- 1Guangdong Key Laboratory for Innovative Development and Utilization of Forest Plant Germplasm, College of Forestry and Landscape Architecture, South China Agricultural University, Guangzhou, China
- 2Faculty of Forestry, University of British Columbia, Vancouver, BC, Canada
Nutrient management in Eucalyptus plantations is critical for wood production and sustainable development. The biogeochemical mechanisms in Eucalyptus plantations are not fully understood due to changes in the spatiotemporal pattern of precipitation and plantation management. The nutrients released from litterfall are important sources of soil nutrition. We measured the seasonal production of various litterfall types and the proportions of their released nutrients in Eucalyptus urophylla × E. grandis plantations under compound fertilization, dry-season irrigation, and a combined compound fertilization and dry-season irrigation treatment. Our results showed that fertilization increased aboveground biomass and annual litterfall production (except leaf), and that the peak of litterfall production occurred in the rainy season. We found that the decomposition rates of leaf were significantly higher than that of twig, which were mainly controlled by stoichiometric characteristics, followed by soil enzyme activity (β-glucosidase, urease, and polyphenol oxidase). Fertilization decreased the carbon: nitrogen ratio and carbon: phosphorus ratio in litter, and increased soil enzyme activities, which accelerates litter decomposition and nutrient release. Dry-season irrigation increased litter decomposition and only affected the proportion of released potassium by changing the carbon: potassium ratio. Fertilization and dry-season irrigation accelerated the nutrient cycle to enhance compensatory growth. These results help to comprehend the effects of forest management on litterfall dynamics and decomposition processes in Eucalyptus plantations with seasonal drought.
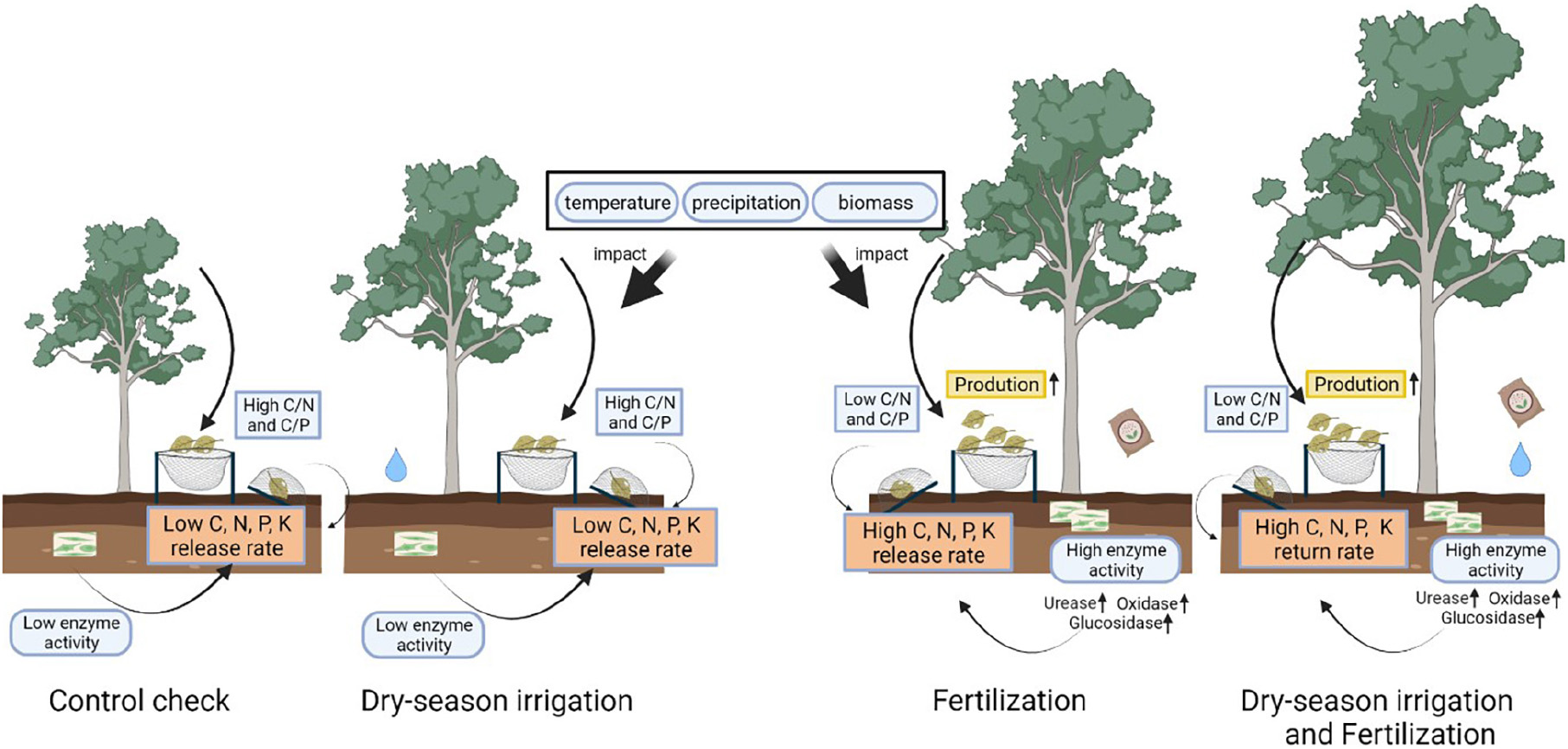
Graphical Abstract. Response of litterfall production and decomposition to fertilization and dry-season irrigation.
Introduction
Litterfall periodically falls to the forest floor and transfers carbon (C) and nutrients into the soil, providing an important biogeochemical process in forest ecosystems (Da Silva et al., 2018; Ni et al., 2021). In tropical forests, over 50% of aboveground net primary productivity is converted to litterfall which transfers nutrient resources to the forest floor, which is beneficial to the sustainable development of forest ecosystems (Camargo et al., 2015; Yin et al., 2019). Additionally, litter in the soil surface can maintain soil fertility by mitigating erosion (Wang et al., 2020) and providing an active environment for soil animals and microorganisms (Cajaiba et al., 2017; Dong et al., 2021). Global climate change has impacted the global hydrological cycle by changing the spatiotemporal pattern of precipitation, water availability, air temperature, air humidity, etc. (Dai, 2013; Dubreuil et al., 2019). Drought can change the nutrient cycle by increasing litterfall production and inhibiting its decomposition rate (Paudel et al., 2015; Yu et al., 2019b). The seasonal drought caused by changes in precipitation pattern (Zhou et al., 2011) in southern China has affected the ecosystem nutrient cycle (Dawoe et al., 2010; Yu et al., 2019b).
Numerous studies show that the shedding process of the aboveground parts of vegetation is affected by vegetation type, species composition, topography, climate, and the physical and chemical properties of soil (Blanco et al., 2008; Tang et al., 2010; Camargo et al., 2015; Kamruzzaman et al., 2019; Gonzalez et al., 2020; Zhu et al., 2021). In the tropical/subtropical forest with seasonal drought, the peak of litterfall production generally occurs in the dry season, which is highly correlated with precipitation (Chave et al., 2010; Paudel et al., 2015). Falling leaves are an important part of litterfall and respond rapidly to environmental changes (Liu et al., 2004). In order to avoid the risk of drought, plants will increase litter production to decrease the loss of water, especially via leaf removal (Liu et al., 2015). In addition, nutrient deficits limit plant growth, resulting in the reduction of litterfall production (Wright et al., 2011). After falling from the plant, litter decomposes in the surface soil and releases nutrients to form organic matter, which is vital to maintaining soil fertility (Cotrufo et al., 2015). Litter decomposition is primarily determined by litter quality, decomposer biodiversity, decomposer activity, and environmental factors. Firstly, different indicators of litter quality determine the decomposability of substrate. The easily decomposed litter is characterized by high nutrient content [nitrogen (N) and/or phosphorus (P)], low stoichiometric characteristics (C:N and/or C:P), and lower recalcitrant polymer content (lignin or holocellulose) (Adair et al., 2008; Mora-Gómez et al., 2020; Hou et al., 2021). Generally, higher contents of soil nutrients were accompanied with high litter quality (Ge et al., 2013). Secondly, environmental factors affect litter decomposition through biodiversity and the activity of soil decomposers. For example the decrease of soil water content caused by drought can reduce the litter decomposition rates by changing the composition of the microbial community and decreasing microbial activity (Petraglia et al., 2019; Liu et al., 2021). Therefore, climate change and forest management (fertilization) not only change the nutrient input from litterfall production, but also affect the released nutrient proportions through litterfall decomposition. Combining litter production and decomposition to assess the nutrient return from litterfall would further realize the variations of soil fertility under the environmental changes.
With its fast growth and high yield, Eucalyptus is widely planted in southern China, covering an area of 4.5 million ha (Xie et al., 2017). Due to the soil in southern China being impoverished (Zhen et al., 2005), compound fertilizers have been applied to meet the fast-growing requirements in the conventionally managed Eucalyptus plantations (Lu et al., 2020). Affected by the fast-growing characteristics and short rotation, the harvesting of Eucalyptus plantations leads to high nutrient export, and results in the decrease of soil fertility (Menegale et al., 2016; Lu et al., 2020). As defined by Li et al. (2020), compensatory growth refers to an organism’s accelerated growth following a disturbance or a period of slow growth caused by unfavorable environmental conditions, such as low temperature or nutrient deprivation. After alleviating nutrient deprivation, compensatory growth caused by fertilization leads to an increase in litterfall production by increasing aboveground biomass (Wright et al., 2011). Moreover, increased litter quality under fertilization positively governs the litter decomposition rates (Hou et al., 2021). Due to Eucalyptus’ high transpiration, fertilizing its plantation will increase transpiration, which reduces soil water content (Hua et al., 2021). The decrease of soil water content has negative effects on aboveground biomass and soil microbial community in Eucalyptus plantations, increasing the litterfall production and reducing the litterfall decomposition (Ribeiro et al., 2002; Nouvellon et al., 2012). Consequently, the reduced growth, increased litterfall production, and decreased decomposition rate are offset or reversed under sufficient water supply. Aboveground biomass is subjected to decreases via drought stress and increases via fertilization. These changes in aboveground biomass can affect the light environment and litter decomposition under the canopy, resulting in further alterations in plantation nutrient cycles (Wright et al., 2011; Liu et al., 2015; Almagro et al., 2017). Nevertheless, the effects of seasonal drought and variations in growth patterns under fertilization on litter deposition are not fully understood. Therefore, exploring the effects of dry season irrigation on nutrient inputs from litterfall in the early growth stage can provide better supporting data for improving nutrient management to ensure healthy growth in the early stage of Eucalyptus plantations under seasonal drought.
In this study, Eucalyptus urophylla × E. grandis, the high-productivity genotypes mainly planted in southern China, was selected to examine the variations of litterfall patterns and decomposition processes under dry-season irrigation and fertilization. The following hypotheses are proposed: (1) the peak of litterfall production occurs in the dry season; (2) fertilization increases the litterfall production, and irrigation decreases the litterfall production; (3) fertilization and irrigation accelerate litter decomposition by increasing litter quality and enzyme activity; (4) fertilization and irrigation promote nutrient release through enhancing soil enzyme activity; and (5) fertilization and irrigation enhance compensatory growth. This research aims to improve forest productivity in Eucalyptus plantations through the enhancement of water and fertilizer management.
Materials and Methods
Study Site
The experimental site is located at the Zengcheng Teaching and Research Bases of South China Agricultural University, Guangzhou (23°14′48N, 113°38′20E), which belongs to a subtropical monsoon climate. According to the data of the Zengcheng meteorological observatory from 1981 to 2010, the annual average temperature is 21.91°C and the annual average precipitation is 2004.4 mm. The seasonal drought in the study site is obvious as its rainfall between October and March only is accounted for 14% of the whole year. The monthly precipitation and the mean air temperature during the experiment are shown in Figure 1.
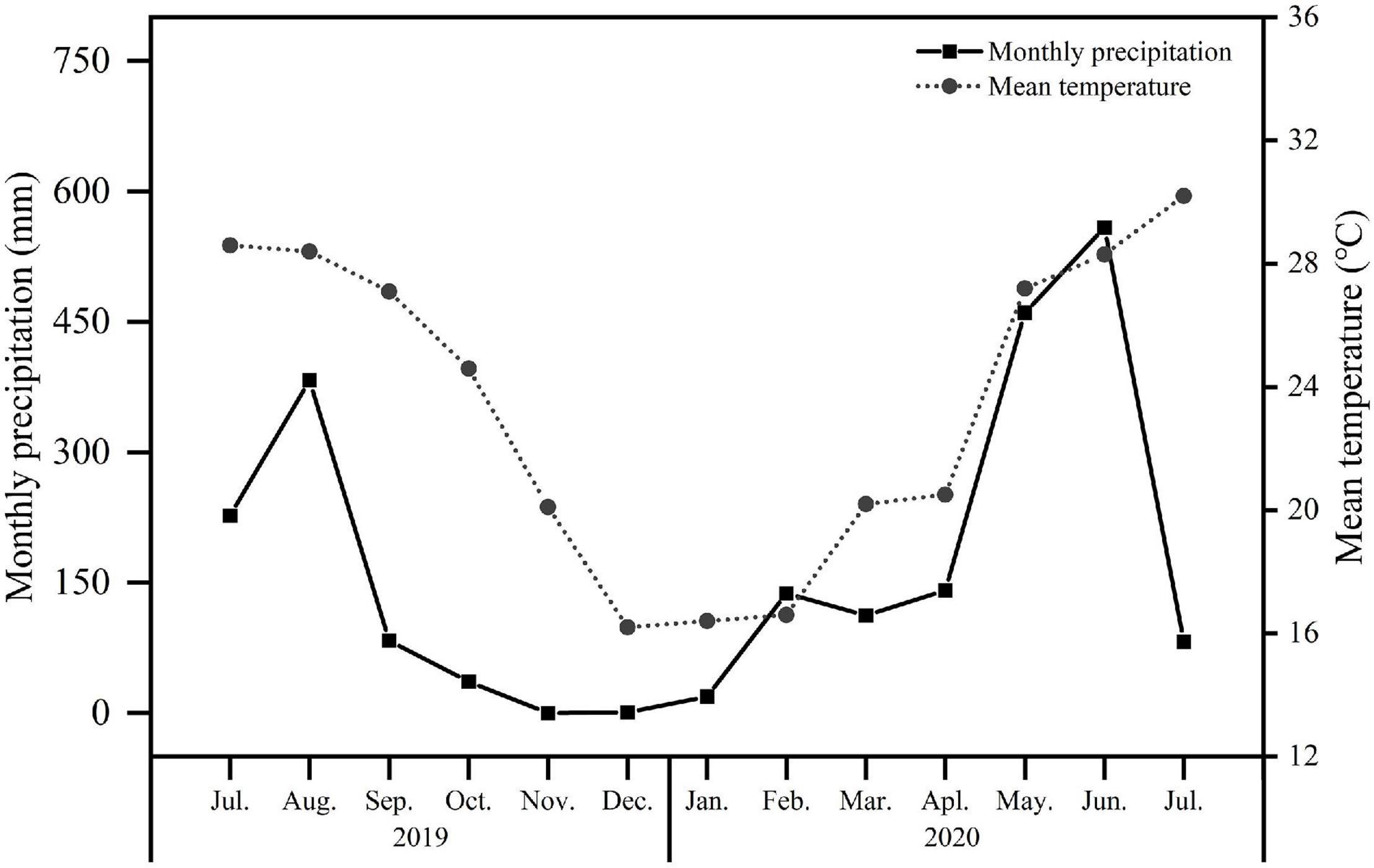
Figure 1. Monthly precipitation and mean temperature during the experimental period from July 2019 to July 2020. The dot with the broken line represented the mean temperature and the square with solid line indicated the monthly precipitation.
Experimental Design
Eucalyptus urophylla × E. grandis was selected as experimental materials and its seedlings (approx. 20 – 35 cm tall) were planted in April 2017 at a density of 3 × 2 m. The forestland was 0.536 ha in total and divided into five terraces with horizontal terraced land preparation method. Each terrace had four treatments (one plot per treatment), which were randomly arranged from 20 to 92 trees for each plot. A total of 20 plots were employed in the present study, and their shapes were described in Yu’s study (Yu et al., 2019a). The experiment was set up as a completely random block design of water and fertilizer. There were four treatments in total: (1) no dry-season irrigation and no fertilizer (CK), (2) dry-season irrigation but no fertilizer (W), (3) no dry-season irrigation but fertilizer (F), and (4) dry-season irrigation and fertilizer. Dry-season irrigation was carried out only from October to March with drip irrigation equipment. Following Yu’s observation (Yu et al., 2019a), the irrigation was given 8 h/week at 4 L/h to ensure the soil relative water content of the soil from 40 cm depth and 40 cm away from the trees was maintained at 90% for 3 days. The fertilizer addition refers to the added amount of Eucalyptus production in southern China (Ministry of Forestry of the People’s Republic of China, 2015). As of July 2020, fertilizer was applied 4 times: base fertilizer, first extra fertilizer, second additional fertilization, and third additional fertilization. The base fertilizer was applied at 400 g/hole in March 2017, which includes N: 24 g, P2O5: 72 g, and K2O: 24 g. The first extra fertilizer was applied at 400 g/hole for F and WF treatments in July 2017, including N: 45 g, P2O5: 21 g, and K2O: 24 g. The second and third additional fertilization for F and WF treatments were, respectively, carried out at 400 g/hole in July 2018 and July 2019, each of which included N: 60 g, P2O5: 28 g, and K2O: 32 g. The initial soil nutrient concentrations (40 cm) were total nitrogen (TN): 0.34 g/kg, total phosphorus (TP): 0.16 g/kg, total potassium (TK): 8.82 g/kg, alkaline hydrolysis nitrogen (AHN): 30.74 mg/kg, available phosphorus (AP): 0.30 mg/kg, and rapidly available potassium (AK): 10.42 mg/kg.
Growth Traits
The diameter at breast height (DBH) and height of all E. urophylla × E. grandis were measured in July 2019 and July 2020. The number of measurements for each treatment were 150 (CK), 189 (W), 146 (F), and 168 (WF). In July 2020, 5 standard trees of each treatment fell to estimate the aboveground biomass. The aboveground part was broken down into stem, twig, leaf, and fruit. All the materials were dried and weighed to calculate the biomass of each organ under various treatments.
Litterfall Deposition and Decomposition
In July 2019, 5 collectors were set in each block using five-point sampling method to measure litterfall deposition, 1 m × 1 m (1 m2) in size, made from mesh gauze (2 mm diameter), with collectors installed at a height of 1 m above the ground to ensure the separation of the mesh gauze from the surface. The litterfall was collected monthly (the end of each month) and classified into organs: leaf, twig, fruit, and bark. The litterfall deposition was monitored from August 2019 to July 2020. All samples were dried in an oven at 65°C to constant weight and then weighed.
In July 2019, fresh litterfall was collected from the plantation’s floor and air-dried to constant weight in a laboratory. Portions of 10 g of leaves and twig samples (cut into 10 cm length) were separately packed in corrosion-resistant plastic bags with 2 mm2 mesh and measuring 25 cm × 25 cm. A 20 leaf bags and 20 twig bags were placed under the litter layer in each plot using five-point methods at the end of July. We planned for 4 successive harvests in each plot during the experiment: 5 leaf bags and 5 twig bags in each plot were collected at 3, 6, 9, 12 months after placing litter bag to measure litter decomposition. After collection, the materials contained in the plastic bags were cleaned with a brush to remove the attachments like weed and soil particles. Afterward, the materials were dried in an oven at 65°C to constant weight (Mt). Leaves and twig litter for each treatment were oven-dried to constant weight at 65°C to determine the ratio between air-dried mass and oven-dried. Then, the ratio was used to convert the weight of initial air-dried litter to the weight of oven-dried litter (M0). The nutrient content of the original samples (C0) and 12 months decomposed samples (Ct) for each treatment were analyzed. Samples for chemical analysis were ground and passed through a 0.5 mm sieve for testing the concentrations of C, nitrogen (N), phosphorus (P), and potassium (K) (refer to soil chemical analysis methods). The mass loss rate (L), proportion of nutrient released (F′), and Olson model were calculated as follows:
Olson negative exponential decay model: y = a × e–kt
In the Olson model: y means the remaining mass rate of litter; a means the fitting parameter; k means the annual decomposition coefficient; t means the decomposition time.
Environmental Factors
The soil water content (SWC) was measured at the end of each month with 15 repeats for each treatment. Soil samples 40 cm deep (40 cm away from the trees) were obtained with a soil drill in three random places of each block. The soil samples were collected in an aluminum box to be weighed and dried at 105°C to constant weight in an oven. Then record the dry weight to compute the SWC. SWC (%) = (fresh weight – dry weight)/(dry weight – box weight) × 100%. The relative soil water content (SWCr) = SWC/field moisture capacity. The field moisture capacity was measured with cutting ring methods (Ministry of Agriculture of the People’s Republic of China, 2010). In July 2019, soil field water-holding capacity of 0 – 20 cm and 20 – 40 cm was measured for each plot. One profile was sampled for each plot, with disturbed and undisturbed soil samples collected from the top soil to 10 and 30 cm. Three undisturbed soil samples were collected from each soil layer with a cutting ring (70 mm diameter and 52 mm height). The disturbed soil samples were collected from the soil around the cutting ring and air dried for 3 days in a laboratory. The undisturbed soil samples were placed in a tray filled with water to a level of 2 – 5 mm below the upper rim of the cutting ring. After air dried for 3 days, the disturbed soil samples sifted by 2 mm sieve and filled with a cutting ring. Next, the undisturbed soil samples were soaked for 24 h, and then put on sand. After the undisturbed soil samples were saturated, the samples were placed on the cutting ring of disturbed soil samples. In order to ensure that the two cutting rings were contacted tightly, a 2 kg brick was placed on the top of the undisturbed soil samples. After the samples were stand for 8 h, 20 – 30 g soil samples from the cutting ring of the wet undisturbed soil samples were collected into an aluminum box of known weight (m0), and then weighed immediately (m1). Finally, the samples in the aluminum box were dried to constant weight (m2) in an oven of 105°C. The formula for field water-holding capacity (FC) is: FC = (m1 – m2) × 1,000/(m2 – m0).
The soil nutrient content was measured once in the dry season (January 2020) and the rainy season (July 2020) with five repeats for each treatment. In each block, a bag of soil samples (0 – 10 cm depth) was taken from 5 places under the litter bags. The soil samples were air-dried for 3 days in the laboratory and then sifted in a mill with 2 mm sieve for testing the concentrations of C, TN, TP, TK, AHN, AP, and AK. Chemical analysis was referenced standard analysis methods (Lu, 2000; Carter and Gregorich, 2007). Organic carbon concentration was determined with the traditional potassium dichromate heating oxidation – volumetric method (Carter and Gregorich, 2007). After sulfuric acid and hydrogen peroxide digestion, the TN concentration was determined by the distillation titration method (Lu, 2000). TP concentration was determined by the vanadium molybdate yellow colorimetric method after digesting with sulfuric acid and hydrogen peroxide (Lu, 2000). TK concentration was determined by flame photometer method after digesting with sulfuric acid and hydrogen peroxide (Lu, 2000). Hydrolytic reduction was carried out in sodium hydroxide and ferrous sulfate solutions, and then the AHN concentration was determined by sulfuric acid titration with boric acid as an indicator (Lu, 2000). After soaking with HCI-HF reagent, AP was determined by the molybdenum antimony colorimetric method. The sample was extracted with ammonium acetate and the AK was determined by flame photometer method (Lu, 2000).
Soil Enzyme Activity
The samples of soil enzyme were collected in January 2020 with 5 repeats for each treatment. In each block, a bag of soil samples (0 – 10 cm depth) was taken from 5 places under the litter bags and stored in an cryogenic container. The soil samples were sifted in a mill with 1 mm sieve in a laboratory. The samples were stored at 4°C and measured within 1 week.
Soil enzyme activity analysis was referenced standard analysis methods. Enzyme activity is expressed as the mass of specific product generated per unit of dry soil weight per unit time. Acid phosphatase activity was analyzed using p-nitrophenyl-phosphate as substrate, and then using a spectrophotometer to measure absorbance at 410 nm to calculate activity (Santos et al., 2018). β -glucosidase activity was analyzed using sodium acetate (pH 5.5) as buffer and using 4-nitrophenyl β-D-glucopyranoside as substrate, and then using a spectrophotometer to measure absorbance at 410 nm to calculate activity (Santos et al., 2018). Urease activity was measured by phenol-sodium hypochlorite colorimetric and assayed at 540 nm, using urea as substrate (Zhen et al., 2019). Using the phenolic amino acid L-3,4-dihydroxy phenylalanine (L-DOPA) as the substrate, polyphenol oxidase activity was analyzed by spectrophotometer at 475 nm (Prosser et al., 2011). Cellulase activity was determined by 3,5-Dinitrosalicylic acid colorimetry Method. The amount of glucose released over 72 h was assayed colorimetrically at 540 nm (Guan, 1986).
Data and Statistical Analysis
Excel 2021 was used for data sorting, SPSS 22.0 was used for ANOVA analysis, Duncan’s multiple comparison analysis, Pearson correlation analysis, and Olson negative exponential decay model. Origin 2021 software was used for principal component analysis (PCA) and making figures.
The annual litterfall production (total or each type) was calculated by adding up the amount of monthly litterfall production in each block. After using Grubbs method to delete outliers, one-way ANOVA following Duncan’s multiple comparison analysis (normal distribution and variance homogeneity of the data have tested) was used to test the effects of treatment on litterfall production, aboveground biomass, percentages of litter remaining mass, C and nutrient concentrations, proportion of C and nutrient released, and soil enzyme activity. Pearson correlation coefficients were estimated between environmental factors and monthly litterfall productions, litter remaining mass, and soil/litter chemical traits, litter nutrient released proportion and soil/litter chemical traits. The Pearson correlation analysis, Olson negative exponential decay model, and PCA were made using the average per plot (n = 5).
Results
Biomass
Fertilization increased the biomass of leaf, twig, and stem 3 years after plantation (Table 1), while dry-season irrigation increased the biomass of stem (P < 0.05). The biomass of stem under dry-season irrigation showed further increase by 14.7% on the basis of fertilization treatment (P < 0.05).
Litterfall Deposition
In E. urophylla × E. grandis plantations under different treatments, the average litterfall productions ranged from 6852.8 kg/ha/yr under CK treatment to 8720.3 kg/ha/yr under WF treatment (Figure 2). The total litterfall productions with fertilizer addition (F and WF) were higher than that with no fertilizer (P < 0.05). Dry-season irrigation had no effect on litterfall production (P > 0.05). Dry-season irrigation increased litterfall production but without forming significant differences. The leaf fraction showed the highest contribution in total litterfall production (average 4733.9 kg/ha/yr), without statistical differences between various treatments. The second largest contribution to total litterfall production was twig (average 1517.5 kg/ha/yr). Fertilization increased the twig (61–71%) and fruit (77–93%) production, while the twig and fruit production did not differ under dry-season irrigation. Barks were significantly different between fertilization and non-fertilization. The PCA depicted similarities/differences between treatments: there were obvious differences between fertilizer (F and WF) and no fertilizer (CK and W), and a small difference between dry-season irrigation and no dry-season irrigation (Figure 3).
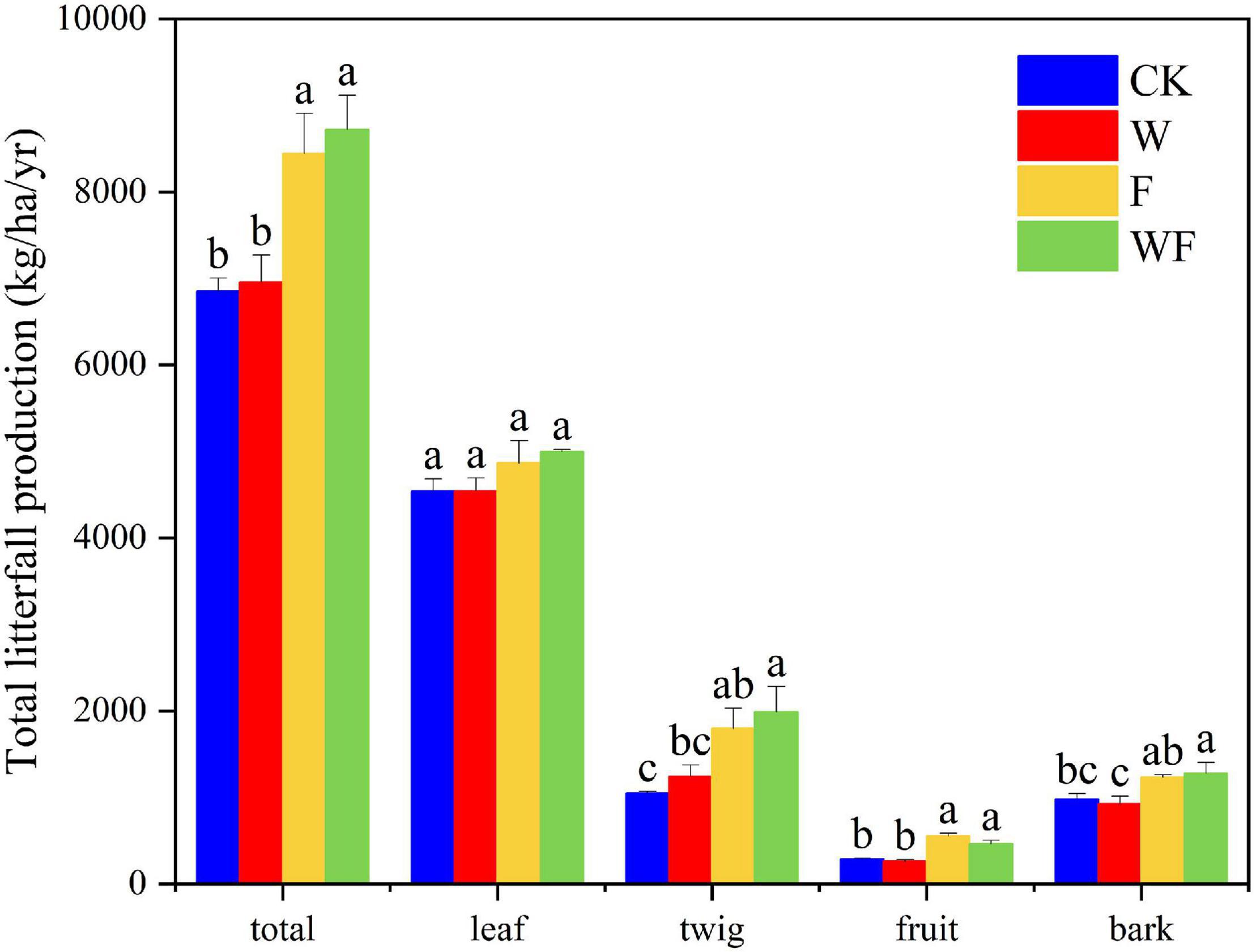
Figure 2. Total litterfall production and components (kg/ha/yr) of E. urophylla × E. grandis from August 2019 to July 2020. The bars in different colors, respectively, indicated CK, W, F, and WF treatment. The error bars indicated SE. The values followed by the different letters are significantly different according to one-way ANOVA (P < 0.05).
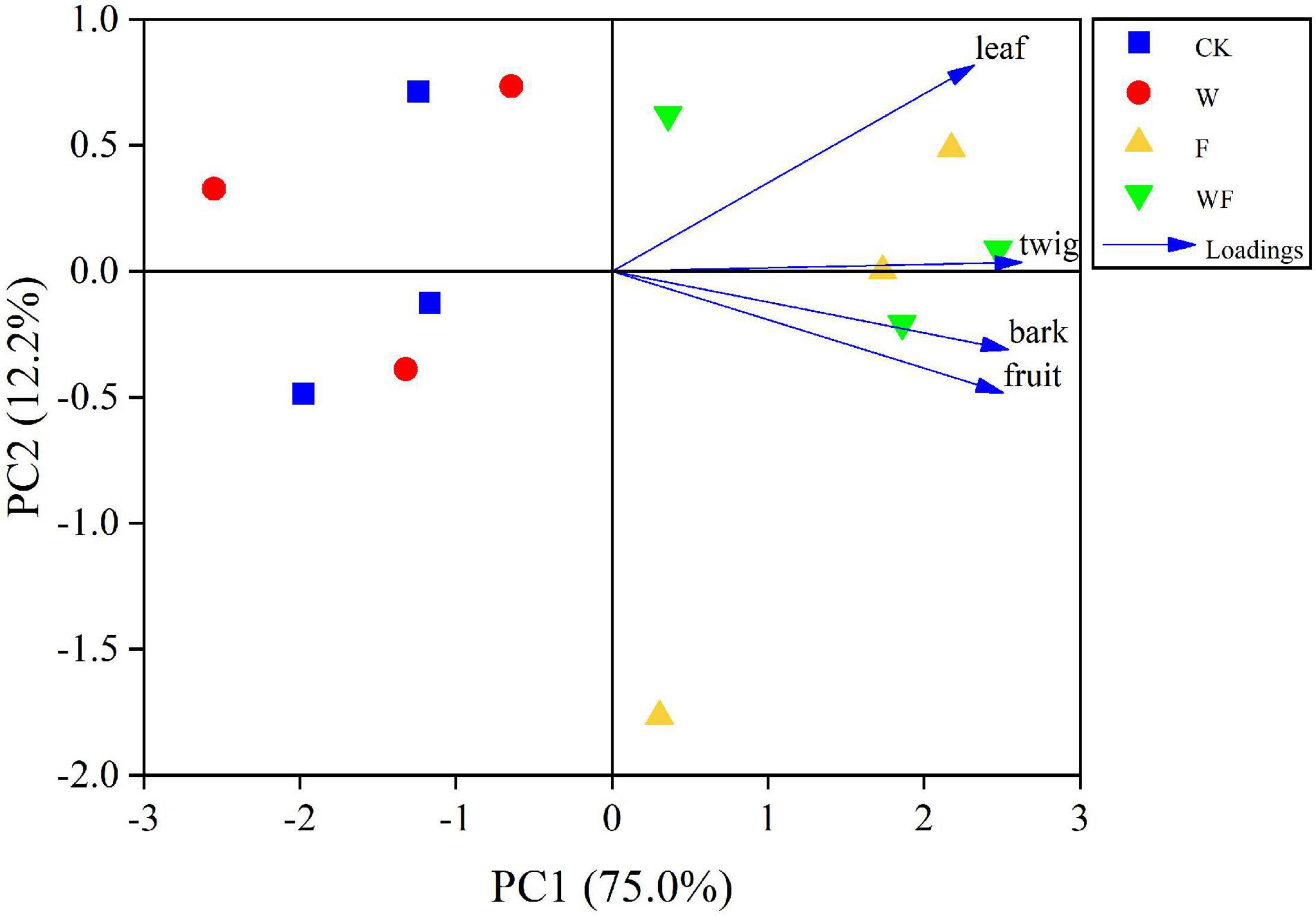
Figure 3. Principal component analysis (PCA) of annual litterfall production (leaf, twig, fruit, and bark) under different treatments.
The litterfall production of all treatments followed a seasonal pattern throughout the experimental period (Figure 4). The deposition over 72% occurred in the rainy season (from April to September), strongly affected by the leaf and twig productions (Supplementary Figure 1). The productions of leaf, twig, and bark peaked in May and fruit in July. In particular, fruit and bark fall rarely occur during the dry season, while leaf and twig are maintained at a low level (Figure 4 and Supplementary Figure 1). In Pearson correlation analysis, litterfall deposition showed positive correlations with temperature and precipitation (Table 2). Temperature had positively correlated with twig and fruit production (P < 0.05), which was not affected by dry-season irrigation and fertilization. Precipitation had positively correlations with total, leaf, twig, and bark production, but the correlation with leaf production disappeared under dry-season irrigation and fertilization.
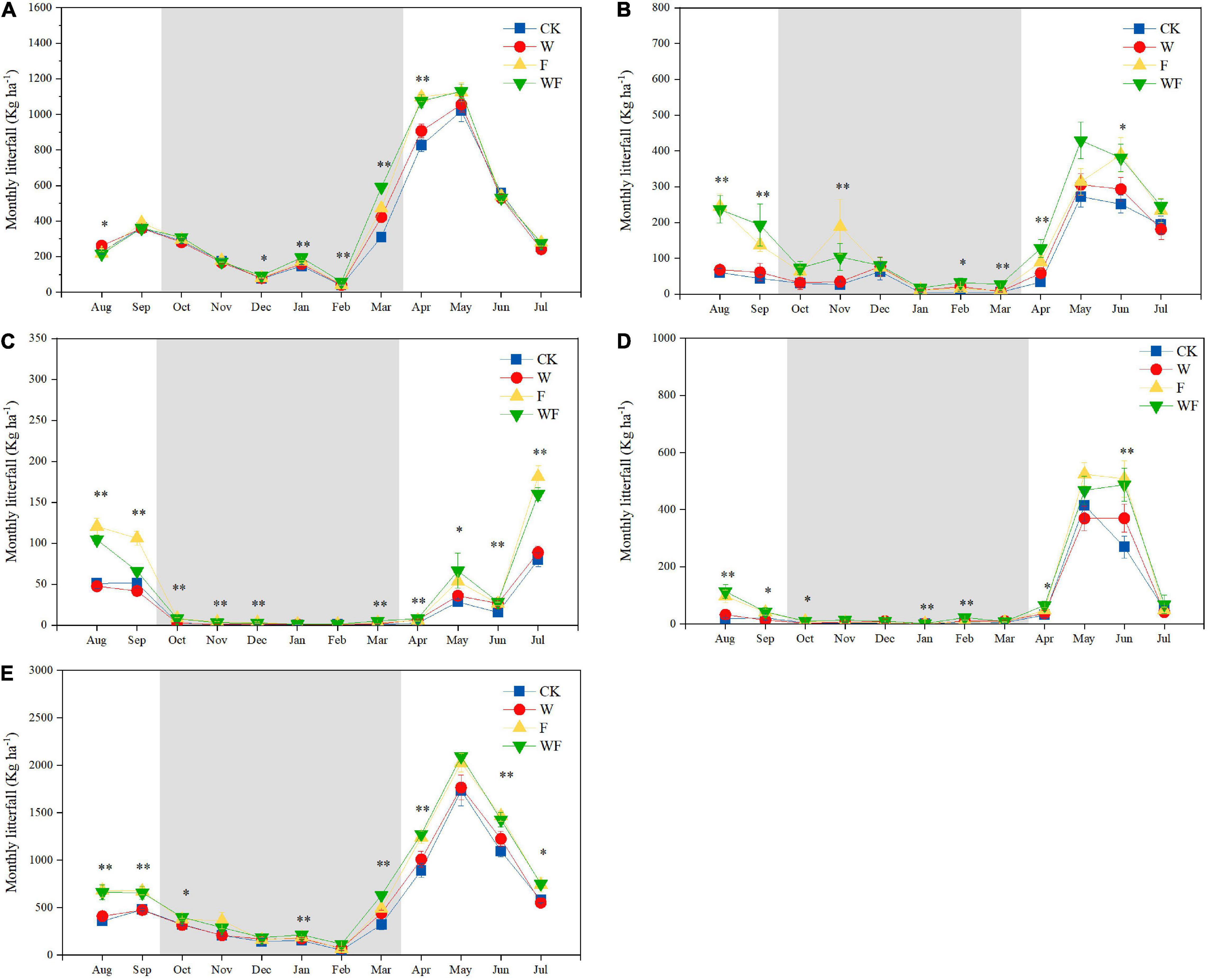
Figure 4. Monthly leaf (A), twig (B), fruit (C), bark (D), and total (E) litter deposition of E. urophylla × E. grandis from August 2019 to July 2020. The gray areas represent the months in the dry season. The P-value showed the results from one-way ANOVA testing for the effects of dry-season irrigation and fertilization, ∗P < 0.05, ∗∗P < 0.01.
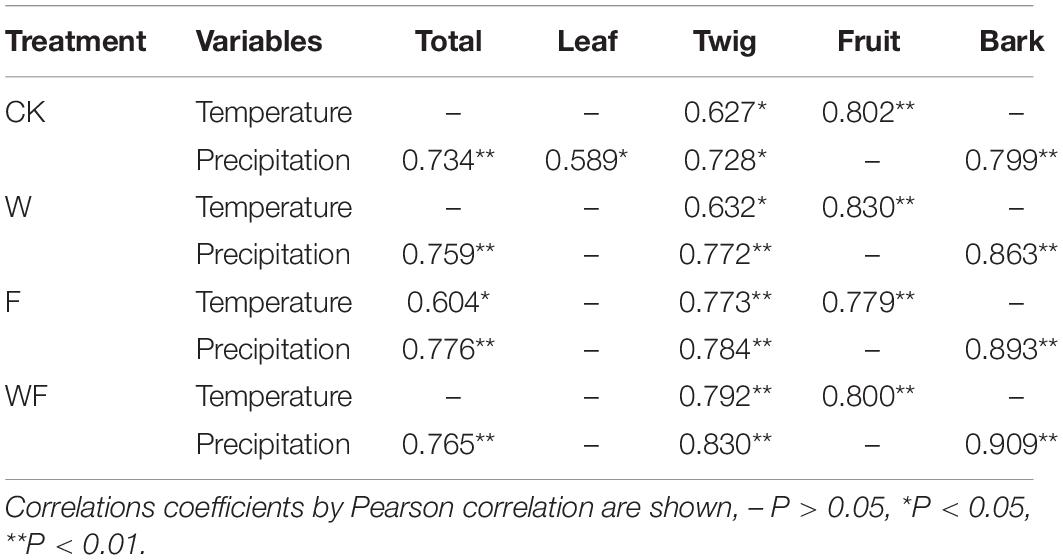
Table 2. Analysis of the correlation between environmental factors and monthly litterfall productions from August 2019 to July 2020.
Litter Decomposition
The Olson negative exponential decay model adequately described the decomposition rates of leaf and twig under different treatments, with R2 values ranging from 0.70 to 0.98 (Table 3). The decomposition rates of the twig were lower than that of the leaf. In the first 90 days, the rates of decomposition under all treatments were more intense than in other periods (Figure 5). After 360 days of decomposition, the average litter decomposition rates of leaf and twig for all treatments were 30 and 12%. Different treatments had significant influences on litter decomposition. Fertilization and dry-season irrigation were beneficial to leaf and twig decomposition (Figure 5), of which under WF treatment attained the highest rates of decomposition with 34% (leaf) and 15% (twig), respectively, after 360 days (P < 0.05). According to the Olson model, the time required to decompose 95% of leaf and twig had been, respectively, reduced by 16.8 to 34% and 20.8 to 43.8% under fertilization and dry-season irrigation. In the experimental period, the remaining mass negatively correlated with initial C, N, P, and K concentration, and positively correlated with C/N, C/P, and C/K (Table 4). In addition, leaf decomposition was related to the activity of β -glucosidase, Urease, and Polyphenol oxidase in the dry season (P < 0.05), while twig decomposition was related to the activity of Urease and Polyphenol oxidase (Table 5).
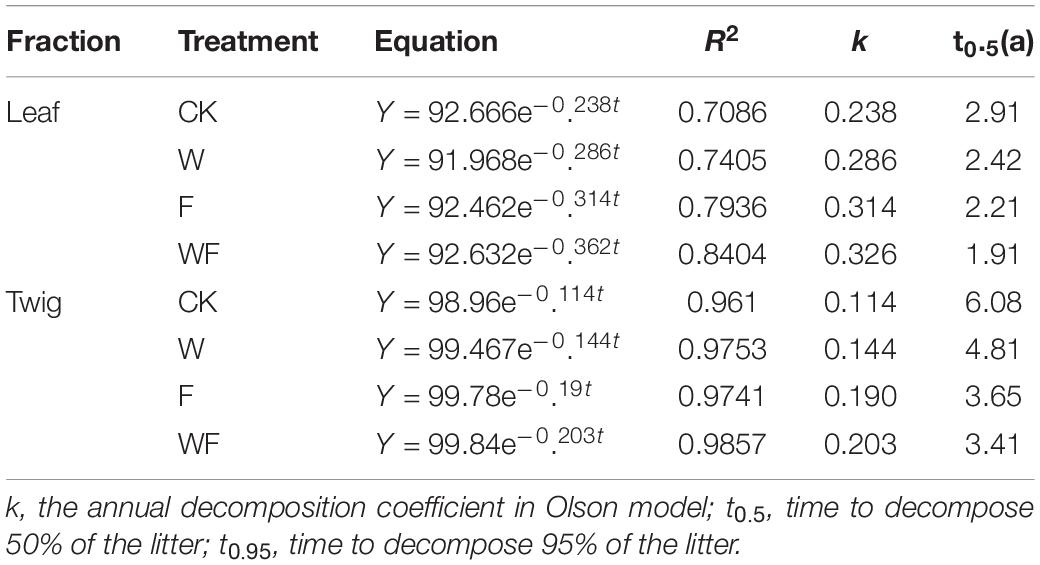
Table 3. Decomposition equations of Olson negative exponential decay model of leaf and twig for all treatments.
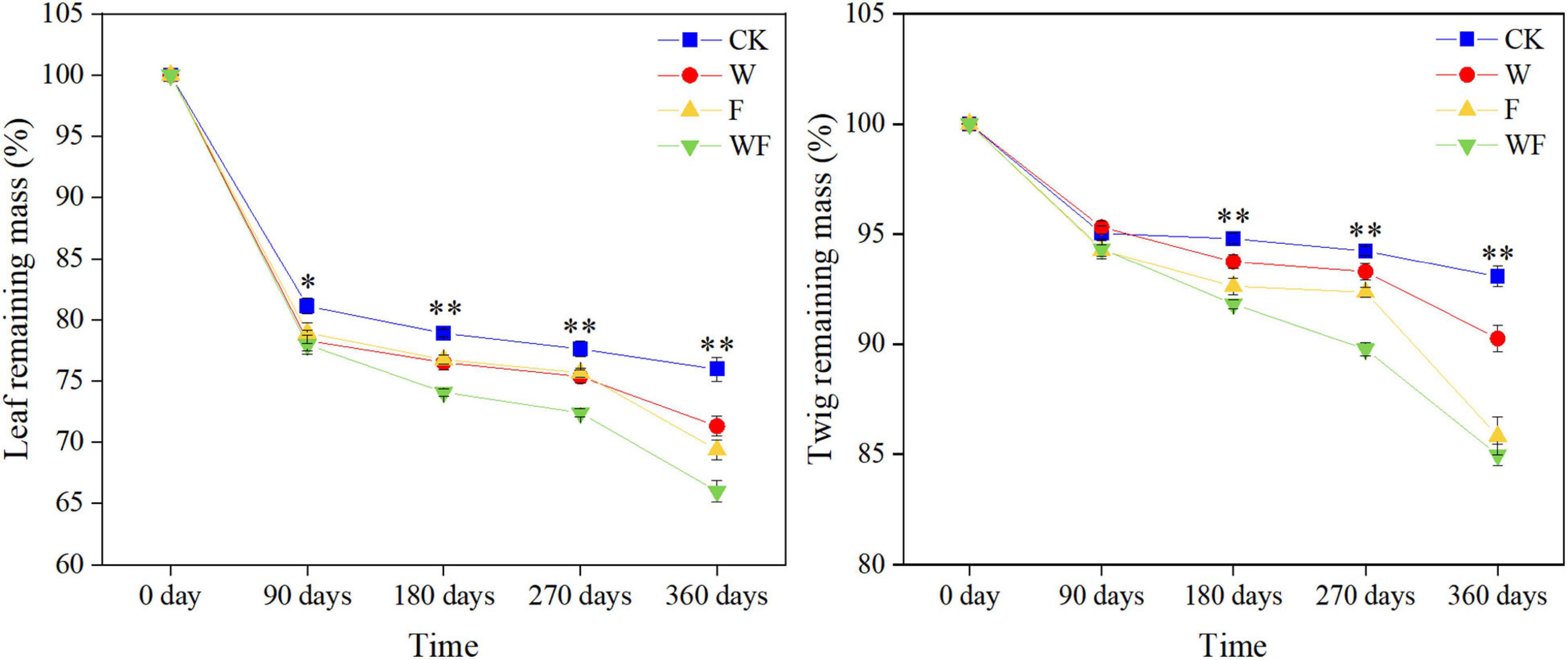
Figure 5. Percentages of remaining mass in leaf and twig under different treatments during the experimental period. The P-value showed the results from one-way ANOVA testing for the effects of dry-season irrigation and fertilization, ∗P < 0.05, ∗∗P < 0.01.

Table 4. Pearson correlation analysis between litter remaining mass (leaf and twig) and initial chemical traits.

Table 5. Pearson correlation analysis between the percentage of remaining mass and enzyme activity of surface soil (10 cm) in the dry season.
Nutrients Released
Most of initial litter C and nutrient concentrations were influenced by fertilization, but not by dry-season irrigation (Table 6). Initial foliar C concentrations increased by 2.6 – 2.7% under fertilization (P < 0.05). Affected by the increase of P concentrations in leaf (26.1 – 27.8%) and twig (48.6 – 65.6%), C/P under fertilization were decreased (leaf, 18.8 – 20.1%; twig, 30.4 – 37.3%). Compared to CK, dry-season irrigation increased foliar K concentration by 55.9 – 58.5%, while fertilization increased the K concentrations of twig by 27.0 – 39.7% (P < 0.05). After decomposing 360 days, C, N, and P concentrations greatly decreased, and their difference narrowed between treatments. Meanwhile, the K concentration of twigs decreased immensely, but foliar K concentrations change little.
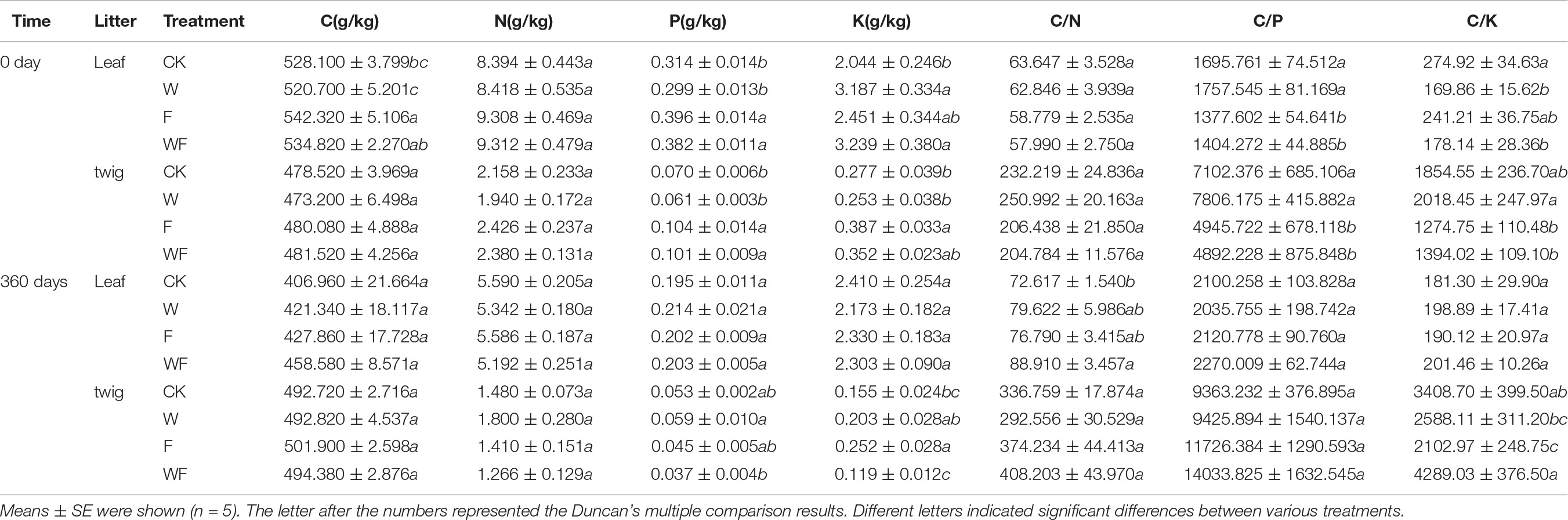
Table 6. C and nutrient concentrations, C/N and C/P ratio of leaf and twig litter under various treatments after 0 and 360 days decomposition.
After 360 days of decomposition, the quantities of C, N, P, and K in leaf and twig decreased for both treatments (Figure 6). In leaf litter, the released proportions of N (16.2 – 18.2%) and P (22.7 – 31.5%) under fertilization were higher than that under non-fertilization (P < 0.05). Compared to non-fertilization, fertilization increased the C release proportion by 111.2 – 145.4%, N release proportion by 46.6 – 72.9%, and P release proportion by 121.5 – 136.4% of twig (P < 0.05), respectively. In particular, the C/N of WF in leaf litter was higher than that of CK (22.4%) and the C/P of WF in twig litter was higher than that of CK (49.7%) and W (34.3%). The released proportions of K were different from that of C, N, and P. Fertilization and dry-season irrigation promoted the release of foliar K, and the effect of dry-season irrigation was greater than fertilization. The release of twig K was inhibited by dry-season irrigation, but the combination of dry-season irrigation and fertilization promoted the release of twig K (P < 0.05).
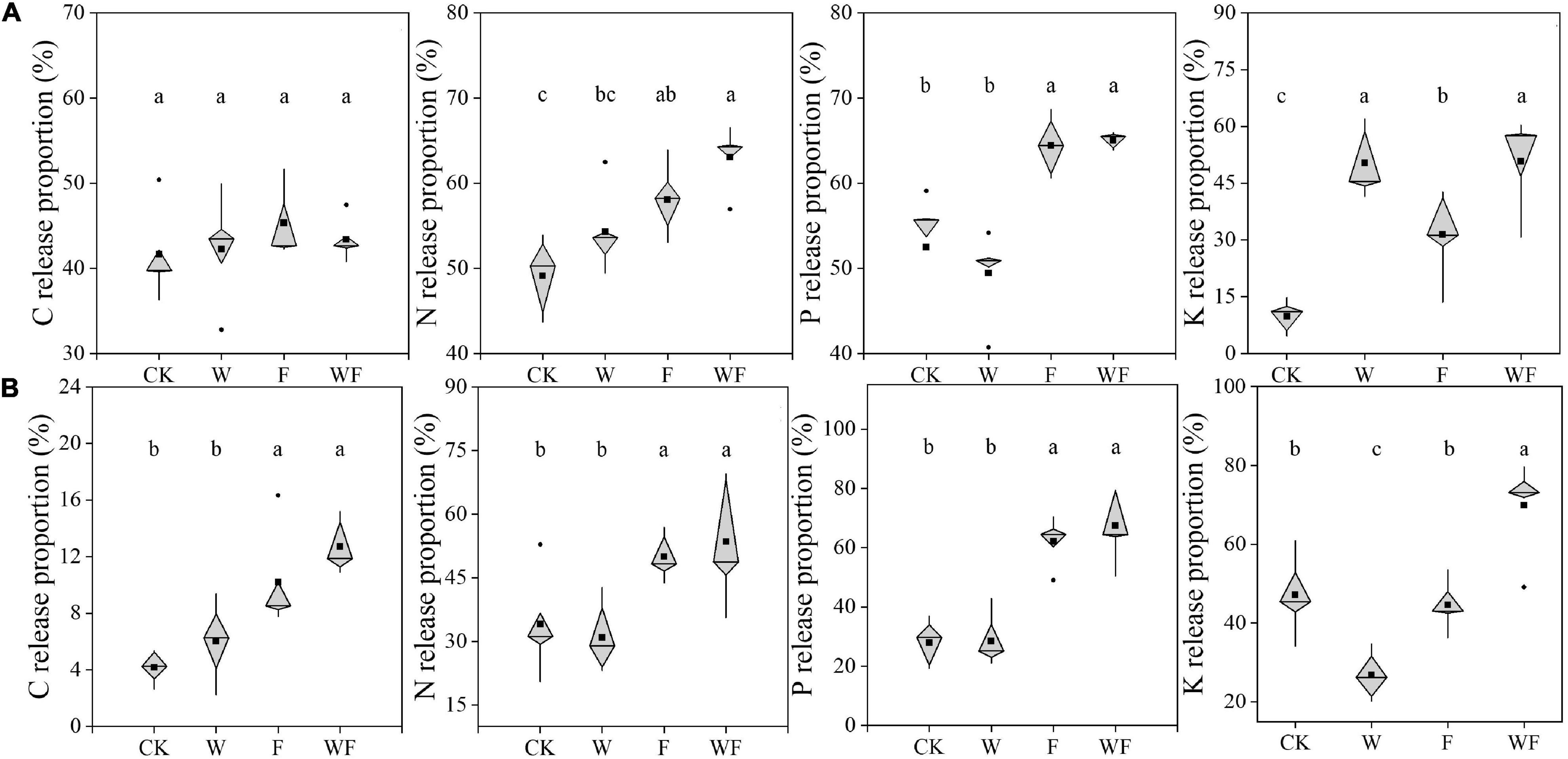
Figure 6. The proportions of nutrient released to soil from leaf (A) and twig (B) litter bags after 360 days (n = 5). Data were shown as boxplots. The center line of the box represents the median, the box represents the inter -quartile range (IQR), the whiskers extend 1.5 times IQR, black dot represents the value outside 1.5 the IQR, and black square represents the mean value. The letters over the boxes represented the Duncan’s multiple comparison results. Different lowercase letters indicated significant differences between various treatments (P < 0.05).
Discussion
Litterfall Production Under Different Treatments
Litterfall production generally exhibits seasonal patterns, which varies with forest types and environmental factors (Staelens et al., 2011; Zhang et al., 2014). The litterfall in the present study was collected from the E. urophylla × E. grandis plantation after 3 years of fertilization and dry-season irrigation. On average, the litterfall productions observed in the present study (6.85 to 8.72 Mg/ha/yr) are close to values of mature Eucalyptus plantations (3.57–6.04 Mg/ha/yr) in Brazil (Barlow et al., 2007; Ribeiro et al., 2018), but higher than that of a E. urophylla × E. grandis plantation (3.09 Mg/ha/yr) in southern China (Liu et al., 2009). In comparison with other studies demonstrating that litterfall production did not change with biomass (Demessie et al., 2012; Xu et al., 2019), the increases of litterfall production in our research are caused by increased biomass (Table 1 and Supplementary Table 1). Our results indicate a higher leaf production, followed by twig, bark, and fruit, which was consistent with the pattern observed in other studies (Da Silva et al., 2018; De Queiroz et al., 2019). Fertilization increases all types of litterfall production except for leaf, but dry-season irrigation had little effect on these (Figure 2). This difference may be related to the fact that fertilization greatly increased aboveground biomass, while dry-season irrigation did not.
The value of litterfall production in tropical/subtropical forests is known to follow a seasonal pattern. Affected by environmental factors and forest types, the monthly dynamics of litterfall showed unimodal, bimodal, and irregular models (Zhang et al., 2014). The variations of total litterfall, leaf, and twig under different treatments show bimodal models, which was consistent with the studies in tropical forests (De Queiroz et al., 2019; Zhu et al., 2021). Our results show leaf litter dominated the temporal dynamics of litterfall. Previous studies have indicated that the peak of leaf abscissions of seasonally dry forests occurred in the dry season, which is the physiological mechanism of drought adaptation (Chave et al., 2010; Zhu et al., 2019, 2021). However, our results indicating that the peak of litterfall production occurred in the mid-rainy season were contrary to our hypothesis, but consistent with other studies of Eucalyptus (Barlow et al., 2007; Cizungu et al., 2014). Although drought stress was observed during dry season in our plantation (Hua et al., 2021), litterfall productions remained at a low level during the dry season. Moreover, dry-season irrigation and fertilization only weaken the correlation between leaf production and precipitation. Litterfall production mainly affected by precipitation (Table 2) may be caused by the heavy rainfall and powerful wind events in the rainy season shaking off the dead hanging tissues (Dawoe et al., 2010).
Litter Decomposition Under Various Treatments
Litter decomposition is an important process of nutrient cycling in ecosystems (Yin et al., 2019). The decomposition of litter is mainly related to the initial nutrient concentrations (Table 4), which is consistent with previous studies that show decomposition rate is primarily determined by its traits. High nutrient concentration and corresponding low carbon: nutrient ratio increases litter attractiveness to microorganisms, which enhances the biodiversity and the activity of soil decomposers, resulting in higher decomposition rates (Zhang et al., 2019; Rawat et al., 2020). Since soil is the main source of plant nutrients, element concentrations in plants are affected by soil chemical properties (Bai et al., 2019). Fertilization increases the initial litter N, P, and K concentrations and decreases the C/N and C/P, while dry-season irrigation has little effect (Table 6). Low C/N and C/P indicates low recalcitrant substrate content and high nutrient content, facilitates microbial activity (Yin et al., 2019; Hou et al., 2021), and degrades organic matter (Zhang et al., 2019; Mora-Gómez et al., 2020). Meanwhile, the organic compounds from litter can influence the diversity and composition of microbial communities, further affecting the decomposition rates (McBride et al., 2020). In the present study, the average decomposition rate of leaf (30%) is much higher than that of twig (14%; Table 3). Compared to twig, the C/N and C/P of leaf are lower (Table 6), which is beneficial to decomposition (Zhang et al., 2019).
Generally, soil microbes secrete enzymes related to their nutrient demands, and enzyme activity is highly correlated with microbial activity (Frey et al., 2004; Li, 2006; Santos et al., 2018). Fertilization increased the activities of β -glucosidase, urease, and polyphenol oxidase, but not acid phosphatase and urease (Supplementary Table 2), indicating that N concentration limits microbial metabolism (Antonious, 2009; Wang et al., 2011). After fertilization, increased polyphenol oxidase activity was conductive to decompose recalcitrant polymers (such as lignin) of litterfall (Allison et al., 2008). Instead of cellulase, microbes in the soil of E. urophylla × E. grandis tend to secrete β -glucosidase to break down cellulose. Dry-season irrigation has little effect on soil enzyme activities during the dry season, which might be related to no differences in surface SWCr caused by high evaporation (Supplementary Tables 2, 3). Contrary to most previous studies (Salazar et al., 2011; Wang et al., 2012), the variation of soil enzyme activities is driven by foliar litter quality rather than soil nutrient (Supplementary Tables 4, 5). The increased soil enzyme activities under fertilization may be caused by diverse substrate availability (Christina and John, 2000; Hu et al., 2006).
Our results indicate that decomposing 50% of leaf litter takes 2.9 years (Table 3), longer than that (2.1 years) in tropical forests (De Queiroz et al., 2019). Compared to other Eucalyptus species, the higher C/N may be the main reason for the slow decomposition rate of E. urophylla × E. grandis litter (Demessie et al., 2012; Cizungu et al., 2014). Fertilization and dry-season irrigation accelerate litter decomposition rates, which is consistent with other studies (Sanaullah et al., 2012; Petraglia et al., 2019; Liu et al., 2021). By contrast with dry-season irrigation, fertilization had greater influences on the decomposition of low quality litter (Liu et al., 2006). After litter quality was increased by fertilization, dry-season irrigation further increased the decomposition rate. In the present study, fertilization stimulates litter decomposition by increasing litter quality and soil enzyme activities (Zhong et al., 2017; Hou et al., 2021; Liu et al., 2021). However, dry-season irrigation promoted litter decomposition with relatively little effect on litter quality or soil enzymes, contrary to previous studies (Prieto et al., 2019; Malik et al., 2020). The promotion of litter decomposition by dry season irrigation may be due to an increase in soil water content, which promotes soluble fraction leaching (Schreeg et al., 2013). These findings may only indicate the short-term effects of dry-season irrigation on litterfall decomposition, and further investigation should be conducted to uncover the underlying mechanisms.
Nutrient Release
In the present study, most leaf nutrients are released under different treatments after decomposing for 360 days, which was similar to Chen’s study Chen et al. (2019). The released proportion of nutrients of twig are much lower than that of leaf, especially the C (Figure 6). The C, N, P, and K are not released at similar proportional rates in twig, leading to the inhibition of C release at the later stage of decomposition (Yin et al., 2019; Hou et al., 2021). High nitrogen concentration (Liu et al., 2021), high labile compounds content (Pei et al., 2019, 2020), and high surface area: volume ratio (Angst et al., 2018) of litter were all beneficial for releasing C and nutrients by improving microbial activity and contact area.
The effect of dry-season irrigation and fertilization on the proportions of released nutrients was mainly driven by the variation of initial stoichiometric characteristics (Supplementary Table 6). We find that fertilization increases the proportions of released N and P by increasing litter C/N and C/P, while dry-season irrigation affects the proportion of released K by increasing foliar C/K and decreasing C/K. This result is consistent with previous studies suggesting that initial stoichiometric characteristics (C/N, C/P, and C/K) were key determinants of nutrient release (Lanuza et al., 2018; Wang et al., 2021). Due to high N concentration suppressing the activity of ligninolytic enzymes, fertilization had little effect on C release of leaf (Frey et al., 2004). In previous studies, irrigation was shown to increase (Sanaullah et al., 2012; Santonja et al., 2017; Zhong et al., 2017), decrease (Walter et al., 2013), or have no effect on decomposition rate (Zhao et al., 2012), which would affect the proportions of released nutrients. However, our results show that dry-season irrigation influences the proportion of released K through C/K, but not the decomposition rate. The variation of initial C/K might be related to the change of K retranslocation under dry-season irrigation (Wan et al., 2021). Over the experimental period, fertilization and dry-season irrigation change the litter decomposition rate by affecting the stoichiometric characteristics of litter. But it is unclear how dry-season irrigation increases decomposition rate without affecting nutrient release. It is necessary to further explore the effects of irrigation on nutrient cycling in Eucalyptus plantations under drier conditions.
Effect of Litterfall on Compensatory Growth
Previous studies on the effects of fertilization and irrigation on Eucalyptus growth have shown increased DBH, height, and biomass (Wright et al., 2011; Hua et al., 2021). In this study, fertilization and dry-season irrigation promoted the growth of DBH, height, and biomass, even though the effects of dry-season irrigation were not significant (Supplementary Table 1). This suggested that some compensatory responses occurred after nutrient and water restrictions were lifted. A high compensatory growth under fertilization may result from the up-regulation of photosynthesis capabilities and leaf biomass. Previous studies at this site have demonstrated that fertilization can increase photosynthesis (Hua et al., 2021) and promotes leaf growth (Yu et al., 2019a). By comparing the variations of biomass and litterfall production, it was found that the rise in biomass was much greater than that in litterfall production, promoting the growth of leaf biomass. Increased leaf biomass is especially beneficial for increasing photosynthetic products, which further enhances compensatory growth (Ma et al., 2020). Although the surface soil relative water content remained at a low level (Supplementary Table 3), dry-season irrigation had little effect on compensatory growth, which may be due to the utilization of deep soil water to alleviate water stress (Asensio et al., 2020). Another strategy that can be used to influence compensatory growth is altering the decomposition rate and release of nutrients in order to accelerate the nutrient cycle. The increase of decomposition rate and nutrient release under fertilization and dry-season irrigation can accelerate the nutrient cycle, and provide sufficient resources for compensatory growth (Van Staalduinen et al., 2010; Fischer et al., 2019).
Conclusion
Our results indicate that litterfall production of E. urophylla × E. grandis follows a seasonal pattern and its peak occurs in the rainy season. Fertilization significantly increases litterfall production (except leaf) while dry-season irrigation does not. Although temperature and rainfall are related to litterfall production, it is unclear whether this is caused by physical impacts (powerful wind or rain) or physiological characteristics. When litter enters topsoil, its decomposition is mainly affected by its traits (C/N, C/P, and C/K), followed by soil enzyme activity. Nutrients, rather than water, are the main factor limiting the litter decomposition in E. urophylla × E. grandis plantation. The positive effects of dry-season irrigation can be reflected only when nutrient limitation is alleviated. However, irrigation has little effect on litter nutrient release, which is contrary to previous studies. Therefore, more water supplement experiments in tropical/subtropical Eucalyptus plantations should be done to realize the effects of dry-season irrigation on the nutrient cycle under seasonal drought. Our finding shows that plantation management not only increased stand productivity, but also played an important role in the nutrient cycle.
Data Availability Statement
The original contributions presented in the study are included in the article/Supplementary Material, further inquiries can be directed to the corresponding author.
Author Contributions
JL and QQ: mainly contribute to the conceptualization of the work, funding acquisition, and investigation. JK, YL, and FH: methodology and analysis. YS, QH, and JK: project administration. WL, YS, JL, and QQ: resources. JK and WL: software. JK: visualization. QQ and QH: writing—original draft preparation. JK, QQ, and GW: writing—review, and editing. All authors have read and agreed to the version of manuscript to be published.
Funding
This research was supported by the National Natural Science Foundation of China (31800527), National Key Research and Development Program of China (2016YFD0600201 and 2016YFD060020102) and China Scholarship Council Grant (202008440171).
Conflict of Interest
The authors declare that the research was conducted in the absence of any commercial or financial relationships that could be construed as a potential conflict of interest.
Publisher’s Note
All claims expressed in this article are solely those of the authors and do not necessarily represent those of their affiliated organizations, or those of the publisher, the editors and the reviewers. Any product that may be evaluated in this article, or claim that may be made by its manufacturer, is not guaranteed or endorsed by the publisher.
Acknowledgments
We thank all members of Guangdong Key Laboratory for Innovative Development and Utilization of Forest Plant Germplasm for suggestions and support during the project.
Supplementary Material
The Supplementary Material for this article can be found online at: https://www.frontiersin.org/articles/10.3389/fevo.2022.919571/full#supplementary-material
References
Adair, E. C., Parton, W. J., Del Grosso, S. J., Silver, W. L., Harmon, M. E., Hall, S. A., et al. (2008). Simple three-pool model accurately describes patterns of long-term litter decomposition in diverse climates. Global Change Biol. 14, 2636–2660. doi: 10.1111/j.1365-2486.2008.01674.x
Allison, S. D., Czimczik, C. I., and Treseder, K. K. (2008). Microbial activity and soil respiration under nitrogen addition in Alaskan boreal forest. Global Change Biol. 14, 1156–1168. doi: 10.1111/j.1365-2486.2008.01549.x
Almagro, M., Martínez-López, J., Maestre, F. T., and Rey, A. (2017). The contribution of photodegradation to litter decomposition in semiarid mediterranean grasslands depends on its interaction with local humidity conditions, litter quality and position. Ecosystems 20, 527–542. doi: 10.1007/s10021-016-0036-5
Angst, Š, Baldrian, P., Harantová, L., Cajthaml, T., and Frouz, J. (2018). Different twig litter (Salix caprea) diameter does affect microbial community activity and composition but not decay rate. FEMS Microbiol. Ecol. 94:y126. doi: 10.1093/femsec/fiy126
Antonious, G. F. (2009). Enzyme activities and heavy metals concentration in soil amended with sewage sludge. J. Environ. Sci. Heal. A 44, 1019–1024. doi: 10.1080/10934520902996971
Asensio, V., Domec, J., Nouvellon, Y., Laclau, J., Bouillet, J., Jordan-Meille, L., et al. (2020). Potassium fertilization increases hydraulic redistribution and water use efficiency for stemwood production in Eucalyptus grandis plantations. Environ. Exp. Bot. 176:104085. doi: 10.1016/j.envexpbot.2020.104085
Bai, K., Lv, S., Ning, S., Zeng, D., Guo, Y., and Wang, B. (2019). Leaf nutrient concentrations associated with phylogeny, leaf habit and soil chemistry in tropical karst seasonal rainforest tree species. Plant Soil 434, 305–326. doi: 10.1007/s11104-018-3858-4
Barlow, J., Gardner, T. A., Ferreira, L. V., and Peres Carlos, A. (2007). Litter fall and decomposition in primary, secondary and plantation forests in the Brazilian Amazon. Forest Ecol. Manag. 247, 91–97. doi: 10.1016/j.foreco.2007.04.017
Blanco, J. A., Imbert, J. B., and Castillo, F. J. (2008). Nutrient return via litterfall in two contrasting Pinus sylvestris forests in the Pyrenees under different thinning intensities. Forest Ecol. Manag. 256, 1840–1852. doi: 10.1016/j.foreco.2008.07.011
Cajaiba, R. L., Périco, E., Caron, E., Dalzochio, M. S., Silva, W. B., and Santos, M. (2017). Are disturbance gradients in neotropical ecosystems detected using rove beetles? a case study in the Brazilian Amazon. Forest Ecol. Manag. 405, 319–327. doi: 10.1016/j.foreco.2017.09.058
Camargo, M., Giarrizzo, T., and Jesus, A. (2015). Effect of seasonal flooding cycle on litterfall production in alluvial rainforest on the middle Xingu River (Amazon basin. Brazil). Braz. J. Biol. 75, 250–256. doi: 10.1590/1519-6984.00514BM
Carter, M. R., and Gregorich, E. G. (2007). Soil Sampling and Methods of Analysis. Canadian Society of Soil Science. Boca Raton, FL: CRC Press, Taylor and Francis Group.
Chave, J., Navarrete, D., Almeida, S., Álvarez, E., Aragão, L. E. O. C., Bonal, D., et al. (2010). Regional and seasonal patterns of litterfall in tropical South America. Biogeosciences 7, 43–55. doi: 10.5194/bg-7-43-2010
Chen, Y., Zhang, Y., Cao, J., Fu, S., Hu, S., Wu, J., et al. (2019). Stand age and species traits alter the effects of understory removal on litter decomposition and nutrient dynamics in subtropical Eucalyptus plantations. Glob. Ecol. Conserv. 20:e693. doi: 10.1016/j.gecco.2019.e00693
Christina, C., and John, D. (2000). Litter quality influences on decomposition, ectomycorrhizal community structure and mycorrhizal root surface acid phosphatase activity. Soil Biol. Biochem. 32, 489–496. doi: 10.1016/s0038-0717(99)00178-9
Cizungu, L., Staelens, J., Huygens, D., Walangululu, J., Muhindo, D., Van Cleemput, O., et al. (2014). Litterfall and leaf litter decomposition in a central African tropical mountain forest and Eucalyptus plantation. Forest Ecol. Manag. 326, 109–116. doi: 10.1016/j.foreco.2014.04.015
Cotrufo, M. F., Soong, J. L., Horton, A. J., Campbell, E. E., Haddix, M. L., Wall, D. H., et al. (2015). Formation of soil organic matter via biochemical and physical pathways of litter mass loss. Nat. Geosci. 8, 776–779. doi: 10.1038/ngeo2520
Da Silva, W. B., Périco, E., Dalzochio, M. S., Santos, M., and Cajaiba, R. L. (2018). Are litterfall and litter decomposition processes indicators of forest regeneration in the neotropics? insights from a case study in the Brazilian Amazon. Forest Ecol. Manag. 429, 189–197. doi: 10.1016/j.foreco.2018.07.020
Dai, A. (2013). Increasing drought under global warming in observations and models. Nat. Clim. Change 3, 52–58. doi: 10.1038/nclimate1633
Dawoe, E. K., Isaac, M. E., and Quashie-Sam, J. (2010). Litterfall and litter nutrient dynamics under cocoa ecosystems in lowland humid Ghana. Plant Soil 330, 55–64. doi: 10.1007/s11104-009-0173-0
De Queiroz, M. G., Da Silva, T. G. F., Zolnier, S., De Souza, C. A. A., De Souza, L. S. B., Steidle Neto, A. J., et al. (2019). Seasonal patterns of deposition litterfall in a seasonal dry tropical forest. Agr. Forest Meteorol. 279:107712. doi: 10.1016/j.agrformet.2019.107712
Demessie, A., Singh, B. R., Lal, R., and Strand, L. T. (2012). Leaf litter fall and litter decomposition under Eucalyptus and coniferous plantations in Gambo District, southern Ethiopia. Acta Agr. Scand. B-S P 62, 467–476. doi: 10.1080/09064710.2011.645497
Dong, X., Gao, P., Zhou, R., Li, C., Dun, X., and Niu, X. (2021). Changing characteristics and influencing factors of the soil microbial community during litter decomposition in a mixed Quercus acutissima Carruth. and Robinia pseudoacacia L. forest in Northern China. Catena 196:104811. doi: 10.1016/j.catena.2020.104811
Dubreuil, V., Fante, K. P., Planchon, O., and Sant’Anna Neto, J. L. (2019). Climate change evidence in Brazil from Köppen’s climate annual types frequency. Int. J. Climatol. 39, 1446–1456. doi: 10.1002/joc.5893
Fischer, R. A., Moreno Ramos, O. H., Ortiz Monasterio, I., and Sayre, K. D. (2019). Yield response to plant density, row spacing and raised beds in low latitude spring wheat with ample soil resources: an update. Field Crop Res. 232, 95–105. doi: 10.1016/j.fcr.2018.12.011
Frey, S. D., Knorr, M., Parrent, J. L., and Simpson, R. T. (2004). Chronic nitrogen enrichment affects the structure and function of the soil microbial community in temperate hardwood and pine forests. Forest Ecol. Manag. 196, 159–171. doi: 10.1016/j.foreco.2004.03.018
Ge, X., Zeng, L., Xiao, W., Huang, Z., Geng, X., and Tan, B. (2013). Effect of litter substrate quality and soil nutrients on forest litter decomposition: a review. Acta Ecol. Sinica 33, 102–108. doi: 10.1016/j.chnaes.2013.01.006
Gonzalez, I., Sixto, H., Rodriguez-Soalleiro, R., and Oliveira, N. (2020). Nutrient contribution of litterfall in a short rotation plantation of pure or mixed plots of Populus alba L. and Robinia pseudoacacia L. Forests 11:1133. doi: 10.3390/f11111133
Hou, S. L., Hättenschwiler, S., Yang, J. J., Sistla, S., Wei, H. W., Zhang, Z. W., et al. (2021). Increasing rates of long-term nitrogen deposition consistently increased litter decomposition in a semi-arid grassland. New Phytol. 229, 296–307. doi: 10.1111/nph.16854
Hu, Y. L., Wang, S. L., and Zeng, D. H. (2006). Effects of single chinese fir and mixed leaf litters on soil chemical, microbial properties and soil enzyme activities. Plant Soil 282, 379–386. doi: 10.1007/s11104-006-0004-5
Hua, L., Yu, F., Qiu, Q., He, Q., Su, Y., Liu, X., et al. (2021). Relationships between diurnal and seasonal variation of photosynthetic characteristics of Eucalyptus plantation and environmental factors under dry-season irrigation with fertilization. Agr. Water Manage 248:106737. doi: 10.1016/j.agwat.2021.106737
Kamruzzaman, M., Basak, K., Paul, S. K., Ahmed, S., and Osawa, A. (2019). Litterfall production, decomposition and nutrient accumulation in Sundarbans mangrove forests, Bangladesh. Forest Sci. Technol. 15, 24–32. doi: 10.1080/21580103.2018.1557566
Lanuza, O., Casanoves, F., Delgado, D., and Van den Meersche, K. (2018). Leaf litter stoichiometry affects decomposition rates and nutrient dynamics in tropical forests under restoration in Costa Rica. Restor. Ecol. 27, 549–558. doi: 10.1111/rec.12893
Li, C., Barclay, H., Roitberg, B., and Lalonde, R. (2020). Forest productivity enhancement and compensatory growth: a review and synthesis. Front. Plant. Sci. 11:575211. doi: 10.3389/fpls.2020.575211
Li, D. (2006). Review of fungal chitinases. Mycopathologia 161, 345–360. doi: 10.1007/s11046-006-0024-y
Liu, C., Westman, C. J., Berg, B., Kutsch, W., Wang, G. Z., Man, R., et al. (2004). Variation in litterfall-climate relationships between coniferous and broadleaf forests in Eurasia. Global Ecol. Biogeogr. 13, 105–114. doi: 10.1111/j.1466-882X.2004.00072.x
Liu, D., Ogaya, R., Barbeta, A., Yang, X., and Peñuelas, J. (2015). Contrasting impacts of continuous moderate drought and episodic severe droughts on the aboveground-biomass increment and litterfall of three coexisting Mediterranean woody species. Global Change Biol. 21, 4196–4209. doi: 10.1111/gcb.13029
Liu, P., Huang, J., Han, X., Sun, O. J., and Zhou, Z. (2006). Differential responses of litter decomposition to increased soil nutrients and water between two contrasting grassland plant species of Inner Mongolia. China. Appl. Soil Ecol. 34, 266–275. doi: 10.1016/j.apsoil.2005.12.009
Liu, S., Behm, J. E., Wan, S., Yan, J., Ye, Q., Zhang, W., et al. (2021). Effects of canopy nitrogen addition on soil fauna and litter decomposition rate in a temperate forest and a subtropical forest. Geoderma 382:114703. doi: 10.1016/j.geoderma.2020.114703
Liu, W., Fan, H., Gao, C., Huang, R., and Su, B. (2009). Litter production and its nutrient fluxes in an age sequence of Eucalyptus plantations. Chinese J. Ecol. 28, 1928–1934.
Lu, H., Xu, J., Li, G., and Liu, W. (2020). Site classification of Eucalyptus urophylla × Eucalyptus grandis plantations in China. Forests 11:871. doi: 10.3390/f11080871
Lu, R. (2000). Soil Agricultural Chemical Analysis Method. China Agricultural. Beijing: Science Press.
Ma, H., Zheng, C., Gao, Y., Baskin, C. C., Sun, H., and Yang, H. (2020). Moderate clipping stimulates over-compensatory growth of Leymus chinensis under saline-alkali stress through high allocation of biomass and nitrogen to shoots. Plant Growth Regul. 92, 95–106. doi: 10.1007/s10725-020-00622-3
Malik, A. A., Swenson, T., Weihe, C., Morrison, E. W., Martiny, J. B. H., Brodie, E. L., et al. (2020). Drought and plant litter chemistry alter microbial gene expression and metabolite production. ISME J. 14, 2236–2247. doi: 10.1038/s41396-020-0683-6
McBride, S. G., Choudoir, M., Fierer, N., and Strickland, M. S. (2020). Volatile organic compounds from leaf litter decomposition alter soil microbial communities and carbon dynamics. Ecology 101:e3130. doi: 10.1002/ecy.3130
Menegale, M., Rocha, J., Harrison, R., Almeida, R., Piccolo, M., Hubner, A., et al. (2016). Effect of timber harvest intensities and fertilizer application on stocks of soil C. N, P, and S. Forests 7:319. doi: 10.3390/f7120319
Ministry of Agriculture of the People’s Republic of China (2010). Soil Testing – Cutting Ring Method for Determination of Field Water – Holding Capacity in Soil. Beijing: Ministry of Agriculture of the People’s Republic of China.
Ministry of Forestry of the People’s Republic of China (2015). Technical Regulation on Management of Eucalypt High-yielding Plantation. Beijing: Ministry of Agriculture of the People’s Republic of China.
Mora-Gómez, J., Boix, D., Duarte, S., Cássio, F., Pascoal, C., Elosegi, A., et al. (2020). Legacy of summer drought on autumnal leaf litter processing in a temporary Mediterranean stream. Ecosystems 23, 989–1003. doi: 10.1007/s10021-019-00451-0
Ni, X., Lin, C., Chen, G., Xie, J., Yang, Z., Liu, X., et al. (2021). Decline in nutrient inputs from litterfall following forest plantation in subtropical China. Forest Ecol. Manag. 496:119445. doi: 10.1016/j.foreco.2021.119445
Nouvellon, Y., Epron, D., Marsden, C., Kinana, A., Le Maire, G., Deleporte, P., et al. (2012). Age-related changes in litter inputs explain annual trends in soil CO2 effluxes over a full Eucalyptus rotation after afforestation of a tropical savannah. Biogeochemistry 111, 515–533. doi: 10.1007/s10533-011-9685-9
Paudel, E., Dossa, G. G. O., Xu, J., and Harrison, R. D. (2015). Litterfall and nutrient return along a disturbance gradient in a tropical montane forest. Forest Ecol. Manag. 353, 97–106. doi: 10.1016/j.foreco.2015.05.028
Pei, G., Liu, J., Peng, B., Gao, D., Wang, C., Dai, W., et al. (2019). Nitrogen, lignin, C/N as important regulators of gross nitrogen release and immobilization during litter decomposition in a temperate forest ecosystem. Forest Ecol. Manag. 440, 61–69. doi: 10.1016/j.foreco.2019.03.001
Pei, G., Liu, J., Peng, B., Wang, C., Jiang, P., and Bai, E. (2020). Nonlinear coupling of carbon and nitrogen release during litter decomposition and its responses to nitrogen dddition. J. Geophys. Res-Biogeo 125:e2019J. doi: 10.1029/2019JG005462
Petraglia, A., Cacciatori, C., Chelli, S., Fenu, G., Calderisi, G., Gargano, D., et al. (2019). Litter decomposition: effects of temperature driven by soil moisture and vegetation type. Plant Soil 435, 187–200. doi: 10.1007/s11104-018-3889-x
Prieto, I., Almagro, M., Bastida, F., and Querejeta, J. I. (2019). Altered leaf litter quality exacerbates the negative impact of climate change on decomposition. J. Ecol. 107, 2364–2382. doi: 10.1111/1365-2745.13168
Prosser, J., Speir, T., Stott, D. E., and Dick, R. (2011). Soil Oxidoreductases and FDA Hydrolysis. Madison, WI: Soil Science Society of America.
Rawat, M., Arunachalam, K., Arunachalam, A., Alatalo, J. M., and Pandey, R. (2020). Predicting litter decomposition rate for temperate forest tree species by the relative contribution of green leaf and litter traits in the Indian Himalayas region. Ecol. Indic. 119:106827. doi: 10.1016/j.ecolind.2020.106827
Ribeiro, C., Madeira, M., and Araújo, M. C. (2002). Decomposition and nutrient release from leaf litter of Eucalyptus globulus grown under different water and nutrient regimes. Forest Ecol. Manag. 171, 31–41. doi: 10.1016/s0378-1127(02)00459-0
Ribeiro, F., Gatto, A., Oliveira, A., Pulrolnik, K., and Neto, S. (2018). Litter dynamics in eucalyptus and native forest in the brazilian cerrado. J. Agr. Sci-Cambridge 10, 29–43. doi: 10.5539/jas.v10n11p29
Salazar, S., Sánchez, L. E., Alvarez, J., Valverde, A., Galindo, P., Igual, J. M., et al. (2011). Correlation among soil enzyme activities under different forest system management practices. Ecol. Eng. 37, 1123–1131.
Sanaullah, M., Rumpel, C., Charrier, X., and Chabbi, A. (2012). How does drought stress influence the decomposition of plant litter with contrasting quality in a grassland ecosystem? Plant Soil 352, 277–288. doi: 10.1007/s11104-011-0995-4
Santonja, M., Fernandez, C., Proffit, M., Gers, C., Gauquelin, T., Reiter, I. M., et al. (2017). Plant litter mixture partly mitigates the negative effects of extended drought on soil biota and litter decomposition in a Mediterranean oak forest. J. Ecol. 105, 801–815. doi: 10.1111/1365-2745.12711
Santos, F. M., Balieiro, F. D. C., Fontes, M. A., and Chaer, G. M. (2018). Understanding the enhanced litter decomposition of mixed-species plantations of Eucalyptus and Acacia mangium. Plant Soil 423, 141–155. doi: 10.1007/s11104-017-3491-7
Schreeg, L. A., Mack, M. C., and Turner, B. L. (2013). Nutrient-specific solubility patterns of leaf litter across 41 lowland tropical woody species. Ecology 94, 94–105. doi: 10.1890/11-1958.1
Staelens, J., Nachtergale, L., De Schrijver, A., Vanhellemont, M., Wuyts, K., and Verheyen, K. (2011). Spatio-temporal litterfall dynamics in a 60-year-old mixed deciduous forest. Ann. Forest Sci. 68, 89–98. doi: 10.1007/s13595-011-0010-5
Tang, J., Cao, M., Zhang, J., and Li, M. (2010). Litterfall production, decomposition and nutrient use efficiency varies with tropical forest types in Xishuangbanna. SW China: a 10-year study. Plant Soil 335, 271–288. doi: 10.1007/s11104-010-0414-2
Van Staalduinen, M. A., Dobarro, I., and Peco, B. (2010). Interactive effects of clipping and nutrient availability on the compensatory growth of a grass species. Plant Ecol. 208, 55–64. doi: 10.1007/s11258-009-9686-0
Walter, J., Hein, R., Beierkuhnlein, C., Hammerl, V., Jentsch, A., Schädler, M., et al. (2013). Combined effects of multifactor climate change and land-use on decomposition in temperate grassland. Soil Biol. Biochem. 60, 10–18. doi: 10.1016/j.soilbio.2013.01.018
Wan, F., Ross-Davis, A. L., Davis, A. S., Song, X., Chang, X., Zhang, J., et al. (2021). Nutrient retranslocation in Larix principis-rupprechtii Mayr relative to fertilization and irrigation. New Forest 52, 69–88. doi: 10.1007/s11056-020-09783-5
Wang, B., Xue, S., Liu, G. B., Zhang, G. H., Li, G., and Ren, Z. P. (2012). Changes in soil nutrient and enzyme activities under different vegetations in the Loess Plateau area, Northwest China. Catena 92, 186–195. doi: 10.1016/j.catena.2011.12.004
Wang, C., Han, G., Jia, Y., Feng, X., Guo, P., and Tian, X. (2011). Response of litter decomposition and related soil enzyme activities to different forms of nitrogen fertilization in a subtropical forest. Ecol. Res. 26, 505–513. doi: 10.1007/s11284-011-0805-8
Wang, L., Chen, Y., Zhou, Y., Xu, Z., Tan, B., You, C., et al. (2021). Environmental conditions and litter nutrients are key determinants of soluble C, N, and P release during litter mixture decomposition. Soil Till Res. 209:104928. doi: 10.1016/j.still.2020.104928
Wang, L., Zhang, G., Zhu, P., and Wang, X. (2020). Comparison of the effects of litter covering and incorporation on infiltration and soil erosion under simulated rainfall. Hydrol. Process 34, 2911–2922. doi: 10.1002/hyp.13779
Wright, S. J., Yavitt, J. B., Wurzburger, N., Turner, B. L., Tanner, E. V. J., Sayer, E. J., et al. (2011). Potassium, phosphorus, or nitrogen limit root allocation, tree growth, or litter production in a lowland tropical forest. Ecology 92, 1616–1625. doi: 10.1890/10-1558.1
Xie, Y., Arnold, R. J., Wu, Z., Chen, S., Du, A., and Luo, J. (2017). Advances in eucalypt research in China. Front. Agr. Sci. Eng. 4:380–390. doi: 10.15302/J-FASE-2017171
Xu, Y., Wang, Z., Zhu, W., and Du, A. (2019). Litterfall and nutrient cycling of Eucalyptus plantation with different ages on Leizhou Peninsula. J. Trop. Subtropical Botany 27, 359–366.
Yin, R., Eisenhauer, N., Auge, H., Purahong, W., Schmidt, A., and Schädler, M. (2019). Additive effects of experimental climate change and land use on faunal contribution to litter decomposition. Soil Biol. Biochem. 131, 141–148. doi: 10.1016/j.soilbio.2019.01.009
Yu, F., Truong, T. V., He, Q., Hua, L., Su, Y., and Li, J. (2019a). Dry season irrigation promotes leaf growth in Eucalyptus urophylla × E. grandis under fertilization. Forests 10:67. doi: 10.3390/f10010067
Yu, S., Mo, Q., Li, Y., Li, Y., Zou, B., Xia, H., et al. (2019b). Changes in seasonal precipitation distribution but not annual amount affect litter decomposition in a secondary tropical forest. Ecol. Evol. 9, 11344–11352. doi: 10.1002/ece3.5635
Zhang, H., Yuan, W., Dong, W., and Liu, S. (2014). Seasonal patterns of litterfall in forest ecosystem worldwide. Ecol. Complex 20, 240–247. doi: 10.1016/j.ecocom.2014.01.003
Zhang, M., Cheng, X., Geng, Q., Shi, Z., Luo, Y., and Xu, X. (2019). Leaf litter traits predominantly control litter decomposition in streams worldwide. Global Ecol. Biogeogr. 28, 1469–1486. doi: 10.1111/geb.12966
Zhao, H., Huang, G., Ma, J., Li, Y., and Zhou, L. (2012). Responses of surface litter decomposition to seasonal water addition in desert. Chinese J. Plant Ecol. 36, 471–482. doi: 10.3724/sp.j.1258.2012.00471
Zhen, Z., Wang, S., Luo, S., Ren, L., Liang, Y., Yang, R., et al. (2005). How different reforestation approaches affect red soil properties in southern China. Land Degrad Dev. 16, 387–396. doi: 10.1002/ldr.650
Zhen, Z., Wang, S., Luo, S., Ren, L., Liang, Y., Yang, R., et al. (2019). Significant impacts of both total amount and availability of heavy metals on the functions and assembly of soil microbial communities in different land use patterns. Front. Microbiol. 10:2293.
Zhong, Y., Yan, W., Wang, R., and Shangguan, Z. (2017). Differential responses of litter decomposition to nutrient addition and soil water availability with long-term vegetation recovery. Biol. Fert. Soils 53, 939–949.
Zhou, G., Wei, X., Wu, Y., Liu, S., Huang, Y., Yan, J., et al. (2011). Quantifying the hydrological responses to climate change in an intact forested small watershed in Southern China. Global Change Biol. 17, 3736–3746. doi: 10.1111/j.1365-2486.2011.02499.x
Zhu, X., Liu, W., Chen, H., Deng, Y., Chen, C., and Zeng, H. (2019). Effects of forest transition on litterfall, standing litter and related nutrient returns: implications for forest management in tropical China. Geoderma 333, 123–134. doi: 10.1016/j.geoderma.2018.07.023
Keywords: fertilization, irrigation, Eucalyptus, litterfall production, decomposition rate, nutrient release
Citation: Kong J, Lin Y, Huang F, Liu W, He Q, Su Y, Li J, Wang G and Qiu Q (2022) Effects of Fertilization and Dry-Season Irrigation on Litterfall Dynamics and Decomposition Processes in Subtropical Eucalyptus Plantations. Front. Ecol. Evol. 10:919571. doi: 10.3389/fevo.2022.919571
Received: 13 April 2022; Accepted: 06 May 2022;
Published: 26 May 2022.
Edited by:
Bernard Roitberg, Simon Fraser University, CanadaReviewed by:
César Marín, Santo Tomás University, ChileXia Zhichao, Hangzhou Normal University, China
Copyright © 2022 Kong, Lin, Huang, Liu, He, Su, Li, Wang and Qiu. This is an open-access article distributed under the terms of the Creative Commons Attribution License (CC BY). The use, distribution or reproduction in other forums is permitted, provided the original author(s) and the copyright owner(s) are credited and that the original publication in this journal is cited, in accordance with accepted academic practice. No use, distribution or reproduction is permitted which does not comply with these terms.
*Correspondence: Quan Qiu, cXFpdUBzY2F1LmVkdS5jbg==