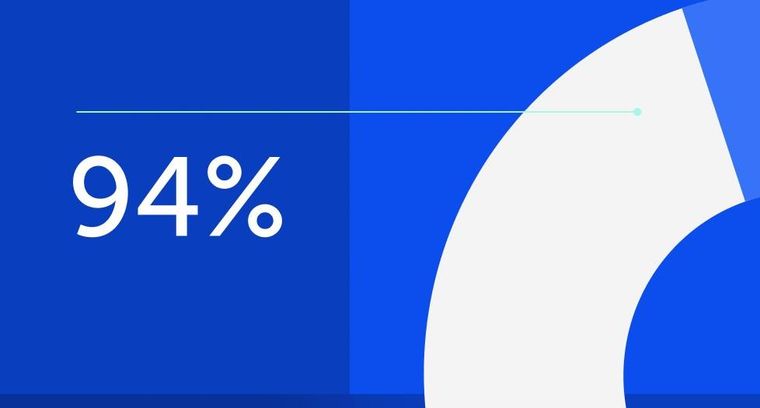
94% of researchers rate our articles as excellent or good
Learn more about the work of our research integrity team to safeguard the quality of each article we publish.
Find out more
ORIGINAL RESEARCH article
Front. Ecol. Evol., 14 July 2022
Sec. Chemical Ecology
Volume 10 - 2022 | https://doi.org/10.3389/fevo.2022.923871
This article is part of the Research TopicInsights in Chemical Ecology: 2022View all 4 articles
Ant colonies have vast and diverse nutritional needs but forager ants have limited mobility to meet these needs. Forager ants would accrue significant energy savings if they were able to sense and orient toward odor plumes of both carbohydrate and protein food sources. Moreover, if worker ants, like other flightless insects, had reduced olfactory acuity, they would not recognize the specific odor signatures of diverse carbohydrate and protein sources, but they may be able to orient toward those odorants that are shared between (macronutrient) food sources. Using the Western carpenter ant, Camponotus modoc, as a model species, we tested the hypotheses that (1) food sources rich in carbohydrates (aphid honeydew, floral nectar) and rich in proteins (bird excreta, house mouse carrion, cow liver infested or not with fly maggots) all prompt long-distance, anemotactic attraction of worker ants, and (2) attraction of ants to plant inflorescences (fireweed, Chamaenerion angustifolium; thimbleberry, Rubus parviflorus; and hardhack, Spiraea douglasii) is mediated by shared floral odorants. In moving-air Y-tube olfactometer bioassays, ants were attracted to two of four carbohydrate sources (thimbleberry and fireweed), and one of four protein sources (bird excreta). Headspace volatiles of these three attractive sources were analyzed by gas chromatography-mass spectrometry, and synthetic odor blends of thimbleberry (7 components), fireweed (23 components), and bird excreta (38 components) were prepared. In Y-tube olfactometer bioassays, synthetic blends of thimbleberry and fireweed but not of bird excreta attracted ants, indicating that only the two floral blends contained all essential attractants. A blend of components shared between thimbleberry and fireweed was not attractive to ants. Our data support the conclusion that C. modoc worker ants can sense and orient toward both carbohydrate and protein food sources. As ants were selective in their responses to carbohydrate and protein resources, it seems that they can discern between specific food odor profiles and that they have good, rather than poor, olfactory acuity.
Ant colonies have vast nutritional needs. Foraging worker ants must meet not only their own nutritional needs but also those of their nestmates. Worker ants require primarily carbohydrates for energy, whereas the queen(s) and brood also require proteins for egg production and larval development, respectively (Markin, 1970; Sorensen and Vinson, 1981; Weeks et al., 2006). To meet these nutritional needs, ants engage in complex and diverse foraging activities. Ants obtain sugary honeydew excretions from hemipteran insects, hunt for insect prey, scavenge for deceased insects, feed on floral and extrafloral nectar as well as pollen, collect plant seeds, harvest plant foliage to cultivate mutualistic fungi, and acquire nutrients from animal excreta and carrion (Hölldobler and Wilson, 1990). The ants’ foraging activities may alter biotic and abiotic characteristics of their habitat, including the plant community composition (Halaj et al., 1997; Macmahon et al., 2000; Swanson et al., 2019).
The means by which foraging ants locate food sources have rarely been studied (Knaden and Graham, 2016). Engaging in certain foraging patterns may increase the likelihood of locating food (Dornhaus and Powell, 2010) but sensing and responding to cues from food sources would make foraging more energy-efficient. Visual cues associated with insect prey seem to guide some foraging ants (Baroni Urbani et al., 1994; Beugnon et al., 2001). Olfactory resource cues guide many foraging insects (Cardé and Willis, 2008; Webster and Cardé, 2017) and – as shown in a few studies – also guide ants (e.g., Zhou et al., 2012; Fischer et al., 2015). Some ants learn to associate odors with food sources, and via trophallaxis pass on food odor information to nestmates (Dupuy et al., 2006; Provecho and Josens, 2009; Nelson et al., 2019; Oberhauser et al., 2019). Innate recognition of certain food odors would expedite the process of locating resources that are reliably present, whereas learned odors may help locate and exploit fleeting resources.
Foraging requires energy expenditures not only for the locomotory physical activity but also for the maintenance of those sensory receptors and nervous tissues that inform foraging activities (Niven and Laughlin, 2008; Dornhaus and Powell, 2010; Elgar et al., 2018). As flightless foragers, ants have limited mobility and would accrue significant energy savings for themselves and for the entire colony, if they were able to track the odor plume from all valuable resources and pinpoint their location from a distance. Specific ant taxa are known to respond to odor cues from specific resources, such as deceased insects (Buehlmann et al., 2014; see below), honeydew (Zhou et al., 2012; Fischer et al., 2015; see below), and floral nectar (Schiestl and Glaser, 2012; De Vega et al., 2014; see below) but to date no study has investigated whether conspecific ants are able to respond to odor cues from multiple macronutrient sources including those consisting of mainly carbohydrates and proteins. This ability would be adaptive because foragers must adjust their foraging activities and priorities in accordance with their colony’s needs. When brood is present, they must collect not only more food but also more proteinaceous food (Cornelius and Grace, 1997; Dussutour and Simpson, 2008, 2009).
Protein-rich food sources such as insect prey, carrion, and animal excreta are often ephemeral. Challenged to locate them quickly, scavenging desert ants, Cataglyphis fortis, use olfaction to find deceased insects (Buehlmann et al., 2014), and the ponerine ant Pachycondyla analis and the formicine ant Crematogaster scutellaris exploit prey odor to locate termite and fig wasp prey, respectively (Schatz et al., 2003; Yusuf et al., 2014). As protein sources commonly release indole – which is a breakdown product of tryptophan and is an indicator of essential amino acid presence (Tomberlin et al., 2016) – many insects, including C. fortis, use indole as a generic semiochemical to locate protein sources (Chaudhury et al., 2015; Zito et al., 2015; Brodie et al., 2016, 2018; Cortez et al., 2017; Zhao et al., 2020). Similarly, workers of C. fortis are attracted to linoleic acid, a necromone indicative of deceased insects (Buehlmann et al., 2014).
Carbohydrates are vital to ant colony survival (Cook et al., 2010; Dussutour and Simpson, 2012; Bazazi et al., 2016; Arganda et al., 2017). Carbohydrates sought by ants originate mainly from floral and extrafloral nectar and sugary honeydew. Previously considered deleterious nectar thieves (Willmer et al., 2009), ants are increasingly documented as floral visitors and pollinators (De Vega et al., 2009; Czechowski et al., 2011; Luo et al., 2012; Ibarra-Isassi and Sendoya, 2016; Kuriakose et al., 2018; Del-Claro et al., 2019; Delnevo et al., 2020). Yet, there are still only a few examples of ant attraction to honeydew or floral and leaf semiochemicals (message bearing chemicals). Workers of both the black garden ant, Lasius niger, and the red-imported fire ant, Solenopsis invicta, are attracted to honeydew excreted by aphids (Zhou et al., 2012; Fischer et al., 2015). Similarly, workers of the African weaver ant, Oecophylla longinoda, respond to leaf odors of cashew trees, Anacardium occidentale, and protect these trees from herbivores in exchange for extrafloral nectar rewards (Wanjiku et al., 2014). Some species of ants respond to herbivore-induced plant volatiles (Agrawal and Dublin-Thaler, 1998; Bruna et al., 2008; Schettino et al., 2017), while others are attracted to floral odors of specific plants (Cytinus hypocistis, Chamorchis alpine), serving as their exclusive pollinators (De Vega et al., 2009, 2014; Schiestl and Glaser, 2012).
If worker ants had reduced olfactory acuity, like other flightless insects (Neupert et al., 2020), they would not likely be able to recognize the specific odor profiles of diverse food sources but might still be able to locate them by responding to key odorants shared between these sources. For example, many inflorescences that provide essential carbohydrates to pollinators share linalool and α-pinene as floral attractants (Knudsen et al., 2006; Nicolson, 2011). Whether foraging ants respond to specific or generic carbohydrate semiochemicals has not yet been investigated.
Western carpenter ants, Camponotus modoc, as a model species in our study, are commonly found in coniferous forests along the west coast of North America (Hansen and Klotz, 2005). They forage on aphid honeydew (Tilles and Wood, 1982; Renyard et al., 2021), scavenge arthropod prey (Hansen and Akre, 1985; Tilles and Wood, 1986), and feed on bird excreta, mammal urine, and carrion (AR pers. obvs). Of the many saccharides present in aphid honeydew, worker ants preferentially consume fructose and sucrose (Renyard et al., 2021) which are widely present also in floral nectar (Blüthgen et al., 2004; Woodring et al., 2004). The carpenter ants’ favorite saccharides also occur in the nectar of fireweed, Chamaenerion angustifolium (Antoń et al., 2017), and are likely present in the nectar of thimbleberry, Rubus parviflorus, which are two plant species common in forest clearings. The ants may less likely encounter hardhack, Spiraea douglasii – which thrives in more riparian habitats – but might still respond to its floral odor when presented with it. Whether carpenter ants are attracted to floral resources has not yet been studied but pollinivory by ants, including Camponotus carpenter ants (Czechowski et al., 2011; Cembrowski et al., 2015), is increasingly observed.
Here, we tested the hypotheses that (1) food sources rich in carbohydrates (aphid honeydew, floral nectar) and rich in proteins (bird excreta, house mouse carrion, cow liver infested or not with fly maggots) all prompt long-distance attraction of worker ants, and (2) attraction of worker ants to inflorescences (fireweed, thimbleberry, and hardhack) is mediated by floral semiochemicals that are shared between these plants.
Nests of C. modoc were collected as previously described by Renyard et al. (2019). Briefly, we excised nine nests from forest logs and maintained them in an outdoor undercover area of the Science Research Annex (Burnaby campus, Simon Fraser University), where they experienced natural light and temperature cycles. We housed ant-infested log sections in large plastic bins connected via clear Nalgene™ tubing to glass aquaria provisioned with food (20% sugar water, apples, meal worms, cockroaches) ad libitum.
Attraction of ants to odor sources was tested in glass Y-tube olfactometers, with odor and control stimuli placed by stratified random assignment (Thompson, 2012) at the orifice of the left or right side arm (Renyard et al., 2019; Figure 1). For each bioassay, we disconnected the Nalgene tubing (see above) from an aquarium and allowed a single outbound ant to walk into a glass holding tube inserted into the Nalgene tubing. We then attached the holding tube to the Y-tube olfactometer via a male/female glass joint and drew air at 0.5 L/min through the olfactometer system with a Neptune Dyna vacuum pump (A.O. Smith, Tipp City, OH, United States). An ant’s first choice of side arm was recorded when she crossed a line 6 cm from a side arm’s orifice. Ants that did not make a choice within 10 min were considered non-responders and were excluded from statistical analyses. We aimed for 30 replicates per experiment but ran fewer replicates if the test stimulus was obviously not attractive, and we ran more replicates if deemed necessary to avoid statistical error type II. Any ant was bioassayed only once. Following bioassays, counter tops and the rubber stopper connecting the vacuum tubing to the holding tube (Figure 1) were cleaned with hexane and ethanol. Y-tubes and holding tubes were cleaned with hot water and soap (Sparkleen, Thermo Fisher Scientific, MA, United States) and dried in an oven for at least 1 h at 100°C. We ran olfactometer experiments during the summer of 2018, 2019, 2020, and 2021.
Figure 1. Graphical illustrations of experimental designs. (A) Set-up for collecting headspace volatiles from natural sources; air was drawn through activated charcoal, a glass chamber containing a natural odor source, and a volatile trap (the adsorbent Porapak in a glass tube). (B) Y-tube olfactometer bioassay station for testing attraction of ants to test stimuli; for each replicate, test stimuli were (i) placed in small plastic bags secured to the side arms of the Y-tube, or (ii) inserted directly into the side arms. Then, the holding tube housing a single bioassay ant was connected to both a vacuum pump and the Y-tube, allowing the ant to walk upwind toward test stimuli. Test stimuli consisted of natural odor sources, headspace volatile extracts (HVEs), and synthetic blends (SBs) of candidate semiochemicals. Aliquots of HVEs, SBs and corresponding solvent control stimuli were pipetted onto pieces of cotton wick placed at the orifice of side arms (see Table 1 for details).
To isolate the effects of aphid/honeydew presence as the test variable for ant attraction, we bioassayed aphid-infested vs. uninfested branches. Branches infested, or not, with Cinara splendens aphids were cut from a Douglas-fir tree, Pseudotsuga menziesii, and inserted into parafilm-covered, water-filled 0.5-dram vials. To ensure that control branches were free of honeydew, they were gently rinsed with water prior to clipping. We then placed one aphid-infested branch and one control branch into separate Ziploc bags (S.C. Johnson and Son, Ltd., Brantford, ON, Canada), cut open one corner of bags to allow air intake, and secured the large opening of bags with a metal hose clamp to the side arms of the Y-tube olfactometer. For each bioassay ant, we used a new aphid-infested branch and a new control branch.
To isolate the inflorescence effect on ant attraction, we bioassayed branches with or without inflorescence, or, a leaf vs. a flower (thimbleberry). Inflorescences and corresponding control branches or leaves of fireweed, thimbleberry, and hardhack were cut from live plants. Each inflorescence and a corresponding control stimulus (see below) were inserted into separate parafilm-covered, water-filled 0.5-dram vials. Hardhack inflorescences consisted of ∼130 individual florets, with similar-sized hardhack branches serving as a control stimulus. For thimbleberry, a single flower and a neighboring leaf served as treatment and control stimuli, respectively. For fireweed, a peduncle with 3–10 flowers and a peduncle with leaves only (control) were tested. Hardhack inflorescences and single thimbleberry flowers, with paired controls, were directedly inserted into side arms of the Y-tube olfactometer. Fireweed inflorescences with paired controls were enclosed in separate Ziploc bags which were then attached to the side arms of Y-tube olfactometers. For each bioassay ant, we tested new plant material.
CO2-euthanized female house mice, Mus musculus, and beef liver from recently slaughtered cows were tested as fresh carrion sources in parallel Y-tube olfactometer experiments. House mice were salvaged from an unrelated experiment which required removal of their uterus followed by freezing. Bisected mice (Exp. 5) and mass-matched beef liver pieces (each ∼6 g) (Exp. 6) were wrapped in cheese cloth and frozen until use. Prior to testing in bioassays, samples were thawed in warm water and then placed in a glass tube (60 mm × 19 mm inner diam.) kept on ice. For each replicate, paired tubes containing either the test sample wrapped in cheese cloth or cheese cloth only (control) were inserted into the side arms of the Y-tube olfactometer.
To obtain aged, maggot-infested carrion (Exp. 7), a 354-mL paper cup (Solo Cup Company, IL, United States) containing a piece of beef liver (2.5 cm × 7.5 cm) was placed into a cage (61 cm × 61 cm × 61 cm; BioQuip®, Compton, CA, United States) with 500 male and female blow flies, Phormia regina, allowing females 4 h to oviposit on the liver. After the 4-h period, the cup was removed, another piece of liver (2.5 cm × 7.5 cm) was added, and the cup was covered with mesh and paper towel and kept in a veiled bin (70.5 cm × 36 cm × 11.5 cm). First-instar maggots were transferred to a glass jar (11 cm × 16.5 cm × 16.5 cm) containing both a chunk of liver (250 g) and wood shavings, and were allowed to develop to 3rd instars which were tested in bioassays. For these bioassays, three 3rd instar maggots, along with the same liver (1-g aliquots) in which they had developed, were enclosed in an 8-layer cheesecloth pouch. Maggot-infested liver pouches and empty control pouches were placed into separate glass tubes (60 mm × 19 mm) and kept on ice in separate coolers prior to bioassays. For each replicate, paired tubes containing either a maggot-infested liver pouch or a blank control pouch were inserted into the side arm of the Y-tube olfactometer. To test the effects of dead mice, fresh and maggot-infested liver, or bird droppings (see below) on ant attraction, we considered air the only appropriate control stimulus as any other ‘control’ odor may have altered the ants’ responses. For each bioassay ant, we tested new stimuli.
Fresh excreta of Ruffs, Calidris pugnax – maintained in SFU’s Animal Care facility for another project – were collected with a scoopula from the ground and placed in a Petri dish. Excreta were homogenized and used within 24 h of collection. On each bioassay day, we placed aliquots of bird excreta (0.5–0.6 g) into glass tubes (60 mm × 19 mm) and covered openings with metal mesh. Paired tubes with, or without (control), bird excreta were placed in separate Styrofoam coolers containing ice. For each replicate, we inserted a glass tube containing bird excreta into one side arm of a Y-tube olfactometer and an empty control tube in the corresponding control side arm. For each bioassay ant, we tested new stimuli.
As fireweed, thimbleberry, and bird excreta attracted ants (see Section “Results”), we collected their headspace volatiles for analyses. Driven by a vacuum pump (Neptune Dyna; A.O. Smith, Tipp City, OH, United States), air was drawn at 1 L min–1 for 16–24 h through activated charcoal, through a glass chamber (41 cm × 17.5 cm diameter) containing the odor source, and finally through a glass tube (14.0 cm × 0.5 cm) filled with Porapak Q adsorbent (200 mg) (Figure 1). Volatiles were desorbed from Porapak Q by flushing it with 2 mL of ether/pentane (1/1).
For fireweed headspace volatile collections, 162 flowers from 20 plants were aerated for 19 h, yielding a total of 3,078 flower-hour equivalents (FHEs) of headspace volatile extract. Aliquots of this extract were tested in behavioral bioassays (Exps. 9–10; below), and extract analyses informed the preparation of a synthetic blend tested in experiment 14.
Thimbleberry headspace flower volatiles were collected on two dates: (1) in 2019 (when most thimbleberry shrubs had already finished blooming), five flowers were aerated for 16 h, yielding a total of 80 FHEs of headspace volatile extract; (2) in 2020, 31 flowers were aerated for 24 h, yielding a total of 744 FHEs of headspace volatile extract. Analysis of the 2019-extract informed the preparation of the synthetic blend tested in experiment 15 (see below).
For headspace volatile collections of bird excreta, 16.6 g of excreta from Ruffs were aerated for 24 h, yielding a total of 397.2 gram-hour equivalents (GHEs) of headspace volatile extract.
Extracts of fireweed, thimbleberry, and bird excreta were concentrated under a nitrogen stream to 200, 130, and 120 μL, respectively, and 2-μL aliquots of each concentrate were analyzed by gas chromatography-mass spectrometry. GC-MS analyses deployed an Agilent GC-MS (Agilent 7890B GC coupled to a 5977A Series MSD; Agilent Technologies Inc., Santa Clara, CA, United States) fitted with a DB-5 column (30 m × 0.25 mm ID.; Agilent Technologies, see above), using helium as the carrier gas (35 cm s–1) and the following temperature program: 50°C (held for 5 min), 10°C per min to 280°C (held for 10 min). Samples were analyzed in split mode (5:1 ratio), with the injector port set to 250°C, the transfer line to 280°C, the MS Quadrupole to 150°C, and the MS source to 230°C. Compounds were identified by comparing their retention indices (Van den Dool and Kratz, 1963) and mass spectra with those of authentic standards.
The sources and purities of authentic chemical standards are listed in Table 2.
Table 2. Chemical numbers (no.; see Figures 3, 4), suppliers, and purities of chemicals tested in Y-tube olfactometer bioassays.
Headspace volatile extracts and synthetic volatile blends were tested in Y-tube olfactometers at doses equivalent to volatiles released from natural test stimuli during 10-min bioassays (see Exps. 3, 4, 8). We also tested synthetic blends at a 10-fold higher dose to account for different release dynamics between synthetic and natural sources. Synthetic blends were formulated in pentane/ether (1/1) and 10-μL aliquots were applied to a piece (1 cm × 1 cm) of cotton dental wick (Richmond Dental & Medical, Charlotte, NC, United States) at the orifice of an olfactometer side arm. In each bioassay, the piece of cotton wick in the control side arm received the corresponding volume (10 μL) of pentane/ether.
Drawing on results that fireweed inflorescences with 3–10 flowers each (median: 7 flowers) attracted ants in 10-min (0.167-h) bioassays (see Section “Results” in Exp. 3), we tested headspace volatile extract in experiment 9 at 1.16 FHEs per replicate (7 flowers × 0.167 h = 1.16 FHEs). Predicting rapid (rather than sustained) release of synthetic volatiles from cotton wicks, we also tested a 10-fold higher dose (11.6 FHEs; Exp. 10).
Drawing on results that one thimbleberry flower was sufficient to attract ants in 10-min bioassays (see Section “Results” in Exp. 4), we were inclined to test headspace volatile extract in experiment 11 at 0.167 FHEs per replicate (1 flower × 0.167 h = 0.167 FHE). However, as fireweed extract was effective only at a 10× higher dose, we instead tested 1.67 FHEs (Exp. 11).
Drawing on results that 0.5 g of bird excreta attracted ants in 10-min bioassays (see Section “Results” in Exp. 8), we tested headspace volatile extract in experiment 12 at 0.084 GHEs per replicate (0.5 g of bird excreta × 0.167 h = 0.084 GHE). Considering that 0.5 g of bird excreta were very attractive to ants (Exp. 8), we tested headspace volatile extract at both a lower dose (0.084 GHE; Exp. 12) and a 10-fold higher dose (0.84 GHE; Exp. 13).
Drawing on results of experiments 9–13, synthetic volatile blends of fireweed inflorescences, thimbleberry flowers, and bird excreta were tested at a 10× dose, comprising 11.6 FHEs, 1.67 FHEs, and 0.84 GHEs, respectively (Table 1). Synthetic blends (SBs) were formulated in pentane/ether (1/1), and 10-μL aliquots of formulations, or of pentane/ether control stimuli, were applied to a cotton wick at the orifice of olfactometer side arms.
As ants were attracted to synthetic volatile blends of fireweed inflorescences and thimbleberry flowers (see Section “Results” of Exps. 14 and 15), we proceeded to test volatiles [(E)-β -caryophyllene, α-humulene] that are shared between these plants. We presented these two compounds at the same 10× dose as tested in experiments 15 (Table 1).
Data (Renyard et al., 2022) were analyzed and graphics prepared using R (V4.0.3; R Core Team, 2020), RStudio (Version 1.4.1103) and Inkscape (Version 1.0.2). Data from all two-choice Y-tube olfactometer experiments were analyzed with a χ2 test against a theoretical 50:50 distribution, under the null hypothesis that treatment stimuli have no effects on the ants’ choices.
In two-choice Y-tube olfactometer experiments (Figure 2), ants preferred fireweed inflorescences to fireweed leaves (χ2 = 4.8, df = 1, n = 30, p = 0.0285), thimbleberry flowers to thimbleberry leaves (χ2 = 4.8286, df = 1, n = 35, p = 0.0280), and bird excreta to clean air (χ2 = 4.84, df = 1, n = 25, p = 0.0278). Ants showed no preference when offered choices between (i) aphid-infested branches and control branches, (ii) hardhack flowers and hardhack leaves, (iii) mouse carrion and clean air, (iii) cow liver and clean air, and (iv) maggot-infested cow liver and clean air (all p > 0.05).
Figure 2. Effect of test stimuli on responses of Camponotus modoc worker ants in Y-tube olfactometer (Figure 1) experiments 1–8, each with 20–35 responding ants. Treatment and control stimuli are indicated above bars and illustrated next to bars. Numbers within bars indicate the number of ants responding to test stimuli and numbers in white inset boxes indicate the number of non-responding ants. An asterisk denotes a significant preference for a test stimulus (Pearson’s χ2 tests: p < 0.05; n. s., not significant).
Headspace volatile extracts of the three sources (fireweed, thimbleberry, and bird excreta) that were attractive to ants proved complex. Thimbleberry headspace volatiles included three hydrocarbons, two alcohols, one ketone, and one methyl ester (Figure 3). Fireweed headspace volatile included six sesquiterpenes, five esters, three alcohols, four ketones, three aldehydes, one triene hydrocarbon, and one methoxy alcohol (Figure 3). Bird excreta headspace volatiles consisted of seven ketones, five alkanes, four acids, three alcohols, three aldehydes, three pyrazines, two sulfides, two nitriles, two esters, one keto-alcohol, one acetate, one monoterpene, one isothiocyanate, one methoxy alcohol, one benzene pyrrole, and one thionitrile (Figure 4). (E)-β-Caryophyllene and α-humulene were shared between thimbleberry and fireweed.
Figure 3. Total ion chromatograms of headspace volatile extracts (HVEs) of thimbleberry (Top) and fireweed (Bottom). Headspace volatiles were adsorbed on Porapak Q (Figure 1) and desorbed with pentane/ether (1/1). HVEs were analyzed by gas chromatography-mass spectrometry (GC-MS; Agilent 7890B GC coupled to a 5977A Series MSD). Names of compounds are listed in Table 2.
Figure 4. Total ion chromatogram of headspace volatile extracts (HVEs) of excreta from Ruffs, Calidris pugnax. Headspace volatiles were adsorbed on Porapak Q (Figure 1) and desorbed with pentane/ether (1/1). HVEs were analyzed by gas chromatography-mass spectrometry (GC-MS; Agilent 7890B GC coupled to a 5977A Series MSD). Names of compounds are listed in Table 2.
When ants in Y-tube olfactometer experiments were offered choices between solvent control stimuli and (i, ii) headspace volatile extracts of fireweed [1× dose (Exp. 9); 10× dose (Exp. 10)], (iii) thimbleberry [10× dose (Exp. 11)], and (iv, v) bird excreta [1× dose (Exp. 12); 10× dose (Exp. 13)], they favored 10× doses of fireweed (Exp. 10: χ2 = 3.9032, df = 1, n = 31, p = 0.0482), thimbleberry (Exp. 11: χ2 = 4.8, df = 1, n = 30, p = 0.0285), and bird excreta (Exp. 13: χ2 = 5.1429, df = 1, n = 28, p = 0.0233; Figure 5). In contrast, they did not prefer 1× doses of fireweed (Exp. 9) and bird excreta (Exp. 12) to solvent controls (each p > 0.05).
Figure 5. Effect of headspace volatile extracts (HVEs) of fireweed, thimbleberry, and bird excreta on responses of Camponotus modoc worker ants in Y-tube olfactometer (Figure 1) experiments 9–12, each with 28–31 responding ants. HVEs in pentane/ether (1/1) were tested at doses equivalent (1×) to volatiles released from natural sources (Exps. 3, 4, 8) or 10-fold higher (10×). The same volume of pentane/ether (1/1) served as control stimulus. Numbers within bars indicate the number of ants responding to test stimuli and numbers within white inset boxes indicate the number of non-responding ants. Asterisks denote a significant preference for a test stimulus (Pearson’s χ2 tests: p < 0.05; n. s., not significant).
When ants in Y-tube olfactometer experiments were offered choices between solvent control stimuli and synthetic volatile blends (10× dose) of fireweed, thimbleberry, and bird excreta, they preferred blends of fireweed (Exp. 14: 5.8276, df = 1, n = 29, p = 0.0158) and thimbleberry (Exp. 15: χ2 = 4.8, df = 1, n = 30, p = 0.0285), but not of bird excreta (Exp. 16: χ2 = 0.030303, df = 1, n = 33, p = 0.86) to solvent controls (Figure 6).
Figure 6. Effect of synthetic blends (SB) of fireweed (FW), thimbleberry (TB), and bird excreta (BE) on responses of C. modoc worker ants in Y-tube olfactometer (Figure 1) experiments 14–16, each with 29–33 responding ants. SBs were formulated in pentane/ether (1/1) and tested at doses equivalent (1×) to volatiles released from natural sources (Exps. 2, 3, 8) or 10-fold higher (10×). The same volume of pentane/ether (1/1) served as control stimulus. Numbers within bars indicate the number of ants responding to test stimuli and numbers within white inset boxes indicate the number of non-responding ants. Asterisks denote a significant preference for a test stimulus (Pearson’s χ2 tests: p < 0.05; n. s., not significant).
When ants in Y-tube olfactometer experiment 17 were offered a choice between a synthetic blend of floral odorants shared between thimbleberry and fireweed [(E)-β-caryophyllene, α-humulene] and a solvent control stimulus, they showed no preference for either test stimulus (χ2 = 2.7931, df = 1, n = 29, p = 0.095; Figure 7).
Figure 7. Effect of a synthetic blend (SB) comprising the two floral odorants [(E)-β-caryophyllene, α-humulene] shared between fireweed (FW) and thimbleberry (TB) on responses of C. modoc worker ants in Y-tube olfactometer (Figure 1) experiment 17. The SB was formulated in pentane/ether (1/1) and tested at a dose 10-fold higher (10×) than volatiles released from thimbleberry (Exp. 4). The same volume of pentane/ether (1/1) served as the control stimulus. Numbers within bars indicate the number of ants responding to test stimuli and the number within the white inset box indicates a single non-responding ant; n.s., not significant; p > 0.05.
Ant colonies have vast and diverse nutritional needs including carbohydrates and proteins (Porter, 1989; Evans and Pierce, 1995; Feldhaar et al., 2007; Blüthgen and Feldhaar, 2010; Mankowski and Morrell, 2014), but forager ants have limited mobility to meet these needs. Foragers face the challenge of not only finding enough nutrients for themselves but also for all of their nestmates including the developing brood (Csata and Dussutour, 2019). Foragers would likely accrue significant energy savings for themselves and for their entire colony if they were able to sense olfactory cues from both carbohydrate and protein sources, and to engage in long-distance orientation toward them. If forager ants had reduced olfactory acuity, like other flightless insects (Neupert et al., 2020), they might not be able to recognize the specific odor profiles of multiple food sources but might still be able to locate them by responding to key odorants shared between food sources. Working with Western carpenter ants as a model species, we show that foragers are capable of long-distance orientation toward both carbohydrate and protein food sources. Foragers were attracted to two of four carbohydrate sources (thimbleberry and fireweed) and to one of four protein sources (bird excreta) that we tested in bioassays. However, a blend of floral odorants shared between thimbleberry and fireweed was not attractive to ants, indicating that select floral odorants, while common among plants, are not attractive to ants when presented outside typical floral odor context. As ants were selective in their responses to carbohydrate and protein food sources, we conclude that they can discern between specific food odor profiles, and that they seem to have good, rather than poor, olfactory acuity.
All four sources of carbohydrates (aphid-infested conifer branches, blooming fireweed, thimbleberry, and hardhack) that we tested in our study would have provided nutritional value to foraging ants. Thus, it is surprising that aphid-infested branches and hardhack did not attract ants. Honeydew, in particular, is consumed by many species of ants – including C. modoc (Tilles and Wood, 1986; Yamamoto and Del-Claro, 2008; Ness et al., 2010; Renyard et al., 2021) – and may constitute a large proportion of an ant’s diet (Domisch et al., 2009; Pekas et al., 2011). That worker ants of L. niger and S. invicta were attracted to honeydew (Zhou et al., 2012; Fischer et al., 2015), but C. modoc workers were not (Figure 2), has at least three plausible explanations: (1) there simply may not have been sufficient honeydew accumulation on the aphid-infested branches; (2) the aphid honeydew, which at the time of excretion is odorless (person obs.), was not yet extensively colonized by exogenous microbes whose volatile metabolites attract natural enemies to aphid colonies (Leroy et al., 2011), and also accounted for attraction of L. niger workers (Fischer et al., 2015); and (3) Cinara aphids colonize tall conifer trees and their honeydew odors, or alarm pheromone signals, may not consistently reach ground-dwelling carpenter ants, providing little opportunity for ants to associate Cinara honeydew odor or aphid pheromones with the presence of aphids and carbohydrate rewards (Verheggen et al., 2012).
Insufficient overlap between habitats colonized by carpenter ants and hardhack may also explain the non-attractiveness of hardhack flowers. Hardhack thrives in open riparian habitats (Pojar et al., 1994) and is less common in areas frequented by carpenter ants (A.R.; pers. observ.), whereas fireweed and thimbleberry are common plant community members of the forest ecosystem (Pojar et al., 1994) inhabited by carpenter ants. Alternatively, the nectar or pollen rewards of hardhack are not sufficiently appealing to, or accessible by, carpenter ants.
Animal-derived nitrogenous sources such as bird excreta, vertebrate urine, and carrion are ephemeral resources. If foraging ants were to rely on chance encounters of these resources, they might not be able to meet the protein requirements of their colony’s egg-laying queen and developing brood. Expectedly then, worker ants were attracted to fresh bird excreta (Figure 2). While ants are known to forage on bird excreta (Kaspari, 1993; Jaffe et al., 2001; Sainz-Borgo, 2015), their olfactory attraction to bird excreta has not previously been reported. Bird excreta are nutritionally valuable to carpenter ants not only as a protein source, but also as a source of uric acid and urea. The ants’ obligate endosymbiont Blochmannia spp. enzymatically breaks down uric acid and urea, and converts urea to both essential and non-essential amino acids (Sauer et al., 2000; Feldhaar et al., 2007). This metabolic capability improves the nutritional intake of the host ants, enables them to persist on otherwise nutrient-deficient diets, and allows them to occupy nutritional niches off-limits to ant community members lacking these endosymbionts (Davidson et al., 2003; Feldhaar et al., 2007; Russell et al., 2009; Hu et al., 2018). The ants’ endosymbiotic ability to process urea as an amino acid precursor may also explain their lack of attraction to other protein sources, such as mouse carrion (Figure 2). Several species of carpenter ants selectively feed on urea (Shetty, 1982; Feldhaar et al., 2007; Menzel et al., 2012), and worker ants of Camponotus terebrans even sift through sand containing urea to acquire it (Petit et al., 2020).
Although our synthetic blend of bird excreta odorants was very complex, it still failed to attract ants in Y-tube olfactometers, indicating that essential constituents were still missing from the blend. These constituents could have been too polar to properly chromatograph [e.g., (bi)acids] or too low in abundance to be detectable in GC-MS analyses.
Over 154 species of ants, including C. modoc carpenter ants (Shean et al., 1993), have been found on or near carrion (Eubanks et al., 2019) but – surprisingly – neither mouse carrion nor cow liver at various stages of decay attracted carpenter ants in our study. The odor profile of decaying carrion dynamically changes in relation to the stage of decay (Dekeirsschieter et al., 2009), and each stage attracts a different guild of scavengers. For example, very fresh carrion attracts blow flies (Brodie et al., 2016), whereas the dry (bone and hair only) stage is attractive to clothes moths (Takács et al., 2001). The carrion stage that is preferentially sought by scavenging ants has not yet been investigated, and we may have presented a suboptimal stage in our bioassays. Alternatively, scavenging ants may prefer invertebrate to vertebrate protein, and vertebrate carrion protein is a suboptimal food source. This explanation is supported by findings that laboratory colonies of S. invicta and Solenopsis geminata had greater brood production and growth when provisioned with insect protein instead of liver protein (Gavilanez-Slone and Porter, 2013; Porter et al., 2015; Lin et al., 2022). It follows that ant assemblies on vertebrate carrion may be motivated primarily by prospective encounters with insect prey, such as fly maggots developing in carrion (Lin et al., 2022). In our study, the liver odor may have masked the (faint) fly maggot odor.
Our prediction that foraging ants are reliant upon a simple olfactory search ‘image’ for nectar odor cues was not supported by the data. The prediction was inspired by previous reports that flightless insects have poor olfactory acuity (Neupert et al., 2020). With a simple search image, ‘featuring’ only those floral constituents that are shared between plants, foraging ants would be able to locate and exploit multiple and diverse nectar sources. This concept seemed particularly appealing because more than 50% of flowering plant families have floral bouquets with overlapping constituents, including α-pinene, benzaldehyde, linalool, and E-β-caryophyllene (Knudsen et al., 2006). E-β-Caryophyllene and α-humulene are shared between fireweed and thimbleberry but this 2-component blend was not attractive to ants. This finding, coupled with (i) reports that ants have hundreds of olfactory receptors (Saad et al., 2018), and (ii) our data showing that foraging carpenter ants were attracted to complete floral odor bouquets of fireweed and thimbleberry but not of hardhack, support the conclusion that carpenter ants recognize specific floral odor blends and discern between them, and thus have good, rather than poor, olfactory acuity.
Western carpenter ants had no prior contact or experience with the carbohydrate and protein sources we tested, indicating innate responses. However, ants in general can learn to associate odors with food rewards (Dupuy et al., 2006; Provecho and Josens, 2009; Nelson et al., 2019; Oberhauser et al., 2019), and thus may be able to opportunistically adjust their foraging activities in accordance with the resources that are currently available in their habitat and that they have learned about. The ants’ disposition to respond to olfactory cues is likely affected by both resource-specific factors such as an optimally attractive stage (see above) and intrinsic ‘ant’ factors such as caste, stage, or hunger (Morgan et al., 2006; Seid and Traniello, 2006; Muscedere et al., 2012; Gadenne et al., 2016). The disposition to respond to olfactory cues is further modulated by shifting barometric pressure (Pellegrino et al., 2013) which could explain the variable number of non-responding ants in our bioassays. Spatio-temporal overlap of food and ant presence, and nutritional value of food, are obvious requisites for odor-mediated foraging responses by ants.
Foragers of Western carpenter ants are attracted to food sources rich in carbohydrates and proteins. The foragers’ ability to sense and orient toward sources of these two macronutrients greatly improves their foraging efficiency. Foragers are not reliant on chance encounters of these resources but can detect them from a distance and move upwind toward them. This ability likely translates into significant energy savings for the entire colony. A complex, rather than simple, olfactory search image seems to guide the foraging activities of ants. They discriminated between odor profiles of three flowering plants, selecting only two (fireweed and thimbleberry), and four protein sources, selecting only one (bird excreta). Moreover, the simple blend of only those two floral odorants shared between fireweed and thimbleberry had no ‘ant appeal.’ All these data support the conclusion that carpenter ants have significant olfactory acuity. With a keen sense of smell, flightless forager ants can efficiently locate valuable nutrient sources and meet the vast and diverse nutritional needs of all their worker nestmates, queen, and developing brood.
The datasets presented in this study can be found in online repositories. The names of the repository/repositories and accession number(s) can be found below: doi: 10.17632/7kj4s38rvn.
AR and GG: conceptualization. AR, RG, SC, CG, GG, JB, and AM: experimental design, methodology, and writing—review and editing. SC, CG, AR, JB, and AM: data collection. RG: analytical chemistry. AR: statistical analysis and graphics. AR and GG: writing—original draft preparation. All authors contributed to the article and approved the submitted version.
This research was supported by a Graduate Fellowship from Simon Fraser University and an Alexander Graham Bell CGSM scholarship from the Natural Sciences and Engineering Research Council of Canada (NSERC) to AR; and an NSERC–Undergraduate Student Research Award (USRA) to CG and a SFU VPR-USRA to SC. This research was further supported by an NSERC–Industrial Research Chair to GG with BASF Canada Inc. and Scotts Canada Ltd. as the industrial sponsors.
The authors declare that this study received funding from BASF Canada Inc. and Scotts Canada Ltd. as the industrial funders. The funders were not involved in the study design, collection, analysis, interpretation of data, the writing of this article or the decision to submit it for publication.
All claims expressed in this article are solely those of the authors and do not necessarily represent those of their affiliated organizations, or those of the publisher, the editors and the reviewers. Any product that may be evaluated in this article, or claim that may be made by its manufacturer, is not guaranteed or endorsed by the publisher.
We thank Michael Gudmundson for field assistance in locating and collecting carpenter ant nests, Grady Ott for generously donating plastic bins for housing carpenter ants, David Lank for providing access to Ruff colonies for collecting bird excreta, Adam Blake and Jaime Chalissery for graphics advice, Laurel Hansen and Robert Higgins for assistance in ant identification, Yonathan Uriel and Eric Maw for assisting with aphid identification, Adriana Ibtisam, Jasper Li, April Lin, Nicholas Low, and Zhanata Almazbekova for help with ant care, Sharon Oliver for some word processing and comments, and two reviewers for constructive feedback.
Agrawal, A. A., and Dublin-Thaler, B. (1998). Induced responses to herbivory in the Neotropical ant-plant association between Azteca ants and Cecropia trees: response of ants to potential inducing cues. Behav. Ecol. Sociobiol. 45, 47–54.
Antoń, S., Denisow, B., Komoń-Janczara, E., and Targoński, Z. (2017). Nectary and gender-biased nectar production in dichogamous Chamaenerion angustifolium (L.) Scop. (Onagraceae). Plant Species Biol. 32, 380–391. doi: 10.1111/1442-1984.12169
Arganda, S., Bouchebti, S., Bazazi, S., Le Hesran, S., Puga, C., Latil, G., et al. (2017). Parsing the life-shortening effects of dietary protein: effects of individual amino acids. Proc. R. Soc. B Biol. Sci. 284, 16–19. doi: 10.1098/rspb.2016.2052
Baroni Urbani, C., Boyan, G. S., Blarer, A., Billen, J., and Musthak Ali, T. M. (1994). A novel mechanism for jumping in the indian ant Harpegnathos saltator (Jerdon) (Formicidae, Ponerinae). Experientia 50, 63–71. doi: 10.1007/BF01992052
Bazazi, S., Arganda, S., Moreau, M., Jeanson, R., and Dussutour, A. (2016). Responses to nutritional challenges in ant colonies. Anim. Behav. 111, 235–249. doi: 10.1016/j.anbehav.2015.10.021
Beugnon, G., Chagné, P., and Dejean, A. (2001). Colony structure and foraging behavior in the tropical formicine ant, Gigantiops destructor. Insectes Soc. 48, 347–351. doi: 10.1007/PL00001788
Blüthgen, N., and Feldhaar, H. (2010). “Food and shelter: how resources influence ant ecology,” in Ant Ecology, eds L. Lach, C. L. Parr, and K. L. Abbot (Oxford: Oxford University Press), 115–136. doi: 10.1093/acprof:oso/9780199544639.003.0007
Blüthgen, N., Gottsberger, G., and Fiedler, K. (2004). Sugar and amino acid composition of ant-attended nectar and honeydew sources from an Australian rainforest. Austral Ecol. 29, 418–429. doi: 10.1111/j.1442-9993.2004.01380.x
Brodie, B. S., Babcock, T., Gries, R., Benn, A., and Gries, G. (2016). Acquired smell? Mature females of the common green bottle fly shift semiochemical preferences from feces feeding sites to carrion oviposition sites. J. Chem. Ecol. 42, 40–50. doi: 10.1007/s10886-015-0658-7
Brodie, B. S., Renyard, A., Gries, R., Zhai, H., Ogilvie, S., Avery, J., et al. (2018). Identification and field testing of floral odorants that attract the rove beetle Pelecomalium testaceum (Mannerheim) to skunk cabbage, Lysichiton americanus (L.). Arthropod. Plant Interact. 12, 591–599. doi: 10.1007/s11829-018-9607-z
Bruna, E. M., Darrigo, M. R., Pacheco, A. M. F., and Vasconcelos, H. L. (2008). Interspecific variation in the defensive responses of ant mutualists to plant volatiles. Biol. J. Linn. Soc. 94, 241–249. doi: 10.1111/j.1095-8312.2008.00962.x
Buehlmann, C., Graham, P., Hansson, B. S., and Knaden, M. (2014). Desert ants locate food by combining high sensitivity to food odors with extensive crosswind runs. Curr. Biol. 24, 960–964. doi: 10.1016/j.cub.2014.02.056
Cardé, R. T., and Willis, M. A. (2008). Navigational strategies used by insects to find distant, wind-borne sources of odor. J. Chem. Ecol. 34, 854–866. doi: 10.1007/s10886-008-9484-5
Cembrowski, A. R., Reurink, G., Arcila Hernandez, L. M., Sanders, J. G., Youngerman, E., and Frederickson, M. E. (2015). Sporadic pollen consumption among tropical ants. Insectes Soc. 62, 379–382. doi: 10.1007/s00040-015-0402-x
Chaudhury, M. F., Zhu, J. J., and Skoda, S. R. (2015). Response of Lucilia sericata (Diptera: Calliphoridae) to screwworm oviposition attractant. J. Med. Entomol. 52, 527–531. doi: 10.1093/jme/tjv054
Clary, K. N., and Back, T. G. (2007). Preparation of unsaturated aminonitriles from the Aza-Morita–Baylis–Hillman reactions of aldimines with penta-2,4-dienenitrile. Synlett 19, 2995–2998. doi: 10.1055/s-2007-990886
Cook, S. C., Eubanks, M. D., Gold, R. E., and Behmer, S. T. (2010). Colony-level macronutrient regulation in ants: mechanisms, hoarding and associated costs. Anim. Behav. 79, 429–437. doi: 10.1016/j.anbehav.2009.11.022
Cornelius, M. L., and Grace, J. K. (1997). Influence of brood on the nutritional preferences of the tropical ant species, Pheidole megacephala (F.) and Ochetellus glaber (Mayr). J. Entomol. Sci. 32, 421–429. doi: 10.18474/0749-8004-32.4.421
Cortez, V., Verdu, J. R., Ortiz, A. J., and Halffter, G. (2017). Identification and evaluation of semiochemicals for the biological control of the beetle Omorgus suberosus (F.) (Coleoptera: Trogidae), a facultative predator of eggs of the sea turtle Lepidochelys olivacea (Eschscholtz). PLoS One 12:e0172015. doi: 10.1371/journal.pone.0172015
Csata, E., and Dussutour, A. (2019). Nutrient regulation in ants (Hymenoptera: Formicidae): a review. Myrmecol. News 29, 111–124. doi: 10.25849/myrmecol.news
Czechowski, W., Marko, B., Eros, K., and Csata, E. (2011). Pollenivory in ants (Hymenoptera: Formicidae) seems to be much more common than it was thought. Ann. Zool. 61, 519–525. doi: 10.3161/000345411X603364
Davidson, D. W., Cook, S. C., Snelling, R. R., and Chua, T. H. (2003). Explaining the abundance of ants in lowland tropical rainforest canopies. Science 300, 969–973. doi: 10.1126/science.1082074
De Vega, C., Arista, M., Ortiz, P. L., Herrera, C. M., and Talavera, S. (2009). The ant-pollination system of Cytinus hypocistis (Cytinaceae), a Mediterranean root holoparasite. Ann. Bot. 103, 1065–1075. doi: 10.1093/aob/mcp049
De Vega, C., Herrera, C. M., and Dötterl, S. (2014). Floral volatiles play a key role in specialized ant pollination. Perspect. Plant Ecol. Evol. Syst. 16, 32–42. doi: 10.1016/j.ppees.2013.11.002
Dekeirsschieter, J., Verheggen, F. J., Gohy, M., Hubrecht, F., Bourguignon, L., Lognay, G., et al. (2009). Cadaveric volatile organic compounds released by decaying pig carcasses (Sus domesticus L.) in different biotopes. Forensic Sci. Int. 189, 46–53. doi: 10.1016/j.forsciint.2009.03.034
Del-Claro, K., Rodriguez-Morales, D., Calixto, E. S., Martins, A. S., and Torezan-Silingardi, H. M. (2019). Ant pollination of Paepalanthus lundii (Eriocaulaceae) in Brazilian savanna. Ann. Bot. 123, 1159–1165. doi: 10.1093/aob/mcz021
Delnevo, N., Van Etten, E. J., Clemente, N., Fogu, L., Pavarani, E., Byrne, M., et al. (2020). Pollen adaptation to ant pollination: a case study from the Proteaceae. Ann. Bot. 126, 377–386. doi: 10.1093/aob/mcaa058
Domisch, T., Finér, L., Neuvonen, S., Niemelä, P., Risch, A. C., Kilpeläinen, J., et al. (2009). Foraging activity and dietary spectrum of wood ants (Formica rufa group) and their role in nutrient fluxes in boreal forests. Ecol. Entomol. 34, 369–377. doi: 10.1111/j.1365-2311.2009.01086.x
Dornhaus, A., and Powell, S. (2010). “Foraging and defence strategies,” in Ant Ecology, eds L. Lach, C. L. Parr, and K. L. Abbot (Oxford: Oxford University Press), 210–230. doi: 10.1093/acprof:oso/9780199544639.003.0012
Dupuy, F., Sandoz, J. C., Giurfa, M., and Josens, R. (2006). Individual olfactory learning in Camponotus ants. Anim. Behav. 72, 1081–1091. doi: 10.1016/j.anbehav.2006.03.011
Dussutour, A., and Simpson, S. J. (2008). Description of a simple synthetic diet for studying nutritional responses in ants. Insectes Soc. 55, 329–333. doi: 10.1007/s00040-008-1008-3
Dussutour, A., and Simpson, S. J. (2009). Communal nutrition in ants. Curr. Biol. 19, 740–744. doi: 10.1016/j.cub.2009.03.015
Dussutour, A., and Simpson, S. J. (2012). Ant workers die young and colonies collapse when fed a high-protein diet. Proc. R. Soc. B Biol. Sci. 279, 2402–2408. doi: 10.1098/rspb.2012.0051
Elgar, M. A., Zhang, D., Wang, Q., Wittwer, B., Pham, H. T., Johnson, T. L., et al. (2018). Insect antennal morphology: the evolution of diverse solutions to odorant perception. Yale J. Biol. Med. 91, 457–469.
Eubanks, M. D., Lin, C., and Tarone, A. M. (2019). The role of ants in vertebrate carrion decomposition. Food Webs 18:e00109. doi: 10.1016/j.fooweb.2018.e00109
Evans, J. D., and Pierce, N. E. (1995). Effects of diet quality and queen number on growth in leptothoracine ant colonies (Hymenoptera: Formicidae). J. N. Y. Entomol. Soc. 103, 91–99.
Feldhaar, H., Straka, J., Krischke, M., Berthold, K., Stoll, S., Mueller, M. J., et al. (2007). Nutritional upgrading for omnivorous carpenter ants by the endosymbiont Blochmannia. BMC Biol. 5:48. doi: 10.1186/1741-7007-5-48
Fischer, C. Y., Lognay, G. C., Detrain, C., Heil, M., Grigorescu, A., Sabri, A., et al. (2015). Bacteria may enhance species association in an ant–aphid mutualistic relationship. Chemoecology 25, 1–10. doi: 10.1007/s00049-015-0188-3
Gadenne, C., Barrozo, R. B., and Anton, S. (2016). Plasticity in insect olfaction: to smell or not to smell? Annu. Rev. Entomol. 61, 317–333. doi: 10.1146/annurev-ento-010715-023523
Gavilanez-Slone, J., and Porter, S. D. (2013). Colony growth of two species of Solenopsis fire ants (Hymenoptera: Formicidae) reared with crickets and beef liver. Fla. Entomol. 96, 1482–1488.
Halaj, J., Ross, D. W., and Moldenke, A. R. (1997). Negative effects of ant foraging on spiders in Douglas-fir canopies. Oecologia 109, 313–322. doi: 10.1007/s004420050089
Hansen, L. D., and Akre, R. D. (1985). Biology of carpenter ants in Washington state. Melanderia 43, 1–65.
Hansen, L. D., and Klotz, J. H. (2005). Carpenter Ants of the United States and Canada. Ithaca, NY: Cornell University Press.
Hu, Y., Sanders, J. G., Łukasik, P., D’Amelio, C. L., Millar, J. S., Vann, D. R., et al. (2018). Herbivorous turtle ants obtain essential nutrients from a conserved nitrogen-recycling gut microbiome. Nat. Commun. 9:964. doi: 10.1038/s41467-018-03357-y
Ibarra-Isassi, J., and Sendoya, S. F. (2016). Ants as floral visitors of Blutaparon portulacoides (A. St-Hil.) mears (Amaranthaceae): an ant pollination system in the Atlantic rainforest. Arthropod. Plant Interact. 10, 221–227. doi: 10.1007/s11829-016-9429-9
Jaffe, K., Caetano, F. H., Sánchez, P., Hernández, J. V., Caraballo, L., Vitelli-Flores, J., et al. (2001). Sensitivity of ant (Cephalotes) colonies and individuals to antibiotics implies feeding symbiosis with gut microorganisms. Can. J. Zool. 79, 1120–1124. doi: 10.1139/cjz-79-6-1120
Kaspari, M. (1993). Removal of seeds from neotropical frugivore droppings–ant responses to seed number. Oecologia 95, 81–88. doi: 10.1007/BF00649510
Knaden, M., and Graham, P. (2016). The sensory ecology of ant navigation: from natural environments to neural mechanisms. Annu. Rev. Entomol. 61, 63–76. doi: 10.1146/annurev-ento-010715-023703
Knudsen, J. T., Eriksson, R., Gershenzon, J., and Ståhl, B. (2006). Diversity and distribution of floral scent. Bot. Rev. 72, 1–120.
Kuriakose, G., Sinu, P. A., and Shivanna, K. R. (2018). Ant pollination of Syzygium occidentale, an endemic tree species of tropical rain forests of the Western Ghats, India. Arthropod. Plant Interact. 12, 647–655. doi: 10.1007/s11829-018-9613-1
Leroy, P. D., Sabri, A., Heuskin, S., Thonart, P., Lognay, G., Verheggen, F. J., et al. (2011). Microorganisms from aphid honeydew attract and enhance the efficacy of natural enemies. Nat. Commun. 2:348. doi: 10.1038/ncomms1347
Lin, C., Tarone, A. M., and Eubanks, M. D. (2022). The discrepancy between fire ant recruitment to and performance on rodent carrion. Sci. Rep. 12:71. doi: 10.1038/s41598-021-04051-8
Luo, C. W., Li, K., Chen, X. M., and Huang, Z. Y. (2012). Ants contribute significantly to the pollination of a biodiesel plant, Jatropha curcas. Environ. Entomol. 41, 1163–1168. doi: 10.1603/EN12042
Macmahon, J. A., Mull, J. F., and Crist, T. O. (2000). Harvester ants (Pogonomyrmex spp.): their community and ecosystem influences. Annu. Rev. Ecol. Syst. 31, 265–291. doi: 10.1146/annurev.ecolsys.31.1.265
Mankowski, M. E., and Morrell, J. J. (2014). Effects of B vitamin deletion in chemically defined diets on brood development in Camponotus vicinus (Hymenoptera: Formicidae). J. Econ. Entomol. 107, 1299–1306. doi: 10.1603/ec13562
Markin, G. P. (1970). Food distribution within laboratory colonies of the argentine ant, Tridomyrmex humilis (Mayr). Insectes Soc. 17, 127–157. doi: 10.1007/BF02223074
Maurer, B., Hauser, A., and Froidevaux, J. C. (1986). (E)-4,8-Dimethyl-1,3,7-nonatriene and (E,E)-4,8,12-trimethyl-1,3,7,11-tridecatetraene, two unusual hydrocarbons from cardamom oil. Tetrahedron Lett. 27, 2111–2112.
Menzel, F., Staab, M., Chung, A. Y. C., Gebauer, G., and Blüthgen, N. (2012). Trophic ecology of parabiotic ants: do the partners have similar food niches? Austral Ecol. 37, 537–546. doi: 10.1111/j.1442-9993.2011.02290.x
Moon, J. K., Kim, J. R., Ahn, Y. J., and Shibamoto, T. (2010). Analysis and anti-Helicobacter activity of sulforaphane and related compounds present in broccoli (Brassica oleracea L .) sprouts. J. Agric. Food Chem. 58, 6672–6677. doi: 10.1021/jf1003573
Morgan, E. D., Keegans, S. J., Tits, J., Wenseleers, T., and Billen, J. (2006). Preferences and differences in the trail pheromone of the leaf-cutting ant Atta sexdens sexdens (Hymenoptera: Formicidae). Eur. J. Entomol. 103, 553–558. doi: 10.14411/eje.2006.075
Moriarty, R. M., and Hou, K. (1984). alpha-Hydroxylation of ketones using o-iodosylbenzoic acid. Tetrahedron Lett. 25, 691–694.
Muscedere, M. L., Johnson, N., Gillis, B. C., Kamhi, J. F., and Traniello, J. F. A. (2012). Serotonin modulates worker responsiveness to trail pheromone in the ant Pheidole dentata. J. Comp. Physiol. A 198, 219–227. doi: 10.1007/s00359-011-0701-2
Nelson, A. S., Acosta, N. C., and Mooney, K. A. (2019). Plant chemical mediation of ant behavior. Curr. Opin. Insect Sci. 32, 98–103. doi: 10.1016/j.cois.2018.12.003
Ness, J., Mooney, K., and Lach, L. (2010). “Ants as mutualists,” in Ant Ecology, eds L. Lach, C. L. Parr, and K. L. Abbot (Oxford: Oxford University Press), 97–114. doi: 10.1093/acprof:oso/9780199544639.003.0006
Neupert, S., McCulloch, G. A., Foster, B. J., Waters, J. M., and Szyszka, P. (2020). Adaptive evolution of olfactory degeneration in recently flightless insects. bioRxiv [Preprint] doi: 10.1101/2020.04.10.035311
Nicolson, S. W. (2011). Bee food: the chemistry and nutritional value of nectar, pollen and mixtures of the two. Afr. Zool. 46, 197–204. doi: 10.1080/15627020.2011.11407495
Nieses, B., and Steglich, W. (1978). Simple method for esterification of carboxylic acids. Angew. Chemie Int. Ed. Eng. 17, 522–524.
Niven, J. E., and Laughlin, S. B. (2008). Energy limitation as a selective pressure on the evolution of sensory systems. J. Exp. Biol. 211, 1792–1804. doi: 10.1242/jeb.017574
Oberhauser, F. B., Schlemm, A., Wendt, S., and Czaczkes, T. J. (2019). Private information conflict: Lasius niger ants prefer olfactory cues to route memory. Anim. Cogn. 22, 355–364. doi: 10.1007/s10071-019-01248-3
Peach, D. A. H., Gries, R., Zhai, H., Young, N., and Gries, G. (2019). Multimodal floral cues guide mosquitoes to tansy inflorescences. Sci. Rep. 9:3908. doi: 10.1038/s41598-019-39748-4
Pekas, A., Tena, A., Aguilar, A., and Garcia-Mari, F. (2011). Spatio-temporal patterns and interactions with honeydew-producing Hemiptera of ants in a Mediterranean citrus orchard. Agric. For. Entomol. 13, 89–97. doi: 10.1111/j.1461-9563.2010.00501.x
Pellegrino, A. C., Peñaflor, M. F. G. V., Nardi, C., Bezner-Kerr, W., Guglielmo, C. G., Bento, J. M. S., et al. (2013). Weather forecasting by insects: modified sexual behaviour in response to atmospheric pressure changes. PLoS One 8:e75004. doi: 10.1371/journal.pone.0075004
Petit, S., Stonor, M. B., Weyland, J. J., Gibbs, J., and Amato, B. (2020). Camponotus ants mine sand for vertebrate urine to extract nitrogen. Austral Ecol. 45, 168–176. doi: 10.1111/aec.12840
Pojar, J., MacKinnon, A., Alaback, P., Antos, J., Goward, T., Lertzman, K., et al. (1994). Plants of Coastal British Columbia: Including Washington, Oregon and Alaska, eds J. Pojar and A. MacKinnon (Vancouver, BC: Lone Pine Publishing).
Porter, S. D. (1989). Effects of diet on the growth of laboratory fire ant colonies (Hymenoptera: Formicidae). J. Kansas Entomol. Soc. 62, 288–291.
Porter, S. D., Valles, S. M., and Gavilanez-Slone, J. M. (2015). Long-term efficacy of two cricket and two liver diets for rearing laboratory fire ant colonies (Hymenoptera: Formicidae: Solenopsis invicta). Fla. Entomol. 98, 991–993.
Provecho, Y., and Josens, R. (2009). Olfactory memory established during trophallaxis affects food search behaviour in ants. J. Exp. Biol. 212, 3221–3227. doi: 10.1242/jeb.033506
R Core Team (2020). R: A Language and Environment for Statistical Computing. Vienna: R Foundation for Statistical Computing.
Renyard, A., Alamsetti, S. K., Gries, R., Munoz, A., and Gries, G. (2019). Identification of the trail pheromone of the carpenter ant Camponotus modoc. J. Chem. Ecol. 45, 901–913. doi: 10.1007/s10886-019-01114-z
Renyard, A., Gries, R., Cooper, S., Gooding, C., Breen, J., Alamsetti, S. K., et al. (2022). R Code and Y-tube Olfactometer Data From: Floral and Bird Excreta Semiochemicals Attract Western Carpenter (London: Mendeley Data). doi: 10.17632/7kj4s38rvn.1
Renyard, A., Gries, R., Lee, J., Chalissery, M., Damin, S., Britton, R., et al. (2021). All sugars ain’t sweet: selection of particular mono-, di- and trisaccharides by western carpenter ants and European fire ants. R. Soc. Open Sci. 8:210804. doi: 10.1098/rsos.210804
Russell, J. A., Moreau, C. S., Goldman-Huertas, B., Fujiwara, M., Lohman, D. J., and Pierce, N. E. (2009). Bacterial gut symbionts are tightly linked with the evolution of herbivory in ants. Proc. Natl. Acad. Sci. U.S.A. 106, 21236–21241. doi: 10.1073/pnas.0907926106
Saad, R., Cohanim, A. B., Kosloff, M., and Privman, E. (2018). GBE Neofunctionalization in ligand binding sites of ant olfactory receptors. Genome Biol. Evol. 10, 2490–2500. doi: 10.1093/gbe/evy131
Sainz-Borgo, C. (2015). Bird feces consumption by fire ant Solenopsis geminata (Hymenoptera: Formicidae). Entomol. News 124, 295–299. doi: 10.3157/021.124.0408
Sauer, C., Stackebrandt, E., Gadau, J., Hölldobler, B., and Gross, R. (2000). Systematic relationships and cospeciation of bacterial endosymbionts and their carpenter ant host species: proposal of the new taxon Candidatus Blochmannia. Int. J. Syst. Evol. Microbiol. 50, 1877–1886. doi: 10.1099/00207713-50-5-1877
Schatz, B., Anstett, M. C., Out, W., and Hossaert-McKey, M. (2003). Olfactive detection of fig wasps as prey by the ant Crematogaster scutellaris (Formicidae; Myrmicinae). Naturwissenschaften 90, 456–459. doi: 10.1007/s00114-003-0457-9
Schettino, M., Grasso, D. A., Weldegergis, B. T., Castracani, C., Mori, A., Dicke, M., et al. (2017). Response of a predatory ant to volatiles emitted by aphid- and caterpillar-infested cucumber and potato plants. J. Chem. Ecol. 43, 1007–1022. doi: 10.1007/s10886-017-0887-z
Schiestl, F. P., and Glaser, F. (2012). Specific ant-pollination in an alpine orchid and the role of floral scent in attracting pollinating ants. Alp. Bot. 122, 1–9. doi: 10.1007/s00035-011-0098-0
Seid, M. A., and Traniello, J. F. A. (2006). Age-related repertoire expansion and division of labor in Pheidole dentata (Hymenoptera: Formicidae): a new perspective on temporal polyethism and behavioral plasticity in ants. Behav. Ecol. Sociobiol. 60, 631–644. doi: 10.1007/s00265-006-0207-z
Shean, B. S., Messinger, L., and Papworth, M. (1993). Observations of differential decomposition on sun exposed v. shaded pig carrion in coastal Washington state. J. Forensic Sci. 38, 938–949.
Shetty, P. S. (1982). Gustatory preferences of ants (Camponotus compressus) for urea and sugars. Experientia 38, 259–260.
Sorensen, A. A., and Vinson, S. B. (1981). Quantitative food distribution studies within laboratory colonies of the imported fire ant, Solenopsis invicta Buren. Insectes Soc. 28, 129–160. doi: 10.1007/BF02223701
Swanson, A. C., Schwendenmann, L., Allen, M. F., Aronson, E. L., Artavia-León, A., Dierick, D., et al. (2019). Welcome to the Atta world: a framework for understanding the effects of leaf-cutter ants on ecosystem functions. Funct. Ecol. 33, 1386–1399. doi: 10.1111/1365-2435.13319
Takács, S., Gries, G., and Gries, R. (2001). Communication ecology of webbing clothes moth: 1. Semiochemical-mediated location and suitability of larval habitat. J. Chem. Ecol. 27, 1535–1546. doi: 10.1023/a:1010486105609
Teders, M., Henkel, C., Anhäuser, L., Strieth-Kalthoff, F., Gómez-Suárez, A., Kleinmans, R., et al. (2018). The energy-transfer-enabled biocompatible disulfide–ene reaction. Nat. Chem. 10, 981–988. doi: 10.1038/s41557-018-0102-z
Terada, Y., Masuda, H., and Watanabe, T. (2015). Structure-activity relationship study on isothiocyanates: comparison of TRPA1-activating ability between allyl isothiocyanate and specific flavor components of wasabi, horseradish, and white mustard. J. Nat. Prod. 78, 1937–1941. doi: 10.1021/acs.jnatprod.5b00272
Tilles, D. A., and Wood, D. L. (1982). The influence of carpenter ant (Camponotus modoc) (Hymentoptera: Formicidae) attendance on the development and survival of aphids (Cinara spp.) (Homoptera: Aphididae) in a giant sequoia forest. Can. Entomol. 114, 1133–1142. doi: 10.4039/Ent1141133-12
Tilles, D. A., and Wood, D. L. (1986). Foraging behaviour of the carpenter ant, Camponotus modoc (Hymenoptera: Formicidae), in a giant sequoia forest. Can. Entomol. 118, 861–867.
Tomberlin, J. K., Crippen, T. L., Wu, G., Griffin, A. S., Wood, T. K., and Kilner, R. M. (2016). Indole: an evolutionarily conserved influencer of behavior across kingdoms. Bioessays 38, 1–12. doi: 10.1002/bies.201600203
Van den Dool, H., and Kratz, P. (1963) A generalization of retention index system including linear temperature programmed gas-liquid partition chromatography. J. Chromatogr A. 11, 463–471. doi: 10.1016/s0021-9673(01)80947-x
Verheggen, F. J., Diez, L., Sablon, L., Fischer, C., Bartram, S., Haubruge, E., et al. (2012). Aphid alarm pheromone as a cue for ants to locate aphid partners. PLoS One 7:e41841. doi: 10.1371/journal.pone.0041841
Wanjiku, C., Khamis, F. M., Teal, P. E. A., and Torto, B. (2014). Plant volatiles influence the African weaver ant-sashew tree mutualism. J. Chem. Ecol. 40, 1167–1175. doi: 10.1007/s10886-014-0512-3
Webster, B., and Cardé, R. T. (2017). Use of habitat odour by host-seeking insects. Biol. Rev. 92, 1241–1249. doi: 10.1111/brv.12281
Weeks, R. D., Wilson, L. T., Vinson, S. B., and James, W. D. (2006). Flow of carbohydrates, lipids, and protein among colonies of polygyne red imported fire ants, Solenopsis invicta (Hymenoptera: Formicidae). Ann. Entomol. Soc. Am. 97, 105–110.
Willmer, P. G., Nuttman, C. V., Raine, N. E., Stone, G. N., Pattrick, J. G., Henson, K., et al. (2009). Floral volatiles controlling ant behaviour. Funct. Ecol. 23, 888–900. doi: 10.1111/j.1365-2435.2009.01632.x
Woodring, J., Wiedemann, R., Fischer, M. K., Hoffmann, K. H., and Völkl, W. (2004). Honeydew amino acids in relation to sugars and their role in the establishment of ant-attendance hierarchy in eight species of aphids feeding on tansy (Tanacetum vulgare). Physiol. Entomol. 29, 311–319. doi: 10.1111/j.0307-6962.2004.00386.x
Yamamoto, M., and Del-Claro, K. (2008). Natural history and foraging behavior of the carpenter ant Camponotus sericeiventris Guerin, 1838 (Formicinae, Campotonini) in the Brazilian tropical savanna. Acta Ethol. 11, 55–65. doi: 10.1007/s10211-008-0041-6
Yusuf, A. A., Crewe, R. M., and Pirk, C. W. W. (2014). Olfactory detection of prey by the termite-raiding ant Pachycondyla analis. J. Insect Sci. 14, 1–10. doi: 10.1673/031.014.53
Zhao, J., Wang, Z., Li, Z., Shi, J., Meng, L., Wang, G., et al. (2020). Development of lady beetle attractants from floral volatiles and other semiochemicals for the biological control of aphids. J. Asia. Pac. Entomol. 23, 1023–1029. doi: 10.1016/j.aspen.2020.08.005
Zhou, A. M., Lu, Y. Y., Zeng, L., Xu, Y. J., and Liang, G. W. (2012). Effects of honeydew of Phenacoccus solenopsis on foliar foraging by Solenopsis invicta (Hymenoptera: Formicidae). Sociobiology 59, 71–80.
Keywords: Hymenoptera, Formicidae, foraging, olfactory cues, semiochemicals, odorants, ant behavior
Citation: Renyard A, Gries R, Cooper SL, Gooding CE, Breen JC, Alamsetti SK, Munoz A and Gries G (2022) Floral and Bird Excreta Semiochemicals Attract Western Carpenter Ants. Front. Ecol. Evol. 10:923871. doi: 10.3389/fevo.2022.923871
Received: 19 April 2022; Accepted: 07 June 2022;
Published: 14 July 2022.
Edited by:
Cesar Rodriguez-Saona, Rutgers, The State University of New Jersey, United StatesReviewed by:
Robert Hanus, Academy of Sciences of the Czech Republic (ASCR), CzechiaCopyright © 2022 Renyard, Gries, Cooper, Gooding, Breen, Alamsetti, Munoz and Gries. This is an open-access article distributed under the terms of the Creative Commons Attribution License (CC BY). The use, distribution or reproduction in other forums is permitted, provided the original author(s) and the copyright owner(s) are credited and that the original publication in this journal is cited, in accordance with accepted academic practice. No use, distribution or reproduction is permitted which does not comply with these terms.
*Correspondence: Asim Renyard, YXNpbS5yZW55YXJkQGdtYWlsLmNvbQ==
†These authors have contributed equally to this work
Disclaimer: All claims expressed in this article are solely those of the authors and do not necessarily represent those of their affiliated organizations, or those of the publisher, the editors and the reviewers. Any product that may be evaluated in this article or claim that may be made by its manufacturer is not guaranteed or endorsed by the publisher.
Research integrity at Frontiers
Learn more about the work of our research integrity team to safeguard the quality of each article we publish.