- 1Comparative Bioacoustics Group, Max Planck Institute for Psycholinguistics, Nijmegen, Netherlands
- 2Department of Clinical Medicine, Center for Music in the Brain, Aarhus University and The Royal Academy of Music Aarhus, Aalborg, Denmark
- 3Department of Neuropsychology and Psychopharmacology, Faculty of Psychology and Neuroscience, Maastricht University, Maastricht, Netherlands
- 4Department of Neuropsychology, Max Planck Institute for Human Cognitive and Brain Sciences, Leipzig, Germany
In the last 20 years, research on turn-taking and duetting has flourished in at least three, historically separate disciplines: animal behavior, language sciences, and music cognition. While different in scope and methods, all three ultimately share one goal—namely the understanding of timed interactions among conspecifics. In this perspective, we aim at connecting turn-taking and duetting across species from a neural perspective. While we are still far from a defined neuroethology of turn-taking, we argue that the human neuroscience of turn-taking and duetting can inform animal bioacoustics. For this, we focus on a particular concept, interhemispheric connectivity, and its main white-matter substrate, the corpus callosum. We provide an overview of the role of corpus callosum in human neuroscience and interactive music and speech. We hypothesize its mechanistic connection to turn-taking and duetting in our species, and a potential translational link to mammalian research. We conclude by illustrating empirical venues for neuroethological research of turn-taking and duetting in mammals.
Turn-Taking and Duetting: From Behavior to Cognition and Neuroscience
No matter the discipline, research on turn-taking and duetting (TTD) is currently a hot topic (e.g., Demartsev et al., 2018; Pika et al., 2018; Benichov and Vallentin, 2020). In the last 20 years (see e.g., Figure 1; Ravignani et al., 2019), there has been an increased and converging interest in TTD in at least three separate disciplines: Animal behavior, language sciences and music cognition (Greenfield et al., 2021). Research into language has explored the nuances of both the semantics and precise timing of turn-taking; because of the short time scales involved in turn-taking in conversation and the comparably slower reactivity of the human nervous system, turn-taking in human speech must be predictive, rather than reactive (Stivers et al., 2009). Some have even argued that turn-taking is at the core of human linguistic abilities (Levinson, 2016). Human music research has experienced a strong empirical turn and likewise explored the behavioral bases of interaction, with prime examples including the dynamics of jazz improvised duetting or string quartet synchronization (Wing et al., 2014; Zeng et al., 2017). Finally, animal behavior has also moved on—at least in bioacoustics—from the exclusive study of behavioral patterns in isolation to a balanced mélange of individuals, duets, and choruses. Catching up with avian bioacoustics (e.g., Benichov and Vallentin, 2020), findings on TTD patterns in mammals are continuously accumulating (Takahashi et al., 2016; Demartsev et al., 2018; Pika et al., 2018; Ravignani, 2019; Ravignani et al., 2019; de Reus et al., 2021).
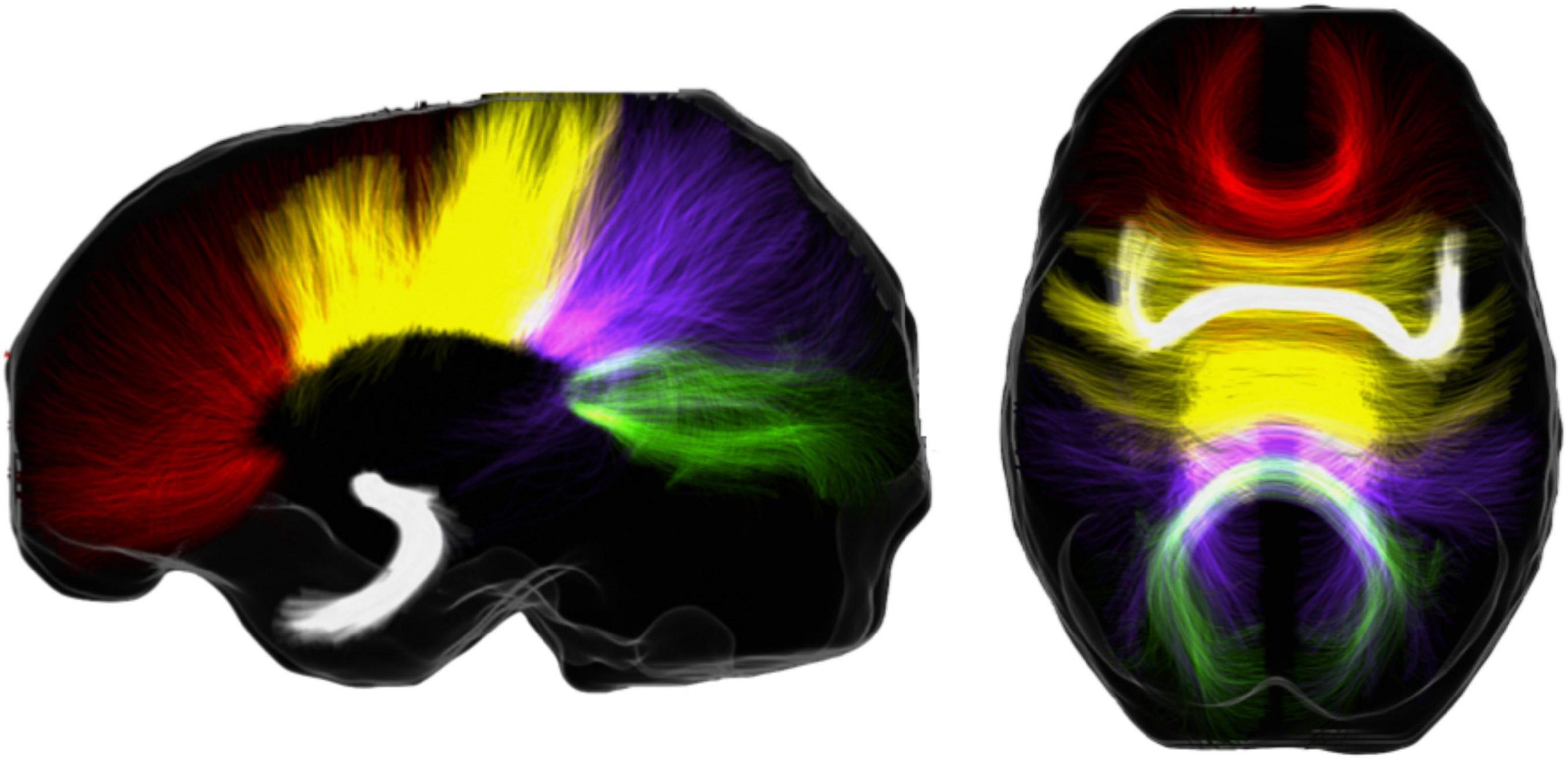
Figure 1. Sagittal (left figure) and axial view (right figure) of the 2 main interhemispheric tracts in humans overlaid on a population template. The tracts included are the corpus callosum with its four geometrical subdivisions (rostrum and genu, in red; truncus or body, in yellow; isthmus, in violet; splenium, in green) and the anterior commissure (in white).
Sometimes in all three disciplines, but especially in animal behavior of TTD, the brain is “black-boxed.” Behavioral patterns are considered and are sometimes interpreted in cognitive terms. However, the mapping of these behaviors to neural processes is still in its infancy. Here we propose that while we are still far from a clear picture in the neuroethology of turn-taking, there are still many potential low-hanging fruits in this research area. In particular, we offer a perspective on a particular structure in the mammalian brain, the corpus callosum (CC, Figure 1), which, we hypothesize, might form basic building blocks of mammalian TTD. We know about the potential neural bases of TTD comparatively much more in humans than any other mammal. Therefore, in the following, we provide an overview of the CC, and its role in human music and spoken language and propose a potential connection between CC and TTD. We discuss the empirical venues its study can open for mammalian comparative TTD research. Of note is that even the human evidence we present is sometimes indirect and speculative; however, we consider building cross-disciplinary bridges to advance valuable knowledge of TTD that has lately received so much attention.
Interhemispheric Communication and Corpus Callosum
In both music and language, an interplay between interhemispheric specialization and communication plays an important role. A key structure enabling and regulating this interplay is the CC, connecting the two hemispheres (Clarke and Zaidel, 1994; Schlaug et al., 1995; Paul et al., 2003; Friederici et al., 2007) and consisting of about 160–190 million fibers (Aboitiz et al., 1992). In music performance, where among others the timing of interactions is key, CC size correlates with musical training (Ozturk et al., 2002). Interhemispheric connectivity has also been linked to musical improvisation: CC may act as a support structure in the lateral perception-action network (Loui, 2018). In language, the CC regulates among others the interplay between syntax/semantics (what to say) and suprasegmental prosody (how to say it) (Friederici et al., 2007). Throughout development, we see that individuals with impaired functionality of the CC may have, among others, impaired TTD-relevant traits (Beens, 1995; Stickles et al., 2002). So, what are the (neuro) biological functions of the CC?
Since the seminal split-brain studies by Sperry (1961) in the fifties and sixties of the last century, we have gained a significant understanding of the role of the CC in interhemispheric brain communication. Specifically, this evidence confirmed that the CC not only transfers information between the two hemispheres of the human brain, but significantly contributes to the development of lateralized function as well as the upkeep of functional integration across the hemispheres (Gazzaniga, 2000; Güntürkün et al., 2020). Despite the lack of general agreement on the functional significance of callosal morphology (Aboitiz et al., 1992), most authors associate a larger callosal area with a better capacity for interhemispheric transfer. A larger diameter and number density of myelinated fibers increase the conduction speed between the two hemispheres, leading to faster and more efficient cross-hemispheric integration and communication (Westerhausen et al., 2006; Horowitz et al., 2015). It is not yet fully understood whether callosal fibers exert an inhibitory or excitatory influence on interhemispheric communication and integration (Bloom and Hynd, 2005); we make no claims here as the particular case of TTD could be explained by both influences. One hypothesis is that the CC provided placental mammals with shorter and more direct pathways between bilateral cortical regions than the anterior commissure, thus speeding up the interhemispheric transfer of information (Buzsáki et al., 2013; Aboitiz, 2017). This could have been even more advantageous in larger brains, such as those of mammals (Aboitiz, 2017; Font et al., 2019).
Human Music and Turn-Taking
Some of the characteristics predicted by the general function of CC have been observed in musicians—individuals with more than 6 years of formal musical training (Zhang et al., 2020)—due to training-related neuroplastic effects on callosal fiber composition and volume (Lee et al., 2003). Musicians exhibit a larger midsagittal callosal size (e.g., Schlaug et al., 1995, 2005) and more organized callosal bundles (e.g., Elmer et al., 2016; Habibi et al., 2018) than musically naive individuals. These findings suggest a positive association between the amount of musical training and the strength of interhemispheric connectivity (Schlaug et al., 1995; Bengtsson et al., 2005; Hyde et al., 2009; Steele et al., 2013; Elmer et al., 2016; Habibi et al., 2018). Stronger cross-hemispheric anatomical connections may explain the enhanced capabilities observed in musicians, as well as in non-musicians (Lumaca et al., 2021) for music perception and performance that rely on high-speed interhemispheric conduction, such as binaural temporal integration, visuo-motor integration, and bimanual motor planning, execution, and control (Gooijers and Swinnen, 2014).
In music, these perceptuo-motor skills are critical for different forms of interpersonal synchrony, including playing jazz in ensembles. Thus, one may hypothesize that the ability of individuals to coordinate their actions in time is associated, and can be predicted by, the microstructural characteristics and the size of their CC. Jazz improvisers, who show a large flexibility and precision in their coordination and joint action, exhibit higher callosal integrity and larger tract volume than classical musicians and non-musicians (Zeng et al., 2017). Conversely, deficits in spatial and temporal interpersonal synchrony have been observed in populations with reduced size of the CC such as Autism Spectrum Disorder (Casanova et al., 2009; Fitzpatrick et al., 2016; Kaur et al., 2018). A recent study with fNIRS on Autism Spectrum Disorder children shows that these deficits are accompanied by reduced symmetrical activations in superior and middle temporal regions compared to typically developed children (Su et al., 2020). This research further supports a key role of interhemispheric brain communication in socially embedded actions.
Human Spoken Language and Turn-Taking
A prime example of interactive human communication, with some potential parallels to mammalian TTD, is language use. Successful communication depends on at least two aspects: the rapid integration of lateralized verbal (segmental) and non-verbal (suprasegmental) information in speech/language comprehension and production (Kotz and Schwartze, 2010, 2016) and swift turn-taking in interpersonal interaction (Holler et al., 2015; Levinson, 2016).
The integration of left-hemispheric segmental and right-hemispheric suprasegmental information necessitates rapid information flow between the two hemispheres (Friederici and Alter, 2004). Such transfer likely engages the commissural fibers crossing through the CC, but the structural and functional differentiation of these fibers indicates that they are topographically organized based on their cortical origin. Diffusion-weighted imaging revealed that while the anterior portions of the CC (genu and truncus) connect the orbital and frontal lobes, the posterior third (isthmus and splenium) link the temporal, parietal, and occipital lobes of the two hemispheres (e.g., Huang et al., 2005; Hofer and Frahm, 2006; Zarei et al., 2006; Dougherty et al., 2007; Park et al., 2008). Empirical evidence in support of such topographical connectivity comes from studies exploring the rapid integration of segmental (syntax) and suprasegmental (prosody) information in patients with CC lesions. Results confirmed a reciprocal speech processing interface between the two hemispheres via the posterior CC that seems to underlie speech/language comprehension (Friederici et al., 2007; Sammler et al., 2010). Recent work with children and adolescents, suffering from developmental agenesis of the corpus callosum, a congenital brain disorder where the axons of the CC are either completely or partially absent (Rauch and Jinkins, 1994; Laìbadi and Beke, 2017), have further confirmed the integrative function of the posterior CC within the language network (Bartha-Doering et al., 2020) and its relation to language abilities (Bartha-Doering et al., 2021). Further evidence also points to interhemispheric information flow for other functions. For example, lesions in the middle and posterior troncus of the CC mainly affect interhemispheric transfer of motor and somatosensory information (Risse et al., 1989; Fabri et al., 2001), while isthmus and splenium lesions mostly affect auditory (Pollmann et al., 2002) and visual transfer (Corballis et al., 2004).
Whether or not turn-taking, a communicative act between two or more speakers, relies on the integrative function of the CC per se or may only relate to the swift integration between segmental and suprasegmental information that speakers and listeners use in communication is currently an open scientific question. Levinson (2016) proposed that turn-taking is at the root of human communication and might derive from three factors. First, turn-taking is one of many means that form human interaction, including non-verbal information such as facial expression, gaze, and gestures. Second, turn-talking might reflect a prototypical form of human interaction as evidenced in pre-lingual infants, and third turn-taking is also found in non-human primates as part of their vocal communicative repertoire. Recently, the cognitive and neural processes underlying turn-taking in humans have also been explored, highlighting the human capacity to anticipate one’s turn to communicate (Holler et al., 2015). Thus, the timely integration of segmental and suprasegmental information while anticipating one’s turn might facilitate swift information flow between a speaker and a listener.
To bridge the gap on how turn-taking might rely on CC interhemispheric connectivity might be best informed by studies on developmental agenesis of the CC. Next to altered language abilities (Bartha-Doering et al., 2021), there are reports on communication deficits that entail pragmatic skills (understanding jokes and non-verbal cues such as emotional vocal and facial expressions; see for example, Brown et al., 2005; Tu et al., 2009). Further, younger children with CC agenesis seem to struggle with initiating and sustaining conversations and lack a basic understanding of social reciprocity and non-verbal communication (Badaruddin et al., 2007). Adults with CC agenesis further exhibit difficulties in social cognition and social behavior (Laìbadi and Beke, 2017). Of note is their atypical facial scanning while observing emotional expressions of others (Bridgman et al., 2014). A speculative conclusion could therefore be that missing out on rapidly detecting and integrating non-verbal information in social interaction affects how children and adults, suffering from CC agenesis, understand and implement turn-taking.
Perspectives for Turn-Taking Research Across Mammals Linking Brain to Behavior
Some building blocks of human TTD may have mechanistic bases in the CC. These building blocks may be potentially analogous or homologous to TTD in other mammals (Fröhlich, 2017; Pika et al., 2018; Anichini et al., 2020; Greenfield et al., 2021). What do we know about TTD mechanisms in other species? Some work has found links between rodent turn-taking behavior and underlying neural mechanisms, highlighting hierarchical, cortical control of this behavior (Okobi et al., 2019). If the interhemispheric neuroscience of TTD in humans is little explored, the non-human animal counterpart is even less so. While, to our knowledge, no mechanistic link has been sought between TTD and their interhemispheric neural bases in other mammals, we consider that combined behavioral evidence in mammals and neural evidence in humans can provide fruitful research directions and predictions for experiments. In other words, we suggest to (1) look for mammalian TTD traits which show strong behavioral similarities to either human music or spoken language; (2) capitalize on human neuroscientific evidence while translating it to other species for which at least some (callosal) brain anatomy is known, and (3) within these species, target and behaviorally test those with maximally divergent predictions in terms of how interhemispheric connectivity should affect TTD features.
Let us consider a concrete example. A recent important finding is that, in mammals, two possible routes of interhemispheric communication exist. While eutherian mammals evolved a corpus callosum for interhemispheric communication, non-eutherian mammals, i.e., monotremes and marsupials (e.g., platypus and kangaroo) do not have this—they use other, potential slower routes for interhemispheric communication (Suárez et al., 2018). In other words, the CC evolved within the last 120 million years, so that non-eutherian mammals and birds use non-callosal structures for interhemispheric communication (Rogers et al., 2013). For instance, monotremes and marsupials mostly use the anterior commissure (see Figure 1; Aboitiz and Montiel, 2003).
This “discontinuity” in mammals provides a powerful testbed for our proposed TTD-CC link; in particular, even in behavioral experiments, we expect to find an evolutionary jump in the TTD phenotype. Within mammals, one often finds pairs of species that have convergently evolved similar behavioral traits or anatomy from two separate ancestors, one marsupial the other placental. Examples of this are, respectively: sugar gliders vs. flying squirrels, marsupial vs. non-marsupial moles, echidnas vs. porcupines. Based on our hypothesis, one could compare TTD abilities between pairs of species, expecting more developed ones in eutherian mammals. Even within eutherians there may be pairs of closely related species one of which duets while the other does not, featuring a variety of social and parental care systems. One could study variation in CC size between pairs of duetting and phylogenetically close non-duetting placental mammals. More generally and going beyond this admittedly simple dichotomy, one could study TTD acoustic phenotypes in species for which tractography data or measures of CC thickness exist, potentially expecting a positive correlation between the two.
In addition, non-invasive cognitive neuroscience techniques could be used to either measure or disrupt interhemispheric connectivity and relate this to behavioral TTD markers. For instance, electroencephalography (EEG) can be used to measure brain responses to sounds or phonatory actions. It appears that interhemispheric EEG coherence correlates with the size of CC and strongly decreases when the CC is damaged (Nielsen et al., 1993; Pogarell et al., 2005). Non-invasive EEG could then be employed in TTD experiments to test whether, as we hypothesize, interhemispheric EEG coherence will positively correlate with behavioral metrics of well-coordinated TTD. In addition, and to obtain more causal inference, techniques such as magnetic stimulation could be used to disrupt TTD patterns and therefore to obtain mechanistic explanations (Fitzgerald et al., 2002; Voineskos et al., 2010).
While we focus on mammals here, it is important to mention that several avian species have very developed TTD capacities (Mann et al., 2006; Pika et al., 2018; Benichov and Vallentin, 2020; Coleman et al., 2021; Kishimoto and Seki, 2022; Norton et al., 2022). Birds achieve this fine timing via developed intra-hemispheric connectivity, often resulting in motor inhibition to avoid overlap (Norton et al., 2022). However, similarly to non-eutherian mammals, birds do not have a CC. How do birds achieve impressive TTD without a CC? We hypothesize that TTD have different neuroanatomical bases in birds and eutherian mammals. While in humans the CC is responsible, and grants high-speed transmission, for most interhemispheric excitation and inhibition, birds rely on non-callosal structures (Rogers et al., 2013), which may still grant fast communication given the relatively smaller brain sizes and hence long distances (Ringo et al., 1994). In other words, we hypothesize that fast interhemispheric connection is achieved in birds via smaller brains and in eutherian mammals via the CC granting similar cross-brain speed irrespective of size (Phillips et al., 2015). This indirectly predicts lack of, or slow, TTD in non-eutherian mammals (and speculatively in large dinosaurs) which have brains on average larger than extant birds but also no CC (Weisbecker and Goswami, 2010; Naumann, 2015; Font et al., 2019).
Conclusion
Our perspective aims at spurring more mammalian TTD research at the behavior-cognition-neuroscience interface. We highlight the value of back-forth human-animal translational approaches, especially important in TTD because the three disciplines have partly solved three parts of the puzzle. The path we propose might become easier now that the field of diffusion analyses is rapidly advancing (e.g., Berns et al., 2015). Compared to a few years ago, diffusion analyses are more sophisticated and their results are getting close to what is observed in vivo. White matter diffusion analysis research may help pinpoint TTD behaviors to specific aspects of micro- (density) or microstructure (volume) and to biologically plausible metrics of connectivity (e.g., fiber bundle capacity). We predict that humans will end up being one example within a plethora of mammalian instances of TTD: The “neural phylogenies” approach we suggest will hopefully provide a roadmap for future integrative work.
Data Availability Statement
The original contributions presented in this study are included in the article/supplementary material, further inquiries can be directed to the corresponding author/s.
Author Contributions
All authors listed have made a substantial, direct, and intellectual contribution to the work, and approved it for publication.
Funding
Center for Music in the Brain is funded by the Danish National Research Foundation (DNRF117). The Comparative Bioacoustics Group is funded by Max Planck Group Leader funding to AR.
Conflict of Interest
The authors declare that the research was conducted in the absence of any commercial or financial relationships that could be construed as a potential conflict of interest.
Publisher’s Note
All claims expressed in this article are solely those of the authors and do not necessarily represent those of their affiliated organizations, or those of the publisher, the editors and the reviewers. Any product that may be evaluated in this article, or claim that may be made by its manufacturer, is not guaranteed or endorsed by the publisher.
References
Aboitiz, F., and Montiel, J. (2003). One hundred million years of interhemispheric communication: the history of the corpus callosum. Braz. J. Med. Biol. Res. 36, 409–420. doi: 10.1590/s0100-879x2003000400002
Aboitiz, F., Scheibel, A. B., Fisher, R. S., and Zaidel, E. (1992). Fiber composition of the human corpus callosum. Brain Res. 598, 143–153. doi: 10.1016/0006-8993(92)90178-C
Anichini, M., de Heer Kloots, M., and Ravignani, A. (2020). Interactive rhythms in the wild, in the brain, and in silico. Can. J. Exp. Psychol. 74:170. doi: 10.1037/cep0000224
Badaruddin, D. H., Andrews, G. L., Bolte, S., Schilmoeller, K. J., Schilmoeller, G., Paul, L. K., et al. (2007). Social and behavioral problems of children with agenesis of the corpus callosum. Child Psychiatry Hum. Dev. 38, 287–302. doi: 10.1007/s10578-007-0065-6
Bartha-Doering, L., Kollndorfer, K., Schwartz, E., Fischmeister, F. P. S., Alexopoulos, J., Langs, G., et al. (2020). The role of the corpus callosum in language network connectivity in children. Dev. Sci. 24:e13031. doi: 10.1111/desc.13031
Bartha-Doering, L., Schwartz, E., Kollndorfer, K., Fischmeiser, F. P. S., Novak, A., Langs, G., et al. (2021). Effect of corpus callosum agenesis on the language network in children and adolescents. Brain Struc. Funct. 226, 701–713. doi: 10.1007/s00429-020-02203-6
Beens, K. D. (1995). The Effects of Interactive Songs on Syllable Production in a Child with Agenesis of the Corpus Callosum.
Bengtsson, S. L., Nagy, Z., Skare, S., Forsman, L., Forssberg, H., and Ullén, F. (2005). Extensive piano practicing has regionally specific effects on white matter development. Nat. Neurosci. 8, 1148–1150. doi: 10.1038/nn1516
Benichov, J. I., and Vallentin, D. (2020). Inhibition within a premotor circuit controls the timing of vocal turn-taking in zebra finches. Nat. Commun. 11, 1–10. doi: 10.1038/s41467-019-13938-0
Berns, G. S., Cook, P. F., Foxley, S., Jbabdi, S., Miller, K. L., and Marino, L. (2015). Diffusion tensor imaging of dolphin brains reveals direct auditory pathway to temporal lobe. Proc. R. Soc. B Biol. Sci. 282:20151203. doi: 10.1098/rspb.2015.1203
Bloom, J. S., and Hynd, G. W. (2005). The role of the corpus callosum in interhemispheric transfer of information: excitation or inhibition? Neuropsychol. Rev. 15, 59–71. doi: 10.1007/s11065-005-6252-y
Bridgman, M. W., Brown, W. S., Spezio, M. L., Leonard, M. K., Adolphs, R., Paul, L. P., et al. (2014). Facial emotion recognition in agenesis of the corpus callosum. J. Neurodev. Disord. 6:32. doi: 10.1186/1866-1955-6-32
Brown, W. S., Paul, L. K., Symington, M., and Dietrich, R. (2005). Comprehension of humor in primary agenesis of the corpus callosum. Neuropsychologia 43, 906–916. doi: 10.1016/j.neuropsychologia.2004.09.008
Buzsáki, G., Logothetis, N., and Singer, W. (2013). Scaling brain size, keeping timing: evolutionary preservation of brain rhythms. Neuron 80, 751–764. doi: 10.1016/j.neuron.2013.10.002
Casanova, M. F., El-Baz, A., Mott, M., Mannheim, G., Hassan, H., Fahmi, R., et al. (2009). Reduced gyral window and corpus callosum size in autism: possible macroscopic correlates of a minicolumnopathy. J. Autism Dev. Disord. 39, 751–764. doi: 10.1007/s10803-008-0681-4
Clarke, J. M., and Zaidel, E. (1994). Anatomical-behavioral relationships: corpus callosum morphometry and hemispheric specialization. Behav. Brain Res. 64, 185–202. doi: 10.1016/0166-4328(94)90131-7
Coleman, M. J., Day, N. F., Rivera-Parra, P., and Fortune, E. S. (2021). Neurophysiological coordination of duet singing. Proc. Natl. Acad. Sci. 118:e2018188118. doi: 10.1073/pnas.2018188118
Corballis, M. C., Corballis, P. M., and Fabri, M. (2004). Redundancy gain in simple reaction time following partial and complete callosotomy. Neuropsychologia 42, 71–81. doi: 10.1016/s0028-3932(03)00152-0
de Reus, K., Soma, M., Anichini, M., Gamba, M., de Heer Kloots, M., Lense, M., et al. (2021). Rhythm in dyadic interactions. Philos. Trans. R. Soc. B 376:20200337. doi: 10.1098/rstb.2020.0337
Demartsev, V., Strandburg-Peshkin, A., Ruffner, M., and Manser, M. (2018). Vocal turn-taking in meerkat group calling sessions. Curr. Biol. 28, 3661–3666. doi: 10.1016/j.cub.2018.09.065
Dougherty, R. F., Ben-Shachar, M., Deutsch, G. K., Hernandez, A., Fox, G. R., and Wandell, B. A. (2007). Temporal-callosal pathway diffusivity predicts phonological skills in children. Pro. Natl. Acad. Sci. 104, 8556–8561. doi: 10.1073/pnas.0608961104
Elmer, S., Hänggi, J., and Jäncke, L. (2016). Interhemispheric transcallosal connectivity between the left and right planum temporale predicts musicianship, performance in temporal speech processing, and functional specialization. Brain Struc. Funct. 221, 331–344. doi: 10.1007/s00429-014-0910-x
Fabri, M., Polonara, G., Pesce, M. D., Quattrini, A., Salvolini, U., and Manzoni, T. (2001). Posterior corpus callosum and interhemispheric transfer of somatosensory information: an fMRI and neuropsychological study of a partially callosotomized patient. J. Cogn. Neurosci. 13, 1071–1079. doi: 10.1162/089892901753294365
Fitzgerald, P. B., Brown, T. L., Daskalakis, Z. J., DeCastella, A., and Kulkarni, J. (2002). A study of transcallosal inhibition in schizophrenia using transcranial magnetic stimulation. Schizophr. Res. 56, 199–209. doi: 10.1016/S0920-9964(01)00222-5
Fitzpatrick, P., Frazier, J. A., Cochran, D. M., Mitchell, T., Coleman, C., and Schmidt, R. C. (2016). Impairments of social motor synchrony evident in autism spectrum disorder. Front. Psychol. 7:1323. doi: 10.3389/fpsyg.2016.01323
Font, E., García-Roa, R., Pincheira-Donoso, D., and Carazo, P. (2019). Rethinking the effects of body size on the study of brain size evolution. Brain Behav. Evol. 93, 182–195. doi: 10.1159/000501161
Friederici, A. D., and Alter, K. (2004). Lateralization of auditory language functions: a dynamic dual pathway model. Brain Lang. 89, 267–276. doi: 10.1016/S0093-934X(03)00351-1
Friederici, A. D., Cramon, D. Y., and Kotz, S. A. (2007). Role of the corpus callosum in speech comprehension: interfacing syntax and prosody. Neuron 53, 135–145. doi: 10.1016/j.neuron.2006.11.020
Fröhlich, M. (2017). Taking turns across channels: conversation-analytic tools in animal communication. Neurosci. Biobehav. Rev. 80, 201–209. doi: 10.1016/j.neubiorev.2017.05.005
Gazzaniga, M. S. (2000). Cerebral specialization and interhemispheric communication. Does the corpus callosum enable the human condition? Brain 123, 1293–1326. doi: 10.1093/brain/123.7.1293
Gooijers, J., and Swinnen, S. P. (2014). Interactions between brain structure and behavior: the corpus callosum and bimanual coordination. Neurosci. Biobehav. Rev.s 43, 1–19. doi: 10.1016/j.neubiorev.2014.03.008
Greenfield, M. D., Honing, H., Kotz, S. A., and Ravignani, A. (2021). Synchrony and rhythm interaction: from the brain to behavioural ecology. Philos. Trans. R. Soc. B 376:20200324. doi: 10.1098/rstb.2020.0324
Güntürkün, O., Ströckens, F., and Ocklenburg, S. (2020). Brain lateralization: a comparative perspective. Physiol. Rev. 100, 1019–1063. doi: 10.1152/physrev.00006.2019
Habibi, A., Damasio, A., Ilari, B., Veiga, R., Joshi, A. A., Leahy, R. M., et al. (2018). Childhood music training induces change in micro and macroscopic brain structure: results from a longitudinal study. Cereb. Cortex 28, 4336–4347. doi: 10.1093/cercor/bhx286
Hofer, S., and Frahm, J. (2006). Topography of the human corpus callosum revisited– comprehensive fibre tractography using diffusion tensor magnetic resonance imaging. NeuroImage 32, 989–994. doi: 10.1016/j.neuroimage.2006.05.044
Holler, J., Kendrick, K. H., Casillas, M., and Levinson, S. C. (2015). Editorial: turn-taking in human communicative interaction. Front. Psychol. 6:1919. doi: 10.3389/fpsyg.2015.01919
Horowitz, A., Barazany, D., Tavor, I., Bernstein, M., Yovel, G., and Assaf, Y. (2015). In vivo correlation between axon diameter and conduction velocity in the human brain. Brain Struc. Funct. 220, 1777–1788. doi: 10.1007/s00429-014-0871-0
Huang, H., Zhang, J., Jiang, H., Wakana, S., Poetscher, L., Miller, M. I., et al. (2005). DTI tractography based parcellation of white matter: application to the mid-sagittal morphology of corpus callosum. NeuroImage 26, 195–205. doi: 10.1016/j.neuroimage.2005.01.019
Hyde, K. L., Lerch, J., Norton, A., Forgeard, M., Winner, E., Evans, A. C., et al. (2009). Musical training shapes structural brain development. J. Neurosci. 29, 3019–3025. doi: 10.1523/JNEUROSCI.5118-08.2009
Kaur, M., Srinivasan, S. M., and Bhat, A. N. (2018). Comparing motor performance, praxis, coordination, and interpersonal synchrony between children with and without Autism Spectrum disorder (ASD). Res. Dev. Disabil. 72, 79–95. doi: 10.1016/j.ridd.2017.10.025
Kishimoto, R., and Seki, Y. (2022). Response timing of budgerigars in a turn-taking task under operant conditioning. Behav Process. 198:104638. doi: 10.1016/j.beproc.2022.104638
Kotz, S. A., and Schwartze, M. (2010). Cortical speech processing unplugged: a timely subcortico-cortical framework. Trends Cogn. Sci. 14, 392–399. doi: 10.1016/j.tics.2010.06.005
Kotz, S. A., and Schwartze, M. (2016). “Motor timing and sequencing in speech production: a general purpose framework,” in Neurobiology of Language, eds G. S. Hickock and S. L. Small (London: Elsevier), 717–723. doi: 10.1016/B978-0-12-407794-2.00057-2
Laìbadi, B., and Beke, A. M. (2017). Mental state understanding in children with agenesis of the corpus callosum. Front. Psychol. 8:94. doi: 10.3389/fpsyg.2017.00094
Lee, D. J., Chen, Y., and Schlaug, G. (2003). Corpus callosum: musician and gender effects. Neuroreport 14, 205–209. doi: 10.1097/00001756-200302100-00009
Levinson, S. C. (2016). Turn-taking in human communication – origins and implications for language processing. Trends Cogn. Sci. 20, 6–14. doi: 10.1016/j.tics.2015.10.010
Loui, P. (2018). Rapid and flexible creativity in musical improvisation: review and a model. Ann. N. Y. Acad. Sci. 1423, 138–145. doi: 10.1111/nyas.13628
Lumaca, M., Baggio, G., and Vuust, P. (2021). White matter variability in auditory callosal pathways contributes to variation in the cultural transmission of auditory symbolic systems. Brain Struct. Funct. 226, 1943–1959. doi: 10.1007/s00429-021-02302-y
Mann, N. I., Dingess, K. A., and Slater, P. J. B. (2006). Antiphonal four-part synchronized chorusing in a neotropical wren. Biol. Lett. 2, 1–4. doi: 10.1098/rsbl.2005.0373
Naumann, R. K. (2015). Even the smallest mammalian brain has yet to reveal its secrets. Brain Behav. Evol. 85, 1–3. doi: 10.1159/000375438
Nielsen, T., Montplaisir, J., and Lassonde, M. (1993). Decreased interhemispheric EEG coherence during sleep in agenesis of the corpus callosum. Eur. Neurol. 33, 173–176. doi: 10.1159/000116928
Norton, P., Benichov, J. I., Pexirra, M., Schreiber, S., and Vallentin, D. (2022). A feedforward inhibitory premotor circuit for auditory–vocal interactions in zebra finches. Proc. Natl. Acad. Sci. 119:e2118448119. doi: 10.1073/pnas.2118448119
Okobi, D. E. Jr., Banerjee, A., Matheson, A. M., Phelps, S. M., and Long, M. A. (2019). Motor cortical control of vocal interaction in neotropical singing mice. Science 363, 983–988. doi: 10.1126/science.aau9480
Ozturk, A. H., Tascioglu, B., Aktekin, M., Kurtoglu, Z., and Erden, I. (2002). Morphometric comparison of the human corpus callosum in professional musicians and non-musicians by using in vivo magnetic resonance imaging. J. Neuroradiol. 29, 29–34.
Park, H. J., Kim, J. J., Lee, S. K., Seok, J. H., Chun, J., Kim, D. I., et al. (2008). Corpus callosal connection mapping using cortical gray matter parcellation and DT-MRI. Hum. Brain Mapp. 29, 503–516. doi: 10.1002/hbm.20314
Paul, L. K., Van Lancker-Sidtis, D., Schieffer, B., Dietrich, R., and Brown, W. S. (2003). Communicative deficits in agenesis of the corpus callosum: nonliteral language and affective prosody. Brain Lang. 85, 313–324. doi: 10.1016/s0093-934x(03)00062-2
Phillips, K. A., Stimpson, C. D., Smaers, J. B., Raghanti, M. A., Jacobs, B., Popratiloff, A., et al. (2015). The corpus callosum in primates: processing speed of axons and the evolution of hemispheric asymmetry. Proc. R. Soc. B Biol. Sci. 282:20151535. doi: 10.1098/rspb.2015.1535
Pika, S., Wilkinson, R., Kendrick, K. H., and Vernes, S. C. (2018). Taking turns: bridging the gap between human and animal communication. Proc. R. Soc. B 285:20180598. doi: 10.1098/rspb.2018.0598
Pogarell, O., Teipel, S. J., Juckel, G., Gootjes, L., Möller, T., Bürger, K., et al. (2005). EEG coherence reflects regional corpus callosum area in Alzheimer’s disease. J. Neurol. Neurosurg. Psychiatry 76, 109–111. doi: 10.1136/jnnp.2004.036566
Pollmann, S., Maertens, M., von Cramon, D. Y., Lepsien, J., and Hugdahl, K. (2002). Dichotic listening in patients with splenial and nonsplenial callosal lesions. Neuropsychology 16:56. doi: 10.1037//0894-4105.16.1.56
Rauch, R. A., and Jinkins, J. R. (1994). “Magnetic resonance imaging of corpus callosum dysgensis,” in Callosal Agenesis: a Natural Split brain?, eds M. Lassonde and M. A. Jeeves (New York, NY: Plenum Press), 83–95. doi: 10.1007/978-1-4613-0487-6_11
Ravignani, A. (2019). Timing of antisynchronous calling: a case study in a harbor seal pup (Phoca vitulina). J. Comp. Psychol. 133:272. doi: 10.1037/com0000160
Ravignani, A., Verga, L., and Greenfield, M. D. (2019). Interactive rhythms across species: the evolutionary biology of animal chorusing and turn-taking. Ann. N. Y. Acad. Sci. 1453, 12–21.
Ringo, J. L., Doty, R. W., Demeter, S., and Simard, P. Y. (1994). Time is of the essence: a conjecture that hemispheric specialization arises from interhemispheric conduction delay. Cereb. Cortex 4, 331–343. doi: 10.1093/cercor/4.4.331
Risse, G. L., Gates, J., Lund, G., Maxwell, R., and Rubens, A. (1989). Interhemispheric transfer in patients with incomplete section of the corpus callosum: anatomic verification with magnetic resonance imaging. Arch. Neurol. 46, 437–443. doi: 10.1001/archneur.1989.00520400097026
Rogers, L. J., Vallortigara, G., and Andrew, R. J. (2013). Divided Brains: the Biology and Behaviour of Brain Asymmetries. Cambridge, MA: Cambridge University Press.
Sammler, D., Kotz, S. A., Eckstein, K., Ott, D. V. M., and Friederici, A. D. (2010). Prosody meets syntax: the role of the corpus callosum. Brain 133, 2643–2655. doi: 10.1093/brain/awq231
Schlaug, G., Jäncke, L., Huang, Y., Staiger, J. F., and Steinmetz, H. (1995). Increased corpus callosum size in musicians. Neuropsychologia 33, 1047–1055. doi: 10.1016/0028-3932(95)00045-5
Schlaug, G., Norton, A., Overy, K., and Winner, E. (2005). Effects of music training on the child’s brain and cognitive development. Ann. N. Y. Acad. Sci. 1060, 219–230. doi: 10.1196/annals.1360.015
Sperry, R. W. (1961). Cerebral organization and behavior. Science 133, 1749–1757. doi: 10.1126/science.133.3466.1749
Steele, C. J., Bailey, J. A., Zatorre, R. J., and Penhune, V. B. (2013). Early musical training and white-matter plasticity in the corpus callosum: evidence for a sensitive period. J. Neurosci. 33, 1282–1290. doi: 10.1523/JNEUROSCI.3578-12.2013
Stickles, J. L., Schilmoeller, G. L., and Schilmoeller, K. J. (2002). A 23-year review of communication development in an individual with agenesis of the corpus callosum. Int. J. Disabil. Dev. Educ. 49, 367–383. doi: 10.1080/1034912022000028349
Stivers, T., Enfield, N. J., Brown, P., Englert, C., Hayashi, M., Heinemann, T., et al. (2009). Universals and cultural variation in turn-taking in conversation. Proc. Natl. Acad. Sci. 106, 10587–10592. doi: 10.1073/pnas.0903616106
Su, W.-C., Culotta, M., Mueller, J., Tsuzuki, D., Pelphrey, K., and Bhat, A. (2020). Differences in cortical activation patterns during action observation, action execution, and interpersonal synchrony between children with or without autism spectrum disorder (ASD): an fNIRS pilot study. PLoS One 15:e0240301. doi: 10.1371/journal.pone.0240301
Suárez, R., Paolino, A., Fenlon, L. R., Morcom, L. R., Kozulin, P., Kurniawan, N. D., et al. (2018). A pan-mammalian map of interhemispheric brain connections predates the evolution of the corpus callosum. Proc. Natl. Acad. Sci. 115, 9622–9627. doi: 10.1073/pnas.1808262115
Takahashi, D. Y., Fenley, A. R., and Ghazanfar, A. A. (2016). Early development of turn-taking with parents shapes vocal acoustics in infant marmoset monkeys. Philos. Trans. R. Soc. B Biol. Sci. 371:20150370. doi: 10.1098/rstb.2015.0370
Tu, S., Doherty, D., Schilmoeller, K. J., and Schilmoeller, G. L. (2009). “Agenesis of the corpus callosum: a literature review,” in International Review of Research in Mental Retardation, Vol. 38, eds S. Tu, D. Doherty, K. J. Schilmoeller, and G. L. Schilmoe (Amsterdam: Elsevier Inc), 171–193. doi: 10.1016/S0074-7750(08)38007-0
Voineskos, A. N., Farzan, F., Barr, M. S., Lobaugh, N. J., Mulsant, B. H., Chen, R., et al. (2010). The role of the corpus callosum in transcranial magnetic stimulation induced interhemispheric signal propagation. Biol. Psychiatry 68, 825–831.
Weisbecker, V., and Goswami, A. (2010). Brain size, life history, and metabolism at the marsupial/placental dichotomy. Proc. Natl. Acad. Sci. 107, 16216–16221.
Westerhausen, R., Kreuder, F., Woerner, W., Huster, R. J., Smit, C. M., Schweiger, E., et al. (2006). Interhemispheric transfer time and structural properties of the corpus callosum. Neurosci. Lett. 409, 140–145.
Wing, A. M., Endo, S., Bradbury, A., and Vorberg, D. (2014). Optimal feedback correction in string quartet synchronization. J. R. Soc. Interface 11:20131125.
Zarei, M., Johansen-Berg, H., Smith, S., Ciccarelli, O., Thompson, A. J., and Matthews, P. M. (2006). Functional anatomy of interhemispheric cortical connections in the human brain. J. Anat. 209, 311–320.
Zeng, T., Przysinda, E., Pfeifer, C., Arkin, C., and Loui, P. (2017). White matter connectivity reflects success in musical improvisation. BioRxiv 218024. doi: 10.1101/218024
Keywords: bioacoustics, brain connectivity, turn-taking, time, music cognition, speech science
Citation: Ravignani A, Lumaca M and Kotz SA (2022) Interhemispheric Brain Communication and the Evolution of Turn-Taking in Mammals. Front. Ecol. Evol. 10:916956. doi: 10.3389/fevo.2022.916956
Received: 10 April 2022; Accepted: 23 June 2022;
Published: 29 July 2022.
Edited by:
Charles T. Snowdon, University of Wisconsin-Madison, United StatesReviewed by:
Giorgio Vallortigara, University of Trento, ItalySebastian Ocklenburg, Ruhr University Bochum, Germany
Copyright © 2022 Ravignani, Lumaca and Kotz. This is an open-access article distributed under the terms of the Creative Commons Attribution License (CC BY). The use, distribution or reproduction in other forums is permitted, provided the original author(s) and the copyright owner(s) are credited and that the original publication in this journal is cited, in accordance with accepted academic practice. No use, distribution or reproduction is permitted which does not comply with these terms.
*Correspondence: Andrea Ravignani, YW5kcmVhLnJhdmlnbmFuaUBtcGkubmw=; Massimo Lumaca, bWFzc2ltby5sdW1hY2FAY2xpbi5hdS5kaw==; Sonja A. Kotz, c29uamEua290ekBtYWFzdHJpY2h0dW5pdmVyc2l0eS5ubA==
†These authors share first authorship