- 1Department of Integrative Biology, University of Texas, Austin, TX, United States
- 2Department of Biology, McMaster University, Hamilton, ON, Canada
- 3Department of Ecology and Evolutionary Biology, University of California, Santa Cruz, Santa Cruz, CA, United States
- 4Institute of Marine Sciences, University of California, Santa Cruz, Santa Cruz, CA, United States
Sexual selection is a powerful diversifier of phenotype, behavior and cognition. Here we compare cognitive-behavioral traits across four reproductive phenotypes (females and three alternative males) of wild-caught ocellated wrasse (Symphodus ocellatus). Both sex and alternative male phenotypes are environmentally determined with sex determination occuring within the first year, and males transition between alternative phenotypes across 2 years (sneaker to satellite or satellite to nesting). We captured 151 ocellated wrasse and tested them on different behavior and cognition assays (scototaxis, shoaling, and two detour-reaching tasks). We found greater divergence across alternative male reproductive phenotypes than differences between the sexes in behavior, problem-solving, and relationships between these traits. Nesting males were significantly less bold than others, while sneaker males were faster problem-solvers and the only phenotype to display a cognitive-behavioral syndrome (significant correlation between boldness and problem-solving speed). Combining these results with prior measurements of sex steroid and stress hormone across males, suggests that nesting and sneaker males represent different coping styles. Our data suggests that transitioning between alternative male phenotypes requires more than changes in physiology (size and ornamentation) and mating tactic (sneaking vs. cooperation), but also involves significant shifts in cognitive-behavioral and coping style plasticity.
Introduction
We are quite familiar with the power of sexual selection as a shaper of traits—be them behavioral, morphological or even bundled together as suites (e.g., life history strategies). Yet the traits that most often come to mind as products of sexual selection are those directly related to reproduction. For instance, the sexually dimorphic tendency for females to be the choosier sex and males to be the competitors for access to mates has long been attributed to fundamental differences in selection on the sexes (Fairbairn et al., 2007; Fairbairn, 2013). Furthermore, the variation in male reproductive phenotypes and the divergent mating tactics that have repeatedly arisen across the animal kingdom is also a predicted outcome of intense intrasexual selection (Oliveira et al., 2008; Neff and Svensson, 2013; Shuster and Wade, 2019). However, few studies have addressed how intrasexual selection shapes differences among individuals in non-reproductive traits. Here we explore how sexual selection affects intraspecific variation in cognition and non-reproductive behavioral profiles between the sexes and among alternative male phenotypes.
Cognitive scientists are becoming more and more aware that sexual selection has a role in driving differences between the sexes in cognitive traits and the behaviors associated with them. Across a wide-range of taxa, studies have found significant differences in females’ and males’ spatial cognitive abilities (Jones et al., 2003; Jonasson, 2005; Carazo et al., 2014; Lucon-Xiccato and Bisazza, 2017), reversal learning abilities (Lucon-Xiccato and Bisazza, 2014; Fuss and Witte, 2019; Triki and Bshary, 2021), problem-solving and innovation (Laland and Reader, 1999; Aplin et al., 2013), and ability to recognize a spatial-temporal pattern (Wallace et al., 2020). It is important to note that in many cases there is no sexual dimorphism in cognitive performance (Healy et al., 1999; Lucon-Xiccato and Bisazza, 2016), and the difficulty of ensuring equal motivation between the sexes in cognition tasks can be daunting (Rowe and Healy, 2014). Nonetheless, when dimorphisms do arise they are often attributed to sexual selection forces and the different means by which males and females maximize their reproductive success (Jacobs, 1996; Jones et al., 2003; Carazo et al., 2014; Lucon-Xiccato and Bisazza, 2016; Cummings, 2018; Keagy et al., 2019). For instance, the sexually dimorphic differences in spatial cognition are often linked to territorial differences. In some rodent species with males securing larger territories than females, males have been shown to have better spatial cognition (FitzGerald, 1986; Galea et al., 1996). Meanwhile in species where females have reproductive tactics involving greater territorial use than males (e.g., brood parasitism by female cowbirds), females have larger hippocampal volumes than males and outperform males in spatial memory tasks (Astié et al., 1998; Hauber et al., 1999; Guigueno et al., 2014).
Sexually dimorphic cognition is not always attributed to sexually dimorphic reproductive roles. Rather, sometimes it is attributed to different behavioral traits that allow each sex to maximize reproductive success (Cummings, 2018). For instance, in three-spined sticklebacks (Gasterosteus aculeatus) bolder individuals solved foraging tasks faster than shyer individuals, and males tended to be bolder than females (Mamuneas et al., 2014). Three-spined stickleback males have also been shown to outperform females in a detour reaching task (that evaluates inhibitory control and cognitive flexibility) and this performance is attributed to males exhibiting less neophobia than females (Keagy et al., 2019). These results raise further questions, such as do males tend to be bolder and less neophobic than females because their reproductive strategies favor those traits? And does this behavioral tendency influence their cognitive performance in certain tasks? The relationship between behavior and cognition (e.g., cognitive-behavioral syndromes or profiles) has become a recent area of investigation to understand sources of cognitive variation between individuals (Exnerová et al., 2010; Carere and Locurto, 2011; Sih and Del Giudice, 2012; Guillette et al., 2017; Lucon-Xiccato and Bisazza, 2017; Dougherty and Guillette, 2018), and the sexes (Cummings, 2018; Etheredge et al., 2018). While Pavlov first proposed a relationship between personality (behavioral types) and learning processes in dogs (Pavlov, 1948; Gray, 1964; Locurto, 2007; Carere and Locurto, 2011), only more recently have these relationships begun to be quantified in birds (Guillette et al., 2011), fishes (Lucon-Xiccato and Bisazza, 2017; Jacquin et al., 2020; Wallace et al., 2020), guinea pigs (Brust and Guenther, 2017) and others [reviewed in Dougherty and Guillette (2018)]. Sometimes the specific relationship between an animal’s behavioral tendencies and its cognitive styles and/or performances makes intuitive sense. If animals explore their environment faster, they might in turn learn more about their environment than slower explorers (Guillette et al., 2009; Sih and Del Giudice, 2012); and variation in these relationships has been shown to have fitness consequences in the wild (Cole et al., 2012; Cole and Quinn, 2012). However, understanding why these relationships might differ between the sexes is still not fully understood. For instance, in great tits (Parus major) males exhibit a positive relationship between exploratory speed and cognitive flexibility, whereas, females show the opposite pattern (Titulaer et al., 2012). Understanding the behavioral and cognitive demands sexual selection is placing on each sex may help to unravel some of these more complicated relationships between how an animal interacts with its environment (behavioral traits/personality) and how well it performs a cognitive task.
While we are learning much by comparing sexual dimorphic patterns across species, a parallel body of work is attempting to understand how sexual selection contributes to variation in cognitive-behavioral traits among individuals of the same sex. Studies across a number of taxa have documented that variation in some male cognitive traits are correlated with continuous variation in sexually selected traits and reproductive success [in birds: Keagy et al. (2009), Boogert et al. (2011), and Chen et al. (2019); in fish: Smith et al. (2015)]; that male cognitive performance increases with greater intrasexual selection [among Drosophila spp.: Hollis and Kawecki (2014) and Smith et al. (2015)], and that artificially selecting for increased male brain size can lead to greater mate search capabilities [in guppies: Kotrschal et al. (2015)]. However, direct comparison of cognitive performance between alternative reproductive male phenotypes (e.g., Griebling et al., 2020), or characterizing their cognitive-behavioral syndromes, is in its nascency.
We focus on a system that is uniquely positioned to explore how sexual selection shapes divergence in cognitive-behavioral profiles. The ocellated wrasse (Symphodus ocellatus) is a marine fish with a complex mating system involving three alternative male phenotypes (Warner and Lejeune, 1985; Alonzo et al., 2000; Alonzo and Warner, 2000a,b) and exhibits extensive reproductive plasticity driven by environment. The largest and most colorful males in the population (“nesting males”) must compete for territories (Alonzo, 2004), build and defend nests made of algae, court and be chosen by females (Alonzo, 2008; Alonzo and Heckman, 2010), compete with sneaker and satellite males to fertilize the eggs spawned by females (Alonzo and Warner, 2000b; Alonzo and Heckman, 2010; Alonzo et al., 2016; Stiver et al., 2018), and care for the developing embryos. The smallest males (“sneakers”) do not build nests, court females, or provide care. Instead, sneakers rush into a nest to release sperm when a female is spawning with a nesting male; and they compete for access to the nest and with other sneakers. “Satellite” males are intermediate in body size and coloration. They do not build nests or provide care, but they do assist the nesting male by chasing away sneakers and by actively bringing females to the nesting male’s nest (Stiver and Alonzo, 2013). As a result of these different selective forces, alternative male types differ in a wide variety of traits including aggressive behavior, gonad size, sperm traits, circulating hormones, and gene expression in the brain (Lejeune, 1985; Warner and Lejeune, 1985; Taborsky et al., 1987; Stiver et al., 2015; Nugent et al., 2016, 2019; Dean et al., 2017).
Both sex (Munday et al., 2006; Pennell et al., 2018) and alternative male phenotype (Alonzo et al., 2000) are environmentally-determined based on growth trajectories. Sex is determined within year 1, with females exhibiting no alternative phenotypes and no evidence of sex change (Warner and Lejeune, 1985). Females choose among available nests, actively defended and maintained by nesting males, and produce a new batch of eggs every 2–3 days throughout the roughly 2 month breeding season (Warner and Lejeune, 1985; Taborsky et al., 1987). On the other hand, males switch between types between years with fast growing males starting out as satellite males and becoming nesting males if they survive to their second year, while slow-growing males reproduce as sneakers in their first year and as satellites in their second (Alonzo et al., 2000). Here we ask whether sexual selection, which has shaped these many differences in reproductive traits between the sexes and among these males types, leads to differences in non-reproductive behaviors, cognition, and cognitive-behavioral syndromes (correlations between behavior and cognitive traits)?
The absence of a genetic polymorphism-based Alternative Reproductive Tactic (ART) system in the ocellated wrasse allows us to test how the reproductive “role” individuals adopt shapes non-reproductive traits. In a system where sexes are determined environmentally, and males transition from one role to another across years, we can identify cognitive-behavioral traits associated with an individual reproductive role rather than genetic predisposition. We can further compare differences between these alternative male roles and females that remain in a single reproductive role throughout their lifetime. Due to the differences in lifetime reproductive strategies between the sexes, researchers have noted sexual dimorphism in some non-reproductive behaviors involving sociability and boldness (Magurran and Macias Garcia, 2000). Variation in male boldness often covaries with reproductive success (Smith and Blumstein, 2008), suggesting that boldness levels are likely to diverge across alternative male reproductive tactics. Cognitive sexual dimorphism observed in spatial learning and problem-solving that is linked to sexual selection processes (Lucon-Xiccato and Bisazza, 2016), is also likely to manifest across alternative male phenotypes that vary in territorial use or innovation to gain access to females. Early neurobiological research suggests males with alternative strategies might be employing different cognitive strategies resulting in differential investment in neocortex size (Dunbar, 1998; Pawlowski and Dunbar, 1999); and neurogenomic work suggests females experiencing multiple male phenotypes engage more genomic synaptic plasticity pathways in the brain than females interacting with a single (coercive) tactic (Lynch et al., 2012; Wang et al., 2014; Cummings and Ramsey, 2015). Lastly, the correlations between behavioral tendencies and cognitive performances [cognitive-behavioral syndromes; Carere and Locurto (2011)] that are beginning to be characterized between the sexes (Titulaer et al., 2012; Etheredge et al., 2018; Wallace et al., 2020) are predicted to diverge across alternative male phenotypes (Carazo et al., 2014). However, to date, direct comparisons of inter and intrasexual variation across the abovementioned axes within a species has yet to be undertaken.
Here, we explore the inter-sexual and intra-sexual divergence across different behavioral features (boldness, sociality, social boldness, and social motivation) along with cognitive measures (problem-solving), and explore the relationships between them (cognitive-behavioral syndromes). In general, we hypothesize that inter-sexual selection will drive greater differences between males and females than variation observed due to intra-sexual selection across males. Specifically, we hypothesize that (i) males (and territorial, nesting males in particular) will be bolder than females, and (ii) females will exhibit greater cognitive flexibility (problem-solving or inhibitory control) than males as has been shown in some fish taxa (Laland and Reader, 1999; Lucon-Xiccato and Bisazza, 2014; Triki and Bshary, 2021), but not others (Keagy et al., 2019). We also hypothesize that (iii) alternative male reproductive roles will have different cognitive-behavioral traits including nesting males exhibiting greater inhibitory control during problem-solving tasks than sneakers due to their parental role (Keagy et al., 2019). Furthermore, we hypothesize that (iv) differences in cognitive-behavioral syndromes between the sexes will be greater than across males types given sexually dimorphic life history strategies. Lastly, we hypothesize that (v) each of the alternative male reproductive phenotypes should show greater variation in cognitive-behavioral syndromes than females due to the plasticity in reproductive-role transitions observed in males. With this system, we have the power to not only determine if males and females differ, and how alternative male phenotypes differ, but also to ask whether the variation we see within the sexes (e.g., across the alternative types) is greater than the variation we see between the sexes.
Materials and Methods
Underwater Observation and Capture of Wild Fish
This research was conducted during the Symphodus ocellatus breeding season (May-June) in 2017 and 2018 in the Baie de Revellata and marine research station La Station de Recherches Sous-Marin et Océanographique (STARESO) near Calvi, Corsica. Fish were first observed and then captured on SCUBA in the rocky reef habitat along roughly 200 m of coastline near the research station. We selected nests that were being actively defended by a nesting male and had one satellite and at least two sneakers present and oriented toward the nest (Alonzo and Warner, 2000a; Alonzo, 2004). The reproductive phenotypes (Females, Nesting Males, Satellite Males, Sneaker Males) are identified by their behavioral and morphological differences (Lejeune, 1985; Alonzo and Warner, 2000a). We then observed the nest for ten minutes to confirm that a nesting male was occupying and defending the nest, females were visiting and spawning in the nest, a satellite was clearly associated with the nest (by assessing whether they remained near the nest and were interacting with other fish at the nest), and that sneaker males were attempting sneak spawning events. Following this observation, we caught the nesting male, satellite, a sneaker and a female associated with the nest using small hand held nets and placed them in bait buckets for transfer back to the research station. Fish were then immediately isolated from other fish and habituated in holding tanks for a minimum of 2 h with freely flowing seawater prior to the sequence of assays described below. Following the three assays (10 min each with 10 min acclimation in between), the fish were released back into the vicinity of their nests prior to sunset on the same day as capture.
Assays
After habituating our wild caught fish for a minimum of 2 h post capture, we measured the four reproductive phenotypes (Females, Nesting Males, Satellite Males, Sneaker Males) across three cognitive and behavioral assays in the same sequence for all individuals: (1) Detour reaching task (an assay to evaluate social motivation as well as problem-solving, inhibitory control, and cognitive flexibility), (2) Shoaling (an assay to measure social tendencies and non-social style), and (3) Scototaxis (an assay to measure anxiety and/or boldness). Each assay included a 10 min habituation period (within a clear cylinder) followed by a 10 min observation period. All assays were conducted in immediate sequence of each other with 10 min time interval between assays. In total, the complete assay sequence for an individual fish took 100 min to completion. Each assay was videotaped from above using a GoPro camera (Hero 5 Black), and all experimental chambers were illuminated from above with fluorescent lights and blue filters to mimic marine spectral irradiance.
Detour Task
For the first assay, we presented each fish with a novel detour-reaching problem-solving task. Fish were placed in a detour-reaching tank (Figure 1C) that included a blue-felted alley (25 cm long × 10 cm wide) that opened up into a wider section (28 cm wide) containing a glass partition (21 cm wide) positioned 10 cm from the alley opening and 8 cm in front of a social reward chamber (a female conspecific within a transparent chamber). For this task, fish were initially placed in a clear habituation tube at the back end of a dark alley for a 10 min habituation period where they were able to observe the social reward at the opposite end of the experimental tank (Figure 1C). After 10 min, the tube was removed and the fish could freely swim throughout the chamber for the following 10 min. The video recordings were then scored by an UT undergraduate student (AR) naive to the research objectives for the following measures including (a) social motivation (time from release to approach of the glass barrier) and (b) problem-solving speed (time from first contact with glass barrier to social reward). To determine whether all phenotypes were equally motivated to approach a female social reward, we compared the latency time to reach the glass barrier (social motivation) between males and females as well as between the three male reproductive phenotypes. We further determined the relationship between social motivation and problem-solving speed by examining correlations between these variables across individuals within each phenotype.
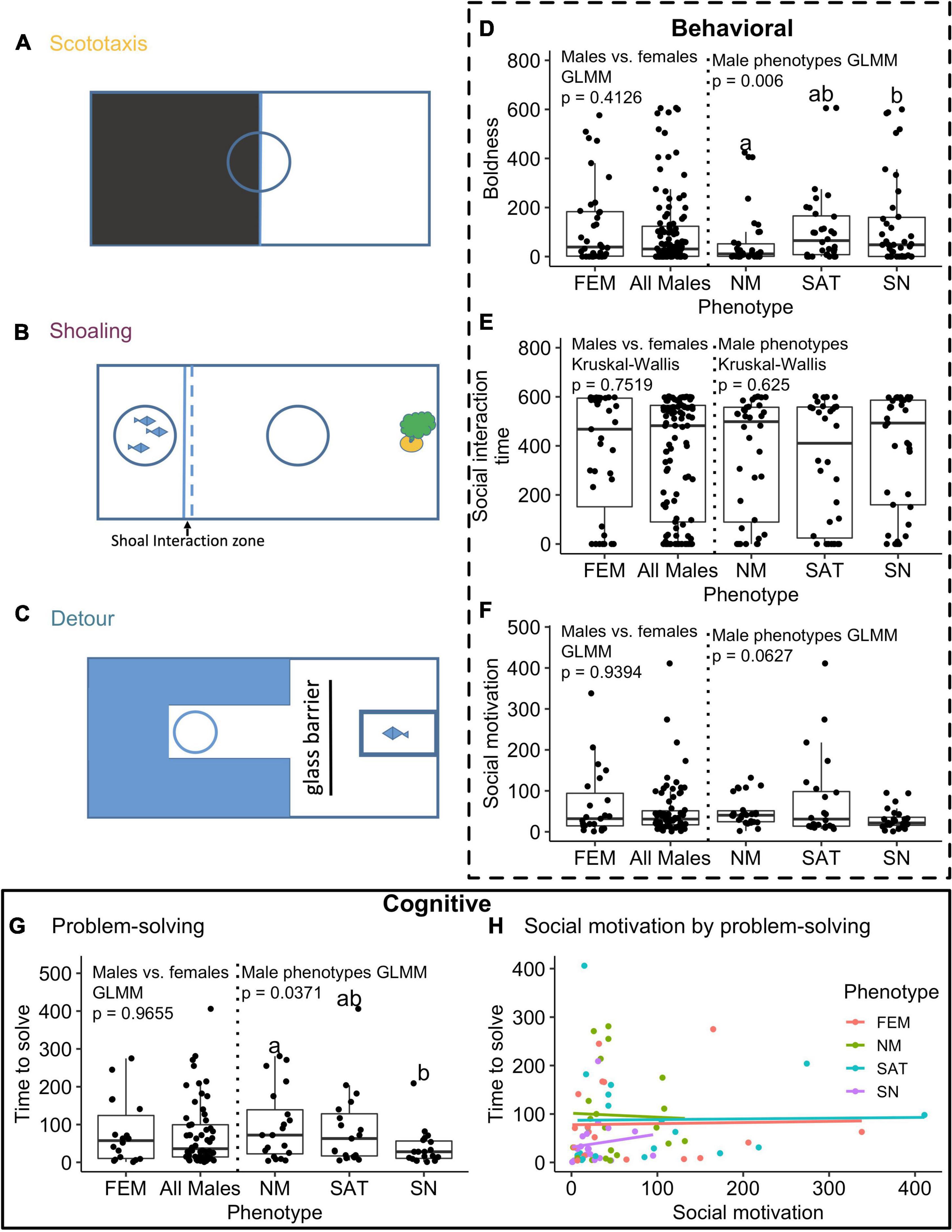
Figure 1. Aerial views of experimental assays (A–C), behavioral metrics (D–F), and cognitive performances (G,H) for all phenotypes. All experimental arenas were 98 cm × 28 cm × 24 cm. Scototaxis assays were run in an aquarium with half of the tank felted in black and the other half felted in white, with habituation cylinder (blue circle) placed at the midline between each half (A). Boldness was evaluated as the time (seconds) spent in the white half of the scototaxis tank (D). Shoaling assays were conducted in a tank with a transparent barrier between a shoal group (three conspecific females in a transparent cylinder) and the region of the tank wherein the focal fish was free to roam (B). The habituation cylinder (blue circle) was placed mid-way between social interaction zone [1 cm away from the shoal barrier (see dotted line) and the plant positioned at the far end of tank]. Shoaling assays were used to measure both social interaction time (seconds) spent within 1 cm of the shoal barrier (E), and social boldness (proportion of time spent in open region of the tank (excluding social interaction zone) relative to time spent in plant). Each focal fish ran through a detour reaching assay wherein fish were released from a habituation cylinder at the back end of an alley and allowed to navigate around a glass barrier to arrive at a social reward [a female conspecific in a transparent container placed at the opposite end of the tank, (C)]. From these detour assays, we collected one behavioral measure: social motivation, latency time (seconds) to reach glass barrier after release from habituation tube, (F), and problem-solving, time (seconds) from barrier to social reward (time to solve) in the detour task, (G). We plot the relationship between social motivation (time to barrier) and problem-solving speed (time from barrier to social reward) in detour task [r = 0.07, p = 0.55, (H)]. Bar graphs (D–G) represent box and whisker plots with midline representing the median value and upper and lower box lines representing upper and lower quartiles, respectively. Statistical comparisons between the sexes and the alternative male reproductive phenotypes (NM, nesting male; SAT, satellite male; SN, sneaker male) were evaluated with either GLMM models or Kruskal-Wallis based on normality criteria. Post-hoc Tukey tests across alternative male mating phenotypes reveal pairwise differences between phenotypes by different letters.
Shoaling
Directly following the first detour task, the focal fish was transferred by a net to the Shoaling tank (98 cm long, Figure 1B) and into an opaque habituation tube centrally positioned between a transparent partition that separated a shoal group (three conspecific females) and a plant at the opposite end of the tank (habituation tube was 20 cm distance from both the shoal group and the plant). After a 10 min habituation period, the tube was removed and the following 10 min of social interactions were recorded. UCSC undergraduate researchers used the software Cowlog to score the videos of the shoaling assay tanks described above. Multiple individuals scored each video and scores were averaged. Scoring started when the acclimation tube was removed from the water and ended after 10 min. We noted when the focal fish was in the “shoal interaction zone” (i.e., the focal individual moved within approximately 1 cm of the barrier between them and the side of the tank with the shoal group), in or near the plant (within approximately 1 cm or in contact with the plant), or in the center of the tank (not near the plant or shoal group). We used the Cowlog output to calculate sociability (the proportion of time spent in the “shoal interaction zone”). To characterize the behavior of the individuals when they were outside of the “shoal interaction zone,” we calculated the proportion of time individuals spent in the open center area relative to time spent in the plant zone as a measure of social boldness. This social boldness measure was evaluated as the proportion of time in the center zone relative to total time away from shoal interaction zone (time in center zone plus time in plant area; see Figure 1A). This measure captures the relative boldness of an individual when it is not interacting in close proximity with others. High social boldness scores are associated with individuals spending a greater proportion of non-social interaction time in the open more exposed areas relative to time spent hiding in the refuge of a plant. Low social boldness scores are associated with individuals who spent the majority of their non-social interaction time in the plant refuge.
Scototaxis
Directly following the shoaling assay, individuals were transferred by handnet into an opaque habituation tube in the center of a scototaxis tank. The scototaxis tank (78 cm long × 28 cm wide) consisted of black and white felted halves (Figure 1A). Scototaxis is a commonly used assay to assess anxiety and boldness levels of smaller prey fish (Maximino et al., 2010; Ramsey et al., 2014; Etheredge et al., 2018) by comparing time spent in the safe (darker, black half) relative to time spent in the more vulnerable zone (brighter, white half). Multiple UCSC undergraduate researchers manually scored the time individual fish spent on the black and white sides of the tank. Scores were averaged across individuals, and we used time spent in the white side of the tank as our measure of boldness.
Analysis Overview
We first examined whether behavioral or cognitive variation within each assay significantly differed by phenotype. We fit generalized linear mixed models in the glmmTMB R package (Brooks et al., 2017), using phenotype as a fixed effect, year of data collection as a random effect, and behavior as the response variable. For each model, we examined the residuals for normality, overdispersion, and the presence of outliers. If these diagnostics showed evidence of poor fit of the model to the data, we attempted the following remedies. We first tried common transformations of the response variable (log + 1 and square root). If common transformations were unsuccessful, for count data we changed the family argument to each of the two negative binomial families within glmmTMB using the default link function, both with and without accounting for zero-inflation using the ziformula argument (as many of the measures we examined were zero-inflated). We then analyzed the residuals from the best model from this analysis as measured by AIC. To examine potential relationships between behavioral tendencies in different contexts (scototaxis and sociability) and relationship to performance across different cognitive tasks (e.g., problem-solving), we generated Spearman rank correlation matrices across the following variables from behavior and cognition assays: social interaction time, social boldness, boldness, social motivation, and time to solve (the problem-solving detour task). We tested significance of each correlation independently with the cor_pmat function from the ggcorrplot package (Kassambara and Kassambara, 2019). We then built generalized linear mixed models that included metrics from each assay as well as interaction terms. As before, year of assay was a random effect in the model. To explore how these diverse interactions between behavior and phenotype might explain variation in cognitive performance, we built generalized linear mixed model to explain cognitive performance (problem-solving in detour task) using behavioral responses and interactions by phenotype as explanatory variables. We then performed backwards model selection based on AIC using the step() function in R to arrive at a simpler model that we use for inference; and tested for multi-collinearity using the check_collinearity function in R. Lastly, as a complimentary approach to our GLMM models, we constructed Linear Discriminant Analyses (LDA) using phenotype as our grouping category and independent measures from each assay to visualize how phenotypes differ by their cognitive-behavioral profiles. We tested whether the loading on the first LDA axis differed by phenotype using a GLMM with phenotype as a fixed effect, year as a random effect, and LDA axis 1 score as the response variable. For all statistical comparisons, we included all four phenotypes (females, sneaker males, satellite males, nesting males) as well as constrained models to include only the three alternative male phenotypes. We used post-hoc tests to evaluate which phenotypes were more distinct from each other.
Results
We collected one of each ocellated wrasse phenotype from 41 different nests. Due to technical difficulties or a lack of participation by focal fish in some assays, we assayed 151 wild-caught ocellated wrasse in total including 38 females, 38 nesting males, 34 satellites, and 41 sneaker males; with final samples sizes varying across assays (N = 97 detour task, N = 140 sociability, N = 143 scototaxis). As expected, the different phenotypes differed in size with the greatest overlap between females and sneaker males. Females ranged in standard length from 43.2 to 68.5 cm with median = 48.5 cm; sneaker males ranged from 38.8 to 64 cm with median = 47.4; satellite males ranged from 49.1 to 68.4 cm with median = 61 cm, and nesting males ranged from 70.3 to 84.2 with a median = 76 cm.
Scototaxis (Boldness)
We found no differences between the sexes (GLMM, X 2 = 0.671, df = 1, p = 0.4126; Figure 1D) in time spent in the white portion (more bold zone) of the scototaxis tank. However, we found highly significant differences in boldness in models including only the three male ARTs (GLMM, n = 107, X 2 = 10.248, df = 2, p = 0.0059), with Tukey post-hoc contrasts showing significant differences between sneaker and nesting males (p = 0.005), and a marginally significant difference between nesting males and satellites (p = 0.053).
Shoaling Assay (Social Tendencies and Social Boldness)
We did not find significant differences between the sexes or across male phenotypes for either shoaling behavioral measure (time interacting with shoal: between sexes: Kruskal Wallis X 2 = 0.0999, df = 1, p = 0.7519; across male phenotypes: Kruskal Wallis X 2 = 0.939, df = 2, p = 0.62; Figure 1E). In addition, we found no significant sex or ART effects on social boldness (between the sexes: Kruskal Wallis X 2 = 1.924, df = 1, p = 0.1654; across male phenotypes: Kruskal Wallis X 2 = 2.3626, df = 2, p = 0.3069; data not shown).
Detour Task (Social Motivation, Cognitive Flexibility, Problem-Solving Abilities)
We first determined whether our social reward (a female conspecific) was equally motivating across all focal fish in our detour reaching task. We found that females were as motivated as males to approach the reward, as latency to approach the glass barrier revealed no significant differences between the sexes (GLMM, n = 97, X 2 = 0.0058, df = 1, p = 0.9394, Figure 1F). Furthermore, we found no significant difference in social motivation across the three male phenotypes with a non-significant trend for satellite males to approach more slowly than nesting or sneaker males (GLMM, n = 74, X 2 = 5.5405, df = 2, p = 0.06265; Figure 1F). We also found no significant difference across phenotypes in the number of individuals who solved the detour task (successfully reached the social reward) with 18 of 38 females, 20 of 38 nesting males, 19 of 34 satellite males, and 19 of 41 sneaker males solving the detour assay (X 2 = 0.894, p = 0.827). Across solvers, we found no significant sex differences in problem-solving speed (log transformed time to solve GLMM, n = 76, df = 1, X 2 = 0.0019, p = 0.9655; Figure 1G). However, when restricting the model to only male phenotypes, we found a significant difference in problem-solving speed across the three male ARTs (GLMM, n = 58, X 2 = 6.587, df = 2, p = 0.037; Figure 1G). Tukey post hoc contrasts revealed significant differences between sneaker and nesting males (p = 0.0456) with sneakers taking less time to solve the detour task than nesting males.
To evaluate whether variation in problem-solving speed was predicted by social motivation, we calculated pearson correlations between social motivation (latency to reach glass barrier) and problem-solving speed (time from barrier to social reward). We found no significant relationship overall (Pearson r = 0.07, p = 0.55) or within any of the four phenotypes (Females: r = 0.03, p = 0.92, Sneakers: r = 0.13, p = 0.58, Satellites: r = 0.02, p = 0.95, Nesting Males: r = −0.03, p = 0.89; Figure 1H). Taken together, these findings indicate that all phenotypes were similarly motivated by a female social reward, and that individual social motivation levels did not explain any of the variation in problem-solving speed across the different phenotypes.
Multivariate Relationships Within and Across Behavior and Cognitive Assays
To identify phenotype-specific cognitive-behavioral profiles, we characterized how the relationship between behavior and cognitive performance differs by phenotypes. We first evaluated Spearman rank correlations across each combination of behavior and cognitive traits (see Figure 2) for each phenotype independently. We note two interesting patterns linking behavioral tendencies to problem-solving performance. First, the significant relationship between boldness and problem-solving speed (time to solve in Detour task) differed across the phenotypes (bolded boxes lower right in each subplot of Figure 2). Sneaker males (r = 0.64, p = 0.005) and satellite males (r = 0.36, p = 0.134) showed a positive relationship between time to solve and scototaxis time, but this relationship was non-existent in nesting males (r = −0.15, p = 0.518) and females (r = −0.05, p = 0.844). Furthermore, social boldness and time to solve showed varying relationships across phenotypes ranging from negative (but non-significant) in females (r = −0.37, p = 0.195) to near-significant and strongly positive in satellite males (r = 0.47, p = 0.09) and non-existent/slightly negative in both nesting males and sneaker males.
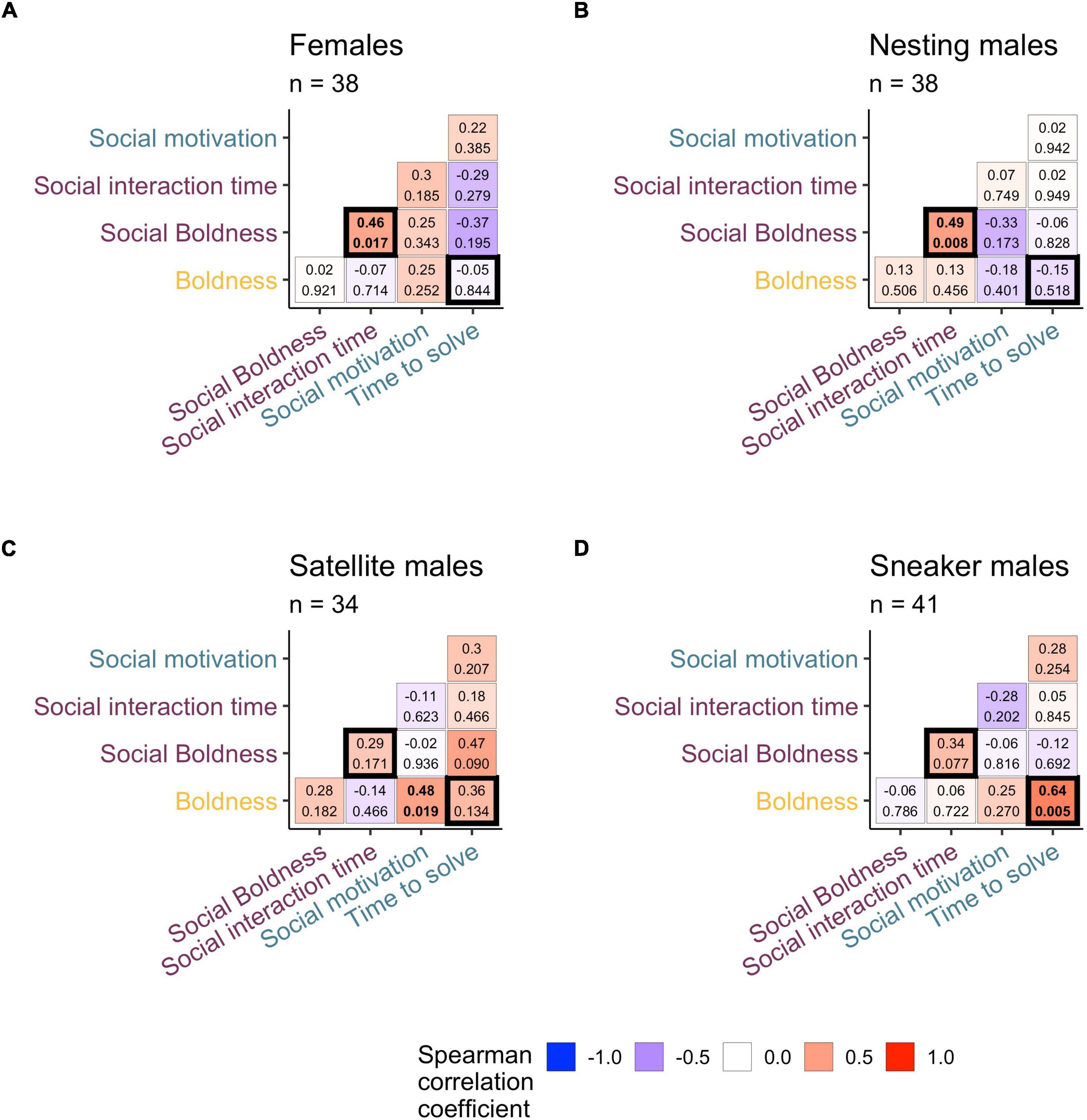
Figure 2. Heatmap of pairwise correlations (spearman) across all behavior metrics and detour problem-solving performance for females (A), nesting males (B), satellite males (C), and sneaker males (D). Each matrix box includes the spearman correlation coefficient on top and the p value on the bottom. Matrix labels are color coded t indicate which behavior assay each measurement was derived from (detour assay in teal, shoaling assay in dark purple, scototaxis in gold). Cells outlined in bold represent significant interaction terms (behavior × phenotype, p < 0.05) in generalized linear mixed models.
In addition, we find interesting patterns of co-varying social behavioral traits that differ across the phenotypes. We note that the strength of relationships between social traits differed across phenotypes. The positive relationship between social interaction time and social boldness was strongest for females (r = 0.46, p = 0.017) and nesting males (r = 0.49, p = 0.008), but less strong in sneaker males (r = 0.34, p = 0.077) and satellite males (r = 0.29, p = 0.171). Note because the social boldness measurement does not include time in the interaction zone but characterizes the time spent when not interacting, these two traits evaluate independent measures of a fish’s social and non-social behavioral traits.
Our final generalized linear mixed models that examined cognitive performance (problem-solving in detour task) as a function of behavioral responses and interactions by phenotype retained a number of behavioral measures. Phenotype (X 2 = 8.3706, p = 0.0389), the interaction between phenotype x boldness (X 2 = 10.0628, p = 0.018), and the interaction between phenotype and social boldness (X 2 = 10.5127, p = 0.0147, Figure 3A) were retained as statistically significant terms in the final model after backwards stepwise selection by AIC. We checked for multicollinearity of the significant variables using the check_collinearity function and found low VIF (variance inflation factor) scores for social boldness (VIF = 4.28) and moderate correlation for boldness (VIF = 7.02). Removal of five (three nesting males, one female, and one satellite male) potentially influential outliers for scototaxis time in white (Figure 3B) only increased the strength of these relationships [phenotype (p = 0.002), boldness (p = 0.004), interaction term of phenotype x boldness (p = 0.003), and interaction term of phenotype x social boldness (p = 0.0003)]. We identified these outliers for time in white using the identify_outliers function from the R package rstatix. Outliers were defined as values above the third quartile + 1.5 times the interquartile range, or values below the first quartile–1.5 times the interquartile range, within each phenotype.
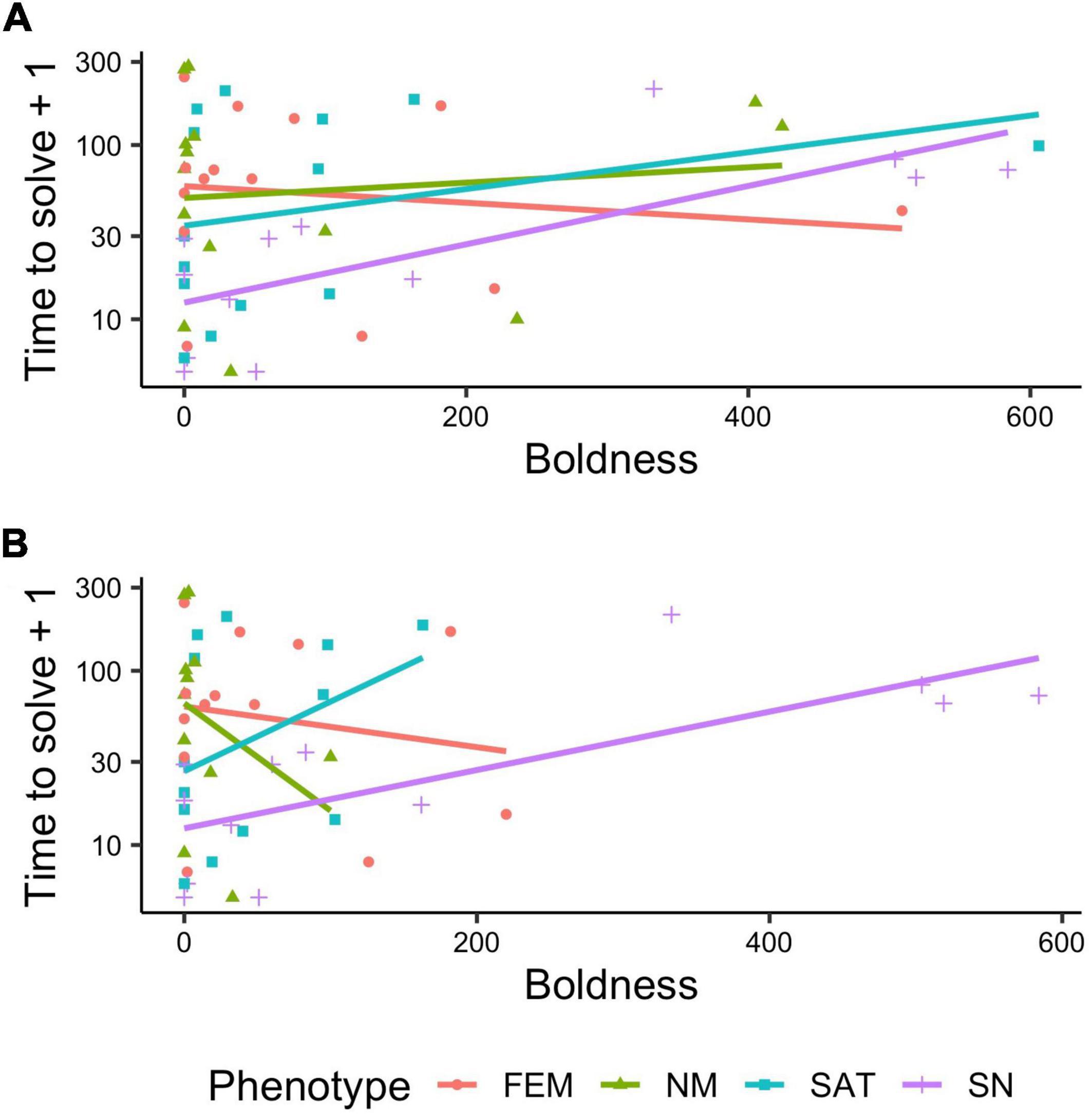
Figure 3. (A) Relationship between boldness and problem-solving speed differs by phenotype. The association between boldness tendencies [x axis, as measured via time (seconds) in white zone of a scototaxis assay] and problem-solving speed (time in seconds) in initial detour reaching task (y axis) for each phenotype: sneaker males (magenta), satellite males (blue), nesting males (green) females (orange). The significant interactions identified with GLMM modeling of boldness (time in white zone of scototaxis trials) by phenotype (p < 0.05) that predicted (A) variation in problem-solving speed (detour task) including all datapoints, (B) with outliers removed.
Visualizing Overall Cognitive-Behavioral Profiles
To visualize the overall cognitive-behavioral profile of the individuals in our experiment, we ran a discriminant function analysis (also known as linear discriminant analysis) in r package MASS (Venables and Ripley, 2002) using log-transformed behavioral metrics (log-transformed values from Figures 1D,E) and problem-solving speed (log-transformed “time to solve” from Detour Task, Figure 1G) inputs from the outlier-removed dataset (from Figure 3B). Discriminant Function assignment performance varied across the four phenotypes (Females: 0.15, Nesting Males: 0.27, Satellite males: 0.15, and Sneaker males: 0.54). Phenotypes significantly differed in DF1 scores (GLMM, X 2 = 19.33, p = 0.0002) and plotting of DF1 and DF2 scores show that sneaker males occupy a much more constrained region in this multivariate cognitive-behavioral space than the other phenotypes (Figure 4). Post-hoc Tukey Contrast analyses on the DF1 scores showed that sneaker males differed from satellite (p = 0.0201) and nesting males (p < 0.001), with a borderline difference between females (p = 0.0523).
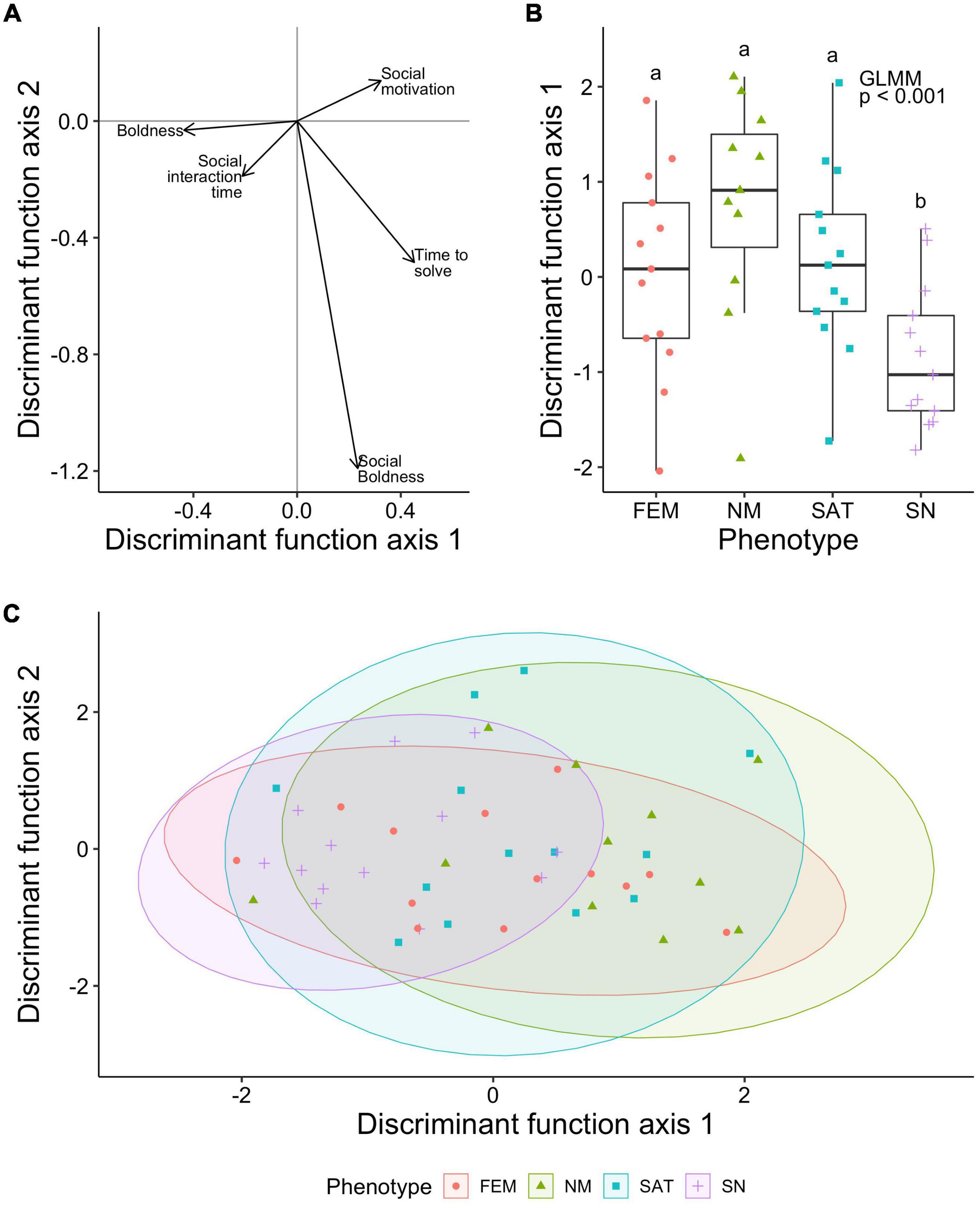
Figure 4. Cognitive-behavioral profiles differ by phenotype. (A) Loadings of the 5 input metrics into a discriminant function analysis onto the two primary axes. (B) Boxplot of the DFA axis 1 scores by phenotype along with GLMM results. Sneaker males significantly differed from the rest of the phenotypes, p < 0.001. Letters above box plots indicate statistical outcomes of tukey contrast analyses with sneaker males being different than all other phenotypes. (C) Plotting the first two axes of the DFA grouped by phenotype. Ellipses represent the 95% confidence intervals for each phenotype.
Discussion
Inter-Sexual Differences in Cognitive-Behavioral Traits
We find that behavioral and cognitive traits, and the relationships between them (cognitive-behavioral syndromes), differ more among alternative types within one sex (males) than between the sexes. Females did not differ significantly from males in any of the non-reproductive behavior attributes or cognitive measures. Contrary to our predictions, we found no detectable differences between the sexes whether evaluated univariately (Figure 1) or as multivariate suites (Figure 4). Specifically, our predictions that males and females would differ in boldness (Figure 1B) or cognitive flexibility (Figure 1G) were not borne out. Females were equally bold, or willing to take a risk and enter the more threatening zone in a non-social assay (white, brighter area in a scototaxis arena), as compared with all the male phenotypes combined. In fact, our prediction that the territorial, nesting males would be the boldest was particularly erroneous, as the nesting male turned out to be the least bold of them all in the scototaxis context.
Male and female ocellated wrasse are met with extremely different challenges during the reproductive season. Males face extreme intra-sexual selection to acquire matings either through nest defense or sneak copulations, meanwhile females’ time budget is largely devoted to foraging and inspecting different male nests. Despite such different selection pressures, we found no sexual dimorphism in problem-solving speed (initial detour task, Figure 1G). Female cognition is predicted to be enhanced in systems with multiple alternative male phenotypes due to the engagement of learning pathways when females are discriminating across types (Cummings and Ramsey, 2015). Several studies have shown females to be faster than males at cognitive tasks involving behavioral flexibility, inhibitory control, and innovation across a number of taxa [in rats: Guillamón et al. (1986); in guppies: Laland and Reader (1999) and Lucon-Xiccato and Bisazza (2017); in blue tits: Aplin et al. (2013)]. However, in other species, it is the male that outperforms females in reversal learning tasks (Fuss and Witte, 2019). Many of these studies have employed a food reward, and since female fecundity is often tied strongly to foraging that may result in the somewhat larger female-bias in performance metrics in these food-motivated tasks. Here we used a social reward. The lack of sexual dimorphism in a problem-solving task with a social reward may be driven by both sexes being equally motivated (Figures 1F,H). Other studies using a social reward in detour reaching tasks in fish have also found no evidence for sexual dimorphism in problem-solving capabilities (Wallace et al., 2020).
Perhaps most surprisingly was our lack of finding sex differences in cognitive-behavioral syndromes. Females did not exhibit distinct correlations between behavioral traits and cognitive performance that differentiated them from males as a whole (Figures 2–4). Divergent relationships between baseline anxiety and/or exploration styles with cognitive performance have been shown in birds (Titulaer et al., 2012) and fishes (Lucon-Xiccato and Dadda, 2016; Etheredge et al., 2018; Wallace et al., 2020), and have been predicted to be a product of strong sexual selection processes (Cummings, 2018). Female ocellated wrasse revealed no significant correlations between behavior metrics and problem-solving speed (Figure 2A), and their specific relationship between boldness and problem-solving speed was less pronounced than any of the alternative male phenotypes (Figures 3A,B). Comparing males and females with a multivariate cognitive-behavioral profile revealed that female ocellated wrasse were statistically similar to both nesting males and satellite males (Figure 4B), suggesting that these three reproductive roles may share similar behavioral styles and processes that shape their problem-solving abilities.
It is interesting to ponder whether the convergence of females, nesting males, and satellite males on similar cognitive-behavioral syndromes may be related to shared reproductive goals. All three of these phenotypes “choose” nests to engage in cooperative reproduction. Nesting males build and guard nests from sneakers and predators and solicit visitations from females while satellite males assist the nesting male in this process. It is noteworthy that nesting males and females expressed nearly identical patterns of social interaction and social boldness in the shoaling assay (Figures 2A,B), for these two phenotypes have the most direct negotiations for reproductive events. Determining whether or not males with similar “social interactions” with females have greater reproductive success than other males will shed light on whether there is a functional benefit for males to share similar social styles to females.
We also found no support for our final prediction that males would show greater variation in cognitive-behavioral syndromes/profiles than females due to the greater plasticity in male reproductive tactics (e.g., transitioning from sneaker to satellite or from satellite to nesting male). In contrast, we observed females having large cognitive-behavioral variation spanning a greater range than some of the male types in cognitive-behavioral space (Figures 4B,C). For instance, females and satellite males exhibited the widest range of DFA1 scores (the primary axis of the multivariate discriminant function analysis), while nesting males and sneaker males appear to occupy opposing ends of this primary axis. Do the roles of sneaking and nesting constrain these phenotypes to a specific cognitive-behavioral relationship? Or is some of this variation related to age? Sneaker males are all first year individuals, nesting males are all second year individuals, and both females and satellite males represent a mixture of both first and second year individuals.
Intra-Sexual Differences in Cognitive-Behavioral Traits
While we observed no sexual dimorphism, we did measure significant differences among the three alternative male reproductive phenotypes in boldness, problem-solving speed, and cognitive-behavioral relationships. Nesting males were the least bold of all male phenotypes, while sneaker males were the boldest (Figure 1D). In the ocellated wrasse, nesting males are the most socially dominant male type with the greatest reproductive success. Nesting males sire roughly two thirds of the offspring, while the satellite and sneakers males at the nest sire the rest (Alonzo and Heckman, 2010; Stiver et al., 2018). The observed difference in boldness levels between nesting and sneaker males is predicted by models focusing on how variation in future reproductive opportunities influences risk-aversion personality traits. Models show that greater caution leads to greater fitness in individuals with higher future reproductive opportunities, whereas greater boldness is favored in individuals with lower future reproductive expectations (Wolf et al., 2007). Hence, the difference in boldness between nesting and sneaker males may be driven by their expectations for future reproductive success.
Divergent life history strategies are expected to lead to divergence in behavioral traits. For instance, several models indicate that polymorphisms in life history strategies should lead to polymorphic behavioral traits including those involving boldness (Wolf et al., 2007; Biro and Stamps, 2008; Réale et al., 2010). Furthermore, theory predicts that the strategic differences that occur in polymorphisms can lead to differences in behavior and cognitive traits that are state-dependent and driven by variation in social interactions experienced by each phenotype in a polymorphism (Wolf and Weissing, 2010). Here we find that the three male phenotypes differ significantly in cognitive-behavioral syndromes (Figures 2–4). It is important to consider that these different male phenotypes are not genetically determined, but rather transition between roles over a lifetime. Unlike a system with genetically determined alternatively reproductive polymorphism where these differences across males would be fixed for life, we have characterized discrete differences across male types in a system where reproductive role is plastic across years. This suggests one of two possible mechanisms maintaining this variation. The first is that cognitive-behavioral syndromes can be reshaped over the lifetime of an adult male. An individual satellite male might be relatively bold in his first year, but if he transitions to a nesting male, this boldness might be reduced (Figure 1D). An individual sneaker male who transitions to a satellite male in year two may trend toward greater social motivation (Figure 1F) while becoming slower at problem-solving (Figure 1G). Alternatively, males may not be changing their behavioral attributes over time but rather the transition of a male from one tactic to another is predicted by behavioral characteristics. For instance, satellite males that are less bold may be the individuals who transition to become nesting males; or sneaker males that are slower at problem-solving might become the satellite males in year two. Only longitudinal studies on individual males can determine which process is maintaining this variation.
Why Are Sneakers so Different From the Rest?
Overall, we found that the sneaker male phenotype differed significantly from all others in boldness (Figure 1D), problem-solving (Figure 1G) and behavioral predictors associated with this cognitive trait (Figures 2, 3). Furthermore, the sneaker male was the only phenotype to significantly differ from all others at a multivariate level, occupying a unique, and potentially more constrained, niche in cognitive-behavioral space (Figures 4B,C). The fast detour solution speeds exhibited by sneakers suggests that males that adopt the sneaker reproductive tactic are better able to innovate or exhibit greater inhibitory control than nesting males that court females, build nests, and defend them. This is contrary to our predictions that the paternal, nesting males would outperform the sneaker male in the inhibitory control aspects of a detour reaching task, as predicted in other paternal care fish systems [e.g., sticklebacks, Keagy et al. (2019)]. However, in fish species with alternative male “sneaker” phenotypes, sneaker males have been shown to have either equal performance in spatial learning abilities relative to territorial dominant males [Rose bitterling, Smith et al. (2015)] or faster at learning associative foraging tasks than the courting phenotype [Xiphophorus multilineatus, Griebling et al. (2020)].
When we combine all measurements from cognition and behavioral assays, we find that the sneaker males are significantly different in cognitive-behavioral syndromes than all other phenotypes (Figure 4). This finding lends support for our predictions that male ARTs would differ in terms of cognitive-behavioral syndromes. However, why are ocellated wrasse sneaker males so different from other male roles as well as females? Is this related to the younger age of sneakers from all other phenotypes, or is the art of “sneaking” a more singular task that involves a more restrictive combination of cognitive and behavioral attributes? A third possibility, which we discuss below, is that the social position of sneaker males in the ocellated wrasse hierarchy leads to divergent coping styles with concomitant variation in behavior and cognition.
Social Dominance, Coping Style, and Cognitive Performance
Here we show the socially dominant ocellated wrasse nesting male are significantly less fast at problem solving than the more subordinate sneaker males. This pattern of the socially dominant exhibiting inferior novel problem -solving abilities than social subordinates has been found in some taxa [crab-eating macaques: Bunnell and Perkins (1980); song sparrows: Boogert et al. (2011)], but not others (Keagy et al., 2009; Chen et al., 2019). Dominant individuals do not underperform in all cognitive tasks. In fact, in many primates (Reader and Laland, 2001), dogs (Pongrácz et al., 2012), and birds (Pravosudov et al., 2003; Langley et al., 2018; Gomes et al., 2020) it is the dominant individuals that exhibit greater associative learning efficiency. However, when environments change (reversal learning) or in novel environments where inhibitory control is required (detour reaching tasks) or innovation it is often the more subordinant individuals that perform better (Reader and Laland, 2001; Mazza et al., 2018).
The tendency for the more aggressive nesting males to be the slowest to solve the detour task is consistent with a proactive coping style. A proactive coping style is associated with highly aggressive individuals with higher testosterone, lower HPA axis, and tendencies to be less attentive to changes in the environment than individuals with a reactive coping style (Koolhaas et al., 1999). Previous physiological measurements of this phenotype have documented higher 11-KT (Nugent et al., 2016), greater aggression (Stiver et al., 2015), and lower HPA activity [lower cortisol, Nugent et al. (2016)] than sneaker males. The relative lack of movement by the nesting males in the scototaxis assays (e.g., decreased “boldness” in a non-social assay) might be similar to the tonic immobility that aggressive, proactive chickens exhibit in open field experiments (Jones et al., 1995). Proactive individuals are predicted to have less inhibitory control and to be less attentive to environmental conditions than reactive individuals. In novel cognitive tasks that involve inhibitory control (e.g., our detour task), proactive types are likely to spend more time pursuing the reward with persistent action (more time spent at glass partition), than identifying novel means to circumvent the problem (Sih and Del Giudice, 2012; Chung et al., 2017; Gomes et al., 2020). The slower growth rates observed in sneaker males along with their higher cortisol levels relative to other ocellated wrasse males and females (Nugent et al., 2016), coupled with the fastest problem-solving times in the novel environment (detour task) are all characteristic of a reactive coping style (Koolhaas et al., 1999; Biro and Stamps, 2008; Réale et al., 2010; Krams et al., 2018; Mazza et al., 2018). Reactive coping styles are expected to perform better at inhibitory control tasks, such as detour reaching, because of their ability to inhibit responses toward irrelevant stimuli (Sih and Del Giudice, 2012), and studies have found negative correlations between boldness and reversal learning performance in a variety of taxa (Mazza et al., 2018). Consistent with this pattern, we found that the least bold sneakers exhibited the fastest problem-solving tendencies (Figures 2D, 3).
Sexual Selection as a Driver of Plasticity in Coping Styles and Cognitive-Behavioral Syndromes
Our finding that alternative male reproductive phenotypes differ in their cognitive-behavioral syndromes and likely coping styles as they transition to different mating tactics suggests the power of sexual selection to shape traits well beyond reproduction. This also suggests intra-individual plasticity in both coping styles and cognitive-behavioral syndromes. Earlier research demonstrated a strong genetic control of both coping-styles (Henry and Stephens, 1977; Koolhaas et al., 1999; Carere et al., 2003; Veenema et al., 2003; Kralj-Fišer et al., 2007) as well as personality or behavioral types (Dingemanse et al., 2002, 2003; Drent et al., 2003; Dingemanse and Réale, 2005; Groothuis and Carere, 2005) in a number of different systems. Here we are finding patterns that suggest the possibility that individual males will transition their behavior, cognition, and coping style physiology to adopt an alternative reproductive role within a lifetime.
As male ocellated wrasse transition from satellite to nesting male, or from sneaker male to satellite male they are putatively shifting behavioral tendencies, problem-solving performance, and shifting the relationship between them to engage in different reproductive roles (Figures 1–4). In addition, they are also transitioning their sex steroid and stress hormone responses. In essence, their transition across alternative reproductive tactics produces a concomitant shift in coping styles. What are the mechanisms regulating this dynamic co-variation in traits? Carere and colleagues (Carere et al., 2010; Carere and Locurto, 2011) put forth multiple possible causal relationships between copying style physiology, behavior, and cognitive responses including the pleiotropic effects of glucocorticoids, direct effects of behavior, and social stress. Carere suggests that social stress is the fundamental basis tying together behavioral types and cognitive performance because personalities likely evolved to navigate social interactions. It is easy to imagine that the three alternative ocellated wrasse male reproductive phenotypes engage in very different forms of social stress, and these different social stressors may indeed activate the glucocorticoid (HPA axis) in different ways. Nesting males engage with all phenotypes with very different behaviors: females (via courtship), satellites (via “encouragement” to serve him), and sneaker males (via actively chasing them away). Meanwhile sneaker males engage with other phenotypes in similar ways that involve covert or hidden actions; and are accustomed to receiving similar treatment (being chased) by nearly all phenotypes. Perhaps this is one reason why their cognitive-behavioral syndromes are the tightest [show the least variation (Figures 4B,C) and strongest correlations (Figures 2, 3)] across all phenotypes.
In summary, we found striking differences in cognitive-behavioral profiles among male types and no evidence of divergence in these profiles between the sexes. Given the fundamental importance of sex differences to so many aspects of biology, it is perhaps surprising that males and females did not differ more in learning, cognition or behavioral profiles in this species. However, on the other hand, when it comes to reproduction males and females must engage in social interactions that require elements of boldness, sociality, and problem-solving. When considering the differences among male types in cognitive behavioral profiles, while we did find striking differences, these differences were also surprising. In systems that include both dominant and subordinate male alternative types, perhaps we should expect great divergence in non-reproductive behavior and cognitive traits as alternative male strategies evolve to circumvent the most successful tactic. Our results show that while sexual selection does shape these patterns, further theoretical and empirical work is clearly needed if we wish to understand in general how sexual selection shapes cognition and behavior or organisms broadly, rather than primarily in the context of reproductive traits and behaviors. Finally, our results demonstrates the immense plasticity that is possible not only among individuals that share a genome, but also that adaptive change in cognition, learning and behavior are possible in striking ways over the lifetime of an individual.
Data Availability Statement
The original contributions presented in this study are included in the article/Supplementary Material, further inquiries can be directed to the corresponding authors.
Ethics Statement
The animal study was reviewed and approved by UCSC IACUC.
Author Contributions
SA and MC conceptualized the research plan. SA provided the funding. SM-R completed the data collection. MC conducted the analyses and drafted the initial version of the manuscript. All authors developed methodology, began experimentation, contributed to the writing and revising of the manuscript, and approved the submitted version.
Funding
SA and SM-R were supported by the National Science Foundation (Grant Number: IOS-1655297 to SA) and funds from the University of California Santa Cruz to SA. MC was supported by the National Science Foundation (Grant Number: IOS-1911826).
Conflict of Interest
The authors declare that the research was conducted in the absence of any commercial or financial relationships that could be construed as a potential conflict of interest.
Publisher’s Note
All claims expressed in this article are solely those of the authors and do not necessarily represent those of their affiliated organizations, or those of the publisher, the editors and the reviewers. Any product that may be evaluated in this article, or claim that may be made by its manufacturer, is not guaranteed or endorsed by the publisher.
Acknowledgments
We thank Kelly Stiver and Jen Hellmann for their assistance in the field as well as Dr. Pierre Lejeune, Alexandre Volpon, Cyril Steibel, and the entire staff at the Station de Recherches Sous-Marines et Oceanographique (STARESO). We thank the following undergraduates at the University of California Santa Cruz: Elin Binck, Yasmine Elshenawi, Carissa Gomez, Aaron Gormley, Lauren Kolyer, Andrew O’Neil, Ari Throckmorton, Lina Vu, and Albert Reyes from the University of Texas at Austin for scoring the videos of the assays. We are also very grateful to Dr. Luke Reding for assistance with figures and statistical analysis.
Supplementary Material
The Supplementary Material for this article can be found online at: https://www.frontiersin.org/articles/10.3389/fevo.2022.929595/full#supplementary-material
References
Alonzo, S. H. (2004). Uncertainty in territory quality affects the benefits of usurpation in a Mediterranean wrasse. Behav. Ecol. 15, 278–285.
Alonzo, S. H. (2008). Female mate choice copying affects sexual selection in wild populations of the ocellated wrasse. Anim. Behav. 75, 1715–1723.
Alonzo, S. H., and Heckman, K. L. (2010). The unexpected but understandable dynamics of mating, paternity and paternal care in the ocellated wrasse. Proc. Biol. Sci. 277, 115–122. doi: 10.1098/rspb.2009.1425
Alonzo, S. H., Taborsky, M., and Wirtz, P. (2000). Male alternative reproductive behaviours in a Mediterranean wrasse, Symphodus ocellatus: evidence from otoliths for multiple life-history pathways. Evol. Ecol. Res. 2, 997–1007.
Alonzo, S. H., Stiver, K. A., and Marsh-Rollo, S. E. (2016). Ovarian fluid allows directional cryptic female choice despite external fertilization. Nat. Commun. 7:12452. doi: 10.1038/ncomms12452
Alonzo, S. H., and Warner, R. R. (2000a). Dynamic games and field experiments examining intra- and intersexual conflict: explaining counterintuitive mating behavior in a Mediterranean wrasse. Symphodus ocellatus. Behav. Ecol. 11, 56–70.
Alonzo, S. H., and Warner, R. R. (2000b). Allocation to Mate Guarding or Increased Sperm Production in a Mediterranean Wrasse. Am. Nat. 156, 266–275.
Aplin, L. M., Sheldon, B. C., and Morand-Ferron, J. (2013). Milk bottles revisited: social learning and individual variation in the blue tit. Cyanistes caeruleus. Anim. Behav. 85, 1225–1232.
Astié, A. A., Kacelnik, A., and Reboreda, J. C. (1998). Sexual differences in memory in shiny cowbirds. Anim. Cogn. 1, 77–82. doi: 10.1007/s100710050011
Biro, P. A., and Stamps, J. A. (2008). Are animal personality traits linked to life-history productivity? Trends Ecol. Evol. 23, 361–368.
Boogert, N. J., Anderson, R. C., Peters, S., Searcy, W. A., and Nowicki, S. (2011). Song repertoire size in male song sparrows correlates with detour reaching, but not with other cognitive measures. Anim. Behav. 81, 1209–1216.
Brooks, M. E., Kristensen, K., Van Benthem, K. J., Magnusson, A., Berg, C. W., Nielsen, A., et al. (2017). glmmTMB balances speed and flexibility among packages for zero-inflated generalized linear mixed modeling. R J. 9, 378–400.
Brust, V., and Guenther, A. (2017). Stability of the guinea pigs personality - cognition - linkage over time. Behav. Process. 34, 4–11. doi: 10.1016/j.beproc.2016.06.009
Bunnell, B. N., and Perkins, M. N. (1980). Performance correlates of social behavior and organization: social rank and complex problem solving in crab-eating macaques (M. fascicularis). Primates 21, 515–523. doi: 10.1007/BF02373840
Carazo, P., Noble, D. W. A., Chandrasoma, D., and Whiting, M. J. (2014). Sex and boldness explain individual differences in spatial learning in a lizard. Proc. R. Soc. B Biol. Sci. 281:20133275. doi: 10.1098/rspb.2013.3275
Carere, C., Caramaschi, D., and Fawcett, T. W. (2010). Covariation between personalities and individual differences in coping with stress: converging evidence and hypotheses. Curr. Zool. 56, 728–740.
Carere, C., Groothuis, T. G. G., Möstl, E., Daan, S., and Koolhaas, J. M. (2003). Fecal corticosteroids in a territorial bird selected for different personalities: daily rhythm and the response to social stress. Horm. Behav. 43, 540–548. doi: 10.1016/s0018-506x(03)00065-5
Carere, C., and Locurto, C. (2011). Interaction between animal personality and animal cognition. Curr. Zool. 57, 491–498.
Chen, J., Zou, Y., Sun, Y. H., and Ten Cate, C. (2019). Problem-solving males become more attractive to female budgerigars. Science 363, 166–167. doi: 10.1126/science.aau8181
Chung, M., Goulet, C. T., Michelangeli, M., Melki-Wegner, B., Wong, B. B. M., and Chapple, D. G. (2017). Does personality influence learning? A case study in an invasive lizard. Oecologia 185, 641–651. doi: 10.1007/s00442-017-3975-4
Cole, E. F., Morand-Ferron, J., Hinks, A. E., and Quinn, J. L. (2012). Cognitive ability influences reproductive life history variation in the wild. Curr. Biol. 22, 1808–1812. doi: 10.1016/j.cub.2012.07.051
Cole, E. F., and Quinn, J. L. (2012). Personality and problem-solving performance explain competitive ability in the wild. Proc. Biol. Sci. 279, 1168–1175. doi: 10.1098/rspb.2011.1539
Cummings, M. E. (2018). Sexual conflict and sexually dimorphic cognition—reviewing their relationship in poeciliid fishes. Behav. Ecol. Sociobiol. 72:73.
Cummings, M. E., and Ramsey, M. E. (2015). Mate choice as social cognition: predicting female behavioral and neural plasticity as a function of alternative male reproductive tactics. Curr. Opin. Behav. Sci. 6, 125–131.
Dean, R., Wright, A. E., Marsh-Rollo, S. E., Nugent, B. M., Alonzo, S. H., and Mank, J. E. (2017). Sperm competition shapes gene expression and sequence evolution in the ocellated wrasse. Mol. Ecol. 26, 505–518. doi: 10.1111/mec.13919
Dingemanse, N. J., Both, C., Drent, P. J., van Oers, K., and van Noordwijk, A. J. (2002). Repeatability and heritability of exploratory behaviour in great tits from the wild. Anim. Behav. 64, 929–938.
Dingemanse, N. J., Both, C., van Noordwijk, A. J., Rutten, A. L., and Drent, P. J. (2003). Natal dispersal and personalities in great tits (Parus major). Proc. Biol. Sci. 270, 741–747. doi: 10.1098/rspb.2002.2300
Dingemanse, N. J., and Réale, D. (2005). Natural selection and animal personality. Behaviour 142, 1159–1184.
Dougherty, L. R., and Guillette, L. M. (2018). Linking personality and cognition: a meta-analysis. Philos. Trans. Soc. Lond. B Biol. Sci. 373:20170282.
Drent, P. J., van Oers, K., and van Noordwijk, A. J. (2003). Realized heritability of personalities in the great tit (Parus major). Proc. Biol. Sci. 270, 45–51. doi: 10.1098/rspb.2002.2168
Etheredge, R. I., Ian Etheredge, R., Avenas, C., Armstrong, M. J., and Cummings, M. E. (2018). Sex-specific cognitive-behavioural profiles emerging from individual variation in numerosity discrimination in Gambusia affinis. Anim. Cogn. 21, 37–53. doi: 10.1007/s10071-017-1134-2
Exnerová, A., Svádová, K. H., Fucíková, E., Drent, P., and Stys, P. (2010). Personality matters: individual variation in reactions of naive bird predators to aposematic prey. Proc. Biol. Sci. 277, 723–728. doi: 10.1098/rspb.2009.1673
Fairbairn, D. J. (2013). Odd Couples: Extraordinary Differences between the Sexes in the Animal Kingdom. Princeton: Princeton University Press.
Fairbairn, D. J., Blanckenhorn, W. U., and Székely, T. (2007). Sex, Size and Gender Roles. Oxford: Oxford University Press.
FitzGerald, R. W. (1986). Sex Differences in Spatial Ability: an Evolutionary Hypothesis and Test. Am. Nat. 127, 74–88.
Fuss, T., and Witte, K. (2019). Sex differences in color discrimination and serial reversal learning in mollies and guppies. Curr. Zool. 65, 323–332. doi: 10.1093/cz/zoz029
Galea, L. A., Kavaliers, M., and Ossenkopp, K. P. (1996). Sexually dimorphic spatial learning in meadow voles Microtus pennsylvanicus and deer mice Peromyscus maniculatus. J. Exp. Biol. 199, 195–200. doi: 10.1242/jeb.199.1.195
Gomes, A. C. R., Guerra, S., Silva, P. A., Marques, C. I., Trigo, S., Boogert, N. J., et al. (2020). Proactive common waxbills make fewer mistakes in a cognitive assay, the detour-reaching task. Behav. Ecol. Sociobiol. 74:31.
Gray, J. A. (1964). “Strength of the nervous system and levels of arousal: A reinterpretation,” in Pavlov’s Typology, ed. J. A. Gray (New York: Macmillan), 289.
Griebling, H. J., Rios-Cardenas, O., Abbott, J., and Morris, M. R. (2020). A study of tactical and sexual dimorphism in cognition with insights for sexual conflict. Anim. Behav. 170, 43–50.
Groothuis, T. G. G., and Carere, C. (2005). Avian personalities: characterization and epigenesis. Neurosci. Biobehav. Rev. 29, 137–150. doi: 10.1016/j.neubiorev.2004.06.010
Guigueno, M. F., Snow, D. A., MacDougall-Shackleton, S. A., and Sherry, D. F. (2014). Female cowbirds have more accurate spatial memory than males. Biol. Lett. 10:20140026. doi: 10.1098/rsbl.2014.0026
Guillamón, A., Valencia, A., Calés, J. M., and Segovia, S. (1986). Effects of early postnatal gonadal steroids on the successive conditional discrimination reversal learning in the rat. Physiol. Behav. 38, 845–849. doi: 10.1016/0031-9384(86)90052-1
Guillette, L. M., Naguib, M., and Griffin, A. S. (2017). Individual differences in cognition and personality. Behav. Process. 134, 1–3.
Guillette, L. M., Reddon, A. R., Hoeschele, M., and Sturdy, C. B. (2011). Sometimes slower is better: slow-exploring birds are more sensitive to changes in a vocal discrimination task. Proc. Biol. Sci. 278, 767–773. doi: 10.1098/rspb.2010.1669
Guillette, L. M., Reddon, A. R., Hurd, P. L., and Sturdy, C. B. (2009). Exploration of a novel space is associated with individual differences in learning speed in black-capped chickadees. Poecile atricapillus. Behav. Process. 82, 265–270. doi: 10.1016/j.beproc.2009.07.005
Hauber, M. E., Clayton, N. S., Kacelnik, A., Reboreda, J. C., and DeVoogd, T. J. (1999). Sexual dimorphism and species differences in HVC volumes of cowbirds. Behav. Neurosci. 113, 1095–1099.
Healy, S. D., Braham, S. R., and Braithwaite, V. A. (1999). Spatial working memory in rats: no differences between the sexes. Proc. Biol. Sci. 266, 2303–2308.
Henry, J. P., and Stephens, P. M. (1977). The social environment and essential hypertension in mice: possible role of the innervation of the adrenal cortex. Prog. Brain Res. 47, 263–276. doi: 10.1016/S0079-6123(08)62731-4
Hollis, B., and Kawecki, T. J. (2014). Male cognitive performance declines in the absence of sexual selection. Proc. Biol. Sci. 281:20132873. doi: 10.1098/rspb.2013.2873
Jacquin, L., Petitjean, Q., Côte, J., Laffaille, P., and Jean, S. (2020). Effects of Pollution on Fish Behavior, Personality, and Cognition: some Research Perspectives. Front. Ecol. Evol. 8:86. doi: 10.3389/fevo.2020.00086
Jonasson, Z. (2005). Meta-analysis of sex differences in rodent models of learning and memory: a review of behavioral and biological data. Neurosci. Biobehav. Rev. 28, 811–825. doi: 10.1016/j.neubiorev.2004.10.006
Jones, C. M., Braithwaite, V. A., and Healy, S. D. (2003). The evolution of sex differences in spatial ability. Behav. Neurosci. 117, 403–411.
Jones, R. B., Blokhuis, H. J., and Beuving, G. (1995). Open-field and tonic immobility responses in domestic chicks of two genetic lines differing in their propensity to feather peck. Br. Poult. Sci. 36, 525–530. doi: 10.1080/00071669508417798
Kassambara, A., and Kassambara, M. A. (2019). Ggcorrplot: Visualization of a Correlation Matrix using ‘ggplot2’. R Package Version 0.1.3. Available online at: https://CRAN.R-project.org/package=ggcorrplot (accessed April 4, 2021).
Keagy, J., Minter, R., and Tinghitella, R. M. (2019). Sex differences in cognition and their relationship to male mate choice. Curr. Zool. 65, 285–293.
Keagy, J., Savard, J.-F., and Borgia, G. (2009). Male satin bowerbird problem-solving ability predicts mating success. Anim. Behav. 78, 809–817.
Koolhaas, J. M., Korte, S. M., De Boer, S. F., Van Der Vegt, B. J., Van Reenen, C. G., Hopster, H., et al. (1999). Coping styles in animals: current status in behavior and stress-physiology. Neurosci. Biobehav. Rev. 23, 925–935. doi: 10.1016/s0149-7634(99)00026-3
Kotrschal, A., Corral-Lopez, A., Amcoff, M., and Kolm, N. (2015). A larger brain confers a benefit in a spatial mate search learning task in male guppies. Behav. Ecol. 26, 527–532. doi: 10.1093/beheco/aru227
Kralj-Fišer, S., Scheiber, I. B. R., Blejec, A., Moestl, E., and Kotrschal, K. (2007). Individualities in a flock of free-roaming greylag geese: behavioral and physiological consistency over time and across situations. Horm. Behav. 51, 239–248. doi: 10.1016/j.yhbeh.2006.10.006
Krams, I., Trakimas, G., Kecko, S., Elferts, D., Krams, R., Luoto, S., et al. (2018). Linking organismal growth, coping styles, stress reactivity, and metabolism via responses against a selective serotonin reuptake inhibitor in an insect. Sci. Rep. 8:8599. doi: 10.1038/s41598-018-26722-9
Laland, K. N., and Reader, S. M. (1999). Foraging innovation in the guppy. Anim. Behav. 57, 331–340.
Langley, E. J. G., van Horik, J. O., Whiteside, M. A., Beardsworth, C. E., and Madden, J. R. (2018). The relationship between social rank and spatial learning in pheasants, Phasianus colchicus: cause or consequence? PeerJ 6:e5738.
Lejeune, P. (1985). Le comportement social des Labridés mediterranéens: etude écoéthologique des comportements reproducteur et sociaux des Labridae méditerranéens des genres Symphodus (Rafinesque 1810) et Coris (Lacepede 1802). Cah. Ethol. Appl. 5, 1–208.
Locurto, C. (2007). Individual differences and animal personality. Comp. Cogn. Behav. Rev. 2, 67–78.
Lucon-Xiccato, T., and Bisazza, A. (2014). Discrimination reversal learning reveals greater female behavioural flexibility in guppies. Biol. Lett. 10:20140206.
Lucon-Xiccato, T., and Bisazza, A. (2016). Male and female guppies differ in speed but not in accuracy in visual discrimination learning. Anim. Cogn. 19, 733–744.
Lucon-Xiccato, T., and Bisazza, A. (2017). Individual differences in cognition among teleost fishes. Behav. Process. 141, 184–195.
Lucon-Xiccato, T., and Dadda, M. (2016). Guppies Show Behavioural but Not Cognitive Sex Differences in a Novel Object Recognition Test. PLoS One 11:e0156589. doi: 10.1371/journal.pone.0156589
Lynch, K. S., Ramsey, M. E., and Cummings, M. E. (2012). The mate choice brain: comparing gene profiles between female choice and male coercive poeciliids. Genes Brain Behav. 11, 222–229. doi: 10.1111/j.1601-183X.2011.00742.x
Magurran, A. E., and Macias Garcia, C. (2000). Sex differences in behaviour as an indirect consequence of mating system. J. Fish Biol. 57, 839–857.
Mamuneas, D., Spence, A. J., Manica, A., and King, A. J. (2014). Bolder stickleback fish make faster decisions, but they are not less accurate. Behav. Ecol. 26, 91–96.
Maximino, C., de Brito, T. M., de Mattos Dias, C. A. G., Gouveia, A., and Morato, S. (2010). Scototaxis as anxiety-like behavior in fish. Nat. Protoc. 5, 209–216.
Mazza, V., Eccard, J. A., Zaccaroni, M., Jacob, J., and Dammhahn, M. (2018). The fast and the flexible: cognitive style drives individual variation in cognition in a small mammal. Anim. Behav. 137, 119–132.
Munday, P. L., Wilson White, J., and Warner, R. R. (2006). A social basis for the development of primary males in a sex-changing fish. Proc. Biol. Sci. 273, 2845–2851. doi: 10.1098/rspb.2006.3666
Neff, B. D., and Svensson, E. I. (2013). Polyandry and alternative mating tactics. Philos. Trans. R. Soc. Lond. B Biol. Sci. 368:20120045.
Nugent, B. M., Stiver, K. A., Alonzo, S. H., and Hofmann, H. A. (2016). Neuroendocrine profiles associated with discrete behavioural variation in Symphodus ocellatus, a species with male alternative reproductive tactics. Mol. Ecol. 25, 5212–5227. doi: 10.1111/mec.13828
Nugent, B. M., Stiver, K. A., Hofmann, H. A., and Alonzo, S. H. (2019). Experimentally induced variation in neuroendocrine processes affects male reproductive behaviour, sperm characteristics and social interactions. Mol. Ecol. 28, 3464–3481. doi: 10.1111/mec.14999
Oliveira, R. F., Taborsky, M., and Jane Brockmann, H. (2008). Alternative Reproductive Tactics: An Integrative Approach. Cambridge: Cambridge University Press.
Pavlov, I. P. (1948). “Scientific study of the so-called psychical processes in the higher animals, 1906,” in Readings in the History of Psychology, ed. W. Dennis (New York, NY: Appleton-Century-Crofts), 425–438.
Pawlowski, B., and Dunbar, R. I. M. (1999). Withholding Age as Putative Deception in Mate Search Tactics. Evol. Hum. Behav. 20, 53–69.
Pennell, M. W., Mank, J. E., and Peichel, C. L. (2018). Transitions in sex determination and sex chromosomes across vertebrate species. Mol. Ecol. 27, 3950–3963.
Pongrácz, P., Bánhegyi, P., and Miklósi, Á (2012). When rank counts — dominant dogs learn better from a human demonstrator in a two-action test. Behaviour 149, 111–132.
Pravosudov, V. V., Mendoza, S. P., and Clayton, N. S. (2003). The relationship between dominance, corticosterone, memory, and food caching in mountain chickadees (Poecile gambeli). Horm. Behav. 44, 93–102. doi: 10.1016/s0018-506x(03)00119-3
Ramsey, M. E., Vu, W., and Cummings, M. E. (2014). Testing synaptic plasticity in dynamic mate choice decisions: n-methyl D-aspartate receptor blockade disrupts female preference. Proc. Biol. Sci. 281:20140047. doi: 10.1098/rspb.2014.0047
Reader, S. M., and Laland, K. N. (2001). Primate innovation: sex, age and social rand differences. Int. J. Primatol. 22, 787–805. doi: 10.1001/jamapediatrics.2018.3074
Réale, D., Garant, D., Humphries, M. M., Bergeron, P., Careau, V., and Montiglio, P.-O. (2010). Personality and the emergence of the pace-of-life syndrome concept at the population level. Philos. Trans. R Soc. Lond. B Biol. Sci. 365, 4051–4063.
Shuster, S. M., and Wade, M. J. (2019). Mating Systems and Strategies. Princeton: Princeton University Press.
Sih, A., and Del Giudice, M. (2012). Linking behavioural syndromes and cognition: a behavioural ecology perspective. Philos. Trans. R. Soc. Lond. B Biol. Sci. 367, 2762–2772.
Smith, B. R., and Blumstein, D. T. (2008). Fitness consequences of personality: a meta-analysis. Behav. Ecol. 19, 448–455.
Smith, C., Philips, A., and Reichard, M. (2015). Cognitive ability is heritable and predicts the success of an alternative mating tactic. Proc. Biol. Sci. 282:20151046. doi: 10.1098/rspb.2015.1046
Stiver, K. A., and Alonzo, S. H. (2013). Does the Risk of Sperm Competition Help Explain Cooperation between Reproductive Competitors? A Study in the Ocellated Wrasse (Symphodus ocellatus). Am. Nat. 181, 373–368. doi: 10.1086/669149
Stiver, K. A., Harris, R. M., Townsend, J. P., Hofmann, H. A., and Alonzo, S. H. (2015). Neural Gene Expression Profiles and Androgen Levels Underlie Alternative Reproductive Tactics in the Ocellated Wrasse,Symphodus ocellatus. Ethology 121, 152–167.
Stiver, K. A., Kindsvater, H. K., Tamburello, N., Heckman, K. L., Klein, J., and Alonzo, S. H. (2018). Intentional multiple mating by females in a species where sneak fertilization circumvents female choice for parental males. J. Fish Biol. 93, 324–333. doi: 10.1111/jfb.13766
Taborsky, M., Hudde, B., and Wirtz, P. (1987). Reproductive Behaviour and Ecology of Symphodus (Crenilabrus) Ocellatus, a European Wrasse With Four Types of Male Behaviour. Behaviour 102, 82–118.
Titulaer, M., van Oers, K., and Naguib, M. (2012). Personality affects learning performance in difficult tasks in a sex-dependent way. Anim. Behav. 102, 82–117.
Triki, Z., and Bshary, R. (2021). Sex differences in the cognitive abilities of a sex-changing fish species Labroides dimidiatus. R. Soc. Open Sci. 8:210239. doi: 10.1098/rsos.210239
Veenema, A. H., Meijer, O. C., de Kloet, E. R., Koolhaas, J. M., and Bohus, B. G. (2003). Differences in basal and stress-induced HPA regulation of wild house mice selected for high and low aggression. Horm. Behav. 43, 197–204.
Venables, W. N., and Ripley, B. D. (2002). Modern Applied Statistics with S. Statistics and Computing. New York, NY: Springer.
Wallace, K. J., Rausch, R. T., Ramsey, M. E., and Cummings, M. E. (2020). Sex differences in cognitive performance and style across domains in mosquitofish (Gambusia affinis). Anim. Cogn. 23, 655–669. doi: 10.1007/s10071-020-01367-2
Wang, S. M. T., Ramsey, M. E., and Cummings, M. E. (2014). Plasticity of the mate choice mind: courtship evokes choice-like brain responses in females from a coercive mating system. Genes Brain Behav. 13, 365–375. doi: 10.1111/gbb.12124
Warner, R. R., and Lejeune, P. (1985). Sex change limited by paternal care: a test using four Mediterranean labrid fishes, genus Symphodus. Mar. Biol. 87, 89–99.
Wolf, M., van Doorn, G. S., Leimar, O., and Weissing, F. J. (2007). Life-history trade-offs favour the evolution of animal personalities. Nature 447, 581–584.
Keywords: coping styles, intrasexual selection, alternative mating tactics, cognitive-behavioral syndromes, cognitive flexibility
Citation: Cummings ME, Marsh-Rollo SE and Alonzo SH (2022) Cognitive-Behavioral Divergence Is Greater Across Alternative Male Reproductive Phenotypes Than Between the Sexes in a Wild Wrasse. Front. Ecol. Evol. 10:929595. doi: 10.3389/fevo.2022.929595
Received: 27 April 2022; Accepted: 20 June 2022;
Published: 14 July 2022.
Edited by:
Sasha Raoul Xola Dall, University of Exeter, United KingdomReviewed by:
Takeshi Takegaki, Nagasaki University, JapanAnuradha Bhat, Indian Institute of Science Education and Research Kolkata, India
Copyright © 2022 Cummings, Marsh-Rollo and Alonzo. This is an open-access article distributed under the terms of the Creative Commons Attribution License (CC BY). The use, distribution or reproduction in other forums is permitted, provided the original author(s) and the copyright owner(s) are credited and that the original publication in this journal is cited, in accordance with accepted academic practice. No use, distribution or reproduction is permitted which does not comply with these terms.
*Correspondence: Molly E. Cummings, bWN1bW1pbmdzQGF1c3Rpbi51dGV4YXMuZWR1; Suzanne H. Alonzo, c2hhbG9uem9AdWNzYy5lZHU=