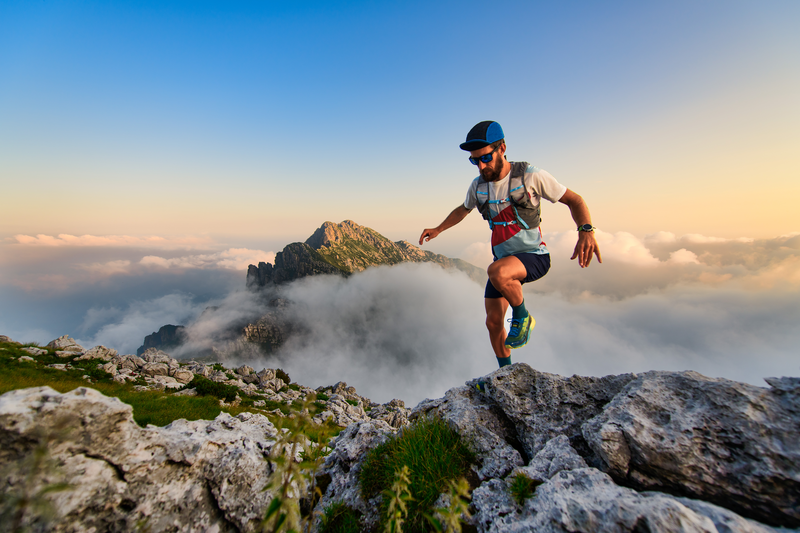
95% of researchers rate our articles as excellent or good
Learn more about the work of our research integrity team to safeguard the quality of each article we publish.
Find out more
ORIGINAL RESEARCH article
Front. Ecol. Evol. , 25 July 2022
Sec. Conservation and Restoration Ecology
Volume 10 - 2022 | https://doi.org/10.3389/fevo.2022.940254
This article is part of the Research Topic Biodiversity Conservation and Ecological Function Restoration in Freshwater Ecosystems View all 16 articles
Many streams and rivers are heterotrophic ecosystems that are highly dependent on cross-ecosystem subsidies such as leaf litter (LL). Terrestrial LL can be consumed by macroinvertebrates and microbes to fuel the detrital-based food webs in freshwaters. To date, our knowledge of LL decomposition in freshwaters is largely based on broadleaved LL, while the patterns and drivers of coniferous leaf-litter (CLL) decomposition in streams and rivers remain poorly understood. Here, we present a global investigation of CLL decomposition in streams and rivers by collecting data from 35 publications. We compared LL breakdown rates in this study with other global-scale studies (including conifers and broadleaved species), between evergreen and deciduous conifers, and between native and invasive conifers. We also investigated the climatic, geographic (latitude and altitude), stream physicochemical characteristics, and experimental factors (e.g., mesh size and experimental duration) in influencing CLL decomposition. We found that the following: (1) LL breakdown rates in this study were 18.5–28.8 and 4.9–16.8% slower than those in other global-scale studies when expressed as per day and per degree day, respectively. Conifer LL in coarse mesh bags, for evergreen and invasive conifers, decomposed 13.6, 10.3, and 10.8% faster than in fine mesh bags, for deciduous and native conifers, respectively; (2) CLL traits, stream physicochemical characteristics, and experimental factors explained higher variations in CLL decomposition than climatic and geographic factors; (3) CLL nutritional quality (N and P), water temperature, and experimental duration were better predictors of CLL decomposition than other predictors in categories of LL traits, stream physicochemical characteristics, and experimental factors, respectively; and (4) total and microbial-mediated CLL breakdown rates showed linear relationships with latitude, altitude, mean annual temperature, and mean annual precipitation. Our results imply that the replacement of native forests by conifer plantation would impose great impacts on adjacent freshwaters by retarding the LL processing rate. Moreover, future climate warming which is very likely to happen in mid- and high-latitude areas according to the IPCC 6th report would accelerate LL decomposition, with a potential consequence of food depletion for detritivores in freshwaters during hot summers.
Leaf-litter (LL) decomposition is one fundamental ecological functioning that links nutrients cycling, food web structure, and energy transfer within and across ecosystems (Anderson and Sedell, 1979; Wallace et al., 1997; Manning et al., 2021). The input of terrestrial LL to freshwaters imposes great impacts on individuals, populations, and communities of macroinvertebrates and microbes (Graça, 2001; Hayer et al., 2022), which can translate the C stored in LL into coarse particulate organic matter and CO2 (Marks, 2019). An increasing number of studies advanced our understanding of LL decomposition in freshwater ecosystems. Many biotic (e.g., LL traits, shredders, and fungi) and abiotic (e.g., water temperature, dissolved oxygen (DO), and latitude) factors have been identified as key drivers of LL decomposition in freshwaters at local, regional, and global scales (Woodward et al., 2012; Tiegs et al., 2019; Xiang et al., 2022). However, most previous studies focused on broadleaved species and ignored the decomposition of coniferous leaf litter (CLL) in freshwaters. For example, Follstad Shah et al. (2017) investigated the temperature sensitivity of LL decomposition in streams at a global scale in which CLL represents only 2.8% of all the cases. On the contrary, the decomposition of CLL in terrestrial ecosystems has been intensively investigated, especially for Pinus, which is the most well-studied genus than any other genus (Berg, 2014).
More studies should be conducted on investigating CLL decomposition in freshwaters, considering that many conifers are globally distributed species; conifers are common and dominant species in many mid- and high-latitude areas (e.g., temperate forests), and coniferous plantation is among one of the biggest forest changes that have invaded in many regions (Richardson and Rejmánek, 2004; Essl et al., 2010; Ferreira et al., 2016). Coniferous LL differs from broadleaf species in many ways such as nutritional quality and LL input time, and the shift between conifers and broadleaf species may have great impacts on communities of macroinvertebrates and microbes (Riipinen et al., 2010; Kanasashi and Hattori, 2011; Ferreira et al., 2017). The lack of information on CLL decomposition in freshwaters is, therefore, unrealistic and may lead to biased results when investigating general patterns of LL decomposition in freshwaters on a global scale. In addition, CLL naturally differs from broadleaved LL in ways of leaf morphology, nutrient concentration (e.g., N and P), chemical (e.g., lignin) and physical (e.g., toughness) characteristics, and, especially, the nutritional quality, which is lower than broadleaved LL (Martínez et al., 2013; Balibrea et al., 2020). Leaf-litter traits refer to characteristics that have correlations with LL decomposability or palatability, which include nutrient concentrations and chemical and physical characteristics in this study. Lower quality (e.g., higher lignin) of LL may influence the responses of LL decomposition to other factors such as water temperature and macroinvertebrate communities (Leroy and Marks, 2006; Fernandes et al., 2012). Therefore, the global patterns and drivers of LL decomposition found in previous large-scale studies may not be applicable for CLL.
To the best of our knowledge, there is no global-scale study of CLL decomposition in freshwaters. However, experiences gained from previous studies may help us predict some general patterns. Leaf-litter traits such as lignin and N have long been acknowledged as driving predictors of LL decomposition in freshwaters (Boyero et al., 2016; Kennedy and El-Sabaawi, 2017; Zhang et al., 2019). Coniferous LL is a low-quality food resource for microbes and detritivores due to the high concentration of lignin and low concentration of N (Richardson et al., 2004). Therefore, we should expect slower LL breakdown rates of conifers than broadleaf species in freshwaters (Graça and Pereira, 1995; Albariño and Balseiro, 2002). However, there are no consistent results regarding which traits are the most important predictors of LL decomposition. Leaf-litter decomposition also has been shown to change along the latitudinal gradient: total, macroinvertebrate-mediated, and microbial-mediated LL decomposition decreased when approaching the poles (Follstad Shah et al., 2017; Boyero et al., 2021) or no significant change of total LL decomposition with increasing absolute latitude (Irons III et al., 1994). These latitudinal patterns of LL decomposition in streams and rivers are usually associated with changes in mean annual temperature (MAT), microbes (e.g., fungi), and macroinvertebrates: lower MAT toward the poles, the highest abundance and richness of fungi at mid-latitude areas, and increasing abundance and richness of shredders toward the poles (Boyero et al., 2011a; Seena et al., 2019). Conifers are mainly distributed in mid- and high-latitude areas (Essl et al., 2010) where the abundance and richness of macroinvertebrates and microbes are relatively high and MAT is low (Seena et al., 2019). Therefore, the latitudinal trend of CLL decomposition may differ from the reported global patterns that included a low proportion of conifers and had a wider distribution range of sampling sites.
Stream physicochemical characteristics such as water temperature and water nutrient concentrations are essential abiotic factors driving LL decomposition in streams (Boyero et al., 2011b; Woodward et al., 2012). A growing number of studies addressed the importance of water temperature in driving LL decomposition in freshwaters (Bernabé et al., 2018; Fenoy et al., 2022). However, most studies suggested accelerated total, microbial-mediated, and macroinvertebrate-mediated LL breakdown rates under warmer temperature conditions (e.g., 1–4°C higher) due to benefited individuals and communities of microbes and detritivores (e.g., stimulated activities and higher density) (Boyero et al., 2011b; Ferreira et al., 2015b; Griffiths and Tiegs, 2016). Some others found no or negative effects of increased water temperature on LL decomposition through the inhibition effects on communities of macroinvertebrates and microbes (Bernabé et al., 2018; Peréz et al., 2021). However, the higher water temperature may reduce the negative effects of LL quality on LL decomposition (Fernandes et al., 2012). Therefore, the observed relationships between water temperature and broadleaved LL breakdown rates may be different from that of conifers due to the lower quality (e.g., higher lignin). Water nutrient concentrations are also closely correlated with LL decomposition in freshwaters. Nutrient concentrations (e.g., NO- 3 and ) in freshwaters worldwide have been increasing and will continue to increase due to intensive anthropogenic activities, such as growing fertilizer and sewage input (Vilmin et al., 2018; Beusen et al., 2022). The responses of LL decomposition to increasing water nutrient concentrations (e.g., NO- 3 and ) may be hump shaped (Woodward et al., 2012) or positive, and this effect was stronger for nutrient-poor LL (e.g., low N) than for nutrient-rich LL (Ferreira et al., 2015a). In addition, nutrient enrichment significantly decreased macroinvertebrate abundance in freshwaters, and this effect was stronger in tropical than in temperate areas (Nessel et al., 2021). Because CLL has lower quality than broadleaved LL, conifers are mainly distributed in temperate regions. Therefore, the relationships between CLL decomposition and water nutrient concentrations may differ from that of broadleaf species.
Here, we collected data on CLL breakdown rates in streams and rivers with associated climatic (MAP, mean annual precipitation; MDTR, mean diurnal temperature range, and MAT), geographic (altitude and latitude), experimental (mesh size, duration, and initial LL mass), and stream physicochemical (e.g., water depth, NO- 3, and ) factors from 35 publications (Supplementary Datasets and Appendix). We aimed to (1) compare the mean values of CLL breakdown rates with that of LL (including conifers and broadleaf species) reported in other global-scale studies and test the effect of mesh size (coarse vs. fine), leaf habit (evergreen vs. deciduous), and invasion status (native vs. exotic) on CLL decomposition; (2) select the driving intrinsic (CLL traits), environmental (climatic and geographic), and experimental factors on CLL breakdown rates; and (3) investigate the responses of CLL breakdown rates to the gradients of MAT, MAP, latitude, and altitude. We predicted that (1) CLL breakdown rates would be lower than other reported LL breakdown rates; (2) LL traits such as N and lignin would have greater impacts on CLL decomposition than other factors; and (3) CLL breakdown rates would increase with increasing MAT and MAP toward the poles but decrease with increasing altitude.
To evaluate the current state of knowledge on CLL decomposition in streams and rivers on a global scale, we built a database by searching online databases (ISI Web of Science and China National Knowledge Infrastructure for papers published in English and Chinese, respectively, prior to January 2022) with the following search terms: “(litter OR leaf) AND (conifer OR needle) AND (stream OR river) AND (breakdown OR decomposition OR decay).” The selected publications were based on several criteria: (1) only studies that were conducted in natural lotic ecosystems (i.e., streams and rivers) or experimental channels adjacent to streams and rivers were included to avoid experiments that were conducted in lentic ecosystems (e.g., lakes and ponds) or mesocosms; (2) the study should have measured LL breakdown rates of at least one conifer species; and (3) studies that measured the breakdown rates of mixed LL species in one leaf-litter bag were excluded due to the LL-mixing effects on LL decomposition (Leroy and Marks, 2006). In total, we screened 541 independent observations (324 and 217 observations in coarse and fine mesh bags, respectively) from 35 publications (Figure 1, Supplementary Datasets). The database included 541 and 428 cases for LL breakdown rates expressed as per day and per degree day, respectively, spanning 105° of latitude and large MAP (310–2,250 mm), MAT (0.36–19.8°C), and altitudinal gradients (1–3,600 m a.s.l.) in five continents.
Figure 1. Global distribution of the 54 sites of coniferous leaf-litter decomposition in streams and rivers. There were 541 and 428 records of coniferous leaf-litter breakdown rates expressed as per day and per degree day, respectively. The colors of circles represent the number of records of leaf-litter breakdown rates (including per day and per degree day) at each site.
Leaf-litter breakdown rates expressed as kd (per day) and kdd (per degree day) were collected from each publication. When kd and/or kdd were not provided in the publication, the following breakdown rate coefficient was used: Wt = We−kt, where WI and Wt are the initial and final dry LL mass, respectively, and t is the incubation time (day) (Bärlocher, 2020), and kdd was calculated by replacing the time (t) in days with the total water temperature accumulated over the experimental period. The total water temperature accumulated over the experimental period was calculated with mean water temperature multiplied by experimental duration in this study. Leaf-litter breakdown rates of the replications at each sampling trial/date were collected if they were provided in the original publication. If not, the mean values of kd or kdd at each sampling date were recorded. For studies that reported LL breakdown rates in both coarse (>1 mm) and fine ( ≤ 1 mm) mesh bags, they represent the total (ktot) and microbial-mediated LL breakdown rates (kmicro), respectively, and macroinvertebrate-mediated LL breakdown rates (kinver) were calculated with Wt as the differences in mass remaining in coarse and fine mesh bags (Hladyz et al., 2010).
Factors that have potential impacts on LL breakdown rates were extracted from the publications. First, climatic and geographic factors including MAT, MAP, MDTR, latitude, longitude, and altitude were recorded. If geographic data (i.e., latitude, longitude, and altitude) were missing in the original study, they were determined by using Google Earth (version 7.1.8.3036). An open climatic dataset (CRU TS v4.04, between 1901 and 2019), which is based on Google Earth on a 0.5° latitude by 0.5° longitude grid (Osborn and Jones, 2014; Harris et al., 2020), was used to obtain the climatic data (MAT, MDTR, and MAP) if they were not provided in the original studies. Second, we collected LL traits including nutritional (e.g., N and P), chemical (e.g., tannin and cellulose), and physical quality (e.g., toughness). We manually extracted LL traits data that were available only in figures. Leaf-litter nutrient concentrations that were recorded as mg/g were transformed into percentages for ease of comparison. Because some studies did not calculate stoichiometry ratios of LL traits, a third LL trait was calculated when two out of the three stoichiometry-related traits were present; for example, the lignin/N ratio was calculated if concentrations of N and lignin were available. Third, experimental factors including a mesh size of LL bags, experimental duration (day), and initial CLL mass (g) were recorded. A mesh size larger than 1 mm that allowed the access of most macroinvertebrates was classified as a coarse mesh size representing total LL breakdown rates, while a mesh size smaller than 1 mm that excluded most macroinvertebrates was classified as a fine mesh size representing microbial-mediated LL breakdown rates (Follstad Shah et al., 2017). Finally, stream physicochemical characteristics that include physical characteristics (e.g., depth, width, and velocity) and stream water quality (e.g., pH, NO- 3, and ) were extracted.
Other information extracted from the publications includes site names of decomposition experiment locations (continent) and plant classification information (species and family name). Plant functional types (PFTs) were also classified as leaf habit (evergreen vs. deciduous) and invasion status (exotic vs. native). The invasion status was determined by checking whether the sampling site is in the species' natural distribution range (http://temperate.theferns.info/) when this information was not provided in the original publication. Data collected from islands that are >1,000 km away from continents (Azores) were not assigned to any continent.
First, a one-way analysis of variance (ANOVA) was conducted to compare the mean values of LL breakdown rates (both kd and kdd) in this study with other global-scale studies (Boyero et al., 2016; Follstad Shah et al., 2017; Zhang et al., 2019; LeRoy et al., 2020). These studies were selected based on several criteria: (1) field experiments or meta-analysis included worldwide data; (2) data of LL breakdown rates should be obtained from natural senesced LL of various species; and (3) the decomposition experiments should be conducted in lotic ecosystems (e.g., streams and rivers). We conducted the analyses with three different datasets: (1) all data combined; (2) data for coarse mesh bags; and (3) data for fine mesh bags. Tukey's honestly significant difference (HSD) was used for the post-hoc multiple comparison in the ANOVA analysis. Second, t-tests were used to investigate whether the CLL breakdown rates differ between coarse and fine mesh bags. The effects of leaf habit and invasion status on CLL breakdown rates were also checked with t-tests. As there were only three studies (37 cases) that had values of macroinvertebrate-mediated LL breakdown rates, we did not conduct any analysis for this variable.
Third, stepwise multiple regression analyses were conducted to investigate the associations of climatic and geographic factors, LL traits, experimental factors, and stream physicochemical characteristics with CLL breakdown rates. We selected factors that were significantly correlated with CLL breakdown rates (Supplementary Table S1). For the selected predictors, any potential collinear predictors were identified and removed (R ≥ 0.70) before running the analyses. If the collinearity was close to the threshold, we further calculated the variance inflation factors (VIFs) to ensure that they would not inflate the variance of models. The predictors were kept when the VIFs were below 4. Toughness and phenol-related traits were excluded in the stepwise multiple regression analyses due to the limited species (<5) that had measurements of these LL traits. The stepwise multiple regression analyses were conducted in two steps: (1) we conducted the analysis for the four categories of predictors individually (model A) and (2) we included all the factors screened in model A (model B). In addition, the included predictors in models A and B should have observations >10 times the number of variables.
Finally, linear models were used to explore the responses of CLL breakdown rates to important predictors selected in the stepwise multiple regression analyses. As some climatic and geographic factors were excluded in the regression analyses (e.g., latitude), we were interested in the latitudinal and altitudinal trends of CLL decomposition in lotic ecosystems and how climate changes (temperature and precipitation) would affect CLL breakdown rates. In this case, all climatic and geographic factors, LL traits including N and P, mean water temperature, and were included in the linear models. These linear models were conducted with three different datasets: all data pooled together and data from coarse and fine mesh bags. This kind of data treatment was aimed to test whether there are differences between the responses of total and microbial-mediated CLL breakdown rates to these predictors. Before conducting all analyses, outliers, normality, and homogeneity of variance were examined, and data were log-transformed to meet normality and homogeneity assumptions. Consequently, CLL breakdown rates, C/P, lignin/N, experimental duration, initial litter mass, velocity, discharge, NO- 3, NH+ 4, , and conductivity were log-transformed. All statistical analyses were carried out using IBM SPSS Statistic 24.0.
We found consistently lower LL breakdown rates of conifers in this study than in other global-scale studies regardless of whether it was expressed as per day or per degree day or whether it was in coarse or fine mesh bags (Figure 2). Mean CLL breakdown rates in this study were −2.37 ± 0.02 (n = 543, mean ± SE, data were log-transformed) and −3.20 ± 0.02 (n = 430, mean ± SE), which were 18.5–28.8 and 4.9–16.8% lower than the reported LL breakdown rates in other global-scale studies when expressed as per day and per degree day, respectively. This trend was similar when data were analyzed separately in coarse and fine mesh bags, except that the mean breakdown rates (per degree day) in coarse mesh bags did not differ from one study (Boyero et al., 2016).
Figure 2. Comparisons of leaf-litter breakdown rates (per day, left; per degree day, right) in this study (A) with other global-scale studies [B, Boyero et al., 2016; C, Follstad Shah et al., 2017; D, LeRoy et al., 2020; E, Zhang et al., 2019]. Box plots summarize the distribution of all values. Box plots indicate the interquartile ranges (box part), 25th and 75th percentiles (lower and upper error bars), median values (red circles in the box), mean values (red triangles in the box), and outliers (black dots). Lowercase letters above each box indicate a significant difference among studies after one-way ANOVA and post-hoc Tukey's t-test (parameters with the same letter are not significantly different between studies, P > 0.05) was used. ***P < 0.001.
We did find that CLL breakdown rates differed between mesh sizes, leaf habits, and invasion status (Figure 3). Coniferous LL decomposed 13.6 and 11.6% faster in coarse than in fine mesh bags when expressed as per day and per degree day, respectively (Figures 3A,D). Unexpectedly, deciduous CLL (Larix) had 11.3% slower breakdown rate (per day) than evergreen species (Figure 3B), and this trend was the same even though data were divided into coarse and fine mesh bags (Supplementary Figures S1A,C). However, the deciduous CLL breakdown rate in fine mesh bags was 4.8% faster than evergreen species when expressed as per degree day (Supplementary Figure S1C) and did not differ in coarse mesh bags nor when data were pooled. Exotic CLL breakdown rates (per day) were 10.6 and 8.7% higher than native species when data were combined (Figure 3C) and in coarse mesh bags (Supplementary Figure S1B), respectively. By contrast, exotic conifers had 3.4% faster breakdown rates, while they had 3.2% slower breakdown rates (per degree day) when data were combined (Figure 3F) and in fine mesh bags (Supplementary Figure S1D), respectively.
Figure 3. Comparisons of leaf-litter breakdown rates (per day, upper; per degree day, lower) between coarse and fine mesh bags (A,D), evergreen and deciduous species (B,E), and native and exotic species (C,F). Box plots summarize the distribution of all values. Box plots indicate the interquartile ranges (box part), 25th and 75th percentiles (lower and upper error bars), median values (circles in the box), and mean values (triangles in the box).
Altitude, MAP, and MDTR were the best-fit climatic and geographic predictors for CLL breakdown rates (per day), which together explained 22.1% of the variation (Table 1), whereas MAT and MDTR only explained 2.8% of the variations in CLL breakdown rates when expressed as per degree day. Nitrogen was the best-fit LL trait predictor, which explained 39.3% of the variations in CLL breakdown rates (per day). When expressed as per degree day, P and N/P became the best-fit LL trait predictors, which together explained 70.4% of the variation in CLL decomposition. The best-fit experimental predictors for CLL breakdown rates were mesh size and experimental duration regardless of whether it was expressed as per day or per degree day. Mean water temperature was the best-fit physicochemical characteristic for the prediction of CLL breakdown rates (per day). By contrast, 81.8% of the variation in CLL breakdown rates (per degree day) was explained by discharge, pH, and minimum water temperature. When the selected four categories of predictors were pooled together, mean water temperature, mesh size, duration, and N concentration of LL were responsible for 23.6% of the variation in CLL breakdown rates (per day), whereas P, experimental duration, and minimum water temperature together explained 91.2% of the variation in CLL breakdown rates (per degree day). However, the best-fit predictors (in each category and with the four categories of predictors pooled) changed when CLL breakdown rates were analyzed individually in coarse and fine mesh bags (Table 1). In addition, when analyzed individually, most of the four groups of predictors showed linear relationships with CLL breakdown rates (per day and per degree day) regardless of whether data were pooled, in coarse or in fine mesh bags (Supplementary Table S1).
Table 1. Results of multiple regressions examining global-scale variations in coniferous leaf-litter breakdown rates (kd, per day; kdd, per degree day).
Coniferous LL breakdown rates (per day) increased with increasing N, P, mean water temperature, , MAT, and MAP, but decreased with increasing MDTR and altitude (Figure 4). There were no latitudinal trends of CLL breakdown rates regardless of whether it was expressed as per day or per degree day when all data were pooled (Figure 4E). However, we found a decreasing trend of CLL breakdown rates toward the poles in coarse mesh bags. On the contrary, CLL breakdown rates increased toward the poles in fine mesh bags (Supplementary Figure S2A). CLL breakdown rates (per day) decreased with increasing altitude when data were pooled (Figure 4I), in coarse and in fine mesh bags (Supplementary Figure S2C). However, CLL breakdown rates (per degree day) increased with increasing altitude in coarse mesh bags (Supplementary Figure S2D). Coniferous LL breakdown rates increased but decreased with increasing MAT and MAP when expressed as per day and per degree day, respectively (Figures 4F,G). However, there were no linear relationships between MAP and CLL breakdown rates (Supplementary Figures S2I,J) and between MAT and CLL breakdown rates (per degree day) in coarse mesh bags (Supplementary Figure S2F). The relationships between MDTR and CLL breakdown rates (per day and per degree day) when all data were pooled were contrary to the relationships between MAT and CLL breakdown rates (Figure 4H). However, CLL breakdown rates (per day) increased but decreased with increasing MDTR in coarse and fine mesh bags, respectively (Supplementary Figure S2G).
Figure 4. (A–I) Relationships between coniferous leaf-litter breakdown rates (per day, circle; per degree day, triangle) and selected important leaf-litter traits (N and P), stream physicochemical characteristics (mean water temperature and ), and geographic and climatic (latitude; MAT, mean annual temperature; MAP, mean annual precipitation; MDTR, mean diurnal temperature range; and altitude) predictors. R values with significant correlations are shown in the figure. ***P < 0.001. Linear fitted lines and 95% confidence intervals are shown.
Our result supports the first hypothesis that LL breakdown rates in this study were significantly lower than that in other global-scale studies (Boyero et al., 2016; Follstad Shah et al., 2017; Zhang et al., 2019; LeRoy et al., 2020). This result advocates many other studies that found lower CLL breakdown rates than broadleaved LL in streams at local, regional, and global scales (Graça and Pereira, 1995; Albariño and Balseiro, 2002; Zhang et al., 2019). Although, some studies claimed that there were no differences in LL breakdown rates between conifers and broadleaved species at local (Ferreira et al., 2017) and global scales (Ferreira et al., 2016). The slower CLL breakdown rate was not surprising because CLL usually has a poorer nutritional quality (e.g., lower N) than other species (Richardson et al., 2004). Microbes and macroinvertebrates prefer a high nutritional quality over less nutritious LL, which resulted in a lower breakdown rate of poor nutritious LL (Gessner and Chauvet, 1994; Balibrea et al., 2020; Oliveira et al., 2022). Another possible explanation may be associated with temperature because most sampling sites in this study were in mid- and high-latitude areas and had no site in tropical areas. Consequently, MAT and water temperature in this study were lower than those mentioned in other large-scale studies, which may contribute to the reduced LL breakdown rates (Boyero et al., 2011b; Follstad Shah et al., 2017). Given that conifer plantation is one of the main types of forest changes that occurred worldwide (Essl et al., 2010; Ferreira et al., 2016; Kawamura et al., 2021), our results, therefore, have implication on predicting the ecological consequences of the replacement of native species by conifer plants (e.g., Pinus) in freshwater ecosystems such as LL decomposition.
As expected, LL traits explained a relatively high proportion of the variation in CLL decomposition. This result is in accordance with many other local and global-scale studies that revealed the predominant role of LL traits in determining LL decomposition in streams and rivers (Leroy and Marks, 2006; Goncalves et al., 2017; Zhang et al., 2019). Also, we found that the controlling LL traits differed depending on whether it was expressed as per day or per degree day and whether in coarse or fine mesh bags. This result adds evidence that there was no consistent conclusion regarding which LL trait is the most important predictor of LL decomposition in freshwaters. Furthermore, our result underscored that LL nutritional quality (N and P) mainly drives the decomposition of CLL in streams and rivers, and the result agrees with the findings for many broadleaved LL and CLL (Kennedy and El-Sabaawi, 2017; Jabiol et al., 2019). Nitrogen and P concentrations are important indicators of LL decomposability. Many researchers found positive effects of N content on LL decomposition in freshwaters through enhanced biomass, diversity, and activity of microbes (e.g., fungi) and macroinvertebrates (Garcia et al., 2012; Jackrel and Wootton, 2015; Pereira and Ferreira, 2021). By contrast, secondary compounds such as lignin can hinder LL decomposition by reducing the abundance and richness of microbes and macroinvertebrates (Gessner and Chauvet, 1994; Leite-Rossi et al., 2016; Balibrea et al., 2020). However, we only found a negative relationship between lignin and CLL breakdown rates in fine mesh bags. Taken together, our results may indicate that microbial-mediated and total CLL decomposition were driven by lignin and the nutritional quality of LL, respectively.
Stream physicochemical characteristics affected CLL decomposition, but the best-fit predictors are context dependent. Specifically, we found stronger impacts of mean water temperature on CLL decomposition than other physicochemical characteristics in fine mesh bags and when all data were pooled. An increasing number of studies addressed the importance of water temperature in driving LL decomposition in freshwaters at local, regional, and global scales (Boyero et al., 2011b; Bernabé et al., 2018; Xiang et al., 2019). As the positive relationships between CLL decomposition and mean water temperature appeared in both coarse and fine mesh bags, the R value was much higher in coarse than in fine mesh bags. We may speculate that the accelerated CLL decomposition at higher water temperature may be attributed to both macroinvertebrate- and microbial-mediated ways. Elevated water temperature can stimulate microbial-mediated LL decomposition in various ways, including stimulating activities, higher biomass, and higher richness of microbes (Ferreira and Chauvet, 2011; Canhoto et al., 2016). Similarly, macroinvertebrates decomposed LL faster under warmer conditions due to higher metabolic rate, stimulated activities, and higher density (Esther et al., 2015; Griffiths and Tiegs, 2016). Although some researchers found negative effects of warmer water temperature on macroinvertebrates and/or microbes (Boyero et al., 2011b; Martínez et al., 2014; Domingos et al., 2015), these negative effects may be weak or were suppressed by the positive effects of warming on LL decomposition. Moreover, we found significant effects of temperature range, minimum water temperature, and maximum water temperature on CLL decomposition. This result highlights that, along with mean water temperature, temperature oscillation should also be considered in future studies (Dang et al., 2009).
By contrast, concentration was the best-fit physicochemical predictor, which showed a positive relationship with CLL decomposition in coarse mesh bags. This result adds evidence to mounting research that suggest accelerated LL decomposition by higher water nutrient concentrations (Woodward et al., 2012; Ferreira et al., 2015a; Manning et al., 2021). Phosphorus is a fundamental and usually limited nutrient in freshwater ecosystems such as streams and rivers (Elser et al., 2007; Dodds and Smith, 2016). Previous studies found that the abundance, density, biomass, and richness of macroinvertebrates may be positively affected by higher nutrient concentrations (Gulis et al., 2006; Bergfur et al., 2007; Pereira et al., 2016) to enhance LL decomposition. We may anticipate faster macroinvertebrate-mediated CLL breakdown rates in future, considering that P concentration in streams and rivers worldwide has been and will continue to increase (Vilmin et al., 2018; Harrison et al., 2019; Beusen et al., 2022). Taken together, our results imply that macroinvertebrate-mediated and microbial-mediated CLL decomposition were differently influenced by stream physicochemical characteristics, and future changes in water temperature and nutrient concentrations should be especially concerned.
Although we found that CLL breakdown rates had linear relationships with gradients of climatic and geographic factors, they explained relatively smaller variations in CLL decomposition in streams and rivers than other predictors (e.g., LL traits). This result agrees with previous studies that found that LL decomposition changed along the gradients of latitude (Irons III et al., 1994; Tiegs et al., 2019; Boyero et al., 2021), altitude (Fabre and Chauvet, 1998; Jinggut and Yule, 2015; Martínez et al., 2016), and temperature (Boyero et al., 2011b). Specifically, we found that CLL breakdown rates increased in fine mesh bags but decreased in coarse mesh bags toward the poles. The result for fine mesh bags is consistent with other large-scale studies, while the result for coarse mesh bags agrees (Tiegs et al., 2019) or disagrees with others (Irons III et al., 1994; Follstad Shah et al., 2017). Our result implies that macroinvertebrate-mediated CLL decomposition was reduced toward the poles and overrode the stimulated microbial-mediated CLL decomposition, which resulted in decreased total CLL breakdown rates. Along the latitudinal gradient, we found increased chemical LL quality (e.g., lower lignin and lignin/N), decreased MAT and mean water temperature, and lower water nutrient concentrations (e.g., NO-3 and ). These changes may have positive (enhanced LL quality) or negative effects (e.g., lower water temperature) on CLL decomposition in streams as evidenced in this study and many others (Woodward et al., 2012; Ferreira et al., 2015b). Therefore, we may speculate that the accelerated microbial-mediated CLL breakdown rates toward the poles may be attributed to the increased chemical LL quality, and this effect may have overridden the negative effects of decreased temperature and water nutrient concentrations on microbes. On the contrary, decreased MAT, mean water temperature, and water nutrient concentrations may have resulted in slower macroinvertebrate-mediated and hence total CLL breakdown rates toward high-latitude areas and suppressed the positive effects of enhanced CLL quality on decomposition.
In addition, we found consistently slower CLL breakdown rates in this study than the reported LL breakdown rates in tropical areas in other studies. This is interesting because previous studies found higher LL breakdown rates in tropical than in high-latitude areas or no differences between the two regions (Irons III et al., 1994; Zhang et al., 2019). This pattern may be true for broadleaved species because many previously published papers focused on broadleaved LL and ignored coniferous species. In fact, conifers are the dominant plants in many mid- and high-latitude areas such as temperate, boreal, and Mediterranean forests (Essl et al., 2010). However, the decomposition of CLL in streams is largely ignored by researchers, and the proportion of conifers was usually low in previous studies (Ferreira et al., 2015a; LeRoy et al., 2020). The low proportion of conifers in global-scale studies may have unintentionally induced higher LL breakdown rates in mid- and high-latitude areas. Therefore, we argue that the inclusion of a proper proportion of conifers may give us a better understanding of the latitudinal trend of LL decomposition in streams and rivers at a global scale, especially for macroinvertebrate-mediated LL decomposition and for temperature-normalized LL breakdown rates.
We also found that the third hypothesis was supported as MAT and MAP positively affected CLL decomposition in both coarse and fine mesh bags in streams, a result following previous studies (Boyero et al., 2011b; Follstad Shah et al., 2017). If the stimulated CLL breakdown rates were induced by the changes in CLL quality associated with increasing MAT and MAP, we should have expected slower CLL breakdown rates. Because CLL quality decreased with increasing MAT and MAP as indicated by higher lignin and lignin/N, and lower LL quality can inhibit LL decomposition through the negative effects on microbes and macroinvertebrates (Hladyz et al., 2009; Kennedy and El-Sabaawi, 2017). Similarly, faster CLL breakdown rates may not be correlated with the changes in detritivore communities associated with changing MAT and MAP. Because abundance and richness of shredders are higher in mid- and high-latitude areas (low MAT and MAP) than in low latitude areas (Boyero et al., 2011a). Alternatively, higher CLL breakdown rates caused by higher MAT may be attributed to the changed microbial communities (e.g., higher diversity) and higher water nutrient concentrations, all of which have shown to boost LL decomposition in streams (Boyero et al., 2011b; Woodward et al., 2012; Fenoy et al., 2022). As we did not have enough cases that included data from both coarse and fine mesh bags to calculate macroinvertebrate-mediated CLL breakdown rates, the relationship between CLL decomposition and MAT was stronger in fine than in coarse mesh bags. We are not sure whether macroinvertebrate-mediated CLL decomposition was stimulated by increasing MAT or not. Our result suggests that future climate change such as warming and higher MAP, which is likely to occur in mid- and high-latitude areas where conifer plants are dominant species (Essl et al., 2010), may lead to faster microbial-mediated and total CLL decomposition in streams.
Moreover, we found negative relationships between CLL breakdown rates and altitude in both coarse and fine mesh bags, while the relationship was positive in coarse mesh bags when expressed as per degree day. This decreasing (or increasing when expressed as per degree day) trend of LL decomposition along altitudinal gradient was also evidenced in other studies (Jinggut and Yule, 2015; Martínez et al., 2016). Because nutritional quality (N and P)increased while decreased with increasing altitude in fine and coarse mesh bags, respectively, the decreased CLL decomposition with increasing altitude in fine mesh bags, therefore, was not controlled by the improved CLL nutritional quality, nor by higher water nutrient concentrations (e.g., NO- 3, ). Rather, the decreased temperature (MAT and mean water temperature) should be responsible for the declined microbial-mediated CLL decomposition (Taylor and Chauvet, 2014). However, both the decreased nutritional quality of CLL and lower temperature may contribute to the decelerated total CLL decomposition.
As expected, we found that mesh size is positively correlated with CLL decomposition in both coarse and fine mesh bags, a result in line with other studies (Zhang et al., 2019). The mesh size of LL bags can be divided into coarse and fine mesh groups to represent total and microbial-mediated LL breakdown rates, respectively. However, this classification is somehow arbitrary and there was no consistent agreement on the standard. Some researchers suggested mesh size larger than 1 mm as coarse mesh (Follstad Shah et al., 2017; Zhang et al., 2019), while others claimed 0.5 mm (Ferreira and Guérold, 2017; Kennedy and El-Sabaawi, 2017). In addition, some researchers used three or more than three categories other than the two categories of mesh size because micro-, meio-, macroinvertebrates, and macroconsumers (e.g., crabs) can contribute to LL decomposition (Moulton et al., 2010; Handa et al., 2014; Wang et al., 2020). Therefore, we suggest that more studies should be conducted on the relationship between mesh size and LL decomposition. The commonly applied 1 or 0.5 mm to differ between coarse and fine mesh bags representing total and microbial-mediated LL breakdown rates may not be able to exclude small size invertebrates (e.g., small body midge) in fine mesh bags. The standard should be adjusted to a smaller size or more size categories may be used. Moreover, CLL breakdown rates decreased with a longer experimental duration, which agrees with many other studies (Ferreira et al., 2015b; Balibrea et al., 2020). Once fallen into freshwaters, LL is processed into three different stages, namely, leaching, conditioning, and fragmentation (Gessner et al., 1999). However, the duration of these stages of CLL was longer than other species (Sakai et al., 2016; Balibrea et al., 2020). Half of the CLL breakdown rates in this study were measured within 70 days, which translates to an average of 77.5% of remaining LL mass. Therefore, we suggested that future studies of CLL decomposition in freshwaters should have a longer duration than that for broadleaved LL.
Coniferous LL breakdown rates were higher in coarse than in fine mesh bags regardless of whether it was expressed as per day or per degree day, which is in accordance with other studies (Whiles and Wallace, 1997; Balibrea et al., 2020). This result suggests that both macroinvertebrates and microbes contributed to the decomposition of CLL. Based on the limited studies that can calculate macroinvertebrate-mediated CLL breakdown rates (37 cases), macroinvertebrates and microbes were responsible for 49.7% and 40.5% of total CLL decomposition, respectively. These values differ from the result in a global study that reported LL breakdown rates in lotic ecosystems at a global scale (unpublished data) and that the contribution of microbes was lower, while macroinvertebrates were higher than that study. Our result also differs from another study that reported higher and lower contributions of macroinvertebrates and microbes on LL decomposition based on biomass, respectively (Hieber and Gessner, 2002). The relatively high contribution of macroinvertebrates to CLL decomposition may be attributed to the high abundance and richness of detritivores in mid- and high-latitude areas (Boyero et al., 2011a) where conifers are abundant. Even though CLL is a low-quality food resource for detritivores in streams (Richardson et al., 2004), CLL can remain longer than broadleaved LL in streams and becomes the major food resource in summer when broadleaved LL are scarce or depleted (Hisabae et al., 2011; Sakai et al., 2016). In addition, CLL can also be utilized as case-building materials by invertebrates due to their refractory nature (Whiles and Wallace, 1997; Richardson et al., 2004; Márquez et al., 2017). These factors may partially explain the relatively high contribution of macroinvertebrates to CLL decomposition in streams.
Coniferous LL decomposition was affected by leaf habit that evergreen CLL decomposed faster than deciduous CLL (Larix). This result is unexpected because evergreen LL usually has a lower quality than broadleaved LL, which may result in slower LL breakdown rates (Kominoski et al., 2011; Ramos et al., 2021). Our result also differs from one early global-scale study that found no difference in broadleaved LL decomposition between evergreen and deciduous species (Zhang et al., 2019). As there was no consistent result regarding whether evergreen CLL had lower quality than deciduous CLL in this study, the slower deciduous CLL breakdown rates were probably because deciduous CLL was decomposed at higher altitude areas where the MAT was lower than the evergreen CLL decomposition sites. Because water temperature is usually positively correlated with LL decomposition in streams. Moreover, we found higher exotic than native CLL breakdown rates in coarse, but not in fine mesh bags, suggesting that only macroinvertebrate-mediated CLL decomposition was influenced by invasion status. This result is in line with another global-scale study (Kennedy and El-Sabaawi, 2017). Exotic CLL had higher nutritional (N) but lower chemical (lignin) quality than native CLL in coarse mesh bags. By contrast, exotic CLL showed both lower nutritional and chemical quality than native CLL in fine mesh bags. Therefore, the differences in CLL quality between native and exotic species may not be the reason why exotic CLL decomposed faster than native species in coarse mesh bags. However, exotic CLL decomposed in streams with higher MAT than native species, which may be responsible for the faster LL breakdown rates (Ferreira et al., 2015b). Our results imply that the environmental conditions (e.g., MAT) may modulate and override the effects of CLL quality on the processing rate in streams and rivers.
In conclusion, we found first that CLL breakdown rates were significantly lower than other species probably due to the lower quality (e.g., lower N and higher lignin) and the lower water temperature. Second, CLL decomposed faster in coarse than in fine mesh bags, and CLL breakdown rates increased with increasing mesh size. This result highlights the importance of macroinvertebrates in driving CLL decomposition, and the commonly used 1 mm or 0.5 mm mesh size to differing total and microbial-mediated LL breakdown rates may have resulted in higher values of the latter. Third, LL traits and stream physicochemical characteristics explained higher variations in CLL decomposition than climatic and geographic factors. Nutritional quality (N and P) of LL, water temperature, and water nutrient concentration () were identified as better predictors of CLL decomposition than other factors. Finally, CLL decomposition was stimulated by higher MAT and MAP. These observed global patterns of CLL decomposition have implications to help predict ecological responses of CLL breakdown rates and their responsive mechanisms to future climate change and the subsequent consequences on macroinvertebrate and microbial communities and global nutrient cycling in freshwater ecosystems.
The raw data supporting the conclusions of this article will be made available by the authors, without undue reservation.
HX and ZZ conceived the ideas and designed methodology. HX collected the data and led the writing of the manuscript. KL, LC, and HY analyzed the data. All authors contributed critically to the drafts and gave final approval for publication.
This work was supported by the fellowship of the China Postdoctoral Science Foundation (2021M692728), the National Natural Science Foundation of China (32101310 and 32071587), the China Scholarship Council (202006625001), the Science Foundation of Science and Technology of Education Department of Jilin Province (JJKH20211293KJ), the Natural Science Foundation of Heilongjiang Province of China (YQ2021C031), the Postdoctoral Scientific Research Developmental Fund of Heilongjiang Province (LBH-Q20166), and the Basal Research Fund for Undergraduate Universities of Heilongjiang Province (2019-KYYWF-0598).
The authors declare that the research was conducted in the absence of any commercial or financial relationships that could be construed as a potential conflict of interest.
All claims expressed in this article are solely those of the authors and do not necessarily represent those of their affiliated organizations, or those of the publisher, the editors and the reviewers. Any product that may be evaluated in this article, or claim that may be made by its manufacturer, is not guaranteed or endorsed by the publisher.
The Supplementary Material for this article can be found online at: https://www.frontiersin.org/articles/10.3389/fevo.2022.940254/full#supplementary-material
Albariño, R. J., and Balseiro, E. G. (2002). Leaf litter breakdown in Patagonian streams: native versus exotic trees and the effect of invertebrate size. Aquat. Conserv. 12, 181–192. doi: 10.1002/aqc.511
Anderson, N. H., and Sedell, J. R. (1979). Detritus processing by macroinvertebrates in stream ecosystems. Annu. Rev. Entomol. 24, 351–377. doi: 10.1146/annurev.en.24.010179.002031
Balibrea, A., Ferreira, V., Balibrea, C., Gonçalves, V., and Raposeiro, P. M. (2020). Contribution of macroinvertebrate shredders and aquatic hyphomycetes to litter decomposition in remote insular streams. Hydrobiologia 847, 2337–2355. doi: 10.1007/s10750-020-04259-1
Bärlocher, F (2020). “Leaf mass loss estimated by the litter bag technique,” in Methods to Study Litter Decomposition: A Practical Guide, eds. Bärlocher, F., Gessner, M. O., and Graça, M. A. S., (Cham: Springer International Publishing) 43–51.
Berg, B (2014). Foliar litter decomposition: a conceptual model with focus on pine (Pinus) litter-a genus with global distribution. Int. Sch. Res. Notices 2014, 838169. doi: 10.1155/2014/838169
Bergfur, J., Johnson, J., Sandin, L., and Goedkoop, W. (2007). Effects of nutrient enrichment on boreal streams: invertebrates, fungi and leaf-litter breakdown. Freshw. Biol. 52, 1618–1633. doi: 10.1111/j.1365-2427.2007.01770.x
Bernabé, T. N., de Omena, P. M., Santos, V. P. d, de Siqueira, V. M., de Oliveira, V. M., and Remero, G. Q. (2018). Warming weakens facilitative interactions between decomposers and detritivores, and modifies freshwater ecosystem functioning. Glob. Change Biol. 24, 3170–3186. doi: 10.1111/gcb.14109
Beusen, A. H. W., Doelman, J. C., Van Beek, L. P. H., Van Puijenbroek, P. J. T. M., Mogollón, J. M., Van Grinsven, H. J. M., et al. (2022). Exploring river nitrogen and phosphorus loading and export to global coastal waters in the Shared Socio-economic pathways. Glob. Environ. Change 72, 102426. doi: 10.1016/j.gloenvcha.2021.102426
Boyero, L., Pearson, R. G., Dudgeon, D., Graça, M. A. S., Gessner, M. O., Albariño, R. J., et al. (2011a). Global distribution of a key trophic guild contrasts with common latitudinal diversity patterns. Ecology 92, 1839–1848. doi: 10.1890/10-2244.1
Boyero, L., Pearson, R. G., Gessner, M. O., Barmuta, L. A., Ferreira, V., Graça, M. A., et al. (2011b). A global experiment suggests climate warming will not accelerate litter decomposition in streams but might reduce carbon sequestration. Ecol. Lett. 14, 289–294. doi: 10.1111/j.1461-0248.2010.01578.x
Boyero, L., Pearson, R. G., Hui, C., Gessner, M. O., Pérez, J., Alexandrou, M. A., et al. (2016). Biotic and abiotic variables influencing plant litter breakdown in streams: a global study. Proc. Royal Soc. B 283, 20152664. doi: 10.1098/rspb.2015.2664
Boyero, L., Pérez, J., López-Rojo, N., Tonin, A. M., Correa-Araneda, F., Pearson, R. G., et al. (2021). Latitude dictates plant diversity effects on instream decomposition. Sci. Adv. 7, eabe7860. doi: 10.1126/sciadv.abe7860
Canhoto, C., Goncalves, A. L., and Baerlocher, F. (2016). Biology and ecological functions of aquatic hyphomycetes in a warming climate. Fungal Ecol. 19, 201–218. doi: 10.1016/j.funeco.2015.09.011
Dang, C. K., Schindler, M., Chauvet, E., and Gessner, M. O. (2009). Temperature oscillation coupled with fungal community shifts can modulate warming effects on litter decomposition. Ecology 90, 122–131. doi: 10.1890/07-1974.1
Dodds, W. K., and Smith, V. H. (2016). Nitrogen, phosphorus, and eutrophication in streams. Inland Waters 6, 155–164. doi: 10.5268/IW-6.2.909
Domingos, C., Ferreira, V., Canhoto, C., and Swan, C. (2015). Warming, and the presence of a dominant shredder, drive variation in decomposer communities in a mountain stream. Aquat. Sci. 77, 129–140. doi: 10.1007/s00027-014-0378-z
Elser, J. J., Bracken, M. E. S., Cleland, E. E., Gruner, D. S., Harpole, W. S., Hillebrand, H., et al. (2007). Global analysis of nitrogen and phosphorus limitation of primary producers in freshwater, marine and terrestrial ecosystems. Ecol. Lett. 10, 1135–1142. doi: 10.1111/j.1461-0248.2007.01113.x
Essl, F., Moser, D., Dullinger, S., Mang, T., and Hulme, P. E. (2010). Selection for commercial forestry determines global patterns of alien conifer invasions. Divers. Distrib. 16, 911–921. doi: 10.1111/j.1472-4642.2010.00705.x
Esther, M. M., Isabel, M., Francesc, O., and Cristina, C. (2015). Effects of increased water temperature on leaf litter quality and detritivore performance: a whole-reach manipulative experiment. Freshw. Biol. 60, 184–197. doi: 10.1111/fwb.12485
Fabre, E., and Chauvet, E. (1998). Leaf breakdown along an altitudinal stream gradient. Arch. Hydrobiol. 141, 167–179. doi: 10.1127/archiv-hydrobiol/141/1998/167
Fenoy, E., Pradhan, A., Pascoal, C., Rubio-Ríos, J., Batista, D., Moyano-López, F. J., et al. (2022). Elevated temperature may reduce functional but not taxonomic diversity of fungal assemblages on decomposing leaf litter in streams. Glob. Change Biol. 28, 115–127. doi: 10.1111/gcb.15931
Fernandes, I., Pascoal, C., Guimarães, H., Pinto, R., Sousa, I., and Cássio, F. (2012). Higher temperature reduces the effects of litter quality on decomposition by aquatic fungi. Freshw. Biol. 57, 2306–2317. doi: 10.1111/fwb.12004
Ferreira, V., Castagneyrol, B., Koricheva, J., Gulis, V., Chauvet, E., and Graça, M. A. S. (2015a). A meta-analysis of the effects of nutrient enrichment on litter decomposition in streams. Biol. Rev. 90, 669–688. doi: 10.1111/brv.12125
Ferreira, V., and Chauvet, E. (2011). Synergistic effects of water temperature and dissolved nutrients on litter decomposition and associated fungi. Glob. Change Biol. 17, 551–564. doi: 10.1111/j.1365-2486.2010.02185.x
Ferreira, V., Chauvet, E., and Canhoto, C. (2015b). Effects of experimental warming, litter species, and presence of macroinvertebrates on litter decomposition and associated decomposers in a temperate mountain stream. Can. J. Fish. Aquat. Sci. 72, 206–216. doi: 10.1139/cjfas-2014-0119
Ferreira, V., Faustino, H., Raposeiro, P. M., and Gonçalves, V. (2017). Replacement of native forests by conifer plantations affects fungal decomposer community structure but not litter decomposition in Atlantic island streams. For. Ecol. Manag. 389, 323–330. doi: 10.1016/j.foreco.2017.01.004
Ferreira, V., and Guérold, F. (2017). Leaf litter decomposition as a bioassessment tool of acidification effects in streams: Evidence from a field study and meta-analysis. Ecol. Indic. 79, 382–390. doi: 10.1016/j.ecolind.2017.04.044
Ferreira, V., Koricheva, J., Pozo, J., and Graça, M. A. S. (2016). A meta-analysis on the effects of changes in the composition of native forests on litter decomposition in streams. For. Ecol. Manag. 364, 27–38. doi: 10.1016/j.foreco.2016.01.002
Follstad Shah, J. J., Kominoski, J. S., Ardon, M., Dodds, W. K., Gessner, M. O., Griffiths, N. A., et al. (2017). Global synthesis of the temperature sensitivity of leaf litter breakdown in streams and rivers. Glob. Change Biol. 23, 3064–3075. doi: 10.1111/gcb.13609
Garcia, L., Richardson, J. S., and Pardo, I. (2012). Leaf quality influences invertebrate colonization and drift in a temperate rainforest stream. Can. J. Fish. Aquat. Sci. 69, 1663–1673. doi: 10.1139/f2012-090
Gessner, M. O., and Chauvet, E. (1994). Importance of stream microfungi in controlling breakdown rates of leaf-litter. Ecology 75, 1807–1817. doi: 10.2307/1939639
Gessner, M. O., Chauvet, E., and Dobson, M. (1999). A perspective on leaf litter breakdown in streams. Oikos 85, 377–384. doi: 10.2307/3546505
Goncalves, J. F., Couceiro, S. R. M., Rezende, R. S., Martins, R. T., Ottoni-Boldrini, B. M. P., Campos, C. M., et al. (2017). Factors controlling leaf litter breakdown in Amazonian streams. Hydrobiologia 792, 195–207. doi: 10.1007/s10750-016-3056-4
Graça, M., and Pereira, A. (1995). The degradation of pine needles in a Mediterranean stream. Arch. Hydrobiol. 134, 119–128. doi: 10.1127/archiv-hydrobiol/134/1995/119
Graça, M. A. S (2001). The role of invertebrates on leaf litter decomposition in streams - a review. Int. Rev. Hydrobiol. 86, 383–393. doi: 10.1002/1522-2632(200107)86:4/5<383::AID-IROH383>3.0.CO;2-D
Griffiths, N. A., and Tiegs, S. D. (2016). Organic-matter decomposition along a temperature gradient in a forested headwater stream. Freshw. Sci. 35, 518–533. doi: 10.1086/685657
Gulis, V., Ferreira, V., and Graca, M. A. S. (2006). Stimulation of leaf litter decomposition and associated fungi and invertebrates by moderate eutrophication: implications for stream assessment. Freshw. Biol. 51, 1655–1669. doi: 10.1111/j.1365-2427.2006.01615.x
Handa, I. T., Aerts, R., Berendse, F., Berg, M. P., Bruder, A., Butenschoen, O., et al. (2014). Consequences of biodiversity loss for litter decomposition across biomes. Nature 509, 218–221. doi: 10.1038/nature13247
Harris, I., Osborn, T. J., Jones, P., and Lister, D. (2020). Version 4 of the CRU TS monthly high-resolution gridded multivariate climate dataset. Sci. Data 7, 109. doi: 10.1038/s41597-020-0453-3
Harrison, J. A., Beusen, A. H. W., Fink, G., Tang, T., Strokal, M., Bouwman, A. F., et al. (2019). Modeling phosphorus in rivers at the global scale: recent successes, remaining challenges, and near-term opportunities. Curr. Opin. Environ. Sustain. 36, 68–77. doi: 10.1016/j.cosust.2018.10.010
Hayer, M., Wymore, A. S., Hungate, B. A., Schwartz, E., Koch, B. J., and Marks, J. C. (2022). Microbes on decomposing litter in streams: entering on the leaf or colonizing in the water? ISME J. 16, 717–725. doi: 10.1038/s41396-021-01114-6
Hieber, M., and Gessner, M. O. (2002). Contribution of stream detrivores, fungi, and bacteria to leaf breakdown based on biomass estimates. Ecology 83, 1026–1038. doi: 10.1890/0012-9658(2002)083[1026:COSDFA]2.0.CO;2
Hisabae, M., Sone, S., and Inoue, M. (2011). Breakdown and macroinvertebrate colonization of needle and leaf litter in conifer plantation streams in Shikoku, southwestern Japan. J. For. Res. 16, 108–115. doi: 10.1007/s10310-010-0210-0
Hladyz, S., Gessner, M. O., Giller, P. S., Pozo, J., and Woodward, G. (2009). Resource quality and stoichiometric constraints on stream ecosystem functioning. Freshw. Biol. 54, 957–970. doi: 10.1111/j.1365-2427.2008.02138.x
Hladyz, S., Tiegs, S. D., Gessner, M. O., Giller, P. S., Rîşnoveanu, G., Preda, E., et al. (2010). Leaf-litter breakdown in pasture and deciduous woodland streams: a comparison among three European regions. Freshw. Biol. 55, 1916–1929. doi: 10.1111/j.1365-2427.2010.02426.x
Irons, J. G. III, Oswood, M. W., Stout, R. J., and Pringle, C. M. (1994). Latitudinal patterns in leaf litter breakdown: is temperature really important? Freshw. Biol. 32, 401–411. doi: 10.1111/j.1365-2427.1994.tb01135.x
Jabiol, J., Lecerf, A., Lamothe, S., Gessner, M. O., and Chauvet, E. (2019). Litter quality modulates effects of dissolved nitrogen on leaf decomposition by stream microbial communities. Microb. Ecol. 77, 959–966. doi: 10.1007/s00248-019-01353-3
Jackrel, S. L., and Wootton, J. T. (2015). Cascading effects of induced terrestrial plant defences on aquatic and terrestrial ecosystem function. Proc. Royal Soc. B 282, 20142522. doi: 10.1098/rspb.2014.2522
Jinggut, T., and Yule, C. M. (2015). Leaf-litter breakdown in streams of East Malaysia (Borneo) along an altitudinal gradient: initial nitrogen content of litter limits shredder feeding. Freshw. Sci. 34, 691–701. doi: 10.1086/681256
Kanasashi, T., and Hattori, S. (2011). Seasonal variation in leaf-litter input and leaf dispersal distances to streams: the effect of converting broadleaf riparian zones to conifer plantations in central Japan. Hydrobiologia 661, 145–161. doi: 10.1007/s10750-010-0520-4
Kawamura, K., Yamaura, Y., Soga, M., Spake, R., and Nakamura, F. (2021). Effects of planted tree species on biodiversity of conifer plantations in Japan: a systematic review and meta-analysis. J. For. Res. 26, 237–246. doi: 10.1080/13416979.2021.1891625
Kennedy, K. T. M., and El-Sabaawi, R. W. (2017). A global meta-analysis of exotic versus native leaf decay in stream ecosystems. Freshw. Biol. 62, 977–989. doi: 10.1111/fwb.12918
Kominoski, J. S., Marczak, L. B., and Richardson, J. S. (2011). Riparian forest composition affects stream litter decomposition despite similar microbial and invertebrate communities. Ecology 92, 151–159. doi: 10.1890/10-0028.1
Leite-Rossi, L. A., Saito, V. S., Cunha-Santino, M. B., and Trivinho-Strixino, S. (2016). How does leaf litter chemistry influence its decomposition and colonization by shredder Chironomidae (Diptera) larvae in a tropical stream? Hydrobiologia 771, 119–130. doi: 10.1007/s10750-015-2626-1
LeRoy, C. J., Hipp, A. L., Lueders, K., Follstad Shah, J. J., Kominoski, J. S., Ardón, M., et al. (2020). Plant phylogenetic history explains in-stream decomposition at a global scale. J. Ecol. 108, 17–35. doi: 10.1111/1365-2745.13262
Leroy, C. J., and Marks, J. C. (2006). Litter quality, stream characteristics and litter diversity influence decomposition rates and macroinvertebrates. Freshw. Biol. 51, 605–617. doi: 10.1111/j.1365-2427.2006.01512.x
Manning, D. W. P., Ferreira, V., Gulis, V., and Rosemond, A. D. (2021). “Pathways, Mechanisms, and Consequences of Nutrient-Stimulated Plant Litter Decomposition in Streams,” in The Ecology of Plant Litter Decomposition in Stream Ecosystems, eds. Swan, C. M., Boyero, L., and Canhoto, C., (Cham: Springer International Publishing) 347–377.
Marks, J. C (2019). Revisiting the fates of dead leaves that fall into streams. Annu. Rev. Ecol. Evol. Syst. 50, 547–568. doi: 10.1146/annurev-ecolsys-110218-024755
Márquez, J. A., Principe, R. E., Martina, L. C., and Albariño, R. J. (2017). Pine needle litter acts as habitat but not as food source for stream invertebrates. Int. Rev. Hydrobiol. 102, 29–37. doi: 10.1002/iroh.201601856
Martínez, A., Larrañaga, A., Pérez, J., Descals, E., Basaguren, A., and Pozo, J. (2013). Effects of pine plantations on structural and functional attributes of forested streams. For. Ecol. Manag. 310, 147–155. doi: 10.1016/j.foreco.2013.08.024
Martínez, A., Larrañaga, A., Pérez, J., Descals, E., and Pozo, J. (2014). Temperature affects leaf litter decomposition in low-order forest streams: field and microcosm approaches. Fems Microbiol. Ecol. 87, 257–267. doi: 10.1111/1574-6941.12221
Martínez, A., Monroy, S., Pérez, J., Larrañaga, A., Basaguren, A., Molinero, J., et al. (2016). In-stream litter decomposition along an altitudinal gradient: does substrate quality matter? Hydrobiologia 766, 17–28. doi: 10.1007/s10750-015-2432-9
Moulton, T. P., Magalhães-Fraga, S. A. P., Brito, E. F., and Barbosa, F. A. (2010). Macroconsumers are more important than specialist macroinvertebrate shredders in leaf processing in urban forest streams of Rio de Janeiro, Brazil. Hydrobiologia 638, 55–66. doi: 10.1007/s10750-009-0009-1
Nessel, M. P., Konnovitch, T., Romero, G. Q., and González, A. L. (2021). Nitrogen and phosphorus enrichment cause declines in invertebrate populations: a global meta-analysis. Biol. Rev. 96, 2617–2637. doi: 10.1111/brv.12771
Oliveira, R., Martinez, A., Goncalves, A. L., and Canhoto, C. (2022). Intra-specific leaf trait variability controls leaf decomposition of Vitis vinifera L. cultivars in streams. Aquat. Ecol. 56, 47–57. doi: 10.1007/s10452-021-09891-0
Osborn, T. J., and Jones, P. D. (2014). The CRUTEM4 land-surface air temperature data set: construction, previous versions and dissemination via Google Earth. Earth Syst. Sci. Data 6, 61–68. doi: 10.5194/essd-6-61-2014
Pereira, A., and Ferreira, V. (2021). Invasion of Native Riparian Forests by Acacia Species Affects In-Stream Litter Decomposition and Associated Microbial Decomposers. Microb. Ecol. 81, 14–25. doi: 10.1007/s00248-020-01552-3
Pereira, A., Geraldes, P., Lima-Fernandes, E., Fernandes, I., Cássio, F., and Pascoal, C. (2016). Structural and functional measures of leaf-associated invertebrates and fungi as predictors of stream eutrophication. Ecol. Indic. 69, 648–656. doi: 10.1016/j.ecolind.2016.05.017
Peréz, J., Ferreira, V., Graça, M. A. S., and Boyero, L. (2021). Litter quality is a stronger driver than temperature of early microbial decomposition in oligotrophic streams: a microcosm study. Microb. Ecol. 82, 897–908. doi: 10.1007/s00248-021-01858-w
Ramos, S. M., Graca, M. A. S., and Ferreira, V. (2021). A comparison of decomposition rates and biological colonization of leaf litter from tropical and temperate origins. Aquat. Ecol. 55, 925–940. doi: 10.1007/s10452-021-09872-3
Richardson, D. M., and Rejmánek, M. (2004). Conifers as invasive aliens: a global survey and predictive framework. Divers. Distrib. 10, 321–331. doi: 10.1111/j.1366-9516.2004.00096.x
Richardson, J. S., Shaughnessy, C. R., and Harrison, P. G. (2004). Litter breakdown and invertebrate association with three types of leaves in a temperate rainforest stream. Arch. Hydrobiol. 159, 309–326. doi: 10.1127/0003-9136/2004/0159-0309
Riipinen, M. P., Fleituch, T., Hladyz, S., Woodward, G., Giller, P., and Dobson, M. (2010). Invertebrate community structure and ecosystem functioning in European conifer plantation streams. Freshw. Biol. 55, 346–359. doi: 10.1111/j.1365-2427.2009.02278.x
Sakai, M., Fukushima, K., Naito, R. S., Natuhara, Y., and Kato, M. (2016). Coniferous needle litter acts as a stable food resource for stream detritivores. Hydrobiologia 779, 161–171. doi: 10.1007/s10750-016-2813-8
Seena, S., Bärlocher, F., Sobral, O., Gessner, M. O., Dudgeon, D., McKie, B. G., et al. (2019). Biodiversity of leaf litter fungi in streams along a latitudinal gradient. Sci. Total Environ. 661, 306–315. doi: 10.1016/j.scitotenv.2019.01.122
Taylor, B. R., and Chauvet, E. E. (2014). Relative influence of shredders and fungi on leaf litter decomposition along a river altitudinal gradient. Hydrobiologia 721, 239–250. doi: 10.1007/s10750-013-1666-7
Tiegs, S. D., Costello, D. M., Isken, M. W., Woodward, G., McIntyre, P. B., Gessner, M. O., et al. (2019). Global patterns and drivers of ecosystem functioning in rivers and riparian zones. Sci. Adv. 5, eaav0486. doi: 10.1126/sciadv.aav0486
Vilmin, L., Mogollón, J. M., Beusen, A. H. W., and Bouwman, A. F. (2018). Forms and subannual variability of nitrogen and phosphorus loading to global river networks over the 20th century. Glob. Planet. Change 163, 67–85. doi: 10.1016/j.gloplacha.2018.02.007
Wallace, J. B., Eggert, S. L., Meyer, J. L., and Webster, J. R. (1997). Multiple trophic levels of a forest stream linked to terrestrial litter inputs. Science 277, 102–104. doi: 10.1126/science.277.5322.102
Wang, F., Lin, D., Li, W., Dou, P., Han, L., Huang, M., et al. (2020). Meiofauna promotes litter decomposition in stream ecosystems depending on leaf species. Ecol. Evol. 10, 9257–9270. doi: 10.1002/ece3.6610
Whiles, M. R., and Wallace, J. B. (1997). Leaf litter decomposition and macroinvertebrate communities in headwater streams draining pine and hardwood catchments. Hydrobiologia 353, 107–119. doi: 10.1023/A:1003054827248
Woodward, G., Gessner, M. O., Giller, P. S., Gulis, V., Hladyz, S., Lecerf, A., et al. (2012). Continental-scale effects of nutrient pollution on stream ecosystem functioning. Science 336, 1438–1440. doi: 10.1126/science.1219534
Xiang, H., Zhang, Y., Atkinson, D., and Sekar, R. (2022). Anthropogenic carrion subsidy and herbicide glyphosate depressed leaf-litter breakdown: effects on environmental health in streams. Front. Environ. Sci. 10, 806340. doi: 10.3389/fenvs.2022.806340
Xiang, H. Y., Zhang, Y. X., Atkinson, D., and Sekar, R. (2019). Combined effects of water temperature, grazing snails and terrestrial herbivores on leaf decomposition in urban streams. Peerj 7, 7580. doi: 10.7717/peerj.7580
Keywords: leaf-litter trait, ecosystem functioning, water temperature, leaf-litter breakdown rate, latitude, freshwater, cross-ecosystem subsidy
Citation: Xiang H, Li K, Cao L, Zhang Z and Yang H (2022) Global patterns and drivers of coniferous leaf-litter decomposition in streams and rivers. Front. Ecol. Evol. 10:940254. doi: 10.3389/fevo.2022.940254
Received: 10 May 2022; Accepted: 04 July 2022;
Published: 25 July 2022.
Edited by:
Naicheng Wu, Ningbo University, ChinaReviewed by:
Kai Chen, Hainan University, ChinaCopyright © 2022 Xiang, Li, Cao, Zhang and Yang. This is an open-access article distributed under the terms of the Creative Commons Attribution License (CC BY). The use, distribution or reproduction in other forums is permitted, provided the original author(s) and the copyright owner(s) are credited and that the original publication in this journal is cited, in accordance with accepted academic practice. No use, distribution or reproduction is permitted which does not comply with these terms.
*Correspondence: Zhenxing Zhang, emhhbmd6eDcyNUBuZW51LmVkdS5jbg==; Haijun Yang, eWFuZ0BuZW51LmVkdS5jbg==
Disclaimer: All claims expressed in this article are solely those of the authors and do not necessarily represent those of their affiliated organizations, or those of the publisher, the editors and the reviewers. Any product that may be evaluated in this article or claim that may be made by its manufacturer is not guaranteed or endorsed by the publisher.
Research integrity at Frontiers
Learn more about the work of our research integrity team to safeguard the quality of each article we publish.