- 1Department of Geosciences, Virginia Polytechnic and State University, Blacksburg, VA, United States
- 2School of Freshwater Sciences, University of Wisconsin, Milwaukee, WI, United States
- 3Centre for Earth Observation Science, University of Manitoba, Winnipeg, MB, Canada
- 4Center for Conservation and Sustainable Development, Missouri Botanical Garden, St. Louis, MO, United States
- 5Department of Anthropology, Washington University in St. Louis, St. Louis, MO, United States
Cave guano deposits represent a relatively untapped paleoecological archive that can provide information about past vegetation, climate, and bat diet over several millennia. Recent research suggests that carbon isotope values (δ13C) measured in guano accumulations from insectivorous bats reflect the relative abundance of C3 and C4 plants on the landscape while nitrogen isotope values (δ15N) may reflect precipitation amount. Together, these proxies can provide useful information for restoration practitioners seeking to understand how plant species composition has changed over time in relation to climate and land use. To better calibrate these proxies for use in restoration, we compared instrumental records of precipitation and satellite imagery of vegetation with isotope values measured in modern bat guano from Mary Lawson Cave, a large limestone cavern located in central Missouri. Mary Lawson Cave hosts a maternity colony of insectivorous gray bats (Myotis grisescens), and as such, contains significant guano accumulations. In the fall of 2018, we collected a 60 cm long guano core that dates to 1999 cal AD at its base. Guano core δ13C values decrease from the base toward the surface (from ~-26 to -27‰) whereas δ15N values increase toward the surface even after accounting for ammonia volatilization (from ~3 to 5‰). Presently, the landscape around Mary Lawson Cave is dominated by a deciduous forest and pasture. Given that the land cover has changed very little over this period, the decline in δ13C values toward the present likely reflects a shift in land management on farms and/or increases in invasive C3 species. Rainfall amounts from nearby Lebanon, Missouri, are significantly positively correlated with guano δ15N values, a relationship that is notably opposite that observed previously in soil and plants. We argue that heavy fertilizer application and significant grazing intensity could lead to the accumulation of large pools of excess labile nitrogen which would be vulnerable to leaching during precipitation events. The relationship between guano δ15N values and precipitation may differ for materials from less agriculturally impacted locations or periods and should be extended into the past with caution.
Introduction
It is becoming increasingly recognized that knowledge of the past can be used to help support present-day biodiversity conservation and restoration as well as both social and ecological resilience in the future (e.g., Dietl and Flessa, 2011; Dietl et al., 2015; Rick and Sandweiss, 2020; Boivin and Crowther, 2021, and references therein). Particularly in the face of rapid environmental change, awareness of the long-term trends and cycles acting within and upon an ecosystem will be invaluable for setting ecological restoration goals and creating management plans (e.g., Wingard et al., 2017). While recent historical data are often readily available from satellite imagery and weather stations, longer-term proxy data can be challenging to acquire, especially for sites where traditional proxy records are lacking or that may have a long land-use history predating the advent of modern instrumentation.
Protected from surface weathering conditions, cave guano deposits can accumulate undisturbed over thousands of years, potentially providing a high-resolution archive of past climate and environment, particularly for low- and mid-latitudes. Accumulating from the detritus of bats and birds that roost in large numbers, these deposits are primarily composed of unconsolidated organic material, such as insect remains (Mizutani et al., 1992a; Bird et al., 2007; Onac et al., 2014). Des Marais et al. (1980) were the first to propose that there could be a connection between guano carbon isotope values and local climate and environment; insectivorous bats feed on insects whose dietary preferences reflect the local vegetation, the distribution of which is ultimately driven by factors such as regional substrates, nutrient availability, climate, and/or human activity. Because carbon isotope (δ13C) values are primarily driven by the photosynthetic pathways of plants, with C4 plants exhibiting higher δ13C values than C3 plants (−12.5 ± 1.1 and −26.7 ± 2.3‰, respectively; Cerling et al., 1997), shifts in guano carbon isotope values may therefore be indicative of landscape-level changes in vegetation between grassland (which contain more C4 species) and forest (which are dominated by C3 plants). This relationship has been used to investigate the paleoenvironment in tropical (Bird et al., 2007; Wurster et al., 2010b, 2017), semi-arid (Wurster et al., 2008, 2010a), and temperate locations (Onac et al., 2014; Forray et al., 2015; Widga and Colburn, 2015). Assuming that bats consume a sufficiently heterogenous sample of local insects, it may also be possible to estimate the relative proportion of C4 biomass on the landscape using an empirical (Wurster et al., 2007) or mass balance (Wurster et al., 2017) approach.
Guano δ15N values, which have not only been linked to bat diet (Mizutani et al., 1992a; Lam et al., 2013; Salvarina et al., 2013) but also to landscape scale N-cycling and/or precipitation (Cleary et al., 2016), can also provide meaningful climatic and environmental information. Cleary et al. (2016) argued that guano δ15N values can be considered system integrators (Robinson, 2001), reflecting the extent to which N is retained in the ecosystem in the vicinity of the cave. An ecosystem with an “open” N-cycle experiences greater N loss relative to internal nitrogen cycling; losses through leaching and N-transformations both favor the loss of 14N, leaving the remaining nitrogen 15N-enriched (Austin and Vitousek, 1998; Robinson, 2001). Precipitation amount (Austin and Vitousek, 1998; Handley et al., 1999; Amundson et al., 2003) and agricultural activities, such as fertilizer application, controlled burning, and grazing intensity (Szpak, 2014), can further modulate the state of the N-cycle. In a core collected in Romania, Cleary et al. (2016) observed lower guano δ15N values during periods of increased fire activity and pasture expansion and a positive correlation between guano δ15N values and precipitation. This relationship with precipitation is notably opposite to the trend observed for soil and plant δ15N values (Austin and Vitousek, 1998; Handley et al., 1999; Amundson et al., 2003) and merits further investigation.
In this study, we seize the opportunity to further investigate the relationships between guano δ13C and δ15N values and environmental variables, including vegetation and precipitation, in a temperate climate. We collected a 60 cm long guano core from Mary Lawson Cave, a large limestone cavern in central Missouri, which is home to a maternity colony of federally endangered, insectivorous gray bats (Myotis grisescens). We sectioned the guano core, used multiple approaches to determine the age of the core, measured guano δ13C and δ15N values, and analyzed their change through time. Because dating estimates showed that the Mary Lawson guano core is very young, the entire period of accumulation corresponded with instrumental records (e.g., weather station precipitation measurements, satellite imagery). By comparing these young guano isotopic records with instrumental records, we aim to both quantify and contextualize the relationships between guano isotope values and environmental variables, such as plant community composition, land management practices, and/or climate. Our goal is to enable future researchers and restoration practitioners to use guano proxy records to help understand how the environment has changed over time and set historically informed restoration goals.
Materials and methods
Study site and subjects
Mary Lawson Cave, a large limestone cavern located in central Missouri, is home to a maternity colony of federally endangered gray bats (M. grisescens) and serves as a minor hibernaculum for Indiana bats (Myotis sodalis). Gray bats are insectivorous and found throughout much of the midwestern and southeastern United States (Tuttle, 1979). Gray bat diet varies considerably by age, sex, and reproductive condition (Brack and LaVal, 2006). At a local scale, gray bats appear to be opportunistic foragers, but on a broader scale, they are more selective, preferring to forage over streams and along woody riparian areas (Brack and LaVal, 2006). Gray bat dietary components are nonetheless correlated with prey abundance (Clawson, 1984; Barclay and Brigham, 1994). Common dietary items include, but are not limited to, mayflies, caddisflies, beetles, flies, and moths/butterflies (Best et al., 1997; Brack and LaVal, 2006). Caves are important to gray bats both in the winter for hibernation and in the spring/summer for raising their young (Martin, 2007). From March/April until early to mid-September, adult females congregate by the thousands to tens of thousands in maternity roosts, such as Mary Lawson Cave, to bear and rear the young (Tuttle, 1976).
To protect the bats in Mary Lawson Cave, the Missouri Department of Conservation installed a gate at the cave entrance in 2004. The gate was further reinforced in 2008. In the early 1970s, a previous cave owner enlarged the cave entrance and installed wiring in preparation for developing Mary Lawson as a show cave (Toole, 1995), but those plans never came to fruition. Based on guano area measurements and roost counts, bat populations in the cave initially increased following the installation of the gate; the gray bat colony grew from an average size of 35,850 between 1990 and 2000 to 71,000 in 2005 (Elliott, 2007). New counting methods using thermal infrared video monitoring suggest that the gray bat population at Mary Lawson consisted of >100,000 individuals in the spring of 2010 (Elliott et al., 2011). But, with the arrival of Geomyces destructans, the fungus that causes white-nose syndrome in bats in Missouri in 2010, the bat population in Mary Lawson Cave has most certainly declined.
Sample collection
In September 2018, we sampled a 60 cm long guano core in the main passage of Mary Lawson Cave. The core was collected from a relatively small guano pile located ~15 m inside the cave entrance. We used a 50-cm-long Russian peat borer (5 cm diameter; Maher, 2006; Forray et al., 2015) and collected material from two adjacent holes (A: 0–50 cm, B: 10–60 cm). The collected cores were labeled and encased in a PVC pipe for transport out of the cave and subsequently stored in a 4°C refrigerator before subsampling.
To provide context for guano core isotope data, we additionally sampled fresh, modern bat guano in June 2019. We laid out plastic sheeting on the ground outside the cave entrance in the evening and returned the following morning to collect fecal pellets that had accumulated on the plastic overnight. The samples were stored in microcentrifuge tubes with silica beads before analysis.
Radiocarbon dating and age model
We sent two untreated, bulk guano samples, one from the bottom (59–60 cm) and one from the middle (32–33 cm) of the core, to the National Ocean Sciences Accelerator Mass Spectrometry (NOSAMS) facility at the Woods Hole Oceanographic Institution for radiocarbon analysis. Given their modern age (see Results), we used the Bomb21NH2 bomb series calibration curve (Hua et al., 2022) in OxCal 4.4 (Bronk Ramsey, 2009) to calibrate the fraction of modern material in the samples to calendar years. We constructed an age model for our core using a weighted linear regression across these two calibrated dates and the date of sampling at the surface.
210Pb and 137Cs age-depth modeling
Given the young radiocarbon dates (see Results), we also employed 137Cs and 210Pb to model the age-depth relationship in the Mary Lawson guano cores. The evidence of 137Cs activity in the bat guano samples (n = 22) was determined by γ-emission (661.7 keV) using a Canberra gamma spectroscopy system with an HPGe well detector (model GCW 4023).
Measurements of 210Bi (n = 3) or 210Po (n = 19) in secular equilibrium with 210Pb were made following the methodology outlined in Waples (2020). Briefly, 207Bi or 209Po yield monitors (Eckert and Ziegler Isotope Products) were added to the weighed samples of bat guano before digestion in concentrated nitric acid. Bismuth was completely separated from lead on an Empore™ anion solid phase extraction (SPE) disk (3 M, 47 mm, product number 2,252, now manufactured by CDS Analytical). The Anion SPE disks were then immediately counted—first for 210Bi via β-decay on a low background gas-flow proportional detector with anticoincidence circuitry (G542 System, Gamma Products), and then for 207Bi via γ-emission to determine the yield. Polonium was plated to copper and α-counted for 210Po and the yield monitor 209Po. The reported activities (±1 SD) include propagated uncertainties in the decay rate, detector efficiencies, and yield. The activity calculations and corrections are fully described by Waples (2020).
The mass accumulation rate (MAR) of bat guano (g cm−2 yr−1) was calculated using the constant flux-constant sedimentation (CF-CS) model (Krishnaswamy et al., 1971; Robbins and Edgington, 1975):
where λ is the decay constant for 210Pb (0.03108 yr−1), C0 is the specific activity of 210Pb at the surface (dpm g−1), and Cm is the specific activity of 210Pb (dpm g−1) at some depth with cumulative mass m (g cm−2; Arias-Ortiz et al., 2018). The density profiles of guano were determined by dividing the total dry weight of each core segment by the volume of each core segment.
Elemental and isotopic analysis
Because of the potential for ammonia volatilization to impact the δ15N values of guano deposits (e.g., Mizutani et al., 1986; McFarlane et al., 1995), we isolated the chitinous component of the guano via solvent extraction for δ15N analyses. Briefly, following Wurster et al. (2017), we ground the samples using a mortar and pestle and removed lipids with 2:1 (v/v) dichloromethane/methanol. We then soaked the samples in 1 M NaOH for 30 min, rinsed them 3x with MilliQ water, and finally soaked the samples for 3 h in 2M HCl before rinsing 3x with MilliQ water and drying them overnight in a 50°C oven.
We conducted elemental and isotopic analyses on bulk (C and N) and solvent-extracted guano (N) at two institutions: Washington University in St. Louis (WUSTL) and Virginia Tech (VT). We analyzed eight samples at both institutions to ensure comparability and we noted the location of analysis for each sample in our comprehensive results table (Supplementary Table 1). At WUSTL, we weighed ~1 mg of dried, homogenized sample into 9 × 5 mm tin capsules for combustion on a Flash 2000 elemental analyzer coupled to a Thermo Delta V Plus continuous-flow isotope ratio mass spectrometer. We used a two-point linear normalization (with USGS 40 and USGS 41) to calibrate isotopic measurements to their respective internationally accepted scales (AIR and VPDB). The analytical precision (1σ) calculated on the repeated measure of an internal standard (n = 3; acetanilide; δ13C = −29.55‰, δ15N = 1.15‰) was <0.1‰ for both δ13C and δ15N. At VT, the samples were also weighed to ~ 1 mg, encased in 9 × 5 mm tin capsules, and analyzed on an Isoprime 100 isotope ratio mass spectrometer coupled with a Vario ISOTOPE Cube elemental analyzer in the Department of Geosciences. The analytical precision (1σ) based on the repeated analyses of a commercial standard (elemental microanalysis urea: δ13C = −48.63‰, δ15N = 57.37) was <0.3‰ for δ13C (n = 10) and <0.2‰ for δ15N (n = 16). We calibrated stable nitrogen isotope compositions to the AIR scale with USGS 25 and USGS 26 via a two-point linear normalization (δ15N = −30.41 and 53.75‰, respectively). Carbon isotope compositions were calibrated to the VPDB scale with IAEA CH 6 and CH 7 also via a two-point linear normalization (δ13C = −10.45 and −32.15‰, respectively). The average sample differences for the eight samples analyzed in both labs were within measurement error (0.3‰ for both δ15N and δ13C).
Precipitation and land use
We downloaded the precipitation data from the Midwestern Regional Climate Center (cli-MATE; https://mrcc.purdue.edu/CLIMATE/). We sourced data from the Lebanon 2W station (37.685, −92.6939, elevation 1,246 ft.), which is located ~20 km away from Mary Lawson Cave in Laclede County, Missouri, as this is the closest station to Mary Lawson Cave with a relatively complete record between 1998 and 2018.
To assess land use trends at a relatively coarse scale, we compiled county-level statistics from the United States Census of Agriculture for the three Missouri counties surrounding Mary Lawson Cave: Camden, Dallas, and Laclede. We looked at the proportion of land on farms together with the proportion of cropland, proportion of woodland, and proportion of land in corn to assess potential changes in the types of vegetation on the broader landscape. Many crops in Missouri have C3 photosynthetic pathways (e.g., soybeans, hay), but corn and sorghum (which is not grown to a significant degree in the counties of interest) are C4 photosynthesizers, and therefore, have higher δ13C values.
We additionally used National Land Cover Database (NLCD) GeoTIFFs provided by the USGS to examine interannual changes in land cover proximate to the cave system where the guano core was collected. The NLCD data provide 30-m spatial resolution classifications of Continental United States (CONUS) land cover updated at 2–3-year intervals. The NLCD land cover datasets are created using optical (e.g., Landsat) satellite imagery to produce a 16-class legend based on a revised version of the Anderson classification system (Anderson et al., 1976). We used all available CONUS GeoTIFF data corresponding to the period covered by the guano core (see Results), covering the years 2001, 2004, 2006, 2008, 2011, 2013, 2016, and 2019. The GeoTIFFs were masked within a 20 and 40 km buffer around the cave system coordinates to isolate land cover within the bats' foraging range. The GeoTIFFs were also masked within the combined boundaries of Camden, Dallas, and Laclede Counties. We then statistically aggregated the various land classes contained within the masked GeoTIFFs to quantify the land cover values and their interannual trends from 2001 to 2019. The GeoTIFF processing and masking were accomplished with QGIS Version 3.10, and statistical analysis on masked GeoTIFFs was accomplished with RStudio Version 1.4, using the raster package for data analysis and ggplot2 for plotting.
Results
Radiocarbon dating and age model
As alluded to above, both samples from the core were modern (Table 1), with the material from the bottom of the core dating to 1999 ±1 cal AD, and the middle to 2008 ±1 cal AD. Guano deposition appears to have been continuous and at a rate of ~3.3 cm/year.
137Cs and 210Pb data
Gamma emission analysis of 22 bat guano samples from the surface (0–1 cm) to the bottom (59–60 cm) of the collected cores found no evidence of 137Cs activity, lending credence to the relatively young (>1,963) 14C-derived age of the core.
210Pb activities in the bat guano profile ranged from 3.3 ± 0.2 to 1.2 ± 0.1 dpm g−1 and tended to decrease with depth (Figure 1, Supplementary Table 1). Sampling overlap between the MLA core (filled circles) and the MLB core (open circles) showed good agreement as did the 210Pb activities derived by 210Bi analysis (triangles) and 210Po analysis (circles).
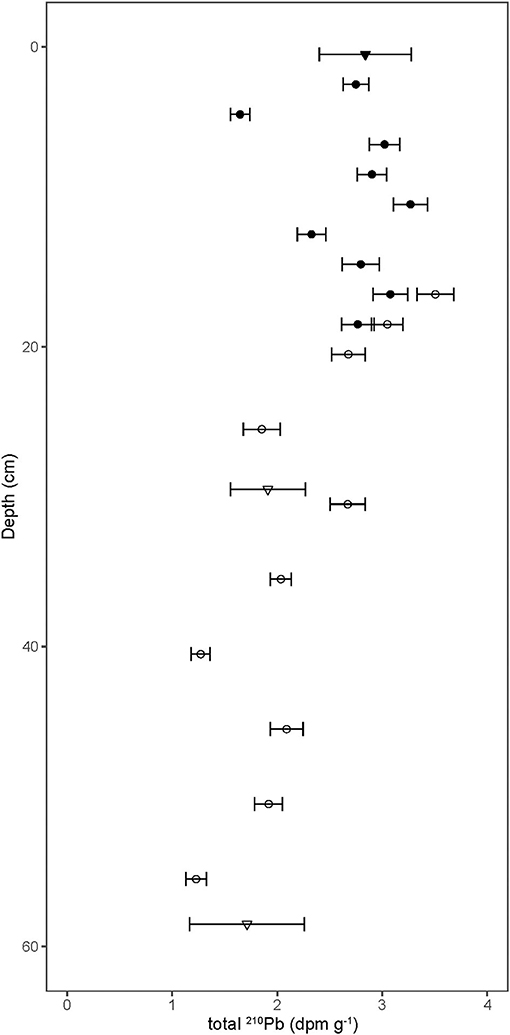
Figure 1. 210Pb activity in bat guano as a function of depth, where the bottom of the core reached the cave floor at 60 cm depth. Error bars show ±1 SD. The MLA core (filled circles) and the MLB core (open circles) show good agreement as do 210Pb activities derived by 210Bi analysis (triangles) and 210Po analysis (circles).
There have been several attempts to date guano cores in caves using 210Pb chronometry—not without controversy (e.g., Gallant et al., 2020; McFarlane and Lundberg, 2021). To use 210Pb as a chronometer of guano accumulation, several system parameters need to be defined. For instance, are inputs of 210Pb to the surface of accumulating guano constant? Is the 210Pb sourced primarily from the food bats consume and feces they produce, or is it through 222Rn decay in the cave chamber? If it is the former, have bat populations remained constant over time? If the latter, has ventilation between the interior and exterior of the cave remained constant? How much of the 210Pb profile is supported by local 226Ra decay? And is the supported 210Pb activity in equilibrium with the 226Ra activity or does a significant fraction of locally produced 222Rn diffuse out of the relatively porous guano pile? Finally, is the only mechanism for 210Pb loss through radioactive decay? Or, is the 210Pb mobile after the deposition? Unfortunately, for this study, most of these parameters are unknown. If we assume that the 14C dating is correct, the 210Pb profile record can be used to constrain the system parameters controlling the deposition and decay of 210Pb in this cave system.
Because the density of the bat guano core was essentially uniform with depth (Figure 2A) and the 14C-derived age of guano decreased linearly with depth (Figure 2B), we can calculate a constant 14C-derived guano accumulation rate of 0.50 ± 0.03 g cm−2 y−1 (Figure 2C). This 14C-derived accumulation rate can be translated to a linear 210Pb decay slope, where the dashed line in Figure 3 corresponds to the expected decay of 210Pb as a function of accumulated mass and a constant guano accumulation rate of 0.50 ± 0.03 g cm−2 y−1. A linear regression of the observed decay of 210Pb (Figure 3, solid line) results in nearly the same slope, with a corresponding guano accumulation rate of g cm−2 y−1. This dates the bottom of the guano core to the year 1998 (±5 years), which is essentially identical to the 14C age.
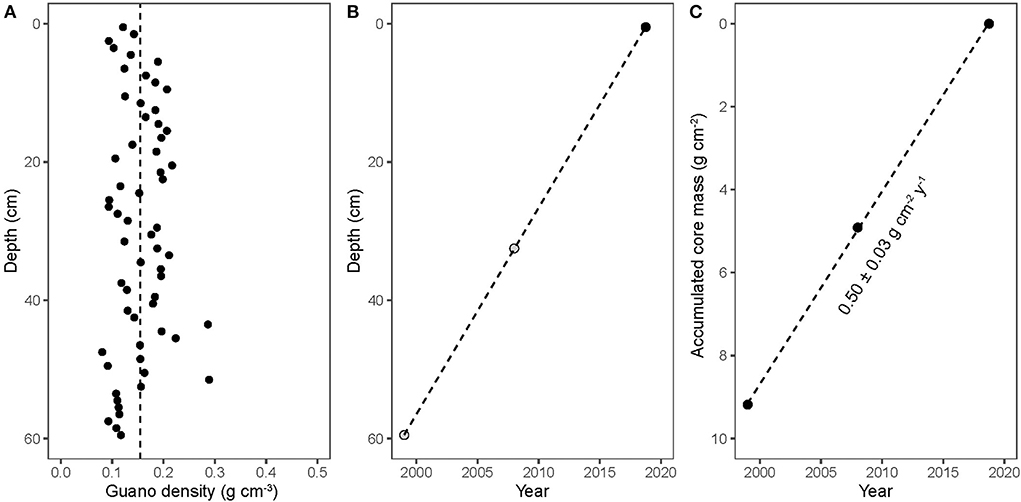
Figure 2. (A) guano density; (B) guano strata dating based on time of collection (black circle) or 14C dating (gray circles); (C) guano strata dating as a function of accumulated core mass. The calculated guano deposition rate was 0.50 ± 0.03 g cm−2 y−1.
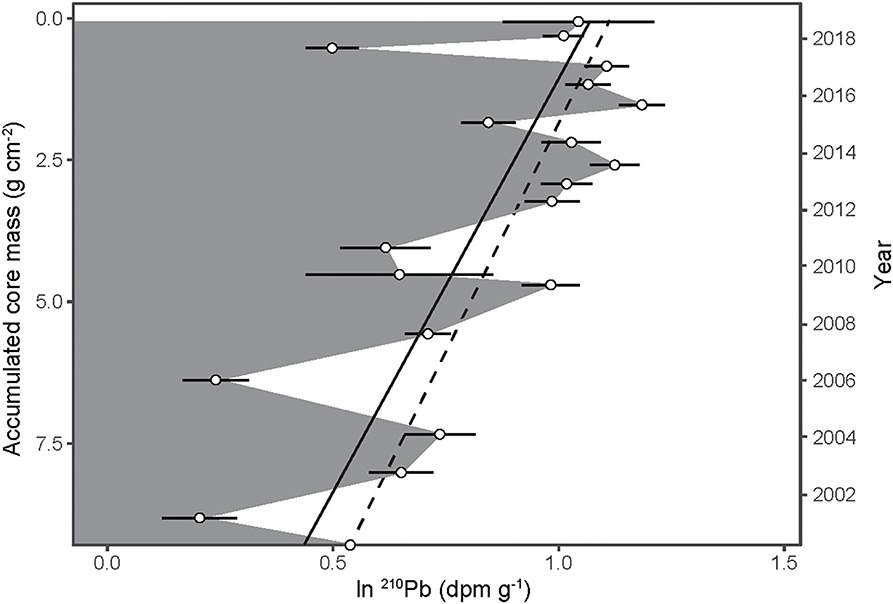
Figure 3. The natural log of 210Pb activity (open circles ±1 SD) as a function of accumulated guano core mass. The slope of the regression is equal to ln(Cm/C0)/m in Equation (1). The corresponding age of each stratum is shown along the right y-axis. The dashed line depicts the expected 210Pb decay slope while the solid line illustrates a linear regression of the observed decay of 210Pb.
Elemental and isotopic data
Fresh gray bat guano collected overnight in June 2019 had a mean δ15N value of 4.7 ± 1.7‰ and a mean δ13C value of −26.7 ± 2.0‰ (n = 35; Supplementary Table 2). Solvent-extracted guano core samples had substantially lower δ15N and marginally lower %N values than bulk guano, suggesting that ammonia volatilization significantly impacted the nitrogen data in this relatively young material (Supplementary Figure 1). All guano core nitrogen results presented henceforth are reported from the solvent-extracted samples. The %C and %N values remain relatively constant throughout the length of the core (mean and standard deviations of 33.4 ± 2.8 and 9.8 ± 1.0%, respectively (Figure 4, Supplementary Table 1). The atomic C:N ratios in the core fluctuate from 3.0 to 5.5; 3.1 ± 0.5). The guano core δ13C values decrease significantly from the base toward the surface (from ~−26 to −27‰; r2 = 0.192, p = 0.00031; Figure 5) whereas the δ15N values increase significantly toward the surface (from ~3 to 5‰; r2 = 0.161, p = 0.00095). There is greater variability in the δ15N values (mean and standard deviation: 4.2 ± 1.2‰) than in the δ13C values (mean and standard deviation: −26.3 ± 0.5‰). The guano core δ13C and δ15N values are not correlated (r2 = 0.0138, p = 0.18).
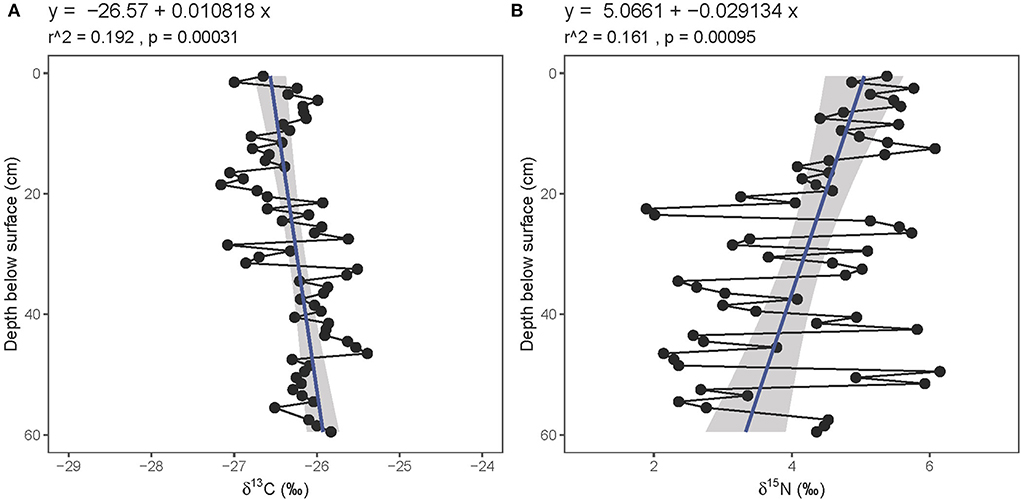
Figure 5. Relationship between guano (A) δ13C (bulk) and (B) δ15N (solvent-extracted) values with depth below surface. Linear regression line (blue) plotted with standard error (gray).
Environmental and land use data
There is no significant trend visible in the Lebanon precipitation data from 1998 to the present. Complete records of monthly and annual precipitation are available from the Lebanon 2W station for all but 2 years between 1998 and 2018. Yearly precipitation averages 109 cm/yr but varies from a high of 214 cm in 2008 to a low of 55.1 cm in 2014. Missouri experienced its wettest year on record in 2008 due in part to a moderate La Niña event (NOAA National Centers for Environmental Information, 2019), while 2014 was a drought year in much of Missouri. Both of these years can be considered outliers based on Cook's distance (Altman and Krzywinski, 2016) and we chose to analyze the data both with and without removing them. Without the two outlier years removed, the precipitation amounts increase slightly toward the present (+1.8 cm/yr; r2 = 0.0593, p = 0.16, Supplementary Figure 2A). With the two outlier years removed, the precipitation amounts increase more strongly toward the present (+2.4 cm/yr; r2 = 0.458, p = 0.0017; Supplementary Figure 2B). We also considered precipitation from the previous year's winter months through the growing season (previous October to present August), which likely contributes more significantly to N availability during the present year growing season (Cleary et al., 2016); the amount of precipitation from October to August increases weakly toward the present with outliers included (+1.4 cm/yr; r2 = 0.0192, p = 0.27, Supplementary Figure 2C) and more strongly with outliers removed (+1.9 cm/yr; r2 = 0.47, p = 0.0029, Supplementary Figure 2D).
The proportion of land on farms from 1997 to 2017 in Camden, Dallas, and Laclede Counties has remained relatively constant (Figure 6A), though farm composition has changed. The proportion of land in crops has declined significantly since 1997 (Figure 6B, r2 = 0.872, p = 0.013) while the proportion of land in pasture and rangeland has increased significantly (Figure 6C, r2 = 0.879, p = 0.012). The proportion of woodland has declined slightly, but not significantly (r2 = 0.627, p = 0.069). Corn is not a major crop in Camden, Dallas, and Laclede Counties, though the proportion of land planted in corn has increased slightly from 0.17% in 1997 to 0.52% in 2017 (Figure 6D). These trends also hold true when Laclede County is considered alone (Supplementary Figure 3). We also found that fertilizer use in Camden, Dallas, and Laclede Counties did not change between 2002 and 2017 (Supplementary Figure 4) nor did the number of common livestock animals in the tri-county area (cattle, horses, sheep, and goats from 1997 to 2017, Supplementary Figure 5A), though the number of livestock in Laclede County alone increased marginally (Supplementary Figure 5B).
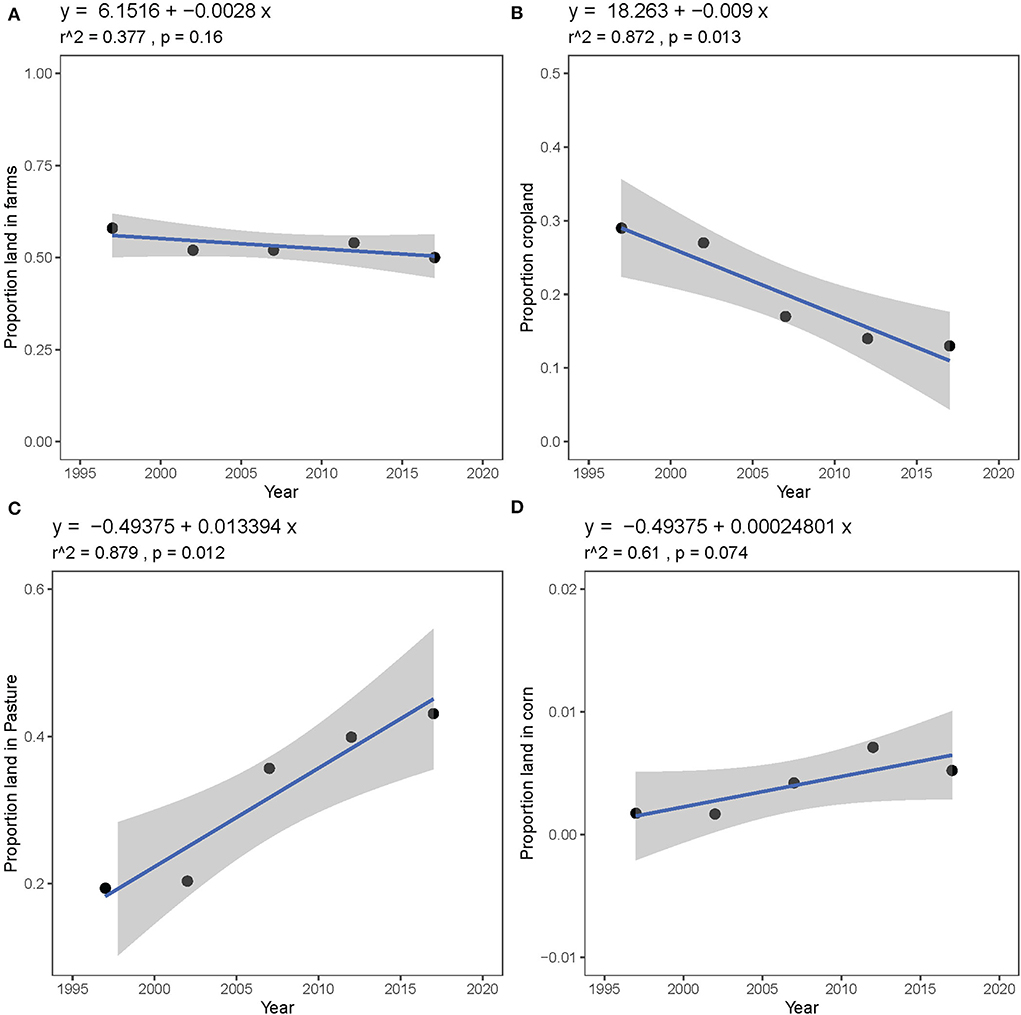
Figure 6. Trends in land use in Camden, Dallas, and Laclede Counties between 1997 and 2017 as reported to the United States Census of Agriculture: (A) proportion of total land area in farms, (B) proportion of farm land planted in crops, (C) proportion of farm land used as pasture or rangeland, and (D) proportion of farm land planted in corn.
A 2001 to 2019 time series analysis of NLCD GeoTIFFs revealed little change in land cover masked within a 20 and 40 km radius around the cave system entrance as well as within the combined boundaries of Camden, Dallas, and Laclede Counties (Figure 7). In all three masks, most of the land cover throughout the time series was primarily deciduous forest and pasture/hay land classes. Deciduous forest exceeded the combined surface area of all the remaining land over classes. The distribution does not change in closer proximity to the cave system, as evidenced by similar land cover surface area distributions within the 20 and 40 km buffers. None of the land classes exhibited notable increases or decreases in surface area between 2001 and 2019.
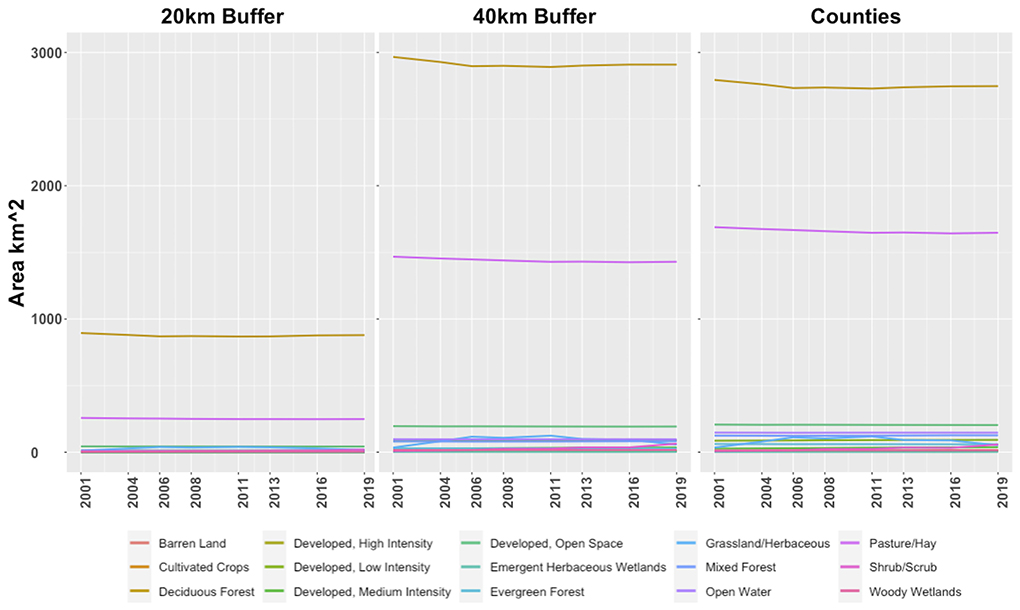
Figure 7. Land class surface areas calculated from NLCD data from 2001 to 2019. Land use classifications are consistent with the NLCD naming convention. The (left) plot depicts land class trends within a 20 km buffer surrounding Mary Lawson Cave; the (center) plot depicts trends within a 40 km buffer; the (right) plot depicts trends within the combined area of Camden, Dallas, and Laclede Counties. Note that the majority of land use is dominated by deciduous forest (gold) and pasture (dark pink). The remaining land classes do not individually exceed 210 km2 of surface area, and thus are somewhat obscured due to overlap in the plots.
Comparison between guano isotope data and environmental variables
Considering previous research on the relationship between guano isotope values and environmental parameters, we evaluated the relationships between guano isotope values and several environmental variables, including precipitation and vegetation type. With the two precipitation outliers removed, neither guano δ13C nor δ15N values are strongly related to the same-year precipitation amount (r2 = 0.108, p = 0.12; r2 = 0.0874, p = 0.14). Guano δ13C values are similarly unrelated to previous year (previous year October to present year August) precipitation amount (r2 = 0.0129, p = 0.3, Figure 8A), but guano δ15N values are significantly positively correlated with precipitation amount from the previous year winter through the growing season (r2 = 0.286, p = 0.023, Figure 8B). These patterns are weakly evident when the precipitation outliers are included (Supplementary Figure 6).
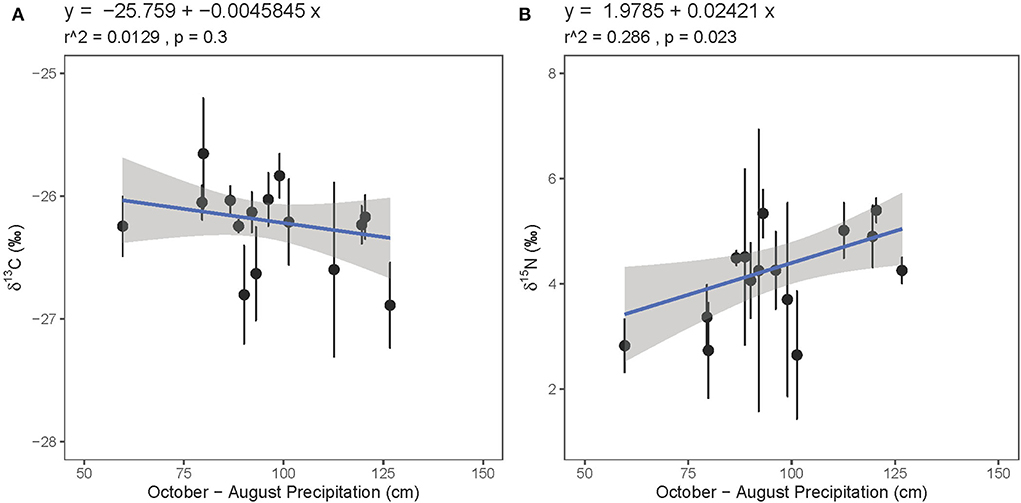
Figure 8. Relationship between bat guano core (A) δ13C and (B) δ15N values with precipitation amount from the previous year through the growing season (October–August).
Discussion
Guano age dating
The similarity between the 14C- and 210Pb-derived ages implies several things about 210Pb accumulation and decay in this cave system. First, it was assumed that the 226Ra and supported 210Pb activities in the guano core were negligible. Any significant supported 210Pb activity would have dramatically increased the apparent decay of unsupported 210Pb, resulting in a decrease in the apparent guano deposition rate and an increase in the overall age of the core—a result incongruent with the 14C and 137Cs chronometers.
Second, 210Pb in the guano core most likely originated from the influx and subsequent decay of 222Rn sourced from outside of the cave (Waring et al., 2021). The annual rate of atmospheric 210Pb deposition at the latitude of Mary Lawson Cave (~37° N) is roughly 1.0 ± 0.6 dpm cm−2 y−1 (Baskaran, 2011). After 19 years of accumulation (i.e., the accumulated age of the core) and concomitant decay, the expected inventory of 210Pb in an area above the cave and exposed to the atmosphere would be 8.2–21.8 dpm cm−2. The measured inventory of 210Pb in the bat guano core was 20.2 dpm cm−2 and within the expected range of atmospheric deposition. Moreover, the more than doubling of the gray bat population between 2000 and 2010 showed no signs of increasing either the guano or 210Pb deposition rate at the site the cores were collected.
Lastly, although 210Pb activities dropped significantly at several time points (e.g., 2001, 2006, and 2011 in Figure 3), there is no evidence of sustained 210Pb mobility in the profile at this site. Additional measurements are needed to determine if the observed decrease in 210Pb at certain strata is repeatable or merely sampling artifacts.
Guano integrity
Although the Mary Lawson guano core is young, it is still important to consider the possibility of post-depositional modification. Guano δ15N values could be altered through ammonia volatilization, whereby the volatile loss of 14N would leave the residual guano enriched in 15N (Mizutani et al., 1986, 1992b; McFarlane et al., 1995). Previous research suggests that this process is driven by the relatively rapid loss of urea (McFarlane et al., 1995), which has a half-life of ~40 years at 25°C (Sigurdarson et al., 2018). Nonetheless, subfossil and fossil guano deposits have been shown to be faithful recorders of the primary δ15N signal, as insect chitin, which makes up ~25-40% of insectivorous bat guano, is very resistant to alteration (McFarlane et al., 2002). We, therefore, chose to solvent extract our guano samples to isolate the chitinous component for nitrogen isotope analysis, thereby removing any overprinting by ammonia volatilization (Supplementary Figure 1). If the solvent-extracted guano were still impacted by post-depositional processes, we would expect to see %N decrease along with an increase in the δ15N values (Bird et al., 2007). We however see no trend in the guano %N values with depth and the average %N values are similar to previously studied unaltered guano deposits (e.g., ~10%, Bird et al., 2007; Cleary et al., 2016). After accounting for ammonia volatilization, any remaining variability within the record can be attributed to primary changes in bat guano isotopic values rather than to post-depositional processes. Additionally, there has been considerable momentum in understanding guano/manure applications as seasonal activities in ancient agricultures using plant nitrogen isotope compositions (e.g., Bogaard et al., 2007; Szpak, 2014). Our results, which illustrate the strong influence ammonia volatilization can have on δ15N values (Supplementary Figure 1), raise awareness of the slowness (over decades, though see Sigurdarson et al., 2018) of ammonia volatilization calling for caution in archaeological interpretation.
Drivers of isotopic change
Guano δ13C values
The guano core δ13C values consistently fall within the range expected for C3 plants, but the small decrease toward the present suggests a possible increase in C3 plants relative to C4 plants that can likely be attributed to changes in the composition of farm and pastureland. Although the satellite data suggest that there were no major changes in land cover during the time represented by the guano core, the data from the Census of Agriculture indicate a shift in farmland allocation; the land area in active cropping declined while the land area in pasture increased (Figure 6). The satellite data do not capture this change, but previous research suggests that the potential for misclassification in the NLCD is particularly high in areas dominated by forest, as is the case in this area of Missouri (Maxwell et al., 2008). Fescue (Festuca spp.) is the primary forage species found in Missouri pastures, often planted in a mixture alongside legume species, such as clovers (Trifolium spp.) to increase feed quality (Glenn et al., 1981; Phelan et al., 2015). Fescue is a perennial, cool-season grass that uses the C3 photosynthetic pathway, whereas the dominant native savanna plants in Missouri are C4 photosynthesizers (e.g., little bluestem, Schizachyrium scoparium; Baskin and Baskin, 2000). The conversion from native savanna or prairie to pasture would not necessarily be accurately discernable in the satellite data (e.g., Wickham et al., 2017), but such a change could still be accompanied by a shift in carbon isotope values if C4 species are replaced with C3 species like fescue. Native grasses may also be replaced by rapidly spreading invasive grasses, such as Bromus inermis (Stotz et al., 2019) or shrubs such as multiflora rose (Rosa multiflora; Moser et al., 2008). Finally, another explanation for the proportional increase in C3 species over time is that the very open, forb-dominated forest understories historically found in the region have increasingly been invaded by Japanese and Bush honeysuckle (Lonicera japonica and L. maackii) over the last 20 years and they now form dense understories in woodland areas in the region (Moser et al., 2008). Such a change would also be unlikely to be detected by satellite data. Though the proportion of corn being planted on farms over this same period increases rather than decreases, the acreage planted in corn is likely too small relative to pasture (1,000s of acres vs. 10,000s) to influence the guano δ13C values. The very low proportion of land planted in corn (<0.01%) is, however, consistent with the estimates of the relative abundance of C3 vs. C4 plants on a landscape using the empirical equations developed by Wurster et al. (2007), which are all <0.1%. The presence of additional native C4 prairie and savanna plants likely make up the difference.
Changes in bat guano δ13C values could also be attributable to ecosystem-scale plant water availability within a temperate, C3-dominated landscape because carbon isotope values of C3 plants are associated with water use efficiency (Forray et al., 2015). Several local (Stewart et al., 1995) and global (Diefendorf et al., 2010; Kohn, 2010) compilations of C3 plant δ13C values show a distinct, non-linear negative relationship between plant δ13C values and mean annual precipitation (MAP), such that δ13C values decrease with increasing MAP. Based on the equation for MAP as a function of δ13C presented by Kohn (2010), the small increase in precipitation amounts we see near Mary Lawson Cave between 1999 and 2018 (+40 cm/yr) could account for a ~0.6‰ decrease in carbon isotope values. This suggests that the ~1‰ decrease in δ13C values in the Mary Lawson guano core could largely be attributed to an increase in precipitation. Nonetheless, we saw no correlation between bat guano δ13C values and precipitation amount at this location (Figure 8A) and the lack of a correlation between guano δ13C and δ15N values in the Mary Lawson core might suggest that changes in these two proxies are attributable to different drivers.
Guano δ15N values
The increase in bat guano δ15N values from the base of the Mary Lawson core toward the surface is slight but it still requires explanation. The changes in bat guano δ15N values are likely attributable to three potential drivers: (1) changes in bat diet, (2) changes in land use or management, and/or (3) changes in precipitation. It is important to try to evaluate the relative influence of these different drivers on bat guano δ15N values so that restoration practitioners and land managers can both appreciate the complexity of this proxy and maximize its utility for achieving restoration goals. Below we explore each of these three potential drivers in detail.
Given that the average increase in δ15N values with each trophic step is around 3–5‰, a shift of ~2‰ could simply be attributable to a trophic shift. Nonetheless, it seems unlikely that bat dietary preferences would change drastically over just 19 years. As stated above, gray bats are insectivorous and they are opportunistic foragers at a local scale, consuming insects roughly in proportion to their abundance (Clawson, 1984; Barclay and Brigham, 1994). Their diets largely consist of mayflies, caddisflies, beetles, flies, and moths/butterflies (Best et al., 1997; Brack and LaVal, 2006). The nitrogen isotope values measured in individual modern gray bat scats range from 0.0 to 6.8‰, suggesting that presently, these bats are consuming a wide range of insects. It is certainly possible that, in the context of declining insects in the Anthropocene (Wagner, 2020), gray bats have had to change their diet as the relative abundance of insects change, though much of the research on insect declines has been conducted in Europe and few studies have focused on the midwestern United States (but see Wepprich et al., 2019). It is also possible that bats have had to adjust the area over which they forage in response to fluctuating population sizes; larger bat populations may have had to travel further afield to find sufficient food. The gray bat population at Mary Lawson Cave increased following the installation of the gate, but it also likely decreased again after the arrival of the fungus that causes white-nose syndrome in bats in 2010. If a change in foraging area contributed to the shift in guano δ15N values in the Mary Lawson core, then we might expect those values to first rise and then fall again as populations declined; instead, we see a roughly linear, though certainly variable, increase in δ15N values toward the present. Future research using compound-specific nitrogen isotope analyses of individual amino acids could provide an opportunity to decipher whether the increase in δ15N values observed here is attributable to a change in bat diet or a change at the base of the food chain (e.g., Whiteman et al., 2019). At present, we suggest that a change in bat diet could contribute to the increasing guano core δ15N values but does not account for the entire signal.
Nitrogen in a given food chain is ultimately derived from the soil N-cycle at the base of the food web, where a complex array of biogeochemical processes lead to N-transformations (e.g., nitrification, denitrification, ammonia volatilization; Högberg, 1997) that impact soil and plant nitrogen isotope values. Agricultural activities, including fertilizer application, fire, and grazing intensity, can also have significant impacts on the nitrogen cycle and soil and plant nitrogen isotope values (Szpak, 2014). The nitrogen isotope values of synthetic fertilizers vary considerably (−5.9 to 6.6‰; Bateman and Kelly, 2007), however, manure often has relatively high δ15N values (mean of 8.1‰; Bateman and Kelly, 2007). Numerous studies now show that the elevated δ15N values characteristic of manure are reflected in plants grown with manure (e.g., Bogaard et al., 2007) such that an increase in the use of manure as fertilizer could explain the increase in the guano core δ15N values. While the Census of Agriculture data from Camden, Laclede, and Dallas counties show a slight increase in the number of acres over which manure is spread (Supplementary Figure 4A), it is likely too small to significantly impact soil and plant δ15N values. Synthetic fertilizer remains much more widely applied in these counties, covering an area of ~150,000 acres vs. just ~25,000 acres (Supplementary Figure 4).
Fire can also affect δ15N values, though studies are mixed on the magnitude and direction of the impact; most commonly, higher foliar δ15N values are initially evident in post-fire vegetation followed by a return to pre-fire values over several years (summarized in Szpak, 2014). For example, vegetation from burned pine forests in California ranged from 4.7 to 8.8‰ higher than that sampled from mature, unburned forest stands (Grogan et al., 2000), suggesting that the magnitude of the difference in δ15N values is comparable to the difference between plants fertilized with manure and plants that are unfertilized. Data on fire location and extent (in acres) are kept and compiled by the Missouri Department of Conservation. These records show that between 2000 and 2018, a total of 39,865 acres burned in Camden, Dallas, and Laclede Counties, but there is no relationship between the acres burned and year either within Laclede County alone or across all three counties (Supplementary Figure 7). Wildfire is, therefore, unlikely to explain the slight increase in bat guano δ15N values at Mary Lawson Cave. It is still possible that some farmers or land managers practiced controlled burning on their properties, which may have impacted guano δ15N values at Mary Lawson Cave, but we do not have the data to support or refute this.
Grazing intensity can additionally increase soil and vegetation δ15N values (Szpak, 2014). Grazing animals add nutrients to plant-soil systems by depositing urine and feces and can further alter nutrient cycling through physical disturbances, such as trampling (Bardgett and Wardle, 2003; Singer and Schoenecker, 2003). Szpak (2014) summarized the studies of plant and soil δ15N values under different grazing intensities, and while the results vary, areas experiencing higher grazing pressure generally show higher soil and plant δ15N values. This increase could potentially be attributable to an increase in the “openness” of the nitrogen cycle, which reflects the relative importance of within-system cycling vs. inputs and outputs; when outputs are large in comparison to internal cycling, soil and plant δ15N values will be higher and nitrogen cycling more open (Austin and Vitousek, 1998). Intensively grazed areas are characterized by both elevated inputs and outputs of nitrogen; they receive direct additions of mineralized or highly labile forms of N, but they also frequently exhibit elevated rates of nitrogen transformation (e.g., ammonification, denitrification, ammonia volatilization, and leaching of ; Högberg, 1997). Still, there is evidence that ecosystem δ15N values increase when inputs, cycling, and outputs are all higher (Högberg et al., 2011, 2014). Given that a large portion of land has been converted from active agriculture to pasture within Camden, Dallas, and Laclede Counties (see Figure 6), if that conversion was accompanied by an increase in livestock, it is possible that greater animal density could be contributing to increasing the baseline δ15N values in the area, as has been observed elsewhere (e.g., Marshall et al., 2018). While the number of livestock animals (cattle, sheep, goats, and horses) did not increase over the tri-county area for this period, it did indeed increase in Laclede County (Supplementary Figure 5).
There is a well-documented negative relationship between soil and plant δ15N values and mean annual precipitation across local, regional, and global scales, such that higher δ15N values commonly correspond with lower amounts of precipitation (Austin and Vitousek, 1998; Handley et al., 1999; Amundson et al., 2003; Swap et al., 2004). Austin and Vitousek (1998) argued that the loss of nitrogen relative to nitrogen cycled between plants and soil would be large in drier sites, as reduced plant demand would leave large pools of relatively labile nitrogen vulnerable to leaching or ammonia volatilization. Because these nitrogen transformations preferentially remove 14N, the remaining soil and plants will have higher δ15N values. Alternatively, in a wet ecosystem, increased production would reduce the size of the standing nitrogen pool and shift the balance such that internal nitrogen cycling would outpace nitrogen loss, resulting in a “closed” nitrogen cycle and lower plant and soil δ15N values (Austin and Vitousek, 1998).
At Mary Lawson Cave, we instead observe a positive correlation between bat guano δ15N values and precipitation from the previous winter suggesting that the nitrogen cycle in this temperate, agriculturally influenced ecosystem opens when precipitation is high. This is consistent with observations at Zidită Cave in Romania, where Cleary et al. (2016) found that drier intervals corresponded with lower δ15N values. The resolution of the Zidită core was not fine enough to quantitatively compare guano δ15N values with precipitation amount, but our data from Mary Lawson Cave show that for every 1‰increase in bat guano δ15N, winter precipitation amount increases by about 13 cm per year. Still, while the relationship is significant, it does not explain all the variation in guano δ15N values (r2 = 0.286 when outliers are excluded), suggesting that climate plays a role in shaping guano δ15N values, but not to the exclusion of other factors, such as agricultural activities. Cleary et al. (2016) posited that the observed positive relationship between guano δ15N values and precipitation amount at Zidită Cave may be specific to the temperate region, rather than arid environments or the tropics, where much of the previous bat guano nitrogen isotope research was conducted (e.g., Mizutani et al., 1992a,b; Wurster et al., 2007). Indeed, historical bat guano δ15N data from more arid Eagle Creek Cave, Arizona, did not correlate with any climatic data (Wurster et al., 2007). We additionally suggest that ecosystems highly impacted by agriculture, like the areas around both Mary Lawson and Zidită Caves, could accumulate relatively large pools of excess labile nitrogen derived from fertilizer application and/or livestock. These nitrogen pools would then be vulnerable to leaching during precipitation events, thereby, flipping the N-cycle open when precipitation amounts increase. This idea is supported by research that suggests that both heavy fertilizer use and significant grazing intensity increase nitrogen leaching (Wachendorf et al., 2005; Hussain et al., 2020). A further possibility is that the pooling of excess, agriculturally derived nitrogen may be more prevalent in temperate regions lending support to the observation made by Cleary et al. (2016). However, the relationship between guano δ15N values and precipitation may differ for materials from less agriculturally impacted locations or periods; we, therefore, encourage caution when extending these records into the past. Future comparison between speleothem oxygen isotope and guano nitrogen isotope data collected in the same cave would help to clarify the guano δ15N and precipitation relationship.
Our results suggest that guano δ15N values provide information about both climate and agricultural practices but will be best interpreted in conjunction with additional proxy records. Guano can additionally trap a variety of pollen grains and charred particles, providing further information about the vegetative landscape and land use surrounding caves. Airborne dust and pollen grains adhere to both insects and bats as they fly (Maher, 2006), ultimately becoming part of the guano record. Using similar methods as for lake and peat cores, the relative abundance of pollen types can be tracked with depth to reconstruct past changes in the composition of local vegetation (Carrión et al., 2006; Maher, 2006; Batina and Reese, 2011; Forray et al., 2015). Microcharcoal serves as a proxy for fire and together with pollen provides information about the interplay between biomass burning (often anthropogenic) and vegetation change (Forray et al., 2015). Should there be paleontological remains associated with guano deposits, they too can provide a record of the local landscape change (Widga and Colburn, 2015). Given the range of possible proxy records obtainable, cave guano deposits offer a unique opportunity to investigate past climate and environment from multiple angles using the same material.
Conclusions and implications for restoration
Both bat guano carbon and nitrogen isotope values provide important information about climate and environment. By comparing isotopic values from a relatively young, high-resolution guano core, we have reaffirmed that guano δ13C values reflect both water availability and the extent of C3 vs. C4 vegetation in the surrounding landscape while guano δ15N values are strongly influenced by climate, agricultural practices, and the interaction between these two factors. Importantly, bat guano records can provide information about past climate and environment in places where paleoecological records were previously unobtainable. The humid tropics, for example, host much of the Earth's biodiversity and play an important role in global climate, but tropical paleoenvironmental records are limited, in large part, due to preservation issues. Bat guano, which can accumulate over millennia, is already providing crucial information about climate and environment in equatorial Southeast Asia as far back as the last glacial maximum (e.g., Wurster et al., 2010b).
Combined with additional proxy records from the same material (e.g., pollen, microcharcoal), bat guano isotopic records can help to clarify the characteristics of ecosystems that occurred in a site before human disturbance, identify the range of ecosystem types that have previously been supported in a particular area, and uncover the long-term ecosystem trajectory. We advocate for paleoecologists and restoration practitioners to collaborate to set goals and maximize the utility of the information these types of records can provide. By analyzing guano core δ13C values, researchers will be able to place quantitative estimates on the relative abundance of C3 and C4 vegetation on the landscape. The inclusion of pollen analyses will help to contextualize these results by providing information about the types of plants present; if changes in guano carbon isotope values are not accompanied by changes in pollen composition, it would suggest that changes in precipitation, rather than plant composition, are driving the isotopic shift. In relatively young accumulations from areas likely to have been impacted heavily by agriculture, guano core δ15N values can provide further information about past precipitation. Future work is, however, necessary to further clarify the relationship between the guano δ15N values, precipitation, and land use.
Particularly now, during the UN Decade on Ecosystem Restoration, restoration plans must incorporate information about the past ecosystems supported by a site as well as the range of climates in which those ecosystems existed. For example, in the eastern and midwestern United States, and in some tropical ecosystems, there is increasing evidence that natural grasslands have been mesified and forested (e.g., Paulson et al., 2016; Arasumani et al., 2019; Bonanomi et al., 2019); restoration efforts that do not take this information into account could erroneously establish forest in a place where grassland would be more appropriate. Bat guano accumulations in the southeastern United States, for example, are presently untapped archives that could provide information about the extent of forest vs. grasslands before European colonization and potentially even before human presence.
Data availability statement
The original contributions presented in the study are included in the article/Supplementary material, further inquiries can be directed to the corresponding author/s.
Author contributions
RR, CE, and XL designed the study. RR, JW, and DJ conducted laboratory work and data analyses. RR, JW, DJ, CE, and XL wrote the paper. All authors contributed to the article and approved the submitted version.
Funding
The funding for this research was provided by the Living Earth Collaborative and the International Center for Energy, Environment and Sustainability. The Virginia Tech Open Access Subvention Fund provided funding to support the publication of this article.
Acknowledgments
We thank Kevin Hedgpeth, Rhonda Rimer, and Kathryn Bulliner of the Missouri Department of Conservation for assistance in the field and for gathering information about the history of Mary Lawson Cave. We also thank Washington University undergraduate students, Mekhi Airhart and Alice Xu, for assistance in the lab and field. Finally, we thank the reviewers and editor for their constructive comments.
Conflict of interest
The authors declare that the research was conducted in the absence of any commercial or financial relationships that could be construed as a potential conflict of interest.
Publisher's note
All claims expressed in this article are solely those of the authors and do not necessarily represent those of their affiliated organizations, or those of the publisher, the editors and the reviewers. Any product that may be evaluated in this article, or claim that may be made by its manufacturer, is not guaranteed or endorsed by the publisher.
Supplementary material
The Supplementary Material for this article can be found online at: https://www.frontiersin.org/articles/10.3389/fevo.2022.929220/full#supplementary-material
References
Altman, N., and Krzywinski, M. (2016). Analyzing outliers: influential or nuisance? Nat. Methods 13, 281–282. doi: 10.1038/nmeth.3812
Amundson, R., Austin, A. T., Schuur, E. A. G., Yoo, K., Matzek, V., Kendall, C., et al. (2003). Global patterns of the isotopic composition of soil and plant nitrogen. Glob. Biogeochem. Cycles 17, 31-1–31-10. doi: 10.1029/2002GB001903
Anderson, J. R., Hardy, E. E., Roach, J. T., and Witmer, R. E. (1976). “A land use and land cover classification system for use with remote sensor data,” in Geological Survey Professional Paper. Washington, DC: United States Government Printing Office. doi: 10.3133/pp964
Arasumani, M., Khan, D., Vishnudas, C. K., Muthukumar, M., Bunyan, M., and Robin, V. V. (2019). Invasion compounds an ecosystem-wide loss to afforestation in the tropical grasslands of the Shola Sky Islands. Biol. Conserv. 230, 141–150. doi: 10.1016/j.biocon.2018.12.019
Arias-Ortiz, A., Masqué, P., Garcia-Orellana, J., Serrano, O., Mazarrasa, I., Marbà, N., et al. (2018). Reviews and syntheses: 210Pb-derived sediment and carbon accumulation rates in vegetated coastal ecosystems setting the record straight. Biogeosciences 15, 6791–6818. doi: 10.5194/bg-15-6791-2018
Austin, A. T., and Vitousek, P. M. (1998). Nutrient dynamics on a precipitation gradient in Hawai'i. Oecologia 113, 519–529. doi: 10.1007/s004420050405
Barclay, R. M. R., and Brigham, R. M. (1994). Constraints on optimal foraging: a field test of prey discrimination by echolocating insectivorous bats. Anim. Behav. 48, 1013–1021. doi: 10.1006/anbe.1994.1334
Bardgett, R. D., and Wardle, D. A. (2003). Herbivore-mediated linkages between aboveground and belowground communities. Ecology 84, 2258–2268. doi: 10.1890/02-0274
Baskaran, M. (2011). Po-210 and Pb-210 as atmospheric tracers and global atmospheric Pb-210 fallout: a Review. J. Environ. Radioact. 102, 500–513. doi: 10.1016/j.jenvrad.2010.10.007
Baskin, J. M., and Baskin, C. C. (2000). Vegetation of limestone and dolomite glades in the Ozarks and midwest regions of the United States. Ann. Missouri Botan. Garden 87:286. doi: 10.2307/2666165
Bateman, A. S., and Kelly, S. D. (2007). Fertilizer nitrogen isotope signatures. Isotopes Environ. Health Stud. 43, 237–247. doi: 10.1080/10256010701550732
Batina, M. C., and Reese, C. A. (2011). A Holocene pollen record recovered from a guano deposit: round Spring Cavern, Missouri, USA: a Holocene pollen record from a guano deposit, Round Spring Cavern, USA. Boreas 40, 332–341. doi: 10.1111/j.1502-3885.2010.00186.x
Best, T. L., Milam, B. A., Haas, T. D., Cvilikas, W. S., and Saidak, L. R. (1997). Variation in diet of the gray bat (Myotis grisescens). J. Mammal. 78, 569–583. doi: 10.2307/1382909
Bird, M. I., Boobyer, E. M., Bryant, C., Lewis, H. A., Paz, V., and Stephens, W. E. (2007). A long record of environmental change from bat guano deposits in Makangit Cave, Palawan, Philippines. Earth Environ. Sci. Trans. R. Soc. Edinburgh 98, 59–69. doi: 10.1017/S1755691007000059
Bogaard, A., Heaton, T. H. E., Poulton, P., and Merbach, I. (2007). The impact of manuring on nitrogen isotope ratios in cereals: archaeological implications for reconstruction of diet and crop management practices. J. Archaeol. Sci. 34, 335–343. doi: 10.1016/j.jas.2006.04.009
Boivin, N., and Crowther, A. (2021). Mobilizing the past to shape a better Anthropocene. Nat. Ecol. Evol. 5, 273–284. doi: 10.1038/s41559-020-01361-4
Bonanomi, J., Tortato, F. R., de Gomes, R. S. R., Penha, J. M., Bueno, A. S., and Peresf, C. A. (2019). Protecting forests at the expense of native grasslands: land-use policy encourages open-habitat loss in the Brazilian cerrado biome. Perspect. Ecol. Conserv. 17, 26–31. doi: 10.1016/j.pecon.2018.12.002
Brack, V., and LaVal, R. K. (2006). Diet of the gray myotis (Myotis grisescens): variability and consistency, opportunism, and selectivity. J. Mammal. 87, 7–18. doi: 10.1644/05-MAMM-A-098R1.1
Bronk Ramsey, C. (2009). Bayesian analysis of radiocarbon dates. Radiocarbon 51, 337–360. doi: 10.1017/S0033822200033865
Carrión, J. S., Scott, L., and Marais, E. (2006). Environmental implications of pollen spectra in bat droppings from southeastern Spain and potential for palaeoenvironmental reconstructions. Rev. Palaeobot. Palynol. 140, 175–186. doi: 10.1016/j.revpalbo.2006.03.007
Cerling, T. E., Harris, J. M., MacFadden, B. J., and Leakey, M. G. others. (1997). Global vegetation change through the Miocene/Pliocene boundary. Nature 389:153. doi: 10.1038/38229
Clawson, R. L. (1984). Investigations of Endangered Indiana Bat and Gray Bat Summer Ecology, Distribution and Status. Missouri Department of Conservation Surveys and Investigation Projects Study. p. 66.
Cleary, D. M., Onac, B. P., Forray, F. L., and Wynn, J. G. (2016). Effect of diet, anthropogenic activity, and climate on δ 15 N values of cave bat guano. Palaeogeogr. Palaeoclimatol. Palaeoecol. 461, 87–97. doi: 10.1016/j.palaeo.2016.08.012
Des Marais, D. J., Mitchell, J. M., Meinschein, W. G., and Hayes, J. M. (1980). The carbon isotope biogeochemistry of the individual hydrocarbons in bat guano and the ecology of the insectivorous bats in the region of Carlsbad, New Mexico. Geochim. Cosmochim. Acta 44, 2075–2086. doi: 10.1016/0016-7037(80)90205-7
Diefendorf, A. F., Mueller, K. E., Wing, S. L., Koch, P. L., and Freeman, K. H. (2010). Global patterns in leaf 13C discrimination and implications for studies of past and future climate. Proc. Natl. Acad. Sci. U.S.A. 107, 5738–5743. doi: 10.1073/pnas.0910513107
Dietl, G. P., and Flessa, K. W. (2011). Conservation paleobiology: putting the dead to work. Trends Ecol. Evol. 26, 30–37. doi: 10.1016/j.tree.2010.09.010
Dietl, G. P., Kidwell, S. M., Brenner, M., Burney, D. A., Flessa, K. W., Jackson, S. T., et al. (2015). Conservation paleobiology: leveraging knowledge of the past to inform conservation and restoration. Annu. Rev. Earth Planet. Sci. 43, 79–103. doi: 10.1146/annurev-earth-040610-133349
Elliott, W. R. (2007). “Gray and Indiana bat population trends in Missouri,” in 2007 National Cave and Karst Management Symposium (St. Louis, MO), 16.
Elliott, W. R., Shiels, D., Colatskie, S., and Dzurick, C. (2011). Gray Bat (Myotis grisescens) Thermal Infrared Monitoring in Missouri, 2008-2011. Missouri Department of Conservation, Resource Science Division, 17.
Forray, F. L., Onac, B. P., Tantău, I., Wynn, J. G., Tămaş, T., Coroiu, I., et al. (2015). A Late Holocene environmental history of a bat guano deposit from Romania: an isotopic, pollen and microcharcoal study. quarter. Sci. Rev. 127, 141–154. doi: 10.1016/j.quascirev.2015.05.022
Gallant, L. R., Grooms, C., Kimpe, L. E., Smol, J. P., Bogdanowicz, W., Stewart, R. S., et al. (2020). A bat guano deposit in Jamaica recorded agricultural changes and metal exposure over the last >4300 years. Palaeogeogr. Palaeoclimatol. Palaeoecol. 538:109470. doi: 10.1016/j.palaeo.2019.109470
Glenn, S., Glenn, B., Rieck, C. E., Ely, D. G., and Bush, L. P. (1981). Chemical quality, in vitro cellulose digestion, and yield of tall fescue forage affected by mefluidide. J. Agric. Food Chem. 29, 1158–1161. doi: 10.1021/jf00108a014
Grogan, P., Burns, T. D., and Chapin, F. S. I. I. I. (2000). Fire effects on ecosystem nitrogen cycling in a Californian bishop pine forest. Oecologia 122, 537–544. doi: 10.1007/s004420050977
Handley, L. L., Austin, A. T., Stewart, G. R., Robinson, D., Scrimgeour, C. M., Raven, J. A., et al. (1999). The 15N natural abundance (δ15N) of ecosystem samples reflects measures of water availability. Austr. J. Plant Physiol. 26:185. doi: 10.1071/PP98146
Högberg, P. (1997). Tansley review no. 95 15 N natural abundance in soil-plant systems. New Phytol. 137, 179–203. doi: 10.1046/j.1469-8137.1997.00808.x
Högberg, P., Johannisson, C., and Högberg, M. N. (2014). Is the high 15N natural abundance of trees in N-loaded forests caused by an internal ecosystem N isotope redistribution or a change in the ecosystem N isotope mass balance? Biogeochemistry 117, 351–358. doi: 10.1007/s10533-013-9873-x
Högberg, P., Johannisson, C., Yarwood, S., Callesen, I., Näsholm, T., Myrold, D. D., et al. (2011). Recovery of ectomycorrhiza after ‘nitrogen saturation' of a conifer forest. New Phytol. 189, 515–525. doi: 10.1111/j.1469-8137.2010.03485.x
Hua, Q., Turnbull, J. C., Santos, G. M., Rakowski, A. Z., Ancapichún, S., De Pol-Holz, R., et al. (2022). Atmospheric radiocarbon for the period 1950–2019. Radiocarbon 64, 723–745. doi: 10.1017/RDC.2021.95
Hussain, M. Z., Robertson, G. P., Basso, B., and Hamilton, S. K. (2020). Leaching losses of dissolved organic carbon and nitrogen from agricultural soils in the upper US Midwest. Sci. Tot. Environ. 734:139379. doi: 10.1016/j.scitotenv.2020.139379
Kohn, M. J. (2010). Carbon isotope compositions of terrestrial C3 plants as indicators of (paleo) ecology and (paleo) climate. Proc. Natl. Acad. Sci. U.S.A. 107, 19691–19695. doi: 10.1073/pnas.1004933107
Krishnaswamy, S., Lal, D., Martin, J. M., and Meybeck, M. (1971). Geochronology of lake sediments. Earth Planet. Sci. Lett. 11, 407–414. doi: 10.1016/0012-821X(71)90202-0
Lam, M. M. -Y., Martin-Creuzburg, D., Rothhaupt, K. -O., Safi, K., Yohannes, E., and Salvarina, I. (2013). Tracking diet preferences of bats using stable isotope and fatty acid signatures of faeces. PLoS ONE 8:e83452. doi: 10.1371/journal.pone.0083452
Maher, L. J. (2006). Environmental information from guano palynology of insectivorous bats of the central part of the United States of America. Palaeogeogr. Palaeoclimatol. Palaeoecol. 237, 19–31. doi: 10.1016/j.palaeo.2005.11.026
Marshall, F., Reid, R. E. B., Goldstein, S., Storozum, M., Wreschnig, A., Hu, L., et al. (2018). Ancient herders enriched and restructured African grasslands. Nature 561, 387–390. doi: 10.1038/s41586-018-0456-9
Martin, C. O. (2007). Assessment of the Population Status of the Gray Bat (Myotis grisescens); Status Review, DoD Initiatives, and Results of a Multi-Agency Effort to Survey Wintering Populations at Major Hibernacula, 2005-2007. Fort Belvoir, VA: Defense Technical Information Center. doi: 10.21236/ADA473199
Maxwell, S. K., Wood, E. C., and Janus, A. (2008). Comparison of the USGS 2001 NLCD to the 2002 USDA census of agriculture for the upper midwest United States. Agric. Ecosyst. Environ. 127, 141–145. doi: 10.1016/j.agee.2008.03.012
McFarlane, D. A., Keeler, R. C., and Mizutani, H. (1995). Ammonia volatilization in a Mexican bat cave ecosystem. Biogeochemistry 30, 1–8. doi: 10.1007/BF02181037
McFarlane, D. A., and Lundberg, J. (2021). Geochronological implications of 210Pb and 137Cs mobility in cave guano deposits. Int. J. Speleol. 50, 239–248. doi: 10.5038/1827-806X.50.3.2391
McFarlane, D. A., Lundberg, J., and Fincham, A. G. (2002). A late Quaternary paleoecological record from caves of southern Jamaica, West Indies. J. Cave Karst Stud. 64, 117–125. Available online at: https://caves.org/pub/journal/PDF/V64/cave_64-02-fullr.pdf
Mizutani, H., Hasegawa, H., and Wada, E. (1986). High nitrogen isotope ratio for soils of seabird rookeries. Biogeochemistry 2, 221–247. doi: 10.1007/BF02180160
Mizutani, H., McFarlane, D. A., and Kabaya, Y. (1992a). Carbon and nitrogen isotopic signatures of bat guanos as record of past environments. Mass Spectrosc. 40, 67–82. doi: 10.5702/massspec.40.67
Mizutani, H., McFarlane, D. A., and Kabaya, Y. (1992b). Nitrogen and carbon isotope study of bat guano core from Eagle Creek Cave, Arizona, USA. Mass Spectrosc. 40, 57–65. doi: 10.5702/massspec.40.57
Moser, W. K., Hansen, M. H., and Nelson, M. D. (2008). “The extent of selected non-native invasive plants on Missouri forestland,” in Proceedings of the 16th Central Hardwoods Forest Conference, 491–505.
NOAA National Centers for Environmental Information. (2019). State of the Climate: El Niño/Southern Oscillation for Annual 2008. Available online at: https://www.ncei.noaa.gov/access/monitoring/monthly-report/enso/200813 (accessed August 18, 2022).
Onac, B. P., Forray, F. L., Wynn, J. G., and Giurgiu, A. M. (2014). Guano-derived δ13C-based paleo-hydroclimate record from Gaura cu Musca Cave, SW Romania. Environ. Earth Sci. 71, 4061–4069. doi: 10.1007/s12665-013-2789-x
Paulson, A. K., Sanders, S., Kirschbaum, J., and Waller, D. M. (2016). Post-settlement ecological changes in the forests of the Great Lakes National Parks. Ecosphere 7:e1490. doi: 10.1002/ecs2.1490
Phelan, P., Moloney, A. P., McGeough, E. J., Humphreys, J., Bertilsson, J., O'Riordan, E. G., et al. (2015). Forage legumes for grazing and conserving in ruminant production systems. Crit. Rev. Plant Sci. 34, 281–326. doi: 10.1080/07352689.2014.898455
Rick, T. C., and Sandweiss, D. H. (2020). Archaeology, climate, and global change in the Age of Humans. Proc. Natl. Acad. Sci. U.S.A. 117, 8250–8253. doi: 10.1073/pnas.2003612117
Robbins, J. A., and Edgington, D. N. (1975). Determination of recent sedimentation rates in Lake Michigian using Pb-210 ad CS-137. Geochim. Cosmochim. Acta 39, 285–304. doi: 10.1016/0016-7037(75)90198-2
Robinson, D. (2001). δ15N as an integrator of the nitrogen cycle. Trends Ecol. Evol. 16, 153–162. doi: 10.1016/S0169-5347(00)02098-X
Salvarina, I., Yohannes, E., Siemers, B. M., and Koselj, K. (2013). Advantages of using fecal samples for stable isotope analysis in bats: evidence from a triple isotopic experiment: stable isotope analysis in bat fecal samples. Rapid Commun. Mass Spectrom. 27, 1945–1953. doi: 10.1002/rcm.6649
Sigurdarson, J. J., Svane, S., and Karring, H. (2018). The molecular processes of urea hydrolysis in relation to ammonia emissions from agriculture. Rev. Environ. Sci. Bio/Technol. 17, 241–258. doi: 10.1007/s11157-018-9466-1
Singer, F. J., and Schoenecker, K. A. (2003). Do ungulates accelerate or decelerate nitrogen cycling? For. Ecol. Manage. 181, 189–204. doi: 10.1016/S0378-1127(03)00133-6
Stewart, G., Turnbull, M., Schmidt, S., and Erskine, P. (1995). 13C Natural abundance in plant communities along a rainfall gradient: a biological integrator of water availability. Funct. Plant Biol. 22:51. doi: 10.1071/PP9950051
Stotz, G. C., Gianoli, E., and Cahill, J. F. (2019). Biotic homogenization within and across eight widely distributed grasslands following invasion by Bromus inermis. Ecology 100, 1–13. doi: 10.1002/ecy.2717
Swap, R. J., Aranibar, J. N., Dowty, P. R., Gilhooly, W. P., and Macko, S. A. (2004). Natural abundance of 13C and 15N in C3 and C4 vegetation of southern Africa: patterns and implications. Glob. Change Biol. 10, 350–358. doi: 10.1111/j.1365-2486.2003.00702.x
Szpak, P. (2014). Complexities of nitrogen isotope biogeochemistry in plant-soil systems: implications for the study of ancient agricultural and animal management practices. Front. Plant Sci. 5:288. doi: 10.3389/fpls.2014.00288
Tuttle, M. D. (1976). Population ecology of the gray bat (Myotis grisescens): factors influencing growth and survival of newly volant young. Ecology 57, 587–595. doi: 10.2307/1936443
Tuttle, M. D. (1979). Status, causes of decline, and management of endangered gray bats. J. Wildl. Manage. 43:1. doi: 10.2307/3800631
Wachendorf, C., Taube, F., and Wachendorf, M. (2005). Nitrogen leaching from 15N labelled cow urine and dung applied to grassland on a sandy soil. Nutr. Cycl. Agroecosyst. 73, 89–100. doi: 10.1007/s10705-005-8313-2
Wagner, D. L. (2020). Insect declines in the anthropocene. Annu. Rev. Entomol. 65, 457–480. doi: 10.1146/annurev-ento-011019-025151
Waples, J. T. (2020). Measuring bismuth-210, its parent, and daughter in aquatic systems. Limnol. Oceanogr. 18, 148–162. doi: 10.1002/lom3.10352
Waring, C., Hankin, S., Solomon, S., Long, S., Yule, A., Blackley, R., et al. (2021). Cave radon exposure, dose, dynamics and mitigation. J. Cave Karst Stud. 83, 1–19. doi: 10.4311/2019ES0124
Wepprich, T., Adrion, J. R., Ries, L., Wiedmann, J., and Haddad, N. M. (2019). Butterfly abundance declines over 20 years of systematic monitoring in Ohio, USA. PLoS ONE 14:e0216270. doi: 10.1371/journal.pone.0216270
Whiteman, J., Elliott Smith, E., Besser, A., and Newsome, S. (2019). A guide to using compound-specific stable isotope analysis to study the fates of molecules in organisms and ecosystems. Diversity 11:8. doi: 10.3390/d11010008
Wickham, J., Stehman, S. V., Gass, L., Dewitz, J. A., Sorenson, D. G., Granneman, B. J., et al. (2017). Thematic accuracy assessment of the 2011 National Land Cover Database (NLCD). Remote Sensing Environ. 191, 328–341. doi: 10.1016/j.rse.2016.12.026
Widga, C., and Colburn, M. (2015). Paleontology and paleoecology of guano deposits in Mammoth Cave, Kentucky, USA. Quarter. Res. 83, 427–436. doi: 10.1016/j.yqres.2015.01.008
Wingard, G. L., Bernhardt, C. E., and Wachnicka, A. H. (2017). The role of paleoecology in restoration and resource management—the past as a guide to future decision-making: review and example from the greater everglades ecosystem, U.S.A. Front. Ecol. Evol. 5:11. doi: 10.3389/fevo.2017.00011
Wurster, C., McFarlane, D., Bird, M., Ascough, P., and Beavan Athfield, N. (2010a). Stable isotopes of subfossil bat guano as a long-term environmental archive: insights from a grand canyon cave deposit. J. Cave Karst Stud. 72, 111–121. doi: 10.4311/jcks2009es0109
Wurster, C. M., Bird, M. I., Bull, I. D., Creed, F., Bryant, C., Dungait, J. A. J., et al. (2010b). Forest contraction in north equatorial Southeast Asia during the Last Glacial Period. Proc. Natl. Acad. Sci. U.S.A. 107, 15508–15511. doi: 10.1073/pnas.1005507107
Wurster, C. M., McFarlane, D. A., and Bird, M. I. (2007). Spatial and temporal expression of vegetation and atmospheric variability from stable carbon and nitrogen isotope analysis of bat guano in the southern United States. Geochim. Cosmochim. Acta 71, 3302–3310. doi: 10.1016/j.gca.2007.05.002
Wurster, C. M., Patterson, W. P., McFarlane, D. A., Wassenaar, L. I., Hobson, K. A., Athfield, N. B., et al. (2008). Stable carbon and hydrogen isotopes from bat guano in the Grand Canyon, USA, reveal Younger Dryas and 8.2 ka events. Geology 36, 683–686. doi: 10.1130/G24938A.1
Wurster, C. M., Rifai, H., Haig, J., Titin, J., Jacobsen, G., and Bird, M. (2017). Stable isotope composition of cave guano from eastern Borneo reveals tropical environments over the past 15,000 cal yr BPalaeogeography, P., Palaeoclimatology. Palaeoecology 473, 73–81. doi: 10.1016/j.palaeo.2017.02.029
Keywords: guano, carbon, nitrogen, time series, precipitation, vegetation, restoration
Citation: Reid REB, Waples JT, Jensen DA, Edwards CE and Liu X (2022) Climate and vegetation and their impact on stable C and N isotope ratios in bat guano. Front. Ecol. Evol. 10:929220. doi: 10.3389/fevo.2022.929220
Received: 26 April 2022; Accepted: 09 August 2022;
Published: 15 September 2022.
Edited by:
Chris Schneider, University of Alberta, CanadaReviewed by:
Christopher Junium, Syracuse University, United StatesMichelle Casey, Towson University, United States
Copyright © 2022 Reid, Waples, Jensen, Edwards and Liu. This is an open-access article distributed under the terms of the Creative Commons Attribution License (CC BY). The use, distribution or reproduction in other forums is permitted, provided the original author(s) and the copyright owner(s) are credited and that the original publication in this journal is cited, in accordance with accepted academic practice. No use, distribution or reproduction is permitted which does not comply with these terms.
*Correspondence: Rachel E. B. Reid, cmVicmVpZCYjeDAwMDQwO3Z0LmVkdQ==