- 1College of Wildlife and Protected Areas, Northeast Forestry University, Harbin, China
- 2National Nature Reserve of Helan Mountains in Ningxia, Yinchuan, China
- 3Chengdu Research Base of Giant Panda Breeding, Chengdu, China
- 4Key Laboratory of Conservation Biology, National Forestry and Grassland Administration, Harbin, China
Studying the coexistence mechanisms of sympatric wildlife helps to shed light on why the earth has so many different species. When ungulates share ranges, food and habitat requirements may partially or fully overlap. Therefore, the aim of this study was to determine how sympatric ungulates share limited resources. Carcasses of 27 adult blue sheep (Pseudois nayaur) and three adult red deer (Cervus elaphus alxaicus) were collected in the Helan Mountains, China. Nutritive indices of plant species foraged and morphometric measurements of the digestive system of the two sympatric ungulates were determined. In addition, 120 passive, infrared motion-triggered cameras recorded spatial overlap and temporal overlap between the two species. Camera trapping revealed relatively limited spatial overlap and significantly different activity rhythms between blue sheep and red deer. Differences were also observed in stomach weight, surface enlargement factor of the rumen, and intestine length between the two species. However, the combined relative weight of the stomach and intestine was not different between species. The low spatiotemporal overlap decreased opportunities for encounters between sympatric blue sheep and red deer, and significant differences in digestive systems allowed the two species to consume different plant species or different parts of the same species. Thus, the two sympatric ungulates coexist harmoniously in the Helan Mountains because of long-term evolutionary behavioral and physiological adaptations that eliminate negative effects on the survival of the other species.
Introduction
Sympatric species live within a defined range of environmental space and constitute the animal community of an area. A complex of biological, environmental, evolutionary, and historical factors determine the coexistence of animals in a given area (Tokeshi, 2009). Generally, morphologically similar or closely related sympatric species are expected to compete for limited resources such as food, space, and shelter (Schoener, 1974; Hofmann et al., 2016; Frey et al., 2017). However, such interspecific competition is detrimental to each species and ultimately reduces species fitness (Forsyth and Hickling, 1998; Ferretti et al., 2015). Competition for resources between sympatric species may even affect the health of offspring because of the negative effects on body weight (Richard et al., 2010). Therefore, interspecific interactions, such as competition, are crucial drivers in structuring animal communities (Hutchinson, 1959). Competition is the central link between limited resources and niche differentiation, and it is not only the result of the former but it is also the cause of the latter. The strong connections among resources, competition, and niches affect species’ coexistence (Xian, 2008). Most of the competition between sympatric species appears to be a mechanism to increase niche partitioning. Thus, sympatric species tend to differ ecologically and have different adaptive strategies or use different spatial, temporal, or feeding dimensions of a niche (Schoener, 1974; Bagchi et al., 2003). Comparative studies of sympatric species are critical to understanding behavioral and ecological adaptations and mechanisms that primarily reduce competition for resources and allow coexistence (Zhou et al., 2013).
Ungulate communities have essential ecological roles in mountain forests by maintaining floral structures and contributing to nutrient cycles (Reyna-Hurtado and Tanner, 2007; Chanchani et al., 2010). Niche segregation associated with differences in ungulate body weight and digestive morphology can explain the species diversity in large herbivore communities (Redjadj et al., 2014). In general, niche segregation is an important mechanism that allows sympatric species to coexist stably and harmoniously. Accordingly, studies on coexistence mechanisms of sympatric mountain ungulates can help shed light on mechanisms controlling the diversity of mountain communities (Rahbek et al., 2019). Typically, ungulate diets depend on physiological factors that determine the food range, such as body weight. By contrast, environmental factors, such as seasonality and interspecific interactions, determine ungulate food availability (Storms et al., 2008). Commonly, relatively large species can utilize relatively low-quality food, and as a result, those species do not need to select sparsely distributed high-quality food, although they do need to consume more low-quality food. By contrast, relatively small species consume less food and therefore can utilize relatively rare food sources of relatively high quality (Bell, 1971; Jarman, 1974; Mueller et al., 2013). The size of the ungulate gastrointestinal tract is a critical element that affects digestive efficiency, and longer digestive tracts result in longer and better processing of low-quality food. Thus, large ungulates can digest food with relatively high levels of fiber, whereas the opposite is true for small ungulates (DÍAz et al., 2007; Ruifrok et al., 2015). Consequently, food selection by ungulates is based on a trade-off between food quality, as determined by protein and energy digestibility and phenolic and terpenoid concentrations, among other factors, and quantity, as determined by feeding efficiency and abundance of host plants, among other factors (Shipley et al., 1998).
Blue sheep (Pseudois nayaur) and red deer (Cervus elaphus alxaicus) are the most abundant and widely distributed ungulates in the Helan Mountains, China (Yu et al., 2004; Cao et al., 2005; Liu et al., 2009). Both species are listed as national key protected class II wildlife of China and the least concerned globally by the International Union for Conservation of Nature (IUCN) (Harris, 2014; Lovari et al., 2018). Blue sheep are distributed throughout the Helan Mountains in various habitats at elevations from 1,400 to 3,556 m above sea level but prefer sparse mountain forests and grasslands below 2,000 m. The mean group size is three to six sheep (Wang et al., 1998; Yu et al., 2004; Cao et al., 2005; Liu et al., 2005a,b). Red deer are mainly distributed in the middle area of the Helan Mountains at a preferred elevation of ∼1,700 m. The deer prefer coniferous mountain forests, sparse mountain forests, and grasslands and occur in groups of 1–15 deer (Liu et al., 2009; Luo et al., 2009). According to the physiological characteristics of the ruminants and the morphological characteristics of the different plants consumed, both species are intermediate between concentrate selectors/browsers and roughage eaters/grazers and therefore can be classified as mixed feeders (Prins and Geelen, 1971; Hofmann, 1989; Schaller, 2000; Shrestha et al., 2005). Because of the demand for similar nutrition, there may be competition for food between sympatric blue sheep and red deer (Darmon et al., 2012). Some studies show that there are differences in body sizes and spatial overlaps in ranges of sympatric blue sheep and red deer in the Helan Mountains, resulting in a relatively high overlap of food resource utilization, especially during winter (Liu et al., 2005a,b, 2009; Luo et al., 2009; Luo, 2011). However, spatiotemporal interactions and adaptations of physiological structures of sympatric blue sheep and red deer are not always estimated properly or simultaneously.
Blue sheep and red deer are dominant ungulates in the Helan Mountains, and habitats of the two species overlap to a certain extent (Liu et al., 2005a,2009; Luo, 2011). Analyses of the coexistence mechanisms of sympatric blue sheep and red deer in the Helan Mountains can reveal how some axes of the two ecological niches differ to reduce competition for resources and allow coexistence. In particular, behavioral data and morphological measurements can help to comprehensively explain some of the mechanisms that allow coexistence based on the external and internal characteristics of each species. Therefore, in this study, the spatiotemporal overlap and gastrointestinal tract differences between sympatric blue sheep and red deer in the Helan Mountains were examined (Supplementary Figures 1, 2). In addition, the mechanisms that allow the two sympatric ungulates with similar resource requirements to coexist were explored from the perspectives of space, time, and food. It was hypothesized that the use of space and activity patterns of blue sheep and red deer would differ to reduce interspecies encounters and potential competition. It was also hypothesized that if the two ungulates did have similar space use and activity patterns, then the two species would consume different plant species or different parts of the same plant species, which would lead to differences in different parts of the gastrointestinal tract. The findings of this study can be a reference for further studies on the coexistence mechanisms of sympatric species.
Materials and methods
Study area
The Helan Mountains National Nature Reserve of the Ningxia Hui Autonomous Region is located in Northwest China (38°21’ to 39°22’N, 105°49’ to 106°42’E; Figure 1). Elevation ranges from 1,085 to 3,556 m a.s.l. The Helan Mountains are surrounded by the Ulan Buh Desert in the north, the Mu Us Desert in the east, and the Tengger Desert in the west, and thus, the elevations and habitats of the mountains differ significantly from adjacent regions. The study area covers approximately 323 km2, which constitutes approximately 11.28% of the nature reserve. The Helan Mountains are an important geographical boundary in Northwest China as the boundary between temperate desert and temperate desert steppe. The mountains are also the watershed between the northwest inflow area and outflow area. In addition, the mountains contain representative and relatively complete natural ecosystems of arid and semiarid regions in China. The mean annual temperature is −0.9°C (high, 25.2°C; low, −31°C), and the mean annual precipitation is 420 mm (Luo, 2011). The Helan Mountains are a typical temperate mountainous forest system characterized by four types of vertically distributed vegetation: mountain steppe forests, mountain sparse forests and grasslands, mountain coniferous forests, and subalpine scrub and meadows (Di, 1987; Di et al., 1988). In addition to blue sheep and red deer, other notable species inhabit the mountains, such as snow leopards (Panthera uncial), goitered gazelles (Gazella subgutturosa), and alpine musk deer (Moschus chrysogaster), although distributions and populations of those species are very limited. There are also red foxes (Vulpes vulpes), Eurasian badgers (Meles meles), and cape hares (Lepus capensis). Because they are the dominant ungulates of the Helan Mountains, the combination of blue sheep and red deer was ideal to study the mechanisms of coexistence in species with similar living space and food requirements.
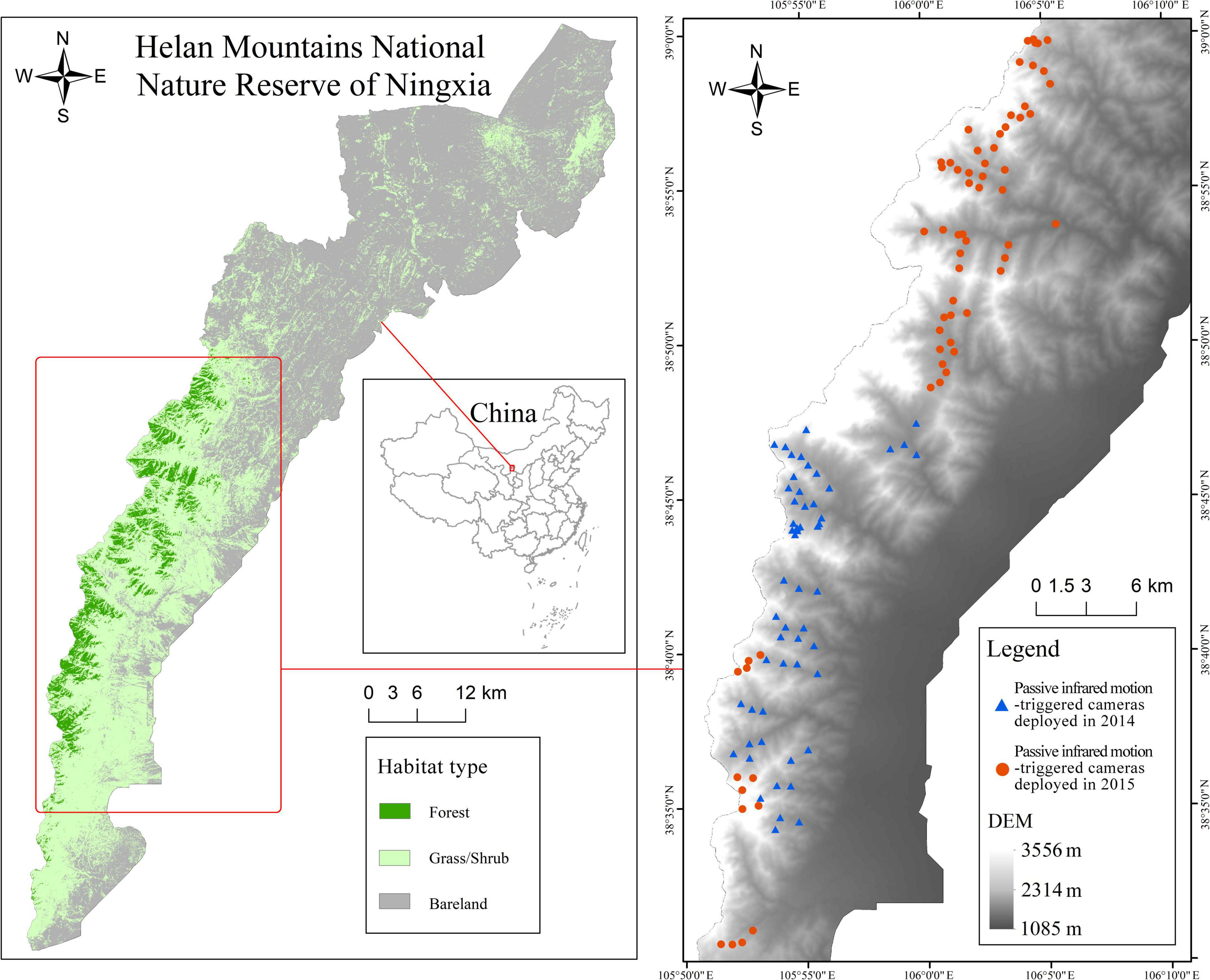
Figure 1. Study area map depicting camera stations and vegetation covers (left, the location of Helan Mountains National Nature Reserve of Ningxia in China and its vegetation covers, which are represented by different colors; right, the location where the passive infrared motion-triggered cameras were deployed to monitor the activity of blue sheep and red deer).
Activity monitoring of blue sheep and red deer
To monitor the activities of the two species, 120 passive, infrared motion-triggered cameras (Ltl 6210 MC; Ltl Acorn Electronics Co., Ltd., Zhuhai, China) were deployed in different habitats (i.e., forest, shrub, grassland) in which blue sheep and red deer co-occurred from October 2014 to October 2015 (Figure 1). To avoid pseudoreplication, the distance between two cameras was always greater than 500 m (Li et al., 2018; Wan et al., 2020). According to the body sizes of blue sheep and red deer and conditions of specific habitats, cameras were bound to tree trunks 50–80 cm aboveground. Cameras were set to work continuously for 24 h a day and to take two photos and a 15-s video when triggered by any passing species. Cameras were checked every 30 days to download data and replace batteries. Fifty-five cameras were set in 2014, 65 cameras in 2015, and the average camera day was 50 days (47 days in 2014 and 53 days in 2015).
Measurements of plants nutrition
Based on the previous research on the diets of blue sheep and red deer in the Helan Mountains (Luo, 2011), plants consumed by each species were collected in the habitats where they co-occurred. To sample plants, transects were randomly arranged in the study area, starting at the valley and ending at the ridge. A quadrat (1 m × 1 m) was set for each 100 m increase in altitude on a transect and there were five to 10 quadrats per transect. Based on fully investigating the feeding habits of blue sheep and red deer (Luo, 2011), parts of the quadrats were selected to collect the main forage plants. Plants in quadrats were identified as species according to the Flora of Helan Mountains (Zhu et al., 2011). Samples of each plant were collected from at least 20 quadrats, and the dry weight of each sample was more than 200 g. Because there were many species in the genera Stipa, Carex, and Artemisia, it was difficult to collect samples of only individual species. Therefore, in each genus, species were mixed with equal dry weights. Stipa included S. breviflora, S. krylovii, and S. grandis; Carex included C. duriuscula, C. lanceolata, and C. korshinskyi; and Artemisia included A. sacrorum, A. frigida, and A. mongolica. And then, the feed proximate analysis (Shen, 2009; Afolabi et al., 2021; Olawuwo et al., 2022) was used to determine nutritive indices of the plants, namely, water (W), crude ash (CA), crude protein (CP), crude fat (CFA), crude fiber (CF), nitrogen-free extract (NFE), neutral detergent fiber (NDF), energy (E), and tannins (T).
Morphometric measurements
Carcasses of 27 adult blue sheep (age: 3–10 years; body weight: 16–46 kg; shoulder height: 62–80 cm) and three adult red deer (age: 2–10 years; body weight: 99–109 kg; shoulder height: 110–130 cm) that died of accidental fall or illness, were hunted by natural predators, or died accidentally at a water source were collected (Supplementary Table 2). Data on carcass external morphologies (i.e., body weight, shoulder height, and body length) and stomach and intestine were collected (Supplementary Table 1). All carcasses were fresh (died within 1–3 days and had not begun to decompose) and taken to the laboratory for measurements as soon as they were found. An electronic scale (accurate to 1 kg) was used to measure body weight, and a steel tape measure (accurate to 1 cm) was used to measure shoulder height and body length. After those measurements, carcasses were placed on a stainless steel dissecting table. Dissecting scissors were used to cut the body cavity, and all digestive organs were taken for measurement and to collect contents. The weight of the stomach and its various parts was measured with an electronic scale (accurate to 1 g). The stomach weight was measured with contents included and then measured again after removing contents. The length of the intestine was measured with a steel tape measure (accurate to 1 cm). Various passages in the stomach and the mastoid on the rumen surface were measured with vernier calipers (accurate to 0.001 cm).
Statistical analyses
Species, date, time, and other information in photos and videos were recorded. Photos and videos of the same species at the same site with an interval of fewer than 30 min were deleted to reduce pseudoreplication and produce independent photos and videos of each species (O’Brien et al., 2003; Liu et al., 2013; Meredith and Ridout, 2014). Spatial overlap between blue and red deer was estimated with the Pianka index, which ranges between 0 (no overlap) and 1 (total overlap) (Pianka, 1974; Delsinne et al., 2007). The equation to calculate the index was the following: Ojk = (Σpij × pik)/(Σpij2 × Σpik2)1/2 where pij and pik are the proportions of records of species j and k, respectively, at different camera trapping stations. The package “overlap” in R was used to estimate the interspecific overlap of temporal activity patterns of blue sheep and red deer by using the Δ coefficient, with Δ = 0, no overlap; Δ = 1, total overlap; and the Δ4 estimator was used when records were > 75 (Ridout and Linkie, 2009; R Core Team, 2013; Meredith and Ridout, 2014). Temporal overlap was considered high when Δ4 > 0.75, intermediate when 0.50 < Δ4 < 0.75, and low when Δ4 < 0.50 (Monterroso et al., 2014). ArcGIS 10.7 (Environmental Systems Research Institute, Inc., Redlands, California, United States) was used to produce a map of spatial overlap. Mann–Whitney U tests in SPSS 20.0 (International Business Machines Corporation, Armonk, New York, United States) were used to analyze differences in the characteristics of the digestive tract between sympatric blue sheep and red deer, namely, stomach weight and weight of its different parts. Origin 2021 (OriginLab Corporation, Northampton, Massachusetts, United States) was used to produce graphs that compared digestive systems and showed food overlap between blue sheep and red deer.
Results
Spatial overlap of blue sheep and red deer
Cameras (n = 55 in 2014; n = 65 in 2015) monitored 6,080 total trap days (Figure 2) (n = 2,600 days in 2014; n = 3,480 days in 2015) from 2014 to 2015 and produced 2,083 independent photos and videos (n = 2,511 of blue sheep, with n = 1,393 in 2014 and n = 1,114 in 2015; n = 292 of red deer, with n = 126 in 2014 and n = 166 in 2015). One hundred and one cameras (84.17%) captured blue sheep (n = 46 in 2014; n = 55 in 2015), 63 cameras (52.5%) captured red deer (n = 25 in 2014; n = 38 in 2015), and 59 cameras (49.17%) captured both blue sheep and red deer (n = 24 in 2014; n = 35 in 2015). The Pianka index evaluated by the proportion of records of each species in different camera stations was 0.258 (0.348 in 2014 and 0.229 in 2015), which reflected relatively low spatial overlap of blue sheep and red deer. In 2014, there was only one camera that only captured red deer; whereas 22 cameras only captured blue sheep. In 2015, three cameras only captured red deer; whereas 20 cameras only captured blue sheep. In 2014, the number of times red deer were captured on camera varied from 0 to 16 (average = 2.21), whereas the number of times blue sheep were captured on camera varied from 0 to 137 (average = 24.51). In 2015, the number of times red deer were captured on camera varied from 0 to 15 (average = 2.55), whereas the number of times blue sheep were captured on camera varied from 0 to 132 (average = 17.14). The camera that captured blue sheep the highest number of times was not the same camera that captured red deer the highest number of times (2014: blue sheep, n = 137 vs. red deer, n = 0; 2015: blue sheep, n = 132 vs. red deer, n = 6). Similarly, the camera that captured red deer the highest number of times was not the same camera that captured blue sheep the highest number of times (2014: red deer, n = 16 vs. blue sheep, n = 28; 2015: red deer, n = 15 vs. blue sheep, n = 14). Overall, distribution and activity intensity were higher for blue sheep than for red deer, with relatively low spatial overlap (Figure 2). Other species of mammals recorded included red foxes (n = 127) and cape hares (n = 55) in 2014 and red foxes (n = 124), cape hares (n = 62), and Eurasian badgers (n = 35) in 2015.
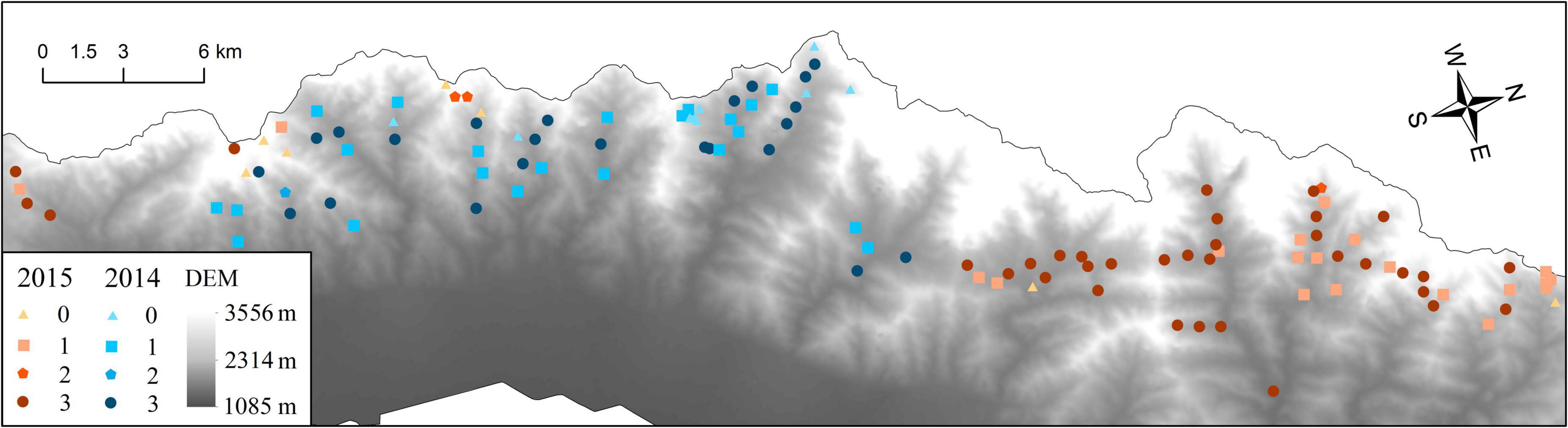
Figure 2. Spatial overlap of blue sheep and red deer (captured condition of cameras: 0 = no blue sheep or red deer were captured, 1 = only blue sheep were captured, 2 = only red deer were captured, and 3 = both blue sheep and red deer were captured).
Temporal overlap of blue sheep and red deer
According to the temporal overlap of blue sheep and red deer (Figure 3), daily activity peaks of both species appeared in the morning and evening. Activity peaks were closer to noon and activity intensity was higher for blue sheep than for red deer. Although similar to the activity pattern of blue sheep, the time between the two activity peaks of red deer was significantly greater than that of blue sheep. Compared with blue sheep, deer daytime activity intensity was also weaker, although the deer nighttime activity intensity was significantly higher. The temporal overlap indicated by the cameras that captured both blue sheep and red deer was Δ4 = 0.598 (Δ4 = 0.527 in 2014; Δ4 = 0.615 in 2015), indicating an intermediate level of temporal overlap between sympatric blue sheep and red deer in the Helan Mountains (Figure 3).
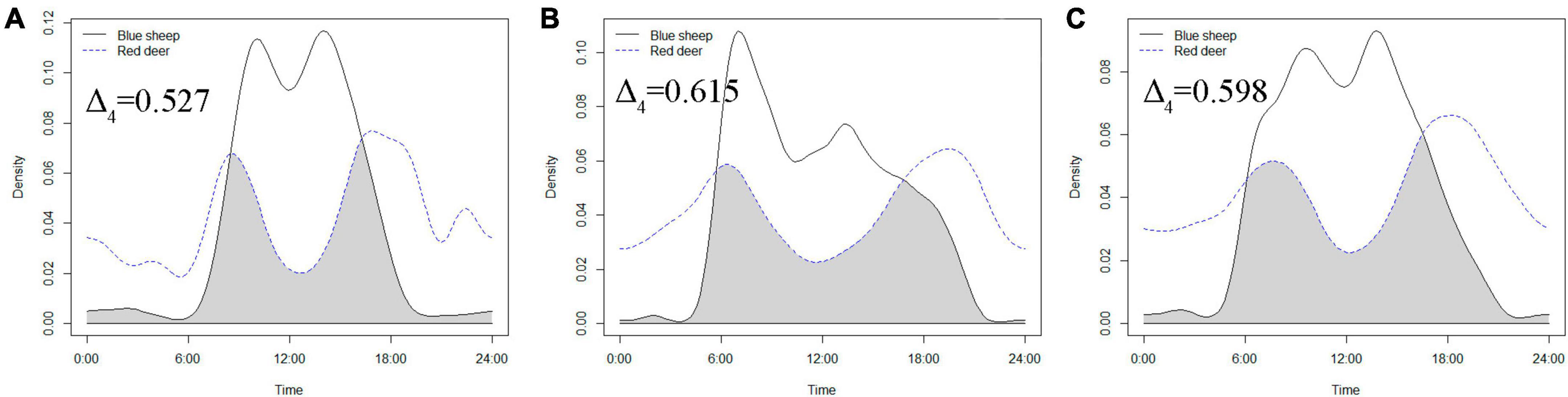
Figure 3. Activity rhythms of blue sheep and red deer in camera traps that captured both species [(A) = activity rhythms of blue sheep and red deer in cameras deployed in 2014, (B) activity rhythms of blue sheep and red deer in cameras deployed in 2015, and (C) activity rhythms of blue sheep and red deer in cameras deployed in 2014 and 2015].
Differences in the digestive system of blue sheep and red deer
Compared with sympatric red deer, stomach tissue weight of blue sheep was significantly lower (Z = −2.907, P = 0.004), as were weights of different stomach parts (rumen–reticulum: Z = −2.907, P = 0.004; omasum: Z = −1.447, P = 0.009; abomasum: Z = −2.849, P = 0.004). Furthermore, there were no differences in the proportion of stomach weight to body weight between species. By contrast, the proportions of weights of different stomach parts to body weights of blue sheep were lower than those of red deer, except for the abomasum (Figure 4A). When evaluating the difference between the contents included in the stomach and its different parts, the difference was significant for only the abomasum weight (contents included) (Z = −2.849, P = 0.004), although only the proportion of abomasum weight (contents included) to body weight was not different between the species. Although the body weight of sympatric blue sheep and red deer was significantly different (Supplementary Table 2), there was no difference in stomach weight (contents included) between species (Figure 4B).
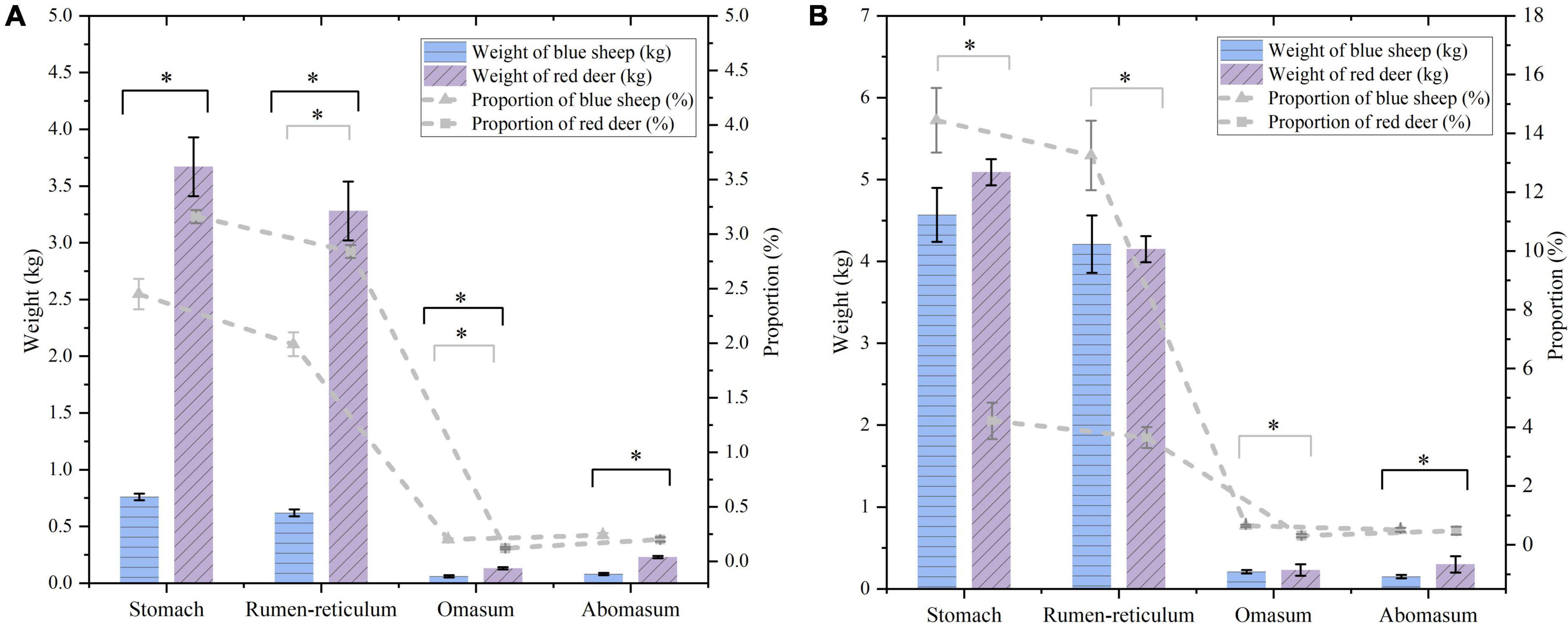
Figure 4. (A) The stomach tissue weight and the proportion of stomach weight to the bodyweight of sympatric blue sheep and red deer (B) the stomach weight (contents included) and the proportion of stomach weight (contents included) to the bodyweight of sympatric blue sheep and red deer (the black and gray error bars represent the standard deviations of the weight and proportion, respectively). *Means there is significant difference between blue sheep and red deer.
When it comes to the size of the various passageways in their stomach, cardias were not significantly different between blue sheep and red deer, whereas the rest of the food passageway was significantly smaller in blue sheep than in red deer. Compared with red deer, the proportion of the size of the omasum–abomasum passage and orifice ileocecal to body length was not different in blue sheep, whereas other proportions of blue sheep were significantly larger than those in red deer (reticulum–omasum passage: Z = −2.220, P = 0.022; cardiac: Z = −2.760, P = 0.001; pylorus: Z = −2.793, P = 0.001) (Supplementary Figure 3).
The overall surface enlargement factor of the rumen was significantly different between blue sheep and red deer (Z = −2.337, P = 0.014). By contrast, papillae number, height, width, and surface enlargement factor were different in different areas of the rumen between the two species (Table 1).
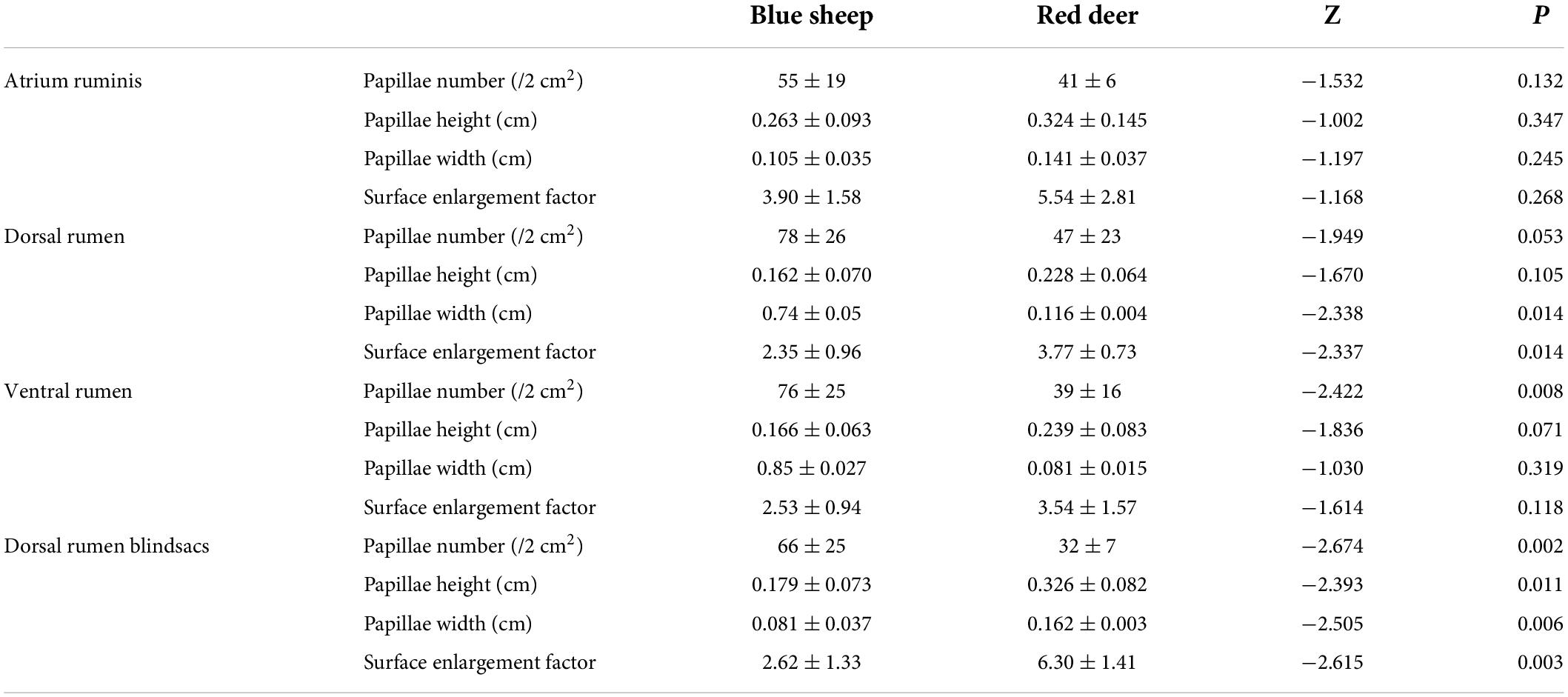
Table 1. The mastoid width, height and number, surface enlargement factor of sympatric blue sheep and red deer.
The proportion of the length of the intestine to the body length of blue sheep was significantly higher than that in red deer (Z = −2.793, P = 0.001). There was no difference in the proportion of the length of the large colon and the rectum to body length between the two species, but there were significant differences in the proportion of the length of the small intestine (Z = −2.806, P < 0.001) and that of the cecum (Z = −2.507, P = 0.006) to body length, with proportions greater in blue sheep than in red deer. The proportion of the length of the small colon to body length was significantly smaller in blue sheep than in red deer (Z = −2.472, P = 0.007, Figure 5A). There were no differences in proportions of the total weight of the intestinal tract or the weights of different parts of the intestine to body weight between sympatric blue sheep and red deer (Figure 5B).
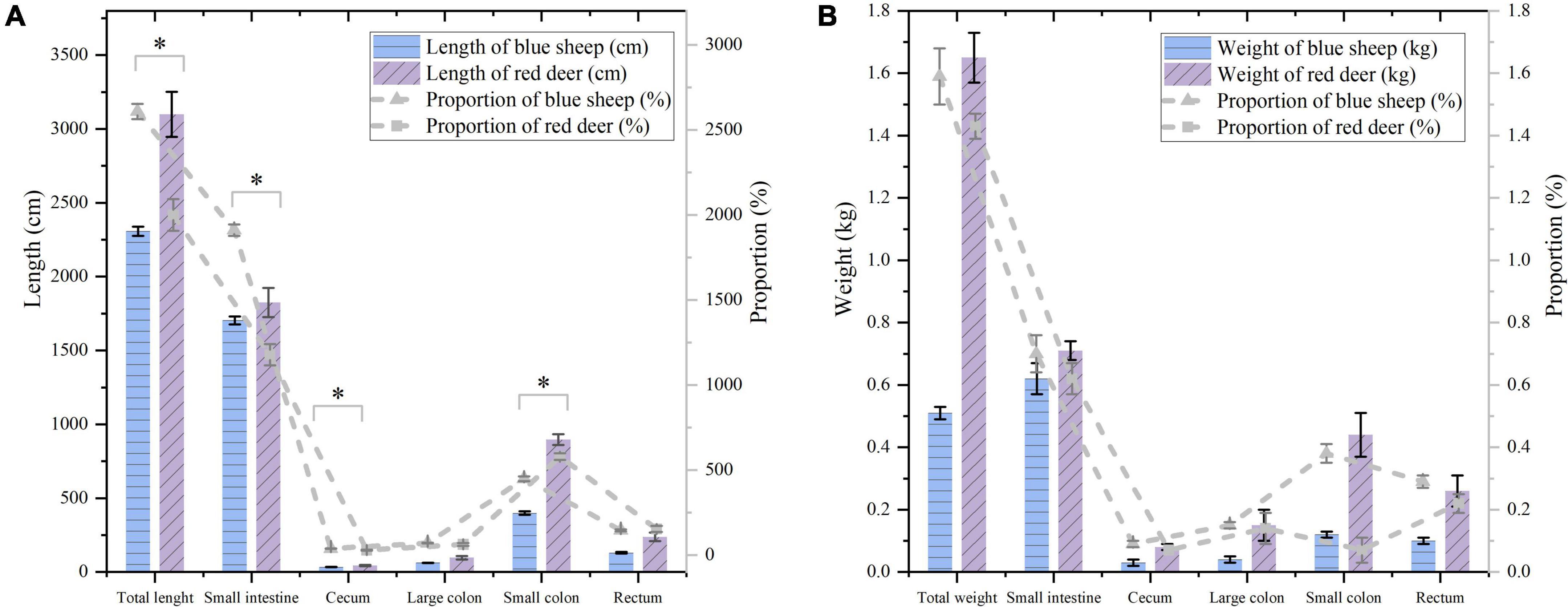
Figure 5. (A) The length of the intestine and the proportion of the length of the intestine to the body length. (B) The weight of the intestine and the proportion of the weight of the intestine to the body weight (the black and gray error bars represent the standard deviations of the weight and proportion, respectively). *Means there is significant difference between blue sheep and red deer.
Discussion
In this study, spatiotemporal overlap and gastrointestinal tract differences between sympatric blue sheep and red deer in the Helan Mountains were examined simultaneously, with the aim to understand behavioral and physiological adaptations that allow the two species to coexist. As hypothesized, the spatiotemporal overlap was relatively low, and different parts of the gastrointestinal tract were significantly different between sympatric blue sheep and red deer. The differences in behavioral and physiological adaptations reduced competition for space and food resources and allowed the two species to successfully coexist in the Helan Mountains, even during winter when food resources are scarce. The findings of this study can provide new insights and ideas for other studies on sympatric species and even for those examining mechanisms to explain species diversity in a given area.
Habitat differentiation is often suggested as the critical mechanism that allows sympatric species to coexist (Schoener, 1974; Lynam et al., 2012; Van Beest et al., 2014). According to previous studies, blue sheep and red deer select different habitats in the Helan Mountains (Liu et al., 2005a,b; Luo et al., 2009). Blue sheep primarily use mountainous sparse forests and grasslands when feeding and resting in different seasons, with no significant preference for slope (Liu et al., 2005b,2009; Luo, 2011). By contrast, red deer prefer mountainous grasslands and coniferous forests, and the slope is an important factor limiting habitat selection (Liu et al., 2009; Luo et al., 2009). Commonly, habitat segregation reduces potential competition between sympatric species and has been documented in several forest-dwelling ungulates (Namgail et al., 2004; Takada et al., 2020). In this study, camera monitoring showed that the spatial overlap of sympatric blue sheep and red deer was relatively low in the Helan Mountains (Figure 2), which reduced competition for spatial resources, such as shelter. As an additional consequence, potential competition for limited food resources was also likely reduced. However, the relatively low spatial overlap might be related to the fact that the population of red deer was much smaller than that of blue sheep in the Helan Mountains in this study, as well as overall. Generally, the population of red deer is approximately 2,000 [2018: n = 2,452; (range: 1,678–3,578) (Huang, 2020)], whereas the population of blue sheep is 15,000–20,000 [2018: n = 10,558; range: 6,784–16,432 (Huang, 2020); in summer 2021: n = 5,176; range: 2,554–10,490; in winter 2021: n = 15,752; range: 7,294–34,017 (Xie et al., 2022)]. The temporal overlap of sympatric blue sheep and red deer was also relatively low, which reduced opportunities for encounters and potential negative interspecific interactions (Figure 3). Typically, temporal niche separation occurs between homogenous species occupying the same habitat (Schoener, 1974; Frey et al., 2017). To summarize, in this study, sympatric blue sheep and red deer showed significant differentiation in activity rhythms, suggesting temporal niche partitioning in order to reduce potential competition. Temporal niche partitioning mechanisms have also been observed within other ungulate communities (Tobler et al., 2009; Darmon et al., 2014; Šprem et al., 2015). Thus, behavioral adaptations were one of the key mechanisms for coexistence between blue sheep and red deer in the Helan Mountains because they led to habitat segregation and temporal niche partitioning, which reduced encounters and potential competition and even negative interspecific interactions.
According to previous research on diets of sympatric blue sheep and red deer in the Helan Mountains, food overlap appears in each season. The number of plant species that overlap was nine in spring, 13 in summer, eight in autumn, and 20 in winter, the highest among the four seasons (Luo, 2011). Only one plant species was the primary reason for food overlap in spring and summer; whereas, in autumn and winter, only three to four plant species primarily accounted for food overlap (Figure 6). Overall, the overlap of the main food species of blue sheep and red deer was relatively low throughout the year, reducing the negative influence of each species had on the other to some extent. Stipa spp. were the favorite species of blue sheep in spring, summer, and autumn, accounting for greater than 30% of the diet (Luo, 2011), even though Stipa nutrition levels were not the highest among primary foods of blue sheep (Table 2). The food preference of blue sheep might simply be due to the high availability of Stipa spp. The food preference of red deer was similar to that of blue sheep (Figure 6). To summarize, plants foraged by blue sheep and red deer were not foods with the highest nutritional values (Table 2 and Figure 6), indicating that the food preference of ungulates is related to both nutrition and availability of plants (Selebatso et al., 2018). Quality and quantity of food resources are often inversely correlated, with those most nutritious tending to be the least common (Demment and Van Soest, 1985; Hansen et al., 2009). In addition, plant growth form is a good indicator of seasonal changes in the nutritive value and palatability of forage plants to ungulates, with young plants more palatable (Zweifel-Schielly et al., 2009; Iversen et al., 2014). Such differences in plant growth form phenology and nutritive value could influence the composition of diets of sympatric ungulates in different seasons (Srivastava and Kumar, 2021). Therefore, with decreases in plant nutrition in the Helan Mountains during winter, the number of species foraged by blue sheep and red deer increased, which has also been shown in other studies on diets of ungulates (Selebatso et al., 2018).
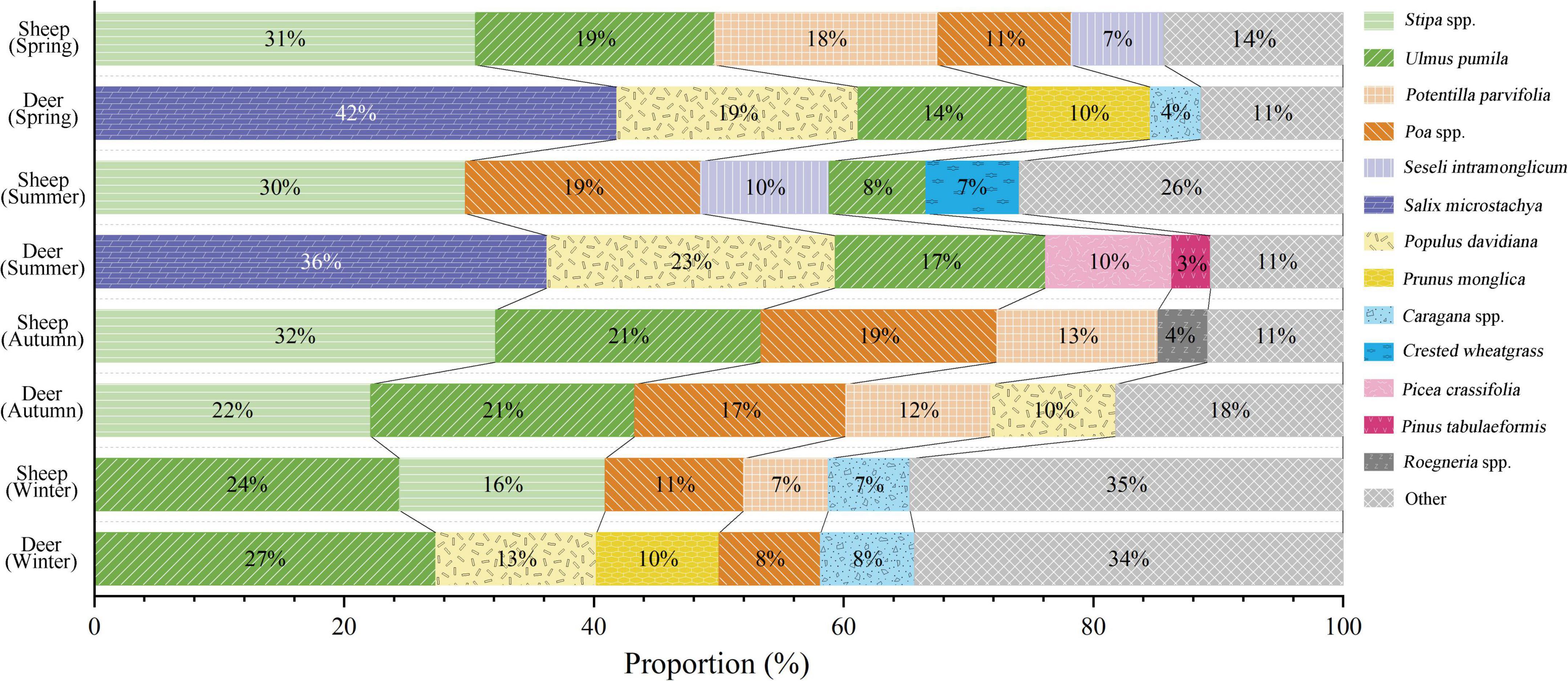
Figure 6. The species of food overlap of blue sheep and red deer in different seasons (Luo, 2011). Different colored and filled color blocks represent different plant species eaten by blue sheep and red deer. The black line between the color blocks distinguishes the first food/second food/eaten by them.
Different feeding strategies (i.e., grazer, browser, mixed feeder) are important in determining the degree of diet overlap between sympatric species (Hofmann, 1989). According to the digestive system morphology in this study, both blue sheep and red deer were more likely to be grazers. The stomach weight of blue sheep was 0.76 ± 0.03 kg (approximately 2.45 ± 0.14% of body weight); whereas the stomach weight of red deer was 3.67 ± 0.26 (approximately 3.16 ± 0.06% of body weight) (Figure 4A). The surface enlargement factors of blue sheep and red deer were 2.85 ± 1.37 and 4.79 ± 1.63, respectively (Table 1). The length of the intestine of blue sheep (Figure 5A) was 26.1 ± 0.5 times the body length, whereas for red deer, it was 20.0 ± 0.93 times the body length, which are results that are different from those of other studies (Prins and Geelen, 1971; Hofmann, 1973, 1985, 1989; Schaller, 2000; Shrestha et al., 2005). The differences might be caused by changes in forage quantity and quality in the Helan Mountains, which produced morphological and functional modifications at different levels in the gastrointestinal tract in blue sheep and red deer (Hofmann, 1989). In fact, the feeding strategies of ungulates are not difficult to change. Because of drastic declines in nutritive value and limited access to graminoids from the growing season to winter, ungulates in high altitudes and latitudes can switch diets and change from a grazing strategy to one of browsing (Suryawanshi et al., 2010; Thompson et al., 2015; Srivastava et al., 2021). Similar conditions likely influence blue sheep and red deer, leading them to switch diets and increase the number of plant species not consumed when food resources are adequate.
In this study, there was no relation between body weight and feeding type of ruminant, because both blue sheep and red deer were grazers, even though body weights differed significantly (blue sheep: 16–46 kg and red deer: 99–106 kg; Supplementary Table 2). In general, many morphophysiological traits do not have a distinct linear relation with a dietary niche (Hofmann, 1989; Hofmann et al., 2008; Clauss et al., 2009). Additionally, ruminants usually do not fit a strictly defined feeding type category, and ruminants with a certain morphophysiology can also ingest forages other than preferred dietary sources (Clauss et al., 2014; Przybyło et al., 2019). In this study, the diet of red deer could change significantly during autumn and winter, when they would be expected to increase feeding on grass. Therefore, the terms “grazer and browser” should be reserved for descriptions of natural diets, whereas morphophysiological types should be described by other terms such as “moose-type” and “cattle-type” (Clauss et al., 2010). In this study, stratification was observed in the rumen–reticulum of both blue sheep and red deer (Table 1), indicating that both should be classified as “cattle-type” (Clauss et al., 2010). “Cattle-type” ruminants can take advantage of various dietary niches, ranging from strictly browsing to strictly grazing (Przybyło et al., 2019). Therefore, the reason blue sheep and red deer coexist harmoniously and adapt to food scarcity in winters in the Helan Mountains might be because they are “cattle-type” ruminants.
The rumen–reticulum in ruminants is the essential part of the gut where most fermentation and digestion occurs (Van Soest, 1994). In this study, the rumen–reticulum of red deer was significantly heavier than that of blue sheep (3.28 ± 0.26 kg vs. 0.62 ± 0.03 kg, respectively) (Figure 4A). In addition, the proportion of rumen–reticulum weight to red deer body weight was significantly higher than that for blue sheep (2.84 ± 0.06% vs. 1.99 ± 0.11%, respectively) (Figure 4A). Consequently, with a relatively large rumen–reticulum, red deer could eat more lignin-rich plants of relatively lower nutritional quality, compared with blue sheep. Additionally, the rumen–reticulum can adjust to variation in dietary energy concentration by changing organ weight and porous surface area, which allow a greater volume to be ingested. Hence, it is not difficult to understand the adaptations that allow the coexistence of sympatric blue sheep and red deer in the Helan Mountains, even during winter with inadequate food resources.
Blue sheep may balance diets by consuming highly digestible, high-carbohydrate plants in combination with plants that are less digestible but with relatively high protein or lipid contents (Aryal et al., 2015). For red deer, proportions and fluctuations of CF and NDF in food were 25–30% and 56–70%, respectively (Chen et al., 1998). Therefore, red deer likely maintain a stable intake of total digestible energy despite significant variation in food composition (Webster et al., 2000; Asher et al., 2011). By balancing diets, both blue and red deer could adapt to the low availability of vegetation in the Helan Mountains in winter. In summary, differences in physiological adaptations of the gastrointestinal tract of sympatric blue sheep and red deer reduced competition for food resources, providing another key to the coexistence of the two species.
Digestive efficiency of ungulates is primarily determined by food quality, food retention time, and food particle size (Clauss et al., 2010). The size of the passage in the stomach affects retention time and flow rate of food particles and thus ruminant efficiency of digestion. In particular, the reticulum–omasum passage directly controls the flow of food particles before allowing them into the omasum (Allen, 1996). Because the fiber content in food of roughage feeders is relatively high, to ensure that food is fully fermented and well absorbed in the rumen–reticulum, the size of the reticulum–omasum passage is relatively small and only food particles smaller than a certain size can pass through. In this study, the diameter of the reticulum–omasum passage (3.65 ± 0.11 cm) was significantly smaller than the omasum–abomasum passage (6.16 ± 0.16 cm) in the stomach of blue sheep (Supplementary Figure 3). The condition was the same in the stomachs of red deer (5.03 ± 0.09 cm vs. 8.81 ± 0.83 cm, respectively) (Supplementary Figure 3). Consequently, the digestive tract after the omasum still retained a large amount of large-particle food, which could enter the intestinal tract through the stomach quickly, thereby improving the absorption rate of the digestive systems of both blue sheep and red deer.
The number and size of rumen papillae and the surface enlargement factor of the rumen can reflect variation in diet quality (Hofmann, 1985; Hofmann et al., 1988; Josefsen et al., 1996). However, because of the relatively few specimens in this study and because there was no separation of rumen data from blue sheep and red deer by season, feeding habits could not be correlated with various rumen parameters, which should be explored further in future studies. Differences were observed in different parts of the rumen between sympatric blue sheep and red deer, which were reflections of differences in adaptability to feeding on different plant species or different parts of the same plant species (Table 1). In some studies, intestinal length does not reflect differences in morphophysiological ruminant types, and it is difficult to interpret relations between morphological changes in hindgut length and forage quality of ruminants (Van Soest, 1994; Pérez et al., 2009). Moreover, conflicting results have been reported on the effects of food quality on hindgut length (Zimmerman et al., 2006). In this study, the total length of the intestinal tract of blue sheep was 26.1 ± 0.5 times the body length, whereas that of red deer was 20.0 ± 0.93 times the body length, and there was no significant difference between the species (Figure 5A). Similarly, relations between intestinal lengths and physiological adaptation could not be inferred because of the limited amount of data. Hence, relations between adaptability and intestinal tracts of blue sheep and red deer need further study. Last, because the number of red deer in the study was much smaller than that of blue sheep, the accuracy of the results was likely affected. The low number might be because only naturally dead blue sheep and red deer were collected instead of hunting live individuals in the Helan Mountains. In addition, the population of red deer in the study area is much smaller than that of blue sheep (Huang, 2020; Xie et al., 2022). Future studies will focus on increasing the number of samples and collecting more natural-death red deer carcasses to increase the significance of the results.
Conclusion
In this study, sympatric blue sheep and red deer appeared in different habitats and areas in the Helan Mountains in China, and even when appearing in the same space, the activity patterns of the two species were significantly different. Thus, the spatial overlap and temporal overlap between sympatric blue sheep and red deer were relatively low in the Helan Mountains, which reduced possible encounters and potential competition for limited resources. Differences were also found in the stomachs and the intestines of the two species, which determined the ability to feed on various species of plants or different parts of the same plant species. Therefore, according to results in this study, blue sheep and red deer appear to have adapted both physically and behaviorally to coexist stably by reducing spatial, temporal, and food acquisition overlap. A similar pattern may also allow other species to coexist sympatrically.
Data availability statement
The raw data supporting the conclusions of this article will be made available by the authors, without undue reservation.
Ethics statement
Ethical review and approval was not required for the animal study because we collected carcasses which died naturally in the protected area when permission were gotten.
Author contributions
ZZL, ZL, and LT conceived the ideas and designed the study and its methods. ZZL performed the data analyses, prepared the figures and tables, and wrote the manuscript. JW and PL conducted the fieldwork and data collection. RK, XH, ZL, and LT interpreted the results and edited the manuscript to its final submitted draft. All authors contributed to the manuscript and approved the submitted version.
Funding
This work was supported by the National Natural Science Foundation of China (Nos. 32071649, 32070519, and 31870512) and the Key Research and Development Program of Ningxia Hui autonomous region, China (No. 2020BEG02001).
Acknowledgments
We thank the assistance supplied by the National Nature Reserve of Helan Mountains in Ningxia in sample collection.
Conflict of interest
The authors declare that the research was conducted in the absence of any commercial or financial relationships that could be construed as a potential conflict of interest.
Publisher’s note
All claims expressed in this article are solely those of the authors and do not necessarily represent those of their affiliated organizations, or those of the publisher, the editors and the reviewers. Any product that may be evaluated in this article, or claim that may be made by its manufacturer, is not guaranteed or endorsed by the publisher.
Supplementary material
The Supplementary Material for this article can be found online at: https://www.frontiersin.org/articles/10.3389/fevo.2022.925465/full#supplementary-material
References
Afolabi, S. S., Oyeyode, J. O., Shafik, W., Sunusi, Z., and Adeyemi, A. A. (2021). Proximate analysis of poultry-mix formed feed using maize bran as a base. Int. J. Anal. Chem. 2021:8894567. doi: 10.1155/2021/8894567
Allen, M. S. (1996). Physical constraints on voluntary intake of forages by ruminants. J. Anim. Sci. 74, 3063–3075. doi: 10.2527/1996.74123063x
Aryal, A., Coogan, S. C., Ji, W., Rothman, J. M., and Raubenheimer, D. (2015). Foods, macronutrients and fibre in the diet of blue sheep (Psuedois nayaur) in the Annapurna Conservation Area of Nepal. Ecol. Evol. 5, 4006–4017. doi: 10.1002/ece3.1661
Asher, G., Stevens, D., Archer, J., Barrell, G. K., Scott, I., Ward, J., et al. (2011). Energy and protein as nutritional drivers of lactation and calf growth of farmed red deer. Livestock Sci. 140, 8–16. doi: 10.1016/j.livsci.2011.02.002
Bagchi, S., Goyal, S., and Sankar, K. (2003). Niche relationships of an ungulate assemblage in a dry tropical forest. J. Mammal. 84, 981–988. doi: 10.1644/BBa-024
Bell, R. H. (1971). A grazing ecosystem in the Serengeti. Sci. Am. 225, 86–93. doi: 10.1038/scientificamerican0771-86
Cao, L., Liu, Z., Wang, X., Hu, T., Zhai, H., and Hou, J. (2005). Winter group size and composition of blue sheep(Pseudois nayaur) in the Helan Mountains, China. Acta Theriol. Sin. 23, 200–204. doi: 10.16829/j.slxb.2005.02.013
Chanchani, P., Rawat, G. S., and Goyal, S. P. (2010). Unveiling a wildlife haven: status and distribution of four Trans-Himalayan ungulates in Sikkim, India. Oryx 44, 366–375. doi: 10.1017/S0030605310000293
Chen, H., Ma, J., Li, F., Sun, Z., Wang, H., and Luo, L. (1998). Seasonal composition and quality of red deer Cervus elaphus diets in northeastern China. Acta Theriol. 43, 77–94. doi: 10.4098/AT.arch.98-6
Clauss, M., Hofmann, R. R., Fickel, J., Streich, W. J., and Hummel, J. (2009). The intraruminal papillation gradient in wild ruminants of different feeding types: implications for rumen physiology. J. Morphol. 270, 929–942. doi: 10.1002/jmor.10729
Clauss, M., Hofmann, R. R., Melleti, M., and Burton, J. (2014). The Digestive System of Ruminants, and Peculiarities of (wild) Cattle. Cambridge: Cambridge University Press.
Clauss, M., Hume, I. D., and Hummel, J. (2010). Evolutionary adaptations of ruminants and their potential relevance for modern production systems. Animal 4, 979–992. doi: 10.1017/S1751731110000388
Darmon, G., Bourgoin, G., Marchand, P., Garel, M., Dubray, D., Jullien, J.-M., et al. (2014). Do ecologically close species shift their daily activities when in sympatry? A test on chamois in the presence of mouflon. Biol. J. Linn. Soc. 111, 621–626. doi: 10.1111/bij.12228
Darmon, G., Calenge, C., Loison, A., Jullien, J. M., Maillard, D., and Lopez, J. F. (2012). Spatial distribution and habitat selection in coexisting species of mountain ungulates. Ecography 35, 44–53. doi: 10.1111/j.1600-0587.2011.06664.x
Delsinne, T., Roisin, Y., and Leponce, M. (2007). Spatial and temporal foraging overlaps in a Chacoan ground-foraging ant assemblage. J. Arid Environ. 71, 29–44. doi: 10.1016/j.jaridenv.2007.02.007
Demment, M. W., and Van Soest, P. J. (1985). A nutritional explanation for body-size patterns of ruminant and nonruminant herbivores. Am. Nat. 125, 641–672. doi: 10.2307/2461476
Di, W., Tian, L., and Li, Z. (1988). The characteristics of Helan Mountains flora of spermatophytes. Acta Bot. Boreali Occidental. Sin. 8, 242–254.
DÍAz, S., Lavorel, S., McIntyre, S., Falczuk, V., Casanoves, F., Milchunas, D. G., et al. (2007). Plant trait responses to grazing–a global synthesis. Glob. Change Biol. 13, 313–341. doi: 10.1111/j.1365-2486.2006.01288.x
Ferretti, F., Corazza, M., Campana, I., Pietrocini, V., Brunetti, C., Scornavacca, D., et al. (2015). Competition between wild herbivores: reintroduced red deer and Apennine chamois. Behav. Ecol. 26, 550–559.
Forsyth, D. M., and Hickling, G. J. (1998). Increasing Himalayan tahr and decreasing chamois densities in the eastern Southern Alps, New Zealand: evidence for interspecific competition. Oecologia 113, 377–382. doi: 10.1007/s004420050389
Frey, S., Fisher, J. T., Burton, A. C., and Volpe, J. P. (2017). Investigating animal activity patterns and temporal niche partitioning using camera-trap data: Challenges and opportunities. Remote Sens. Ecol. Conserv. 3, 123–132. doi: 10.1002/rse2.60
Hansen, B. B., Aanes, R., Herfindal, I., Saether, B.-E., and Henriksen, S. (2009). Winter habitat–space use in a large arctic herbivore facing contrasting forage abundance. Polar Biol. 32, 971–984. doi: 10.1007/s00300-009-0597-2
Hofmann, G. S., Coelho, I. P., Bastazini, V. A. G., Cordeiro, J. L. P., and de Oliveira, L. F. B. (2016). Implications of climatic seasonality on activity patterns and resource use by sympatric peccaries in northern Pantanal. Int. J. Biometeorol. 60, 421–433. doi: 10.1007/s00484-015-1040-8
Hofmann, R. R. (1973). The Ruminant Stomach. Stomach Structure and Feeding Habits of East African Game Ruminants. Nairobi: East African Literature Bureau.
Hofmann, R. R. (1985). “Digestive physiology of the deer-Their morphophysiological specialisation and adaptation,” in Proceedings of the Biology of deer production. Proceedings of an International Conference held at Dunedin, New Zealand.
Hofmann, R. R. (1989). Evolutionary steps of ecophysiological adaptation and diversification of ruminants: a comparative view of their digestive system. Oecologia 78, 443–457. doi: 10.1007/BF00378733
Hofmann, R. R., Dobson, A., and Dobson, M. (1988). Morphophysiological Evolutionary Adaptations of the Ruminant Digestive System. Aspects of Digestive Physiology in Ruminants. Ithaca: Cornell University Press.
Hofmann, R. R., Streich, W. J., Fickel, J., Hummel, J., and Clauss, M. (2008). Convergent evolution in feeding types: salivary gland mass differences in wild ruminant species. J. Morphol. 269, 240–257. doi: 10.1002/jmor.10580
Huang, S. (2020). The Population and the Influence of Observers of Blue Sheep and Red Deer in Helan Mountain Reserve, Inner Mongolia. Master’s thesis. Harbin: Northeast Forestry University.
Hutchinson, G. E. (1959). Homage to Santa Rosalia or why are there so many kinds of animals? Am. Nat. 93, 145–159.
Iversen, M., Fauchald, P., Langeland, K., Ims, R. A., Yoccoz, N. G., and Bråthen, K. A. (2014). Phenology and cover of plant growth forms predict herbivore habitat selection in a high latitude ecosystem. PLoS One 9:e100780. doi: 10.1371/journal.pone.0100780
Jarman, P. (1974). The social organisation of antelope in relation to their ecology. Behaviour 48, 215–267. doi: 10.1163/156853974x00345
Josefsen, T. D., Aagnes, T. H., and Mathiesen, S. D. (1996). Influence of diet on the morphology of the ruminal papillae in reindeer calves (Rangifer tarandus tarandus L.). Rangifer 16, 119–128. doi: 10.7557/2.16.3.1205
Li, J., Xu, H., Wan, Y., Sun, J., Li, S., and Cai, L. (2018). Progress in Construction of China Mammal Diversity Observation Network (China BON-Mammals). J. Ecol. Rural Environ. 34, 12–19.
Liu, X., Wu, P., Songer, M., Cai, Q., He, X., Zhu, Y., et al. (2013). Monitoring wildlife abundance and diversity with infra-red camera traps in Guanyinshan Nature Reserve of Shaanxi Province, China. Ecol. Indic. 33, 121–128.
Liu, Z., Cao, L., Wang, X., Li, T., and Li, Z. (2005a). Winter Bed-Site Selection by Blue Sheep(Pseudois nayaur) in Helan Mountains Ningxia, China. Acta Theriol. Sin. 25, 1–8. doi: 10.16829/j.slxb.2005.01.001
Liu, Z., Wang, X., Li, Z., Cui, D., and Li, X. (2005b). Comparison of Seasonal Feeding Habitats by Blue Sheep(Pseudois nayaur) During Winter and Spring in Helan Mountain, China. Zool. Res. 26, 580–589. doi: 10.3321/j.issn:0254-5853.2005.06.002
Liu, Z., Zhang, M., Li, Z., Hu, T., and Zhai, H. (2009). Feeding and bedding habitat seleciton by red deer(Cervuselaphus alxaicus) during winter in the Helan Mountains, China. Acta Theriol. Sin. 29, 133–141. doi: 10.16829/j.slxb.2009.02.005
Lovari, S., Lorenzini, R., Masseti, M., Pereladova, O., Carden, R. F., Brook, S. M., et al. (2018). Cervus elaphus (errata version published in 2019). The IUCN Red List of Threatened Species 2018. Gland: IUCN
Luo, Y. (2011). Compared Study on the Diet, Habitat Selection of Sympatric Blue sheep(Pseudois nayaur) and Red deer(Cervus elaphus alxaicus) in Helan Mountains, China. Ph.D. thesis. Harbin: Northeast Forestry University.
Luo, Y., Zhang, M., Liu, Z., Li, Z., Hu, T., and Zhai, H. (2009). Winter and spring habitat selection of Red deer (Cervus elaphus alxaicus) in the Helan Mountains, China. Acta Ecol. Sin. 229, 2757–2763. doi: 10.1016/S1874-8651(10)60059-2
Lynam, A. J., Tantipisanuh, N., Chutipong, W., Ngoprasert, D., Baker, M. C., Cutter, P., et al. (2012). Comparative sensitivity to environmental variation and human disturbance of Asian tapirs (Tapirus indicus) and other wild ungulates in Thailand. Integ. Zool. 7, 389–399. doi: 10.1111/1749-4877.12002
Meredith, M., and Ridout, M. (2014). Overview of the overlap package. Available online at: https://cran.r-project.org/web/packages/overlap/overlap.pdf (accessed December 23, 2018).
Monterroso, P., Alves, P. C., and Ferreras, P. (2014). Plasticity in circadian activity patterns of mesocarnivores in Southwestern Europe: implications for species coexistence. Behav. Ecol. Sociobiol. 68, 1403–1417. doi: 10.1007/s00265-014-1748-1
Mueller, D. W. H., Codron, D., Meloro, C., Munn, A., Schwarm, A., Hummel, J., et al. (2013). Assessing the Jarman-Bell Principle: scaling of intake, digestibility, retention time and gut fill with body mass in mammalian herbivores. Comp. Biochem. Physiol. Mol. Integ. Physiol. 164, 129–140. doi: 10.1016/j.cbpa.2012.09.018
Namgail, T., Fox, J. L., and Bhatnagar, Y. V. (2004). Habitat segregation between sympatric Tibetan argali Ovis ammon hodgsoni and blue sheep Pseudois nayaur in the Indian Trans-Himalaya. J. Zool. 262, 57–63. doi: 10.1017/S0952836903004394
O’Brien, T. G., Kinnaird, M. F., and Wibisono, H. T. (2003). Crouching tigers, hidden prey: sumatran tiger and prey populations in a tropical forest landscape. Anim. Conserv. 6, 131–139.
Olawuwo, O., Abdalla, M. A., Mühling, K., and McGaw, L. J. (2022). Proximate analysis of nutrients and in vitro radical scavenging efficacy in selected medicinal plant powders with potential for use as poultry feed additives. South Afr. J. Bot. 146, 103–110.
Pérez, W., Lima, M., and Clauss, M. (2009). Gross anatomy of the intestine in the giraffe (Giraffa camelopardalis). Anat. Histol. Embryol. 38, 432–435. doi: 10.1111/j.1439-0264.2009.00965.x
Pianka, E. R. (1974). Niche overlap and diffuse competition. Proc. Nat. Acad. Sci. U.S.A. 71, 2141–2145. doi: 10.1073/pnas.71.5.2141
Prins, R., and Geelen, M. (1971). Rumen characteristics of red deer, fallow deer, and roe deer. J. Wildlife Manage. 35, 673–680. doi: 10.2307/3799772
Przybyło, M., Hummel, J., Ortmann, S., Codron, D., Kohlschein, G.-M., Kilga, D., et al. (2019). Digesta passage in nondomestic ruminants: separation mechanisms in ‘moose-type’and ‘cattle-type’species, and seemingly atypical browsers. Comp. Biochem. Physiol. A Mol. Integ. Physiol. 235, 180–192. doi: 10.1016/j.cbpa.2019.06.010
R Core Team (2013). R: A language and environment for statistical computing. Vienna: R Foundation for Statistical Computing.
Rahbek, C., Borregaard, M. K., Antonelli, A., Colwell, R. K., Holt, B. G., Nogues-Bravo, D., et al. (2019). Building mountain biodiversity: Geological and evolutionary processes. Science 365, 1114–1119. doi: 10.1126/science.aax0151
Redjadj, C., Darmon, G., Maillard, D., Chevrier, T., Bastianelli, D., Verheyden, H., et al. (2014). Intra-and interspecific differences in diet quality and composition in a large herbivore community. PLoS One 9:e84756. doi: 10.1371/journal.pone.0084756
Reyna-Hurtado, R., and Tanner, G. W. (2007). Ungulate relative abundance in hunted and non-hunted sites in Calakmul Forest (Southern Mexico). Biodivers. Conserv. 16, 743–756. doi: 10.1007/s10531-005-6198-7
Richard, E., Gaillard, J. M., Said, S., Hamann, J. L., and Klein, F. (2010). High red deer density depresses body mass of roe deer fawns. Oecologia 163, 91–97. doi: 10.1007/s00442-009-1538-z
Ridout, M. S., and Linkie, M. (2009). Estimating overlap of daily activity patterns from camera trap data. J. Agric. Biol. Environ. Stat. 14, 322–337. doi: 10.1198/jabes.2009.08038
Ruifrok, J. L., Janzen, T., Kuijper, D. P., Rietkerk, M., Olff, H., and Smit, C. (2015). Cyclical succession in grazed ecosystems: the importance of interactions between different-sized herbivores and different-sized predators. Theor. Popul. Biol. 101, 31–39. doi: 10.1016/j.tpb.2015.02.001
Schoener, T. W. (1974). Resource partitioning in ecological communities. Science 185, 27–39. doi: 10.1126/science.185.4145.27
Selebatso, M., Maude, G., and Fynn, R. W. (2018). Adaptive foraging of sympatric ungulates in the Central Kalahari Game Reserve, Botswana. Afr. J. Wildlife Res. 48, 1–12. doi: 10.3957/056.048.023005
Shen, G. (2009). Food composition, Feeding and utritional Adaption Strategies of Tibetan Red Deer (Cervus elaphus wallichi) during the Green Grass Period in Tibet. Master’s thesis. Harbin: Northeast Forestry University.
Shipley, L., Blomquist, S., and Danell, K. (1998). Diet choices made by free-ranging moose in northern Sweden in relation to plant distribution, chemistry, and morphology. Can. J. Zool. 76, 1722–1733. doi: 10.1139/z98-110
Shrestha, R., Wegge, P., and Koirala, R. A. (2005). Summer diets of wild and domestic ungulates in Nepal Himalaya. J. Zool. 266, 111–119. doi: 10.1017/s0952836905006527
Šprem, N., Zanella, D., Ugarković, D., Prebanić, I., Gančević, P., and Corlatti, L. (2015). Unimodal activity pattern in forest-dwelling chamois: typical behaviour or interspecific avoidance? Eur. J. Wildlife Res. 61, 789–794. doi: 10.1007/s10344-015-0939-z
Srivastava, T., and Kumar, A. (2021). Seasonal forage and diet quality in two subtropical ungulates in the Himalaya. Eur. J. Wildlife Res. 67, 1–10. doi: 10.1007/s10344-021-01518-x
Srivastava, T., Kumar, A., Kumar, V., and Umapathy, G. (2021). Diet drives differences in reproductive synchrony in two sympatric mountain ungulates in the Himalaya. Front. Ecol. Evol. 9:647465. doi: 10.3389/fevo.2021.647465
Storms, D., Aubry, P., Hamann, J.-L., Saïd, S., Fritz, H., Saint-Andrieux, C., et al. (2008). Seasonal variation in diet composition and similarity of sympatric red deer Cervus elaphus and roe deer Capreolus capreolus. Wildlife Biol. 14, 237–250.
Suryawanshi, K. R., Bhatnagar, Y. V., and Mishra, C. (2010). Why should a grazer browse? Livestock impact on winter resource use by bharal Pseudois nayaur. Oecologia 162, 453–462. doi: 10.1007/s00442-009-1467-x
Takada, H., Ohuchi, R., Watanabe, H., Yano, R., Miyaoka, R., Nakagawa, T., et al. (2020). Habitat use and the coexistence of the sika deer and the Japanese serow, sympatric ungulates from Mt. Asama, central Japan. Mammalia 84, 503–511. doi: 10.1515/mammalia-2019-0150
Thompson, I. D., Wiebe, P. A., Mallon, E., Rodgers, A. R., Fryxell, J. M., Baker, J. A., et al. (2015). Factors influencing the seasonal diet selection by woodland caribou (Rangifer tarandus tarandus) in boreal forests in Ontario. Can. J. Zool. 93, 87–98. doi: 10.1139/cjz-2014-0140
Tobler, M. W., Carrillo-Percastegui, S. E., and Powell, G. (2009). Habitat use, activity patterns and use of mineral licks by five species of ungulate in south-eastern Peru. J. Trop. Ecol. 25, 261–270. doi: 10.1017/S0266467409005896
Tokeshi, M. (2009). Species Coexistence: Ecological and Evolutionary Perspectives. Hoboken, NJ: John Wiley & Sons.
Van Beest, F. M., McLoughlin, P. D., Vander Wal, E., and Brook, R. K. (2014). Density-dependent habitat selection and partitioning between two sympatric ungulates. Oecologia 175, 1155–1165. doi: 10.1007/s00442-014-2978-7
Van Soest, P. J. (1994). Nutritional ecology of the ruminant. New York, NY: Cornell university press.
Wan, Y., Li, J., Yang, X., Li, S., and Xu, H. (2020). Progress of the China mammal diversity observation network (China BON-Mammal) based on camera-trapping. Biodivers. Sci. 28, 1115–1124.
Wang, X., Liu, Z., Xu, H., Li, M., and Li, Y. (1998). The Blue Sheep population ecology and its conservation in Helan Mountain, China. Chin. Biodivers. 6, 1–5. doi: 10.1111/1749-4877.12422
Webster, J., Corson, I., Littlejohn, R., Masters, B., and Suttie, J. (2000). Effect of diet energy density and season on voluntary dry-matter and energy intake in male red deer. Anim. Sci. 70, 547–554. doi: 10.1017/S1357729800051894
Xian, D. (2008). The role of competition in coexistence among species. For. Invent. Plann. 33, 97–102.
Xie, J., Meng, D., Li, Z., Zhang, Z., Liu, Z., and Teng, L. (2022). Population on size and strueture of blue sheep in helan mountains national nature reserves, ningxia. Acta Ecol. Sin. 42, 1–8.
Yu, Y., Guo, S., Bai, Q., Li, Z., Hu, T., and Lu, H. (2004). The Seasonal Change of Blue Sheep Population Structure in Helanshan Mountains. Acta Theriol. Sin. 24, 200–204. doi: 10.16829/j.slxb.2004.03.003
Zhou, Q., Wei, H., Tang, H., Huang, Z., Krzton, A., and Huang, C. (2013). Niche separation of sympatric macaques, Macaca assamensis and M. mulatta, in limestone habitats of Nonggang, China. Primat. J. Primatol. 55, 125–137. doi: 10.1007/s10329-013-0385-z
Zimmerman, T. J., Jenks, J. A., and Leslie, D. M. Jr. (2006). Gastrointestinal morphology of female white-tailed and mule deer: effects of fire, reproduction, and feeding type. J. Mammal. 87, 598–605. doi: 10.1644/05-mamm-A-356R1.1
Keywords: mountain ungulates, mountain diversity, Pseudois nayaur, Cervus elaphus alxaicus, Helan Mountains, behavioral adaptations, physiological adaptations
Citation: Li Z, Wang J, Khattak RH, Han X, Liu P, Liu Z and Teng L (2022) Coexistence mechanisms of sympatric ungulates: Behavioral and physiological adaptations of blue sheep (Pseudois nayaur) and red deer (Cervus elaphus alxaicus) in Helan Mountains, China. Front. Ecol. Evol. 10:925465. doi: 10.3389/fevo.2022.925465
Received: 21 April 2022; Accepted: 05 July 2022;
Published: 27 July 2022.
Edited by:
Lin Zhang, Hubei University of Chinese Medicine, ChinaReviewed by:
Carlos Alberto Lopez Gonzalez, Universidad Autónoma de Querétaro, MexicoSongtao Guo, Northwest University, China
Tongzuo Zhang, Northwest Institute of Plateau Biology (CAS), China
Copyright © 2022 Li, Wang, Khattak, Han, Liu, Liu and Teng. This is an open-access article distributed under the terms of the Creative Commons Attribution License (CC BY). The use, distribution or reproduction in other forums is permitted, provided the original author(s) and the copyright owner(s) are credited and that the original publication in this journal is cited, in accordance with accepted academic practice. No use, distribution or reproduction is permitted which does not comply with these terms.
*Correspondence: Zhensheng Liu, zhenshengliu@163.com; Liwei Teng, tenglw1975@163.com