- 1Department of Biological Sciences, University of Toronto, Toronto, ON, Canada
- 2Ecology and Biodiversity, Faculty of Science, Institute of Environmental Biology, Utrecht University, Utrecht, Netherlands
- 3Department of Biological Sciences, California State University, Los Angeles, Los Angeles, CA, United States
Urbanization is a strong driver of plant diversity and may have complex effects on developed ecosystems. Nevertheless, it remains unclear whether urban environments increase or decrease plant biodiversity compared with rural environments. Further, it is also unclear how non-native plant species contribute to spatial diversity patterns and ecosystem services. Better understanding these diversity drivers across gradients of urbanization has the potential to enhance native species conservation (e.g., targeted restoration activities), leading to positive feedbacks for broader promotion of biodiversity and societal benefits (e.g., links with native biodiversity and human health). In this study, we hypothesized that for plant species in unmanaged grasslands, urbanization would lead to declines in diversity at both small and medium scales. We established a network of remnant grassland sites across an urban to rural gradient in Los Angeles, CA, USA. Across this gradient we assessed patterns of alpha and beta diversity during the 2019 growing season. We found that local plant alpha diversity in remnant grasslands declined in urban landscapes (measured by surrounding percent development) due mostly to loss of native species. However, at intermediate scales across unmanaged parks and greenspaces, we saw increases in beta diversity at more urban locations. This was possibly due to the patchy dominance of different exotic species at urban locations; whereas, in rural locations non-native and native species were common across plots. Conservation is often informed by examinations of large scale, city-wide assessment of diversity, however, our results show that urban plant diversity, particularly native species, is affected at all spatial scales and beta-diversity can add important insights into how to manage urban ecosystems. Conservation that accounts for alpha and beta diversity may promote “virtuous cycle” frameworks where the promotion and protection of biodiversity simultaneously reduces the negative effects of invasion.
Introduction
Urbanization is the most comprehensive form of land-use alteration, resulting in environments that are radically different from less developed areas (Shochat et al., 2006). According to some models, global urban land cover is set to increase by 78 to 171% by 2050 (Huang et al., 2019). Furthermore, cities are often created in biodiversity hotspots, resulting in profound losses of global vegetation land cover (Myers et al., 2000). A shift from natural to urban land cover can result in myriad changes including an increase in impervious surfaces (Stewart and Oke, 2012), reduced soil water absorption and increased flooding (Scalenghe and Marsan, 2018), increased irradiative heating (urban heat islands; Taha, 2017), increased habitat disturbance (Knapp et al., 2008), and changes in soil characteristics (Pickett et al., 2001; Kowarik, 2011).
Beyond the physical implications of urbanization, past work has indicated that urbanization can have strong biotic effects as well. In particular, urbanization may favor invasive exotic species over native species (McKinney, 2006, 2008; Wania et al., 2006; Avolio et al., 2019), but this effect is not consistent and depends on city-specific conditions (Kowarik, 2011). The mechanisms underlying the relationship between urbanization and exotic invasion are still unclear, in part due to many urban biodiversity studies being conducted in highly managed city parks and greenspaces with relatively few studies occurring in unmanaged, remnant urban locations (Avolio et al., 2019; Knight et al., 2021). Developing a better understanding of these mechanisms should be a conservation priority, as in remnant natural areas there can be a high proportion of plant cover by invasive, exotic species (Avolio et al., 2019). There remains a need for robust biodiversity assessments focused on remnant locations.
Here, we focus on three potential mechanisms for increases in exotic species in urban environments. First, exotic species introductions may be higher in urban areas (McKinney, 2006, 2008). Tait et al. (2005) found that in Adelaide, Australia plant species richness increased by 46% from 1836 to 2002 due to the introduction of exotic plant species outpacing extinctions. Second, urban development modifies natural habitats. This modification often results in the loss of native species with high habitat specialization (Knapp and Ingolf, 2009). Third, urban environments may be especially stressful with higher temperatures, increased drought, and widespread pollutants (Calfapietra and Pen, 2015; English and Wright, 2021). This may benefit certain invasive exotics that favor these conditions. For example, species that can exploit anthropogenic nitrogen deposition and tolerate higher thresholds of water stress (Valliere et al., 2017). Past work has shown that exotic Mediterranean grasses (primarily Avena barbata and several species of Bromus) in California may have become particularly invasive due to their ability to tolerate stress and disturbance (D’Antonio et al., 2007). The primary forces that led to these exotic species becoming dominant were better adaptations to drought, intensification of crop agriculture, and the intense year-round grazing pressure that occurred during the 1860s–1880s. Conversely, native species may be more closely adapted to historical conditions and/or less stressful conditions that more closely mirror those found in rural locations.
Exotic species invasions in urban environments are also correlated with both increased and decreased biodiversity. The effect of exotic species appears to depend on the level of urbanization, taxa, biodiversity metric considered (e.g., alpha vs. beta), and other local variables (McKinney, 2006; Schwarz et al., 2017). For example, a moderate level of urbanization (e.g., suburban neighborhoods) may increase the overall number of species (alpha diversity) because exotic species gains outpace native species losses. In fact, past work has shown that plant communities in the transition zone between the urban core and the city outskirts foster the highest levels of diversity (Zerbe et al., 2003). The level of disturbance in the urban core is too high for many plant species to grow (Hahs and McDonnell, 2006; McKinney, 2006) and the rural outskirts experience competitive exclusion from dominant, well-established native species (D’Antonio et al., 2007). Moderately urban areas act as a sort of Goldilocks zone where there is high enough disturbance to disrupt competitive exclusion by dominant species, but not enough disturbance to inhibit the growth of species. Moderately urbanized areas are also often suburban neighborhoods where introductions of exotic species are the highest (due to gardening and horticulture, Kowarik, 1995; McKinney, 2008). Additionally, these urban areas can have high heterogeneity between locations, fostering different plant assemblages at small scales (McKinney, 2008; Clarke et al., 2013). Consequently, we may see the highest levels of alpha diversity (the total number of species at a site) and beta diversity (species turnover between plots) in these suburban areas.
Alternatively, should dominant exotic species respond positively to urbanization, alpha and beta diversity decline with increasing urbanization. Specifically, if all levels of urbanization favor exotic species and these species tend to dominate and outcompete all others (exotic and native), this could drive both alpha and beta diversity down. For example, Southern California experiences unusually high levels of nitrogen deposition such that annual fluxes of nitric oxide (NO) from high-deposition chaparral and forested areas in the Los Angeles air basin are similar to those of fertilized croplands (Fenn et al., 2003). Valliere et al. (2017) found that in Southern California, higher nitrogen deposition reduced native plant cover and concomitantly increased cover and biomass of non-native annuals. For dominant exotic species that respond positively to these environmental changes, urbanization may increase the rate of invasion, and the invasive species may then outcompete remaining natives and/or less stress-adapted exotics.
While alpha diversity may increase or decrease with urbanization intensity, beta diversity is likely highest in suburban environments. Suburban environments support the highest habitat heterogeneity because novel urban habitats are interspersed with historical habitat remnants. The combination of habitat types may maintain or increase species richness of both exotic and native species (Pysek, 1993; Pickett et al., 2001). In addition to habitat heterogeneity, patchy local extinctions may drive variation in species composition in urban habitats (Niemelä, 1999; McKinney, 2002). Extinctions occur due to habitat degradation and destruction, causing species with poor dispersal ability to become isolated or have their habitat patch further decreased in size. Consequently, due to low integration between urban patches, there can be high variation in colonization and extinction at sites resulting in high urban beta diversity.
Typically, urban biodiversity studies are conducted on managed properties (Godefroid and Koedam, 2007; Walker et al., 2009; Avolio et al., 2019). This is standard experimental practice considering the constraints of most western cities: urban developers deconstruct landscapes and replace natural areas with tightly managed non-native vegetation to create lawns and other urban landscapes (Walker et al., 2009; Faeth et al., 2011). The result of this is that urban ecosystems often have very little unmanaged land where species recruitment can naturally occur. However, due to its unique geography, Los Angeles may be an exception. There are 886,443 acres of protected public lands in Los Angeles County, 34% of total County land (Gold et al., 2015). While the abundance of protected area varies with topography, there remains a significant amount of the Los Angeles region that does not have direct human management regimes applied to it and has never been developed. The management of these spaces should be of high concern, as invasion by Mediterranean grasses pose a threat to native species and ecosystems. For example, as of 2018, herbaceous cover represented roughly 31% of the Angeles National Forest (directly adjacent to the city of Los Angeles, CA, USA). This is a high-traffic forest that was historically dominated by native chaparral (Park et al., 2018). A large portion of this invasion is by species in three genera: Avena, Bromus, and Brassica. Species in each of these genera have been shown to reduce the diversity and abundance of native species. Some of these exotic species have a seed bank density an order of magnitude greater than native forbs and shrubs (Cox and Allen, 2008; Abella et al., 2011; Vallejo et al., 2012). Consequently, Los Angeles may be a novel system to examine and develop assessments for natural dynamics of biodiversity along an urban to rural gradient without confounding urbanization with management.
Better understanding the diversity dynamics between native and invasive species across urban gradients has the potential to highlight interventions that could enhance native species (e.g., targeted restoration activities), eventually leading to positive feedbacks for broader promotion of biodiversity and societal benefits (e.g., links with native biodiversity and human health; Dean et al., 2011; Morrison, 2016). While limited, there have been studies linking access to greenspace and access to biodiverse urban locations with the physical, mental, and social health of surrounding communities (Marselle et al., 2021). Given that large, unmanaged grasslands are uncommon in urban areas, the management of native species and biodiversity in these systems could provide novel benefits to the local non-human and human communities.
Here, we assess how exotic species, native species, and overall grassland plant diversity (both alpha and beta) change across an urban-to-rural gradient in Los Angeles, CA, USA. Specifically, we had two objectives: to investigate (a) how urbanization affects different plant diversity metrics and (b) differences in how native and exotic species respond to urbanization. To address these, we established a network of unmanaged grasslands distributed across the greater Los Angeles area. We used this network of grassland patches situated within open spaces across an urban to rural gradient to test the following hypotheses: (H1) native plant species decline and exotic species increase with increasing urbanization, (H2) alpha diversity (Shannon’s index) of plants peaks in moderately developed areas (due to introduction of exotic species outpacing the exclusion of natives) but declines in our most developed areas, and (H3) beta diversity of plants peaks in moderately developed areas due to novel urban conditions increasing the recruitment of novel exotic species in combination with the local extinction of native species.
Materials and methods
Study area
The study area encompassed the greater Los Angeles area, covering over 10,000 km2 ranging from the San Gabriel mountain range to the Santa Ana mountain range to the south, and the Los Angeles county barrier to the east (Figure 1A). All locations exist within a Mediterranean climate associated with moderately wet winters with cool temperatures and dry summers with high temperatures (Gómez et al., 2004). Precipitation during the growing season (November–April) from 1969 to 2018 averaged 614.68 mm and ranged between 167.64 and 1,513.84 mm (PRISM Climate Group, Oregon State University). Mean surface temperature over the same period was 6.9°C and ranged from 4.6 to 8.4°C.
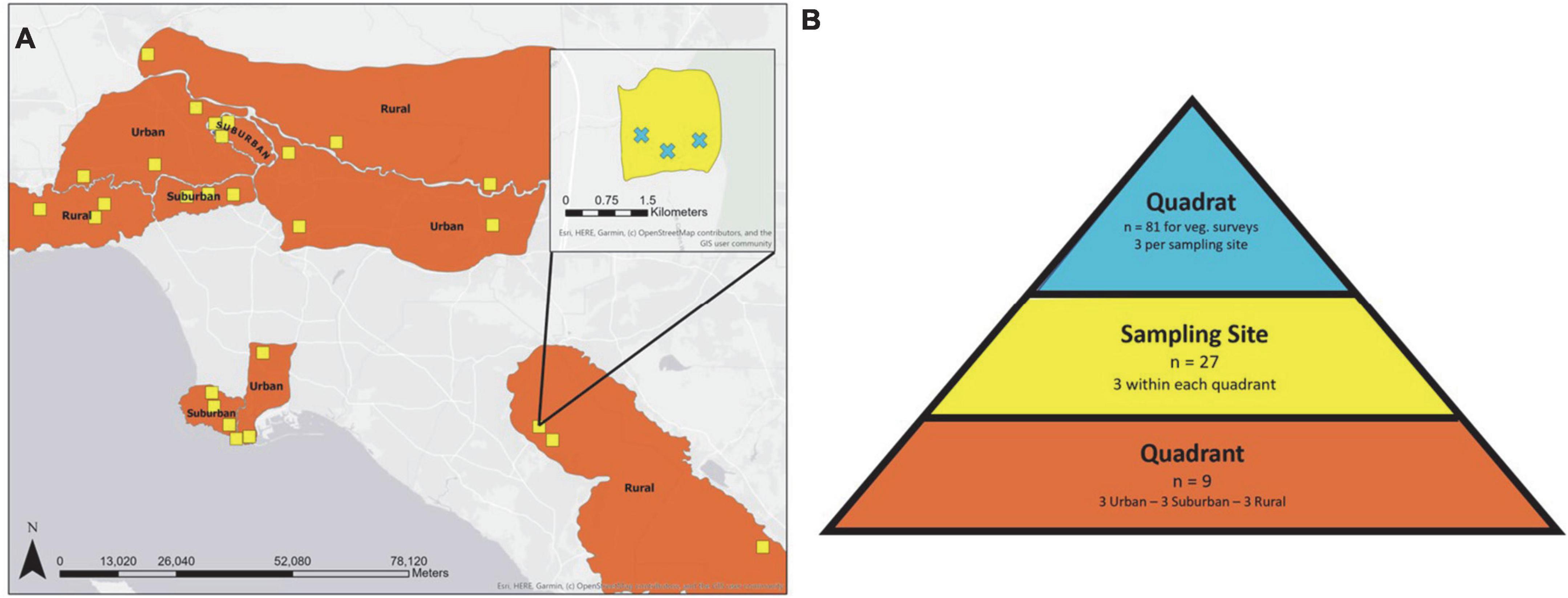
Figure 1. (A) Map of sampling sites across the greater Los Angeles region. Orange polygons depict quadrants, yellow squares depict sampling sites, and blue crosses depict 1 m × 1 m quadrats (only one site shown in figure). (B) Conceptual figure of our sampling design with colors corresponding to panel (A) where orange depicts quadrants, yellow depicts sampling sites, and blue depicts quadrats.
Site selection
Potential field locations were identified using ArcMap (Version 10.5) where we selected within a range of a 5–30% slope, ≤1,200 m elevation, ≥1,400 m2 patch size, and south-facing aspect within Los Angeles County, Orange County, and the western 1/3 of Riverside County. In order to sample across urbanization levels, we identified 16 quadrants throughout the greater Los Angeles region based on neighborhood boundaries. Each of these quadrants was classified based on percent impervious surfaces within the neighborhood polygon. This resulted in a range from rural or undeveloped areas with less than 20% impervious surface area, moderate levels of urbanization ranging from 20 to 50% impervious surface area, to “hardscape” areas with over 50% impervious surface cover. These “hardscaped” areas are highly fragmented and thus we do not have field sites with higher than 66% surrounding impervious surface.
We then randomly chose nine quadrants from the 16 identified: three quadrants were in rural areas, three were in suburban areas, and three were in urban areas. Within each of these quadrants, all green spaces larger than 100 square meters were identified, delineated using GIS, and three local sampling sites were randomly selected from these. Sampling sites were then ground-truthed to confirm that they were unmanaged and unmaintained grasslands. If a site was actively managed by the community or a designated land manager (e.g., mowing or native species planting), it was removed from the dataset and replaced with either a nearby site (first choice) or a newly randomly selected site (if a nearby site was unavailable). This resulted in a total of 27 locations. For each site we also measured elevation and distance to nearest coastline as two other drivers of community composition and diversity along our urban gradient.
Plant diversity survey
In April 2019, we determined species identity and quantified the abundance of all plant species at each of our sites. At each of the 27 locations, three 1 m× 1 m quadrats were selected (for a total of 81 plots across the gradient) using the generate random points tool for our site layer in ArcMaps. In each of these quadrats all plants in all taxa were identified to the species level (Supplementary Table 1). In order to estimate abundance, we visually determined percent cover of each individual species in the plot using a plot grid to increase the accuracy of our estimates. At each of the three plots within a location, we used our species abundance and evenness data to determine plot diversity using the Shannon diversity index (Shannon and Weaver, 1964). We also collected soil cores from a depth of 1 ft with a 1-inch diameter. Soil cores were stored in a freezer after collection and were sent to the UC Davis Analytical Lab in August 2021. Samples were analyzed for nitrogen content in the form of nitrate (NO3) and ammonium (NH4).
Data analysis
We used a model selection approach to assess the best-fitting model for what was driving alpha diversity (Supplementary Equation 1a) and ranked the candidate models with AIC to determine the best-fitting model. This model selection was conducted to account for other environmental factors that may affect the diversity in our plots. We fit linear mixed effects models that included continuous fixed effects of percent development (impervious surface area in a 2 km buffer around each site), elevation (Molina-Venegas et al., 2016), distance from coast (Stromberg et al., 2001), NO3, and NH4 (Valliere et al., 2017) for all of our 27 sites. We included these additional effects to account for other drivers of diversity in Mediterranean grasslands. Additionally, due to our nested design, we included site location nested within quadrant as a random effect given the spatial blocking of our study (plots at each site will have similar conditions to one another and sites located within the same quadrants of the city will have similar conditions; Table 1). Our sites are co-located along the rural to urban gradient because unmanaged grasslands are spatially clumped within this gradient. Because of this design, there is spatial autocorrelation in our sites that is inherently related to the gradient that we are examining. To control for this to the best of our ability, we added blocking variables for the site to the random effect structure of our model. This cannot completely control for spatial autocorrelation but does control for severe autocorrelation within sites. Since percent development and elevation were correlated variables (Supplementary Figure 1), we removed elevation from subsequent analyses while keeping percent development due to the nature of our hypotheses.
To address our first hypothesis, we calculated Spearman’s correlation coefficient on total, native, and the three most common species (Table 2, and Supplementary Equation 1c) against the continuous measure of percent development around each site. These three species (A. barbata, B. diandrus, B. reubens) are invasive exotics known to be detrimental to local ecosystems and were the most abundant across our plots. We wanted to determine if development might be enabling their dominance. Additionally, we ran mixed-effects ANCOVAs using the same random effects structure as above to account for spatial blocking of our study design. We assessed the correlation between our three most dominant invasive species (above) and alpha and beta diversity in our plots.
To address our second and third hypotheses, we analyzed the effects of urbanization on species diversity and abundance, as well as the presence of native and exotic species. We assessed the effects of all fixed and random effects on alpha diversity (Shannon diversity index), beta diversity among the three quadrats at each site (betapart Package in R, version 1.5.1; Orme, 2012), number of native species, and number of exotic species.
Results
Effects of urban gradient
We found a total of 52 species across all grasslands. At each site there were 7.63 ± 2.66 total species, 6.67 ± 1.96 exotic species, and 1 ± 1.32 native species. Our best-fit model showed that the combination of percent development and NO3 best explained alpha diversity (Table 1 and Supplementary Equation 1b). This model was used in all subsequent analyses. We found that percent development had a negative effect on alpha diversity [F(1,12) = 7.24, p = 0.02] and a positive effect on beta diversity [F(1,12) = 4.50, p = 0.055] across our urban gradient (Figure 2).
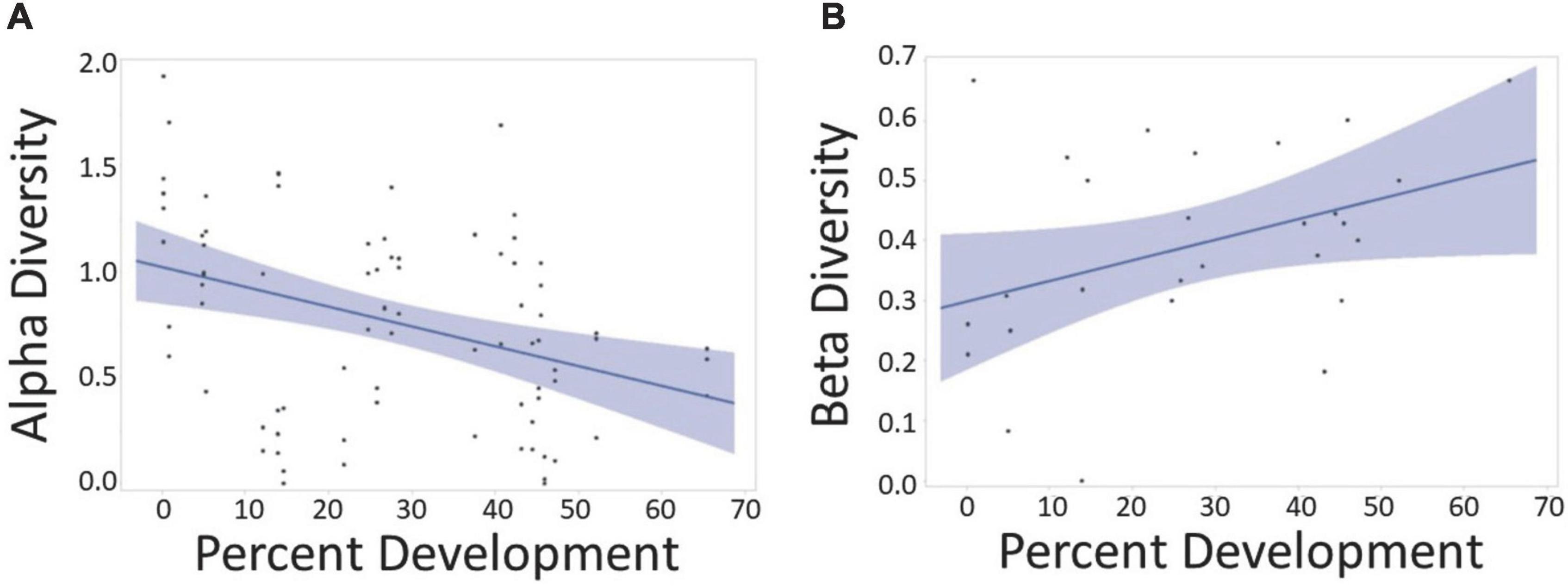
Figure 2. Percent development around a 2 km radius of field sites against alpha and beta diversity. (A) Plot showing alpha diversity where each data point represents Shannon’s diversity at one of the three quadrats at each site. As surrounding percent development increased, alpha diversity decreased [F(1,12) = 7.24, p = 0.020]. (B) Plot showing beta diversity where data points reflect beta diversity across each site. As percent development increased, beta diversity increased [F(1,12) = 4.50, p = 0.055].
The overall number of species in each quadrat was negatively affected by percent development [Figure 3A, F(1,12) = 6.05, p = 0.03]. Native species were negatively affected by percent development [Figure 3B, F(1,12) = 8.70, p = 0.012] whereas exotic species were not affected [Figure 3C, F(1,12) = 3.15, p = 0.10]. There were no other significant relationships between abiotic factors and community composition.
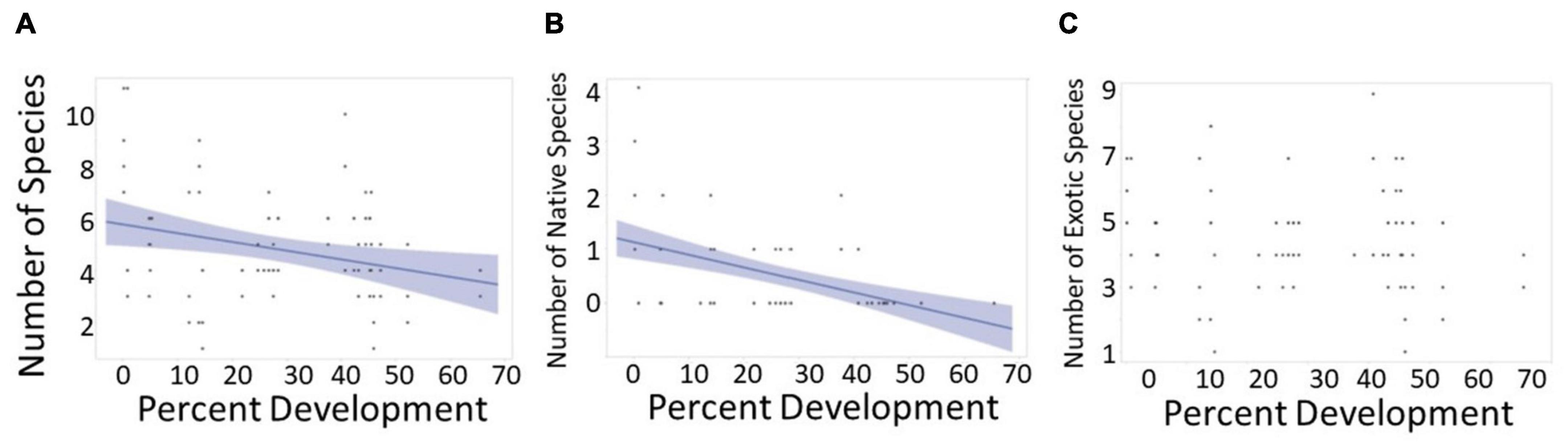
Figure 3. The effect of number of overall species, native species, and most common exotics vs. percent development. (A) Percent development had an effect on the overall number of species at each quadrat [F(1,12) = 6.05, p = 0.030]. Spearman’s correlation coefficient showed this to be a weak negative effect (rs = –0.28, n = 81, p = 0.01]. (B) Percent development had an effect on native species [F(1,12) = 8.70, p = 0.012] which Spearman’s correlation coefficient found to be a moderately strong negative monotonic correlation (rs = –0.47, n = 81, p < 0.001). (C) Percent development did not have an effect on exotic species [F(1,12) = 3.15, p = 0.10].
Species-specific responses to urban gradient
The three most abundant species were all exotic annual grasses (Table 2). The next most abundant species was the exotic annual herb Brassica nigra which covered an average of 1.4% of all quadrats. Two exotic grasses (B. diandrus and B. reubens) were correlated with decreased alpha diversity of the plots (Supplementary Figure 2). None of the invasive exotic grasses were correlated with beta diversity.
Discussion
We found that plant diversity in unmanaged grasslands in Southern California was affected by urbanization. In addressing our first hypothesis concerning how native and exotic plant species respond to urbanization, consistent with previous literature (Avolio et al., 2019), we saw a reduction in native species with increasing levels of development. However, we did not see an increase in exotic species with increasing development in our unmanaged site network. Instead, we saw that exotic species were equally well represented in Southern Californian grasslands at both low and high levels of development. This resulted in an overall reduction in alpha diversity across our gradient as only native species were lost in the more urban sites with no new exotic species appearing in more urban sites, contrary to our expectations (H2). Lastly, our prediction that beta diversity would be highest in suburban areas was moderately supported; we found the highest levels of beta diversity in our most urban sites, though these sites were still more suburban than what past studies likely deemed the urban core (McKinney, 2008).
Contrary to previous studies of plant diversity across managed urban gradients (McKinney, 2008), we did not find that species diversity peaked in moderately developed areas. Comparing our most rural sites to our most urban, there was an average loss of 2.5 species at the site level constituting a 41.6% loss of diversity in our most urban plots. In contrast to other studies of managed urban greenspaces (Walker et al., 2009; La Sorte et al., 2014), remnant grasslands in our study did not appear to have any locally available horticultural species colonizing them (Supplementary Table 1). Additionally, our results differ from studies of urban plant biodiversity in coastal sage scrub communities that show remnant natural areas hosting a majority of native species compared to exotic invasive species (Avolio et al., 2019).
Unmanaged grassland communities across the Los Angeles area consequently appear to be an ecosystem particularly affected by exotic invasion and biotic homogenization. Consequently, Los Angeles offers a unique opportunity to assess the effect of urbanization on ecological assembly in an unmanaged context. Our sites were not specifically managed in any way. Instead, these grasslands are likely undergoing succession just like their rural counterparts, the only difference being the environmental gradients that result from urbanization (e.g., nitrogen, temperature, and habitat fragmentation). This may contribute to the homogenization of urban locations, as exotic species in California grasslands can be very successful at expanding into ranges where resource limitation is alleviated (e.g., urban areas that experience fertilizer runoff and increased nitrogen deposition; Bettez and Groffman, 2013; Eskelinen and Harrison, 2015). The success of exotic species under these urban conditions consequently leads to native species being limited to marginal habitats. Future restoration in these systems should prioritize soil recovery and revegetation to facilitate establishment of native plant species (Beltran et al., 2014). Additionally, this may be evidence of “extinction debt” common to urban areas where native species go locally extinct over long time periods due to disturbance (Hahs et al., 2009; du Toit et al., 2016). The effects of declining habitat connectivity in similar semi-natural grassland diversity have been realized after 50–100 years (Lindborg and Eriksson, 2004), similar to the surrounding landscape history of our field sites.
One other likely reason for the discrepancy between our results and previous studies is that our network of sites included locations with a maximum of 66% development. Thus, our study does not examine the true “urban core” the way past urban biodiversity surveys have (McKinney, 2008; Supplementary Figure 3). We are consequently not capturing truly hardscaped areas given that past studies have included sites up to 95% development (Yan et al., 2019). Furthermore, past surveys of urban diversity have often used qualitative metrics for developments and lack a quantitative measure of development around sites (Hope et al., 2008; Walker et al., 2009; Avolio et al., 2019). The differences in our results may result from differences in how urbanization levels are defined. Additionally, because our unmanaged grasslands are clumped along the rural to urban gradient, there is likely some spatial autocorrelation in our data that we are unable to address. We do not, however, believe that this spatial autocorrelation is likely to alter our results.
We found that beta diversity steadily increased with increasing urbanization across our gradient. This might be driven by patchy extinctions of subordinate species coupled with dominant exotics that dominate across different landscape settings. For example, our most urbanized site was California State University- Dominguez Hills located in the city of Carson. This site is on the campus of a highly developed public university and is surrounded by 65.4% development. At this location, we identified seven species, none of which were native species. However, each of the three plots at this site were dominated by a different exotic species and the subordinate species in this community differed from one quadrat to another, leading to greater beta diversity (Figure 4). Conversely our least developed site was the Santa Rosa Plateau Ecological Preserve in the city of Murrieta. The Santa Rosa Plateau is a preserved area surrounded by 0.2% development. We identified 12 species where 4 were native to the region. There was relatively high diversity in each plot, but also nearly identical species in each of the three quadrats.
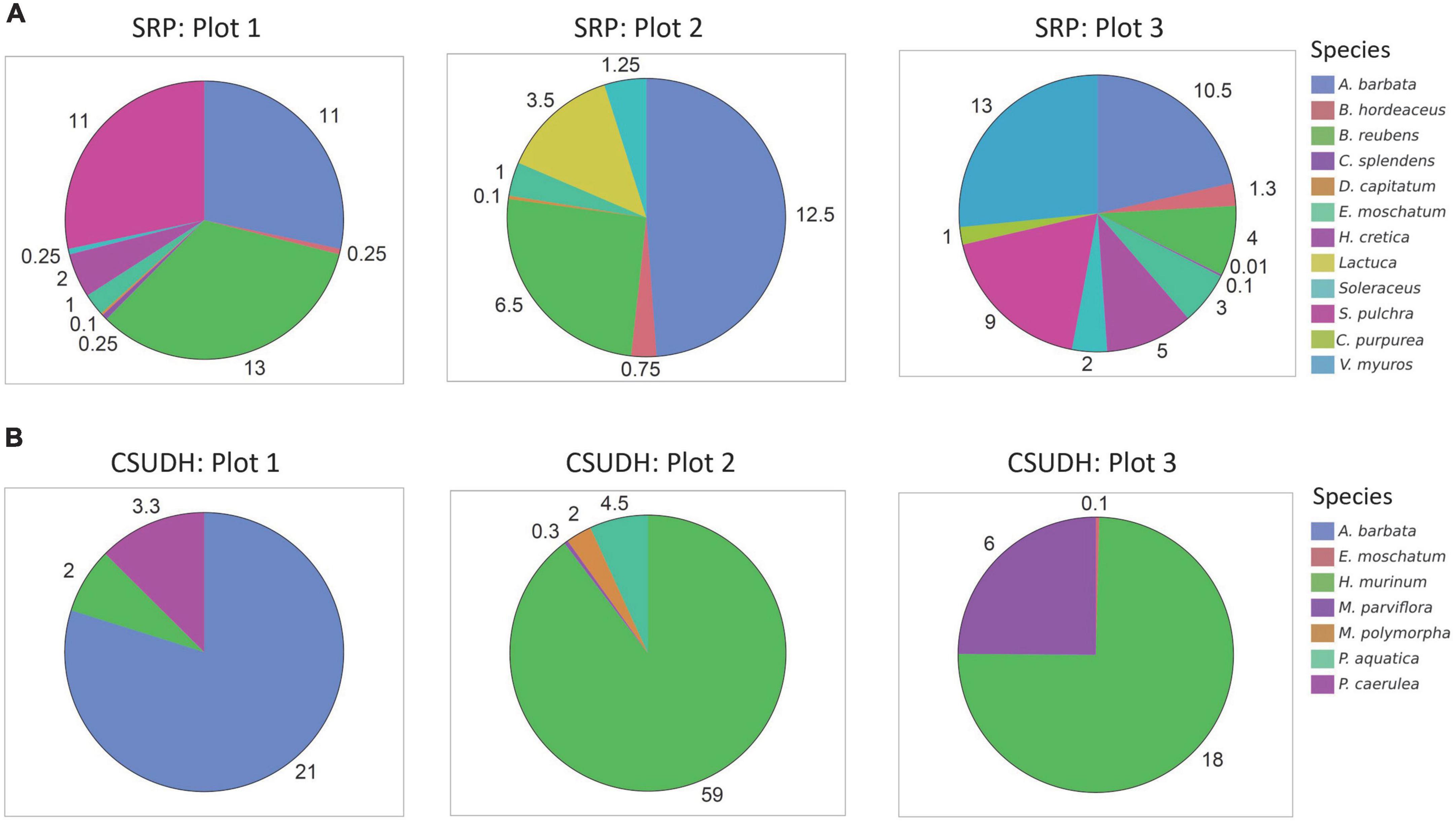
Figure 4. Pie charts showing the species distributions at each quadrat at two of our field sites. (A) Percent abundance of present species at our least developed site, the Santa Rosa Plateau Ecological Reserve. At these plots there was relatively high diversity, but nearly identical species in each of the three quadrats. (B) Percent abundance of present species at our most developed site, California State University- Dominguez Hills. At these plots there were no native species present, with different exotic species dominating quadrats.
While we did not survey the most highly developed areas (e.g., >66% impervious surfaces), our findings are consistent with research showing that moderately developed areas often foster higher levels of beta diversity (Rebele, 1994; La Sorte et al., 2014). Urban landscapes have a large variety of habitat types and ecological communities associated to each (Norton et al., 2016). Further, previous work has shown that rare native species can go locally extinct with urbanization (Kühn and Klotz, 2006) compared to exotic species that show lower levels of turnover (La Sorte et al., 2014). Across our urban gradient we may see an increase in beta diversity as we move from higher levels of species, namely native species, to more urban areas where these species become rare and are thus more likely to go locally extinct. Importantly, our results suggest that fine-scale surveys of beta diversity patterns are essential to our understanding of larger scale patterns of diversity and how to conserve regional diversity. Examinations of urban beta diversity have the potential to spatially inform conservation practices such as protected area selection and where corridor and dispersal facilitation could be beneficial (Socolar et al., 2016).
Future examinations of plant diversity could benefit from including more comprehensive biodiversity metrics not just limited to the inclusion of beta diversity. The inclusion of multiple metrics may allow for more comprehensive and interesting investigations into how different diversity metrics interact with one another. For example, assessments of functional diversity can provide unique insights into ecosystem stability as a supplement to phylogenetic diversity. This can be beneficial as phylogenetic diversity alone may be an imprecise proxy for assessing the functional diversity of urban plant communities (Lososová et al., 2016). In turn, the use of these approaches can aid land managers in supporting conservation efforts following “virtuous cycle” frameworks where the promotion and protection of biodiversity could simultaneously reduce the negative effects of invasion.
We believe the greater Los Angeles area would be an ideal location for these future studies given the unique and interwoven availability of this system. While managed grasslands in the form of yards and parks are more ubiquitous across many urban areas, large unmanaged urban grasslands remain novel. Promoting biodiversity in these areas should be a potential conservation priority given the unique potential they have to provide local communities with a generally inaccessible ecosystem type. Having native species more widely accessible to communities may in turn increase attention to preserving these species, creating a cycle where attention increases availability.
Conclusion
Across remnant grasslands in Los Angeles, our data show that alpha diversity is decreasing across a rural to urban gradient, driven by losses in native species. However, possibly due to stochastic local extinctions, beta diversity in our most urban sites was higher than nearby rural areas. While we can only speculate on the mechanism, native species were negatively affected along our development gradient while the number of exotic species remained constant. Future conservation at these urban locations should prioritize proven restoration efforts such as soil recovery and revegetation to promote native species. Transitioning into more developed areas across our gradient, exotic species were not introduced into these systems in higher proportions but rather those already present appeared to displace native species. We suggest that conservation efforts should utilize multiple biodiversity metrics, including beta diversity, to aid our understanding of how biodiversity patterns operate at different scales and supporting efforts that utilize “virtuous cycle” conservation frameworks.
Data availability statement
The raw data supporting the conclusions of this article will be made available by the authors, without undue reservation.
Author contributions
AW conceived the experiment and provided partial funding. JE and AW established the experimental design. JE collected the data, analyzed the data, and wrote the manuscript. KB and EW advised on data analysis. JE, AW, KB, and EW contributed to manuscript writing. All authors contributed to the article and approved the submitted version.
Funding
This work was supported by a NOAA CIMEC Education Outreach Grant (ID #231688) as well as startup funds provided by California State University, Los Angeles awarded to Alexandra Wright.
Acknowledgments
We thank Brian Hsieh, Daniel Guzman, Bryan Miranda and Miles Kilpatrick for field assistance and data collection. Thanks to Beatriz Aguirre, Regina Mae Francia, and Sam Watson for the support. We thank Steve LaDochy for their comments on the manuscript. We also thank Justin Valliere for help with species identification.
Conflict of interest
The authors declare that the research was conducted in the absence of any commercial or financial relationships that could be construed as a potential conflict of interest.
Publisher’s note
All claims expressed in this article are solely those of the authors and do not necessarily represent those of their affiliated organizations, or those of the publisher, the editors and the reviewers. Any product that may be evaluated in this article, or claim that may be made by its manufacturer, is not guaranteed or endorsed by the publisher.
Supplementary material
The Supplementary Material for this article can be found online at: https://www.frontiersin.org/articles/10.3389/fevo.2022.921472/full#supplementary-material
References
Abella, S. R., Lee, A. C., and Suazo, A. A. (2011). ‘Effects of burial depth and substrate on the emergence of bromus rubens and brassica tournefortii’. Bull. South. Calif. Acad. Sci. 110, 17–24. doi: 10.3160/0038-3872-110.1.17
Avolio, M. L., Pataki, D. E., Jenerette, G. D., Pincetl, S., Clarke, L. W., Cavender-Bares, J., et al. (2019). ‘Urban plant diversity in Los Angeles, California: Species and functional type turnover in cultivated landscapes’. Plants People Planet 2, 144–156. doi: 10.1002/ppp3.10067
Beltran, R. S., Kreidler, N., Van Vuren, D. H., Morrison, S. A., Zavaleta, E. S., Newton, K., et al. (2014). ‘Passive recovery of vegetation after herbivore eradication on santa cruz island, California’. Restor. Ecol. 22, 790–797. doi: 10.1111/rec.12144
Bettez, N. D., and Groffman, P. M. (2013). ‘Nitrogen deposition in and near an urban ecosystem’. Environ. Sci. Technol. 47, 6047–6051. doi: 10.1021/es400664b
Calfapietra, C., and Pen, J. (2015). ‘Urban plant physiology : Adaptation-mitigation strategies under permanent stress’. Trends Plant Sci. 20, 72–75. doi: 10.1016/j.tplants.2014.11.001
Clarke, L. W., Jenerette, G. D., and Davila, A. (2013). ‘The luxury of vegetation and the legacy of tree biodiversity in Los Angeles, CA’. Landsc. Urban Plan. 116, 48–59. doi: 10.1016/j.landurbplan.2013.04.006
Cox, R. D., and Allen, E. B. (2008). ‘Composition of soil seed banks in southern California coastal sage scrub and adjacent exotic grassland’. Plant Ecol. 198, 37–46. doi: 10.1007/s11258-007-9383-9
D’Antonio, C. M., Malmstrom, C., Reynolds, S. A., and Gerlach, J. (2007). “‘Ecology of Invasive Non-native Species in California Grassland’,” in California Grasslands: Ecology and Management, eds M. R. Stromberg, J. D. Corbin, and C. M. D’Antonio (Berkeley: University of California Press), 67–83.
Dean, J., van Dooren, K., and Weinstein, P. (2011). ‘Does biodiversity improve mental health in urban settings?’. Med. Hypotheses 76, 877–880. doi: 10.1016/j.mehy.2011.02.040
du Toit, M. J., Kotze, D. J., and Cilliers, S. S. (2016). ‘Landscape history, time lags and drivers of change: Urban natural grassland remnants in Potchefstroom, South Africa’. Landsc. Ecol. 31, 2133–2150. doi: 10.1007/s10980-016-0386-6
English, J., and Wright, A. J. (2021). The effect of urban temperature gradients on grassland microclimate amelioration in Los Angeles, USA. Appl. Veg. Sci. 1–11. doi: 10.1111/avsc.12556
Eskelinen, A., and Harrison, S. (2015). ‘Erosion of beta diversity under interacting global change impacts in a semi-arid grassland’. J. Ecol. 103, 397–407. doi: 10.1111/1365-2745.12360
Faeth, S. H., Bang, C., and Saari, S. (2011). ‘Urban biodiversity: Patterns and mechanisms’. Ann. N. Y. Acad. Sci. 1223, 69–81. doi: 10.1111/j.1749-6632.2010.05925.x
Fenn, M. E., Baron, J. S., Allen, E. B., Rueth, H. M., Nydick, K. R., Geiser, L., et al. (2003). ‘Ecological effects of nitrogen deposition in the western United States’. Bioscience 53, 404–420. doi: 10.1641/0006-35682003053[0404:EEONDI]2.0.CO;2
Godefroid, S., and Koedam, N. (2007). ‘Urban plant species patterns are highly driven by density and function of built-up areas’. Landsc. Ecol. 22, 1227–1239. doi: 10.1007/s10980-007-9102-x
Gold, M., Pincetl, S., and Federico, F. (2015). ‘2015 Environmental Report Card for Los Angeles County’. Los Angeles: UCLA.
Gómez, L., Zamora, R., Hódar, J. A., and Gómez, J. M. (2004). ‘Applying plan facilitation to forest restoration in mediterranean ecosystems: A meta-analysis of the shrubs as nurse plants’. Ecol. Appl. 14, 1118–1138. doi: 10.1890/03-5084
Hahs, A. K., and McDonnell, M. J. (2006). ‘Selecting independent measures to quantify Melbourne’s urban-rural gradient’. Landsc. Urban Plan. 78, 435–448. doi: 10.1016/j.landurbplan.2005.12.005
Hahs, A. K., McDonnell, M. J., McCarthy, M. A., Vesk, P. A., Corlett, R. T., Norton, B. A., et al. (2009). ‘A global synthesis of plant extinction rates in urban areas’. Ecol. Lett. 12, 1165–1173. doi: 10.1111/j.1461-0248.2009.01372.x
Hope, D., Gries, C., Zhu, W., Fagan, W. F., Redman, C. L., Grimm, N. B., et al. (2008). ‘Socioeconomics drive urban plant diversity’. Proc. Natl. Acad. Sci. U.S.A. 100, 339–347. doi: 10.1007/978-0-387-73412-5_21
Huang, K., Li, X., Liu, X., and Seto, K. C. (2019). ‘Projecting global urban land expansion and heat island intensification through 2050’. Environ. Res. Lett. 14:114037. doi: 10.1088/1748-9326/ab4b71
Knapp, S., and Ingolf, K. (2009). ‘Changes in the functional composition of a Central European urban flora over three centuries’. Perspect. Plant Ecol. Evol. Syst. 12, 235–244. doi: 10.1016/j.ppees.2009.11.001
Knapp, S., Kühn, I., Wittig, R., Ozinga, W. A., Poschlod, P., and Klotz, S. (2008). ‘Urbanization causes shifts in species’ trait state frequencies’. Preslia 80, 375–388. doi: 10.3390/ani11113226
Knight, T., Price, S., Bowler, D., Hookway, A., King, S., Konno, K., et al. (2021). ‘How effective is “greening” of urban areas in reducing human exposure to ground-level ozone concentrations, UV exposure and the “urban heat island effect”? An updated systematic review’. Environ. Evid. 10:12. doi: 10.1186/s13750-021-00226-y
Kowarik, I. (1995). “‘Time lags in biological invasions with regard to the success and failure of alien species’,” in Plant Invasions—General Aspects and Special Problems, eds P. Pysek, K. Prach, and M. W. P. Rejmánek (Amsterdam: SPB Academic), 85–103.
Kowarik, I. (2011). ‘Novel urban ecosystems, biodiversity, and conservation’. Environ. Pollut. 159, 1974–1983. doi: 10.1016/j.envpol.2011.02.022
Kühn, I., and Klotz, S. (2006). ‘Urbanization and homogenization - comparing the floras of urban and rural areas in Germany’. Biol. Conserv. 127, 292–300. doi: 10.1016/j.biocon.2005.06.033
La Sorte, F. A., Myla, A., Nicholas, W., Laura, C.-G., Cilliers, S. C., and Clarkson, B. D. (2014). ‘Beta diversity of urban floras among European and non-European cities’. Glob. Ecol. Biogeogr. 23, 769–779. doi: 10.1111/geb.12159
Lindborg, R., and Eriksson, O. (2004). ‘Historical Landscape Connectivity Affects Present Plant’. Ecol. Soc. Am. 85, 1840–1845.
Lososová, Z., Čeplová, N., Chytrý, M., Tichý, L., Danihelka, J., Fajmon, K., et al. (2016). ‘Is phylogenetic diversity a good proxy for functional diversity of plant communities? A case study from urban habitats’. J. Veg. Sci. 27, 1036–1046. doi: 10.1111/jvs.12414
Marselle, M. R., Cook, P. A., Bonn, A., and Lindley, S. J. (2021). ‘Biodiversity and health in the urban environment’. Curr. Environ. Health Rep. 8, 146–156. doi: 10.1007/s40572-021-00313-9
McKinney, M. L. (2006). ‘McKinney, M. L. Urbanization as a major cause of biotic homogenization. Urbanization as a major cause of biotic homogenization’. Biol. Conserv. 127, 247–260. doi: 10.1016/j.biocon.2005.09.005
McKinney, M. L. (2008). ‘Effects of urbanization on species richness: A review of plants and animals’. Urban Ecosyst. 11, 161–176. doi: 10.1007/s11252-007-0045-4
Molina-Venegas, R., Abelardo, A., Sébastien, L., and Juan, A. (2016). ‘How soil and elevation shape local plant biodiversity in a mediterranean hotspot’. Biodivers. Conserv. 25, 1133–1149. doi: 10.1007/s10531-016-1113-y
Morrison, S. A. (2016). ‘Designing virtuous socio-ecological cycles for biodiversity conservation’. Biol. Conserv. 195, 9–16. doi: 10.1016/j.biocon.2015.12.022
Myers, N., Mittermeier, R. A., Mittermeier, C. G., da Fonseca, G. A., and Kent, J. (2000). ‘Biodiversity hotspots for conservation priorities’. Nature 403, 853–858.
Niemelä, J. (1999). ‘Ecology and urban planning’. Biodivers. Conserv. 8, 119–131. doi: 10.1023/A:1008817325994
Norton, B. A., Evans, K. L., and Warren, P. H. (2016). ‘Urban biodiversity and landscape ecology: Patterns, processes and planning’. Curr. Landsc. Ecol. Rep. 1, 178–192. doi: 10.1007/s40823-016-0018-5
Orme, D. L. (2012). ‘betapart : An R package for the study of beta diversity’. Methods Ecol. Evol. 3, 808–812. doi: 10.1111/j.2041-210X.2012.00224.x
Park, I. W., Hooper, J., Flegal, J. M., and Jenerette, G. D. (2018). ‘Impacts of climate, disturbance and topography on distribution of herbaceous cover in Southern California chaparral: Insights from a remote-sensing method’. Divers. Distrib. 24, 497–508. doi: 10.1111/ddi.12693
Pickett, S. T. A., Cadenasso, M. L., Grove, M. J., Nilon, C. H., Pouyat, R. V., Zipperer, W. C., et al. (2001). ‘Urban ecological systems: Linking terrestria ecological, physical, and socioeconomic components of metropolitan areas’. Annu. Rev. Ecol. Syst. 32, 127–157.
Pysek, P. (1993). ‘Factors affecting the diversity of flora and vegetation in central European settlements’. Vegetatio 106, 89–100.
Rebele, F. (1994). ‘Urban ecology and special features of urban ecosystems’. Glob. Ecol. Biogeogr. Lett. 4, 173–187.
Scalenghe, R., and Marsan, F. A. (2018). ‘The anthropogenic sealing of soils in urban areas’. Landsc. Urban Plan. 90, 1–10. doi: 10.1016/j.landurbplan.2008.10.011
Schwarz, N., Moretti, M., Bugalho, M. N., Davies, Z. G., Haase, D., Hack, J., et al. (2017). ‘Understanding biodiversity-ecosystem service relationships in urban areas : A comprehensive literature review’. Ecosyst. Serv. 27, 161–171. doi: 10.1016/j.ecoser.2017.08.014
Shannon, C., and Weaver, W. (1964). The Mathematical Theory Of Communication. Champaign: The University of Illinois Press.
Shochat, E., Paige, W., Stanley, F., Nancy, M., and Diane, H. (2006). ‘From patterns to emerging processes in mechanistic urban ecology’. Trends Ecol. Evol. 21, 186–191. doi: 10.1016/j.tree.2005.11.019
Socolar, J. B., Gilroy, J. J., Kunin, W. E., and Edwards, D. P. (2016). ‘How should beta-diversity inform biodiversity conservation?’. Trends Ecol. Evol. 31, 67–80. doi: 10.1016/j.tree.2015.11.005
Stewart, I. D., and Oke, T. R. (2012). ‘Local climate zones for urban temperature studies’. Bull. Am. Meteorol. Soc. 93, 1879–1900. doi: 10.1175/BAMS-D-11-00019.1
Stromberg, M. R., Kephart, P., and Yadon, V. (2001). ‘Composition, invasibility, and diversity in coastal California Grasslands’. Calif. Bot. Soc. 48, 236–252.
Taha, H. (2017). ‘Characterization of urban heat and exacerbation: Development of a heat island index for California’. Climate 5:59. doi: 10.3390/cli5030059
Tait, C. J., Daniels, C. B., and Hill, R. S. (2005). ‘Changes in species assemblages within the adelaide metropolitan area, Australia, 1836- 2002’. Ecol. Appl. 15, 346–359.
Vallejo, V. R., Allen, E. B., Aronson, J., Pausas, J. G., Cortina, J., Gutierrez, J., et al. (2012). “Restoration of Mediterranean-Type Woodlands and Shrublands,” in Restoration Ecology: The New Frontier. Second, eds J. van Andel and J. Aronson (Hoboken: Blackwell Publishing Ltd), 130–144. doi: 10.1002/9781118223130.ch11
Valliere, J. M., Irina, I. C., Santiago, L., and Allen, E. B. (2017). ‘High N, dry : Experimental nitrogen deposition exacerbates native shrub loss and nonnative plant invasion during extreme drought’. Glob. Change Biol. 23, 4333–4345. doi: 10.1111/gcb.13694
Walker, J. S., Grimm, N. B., Briggs, J. M., Gries, C., and Dugan, L. (2009). ‘Effects of urbanization on plant species diversity in central Arizona’. Res. Commun. 7, 465–470. doi: 10.1890/080084
Wania, A., Ingolf, K., and Klotz, S. (2006). ‘Plant richness patterns in agricultural and urban landscapes in Central Germany — spatial gradients of species richness’. Landsc. Urban Plan. 75, 97–110. doi: 10.1016/j.landurbplan.2004.12.006
Yan, Z., Teng, M., He, W., Liu, A., Li, Y., and Wang, P. (2019). ‘Impervious surface area is a key predictor for urban plant diversity in a city undergone rapid urbanization’. Sci. Total Environ. 650, 335–342. doi: 10.1016/j.scitotenv.2018.09.025
Keywords: urban ecology, alpha diversity (α), beta diversity (β), conservation, California grasslands
Citation: English J, Barry KE, Wood EM and Wright AJ (2022) The effect of urban environments on the diversity of plants in unmanaged grasslands in Los Angeles, United States. Front. Ecol. Evol. 10:921472. doi: 10.3389/fevo.2022.921472
Received: 15 April 2022; Accepted: 10 October 2022;
Published: 31 October 2022.
Edited by:
Federico Morelli, Czech University of Life Sciences Prague, CzechiaReviewed by:
Piotr Tryjanowski, Poznań University of Life Sciences, PolandJohn Morgan, La Trobe University, Australia
Copyright © 2022 English, Barry, Wood and Wright. This is an open-access article distributed under the terms of the Creative Commons Attribution License (CC BY). The use, distribution or reproduction in other forums is permitted, provided the original author(s) and the copyright owner(s) are credited and that the original publication in this journal is cited, in accordance with accepted academic practice. No use, distribution or reproduction is permitted which does not comply with these terms.
*Correspondence: John English, j.english@mail.utoronto.ca