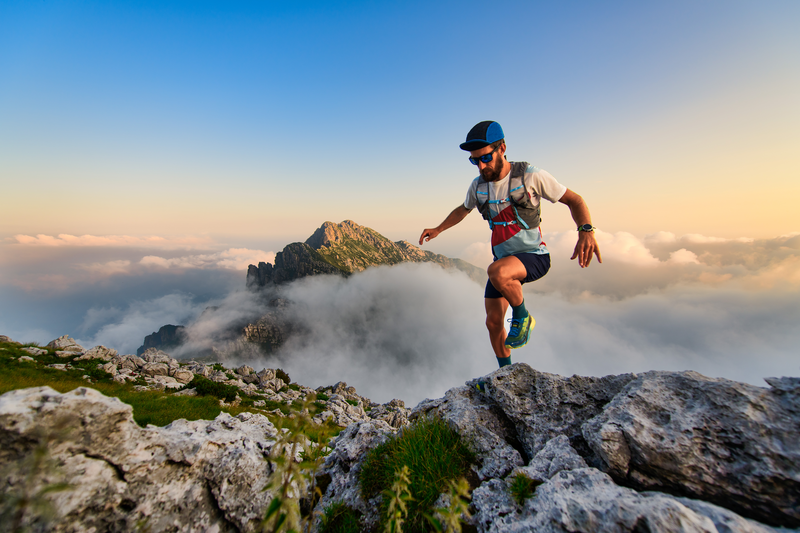
94% of researchers rate our articles as excellent or good
Learn more about the work of our research integrity team to safeguard the quality of each article we publish.
Find out more
ORIGINAL RESEARCH article
Front. Ecol. Evol. , 11 August 2022
Sec. Chemical Ecology
Volume 10 - 2022 | https://doi.org/10.3389/fevo.2022.930665
Mosquitoes use chemical codes to locate and discriminate among vertebrate hosts to obtain a blood meal. Recent advances have allowed for the identification of the chemical codes used by mosquitoes to locate and discriminate humans from other vertebrate hosts. Humans are incidental “dead-end” hosts for the West Nile virus, which is maintained in an enzootic cycle, primarily through its transmission between infected birds by Culex mosquitoes. Host-seeking Culex mosquitoes are attracted to the odor of chicken, which are used in sentinel traps to monitor West Nile virus transmission. Using combined gas chromatography and electroantennography and mass spectrometry we identify a blend of volatile organic compounds present in chicken emanates, including mostly salient bioactive compounds previously identified in human emanates. When released at their identified ratios, this blend elicits behavioral responses of Culex pipiens molestus and Culex quinquefasciatus similar to that to the natural chicken odor. Tested under field conditions, this blend attract Culex spp. and other species of mosquitoes using birds among their hosts. This study provides evidence for conserved chemical codes for resource location by mosquitoes, and highlights the intricate role of CO2 for host-seeking mosquitoes. The identification of conserved chemical codes, which drive innate preference behaviors that are fundamental for survival and reproduction, provides important substrates for future control interventions targeting disease vector mosquitoes.
Mosquitoes blood feed from a wide range of vertebrate host species, whereby they can transmit diseases between intra- and interspecific hosts. For many of these diseases, e.g., West Nile fever, non-human animals are the primary hosts, and human infections are incidental and often “dead-end” in nature (Farajollahi et al., 2011). West Nile fever is caused by a virus, which is maintained in an enzootic cycle, primarily through its transmission between infected birds by Culex mosquitoes (Chancey et al., 2015). While recent technical advances allow for the identification of the chemical codes used by mosquitoes to locate and discriminate humans from other vertebrate hosts (Bowen, 1991; Takken and Knols, 1999; Takken and Verhulst, 2013; McBride et al., 2014; Omondi et al., 2019; Zhao et al., 2020), the mechanism by which Culex mosquitoes select birds for blood feeding is poorly understood. Understanding the chemical codes used by mosquitoes for host selection, and how olfactory signaling and reception co-evolve, is fundamental for assessing how natural selection shapes adaptive behaviors (Lyimo and Ferguson, 2009; Takken and Verhulst, 2013). For applied purposes, these codes may be exploited in the ongoing development of novel vector management strategies.
Sentinel chickens are commonly used to monitor West Nile virus transmission (Chaskopoulou et al., 2013), as several Culex species prefer to blood feed on these birds (Elizondo-Quiroga et al., 2006; Gomes et al., 2013; Brugman et al., 2018). Culex mosquitoes are attracted to chicken odor, using volatile organic compounds for both host location and discrimination (Bernier et al., 2008; Cooperband et al., 2008; Spanoudis et al., 2020), the majority of which are saturated aldehydes (Bernier et al., 2008; Cooperband et al., 2008; Syed and Leal, 2009). Aldehydes, a common class of volatile organic compounds, are used by mosquitoes as aggregation pheromones, as well as nectar-, host-, and oviposition site-kairomones, demonstrating a likely parsimonious mechanism underlying the associated behaviors (e.g., Bernier et al., 2008; Nyasembe and Torto, 2014; Wondwosen et al., 2016; Ignell and Hill, 2020; Mozûraitis et al., 2020). Additional volatile organic compounds, however, are often needed to elicit attraction of mosquitoes, and to enable discrimination among resources (Takken and Knols, 1999; Ignell and Hill, 2020). Presented in proper ratios, such odor blends often decrease the threshold of behavioral response compared to that of single component attractants (Takken and Knols, 1999; Ignell and Hill, 2020). The response threshold may further be decreased by the presence of carbon dioxide (CO2), or a CO2 receptor agonist, which is critical for synergizing or gating the behavioral response of host-seeking mosquitoes to host volatile organic compounds (Gillies, 1980; Tauxe et al., 2013; Spanoudis et al., 2020).
Culex pipiens and Culex quinquefasciatus are the main vectors of West Nile virus in Europe and North America, respectively (Bernard and Kramer, 2001; Zeller and Schuffenecker, 2004). Although these species, especially the two biotypes of Culex pipiens, pipiens and molestus, blood feed on a distinct, or in some cases partially overlapping, range of host species, they are all attracted to the sentinel birds, and frequently feed on various bird species (Vinogradova, 2000; Apperson et al., 2004; Molaei et al., 2006; Roiz et al., 2012; Gomes et al., 2013; Rizzoli et al., 2015). Furthermore, behavioral analysis demonstrates that Cx. p. molestus and Cx. quinquefasciatus respond similarly to chicken odor (Spanoudis et al., 2020), suggesting that the detection of a signature chemical code within the chicken “volatilome” is conserved among these closely related species (Weitzel et al., 2009). In this study, we identify a blend of volatile organic compounds, which when released in their identified released ratios, and at relevant rates, reflects the behavioral response of Cx. p. molestus and Cx. quinquefasciatus to natural chicken odor. Tested under field conditions, this blend attracts Culex mosquitoes, and other species of mosquitoes, that include birds within their host range. Investigations under laboratory and field conditions clarify the mechanism by which mosquitoes may use CO2 for host location. We discuss these results in the perspective of conservation of chemical codes used by mosquitoes to locate vertebrate hosts, and how these can be exploited for developing novel control tools, especially against Culex mosquitoes.
Culex quinquefasciatus (Thai strain) and Cx. p. molestus (provided by Benaki Phytopathological Institute, Kifissia, Greece) were used for electrophysiological and behavioral analyses. The mosquitoes were reared at 27 ± 2°C, 70 ± 2% relative humidity (RH), and at a 12 h:12 h light:dark (L:D) photoperiod, as previously described (Spanoudis et al., 2020). Four-to-five days post-emergence, adult female Cx. quinquefasciatus were selected for the experiments. In contrast, autogenous Cx. p. molestus females were first allowed to oviposit to induce host seeking (Vinogradova, 2000; Spanoudis et al., 2020), and then used for the experiments, at 8–10 days post-adult emergence.
Headspace volatile extracts were collected in the field from live, male and female domestic chickens (Gallus gallus domesticus v. Golden Rocket; n = 6 and 7, respectively). Permission to handle birds was provided by the Greek Ministry of Environment and Energy (Department of Management of Wildlife and Hunting, Athens: 17-4-2013/129493/1072), in compliance with Directive 2010/63/EU in Europe. Individual birds were placed in a plastic cage (48 cm × 28 cm × 28 cm), covered with a heat-sealed oven bag (FoodSaver® Brand, United Kingdom), with an empty cage serving as a control. Charcoal-filtered air was introduced into the cages via a pump (1 l min–1; 12 V, KNF-Neuberger, Freiburg, Germany), and then extracted (1 l min–1) through a splitter connected to three adsorbent columns for 2.5 h. The columns were made of Teflon tubing (4 cm × 0.3 cm id), containing 40 mg Porapak Q (80/100 mesh, Alltech, Deerfield, IL, United States) between polypropylene wool plugs and Teflon stoppers. The columns were rinsed with 1 ml each of methanol (purity > 99.8%, Chem-Lab NV, Zedelgem, Belgium), acetone (purity > 99.5%, Chem-Lab NV, Zedelgem, Belgium) and pentane (purity > 99%, Chem-Lab NV, Zedelgem, Belgium) before use. The adsorbed volatiles were eluted with 600 μl of pentane (puriss p.a., Sigma-Aldrich Chemie GmbH, Steinheim, Germany). The extracts were pooled, concentrated to 50% of their original volume, and then stored in sealed glass vials at −20°C until used for behavioral, electrophysiological and chemical analyses.
Antennal responses of host-seeking Cx. quinquefasciatus and Cx. p. molestus to the chicken headspace extract were analyzed using combined gas chromatography and electroantennographic detection (GC-EAD). Volatile organic compounds were separated on an Agilent Technologies 6890 GC (Santa Clara, CA, United States), equipped with an HP-5 column (30 m × 0.25 mm id, fused silica, 0.25 μm film thickness, Agilent Technologies), with hydrogen as the mobile phase, at an average linear flow rate of 45 cm s–1. Each sample (2 μl) was injected splitless (30 s, injector temperature 225°C), and the GC oven temperature programmed from 35°C (3 min hold) at 10°C min–1 to 290°C (10 min hold). At the GC effluent, nitrogen was added and split 1:1 in a Gerstel 3D/2 low dead volume four-way cross (Gerstel, Mülheim, Germany) between the flame ionization detector and the EAD. The GC effluent capillary for the EAD passed through a Gerstel ODP-2 transfer line, which tracked the GC oven temperature, into a glass tube (10 cm × 7 mm ID), where the effluent was mixed with charcoal-filtered, humidified air (1.5 l min–1). The antenna was placed 0.5 cm from the outlet of this tube. The antennal preparation was made by inserting the distal end of the antenna, after cutting the distal segment, into a recording glass electrode filled with Beadle–Ephrussi Ringer, while the reference electrode, filled with Beadle–Ephrussi Ringer, was inserted into the capsule of the excised head and grounded. The recording electrode was connected to a pre-amplifier probe (10×) and then to a high impedance DC amplifier interface box (IDAC-2; Syntech, Kirchgarten, Germany). For each Culex species, 10 recordings were performed.
The pooled and concentrated chicken headspace extract was analyzed twice by combined gas chromatography and mass spectrometry (GC-MS), using two different fused silica capillaries (60 m, ID 250 μm; df = 0.25 μm) coated with either HP-5MS UI (Agilent Technologies) on an Agilent 6890-5975 GC-MS, or with DB-Wax (J&W Scientific, Folsom, CA, United States) on an Agilent 7890-5977 GC-MS (Agilent Technologies). Both GC-MS setups were operated in the electron impact ionization mode at 70 eV. Helium was used as the mobile phase at an average linear flow rate of 35 cm s–1. The volume injected and the temperature programs were the same as those used for the GC-EAD analysis. The volatile organic compounds were identified according to retention times (Kovat’s indices) and mass spectra, in comparison with custom made and NIST14 libraries (Agilent), and confirmed by co-injection of authentic standards: m-xylene (CAS no. 108-38-3; Aldrich, 99%), heptanal (CAS no. 111-71-7; Aldrich, 95%), 3-octanone (CAS no. 106-68-3; Aldrich, 98%), benzaldehyde (CAS no. 100-52-7; Aldrich, 99%), sulcatone (CAS no. 110-93-0; Fluka, 96%), octanal (CAS no. 124-13-0; Aldrich, 99%), R-(+)-limonene (CAS no. 5989-27-5; Aldrich, 97%), p-cresol (CAS no. 106-44-5; Aldrich, 99%), non-anal (CAS no. 124-19-6; Aldrich, 95%), decanal (CAS no. 112-31-2; Aldrich, 92%) and geranyl acetone (CAS no. 689-67-8; Aldrich, 95%). R-(+)-limonene was the only limonene enantiomer eliciting EAD activity. For the approximation of release rate, 100 ng of heptyl acetate (99.8% chemical purity; Aldrich) was added as an internal standard to a 20 μl aliquot out of the total 400 μl headspace extract.
Behavioral assays were conducted in a Y-tube olfactometer (Spanoudis et al., 2020). The olfactometer was illuminated with red light from above at 40 lux. A charcoal-filtered and humidified air stream (25 ± 2°C, 65 ± 2% RH) flowed through the bioassay at 30 cm s–1. When required, CO2 was delivered in 1 s on-off pulses at 600 ppm via a stimulus controller (SEC-2/b, Syntech). The consistency in amplitude, and the structure of the pulsed stimuli was visualized using a mini-photo ionization detector (PID; Aurora Scientific, Aurora, Ontario, Canada), and the concentration of CO2 measured at the downwind and upwind end of the assay via a CO2 analyzer (LI-820, LICOR Biosciences, Lincoln, NE, United States) (Majeed et al., 2017).
The behavioral activity of the mosquitoes was analyzed in response to both a synthetic blend and a pooled natural headspace volatile extract of chicken odor. The synthetic blend (stock: 1 μl heptanal; 5 μl octanal; 50 μl non-anal; 10 μl decanal; 5 μl 3-octanone; 7 μl sulcatone; 2 μl benzaldehyde diluted in 2 ml pentane), diluted in pentane, mimicked the composition and ratio of the bioactive compounds identified in the pooled natural extracts of chickens (Supplementary Figure 1). Dose-response assays, in which the ratio among the compounds within the blend was maintained, were conducted in the Y-tube olfactometer for both species, with pentane as a control. Preference for either the pooled natural headspace volatiles, or the synthetic odor blend, of chickens were assessed in the Y-tube olfactometer, using the dose identified to elicit the highest behavioral response, respectively (Spanoudis et al., 2020; this study). Subtractive bioassays followed to determine the relative activity of the identified components, using the dose of the synthetic odor blend, which elicited the highest behavioral response as a basis. For all experiments, the synthetic odor blend, the headspace volatile extract or the pentane control were pipetted (100 μl) onto a piece of filter paper (55 mm, Munktell, Munksjö, Sweden), which was suspended from a 5 cm wire coil at the upwind end of the assays. These filter papers were renewed before each experimental replicate.
Prior to the behavioral experiments, females of both species were starved for 8 h, and only given access to water. Two hours before the experiments, females were collected and transferred in groups of three to release cages to acclimatize. At the time for experiments, a release cage was placed at the downwind end of the assays, where the insects were allowed 3 min to adapt, before the butterfly valve of the release cage was opened. Thereafter, the choice of each mosquito was observed for a maximum of 4 min. Attraction to either treatment or control was analyzed as the proportion of mosquitoes that made source contact within this time. Data collected from the Y-tube olfactometer is presented as a Preference Index (PI), calculated as (T − C)/(T + C), where T is the number of mosquitoes associated with the test odor, and C the number of mosquitoes associated with the control. Ten replicates were conducted for each experimental condition (30 individuals in total), with dose-response experiment (with doses tested consecutively, starting with the lowest dose) and control flights performed each day. All behavioral experiments were conducted during host seeking time, in the early part of scotophase (Zeitgeber time 15 ± 2 h) (Yee and Foster, 1992).
The effect of dose of the synthetic blend, as well as the presence and absence of CO2, on the preference of Cx. p. molestus and Cx. quinquefasciatus was estimated using binomial regression of the generalized linear model GLM; JMP Pro version 13 SAS Institute Inc., Cary, NC, United States). Two sample t-tests were used to identify significant differences in the attraction to the chicken extract and the synthetic blend for each mosquito species separately. Responses of preference of Cx. p. molestus and Cx. quinquefasciatus to the synthetic and subtractive blends were analyzed using a generalized linear model followed by Dunnett’s post-hoc tests.
Field experiments were conducted in the urban area of Sindos, Thessaloniki, Greece (40.67°N, 22.79°E), and in Cedar Key Scrub State Reserve, FL, United States (29.11°N, 83.2°W). The experimental design at both field sites followed a Latin square, in which treatments were randomly assigned on the first day, and then rotated to minimize location bias over the following days. In Sindos, the experiments were conducted in August 2015, using four Encephalitis Vector Survey (EVS) mosquito traps (BioQuip Products, Inc., Rancho Dominguez, CA, United States) that were rotated daily, for a total of 16 days, so that each trap (treatment and control) was tested four times at all selected sites. The distance between traps was approximately 100 m. In the Cedar Key Scrub State Reserve, the experiments were conducted in December 2017, using sixteen Centre of Disease Control (CDC) traps (John W. Hock Company, Gainesville, FL, United States), located at 2 sub-sites, with eight traps at each site, spaced at least 25 m apart, and rotated daily within the individual sub-site, for a total of 8 days. The traps were placed in the cover of vegetation or next to residences, approximately 1 m above ground level.
The traps were baited with combinations of CO2 and/or the synthetic odor blend, with heptane (99% purity, Merck, Darmstadt, DE) as a control. Dry ice, either 1 kg (United States) or 1.5 kg (Greece) served as the source of CO2, which was contained and released from insulated dry ice dispensers (BioQuip Products, Inc.). The synthetic odor blend (4 ml), with 400 ng min–1 of decanal within the blend, and the solvent control (4 ml) were released by diffusion from wick dispensers (Karlsson et al., 2013). The dispensers were made from a 4 ml glass vial with a pinhole in the center of the cap through which a cotton wick encased in Teflon protruded into the air. Heptane was used to allow for the release of compounds throughout the course of the experiment. The dispensers were hung on the outside of the traps close to the trap entrance. Only a small amount of dry ice remained in the dispenser in the following morning. Each trap was set to operate from 2 h before sunset until 2 h after sunrise. Insects caught in each trap were collected daily, placed in between paper towels, and then stored at −20°C. In the laboratory, the collected mosquitoes were enumerated and identified to species level, when possible, under a microscope using morphological identification keys (Snow, 1990; Darsie<suffix>Jr.</suffix>, and Morris, 2003; Darsie and Ward, 2005).
For the field evaluation of the chicken blend, a mixed effects model (JMP® Pro 12.0.1. SAS Institute Inc., Cary, NC, United States) was used to identify significant differences between the treatments used to collect mosquitoes in the field. The number of mosquitoes collected was added into the model as the response value. Treatment was added to the model as a fixed effect, as was the two different sub-sites used in the Cedar Key Scrub State Reserve. Day, as well as trap position, were selected as random effects in the model. The two sub-sites were nested within the model to account for potential differences at these sites. An all pairwise comparison t-test was used to identify significant differences between the treatments. The F and P-value for the test is here reported.
Cyclopentanone has previously been shown to act as a CO2 receptor agonist, eliciting attraction and increasing trap capture of Cx. quinquefasciatus in semi-field enclosures (Tauxe et al., 2013). To assess whether cyclopentanone could be a viable option to replace CO2, we initially validated its effect on Cx. quinquefasciatus, in the presence and absence of the synthetic chicken odor blend, using a straight tube olfactometer (Majeed et al., 2017). As a control, mosquitoes were flown to CO2, in the presence and absence of the synthetic chicken odor. Cyclopentanone (≥ 99%, Sigma-Aldrich Chemie GmbH), 1% or 10% diluted in pentane, was released from a wick dispenser at a rate of 8 μl min–1. Wick dispensers, containing the synthetic chicken odor or cyclopentanone, were placed within a glass wash bottle (250 ml; Lenz Laborglas, Wertheim, Germany). Charcoal-filtered and humidified air (0.3 l min–1) was passed through the wash bottle and delivered via low-density polyethylene tubing at the upwind end of the olfactometer. When applicable, air (0.5 l min–1) mixed with pulses (1 s on and 1 s off intervals) of 600 ppm CO2, using a stimulus controller (SEC-2/b. Syntech, Buchenbach, Germany), was delivered through a hole (1 cm i.d.) at the upwind end of the olfactometer via low-density polyethylene tubing (0.4 cm i.d.) (Majeed et al., 2014). The concentration of CO2, at the release was measured using a CO2 analyzer (LI-820, Licor Bioscience, United States).
Prior to and during the experiment, female Cx. quinquefasciatus were kept under the same condition as mentioned above. Five mosquitoes were placed in a release cage and left for 30 s to acclimatize at the downwind end of the olfactometer, after which the butterfly valve of the cage was opened. After 2 min the number of females reaching the upwind end was recorded, and the proportion of mosquitoes reaching the other side was calculated. A total of 10 replicates were performed. A nominal logistic fit model (JMP® Pro 12.0.1. SAS Institute Inc., Cary, NC, United States) was used to evaluate any significant differences in the proportion of female Cx. quinquefasciatus responding to the chicken blend and CO2/cyclopentanone. The treatment was used as the dependent variable in the model, weighted by the proportion of females, and dose was set as the independent fixed effect in the model. The χ2 and P-value from the likelihood ratio test is reported.
Field experiments, assessing cyclopentanone as a CO2 replacement (10% diluted in heptane), were subsequently conducted in Cedar Key Scrub State Reserve, FL, United States, in December 2018. The design of the experiment was similar to that described above, with the addition of dispensers containing two different doses of the synthetic chicken odor (1:10, reflecting the release rate used for the field evaluation of the blend, and a decadic dilution of this, 1:100). Dispensers containing cyclopentanone were hung next to those containing the synthetic chicken odor, where applicable. A mixed effects model, as described above, was used to evaluate these results.
Combined GC-EAD analysis, using the antennae of either Cx. p. molestus or Cx. quinquefasciatus as a biological detector, revealed consistent responses to 14 bioactive volatile organic compounds in the pooled headspace extract of chickens (Figure 1A), which were not present in the control. Of the bioactive compounds eliciting antennal responses, eight elicited a response in both species, while six elicited responses in either Cx. p. molestus (an unidentified dimethylheptene) or Cx. quinquefasciatus (R-(+)-limonene, unidentified branched C12- and C15-isomers, p-cresol, and geranyl acetone). Of the eight compounds found to elicit a response in both species, we were unable to identify an alkane, a methyloctane. The overall volatile release rate of bioactive compounds was approximately 100 ng min–1, with non anal, decanal, and octanal as the most abundant compounds (Figure 1A).
Figure 1. Host-seeking Culex pipiens molestus and Culex quinquefasciatus respond to chicken odor. (A) Combined gas chromatography and electroantennographic detection (GC-EAD) analyses of the two mosquito species identify the bioactive volatile organic compounds present in the headspace of chickens. Electroantennographic detection traces depict voltage changes (mV) in response to the bioactive compounds eluting from the GC, which are registered by the flame ionization detector. Asterisks indicate non-reproducible physiological responses. The identity and release rate of the bioactive compounds are shown to the right. u/i: unidentified volatile organic compound. (B,C) A synthetic blend composed of the bioactive compounds, in their natural ratio (A), elicited attraction (positive preference indices) of both Culex spp. in a Y-tube olfactometer, which was dependent on the presence or absence of carbon dioxide (CO2), as demonstrated using binomial regression. Mosquitoes demonstrated no significant preference for either side of the Y-tube olfactometer in the presence of a control (pentane) (C vs. C) or in the presence of CO2 in one of the upwind arms (CO2 vs. C) as shown on the left of the graph. The preference index was calculated as (T − C)/(T + C), where T is the number of mosquitoes associated with the test odor, and C the number of mosquitoes associated with the control. (D) The two Culex species were unable to discriminate between the natural headspace (filled) and the synthetic blend (open) of chicken odor, when assessed in a two-choice olfactometer (two sample t-test).
A synthetic blend of the seven shared GC-EAD-bioactive compounds identified in the pooled headspace extract of chickens, in their identified ratio (Figure 1A; indicated in bold italics), elicited dose-dependent attraction of both Cx. p. molestus (F = 2.498, df = 4, P = 0.05; Figure 1B) and Cx. quinquefasciatus (F = 2.479, df = 4, P = 0.05; Figure 1C). The presence of CO2 elicited a similar dose-dependent attraction of Cx. p. molestus to the synthetic blend (F = 2.851, df = 4, P = 0.034), a response that was not significantly different from that to the synthetic blend alone (X2 = 2.995; df = 1; P = 0.084). In contrast, for Cx. quinquefasciatus, the presence of CO2 elicited an aversive response to the synthetic blend within the full range of doses tested (X2 = 3.7, df = 1, P < 0.05). To further compare the potency of the synthetic chicken odor blend to attract the two Culex species, this was tested against the pooled natural headspace extract (released at 17 min equivalents; Spanoudis et al., 2020) in a Y-tube olfactometer, revealing no significant difference for neither Cx. p. molestus (t = 0.126; P = 0.901) nor Cx. quinquefasciatus (t = 0.941; P = 0.354) (Figure 1D).
The subtraction of individual components from the full synthetic blend, released at a dose of 10–3, generally reduced the attraction of host-seeking Culex mosquitoes significantly, when compared to the attraction response to the full blend, with the exception of the removal of non anal in the case of Cx. p. molestus and Cx. quinquefasciatus and 3-octanone of Cx. quinquefasciatus (Figures 2A,B).
Figure 2. Behavioral responses of host-seeking Culex pipiens molestus (A) and Culex quinquefasciatus (B) to subtractive blends based on chicken odor. Attraction to the full chicken blend was generally significantly reduced compared to that of the full chicken odor blend, as demonstrated using a generalized linear model followed by Dunnett’s post-hoc tests. Different lowercase letters indicate significant differences in attraction between the subtractive blend and the full chicken odor blend. The preference index was calculated as (T − C)/(T + C), where T is the number of mosquitoes associated with the test odor, and C the number of mosquitoes associated with the control. Error bars denote standard error of the mean.
The efficacy of the synthetic chicken odor to attract mosquitoes under field conditions was assessed at two geographical sites, Sindos, Thessaloniki (Greece) and Cedar Key Scrub State Reserve (FL, United States) (Figures 3A,B), each with disparate mosquito fauna. At each site, traps baited with the synthetic odor blend, in combination with CO2, caught significantly higher number of mosquitoes compared to the other treatments (Thessaloniki: F = 53.76, df = 3, P < 0.0001; Cedar Key Scrub State Reserve: F = 64.49, df = 3, P < 0.0001; Figures 3A,B). When trap captures from Thessaloniki were analyzed at the genus level, a significantly higher number of Culex, Anopheles, and Culiseta mosquitoes were found in traps baited with the synthetic odor blend, in combination with CO2, in comparison to the other lure types (Figure 3A). Similarly, a significantly higher number of Culex mosquitoes were caught in traps baited with the synthetic odor blend, in combination with CO2, at Cedar Key Scrub State Reserve (Figure 3B). The predominant species caught in Thessaloniki and at Cedar Key Scrub State Reserve were Cx. pipiens (80%) and Cx. salinarius (72%), respectively, both of which include birds in their host ranges (Tables 1, 2). Several of the other species caught in traps baited with the synthetic odor blend, in combination with CO2, have also been reported to display similar host preferences (Tables 1, 2). Interestingly, a higher number of the annelid specialist, Uranotaenia sapphirina, were caught in traps baited with the synthetic odor blend alone, compared to the other trap types at Cedar Key Scrub State Reserve (Table 2).
Figure 3. Field assessment of the synthetic chicken odor. Deployment of traps (°) in (A) Sindos, Thessaloniki (Greece) and (B) Cedar Key Scrub State Reserve (FL, United States). At both sites, the synthetic chicken odor blend, when combined with carbon dioxide (CO2), significantly enhanced the abundance and diversity of mosquito species that include birds in their diet, when compared to CO2-baited and control traps, when analyzed using a mixed effects model. In the absence of CO2, traps baited with the synthetic chicken odor blend caught similar number of mosquitoes as the control traps, as shown using a pairwise comparison t-test. Lowercase letters indicate significant differences by P < 0.05. Error bars denote standard error of the mean (A: N = 16; B: N = 32).
Table 1. Species and number of mosquitoes caught in Sindos, Thessaloniki (Greece) using odor-baited Encephalitis Vector Survey mosquito traps.
Table 2. Species and number of mosquitoes caught at Cedar Key Scrub State Reserve (FL, United States) using odor-baited Center of Disease Control mosquito traps.
Under laboratory conditions, CO2 and cyclopentanone, when presented at a concentration of 10%, alone or in combination with the synthetic chicken odor had a significant effect on the attraction of host-seeking Cx. quinquefasciatus (χ2 = 79.92, df = 5, P < 0.0001) (Figure 4A). An additive significant effect was observed when combining either CO2 or cyclopentanone with the synthetic chicken odor (Figure 4A).
Figure 4. Differential response of mosquitoes to the carbon dioxide (CO2) receptor agonist, cyclopentanone. (A) Under laboratory conditions, cyclopentanone (CP) reproduced the behavioral response of Culex quinquefasciatus to CO2 in the presence or absence of the synthetic chicken odor blend. A nominal logistic fit model was used for statistical analysis. Lowercase letters indicate significant differences by odd ratios (likelihood ratio test) in pairwise comparisons, P < 0.05. Error bars denote standard error of the mean (N = 10). (B) Tested under field conditions at Cedar Key Scrub State Reserve (FL, United States) (Figure 2B), traps baited with cyclopentanone, in combination with the synthetic chicken odor, caught significantly overall higher number of Uranataenia sapphirine and Uranataenia lowii when compared to traps baited with cyclopentanone alone, and to control traps, when analyzed using a mixed effects model. The two Uranataenia species were also attracted to, and caught in, traps baited with CO2 and a low dose of the synthetic chicken odor blend. Lowercase letters indicate significant differences by, P < 0.05. Error bars denote standard error of the mean (N = 9).
In the field, an overall significant effect was observed on trap captures when CO2 and the receptor agonist cyclopentanone were evaluated together with the synthetic chicken odor in the field at Cedar Key Scrub State Reserve (F-ratio = 23.51, df = 8, P < 0.0001). However, the CO2 receptor agonist, cyclopentanone, had no significant effect on the trap captures of available Culex and Anopheles species, when presented alone or in combination with the synthetic chicken odor (P > 0.05). Thus, the observed overall significant effect on trap captures at Cedar Key Scrub State Reserve was attributed mainly to the performance of CO2-baited traps, which, in the presence and absence of the synthetic chicken odor, caught a significantly higher number of Culex mosquitoes compared to the other trap types (P < 0.0001). The species composition of Culex and Anopheles mosquitoes caught in the traps reflected that presented in Table 2 (data not shown). The only species caught in significantly higher numbers in traps baited with the CO2 receptor agonist in combination with the synthetic chicken odor were U. sapphirinia and U. lowii, specialist feeders on annelids and amphibians, respectively, both of which were also caught in significant numbers in traps baited with CO2 in combination with a low release rate of the synthetic chicken odor (Figure 4B).
Conserved chemical codes, consisting of a complex blend of salient volatile organic compounds, drive long-range host seeking and discrimination in mosquitoes (Ignell and Hill, 2020; Zhao et al., 2020). Evidence provided in this study emphasizes that the physiological and behavioral response to such codes is selected for through purifying selection, or has evolved convergently, as both closely and distantly related taxa of mosquitoes were attracted to the chemical code of chicken odor, when presented in the background of CO2. The CO2 receptor agonist, cyclopentanone, while failing to gate the response of bird-preferring mosquitoes to the synthetic chicken odor blend in the field, significantly increased the trap capture of two highly specialized mosquito species. This highlights the intricate role of CO2 for host-seeking mosquitoes. The identification of conserved chemical codes, which drive innate preference behaviors that are fundamental for survival and reproduction, provides important substrates for future control interventions targeting disease vector mosquitoes.
The chemical code of chicken odor for a mosquito is composed of a single chicken-specific bioactive volatile organic compound (3-octanone), and several salient bioactive volatile organic compounds, which previously were identified from other mosquito resources, e.g., human emanates, and demonstrated to elicit physiological and behavioral responses in mosquitoes, albeit at different relative ratios (Omondi et al., 2019; Ignell and Hill, 2020). Consistent with previous analyses of vertebrate host odor, chicken odor is enriched in aliphatic aldehydes, but demonstrates an apparent lower abundance of the long-chain aldehyde, decanal, and the ketone, sulcatone, when compared to human odor (Omondi et al., 2019; Zhao et al., 2020). Notably, non anal, to which Culex mosquitoes have an acute sensitivity (Syed and Leal, 2009), appears to be redundant for eliciting host seeking. The short-chain aliphatic aldehydes and sulcatone, as well as benzaldehyde, are common volatile organic constituents of bird odor (Campagna et al., 2011), and a recent analysis of the odor profile of quail, which plays an important role in the ecology of mosquito-borne encephalitis (Reisen et al., 2006), demonstrate similar relative abundance of the salient bioactive volatile organic compounds identified in chickens (this study; Zhao et al., 2020). Available data thus suggest that mosquitoes that include birds in their diet, either as specialist or generalist feeders, can distinguish birds from humans, and other animals, by their unique odor bouquet, in terms of blend composition and ratio of volatile organic compounds.
Culex p. molestus and Cx. quinquefasciatus are members of the enigmatic Cx. pipiens species complex, which demonstrate differential traits, e.g., host and feeding site preferences, reflected in differential gene expression and nucleotide polymorphisms, including genes involved in odorant detection (Aardema et al., 2021; Kang et al., 2021; Gu et al., 2022). Comparative GC-EAD analyses of Cx p. molestus and Cx. quinquefasciatus revealed conserved, as well as unique, receptivity to host odorants between the two species, likely augmented by changes in the function, and/or in the presence/absence or abundance of subsets of chemosensory genes, including odorant receptors (Gu et al., 2022). Future functional characterization of the divergently expressed odorant receptors identified in Cx p. molestus and Cx. quinquefasciatus by Gu et al. (2022) will be pertinent for identifying the molecular mechanism underlying host selection and discrimination in these species. Considering that divergent taxa of mosquitoes, including Cx. quinquefasciatus, An. coluzzii, and Aedes aegypti, all respond to the aliphatic aldehydes, benzaldehyde, the ketones, sulcatone and geranyl acetone, as well as limonene, that are present in chicken and human emanates (this study; Omondi et al., 2019; Zhao et al., 2020), suggests that odorant receptor function has evolved convergently. Whether the lack of sensitivity to geranyl acetone and limonene, two compounds present in human odor emanates (Omondi et al., 2019; Zhao et al., 2020), is due to a loss of function in Cx. p. pipiens, and makes Cx. quinquefasciatus more prone to be attracted to humans for blood feeding compared to Cx. p. molestus awaits further analysis.
Carbon dioxide is a known activator and attractant, as well as gates the response of mosquitoes to other host odors (McMeniman et al., 2014). While CO2 decreases or inhibits the behavioral response of Cx. p. molestus and Cx. quinquefasciatus, respectively, in a Y-tube assay (this study, Spanoudis et al., 2020), this was not observed using a no-choice assay or under field conditions, emphasizing the stealthy host-approach behavior of these species (Edman and Scott, 1987; Cooperband and Cardé, 2006). While the CO2 receptor agonist, cyclopentanone, attracted Cx. quinquefasciatus at a comparable level as CO2 under laboratory conditions, supporting previous observations (Tauxe et al., 2013), it failed to lure mosquitoes, with the notable exception of U. sapphirinia and U. lowii, in the field. This may be due to different volatility of CO2 and cyclopentanone. The fact that these specialist feeders on annelids and amphibians (Reeves et al., 2018) are caught in significantly higher numbers in traps baited with the CO2 receptor agonist and a synthetic odor blend derived from chickens, than in equivalent traps baited with CO2 and the synthetic blend, suggests that (a) they are acutely tuned to the low levels of CO2 emitted by these animals, and (b) tuned to at least some of the salient volatile compounds in this synthetic odor blend. In support of the latter, chemical analyses of frog odor (Smith et al., 2000; Brunetti et al., 2015, 2019) have demonstrated the presence of the short-chain aldehydes, sulcatone and limonene identified in chicken emanates. The presence of these, and other volatile organic compounds identified in vertebrate host odor demonstrates substantial chemical parsimony, and a strong adaptation pressure on mosquitoes to use these signals to locate resources.
While recent successes in vector control have led to major reductions in vector-borne diseases, there are still many examples of resurgence and spread of vector populations, exacerbated by increased insecticide resistance (Amelia-Yap et al., 2018; Riveron et al., 2018), global trade (Lillepold et al., 2019), travel (Lillepold et al., 2019) and climate change (Rocklov and Dubrow, 2020; Colon-Gonzalez et al., 2021; Ligsay et al., 2021). Odor-baited traps are deemed a viable component for future integrated vector management programmes, which can be used for both surveillance and control of vector mosquitoes (Mafra-Neto and Dekker, 2019). Challenges overcome in this study have allowed for the identification of a chemical code, and a formulation mimicking the release rate and ratio of compounds within the code, which attracts a wide range of mosquito taxa, including vectors of the West Nile virus, for which no efficient attractant is currently available. Further optimization of the blend may, however, be required for increased species selectivity. Additional obstacles to overcome to make the current lure, and other emerging odor-based lures available for integrated vector management is product registration, which is hampered by cost and stringent regulatory bureaucracy, to allow the product to be adopted by national programs or other stakeholders.
The original contributions presented in this study are included in the article/Supplementary material, further inquiries can be directed to the corresponding author.
RI conceived, designed the study, and wrote the manuscript. CS and SA collected the headspace volatiles. CS and EI performed the laboratory behavioral assays. BW performed the electrophysiological analysis and with the assistance of GB the chemical analysis. CS and SA performed the field experiments in Greece, whereas EI, with guidance of DK performed the field experiments in USA. CS, SA, and EI performed the statistical analyses. All authors had critically read and approved the final draft of the manuscript.
This research was mainly funded by the Swedish Research Council, Formas, and partially funded by the Foundation Lars Hiertas Minne (granted to SA) and the Hellenic State Scholarships Foundation in the framework of the Operational Programme “Education and Life Long Learning” within the National Strategic Reference Framework (2007–2013), co-financed by the European Social Fund and the Public Investments Programme (grant no. 18676 to CS).
The authors declare that the research was conducted in the absence of any commercial or financial relationships that could be construed as a potential conflict of interest.
All claims expressed in this article are solely those of the authors and do not necessarily represent those of their affiliated organizations, or those of the publisher, the editors and the reviewers. Any product that may be evaluated in this article, or claim that may be made by its manufacturer, is not guaranteed or endorsed by the publisher.
The Supplementary Material for this article can be found online at: https://www.frontiersin.org/articles/10.3389/fevo.2022.930665/full#supplementary-material
Supplementary Figure 1 | Relative proportion of individual components emitted from filter paper, wick dispensers and the natural headspace extracts of chicken. Emission of volatile organic compounds from filter paper was assessed using solid phase microextraction for 4 min (n = 3).
Aardema, M. L., Campana, M. G., Wagner, N. E., Ferreira, F. C., and Fonseca, D. M. (2021). A gene-based capture assay for surveying patterns of genetic diversity and insecticide resistance in a worldwide group of invasive mosquitoes. bioRxiv [Preprint] doi: 10.1101/2021.08.24.457535
Alto, B. W., Connelly, C. R., O’Meara, G. F., Hickman, D., and Karr, N. (2014). Reproductive biology and susceptibility of Florida Culex coronator to infection with West Nile virus. Vector Borne Zoonotic Dis. 14, 606–614. doi: 10.1089/vbz.2013.1501
Amelia-Yap, Z. H., Chen, C. D., Sofian-Azirun, M., and Low, V. L. (2018). Pyrethroid resistance in the dengue vector Aedes aegypti in Southeast Asia: Present situation and prospects for management. Parasit. Vectors 11:332. doi: 10.1186/s13071-018-2899-0
Apperson, C. S., Hassan, H. K., Harrison, B. A., Savage, H. M., Aspen, S. E., Farajollahi, A., et al. (2004). Host feeding patterns of established and potential mosquito vectors of West Nile virus in the eastern United States. Vector Borne Zoonotic Dis. 4, 71–82. doi: 10.1089/153036604773083013
Balenghien, T., Fouque, F., Sabatier, P., and Bicout, D. J. (2006). Horse-, bird-, and human-seeking behavior and seasonal abundance of mosquitoes in a West Nile virus focus of southern France. J. Med. Entomol. 43, 936–946. doi: 10.1603/0022-2585(2006)43[936:hbahba]2.0.co;2
Bernard, K. A., and Kramer, L. D. (2001). West Nile virus activity in the United States, 2001. Viral Immunol. 14, 319–338.
Bernier, U. R., Allan, S. A., Quinn, B. P., Kline, D. L., Barnard, D. R., and Clark, G. G. (2008). Volatile compounds from the integument of White Leghorn chickens (Gallus gallus domesticus L.): Candidate attractants of ornithophilic mosquito species. J. Sep. Sci. 31, 1092–1099. doi: 10.1002/jssc.200700434
Boreham, P. F. L., and Garrett-Jones, C. (1973). Prevalence of mixed blood meals and double feeding in a malaria vector (Anopheles sacharovi Favre). Bull. World Health Organ. 48:605.
Bowen, M. F. (1991). The sensory physiology of host-seeking behavior in mosquitoes. Annu. Rev. Entomol. 36, 139–158.
Braverman, Y., Kitron, U., and Killick-Kendrick, R. (1991). Attractiveness of vertebrate hosts to Culex pipiens (Diptera: Culicidae) and other mosquitoes in Israel. J. Med. Entomol. 28, 133–138. doi: 10.1093/jmedent/28.1.133
Brugman, V. (2016). Host selection and feeding preferences of farm-associated mosquitoes (Diptera: Culicidae) in the United Kingdom. [dissertation thesis]. London: London School of Hygiene & Tropical Medicine.
Brugman, V. A., Hernández-Triana, L. M., Prosser, S. W., Weland, C., Westcott, D. G., Fooks, A. R., et al. (2015). Molecular species identification, host preference and detection of myxoma virus in the Anopheles maculipennis complex (Diptera: Culicidae) in southern England, UK. Parasit. Vectors. 8:421. doi: 10.1186/s13071-015-1034-8
Brugman, V., Hernández-Triana, L., Medlock, J., Fooks, A., Carpenter, S., and Johnson, N. (2018). The role of Culex pipiens L. (Diptera: Culicidae) in virus transmission in Europe. Int. J. Environ. Res. Public Health. 15, 389–419. doi: 10.3390/ijerph15020389
Brunetti, A. E., Lyra, M. L., Melo, W. G., Andrade, L. E., Palacios-Rodríguez, P., Prado, B. M., et al. (2019). Symbiotic skin bacteria as a source for sex-specific scents in frogs. Proc. Natl. Acad. Sci. U.S.A. 116, 2124–2129. doi: 10.1073/pnas.1806834116
Brunetti, A. E., Merib, J., Carasek, E., Caramão, E. B., Barbará, J., Zini, C. A., et al. (2015). Frog volatile compounds: Application of in vivo SPME for the characterization of the odorous secretions from two species of Hypsiboas treefrogs. J. Chem. Ecol. 41, 360–372. doi: 10.1007/s10886-015-0564-z
Campagna, S., Mardon, J., Celerier, A., and Bonadonna, F. (2011). Potential semiochemical molecules from birds: A practical and comprehensive compilation of the last 20 years studies. Chem. Senses. 37, 3–25. doi: 10.1093/chemse/bjr067
Chancey, C., Grinev, A., Volkova, E., and Rios, M. (2015). The global ecology and epidemiology of West Nile virus. Biomed. Res. Int. 2015, 376230.
Chaskopoulou, A., Dovas, C. I., Chaintoutis, S. C., Kashefi, J., Koehler, P., and Papanastassopoulou, M. (2013). Detection and early warning of West Nile Virus circulation in Central Macedonia, Greece, using sentinel chickens and mosquitoes. Vector Borne Zoonotic Dis. 13, 723–732. doi: 10.1089/vbz.2012.1176
Colon-Gonzalez, F. J., Sewe, M. O., Tompkins, A. M., Sjodin, H., Casallas, A., Rocklov, J., et al. (2021). Projecting the risk of mosquito-borne diseases in a warmer and more populated world: A multi-model, multi-scenario intercomparison modelling study. Lancet Planet Health 5, 404–414. doi: 10.1016/S2542-5196(21)00132-7
Cooperband, M. F., and Cardé, R. T. (2006). Orientation of Culex mosquitoes to carbon dioxide-baited traps: Flight manoeuvres and trapping efficiency. Med. Vet. Entomol. 20, 11–26. doi: 10.1111/j.1365-2915.2006.00613.x
Cooperband, M. F., McElfresh, J. S., Millar, J. G., and Carde, R. T. (2008). Attraction of female Culex quinquefasciatus say (Diptera: Culicidae) to odors from chicken feces. J. Insect Physiol. 54, 1184–1192. doi: 10.1016/j.jinsphys.2008.05.003
Darsie, R. F. Jr., and Morris, C. D. (2003). Keys to the adult females and fourth instar larvae of the mosquitoes of Florida (Diptera: Culicidae), Volume 1 (revised). Tallahassee, FL: Florida Mosquito Control Association.
Darsie, R. F., and Ward, R. A. (2005). Identification and geographical distribution of the mosquitoes of North America, North of Mexico. Gainesville, FL: University Press of Florida.
Edman, J. D. (1971). Host-feeding patterns of Florida mosquitoes I. Aedes, Anopheles, coquillettidia, Mansonia and Psorophora. J. Med. Entomol. 8, 687–695.
Edman, J. D. (1974). Host-feeding patterns of Florida mosquitoes: III. Culex (Culex) and Culex (Neoculex). J. Med. Entomol. 11, 95–104.
Edman, J. D., and Scott, T. W. (1987). Host defensive behaviour and the feeding success of mosquitoes. Int. J. Trop. Insect Sci. 8, 617–622.
Edman, J. D., and Taylor, D. J. (1968). Culex nigripalpus: Seasonal shift in the bird-mammal feeding ratio in a mosquito vector of human encephalitis. Science 161, 67–68. doi: 10.1126/science.161.3836.67
Edman, J. D., Webber, L. A., and Kale, H. W. (1972). Host-feeding patterns of Florida mosquitoes II. Culiseta. J. Med. Entomol. 9, 429–434. doi: 10.1093/jmedent/9.5.429
Elizondo-Quiroga, A., Flores-Suarez, A., Elizondo-Quiroga, D., Ponce-Garcia, G., Blitvich, B. J., Contreras-Cordero, J. F., et al. (2006). Host-feeding preference of Culex quinquefasciatus in Monterrey, northeastern Mexico. J. Am. Mosq. Control Assoc. 22, 654–661. doi: 10.2987/8756-971X(2006)22[654:HPOCQI]2.0.CO;2
Farajollahi, A., Fonseca, D. M., Kramer, L. D., and Kilpatrick, A. M. (2011). “Bird biting” mosquitoes and human disease: A review of the role of Culex pipiens complex mosquitoes in epidemiology. Infect. Genet. Evol. 11, 1577–1585. doi: 10.1016/j.meegid.2011.08.013
Gillies, M. T. (1980). The role of carbon dioxide in host-finding by mosquitoes (Diptera: Culicidae): A review. Bull. Entomol. Res. 70, 525–532.
Gomes, B., Sousa, C. A., Vicente, J. L., Pinho, L., Calderón, I., Arez, E., et al. (2013). Feeding patterns of molestus and pipiens forms of Culex pipiens (Diptera: Culicidae) in a region of high hybridization. Parasit. Vectors. 6, 93–102. doi: 10.1186/1756-3305-6-93
Greenberg, J. A., Lujan, D. A., DiMenna, M. A., Wearing, H. J., and Hofkin, B. V. (2013). Identification of blood meal sources in Aedes vexans and Culex quinquefasciatus in Bernalillo County. New Mexico. J. Insect Sci. 13:75. doi: 10.1673/031.013.7501
Gu, Z. Y., Gao, H. T., Yang, Q. J., Ni, M., Li, M. J., Xing, D., et al. (2022). Screening of olfactory genes related to blood-feeding behaviors in Culex pipiens quinquefasciatus and Culex pipiens molestus by transcriptome analysis. PLoS Negl. Trop. Dis. 16:e0010204. doi: 10.1371/journal.pntd.0010204
Ignell, R., and Hill, S. R. (2020). Malaria mosquito chemical ecology. Curr. Opin. Insect Sc. 40, 6–10.
Kang, D. S., Kim, S., Cotten, M. A., and Sim, C. (2021). Transcript assembly and quantification by RNA-Seq reveals significant differences in gene expression and genetic variants in mosquitoes of the Culex pipiens (Diptera: Culicidae) complex. J. Med. Entomol. 58, 139–145. doi: 10.1093/jme/tjaa167
Karlsson, M. F., Birgersson, G., Witzgall, P., Lekfeld, J. D., Punyasiri, N. P., and Bengtsson, M. (2013). Guatemalan potato moth Tecia solanivora distinguish odour profiles from qualitatively different potatoes Solanum tuberosum L. Phytochemistry 85, 72–81. doi: 10.1016/j.phytochem.2012.09.015
Kenawy, M. A., Beier, J. C., Zimmerman, J. H., Said, S. E., and Abbassy, M. M. (1987). Host-feeding patterns of the mosquito community (Diptera: Culicidae) in Aswan Governorate, Egypt. J. Med. Entomol. 24, 35–39. doi: 10.1093/jmedent/24.1.35
Kurucz, K., Kepner, A., Krtinic, B., Hederics, D., Foldes, F., Brigetta, Z., et al. (2018). Blood-meal analysis and avian malaria screening of mosquitoes collected from human-inhabited areas in Hungary and Serbia. J. Eur. Mosq. Control Assoc. 36, 3–13.
Ligsay, A., Telle, O., and Paul, R. (2021). Challenges to mitigating the urban health burden of mosquito-borne diseases in the face of climate change. Int. J. Environ. Res. Public Health 18, 5035–5046. doi: 10.3390/ijerph18095035
Lillepold, K., Rocklöv, J., Liu-Helmersson, J., Sewe, M., and Semenza, J. C. (2019). More arboviral disease outbreaks in continental Europe due to the warming climate? J. Travel Med. 26:taz017. doi: 10.1093/jtm/taz017
Lyimo, I. N., and Ferguson, H. M. (2009). Ecological and evolutionary determinants of host species choice in mosquito vectors. Trends Parasitol. 25, 189–196. doi: 10.1016/j.pt.2009.01.005
Mackay, A. J., Kramer, W. L., Meece, J. K., Brumfield, R. T., and Foil, L. D. (2014). Host feeding patterns of Culex mosquitoes (Diptera: Culicidae) in East Baton Rouge Parish, Louisiana. J. Med. Entomol. 47, 238–248. doi: 10.1603/me09168
Mafra-Neto, A., and Dekker, T. (2019). Novel odor-based strategies for integrated management of vectors of disease. Curr. Opin. Insect Sci. 34, 105–111. doi: 10.1016/j.cois.2019.05.007
Majeed, S., Hill, S. R., and Ignell, R. (2014). Impact of elevated CO2 background levels on the host-seeking behaviour of Aedes aegypti. J. Exp. Biol. 217, 598–604.
Majeed, S., Hill, S. R., Dekker, T., and Ignell, R. (2017). Detection and perception of generic host volatiles by mosquitoes: Responses to CO2 constrains host-seeking behaviour. R. Soc. Open Sci. 4:170189. doi: 10.1098/rsos.170189
McBride, C. S., Baier, F., Omondi, A. B., Spitzer, S. A., Lutomiah, J., Sang, R., et al. (2014). Evolution of mosquito preference for humans linked to an odorant receptor. Nature 515, 222–227. doi: 10.1038/nature13964
McMeniman, C. J., Corfas, R. A., Matthews, B. J., Ritchie, S. A., and Vosshall, L. B. (2014). Multimodal integration of carbon dioxide and other sensory cues drives mosquito attraction to humans. Cell 156, 1060–1071. doi: 10.1016/j.cell.2013.12.044
Medlock, J. M., and Vaux, A. G. (2011). Assessing the possible implications of wetland expansion and management on mosquitoes in Britain. Eur. Mosquito Bull. 29, 38–65.
Mendenhall, I. H., Bahl, J., Blum, M. J., and Wesson, D. M. (2012). Genetic structure of Culex erraticus populations across the Americas. J. Med. Entomol. 49, 522–534.
Molaei, G., Andreadis, T. G., Armstrong, P. M., Anderson, J. F., and Vossbrinck, C. R. (2006). Host feeding patterns of Culex mosquitoes and West Nile virus transmission, northeastern United States. Emerg. Infect. Dis. 12, 468–474. doi: 10.3201/eid1203.051004
Mozûraitis, R., Hajkazemian, M., Zawada, J. W., Szymczak, J., Pålsson, K., Sekar, V., et al. (2020). Male swarming aggregation pheromones increase female attraction and mating success among multiple African malaria vector mosquito species. Nat. Ecol. Evol. 4, 1395–1401. doi: 10.1038/s41559-020-1264-9
Murphey, F. J., Burbutis, P. P., and Bray, D. F. (1967). Bionomics of Culex salinarius Coquillett II. Host acceptance and feeding by adult females of C. salinarius and other mosquito species. Mosq. News 27, 366–374.
Nyasembe, V. O., and Torto, B. (2014). Volatile phytochemicals as mosquito semiochemicals. Phytochem. Lett. 8, 196–201.
Omondi, A. B., Ghaninia, M., Dawit, M., Svensson, T., and Ignell, R. (2019). Age-dependent regulation of host seeking in Anopheles coluzzii. Sci. Rep. 9:9699. doi: 10.1038/s41598-019-46220-w
Ree, H. I., Yong, T. S., and Hwang, U. W. (2005). Identification of four species of the Anopheles hyrcanus complex (Diptera: Culicidae) found in Korea using species-specific primers for Polymerase Chain Reaction Assay. Med. Entomol. Zool. 56, 201–205.
Reeves, L. E., Holderman, C. J., Blosser, E. M., Gillett-Kaufman, J. L., Kawahara, A. Y., Kaufman, P. E., et al. (2018). Identification of Uranotaenia sapphirina as a specialist of annelids broadens known mosquito host use patterns. Commun. Biol. 1:92. doi: 10.1038/s42003-018-0096-5
Reisen, W. K., Martinez, V. M., Fang, Y., Garcia, S., Ashtari, S., Wheeler, S. S., et al. (2006). Role of California (Callipepla californica) and Gambel’s (Callipepla gambelii) quail in the ecology of mosquito-borne encephalitis viruses in California, USA. Vector Borne Zoonotic Dis. 6, 248–260. doi: 10.1089/vbz.2006.6.248
Riveron, J. M., Tchouakui, M., Mugenzi, L., Menze, B. D., Chiang, M. C., and Wondji, C. S. (2018). “Insecticide resistance in malaria vectors: An update at a global scale,” in Towards malaria elimination-a leap forward, eds S. Manguin and V. Dev (London: IntechOpen), 149–173.
Rizzoli, A., Bolzoni, L., Chadwick, E. A., Capelli, G., Montarsi, F., Grisenti, M., et al. (2015). Understanding West Nile virus ecology in Europe: Culex pipiens host feeding preference in a hotspot of virus emergence. Parasit. Vectors 8:213. doi: 10.1186/s13071-015-0831-4
Rocklov, J., and Dubrow, R. (2020). Climate change: An enduring challenge for vector-borne disease prevention and control. Nat. Immunol. 21, 479–483.
Roiz, D., Vazquez, A., Rosà, R., Muñoz, J., Arnoldi, D., Rosso, F., et al. (2012). Blood meal analysis, flavivirus screening, and influence of meteorological variables on the dynamics of potential mosquito vectors of West Nile virus in northern Italy. J. Vector Ecol. 37, 20–28. doi: 10.1111/j.1948-7134.2012.00196.x
Shahhosseini, N., Friedrich, J., Moosa-Kazemi, S. H., Sedaghat, M. M., Kayedi, M. H., Tannich, E., et al. (2018). Host-feeding patterns of Culex mosquitoes in Iran. Parasit. Vectors 11:669.
Smith, B. P., Zini, C. A., Pawliszyn, J., Tyler, M. J., Hayasaka, Y., Williams, B., et al. (2000). Solid-phase microextraction as a tool for studying volatile compounds in frog skin. Chem. Ecol. 17, 215–225.
Spanoudis, C. G., Andreadis, S. S., Bray, D. P., Savopoulou-Soultani, M., and Ignell, R. (2020). Behavioural response of the house mosquitoes Culex quinquefasciatus and Culex pipiens molestus to avian odours and its reliance on carbon dioxide. Med. Vet. Entomol. 34, 129–137. doi: 10.1111/mve.12429
Syed, Z., and Leal, W. S. (2009). Acute olfactory response of Culex mosquitoes to a human-and bird-derived attractant. Proc. Natl. Acad. Sci. U.S.A. 106, 18803–18808. doi: 10.1073/pnas.0906932106
Takken, W., and Knols, B. G. (1999). Odor-mediated behavior of Afrotropical malaria mosquitoes. Annu. Rev. Entomol. 44, 131–157. doi: 10.1146/annurev.ento.44.1.131
Takken, W., and Verhulst, N. O. (2013). Host preferences of blood-feeding mosquitoes. Annu. Rev. Entomol. 58, 433–453.
Tauxe, G. M., MacWilliam, D., Boyle, S. M., Guda, T., and Ray, A. (2013). Targeting a dual detector of skin and CO2 to modify mosquito host seeking. Cell 155, 1365–1379. doi: 10.1016/j.cell.2013.11.013
Vinogradova, E. B. (2000). Culex pipiens mosquitoes: Taxonomy, distribution, ecology, physiology, genetics, applied importance and control. Sofia: Pensoft Publishers.
Weitzel, T., Collado, A., Jöst, A., Pietsch, K., Storch, V., and Becker, N. (2009). Genetic differentiation of populations within the Culex pipiens complex and phylogeny of related species. J. Am. Mosq. Control Assoc. 25, 6–17. doi: 10.2987/08-5699.1
Wondwosen, B., Birgersson, G., Seyoum, E., Tekie, H., Torto, B., Fillinger, U., et al. (2016). Rice volatiles lure gravid malaria mosquitoes, Anopheles arabiensis. Sci. Rep. 6:37930. doi: 10.1038/srep37930
Yee, W. L., and Foster, W. A. (1992). Diel sugar-feeding and host-seeking rhythms in mosquitoes (Diptera: Culicidae) under laboratory conditions. J. Med. Entomol. 29, 784–791.
Yurttas, H., and Alten, B. (2006). Geographic differentiation of life table attributes among Anopheles sacharovi (Diptera: Culicidae) populations in Turkey. J. Vector Ecol. 31, 275–284. doi: 10.3376/1081-1710(2006)31[275:gdolta]2.0.co;2
Zeller, H. G., and Schuffenecker, I. (2004). West Nile virus: An overview of its spread in Europe and the Mediterranean basin in contrast to its spread in the Americas. Eur. J. Clin. Microbiol. Infect. Dis. 23, 147–156. doi: 10.1007/s10096-003-1085-1
Keywords: chemical ecology, Culicidae, host seeking, electrophysiology, chemical analysis, kairomone
Citation: Spanoudis CG, Wondwosen B, Isberg E, Andreadis SS, Kline DL, Birgersson G and Ignell R (2022) The chemical code for attracting Culex mosquitoes. Front. Ecol. Evol. 10:930665. doi: 10.3389/fevo.2022.930665
Received: 28 April 2022; Accepted: 11 July 2022;
Published: 11 August 2022.
Edited by:
Cesar Gemeno, Universitat de Lleida, SpainReviewed by:
Madelien Wooding, University of Pretoria, South AfricaCopyright © 2022 Spanoudis, Wondwosen, Isberg, Andreadis, Kline, Birgersson and Ignell. This is an open-access article distributed under the terms of the Creative Commons Attribution License (CC BY). The use, distribution or reproduction in other forums is permitted, provided the original author(s) and the copyright owner(s) are credited and that the original publication in this journal is cited, in accordance with accepted academic practice. No use, distribution or reproduction is permitted which does not comply with these terms.
*Correspondence: Rickard Ignell, cmlja2FyZC5pZ25lbGxAc2x1LnNl
Disclaimer: All claims expressed in this article are solely those of the authors and do not necessarily represent those of their affiliated organizations, or those of the publisher, the editors and the reviewers. Any product that may be evaluated in this article or claim that may be made by its manufacturer is not guaranteed or endorsed by the publisher.
Research integrity at Frontiers
Learn more about the work of our research integrity team to safeguard the quality of each article we publish.