- Department of Archaeology and Art History, Seoul National University, Seoul, South Korea
Rice is currently the staple food for over 3.5 billion people and is arguably the most important crop exploited by humans. Understanding how we came to the point where a single crop dominates the lives of almost half of the Earth’s population has major significance for our future, even more so given the climatic instability we face today, as rice is a cereal that is dependent on water to an extreme degree. In this study, the nature of early rice agriculture in South Asia is explored, looking at how this critical crop may have begun to be exploited, cultivated, and then brought under agricultural regimes during the long span between c.6500 and 1500 BC. There is now clear evidence for early Holocene cultivation of rice in the Middle Gangetic plains of northern India, but there is still considerable debate about the timing of when this cultivation began and whether it involved domestication of rice. By 3200 BC, however, rice agriculture was present outside the Ganges in the Indus Civilization. The data show accelerated domestication in the Indus environment and agricultural systems that played a part in later hybridization with the arrival of Chinese rice. Understanding how this move from its place of origin to a new environment may have become entangled in the domestication pathways of South Asia rice prior to the arrival of Chinese rice c.1500 BC are important to the overall rice story, as they play into modern concerns relating to biodiversity and different ways of growing and watering rice.
Introduction
The process of rice domestication and the social and economic implications of its early use remain hotly debated. Modern domesticated Asian rice, Oryza sativa L., which can be subdivided genetically into subspp. indica, japonica, and aus (Grist, 1965; Chang, 1976; Oka, 1988; Garris et al., 2005; McNally et al., 2009; Schatz et al., 2014; Civáň et al., 2015; Travis et al., 2015; Choi et al., 2017), has a complex history, as it is the product of repeated instances of hybridization1.
While there has been considerable discussion on the origins of rice agricultural systems in China (the japonica story), the story of rice in South Asia remains less clear. The ancestor for indica (proto or otherwise) is nivara, a type of wild rice that originates in the Gangetic Plains of northern India. There is evidence of early Holocene cultivation of rice in the Middle Gangetic plains of northern India, but there is still considerable debate about the timing of when this cultivation began and whether it was entangled with the domestication of rice.
This region currently relies heavily on rice as a staple food, as does much of the world. Rice is currently the staple food for over 3.5 billion people and is thus arguably the most important crop exploited by humans. It is estimated that, by 2050 (relative to 2018), the amount of rice needed to sustain current demand will have to increase by 25%. Modern rice agriculture is based on a limited number of highly intensive water-demanding systems. As Consultative Group for International Agricultural Research (CGIAR) has pointed out, the heavy water footprint and resource-intensive nature of rice agriculture today needs to change as it is not sustainable. This creates a central question for those involved in both the archeology of rice agriculture and sustainability studies and Anthropocene debates (among others): How does a single crop attain such a predominance in our daily diets and lives and come to shape the land use, subsistence, foodways, cultures, and perhaps even climate and environment of much of planet?
In this study, pathways to rice domestication and the diversity of agricultural strategies that accompany this are explored. Rather than focusing on the Chinese japonica narratives and the later hybridization of japonica in South Asia c.1500 BC, this study adds to the debate instead by outlining how the pathways to rice domestication in South Asia c.6500–1500 BC have been researched in light of agricultural and cultivation systems and how new data from multiple proxies and new regions can and are not only being used to fill in gaps in our narratives but are also raising new questions. While rice use in South Asia began in the Ganges, new data from the Indus Civilization to the west of the Gangetic region, once described as an area familiar with but not focused on or perhaps even unwilling to adopt rice agriculture (Fuller and Madella, 2002), encourage us to ask new questions about how the use of this novel plant in new agricultural systems outside its native ecology may have impacted the domestication pathways of rice before the arrival of the fully domesticated japonica (Figure 1). The Indus Civilization covered an area that is today modern Pakistan and northwest India and stretched up to Afghanistan. It was one of the Old World Bronze Age civilizations with an established agricultural system that had roots stretching back to the Neolithic of Mehrgarh. Crops included Near Eastern wheat, barley and winter pulses, South Asian native millets and pulses, and African pulses and millets, as well as a variety of oil/fiber crops and fruits (see Pokharia and Srivastava, 2013; Petrie and Bates, 2017; Bates, 2019a).
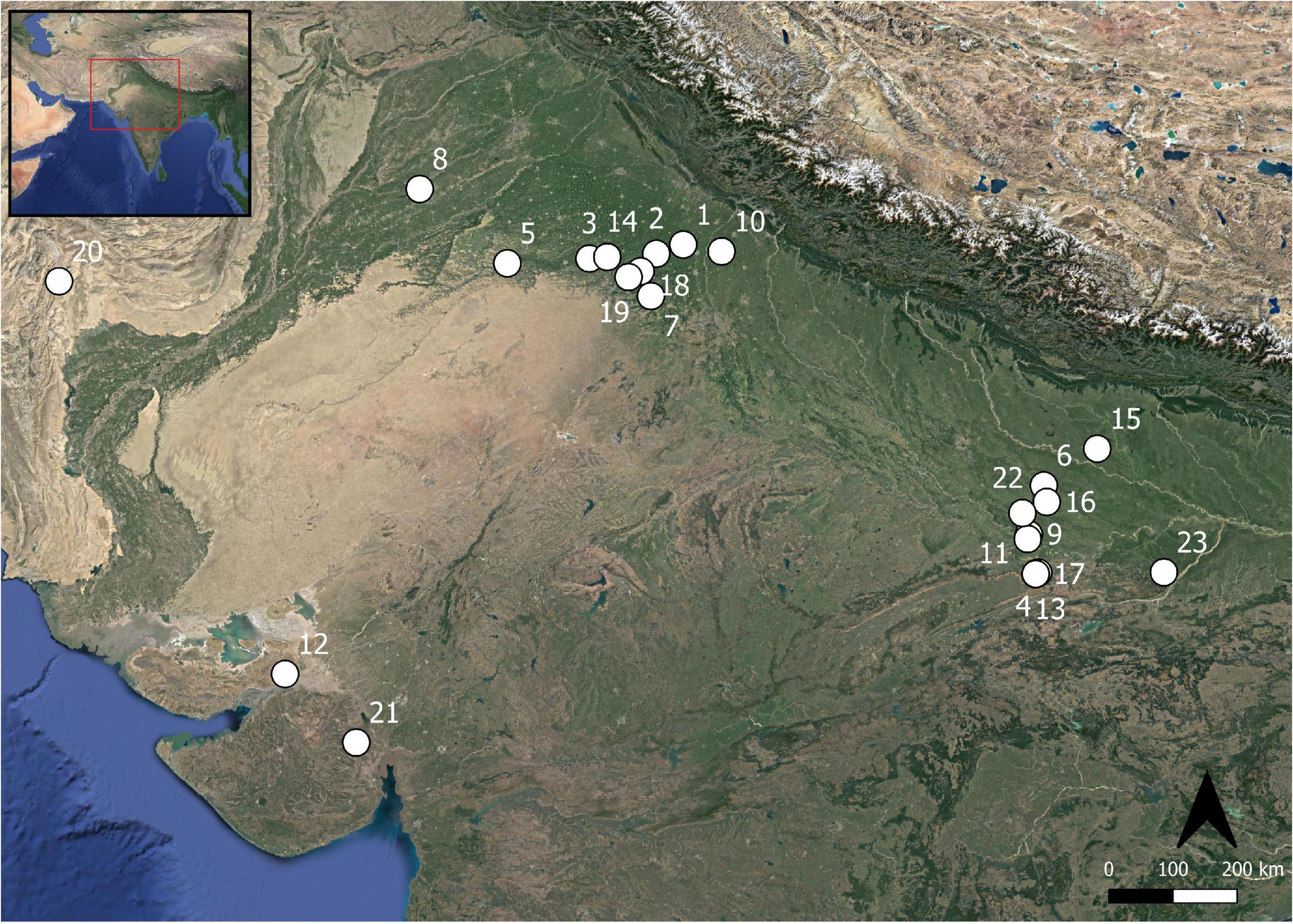
Figure 1. Map of sites discussed in this study. (1) Bahola, (2) Balu, (3) Banawali, (4) Chopani Mando, (5) Dabli vas Chugta, (6) Damdama, (7) Farmana, (8) Harappa, (9) Hetapatti, (10) Hulas, (11) Jhusi, (12) Kanmer, (13) Koldihwa, (14) Kunal, (15) Lahuradewa, (16) Mahadaha, (17) Mahagara, (18) Masudpur I, (19) Masudpur VII, (20) Pirak, (21) Rangpur, (22) Sarai Nahar Rai, and (23) Senuwar 2.
To this end, the study asks the following question: What role could introducing a wild/semi-domesticated type of rice into agricultural systems in novel ecologies of the Indus region have had on the overall domestication pathways of nivara and (proto-) indica? By focusing not just on the earliest instances of South Asian rice use, it will expand the horizons for understanding this complex cereal. In addition, the diverse and alternative agricultural methods exploited along the pathways to domestication could provide ideas for the future to help us move away from the restricted and harmfully intensive systems that are used in recent times.
Besides thinking about the impact of new agricultural environments on rice domestication in South Asian prehistory, the study also asks the following question: What data are available to think about early South Asian rice use, and do the different proxies used to think about rice domestication create different narratives about rice domestication? Why are these narratives sometimes at odds with one another, and what additional data are needed to fill the gaps in our existing datasets and bring the narratives into consensus with one another?
The study is structured around the proto-indica hypothesis debate, which is introduced briefly before being unpacked through a discussion of the ecological variability of wild rice and the impact of this on narratives on rice domestication. The types of data used in Gangetic rice reconstructions are then outlined, followed by a review of the diversity of proxies and data (biogeographic distribution, genetics, and archaeobotany) used to build different hypotheses. Indus archeobotanical datasets are then introduced, and how rice has been theorized within these datasets, followed by rethinking and retheorizing of early rice domestication in light of niche construction theory in novel environments. The study is concluded by considering not only the role of novel Indus agriculture and ecological settings and different proxies on our understanding of early rice domestication pathways in South Asia, but also the implications the different narratives have for thinking about rice agricultural intensification in later historic and even modern settings.
Through this review, the study addresses the gap in the archeological record by exploring how South Asian rice grew, how and why it was incorporated into local gathering and cultivation systems, how much of the local dryland agricultural systems were retained when paddy/wetland rice was introduced, and how the nuanced agricultural systems of the Indus and Ganges were entangled within this past, present, and future. In doing so, the implications that this understanding has for the variation we have lost in our modern agricultural systems are considered.
The Proto-Indica Hypothesis: Introducing the Debate
Japonica rice cultivation began in the Yangtze Valley in c.9000 BP (Fuller et al., 2009, 2010; Gross and Zhao, 2014; Allaby et al., 2017; Gutaker et al., 2020) based on archeobotanical data, and we can also see that key genes associated with domestication were fixed in the population as a result of previous studies (Fuller, 2011; Huang et al., 2012; Silva et al., 2018; Gutaker et al., 2020). However, hybridization between japonica and another rice, the South Asian indica, is the reason we have our modern rice (Fuller et al., 2010; Gross and Zhao, 2014; Silva et al., 2018), with later developments leading to other sub-varieties and subspecies. The two types, japonica and indica, have two clearly distinct wild sources as shown by both chloroplast genes (Tang et al., 2004) and nuclear genome data (e.g., Ohtsubo et al., 2004; Vitte et al., 2004; Londo et al., 2006; Kovach et al., 2007; McNally et al., 2009; He et al., 2011; Civáň et al., 2015; Choi et al., 2017).
The origins of indica, unlike japonica, are debated, and a prominent hypothesis that has arisen is the “proto-indica hypothesis” (Fuller et al., 2010; Fuller, 2011; Castillo et al., 2015; Silva et al., 2018). The hypothesis argues that the “independent rice tradition in north India […] never […] proceeded on its own to full domestication” until the arrival of japonica rice in the second millennium BC (Fuller, 2011, p. 82). It proposes that, while a semi-domesticated type of rice existed in South Asia prior to 2000–1500 BC, it was not fully domesticated and that it required Chinese domesticated japonica genes to reach full domestication. This semi-domesticated indica ancestor, proto-indica as Fuller et al. (2010; see also Fuller, 2011; Castillo et al., 2015; Silva et al., 2018) termed it, was under pre-domestication cultivation. By back-crossing and transference of domesticated traits with fully domesticated japonica, indica evolved (Fuller et al., 2010; Fuller, 2011; Castillo et al., 2015; Silva et al., 2018). Originally, Fuller et al. (2010) placed the hybridization event at c.1500 BC, but more recently, Silva et al. (2018) suggested that we might need to move this back to c.2000 BC. The hypothesis was developed out of a slightly different idea from Sang and Ge (2007), who proposed that two separately domesticated rice lineages later exchanged domestication genes; however, Fuller et al. (2010) regarding the proto-indica hypothesis argue that, rather than two fully domesticated rice lineages hybridizing, wild/semi-domesticated indica was hybridized with the fully domesticated japonica.
Some genetic data support the proto-indica hypothesis [refer to Sweeney et al. (2007) on pericarp mutations and summaries in Silva et al. (2018)] as this study will unpack and suggest that there was a hybridization event between japonica and (proto-)indica at the start of the second millennium BC (Molina et al., 2011; Castillo et al., 2015; Silva et al., 2018). However, controversy arises from other genetic data and the archaeological record (unpacked below) regarding what exactly the domesticated japonica was meeting. It remains an open question as to whether the rice in South Asia from c.2000 to 1500 BC was a wild/semi-domesticated or fully domesticated type. This relates to what kind of exploitation of rice (nivara, indica, or proto-indica) was occurring in the period between the first exploitation and the arrival of japonica in the second millennium BC and how the domestication pathways of rice in the period of the 7th millennium to 2nd millennium BC unfolded, and within this, water was a key component of the system.
Dry Versus Wet Rice: Ecological Response to Drought Stress
Water is a critical variable in modern rice agricultural systems (White, 1995) and in narratives on rice domestication. There are numerous ways rice can be grown; Kingwell-Banham (2019) outlines the spectrum ranges across the dry to the wet systems. Dry systems can be defined as those systems using < 800 mm of water, while wet systems are those using > 1000 mm, although there is a bit of a blurred zone in between (Kingwell-Banham, 2019). Within this spectrum, there are ways that one can grow rice by manipulation of water and the agricultural environment: dry-upland systems, dry-groundwater fed or lowland rainfed systems; wet flooded or decrue systems, paddyrice, and deepwater rice. Each system exploits and manipulates the water environment of rice, changing the ecology around it (Figure 2).
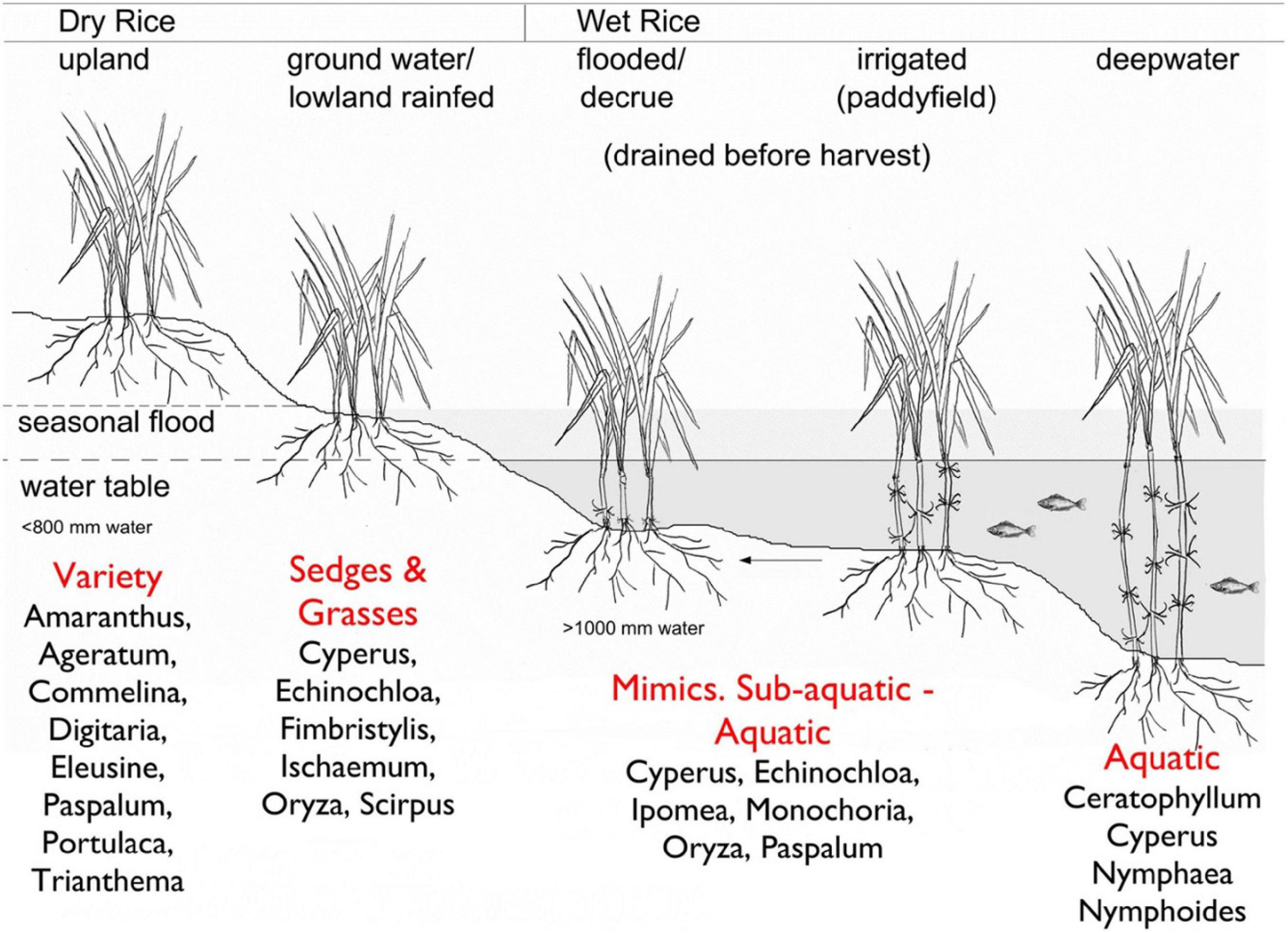
Figure 2. From Kingwell-Banham (2019, Figure 3) “rice cultivation systems classified according to elevation and water availability, with example weed profiles (as per Fuller et al., 2010).” This shows the wide range of ecological settings for rice, and the diversity of weed ecologies that can cohabit them. This Figure has been reprinted following a Creative Commons Attribution 4.0 International License (http://creativecommons.org/licenses/by/4.0/), and has been reprinted following the study of Kingwell-Banham (2019) Dry, rainfed or irrigated? Re-evaluating the role and development of rice agriculture in Iron Age-Early Historic South India using archeobotanical approaches. Archaeological and Anthropological Sciences (https://doi.org/10.1007/s12520-019-00795-7).
This is particularly relevant to the domestication narrative, as wild rice yields are linked to mild water stress. When seasonally water-stressed, the plant produces greater yield. The extent humans can use this, however, is related to the species of rice present, its native habitat, and its sensitivity to water stress. The two wild rice species of relevance for this study are nivara and rufipogon (refer to Oka, 1988; Vaughan et al., 2008a; Choi et al., 2017; for details on genetic discussions).
Nivara is the wild rice of South Asia. It is an annual grass of seasonally monsoon-inundated ponds and produces high grain yields when the monsoon ends. The natural stress response to a natural drying trigger has led to Fuller and Qin (2009), p. 90) and Fuller (2011) arguing that it would not only have been an attractive high yield resource but that there would have been little need to artificially stress the plant to make it produce more grains as the seasonal nature of the water environment already did that. Ethnographic data also suggest that nivara is harvested in its wild form at present by gathering bundles of panicles together in their immature form to reduce loss of grains due to shattering, thus potentially reducing selective pressures for non-shattering grain types (Fuller and Qin, 2009). The high productivity and predictability of nivara meant that it could be effectively exploited in its wild state (Fuller and Qin, 2009). This would have discouraged intensive cultivation and, therefore, any selective pressures being placed on it by people would eventually lead to domestication.
We can place this hypothesis in contrast with that proposed for wild rice in China, rufipogon. As a perennial grass that grows permanently on wet soil, often far from shore, it produces unpredictable yields year by year, as the water stress that encourages yields is not regular or constant (Fuller and Qin, 2009, p. 90). In addition, it can reproduce through grains or by tillering. Vegetative growth is often more common than grain production (Fuller, 2011, p. 80), and it only prefers to make more grains if there is a drought. Based on this ecological response to drought stress, Fuller and Qin (2009), p. 90) and Fuller (2011, p. 80) have argued that humans could have manipulated soils and water conditions to create an artificial drought at a certain point during growth, thus forcing the plant to favor grain over vegetative tissue production, and to ensure a reliable and predictable yield. The added benefit is that, in order to stress the plant, it would need to be closer to shore, making it more accessible and easier to use. Fuller (2011) argues that over time this would have led to selection for annual, higher-yielding, larger-grained plants and eventually to full domestication as japonica rice by entanglement of human choices, ecological manipulations, and plant responses and needs.
Therefore, while Chinese rice required domestication of rufipogon to japonica through change in its water requirements and habitat in order for it to be a profitable crop, Indian nivara rice could be harvested without any intensive cultivation or selection by habitat alteration (Fuller and Qin, 2009; Fuller et al., 2010; Fuller, 2011). This is, however, a hypothesis based on ecological requirements of and reactions to water conditions of wild rice taxa, with some ethnographic data to support it. It needs support from archaeobotanical data to show that people in different places with different taxa were interacting differently with these taxa, that the way rice changed was different depending on the taxa and the ways people were manipulating the growing conditions to alter yields, and that this all occurred simultaneously. While increasingly we see this kind of evidence occurring in China (e.g., Zheng et al., 2007; Fuller and Qin, 2009; Fuller et al., 2009; Zhang et al., 2012; Deng et al., 2015; Crawford et al., 2016), the data for early rice use from South Asia are less clear, as this study outlines, and consideration of what is actually happening in terms of the rice and its cultivation/agricultural setting is needed to test the proto-indica hypothesis.
Origins of Rice in the Ganges
Water is an important factor in rice ecology, but it is also important to other plants and animals, and in the Gangetic region of north India, it was a key element in the Mesolithic way of life. We see early Mesolithic sites located on or near water bodies. Sites like Lahuradewa were located on lake edges, while others like Chopani Mando were located on the banks of rivers (Pandey, 1990; Pal, 2016). The wetlands acted as the nexus of floral and faunal resources, taking advantage of water and fertile lands. While Mesolithic Lake Culture peoples were not specialized fisherfolk and were instead focused on broad-spectrum hunting strategies (Pandey, 1990), aquatic resources would have been an important and seasonally stable resource (Pal, 2016). Dentition microwear from human remains and the lithic microwear show a coarse diet consistent with hunting, foraging, and gathering (Lukacs and Pal, 1993; Lukacs, 2016), which is reflected in zooarchaeological and archeobotanical remains. At the site of Mahadaha and Damdama, wild cattle, gaur, nilgai, chital, hog deer, wild pig, hippopotamus, and a variety of wet-land bird and reptile species have been identified (Chattopadaya, 2002; Joglekar et al., 2003; Pal, 2016). Pal (2016) notes that the microwear analysis on lithics shows diversity of materials being exploited, from hides to meat to plant matter to bone, antler, and wood. The wetlands were the natural habitat for wild nivara rice, and it is unsurprising then that these are areas where we find the earliest dated rice in South Asia2.
The earliest evidence for rice use is dated to 6409 BC (8359 cal BP) at Lahuradewa in the Middle Ganges (Tewari et al., 2008, p. 350) in the form of charred grains. There has been some suggestion that rice use may stretch as far back as the 8th or 9th millennium BC (PRL3031 9570 ± 120 uncal BP, Tewari et al., 2008), but these dates are disputed (refer to Fuller et al., 2010), and it is more generally accepted that occupation and incipient rice use began at Lauhradewa in the 7th millennium BC (Tewari et al., 2003, 2005, 2006; Saraswat, 2004, 2005; Singh, 2005a,b; Pokharia, 2011).
Charred rice grains (n = 26; Tewari et al., 2008) were found in the earliest phase of Lahuradewa, site phase 1A. Tewari et al. (2008) have suggested that this rice was domesticated based on morphometrics of the grains, but this has been contested (e.g., Fuller et al., 2010; also refer to the detailed blog discussion that Fuller outlined in 20093). Grains are not a particularly diagnostic feature in rice domestication (Thompson, 1996; Harvey, 2006); instead, other features like changes in the spikelet base shattering structure would provide more secure evidence. As a result of this, Fuller et al. (2010) have argued that the grain morphometrics at Lahuradewa overlap with those of wild rice and that the rice exploitation at Lahuradewa represents not agriculture using domesticates but part of (perhaps the beginning of) the long history of wild rice use/cultivation in South Asia. The nuances of terms like “agriculture:” and “cultivation” and their theoretical baggage will be unpacked below.
Four spikelet bases were found at Lahuradewa in the early levels that Tewari et al. (2006, p. 49, 2008; refer also to Tewari et al., 2008; Pokharia, 2011) contest are domesticated in form. However, this was an extremely low number of spikelet bases, even if they do have attached chaff and grains that are still intact, suggesting good preservation. This tiny data set is difficult to use to argue anything, and a study on other small botanical objects has shown that things of this tiny size can move profiles up and down (Motuzaite-Matuzeviciute et al., 2013). Five hundred-μm spikelet bases may be contamination from later levels; more are needed to make a stronger argument related to both the overall domestication status of rice at the site and especially to any domestication pattern in the region over time (cf. Fuller et al., 2010). Fuller, in his 2009 blog post (see footnote 3), made the point that the Figures from Tewari et al. (2008), rather than showing domesticated type bases, appear to show immature spikelets with long rachilla still attached as a key feature (e.g., Tewari et al., 2008, Figure 16.3), while others shown in the Figure were of wild types. This combination of wild and immature types is not indicative of a domesticate harvest or a domestication process but instead a wild cultivation practice targeting grains that do not fall off the panicle easily. This could lead, through unconscious selection biases, to domestication, but, in itself as a small dataset (n = 4), it does not indicate domestication (let along domesticates). In addition, with his critiques of the grain morphometrics [stated in both the blogpost and in peer-reviewed articles like Fuller et al. (2010) among others] and the contention that the husk fragments and impressions in ceramics showing a domesticated double-peak husk pattern (see Tewari et al., 2008) have not been tested by other datasets of modern experiments, Fuller argues that the data at Lahuradewa are not evidence for early domestication, domesticated and extension agriculture, but instead for wild cultivation. I am inclined to argue that what we see is the beginning of a long process of the domestication pathway, with selection of immature grains by green harvesting that could lead to domestication, making Lahuradewa an exciting site in the rice story for South Asia.
Beyond macrobotanical remains, phytoliths were used to support arguments about rice domestication and use at Lahuradewa. Rice phytoliths were found in lake cores at Lahuradewa (Tewari et al., 2008). Tewari et al. (2008) argued that these included a mix of “wild types” in the earliest parts of the core alongside “domesticated types” c.7010 ± 170 BP (Tewari et al., 2008) and that this continues throughout. However, determining wild/domesticated rice from phytoliths is not simple. There have been arguments for the use of scale presence on bulliform phytoliths to look at domestication; bulliforms with more than nine scales on the lateral side and of a large size have been argued to be of a domesticated type (Zheng et al., 2003; Lu et al., 2008; Wu et al., 2014; Ball et al., 2016; Ma et al., 2016; Zuo et al., 2017), but this has been shown to be complicated by the variable reactions that phytoliths have to changes in evapotranspiration rates and CO2 concentrations (Ge et al., 2010; Issaharou-Matchi et al., 2016). Caution is therefore needed before using rice phytoliths to look at domestication (Pearsall et al., 1995; Fuller and Qin, 2009; Gu et al., 2013). The sample location, the lake adjacent to the site, is also not directly linked to human occupation activity or levels in the site. The core has been dated, but determining whether phytoliths show a background signature of rice presence in the environment or human use of phytoliths is complicated by the sample location, the natural habitat of nivara rice.
Lahuradewa is often cited as the best evidence for early rice use regardless of which side of the domesticated/wild argument one falls on, but similarly early finds of rice have been made at the Mesolithic sites of Damdama (Kajale, 1990) and Chopani Mando (where rice grain impressions in pottery have been identified (Sharma and Misra, 1980) and are suggested as wild-type) and, slightly later, at the Neolithic sites of Jhusi and Hetapatti (Sharma and Misra, 1980; Varma et al., 1985; Kajale, 1990; Pal, 2008, 2016). Again, the dates from these sites have been debated (Fuller et al., 2010). Lahuradewa phase 1B rice was an established and dominant part of the archeobotanical assemblage at the site. Rice, regardless of its domesticated or wild status, became critical to the economy of the people (refer to Pokharia, 2011). The domesticated status of the rice, however, in 1B faces similar challenges that the rice from the earliest phase does: low numbers, reliance on morphometrics, and few spikelet bases. Non-native crops appear in phase 1B including wheat, barley, and lentils, non-native animals such as sheep/goat are also present, and Indus-like ceramics are seen (dish-on-stand shapes for example) (refer to Tewari et al., 2008; Pokharia, 2011). The barley at the site has been dated to 4300 years BP (Tewari et al., 2008; Pokharia, 2011), and overall, phase 1B dates to 4170 ± 180 BP (cal. 2700 BC) (Pokharia, 2011).
Exactly what the systems of exploitation were in the Ganges during this Mesolithic period remains an area that needs further exploration. As noted in hypothetical scenarios laid out by scholars like Fuller and Qin (2009), Fuller (2011), and Kingwell-Banham (2019), we can see the importance of understanding whether the water environment was being manipulated or not, whether rice gathering was being intensified, and whether cultivation strategies were being introduced that might have changed the way the rice was growing and the conditions it was growing. It is not until we get to the third millennium BC that we have discussions about such patterns occurring, and this is outside the natural growing range of nivara rice, far to the west in the Indus Civilization (Bates et al., 2017a; Petrie and Bates, 2017) and slightly later in the second millennium at sites in the Middle Ganges like Senuwar 2, Koldihwa, and Mahagara (Harvey et al., 2003; Harvey and Fuller, 2005; Harvey, 2006; Fuller et al., 2010). How this movement outside the natural ecological setting and environment of wild rice impacts rice domestication needs to be explored further, but first it is important to lay out what kinds of data have been used to think about the South Asian rice story and how this impacts the coherence of any narratives being formed.
The Gap Between Datasets: How the Different Proxies Used Impact the Narratives for South Asian Rice
Vaughan et al. (2008b; refer also to Vaughan, 1994; Gao, 2003; Vaughan et al., 2005; Sang and Ge, 2007) have outlined the biogeographic distribution of modern nivara rice. As an annual type of rice that inbreeds frequently, nivara has a fairly restricted distribution and is most common in regions of South and Southeast Asia with strong dry seasons. These regions tend to feature grasslands with seasonal pools where nivara is found at the margins of the pools or water. Nivara also tends to be found in disturbed habitats (Vaughan et al., 2008b), as these water-marginal regions are loci where multiple species congregate for water and exploit wetland resources. Vaughan et al. (2008b) even go as far as suggesting that nivara’s focus on seed production over vegetative production, as well as being water-stress related, may be linked with trampling and over-grazing.
Highlighted as regions of nivara growth are the Yamuna and Ganges basins, the east coast of South Asia, and some more patchy regions of the west coast (Vaughan et al., 2008b; also refer to Fuller et al., 2010). Fuller et al. (2010), however, note that modern distributions of wild rice are likely not to fully represent ancient distributions as climate change and human agriculture (particularly post the Green Revolution) as well as other land uses will have altered these. The changes in these regional distributions are not likely to have been expansions but shrinkages, and therefore what this suggests is that the Ganges-Yamuna floodplains are likely to be a region where early rice exploitation could have begun, as wild rice is still found there today and would likely have also been present in the past. Extending west beyond these is unlikely because of environmental constraints: temperature and rainfall differences as well as wetland differences suggest that the regions to the west in places like the Indus river basin and the interfluve are not particularly suitable for wild rice. There is also a lack of wild rice progenitors in the area. Even with Fuller et al.’s (2010) caveat of altered distributions due to climate change and agricultural land use alterations, it does not seem likely that any shrinkage in natural distribution would have led to there being a wider distribution of nivara to the west. Rice being used in the Indus region seems to be unlikely to have been occurring outside of an agricultural setting with a (semi-) domesticated species.
Genetic evidence for early rice domestication is highly complex and remains a subject of constant discovery in no short part to the complexity of both the rice genetic structure and the hybridization of rice species over time. What can be noted is that, in c.200k-80 kya, there was a divergence between lineages of nivara and japonica based on whole chloroplast genome data (Tang et al., 2004; refer also to Ohtsubo et al., 2004; Garris et al., 2005; Londo et al., 2006; McNally et al., 2009 for other genetic evidence). Nuclear genome data put this at roughly 100 kya, tallying with the whole chloroplast data (Ma and Bennetzen, 2004; Vitte et al., 2004).
There have also been recent and frequent hybridizations between cultivar4 lineages (Sang and Ge, 2007; McNally et al., 2009), although as Fuller et al. (2010) summarize, there are many possible points in history that this could have occurred. One of these is hypothesized to have been between a (fully domesticated) japonica and a (wild or semi-domesticated) nivara/indica lineage of c.1500–2000 BC and is formative to the proto-indica hypothesis and the role that japonica has played in changing rice agriculture in the sub-continent during early historic periods (refer to Sang and Ge, 2007; and the use of this by Coningham, 1995).
Genetically this proto-indica hypothesis relies on several genes, but, in particular, sh4 and qsh1 (see Fuller et al., 2010). Non-shattering of ears/panicles is a critical trait in the domestication story of cereals, and in rice, this is controlled by six quantitative trait loci (Xiong et al., 1999; Cai and Morishima, 2002; Li et al., 2006a,b; Lin et al., 2007), but sh4 and qsh1 are the focus of discussions (Fuller et al., 2010). qsh1 is found only in some of the temperate japonica rice types and is derived from a haplotype found in some rufipogon (Konishi et al., 2006). sh4, on the other hand, is a recessive mutation shared widely across japonica, aus, and indica rice (Li et al., 2006b; Lin et al., 2007). sh4 has been argued by Fuller and Qin (2009) to have been part of japonica/indica domestication and to have been introduced to India after the long period of rice use/cultivation (the “proto-indica phase” or proto-indica cultivars). This is part of the hypothesis of proto-indica – that genes of domestication, that carry with them domestication syndrome traits including the non-shattering spikelet bases, are introduced after the arrival of Chinese domesticated japonica rice of c.2000-1500 BC.
This is somewhat debated by Zhang et al. (2009), who suggested that sh4 was a rapidly evolving gene after domestication and that, by hybridization between cultivated rice types, this would have become widespread quickly. sh4 would not have been part of the initial evolution of non-shattering rice and thus is not a crucial piece of genetic evidence for a (proto-)indica-japonica hybridization story. sh4 demonstrates the complexity of the genetic evidence available in both discussing the rice domestication story and in dating or identifying key moments like the hybridization between (proto-)indica and japonica.
Looking at Fuller et al. (2010, Figure 3), it becomes even more apparent how complex the narrative based on the genetic evidence can get and how there remain gaps and questions to be addressed (Figure 3). There is a rough timeline placed in Fuller et al.’s (2010, Figure 3) of “The Holocene,” although it is not made clear what the speed or divisions of this timeline are, as genetics is complex when it comes to dating events. The domesticated gene pool of japonica [shown carrying sh4 as per Fuller et al.’s (2010) hypothesis, although refer to Zhang et al. (2009)] is shown on an evolutionary pathway toward more modern japonica varieties. At some point, additional genes are expressed or become introduced to this domesticated japonica gene pool: Prog1 (erect growth), rc (white grain pericarp), sw5 (wider grains), qsh1 (non-shattering), and wxy (low amylase waxy/glutinous). It is not clear in the Figure when these happened, but the Figure suggests that, while carrying sh4, rc, and Prog1, japonica meets with a wild cultivated proto-indica (assumed to be nivara under cultivation) and a wild gene pool of nivara, hybridizes and forms a new wild nivara (presumably by carrying the new genes? The Figure is unclear), and what the Figure labels as “dW” (code for domesticated wild type, presumably a semi-domesticated or wild type under cultivation) proto indica. This proto-indica, now carrying sh4, rc, and Prog1, backcrosses under hybridization with wild cultivated proto-indica not carrying the genes (the nivara from earlier) to form domesticated indica. As can be seen from this description, it is extremely complicated trying to parse out the possible routes and moments in rice genetics for indica/nivara. Exactly what the status of the “proto-indica” is in this Figure is also unclear, whether this is semi-domesticated with genes of domestication being fixed from cultivation acts or if it is simply a fully wild thing under some form of human cultivation with no changes resulting from that. How nivara, rather than rufipogon/japonica, reacts to cultivation remains open to genetic exploration.
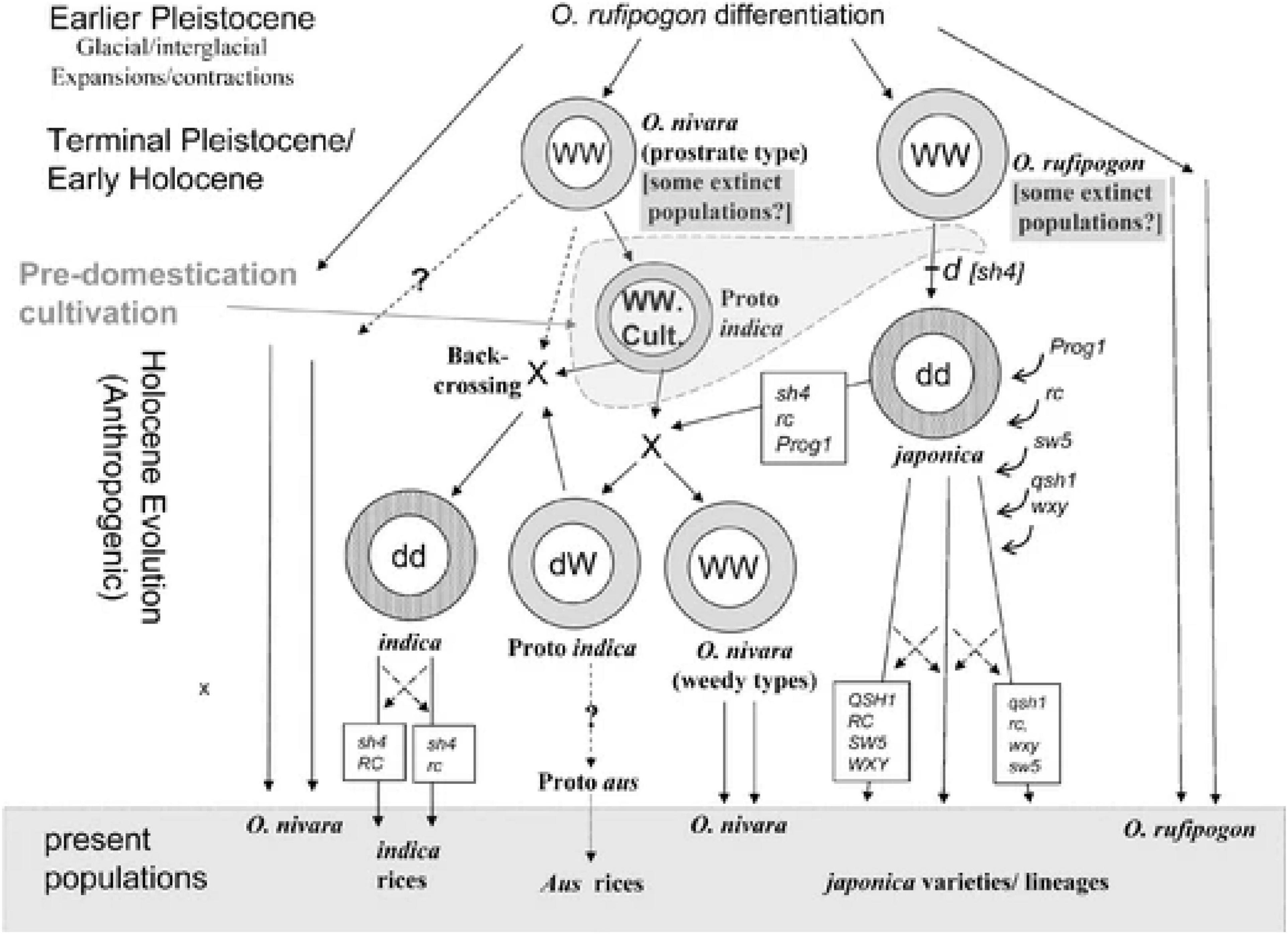
Figure 3. From Kingwell-Banham (2019, Figure 3) captioned “A simplified diagram of the main evolutionary pathways to domesticated Asian rice, with selected key mutations indicated, differentiating wild-type dominant form with all capital letters and domesticated type recessive alleles with lowercase letters. Mutations: sh4,non-shattering; Prog1, erect growth; rc, white grain pericarp; sw5, wider grains; qsh1, further non-shattering; wxy, waxy/glutinous rice (low amylase); WW, wild gene pool; dd, domesticated gene pool; cult., cultivated; X, major hybridization event; crossed arrows indicate continued gene flow (introgression).” This figure from Kingwell-Banham (2019, Figure 3) shows the complexity of rice domestication and hybridization and the way the proto-indica hypothesis is conceived Fuller et al. (2010). Reprinted with permission from Springer Nature Customer Service Centre GmbH (the Licensor): Springer Nature, ARCHAEOLOGICAL AND ANTHROPOLOGICAL SCIENCES. Consilience of Genetics and Archaeobotany in the Entangled History of Rice, Fuller et al. (2010). Article hyperlink: https://doi.org/10.1007/s12520-010-0035-y (Journal homepage hyperlink: https://www.springer.com/journal/12520).
The genetics then is complex, and it is unclear what exactly is happening in South Asia, only that, at some point in the past, it seems likely that genes from japonica like sh4 and qsh1 have been introduced, along with others linked with larger grain size and pericarp changes (e.g., Jin et al., 2008; Tan et al., 2008). The archeobotanical evidence is equally complex. While the Chinese data are more fleshed out (refer to Fuller et al., 2010 for a summary), the data on South Asia are less comprehensive.
The macrobotanical evidence for rice domestication is hampered by a grain that shows vast variation between modern cultivars and between wild populations, often ecologically and climatically linked. Trends toward larger grains over time in South Asia can be seen (Fuller et al., 2010, Figures 5b,c), but there is a regional variation that is potentially related to both ecology and gene flow between wild and domestic populations over time (Fuller et al., 2010). This all links back to the critiques by Fuller (2011; refer also to Fuller et al., 2010) of the morphometrics at Lahuradewa. Not only was the sample size small in phase 1A at the site (Tewari et al., 2008; Fuller, 2011; Pokharia et al., 2011), but the grain size range overlaps with both wild and domesticated populations, so more studies are needed to be conducted to disentangle this.
Beyond thinking about grain size changes, grain presence can be used to show the potential for people to have been using rice as food, but rice (or any other food item) alone does not show gathering/cultivation/agricultural strategy. More information on associated weeds of cultivation is needed to look at how these plants might have been tended or even grown. Weed analysis on early rice in South Asia is rare, with only later period assessments, for example, by Fuller and Qin (2009), looking at changes across the hypothesized japonica hybridization period as an example, and studies on the Indus looking at rice agricultural systems (Bates et al., 2017a) being examples of this [refer also to Morrison, 2016; Kingwell-Banham et al., 2018; Naik et al., 2019; in south and east and Wolff et al. (2022) for a discussion of the state of study on weeds in archeology]. Phytoliths have been used to look at weed floras associated with changing rice watering regimes in China and Odisha (Weisskopf et al., 2014, 2015) but have yet to be applied to Gangetic rice domestication periods. While phytoliths have been looked at in the Lahuradewa lake (Tewari et al., 2003, 2006, 2008), this was more a matter of whether there was domesticated rice present, and as already noted earlier in this article, this is a complex and problematic question.
An alternative approach for looking at grains for rice domesticated status is to look at spikelet bases. The 500-μm chaff elements that attach the grain spikelet to the panicle have been an uncommon find generally in archeobotany due to their size (Fuller et al., 2010). Spikelet bases are the clearest evidence of domesticated status due to morphological changes associated with shattering/non-shattering panicles (Thompson, 1996; Sato, 2002; Harvey, 2006). Thompson (1996) argued that there should be three categories considered: wild, domesticated, and immature. Wild types have a straight profile at their bases with smooth and round abscission scars and small distinct vascular pores resulting from shattering (Fuller and Qin, 2008, 2009). Domesticated types have larger and more irregular pores, an uneven profile that is dimpled in appearance and is overall less symmetrical. This is a result of threshing to separate non-shattering spikelets (Fuller and Qin, 2008, 2009). Immature spikelet bases also considered as grains will be harvested green in order to reduce the likelihood of yield loss, and immature spikelet bases have protruding vascular bundles from the remnant of the attached rachilla (Fuller and Qin, 2008, 2009). Although exceptionally small compared with grains, spikelet bases can be found using a fine mesh on flotation samples. Large numbers of these are needed to think about the processes of domestication. In South Asia, however, like grain, weed, and phytolith analysis, the number of spikelet bases available for analysis increases later in time than we look. While there are couples available from Lahuradewa in the early phases, these are extremely small in number (n = 4, Tewari et al., 2008), and their domesticated status is disputed (see Fuller, 2011). It is only in the Indus c.3200 BC far to the west (Bates et al., 2017a) and, in later periods, in the Ganges at sites like Mahagara (Harvey et al., 2003; Harvey and Fuller, 2005; Harvey, 2006; Fuller et al., 2010) that we see larger numbers that can be discussed in terms of proportional changes in spikelet base types in order to think about domestication processes.
As ever, dating of material remains a challenge. Genetic changes have already been noted to be highly complex to date “was the arrival of japonica-carried genes sometime in c.1500–2000 BC or more recently? At what pace does this occur?” Radiocarbon dates for the Mesolithic of the Ganges have been typically based on the bone (see for example Agrawal and Kusumgar, 1975; Rajagopalan et al., 1982; Indian Archaeology a Review, 1989-90 for dates from Mahadada, Sarai Nahar Rai, and Damdama, respectively), often with a wide variation in results. With Lahuradewa providing dates specifically for early rice, and with the dates being highly controversial (see earlier discussion in this study), more dates on non-bone and on rice specifically are required to explore both the dates of the Mesolithic and of the use of rice.
What this all implies then is that while we might argue that early rice use began in the Ganges-Yamuna plains, based on the limited archeobotanical data, we have available and modern biogeographic rice distributions, how the rice was used, whether it was part of a domestication story or not, and exact dates of this use remain open for discussion. Whether this was gathering, cultivation, or agriculture is also highly debated, and how this developed over time is poorly understood. Genetically, the picture is not helped by a complex hybridization and backcrossing picture, and while it seems likely that many of the genes related to rice domesticated status came from introduced japonica, exactly when this happened is not at all clear. Indeed, what the exact status of nivara was, when it met japonica, whether this was “proto-indica” (semi-domesticated or wild under cultivation, both sensu Fuller et al., 2010) remains open for debate.
Instead, most comprehensive data for rice domestication and cultivation/use in early South Asia come not from the Ganges region but from far to the west in the Indus region, despite this being outside the natural ecological zone for wild rice. This is later in date than the earliest material from the Ganges (that at Lahuradewa and similar but less well-dated sites like Jhusi, Hetapatti, and Chopani Mando). Open for debate then is what happens to this “proto-indica”/nivara under the new ecological settings it finds itself in the Indus Civilization. It is to this that we can turn to think about how new ecological conditions, both environmentally and agriculturally, might have affected this plant during its long-protracted pathway to domestication.
Available but Not Fully Adopted? The Indus Rice Story
While rice use in India probably began in the Gangetic region, the largest body of early evidence we have comes from far to the west in the Indus Civilization region c.3200–1500 BC. The evidence for rice use in the Indus Civilization has traditionally been highly contentious. Fuller and Madella (2002, pp. 336–337) have argued that “rice was available as a crop […] but not adopted” and that “there is no reason as yet to believe it was an important crop,” while Fuller and Qin (2009) have argued that there is no evidence of rice agriculture until the Late Harappan period c.2000 BCE, when it is likely that the japonica rice arrived. However, there has been a long tradition of rice data from Indus sites, and it has been strengthened over time with new data especially since these statements were published.
The evidence first cited by scholars for rice use by Indus peoples was based on impressions of grains in ceramics. These were found in Gujarat and Rajasthan (e.g., Ghosh and Lal, 1963; Vishnu-Mittre and Savithri, 1975), although these have been questioned (refer to Fuller, 2002) given the complexities in using impressions as evidence for species or even genus level identifications. Since then, grains have become more common finds. Rice grains have been found at Early and Mature Harappan Balu and Early Harappan Kunal (Saraswat and Pokharia, 2002, 2003), Mature Harappan Banawali (Lone et al., 1987), and Late Harappan Hulas (Saraswat, 1993), although all in relatively small numbers. This is also the case at Kanmer (Pokharia et al., 2011, Table 3), although as will be noted momentarily, there is some concern about the dating of these. Grains have also been found at Harappa, Rangpur, Farmana, and Pirak (refer to summary in Bates, 2019a). Macrobotanical evidence (Weber, 1997, 2003) is supported by phytolith data from Harappa (Fujiwara et al., 1992; Fujiwara, 1993; Madella, 1997, 2003), which pushes rice presence at the site back to the Mature Harappan. Larger numbers of grains have been found alongside spikelet bases at the sites of Masupdur I, Masupdur VII, Bahola, and Dabli Vas Chugta in Rajasthan and Haryana (Petrie et al., 2016; Bates et al., 2017a).
This is, therefore, a growing dataset that suggests that far from rice merely being available to Indus peoples, it was, in fact, adopted and incorporated into the agricultural assemblage, at least at some sites (Petrie et al., 2016; Bates et al., 2017a; Petrie and Bates, 2017). One critique of rice presence at Indus sites has traditionally been the poor dating of this and other summer kharif crops. For example, at sites like Banawali, while rice presence has been placed in the Indus period, this is based on their discovery in phases with Indus ceramics and on radiocarbon from other cereals in these phases rather than directly on the rice itself, and some of the “relative” absolute dates returned problematic ranges. At Banawali, directly dated wheat in proximity to rice that was found at Mature Harappan levels returned a date of cal AD 80-231 (Saraswat et al., 2000; Saraswat and Pokharia, 2003). At Mature Harappan Kunal, direct dates on wheat grains came back as 1500-1311 cal BC (Saraswat and Pokharia, 2003), raising questions about the date of rice in the same stratigraphic context. Motuzaite-Matuzeviciute et al. (2013) have shown that millets can very easily move throughout a profile because of acts like bioturbation and soil profile movement. With the late dates on even large materials like wheat that have been thought to be relatively stable in a soil profile in proximity to rice grains that are being disputed as Harappan or not, it required researchers to gain more secure dates on the rice themselves from Indus sites to show that they were not later period contaminants. While these issues may not have applied to all “relative” absolutely dated rice at all sites, it required some directly dated rice grains to support arguments that rice was used by Indus peoples and was not just a contaminant from later occupations at sites.
At Kanmer, direct dating of rice was attempted to prove or disprove the Indus peoples’ use of rice (Pokharia et al., 2011). The rice, however, produced dates that were much later in time for the phases they were found in [PLD-16351 AD 335 (95.4%) AD 425 and PLD-16353 AD 321 (80.1%) AD 410; Pokharia et al., 2011: 1836], suggesting they may have been contaminants from later stratigraphic layers (Pokharia et al., 2011). At the sites of Masupdur VII and Masupdur I, however, we have clear and direct evidence for rice use in the Indus period along with other summer kharif crops (Petrie et al., 2016). The dates of rice from Masudpur VII came back to the Late Harappan with dates of 1886-1695 cal BC (prob. 95.4% OXA28661_571) and from Masupdur I the dates were Mature Harappan at 2431-2144 cal BC (prob. 95.5%, OXA28663_231) (Petrie et al., 2016). Rice was also found in context with multiple directly dated Early Harappan crops (Petrie et al., 2016). The crops include small millets like Echinochloa cf colona and Setaria cf. pumila, mung bean, and urd bean (Vigna radiata and mungo), horsegram (Macrotyloma uniflorum), and cereals like wheat and barley as well as winter pulses (Petrie et al., 2016; Bates et al., 2017a,b, c; Petrie and Bates, 2017). The dates on these crops in association with rice suggest we can push back the dating of summer crops at the site into the Early Harappan (Petrie et al., 2016), supporting the arguments made by Ghosh and Lal (1963), Vishnu-Mittre and Savithri (1975), Weber (1991; 1997; 1999; 2003), Fujiwara et al. (1992); Fujiwara (1993), Madella (1997, 2003), Saraswat et al. (2000); Saraswat (2002), Saraswat and Pokharia (2002, 2003), Weber and Fuller (2008), Kashyap and Weber (2010; 2013; 2016), Fuller (2011); Pokharia et al. (2011)Pokharia et al. (2014); Weber et al. (2011), Fuller and Murphy (2014); Kingwell-Banham et al. (2015), and Weber and Kashyap (2016), among others, regarding rice and kharif crop use in the Indus from the earliest periods.
The presence of rice at sites in the Indus suggests that it was traded from its original environment to a new one and brought in the agricultural systems of the Indus. The Indus was one of the largest Bronze Age civilizations (Childe, 1950; Kenoyer, 1998; Possehl, 1999; Wright, 2010), covering an area that includes modern day Pakistan and northern India and extending into Afghanistan with the site of Shortughai. This vast region incorporated a great diversity in environments and ecologies, as well as multiple river systems and two rainfall systems (winter westerlies and the Indian Summer Monsoon). Alongside this is the increasing recognition of the internal nuances in cultural expressions of “being Indus” (refer for example to those outlined in Kenoyer, 1998; Possehl, 2002; Petrie, 2013; Parikh and Petrie, 2019; among many others). This vast diversity in both the natural and cultural world is also reflected in agricultural systems, and far from the “dull and homogenous” world once modeled by Wheeler (1950), we are now seeing multiple agricultural strategies deployed by farmers across this complex region (e.g., Vishnu-Mittre, 1974; Vishnu-Mittre and Savithri, 1982; Saraswat, 1992; Reddy, 1997, 2003; Weber, 2003; Weber and Fuller, 2008; Pokharia et al., 2011, 2014; Weber et al., 2011; García-Granero et al., 2015, 2016, 2017, 2022; Petrie et al., 2016; Bates et al., 2017a,b, c, 2021; Petrie and Bates, 2017; Bates, 2019b,2020; Chakraborty et al., 2020; Suryanarayan et al., 2021). This includes the use of rabi (winter) crops like wheat and barley that might have been monocropped in places like Harappa (see Weber, 2003 for example), summer monsoon season kharif millets that could be maslin intercropped and grown in the dry region of Gujarat (refer to Reddy, 1997, 2003; García-Granero et al., 2016, 2017), and even a third season of the dry summer zaid with kitchen garden plants grown between these (refer to Bates, 2020). More systems than this were deployed, outlined in Petrie and Bates (2017) through the complex multicropping options open to the Indus as a result of the diversity available in both the range of crops available to farmers and the mosaic of environments and ecologies they were exploiting, as well as economic choices they were making and negotiating as part of this complex system (refer for example to Bates and Choi under review).
With rice now incorporated into these agricultural systems, people have sought to explore what rice as a potential food item might have meant to different elements of Indus society, as we see it distributed in specific parts of the Indus rather than ubiquitously across the region. Madella (2014, p. 230) has explored how rice might have been viewed by Indus people, and how the role of rice changed over time throughout the Indus period. At urban sites during the Mature Harappan period, rice was a secondary and rare crop (Weber, 2003; Madella, 2014), while by the Late Harappan period and certainly in post-Indus periods it was becoming a staple. Madella (2014) argues that at urban sites rice may have been a sought-after product during the Mature Harappan period, explaining its appearance at Harappa, outside its natural habitat and in only small quantities. He argues that rice only became a staple when its status as a rare crop was lost as superior varieties were introduced in c.2000–1900 BC [as Madella (2014) hypothesizes like Silva et al. (2018) that japonica hybridization should be brought forward to this point], and as diversification in agricultural strategies occurred during the Late Harappan period and in post-Harappan periods. This can be contrasted with the rural village sites, where rice was available often in abundance, especially in the eastern region (Bates et al., 2017a; Petrie and Bates, 2017). At sites like Masudpur VII and Masudpur I, rice was a staple, a regular part of the diet from the earliest stages of the agricultural strategy, and does not necessarily fit the “luxury” category theorized by Madella (2014). At these sites, rice was adopted in agricultural systems despite it not being native to the region, a new and unknown crop for people to explore and exploit but also a new environmental setting for the rice itself to adapt to.
With rice being incorporated into this new agricultural system, it is interesting to think about how this hypothetically wild or semi-domesticated crop (nivara or proto-indica) not only adapted to the new agricultural system in which it was placed but also what impact such systems might have had on the domestication pathways of nivara/proto-indica. The move outside the native habitat and ecology of nivara/proto-indica and out of cultivation systems into a fully agricultural system could potentially have had much strong domestication selection pressures on this crop and led to changes at a new pace and to new extents on this rice plant, and therefore this shift needs to be incorporated into the (proto-)indica narrative.
Domestication and the Role of New Agricultural Environments
In the Indus archeobotanical data, we see evidence of the rice domestication process (Figure 4). Rice spikelet bases were found at the sites of Masudpur I, Masudpur VII, and Bahola (Bates et al., 2017a). As outlined above, having spikelet bases is the most secure way to think about domestication, and having an assemblage with enough spikelet bases to form data patterns is needed to look for change over time. At these sites this was possible. Spikelet bases from the Mature Harappan phases at Masudpur I and VII were predominantly wild-type based on abscission scars (Thompson, 1996; Harvey and Fuller, 2005; Harvey, 2006; Bates et al., 2017a). These were not the only spikelet bases though; significant proportions of the domesticated type were also present (9 and 10% respectively) [Bates et al., 2017a, misreported or calculated differently in Silva et al. (2018) as 11.2 and 20.5%].
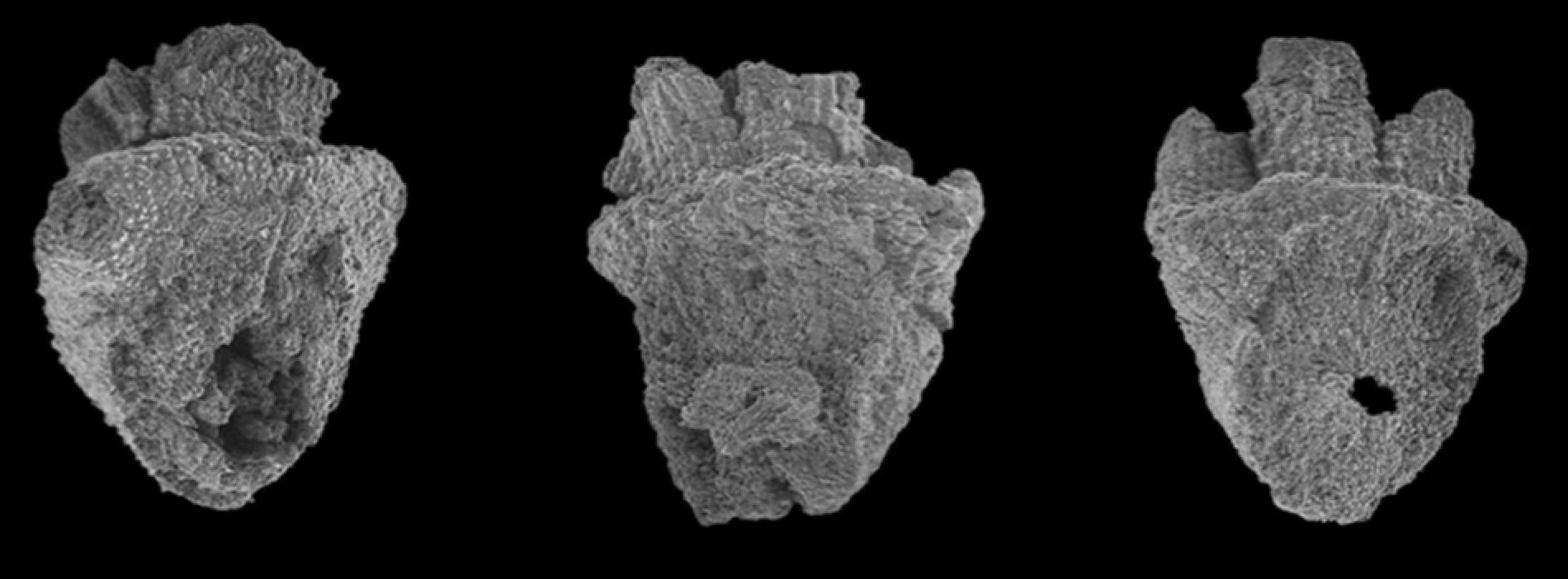
Figure 4. Rice spikelet bases from Masudpur I showing domesticated, wild, and immature types. Authors own work, originally published in Kingwell-Banham (2019, Figure 3). Figure 4 has been reprinted following a Creative Commons Attribution 4.0 International License (http://creativecommons.org/licenses/by/4.0/), and has been reprinted following the study of Bates et al. (2017a). Approaching Rice Domestication in South Asia: New Evidence from Indus Settlements in Northern India. Journal of Archaeological Science 78, 193–201. https://doi.org/10.1016/j.jas.2016.04.018.
This suggests that some selection for the non-shattering domestication trait was taking place in northern India by the time Indus peoples began using rice. Thinking back to the genetic debates, it is a timely reminder of the complexity of dealing with non-shattering trait genes. While the focus has been on sh4 and qsh1, which are traits that developed in japonica, there are four other genes associated with non-shattering rice, and it might be useful to look at nivara again with these in mind and consider whether these could have been part of the domestication story and have led to full domestication before the introduction of novel genes from China. Alternatively, as domestication is not a simply unilinear pathway, the Indus story could be a dead end, with nivara non-shattering genes not contributing to the later indica narrative.
We see a spikelet base change pattern developing into Late Harappan and post-Indus Painted Grey Ware contexts at Masudpur VII and Bahola showing predominantly domesticated-type assemblages (28.6% with a high proportion of immature spikelets still present and no wild type at Masudpur VII and 33.2% domesticated type at Bahola, 6.9% wild, and the rest unidentifiable). Comparing the proportion of identifiable spikelet bases [which Silva et al. (2018) estimated to be > 75%] with Fuller et al.’s (2014) Chinese assemblages, Silva et al. (2018) suggested that the data from Bahola (and thus by extension Late Harappan Masudpur VII) show the end of the process of domestication or a point where we can say the grains are domesticated by hybridization with incoming japonica. This raises a further question then: is the Bahola and Masupdur VII rice a result of an Indus pathway to indica domestication (and then in 1500 BC it was hybridized with japonica to introduce new genes like sh4) or is the Indus data evidence of a near replacement of a proto-indica in 2,000 BC by the arriving japonica?
Silva et al. (2018) opted to argue that the Indus spikelet base data require us to modify the proto-indica hypothesis: there was an incipient local domestication process in northern India, but that did not reach full domestication, and that we need to move the hybridization even a little further back in time to the Late Harappan period, placing it at 2,000 BC rather than 1500 BC, but further modeling (and data) is needed (Silva et al., 2018). This must remain a hypothesis, however, because it relies on only two genes for non-shattering (sh4 and qsh1), rather than thinking about other possible non-shattering genes that could have been locally fixed and replaced or not yet studied in as much depth [refer also to Zhang et al. (2009) for critique on the over-focus on sh4], and on the uncertain genetic timeline and modeling of the genetic clock and archeobotanical movements of japonica [refer to Silva et al. (2018) for hypothetical modeling].
As outlined above, the genetic data do not exactly help us with this, messy as the evidence is. Grain size morphometrics does not help either, and we lack good evidence for the movement of the (japonica) rice through regions that might link China and India (beyond the scope of this article to outline all the evidence).
Rather than focus on whether the rice used in the Indus reaches full domestication or not, it is perhaps a more fruitful line of inquiry to ask why under the Indus agriculture system we see an acceleration of domestication syndrome (certainly with regard to non-shattering traits)? For the previous c.3000 years between its first use in the Ganges at Lahuradewa and the first arrival in the Indus in c.3200 BC at sites like Masudpur VII, we see little to suggest change (although the record is, as outlined, patchy). It remained likely a wild thing if we are to go on the evidence of the predominantly wild spikelet bases seen in the earliest levels at the Indus sites. However, by the end of the Indus period, we see predominantly domesticated types, with a gradual change from wild to domesticated over time. What might have caused this?
Domestication is an extension of evolutionary pressures placed on plants (and animals) as they become entangled in the human “domestic habitat.” Larson et al. (2014, p. 6140) define it as “a complex process along a continuum of human, plant, and animal relationships that often took place over a long time period and was driven by a mix of ecological, biological, and human cultural factors,” which result in what Fuller and Hildebrand (2013, p. 508) characterize as “genetic and morphological changes on the part of the plant [and animal] population in response to selective pressures imposed by cultivation.” It is distinct from the acts of agriculture, which Harris (2007) defines as coming from the Latin ager (a field) and colo (to cultivate), suggesting that “agriculture” should be used as a specific term related to the tillage of land for crop production typically involving domesticates. He also distinguishes “cultivation” from agriculture by defining cultivation as a general term for all forms of plant growth-promoting activities, thereby including both hunter-gatherer plant management and more complex deliberate planting and tending of plants (Harris, 2007, p. 25).
It has been noted that domestication, as a long and entangled process of developing relationships between domesticators and domesticates, usually happens as interactions and behaviors change, i.e., with sickle harvesting leading to unconscious selection for seeds that do not fall off the stalk in cereals and development of non-shattering ear traits (Larson et al., 2014). This niche construction and entanglement of humans and plants and humans and animals created coevolutionary relationships, with human niche constructors as a critical component in the domestication pathway of many plants (and animals) (Smith, 2011a). The prolonged period of “pre-domestication cultivation” before the evolution of full domesticates and development of agriculture has been a prime period for studies on domestication narratives (Willcox, 1999; Kahlheber and Neumann, 2007; Willcox et al., 2008; Piperno, 2011; Willcox and Stordeur, 2012; Yang et al., 2012; Fuller et al., 2014; Larson et al., 2014).
Agriculture and agricultural settings are therefore not the usual locale for the initial domestication of plants and animals, simply because by the time we are looking at agricultural settings, most things have been domesticated, with the full suite of domesticated syndrome traits, if not fixed, is then well on the way to being fixed. We do see subsequent changes in agriculture, i.e., the development of secondary traits like glutinous versus non-glutinous rice or breeds in animals. These processes have accelerated especially since the 18th century AD with the industrial agricultural revolution (Ahmad et al., 2020), but as a site of initial domestication agricultural settings, as they already involve domesticates, are not usually explored.
The role of humans as niche constructors, however, and complex pathways of rice in South Asia, might suggest that we have a different set of processes and pathways occurring. Rice, like all cereals, involves a slow process of change (e.g., Purugganan and Fuller, 2011; Fuller et al., 2014; Larson et al., 2014). The rice in South Asia would seem to take an even longer domestication pathway than most cereals, rather than just 2–4,000 years, and it seems to take up to 5–6,000 years between the first use of rice in the Ganges and the arrival of japonica and the fixing of non-shattering genes sh4 and qsh1. These changes, however, have variable paces, there is potentially a prolonged period of pre-domestication cultivation with the Gangetic Mesolithic-Neolithic, but in the Indus data, we see an increase in the rate of change, with other non-shattering traits evolving over just a couple of millennia. While this has to be a caveat of the recognition of the patchy nature of the Gangetic data, the lack of spikelet bases, for example, and the low number of sites with rice evidence, it might be suggested that the movement of rice from the cultivation context of the Ganges into the agricultural setting of the Indus played a role in changing the pace of domestication.
Domestication, as a sustained multi-generational coevolutionary relationship between two species with each undergoing changes that enhance the benefits each party derives from the relationship and/or that make further investment in the relationship more attractive to its partner (Zeder, 2017, p. 4), can be brought into the realm of agriculture and potentially accelerated by the dramatic niche modifications offered in this novel artificial ecological setting. Zeder (2017) has argued that novel environments are likely to induce plastic responses with greater speed and more often than those seen naturally through mutation. Mutation is the result of a slow process of selection and transmission reliant initially on one individual and their reproductive fitness, while niche-constructed changes (and likely other environmental ones too) affect numerous individuals and do not necessarily need strong positive selection, just an inducing factor (West-Eberhard, 2005a,b; Zeder, 2017). Anthropogenic-induced traits occur two times as fast as non-anthropogenic drivers (Zeder, 2017), so when a plant (or animal) is introduced to a new environment, including one such as an agricultural setting, we have the potential to see rapid, population-wide changes in domesticated candidate species. When thinking about this in an agricultural setting, we might also argue that the potential for change is also increased and if a wild type should be brought under agricultural-scale cultivation.
The established agricultural setting of the Indus and the locality of this outside the climatic and ecological niche of the Ganges-Yamuna plains changed the interactions the rice plants would have had both with their environment and with humans dramatically. Acts like water management, tilling, and planting controls are heightened under agriculture in a way that is not seen in pre-domestication cultivation. Trying to bring a semi-wild type of rice within the bounds of the established Indus agricultural realm would likely have led to a much faster set of changes than we see before when the rice was subject to less intense interactions in its natural setting, being cultivated and tended to under a less intensive situation. From the point of view of niche construction and humans as niche constructors, the evolutionary impacts of ecosystem engineering activities that humans do can be a strong modifying and selective pressure that acts on present and future generations living in the altered niches (Odling-Smee et al., 2003; Post and Palkovacs, 2009; Crawford, 2011; Kendal et al., 2011; Smith, 2011a,b; Zeder, 2012a,b, 2015, 2017; Beddows et al., 2016; Laland et al., 2016).
Discussion
The role of humans as novel niche constructors means that the impacts they can have on plant evolutionary pathways are vast, leading to the domestication trajectories we see continuing to this day. This study asked the question “what role could introducing a wild/semi-domesticated type of rice into agricultural systems in the novel ecologies of the Indus region have had on the overall domestication pathways of nivara and (proto-)indica?” With the nivara rice, the movement of this plant outside its natural habitat into the stark contrast of an already established agricultural setting is likely to have had an accelerating effect on domestication processes. We can infer this from the spikelet base morphologies that we are beginning to see in the Indus archeobotanical datasets. This is tempered by the massive gaps in the datasets surrounding South Asian early rice use; it is highly likely that the rice domestication story began in the Ganges as far back as Lahuradewa, Chopani Mando, Hetapatti, Jhusi, and other sites, but there remain many gaps related to exactly what happened after the initial use, how fast any process of domestication was, and what state the rice was in when it moved out of the Ganges. Based on the data we currently have, archeobotanical, genetic, and ecological, it seems likely that rice used in the Gangetic Mesolithic period would not have led to much change and that, when it was traded into the Indus region, it would have been in a wild or semi-wild state. As the spikelet bases at the north-east Indus sites attest, it is after this introduction to new agricultural conditions that we see an increase in non-shattering traits appearing. Then, in 2000 BC, either we have incipient domestication reach its zenith or, as Silva et al. (2018) argue, we need to move the japonica arrival back by 500 years and assume that this is when the sh4 genes were introduced.
One thing that might also be noted is that the watering regimes in the Indus agricultural system might be considered somewhat similar to those in the Ganges ecological setting to which rice was accustomed. Weed ecological data show that rice in the Indus was grown not under intensive watering conditions but as semi-wet, semi-dry, or in marginal environments (Bates et al., 2017a). The presence of both water-loving and drought-tolerant weeds and those that have no particular preference suggests that rice was not grown under a wet (>1,000 mm) system but more of a dry system (<800 mm) (refer to Kingwell-Banham, 2019), perhaps dry groundwater-fed and reliant on seasonal flooding with some kind of water management to ensure that rice reached its required needs (with rice being more water greedy than other cereals). However, this was certainly not the paddy systems that have today come to dominate (Bates et al., 2017a; refer also to Fuller and Qin, 2009). What did change when rice was used in the Indus, however, from the cultivation systems that rice would have been handled under in the Gangetic Mesolithic-Neolithic was more intensive management and sowing of it on a regular basis in specific seasonal rhythms timed to farmers’ needs and not plants’ ecological cycles. Farmers would have been growing in their own tempo, somewhat guided by the plant and the environment but certainly at a more regulated and regimented pace, and making decisions based on their own understanding and experience of already domesticated cereals like wheat, barley, and millets. They would have been familiar with non-shattering cereals, with selection for specific aspects of a crop they preferred, and while much of this would have still been an unconscious selection bias, it would have led to a faster pace of change.
Another aspect that we might also note as being of interest is that this would have remained a relatively low-yielding crop (Fuller, 2020), as the nivara or the proto-indica in the Indus was grown under a dry farming system. Fuller (2020, p. 97) has estimated that, with dry rice, one needs roughly 17 ha to feed 50 people. Furthermore, he has argued that, for a village of 3–4 ha, one would need to set aside 53–71 ha for rice if it is the sole staple (Fuller, 2020, p. 97). This might explain why we never see rice as the sole Indus staple crop at Masudpur VII, Masudpur I, and Bahola (all small sites roughly 1–6 ha), while an important staple rice is not the only crop being used and rather was found alongside wheat, barley, and millets all as important staple foods (Bates et al., 2017a; Petrie and Bates, 2017). Dry rice is, however, less labor-intensive than wet rice (Fuller, 2020), and Fuller (2020) argues that this might have resulted in different trajectories in the agricultural strategies for dry and wet rice economies over time. Land-limited and labor-limited systems result from different rice watering systems, and a parallel might be drawn with Northeast Thailand for Indus rice systems and contrasted with China (refer to Fuller, 2020; refer also to Castillo, 2011; Castillo et al., 2018). The less intensive and geographically expansive systems of dry rice are more suited to smaller villages than the intensive smaller units of wet paddy rice that are labor-demanding but can support larger populations (Fuller, 2020). This might explain why under the Indus regime rice remained a village staple and an urban exotic; it was still a dry cropped, labor-limited system that was more suited for village economies than for urban sustenance (this links back to Madella, 2014).
Beyond the nuances of Indus rice economies, the data we have do suggest that the Indus archeobotanical evidence has both filled a gap in the time between the first use of rice in the 7th millennium BC and the hypothesized arrival of japonica in c.1500 BC. However, as this study also asked, what data do we have available to think about early South Asian rice use, and do the different proxies used to think about rice domestication create different narratives on rice domestication? Why are these narratives sometimes at odds with one another, and what additional data are needed to fill the gaps in our existing datasets and bring the narratives into consensus with one another? We can note from this review that there remain several gaps in the datasets, and that the types of proxy chosen can steer the narratives through their variable time frames (e.g., genetic data having less certain timeframes compared with the more specific archeobotanical data) and availability (e.g., genetic data are more readily available as modern samples can be accessed, while archeobotanical data have a patchy record in South Asia). Some of the gaps have been partially filled with the data on the Indus from c.3200–1500 BC, which address not only a chronological gap but also raise new questions that need to be addressed by more archeobotanical and genetic analyses:
- How much rice was available in the Gangetic Mesolithic (and later Neolithic) and at what specific dates? By archeobotanical analysis, we need both more and securely dated samples from the time frame between the 7th millennium and the 3rd millennium in the Ganges region to support the data we already have for early Ganges rice use and to see how this developed over time.
- What was the domesticated status of early rice? Alongside dated materials and genetic studies, we need fine-mesh screening to look for more spikelet bases to support the discussions on rice domestication in this period and look at rates of change in the non-shattering status over time.
- How was early rice used in the Ganges? We need both weeds and phytoliths to think about the cultivation strategies of Mesolithic-Neolithic rice use and consider how this might have influenced rice domestication pathways.
These three questions remain a priority for Gangetic rice studies and can be expanded to the Indus. We might ask, how does rice move from the Ganges to the Indus and exactly when? We need data from pre-Indus sites in the Indus region, more Indus sites with rice and spikelet bases, and sites in the region between the Indus and Gangetic regions to link them up. We should also ask how and when does japonica reach South Asia; more and securely dated evidence is needed on possible routes for japonica moving from China to South Asia to help with this, and more genetic information on nivara is needed to bolster the story. There have been some interesting discussions of aDNA (Castillo et al., 2015), and this could be expanded onto nivara/(proto-)indica should preservation be good enough.
This is an ambitious program of study, but one that builds on the foundations established from the many references included throughout this article. It builds on the two framing questions of this study as well: what was the impact of rice being moved around the variable ecologies and cultivation/agricultural settings of South Asia, and what are the impacts of filling in the data gaps on our narratives? Exploring how the proto-indica story developed and why dry rice that clearly supported villages and towns in a complex civilization like the Indus but is no longer used extensively today in favor of the labor and water-intensive paddy rice is important in light of some of the concerns related to Anthropocene impacts on the plant and global food sustainability. Methane outputs and other anthropogenic gasses (Anselman and Crutzen, 1989; Neue, 1993; Ruddiman and Thompson, 2001; Ruddiman et al., 2008; Li et al., 2009; Fuller, 2011; Fuller and Weisskopf, 2011; Fuller et al., 2011) are increased by the focus on paddy, and have, over time, potentially added to our impact on climate change (Fuller, 2011; Fuller and Weisskopf, 2011; Fuller et al., 2011). Watering strategies are also potentially affecting the health of rice consumers (e.g., flooded rice paddies may be increasing arsenic content in grains; Fao NewsRoom 2007, 2018).
While paddy systems can produce high rice yields that can be exported en masse and are critical to food supply chains and should not be abandoned, they should not be considered the only system that can be used to produce food in all circumstances. More diverse strategies need to be implemented, thinking back to local food strategies that are more sustainable for the local environment in which they are set. Such an approach diversifies the agricultural system in the face of food sustainability, anthropogenic impacts on the land and climate, and in terms of species diversity (both rice types but also their companion weeds and accompanying fauna). We are seeing this with calls for crop diversification away from paddy, i.e., by Anantha et al. (2021) and Bhogal and Vatta (2021) [although refer to Sinha (2022) on the political role of paddy and recent concerns about global supply chains]. Such calls do not necessarily have to mean the end of rice or of paddy (and cannot give the concerns over global food supply maintenance) but perhaps a change toward the inclusion of other ancient ways of growing or even inclusion of older types of rice (e.g., Green et al., 2020).
Post-Script: Changing Water and Later Agricultural Strategies
While the debate about early rice has been fraught and remains patchy, there is a post-script to be added: What happens after the Indus, after the period when japonica has been introduced, and can we can that we have fully domesticated indica with Chinese wetland rice genes present? How does this affect both agricultural production and land use?, a question that links back to the final point on land use and urban food supply. The role of watering and rice has been central to many discussions of historical period archaeological debates as well as prehistoric ones, as seen in studies by Morrison (1994, 2016), Risberg et al. (2002); Sinopoli et al. (2010), Gilliland et al. (2013), and Murphy et al. (2018), among many others.
Coningham (1995, pp. 66–67) hypothesized that the post-Indus period saw changes in the methods of growing crops, particularly rice, with a shift from dry to wet-land rice. Such a change would have big impacts on harvest yields and carrying capacities (Fuller, 2020). Fuller (2020) has used ethnographic and historic data to estimate the carrying capacities of different rice systems, which are roughly divided into wet and dry. With examples from pre-industrial Borneo, India, and Sumatra showing dry rice systems with yields ranging from 229–1,500 kg/ha compared with Han Dynasty China and 19th century Japan and Southeast Asia wet rice having yields of 1,000–2,000 kg/ha (Heston, 1973; Bray, 1986; Sherman, 1990; Ellis and Wang, 1997; Barton, 2012; Qin and Fuller, 2019), Fuller (2020) notes that there is already a disparity in how much food can be produced across wet and dry rice systems. Combining this, it can be argued that, under pre-industrial systems, wet rice could support up to 14,000 people and that dry rice could support, at most, 2,500–3,000 people (the carrying capacity) (Fuller, 2020). With greater yields (kg per ha) and carrying capacity, it has therefore been assumed that the arrival of japonica and accompanying wet systems would lead to the ability to support even larger urban centers with rice as the staple crop (Coningham, 1995, pp. 66–67) (this too can be debated; we have little evidence that rice was the initial staple crop, and what the role of wheat and barley was in the Early Historic periods).
This is again based on the ecological differences for rice and its growing preferences. Coningham’s (1995) argument assumed that when japonica arrived and hybridized with the (proto-)indica in the region, this would have brought with it not only the water-stress response genes (Fuller and Qin, 2009; Fuller, 2011; Weisskopf et al., 2014, 2015) but also a necessity to use irrigated paddy fields. Furthermore, Coningham (1995) assumed that the necessity of using a new agricultural system would, in turn, lead to higher yields and, thus, to greater food supplies and surplus, and it was this that drove secondary (and larger) urbanization. However, Fuller and Qin (2009) have noted that, while japonica and (proto-)indica do have different life cycles, they are also both species of rice, and all types of rice have higher water requirements than other cereals and generally prefer wet conditions overall and can also exploit a range of conditions; there is no prerequisite for one particular type of rice having to be grown in paddy, for example (refer to Kingwell-Banham, 2019). Countering Coningham (1995), they suggested that, when hybridization happened, it did not automatically lead to wetland irrigated paddy rice, and japonica’s arrival did not necessarily bring with it wet rice systems, but that people could still retain a range of options on how to grow rice, from wet to dry (Fuller and Qin, 2009), and one only has to look to diversity in South Asia today to see this happening. They note, though, that this diversity is mostly supplanted by paddy today, but it is still practiced in some areas, and it is important to note that paddy did not completely replace it.
Furthermore, Fuller and Qin (2009) went on to demonstrate this by looking at the weed data, looking at whether the conditions of the rice agriculture shifted from dry to wet. No pattern of the shift from dry rice to wet rice systems was seen, and more mixed agricultural watering systems were noted (Fuller and Qin, 2009). This seems to be a continuation of the patterns seen in the Indus period that preceded it; neither a predominantly wetland nor a predominantly dryland cultivation practice was carried out across any one phase of the Indus and into the post-Indus Painted Grey Ware period at the sites where rice assemblages were analyzed (Bates et al., 2017a).
Therefore, contrary to the hypothesized sudden impact of japonica-indica hybridization and for a paddy-induced secondary urbanization of South Asia, it seems that rice continued to be exploited in either marginal wet-dry environments or in a mix of wet and dry cultivation systems, as noted in Fuller and Qin (2009) and Weisskopf et al. (2014, 2015) and as further illustrated in Bates et al. (2017a).
Rice, it seems, is a crop that continues to confound and provide patterns of data that show the diversity of ways it can be used. The nuanced picture of early rice is one that will continue to unfold as more data become available. The post-1500 BC narrative of rice use and the role of irrigation and the water management story link back to the discussions of water and agricultural sustainability in future rice systems. This diversity, while seen in South Asia today, is gradually being lost to more intensive paddy systems as the demand for rice yields increases. More studies on how early rice was grown and how it was watered and used to maintain urban systems as part of diverse crop packages will perhaps provide one possible solution to the growing food and water crises we see developing globally and within South Asia in recent times.
Author Contributions
The author confirms being the sole contributor of this work and has approved it for publication.
Funding
This research was supported by the Research Grants for Asian Studies funded by Seoul National University Asia Center (SNUAC) in 2022. The project is entitled “Meso-Rice: testing the early rice domestication hypothesis in the Ganges Mesolithic and Neolithic” and runs from 2021 to 2022.
Conflict of Interest
The author declares that the research was conducted in the absence of any commercial or financial relationships that could be construed as a potential conflict of interest.
Publisher’s Note
All claims expressed in this article are solely those of the authors and do not necessarily represent those of their affiliated organizations, or those of the publisher, the editors and the reviewers. Any product that may be evaluated in this article, or claim that may be made by its manufacturer, is not guaranteed or endorsed by the publisher.
Acknowledgments
The study was completed at Seoul National University, Department of Archaeology and Art History where the author is an Assistant Professor of Archaeological Science. She is grateful for the support shown to her by the department. Figure 2 has been reprinted following a Creative Commons Attribution 4.0 International license (http://creativecommons.org/licenses/by/4.0/), and has been reprinted following the study of Kingwell-Banham (2019), Dry, rainfed or irrigated? Reevaluating the role and development of rice agriculture in Iron Age-Early Historic South India using archaeobotanical approaches. Archaeological and Anthropological Sciences. https://doi.org/10.1007/s12520-019-00795-7. Figure 3 has been reprinted with permission from Springer Nature Customer Service Centre GmbH (the Licensor): Springer Nature, Archaeological and Anthropological Sciences. Consilience of genetics and archeobotany in the entangled history of rice, Fuller et al. (2010). Article hyperlink: doi: 10.1007/s12520-010-0035-y (Journal homepage hyperlink: https://www.springer.com/journal/12520). Figure 4 has been reprinted following a Creative Commons Attribution 4.0 International license (http://creativecommons.org/licenses/by/4.0/), and has been reprinted following the study of Bates et al. (2017a), Approaching rice domestication in South Asia: New evidence from Indus settlements in northern India. Journal of Archaeological Science 78, 193–201. https://doi.org/10.1016/j.jas.2016.04.018.
Footnotes
- ^ The nivara, indica, rufipogon, japonica, and aus “types” of rice are not included as species or subspecies, although they are referred to as such variably in text depending on the authors. The decision to simply leave them as “type” without a Latin binomial is based on The Plant List, which suggests that these are synonyms of O. sativa, i.e., O. indica, or O. sativa subsp. Indica, are listed as synonyms for O. sativa.
- ^ Premathilake (2006) has argued that the first possible evidence for cultivated rice was seen in Sri Lanka much earlier than c.13 kya. This is based on microcharcoal evidence that is interpreted as showing clearance activities and pollen showing “rice.” Pollen data are notoriously difficult for identifying to genus or species, and Poaceae is particularly difficult to get to such levels. This has been taken to even further extremes to suggest “agriculture” of rice, and at 11 kya oats and barley, on the basis of pollen and microcharcoal (Premathilake and Risberg, 2003; Premathilake, 2006; Premathilake and Hunt, 2017) and the contested nature of this evidence, questions on whether pollen can indeed be identified at the genus level, let alone the species level (domesticated rice for example), have led to this study setting aside this debate for the sake of clarity. It might be better to argue that the Sri Lankan data show perhaps low-level food production, maybe akin to that in the Gangetic region where rice was also used at an early date, but until other proxies are also found, it is not going to be discussed further here.
- ^ http://archaeobotanist.blogspot.com/2009/06/indian-archaeobotany-watch-lahuradewa.html
- ^ Cultivar is a term referring to a plant produced in cultivation, bred specifically for traits, for example through careful seed control. Cultivars can also occur in the wild, but more commonly result from human manipulation. Not all cultivated plants are cultivars. The ICNCP defines “A cultivar is an assemblage of plants that (a) has been selected for a particular character or combination of characters, (b) is distinct, uniform and stable in those characters, and (c) when propagated by appropriate means, retains those characters” (ICNCP 2009).
References
Agrawal, D. P., and Kusumgar, S. (1975). Tata institute radiocarbon date list XI. Radiocarbon 17, 219–225.
Ahmad, H. I., Ahmad, M. J., Jabbir, F., Ahmar, S., Ahmad, N., Elokil, A. A., et al. (2020). The domestication makeup: evolution, survival, and challenges. Front. Ecol. Evol. 8:103. doi: 10.3389/fevo.2020.00103
Allaby, R. G., Stevens, C., Lucas, L., Maeda, O., and Fuller, D. Q. (2017). Geographic mosaics and changing rates of cereal domestication. Philos. Trans. R. Soc. B 372:20160429. doi: 10.1098/rstb.2016.0429
Anantha, K. H., Garg, K. K., Petrie, C. A., and Dixit, S. (2021). Seeking sustainable pathways for fostering agricultural transformation in peninsular India. Environ. Res. Lett. 16:044032. doi: 10.1088/1748-9326/abed7b
Anselman, I., and Crutzen, P. J. (1989). Global distribution of natural freshwater wetlands and rice paddies, their net primary productivity, seasonality and possible methane emissions. J. Atmospheric Chem. 8, 307–358.
Ball, T., Chandler-Ezell, K., Dickau, R., Duncan, N., Hart, T. C., Iriarte, J., et al. (2016). Phytoliths as a tool for investigations of agricultural origins and dispersals around the world. J. Archaeol. Sci. 68, 32–45. doi: 10.1016/j.jas.2015.08.010
Barton, H. (2012). The reversed fortunes of sago and rice, Oryza sativa, in the rainforests of Sarawak, Borneo. Quat. Int. 249, 96–104. doi: 10.1016/j.quaint.2011.03.037
Bates, J. (2019a). The published archaeobotanical data from the indus civilisation, South Asia, c.3200–1500BC. J. Open Archaeol. Data 7:5. doi: 10.5334/joad.57
Bates, J. (2019b). Oilseeds, spices, fruits and flavour in the indus civilisation. J. Archaeol. Sci. Rep. 24, 879–887. doi: 10.1016/j.jasrep.2019.02.033
Bates, J. (2020). Kitchen gardens, wild forage and tree fruits: a hypothesis on the role of the Zaid season in the indus civilisation (c.3200-1300 BCE). Archaeol. Res. Asia 21:100175. doi: 10.1016/j.ara.2019.100175
Bates, J., Petrie, C. A., and Singh, R. N. (2017a). Approaching rice domestication in South Asia: new evidence from Indus settlements in northern India. J. Archaeol. Sci. 78, 193–201.
Bates, J., Petrie, C. A., and Singh, R. N. (2017b). Cereals, calories and change: exploring approaches to quantification in Indus archaeobotany. Archaeol. Anthropol. Sci. 10, 1703–1716. doi: 10.1007/s12520-017-0489-2
Bates, J., Singh, R. N., and Petrie, C. A. (2017c). Exploring Indus crop processing: combining phytolith and macrobotanical analyses to consider the organisation of agriculture in northwest India c. 3200–1500 bc. Veg. Hist. Archaeobotany 26, 25–41. doi: 10.1007/s00334-016-0576-9
Bates, J., Petrie, C. A., Ballantyne, R., Lancelotti, C., Saraswat, K. S., Pathak, A., et al. (2021). Cereal grains and grain pulses: reassessing the archaeobotany of the indus civilisation and painted grey ware period occupation at alamgirpur district meerut U.P. Indian J. Archaeol. 6, 495–522.
Beddows, P. A., Glover, J. B., Rissolo, D., Carter, A. M., Jaijel, R., Smith, D. M., et al. (2016). the proyecto costa escondida: recent interdisciplinary research in search of freshwater along the north coast of quintana roo, Mexico. Wiley Interdiscip. Rev. Water 3, 749–761.
Bhogal, S., and Vatta, K. (2021). Can crop diversification be widely adopted to solve the water crisis in Punjab? Curr. Sci. 120:1303. doi: 10.18520/cs/v120/i8/1303-1307
Bray, F. (1986). The Rice Economies: Technology and development in Asian societies. Oxford: Blackwell.
Cai, H., and Morishima, H. (2002). QTL clusters reflect character associations in wild and cultivated rice. Theor. Appl. Genet. 104, 1217–1228. doi: 10.1007/s00122-001-0819-7
Castillo, C. (2011). Rice in Thailand: the archaeobotanical contribution. Rice 4, 114–120. doi: 10.1007/s12284-011-9070-2
Castillo, C. C., Higham, C. F. W., Miller, K., Chang, N., Douka, K., Higham, T. F. G., et al. (2018). Social responses to climate change in Iron Age north-east Thailand: new archaeobotanical evidence. Antiquity 92, 1274–1291. doi: 10.15184/aqy.2018.198
Castillo, C. C., Tanaka, K., Sato, Y.-I., Ishikawa, R., Bellina, B., Higham, C., et al. (2015). Archaeogenetic study of prehistoric rice remains from Thailand and India: evidence of early japonica in South and Southeast Asia. Archaeol. Anthropol. Sci. 8, 523–543. doi: 10.1007/s12520-015-0236-5
Chakraborty, K. S., Slater, G. F., Miller, H. M.-L., Shirvalkar, P., and Rawat, Y. (2020). Compound specific isotope analysis of lipid residues provides the earliest direct evidence of dairy product processing in South Asia. Sci. Rep. 10:16095. doi: 10.1038/s41598-020-72963-y
Chang, T.-T. (1976). The origin, evolution, cultivation, dissemination, and diversification of Asian and African rices. Euphytica 25, 425–441. doi: 10.1007/BF00041576
Chattopadaya, U. C. (2002). “Researches in archaeozoology of the Holocene period (Including the Harappan tradition in India and Pakistan),” in Indian Archaeology in Retrospect. Archaeology and Interactive Disciplines, eds S. Settar and R. Korisettar (New Delhi: Manohar).
Choi, J. Y., Platts, A. E., Fuller, D. Q., Hsing, Y.-I., Wing, R. A., and Purugganan, M. D. (2017). The rice paradox: multiple origins but single domestication in Asian rice. Mol. Biol. Evol. 34, 969–979. doi: 10.1093/molbev/msx049
Civáň, P., Craig, H., Cox, C. J., and Brown, T. A. (2015). Three geographically separate domestications of Asian rice. Nat. Plants 1:15164. doi: 10.1038/nplants.2015.164
Coningham, R. A. E. (1995). “Dark age or continuum? an archaeological analysis of the second emergence of urbanism in South Asia,” in The Archaeology of Early Historic South Asia, ed. F. R. Allchin (Cambridge: Cambridge University Press).
Crawford, G. W. (2011). Advances in understanding early agriculture in Japan. Curr. Anthropol. 52, S331–S345. doi: 10.1086/658369
Crawford, G. W., Chen, X., Luan, F., and Wang, J. (2016). People and plant interaction at the Houli Culture Yuezhuang site in Shandong Province. China. Holocene 26, 1594–1604. doi: 10.1177/0959683616650269
Deng, Z., Qin, L., Gao, Y., Weisskopf, A. R., Zhang, C., and Fuller, D. Q. (2015). From early domesticated rice of the middle yangtze basin to millet, rice and wheat agriculture: archaeobotanical macro-remains from Baligang, Nanyang Basin, Central China (6700–500 BC). PLoS One 10:e0139885. doi: 10.1371/journal.pone.0139885
Ellis, E. C., and Wang, S. M. (1997). Sustainable traditional agriculture in the Tai Lake Region of China. Agric. Ecosyst. Environ. 61, 177–193. doi: 10.1016/S0167-8809(96)01099-7
Fujiwara, H. (1993). “Research into the history of rice cultivation using plant opal analysis,” in Current Research in Phytolith Analysis: Applications in Archaeology and Palaeoecology, eds D. M. Pearsall and D. R. Piperno (Philadelphia, PA: University of Pennsylvania Museum).
Fujiwara, H., Mughal, M. R., Sasaki, A., and Matano, T. (1992). Rice and Ragi at Harappa: preliminary results by plant opal analysis. Pak. Archaeol. 27, 129–142.
Fuller, D. Q. (2002). “Fifty years of archaeobotanical studies in india: laying a solid foundation,” in Indian Archaeology in Retrospect III: Archaeology and Interactive Disciplines, eds S. Settar and R. Korisettar (New Delhi.: Manohar).
Fuller, D. Q. (2011). Pathways to asian civilizations: tracing the origins and spread of rice and rice cultures. Rice 4, 78–92. doi: 10.1007/s12284-011-9078-7
Fuller, D. Q. (2020). Transitions in productivity: rice intensification from domestication to urbanisation. Archaeol. Int. 23, 88–103. doi: 10.14324/111.444.ai.2020.08
Fuller, D. Q., and Hildebrand, E. (2013). “Domesticating plants in Africa,” in The Oxford Handbook of African Archaeology, eds P. Mitchell and P. Land (Oxford: Oxford University Press).
Fuller, D. Q., and Madella, M. (2002). “Issues in harappan archaeobotany: retrospect and prospect,” in Indian Archaeology in Retrospect II: Protohistory, eds S. Settar and R. Korisettar (New Delhi: Manohar).
Fuller, D. Q., and Murphy, C. (2014). Overlooked but not forgotten: india as a center for agricultural domestication. Gen. Anthropol. 21, 1–8. doi: 10.1111/gena.01001
Fuller, D. Q., Sato, Y.-I., Castillo, C., Qin, L., Weisskopf, A., Kingwell-Banham, E., et al. (2010). Consilience of genetics and archaeobotany in the entangled history of rice. Archaeol. Anthropol. Sci. 2, 115–131. doi: 10.1007/s12520-010-0035-y
Fuller, D. Q., and Qin, L. (2008). Immature rice and its archaeobotanical recognition: a reply to pan. Antiq. Proj. Gallery 82.
Fuller, D. Q., and Qin, L. (2009). Water management and labour in the origins and dispersal of Asian rice. World Archaeol. 41, 88–111. doi: 10.1080/00438240802668321
Fuller, D. Q., and Weisskopf, A. (2011). The early rice project: from domestication to global warming. Archaeol. Int. 13, 44–51. doi: 10.5334/ai.1314
Fuller, D. Q., Denham, T., Arroyo-Kalin, M., Lucas, L., Stevens, C. J., Qin, L., et al. (2014). Convergent evolution and parallelism in plant domestication revealed by an expanding archaeological record. Proc. Natl. Acad. Sci. U S A. 111, 6147–6152. doi: 10.1073/pnas.1308937110
Fuller, D. Q., Qin, L., Zheng, Y., Zhao, Z., Chen, X., Hosoya, L. A., et al. (2009). The domestication process and domestication rate in rice: spikelet bases from the lower yangtze. Science 323, 1607–1610. doi: 10.1126/science.1166605
Fuller, D. Q., van Etten, J., Manning, K., Castillo, C., Kingwell-Banham, E., Weisskopf, A., et al. (2011). The contribution of rice agriculture and livestock pastoralism to prehistoric methane levels: an archaeological assessment. Holocene 21, 743–759. doi: 10.1177/0959683611398052
Gao, L. (2003). The conservation of Chinese rice biodiversity: genetic erosion, ethnobotany and prospects. Genet. Resour. Crop Evol. 50, 17–32. doi: 10.1023/A:1022933230689
García-Granero, J. J., Gadekar, C. S., Esteban, I., Lancelotti, C., Madella, M., and Ajithprasad, P. (2017). What is on the craftsmen’s menu? plant consumption at Datrana, a 5000-year-old lithic blade workshop in North Gujarat, India. Archaeol. Anthropol. Sci. 9, 251–263.
García-Granero, J. J., Lancelotti, C., and Madella, M. (2015). A tale of multi-proxies: integrating macro- and microbotanical remains to understand subsistence strategies. Veg. Hist. Archaeobotany 24, 121–133. doi: 10.1007/s00334-014-0486-7
García-Granero, J. J., Lancelotti, C., Madella, M., and Ajithprasad, P. (2016). Millets and Herders: the origins of plant cultivation in semiarid North Gujarat (India). Curr. Anthropol. 57, 149–173.
García-Granero, J. J., Suryanarayan, A., Cubas, M., Craig, O. E., Cárdenas, M., Ajithprasad, P., et al. (2022). Integrating lipid and starch grain analyses from pottery vessels to explore prehistoric foodways in Northern Gujarat, India. Front. Ecol. Evol. 10:840199. doi: 10.3389/fevo.2022.840199
Garris, A. J., Tai, T. H., Coburn, J., Kresovich, S., and McCouch, S. (2005). Genetic structure and diversity in Oryza sativa L. Genetics 169, 1631–1638. doi: 10.1534/genetics.104.035642
Ge, Y., Jie, D., Guo, J., Liu, H., and Shi, L. (2010). Response of phytoliths in Leymus chinensis to the simulation of elevated global CO2 concentrations in Songnen Grassland. China. Chin. Sci. Bull. 55, 3703–3708. doi: 10.1007/s11434-010-4123-2
Ghosh, S. S., and Lal, K. (1963). Plant remains from rangpur and other explorations in Gujarat. Anc. India 1, 161–175.
Gilliland, K., Simpson, I. A., Adderley, W. P., Burbidge, C. I., Cresswell, A. J., Sanderson, D. C. W., et al. (2013). The dry tank: development and disuse of water management infrastructure in the Anuradhapura hinterland, Sri Lanka. J. Archaeol. Sci. 40, 1012–1028. doi: 10.1016/j.jas.2012.09.034
Green, A. S., Dixit, S., Garg, K. K., Sandya, N., Singh, G., Vatta, K., et al. (2020). An interdisciplinary framework for using archaeology, history and collective action to enhance India’s agricultural resilience and sustainability. Environ. Res. Lett. 15:105021. doi: 10.1088/1748-9326/aba780
Gross, B. L., and Zhao, Z. (2014). Archaeological and genetic insights into the origins of domesticated rice. Proc. Natl. Acad. Sci. U.S.A. 111, 6190–6197. doi: 10.1073/pnas.1308942110
Gu, Y., Zhao, Z., and Pearsall, D. M. (2013). Phytolith morphology research on wild and domesticated rice species in East Asia. Quat. Int. 287, 141–148. doi: 10.1016/j.quaint.2012.02.013
Gutaker, R. M., Groen, S. C., Bellis, E. S., Choi, J. Y., Pires, I. S., Bocinsky, R. K., et al. (2020). Genomic history and ecology of the geographic spread of rice. Nat. Plants 6, 492–502. doi: 10.1038/s41477-020-0659-6
Harris, D. R. (2007). “Agriculture, cultivation and domestication: exploring the conceptual framework of early food production,” in Rethinking Agriculture: Archaeological and Ethnoarchaeological Perspectives, eds T. Denham, J. Iriarte, and L. Vrydaghs (Walnut Creek, CA: Left Coast).
Harvey, E. L. (2006). Early Agricultural Communities in Northern and Eastern India: an archaeobotanical investigation. Ph D thesis, London: UCL.
Harvey, E. L., and Fuller, D. Q. (2005). Investigating crop processing using phytolith analysis: the example of rice and millets. J. Archaeol. Sci. 32, 739–752. doi: 10.1016/j.jas.2004.12.010
Harvey, E. L., Fuller, D. Q., Pal, J. N., and Gupta, M. C. (2003). “Early agriculture of the Neolithic Vindhyas (North Central India),” in South Asian Archaeology 2003 eds U. Franke-Vogt and H.-J. Weisshaar, Deutsches Archaeologishes Institut: Achen.
He, Z., Zhai, W., Wen, H., Tang, T., Wang, Y., Lu, X., et al. (2011). Two evolutionary histories in the genome of rice: the roles of domestication genes. PLoS Genet. 7:e1002100. doi: 10.1371/journal.pgen.1002100
Heston, A. W. (1973). Official yields per acre in India, 1886-1947; some questions of interpretation. Indian Econ. Soc. Hist. Rev. 10, 303–332. doi: 10.1177/001946467301000401
Huang, X., Kurata, N., Wei, X., Wang, Z.-X., Wang, A., Zhao, Q., et al. (2012). A map of rice genome variation reveals the origin of cultivated rice. Nature 490, 497–501. doi: 10.1038/nature11532
Issaharou-Matchi, I., Barboni, D., Meunier, J.-D., Saadou, M., Dussouillez, P., Contoux, C., et al. (2016). Intraspecific biogenic silica variations in the grass species Pennisetum pedicellatum along an evapotranspiration gradient in South Niger. Flora - Morphol. Distrib. Funct. Ecol. Plants 220, 84–93. doi: 10.1016/j.flora.2016.02.008
Jin, J., Huang, W., Gao, J.-P., Yang, J., Shi, M., Zhu, M.-Z., et al. (2008). Genetic control of rice plant architecture under domestication. Nat. Genet. 40, 1365–1369. doi: 10.1038/ng.247
Joglekar, P. P., Misra, V. D., Pal, J. N., and Gupta, M. C. (2003). Mesolithic Mahadaha: The Faunal Remains. Allahbad: University of Allahabad.
Kahlheber, S., and Neumann, K. (2007). “The development of plant cultivation in semi-arid West Africa,” in Rethinking Agriculture: Archaeological and Ethnoarchaeological Perspectives, eds T. P. Denham, J. Iriarte, and L. Vrydaghs (Walnut Creek: Left Coast Press).
Kajale, M. D. (1990). “Some Initial Observations on Palaeobotanical Evidence for Mesolithic Plant Economy from Excavations at Damdama, Pratapgarh, Uttar Pradesh,” in Adaptation and Other Essays, eds N. C. Ghosh and S. Chakrabarti (Santiniketan: Viswa Bharti).
Kashyap, A., and Weber, S. A. (2010). Harappan plant use revealed by starch grains from Farmana, India. Antiq. Proj. Gallery 84:326.
Kashyap, A., and Weber, S. A. (2013). “Starch grain analysis and experiments provide insights into harappan cooking practices,” in Connections and Complexity, New Approaches to the Archaeology of South Asia, eds S. A. Abraham, P. Gullapalli, T. P. Raczek, and U. Z. Rizvi (London: Routledge, Taylor & Francis Group).
Kashyap, A., and Weber, S. A. (2016). “Preliminary analysis of starch from cattle tooth at Farmana: implications for Indus ethnobotany,” in The Harappa Archaeological Research Project 2001-2007: Documentation, Analysis and New Excavations, ed. R. P. Wright (Baltimore, MD: National Agricultural Library).
Kendal, J., Tehrani, J. J., and Odling-Smee, J. (2011). Human niche construction in interdisciplinary focus. Philos. Trans. R. Soc. B Biol. Sci. 366, 785–792. doi: 10.1098/rstb.2010.0306
Kingwell-Banham, E. (2019). Dry, rainfed or irrigated? Reevaluating the role and development of rice agriculture in Iron Age-Early Historic South India using archaeobotanical approaches. Archaeol. Anthropol. Sci. 11, 6485–6500. doi: 10.1007/s12520-019-00795-7
Kingwell-Banham, E., Harvey, E. K., Mohanty, R. K., and Fuller, D. Q. (2018). Archaeobotanical investigations into golbai sasan and gopalpur, two neolithic-chalcolithic settlements of Odisha. Anc. Asia 9:5. doi: 10.5334/aa.164
Kingwell-Banham, E., Petrie, C. A., and Fuller, D. Q. (2015). “Early agriculture in South Asia,” in The Cambridge World History, eds G. Barker and C. Goucher (Cambridge: Cambridge University Press).
Konishi, S., Izawa, T., Lin, S. Y., Ebana, K., Fukuta, Y., Sasaki, T., et al. (2006). An SNP caused loss of seed shattering during rice domestication. Science 312, 1392–1396. doi: 10.1126/science.1126410
Kovach, M. J., Sweeney, M. T., and McCouch, S. R. (2007). New insights into the history of rice domestication. Trends Genet. 23, 578–587. doi: 10.1016/j.tig.2007.08.012
Laland, K., Matthews, B., and Feldman, M. W. (2016). An introduction to niche construction theory. Evol. Ecol. 30, 191–202. doi: 10.1007/s10682-016-9821-z
Larson, G., Piperno, D. R., Allaby, R. G., Purugganan, M. D., Andersson, L., Arroyo-Kalin, M., et al. (2014). Current perspectives and the future of domestication studies. Proc. Natl. Acad. Sci. U S A. 111, 6139–6146. doi: 10.1073/pnas.1323964111
Li, C., Zhou, A., and Sang, T. (2006a). Genetic analysis of rice domestication syndrome with the wild annual species, Oryza nivara. New Phytol. 170, 185–194. doi: 10.1111/j.1469-8137.2005.01647.x
Li, C., Zhou, A., and Sang, T. (2006b). Rice domestication by reducing shattering. Science 311, 1936–1939. doi: 10.1126/science.1123604
Li, X., Dodson, J., Zhou, J., and Zhou, X. (2009). Increases of population and expansion of rice agriculture in asia, and anthropogenic methane emissions since 5000 BP. Quat. Int. 202, 41–50.
Lin, Z., Griffith, M. E., Li, X., Zhu, Z., Tan, L., Fu, Y., et al. (2007). Origin of seed shattering in rice (Oryza sativa L.). Planta 226, 11–20. doi: 10.1007/s00425-006-0460-4
Londo, J. P., Chiang, Y.-C., Hung, K.-H., Chiang, T.-Y., and Schaal, B. A. (2006). Phylogeography of Asian wild rice, Oryza rufipogon, reveals multiple independent domestications of cultivated rice, Oryza sativa. Proc. Natl. Acad. Sci. U S A. 103, 9578–9583. doi: 10.1073/pnas.0603152103
Lone, F. A., Khan, M., and Buth, G. M. (1987). Plant remains from Banawali, Haryana. Curr. Sci. 56, 837–838.
Lu, H., Liu, Z., Wu, N., Berné, S., Saito, Y., Liu, B., et al. (2008). Rice domestication and climatic change: phytolith evidence from East China. Boreas 31, 378–385. doi: 10.1111/j.1502-3885.2002.tb01081.x
Lukacs, J. R. (2016). “Mesolithic foragers of the ganges plain and adjoining hilly,” in A Companion to South Asia in the Past, Blackwell Companions to Anthropology, eds G. R. Schug, S. R. Walimbe, and K. A. R. Kennedy (Chichester: John Wiley & Sons).
Lukacs, J. R., and Pal, J. N. (1993). Mesolithic subsistence in North India: inferences from dental attributes. Curr. Anthropol. 34, 745–765. doi: 10.1086/204220
Ma, J., and Bennetzen, J. L. (2004). Rapid recent growth and divergence of rice nuclear genomes. Proc. Natl. Acad. Sci. U S A. 101, 12404–12410. doi: 10.1073/pnas.0403715101
Ma, Y., Yang, X., Huan, X., Wang, W., Ma, Z., Li, Z., et al. (2016). Rice bulliform phytoliths reveal the process of rice domestication in the Neolithic Lower Yangtze River region. Quat. Int. 426, 126–132. doi: 10.1016/j.quaint.2016.02.030
Madella, M. (1997). “Phytolith analysis from the indus valley site of Kot Diji, Sindh, Pakistan,” in Proceedings of the Archaeological Sciences 1995: Proceedings of a Conferene on the Application of Scientific Techniques to the Study of Archaeology, eds A. Sinclair, E. Slater, and J. Gowlett (Oxford: Oxbow Books).
Madella, M. (2003). “Investigating agriculture and environment in south Asia: present and future contributions of opal phytoliths,” in Indus Ethnobiology: New Persepctives from the Field, eds S. A. Weber and W. R. Belcher (Oxford: Lexington Books).
Madella, M. (2014). “Of crops and food, a social perspective on rice in the indus civilisation,” in Ancient Plants and People, Contemporary Trends in Archaeology, eds M. Madella, C. Lancelotti, and M. Savard (Tuscon: University of Arizona Press).
McNally, K. L., Childs, K. L., Bohnert, R., Davidson, R. M., Zhao, K., Ulat, V. J., et al. (2009). Genomewide SNP variation reveals relationships among landraces and modern varieties of rice. Proc. Natl. Acad. Sci. U S A. 106, 12273–12278. doi: 10.1073/pnas.0900992106
Molina, J., Sikora, M., Garud, N., Flowers, J. M., Rubinstein, S., Reynolds, A., et al. (2011). Molecular evidence for a single evolutionary origin of domesticated rice. Proc. Natl. Acad. Sci. U.S.A. 108, 8351–8356. doi: 10.1073/pnas.1104686108
Morrison, K. D. (1994). The intensification of production: archaeological approaches. J. Archaeol. Method Theory 1, 111–159. doi: 10.1007/BF02231414
Morrison, K. D. (2016). “From millets to rice (and Back Again?): cuisine, cultivation and health in early South India,” in A Companion to South Asia in the Past, eds G. Robbins Schug and S. R. Walimbe (New York, NY: Wiley Blackwell).
Motuzaite-Matuzeviciute, G., Staff, R. A., Hunt, H. V., Liu, X., and Jones, M. K. (2013). The early chronology of broomcorn millet Panicum miliaceum in Europe. Antiquity 87, 1073–1085. doi: 10.1017/S0003598X00049875
Murphy, C., Weisskopf, A., Bohingamuwa, W., Adikari, G., Perera, N., Blinkhorn, J., et al. (2018). Early agriculture in Sri Lanka: new Archaeobotanical analyses and radiocarbon dates from the early historic sites of Kirinda and Kantharodai (Kandarodai). Archaeol. Res. Asia 16, 88–102. doi: 10.1016/j.ara.2018.06.001
Naik, S. S., Patnaik, J. K., Kingwell-Banham, E., Murphy, C., and Fuller, D. Q. (2019). Archaeobotanical studies at Suabarei, Puri district, Odisha, India. Curr. Sci. 116:8.
Odling-Smee, J., Laland, K. N., and Feldman, M. W. (2003). Niche Construction: the Neglected Process in Evolution. Monographs in Population Biology 37. Princeton, NJ: Princeton University Press.
Ohtsubo, H., Cheng, C., Ohsawa, I., Tsuchimoto, S., and Ohtsubo, E. (2004). Rice retroposon p-SINE1 and origin of cultivated rice. Breed. Sci. 54, 1–11. doi: 10.1270/jsbbs.54.1
Pal, J. N. (2008). The early farming culture of the middle ganga plain with special reference to the excavations at Jhusi and Hetapatti. Pragdhara 18, 263–281.
Pal, J. N. (2016). “Mesolithic foragers of the ganges plain and adjoining hilly,” in A Companion to South Asia in the Past, Blackwell Companions to Anthropology, eds G. R. Schug, S. R. Walimbe, and K. A. R. Kennedy (Chichester: John Wiley & Sons).
Pandey, J. N. (1990). Mesolithic in the middle ganga valley. Bull. Deccan Coll. Res. Inst. 49, 311–316. doi: 10.1007/BF02704749
Parikh, D., and Petrie, C. A. (2019). ‘We are inheritors of a rural civilisation’: rural complexity and the ceramic economy in the Indus Civilisation in northwest India. World Archaeol. 51, 252–272. doi: 10.1080/00438243.2019.1601463
Pearsall, D. M., Piperno, D. R., Dinan, E. H., Umlauf, M., Zhao, Z., and Benfer, R. A. (1995). Distinguishing Rice (Oryza sativa Poaceae) from Wild Oryza Species through phytolith analysis: results of preliminary research. Econ. Bot. 49, 183–196.
Petrie, C. A. (2013). “Taxila,” in History of Ancient India III: The Texts and Political History and Administration till c.200 BC, eds D. K. Chakrabarti and M. Lal (Delhi: Vivekananda International Foundation and Ayran Books International).
Petrie, C. A., and Bates, J. (2017). Multi-cropping”, intercropping and adaptation to variable environments in indus South Asia. J. World Prehistory 30, 81–130. doi: 10.1007/s10963-017-9101-z
Petrie, C. A., Bates, J., Higham, T., and Singh, R. N. (2016). Feeding ancient cities in South Asia: dating the adoption of rice, millet and tropical pulses in the Indus civilisation. Antiquity 90, 1489–1504. doi: 10.15184/aqy.2016.210
Piperno, D. R. (2011). The origins of plant cultivation and domestication in the new world tropics: patterns, process, and new developments. Curr. Anthropol. 52, S453–S470. doi: 10.1086/659998
Pokharia, A. K. (2011). Palaeoethnobotany at lahuradewa: a contribution to the 2nd millennium BC agriculture of the Ganga Plain, India. Curr. Sci. 101, 1569–1578.
Pokharia, A. K., and Srivastava, C. (2013). Current status of archaeobotanical studies in harappan civilization: an archaeological perspective. Herit. J. Multidiscip. Stud. Archaeol. 1, 118–137.
Pokharia, A. K., Kharakwal, J. S., and Srivastava, A. (2014). Archaeobotanical evidence of millets in the Indian subcontinent with some observations on their role in the Indus civilization. J. Archaeol. Sci. 42, 442–455. doi: 10.1016/j.jas.2013.11.029
Pokharia, A. K., Kharakwal, J. S., Rawat, R. S., Osada, T., Nautiyal, C. M., and Srivastava, A. (2011). Archaeobotany and archaeology at Kanmer, a Harappan site in Kachchh, Gujarat: evidence for adaptation in response to climatic variation. Curr. Sci. 100, 1833–1846.
Possehl, G. L. (1999). Indus Age: the beginnings. Philadelphia, PA: University of Pennsylvania Press.
Possehl, G. L. (2002). The Indus Civilization: a Contemporary Perspective. Walnut Creek: AltaMira Press.
Post, D. M., and Palkovacs, E. P. (2009). Eco-evolutionary feedbacks in community and ecosystem ecology: interactions between the ecological theatre and the evolutionary play. Philos. Trans. R. Soc. B Biol. Sci. 364, 1629–1640. doi: 10.1098/rstb.2009.0012
Premathilake, R. (2006). Relationship of environmental changes in central Sri Lanka to possible prehistoric land-use and climate changes. Palaeogeogr. Palaeoclimatol. Palaeoecol. 240, 468–496. doi: 10.1016/j.palaeo.2006.03.001
Premathilake, R., and Hunt, C. O. (2017). Late Pleistocene humans used rice in Sri Lanka: Phytolith investigation of the deposits at Fahien rock shelter. J. Hum.-Soc. Sci. 17, 19–29.
Premathilake, R., and Risberg, J. (2003). Late quaternary climate history of the horton plains, central Sri Lanka. Quat. Sci. Rev. 22, 1525–1541. doi: 10.1016/S0277-3791(03)00128-8
Purugganan, M. D., and Fuller, D. Q. (2011). Archaeological data reveal slow rates of evolution during plant domestication: rates of phenotypic evolution during domestication. Evolution 65, 171–183. doi: 10.1111/j.1558-5646.2010.01093.x
Qin, L., and Fuller, D. Q. (2019). “Why rice farmers don’t sail: coastal subsistence traditions and maritime trends in early China,” in Prehistoric Maritime Cultures and Seafaring in East Asia, The Archaeology of Asia-Pacific Navigation, eds C. Wu and B. V. Rolett (Singapore: Springer).
Rajagopalan, G., Vishnu-Mittre, Sekar, B., and Mandal, T. K. (1982). Birbal sahni institute radiocarbon measurements III. Radiocarbon 24, 45–53.
Reddy, S. N. (1997). If the threshing floor could speak: integration of agriculture and pastoralism during the late harappan in Gujarat, India. J. Anthropol. Archaeol. 16, 162–187.
Reddy, S. N. (2003). “Discerning palates of the past: an ethnoarchaeological study of crop cultivation and plant usage in India, ethnoarchaeological series,” in Proceedings International Monographs in Prehistory, (Ann Arbor, Mich).
Risberg, J., Myrdal-Runebjer, E., and Miller, U. (2002). Sediment and soil characteristics and an evaluation of their applicability to the irrigation history in Sigiriya, Sri Lanka. J. Nord. Archaeol. Sci. 13, 27–42.
Ruddiman, W. F., and Thompson, J. S. (2001). The case for human causes of increased atmospheric CH4 over the last 5000 years. Quat. Sci. Rev. 20, 1769–1777.
Ruddiman, W. F., Guo, X., Zhou, H., and Wu, Y. (2008). Early rice farming and anomalous methane trends. Quat. Sci. Rev. 27, 1291–1295. doi: 10.1038/nature09739
Sang, T., and Ge, S. (2007). Genetics and phylogenetics of rice domestication. Curr. Opin. Genet. Dev. 17, 533–538. doi: 10.1016/j.gde.2007.09.005
Saraswat, K. S. (1992). Archaeobotanical remains in ancient cultural and socio-economic dynamics of the indian subcontinent. Palaeobotanist 40, 514–545.
Saraswat, K. S. (2002). Balu (29o40’ N; 76o22’ E), District Kaithal. Indian Archaeol. Rev. 1996–7, 198–203.
Saraswat, K. S. (2004). “Plant economy of early farming communities,” in Early Farming Communities of the Kaimur (Excavations at Senuwar), ed. B. P. Singh (Jaipur: Publication Scheme).
Saraswat, K. S. (2005). Agricultural background of the early farming communities in the middle ganga plain. Pragdhara 15, 145–177.
Saraswat, K. S., and Pokharia, A. K. (2002). Harappan plant economy at ancient Balu, Haryana. Pragdhara 12, 153–172.
Saraswat, K. S., and Pokharia, A. K. (2003). Palaeoethnobotanical investigations at early harappan Kunal. Pragdhara 13, 105–140.
Saraswat, K. S., Srivastava, C., and Pokharia, A. K. (2000). Palaeobotanical and pollen analytical investigations. Indian Archaeol. Rev. 1994–1995, 96.
Sato, Y. (2002). “Origin of rice cultivation in the Yangtze River Basin,” in The Origins of Pottery and Agriculture, ed. Y. Yasuda (New Delhi: Lustre), 143–150.
Schatz, M. C., Maron, L. G., Stein, J. C., Wences, A. H., Gurtowski, J., Biggers, E., et al. (2014). Whole genome de novo assemblies of three divergent strains of rice, Oryza sativa, document novel gene space of aus and indica. Genome Biol. 15:506. doi: 10.1186/s13059-014-0506-z
Sharma, G. B., and Misra, B. B. (1980). Excavations at Chopani-Mando (Belan Valley) 1977-1979?: Epipalaeolithic to Protoneolithic. Allahbad: University of Allahabad.
Sherman, D. G. (1990). Rice, Rupees, and Ritual: Economy and Society Among the Samosir Batak of Sumatra. Stanford: Stanford University Press.
Silva, F., Weisskopf, A., Castillo, C., Murphy, C., Kingwell-Banham, E., Qin, L., et al. (2018). A tale of two rice varieties: modelling the prehistoric dispersals of japonica and proto- indica rices. Holocene 28, 1745–1758. doi: 10.1177/0959683618788634
Singh, I. B. (2005a). Quaternary palaeoenvironments of the ganga plain and anthropogenic activity. Man Environ. 30, 1–35.
Singh, I. B. (2005b). Landform development and palaeovegetation in Late Quaternary of the Ganga Plain: implicatrions for anthropogenic activity. Pragdhara 15, 5–31.
Sinha, S. (2022). From cotton to paddy: political crops in the Indian Punjab. Geoforum 130, 146–154. doi: 10.1016/j.geoforum.2021.05.017
Sinopoli, C., Morrison, K. D., Bauer, A., Kelly, G., Johansen, P. G., Trivedi, M., et al. (2010). Production, Consumption and Political Transformation in Late Prehistoric/Early Historic South India: Final Report, NSF Grant BCS-0350803. Alexandria, VA: National Science Foundation.
Smith, B. D. (2011a). General patterns of niche construction and the management of ‘wild’ plant and animal resources by small-scale pre-industrial societies. Philos. Trans. R. Soc. B Biol. Sci. 366, 836–848. doi: 10.1098/rstb.2010.0253
Smith, B. D. (2011b). A cultural niche construction theory of initial domestication. Biol. Theory 6, 260–271. doi: 10.1007/s13752-012-0028-4
Suryanarayan, A., Cubas, M., Craig, O. E., Heron, C. P., Shinde, V. S., Singh, R. N., et al. (2021). Lipid residues in pottery from the indus civilisation in Northwest India. J. Archaeol. Sci. 125:105291. doi: 10.1016/j.jas.2020.105291
Sweeney, M. T., Thomson, M. J., Cho, Y. G., Park, Y. J., Williamson, S. H., Bustamante, C. D., et al. (2007). Global dissemination of a single mutation conferring white pericarp in rice. PLoS Genet. 3:e133. doi: 10.1371/journal.pgen.0030133
Tan, L., Li, X., Liu, F., Sun, X., Li, C., Zhu, Z., et al. (2008). Control of a key transition from prostrate to erect growth in rice domestication. Nat. Genet. 40, 1360–1364. doi: 10.1038/ng.197
Tang, J., Xia, H., Cao, M., Zhang, X., Zeng, W., Hu, S., et al. (2004). A comparison of rice chloroplast genomes. Plant Physiol. 135, 412–420. doi: 10.1104/pp.103.031245
Tewari, R., Srivastava, K. K., Singh, K. K., Vinay, R., Trivedi, R. K., and Singh, G. C. (2005). Recently excavated sites in the Ganga Plain and North Vindhyas: some observations regarding the pre-urban context. Pragdhara 15, 39–49.
Tewari, R., Srivastava, R. K., Saraswat, K. S., Singh, I. B., and Singh, K. K. (2008). Early farming at lahuradewa. Pragdhara 18, 347–373. doi: 10.1016/j.jas.2016.04.018
Tewari, R., Srivastava, R. K., Singh, K. K., Saraswat, K. S., and Singh, I. B. (2003). Preliminary report of the excavation at Lahuradewa, District Sant KabirNagar, U.P. 2001–2002. wider archaeological implications. Pragdhara 13, 37–68.
Tewari, R., Srivastava, R. K., Singh, K. K., Saraswat, K. S., Singh, I. B., Chauhan, M. S., et al. (2006). Second preliminary report of the excavations at lahuradewa district Sant Kabir Nagar, U.P.?: 2002-2003-2004 & 2005-06. Pragdhara 16, 35–68.
Thompson, G. B. (1996). “Ethnographic models for interpreting rice remains,” in The Excavations at Khok Phanom Di, a Prehistoric Site in Central Thailand, eds C. Higham and R. Thosarat (London: The Society of Antiquaries of London), 119–150.
Travis, A. J., Norton, G. J., Datta, S., Sarma, R., Dasgupta, T., Savio, F. L., et al. (2015). Assessing the genetic diversity of rice originating from Bangladesh, Assam and West Bengal. Rice 8:35. doi: 10.1186/s12284-015-0068-z
Varma, R. K., Misra, V. D., Pandey, J. N., and Pal, J. N. (1985). A preliminary report on the excavations at Damdama (1982-1984). Man Environ. 9, 45–65.
Vaughan, D. A., Kadowaki, K., Kaga, A., and Tomooka, N. (2005). On the phylogeny and biogeography of the genus Oryza. Breed. Sci. 55, 113–122. doi: 10.1270/jsbbs.55.113
Vaughan, D. A., Lu, B., and Tomooka, N. (2008a). Was Asian rice (Oryza sativa) domesticated more than once? Rice 1, 16–24.
Vaughan, D. A., Lu, B.-R., and Tomooka, N. (2008b). The evolving story of rice evolution. Plant Sci. 174, 394–408. doi: 10.1016/j.plantsci.2008.01.016
Vishnu-Mittre Savithri, R. (1975). Supposed remains of rice (Oryza sp.) in the terracotta cakes and Pai at Kalibangan, Rajasthan. Palaeobotanist 22, 124–126.
Vishnu-Mittre Savithri, R. (1982). “Food economy of the harappans,” in Harappan Civilisation, ed. G. L. Possehl (New Delhi: Oxford and IBH Publishing Co. Pvt. Ltd.), 205–221.
Vishnu-Mittre. (1974). “Palaeobotanical evidence in India,” in Evolutionary Studies in World Crops: Diversity and Change in the Indan Subcontinent, ed. J. Hutchinson (Cambridge: Cambridge University Press), 3–30. doi: 10.1038/srep10379
Vitte, C., Ishii, T., Lamy, F., Brar, D., and Panaud, O. (2004). Genomic paleontology provides evidence for two distinct origins of Asian rice (Oryza sativa L.). Mol. Genet. Genomics 272, 504–511. doi: 10.1007/s00438-004-1069-6
Weber, S. A. (1991). Plants and Harappan Subsistence: an Example of Stability and Change from Rojdi. New Delhi: Oxford and IBH Publishing Co. Pvt. Ltd.
Weber, S. A. (1997). “Harappa archaeobotany: a model for subsistence,” in South Asian Archaeology 1995, eds B. Allchin and F. R. Allchin (New Delhi: Oxford and IBH Publishing Co. Pvt. Ltd.), 115–117.
Weber, S. A. (1999). Seeds of urbanism: palaeoethnobotany and the Indus Civilization. Antiquity 73, 813–826.
Weber, S. A. (2003). “Archaeobotany at harappa: indications for change,” in, Indus Ethnobiology: New Persepctives from the Field. eds S. A. Weber and W. R. Belcher, (Lanham: Lexington Books).
Weber, S. A., and Fuller, D. Q. (2008). Millets and their role in early agriculture. Pragdhara 18, 69–90.
Weber, S. A., Kashyap, A., and Mounce, L. (2011). “Archaeobotany at farmana: new insights into harappan plant use strategies,” in Excavations at Farmana, District Rohtak, Haryana, India, 2006-8, eds V. Shinde, T. Osada, and M. Kumar (Kyoto: Research Institute for Humanity and Nature), 808–825.
Weber, S., and Kashyap, A. (2016). The vanishing millets of the Indus civilization. Archaeol. Anthropol. Sci. 8, 9–15. doi: 10.1007/s12520-013-0143-6
Weisskopf, A., Deng, Z., Qin, L., and Fuller, D. Q. (2015). The interplay of millets and rice in Neolithic central China: integrating phytoliths into the archaeobotany of Baligang. Archaeol. Res. Asia 4, 36–45. doi: 10.1016/j.ara.2015.10.002
Weisskopf, A., Harvey, E., Kingwell-Banham, E., Kajale, M., Mohanty, R., and Fuller, D. Q. (2014). Archaeobotanical implications of phytolith assemblages from cultivated rice systems, wild rice stands and macro-regional patterns. J. Archaeol. Sci. 51, 43–53. doi: 10.1016/j.jas.2013.04.026
West-Eberhard, M. J. (2005a). Developmental plasticity and the origin of species differences. Proc. Natl. Acad. Sci. 102, 6543–6549. doi: 10.1073/pnas.0501844102
West-Eberhard, M. J. (2005b). Phenotypic accommodation: adaptive innovation due to developmental plasticity. J. Exp. Zoolog. B Mol. Dev. Evol. 304B, 610–618. doi: 10.1002/jez.b.21071
Wheeler, M. (1950). Five Thousand Years of Pakistan: an Archaelogical Outline. London: Royal India and Pakistan Society.
White, J. C. (1995). Modelling the development of early rice agriculture: ethnoecological perspectives from Northeast Thailand. Asian Perspect. 34, 37–67.
Willcox, G. (1999). “Agrarian change and the beginnings of cultivation in the Near East: evidence from wild progenitors, experimental cultivation and archaeobotanical data,” in The Prehistory of Food. Appetites for Change, eds C. Gosden and J. Hather (London: Routledge), 478–500.
Willcox, G., and Stordeur, D. (2012). Large-scale cereal processing before domestication during the tenth millennium cal BC in northern Syria. Antiquity 86, 99–114. doi: 10.1017/S0003598X00062487
Willcox, G., Fornite, S., and Herveux, L. (2008). Early Holocene cultivation before domestication in northern Syria. Veg. Hist. Archaeobotany 17, 313–325. doi: 10.1007/s00334-007-0121-y
Wolff, A. C., Westbrook, A. S., and DiTommaso, A. (2022). In the ruins: the neglected link between archaeology and weed science. Weed Sci. 70, 135–143. doi: 10.1017/wsc.2022.11
Wright, R. P. (2010). The Ancient Indus: Urbanism, Economy, and Society, Case Studies in Early Societies. New York, NY: Cambridge University Press.
Wu, Y., Jiang, L., Zheng, Y., Wang, C., and Zhao, Z. (2014). Morphological trend analysis of rice phytolith during the early Neolithic in the Lower Yangtze. J. Archaeol. Sci. 49, 326–331. doi: 10.1016/j.jas.2014.06.001
Xiong, L. Z., Liu, K. D., Dai, X. K., Xu, C. G., and Zhang, Q. (1999). Identification of genetic factors controlling domestication-related traits of rice using an F2 population of a cross between Oryza sativa and O. rufipogon. Theor. Appl. Genet. 98, 243–251. doi: 10.1007/s001220051064
Yang, X., Wan, Z., Perry, L., Lu, H., Wang, Q., Zhao, C., et al. (2012). Early millet use in northern China. Proc. Natl. Acad. Sci. U S A. 109, 3726–3730. doi: 10.1073/pnas.1115430109
Zeder, M. A. (2012a). “Pathways to animal domestication,” in Biodiversity in Agriculture: Domestication, Evolution, and Sustainability. Presented at the Harlan Symposium, eds J. R. Harlan and P. L. Gepts (Cambridge, NY: Cambridge University Press).
Zeder, M. A. (2012b). The broad spectrum revolution at 40: resource diversity, intensification, and an alternative to optimal foraging explanations. J. Anthropol. Archaeol. 31, 241–264. doi: 10.1016/j.jaa.2012.03.003
Zeder, M. A. (2015). Core questions in domestication research. Proc. Natl. Acad. Sci. U S A. 112, 3191–3198. doi: 10.1073/pnas.1501711112
Zeder, M. A. (2017). Domestication as a model system for the extended evolutionary synthesis. Interface Focus 7:20160133. doi: 10.1098/rsfs.2016.0133
Zhang, J., Lu, H., Gu, W., Wu, N., Zhou, K., Hu, Y., et al. (2012). Early mixed farming of millet and rice 7800 years ago in the middle yellow river region. China. PLoS One 7:e52146. doi: 10.1371/journal.pone.0052146
Zhang, L.-B., Zhu, Q., Wu, Z.-Q., Ross-Ibarra, J., Gaut, B. S., Ge, S., et al. (2009). Selection on grain shattering genes and rates of rice domestication. New Phytol. 184, 708–720. doi: 10.1111/j.1469-8137.2009.02984.x
Zheng, Y., Matsui, A., and Fujiwara, H. (2003). Phytoliths of rice detected in the neolithic sites in the valley of the Taihu Lake in China. Environ. Archaeol. 8, 177–183. doi: 10.1179/env.2003.8.2.177
Zheng, Y., Sun, G., and Chen, X. (2007). Characteristics of the short rachillae of rice from archaeological sites dating to 7000 years ago. Chin. Sci. Bullentin 52, 1654–1660.
Keywords: rice, South Asia, Mesolithic, Ganges, domestication, Indus Civilization
Citation: Bates J (2022) The Fits and Starts of Indian Rice Domestication: How the Movement of Rice Across Northwest India Impacted Domestication Pathways and Agricultural Stories. Front. Ecol. Evol. 10:924977. doi: 10.3389/fevo.2022.924977
Received: 21 April 2022; Accepted: 18 May 2022;
Published: 05 July 2022.
Edited by:
Petra Vaiglova, Washington University in St. Louis, United StatesReviewed by:
Henry Lamb, Aberystwyth University, United KingdomCarolina Jiménez Arteaga, Pompeu Fabra University, Spain
Copyright © 2022 Bates. This is an open-access article distributed under the terms of the Creative Commons Attribution License (CC BY). The use, distribution or reproduction in other forums is permitted, provided the original author(s) and the copyright owner(s) are credited and that the original publication in this journal is cited, in accordance with accepted academic practice. No use, distribution or reproduction is permitted which does not comply with these terms.
*Correspondence: Jennifer Bates, amJhdGVzMDFAc251LmFjLmty