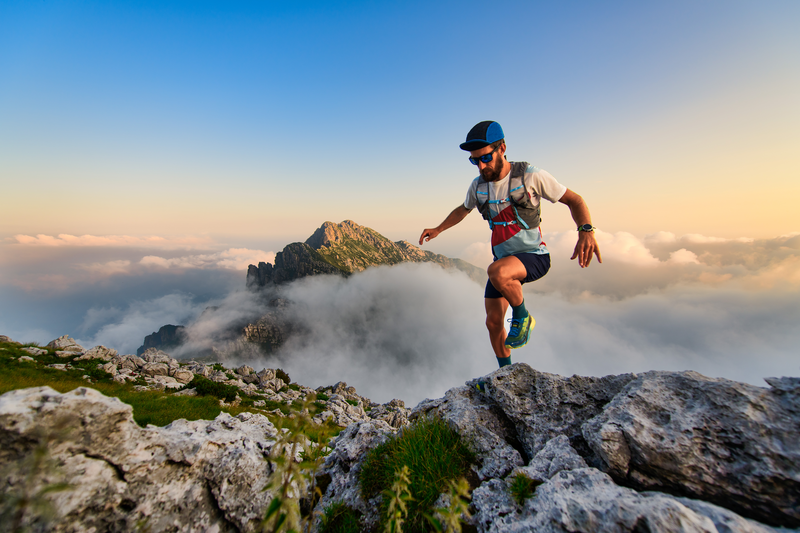
94% of researchers rate our articles as excellent or good
Learn more about the work of our research integrity team to safeguard the quality of each article we publish.
Find out more
BRIEF RESEARCH REPORT article
Front. Ecol. Evol. , 22 July 2022
Sec. Behavioral and Evolutionary Ecology
Volume 10 - 2022 | https://doi.org/10.3389/fevo.2022.939408
This article is part of the Research Topic Social Functions of Bat Vocalizations View all 15 articles
Behavioral traits play a major role in the successful adaptation of wildlife to urban conditions. We investigated and compared the acoustic behavior of free ranging bats in rural (Havelland, Brandenburg) and urban (Berlin city center) green areas (n = 6 sites) to assess possible effects of urbanization on bat vocalizations using automated real-time recordings from May to October 2020 and 2021. We show that foraging and social call activity of commonly occurring bat species was lower in urban areas compared to rural areas. We present data on rural-urban variation in acoustic parameters of echolocation and Type D social calls (produced during flight) using the example of the common pipistrelle Pipistrellus pipistrellus. Calls from urban sites revealed significantly higher end and peak frequencies compared to rural site calls. In addition, urban social calls present a higher degree of complexity as they structurally differed from rural social calls with regard to assemblage and number of call components. Moreover, urban social calls were emitted in a presumably different context than rural calls: antagonistic social calls in urban areas were detected throughout the year and in the acoustic absence of conspecifics and heterospecifics. Our results provide evidence for the ability of P. pipistrellus to modulate temporal and spectral features of echolocation and social calls, as well as patterns of social call production, in order to compensate for constraints imposed by the urban acoustic environment. We suggest that this acoustic behavioral plasticity plays a major role in the degree of adaptation of insectivorous bats to urban habitats.
Urban environments pose various challenges to wildlife, including alteration in disturbance levels (e.g., light or noise) and resource abundance (Garcia et al., 2017). Especially increasing noise and light levels associated with urban development have been identified as the key stressors for animal communities (Longcore and Rich, 2004; Russo and Ancillotto, 2015). Urban habitats are characterized by anthropogenically produced sounds, mainly deriving from traffic and construction works (Warren et al., 2006; Cardoso et al., 2018). Thus, the “urban soundscape”, i.e., the auditory dimension of the urban landscape (Southworth, 1969), is louder and temporally more consistent than most natural sources of noise (Brumm and Slabbekoorn, 2005). While some species are unable to respond to human-induced environmental change (“evolutionarily novel environments”), others quickly adapt to – or even benefit from – cities (=“synanthropic generalist species” or “urban exploiters”, Blair, 1996; Shochat et al., 2006; Lowry et al., 2012). Specific traits or trait combinations are crucial for species to cope with environmental alterations imposed by urbanization. One such important trait is behavioral flexibility (Slabbekoorn and Peet, 2003; Vardi and Berger-Tal, 2022). Individuals occupying urban habitats can exhibit different behavioral responses than conspecifics living in less altered habitats (Miranda, 2017). Some species that use acoustic signals for foraging and communication compensate or minimize the loss in sound transmission caused by anthropogenic noise to adapt to the acoustic properties of an urban area (“acoustic adaptation hypothesis”, Morton, 1975). Songbirds provide an example for such a response to the stressful soundscape of the city. European urban great tits (Parus major) have shorter calls and sing with higher minimum frequencies to increase transmission probability in a noisy environment (Slabbekoorn and den Boer-Visser, 2006). Whether this strategy also applies to urban bats has not yet gained much attention in the scientific literature. While impacts of habitat structure on bat signal design have already been discussed intensively with regard to ecological speciation (e.g., Obrist, 1995; Schnitzler and Kalko, 2001; Luo et al., 2019), we are not aware of any prior studies explicitly investigating the impact of urbanization on bat signal design.
Urban and rural habitats differ across a variety of features (Isaksson et al., 2018), e.g., in terms of the availability and distribution of food resources. For example, the urban heat island effect (Hulley, 2012) causes insects in cities to occur locally concentrated in the same patches over many consecutive nights (Meineke et al., 2013). The most common species of insectivorous bats in cities are those that forage socially on these concentrated prey insects, e.g., found in urban parks and green areas (Threlfall et al., 2017). Thus, rural-urban differences in resource availability may not only lead to changes in individual behavior and space use but also group social dynamics (Russo and Ancillotto, 2015), i.e., how individuals associate or engage in group behaviors in urban environments (Dechmann et al., 2009; Egert-Berg et al., 2018). For example, foraging in groups improves the ability to locate and communicate about sources of food, to avoid predators, and to overcome competition (Sol et al., 2013). Group hunting bat species use foraging-associated vocalizations (“in-flight social calls” of Type D, Bohn and Gillam, 2018; Springall et al., 2019) to either recruit conspecifics to food patches (i.e., food advertisement hypothesis) or defend food resources against competitors (food defense hypothesis, Barlow and Jones, 1997). These in-flight social calls serve a function in interspecific communication (Bohn and Gillam, 2018) and it is expected that they are emitted more frequently when several individuals of the same species are present (Budenz et al., 2009). Given that in urban environments, food resources are more concentrated compared to rural regions, adaptations of bats to the stressful city should also be reflected in this social communication.
Here, we investigate the acoustic behavior of free ranging bats in a rural landscape of Brandenburg and the urban core of Berlin (Germany). We hypothesize that urban environments do not only affect community parameters (richness, diversity, overall and species-specific activity), but also the acoustic behavior of bats. Bat species assigned to the guild of “edge-space aerial foragers” are known for their large repertoire of echolocation signals while foraging (Denzinger and Schnitzler, 2013). We predict that members of this guild, which form part of the rural and the urban bat community, display more variable sound emission patterns (higher variability in temporal and spectral features of echolocation calls) in the city center compared to the rural region. Given the increased sensory challenges in urban environments (buildings, soundscape), we assume that these species will show similar modifications in call structure as foraging bat species responding to the acoustic constraints of highly cluttered habitats (producing higher-frequency and shorter signals, Schnitzler and Kalko, 2001). We furthermore hypothesize that the changed availability of foraging resources and the changed community structure in urban habitats also affect social communication of edge-space foraging species. In the light of their group hunting behavior and their foraging-associated vocalizations, we predict that species of the urban community belonging to this foraging guild display measurable changes in social call structure and complexity.
We used a consistent passive acoustic sampling design over 324 sampling nights and 33,901 full-spectrum recordings to address the following objectives: (1) to identify and compare functional vocalization types of commonly occurring bat species (orientation, foraging, and social communication) between urban and rural areas, (2) to investigate aspects of possible behavioral adaptations and acoustic flexibility of selected urban bat species, and (3) to quantify variation in seasonal, temporal and structural patterns of social call production between rural and urban bats.
We passively recorded free flying bats on three green areas in the city center of Berlin (urban) and on three green areas approx. 70 km to the West in the rural Havelland region of Brandenburg (non-urban), Germany (Supplementary Figure 1 and Supplementary Table 1). A total of 54 weekly surveys (repeated measurements) were conducted per site using stationary calibrated acoustic recorders with omnidirectional ultrasonic microphone (batcorder types 2.0 and 3.0; ecoObs GmbH, Nuremberg, Germany). To account for the influence of habitat type on echolocation behavior, site locations of each category (urban/rural) were selected to have similar habitat features surrounding them (Supplementary Table 1). We sampled data during adequate weather conditions (no rain and/or low wind speed) and deployed devices oriented away from clutter (no foliage, branches, buildings, etc.) to avoid echoes. Due to logistical reasons, two sites (one urban and one rural site) were sampled in parallel each night. Each site was monitored once per week between May and October 2020 and 2021 from 60 min before sunset to 60 min after sunrise so that all locations were sampled for the same amount of time. We made full spectrum recordings in .raw format [sampling rate: 500 kHz, record quality: 20, amplitude resolution: 16 bit, threshold amplitude (sensitivity): −36 dB, post trigger: 400 ms, threshold frequency (sensitivity): 16 kHz].
Echolocation call sequences were firstly analyzed by the automated identification software BatIdent (ecoObs GmbH, Nuremberg, Germany) followed by manual inspection of displayed color spectrograms (sampling frequency 500 Khz, FFT size 256, hamming window, overlap 75%; raw files had been converted to .wav files) using the software BatSound ver. 4.1.4 (Pettersson Elektronik AB, Uppsala, Sweden). We post-validated files comparing measured parameters on spectrograms (shape, peak frequency, duration, and intervals) with those available from the literature (see Supplementary Material). Overall bat activity and species-specific activity was defined as the number of recorded call sequences per sampling site per hour during one night of monitoring (Starik et al., 2018). A call sequence was defined as a consecutive sequence of individual bat calls, composed of at least two pulses (Thomas and West, 1989). When the time interval between calls exceeded the post trigger time of 400 ms, successive sequences were discriminated. Call sequences with more than 10 calls per recording were explored for behavioral characteristics of species occurring in both rural and urban habitats. Foraging activity was defined as the number of times a feeding “buzz” occurred per night. We determined the social call rate for every site as the total number of social calls per night. We followed the related classification systems of Pfalzer and Kusch (2003) and Middleton et al. (2014) to allocate social calls to types [A (aggressive), B (distress), C (isolation, tandem), D (agonistic, song-like)] according to signal structure in the spectrogram.
As specific characteristics of echolocation pulses surrounding social calls enable us to reliably assign social calls to species, only social calls recorded within echolocation sequences were considered (“in-flight social calls”). To explore possible behavioral changes in the acoustic behavior between urban and rural bats, we investigated the extent of intraspecific variability by sound analysis of a subsample of echolocation and social calls performed with the software BatSound ver. 4.1.4 (Pettersson Elektronik AB, Uppsala, Sweden). Because P. pipistrellus was the most abundant species on both urban and rural study sites and because social calls of this species can be clearly distinguished from other species, we focused this analysis solely on this species.
To reduce pseudoreplication, we did not use consecutive calls for call structure analysis. Instead, we analyzed calls from three temporally independent echolocation sequences (with at least three pulses) for each sample night per site (n = 54 × 3 = 162 sequences per site = 162 × 6 = 972 sequences containing 3,469 different echolocation calls). In addition, three temporally independent sequences containing social calls per site per month were used for spectral analysis (n = 12 × 3 = 36 sequences per site = 6 × 36 = 216 sequences containing 506 different social calls). From each echolocation and social call, we measured the following variables: start (fstart) and end (fend) frequencies (for multiharmonic social calls taken from the fundamental component), frequency of maximum energy (fmaxE), and duration. In addition, for echolocation call sequences we determined inter-pulse interval (IPI) and for social calls the number of call components. Frequency values (in kHz) were taken from spectrograms except for “fmaxE,” which was taken from the power spectrum. Time measurements were taken in ms from oscillograms. Measured parameters for echolocation sequences were derived from search-phase calls.
There are different structural categories (types) of social calls in Pipistrellus pipistrellus (Pfalzer and Kusch, 2003). Here we focus on the complex social calls of Type D consisting of several multi-harmonic frequency-modulated syllables (Budenz et al., 2009), which are interspersed between echolocation signals. These calls are either associated with agonistic (intraspecific interactions while foraging, e.g., competition) or advertisement (songflight) behavior (e.g., Budenz et al., 2009; Götze et al., 2020). From all recorded Type D social calls (n = 3,487), we attempted to separate non-mating social calls (e.g., “agonistic calls” emitted during foraging and/or commuting) from “advertisement” or “songflight” calls (i.e., comprising of a longer sequence used by male bats whilst trying to attract a mate) according to Sachteleben and von Helversen (2006). We used the calling rate to distinguish between agonistic and advertisement calls: call series were defined as agonistic if calls were emitted ≤4 times in a series. In addition, agonistic calls used for competing over prey items are frequently followed by a terminal buzz, while advertisement/songflight calls are not. Call series were categorized as advertisement behavior if they comprised at least 10 calls at regular intervals of 0.8 ms. Call sequences with more than 4 and less than 10 calls (n = 109) were not used. We investigated the seasonal distribution of both call types by plotting the nightly social call rate per month. Although recordings revealed signals of up to three individuals at the same time (determined using the pulse interval), the true number of recorded bats remains unknown. Nonetheless, we sought to make tentative assumptions of acoustic interference from vocalizing conspecifics and heterospecifics on agonistic social call production and calculated the total proportion of recordings containing agonistic calls depending on the presence of other P. pipistrellus individuals or other co-occurring species (Eptesicus serotinus or Nyctalus noctula) over all sampling nights. We furthermore investigated the structure of social calls by calculating the proportional nightly distribution of recorded sequences with only one or with multiple social calls. Also, we calculated the proportional nightly distribution of recorded social call sequences differentiated by the number of syllables.
In all analyses, rural sites were pooled as were urban sites. For statistical analysis, we used non-parametric pairwise comparisons between pooled data for urban and rural sites. Species diversity (Shannon diversity index H) was calculated for each night at each sampling site. Medians for H, overall and species-specific bat activity, foraging activity, and social call rate were compared between the years 2020 and 2021 for all sites using Mann–Whitney U-test or Kruskal–Wallis test. Since no statistical differences between years were detected for any site, data from both study years were pooled to obtain a general pattern of overall bat activity. To determine, whether bat activity, foraging activity or social call rate, as well as echolocation and social call parameters, differed between urban and rural sites, we used the Mann–Whitney U-test. For all statistical tests, the significance level was set to α = 0.05.
Based on 12,304 (city) and 21,597 (rural) full-spectrum recordings over 324 sampling nights, we detected four bat species (and one sonotype) in the city center: Pipistrellus pipistrellus, Eptesicus serotinus, Nyctalus noctula, and Vespertilio murinus. On rural sites, five additional species (Myotis nattereri, Myotis daubentonii, Nyctalus leisleri, Pipistrellus pygmaeus, Pipistrellus nathusii) and three sonotypes have been recorded (Figure 1A). Sonotypes are Nycmi (Nyctalus leisleri, Eptesicus serotinus, Vespertilio murinus), Mkm (Myotis brandtii, Myotis mystacinus), and Plecotus (Plecotus auritus, Plecotus austriacus). Pipistrellus pipistrellus was the most frequently detected species in the entire study area both on urban (9,328 recordings) and rural (10,288 recordings) study sites.
Figure 1. Community-characterizing attributes and functional vocalization types on urban and rural study sites (n = 162 paired sample nights). (A) Boxplots of species diversity as measured by Shannon’s H. (B) Overall and species-specific bat activity (recordings/h), foraging activity (feeding buzzes/night), and social call rate1 (calls/night); medians are indicated by the center point of the boxes and interquartile ranges by the extent of the whiskers. 1All social call types. *P < 0.05.
Our data indicate an overall significant decrease in mean species diversity (z = 15.49, P < 0.001, N = 162, Figure 1A) on urban sites compared to rural sites. While overall bat activity did not differ between urban and rural sites (z = 12.71, P = 0.001, N = 162) foraging activity was significantly lower (z = 5.76, p = 0.014, N = 162) and social call rate significantly higher (z = 2.41, P < 0.001, N = 162) on urban sites compared to rural sites. On the species level, we found a significant increase in activity levels of urban exploiters P. pipistrellus (z = 1.89, P = 0.0069, N = 162) and E. serotinus (z = 2.67, P = 0.0092, N = 162) compared to their non-urban counterparts (Figure 1B). For N. noctula, however, activity levels were significantly lower in urban compared to non-urban habitats (z = 6.29, P = 0.0001, N = 162). About 65% of the recordings (16,664 out of 25,357 sequences) from these three species occurring at both urban and rural sites consisted of more than 10 calls and were suitable for further investigating the functional call types of the recorded sequences. For P. pipistrellus, E. serotinus, and N. noctula we observed a decreased foraging activity at urban study sites which revealed statistical significance for N. noctula (z = 6.82, P < 0.001, N = 162) (Figure 1B). Further, we found a clear difference in the median social call rate for P. pipistrellus (z = 5.69, P < 0.001), which was approximately 10-fold higher for the urban sites compared to the rural sites.
The acoustic behavior of P. pipistrellus subsequently was investigated in more detail. Analyzed search phase calls from urban and rural sites showed clear differences with respect to several spectral characteristics: the average end frequency of P. pipistrellus calls was significantly higher in the city compared to rural sites (z = 32.933, P < 0.001) (Table 1). The same pattern applies to the average peak frequency (fmaxE) (z = 20.756, P < 0.001) (Table 1). We found no such shifts in start frequency (z = 0.496, P = 0.624). Search phase calls differed also with regard to temporal characteristics. Calls from urban sites were shorter in duration (z = 38.024, P < 0.001) and involved shorter inter pulse intervals (z = 38.34, P < 0.001) compared to calls recorded on rural study sites.
Table 1. Mean ± SD for time and frequency variables of echolocation and social calls of urban and rural Pipistrellus pipistrellus with probability level for significance of differences (Mann–Whitney U-test).
Type D calls were the only social call type recorded during the study. These social calls consisted of 3–5 multiharmonic and frequency-modulated syllables with start frequencies of 35.22 ± 1.39 kHz (urban) and 34.94 ± 3.27 kHz (rural). We found a significant increase in average peak frequency (z = 22.097, P < 0.001) and minimum frequency (z = 25.587, P < 0.001) and a significant decrease of call duration (z = 46.364, P < 0.001) in social calls of urban bats compared to social calls of rural bats (Table 1).
The majority of social calls were emitted during foraging flight, indicated by feeding buzzes. On urban sites, agonistic social calls were recorded almost throughout the entire activity season from May to October (Figure 2A) and were evenly distributed over 78% of all recording nights. The seasonal agonistic social call rate on rural sites followed a different pattern with an increasing intensity from late spring onward (almost no social calls in May), a peak in July followed by a decreasing intensity to almost zero social calls in autumn (October). Overall, the agonistic social call rate was approximately ten-fold lower on the rural sites compared to the urban sites, even at its maximum level in August. The variation in the social call rate across nights was notably higher on the rural sites, especially from July to September. At urban sites, advertisement calls were documented in 29% of nightly samples in June and until late in October, whereas at rural sites advertisement calls were registered in very low numbers and exclusively in August and September.
Figure 2. Context of P. pipistrellus social call production in urban and rural green areas. (A) Boxplots of median social call rates indicating agonistic and advertisement behavior over the sample months. Social call rate, number of social calls per night. (B) Proportion of Type D foraging associated (agonistic) call sequences in relation to the presence of conspecifics (P × P) and heterospecifics (P × N, P × E, P × other; P, P. pipistrellus; N, N. noctula; E, E. serotinus). *P < 0.05.
Social call structure of foraging associated (agonistic) call sequences showed a strong divergence between recorded sequences from urban and rural sites (Figure 2B): on urban sites, more than 50% of these recordings only contained calls from a single P. pipistrellus individual. Recordings that included calls from conspecifics (other P. pipistrellus) or heterospecifics (E. serotinus and N. noctula) each only made up 10%. On the rural sites, in contrast, only 20% of the agonistic call sequences neither contained calls from conspecifics nor heterospecifics. Agonistic call sequences containing calls of conspecifics made up more than 50% of the recordings and call sequences containing calls from E. serotinus, N. noctula or other species accounted for less than 10% of the recordings.
At urban sites, the social call structural pattern (Figure 3A) markedly changed after the first half of the night. While 87.8% of Type D foraging associated call sequences in the period from 1 h before sunset to 3 h after sunset contained multiple subsequent calls, recordings with only one call per sequence were rarely registered (Figure 3B). During the second half of the night only 36.7% of recordings were characterized by multiple calls per recording. In the early morning hours (8–9 h after sunset), the proportion in the number of recordings with multiple (43.4%) and single calls (51.2%) was similar. At the rural sites, recordings characterized by only one social call per recording were almost equally distributed over the night hours; only in the period 3–5 h after sunset a comparable number of recordings of both types has been registered (Figure 3B).
Figure 3. Structural patterns of P. pipistrellus’ Type D social calls on urban and rural study sites. Examples of different composition (single vs. multiple calls within a sequence) and call types (3, 4, and 5 syllables); green boxes show social calls interspersed between echolocation calls (A). Nightly proportional distribution of social call sequences with single calls (white diamonds) and multiple calls (dark diamonds) (B), and nightly proportional distribution of sequences with different syllables (C).
Type D foraging associated call sequences from urban sites and rural sites also differed with regard to call structure (number of call components, i.e., syllables) (Figure 3C): from 1 h before sunset to 7–8 h after sunset, recordings were characterized by 4- or 5-syllabic calls. On the rural sites, recordings were characterized mainly by 3-syllabic calls, while 4- or 5-syllabic calls were registered in only 10–30% of the recordings independent of the time of night.
The aim of this study was to investigate and compare the acoustic behavior of free ranging bats in rural (Brandenburg district) and urban (Berlin city center) green areas to assess possible effects of urbanization on bat vocalizations. We showed that bat species richness and diversity were significantly lower in urban areas compared to rural sites (Figure 1) and that several species were not detected in urban environments (“urban losers”), while activity levels of remaining species increased (“urban exploiters”). Urban settings often exhibit a higher degree of structural complexity compared to rural environments, implying more complex sensory demands on echolocating bats (Corcoran and Moss, 2017). Thus, we hypothesized that urban exploiters display more variable sound emission patterns in the city center compared to the rural region. Using the vocalizations of the common pipistrelle (Pipistrellus pipistrellus), we show that echolocation calls recorded at urban sites differ from those recorded at rural sites. Pipistrellus pipistrellus hunts in a range of habitat types, e.g., in parks, in woodland, over water, over open fields, and near clutter (e.g., vegetation), where mainly Diptera (e.g., mosquitos) are caught by aerial hawking (Racey and Swift, 1985). Wings and echolocation frequency are well adapted to foraging with high maneuverability along tree lines and edges of forests (Norberg and Rayner, 1987), suggesting optimal conditions to forage in urban areas with similar “edge terrains”. Urban calls are significantly shorter in duration with higher peak and terminal frequencies compared to calls recorded in rural areas (Table 1). Despite being statistically significant, data from urban and rural bat calls are characterized by large standard deviations (SDs; Table 1). These large SDs are an expression of the ability of Pipistrellus pipistrellus to modulate calls along a wide continuum, reflecting behavioral adaptation to different habitat conditions among individuals (Fenton, 1994). This plasticity in echolocation behavior confirms that bats foraging in edge-space habitats, such as Pipistrellus pipistrellus, show relatively high variability in call parameters (Schnitzler and Kalko, 2001; Boonman and Schnitzler, 2004).
To further interpret our findings, two explanations seem conceivable. Changes in echolocation call parameters might be a result of (1) changes in habitat parameters according to the acoustic adaptation hypothesis (AAH; Morton, 1975) and/or (2) changes in conspecific activity and community composition. Following the AAH, it can be assumed that signals may be “improved” for optimal transmission and/or recognition in order to overcome the constraints imposed by the soundscape of the city, the physical structure of the urban habitats (e.g., buildings) and the resulting complex interaction of these factors. In the light of the assumption that inter-individual interactions lead to the observed differences in echolocation call parameters, two different theories exist: while the attention hypothesis is based on the idea that changes in call parameters are linked with exploring nearby conspecifics (Götze et al., 2020), the jamming avoidance hypothesis assumes that changes in call parameters occur to reduce acoustic interference with other echolocating bats (e.g., Bartonička et al., 2007; Necknig and Zahn, 2011).
Surprisingly, we found that a certain type of social calls (type D emitted during flight) is also modified in urban P. pipistrellus. These calls are characterized by significantly higher end and peak frequencies and longer durations than those from rural sites (Table 1). Moreover, we show that social calls in urban areas are not only altered with regard to spectral and temporal characteristics, but also concerning quantity, quality, and seasonal distribution. In fact, the urban social call rate is about as twice as high compared to rural areas (Figure 1). This effect is particularly pronounced with regard to agonistic call sequences (Figure 2). But what are the implications of these significant differences? Comparable findings are scarce, as social calls are mainly studied in or in the vicinity of roosts and only rarely in free-flying individuals in the field (Bohn and Gillam, 2018; Springall et al., 2019).
The observed changes in social call behavior are mainly related to agonistic foraging interactions of the common pipistrelle. Agonistic territorial behavior occurs when a particular resource is clumped (Racey and Swift, 1985; Chaverri et al., 2018). This applies to urban environments: due to microclimatic changes compared to rural areas, insects in cities show extended flight phenologies, beginning already in early spring and lasting until late autumn (Merckx et al., 2021). However, they occur locally concentrated in the same patches over many consecutive nights (Meineke et al., 2013). In rural areas, insects are characterized by a much shorter seasonality but are more evenly distributed over the landscape compared to the city. This different pattern in resource availability explains the high agonistic social call rate of P. pipistrellus from May throughout September over the entire period of insect availability in the city (constant availability but spatially very clumped). In rural areas, by contrast, agonistic social call rate of bats was generally much lower (food resource not spatially clumped), even at times of high food availability during summer months. Besides seasonal and quantitative effects, agonistic calls differ also in terms of quality. Recordings from urban areas contain several subsequent calls of 4–5 syllables, while the majority of recordings from rural areas are characterized by single calls of 3 syllables, suggesting a higher complexity of urban P. pipistrellus calls. Furthermore, social calls in urban areas are emitted in a presumably different context compared to rural areas: the majority of agonistic call sequences from urban areas is emitted in the acoustic absence of conspecifics. “Acoustic absence” does not imply real absence but could rather indicate single individuals emitting social calls to delimit the spatially limited (clumped) food resources in urban areas against conspecifics. Differently structured calls could encode different information, e.g., individually distinct signatures facilitating to explore nearby conspecifics. This could be useful to mediate subsequent interactions when multiple bats are present at the same foraging site. We suggest that bats in urban areas advertise their presence with more complex calls to repel other individuals and claim food and territory. This would result in reduced competition while facilitating location of prey in an eased acoustic and physical environment (Cvikel et al., 2015). Following Barlow and Jones (1997) we suggest that P. pipistrellus increases emission of social calls when foraging in areas with low insect densities or clumped distribution and that these calls result in decreased activity of other bats (conspecifics and heterospecifics) in the very same area.
However, changes in social call behavior of urban Pipistrellus pipistrellus are not only limited to agonistic calls, but also to advertisement calls. Advertisement calls were detected much earlier in the recordings from urban areas (June) compared to rural areas (August). Usually, songflight behavior peaks in the mating period. For example, Sachteleben and von Helversen (2006) found the greatest number of songflights of P. pipistrellus to occur in September. Although urban microclimate-induced shifts in courtship activity could be a possible explanation for the observation of advertisement calls in June in our study (late spring mating, Jahelková, 2011), we suggest that the songflight calls emitted outside the mating period in cities may serve another social function. For example, these calls may be related to (early) summer swarming behavior of pipistrelles (Sendor et al., 2000) in the urban environment, which is supposed to maximize reproductive success through transfer of information on hibernacula.
In summary, we can confirm that the opportunistic common pipistrelle, known for its ability to adapt to urban environments (Lintott et al., 2016; Straka et al., 2019), is ecologically highly flexible (Dietz et al., 2009). Here, we show that this ecological flexibility goes along with a high degree of flexibility in social vocal behavior. However, there are two interdependent aspects that cannot be considered separately. Changes in vocalization behavior in urban settings can be the result of (1) environmental changes and a more complex urban environment (i.e., top-down processes: noise levels, changes in light conditions, spatial complexity of structures, changes in food availability) and/or (2) changes in the community structure of urban populations (i.e., bottom-up processes: fewer species). At this point, it is not possible to clarify, whether the observed acoustic flexibility of P. pipistrellus is related to environmental or community changes or a combination of both.
It remains unclear, whether the observed differences in acoustic behavior (echolocation and social) are a consequence of the mentioned intraspecific variability (plasticity), or possibly even reflect adaptive evolution. Evidence suggests that selection can cause evolutionary shifts within only a few generations, leading to rapid microevolution with substantial implications (Miranda, 2017; Liker, 2020). Divergence in acoustic behavior may emerge as a result of either direct ecological selection, genetic drift, cultural drift, or indirect ecological selection (Jiang et al., 2015). Fundamental changes in food resources could induce adaptations at several levels and bats could respond quickly by modifying their food preference and feeding behavior in urban environments. This could lead to geographical variation of call parameters at different spatial scales (Jiang et al., 2015; López-Baucells et al., 2018). Several urban characteristics may exert strong and sometimes new selection pressures on organisms. We have shown that social calls of the common pipistrelle are structurally more complex in urban areas compared to rural areas. This illustrates, that urban environments can alter environmental and physiological conditions that are key for the production and maintenance of signal quality. Thus, urbanization may generally have a strong impact on the expression and effectiveness of animal signals, such as mating signals. Urbanization is therefore likely to lead to changes in sexual selection pressures (Cronin et al., 2022), resulting in signal divergence between urban and non-urban populations and finally initiating possible speciation processes (Halfwerk et al., 2019; Halfwerk, 2021).
On the basis of our results, the following perspectives for future studies emerge: Specific environmental factors (noise, light, etc.) vary across urban and rural green areas and bats seem to be able to adjust their vocal behavior to these specific local conditions. Thus, studying intermediate environments between urban and rural habitats may help to assess the processes that lead to the changes in echolocation and social call design. Our study gives reason to more explicitly focus on the social-communicative aspects of species’ adaptations to evolutionary novel environments, such as urban environments. Our study also encourages to explore, whether social organization (group size, cooperative foraging behavior, etc.) acts as a driver of acoustic phenotype plasticity and communicative complexity (Luo et al., 2017; Knörnschild et al., 2019). Changes in communication behavior as a response to urbanization seem to strongly depend on the overall species community. As this interspecific community-level social structure affects the distribution of species in space and time (Goodale et al., 2010), it seems worthwhile considering multiple species to identify the main urban ecological processes that affect ecological interactions.
The raw data supporting the conclusions of this article will be made available by the authors, without undue reservation.
Ethical review and approval was not required for the animal study because we passively collected data using only sound recordings and as we did not conduct any collection or handling of animals, our research was not subject to institutional or governmental regulations. Field studies did not disturb endangered or protected species. No privately owned or protected land was accessed during the recordings.
NS and TG designed the study and wrote the manuscript. NS collected the data, conducted the acoustic analyses, and performed the data analyses. Both authors contributed to the article and approved the submitted version.
The authors declare that the research was conducted in the absence of any commercial or financial relationships that could be construed as a potential conflict of interest.
All claims expressed in this article are solely those of the authors and do not necessarily represent those of their affiliated organizations, or those of the publisher, the editors and the reviewers. Any product that may be evaluated in this article, or claim that may be made by its manufacturer, is not guaranteed or endorsed by the publisher.
We thank the Zwillenberg-Tietz Stiftung (www.zwillenberg-tietz-stiftung.de) for providing technical equipment to NS (affiliated with Deutsche Fledermauswarte e.V.). We also thank M. Fritze for his advice during fieldwork. Finally, we wish to thank the reviewers for their valuable comments and helpful feedback to improve this manuscript.
The Supplementary Material for this article can be found online at: https://www.frontiersin.org/articles/10.3389/fevo.2022.939408/full#supplementary-material
Barlow, K. E., and Jones, G. (1997). Function of pipistrelle social calls: field data and a playback experiment. Anim. Behav. 53, 991–999. doi: 10.1006/anbe.1996.0398
Bartonička, T., Řehák, Z., and Gaisler, J. (2007). Can pipistrelles, Pipistrellus pipistrellus (Schreber, 1774) and Pipistrellus pygmaeus (Leach, 1825), foraging in a group, change parameters of their signals? J. Zool. 272, 194–201. doi: 10.1111/j.1469-7998.2006.00255.x
Blair, R. B. (1996). Land use and avian species diversity along an urban gradient. Ecol. Appl. 6, 506–519.
Bohn, K. M., and Gillam, E. H. (2018). In-flight social calls: a primer for biologists and managers studying echolocation. Can. J. Zool. 96, 787–800.
Boonman, A., and Schnitzler, H. U. (2004). Frequency modulation patterns in the echolocation signals of two vespertilionid bats. J. Comp. Physiol. A Neuroethol. Sens. Neural Behav. Physiol. 191, 13–21. doi: 10.1007/s00359-004-0566-8
Brumm, H., and Slabbekoorn, H. (2005). Acoustic communication in noise. Adv. Study Behav. 35, 151–209. doi: 10.1016/S0065-3454(05)35004-2
Budenz, T., Heib, S., and Kusch, J. (2009). Functions of bat social calls: the influence of local abundance, interspecific interactions and season on the production of pipistrelle (Pipistrellus pipistrellus) type D social calls. Acta Chiropt. 11, 173–182. doi: 10.3161/150811009X465794
Cardoso, G. C., Hu, Y., and Francis, C. D. (2018). The comparative evidence for urban species sorting by anthropogenic noise. R. Soc. Open Sci. 5:172059. doi: 10.1098/rsos.172059
Chaverri, G., Ancillotto, L., and Russo, D. (2018). Social communication in bats. Biol. Rev. 93, 1938–1954. doi: 10.1111/brv.12427
Corcoran, A. J., and Moss, C. F. (2017). Sensing in a noisy world: lessons from auditory specialists, echolocating bats. J. Exp. Biol. 220, 4554–4566. doi: 10.1242/jeb.163063
Cronin, A. D., Smit, J. A., Muñoz, M. I., Poirier, A., Moran, P. A., Jerem, P., et al. (2022). A comprehensive overview of the effects of urbanisation on sexual selection and sexual traits. Biol. Rev. Camb. Philos. Soc. doi: 10.1111/brv.12845 [Epub ahead of print].
Cvikel, N., Levin, E., Hurme, E., Borissov, I., Boonman, A., Amichai, E., et al. (2015). On-board recordings reveal no jamming avoidance in wild bats. Proc. R. Soc. Lond. B Biol. Sci. 282:20142274. doi: 10.1098/rspb.2014.2274
Dechmann, D. K. N., Heucke, S. L., Giuggioli, L., Safi, K., Voigt, C. C., and Wikelski, M. (2009). Experimental evidence for group hunting via eavesdropping in echolocating bats. Proc. R. Soc. Lond. B Biol. Sci. 276, 2721–2728. doi: 10.1098/rspb.2009.0473
Denzinger, A., and Schnitzler, H. U. (2013). Bat guilds, a concept to classify the highly diverse foraging and echolocation behaviors of microchiropteran bats. Front. Physiol. 4:164. doi: 10.3389/fphys.2013.00164
Dietz, C., von Helversen, O., and Nill, D. (2009). Bats of Britain. Europe and Northwest Africa. London: A & C Black.
Egert-Berg, K., Hurme, E. R., Greif, S., Goldstein, A., Harten, L., and Herrera, M. L. G. (2018). Resource ephemerality drives social foraging in bats. Curr. Biol. 28, 3667–3673.e5. doi: 10.1016/j.cub.2018.09.064
Fenton, M. B. (1994). Assessing signal variability and reliability: ‘to thine ownself be true’. Anim. Behav. 47, 757–764. doi: 10.1006/anbe.1994.1108
Garcia, C. M., Suárez-Rodríguez, M., and López-Rull, I. (2017). “Becoming citizens: avian adaptations to urban life,” in Ecology and Conservation of Birds in Urban Environments, eds E. Murgui and M. Hedblom (Basel: Springer), 91–112. doi: 10.1007/978-3-319-43314-1
Goodale, E., Beauchamp, G., Magrath, R. D., Nieh, J. C., and Ruxton, G. D. (2010). Interspecific information transfer influences animal community structure. Trends Ecol. Evol. 25, 354–361.
Götze, S., Denzinger, A., and Schnitzler, H. U. (2020). High frequency social calls indicate food source defense in foraging Common pipistrelle bats. Sci. Rep. 10:5764. doi: 10.1038/s41598-020-62743-z
Halfwerk, W. (2021). How should we study urban speciation? Front. Ecol. Evol. 8:573545. doi: 10.3389/fevo.2020.573545
Halfwerk, W., Blaas, M., Kramer, L., Hijner, N., Trillo, P. A., Bernal, X. E., et al. (2019). Adaptive changes in sexual signalling in response to urbanization. Nat. Ecol. Evol. 3, 374–380. doi: 10.1038/s41559-018-0751-8
Hulley, M. E. (2012). “The urban heat island effect: causes and potential solutions,” in Metropolitan Sustainability - Understanding and Improving the Urban Environment, ed. F. Zeman (Cambridge: Woodhead Publishing), 79–98.
Isaksson, C., Rodewald, A. D., and Gil, D. (2018). Behavioural and ecological consequences of urban life in birds. Front. Ecol. Evol. 6:50. doi: 10.3389/fevo.2018.00050
Jahelková, H. (2011). Unusual social calls of Nathusius’ pipistrelle (Vespertilionidae, Chiroptera) recorded outside the mating season. J. Vertebr. Biol. 60, 25–30.
Jiang, T., Wu, H., and Feng, J. (2015). Patterns and causes of geographic variation in bat echolocation pulses. Integr. Zool. 10, 241–256. doi: 10.1111/1749-4877.12129
Knörnschild, M., Fernandez, A. A., and Nagy, M. (2019). Vocal information and the navigation of social decisions in bats: is social complexity linked to vocal complexity? Funct. Ecol. 34, 322–331. doi: 10.1111/1365-2435.13407
Liker, A. (2020). Biologia Futura: adaptive changes in urban populations. Biol. Futur. 71, 1–8. doi: 10.1007/s42977-020-00005-9
Lintott, P. R., Barlow, K., Bunnefeld, N., Briggs, P., Gajas Roig, C., and Park, K. J. (2016). Differential responses of cryptic bat species to the urban landscape. Ecol. Evol. 6, 2044–2052. doi: 10.1002/ece3.1996
Longcore, T., and Rich, C. (2004). Ecological light pollution. Front. Ecol. Environ. 2, 191–198. doi: 10.1890/1540-92952004002[0191:ELP]2.0.CO;2
López-Baucells, A., Torrent, L., Rocha, R., Pavan, A. C., Bobrowiec, P. E. D., and Meyer, C. F. (2018). Geographical variation in the high-duty cycle echolocation of the cryptic common mustached bat Pteronotus cf. rubiginosus (Mormoopidae). Bioacoustics 27, 341–357. doi: 10.1080/09524622.2017.1357145
Lowry, H., Lill, A., and Wong, B. B. (2012). Behavioural responses of wildlife to urban environments. Biol. Rev. 88, 537–549. doi: 10.1111/brv.12012
Luo, B., Huang, X., Li, Y., Lu, G., Zhao, J., Zhang, K., et al. (2017). Social call divergence in bats: a comparative analysis. Behav. Ecol. 28, 533–540. doi: 10.1093/beheco/arw184
Luo, B., Leiser-Miller, L., Santana, S. E., Zhang, L., Liu, T., Xiao, Y., et al. (2019). Echolocation call divergence in bats: a comparative analysis. Behav. Ecol. Sociobiol. 73:154. doi: 10.1007/s00265-019-2766-9
Meineke, E. K., Dunn, R. R., Sexton, J. O., and Frank, S. D. (2013). Urban warming drives insect pest abundance on street trees. PLoS One 8:e59687. doi: 10.1371/journal.pone.0059687
Merckx, T., Nielsen, M. E., Heliölä, J., Kuussaari, M., Pettersson, L. B., Pöyry, J., et al. (2021). Urbanization extends flight phenology and leads to local adaptation of seasonal plasticity in Lepidoptera. Proc. Natl. Acad. Sci. U.S.A. 118:e2106006118. doi: 10.1073/pnas.2106006118
Middleton, N., Froud, A., and French, K. (2014). Social Calls of the Bats of Britain and Ireland. London: Pelagic Publishing Ltd.
Miranda, A. C. (2017). “Mechanisms of behavioural change in urban animals: the role of microevolution and phenotypic plasticity,” in Ecology and Conservation of Birds in Urban Environments, eds E. Murgui and M. Hedblom (Basel: Springer), 113–132. doi: 10.1007/978-3-319-43314-1_7
Morton, E. S. (1975). Ecological sources of selection on avian sounds. Am. Nat. 109, 17–34. doi: 10.1086/282971
Necknig, V., and Zahn, A. (2011). Between-species jamming avoidance in Pipistrelles? J. Comp. Physiol. A Neuroethol. Sens. Neural Behav. Physiol. 197, 469–473. doi: 10.1007/s00359-010-0586-5
Norberg, U. M., and Rayner, J. M. (1987). Ecological morphology and flight in bats (Mammalia; Chiroptera): wing adaptations, flight performance, foraging strategy and echolocation. Philos. Trans. R. Soc. B Biol. Sci. 316, 335–427.
Obrist, M. K. (1995). Flexible bat echolocation: the influence of individual, habitat and conspecifics on sonar signal design. Behav. Ecol. Sociobiol. 36, 207–219. doi: 10.1007/BF00177798
Pfalzer, G., and Kusch, J. (2003). Structure and variability of bat social calls: implications for specificity and individual recognition. J. Zool. 261, 21–33. doi: 10.1017/S0952836903003935
Racey, P. A., and Swift, S. M. (1985). Feeding ecology of Pipistrellus pipistrellus (Chiroptera: Vespertilionidae) during pregnancy and lactation. I. Foraging behaviour. J. Anim. Ecol. 54, 205–215. doi: 10.2307/4631
Russo, D., and Ancillotto, L. (2015). Sensitivity of bats to urbanization: a review. Mamm. Biol. 80, 205–212. doi: 10.1016/j.mambio.2014.10.003
Sachteleben, J., and von Helversen, O. (2006). Songflight behaviour and mating system of the pipistrelle bat (Pipistrellus pipistrellus) in an urban habitat. Acta Chiropt. 8, 391–401. doi: 10.3161/150811006779398609
Schnitzler, H. U., and Kalko, E. K. (2001). Echolocation by insect-eating bats: we define four distinct functional groups of bats and find differences in signal structure that correlate with the typical echolocation tasks faced by each group. Bioscience 51, 557–569.
Sendor, T., Kugelschafter, K., and Simon, M. (2000). Seasonal variation of activity patterns at a pipistrelle (Pipistrellus pipistrellus) hibernaculum. Myotis 38, 91–109.
Shochat, E., Warren, P. S., Faeth, S. H., McIntyre, N. E., and Hope, D. (2006). From patterns to emerging processes in mechanistic urban ecology. Trends Ecol. Evol. 21, 186–191. doi: 10.1016/j.tree.2005.11.019
Slabbekoorn, H., and den Boer-Visser, A. (2006). Cities change the songs of birds. Curr. Biol. 16, 2326–2331.
Slabbekoorn, H., and Peet, M. (2003). Birds sing at a higher pitch in urban noise. Nature 424, 267–267. doi: 10.1038/424267a
Sol, D., Lapiedra, O., and González-Lagos, C. (2013). Behavioural adjustments for a life in the city. Anim. Behav. 85, 1101–1112.
Springall, B. T., Li, H., and Kalcounis-Rueppell, M. C. (2019). The in-flight social calls of insectivorous bats: species specific behaviors and contexts of social call production. Front. Ecol. Evol. 7:441. doi: 10.3389/fevo.2019.00441
Starik, N., Göttert, T., Heitlinger, E., and Zeller, U. (2018). Bat community responses to structural habitat complexity resulting from management practices within different land use types—a case study from north-eastern Germany. Acta Chiropt. 20, 387–405. doi: 10.3161/15081109ACC2018.20.2.010
Straka, T. M., Wolf, M., Gras, P., Buchholz, S., and Voigt, C. C. (2019). Tree cover mediates the effect of artificial light on urban bats. Front. Ecol. Evol. 7:91. doi: 10.3389/fevo.2019.00091
Thomas, D. W., and West, S. D. (1989). “Sampling methods for bats,” in Wildlife Habitat Relationships: Sampling Procedures for Pacific Northwest Vertebrates, Gen. Tech. Rep. PNW-GTR-243, eds L. F. Ruggiero and A. B. Carey (Portland, OR: U.S. Department of Agriculture, Forest Service, Pacific Northwest Research Station), 1–20.
Threlfall, C. G., Mata, L., Mackie, J. A., Hahs, A. K., Stork, N. E., Williams, N. S., et al. (2017). Increasing biodiversity in urban green spaces through simple vegetation interventions. J. Appl. Ecol. 54, 1874–1883. doi: 10.1111/1365-2664.12876
Vardi, R., and Berger-Tal, O. (2022). Environmental variability as a predictor of behavioral flexibility in urban environments. Behav. Ecol. 33, 573–581. doi: 10.1093/beheco/arac002
Keywords: acoustic flexibility, common pipistrelle, intra- and interspecific communication, signal design, urbanization
Citation: Starik N and Göttert T (2022) Bats adjust echolocation and social call design as a response to urban environments. Front. Ecol. Evol. 10:939408. doi: 10.3389/fevo.2022.939408
Received: 09 May 2022; Accepted: 01 July 2022;
Published: 22 July 2022.
Edited by:
Mirjam Knörnschild, Leibniz-Institut für Evolutions- und Biodiversitätsforschung, GermanyReviewed by:
Olga Heim, Leibniz Institute for Zoo and Wildlife Research (LG), GermanyCopyright © 2022 Starik and Göttert. This is an open-access article distributed under the terms of the Creative Commons Attribution License (CC BY). The use, distribution or reproduction in other forums is permitted, provided the original author(s) and the copyright owner(s) are credited and that the original publication in this journal is cited, in accordance with accepted academic practice. No use, distribution or reproduction is permitted which does not comply with these terms.
*Correspondence: Nicole Starik, bmljb2xlLnN0YXJpa0BodS1iZXJsaW4uZGU=
Disclaimer: All claims expressed in this article are solely those of the authors and do not necessarily represent those of their affiliated organizations, or those of the publisher, the editors and the reviewers. Any product that may be evaluated in this article or claim that may be made by its manufacturer is not guaranteed or endorsed by the publisher.
Research integrity at Frontiers
Learn more about the work of our research integrity team to safeguard the quality of each article we publish.