- 1School of Life Sciences, Arizona State University, Tempe, AZ, United States
- 2Schools of Geographical Science and Urban Planning and Sustainability, Arizona State University, Tempe, AZ, United States
- 3Department of Environmental Science, Barnard College, New York, NY, United States
Introduction: Integrated social and ecological processes shape urban plant communities, but the temporal dynamics and potential for change in these managed communities have rarely been explored. In residential yards, which cover about 40% of urban land area, individuals make decisions that control vegetation outcomes. These decisions may lead to relatively static plant composition and structure, as residents seek to expend little effort to maintain stable landscapes. Alternatively, residents may actively modify plant communities to meet their preferences or address perceived problems, or they may passively allow them to change. In this research, we ask, how and to what extent does residential yard vegetation change over time?
Methods: We conducted co-located ecological surveys of yards (in 2008, 2018, and 2019) and social surveys of residents (in 2018) in four diverse neighborhoods of Phoenix, Arizona.
Results: 94% of residents had made some changes to their front or back yards since moving in. On average, about 60% of woody vegetation per yard changed between 2008 and 2018, though the number of species present did not differ significantly. In comparison, about 30% of woody vegetation changed in native Sonoran Desert reference areas over 10 years. In yards, about 15% of woody vegetation changed on average in a single year, with up to 90% change in some yards. Greater turnover was observed for homes that were sold, indicating a “pulse” of management. Additionally, we observed greater vegetation turnover in the two older, lawn-dominated neighborhoods surveyed despite differences in neighborhood socioeconomic factors.
Discussion: These results indicate that residential plant communities are dynamic over time. Neighborhood age and other characteristics may be important drivers of change, while socioeconomic status neither promotes nor inhibits change at the neighborhood scale. Our findings highlight an opportunity for management interventions, wherein residents may be open to making conservation-friendly changes if they are already altering the composition of their yards.
Introduction
Cities globally are rapidly expanding, with most urban land under human management (Foley et al., 2005; Seto et al., 2011). Management choices in public and private spaces can improve biodiversity outcomes and human wellbeing (Dearborn and Kark, 2010; Hartig et al., 2014). In particular, management of urban plant communities can increase the provision of cultural services, wildlife habitat, and cooling services (Jenerette et al., 2011; Nesbitt et al., 2017). As interest in these ecosystem services grows, understanding how and why urban land managers, including individual residents, incorporate new plant communities or change existing ones will inform ways to increase biodiversity and other service provision in urban areas.
Natural plant communities are dynamic, changing randomly or directionally over time through processes of disturbance and succession, as well as in response to trophic interactions and other environmental drivers (Clements, 1916; Pickett et al., 2009; Pulsford et al., 2016). Urbanization also changes vegetation communities, including through slow, continued species loss over time following the initial effects of land conversion, for example from native desert or forest to a new residential subdivision (Nowak and Walton, 2005; Rogers et al., 2009; Walker et al., 2009; Dolan et al., 2011; Ziter et al., 2017). Changes within urban land uses alter vegetation, as with modifications to greenspace size or shape over time (reviewed in Gaston et al., 2013) or land abandonment and revitalization (Pearsall and Christman, 2012; Johnson et al., 2018). The temporal dynamics of urban tree cover have been well studied relative to other types of vegetation, with declining canopy cover over time recorded in many cities (Nowak and Greenfield, 2012, 2018; Roman et al., 2017, 2018; Guo et al., 2018; Smith et al., 2019). However, the extent to which urban plant communities experience changes in composition outside of land-use conversion effects has rarely been evaluated.
Residential yards compose 25%–40% of land area in cities (Loram et al., 2007; Mathieu et al., 2007), and in the United States, they represent a homogeneous, continental-scale macrosystem with relatively similar biophysical properties compared to natural ecosystems (Groffman et al., 2017). However, individual management decisions made by residents (i.e., homeowners or renters who reside in a home) influence local-scale ecological outcomes, such as plant resources available to support wildlife. Numerous studies have addressed the range of attitudinal and social-economic forces driving land manager decisions in urban, residential, and other private land-management contexts, and the ways these decisions shape existing landscapes (Larson et al., 2010; Drescher et al., 2017; Wheeler et al., 2020). However, the extent to which land manager decisions drive temporally dynamic urban plant communities over time has not been evaluated.
Following what we know about individuals’ landscape management choices, residents may manage their yards to maintain structural and compositional consistency over time to align with local norms and individual preferences. Residents overwhelmingly manage yards to maintain a neat, esthetically pleasing appearance (Larson et al., 2009; Nassauer et al., 2009; Larson and Brumand, 2014), which requires regular maintenance such as pruning and replacement of dead plants. Additionally, residents tend to prioritize low-maintenance yard landscaping, suggesting that they may choose to expend minimal effort by maintaining existing vegetation rather than choosing new plants (Martin et al., 2003; Larson et al., 2009; Conway, 2016). If residents manage yards for structural consistency over time and a consistent community composition, they may replace removed plants with the same species or type of vegetation, resulting in little change over time. Normative pressures and regulations that enforce yard conformity with the neighborhood may also prevent major vegetation change and require upkeep of consistent landscaping (Nassauer et al., 2009; Blaine et al., 2012; Hunter and Brown, 2012). These social pressures are particularly influential in the visible front yard, where neighbors are perceived as more likely to view and critique yard management choices (Zmyslony and Gagnon, 1998; Hunter and Brown, 2012). For example, the normatively prescribed residential lawn requires the maintenance of a consistent composition, height, and color (Robbins, 2007; Burr et al., 2018), reducing variability or change.
In addition to normative pressures, neighborhood and resident characteristics may impact the ways in which yard vegetation changes over time. Socioeconomic status is a well-established predictor of residential plant diversity and abundance (Hope et al., 2003; Grove et al., 2014; Avolio et al., 2015; Gerrish and Watkins, 2018; Leong et al., 2018) and is also related to tree survival (Roman et al., 2014; Ko et al., 2015). Neighborhoods with greater financial resources could have less dynamic vegetation because residents are able to maintain consistent plant communities over time. Conversely, they may have more dynamic vegetation, because residents can afford to make potentially expensive changes like adding and removing trees. In older, established neighborhoods, the decisions of previous residents continue to influence present-day landscapes, while in newer neighborhoods developer decisions may be particularly impactful (Larson et al., 2017; Roman et al., 2018). These contextual factors are likely to impact the extent to which vegetation remains static through time.
While residents may manage for consistency, evidence also suggests that yard landscaping experiences significant shifts over time. For example, yards in the Phoenix metropolitan area have changed from lawn-dominated landscape types to low water-use landscapes over the past several decades (Frost, 2016). This change has been gradual compared to a similar transformation in California wherein lawns were removed in response to drought, short-term water restrictions, and lawn replacement programs (Pincetl et al., 2019). Additionally, residential plant communities have changed in response to economic disturbances resulting in home foreclosures and reduced management (Ripplinger et al., 2017). Change over time is also indicated by the increasing degree to which residential yards match resident preferences with longer duration of residence in the home (Kendal et al., 2012a). Research on environmental behaviors and the persistence of habit has identified a window of opportunity for change in the 3 months following residents moving into homes, suggesting the possibility of rapid change in yard characteristics when a home is sold (Verplanken and Roy, 2016). Thus, it is clear that social, ecological, and climatic disturbances are able to provoke change in urban vegetation, as they do in natural landscapes (Collins et al., 2011).
Understanding the ways in which managed urban vegetation compares to dynamic natural communities requires a social-ecological approach. To structure our exploration of how managed urban plant communities change over time, we considered change and disturbance as characterized in two ways: long-term, chronic change (“presses”), and short-term, discrete change (“pulses”), which can include both ecological and social elements (Supplementary Figure S1; Collins et al., 2011). Examples of press disturbances within a residential yard context include shifts in dominant norms and landscape fashion, climate change, and household demographic change. Pulse disturbances include damage from storms or other discrete events, changes in the land manager through home sales or rental turnovers, abrupt shifts in household economic condition or leisure time through changes in employment, and changes in incentive or regulatory structures around residential yard management. All of these disturbances could affect yard plant community composition, either by testing the stability of the landscape if maintained for consistency, or by provoking small management events (e.g., planting or removing a single plant) or large management events (e.g., re-landscaping an entire yard). While ecological disturbance events may act directly on the plant community (e.g., trees blown down during a storm or killed by pests), social disturbances act indirectly through either pulse or press changes in management behavior.
In this paper, we ask four questions about change over time in residential yard vegetation:
1. How much do residents change their yard landscaping?
2. How dynamic are yard plant communities as a result, in comparison to natural plant communities?
3. To what extent does change occur as “pulses” of change in response to discrete disturbances?
4. How do neighborhood-scale characteristics impact yard vegetation dynamics?
To address these questions, we conducted a paired social and ecological survey of residential yards in four neighborhoods of Phoenix, Arizona. We evaluated front yard woody plant community turnover between 2008, 2018, and 2019 to characterize patterns of vegetation change, and compared turnover with resident-reported characteristics. We compared turnover in residential yards to turnover in surrounding desert communities to contextualize our findings within the local natural landscape.
Materials and methods
Study area and sample selection
Phoenix, Arizona is located in the Sonoran Desert in the southwestern United States. Annual rainfall is low in this semi-arid region and summer temperatures are high, with an annual average of 20 cm of precipitation and 109 days over 100°F (38°C; NOAA, 2019a,b). The Phoenix–Mesa–Scottsdale metropolitan area is currently among the fastest growing cities in the United States (U.S. Census Bureau, 2019). With rapid population growth has come rapid development, previously on agricultural land but increasingly on newly converted desert. Phoenix has historically been considered an oasis in the desert, with abundant grassy landscaping despite its arid context (Hirt et al., 2008). However, alternative desert-like xeric yards are increasingly common, especially in new developments (Larsen and Harlan, 2006; Walker et al., 2009; Frost, 2016). Due to the changing characteristics of new construction and the rapid influx of people, the city of Phoenix may be more likely to experience landscape change over time than other cities.
We sampled yards within four neighborhoods located along a north-to-south transect in the city of Phoenix (see Larson et al., 2010; Larson and Brumand, 2014; Wheeler et al., 2022a). Neighborhoods were originally selected as part of the Central Arizona-Phoenix Long-Term Ecological Research (CAP LTER) Phoenix Area Social Survey project (Larson et al., 2010, 2017), with boundaries defined by 2000 U.S. Census block groups.
The four neighborhoods used in this study were selected to represent different combinations of socioeconomic status and dominant landscape type. Two sampled neighborhoods had primarily mesic-type landscaping with mowed turfgrass lawns and two were primarily xeric, and in each of these landscape groupings one neighborhood had higher median income and educational attainment and one lower (Table 1, Figure 1). Due to the historic grassy character of Phoenix and the more recent shift to desert-like landscaping, the two mesic neighborhoods are also older than the xeric neighborhoods (Table 1). Notably for this study, one neighborhood (“Historic Palms District”) is a designated historic district, and in the early to mid 1900s was considered the “best and most exclusive residential district in Phoenix… highly restricted… occupied by the more affluent business and professional men” (Nelson et al., 2020). Thus, this neighborhood has a long history of affluence and privilege not shared by the other three neighborhoods. Current historic protections restrict visible architectural change to the homes. Palm trees (Washingtonia spp.) are maintained in the strip of lawn between the sidewalk and road in this neighborhood, but other vegetation is not restricted by the historic designation. However, strong normative pressures to maintain historic character (including lawns) have been observed in this particular neighborhood (Larson and Brumand, 2014).
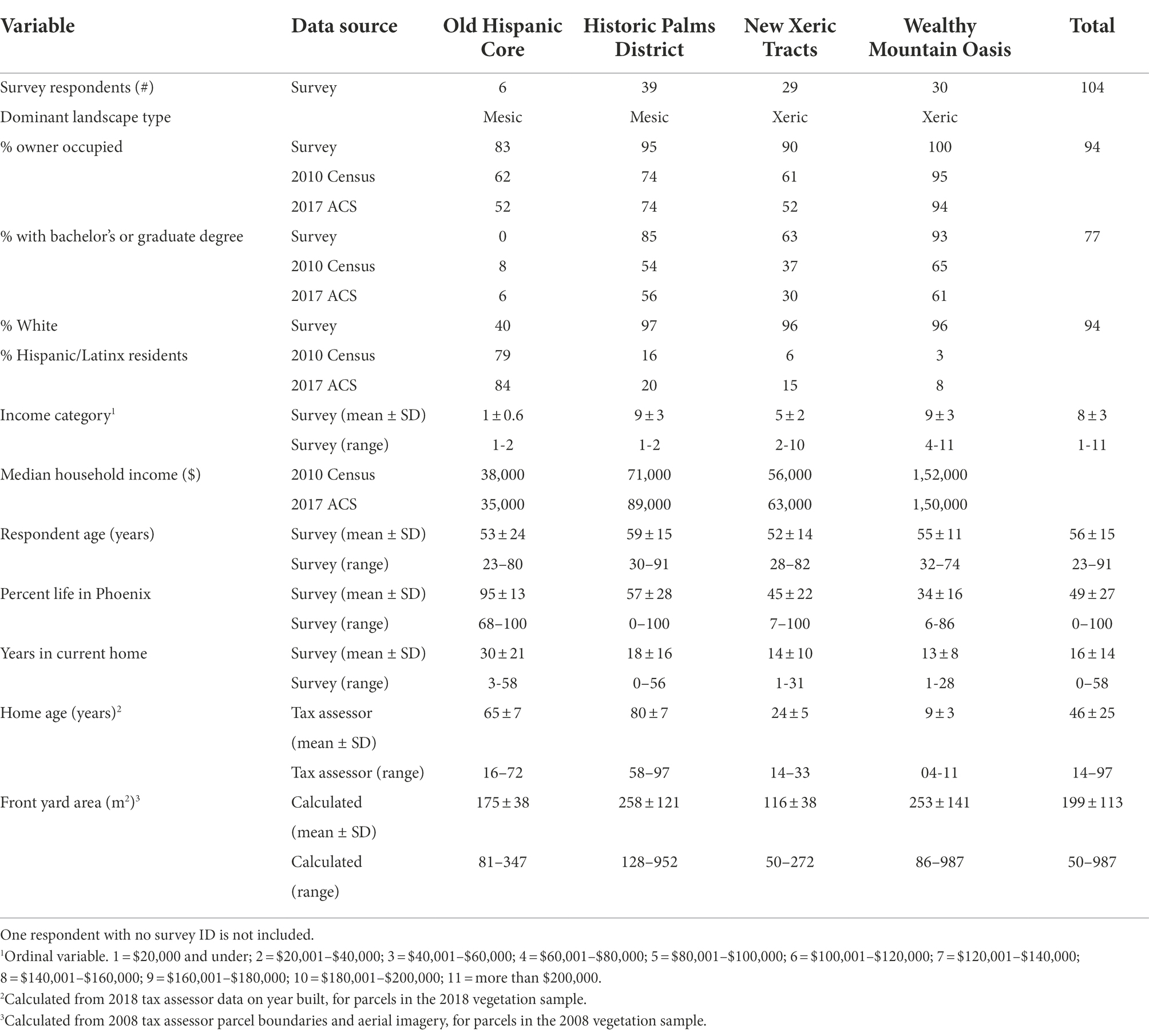
Table 1. 2018 social survey respondent demographics by neighborhood, and neighborhood demographics from the 2010 U.S. Census and the 2017 American Community Survey for the most closely overlapping census tract for each sample neighborhood.
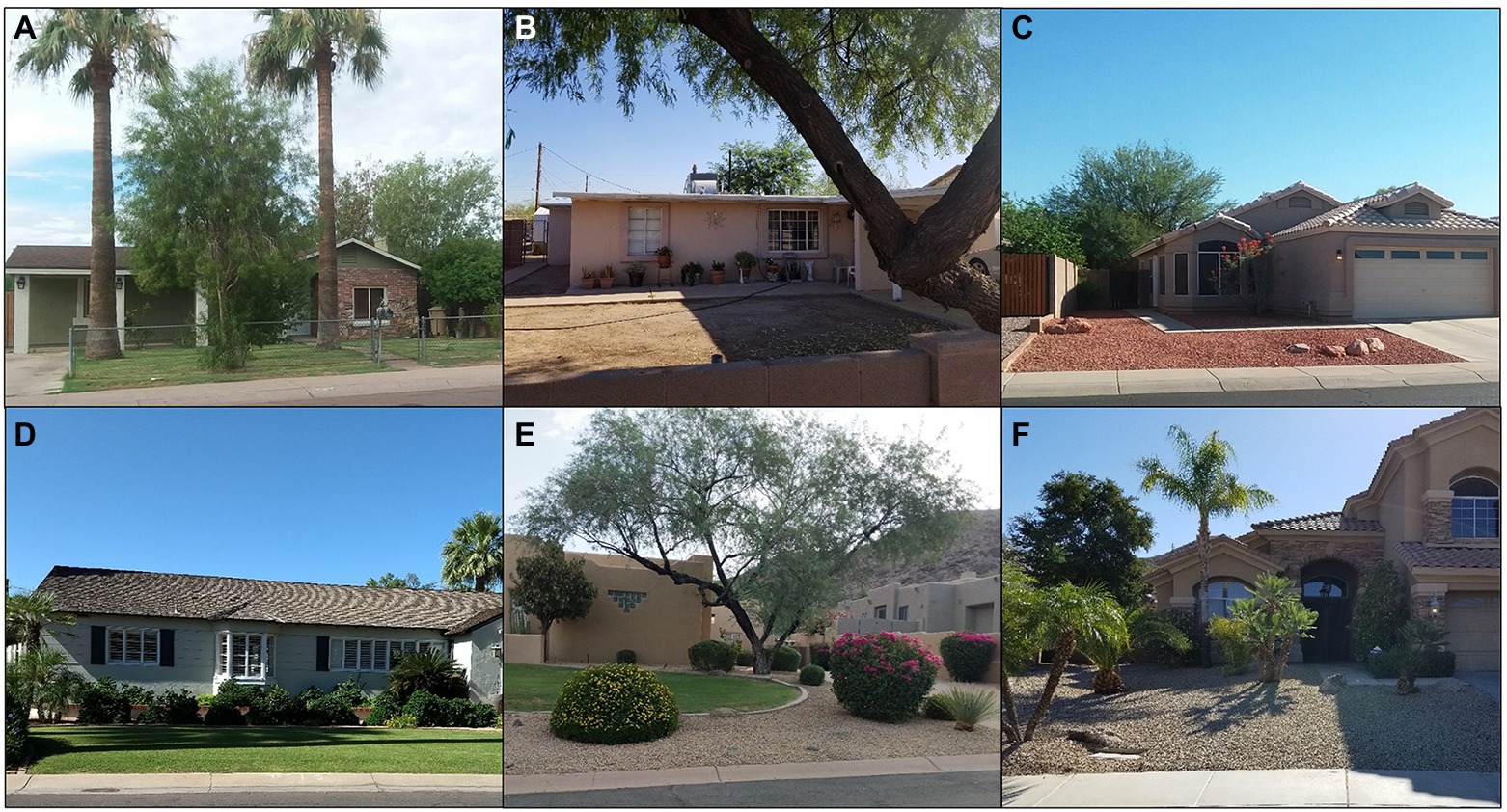
Figure 1. Photos of six yards included in this study, from 2018. (A) Old Hispanic Core mesic yard; (B) Old Hispanic Core bare yard; (C) New Xeric Tracts xeric yard; (D) Historic Palms District mesic yard; (E) Wealthy Mountain Oasis oasis yard; (F) Wealthy Mountain Oasis xeric yard.
Within each selected neighborhood, we randomly chose ~100 parcels to sample using Maricopa County Tax Assessor records. We obtained the construction year and last sale date for each chosen parcel from tax assessor records. We also calculated front yard area for each parcel using ArcGIS to match Tax Assessor-defined parcel boundaries with 2005 aerial photos (0.3 m resolution) and 2009 satellite images from Google Maps. Front yards were manually outlined to calculate yard area (Table 1).
We sampled front yard vegetation in 2008 and 2018 to measure change over a period of 10 years, and again in 2019 to capture the rate of change in a single year. 428 parcels were sampled in 2008 (April–August), 417 of these parcels were resampled in 2018 (May–June) and 100 parcels were sampled a third time in 2019 (September). In 2018, we also surveyed residents to understand their yard management behavior and attitudes. Some parcels were not resampled because they could not be definitively relocated or were inaccessible at the time of sampling.
Resident social survey
To address our first research question, we surveyed residents to understand the extent to which they had changed their front and back yards, relative to their duration of residence in the home. In June 2018, we contacted 425 of the 428 households for which vegetation was surveyed in 2008 (omitting three households with 2008 addresses that could not be uniquely identified). Each household received a printed survey booklet, stamped and addressed return envelope, and cover letter with English on one side and a Spanish translation on the other side. Letters included instructions to request a Spanish language survey. Households were sent a follow-up postcard 1 week after the survey was mailed to thank respondents and remind others to return the survey. As an incentive for participation, 15 $25 Visa gift cards were raffled among respondents, detailed in the cover letter. A total of 105 surveys were completed and returned, yielding a response rate of 25.7% (excluding 16 surveys returned as vacant or undeliverable; Table 2).
In the survey, we asked residents how long they had lived in the home and the extent to which they had made changes to the front and back yard. Responses were (1) I have made no changes; (2) I have made a few changes; (3) I have made a lot of changes; and (4) I have completely redone my yard. We also asked whether residents had planted or removed any trees.
Residential vegetation surveys
To address our second, third, and fourth research questions, we surveyed front yard vegetation in 2008, 2018, and 2019. Front yards were surveyed from the front sidewalk or from the street where there was no sidewalk. We did not enter the property unless invited by the resident. Vegetation in the strip of ground between the front sidewalk and the street, where present, was not considered as part of the front yard (Supplementary Figure S2). For each yard, we determined the overall yard typology and inventoried yard vegetation. Based on previous research (Larsen and Harlan, 2006; Larson et al., 2009), the yard typologies were broad classes defined as: mostly grass with some trees and other plants (“mesic”), some grass and some crushed rock with or without trees and other plants (“oasis”), mostly crushed rock with desert-like trees and other plants (“xeric”), mostly patio area with trees and other plants in pots (“paved”), and mostly bare dirt with little vegetation (“bare”; Figure 1).
Due to our focus on change over a 10-year period, the difference in seasonal timing of 2019 sampling, and our sidewalk survey method, our sampling focused on perennial vegetation only, omitting herbaceous and grass species as they may be replaced on much shorter time scales (e.g., annual flowers which have often died before our 2008 and 2018 summer sampling period). We included all trees, shrubs, cacti and other succulents, and vines, as well as some perennial subshrubs, such as Asparagus densiflorus, Asclepias subulata, Canna × generalis, and Strelitzia sp. (hereafter, “woody plants”; Supplementary Table S1).
We identified front yard woody plants to the lowest possible taxon from the front sidewalk using gross morphological features and knowledge of locally available horticultural species. Individuals of each species were counted and recorded, which was possible due to the relatively low density of planting in most yards (Figure 1). Field team leaders from 2008, 2018, and 2019 compared plant survey methods to ensure consistency in sampling effort. Although we identified many individuals to species in the field, we conducted analyses at the genus level due to the difficulty of consistently distinguishing common cultivars and hybrids. Some individuals were left as unknowns (4%–5% of woody individuals per year) if they could not be seen well enough to identify, often for plants that were small, recently pruned, far from the front sidewalk, or lacking visible identifying characteristics. Photos of each unknown taxon in 2018 were checked against the 2008 species list for the yard to confirm that they were not any species present in 2008. In 2019, we re-surveyed yards using the 2018 species list in the field and recording species abundance and any changes in composition.
Species nomenclature follows The Plant List (2013), with the exception of the common palo verde tree, which was consistently identified as Parkinsonia sp. although some species are classified in The Plant List as Parkinsonia and some as Cercidium.
Reference vegetation surveys
To contextualize our findings, we used vegetation data from the CAP LTER Ecological Survey of Central Arizona conducted in 2005 and 2015 (Childers et al., 2018). The sampling effort included vegetation surveys in ~200 30 m × 30 m sampling plots distributed in a stratified random pattern across metropolitan Phoenix. We included only plots which were sampled in both years with the same dominant land use type: urban, to evaluate whether the sampled residential yards represent urban land uses more broadly, or native Sonoran Desert, to compare against natural vegetation turnover rates. Urban land uses included residential, industrial, commercial, open (i.e., golf courses, parks, and vacant land), and transportation uses (i.e., highways, roads, and airports). This resulted in a total of 94 plots (37 desert, 57 urban). In each survey, all trees and other perennial vegetation within the plot were identified to species and the number of individuals was recorded.
Residential yard analysis
One parcel with a returned social survey was excluded from the vegetation data due to inability to view the entire front yard. An additional returned survey had no sample ID and so could not be linked with vegetation data. Three yards with returned social surveys could not be resurveyed in 2019. Thus, a total of 100 parcels had the complete dataset of 2008, 2018, and 2019 vegetation data and 2018 social survey responses, 103 parcels had 2008 and 2018 vegetation data with social survey responses, and 416 parcels had vegetation data for 2008 and 2018 (Table 2). All data are available online (Wheeler et al., 2022b). All analyses were conducted in R, version 3.6.1 (R Core Team, 2019).
Survey respondents were primarily homeowners, with relatively high income and education compared to census demographics (Table 1). Therefore, caution should be taken in broadly generalizing resident-reported change. However, front yard vegetation change was measured in a representative sample of the four focal neighborhoods, and thus these measures include a broad range of resident and parcel characteristics (Table 1).
To find vegetation community change over time (hereafter “turnover”), we calculated Bray–Curtis dissimilarity for each yard against itself from 2008 to 2018 (10-year dissimilarity) and from 2018 to 2019 (1-year dissimilarity). Dissimilarity was calculated at the genus level for woody plants only, excluding unidentified individuals, and is a conservative estimate of change given that replacement of an individual with a new individual in the same genus would not be considered a change. Results for Bray-Curtis and Jaccard dissimilarity showed comparable trends (Supplementary Table S2); therefore, only Bray–Curtis dissimilarity is reported here. To compare our residential front yards with natural vegetation and broader urban vegetation, we compared trees, shrubs, succulents, and vines at the genus level between reference surveys in 2005 and 2015 and calculated 10-year Bray–Curtis dissimilarity.
We compared the distributions of dissimilarity values for residential front yards between time periods with a two-sample Kolmogorov–Smirnov test using the ks.test function. We also compared the dissimilarity distribution of desert reference plots with urban reference plots and with our residential front yards using a two-sample Kolmogorov–Smirnov test.
In addition to dissimilarity metrics, we calculated the generic richness of each yard as the number of unique woody genera present in order to test for changes in diversity over time. We conducted a Student’s t-test using the t.test function to test whether change in generic richness of yards differed significantly from zero over the 10-year and 1-year periods.
To evaluate the impact of pulse changes and address our third research question, we considered two pulse-type changes that we expect to result in high plant community turnover in residential yards: change in yard typology and change in ownership. Yard typology change came from our field survey classifications and largely reflected change in dominant groundcover type (i.e., lawn, crushed rock, bare dirt, or paving). Change in ownership was obtained from tax assessor most recent sale dates obtained at the time of field surveys in 2018. We compared Bray–Curtis dissimilarity for yards that had or had not changed typology or ownership between 2008 and 2018 using ordinary least squares (OLS) regression. We also compared neighborhoods in this model to test whether vegetation turnover differed by neighborhood, and included an interaction between typology change and neighborhood to determine whether differences in neighborhood dominant typology and socioeconomic status altered the effect of typology change (e.g., typology change may more often correspond with large-scale planting in wealthier neighborhoods). We also compared generic richness by neighborhood and year (2008 and 2018) using a two-way ANOVA with Tukey post-hoc test. OLS model residuals were normally distributed (Shapiro–Wilk test, p = 0.3). We ran a Tukey post-hoc test for pairwise comparisons of neighborhoods following OLS modeling.
Results
RQ1: Reported yard change
Most surveyed residents reported having made at least a few changes to their front yard (~75% of respondents) or back yard (~90%) since moving into their home, and only 6% of respondents reported having made no changes to either the front or the back yard (Figure 2). Respondents who had lived in the home for <5 years had made only slightly fewer changes than the overall sample, with 66% reporting changes to the front yard and 79% to the back yard. Considering the tree community alone, over 60% of respondents had both planted and removed at least one tree since moving into their home, and 16% had neither planted nor removed a tree.
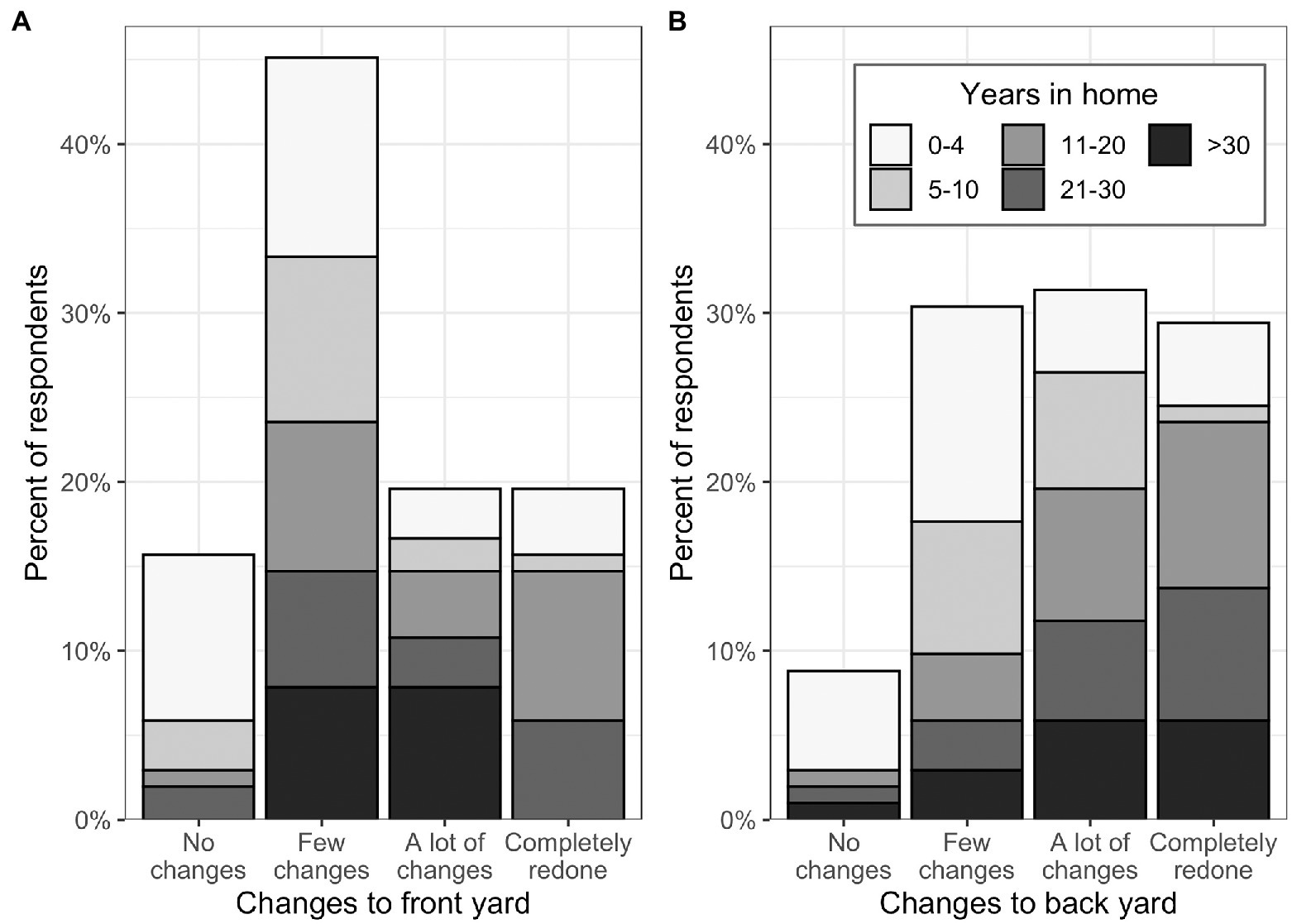
Figure 2. Reported amount of change made to the (A) front yard and (B) back yard in the time the respondent had lived at their current home (shown by shades of gray). N = 102.
RQ2: Amount of vegetation change in residential front yard and reference plant communities
The mean Bray–Curtis dissimilarity of a front yard with itself between 2008 and 2018 was 0.58 for all woody vegetation (equivalent to 58% turnover; median = 0.60; Figure 3). Only 3 yards (0.7% of sample) had the same vegetation composition in both years, while 33 yards (8% of sample) experienced complete turnover. Measured dissimilarity for yards across 2008 and 2018 was somewhat higher where residents reported having made more changes, though this difference was not statistically significant (Supplementary Figure S3).
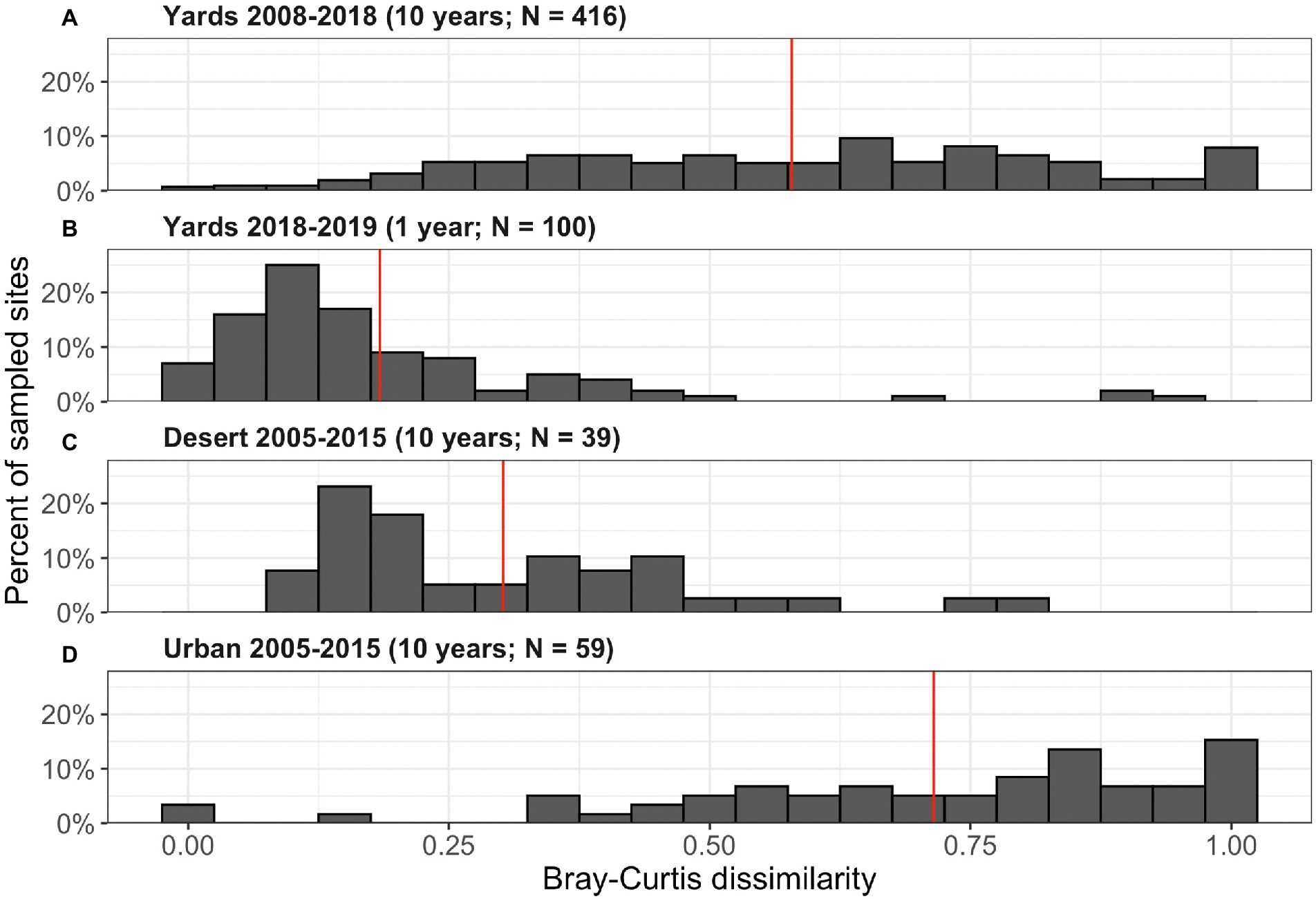
Figure 3. Bray–Curtis dissimilarity of woody vegetation at the genus level in residential yards and in desert and urban plots surveyed as part of the Ecological Survey of Central Arizona. Panels show dissimilarity in (A) residential yards from 2008 to 2018; (B) residential yards from 2018 to 2019; (C) desert plots from 2005 to 2015; and (D) urban plots from 2005 to 2015. Dissimilarity of 1 indicates complete turnover of the woody plant community. Red lines show mean dissimilarity values for each panel. Distributions differ significantly (Two-sample Kolmogorov–Smirnov test; A–B: p < 0.0001; A–C: p < 0.0001; A–D: p = 0.0003; C–D: p < 0.0001; B–C and B–D not tested).
Yard plant richness did not significantly change from 2008 to 2018 (t-test, p = 0.13, N = 416), with a median of seven woody plant genera per yard in both years. There was also no significant change in richness from 2018 to 2019 (t-test, p = 0.09, N = 100), with a median of eight woody plant genera in both years.
The genera that experienced the greatest decline in abundance from 2008 to 2018 were all common garden plants found in the area: Carissa (all Carissa macrocarpa, natal plum), Nerium (all Nerium oleander, oleander), Leucophyllum (multiple species and cultivars), and Bougainvillea (Bougainvillea spectabilis and cultivars, bougainvillea) all declined by more than 100 individuals in 2018 compared to 2008 (Supplementary Figure S4). The genera that increased most in abundance were typical of xeric, low water use landscaping, with at least 100 more Aloe and Agave individuals in 2018 compared to 2008.
In a single year, yards experienced 18% turnover on average, significantly lower than over a 10-year period (Two-sample Kolmogorov–Smirnov test with 100 resampled yards only, D = 0.66, p < 0.0001; Figure 3). However, few yard plant communities remained exactly the same between 2018 and 2019, with only 7% of yards experiencing no change in woody plant composition (Figure 3). Meanwhile, 5% of yards experienced over 50% turnover during this single year.
Urban reference sites experienced 73% woody vegetation turnover in a 10 year period on average, significantly higher than our measured residential yards (Two-sample Kolmogorov–Smirnov test; D = 0.30, p = 0.0003; Figure 3). Desert reference woody plant communities had only 30% community turnover in a 10-year period, significantly lower than both urban reference plots (Two-sample Kolmogorov–Smirnov test; D = 0.75, p < 0.0001; Figure 3) and our sampled residential yards (Two-sample Kolmogorov–Smirnov test; D = 0.52, p < 0.0001).
RQ3: Influence of pulse changes
Out of our sample of 416 yards, 138 were sold between 2008 and 2018 and 98 experienced a change in yard typology (based on dominant ground cover type). 27% of yards that were sold also changed typology, and 38% of yards that changed typology were also sold (37 yards with both changes). Vegetation turnover was higher in yards that experienced these “pulse” changes than in those that did not (Table 3, Figure 4). Yards with changes in typology (e.g., mesic lawn to xeric or bare) experienced significantly higher turnover of woody vegetation compared to yards that did not change typology (OLS; p = 0.002), and homes that had sold in the period from 2008 to 2018 also experienced greater turnover (OLS; p < 0.0001).
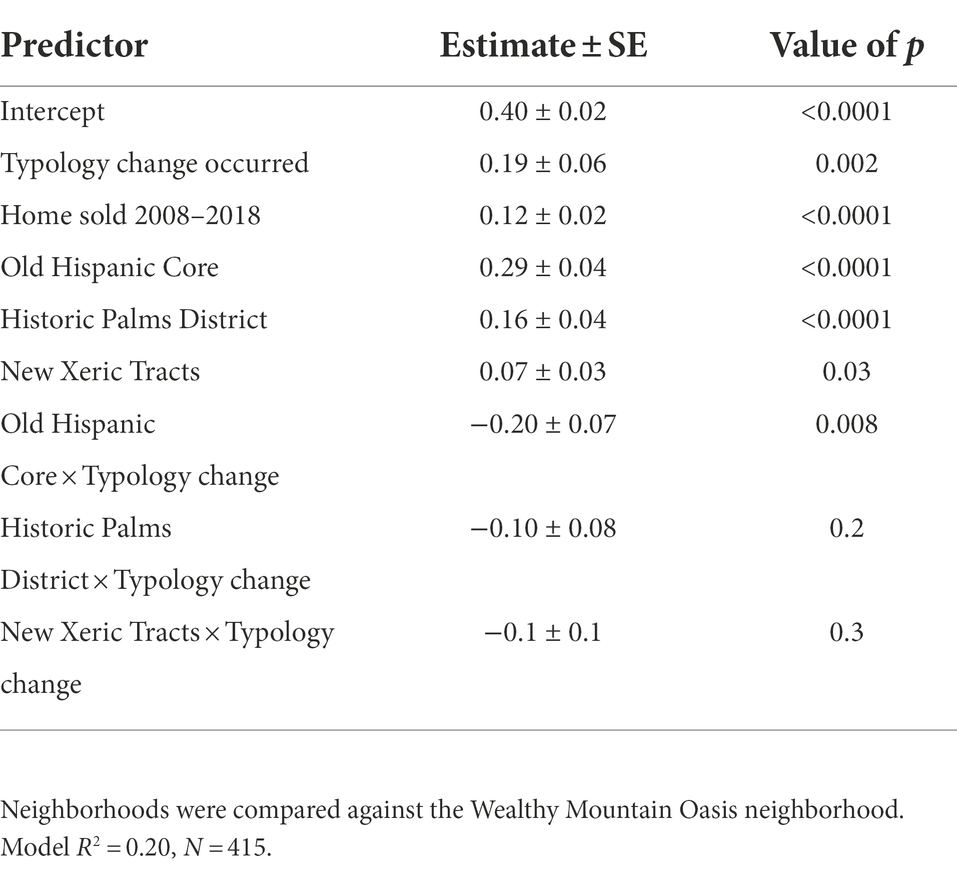
Table 3. Predictors of Bray–Curtis dissimilarity for front yard vegetation communities from 2008 to 2018, including interactions between neighborhood and typology change.
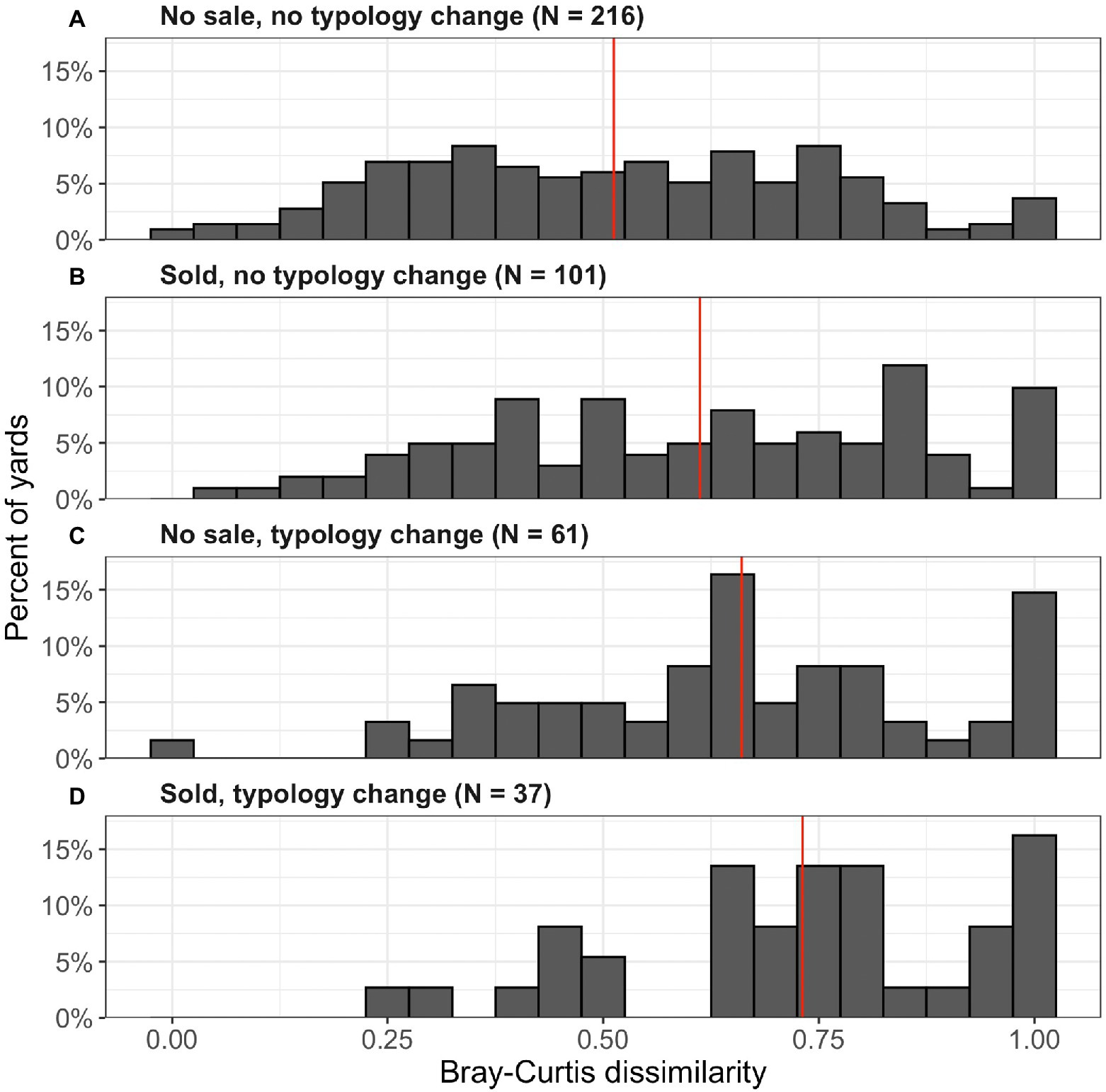
Figure 4. Bray–Curtis dissimilarity of woody vegetation at the genus level for front yards from 2008 to 2018 that have or have not changed ownership or typology from 2008 to 2018. Panels show yards that experienced (A) neither a change in ownership nor a typology change; (B) change in ownership but not typology; (C) change in typology but not ownership; and (D) change in both ownership and typology. Red lines show mean dissimilarity values for each panel.
RQ4: Neighborhood comparisons
Turnover differed by neighborhood, with greater vegetation community dissimilarity over time in the two older and primarily grassy neighborhoods (Old Hispanic Core and Historic Palms District) compared to the newer and primarily xeric neighborhoods (Table 3, Figure 5). Paired neighborhoods with the same dominant landscape type but different socioeconomic characteristics (i.e., Old Hispanic Core vs. Historic Palms District, and Wealthy Mountain Oasis vs. New Xeric Tracts) did not differ significantly in vegetation turnover (Figure 5). Change in yard typology resulted in significantly less vegetation turnover in the Old Hispanic Core compared to the Wealthy Mountain Oasis (OLS; p = 0.008; Table 3).
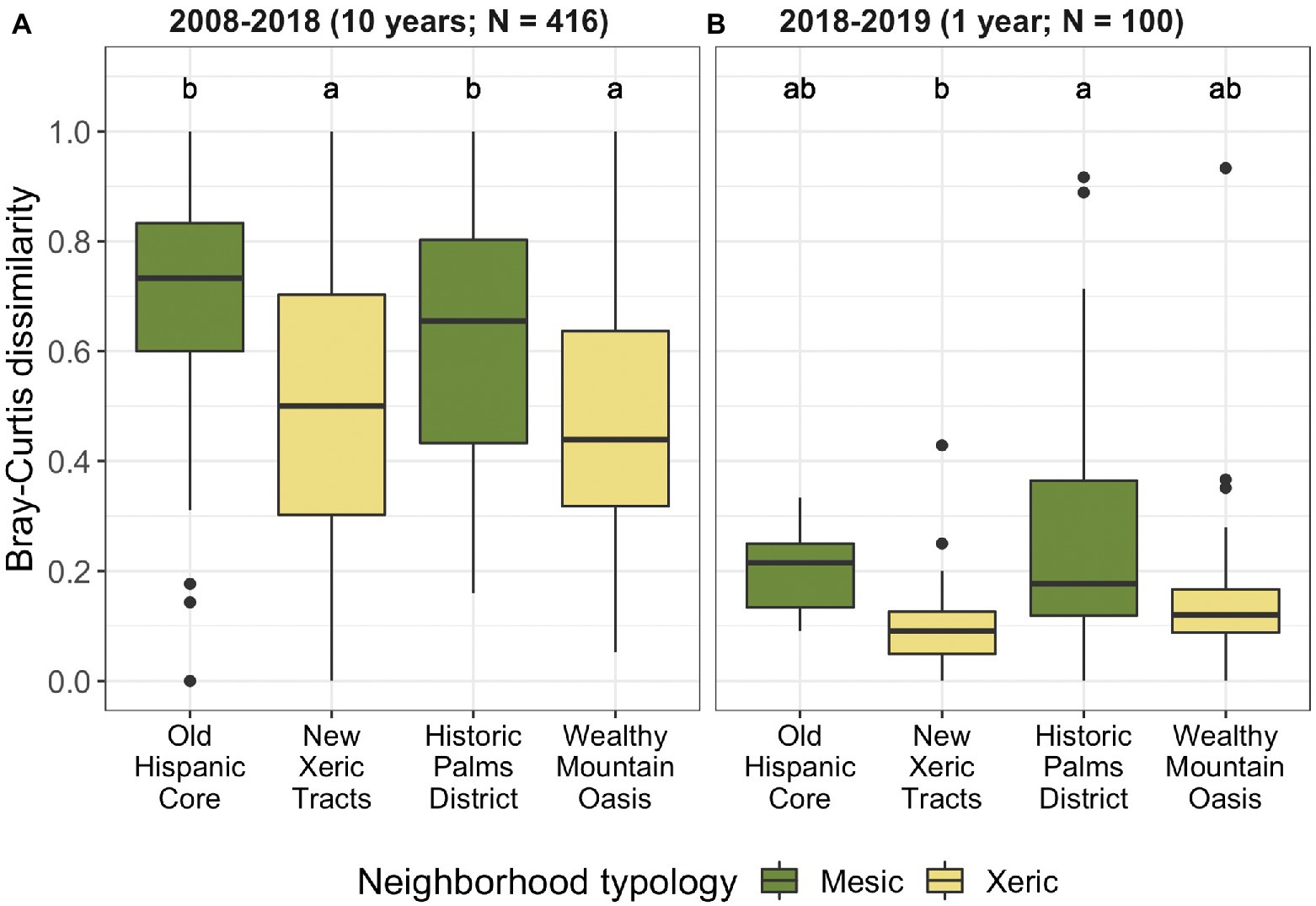
Figure 5. Change in Bray–Curtis dissimilarity of woody vegetation at the genus level by neighborhood from (A) 2008 to 2018 and (B) 2018 to 2019. Annotations above boxes indicate significant differences in dissimilarity between neighborhoods, as determined by Tukey test following OLS modeling. Box colors show the neighborhood dominant typology, either mesic (lawn-dominated) or xeric (desert-like). Neighborhood median income increases along the x-axis.
While genus richness did not change over time, it was significantly lower in the two lower-income neighborhoods (Old Hispanic Core and New Xeric Tracts) compared to the two higher-income neighborhoods (Two-way ANOVA with Tukey post-hoc test; Figure 6; Supplementary Table S4).
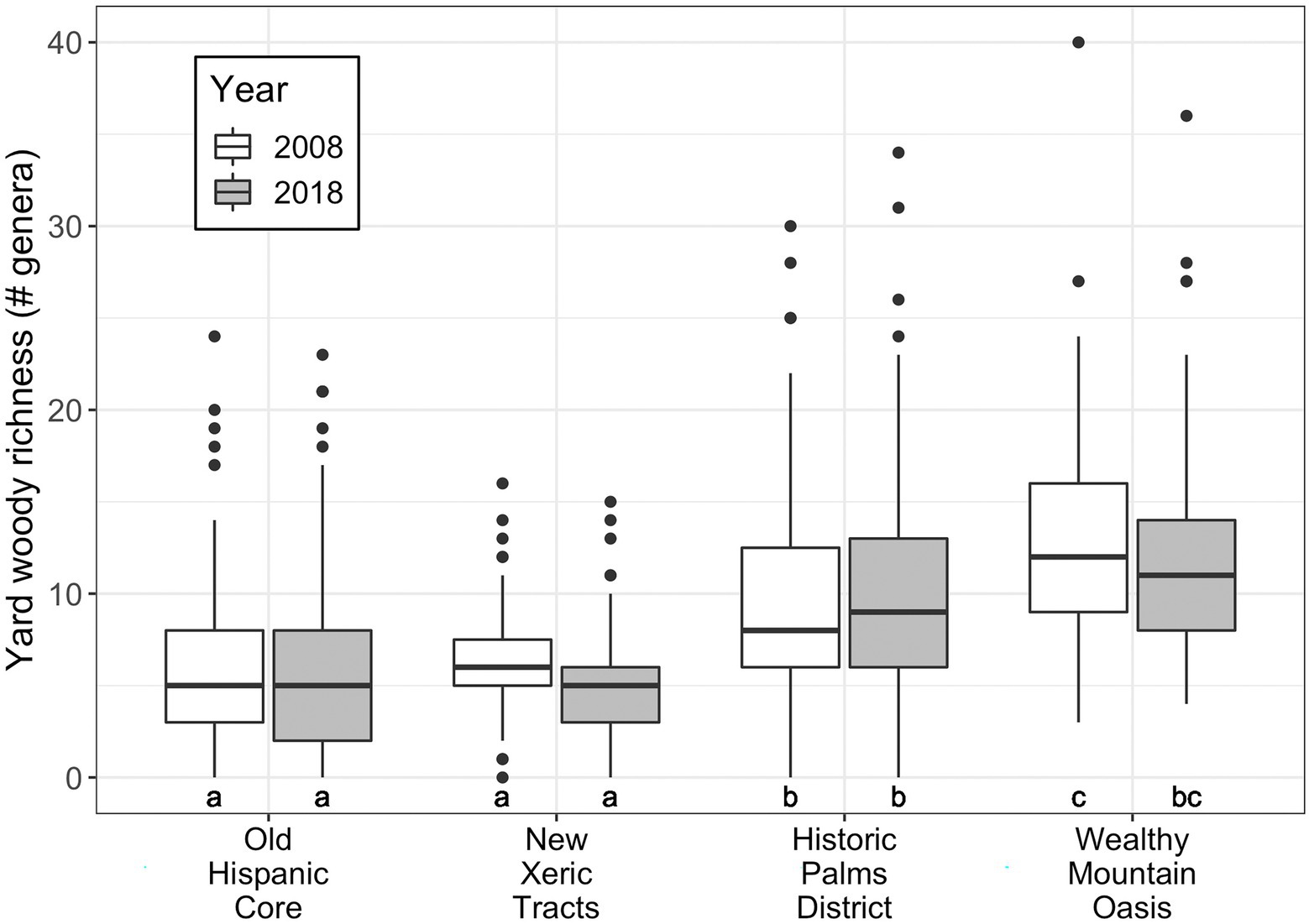
Figure 6. Woody generic richness by neighborhood in 2008 and 2018. Annotations below boxes indicate significant differences in richness between neighborhoods and years, as determined by Tukey test following two-way ANOVA (Supplementary Table S4). Neighborhood median income increases along the x-axis.
Discussion
The residential yard plant communities compared in this study experienced high rates of change relative to natural landscapes, with residents altering even the long-lived woody components of their yards on a decadal and annual time scale. Nearly all residents reported making changes, even those who had not lived in the home for very long, and the measured woody plant communities in their front yards showed the impact of this active management. In a 10-year period, the front yard woody plant communities experienced nearly twice as much turnover as natural desert communities. While previous literature has highlighted the importance of normative pressures and desires for low maintenance in resident landscaping decisions, which we expected to result in low turnover, we did not find static plant communities over time. Instead, we found highly dynamic communities.
One potential explanation for high turnover in the residential plant community compared to native desert is higher plant mortality in cities compared to native ecosystems. Higher urban mortality may be due to the combination of residents’ actions and urban conditions. The unique stressors of the urban environment—such as compacted soil, overwatering, and increased prevalence of disease (Scharenbroch et al., 2005; Tubby and Webber, 2010; Cook et al., 2012; Roman et al., 2014)—can lead to heightened mortality, which could lead residents to replace plants. In addition, urban work has documented relatively short lifespans for trees, especially for street trees (Roman and Scatena, 2011; Widney et al., 2016; Smith et al., 2019). In other studies, residents who removed trees most often reported that the reason to do so was that the tree was dead or in poor health (Conway, 2016; Avolio et al., 2018). Thus, while we did not track individual plant mortality, we expect high mortality of urban woody plant communities is likely a key driver of turnover.
High turnover could also result from residents choosing to plant or remove vegetation regardless of plant health, in response to local norms, personal preferences, or conflict with the desired use of a space (Larson et al., 2009; Kendal et al., 2012b; Kirkpatrick et al., 2013; Conway, 2016). In Phoenix, xeric landscaping is broadly replacing lawns in support of water conservation (Frost, 2016). Thus, the observed removal of typical landscape shrubs like natal plum and oleander, and increase in succulents like aloe and agave, likely reflects a shift in overall landscape trends. While all four of the declining shrubs are drought tolerant and grow well in dry Phoenix landscapes, they are often found in mesic, grassy landscapes as well as more xeric ones. Likewise, aloe species and many agave species planted are not native to the Sonoran Desert, but as succulents may better fit residents’ imagined desert palette for low water use, low maintenance landscapes. The reduction in common shrubs and increase in select succulent genera suggests a slow shift in landscaping fashion over time. Further research should explore the relative importance of mortality and resident choice as mechanisms to explain the high turnover observed.
The pulse events of home sale and yard typology change each increased vegetation turnover in residential yards, supporting the idea that social-ecological pulse events can drive change over time. Additionally, a small number of yards experienced high turnover in a single year, suggesting the occurrence of a pulse of management. This evidence shows the importance of pulse events as another driver of the overall high turnover observed. We also expect that ecological pulse disturbances such as severe storms would result in increased turnover (Conway and Yip, 2016). The impact of pulse disturbances indicates a window of opportunity for yard change, wherein conservation messages such as encouraging residents to plant drought-tolerant or biodiversity-supporting vegetation may be more impactful if aligned with disturbances such as home sales.
Residential vegetation turnover was high, but yard diversity did not significantly change over the year or decade. The relative stability of total genus richness in combination with the observed community turnover suggests that replacement of plants with different taxa is most common, with a similar level of diversity maintained over time in most yards. While yards have not become more diverse over time, they also have not lost species. Thus, inequitable distributions of urban biodiversity throughout an urban area, such that lower income communities are often associated with lower biodiversity, may persist over time in spite of high turnover, at least on a decadal time scale (Clarke et al., 2013).
We found that turnover patterns can be partially explained by neighborhood characteristics, such as dominant landscape type and neighborhood age, but the mechanisms driving change in different neighborhoods deserve more study. The two older, lawn-dominated mesic neighborhoods in our study had higher vegetation turnover than did the two newer, xeric neighborhoods. Neighborhood contexts, including home age and local governance, have often been identified as important predictors of vegetation diversity, density, and cover (Hope et al., 2003; Lowry et al., 2012; Clarke et al., 2013; Aronson et al., 2014). In this study, dominant neighborhood landscape type and neighborhood age covaried, and either factor could drive the observed higher turnover in the mesic, older neighborhoods. One explanation of higher turnover in the mesic-style neighborhoods could be greater planting of species that match the lush character of the landscaping but are unsuitable for the desert climate, leading to high mortality. Alternatively, neighborhood age could drive the observed turnover pattern if replacement of older, outdated landscaping or oversized vegetation leads to greater vegetation change (Kirkpatrick et al., 2013). Regardless of the mechanism, higher turnover in these grassy neighborhoods indicates that the normatively prescribed consistent management of residential lawns does not extend to static management of woody plant communities in these yards. Similarly, the historic restrictions on change in the Historic Palms District did not prevent turnover in the woody plant community. Additional research to distinguish the factors driving change would clarify whether these mechanisms both contribute to turnover or whether one is dominant.
Our findings suggest that while wealthier neighborhoods and residents may have more plant diversity, as expected based on the luxury effect (Hope et al., 2003; Gerrish and Watkins, 2018), a wide range of neighborhoods experience high vegetation turnover. Thus, change is not dependent on residents making expensive updates to their yard landscaping, nor does it arise primarily through neglect and natural turnover. However, similar rates of change may obscure different types of change. For example, typology change in the Old Hispanic Core neighborhood was often of lawn to bare dirt as lawns were allowed to die, which could result in death of other nearby vegetation as well. Meanwhile, in the Historic Palms District, typology conversions were usually of lawn to xeric landscaping, which likely included planting of new desert species. Both instances involve high vegetation turnover, but for different reasons and with different outcomes. Further characterization of the types of change that are dominant in different urban contexts and whether they lead to meaningful shifts in plant community function or ecosystem service provision is an important next step in describing vegetation dynamics in urban spaces.
In this study, we considered only vegetation in residential front yards. However, we expect that patterns of vegetation turnover may be higher in private back yards. Most of our survey respondents reported having made changes to the front yard, but even more had changed the back yard. Management in front and back yards may differ due to the influence of normative pressures and resident use of public front compared to private back yards (Larsen and Harlan, 2006; Nassauer et al., 2009; Larson and Brumand, 2014; Locke et al., 2018; Ossola et al., 2019), which could affect vegetation turnover if dying plants are more quickly removed or replaced in the front, or if desired changes can be more freely made in the back. Additionally, front yards may be constrained by formal regulations, especially for yards regulated by homeowners’ associations (Martin et al., 2003; Harris et al., 2012; Turner and Stiller, 2020). While we did not specifically address the effect of normative constraints or formal regulations in this study, future work comparing front and back yard rates of vegetation change would be well suited to explore the effects of social norms and front yard regulations that may alter vegetation management behavior.
We examined vegetation turnover in a single, semi-arid city and over one long and one short time period, but suggest that these results may be broadly generalizable. Cities in a more mesic environment may see vegetation turnover rates more similar to their respective natural environments, if the urban environment is less extreme in terms of temperature and water availability. However, a study of tree mortality in Boston rural forests compared to urban street trees found much higher mortality among street trees (Smith et al., 2019) and another study estimated average street tree lifespan across a range of cities at 28 years (Roman and Scatena, 2011). These findings suggest that urban vegetation in a variety of climates experiences stressors leading to greater mortality rates. Our study was conducted from 2008 to 2018, a time period corresponding with a major economic downturn (2008–2009). Other research in this region showed vegetation responses to this large-scale event and linked vegetation change to changing economic conditions (Ripplinger et al., 2016, 2017). While we expect that this economic event also influenced our sample sites, we further show non-zero turnover in the year from 2018 to 2019, revealing that vegetation change is not a unique feature of the 2008–2009 period of economic instability. Thus, we suggest that the dynamic community we observed is not unique to this time and place, although further research in other contexts should seek to validate these conclusions.
Research implications
To date, few studies have investigated change over time in urban vegetation outside of urban forestry and land use change. This study highlights fruitful avenues for future work, including exploring how home sales and other major management changes drive turnover in urban plant communities; investigating shifts in urban plant composition over time toward native plants or plants that are perceived as locally appropriate; the role of plant mortality and urban conditions in shaping community dynamics; the generality of the patterns in turnover observed here across climates and types of urban land use; and the impacts of high vegetation turnover on urban ecosystem service provision.
Further research into the drivers of the high plant turnover observed here will help guide actions to harness vegetation change for positive outcomes. Techniques to reduce turnover caused by mortality from poor management, such as educational campaigns and young tree care assistance, can promote increased canopy cover and ecosystem service provision by larger, older trees (Roman et al., 2015). Resident interest in biodiversity support and habitat creation could be put into action through educational and normative efforts to direct change toward expanding ecosystem service provision (van Heezik et al., 2020; Larson et al., 2022). Further research distinguishing the mechanisms driving change and the qualitative nature of changes made over time should inform future incentives, regulations, and programming aimed at driving change in residential landscapes (Pincetl et al., 2019; van Heezik et al., 2020).
In continued research on residential yards, particular attention should be paid to historically disadvantaged communities that may live in neighborhoods with less vegetation, may hold differing values or preferences for yard vegetation, and may be underrepresented in sampling efforts (Landry and Chakraborty, 2009; Yue et al., 2012; Heberlein, 2013; Kirkpatrick et al., 2013). External factors such as plant cost and availability, incentivization programs, and local regulations interact with internal drivers including social norms, personal values, and personal capabilities to shape the paths from preferences to outcomes. With a better understanding of these pathways, conservation measures can direct resident behaviors toward positive change for biodiversity and human wellbeing outcomes, including equitable access to the benefits provided by urban vegetation.
Data availability statement
The datasets presented in this study can be found online at: EDI Data Portal: https://doi.org/10.6073/pasta/a94873b72bce159817469f2b65c183ce.
Ethics statement
The studies involving human participants were reviewed and classified as exempt pursuant to Federal Regulations 45CFR46 by Arizona State University (ID: 662) IRB—Social & Behavioral Research Group. The participants provided their written informed consent to participate in this study.
Author contributions
MW and SH conceptualized the project idea. EC initialized the study and collected 2008 field data. MW collected 2018 and 2019 field data, conducted data analyses, and drafted the initial manuscript. MW and KL designed and implemented the social survey. MW, SH, KL, and EC reviewed and revised the manuscript. All authors contributed to the article and approved the submitted version.
Funding
This material is based upon work supported by the National Science Foundation under grant numbers DEB-1832016, DEB-1637590, DEB-1026865, and DEB-0423704 (Central Arizona-Phoenix Long-Term Ecological Research Program, CAP LTER), and under grant number DEB-1638725 (Alternative Futures for the American Residential Macrosystem). Additional funding from the Arizona State University Graduate & Professional Student Association and from the Lisa Dent Memorial Fellowship also supported this work.
Acknowledgments
We are grateful to the Phoenix residents who returned our surveys and discussed their yards with us during our ecological surveys. Yard surveys were conducted with assistance from Laura Steger, Miranda Bernard, Dena Bergman, Alicia Flores, Brittany Strobel, Michele Clark, Kinley Ragan, Jeff Brown, Julianne Vittal, and Matthew Salem. Thanks to Jeannine Cavender-Bares and Nancy Grimm for early comments on this work. This study was originally presented as part of a dissertation (Wheeler, 2020).
Conflict of interest
The authors declare that the research was conducted in the absence of any commercial or financial relationships that could be construed as a potential conflict of interest.
Publisher’s note
All claims expressed in this article are solely those of the authors and do not necessarily represent those of their affiliated organizations, or those of the publisher, the editors and the reviewers. Any product that may be evaluated in this article, or claim that may be made by its manufacturer, is not guaranteed or endorsed by the publisher.
Supplementary material
The Supplementary material for this article can be found online at: https://www.frontiersin.org/articles/10.3389/fevo.2022.944803/full#supplementary-material
References
Aronson, M. F. J., la Sorte, F. A., Nilon, C. H., Katti, M., Goddard, M. A., Lepczyk, C. A., et al. (2014). A global analysis of the impacts of urbanization on bird and plant diversity reveals key anthropogenic drivers. Proc. R. Soc. B Biol. Sci. 281:20133330. doi: 10.1098/rspb.2013.3330
Avolio, M. L., Pataki, D. E., Pincetl, S., Gillespie, T. W., Jenerette, G. D., and McCarthy, H. R. (2015). Understanding preferences for tree attributes: the relative effects of socio-economic and local environmental factors. Urban Ecosyst. 18, 73–86. doi: 10.1007/s11252-014-0388-6
Avolio, M. L., Pataki, D. E., Trammell, T. L. E., and Endter-Wada, J. (2018). Biodiverse cities: the nursery industry, homeowners, and neighborhood differences drive urban tree composition. Ecol. Monogr. 88, 259–276. doi: 10.1002/ecm.1290
Blaine, T. W., Clayton, S., Robbins, P., and Grewal, P. S. (2012). Homeowner attitudes and practices towards residential landscape management in Ohio, USA. Environ. Manag. 50, 257–271. doi: 10.1007/s00267-012-9874-x
Burr, A., Hall, D. M., and Schaeg, N. (2018). The perfect lawn: exploring neighborhood socio-cultural drivers for insect pollinator habitat. Urban Ecosyst. 21, 1123–1137. doi: 10.1007/s11252-018-0798-y
Childers, D. L., Grimm, N. B., Hope, D., Kaye, J., Martin, C., McIntrye, N., et al. (2018). Ecological Survey of Central Arizona: A Survey of Key Ecological Indicators in the Greater Phoenix Metropolitan Area and Surrounding Sonoran Desert, Ongoing Since 1999. Available at: https://sustainability.asu.edu/caplter/data/view/knb-lter-cap.652/ (Accessed December 13, 2019).
Clarke, L. W., Jenerette, G. D., and Davila, A. (2013). The luxury of vegetation and the legacy of tree biodiversity in Los Angeles, CA. Landsc. Urban Plan. 116, 48–59. doi: 10.1016/j.landurbplan.2013.04.006
Clements, F. E. (1916). Plant Succession: An Analysis of the Development of Vegetation. Washington: Carnegie Institution of Washington.
Collins, S. L., Carpenter, S. R., Swinton, S. M., Orenstein, D. E., Childers, D. L., Gragson, T. L., et al. (2011). An integrated conceptual framework for long-term social-ecological research. Front. Ecol. Environ. 9, 351–357. doi: 10.1890/100068
Conway, T. M. (2016). Tending their urban forest: residents’ motivations for tree planting and removal. Urban For. Urban Green. 17, 23–32. doi: 10.1016/j.ufug.2016.03.008
Conway, T. M., and Yip, V. (2016). Assessing residents’ reactions to urban forest disservices: a case study of a major storm event. Landsc. Urban Plan. 153, 1–10. doi: 10.1016/j.landurbplan.2016.04.016
Cook, E. M., Hall, S. J., and Larson, K. L. (2012). Residential landscapes as social-ecological systems: a synthesis of multi-scalar interactions between people and their home environment. Urban Ecosyst. 15, 19–52. doi: 10.1007/s11252-011-0197-0
Dearborn, D. C., and Kark, S. (2010). Motivations for conserving urban biodiversity. Conserv. Biol. 24, 432–440. doi: 10.1111/j.1523-1739.2009.01328.x
Dolan, R. W., Moore, M. E., and Stephens, J. D. (2011). Documenting effects of urbanization on flora using herbarium records. J. Ecol. 99, 1055–1062. doi: 10.1111/j.1365-2745.2011.01820.x
Drescher, M., Warriner, G. K., Farmer, J. R., and Larson, B. M. H. (2017). Private landowners and environmental conservation: A case study of socialpsychological determinants of conservation program participation in Ontario. Ecol. Soc. 22. doi: 10.5751/ES-09118-220144
Foley, J. A., DeFries, R., Asner, G. P., Barford, C., Bonan, G., Carpenter, S. R., et al. (2005). Global consequences of land use. Science 309, 570–574. doi: 10.1126/science.1111772
Gaston, K. J., Ávila-Jiménez, M. L., and Edmondson, J. L. (2013). Managing urban ecosystems for goods and services. J. Appl. Ecol. 50, 830–840. doi: 10.1111/1365-2664.12087
Gerrish, E., and Watkins, S. L. (2018). The relationship between urban forests and income: a meta-analysis. Landsc. Urban Plan. 170, 293–308. doi: 10.1016/j.landurbplan.2017.09.005
Groffman, P. M., Avolio, M., Cavender-Bares, J., Bettez, N. D., Grove, J. M., Hall, S. J., et al. (2017). Ecological homogenization of residential macrosystems. Nature Ecol. Evol. 1:0191. doi: 10.1038/s41559-017-0191
Grove, J. M., Locke, D. H., and O’Neil-Dunne, J. P. M. (2014). An ecology of prestige in new York City: examining the relationships among population density, socio-economic status, group identity, and residential canopy cover. Environ. Manag. 54, 402–419. doi: 10.1007/s00267-014-0310-2
Guo, T., Morgenroth, J., and Conway, T. (2018). Redeveloping the urban forest: the effect of redevelopment and property-scale variables on tree removal and retention. Urban For. Urban Green. 35, 192–201. doi: 10.1016/j.ufug.2018.08.012
Harris, E. M., Polsky, C., Larson, K. L., Garvoille, R., Martin, D. G., Brumand, J., et al. (2012). Heterogeneity in residential yard care: evidence from Boston, Miami, and Phoenix. Hum. Ecol. 40, 735–749. doi: 10.1007/s10745-012-9514-3
Hartig, T., Mitchell, R., de Vries, S., and Frumkin, H. (2014). Nature and health. Annu. Rev. Public Health 35, 207–228. doi: 10.1146/annurev-publhealth-032013-182443
Heberlein, T. A. (2013). Navigating environmental attitudes. Conserv. Biol. 26, 583–585. doi: 10.1093/acprof:oso/9780199773329.001.0001
Hirt, P., Gustafson, A., and Larson, K. L. (2008). The mirage in the valley of the sun. Environ. Hist. 13, 482–514.
Hope, D., Gries, C., Zhu, W., Fagan, W. F., Redman, C. L., Grimm, N. B., et al. (2003). Socioeconomics drive urban plant diversity. PNAS Nexus 100, 8788–8792. doi: 10.1073/pnas.1537557100
Hunter, M. C. R., and Brown, D. G. (2012). Spatial contagion: gardening along the street in residential neighborhoods. Landsc. Urban Plan. 105, 407–416. doi: 10.1016/j.landurbplan.2012.01.013
Jenerette, G. D., Harlan, S. L., Stefanov, W. L., and Martin, C. A. (2011). Ecosystem services and urban heat riskscape moderation: water, green spaces, and social inequality in Phoenix, USA. Ecol. Appl. 21, 2637–2651. doi: 10.1890/10-1493.1
Johnson, A. L., Borowy, D., and Swan, C. M. (2018). Land use history and seed dispersal drive divergent plant community assembly patterns in urban vacant lots. J. Appl. Ecol. 55, 451–460. doi: 10.1111/1365-2664.12958
Kendal, D., Williams, K. J. H., and Williams, N. S. G. (2012a). Plant traits link people’s plant preferences to the composition of their gardens. Landsc. Urban Plan. 105, 34–42. doi: 10.1016/j.landurbplan.2011.11.023
Kendal, D., Williams, N. S. G., and Williams, K. J. H. (2012b). A cultivated environment: exploring the global distribution of plants in gardens, parks and streetscapes. Urban Ecosyst. 15, 637–652. doi: 10.1007/s11252-011-0215-2
Kirkpatrick, J. B., Davison, A., and Daniels, G. D. (2013). Sinners, scapegoats or fashion victims? Understanding the deaths of trees in the green city. Geoforum 48, 165–176. doi: 10.1016/j.geoforum.2013.04.018
Ko, Y., Lee, J. H., McPherson, E. G., and Roman, L. A. (2015). Factors affecting long-term mortality of residential shade trees: evidence from Sacramento, California. Urban For. Urban Green. 14, 500–507. doi: 10.1016/j.ufug.2015.05.002
Landry, S. M., and Chakraborty, J. (2009). Street trees and equity: evaluating the spatial distribution of an urban amenity. Environ Plan A 41, 2651–2670. doi: 10.1068/a41236
Larsen, L., and Harlan, S. L. (2006). Desert dreamscapes: residential landscape preference and behavior. Landsc. Urban Plan. 78, 85–100. doi: 10.1016/j.landurbplan.2005.06.002
Larson, K. L., and Brumand, J. (2014). Paradoxes in landscape management and water conservation: examining neighborhood norms and institutional forces. Cities Environ. 7:6
Larson, K. L., Casagrande, D., Harlan, S. L., and Yabiku, S. T. (2009). Residents’ yard choices and rationales in a desert city: social priorities, ecological impacts, and decision tradeoffs. Environ. Manag. 44, 921–937. doi: 10.1007/s00267-009-9353-1
Larson, K. L., Cook, E., Strawhacker, C., and Hall, S. J. (2010). The influence of diverse values, ecological structure, and geographic context on residents’ multifaceted landscaping decisions. Hum. Ecol. 38, 747–761. doi: 10.1007/s10745-010-9359-6
Larson, K. L., Hoffman, J., and Ripplinger, J. (2017a). Legacy effects and landscape choices in a desert city. Landsc. Urban Plan. 165, 22–29. doi: 10.1016/j.landurbplan.2017.04.014
Larson, K. L., Lerman, S. B., Nelson, K. C., Narango, D. L., Wheeler, M. M., Groffman, P. M., et al. (2022). Examining the potential to expand wildlife-supporting residential yards and gardens. Landsc Urban Plan 222:104396. doi: 10.1016/j.landurbplan.2022.104396
Larson, K. L., Andrade, R., York, A., Childers, D., Coseo, P., Earl, S., et al. (2017b). “The Phoenix area social survey IV: Linking social and biophysical dynamics in urban neighborhoods,” in A Report from the Central Arizona–Phoenix Long-Term Ecological Research (CAP LTER) Project. Tempe, Arizona.
Leong, M., Dunn, R. R., and Trautwein, M. D. (2018). Biodiversity and socioeconomics in the city: a review of the luxury effect. Biol. Lett. 14:20180082. doi: 10.1098/rsbl.2018.0082
Locke, D. H., Avolio, M., Trammell, T. L. E., Roy Chowdhury, R., Morgan Grove, J., Rogan, J., et al. (2018). A multi-city comparison of front and backyard differences in plant species diversity and nitrogen cycling in residential landscapes. Landsc. Urban Plan. 178, 102–111. doi: 10.1016/j.landurbplan.2018.05.030
Loram, A., Tratalos, J., Warren, P. H., and Gaston, K. J. (2007). Urban domestic gardens (X): the extent & structure of the resource in five major cities. Landsc. Ecol. 22, 601–615. doi: 10.1007/s10980-006-9051-9
Lowry, J. H., Baker, M. E., and Ramsey, R. D. (2012). Determinants of urban tree canopy in residential neighborhoods: household characteristics, urban form, and the geophysical landscape. Urban Ecosyst. 15, 247–266. doi: 10.1007/s11252-011-0185-4
Martin, C. A., Peterson, K. A., and Stabler, L. B. (2003). Residential landscaping in Phoenix, Arizona, U.S.: practices and preferences relative to covenants, codes, and restrictions. J. Arboric. 29, 9–17.
Mathieu, R., Freeman, C., and Aryal, J. (2007). Mapping private gardens in urban areas using object-oriented techniques and very high-resolution satellite imagery. Landsc. Urban Plan. 81, 179–192. doi: 10.1016/j.landurbplan.2006.11.009
Nassauer, J. I., Wang, Z., and Dayrell, E. (2009). What will the neighbors think? Cultural norms and ecological design. Landsc. Urban Plan. 92, 282–292. doi: 10.1016/j.landurbplan.2009.05.010
Nelson, R. K., Winling, L., Marciano, R., and Connolly, N. (2020). Mapping Inequality, American Panorama. Available at: https://dsl.richmond.edu/panorama/redlining/#loc=12/33.463/-112.152&city=phoenix-az (Accessed May 6, 2020).
Nesbitt, L., Hotte, N., Barron, S., Cowan, J., and Sheppard, S. R. J. (2017). The social and economic value of cultural ecosystem services provided by urban forests in North America: a review and suggestions for future research. Urban For. Urban Green. 25, 103–111. doi: 10.1016/j.ufug.2017.05.005
NOAA (2019a) Data Tools: 1981–2010 Normals. National Climate Data Center. Available at: https://www.ncdc.noaa.gov/cdo-web/datatools/normals (Accessed April 18, 2019).
NOAA (2019b) Extreme Temperature Facts for Phoenix and Yuma. National Weather Service. Available at: https://www.wrh.noaa.gov/psr/climate/extremeTemps.php (Accessed April 18, 2019).
Nowak, D. J., and Greenfield, E. J. (2012). Tree and impervious cover change in U.S. cities. Urban For. Urban Green. 11, 21–30. doi: 10.1016/j.ufug.2011.11.005
Nowak, D. J., and Greenfield, E. J. (2018). Declining urban and community tree cover in the United States. Urban For. Urban Green. 32, 32–55. doi: 10.1016/j.ufug.2018.03.006
Nowak, D. J., and Walton, J. T. (2005). Projected urban growth (2000-2050) and its estimated impact on the US forest resource. J. For. 103, 383–389. doi: 10.1093/jof/103.8.383
Ossola, A., Locke, D., Lin, B., and Minor, E. (2019). Greening in style: urban form, architecture and the structure of front and backyard vegetation. Landsc. Urban Plan. 185, 141–157. doi: 10.1016/j.landurbplan.2019.02.014
Pearsall, H., and Christman, Z. (2012). Tree-lined lanes or vacant lots? Evaluating non-stationarity between urban greenness and socio-economic conditions in Philadelphia, Pennsylvania, USA at multiple scales. Appl. Geogr. 35, 257–264. doi: 10.1016/j.apgeog.2012.07.006
Pickett, S. T. A., Cadenasso, M. L., and Meiners, S. J. (2009). Ever since Clements: from succession to vegetation dynamics and understanding to intervention. Appl. Veg. Sci. 12, 9–21.
Pincetl, S., Gillespie, T. W., Pataki, D. E., Porse, E., Jia, S., Kidera, E., et al. (2019). Evaluating the effects of turf-replacement programs in Los Angeles. Landsc. Urban Plan. 185, 210–221. doi: 10.1016/J.LANDURBPLAN.2019.01.011
Pulsford, S. A., Lindenmayer, D. B., and Driscoll, D. A. (2016). A succession of theories: purging redundancy from disturbance theory. Biol. Rev. 91, 148–167. doi: 10.1111/brv.12163
R Core Team (2019) ‘R: A Language and Environment for Statistical Computing.’ Vienna, Austria: R Foundation for Statistical Computing. Available at: https://www.r-project.org/
Ripplinger, J., Collins, S. L., York, A. M., and Franklin, J. (2017). Boom-bust economics and vegetation dynamics in a desert city: how strong is the link? Ecosphere 8:e01826. doi: 10.1002/ecs2.1826
Ripplinger, J., Franklin, J., and Collins, S. L. (2016). When the economic engine stalls – a multi-scale comparison of vegetation dynamics in pre- and post-recession Phoenix, Arizona, USA. Landsc. Urban Plan. 153, 140–148. doi: 10.1016/j.landurbplan.2016.05.009
Robbins, P. (2007). Lawn people: How grasses, weeds, and chemicals make us who we are. Philadelphia, PA: Temple University Press.
Rogers, D. A., Rooney, T. P., Hawbaker, T. J., Radeloff, V. C., and Waller, D. M. (2009). Paying the extinction debt in southern Wisconsin forest understories. Conserv. Biol. 23, 1497–1506. doi: 10.1111/j.1523-1739.2009.01256.x
Roman, L. A., Battles, J. J., and McBride, J. R. (2014). Determinants of establishment survival for residential trees in Sacramento County, CA. Landsc. Urban Plan. 129, 22–31. doi: 10.1016/j.landurbplan.2014.05.004
Roman, L. A., Fristensky, J. P., Eisenman, T. S., Greenfield, E. J., Lundgren, R. E., Cerwinka, C. E., et al. (2017). Growing canopy on a college campus: understanding urban forest change through archival records and aerial photography. Environ. Manag. 60, 1042–1061. doi: 10.1007/s00267-017-0934-0
Roman, L. A., Pearsall, H., Eisenman, T. S., Conway, T. M., Fahey, R. T., Landry, S., et al. (2018). Human and biophysical legacies shape contemporary urban forests: a literature synthesis. Urban For. Urban Green. 31, 157–168. doi: 10.1016/j.ufug.2018.03.004
Roman, L. A., and Scatena, F. N. (2011). Street tree survival rates: meta-analysis of previous studies and application to a field survey in Philadelphia, PA, USA. Urban For. Urban Green. 10, 269–274. doi: 10.1016/j.ufug.2011.05.008
Roman, L. A., Walker, L. A., Martineau, C. M., Muffly, D. J., MacQueen, S. A., and Harris, W. (2015). Stewardship matters: case studies in establishment success of urban trees. Urban For. Urban Green. 14, 1174–1182. doi: 10.1016/j.ufug.2015.11.001
Scharenbroch, B. C., Lloyd, J. E., and Johnson-Maynard, J. L. (2005). Distinguishing urban soils with physical, chemical, and biological properties. Pedobiologia 49, 283–296. doi: 10.1016/j.pedobi.2004.12.002
Seto, K. C., Fragkias, M., Güneralp, B., and Reilly, M. K. (2011). A meta-analysis of global urban land expansion. PLoS One 6:e23777. doi: 10.1371/journal.pone.0023777
Smith, I. A., Dearborn, V. K., and Hutyra, L. R. (2019). Live fast, die young: accelerated growth, mortality, and turnover in urban street trees. PLoS One 14:e0215846. doi: 10.1371/journal.pone.0215846
The Plant List (2013). The Plant List. Version 1.1, Internet. Available at: http://www.theplantlist.org/
Tubby, K. V., and Webber, J. F. (2010). Pests and diseases threatening urban trees under a changing climate. Forestry 83, 451–459. doi: 10.1093/forestry/cpq027
Turner, V. K., and Stiller, M. (2020). How do homeowners associations regulate residential landscapes? An analysis of rule structure and content in Maricopa County (AZ). J. Am. Plan. Assoc. 86, 25–38. doi: 10.1080/01944363.2019.1665474
U.S. Census Bureau (2019). ‘New Census Bureau Estimates Show Counties in South and West Lead Nation in Population Growth’. Available at: https://www.census.gov/newsroom/press-releases/2019/estimates-county-metro.html
van Heezik, Y., Freeman, C., Davidson, K., and Lewis, B. (2020). Uptake and engagement of activities to promote native species in private gardens. Environ. Manag. 66, 42–55. doi: 10.1007/s00267-020-01294-5
Verplanken, B., and Roy, D. (2016). Empowering interventions to promote sustainable lifestyles: testing the habit discontinuity hypothesis in a field experiment. J. Environ. Psychol. 45, 127–134. doi: 10.1016/j.jenvp.2015.11.008
Walker, J. S., Grimm, N. B., Briggs, J. M., Gries, C., and Dugan, L. (2009). Effects of urbanization on plant species diversity in Central Arizona. Front. Ecol. Environ. 7, 465–470. doi: 10.1890/080084
Wheeler, M. M. (2020). Urban Ecology for Conservation: Evaluating Social and Ecological Drivers of Biodiversity Change Over Time. Tempe, Arizona: Arizona State University.
Wheeler, M. M., Cook, E. M., Larson, K. L., and Hall, S. J. (2022b). “Phoenix, Arizona (USA) residential yard management and vegetation change over time: 2008-2019,” in Environmental Data Initiative, p. ver. 1. doi: 10.6073/pasta/a94873b72bce159817469f2b65c183ce
Wheeler, M. M., Larson, K. L., and Andrade, R. (2020). ‘Attitudinal and structural drivers of preferred versus actual residential landscapes in a desert city’, urban ecosystems. Urban Ecosyst. 23, 659–673. doi: 10.1007/s11252-020-00928-0
Wheeler, M. M., Larson, K. L., Bergman, D., and Hall, S. J. (2022a). Environmental attitudes predict native plant abundance in residential yards. Landsc Urban Plan 224:104443. doi: 10.1016/j.landurbplan.2022.104443
Widney, S., Fischer, B. C., and Vogt, J. (2016). Tree mortality undercuts ability of tree-planting programs to provide benefits: results of a three-city study. Forests 7:65. doi: 10.3390/f7030065
Yue, C., Hurley, T., and Anderson, N. O. (2012). Heterogeneous consumer preferences for native and invasive plants: evidence from experimental auctions. HortScience 47, 1091–1095. doi: 10.21273/HORTSCI.47.8.1091
Ziter, C., Graves, R. A., and Turner, M. G. (2017). How do land-use legacies affect ecosystem services in United States cultural landscapes? Landsc. Ecol. 32, 2205–2218. doi: 10.1007/s10980-017-0545-4
Keywords: environmental change, conservation, residential yards, ecosystem dynamics, urban
Citation: Wheeler MM, Larson KL, Cook EM and Hall SJ (2022) Residents manage dynamic plant communities: Change over time in urban vegetation. Front. Ecol. Evol. 10:944803. doi: 10.3389/fevo.2022.944803
Edited by:
Salman Qureshi, Humboldt University of Berlin, GermanyReviewed by:
Meghan Avolio, Johns Hopkins University, United StatesLea Johnson, Longwood Gardens, United States
Copyright © 2022 Wheeler, Larson, Cook and Hall. This is an open-access article distributed under the terms of the Creative Commons Attribution License (CC BY). The use, distribution or reproduction in other forums is permitted, provided the original author(s) and the copyright owner(s) are credited and that the original publication in this journal is cited, in accordance with accepted academic practice. No use, distribution or reproduction is permitted which does not comply with these terms.
*Correspondence: Megan M. Wheeler, bW13aGVlbGVyM0BnbWFpbC5jb20=