact of land use changes and habitat fragmentation on the eco-epidemiology of tick-borne diseases. J. Med. Entomol. 58, 1546–1564. doi: 10.1093/jme/tjaa209
European Union (2016). European Red List of Habitats Part 2. Terrestrial and freshwater habitats. Available online at: https://ec.europa.eu/environment/nature/knowledge/pdf/terrestrial_EU_red_list_report.pdf (accessed May 16, 2022).
Fedele, G., Locatelli, B., Djoudi, H., and Colloff, M. J. (2018). Reducing risks by transforming landscapes: Cross-scale effects of land-use changes on ecosystem services. PLoS One 13:e0195895. doi: 10.1371/journal.pone.0195895
Fernández García, F. (2005). “La quiebra del modelo histórico en la Montaña Cantábrica,” in La montaña cantábrica. Una montaña viva, ed. C. Delgado Viña (Santander: Parlamento de Cantabria y Universidad de Cantabria), 79–96.
Fernández García, F. (2010). La representación gráfica de los paisajes rurales: Cuestiones de método. Ería 83, 237–239. doi: 10.17811/er.83.2010.237-259
Ferreira, L. M. M., Celaya, R., Benavides, R., Jáuregui, B. M., García, U., Sofia Santos, A., et al. (2013). Foraging behaviour of domestic herbivore species grazing on heathlands associated with improved pasture areas. Lives. Sci. 155, 373–383. doi: 10.1016/j.livsci.2013.05.007
Flanigan, S., Blackstock, K., and Hunter, C. (2014). Agritourism from the perspective of providers and visitors: a typology-based study. Tour. Manage. 40, 394–405. doi: 10.1016/j.tourman.2013.07.004
Fulé, P. Z. (2008). Does it make sense to restore wildland fire in changing climate? Restor. Ecol. 16, 526–531. doi: 10.1111/j.1526-100X.2008.00489.x
Gallant, A. L., Loveland, T. R., Sohl, T. L., and Napton, D. E. (2004). Using an ecoregion framework to analyze land-cover and land-use dynamics. Environ. Manage. 34, 89–110. doi: 10.1007/s00267-003-0145-3
García-Llamas, P., Geijzendorffer, I. R., García-Nieto, A. P., Calvo, L., Suárez-Seoane, S., and Cramer, W. (2019). Impact of land cover change on ecosystem service supply in mountain systems: a case study in the Cantabrian Mountains (NW of Spain). Reg. Environ. Change 19, 529–542. doi: 10.1007/s10113-018-1419-2
Gerard, F., Petit, S., Smith, G., Thomson, A., Brown, N., Manchester, S., et al. (2010). Land cover change in Europe between 1950 and 2000 determined employing aerial photography. Prog. Phys. Geogr. Earth Env. 34, 183–205. doi: 10.1177/0309133309360141
González Díaz, J. A., Celaya, R., Fraser, M. D., Osoro, K., Ferreira, L. M. M., Fernández García, F., et al. (2018). “Agroforestry systems in northern Spain. The role of land management and socioeconomy in the dynamics of landscapes,” in Agroforestry: Anecdotal to Modern Science, eds J. Dagar and V. Tewari (Berlin: Springer), 189–215. doi: 10.1007/978-981-10-7650-3_7
González-Varela, G., and González-Fernández, A. J. (2006). El chancro del castaño en asturias. Tecnol. Agroaliment. 3, 45–47.
Guswa, A. J., Hamel, P., and Dennedy-Frank, P. J. (2017). Potential effects of landscape change on water supplies in the presence of reservoir storage. Water Resour. Res. 53, 2679–2692. doi: 10.1002/2016WR019691
Holdenrieder, O., Pautasso, M., Weisberg, P. J., and Lonsdale, D. (2004). Tree diseases and landscape processes: The challenge of landscape pathology. Trends Ecol. Evol. 19, 446–452. doi: 10.1016/j.tree.2004.06.003
Huber, L., Schirpke, U., Marsoner, T., Tasser, E., and Leitinger, G. (2020). Does socioeconomic diversification enhance multifunctionality of mountain landscapes? Ecosyst. Serv. 44:101122. doi: 10.1016/j.ecoser.2020.101122
Jiménez-Calderón, J. D., Martínez-Fernández, A., Soldado, A., González, A., Vicente, F., Jiménez-Calderón, J. D., et al. (2020). Faba bean-rapeseed silage as substitute for Italian ryegrass silage: effects on performance and milk quality of grazing dairy cows. Anim. Product. Sci. 60, 913–922. doi: 10.1071/AN17905
Lang, S., and Tiede, D. (2003). “Late-1500071036-1500071036 extension für arcgis-vektorbasiertes tool zur quantitativen landschaftsstrukturanalyse 1 einleitung und motivation,” in Procedings of the ESRI european user conference 2003, Innsbruck, 1–10.
Lasanta, T. (2002). Los sistemas de gestión en el Pirineo central español durante el siglo XX: del aprovechamiento global de los recursos a la descoordinación espacial en los usos del suelo. Ager 2, 173–195.
Lasanta, T., De, U., and Rioja, L. (2014). La contribución de una pequeña estación de esquí al desarrollo de su entorno: el caso de Valdezcaray (La Rioja). Cuad. Turismo 33, 151–172.
Lasanta, T., Errea, M. P., and Nadal-Romero, E. (2017). Traditional agrarian landscape in the mediterranean mountains. a regional and local factor analysis in the central spanish pyrenees. Land Degrad. Dev. 28, 1626–1640. doi: 10.1002/ldr.2695
Lasanta, T., González-Hidalgo, J. C., Vicente-Serrano, S. M., and Sferi, E. (2006). Using landscape ecology to evaluate an alternative management scenario in abandoned Mediterranean mountain areas. Landsc. Urban Plann. 78, 101–114. doi: 10.1016/j.landurbplan.2005.06.003
Lasanta, T., Nadal-Romero, E., and Arnáez, J. (2015). Managing abandoned farmland to control the impact of re-vegetation on the environment. the state of the art in Europe. Environ. Sci. Policy 52, 99–109. doi: 10.1016/j.envsci.2015.05.012
López Fernández, A., León Suarz, B., and Ramos López, J. E. (2006). El agua y los bosques de Redes, La esencia del Paraíso Natural. Oviedo: Consejería de Medio Ambiente.
López Fernández, B. M. (2016). Poblamiento y declive demográfico en Asturias, 2000-2014. Eriìa 99, 95–108. doi: 10.17811/er.99.2016.95-108
MAAMA (2012). Ministerio de Agricultura, Alimentación y Medio Ambiente: Cuarto inventario forestal nacional del Principado de Asturias. Madrid: Centro de Publicaciones.
Mantale, P. (2010). Introduction: a special focus on shrinking regions. Soc. Sci. Jpn. J. 13, 183–185. doi: 10.1093/ssjj/jyq064
Midha, N., and Mathur, P. K. (2010). Assessment of forest fragmentation in the conservation priority Dudhwa landscape, India using FRAGSTATS computed class level metrics. J. Indian. Soc. Remot. 38, 487–500. doi: 10.1007/s12524-010-0034-6
Mijatović, D., van Oudenhoven, F., Eyzaguirre, P., and Hodgkin, T. (2013). The role of agricultural biodiversity in strengthening resilience to climate change: Towards an analytical framework. Int. J. Agr. Sustain. 11, 95–107. doi: 10.1080/14735903.2012.691221
Morán-Ordóñez, A., Bugter, R., Suárez-Seoane, S., de Luis, E., and Calvo, L. (2013). Temporal changes in socio-ecological systems and their impact on ecosystem services at different governance scales: a case study of heathlands. Ecosystems 16, 765–782. doi: 10.1007/s10021-013-9649-0
Moreira, F., Vaz, P., Catry, F., and Silva, J. S. (2009). Regional variations in wildfire susceptibility of land-cover types in Portugal: Implications for landscape management to minimize fire hazard. Int. J. Wildl. Fire 18, 563–574. doi: 10.1071/WF07098
Moreno, G., Aviron, S., Berg, S., Crous-Duran, J., Franca, A., de Jalón, S. G., et al. (2018). Agroforestry systems of high nature and cultural value in Europe: provision of commercial goods and other ecosystem services. Agroforest. Syst. 92, 877–891. doi: 10.1007/s10457-017-0126-1
Nair, P. K. R., Kumar, B. M., and Nair, V. D. (2021). Classification of Agroforestry Systems. An Introduction to Agroforestry. Berlin: Springer. doi: 10.1007/978-3-030-75358-0
O’Rourke, E., Charbonneau, M., and Poinsot, I. (2016). High nature value mountain farming systems in Europe: case studies from the atlantic pyrenees, france and the kerry uplands, Ireland. J. Rural Stud. 46, 47–59. doi: 10.1016/j.jrurstud.2016.05.010
Ortega Valcárcel, J. (2004). “La transición rural en España el campo en la sociedad urbanizada,” in El futuro de los espacios rurales, ed. S. Nogués Linares (Cantabria: Universidad de Cantabria), 89–114.
Otero, I., Marull, J., Tello, E., Diana, G. L., Pons, M., Coll, F., et al. (2015). Questioning the restorative character of the forest transition in the Mediterranean. Ecol. Soc. 20, 1–15. doi: 10.5751/ES-07378-200207
Pereira, P., Bogunovic, I., Zhao, W., and Barcelo, D. (2021). Short-term effect of wildfires and prescribed fires on ecosystem services. Curr. Opin. Env Sci. Health 22:100266. doi: 10.1016/j.coesh.2021.100266
Plieninger, T., and Huntsinger, L. (2018). Complex rangeland systems: integrated social-ecological approaches to silvopastoralism. Rang. Ecol. Manag. 71, 519–525. doi: 10.1016/j.rama.2018.05.002
Pornaro, C., Spigarelli, C., Pasut, D., Ramanzin, M., Bovolenta, S., Sturaro, E., et al. (2021). Plant biodiversity of mountain grasslands as influenced by dairy farm management in the Eastern Alps. Agr. Ecosyst. Environ. 320:107583. doi: 10.1016/j.agee.2021.107583
Prados, M. J. (2009). Naturbanization: New identities and processes for rural-natural areas. London: Taylor & Francis.
Preiss, E., Martin, J. L., and Debussche, M. (1997). Rural depopulation and recent landscape changes in a Mediterranean region: Consequences to the breeding avifauna. Landsc. Ecol. 12, 51–61. doi: 10.1007/BF02698207
Rey Poveda, A., Cebrián Villar, M., and Ortega Osona, J. A. (2009). Despoblamiento y envejecimiento en Castilla y León durante el siglo XX: análisis a través de la emigración femenina y la pérdida de nacimientos. Ager 8, 113–150.
Rosa García, R., Celaya, R., García, U., and Osoro, K. (2012). Goat grazing, its interactions with other herbivores and biodiversity conservation issues. Small Rumin. Res. 107, 49–64. doi: 10.1016/j.smallrumres.2012.03.021
Rosa García, R., Fraser, M. D., Celaya, R., Ferreira, L. M. M., García, U., and Osoro, K. (2013). Grazing land management and biodiversity in the Atlantic European heathlands: a review. Agroforest. Syst. 87, 19–43. doi: 10.1007/s10457-012-9519-3
Rust, A. J., Randell, J., Todd, A. S., and Hogue, T. S. (2019). Wildfire impacts on water quality, macroinvertebrate, and trout populations in the Upper Rio Grande. Forest Ecol.Manag. 453:117636. doi: 10.1016/j.foreco.2019.117636
SADEI (1987). Campaña de saneamiento ganadero 1986. Datos estadísticos. Madrid: Servicio de Publicaciones del Principado de Asturias.
Seddon, N., Chausson, A., Berry, P., Girardin, C. A. J., Smith, A., and Turner, B. (2020). Understanding the value and limits of nature-based solutions to climate change and other global challenges. Philos. Trans. R. Soc. Biol.Sci. 375:20190120. doi: 10.1098/rstb.2019.0120
Seidl, R., Klonner, G., Rammer, W., Essl, F., Moreno, A., Neumann, M., et al. (2018). Invasive alien pests threaten the carbon stored in Europe’s forests. Nat. Commun. 9:1626. doi: 10.1038/s41467-018-04096-w
Stoate, C., Báldi, A., Beja, P., Boatman, N. D., Herzon, I., van Doorn, A., et al. (2009). Ecological impacts of early 21st century agricultural change in Europe – a review. J. Environ. Manage. 91, 22–46. doi: 10.1016/j.jenvman.2009.07.005
Straubinger, C., Reisch, C., and Poschlod, P. (2021). The influence of historical management on the vegetation and habitat properties of semi-dry grassland. Agr. Ecosyst. Environ. 320:107587. doi: 10.1016/j.agee.2021.107587
Streifeneder, T. (2016). Agriculture first: assessing European policies and scientific typologies to define authentic agritourism and differentiate it from countryside tourism. Tour. Manage. 20, 251–264. doi: 10.1016/j.tmp.2016.10.003
Vázquez, A., and Díaz, T. E. (2006). Parque Natural de Redes: Tierras de bosques y urogallos. Oviedo: Ediciones Novel.
Keywords: land abandonment, depopulation, land use, extensification, livestock, landscape (gamma) diversity, rural development, mountain
Citation: González Díaz JA, González Díaz B and Rosa-García R (2022) Role of socioeconomy and land management in the evolution of agrosilvopastoral landscapes in Northern Spain: The case study of Redes Biosphere Reserve. Front. Ecol. Evol. 10:949093. doi: 10.3389/fevo.2022.949093
Received: 20 May 2022; Accepted: 25 July 2022;
Published: 31 August 2022.
Edited by:
Anita Diaz, Bournemouth University, United KingdomReviewed by:
Caiyun Luo, Chinese Academy of Sciences (CAS), ChinaRafael Mata-Olmo, Autonomous University of Madrid, Spain
Ramón Reiné, University of Zaragoza, Spain
Copyright © 2022 González Díaz, González Díaz and Rosa-García. This is an open-access article distributed under the terms of the Creative Commons Attribution License (CC BY). The use, distribution or reproduction in other forums is permitted, provided the original author(s) and the copyright owner(s) are credited and that the original publication in this journal is cited, in accordance with accepted academic practice. No use, distribution or reproduction is permitted which does not comply with these terms.
*Correspondence: Rocío Rosa-García, cm9jaW9yQHNlcmlkYS5vcmc=