- 1Department of Archaeology, Faculty of History, Vilnius University, Vilnius, Lithuania
- 2Department of History, Faculty of Humanities, Kyrgyz Manas University, Bishkek, Kyrgyzstan
- 3Faculty of Archaeology, Leiden University, Leiden, Netherlands
This paper presents archaeobotanical research results from an occupation horizon of the Chap II site left by the earliest known farming community in the Central Tien Shan mountains in the current territory of Kyrgyzstan. The archaeobotanical samples were recovered from well-defined contexts in domestic waste pits, house floors, fireplaces, and an oven, all of which date to a narrow period of occupation between 2474 and 2162 cal BCE (based on n-14 AMS dates). The archaeobotanical assemblage is dominated by the SW package crops of bread wheat and naked barley. Those are the only species to have progressed further east across the mountain ranges of Central Asia during the earliest wave of crop dispersal. However, other species in small quantities were also identified at the Chap II site, such as T. durum/turgidum and T. carthlicum, possibly glume wheats and hulled barley. Here, we argue that the dominant compact morphotypes seen only in bread wheat and naked barley caryopses hint toward a selection for the specific adaptive traits of cultigens that enabled successful agriculture in high-altitude ecogeographies. Large variations in cereal caryopses size possibly indicate that crops endured stress (e.g., insufficient nutrients, water, or other) during plant development. More research is needed for a better understanding of the developmental plasticity between different crop species and the formation of unique landraces in diverse environmental niches in the past.
Introduction
The adaptation of plant species to novel ecotones as they dispersed away from their centers of domestication has been a subject of many scientific studies (Coward et al., 2008; Spengler et al., 2016; Fuller and Lucas, 2017; Stevens and Fuller, 2017). These adaptation processes are complex and multi-dimensional for the successful adaptation and cultivation of novel crops in new environmental circumstances under different climatic and day length regimes. When and what species first reached any given novel geographical regions very much depends on plant adaptability, genetic changes in the crops themselves, changes in the crop package which involve new additional crops that allow minimization of agricultural risk, human culinary preferences, and the human ability to facilitate crop cultivation with plowing, irrigation, or manuring (Liu et al., 2016; Fuller and Lucas, 2017; Lister et al., 2018; Motuzaite Matuzeviciute, 2018). To assess how well crops adapt to novel environments from archaeobotanical perspectives, we can look at several criteria such as crop biodiversity or cereal caryopses dimensionality. It has previously been shown that crop response to climatic stresses is expressed in high variability of caryopses sizes (Motuzaite Matuzeviciute et al., 2015, 2018, 2021a) or reduction in the overall caryopses size (Fuller et al., 2017). On the contrary, the reduction of caryopses size in wheat and barley in monsoonal China has led researchers to infer the influence of culinary preferences (Liu et al., 2016; Ritchey et al., 2021).
The Chap II site is situated at the highest elevation ecocline for successful cereal cultivation at 2000 m.a.s.l in the Tien Shan Mountains of Kyrgyzstan (Figure 1). The agriculture in the Kochkor valley is currently concentrated on the valley bottom, as surrounding slopes promptly increase in elevation. In the summer, the south-facing slopes can receive snowstorms at 2,600 m.a.s.l., drawing the line for successful cereal cultivation. Currently, the main cultivated species in the vicinity of Chap archaeological site are spring and winter barley (mostly naked varieties), bread wheat, lucerne grass, and potatoes. The key limiting factor of agriculture in high-altitude valleys—such as Kochkor—are unstable climates that can generate strong winds, rain storms, water shortages, and short growing seasons due to the continental climate. Therefore, current agricultural practices around the Chap II site are highly susceptible to minor climatic fluctuations and therefore can only be successful by targeting crop species that are adapted to local environments, along with facilitating their growth via manuring and irrigation.
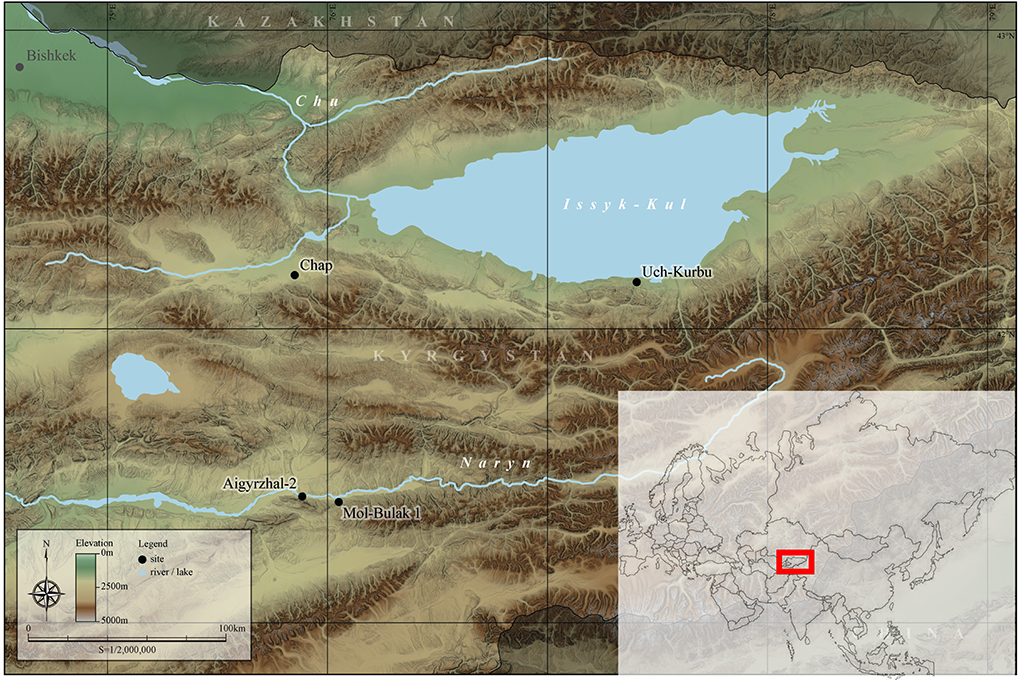
Figure 1. Map of Kyrgyzstan showing Chap and other sites mentioned in the text. The red rectangle marks the position of the Kyrgyzstan on the Eurasian map. SRTM (NASA) for DEM, Digital Chart of the World (DCW) for water surface, Global Administrative Areas (GADM) for boundaries.
The occupation horizon of the Chap II site was formed by the first farming communities that settled the highland valleys of Central Tien Shan during the second half of the 3rd millennium BCE (Motuzaite Matuzeviciute et al., 2021a). Previous research at the Chap II site has shown that southwest Asian cereal crops facilitated high-elevation agriculture in the Central Tien Shan; the crop species consisted mainly of free-threshing wheat species and naked barley varieties but also possibly some glume wheat and hulled barleys (Motuzaite Matuzeviciute et al., 2020a). The reported values of bread wheat caryopses (n-311) ranged between 6 and 2.4 mm (mean values 3.9 mm) in length, 4.2 and 1.8 mm in width (mean values 2.9 mm), and 3.4 and 1.3 mm in depth (mean values 2.2 mm), while naked barley (n-256) caryopses ranged between 6.4 and 2.7 mm in length (mean values 4.3 mm), 4.3 and 1.8 mm in width (mean values 3 mm), and 3.5 and 1.2 mm in depth (mean values 2.3 mm) (Motuzaite Matuzeviciute et al., 2021a). Such large variation in caryopses size is usually associated with environmental conditions during crop development that is common for many plant species (Salisbury, 1974; Goodman and Brown, 1988; Moles et al., 2005). Plant exposure to heat, for example, can decrease seed size during development (Reed et al., 2022). Previous studies have suggested that the variability in cereal caryopses can arise from unstable temperatures and/or strong winds and rains, which can enable the survival of certain landraces while eliminating others over time (Motuzaite Matuzeviciute et al., 2018).
The previously published archaeobotanical assemblage from the Chap II site constitutes the largest in the Central Tien Shan to date (Motuzaite Matuzeviciute et al., 2021a). Yet, all previously analyzed archaeobotanical remains at this site were recovered just from two adjacent ash pits. These pits were recovered during the last days of the 2019 archeological excavation after opening a 2 x 2 m test pit, raising many questions regarding the representativity of the analyzed contexts and the function of the pits themselves. It was not clear whether the pits constituted a part of a wider domestic context or were ritual deposits, as has been previously suggested at contemporaneous sites in the Semirechye region of Kazakhstan (Frachetti, 2014). During the Chap II archeological excavations in the year 2020–2021, an extensive horizon of Chap II occupation was recovered. Additional archaeobotanical samples were taken from fireplaces, clay ovens, domestic pits, burial pits (dug into the oven), and house floors, suggesting domestic occupation horizons.
This paper presents extensive and detailed archaeobotanical research results that, together with new radiocarbon dates, have facilitated the development of a much broader picture of agricultural practices, the paleoenvironment, and plant adaptation during the earliest episodes of crop cultivation in the Tien Central Shan Mountains.
Methods
Archaeobotany
The archaeobotanical samples were collected from the domestic occupation horizon of the Chap II site, targeting fireplaces, a clay oven, domestic waste pits, and house floors, all of which were distributed at a depth between 170 and 300 cm below the present-day surface (Figure 2). A total of 550 l of sediments were floated from 12 archeological contexts collected during the 2020–2021 excavations, while 136 l were collected during the 2019 excavation (previously published in Motuzaite Matuzeviciute et al. (2020a) and added to Table 1). For sediment flotation, we used a 0.3 mm sieve size and water from the irrigation channel at the Kara Suu village. We also collected additional sediment samples from every context (40 l bag), which are currently stored as archival material at the excavation site. After sediments were floated using the bucket flotation method, the dried flotation samples were transported to the Bioarchaeology Research Center of Vilnius University, where they are currently archived. During the sorting, each sample was collected in a stack of three sieves of the following mesh sizes to facilitate the analysis of the material: 2 mm, 0,6 mm, and 0,14 mm. The finest fraction was collected in a separate container at the bottom of the sieves. This fraction was not analyzed, as it was mainly composed of very fine silt size sediment and/or charcoal “dust.” The stereomicroscope and reference collection of the Bioarchaeology Research Center, including botanical seed atlases, such as Cappers et al. (2006) and Martin and Barkley, 1961; were used to identify and quantify both wild and domesticated plant taxa. In addition, for the identification of the cereal crop caryopses, rachis internodes, and glume bases were relied on identification criteria as presented by Jacomet and Schlichtherle (1984), Zohary and Hopf (2000), Hillman (2001), Hillman et al. (1996), Renfrew (1973), and Jacomet (2006), Neef et al. (2012). In addition, for the identification of wheat rachis internodes to species, we consulted with archaeobotanist Nana Rusishvili from the Georgian National Museum and the botanist and wheat specialist Marine Mosulishvili from Ilia State University, Georgia. A more detailed description of the crop identification and quantification criteria applied is found in the results section.
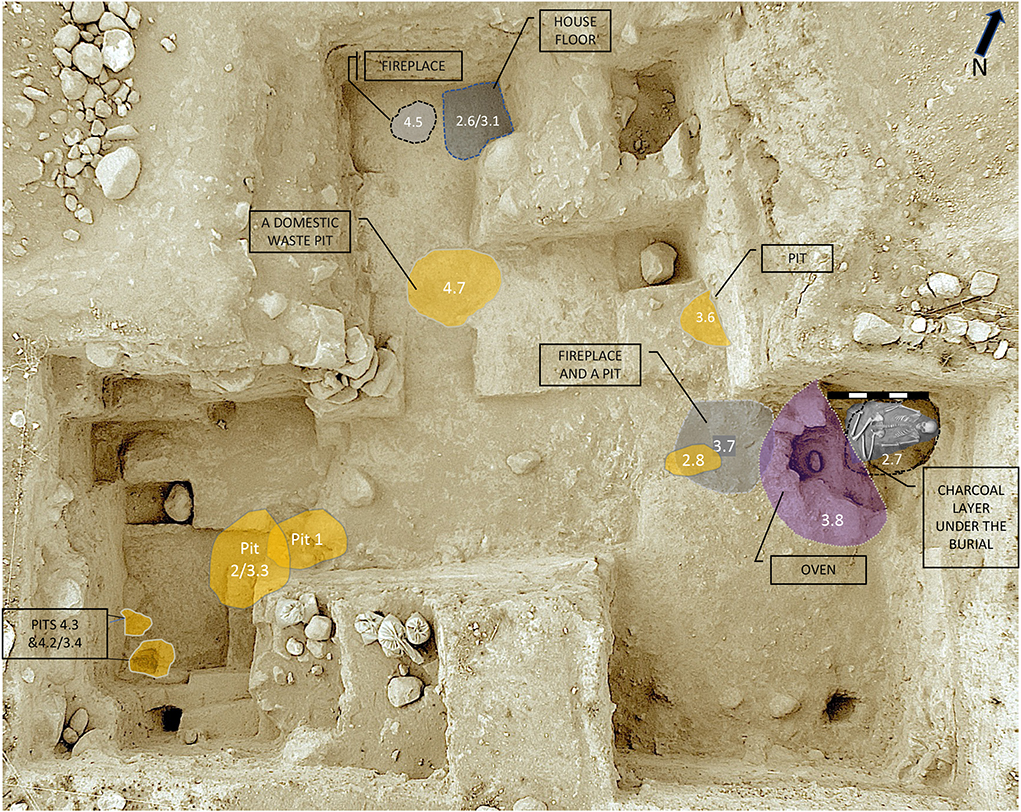
Figure 2. The aerial photography of Chap II excavation area with marked contexts of archaeobotanical samples as listed in Table 1 (photograph by Kunbolot Akmatov).
Radiocarbon dating
Radiocarbon dating of carbonized grains, animal bones, and human bones was performed by the accelerator mass spectrometer (AMS) method. The dating was performed at the Center for Physical Science and Technology in Vilnius. Radiocarbon results were calibrated using the IntCal20 calibration curve (Bronk Ramsey, 2009; Reimer et al., 2020) (Table 2). Bayesian modeling of the Chap II site archeological contexts was modeled in OxCal v. 4.4.4 according to their stratigraphic positions (n-14) together with the Chap I site (n-10) (Bronk Ramsey, 2021). The previously published dates from the Chap I site, which is situated above the occupation horizon of Chap II, were used for comparison and visualization of the Chap occupations phases as these two phases together are published for the first time (SOM1). Date ranges are reported as 95% posterior probabilities on individual dates and the start and end dates of site occupations.
Results
Archaeobotany
All archaeobotanically analyzed contexts contained large quantities of cultivated cereal grains, grain fragments, and cereal chaff (Table 1). The barley grains and chaff were of two varieties—naked (Hordeum vulgare var. nudum) and hulled (Hordeum vulgare var. vulgare). The naked forms constituted the absolute majority of all barleys and belonged to compact morphotypes that are highly variable in size (Figures 3A–D,G, 4). We also identified fragments of rachis internodes belonging to naked barley varieties, as characterized by the “spiky” attachment platform on the central floret (Motuzaite Matuzeviciute et al., 2021b) (Figures 5A–C). We also found some hulled varieties of barley as seen from the barley caryopses that still had glumes attached (Figure 3E) and from rachis internodes that contained glumes on the side of the glume base platform but none in the middle.
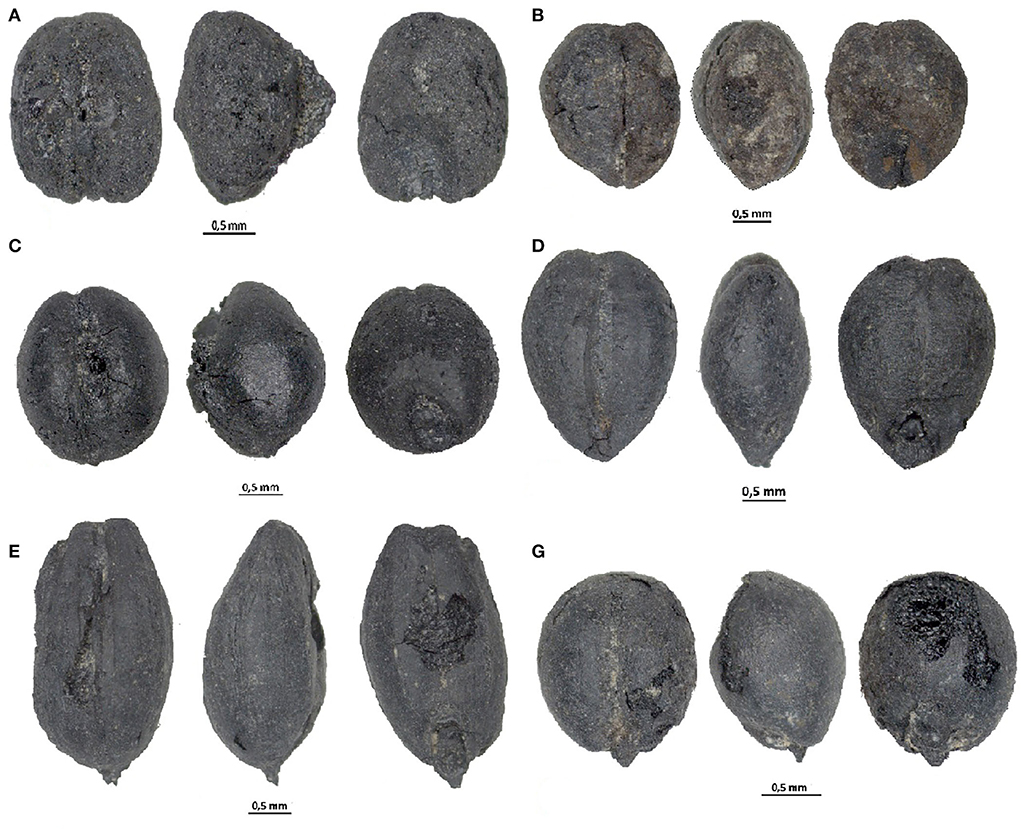
Figure 3. Barley varieties from Chap II site: compact naked barley morphotypes (Hordeum vulgare var. nudum) (A–D,G) and hulled barley (Hordeum vulgare var. vulgare) (E).
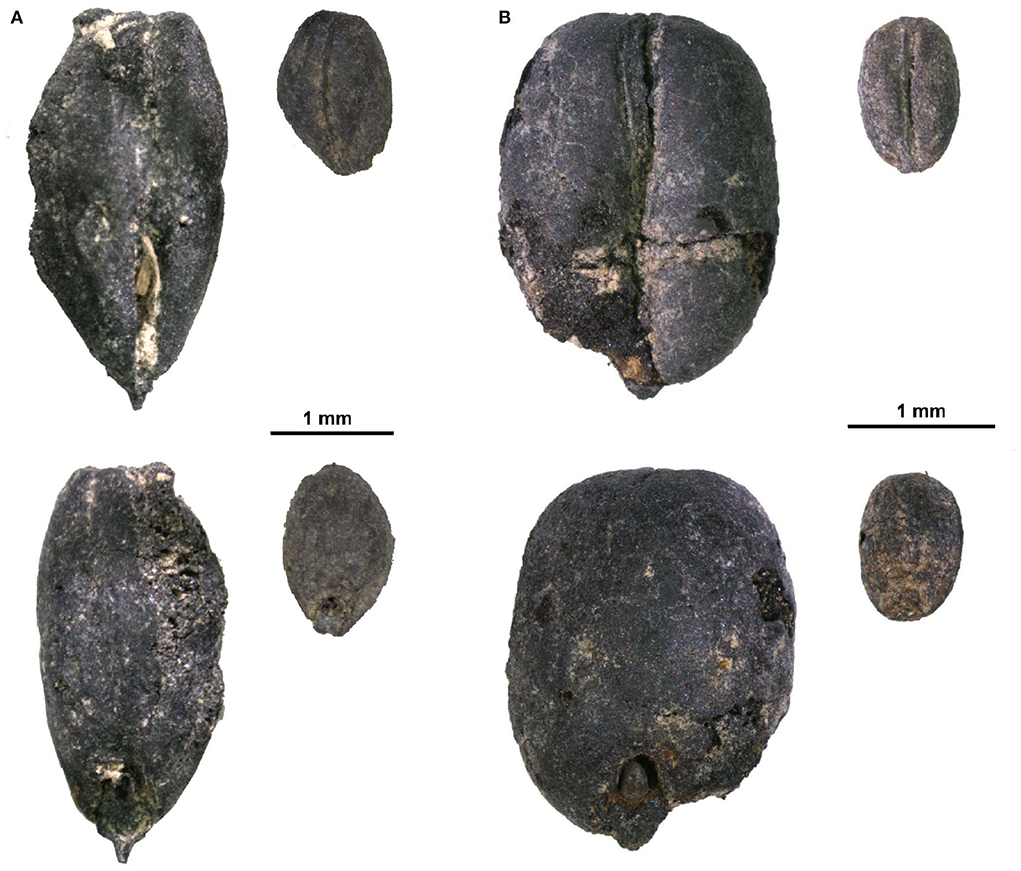
Figure 4. Variation in size between caryopses of the same species of barley (A) and wheat (B) at Chap II site.
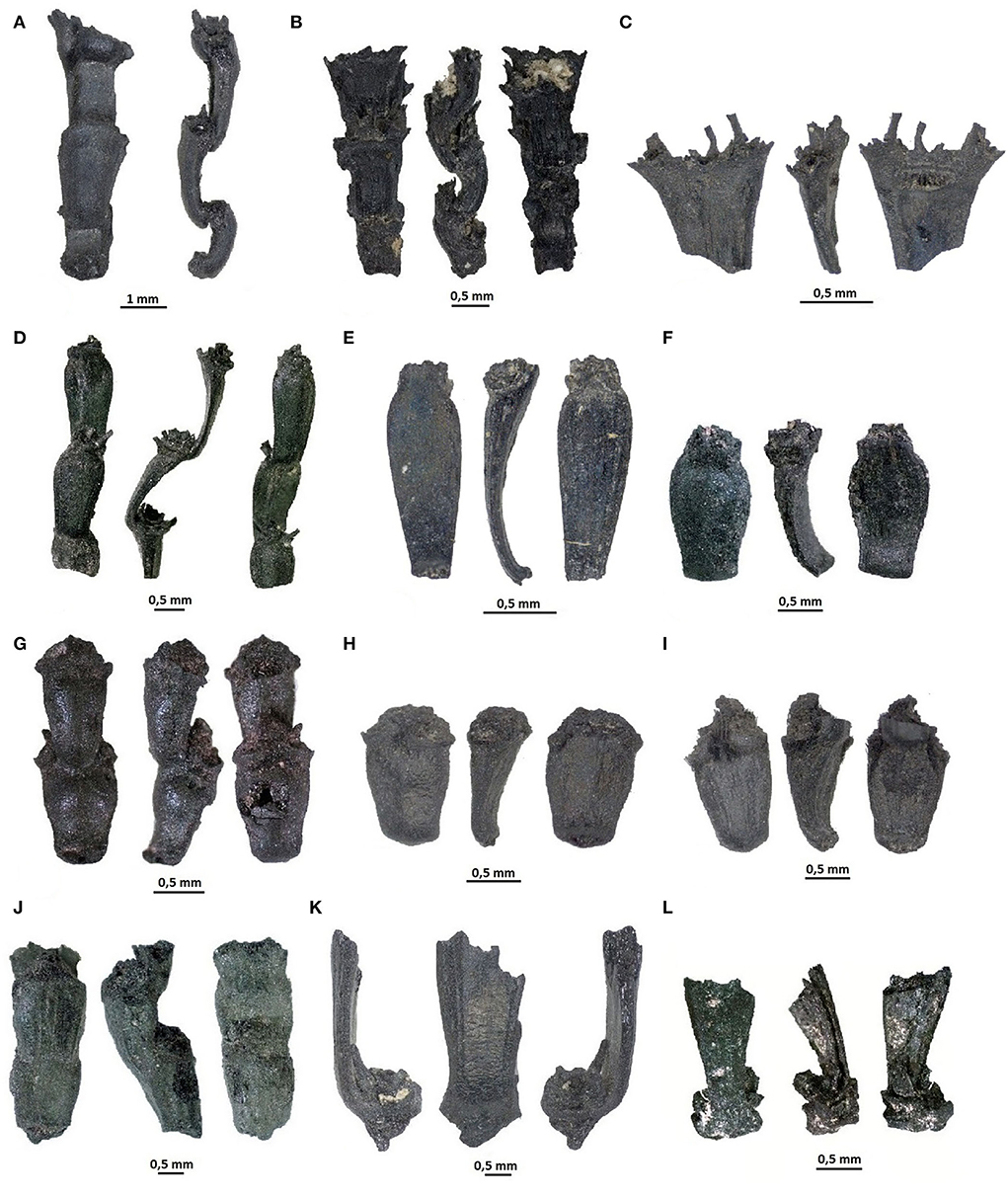
Figure 5. Rachis internodes from Chap II site of Hordeum vulgare var. nudum (A–C), Triticum aestivum (D, F), Titicum carthlicum (E), tetraploid free-threshing wheats cf. Triticum durum/turgidum (G–J), and rachis internodes of possibly glume wheats (K,L).
The free-threshing wheat species constituted the second major component of all cultivated cereal remains in all analyzed assemblages, belonging to the free-threshing species of Triticum aestivum/durum/turgidum (Figures 6A,B). The majority of rachis internodes belonged to bread wheat and contained a characteristic “shield shape” or curved rachis (Figures 5D,F). The widest part of such rachises is just above the middle portion, without a swelling below the glumes (Hillman et al., 1996; Hillman, 2001; Jacomet, 2006). However, some rachis internodes also belonged to other free-threshing wheat species, which did not fit into the shield shape rachis internode category. The former group of rachis internodes had straight side contours where the maximum width of the rachis was located in the upper portion; these internodes also contained a distinct swelling below the glume insertion (Figures 5G–J). Such rachises were attributed to Triticum durum/turgidum species (Table 1). Having internodes that belonged to more than one free-threshing wheat species, it was impossible to attribute free-threshing wheat caryopses to particular wheat species. Therefore, all caryopses belonging to free-threshing wheats were clustered as Triticum aestivum/durum/turgidum according to the spherical caryopses. Similarly to barley, the free-threshing wheat grains possessed highly compact caryopses morphotypes with a strikingly large variation in grain size (Figure 4).
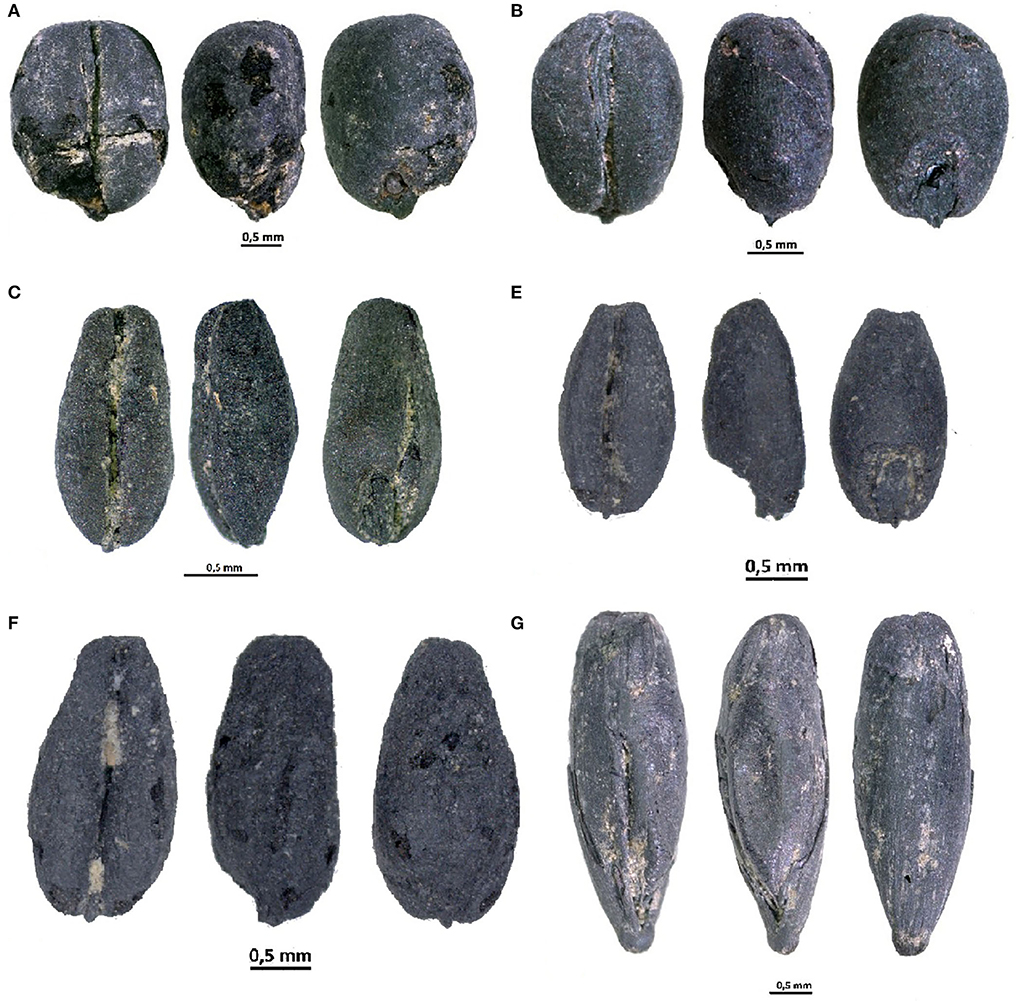
Figure 6. Caryopses of Triticum aestivum (A,B), Triticum sp. (cf. glume/ tetraploid wheat or T. carthlicum) (C,E,F), and Avena sp. (G).
In addition, a rachis internode belonging to Triticum carthlicum, which contains very characteristic elongated rachis internodes with visible veneration, was identified for the first time (Figure 5E). The caryopses of this free-threshing species of wheat were more elongated than Triticum durum/turgidum caryopses and contained a deeper ventral furrow and higher dorsal keel, similar to emmer glume wheat. Other wheat species, likely glume wheats, were probably also present in the Chap II assemblage, as seen from the recovered glume bases with visible striations (Figures 5K,L) and more elongated caryopses with a pronounced keel (Figures 6C,E,F). Therefore, such wheat caryopses were clustered together with Triticum carthlicum, which is known to be present in the assemblage. Among other cultivated crops, the remains of oat (Avena sp.) (Figure 6G) and one poppy (Papaver sp.) were identified. Yet, those solitary finds do not allow us to make any further interpretation or identification of species.
Thousands of fragmented cereals, counted as “Cerealia,” were recovered from food preparation places or waste pits. They were found together with carbonized food fragments characterized by porous or amorphous-like matrices and burned, lumpy conglomerates (Figure 7). These charred food pieces probably represent the remains of bread or porridge. Yet, future analysis using SEM microscopy needs to be done to allow further identification of these conglomerates.
The wild plants belonged to 14 plant families and over 56 species (Table 1) as more than one species within a genus of Galium or Carex were noted. The prevailing wild plant remains belonged to Amaranthaceae family, dominated by Chenopodium sp. (goosefoot). The second and third most abundant plant genus is the Cyperaceae family, represented by at least five Carex sp. (sedges), and the Rubiaceae family represented by a few species of Galium sp. (Table 1, Figure 8). The Caryophyllaceae family is represented by Silene noctiflora and Vaccaria sp.; the Fabaceae family is represented by the Melilotus, Medicago, Trifolium species. It was not always possible to separate Melilotus/Medicago/Trifolium sp. apart as charring frequently distorted the seed shape and size. Among the Poaceae family, cf. Eragrostis sp. (both caryopses and florescence) was the most frequently found in most of the analyzed contexts.
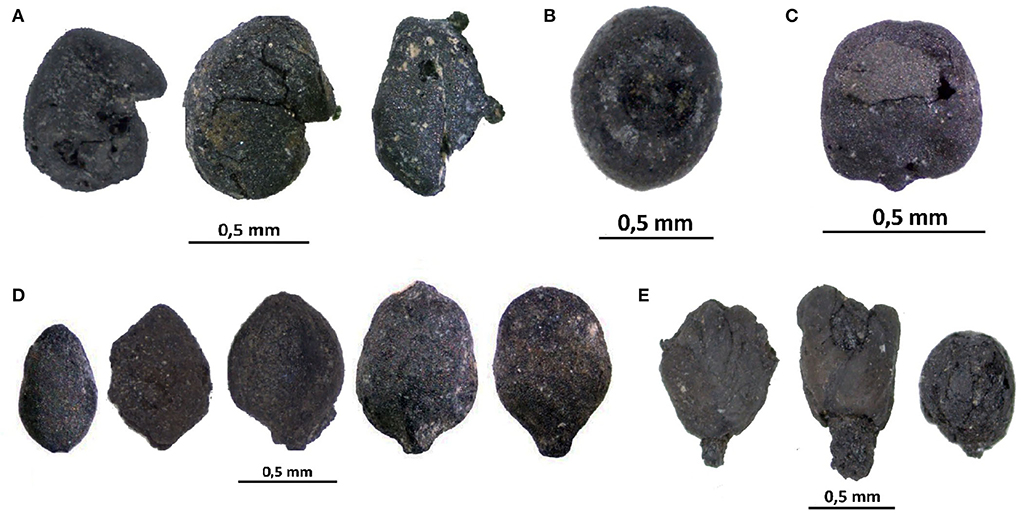
Figure 8. Most dominant wild plant taxa from Chap II site: Medicago/Melilotus spp. (A), Galium sp. (B), Eragrostis sp. (C), Carex spp. (D), and Eragrostis sp. inflorescence (E).
Taphonomy
The analyzed assemblage represents food cooking and crop processing and domestic waste discharge locations that contain preserved carbonized plant remains. The taphonomic conditions were very good for preserving charred plant remains at Chap II, as not only cereal grains and chaff were present, but also fragile fluorescence and thorns were abundantly found in the archaeobotanical assemblages. Future experimental work should be conducted to identify whether the plant remains preserve better in high-altitude environments due to reduced oxygen conditions during charring than in lowland sites.
The wood charcoals were not quantified at the Chap II site, but they were present in all analyzed contexts. The largest fragments of wood charcoal (up to 1 cm in size) were recovered in fireplaces and oven as well as in the pit 4.3. An anoxic condition in the enclosed ovens undoubtedly had an influence on wood preservation. No pellets of carbonized sheep dung, as previously abundantly recovered at Chap I site (Motuzaite Matuzeviciute et al., 2020b), were noted at Chap II site, and it is likely that wood rather than dung was the main fuel source here.
Unusually, high quantities (over 2,000 fragments) of cereal fragments were identified in pit 2 (that is the same context as 3.7) (Figure 2). This could indicate a waste dump from the fireplace where some sort of crop processing activities took place. More detailed analysis is needed to identify whether the fragmentation of cereal is a part of taphonomic process of fragmentation during entire grain charring or a part of food preparation activity, such as course porridge or bulgur wheat-type food.
Small seeded plants were identified together with larger caryopses of domesticated grasses in all contexts. That would infer that the domestic waste where crop processing and cooking activities took place was discarded into fires and later into waste pits. The presence of wild plant seeds in all the contexts could also indicate that along with wood, dried animal dung could have also been used as a fuel (although no pellets were identified).
Radiocarbon dating
Radiocarbon dates modeled with Bayesian statistics from the Chap II site all fell into a very tight occupation phase dated between the 2474–2302 cal BCE period and finished between 2336–2162 cal BCE. Despite differences in stratigraphy, where fireplace 3.7/2.8 was located higher than the oven/hearth 3.8, and where the human grave buried inside of the abandoned oven was dug at a later date, it looks like all activities at the site took place between 2474–2162 cal BCE (Table 2, Figure 9). The radiocarbon dates from the Chap I sites were also included in the Bayesian statistics model, clearly showing an occupation horizon from over a thousand years later, which started between 1102–926 cal BCE and ended 916–816 cal BCE (SOM 1).
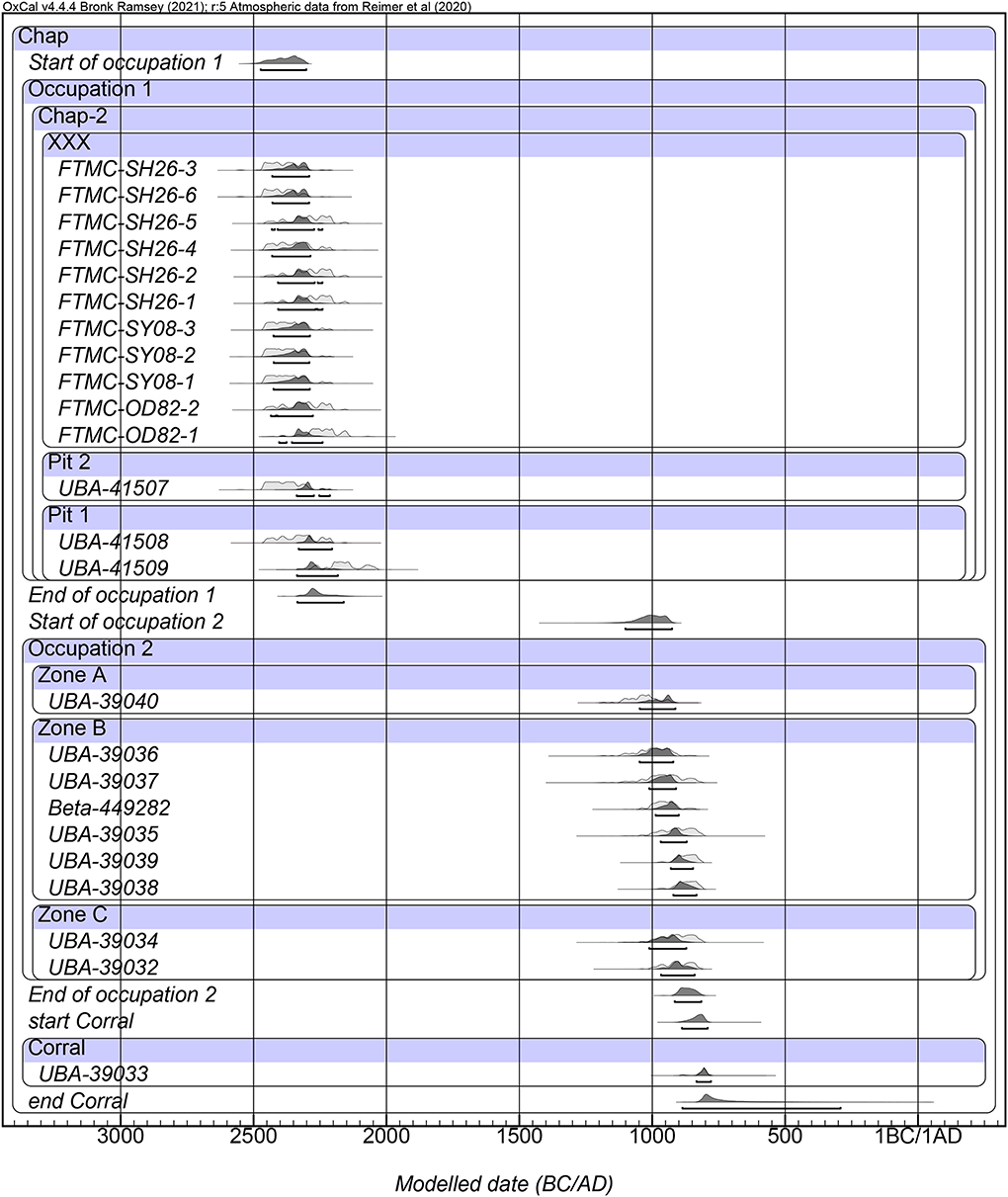
Figure 9. Radiocarbon chronology for Chap II. Modeled radiocarbon dates measured on plant seeds, human, and faunal bone from Chap II in relation to the later occupational period of Chap I. Three AMS dates from Chap II and all dates from the Chap I site were previously published (Motuzaite Matuzeviciute et al., 2020a,b).
Discussion
Chap II represents the earliest settlement site on the southeastern fringes of Central Asia to have revealed the established agricultural practices in the region. The Chap II site shows a broad assemblage of cultigens consisting of at least three species of free-threshing wheats and possibly glume wheats, naked, and some hulled barleys. Although the free-threshing wheats were not differentiated into species, it is most likely that the majority of free-threshing grains belong to bread wheat (Triticum aestivum), while the majority of barley belong to naked varieties. The solitary finds of oat and poppy identified only to genus level likely represent wild species. The cereal chaff remains, belonging to hulled and naked barleys, free-threshing and possibly glume wheats, show local cereal cultivation and processing.
The Chap II assemblage represents the initial array of cultigens that left southwest Asia and dispersed to the east following the Inner Asian Mountain Corridor. As this southwest crop package continued to journey further east and north across subsequent mountain ranges, only hexaploid free-threshing wheat (Triticum aestivum) and naked barley (Hordeum vulgare var. nudum) have been found across the other side of the mountain range, at 3rd millennium BCE sites in present-day China (Flad et al., 2010; Liu et al., 2016; Stevens et al., 2016; Zhou et al., 2020). From this period, hulled barley or glume wheats have also been found in the foothill sites of Tajikistan and Kashmir (Spengler and Willcox, 2013; Betts et al., 2019; Yatoo et al., 2020). Yet, in most archaeobotanical assemblages from foothill or mountain valley sites of Central Asia, naked barley and bread wheat dominate the recovered assemblages (Spengler et al., 2014; Motuzaite Matuzeviciute et al., 2020a). Although future and more extensive studies, especially in western China, might change this current pattern, it appears that during the 3rd millennium BCE, the crop package on the western side of the mountain chain contained a much wider array of southwestern crop packages than that identified on the eastern side. This could possibly suggest that the mountain highlands acted as a filter for a selective set of species. Only those landraces of naked barley and bread wheat were selected that provided an adaptive advantage to mountain environments as they dispersed into novel ecogeographies. The selection of compact caryopses morphotypes for free-threshing wheats and naked barley is clear at the Chap II site. Yet, Chap II site is not unique in this respect, as the compact morphotypes of bread wheat and naked barley were also identified at other high-altitude sites in the Tien Shan, Dzhungar Alatau, and Altai Mountains dated between the 3rd and 2nd millennium BCE (Figure 1) (Motuzaite Matuzeviciute et al., 2021a) as well as in other ecogeographies of western Central Asia and India that are dated earlier than the assemblages in the mountainous regions of eastern Central Asia (Weber, 1991; Moore et al., 1994; Miller, 1999, 2003). Therefore, it should be emphasized that such compact morphotypes of wheat and barley did not evolve in the mountains but were selected as preferred morphotypes during the pioneer crop dispersal from the west further to the east. Very little is known about where those compact wheats originated, what their growing requirements were, and whether those varieties are related to modern landraces of wheat that are cultivated in Central Asia (Spengler, 2019).
The observations of barley landraces across the world collected by botanist A. Vavilov show unique adaptations of barley to specific ecogeographies, and the barleys in Central Asia, in particular, are characterized by being diverse in their morphological and adaptive characteristics (Knüpffer et al., 2003). According to modern analogies, the barley or wheat that possess compact and spherical grains usually, but not always, have short compact plant morphology. For example, the East Asiatic barleys are generally characterized by short straw (dwarfness) containing short spikes, and small, almost spherical caryopses, while the dwarf wheats (Triticum aestivum L. ssp. sphaerococcum) also have compact grain morphotypes (see, e.g., Knüpffer et al., 2003; Mori et al., 2013; Skrajda-Brdak et al., 2020). Landraces of barleys from Syria and Palestine are short and characterized by compact, very rigid spikes, and a strong waxy cover on culm and leaves, while barleys in Tibet at 4,500 m.a.s.l. contain dense spikes and small-to-medium-sized caryopses, tall plants that are extraordinarily prostrate, with thick straw, and long, very broad leaves, moderately resistant to lodging (Knüpffer et al., 2003). Compared to non-compact forms, compact varieties of cereals exhibit greater standing power against extreme weather conditions, such as strong winds and intense rains that can cause lodging and stem breakage (Motuzaite Matuzeviciute et al., 2018, 2021a; Spengler, 2019).
Again, we speculate that the selection of adaptive to mountainous or any marginal environments properties, such as reduced tillering, lodging, thick straw, drought-resistant waxy forms, or breaking of photoperiod sensitivity, could have resulted in the caryopses compact morphotypes as we see in Chap II and other archeological sites. The evolution of more compact grain morphotypes in wheat and barley could have resulted from pleiotropic effects in selecting plants that were advantageous for crop adaptation in marginal environments.
As was already mentioned, at the Chap II site, the resilience of naked barley and bread wheat in mountainous environments can be seen not only from the compact grain morphotypes but also from the large variation in cereal grain sizes (Figure 4). It should be mentioned that the Chap II site for this matter is not an exception. Large variability in caryopses sizes was detected in all archaeobotanical samples from the mountain regions of the Central Tien Shan (Motuzaite Matuzeviciute et al., 2018). The high variation in compact caryopses size in both bread wheat and naked barley varieties at the Chap II and other sites of the Central Tien Shan can be linked with thermal and water stress at the stage of grain filling during the summer (Magliano et al., 2014) or varying soil fertility in cultivated fields (van Bommel et al., 2021). Previous experimental research has shown that plants experiencing physiological stress from differences in water availability, ambient temperature, or amount of nutrients during grain filling resulted in grain size diminution, due to interference in the deposition of carbohydrates in the grains (Nicolas et al., 1984; Blum, 1996a,b, 1998). Furthermore, the growth rate per grain depends also on the floret position within the ear (Sofield et al., 1977). Therefore, variation of grain size, as seen in the archaeobotanical assemblage, could also be from large grain differences within the same ear (Reed et al., 2019).
Along with adaptability to the local environment, naked barley and bread wheat were preferred by pioneer farmers probably also due to the simplicity of their threshing and culinary properties as human food. Knüpffer et al. (2003) note from landrace examples in diverse ecogeographies that in the high-altitude regions, the naked barleys were selected by mountain populations due to their greater cold-hardiness. The “naked” or “hulless” barley caryopses could also be an important trait for cultivation in high altitudes due to its ability to produce purple or black pigmented caryopses caused by anthocyanins (Zhang et al., 2021). These compounds are antioxidants and have protective properties against damage caused by high levels of UV radiation at high altitude. In turn, the intensive UV light eliminated various fungal diseases that naked barley caryopses are prone to in lower altitudes. Anthocyanin-rich purple highland barley also provides multiple health benefits to humans (Zhang et al., 2021). It was suggested that naked barley in the highlands produces higher grain yields, generates more overall biomass, and matures faster than hulled varieties (Ghimire et al., 2019).
The reasons why other species such as hulled barley or glume wheats were filtered out along the way during the initial stage of crop dispersal are probably due to their poorer adaptability to mountainous ecosystems during their early stages of dispersal, and their inability to adapt to seasonal climatic changes. Genetic changes in plants through alteration in flowering and length of the growing season can have a dramatic effect on a crop's ability to grow successfully in novel environments (Fuller and Lucas, 2017). The seasonal pattern of free-threshing wheat and naked barley planting at the Chap II site was probably unsuitable for other wheat species and hulled barley varieties; therefore, they were found in such small quantities. It is likely that the dominant species of the pioneer crops were planted just during the autumn, as winter crops, or just in spring as summer crops. The seasonality pattern of crop planting became much more variable during the 1st millennium BCE with both winter and summer cereals present (Lister et al., 2018; Motuzaite Matuzeviciute et al., 2021b). During the 1st millennium BCE, both hulled and naked barleys are frequently identified in archaeobotanical assemblage of the Chap I site (Motuzaite Matuzeviciute et al., 2021b). Previous research using a niche model to examine the impact of changing temperatures across a wider geographical scope on the human ability to cultivate different crop species has shown a strong correlation between temperature variation and crop transmission across time and space (d'Alpoim Guedes and Bocinsky, 2018).
It is interesting that out of 686 l of sediment samples from multiple archeological contexts floated and analyzed at the Chap II site, no evidence of broomcorn or foxtail millets was found. On the cont, broomcorn millet was recovered at the contemporaneous site of Begash located at 900 m.a.s.l. in the neighboring region of Semirechye (southeastern Kazakhstan) (Frachetti et al., 2010). Both broomcorn and foxtail millets and wild Panicoid grasses appear in Kyrgyzstan at the sites of Uch-Kurbu (in a layer dated to 1366–1124 BCE) and Chap I (dated to c. 1065–825 BCE) (Motuzaite Matuzeviciute et al., 2018, 2020b). Stable isotope analysis of both human and animal bone collagen showed that during the 3rd millennium BCE in Kyrgyzstan, only solitary individuals were eating C4 plants, while between 1300 and 1000 BCE there was a clear increase in C4 plant consumption among both humans and animals as millet became a frequent dietary source for the ancient population of Kyrgyzstan (Motuzaite Matuzeviciute et al., 2022). Here, we can support a previous argument (Motuzaite Matuzeviciute et al., 2020a) that the definitive absence of millet at Chap II at 2000 m.a.s.l. altitude during the second half of the 3rd millennium BCE represents an elevational ceiling for millet cultivation, likely influenced by climatic conditions during this particular episode of farming establishment.
Although it is outside of the scope of this paper to discuss in detail ecological niches and adaptation of wild plant taxa at the Chap II site, some of which probably traveled together with the agricultural package, instead we only focus on dominant plant taxa that infer human action in facilitating crop adaptation in alpine ecosystems. During the occupation of the Chap II site, wild plant taxa reveal a set of well-watered meadow vegetation dominated by sedges (Carex sp.) and various legumes (Melilotus/Medicago/Trifolium sp.). Goosefoot (Chenopodium sp.) was also frequent together with bedstraw (Galium sp. genus). The majority of Galium and Chenopodium genus plants of Central Asia inhabit nitrogen-rich, often moist, meadows modified by intensive agricultural and pastoralist activities (Fisyunov, 1984; Spengler, 2018). Together with a favorable and more humid climate, it is plausible that irrigation technologies were also present in the Chap II surroundings to facilitate successful crop cultivation in the highlands of the Tien Shan. Similar vegetation consisting of alfalfa (Medicago sativa) also called lucerne of the legume family, together with foxtail and barnyards grasses, currently grows in well-irrigated meadows in the Kochkor valley, while sedges nowadays are frequently found along water irrigation channels. Research by Rouse et al. (2021) has shown the past existence of complex ancient irrigation channels in the Kochkor valley. Although their dating is not clear, the existence of ancient irrigation systems demonstrates the importance of water management systems in the past for maintaining agriculture in the valley.
Paleoclimatic data from this part of the Tien Shan mountains show an increase in moisture at the onset of agricultural dispersal around 4000 BCE (Chen et al., 2016). Within the humid episodes that likely facilitated agriculture dispersal in the mountain highlands, several rapid cold episodes were detected at around ~2200 BCE and ~700 BCE (Leroy and Giralt, 2021; Spate et al., 2022). The identified cooling episodes roughly overlap with the abandonment of the Chap II and Chap I sites (Figure 9). Yet, further research needs to be done to understand the reasons for the site abandonment and the influence of the changing climate on these events. The populations in the high-altitude mountains are particularly sensitive to cold spells and long-term climatic changes as they inhabited marginal territories situated at the edge of the agricultural limit.
Conclusion
The archaeobotanical assemblage of Chap II represents the earliest known occupation by an agropastoral community in the Central Tien Shan at 2,000 m.a.s.l. dated between 2474 and 2162 cal BCE (AMS n-14). It also represents the largest assemblage of cultivated plant species, their chaff, and wild plant taxa in the region showing local crop production and processing. The archaeobotanical samples were obtained from 12 contexts totaling 680 l of floated sediment. Therefore, the Chap II site represents a role model site for understanding agricultural resilience and adaptation by the first farmers in the Central Tien Shan mountains.
The Chap II site constitutes the eastern pocket of the southwest agricultural package within the mountains of Central Asia dominated by compact bread wheat species and naked barley, while other free-threshing wheats belonging to T. turgidum/durum and T. carthlicum along with hulled barley, probably glume wheat were also present. Therefore, the Chap II site represents a tipping point where the wider array of SW Asian cultigens gets reduced just to two species as agriculture advanced further into the territories of present-day China along the IAMC during the 3rd millennium BCE.
While it is beyond archaeobotanical research methods, it could be only speculated what adaptive qualities of bread wheat and naked barley provided these species with the necessary resilience to survive in high-altitude mountainous climates and withstand strong winds, rainstorms, and other climatic stressors. The environmental stress and climatic variability that early crops were affected by can be observed from the large variability in caryopses size among plants. Other genetic and phenotypic changes, invisible in archaeobotanical datasets, such as adaptation to growing season and resistance to diseases, thick straw, and sturdy plant morphotype could have given an advantage to naked barley and bread wheat crops in high altitudes.
In addition, the pioneer farming systems in the mountain zones of Central Asia were likely facilitated by additional watering and possibly manuring of crops. Yet, further research measuring carbon (δ13C) and nitrogen (δ15N) isotopic compositions of cereal grain and changes in stable isotope values over time could help to validate this claim.
Data availability statement
The original contributions presented in the study are included in the article/supplementary material, further inquiries can be directed to the corresponding author.
Author contributions
GMM is a PI of the project, she has conducted the archaeobotanical research, analyzed archaeobotanical data, and has written the paper. MU conducted archaeobotanical sample sorting and identification. RK conducted archaeobotanical sample sorting, and compiled figures. KT has contributed to research design. All authors contributed to the article and approved the submitted version.
Funding
This project has received funding from the European Social Fund project No. 09.3.3-LMT-K-712-01-0002 under a grant agreement with the Research Council of Lithuania (LMTLT).
Acknowledgments
We would like to express our gratitude to Taylor Hermes for helping to generate the Bayesian statistics model that is used in this manuscript. We would also like to thank the editor AM and both reviewers AL and NM for their accurate and constructive comments.
Conflict of interest
The authors declare that the research was conducted in the absence of any commercial or financial relationships that could be construed as a potential conflict of interest.
Publisher's note
All claims expressed in this article are solely those of the authors and do not necessarily represent those of their affiliated organizations, or those of the publisher, the editors and the reviewers. Any product that may be evaluated in this article, or claim that may be made by its manufacturer, is not guaranteed or endorsed by the publisher.
References
Betts, A., Yatoo, M., Spate, M., Frazer, J., Kaloo, Z., Younus, R., et al. (2019). The northern neolithic of the Western Himalayas: new research in the Kashmir valley. Archaeol. Res. Asia. 18, 17–39.
Blum, A. (1996a). “Constitutive traits affecting plant performance under stress,” Edmeades, G. O., Banziger, M., Mickelson, H. R., and Pena-Valdivia, C. B. (Mexico: International Maize and Wheat Improvement Center) p. 131–135.
Blum, A. (1996b). Crop responses to drought and the interpretation of adaptation. Plant Growth Regul. 20, 135–148.
Blum, A. (1998). Improving wheat grain filling under stress by stem reserve mobilisation. Euphytica. 100, 77–83.
Bronk Ramsey, C. (2021). OxCal version 4.4.2. Available online at: https://c14.arch.ox.ac.uk (accessed 18 April 2021).
Cappers, R. T. J., Bekker, R. M., and Jans, J. E. A. (2006). Digitale zadenatlas van Nederland (Digital Seed Atlas of the Netherlans). Groningen: Barkhuis Publishing and Gronigen University Library.
Chen, F., Jia, J., Chen, J., Li, G., Zhang, X., Xie, H., et al. (2016). A persistent Holocene wetting trend in arid central Asia, with wettest conditions in the late Holocene, revealed by multi-proxy analyses of loess-paleosol sequences in Xinjiang, China. Quat. Sci. Rev. 146, 134–146. doi: 10.1016/j.quascirev.2016.06.002
Coward, F., Shennan, S., Colledge, S., Conolly, J., and Collard, M. (2008). The spread of Neolithic plants economies from the Near East to northwest Europe: a phylogenetic analysis. J. Archaeol. Sci. 35, 42–56.
d'Alpoim Guedes, J., and Bocinsky, R. K. (2018). Climate change stimulated agricultural innovation and exchange across Asia. Sci. Adv. 4, eaar4491. doi: 10.1126/sciadv.aar4491
Flad, R., Shuicheng, L., Xiaohong, W., and Zhijun, Z. (2010). Early wheat in China: results from new studies at Donghuishan in the Hexi Corridor. The Holocene. 20, 955–965.
Frachetti, M. (2014). “Seeds for the soul: ideology and diffusion of domesticated grains across Inner Asia.,” in Reconfiguring the Silk Road: New Research on East–West Exchange in Antiquity (Philadelphia, PA: University of Pennsylvania Museum of Archaeology and Anthropology) p. 41–54.
Frachetti, M. D., Spengler, R. N., Fritz, G. J., and Mar'yashev, A. N. (2010). Earliest direct evidence for broomcorn millet and wheat in the central Eurasian steppe region. Antiquity. 84, 993–1010.
Fuller, D. Q., Colledge, S., Murphy, C., and Stevens, C. J. (2017). Sizing up cereal variation: patterns in grain evolution revealed in chronological and geographical comparisons. Universidad del País Vasco. p. 131–150.
Fuller, D. Q., and Lucas, L. (2017). Adapting crops, landscapes, and food choices: patterns in the dispersal of domesticated plants across Eurasia. Cambridge: Cambridge University Press.
Ghimire, K. H., Joshi, B. K., Gurung, R., Palikhey, E., Pudasaini, N., and Parajuli, A. (2019). Adaptability of naked barley landraces in mountain agro-ecosystem of Nepal. J. Nep. Agric. 5, 34–42. doi: 10.3126/jnarc.v5i1.22064
Goodman, M. M., and Brown, W. L. (1988). “Races of Corn,” in Corn and Corn Improvement. John Wiley and Sons. Ltd. p. 33–79. doi: 10.2134/agronmonogr18.3ed.c2
Hillman, G. (2001). “Archaeology, percival, and the problems of identifying wheat remains,” in The Linnean Speciaal Issue no 3. Wheat Taxonomy: The Legacy of John Percival, eds P. D. S. Caligari and P. D. Brandham (London: Academic Press Limited), 27–36.
Hillman, G. C., Mason, S., de Moulins, D., and Nesbitt, M. (1996). Identification of archaeological remains of wheat: the 1992 London workshop. J. Assoc. Environ. Archaeol. 12: 195–209.
Jacomet, S. (2006). Identification of Cereal Remains From Archaeological Sites. 2nd ed. Basel: Basel University.
Jacomet, S., and Schlichtherle, H. (1984). “Der kleine Pfahlbauweizen Oswald Heer's: neue Untersuchungen zur Morphologie neolithischer Nacktweizen-Ähren,” in Plants and ancient man. Proceedings of the sixth symposium of the international work grop for palaeoethnobotany 1983 in Groningen, van Zeist, W., and Casparie, W. A. (eds). Rotterdam. p. 153–176.
Knüpffer, H., Terentyeva, I., Hammer, K., Kovaleva, O., and Sato, K. (2003). “Ecogeographical diversity–a Vavilovian approach,” in Diversity in barley, eds. R. von Bothmer, T. van Hintum, H. Knüpffer, and K. Sato (Amsterdam: Elsevier), 53–76.
Leroy, S. A., and Giralt, S. R. (2021). Humid and cold periods in the last 5600 years in Arid Central Asia revealed by palynology of Picea schrenkiana from Issyk-Kul. The Holocene. 31. doi: 10.1177/0959683620972776
Lister, D. L., Jones, H., Oliveira, H. R., Petrie, C. A., Liu, X., Cockram, J., et al. (2018). Barley heads east: Genetic analyses reveal routes of spread through diverse Eurasian landscapes. PLoS ONE. 13, e0196652.
Liu, X., Lister, D. L., Zhao, Z., Staff, R. A., Jones, P. J., Zhou, L., et al. (2016). The virtues of small grain size: Potential pathways to a distinguishing feature of Asian wheats. Quaternary Int. 426, 107–119.
Magliano, P. N., Prystupa, P., and Gutiérrez-Boem, F. H. (2014). Protein content of grains of different size fractions in malting barley. J. Inst. Brew. 120, 347.
Martin, A. C., and Barkley, W. D. (1961). Seed Identification Manual. Berkeley, CA: University of California Press.
Miller, N. (2003). “The use of plants at Anau North,” in A Central Asian village at the dawn of civilization, excavations at Anau, Turkmenistan, Hiebert, F. T., and Kurdansakhatov, K. (eds). (Philadelphia: University of Pennsylvania Museum) p. 127–138.
Miller, N. F. (1999). Agricultural development in western Central Asia in the Chalcolithic and Bronze Ages. Veg. Hist. Archaeobot. 8, 13–19.
Moles, A. T., Ackerly, D. D., Webb, C. O., Tweddle, J. C., Dickie, J. B., and Westoby, M. (2005). A brief history of seed size. Science. 307, 576–580. doi: 10.1126/science.1104863
Moore, K. M., Miller, N. F., Hiebert, F. T., and Meadow, R. H. (1994). Agriculture and herding in the early oasis settlements of the Oxus Civilization. Antiquity. 68, 418–427. doi: 10.1017/S0003598X00046767
Mori, N., Ohta, S., Chiba, H., Takagi, T., Niimi, Y., Shinde, V., et al. (2013). Rediscovery of Indian dwarf wheat (Triticum aestivum L. ssp. sphaerococcum (Perc.) MK.) an ancient crop of the Indian subcontinent. Genet. Resour. Crop Evol. 60, 1771–1775. doi: 10.1007/s10722-013-9994-z
Motuzaite Matuzeviciute, G., Abdykanova, A., Kume, S., Nishiaki, Y., and Tabaldiev, K. (2018). The effect of geographical margins on cereal grain size variation: case study for highlands of Kyrgyzstan. J. Archaeol. Sci. 20, 400–410.
Motuzaite Matuzeviciute, G., Hermes, T. R., Mir-Makhamad, B., and Tabaldiev, K. (2020a). Southwest Asian cereal crops facilitated high-elevation agriculture in the central Tien Shan during the mid-third millennium BCE. PLoS ONE. 15, e0229372. doi: 10.1371/journal.pone.0229372
Motuzaite Matuzeviciute, G., Mir-Makhamad, B., and Spengler, R. N. I. (2021a). Interpreting diachronic size variation in prehistoric Central Asian cereal grains. Front. Ecol. Evol. 9. doi: 10.3389/fevo.2021.633634
Motuzaite Matuzeviciute, G., Mir-Makhamad, B., and Tabaldiev, K. (2021b). The first comprehensive archaeobotanical analysis of prehistoric agriculture in Kyrgyzstan. Veget Hist. Archaeobot. doi: 10.1007/s00334-021-00827-0
Motuzaite Matuzeviciute, G., Preece, R., Wang, S., Colominas, L., Ohnuma, K., Kume, S., et al. (2015). Ecology and subsistence at the Mesolithic and Bronze Age site of Aigyrzhal-2, Naryn valley, Kyrgyzstan. Quat. Int. 437, 35–49.
Motuzaite Matuzeviciute, G., Tabaldiev, K., Hermes, T., Ananyevskaya, E., Grikpedis, M., Luneau, E., et al. (2020b). High-altitude agro-pastoralism in the Kyrgyz Tien Shan: new excavations of the Chap Farmstead (1065–825 cal b.c.). J. Field Archaeol. 45, 29–45. doi: 10.1080/00934690.2019.1672128
Motuzaite Matuzeviciute, G. M. (2018). The possible geographic margin effect on the delay of agriculture introduction into the East Baltic. Eesti Arheoloogia Ajakiri. 22, 149–162.
Motuzaite Matuzeviciute, G. M., Ananyevskaya, E., Sakalauskaite, J., Soltobaev, O., and Tabaldiev, K. (2022). The integration of millet into the diet of Central Asian populations in the third millennium BC. Antiquity. 1–15. doi: 10.15184/aqy.2022.23
Neef, R., Cappers, R. T. J., and Bekker, R. M. (2012). Digital Atlas of Economic Plants in Archaeology. Eelde: Barkhuis.
Nicolas, M., Gleadow, R., and Dalling, M. (1984). Effects of drought and high temperature on grain growth in wheat. Aust. J. Plant Physiol. 11, 66.
Reed, K., Sabljić, S., Šoštarić, R., and Essert, S. (2019). Grains from ear to ear: the morphology of spelt and free-threshing wheat from Roman Mursa (Osijek), Croatia. Veget Hist Archaeobot 28, 623–634. doi: 10.1007/s00334-019-00719-4
Reed, R. C., Bradford, K. J., and Khanday, I. (2022). Seed germination and vigor: ensuring crop sustainability in a changing climate. Heredity. 128, 450–459. doi: 10.1038/s41437-022-00497-2
Reimer, P. J., Austin, W. E. N., Bard, E., Bayliss, A., Blackwell, P. G., Ramsey, C. B., et al. (2020). The IntCal20 Northern hemisphere radiocarbon age calibration curve (0–55 cal kBP). Radiocarbon. 62, 725–757. doi: 10.1017/RDC.2020.41
Renfrew, J. M. (1973). Palaeoethnobotany: The Prehistoric Food Plants of the Near East and Europe. : London: Methuenand, Co LTD.
Ritchey, M. M., Sun, Y., Motuzaite Matuzeviciute, G., Shoda, S., Pokharia, A. K., Spate, M., et al. (2021). The wind that shakes the barley: the role of East Asian cuisines on barley grain size. World Archaeol. 53, 287–304. doi: 10.1080/00438243.2022.2030792
Rouse, L. M., Tabaldiev, K., and Matuzeviciute, G. M. (2021). Exploring Landscape Archaeology and UAV-Based Survey in the Kochkor Valley, Kyrgyzstan. J. Field Archaeol. 1–22. doi: 10.1080/00934690.2021.1945744
Salisbury, E. J. (1974). Seed size and mass in relation to environment. Proc. Royal Soc. 186, 83–88. doi: 10.1098/rspb.1974.0039
Skrajda-Brdak, M., Konopka, I., Tańska, M., Szczepanek, M., Sadowski, T., and Rychcik, B. (2020). Low molecular phytochemicals of Indian dwarf (Triticum sphaerococcum Percival) and Persian wheat (T. carthlicum Nevski) grain. J. Cereal Sci. 91, 102887. doi: 10.1016/j.jcs.2019.102887
Sofield, I., Evans, L. T., Cook, M. G., and Wardlaw, I. F. (1977). Factors influencing the rate and duration of grain filling in wheat. Functional Plant Biol. 4, 785–797. doi: 10.1071/pp9770785
Spate, M., Leipe, C., and Motuzaite Matuzeviciute, G. (2022). Reviewing the palaeoenvironmental record to better understand long-term human-environment interaction in inner asia during the late Holocene. Front. Ecol. Evol. 10.
Spengler, R. N. (2018). Dung burning in the archaeobotanical record of West Asia: where are we now? Veg. Hist. Archaeobot. 28, 215–227.
Spengler, R. N. (2019). Fruit from the Sands: The Silk Road Origins of the Foods We Eat. First edition. Oakland, California: University of California Press.
Spengler, R. N., Frachetti, M. D., Doumani, P., Rouse, L., Cerasetti, B., Bullion, E., et al. (2014). Early agriculture and crop transmission among Bronze Age mobile pastoralists of Central Asia. Proc. R. Soc. 281, 1–7.
Spengler, R. N., Ryabogina, N., Tarasov, P. E., and Wagner, M. (2016). The spread of agriculture into northern Central Asia: timing, pathways, and environmental feedbacks. The Holocene. 26, 1527–1540.
Spengler, R. N., and Willcox, G. (2013). Archaeobotanical results from Sarazm, Tajikistan, an Early Bronze Age Settlement on the edge: agriculture and exchange. Environ. Archaeol. 18, 211–221.
Stevens, C. J., and Fuller, D. Q. (2017). The spread of agriculture in eastern Asia: archaeological bases for hypothetical farmer/language dispersals. Lang. Dyn. Chang. 7, 152–186. doi: 10.1163/22105832-00702001
Stevens, C. J., Murphy, C., Roberts, R., Lucas, L., Silva, F., and Fuller, D. Q. (2016). Between China and South Asia: a Middle Asian corridor of crop dispersal and agricultural innovation in the Bronze Age. The Holocene. 26, 1541–1555.
van Bommel, D., Bruins, H. J., Lazarovitch, N., and van der Plicht, J. (2021). Effect of dung, ash and runoff water on wheat and barley grain sizes and stable isotope ratios: Experimental studies in ancient desert agriculture (Negev, Israel). J. Archaeol. Sci. 39, 103172. doi: 10.1016/j.jasrep.2021.103172
Weber, S. A. (1991). Plants And Harappan Subsistence: An Example Of Stability And Change From Rojdi. New York (NY): Routledge.
Yatoo, M. A., Spate, M., Betts, A., Pokharia, A. K., and Shah, M. A. (2020). New evidence from the Kashmir Valley indicates the adoption of East and West Asian crops in the western Himalayas by 4400 years ago. Quat. Sci. Rev. 2, 100011. doi: 10.1016/j.qsa.2020.100011
Zhang, Y., Yin, L., Huang, L., Tekliye, M., Xia, X., Li, J., et al. (2021). Composition, antioxidant activity, and neuroprotective effects of anthocyanin-rich extract from purple highland barley bran and its promotion on autophagy. Food Chem. 339, 127849. doi: 10.1016/j.foodchem.2020.127849
Zhou, X., Yu, J., Spengler, N. R., Shen, H., Zhao, K., Ge, J., et al. (2020). 5,200-year-old cereal grains from the eastern Altai Mountains redate the trans-Eurasian crop exchange. Nat. Plants. 6, 78–87.
Keywords: crop resilience, crop adaptation, pioneer crop package, Central Asia, archaeobotany, Bronze Age, earliest farmers, agropastoralists
Citation: Motuzaite Matuzeviciute G, van Unen M, Karaliute R and Tabaldiev K (2022) The resilience of pioneer crops in the highlands of Central Asia: Archaeobotanical investigation at the Chap II site in Kyrgyzstan. Front. Ecol. Evol. 10:934340. doi: 10.3389/fevo.2022.934340
Received: 02 May 2022; Accepted: 07 September 2022;
Published: 17 October 2022.
Edited by:
Anna Maria Mercuri, University of Modena and Reggio Emilia, ItalyReviewed by:
Alexandra Livarda, Catalan Institute of Classical Archaeology, SpainNaomi Miller, University of Pennsylvania, United States
Copyright © 2022 Motuzaite Matuzeviciute, van Unen, Karaliute and Tabaldiev. This is an open-access article distributed under the terms of the Creative Commons Attribution License (CC BY). The use, distribution or reproduction in other forums is permitted, provided the original author(s) and the copyright owner(s) are credited and that the original publication in this journal is cited, in accordance with accepted academic practice. No use, distribution or reproduction is permitted which does not comply with these terms.
*Correspondence: Giedre Motuzaite Matuzeviciute, Z2llZHJlLm1vdHV6YWl0ZUBnbWFpbC5jb20=
†ORCID: Giedre Motuzaite Matuzeviciute orcid.org/0000-0001-9069-1551
Manon van Unen orcid.org/0000-0002-2174-248X
Ruta Karaliute orcid.org/0000-0002-8733-2117
Kubatbek Tabaldiev orcid.org/0000-0002-6679-8030