- 1Botanical Gardens, Osaka Metropolitan University, Osaka, Japan
- 2Faculty of Education, Kyoto University of Education, Kyoto, Japan
- 3Department of Chemical and Biological Sciences, Japan Women’s University, Tokyo, Japan
Late Silurian to early Devonian lycophytes had prostrate aerial axes, while subordinate organs or subterranean axes were formed around the dichotomies of the axes. The subterranean axes are hypothesized to have evolved into root-bearing axes (rhizophores) and roots in extant Selaginellaceae and Lycopodiaceae, respectively. Consistent with this hypothesis, rhizophores are formed on the dichotomies of shoots in Selaginellaceae. However, it has remained unclear whether roots are borne in the same position in Lycopodiaceae. In addition, roots form endogenously in the stem, but no data are available regarding the tissues in stem from which they arise. In this study, we tracked the root development in the clubmoss, Lycopodium clavatum, based on anatomical sections and 3D reconstructed images. The vascular tissue of the stem is encircled by ground meristem, which supplies cortical cells outwardly by periclinal divisions. A linear parenchymatous tissue is present on the ventral side of vascular cylinder, which we call “ventral tissue” in this study. We found that root primordia are formed endogenously on the ventral side of stem, possibly from the ventral tissue. In addition, roots always initiate at positions close to dichotomies of stem. The root-initiating position supports the suggestion that Lycopodium roots share a body plan with the subterranean organs of the hypothesized ancestry.
Introduction
Plants have evolved various structures for terrestrial life since they first invaded the land during the Ordovician period (Wellman and Gray, 2000; Morris et al., 2018). A prominent example of such an invention that occurred in the Devonian period is the vascular plant root, which facilitates nutrient absorption from, and provides an anchor to, substrate (Kenrick and Strullu-Derrien, 2014; Stein et al., 2020). The origin of roots triggered a decline in atmospheric carbon dioxide through weathering of the Earth’s surface (Le Hir et al., 2011), which in turn would have forced plants to evolve further innovations (Beerling et al., 2001; Fujinami et al., 2020). Two major clades of extant vascular plants, lycophytes and euphyllophytes, acquired roots independently (Raven and Edwards, 2001; Friedman et al., 2004; Fujinami et al., 2017, 2020; Hetherington and Dolan, 2018, 2019) with lycophytes acquiring them earlier (Matsunaga and Tomescu, 2016).
Extant lycophytes include three families, i.e., Lycopodiaceae (clubmosses), Selaginellaceae (spikemosses), and Isoetaceae (quillworts), which have highly diverse body plans (Figure 1). The Lycopodiaceae consists of the urostachyan clade (Huperzioideae) characterized by isotomously dichotomizing erect shoots, and the rhopalostachyan clade (Lycopodioideae and Lycopodielloideae) with anisotomously dichotomizing prostrate shoots (Sporne, 1962; Gifford and Foster, 1989; Øllgaard, 2015). Roots are borne endogenously in the stem near the shoot apex in both clades (Figures 1A,B), whereas they elongate through the cortex toward the base of the parent shoot in the former clade (Stokey, 1907; Roberts and Herty, 1934). Selaginellaceae and Isoetaceae form a clade, which is characterized by ligule, heterospory, and endosporic gametophytes (Eames, 1977; Gifford and Foster, 1989; Christenhusz et al., 2017). Selaginellaceae has a unique organ, the rhizophore, which arises exogenously from the angle meristem in the dichotomy of the shoots (Figures 1C,D). The rhizophore grows downward and terminates to produce roots endogenously, but does not form any leaves laterally or a cap-like structure covering the apex (Imaichi and Kato, 1989; Banks, 2009). Therefore, it is distinct from shoots and roots despite its axial nature, which is also suggested by differences in gene expression profiles among these organs (Mello et al., 2019). Isoetaceae has a cormous body, which bears a tuft of quill-like leaves from the upper part and a tuft of rootlets from the lower part (Figures 1E,F; Foster and Gifford, 1959; Bierhorst, 1971; Gifford and Foster, 1989). Rootlets are formed endogenously in regularly arranged rows called orthostichies (Paolillo, 1963, 1982; Bierhorst, 1971; Yi and Kato, 2001).
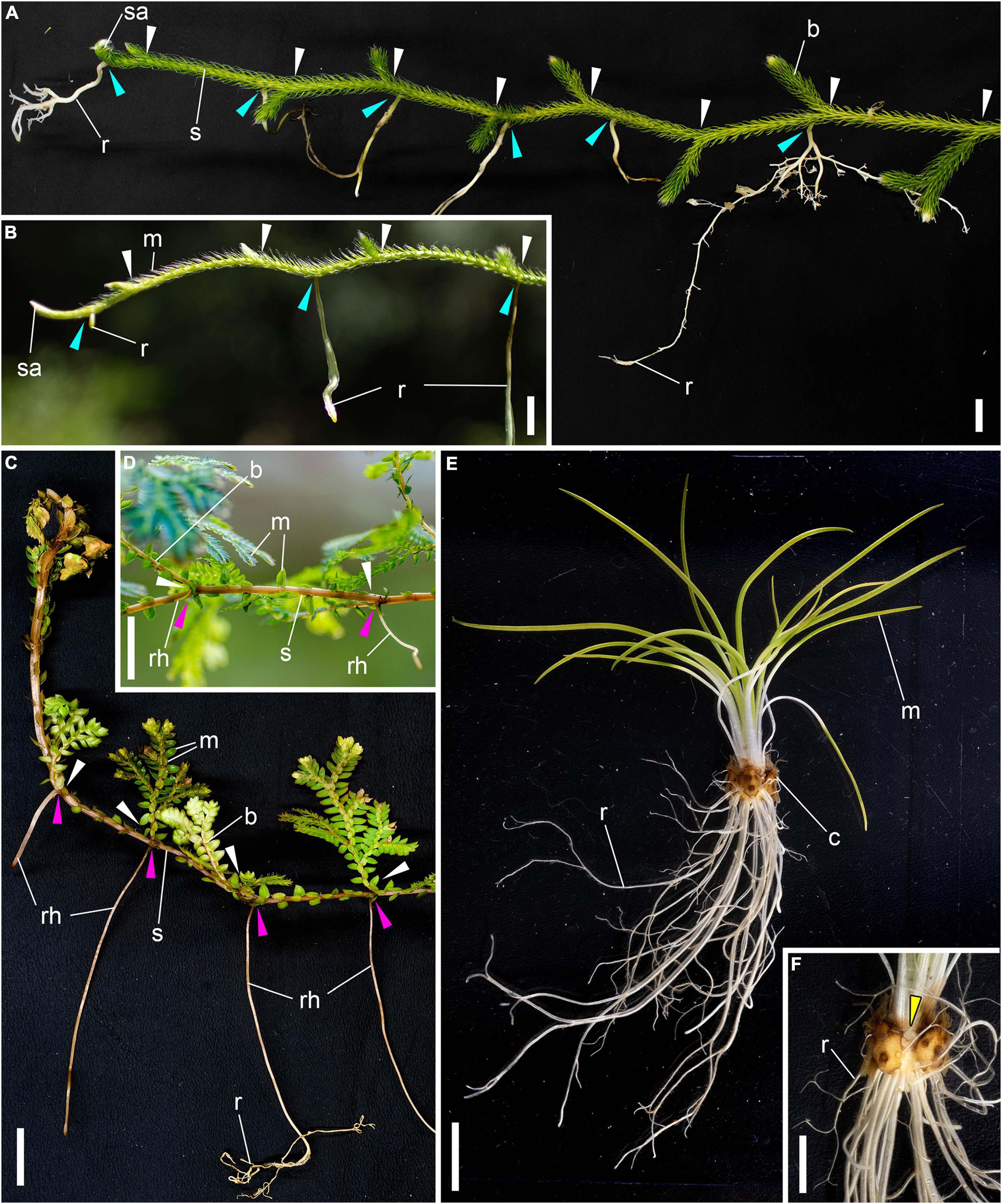
Figure 1. Body architectures of extant lycophytes. (A,B) Lycopodium clavatum (Lycopodiaceae). (A) Dorsal view. (B) Lateral view. White arrows: dichotomies of shoots; blue arrows: emergence positions of roots endogenously from parent shoot. (C,D) Selaginella uncinata (Desv. ex Poir.) Spring (Selaginellaceae). Lateral views. White arrows: dichotomies of shoots; pink arrows: exogenous branching points of rhizophores. (E,F) Isoetes pseudojaponica M.Takamiya, Mitsu.Watan. et K.Ono (Isoetaceae). (E) Lateral view. (F) The opposite side of (E). Yellow arrow: furrow of corm. b, lateral branch (shoot); c, corm; m: microphyll; r, root (rootlets in Isoetes); rh, rhizophore; s, shoot (main axis); sa, shoot apex. Scale bars, 10 mm in (A–E), 5 mm in (F).
The extant lycophyte families are sister groups to the fossil order Drepanophycales (Hueber, 1992; Kenrick and Crane, 1997) or Drepanophycales plus zosterophylls (Gensel, 1992; Kenrick and Crane, 1997), which flourished during the late Silurian to early Devonian periods. Drepanophycaleans have aerial shoot systems with dichotomies that resemble those of extant Lycopodiaceae (Kidston and Lang, 1920, 1921; Andrews, 1961; Kerp et al., 2013), although the “leaves” lack a distinct veinlet in many drepanophycaleans (Hueber, 1992; Kenrick and Crane, 1997). Meanwhile, they have subterranean axial organs (root-bearing axes sensu Matsunaga and Tomescu (2016, 2017) and Hetherington et al. (2021)), which branch exogenously from the aerial shoot axis. Rooting axes without a cap-like structure are born anisotomously from the subterranean axes (Hetherington and Dolan, 2018; Hetherington et al., 2021). In some drepanophycaleans, an aerial shoot axis gives rise to two daughter shoots by anisotomous dichotomy and one daughter shoot further branches anisotomously to form the subterranean axis and a shoot (Gensel et al., 2001; Matsunaga et al., 2017). As the second anisotomy occurs before elongation of the daughter shoot, the subterranean axis is placed quite close to the branching point of shoot axes. In addition, zosterophylls form subaxillary tubercles or subordinate organs around the dichotomy of prostrate aerial axes, which can be compared to the subterranean axis of Drepanophycales (Høeg, 1942; Banks and Davis, 1969; Edwards, 1970, 1994; Edwards and Kenrick, 1986; Hass and Remy, 1986; Kenrick and Edwards, 1988; Edwards et al., 1989; Kenrick and Crane, 1997; Matsunaga et al., 2017). These fossils suggest that lycophytes share a body plan in which each dichotomy of the aerial axes has the ability to form a downwardly growing organ (Matsunaga and Tomescu, 2015).
It is suggested that the subterranean axis would have evolved into the rhizophore or similar organs from which roots are borne (Matsunaga and Tomescu, 2016, 2017; Hetherington et al., 2021). As this hypothesis implies, the Selaginella rhizophore is formed on the dichotomy of shoot axes (Jernstedt et al., 1992; Banks, 2009; Matsunaga et al., 2017). On the other hand, the subterranean axis is hypothesized to be an ancestral organ of the root in some lycophytes (Gensel and Berry, 2001; Gensel et al., 2001). As a pair of daughter shoots and the subterranean axis are formed by branching of a parent shoot (see above), this hypothesis suggests that shoot and root should share some developmental traits in these lineages (Gensel et al., 2001). Roots of Lycopodiaceae would represent the products of such evolution, because root and shoot apical meristems likely have the same mechanisms for branching and maintenance of apical growth (Fujinami et al., 2021). Therefore, it is expected that the dichotomy of stems is the site of root formation in Lycopodiaceae, although a developmental study on mature plants of Diphasiastrum complanatum (L.) Holub noted that roots seem to emerge from the stem regardless of the dichotomies (Roberts and Herty, 1934). However, stems elongate considerably before roots are projected from the parent stem (Roberts and Herty, 1934; Freeberg and Wetmore, 1967), which makes it difficult to infer a position of the origin based on the mature root. Therefore, reexaminations are necessary to determine where root primordia are formed in the stems of Lycopodiaceae.
In this study, we conducted histological observations on shoot apices of Lycopodium clavatum L. (Lycopodiaceae) in which root primordia should be formed (Roberts and Herty, 1934; Gifford and Foster, 1989). First, we focused on the tissue in which root primordia develop in the stem to reevaluate the long-standing view that root primordia initiate in the ventral part of the pericycle in this family (Roberts and Herty, 1934). Second, we examined if roots initiate in the proximity of dichotomies of stems. To this end, we measured the distances between root primordia and dichotomies based on serial sections of shoot tips, as well as reconstructed histological 3D images of shoot apex. Based on these observations, we confirm that the dichotomies of stems have the ability to form subterranean organs in extant Lycopodiaceae.
Materials and methods
Sample collection and observation
Shoots of Lycopodium clavatum were collected in following localities in Japan: Umegahata, Kyoto and Kawakami, Nara. Voucher specimens were stored in the Vascular Plants Collection of the National Museum of Nature and Science (TNS). Collected shoots with leaves removed were cut into small pieces and fixed in FAA (37% formaldehyde: glacial acetic acid: 50% ethanol = 1: 1: 18). The shoot tips were embedded in Technovit 7100 (Heraeus Kulzur, Wehrheim, Germany) or Paraplast plus (Sigma-Aldrich, St Louis, MO, USA) after dehydration in a graded ethanol series. The embedded samples were cut into sections 3–10 μm thick on a rotary microtome. The plastic sections were stained with safranin, toluidine blue O, and orange G (Jernstedt et al., 1992). The paraffin sections were stained by Mayer’s Hematoxylin and safranin. Then they were observed using an Olympus BX53 microscope (Olympus Corporation, Tokyo, Japan). Photographs were taken with an Olympus DP74 digital camera under the control of the Olympus cellSens Standard software (Olympus Corporation). The most sections were cut transversely to the main stem in this study (Figures 2–5). Their relative positions in a longitudinal section are shown in Supplementary Figure 1A.
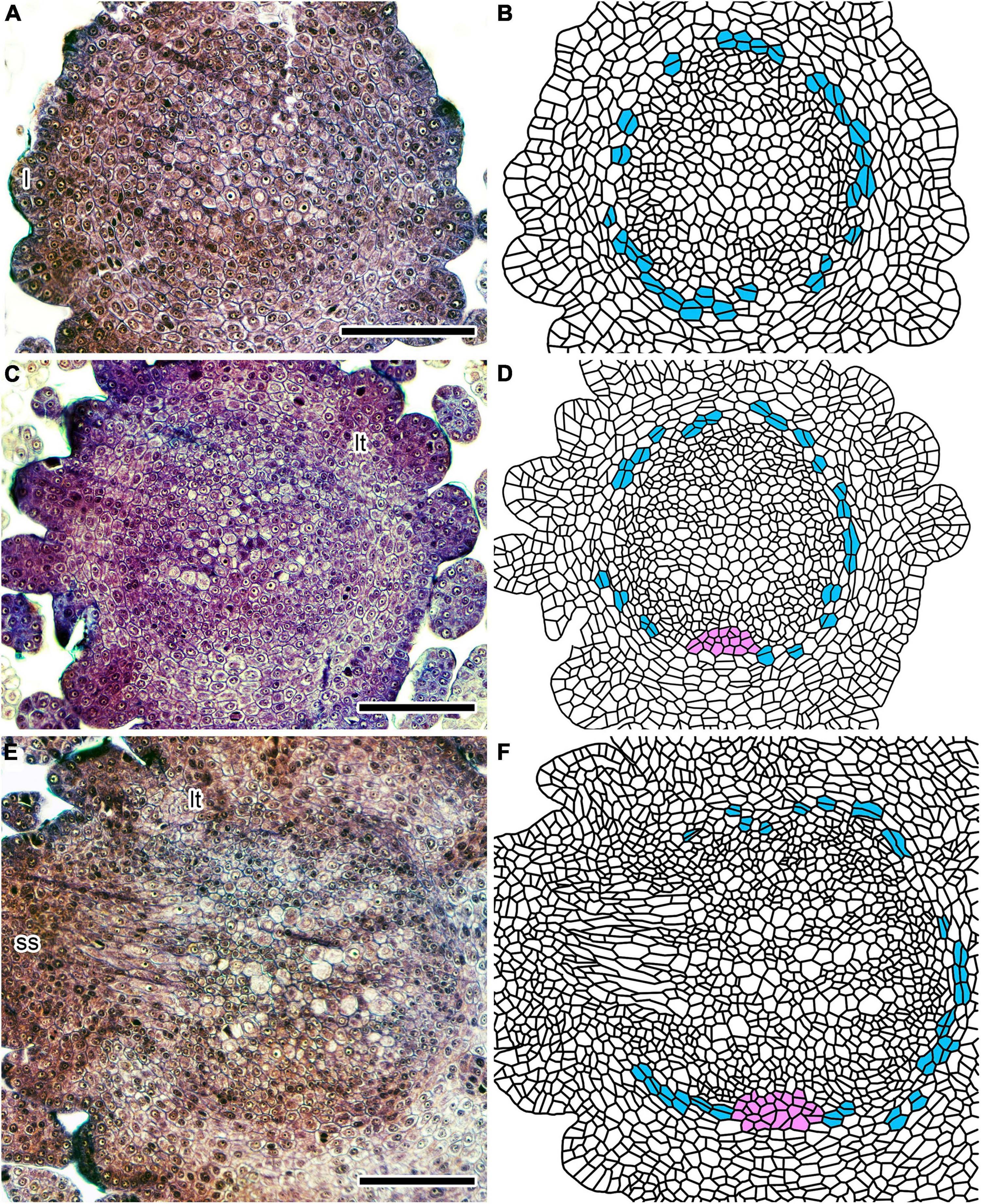
Figure 2. Tissue differentiation processes in an immature Lycopodium clavatum shoot (sample #P1) within 1 mm from the shoot apex. (A,C,E) Cross-sections at 175, 235, and 360 μm from the shoot apex, respectively. (B,D,F) Line drawings of sections on the left. Cells encircling vascular tissue (blue) divide periclinally to supply cortical cells. A mass of parenchymatous cells (ventral tissue) is colored in pink. Scale bars, 100 μm. ss, small shoot; l, leaf primordium; lt, leaf trace.
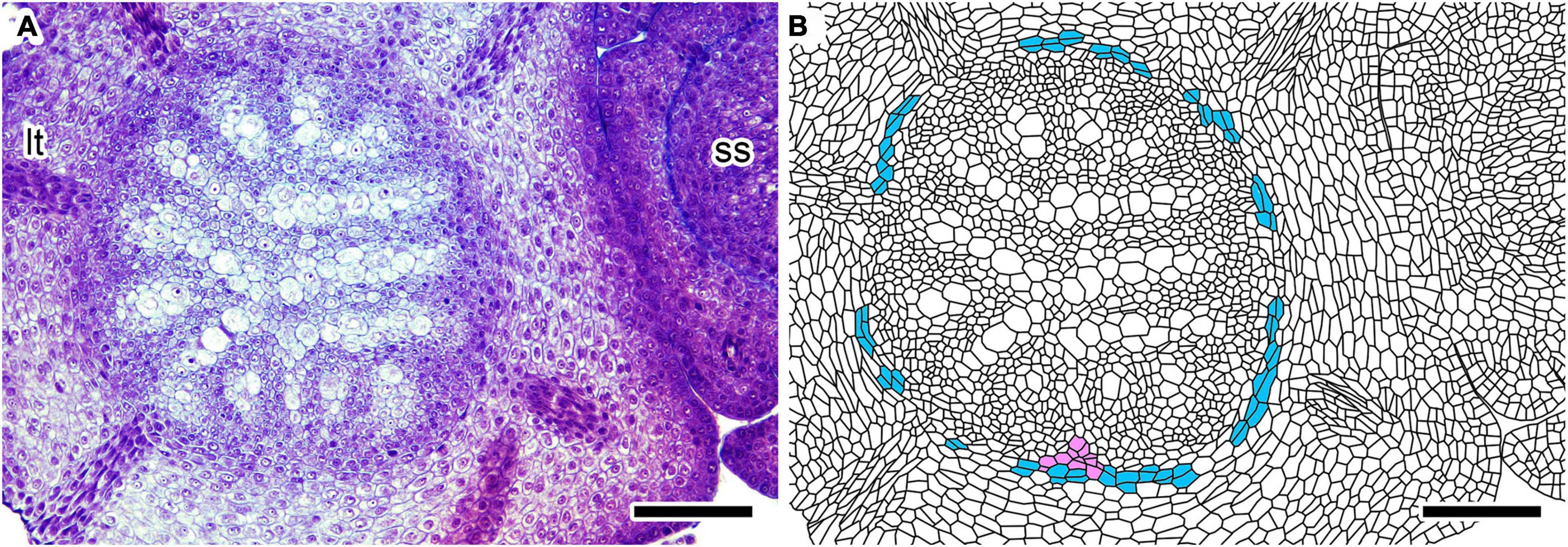
Figure 3. Cross-section of an immature Lycopodium clavatum shoot (sample #P1) at 665 μm from the shoot apex. (A) Original section. (B) Line drawing of (A). See color legend for Figure 2. Scale bars, 100 μm. ss, small shoot; lt, leaf trace.
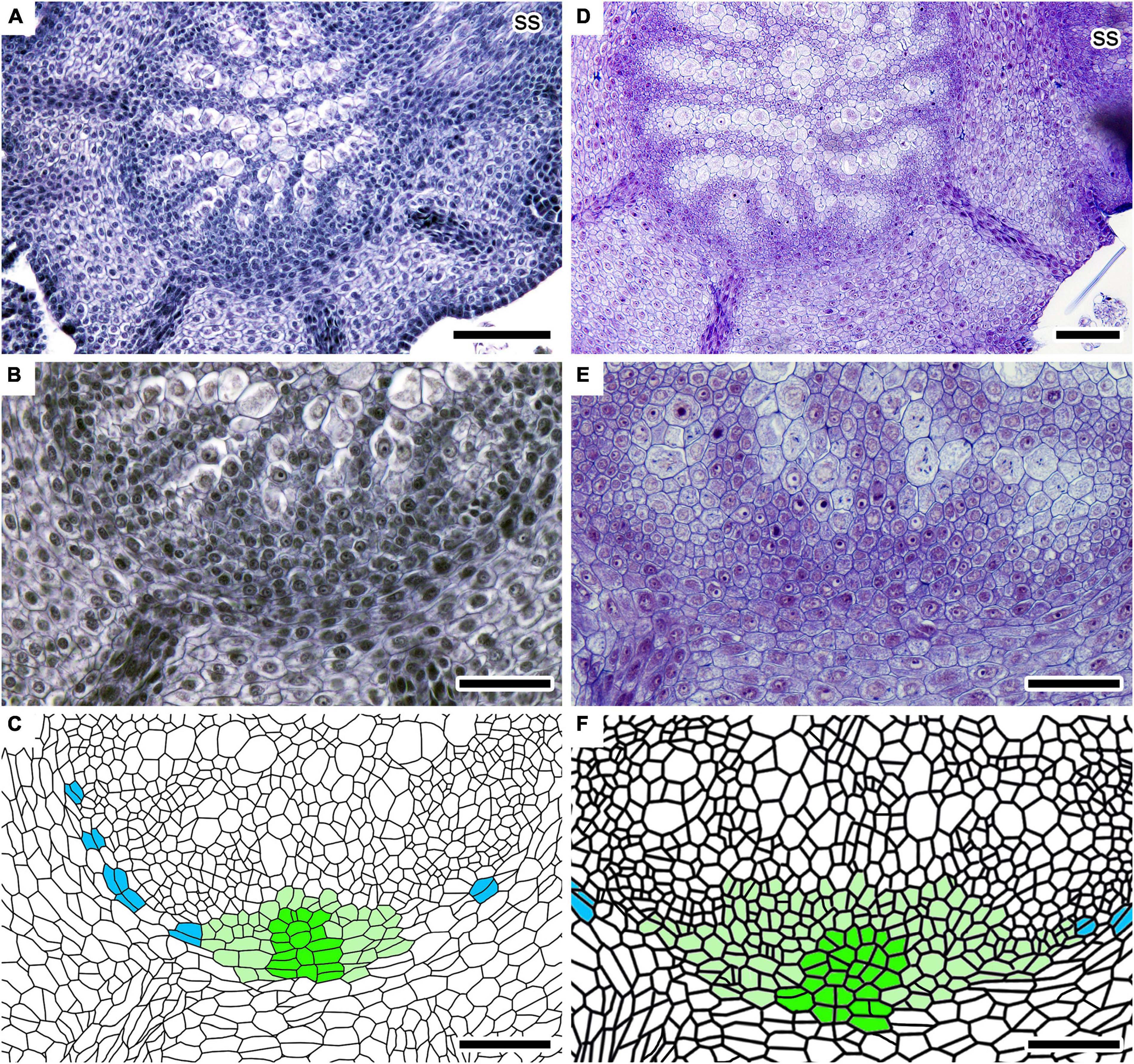
Figure 4. Root initiation processes in two independent shoots of Lycopodium clavatum. (A–C) In sample #2–35. Cross-sections at 630 μm from the shoot apex. (B) Close-up of (A) showing root primordium. (C) Line drawing of (B). A row of initial cells and cell files radiating from it are colored in dark green, while other cells of root primordium in light green. Periclinally divided cells in ground meristem are colored in blue. (D–F) In sample #P3. Cross-sections at 1,268 μm from the shoot apex. (E) Close-up of (D) showing root primordium. (F) Line drawing of (E). See (C) for color legend. Scale bars, 100 μm in (A,D); 50 μm in (B,C,E,F). ss, small shoot.
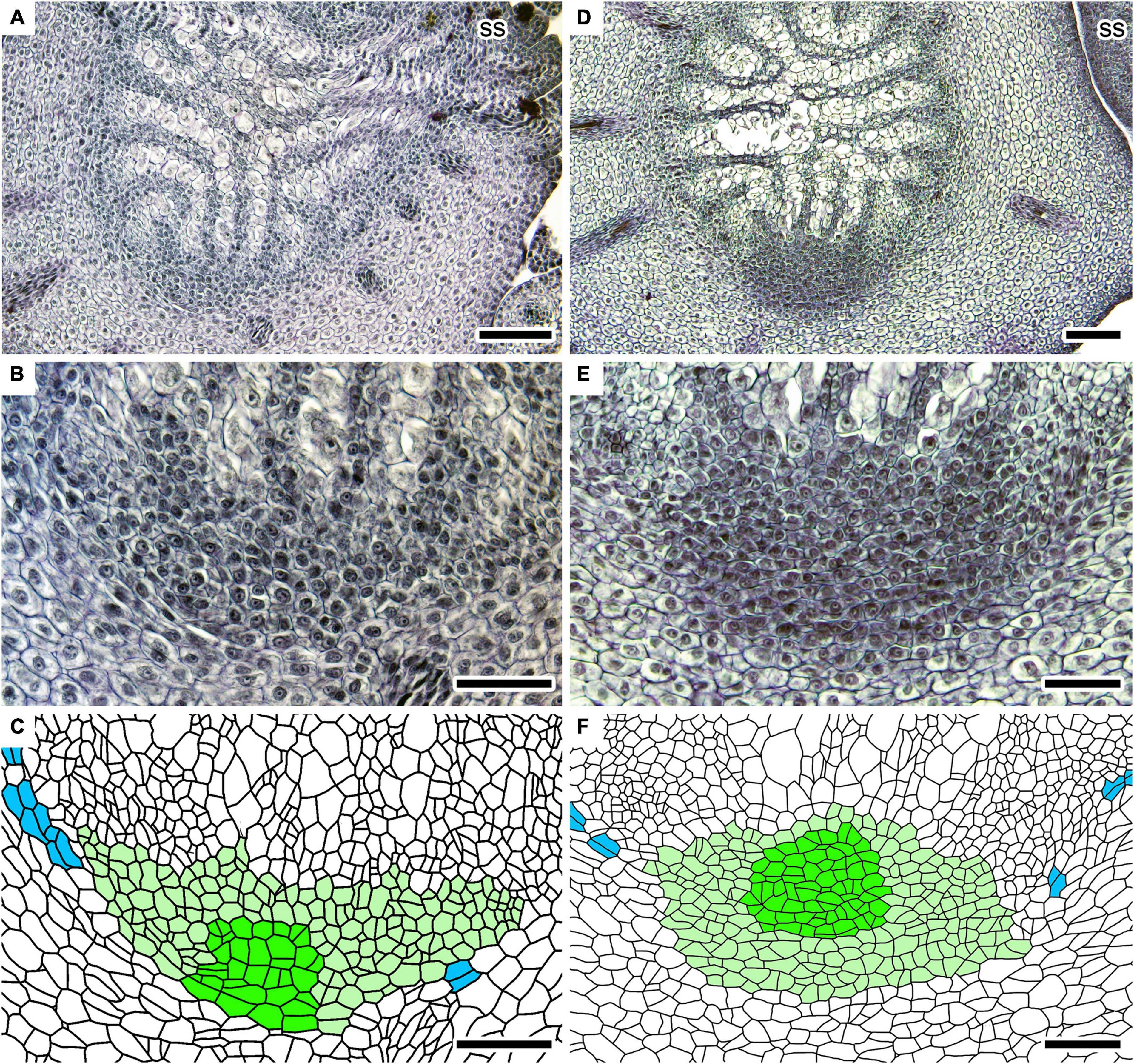
Figure 5. Root developing processes in two independent shoots of Lycopodium clavatum. (A–C) In sample #2–11. Cross-sections at 1,040 μm from the shoot apex. (B) Close-up of (A) showing root primordium. (C) Line drawing of (B). (D–F) In sample #2–33. Cross-sections at 1,450 μm from the shoot apex. (E) Close-up of (D) showing root primordium. (F) Line drawing of (E). Scale bars, 100 μm in (A,D); 50 μm in (B,C,E,F). ss: small shoot. See Figure 4 for color legend in line drawings.
We collected one shoot from each individual. A total of 13 shoots were observed by serial plastic section, and 6 of the 13 shoots were used for 3D reconstruction (see below). In addition, 44 shoots embedded in paraffin were observed, and 37 root primordia were found in 31 shoots (Supplementary Tables 1, 2; see below). No roots were found around the first nor second branching points in remaining 13 shoots.
3D reconstruction
Photographs of serial sections were layered in Adobe Photoshop 2021 (Adobe Inc., San Jose, CA, USA) and rotated so that the tracheary elements of the plectostele overlapped. Each layer was rasterized and background other than plant material was erased using the quick selection tool. Then the background area within the canvas was filled with white (RGB 255:255:255) to ensure that ImageJ (Schneider et al., 2012) could adequately recognize the canvas size. Highly stained areas expanding around the center of a young stem were semi-automatically filled with light green (RGB 83:244:131) using the Paint Bucket tool (Mode: Normal; Opacity: 100%; Tolerance: 10–45; Contiguous). Each edited layer was saved as an uncompressed image in PNG format.
The images were imported into ImageJ Fiji (Schindelin et al., 2012) as an image sequence. Color Deconvolution 2 (Landini et al., 2020) was used to produce a series of images in which the light green area was extracted. The image sequence was binarized with a dark background (RGB 0:0:0), while internal structures of the stem (RGB 255:255:255) were painted with tools implemented in Adobe Photoshop, i.e., the roots in light blue (RGB 0:157:255), the vascular cylinder of lateral shoots in magenta (RGB 255:0:102), and leaf traces in dark green (RGB 2:118:10). The ventral tissue (see section “Results” for details) was also painted in three shoots in which the most sections were available for the apical part. However, the tissue could not be painted on the images with light green area extracted because part of it was not extracted correctly. Therefore, we painted it in cyan (RGB 0:255:242) in the original (i.e., unprocessed) photographs and composited the colored part onto the processed images of the image sequence. The artificially colored image sequence was opened in ImageJ to reconstruct a 3D image with 3D Viewer (Schmid et al., 2010) after complementing missing or spoiled sections by blank images with a dark background. In the 3D reconstruction, the units of pixel height and pixel width were converted into micrometers and the voxel depth was specified by the thickness of each section.
Measurements of root–dichotomy distances and inter-dichotomy lengths
Distances to the nearest dichotomy were measured for root primordia which were found around the youngest and second youngest dichotomies because root initiating points could be displaced as stem grows. The distance hereafter called “root–dichotomy distance.” For the same reason, we did not include root primordia for the analyses which were found in the inter-dichotomies with ≥2 mm in length. Based on these criteria, we used 25 root primordia in 24 shoots for the analyses among 37 root primordia in 31 shoots (Supplementary Tables 1, 2). Among the 25 root primordia, 5 are close to the youngest dichotomy, while 20 to the second youngest one (Supplementary Table 1). However, the 25 root primordia were analyzed altogether because the number of primordia observed was too small for the youngest dichotomy.
All root primordia were located between the nearest dichotomy and the midpoint of the inter-dichotomy on which the primordia are located. Therefore, we evaluated whether a root primordium is close to the midpoint or the dichotomy by comparing a root–dichotomy distance with a quarter length of the inter-dichotomy. Differences between them were statistically tested by t-test. To facilitate comparison among root primordia of different shoots, we also calculated relative root–dichotomy distances in which inter-dichotomy lengths were set to 100.
The exact branching point of shoots is defined here as the intersection between each center line of diverging vascular cylinders. We considered the intersection point of the two vascular cylinders to be the median section in the series of sections that encompassed the connection between the two vascular cylinders. Similarly, we considered the branching point of a root to be represented by the median section of the series of sections encompassing the extent of a root primordium. Then the distances between the two points were calculated based on the number of sections between them (Figure 6A). Lengths of inter-dichotomies were also calculated based on the estimated branching points of shoots. For the first inter-dichotomies, lengths were measured from the apex of the large shoot to the first dichotomy.
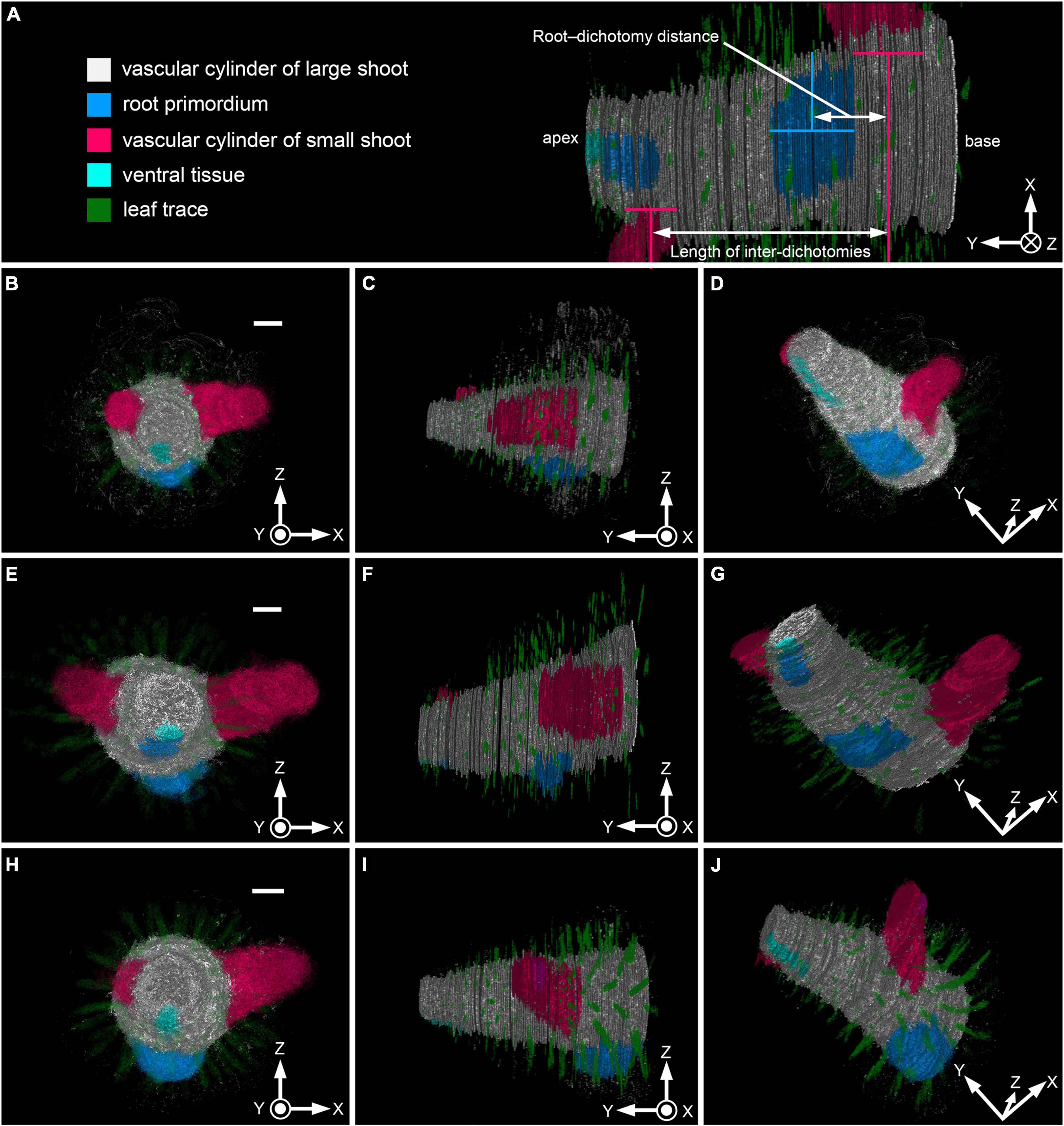
Figure 6. 3D images of vascular cylinders and root primordium in Lycopodium clavatum shoots. Sample numbers correspond to those in Supplementary Tables 1, 2. (A) Color legends and diagrammatic representation of the measurements. 3D image of sample #P3 viewed from below. (B,E,H) Front view. (C,F,I) Side view. (D,G,J) Oblique downward view. (B–D) 3D images of shoots shown in Figures 2A–F (sample # P1). A total of 222 images were used for the reconstruction, which included 31 blanks. Each section is 5 μm thick. (E–G) 3D images based on shoot shown in Figures 3C,D (sample # P3). A total of 313 images, including 120 blanks, were used. Each section is 5 μm thick. (H–J) 3D images based on sample # P5, consisting of 260 images, including 44 blanks. Each section is 4 μm thick. Scale bars, 200 μm in (B,E,H). Coordinate axes (X, Y, and Z) are indicated on the bottom-right corner of each panel. Concentric circle: axis perpendicularly out of the panel plane; circle with cross-mark: axis perpendicularly into the panel plane.
Results
Anatomical observations on root development in stem tissue
The incipient vascular cylinder starts to differentiate in the center of the stem 150–200 μm from the apex (Figures 2A,B; see also Supplementary Figure 1A, which shows relative positions of cross-sections in Figure 2 along the longitudinal axis). Although tissues are not distinctly differentiated in the immature stele, less-stained and vacuolated cells denote positions of metaxylem strands (Figures 2A,B). The characteristic pattern of the plectostele with alternating primary xylem and phloem becomes evident in the immature vascular tissue 200–300 μm from the apex (Figures 2C,D). The cross-sectioned vascular cylinder has a circular outline, except for the ventral side where a parenchymatous tissue interrupts the circle of the outline for 90–100 μm of width (Figures 2C,D); hereafter, we call this “ventral tissue.” The ventral tissue consists of isodiametric cells, but less stained than those of the vascular tissue. These cellular characters also distinguish the ventral tissue from the cortex, although both tissues are similar to each other in the parenchymatous nature. In cross-sections further below, the diameter of the vascular cylinder increases and vascular tissue differentiates progressively. However, the width of the ventral tissue remains almost unchanged (Figures 2E,F at 430 and Figures 3A,B at 665 μm from the apex, respectively).
The vascular cylinder is encircled by a layer of parenchymatous cells which supply cortical cells outwardly by periclinal cell divisions (Figures 2, 3). These cells are rectangular in shape in contrast to the isodiametric shape of the vascular cells (Figures 2, 3). In addition, they are larger and less stained than the vascular cells juxtaposing to them. These cellular differences make a distinct boundary between the vascular tissue and the layer of periclinally dividing cells; therefore, we call the cell layer “ground meristem” following previous studies (Wetmore, 1943; Foster and Gifford, 1959). It was suggested in a previous study that vascular tissue is encircled by pericycle and endodermis in stems of Diphasiastrum complanatum (Roberts and Herty, 1934). Ground meristem in this study may correspond to endodermis of the previous study, although the study did not provide structural details of these tissues. However, even if this correlation would be the case, “ground meristem” is used here because cortex is still developing in young stems. We could not clearly discern layer(s) assignable to the pericycle between the ground meristem and the vascular tissue (Figures 2, 3).
Stem branches anisotomously to form large and small shoots. The vascular cylinder of the youngest small shoot is branched from that of large shoot in lateral or slightly dorsal positions (Figures 2E,F, 4A). A young root primordium is often found around the youngest or second youngest dichotomies of the stems (Supplementary Figures 1B,C; see next section for details). The root primordium is formed on the ventral side of the vascular cylinder of large shoot where the ventral tissue should be present (Figures 4, 5). Several xylem and phloem elements elongate downwardly and connect to the root primordium (Figures 4, 5). At the center of the youngest root primordium which we could recognize in this study, several cell files radiate from a row of possible initial cells (Figures 4B,C). The number of central rows, as well as that of radiating cell files, increases in slightly larger root primordium (Figures 4E,F). These numbers further increase as the root primordium grows (Figures 5B,C). As the root primordium enters the cortex, radiating cell files also become evident on the side facing the cortex (Figures 5E,F).
The root primordium extends obliquely toward the shoot tip in later stages (Supplementary Figure 2). Intercellular spaces form schizogenously between the root primordium and the cortex as the root primordium grows (Supplementary Figures 2A–C). The root cap becomes recognizable at the tip of root before it emerges from the parent stem (Supplementary Figure 2D).
The vascular tissue is almost matured ca. 1,500 μm from the apex (Supplementary Figures 1A,D). The ground meristem supplies cortical cells less actively at this level. The ventral depression of the vascular cylinder is still recognizable along with the intervening ventral tissue (Supplementary Figure 1D). However, incipient root primordia are not found below this level.
Root initiating position relative to dichotomy
We reconstructed 3D images of internal tissues of six young shoot axes which were collected from different individuals (Figure 6, Supplementary Figure 3 and Supplementary Videos 1–6) to visualize the positions of root primordia, ventral tissue and the dichotomies of stems precisely. These images showed that the ventral tissue runs linearly on the ventral side of the vascular cylinder of the large shoot. Some root primordia were found directly on the ventral face of the dichotomy (Figures 6B–D, Supplementary Figures 3A–C and Supplementary Videos 1, 4), while others were found slight apically (Figures 6E–G, Supplementary Figures 3D–F and Supplementary Videos 2, 5) or basally (Figures 6H–J, Supplementary Figures 3G–I and Supplementary Videos 3, 6) relative to the dichotomy.
One root primordium is usually found between adjacent dichotomies. While two root primordia are rarely found in an inter-dichotomy (Sample #S2–15 and S2–23; Supplementary Tables 1, 2), each dichotomy has only one root primordium nearby. In addition, we did not find more than two roots per inter-dichotomy. Root–dichotomy distances range from 10 to 332 μm and average distance is 107.1 ± 19.2 μm. Quarter lengths of inter-dichotomy vary from 72.5 to 481.5 μm with an average of 203.7 ± 24.8 μm (Figure 7A and Supplementary Table 3). The 95% confidence intervals are not overlapped between the root–dichotomy distances (107.1 ± 37.5 μm) and quarter inter-dichotomy lengths (203.7 ± 48.6 μm). The root–dichotomy distance is significantly shorter than the quarter length of inter-dichotomies (p = 0.00025 < 0.001; Figure 7A and Supplementary Table 3). A total of 23 of 25 root primordia are found within apical or basal quarter of the inter-dichotomies (Figure 7B).
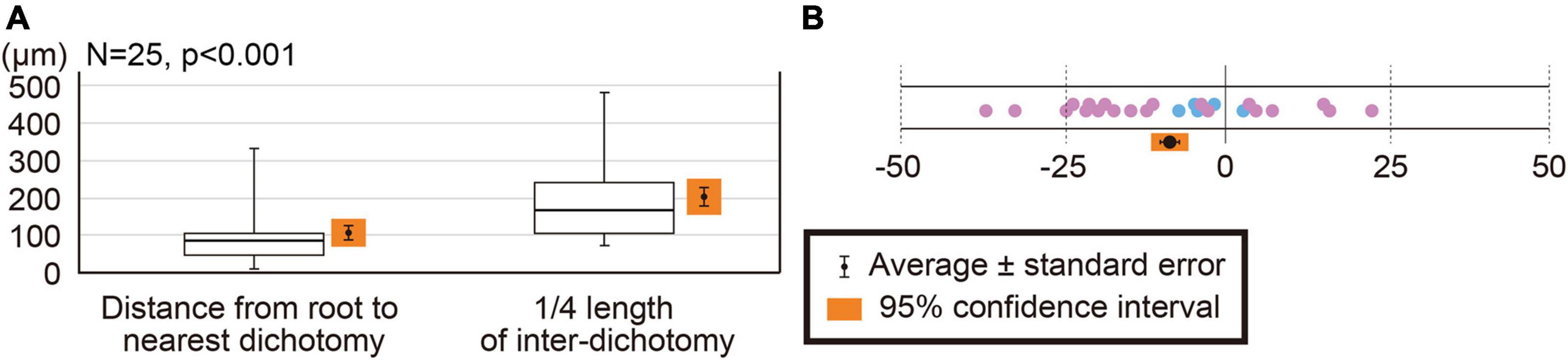
Figure 7. Comparison of root-dichotomy distances to quarter lengths of inter-dichotomies. (A) Box-whisker plots showing root-dichotomy distances and quarter lengths of inter-dichotomies. Bold lines show medians. (B) Relative root-dichotomy distances. Positions of dichotomies are set to zero, while lengths of inter-dichotomies to 100. Negative values indicate that the root primordium formed apical to a dichotomy. Root primordia associated with the first dichotomy are indicated by blue circles, while those with the second one by pink.
Relative root–dichotomy distances are larger in the second youngest dichotomy than in the youngest one (Figure 7B). This implies that elongation speed is not uniform over the entire inter-dichotomy. Such lack of uniformity would enhance the variability in the root–dichotomy distances in older inter-dichotomies, as actually observed in roots emerging outside of the parent stem (Figures 1A,B).
Discussion
The subterranean organ forms on the dichotomy in lycophytes
Selaginella forms the rhizophore in the vicinity of the branching angle of stems and the rhizophore grows downward to bear roots endogenously (Imaichi and Kato, 1989; Jernstedt et al., 1992; Figures 1C,D). Although organs identical to the rhizophore are not known in other lycophytes, Drepanophycales and zosterophylls have similar subterranean organs that grow downward from dichotomies of prostrate axes (Matsunaga et al., 2017). For example, the subterranean organ forms proximal to the dichotomy in Crenaticaulis, Gosslingia, Deheubarthia, and Bathurstia (Banks and Davis, 1969; Edwards, 1970; Edwards et al., 1989; Kotyk and Basinger, 2000; Matsunaga et al., 2017). On the other hand, it branches from either of the axes which have just dichotomized in Sengelia (Matsunaga and Tomescu, 2016, 2017) and Asteroxylon (Hetherington et al., 2021). In this study, we found that roots form around the dichotomies in Lycopodium (Figures 6, 7). This finding supports the hypothesis that the Lycopodium root were derived from the subterranean organ of the fossil lycophytes (Fujinami et al., 2021).
Although the precise positions varies among the subterranean organs, it should be noted that these organs consistently formed close to the dichotomies of prostrate axes. That is, fossil and extant lycophytes share this body plan (but see below for Isoetaceae). It is postulated that redirection of auxin flow from basipetal to acropetal occurred in the dichotomies in Selaginella (Matsunaga et al., 2017). In addition, auxin promotes root fate through transient rhizophore stage in the angle meristem of Selaginella (Mello et al., 2019). These findings support the idea that the dichotomies of lycophytes have a conserved role in regulating auxin concentration and/or flow, which promotes the development of the subterranean organs (Matsunaga and Tomescu, 2015).
Future perspective to understand a common body plan in lycophytes
Isoetes (monotypic genus of extant Isoetaceae) seems to have a different body plan from those of other lycophytes in the cormous body from which leaves and rootlets are born (Paolillo, 1963, 1982; Bierhorst, 1971). The corm has one or three furrows on the lower surface, which divide the corm into lobes (Figures 1E,F). Rootlets are repeatedly produced from the rootlet-producing meristem (or basal meristem), which develops along the furrows, while old rootlets are excised from the corm. As a result, rootlet scars are arranged regularly on both sides of the furrow (Bierhorst, 1971; Paolillo, 1982). It has been suggested that rootlets are modified leaves (Karrfalt, 1980; Stubblefield and Rothwell, 1981) in rhizomorphic lycophytes (sensu Kenrick and Crane, 1997) including Isoetaceae, while transcriptome data in Isoetes did not provide a clue supporting the homology between rootlets and leaves (Hetherington et al., 2020).
The Lycopodium roots develop on the ventral side of the differentiating vascular cylinder (Figures 4, 5) where the ventral tissue extends linearly (Figures 2–6). However, we did not observe the ventral tissue and root primordium simultaneously in a cross-section. This observation implies that at least a part of the ventral tissue transforms into the root primordium. Young root primordia could be recognized by a row of possible initial cells in Lycopodium, but it is difficult to identify primordia in younger stages at this moment. In addition, we could not elucidate if the ventral tissue is an independent tissue or a part of other tissue (e.g., vascular or cortical tissues). Nevertheless, it should be noted that Lycopodium and Isoetes have a linear zone in which roots or rootlets are born. Finding makers to identify the root primordia and tissue layers at very initial stage is crucial to understand how the ventral tissue contributes to the root formation in Lycopodium, as well as to compare the ventral tissue and rootlet-producing meristem of Isoetes.
Data availability statement
The original contributions presented in this study are included in the article/Supplementary material, further inquiries can be directed to the corresponding author.
Author contributions
YI, RF, and TY collected anatomical data on Lycopodium root development and TY and RI analyzed them. YI reconstructed 3D images of young shoots. TY designed experiments and the conceptual framework. YI and TY prepared the original draft of the manuscript. All authors polished the manuscript.
Funding
This study was funded by the Japan Society for the Promotion of Science (JSPS) KAKENHI grants (no. 18K06380 to RF and nos. 18H02495 and 21H02553 to TY and RF), and MEXT Promotion of Distinctive Joint Research Center Program (JPMXP0622716984 to TY).
Acknowledgments
We thank Kento Terada for his technical supports on 3D reconstruction. We also thank staffs of Botanical Gardens, Osaka Metropolitan University for cultivation of our materials.
Conflict of interest
The authors declare that the research was conducted in the absence of any commercial or financial relationships that could be construed as a potential conflict of interest.
Publisher’s note
All claims expressed in this article are solely those of the authors and do not necessarily represent those of their affiliated organizations, or those of the publisher, the editors and the reviewers. Any product that may be evaluated in this article, or claim that may be made by its manufacturer, is not guaranteed or endorsed by the publisher.
Supplementary material
The Supplementary Material for this article can be found online at: https://www.frontiersin.org/articles/10.3389/fevo.2022.930167/full#supplementary-material
References
Banks, H. P., and Davis, M. R. (1969). Crenaticaulis, a new genus of Devonian plants allied to Zosterophyllum, and its bearing on the classification of early land plants. Am. J. Bot. 56, 436–449. doi: 10.2307/2440821
Banks, J. A. (2009). Selaginella and 400 million years of separation. Annu. Rev. Plant Biol. 60, 223–238. doi: 10.1146/annurev.arplant.59.032607.092851
Beerling, D. J., Osborne, C. P., and Chaloner, W. G. (2001). Evolution of leaf-form in land plants linked to atmospheric CO2 decline in the late Palaeozoic era. Nature 410, 352–354. doi: 10.1038/35066546
Christenhusz, M. J. M., Fay, M. F., and Chase, M. W. (2017). Plants of the world: An illustrated encyclopedia of vascular plants. Chicago, IL: Univ. of Chicago Pr. doi: 10.7208/chicago/9780226536705.001.0001
Eames, A. J. (1977). Morphology of vascular plants –lower groups (Psilophytales to Filicales). New York, NY: Robert E. Krieger Publishing Company.
Edwards, D. (1970). Further observations on the Lower Devonian plant, Gosslingia breconensis Heard. Philos. Trans. R. Soc. Lond. B Biol. Sci. 258, 225–243. doi: 10.1098/rstb.1970.0034
Edwards, D. (1994). “Toward an understanding of pattern and process in the growth of early vascular plants,” in Shape and form in plants and fungi, eds D. S. Ingram and A. Hudson (London: Academic Press), 39–59.
Edwards, D., and Kenrick, P. (1986). A new zosterophyll from the Lower Devonian of Wales. Bot. J. Linn. Soc. 92, 269–283. doi: 10.1111/j.1095-8339.1986.tb01432.x
Edwards, D., Kenrick, P., and Carluccio, L. M. (1989). A reconsideration of cf. Psilophyton princeps (Croft & Lang, 1942), a Zosterophyll widespread in the Lower Old Red Sandstone of South Wales. Bot. J. Linn. Soc. 100, 293–318. doi: 10.1111/j.1095-8339.1989.tb01723.x
Foster, A. S., and Gifford, E. M. Jr. (1959). Comparative morphology of vascular plants. San Francisco, CA: W. H. Freeman and company.
Freeberg, J. A., and Wetmore, R. H. (1967). The Lycopsida –a study in development. Phytomorphology 17, 78–91.
Friedman, W. E., Moore, R. C., and Purugganan, M. D. (2004). The evolution of plant development. Am. J. Bot. 9, 1726–1741. doi: 10.3732/ajb.91.10.1726
Fujinami, R., Nakajima, A., Imaichi, R., and Yamada, T. (2021). Lycopodium root meristem dynamics supports homology between shoots and roots in lycophytes. New Phytol. 229, 460–468. doi: 10.1111/nph.16814
Fujinami, R., Yamada, T., and Imaichi, R. (2020). Root apical meristem diversity and the origin of roots: Insights from extant lycophytes. J. Plant Res. 133, 291–296. doi: 10.1007/s10265-020-01167-2
Fujinami, R., Yamada, T., Nakajima, A., Takagi, S., Idogawa, A., Kawakami, E., et al. (2017). Root apical meristem diversity in extant lycophytes and implications for root origins. New Phytol. 215, 1210–1220. doi: 10.1111/nph.14630
Gensel, P. G. (1992). Phylogenetic relationships of the zosterophylls and lycopsids: Evidence from morphology, paleoecology, and cladistic methods of inference. Ann. Mo. Bot. Gard. 79, 450–473. doi: 10.2307/2399750
Gensel, P. G., and Berry, C. M. (2001). Early lycophyte evolution. Am. Fern J. 91, 74–98. doi: 10.1640/0002-8444(2001)091[0074:ELE]2.0.CO;2
Gensel, P. G., Kotyk, M. E., and Basinger, J. F. (2001). “Morphology of above- and below- ground structures in Early Devonian (Pragian-Emsian) plants,” in Plants invade the land, eds P. G. Gensel and D. Edwards (New York, NY: Columbia University Press), 83–102. doi: 10.7312/gens11160-006
Gifford, E. M. Jr., and Foster, A. S. (1989). Morphology and evolution of vascular plants, 3rd Edn. New York, NY: W. H. Freeman and Company.
Hass, H., and Remy, W. (1986). Angular-organe – Ausdruck einer wenig beachteten morphogenetischen Strategie devonischer Pflanzen. Argumenta Palaeobot. 7, 155–177.
Hetherington, A. J., and Dolan, L. (2018). Stepwise and independent origins of roots among land plants. Nature 561, 235–238. doi: 10.1038/s41586-018-0445-z
Hetherington, A. J., and Dolan, L. (2019). Rhynie chert fossils demonstrate the independent origin and gradual evolution of lycophyte roots. Curr. Opin. Plant Biol. 47, 119–126. doi: 10.1016/j.pbi.2018.12.001
Hetherington, A. J., Bridson, S. L., Jones, A. L., Hass, H., Kerp, H., and Dolan, L. (2021). An evidence-based 3D reconstruction of Asteroxylon mackiei, the most complex plant preserved from the Rhynie chert. eLife 10:e69447. doi: 10.7554/eLife.69447
Hetherington, A. J., Emms, D. M., Kelly, S., and Dolan, L. (2020). Gene expression data support the hypothesis that Isoetes rootlets are true roots and not modified leaves. Sci. Rep. 10:21547. doi: 10.1038/s41598-020-78171-y
Høeg, O. A. (1942). The Downtonian and Devonian flora of Spitsbergen. Nor. Svalbard Ishavs Unders. Skr. 83, 1–228.
Hueber, F. M. (1992). Thoughts on the early lycopsids and zosterophylls. Ann. Mo. Bot. Gard. 79, 474–499. doi: 10.2307/2399751
Imaichi, R., and Kato, M. (1989). Developmental anatomy of the shoot apical cell, rhizophore and root of Selaginella uncinata. Bot. Mag. Tokyo 102, 369–380. doi: 10.1007/BF02488120
Jernstedt, J. A., Cutter, E. G., Gifford, E. M., and Lu, P. (1992). Angle meristem origin and development in Selaginella martensii. Ann. Bot. 69, 351–363. doi: 10.1093/oxfordjournals.aob.a088352
Karrfalt, E. E. (1980). A further comparison of Isoetes roots and stigmarian appendages. Can. J. Bot. 58, 2318–2322. doi: 10.1139/b80-268
Kenrick, P., and Crane, P. R. (1997). The origin and early diversification of land plants –a cladistic study. Washington, DC: Smithsonian Institution Press.
Kenrick, P., and Edwards, D. (1988). A new zosterophyll from a recently discovered exposure of the Lower Devonian Senni Beds in Dyfed, Wales. Bot. J. Linn. Soc. 98, 97–115. doi: 10.1111/j.1095-8339.1988.tb01698.x
Kenrick, P., and Strullu-Derrien, C. (2014). The origin and early evolution of roots. Plant Physiol. 166, 570–580. doi: 10.1104/pp.114.244517
Kerp, H., Wellman, C. H., Krings, M., Kearney, P., and Hass, H. (2013). Reproductive organs and in situ spores of Asteroxylon mackiei Kidston & Lang, the most complex plant from the Lower Devonian Rhynie chert. Int. J. Plant Sci. 174, 293–308. doi: 10.1086/668613
Kidston, R., and Lang, W. H. (1920). On Old Red Sandstone plants showing structure, from the Rhynie chert bed, Aberdeenshire. Part III. Asteroxylon mackiei, Kidston and Lang. Trans. R. Soc. Edinb. 52, 643–680. doi: 10.1017/S0080456800004506
Kidston, R., and Lang, W. H. (1921). On Old Red Sandstone plants showing structure, from the Rhynie chert bed, Aberdeenshire. Part IV. Restorations of the vascular cryptogams, and discussion of their bearing on the general morphology of the Pteridophyta and the origin of the organisation of land plants. Trans. R. Soc. Edinb. 52, 831–854. doi: 10.1017/S0080456800004506
Kotyk, M. E., and Basinger, J. F. (2000). The Early Devonian (Pragian) zosterophylls Bathurstia denticulata Hueber. Can. J. Bot. 78, 193–207. doi: 10.1139/b99-179
Landini, G., Martinelli, G., and Piccinini, F. (2020). Colour deconvolution: Stain unmixing in histological imaging. Bioinformatics 37, 1485–1487. doi: 10.1093/bioinformatics/btaa847
Le Hir, G., Donnadieu, Y., Goddéris, Y., Meyer-Berthaud, B., Ramstein, G., and Blakey, R. C. (2011). The climate change caused by the land plant invasion in the Devonian. Earth Planet Sci. Lett. 310, 203–212. doi: 10.1016/j.epsl.2011.08.042
Matsunaga, K. K. S., and Tomescu, A. M. F. (2015). Developmental determinants, morphology, and the fossil record – updating paradigms in the evolution of lycophyte rooting structures. Available online at: http://2015.botanyconference.org/engine/search/index.php?func=detail&aid=453 (accessed October 4, 2022).
Matsunaga, K. K. S., and Tomescu, A. M. F. (2016). Root evolution at the base of the lycophyte clade: Insights from an Early Devonian lycophyte. Ann. Bot. 117, 585–598. doi: 10.1093/aob/mcw006
Matsunaga, K. K. S., and Tomescu, A. M. F. (2017). An organismal concept for Sengelia radicans gen. et sp. nov. – morphology and natural history of an Early Devonian lycophyte. Ann. Bot. 119, 1097–1113. doi: 10.1093/aob/mcw277
Matsunaga, K. K. S., Cullen, N. P., and Tomescu, A. M. F. (2017). Vascularization of the Selaginella rhizophore: Anatomical fingerprints of polar auxin transport with implications for the deep fossil record. New Phytol. 216, 419–428. doi: 10.1111/nph.14478
Mello, A., Efroni, I., Rahni, R., and Birnbaum, K. D. (2019). The Selaginella rhizophore has a unique transcriptional identity compared with root and shoot meristems. New Phytol. 222, 882–894. doi: 10.1111/nph.15630
Morris, J. L., Puttick, M. N., Clark, J. W., Edwards, D., Kenrick, P., Pressel, S., et al. (2018). The timescale of early land plant evolution. Proc. Natl. Acad. Sci. U.S.A. 115, E2274–E2283. doi: 10.1073/pnas.1719588115
Øllgaard, B. (2015). Six new species and some nomenclatural changes in neotropical Lycopodiaceae. Nord. J. Bot. 33, 186–196. doi: 10.1111/njb.00652
Paolillo, D. J. Jr. (1963). The developmental anatomy of Isoetes, Vol. 31. Urbana, IL: University of Illinois Press. doi: 10.5962/bhl.title.50237
Paolillo, D. J. Jr. (1982). Meristems and evolution: Developmental correspondence among the rhizomorphs of the lycopsids. Am. J. Bot. 69, 1032–1042. doi: 10.1002/j.1537-2197.1982.tb13347.x
Raven, J. A., and Edwards, D. (2001). Roots: Evolutionary origins and biogeochemical significance. J. Exp. Bot. 52, 381–401. doi: 10.1093/jexbot/52.suppl_1.381
Roberts, E. A., and Herty, S. D. (1934). Lycopodium complanatum var. flabelliforme Fernald: Its anatomy and a method of vegetative propagation. Am. J. Bot. 21, 688–697. doi: 10.2307/2436286
Schindelin, J., Arganda-Carreras, I., Frise, E., Kaynig, V., Longair, M., Pietzsch, T., et al. (2012). Fiji: An open-source platform for biological-image analysis. Nat. Methods 9, 676–682. doi: 10.1038/nmeth.2019
Schmid, B., Schindelin, J., Cardona, A., Longair, M., and Heisenberg, M. (2010). A high-level 3D visualization API for Java and ImageJ. BMC Bioinformatics 11:274. doi: 10.1186/1471-2105-11-274
Schneider, C. A., Rasband, W. S., and Eliceiri, K. W. (2012). NIH image to ImageJ: 25 years of image analysis. Nat. Methods 9, 671–675. doi: 10.1038/nmeth.2089
Stein, W. E., Berry, C. M., Morris, J. L., Hernick, L. V., Mannolini, F., Ver Straeten, C., et al. (2020). Mid-Devonian Archaeopteris roots signal revolutionary change in earliest fossil forests. Curr. Biol. 30, 421–431. doi: 10.1016/j.cub.2019.11.067
Stubblefield, S. P., and Rothwell, G. W. (1981). Embryology and reproductive biology of Bothrodendrostrobus mundus (Lycopsida). Am. J. Bot. 68, 625–634. doi: 10.1002/j.1537-2197.1981.tb12394.x
Wellman, C. H., and Gray, J. (2000). The microfossil record of early land plants. Philos. Trans. R. Soc. Lond. B Biol. Sci. 355, 717–732. doi: 10.1098/rstb.2000.0612
Keywords: root, lycophytes, Lycopodium, Isoetes, subterranean organ
Citation: Ito Y, Fujinami R, Imaichi R and Yamada T (2022) Shared body plans of lycophytes inferred from root formation of Lycopodium clavatum. Front. Ecol. Evol. 10:930167. doi: 10.3389/fevo.2022.930167
Received: 27 April 2022; Accepted: 05 October 2022;
Published: 20 October 2022.
Edited by:
Alexandru Tomescu, Cal Poly Humboldt, United StatesReviewed by:
Edyta M. Gola, University of Wrocław, PolandJill Harrison, University of Bristol, United Kingdom
Copyright © 2022 Ito, Fujinami, Imaichi and Yamada. This is an open-access article distributed under the terms of the Creative Commons Attribution License (CC BY). The use, distribution or reproduction in other forums is permitted, provided the original author(s) and the copyright owner(s) are credited and that the original publication in this journal is cited, in accordance with accepted academic practice. No use, distribution or reproduction is permitted which does not comply with these terms.
*Correspondence: Toshihiro Yamada, cHRpbG9AbmlmdHkuY29t