- Department of Biology, Stanford University, Stanford, CA, United States
The multi-faceted impacts of the Anthropocene are increasingly modifying natural ecosystems and threatening biodiversity. Can small protected spaces conserve small mammal diversity across spatial and temporal scales of human impact? We identified small mammal remains from modern raptor pellets and Holocene archeological sites along a human modification gradient in the San Francisco Bay Area, CA and evaluated alpha and beta diversity across sites and time periods. We found that Shannon diversity, standardized species richness, and evenness decrease across modern sites based on level of human modification, with no corresponding change between Holocene sites. Additionally, the alpha diversity of modern sites with moderate and high levels of human modification was significantly lower than the diversity of modern sites with low levels of human modification as well as all Holocene sites. On the other hand, the small mammal communities from Jasper Ridge Biological Preserve, a small protected area, retain Holocene levels of alpha diversity. Jasper Ridge has also changed less over time in terms of overall community composition (beta diversity) than more modified sites. Despite this, Holocene and Anthropocene communities are distinct regardless of study area. Our results suggest that small mammal communities today are fundamentally different from even a few centuries ago, but that even relatively small protected spaces can partially conserve native faunal communities, highlighting their important role in urban conservation.
Introduction
The Anthropocene (here defined as 1950 CE to present) is characterized by extreme human impacts including rapid climatic change, pollution, defaunation, and landscape modification (Barnosky, 2014; Dirzo et al., 2014; Lewis and Maslin, 2015; Zalasiewicz et al., 2017). In this time of global change, we must leverage long-term and broad-scale biodiversity records to determine how to retain species in heavily modified environments, with the goal of informing conservation policy (Fraixedas et al., 2022). Habitat alteration and urbanization, specifically, fragment once-continuous natural landscapes and threaten biodiversity. A long-standing debate in conservation is how large protected areas must be in order to buffer species loss in increasingly modified landscapes (Gaston et al., 2008). Many studies have shown that larger reserves do a better job of conserving biodiversity, suggesting that “megareserves” may be necessary for the lasting protection of species and ecological processes (Newmark, 1987; Terborgh and Soule, 1999; Brashares et al., 2001; Gurd et al., 2001; Peres, 2005; Friedlander et al., 2007; Gaston et al., 2008). However, in places where the protection of large swaths of land is untenable, as in many human-dominated landscapes, small reserves may still be useful in harboring native biodiversity and ecosystem functions (Cowling and Bond, 1991). In such cases, can small protected spaces act as reservoirs for biodiversity across dynamic spatial and temporal gradients of human impact?
Jasper Ridge Biological Preserve (named “Ootchamin Ooyakma” by the Muwekma Ohlone; hereafter called Jasper Ridge) is a small protected area in Woodside, CA located only a few kilometers from Stanford University and the city of Palo Alto. Jasper Ridge is separated from these urban areas by open agricultural lands, golfing greens, and suburban housing and businesses (Figure 1). We surveyed small mammals along this gradient of human modification and compared them to their Holocene abundances using local zooarcheological data. We aimed to differentiate the spatial and temporal effects of human modification on small mammal communities in the Anthropocene. This is directly relevant to conservation because it will distinguish the specific impacts of different land management practices versus the broader-scale changes intrinsic to the Anthropocene which are beyond the scope of management. We ask: How has the Anthropocene impacted small mammals across gradients of time and human modification in terms of species richness, evenness, and community composition in the San Francisco Bay Area?
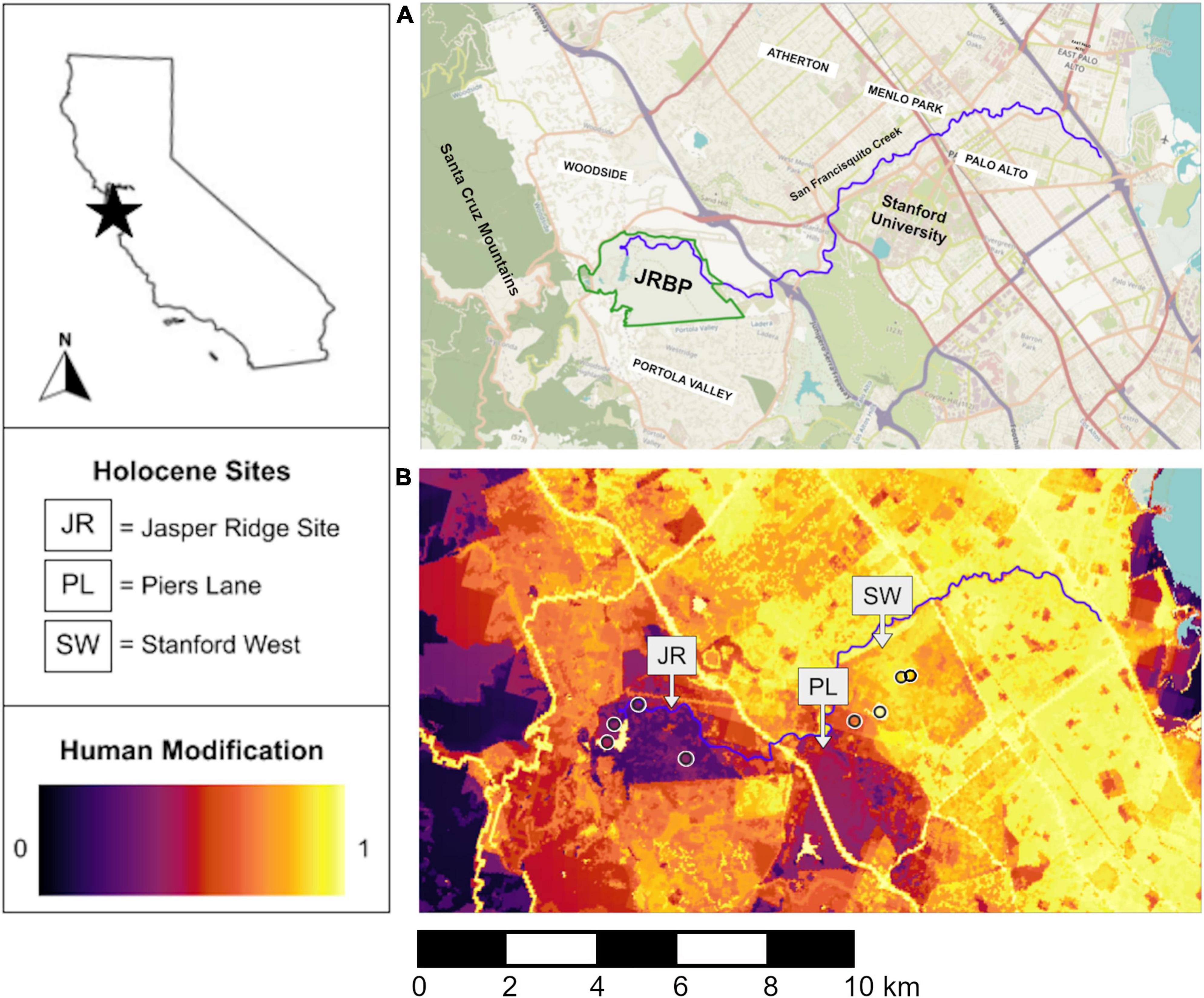
Figure 1. (A) Street view (JRBP, Jasper Ridge Biological Preserve) and (B) human modification maps of the study region. Anthropocene pellet collection sites are represented as open circles in (B). The human modification map is on a scale from 0 to 1, where 0 is pristine wilderness and 1 is completely transformed landscapes (Theobald, 2013).
Small mammals are ideal subjects for spatiotemporal studies due to their habitat specificity, small individual geographic ranges, population abundance, and ubiquity both today and in the recent fossil record (Barnosky, 1994; Yasuda et al., 2003). These factors, in addition to their low trophic position, also make small mammals excellent indicators of overall ecosystem health and integrity, with broad conservation implications (Avenant, 2011). Small mammals are increasingly being recognized for their critical role as hosts for pathogens of zoonotic potential (Young et al., 2014). Additionally, small mammals escaped the size-biased extinctions of the late-Quaternary and generally have low extinction risk due to their high fecundity, abundance, and growth rate (Lyons et al., 2004; Cardillo et al., 2005; Blois et al., 2010; Dirzo et al., 2014; Smith et al., 2018). As a result, they have remained taxonomically stable in many places of the American West over thousands of years (Kirkland, 1990; McGill et al., 2005; Rowe, 2007; Hadly et al., 2009; Blois et al., 2010; Terry, 2010; Rowe and Terry, 2014; Stegner, 2016; Fox, 2020). Despite their relative resilience to extinction, small mammal community composition may be altered by environmental change and human impacts in other, less obvious ways. Extinctions are the most dramatic and conspicuous losses of diversity and are therefore the focus of many studies on biodiversity decline in the Anthropocene (e.g., Barnosky et al., 2011). However, reductions in population and community-level diversity may reveal declines in ecosystem stability and may precede and predict extinctions (Blois et al., 2010; Ceballos et al., 2017). Therefore, tracking small mammal diversity over temporal gradients can elucidate the magnitude of human impacts on even robust species, with implications for ecosystem function (Naeem et al., 1994).
The Quaternary small mammal fossil record has shown that changes in species relative abundances in general, and declining evenness in particular, may be important proxies for identifying disturbance over temporal gradients (Blois et al., 2010; López-García et al., 2013; Tammone et al., 2020; Schap et al., 2021). Specifically, anthropogenic impacts tend to favor non-native, human-commensal, and disturbance-tolerant small mammal species at the cost of more sensitive native species (Bolger et al., 1997; Sauvajot et al., 1998; Clark and Bunck, 2011; Balestrieri et al., 2019). Species richness may therefore be maximized in areas of intermediate disturbance where small mammals with different tolerances can co-occur, though the dominance of disturbance-tolerant taxa may reduce evenness in impacted areas (Racey and Euler, 1982; Avenant, 2011). A study of butterfly and bird community composition from Jasper Ridge to Palo Alto in the late 1990s found that both groups experienced peaks in Shannon diversity and species richness at intermediate levels of development, with a filtering effect on some habitat specialists in highly modified sites (Blair, 1999). A recent resampling of birds from the same sites found dramatic population declines across all landscapes with some notable increases in disturbance-tolerant passerines, although declines were partially buffered in less disturbed areas (J. Wright-Ueda, in prep). We hypothesized that small mammal communities along this gradient would experience richness peaks at moderately impacted sites while evenness would decline with increasing modification due to the dominance of human-commensal small mammal species.
While humans have been modifying environments for at least 12,000 years in North America (Ellis et al., 2021), Holocene paleontological (i.e., non-human associated) and archeological (i.e., human-associated) deposits pre-date the accelerated landscape alteration and population growth of the Anthropocene and can provide a window into the pre-European past (Amorosi et al., 1996; Hadly, 1996; Dietl and Flessa, 2011; Lyman, 2012; Wolverton and Lyman, 2012; Lyman, 2012; Rick and Lockwood, 2013; Dietl et al., 2015; Kidwell, 2015; Barnosky et al., 2017). However, Holocene specimens from the rich archeological record of North America are underutilized for their conservation potential (Wolverton and Lyman, 2012; Lyman, 2012). To leverage zooarcheological specimens in conservation decision-making, we must ascertain whether differences between modern small mammal communities and their archeofaunal records indicate environmental change. Beta diversity mismatch between living and subfossil communities of marine (Kidwell, 2007), lacustrine (Michelson et al., 2018), and terrestrial (Terry, 2009) fauna have all been shown to record human impact. One study in the Great Basin showed that the small mammal community from a site that had undergone human modification was more dissimilar to its paleontological baseline than a less modified site was to its baseline (Terry, 2010; Rowe et al., 2014). We apply a similar method to determine whether mismatches between zooarcheological and modern small mammal communities can capture landscape alteration between the late Holocene and Anthropocene in a more urbanized context. We additionally test whether small mammal remains from archeological sites can record ecological differences in the local environment by tracking indicator species at each Holocene site. If the indicator species match with habitat differences between sites, it will suggest that small mammal zooarcheological records can act as an effective and efficient tool for providing paleoenvironmental baselines.
Study area
The San Francisco Bay Area (hereafter the Bay Area) is a biodiversity hotspot which has experienced rapid urbanization, agricultural intensification, and extensive human population growth in the past few decades (Myers et al., 2000). However, the Bay Area also has many protected spaces due to public support for open-space conservation (Rissman and Merenlender, 2008; Walker, 2009). The proximity of both highly urbanized and protected spaces allows for gradients of human disturbance across short geographical distances (Blair, 1999; Connor et al., 2002). As part of the recent 30 × 30 initiative, California is actively looking for ways to better steward its existing protected spaces and prioritize where to create new ones to both maximize native biodiversity and meet human needs, including access to nature (Draft Pathways to 30x30, 2021). Therefore, research into the use of small preserves as biodiversity reservoirs in urban and suburban landscapes is of immediate and urgent utility for both policy-making and conservation science.
Here we focus on lands in the south Bay Area which are owned by Stanford University (hereafter Stanford Lands). This area underwent documented changes since the arrival of Europeans around 1769. By 1793 the Spanish Governor of California had banned the use of fire as a land management tool, ceasing thousands of years of controlled burns conducted by the native Ohlone people and causing a dramatic shift in the prevailing fire regime of the region (Bocek and Reese, 1992; Anderson, 2005). The introduction of European grasses and grazing by cattle, sheep, and horses have also dramatically changed the landscape (Bocek and Reese, 1992). The south Bay Area, including Stanford Lands, experienced heavy logging in the 19th century, which decimated local old growth forests and introduced non-native conifers and hardwoods such as eucalyptus (Bocek and Reese, 1992). We therefore expected differences in small mammal communities between the pre-colonial Holocene and today due to historical land modification. However, we predicted that differences would be less pronounced at sites that are under less pressure from human impacts today.
Materials and methods
Anthropocene pellet collection and data
We estimated modern small mammal community composition using bones and teeth dissected from raptor pellets. While we did not monitor roosts, the size and morphology of the pellets are consistent with those produced by Tyto alba. Barn owls (T. alba) have been shown to sample small mammal communities in proportion to their local abundance and offer less biased subsamples of local small mammal diversity than other common survey practices (Hadly, 1999; Andrade et al., 2016). Additionally, a recent study showed that raptor pellets accurately reflect local small mammal community composition regardless of raptor identity (Viteri et al., 2021).
Pellets were collected intermittently from 1999 to 2021 along a short (∼8 km long) human modification gradient on Stanford Lands (Figure 1). The foraging radius of barn owls averages 2.5–3 km, so although there may be some overlap between the closest sites, we expected variation in diet even across this small spatial scale (Taberlet, 1983; Purger and Szép, 2022). We analyzed raptor pellets from three study areas—within Jasper Ridge (JR), on Stanford Campus (SC), or in the middle (MI)—which approximately correspond to the location of the three included archeological deposits (Figure 1). We identified small mammal (here defined as mammals the size of Lepus californicus or smaller) craniodental remains (number of identified specimens = NISP) for each species at each collection site and year using the comparative collections in the Hadly lab at Stanford University and Gilbert (1980) as references.
Holocene sites and data
We used subfossil records from three late-Holocene archeological sites to provide a baseline of past small mammal community composition on Stanford Lands (Figure 1). For all three sites, we identified small mammal specimens both from previously sorted faunal remains as well as unsorted heavy fraction material for each excavation unit and level (depth). We taxonomically identified craniodental remains using the same methods as for the Anthropocene materials.
All three Holocene sites are cemetery and occupation midden deposits situated along San Francisquito Creek, which drains directly into the San Francisco Bay. Faunal remains in these sites are primarily cultural materials accumulated by the ancestral Ohlone, but “natural” processes such as small mammal burrowing and bone deposition by raptors and other carnivores likely also contributed to the small mammal component of the sites (Bocek, 1986). We therefore expected a possible overrepresentation of both larger, calorie-rich small mammals that may have been actively hunted by the Ohlone (such as leporids) and strongly fossorial species with may have been intrusive to the deposit (such as gophers) relative to raptor accumulations. These potential biases are discussed further below. During the Holocene, these sites were within a continuous riparian woodland along this drainage. They span a slight elevation gradient with the Jasper Ridge Site being the highest (73 m above sea level), Stanford West being the lowest (28 m above sea level), and Piers Lane falling in between (52 m above sea level) (Bocek, 1987). All materials from these sites are stored and accessioned at the Stanford University Archeology Collections. While the sites were excavated at different times with overlapping but non-identical personnel, the collection methodology was largely consistent between them. However, differences in the sieve size used to screen materials between sites have the potential to impact the recovery of very small mammal remains (Shaffer and Sanchez, 1994). This bias was avoided by reprocessing all the material that went through the sieves in the lab.
The Jasper Ridge Site (CA-SMA-204), located in the foothills of the Santa Cruz Mountains, is 1.4 km downstream of the confluence of San Francisquito Creek and Bear Creek (Bocek, 1987; Figure 1). Historically, this site would have been adjacent to open grassland, mixed hardwood forest, and chaparral (Kelly et al., 2005). Radiocarbon dates range from 1,850 (±30) to 145 (±20) years before present (ybp) (Viteri and Hadly, 2021, preprint). Materials from this site were excavated in twenty-four 1 × 2-meter units and 10 cm levels and passed through a 1/4 inch screen (Bocek, 1987). The identifiable remains larger than 1/4 inch were sorted and analyzed by Bocek (1987) while smaller matrix was divided into heavy and light fractions via flotation and left unsorted. From 2017 to 2020, we verified the existing small mammal identifications and sorted the heavy fraction material to identify additional small mammal remains.
The Piers Lane Site is located in Stanford, California on the southern bank of San Francisquito Creek between the Jasper Ridge and Stanford West sites (Figure 1). The site was excavated by Laura Jones, Stanford University Archeologist, and her team in 2015. In the Holocene, Piers Lane would have been next to chaparral, oak woodlands, and oak grasslands (Brown, 1964). Radiocarbon dating of charcoal resulted in dates of 2,470 (± 30) and 220 (± 30) ybp from depths of ∼1 m and 40 cm, respectively (Laura Jones, personal communication). Eighteen units were excavated in 10 cm levels until sterile soil was reached (∼1.5 m) and passed through a 1/8 inch screen. For each excavation level and unit, faunal material larger than 1/8 inch was separated by identifiable vs. unidentifiable bone while smaller material was separated by flotation. We sorted and identified small mammal remains from both the identifiable bone bags and heavy fraction materials.
Stanford West is a large site (∼12,000 m2) in Palo Alto, CA, United States (Figure 1) that was excavated in the early 1990s following the same methods as the Jasper Ridge Site excavation (Bocek, 1987). The site would have been adjacent to oak savannah, grasslands, chaparral, and marshland (Brown, 1964). The area around this site is currently used as housing for Stanford University affiliates. Radiocarbon dates for this site yielded a maximum date of 3,190 (±200) ybp and a minimum date of 440 (±90) ybp from 160 and 90 cm below surface, respectively, (Laura Jones, personal communication). We sorted and identified small mammal remains from both the separated faunal bags and heavy fraction materials for each excavation unit and level.
Gradient of human modification
We used a high-resolution map of human modification produced by Theobald (2013) to quantify modern gradients of human impacts. This metric ranges from 0 to 1, where 0 approximates natural environments with no human modification and 1 is completely transformed habitat dominated by the built environment (Theobald, 2013; Theobald et al., 2020). The human modification metric incorporates both the footprint and magnitude of impact for various stressors including land cover type, land use, and roads (Theobald, 2013). For each Anthropocene site we calculated the average human modification value in a 1-km radius around the pellet sampling location as in Kross et al. (2016) and Hindmarch and Elliott (2015) using QGIS (QGIS.org, 2022, version 3.2.0; Figure 1).
The “Jasper Ridge” study area sits within Jasper Ridge Biological Preserve and contains our least modified sites. The preserve is a modest 4.9 km2 of land but encompasses many habitat types including chaparral, serpentine grassland, redwood forest, and oak woodland (Holl, 2003). The preserve is closed to the public but supports the day-time presence of researchers and a small staff. The human modification scores of the Jasper Ridge pellet collection sites range from 0.392 to 0.489. Jasper Ridge has a history of human disturbance prior to becoming a nature preserve in 1973, including use as a recreational area during much of the 20th century (Bocek and Reese, 1992). The preserve also contains Searsville Reservoir, which captures streams in the San Francisquito drainage that would have flowed freely prior to the 1890s. This history of human disturbance and the modification of the landscape by the Searsville Dam is likely why the human modification scores at Jasper Ridge are not lower (Salafsky et al., 2008; Theobald, 2013). Even within the region, more “pristine” spaces exist to the west, but none that are contiguous with this small island of protected lands surrounded by residential and suburban areas (Figure 1).
Our “Middle” study area, both in terms of geography and human modification level, is Stanford’s Student Observatory. The Observatory is the modern site closest to Piers Lane, located between Jasper Ridge and Stanford campus and representing moderate human impact (Human Impact Score = 0.698; Figure 1). This site is exceptional due to the presence of two owl boxes, under which many years of small mammal bones have accumulated. The Observatory sits in a small patch of oak woodland bordering a popular hiking area, the Dish, on one side and Stanford campus on the other (Figure 1). While this area is not barred from public access, the observatory itself is not regularly open to the public. The nearby Dish, however, is frequented by ∼600,000 people annually, although they are restricted to trails during daylight hours and dogs are not allowed (Stanford University, 2022).
The “Stanford Campus” study area includes three collection sites on the main campus of Stanford University, which supports ∼37,000 researchers, staff, and students, many of whom live on or nearby the campus (Stanford University, 2022). The landscape is dominated by buildings and roads and is frequently under construction, with ensuing noise and dust. Although there are green spaces, they are typically highly manicured with non-native plants. The human modification scores of the Stanford campus sites range from 0.805 to 0.845.
Analytical methods
To assess the relationship between the degree of landscape alteration and small mammal diversity, we first calculated small mammal abundances at each Anthropocene collection site and excluded sites with low sample size (NISP < 50). We then ran linear regressions between the human modification scores of each collection site and three alpha diversity metrics: Shannon diversity, standardized species richness, and evenness. Shannon diversity is a widely used alpha diversity metric which incorporates both species richness and evenness. However, we also independently evaluated both richness and evenness with other metrics to understand their individual contributions. We calculated standardized species richness using shareholder quorum subsampling (SQS) with a quorum level of 0.7 and 1,000 iterations (Alroy, 2010). SQS is a “fair” sampling technique that yields the number of species you would find at each site with a fixed quorum, or coverage, of the underlying abundance distribution (Alroy, 2010). We also calculated taxon evenness using the probability of interspecific encounter (PIE), following the methods of Davis (2005) and Stegner (2016). Both SQS and PIE are independent of sample size, allowing for more robust comparison across differently sized groups.
We then compared alpha and beta diversity of Anthropocene and Holocene sites to assess whether diversity changed within or between time bins and study areas. While we recognize that the distinct pathways leading to small mammal preservation in the Anthropocene vs. Holocene sites, we believe they are comparable because humans and raptors are thought to sample small mammals opportunistically and locally (Simms, 1987; Smith, 2003; Andrade et al., 2016; Viteri et al., 2021). We grouped the Anthropocene sites by study area (Jasper Ridge, Middle, and Stanford Campus) and collection year, except Middle, which was divided into two collection months in order to have replicates. When Anthropocene and Holocene sites were compared, we combined Holocene excavation levels into 30-cm bins to increase sample sizes and make them more comparable to those of Anthropocene sites. Low sample size groups (NISP < 50) were dropped from the analysis. We also removed particular taxa that may have different biases between Holocene and Anthropocene sampling when comparing between time bins. Sciurids were removed since nocturnal owls rarely overlap temporally with diurnal mammals, and therefore miss that component of local diversity. Pocket gophers (Thomomys bottae) were also excluded since their remains are partially intrusive in Bay Area archeological sites and are therefore overrepresented in Holocene deposits (Bocek, 1986; Viteri and Hadly, 2021, preprint). When comparing solely between Holocene assemblages, we included sciurids and gophers, binned by excavation unit, and dropped units that had a sample size below NISP = 25 based on rarefaction.
We evaluated Shannon diversity, SQS, and PIE for each Holocene site and Anthropocene study area. We also compared the Bray-Curtis dissimilarities of Holocene sites and their Anthropocene geographical equivalents in order to test whether community turnover was more pronounced in more modified study areas. To account for zero-inflated data and different sample sizes, we standardized our abundance data using a Hellinger transformation (Legendre and Gallagher, 2001). Since data did not meet the criteria of homoscedasticity and normal distribution, we tested for global differences between groups using a Kruskal–Wallis test and pairwise differences using a Wilcoxon test with Holm p-value correction.
We tested for significant differences between groups using permutational multivariate analysis of variance (PERMANOVA) with Bray-Curtis dissimilarities. We used Holm p-value corrections and tested for overdispersion within our groupings using the betadisper function in vegan (Oksanen, 2019). When multiple groupings were compared, we tested for pairwise differences using the adonis.pair function in the EcolUtils package (Salazar, 2020). We visualized community differences with non-metric multidimensional scaling (NMDS). NMDS is an ordination technique used for non-parametric data. We used the metaMDS function from the vegan package (Oksanen, 2019) which automatically rotates the NMDS axes such that NMS axis 1 explains the dominate source of variation, as in other common ordination methods such as principal component analysis. We used 100 random starts to ensure that a stable solution was reached for each NMDS plot.
In order to understand which small mammal species characterize each study area we performed an indicator value analysis using the indval function from the R labdsv package (Roberts, 2019). All analyses were done using the R program for statistical computing (version 3.6.1; R Core Team, 2019).
Results
We identified a total of 4,751 small mammal craniodental elements from the Holocene sites and 11,531 from the Anthropocene pellet accumulations (Table 1). Four taxa—Rattus spp., Mus musculus, Neurotrichus gibbsii, and Sorex cf. ornatus—were found in Anthropocene but not Holocene samples, while only one species, Chaetodipus californicus, was recovered exclusively in the Holocene deposits (Table 1). We found that small mammal Shannon diversity, standardized species richness, and evenness decrease with increasing levels of human modification across Anthropocene collection sites (p-values: 0.026, 0.020, and 0.021 respectively) (Figure 2). We also found that Anthropocene small mammal communities from the Middle and Stanford Campus study areas are less rich and less even than Jasper Ridge and Holocene sites in all cases (Figure 3). While significant p-values differentiating Anthropocene Stanford Campus from Holocene sites and Anthropocene Jasper Ridge are reported in Figure 3, none of these splits are significant with Holm p-value corrections, likely due to the number of pairwise comparisons. However, when Holocene sites are lumped (due to their virtual equivalence), the Stanford Campus study area is significantly different from the Holocene sites in terms of Shannon diversity (p-value = 0.052), SQS (p-value = 0.0087), and PIE (p-value = 0.033) and Anthropocene Jasper Ridge in terms of Shannon diversity (p-value = 0.079) and SQS (p-value = 0.0159) with Holm p-value correction. With and without Holm p-value corrections, there is no significant difference in any alpha diversity metric across Holocene sites or between Anthropocene Jasper Ridge and the Holocene sites (Figure 3). Additionally, the Holocene fauna of Jasper Ridge are more similar (lower Bray-Curtis dissimilarity) to the modern Jasper Ridge fauna than Holocene vs. Anthropocene communities of the more impacted study areas (Figure 4). Only Stanford and Jasper Ridge are significantly different with Holm p-value correction (p-value = 0.048).
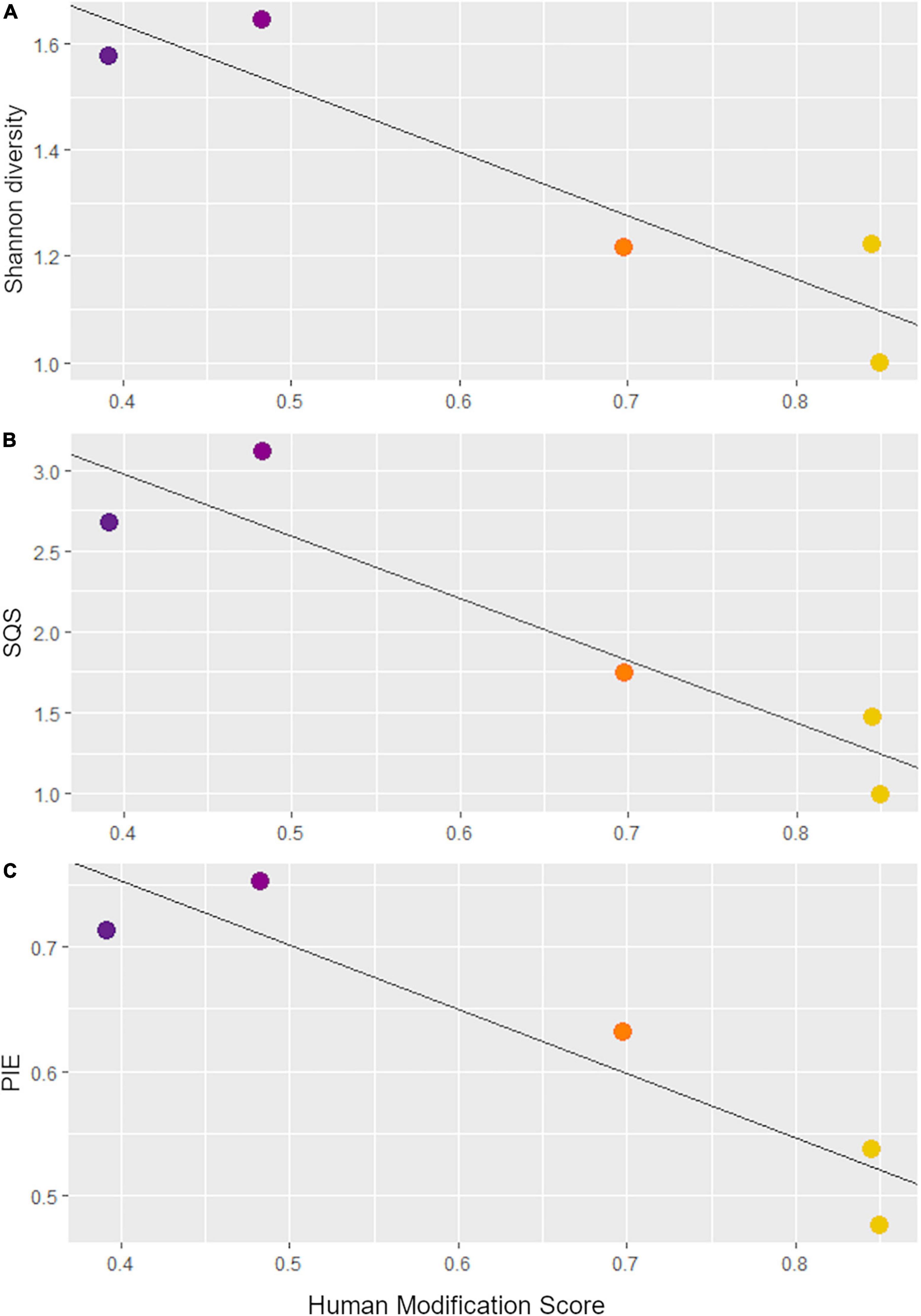
Figure 2. Linear regressions of the human modification scores for each Anthropocene collection site versus (A) Shannon diversity, (B) shareholder quorum subsampling (with a quorum of 0.7 and 1,000 iterations), and (C) probability of interspecific encounter values. Points are colored based on their human modification score according to the scale in Figure 1.
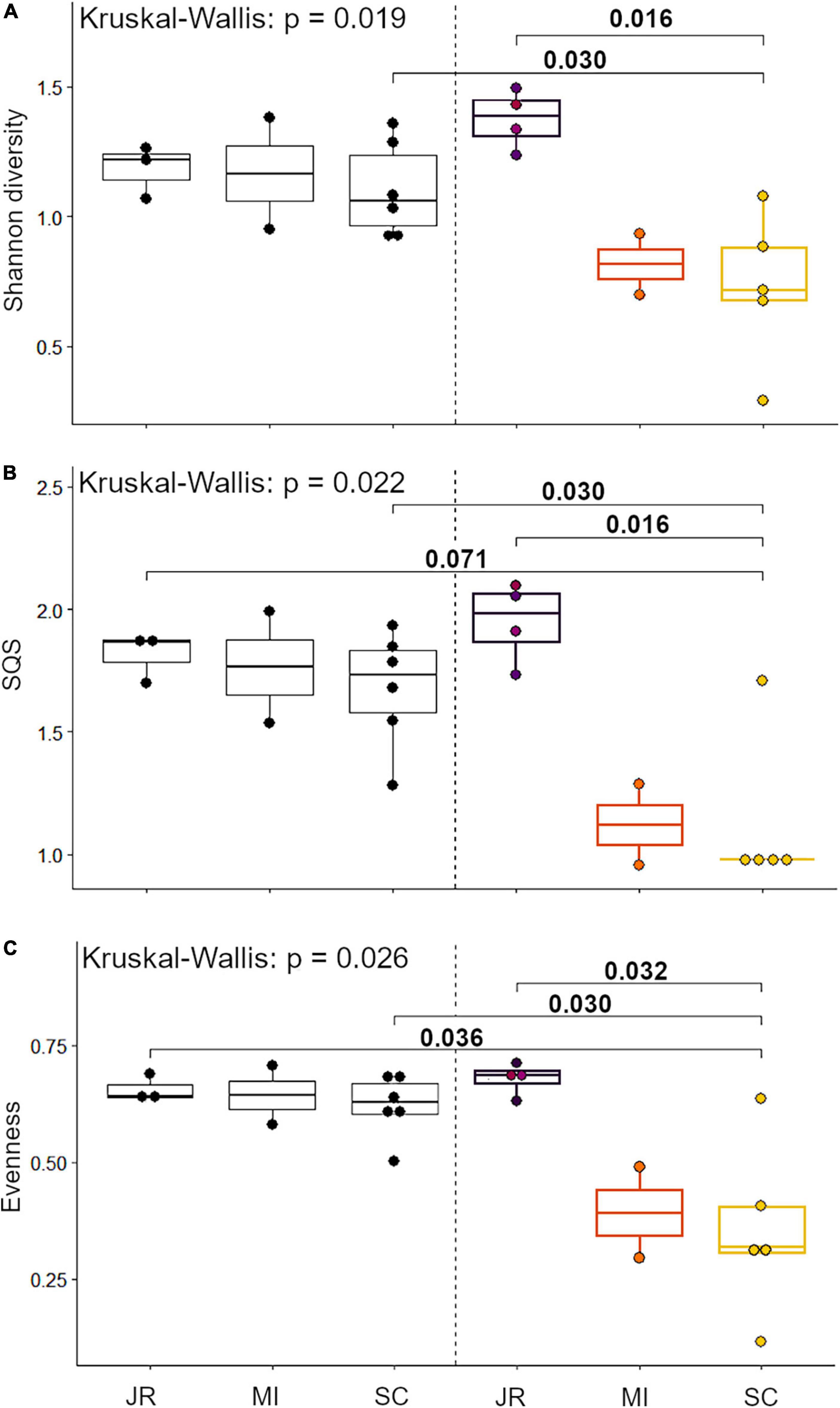
Figure 3. Quantification of alpha diversity using (A) Shannon diversity, (B) shareholder quorum subsampling (with a quorum of 0.7 and 1,000 iterations), and (C) probability of interspecific encounter across Holocene and Anthropocene sites (JR, Jasper Ridge; MI, Middle, SC, Stanford Campus). Anthropocene points colored based on human modification scores according to the scale in Figure 1. Scores and colors were averaged when multiple collection sites were binned. Pairwise comparisons done using a Wilcoxon test.
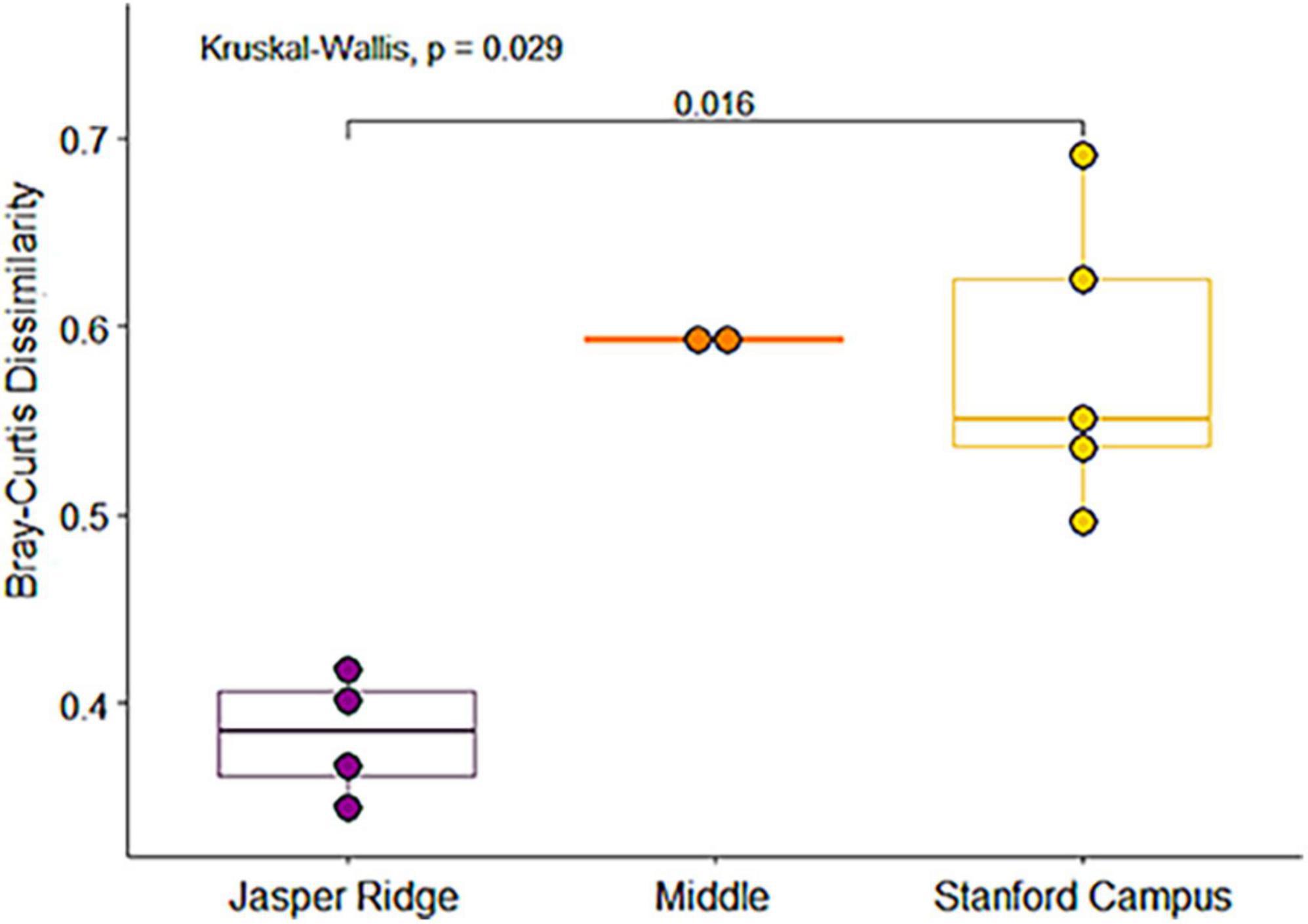
Figure 4. Boxplot of Bray-Curtis dissimilarities between Anthropocene collection sites and their corresponding Holocene archeological site by study area. Pairwise significance based on a Wilcoxon test.
The Holocene sites are significantly different from each other in terms of community composition based on PERMANOVA (global p-value = 0.0010; pairwise p-values = JR vs. PL: 0.079; JR vs. SW: 0.0030; PL vs. SW; 0.0060). However, the assumption of homogeneity of dispersion between groups is not met (betadisper p-value = 0.0069). However, visual comparison with NMDS shows that the groups are indeed distinct, especially the Jasper Ridge and Stanford West sites (Figure 5A).
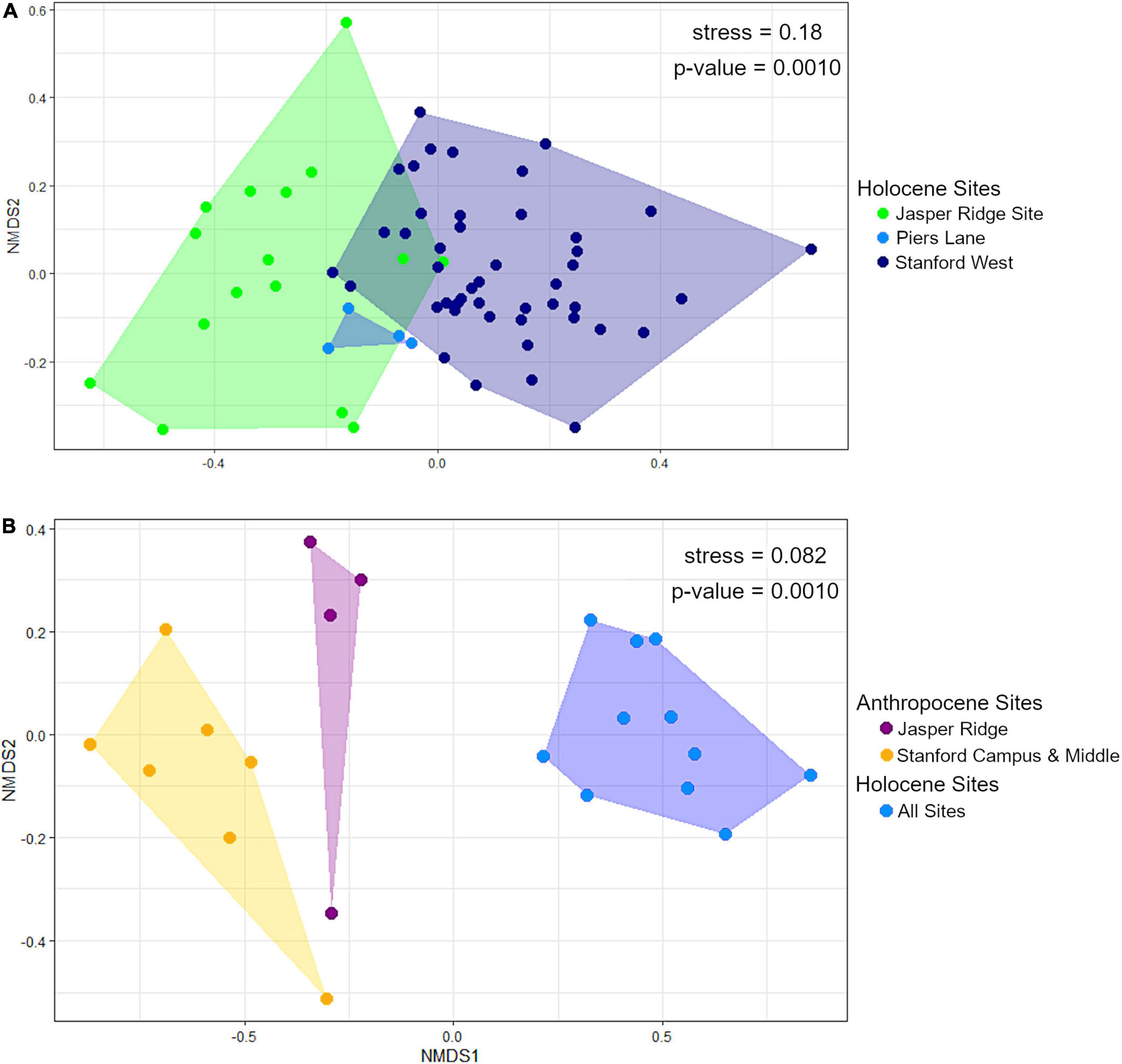
Figure 5. NMDS plots of (A) Holocene sites by unit, and (B) Anthropocene (binned by collection year) and Holocene (binned in 30-cm levels) sites. For panel (B). Anthropocene Stanford campus and Anthropocene Middle as well as all Holocene sites are grouped together because they are not significantly different from each other.
Holocene and Anthropocene communities cluster together regardless of collection locality (p-value = 0.0010) and pass the homogeneity of variance assumption (betadisper p-value = 0.67). When further subdivided by study area, the Anthropocene Jasper Ridge and Stanford Campus sites are distinct from each other, but the Middle sites are nested within the Stanford Campus ones. Holocene communities are also not significantly different from each other once gophers and sciurids are removed (Figure 5B), likely because these species partially drove their separation in Figure 5A. Stanford Campus and Middle as well as all Holocene sites are therefore combined for NMDS visualization and further analysis (Figure 5B). Together, the Anthropocene Stanford Campus and Middle study areas are significantly different from Anthropocene Jasper Ridge (p-value = 0.0070) and all Anthropocene samples are distinct from the Holocene ones (p-value = 0.0030) with Holm correction. The homogeneity of dispersion assumption is met (betadisper p-value = 0.38). While the Anthropocene sites cluster together, the Jasper Ridge pellets fall more closely along NMDS axis 1 to the Holocene sites than the more impacted Middle and Stanford Campus collection sites (Figure 5B).
The indicator value analysis for Holocene-only communities showed that abundant Microtus californicus characterizes the Jasper Ridge Site, while Neotoma fuscipes and Chaetodipus californicus characterize Piers Lane, and Thomomys bottae characterizes Stanford West. The significant indicator taxa for Anthropocene and Holocene groups are: Rattus spp., Mi. californicus, and Mus musculus for the Anthropocene Stanford Campus and Middle sites, Reithrodontomys megalotis and Sorex cf. ornatus for Anthropocene Jasper Ridge, and Leporidae and N. fuscipes for Holocene sites.
Discussion
The species richness of small mammal communities declines across a gradient of human modification in our study system (Figure 2). This is in contrast to previous studies which suggest that areas with moderate disturbance have the highest diversity (e.g., Racey and Euler, 1982). These results are also counter to Blair’s (1999) study of insect and bird diversity across this same gradient, which found that areas with intermediate modification maximized species richness and Shannon diversity in both taxonomic groups. We also confirm that small mammal evenness decreases with increasing modification (Figure 2), likely due to the dominance of disturbance-tolerant and human-commensal species which are associated with more modified sites. This result highlights the importance of including evenness in biodiversity measurements as an indicator of human impacts.
All three Holocene sites have consistently species-rich and even small mammal communities in comparison to impacted Anthropocene sites (Figure 3). Despite these similarities, the sites are distinct in terms of overall community composition in ways that track with their local environments prior to European modification (Figure 5A). While all sites were primarily located in riparian woodlands, the Jasper Ridge Site would have been the closest to open grasslands (Kelly et al., 2005). Therefore, the abundance of Microtus californicus, a grassland-associated species, tracks with the local habitat (Heske et al., 1984). The chaparral and oak woodland habitats near Piers Lane correspond well with the affinities of its indicator species, Neotoma fuscipes and Chaetodipus californicus (Brown, 1964; Carraway and Verts, 1991; Heske et al., 1997; Chaudhary et al., 2021). Thomomys bottae, the indicator species for Stanford West, is found in many open environments with loose, deep soil but is especially characteristic of ecotones between chaparral and grasslands (Quinn, 1990). This matches with the long oak grassland/chaparral boundary adjacent to Stanford West (Brown, 1964). The significant differences in small mammal communities between Holocene sites (Figure 5A) in combination with the correspondence of indicator species with local habitat variation suggests that small mammal remains from archeological sites retain an environmental signal. Therefore, small mammal relative abundances from Holocene archeological sites may be useful in the reconstruction of past environments.
Although the Holocene sites retain environmental variation, we found that there are much larger differences in alpha diversity today than in the Holocene across the same spatial scales, showing that human habitat transformation today is more impactful to small mammal richness and evenness than is natural habitat variation in this region (Figure 2). However, despite being a small preserve with a history of disturbance, Anthropocene Jasper Ridge retains Holocene levels of alpha diversity (Figure 3). Our sites with both high and moderate levels of modification have lower diversity values than all Holocene and Jasper Anthropocene communities, although the low replicates of Anthropocene and Holocene Middle prevent statistical significance (Figure 3). Anthropocene Jasper Ridge also matches its Holocene community composition much more closely than both the Middle and Stanford Campus communities resemble theirs (Figure 4). This both confirms that Jasper Ridge is able to partially conserve small mammal diversity and shows that greater discordance between archeological baselines and modern small mammal communities reflects higher levels of human modification. However, even Jasper Ridge, which has been protected for decades, has fundamentally distinct small mammal communities today than in the Holocene (Figure 5B). While Jasper Ridge small mammal communities have changed less over time than the more impacted sites (Figure 4), they are more similar to other Anthropocene sites than they are to Holocene Jasper Ridge communities (Figure 5B).
More modified Anthropocene sites (Stanford Campus and Middle) are characterized by the presence of non-native and human-commensal species (Rattus spp. and Mus musculus) as well as native disturbance-tolerant species (Mi. californicus), while Anthropocene Jasper Ridge is characterized by Sorex cf. ornatus which is more sensitive to disturbance (Sauvajot et al., 1998; Clark and Bunck, 2011; Quinn et al., 2018; Balestrieri et al., 2019). The other indicator species for Jasper Ridge, Reithrodontomys megalotis, is a grassland-associated species, which likely reflects the abundance of pellets collected from the preserve’s serpentine grassland. R. megalotis abundances are limited by Mi. californicus where the two species overlap (Heske et al., 1984). Therefore, the local abundance of R. megalotis may point to lower Mi. californicus numbers in serpentine grasslands, a possibility which has been reported earlier (Jasper Ridge Annual Report 2007–2008). These grasslands are characterized by native grasses and forbs rather than the exotic flora of non-serpentine grasslands in the rest of the preserve (Moloney et al., 1992). The serpentine grasslands at Jasper Ridge may also be too xeric to be optimal for Mi. californicus (Lawrence, 1966; Laurance and Coan, 1987). Due to the average foraging radius of barn owls (2.5–3 km), there may be some possible overlap between pellet collection sites (Taberlet, 1983; Purger and Szép, 2022). This may especially explain the nested relationship between the Middle and Stanford Campus sites. However, since the Jasper Ridge and Stanford Campus collections sites are ∼6 km from each other, their overlap is likely to be minimal. Additionally, the close correlations between all alpha diversity metrics and human modification scores as well as the matching of indicator species which each study area’s level of disturbance and environment suggest that our results are robust.
Three of the Anthropocene indicator taxa—Rattus spp., Mus musculus, and Sorex cf. ornatus—in addition to Neurotrichus gibbsii are only found in the Anthropocene samples (Table 1). Since Rattus spp. and Mu. musculus were introduced post-colonially, we would not expect to find them in the archeological deposits. We presume that N. gibbsii and Sorex cf. ornatus, native small mammals, were missed from the Holocene deposits due to their small body sizes and low natural abundances, which is reflected in their poor fossil records. However, both species occupy a similar ecological niche, so their joint absence is notable (Dalquest and Orcutt, 1942). A potential explanation is that N. gibbsii and Sorex ornatus thrive in low, dense vegetation that may have been rare around the Holocene sites due to frequent controlled burns by the Ohlone (Owen and Hoffmann, 1983; Anderson, 2005). They additionally both primarily subsist on earthworms and other soil invertebrates, so their absence may reflect changes in the soil biota. One species, Chaetodipus californicus, is found in Holocene but not Anthropocene sites, suggesting that it may have been extirpated from the area. C. californicus is typically found at edges of chaparral which have largely been removed at the Middle and Stanford Campus study areas where C. californicus was found in the Holocene (Table 1; Quinn, 1990; Chaudhary et al., 2021). In general, chaparral was much more extensive in the region in the past (Cooper, 1926). Notebooks by Joseph Grinnell of the University of California Museum of Vertebrate Zoology show that he trapped C. californicus on Stanford Lands in the early 1900s (Grinnell, 1901). Additionally, a trapping survey at Jasper Ridge in 2007 documented this species, but also reports that it was absent in decades of prior small mammal studies (Jasper Ridge Annual Report 2007–2008). This suggests that while C. californicus may still be found in the study region, it may persist in low abundances and its presence has likely not been continuous over time. Unlike many other small mammals in this region, C. californicus is mainly solitary and has low intrinsic rates of population growth, making it more vulnerable to disturbance (Chaudhary et al., 2021). They are also granivores, so a paucity of seeds in the mowed lawns of Stanford Campus may also contribute to their absence there (Chaudhary et al., 2021).
Our modern sites do not include the full range of habitat modification in the Bay Area, with our “pristine” study area having experienced historical disturbance and our “urban” location being fairly suburban (Figure 1). However, this serves to strengthen our findings as even this conservative human modification gradient produces stark contrasts in mammal communities. We expect that a more inclusive human impact gradient (from highly “pristine” to highly urban) would have even more extreme differences in small mammal community composition.
One potential source of bias in our dataset is the different taphonomic biases between the Holocene and Anthropocene samples. It is unclear how many of the small mammal remains in open-air archeological deposits were accumulated by people vs. other processes. Many zooarcheologists exclude mammals smaller than rabbits in their interpretations of human foraging patterns because they assume that they are not significant components of past human diets (Pastron et al., 1988; Broughton, 2002; Wake, 2012). Bocek (1987) argued that rodents were likely disproportionately added via non-human processes at the Jasper Ridge Site because only 2% of their remains were burnt vs. 24% of other identifiable bone (Bocek, 1987). If small mammals were primarily added to the Holocene record by raptors, as is true of some other open-air archeological sites (López and Chiavazza, 2019), this would make the archeological deposits quite comparable to the Anthropocene ones. However, even if small mammals mainly entered the archeological record as a result of human harvesting, they represent low biomass per sampling effort and would likely be sampled locally and opportunistically (Bocek, 1987; Simms, 1987; Smith, 2003). Therefore, we assume that small mammal archeofaunal remains largely reflect their local relative abundances at their time of deposition.
Our recent manuscript has demonstrated that small mammal remains from raptor pellets also closely reflect the local environment (Viteri et al., 2021). We therefore argue that human-deposited and raptor-deposited sites are largely comparable. However, we find some possible exceptions to this argument. The small mammal taxa which characterize the Holocene sites, N. fuscipes and leporids, could suggest an overrepresentation of larger small mammal taxa which may have been actively foraged by humans. Neotoma fuscipes and leporids represent much more substantial meals than some of the other small mammals included in this study, and there is ethnographic evidence that they were eaten by people in this region of California (Bocek, 1987). However, when leporids and N. fuscipes are removed from the ordination analysis, Holocene and Anthropocene sites are still found to be distinct (p-value = 0.001). Additionally, the trend of declining N. fuscipes and leporids across gradients of human impacts is retained in the modern landscape, with numbers decreasing for both taxa from Jasper Ridge to Stanford Campus (Table 1). We therefore believe this to be a true signal of the Anthropocene. The local leporid species, Syvilagus bachmani and Lepus californicus, are chaparral-associated animals and therefore their declining modern abundance may reflect the well-documented decline in chaparral in this region over time (Bocek, 1987; Bocek and Reese, 1992). Their Holocene abundances may also be tied to the frequency of controlled burns set by the Ohlone, as jackrabbits have been found to become ∼4.5 times more numerous in recently burned spaces (Biswell, 1967). A greater pre-European abundance of N. fuscipes, a woodland-associated species, also makes sense due to the intense logging of the 19th century in this region (Carraway and Verts, 1991; Bocek and Reese, 1992).
Small mammal remains from late-Holocene fossil deposits have been shown to generally match the composition of living communities as well as surface collections of raptor pellets, showing that there is not an inherent bias in preservation (Terry, 2010). High live-dead agreement across temporal scales has been found both theoretically and practically (Terry, 2008, 2010). However, century-scale time-averaging can enrich richness and evenness in comparison to living communities (Terry, 2008, 2010). While this is not a problem when comparing Holocene sites to each other, this could be an issue when directly comparing our Anthropocene and Holocene samples. We reduced this bias by pooling our pellet samples across years (increasing time-averaging) and dividing our Holocene samples by collection levels (reducing time-averaging). However, there is still a mismatch, with the Holocene levels spanning much more time than Anthropocene samples. If a bias exists as a result, it could suggest that the Holocene evenness and richness values are artificially elevated, making the high Anthropocene Jasper Ridge values even more remarkable.
While differences in small mammal communities between the Holocene and today are reflective of broad-scale environmental change over time, they also reflect land stewardship by indigenous communities. Native Californians have lived in this region for thousands of years and continue to cultivate their traditional practices here today (Severson et al., 2022). The ancestors of the native peoples of this region, the Muwekma Ohlone, actively managed plants and animals across all three archeological sites during the Holocene (Bocek and Reese, 1992). These traditional ecological practices, such regular controlled burning of vegetation and the cultivation of native plants for traditional uses, were disrupted by European colonization (Anderson, 2005). Therefore, the compositional differences between modern and Holocene faunal communities have been shaped by human land management practices (Ohlone practices vs. European practices) in this region over time.
An important question in urban conservation is how best to “re-green” spaces. Despite being a small preserve, Jasper Ridge harbors significant habitat heterogeneity (Holl, 2003), which may contribute to its ability to protect native small mammal species even as non-native plant and animal species have proliferated. Jasper Ridge retains almost all of the native animals present a millennium ago, minus some megacarnivores and megaherbivores (Leempoel et al., 2020; Meyer et al., 2020). Additionally, the boundary of Jasper Ridge is semi-permeable and its residential surroundings allow some wildlife movement, ultimately connecting the reserve to other protected areas in the nearly Santa Cruz Mountains. We can also learn lessons from Stanford Campus, whose many green spaces are full of non-native species and subject to frequent modification. In particular, we believe the high proportion of lawns, including golfing greens, has led to the dominance of a disturbance-tolerant grassland-specialist (Microtus californicus) and a loss of the granivorous pocket mouse. Our results show that not all “green” spaces are equal, and that places with less human disturbance as well as more native vegetation, connectivity, and habitat heterogeneity will best preserve native fauna. Importantly, small preserves in urbanized landscapes may act as important sources of biodiversity for the colonization of new protected spaces. If these preserves can harbor native species in otherwise degraded environments, they may be able to supply species to neighboring lands.
We also show that space cannot always substitute for time in ecological studies. While many studies use protected spaces as a proxy for faunal community compositions prior to human modification (Pickett, 1989), we find that today’s communities are distinct from those of the past, regardless of protection status. Our results support previous critiques of space-for-time substitutions which argue that this widely used method can vastly underestimate human impacts on today’s ecosystems (França et al., 2016; Damgaard, 2019; Attinello, 2021). Therefore, if we are to understand how modern faunal communities have changed over time, we must look to the fossil or historical record rather than existing “natural” spaces as baselines.
Conclusion
Here, we demonstrate that modern small mammal communities are fundamentally distinct from even a few centuries ago, showing the importance of utilizing fossil and subfossil baselines to understand the impacts of humans on even the most resilient members of ecosystems. More optimistically, our results demonstrate that even a relatively small, protected space can at least partially protect native faunal communities, highlighting their important role in urban conservation. While biological preserves can buffer biodiversity change across spatiotemporal gradients of human impact, they cannot completely mitigate the overwhelming signal of the Anthropocene on today’s ecosystems.
Data availability statement
The original contributions presented in the study are included in the article/supplementary material, further inquiries can be directed to the corresponding author.
Ethics statement
Ethical review and approval was not required for the animal study because no living animals were used.
Author contributions
MV and EH collaboratively developed the project and questions and collected raptor pellets. MV sorted and identified the small mammal remains from all three archeological sites and analyzed all small mammal data using RStudio. MV wrote the manuscript with edits from EH. Both authors contributed to the article and approved the submitted version.
Acknowledgments
We would like to thank many interns for their help sorting small mammal remains including: Jayela Lopez, Katherine Recinos, Carson Conley, Mahpiya Vanderbilt, and Liraz Bistriz. We would also like to thank the efforts of Jasper Ridge docent Targe Lindsay in collecting and dissecting pellets. Thanks to the staff of Jasper Ridge Biological Preserve, especially Nona Chiariello, Steve Gomez, and Trevor Hebert, and the staff of the Stanford University Archeology Collections, including Christina Hodge and Veronica Jacobs-Edmondson. Thank you to David Theobald for permission to use the human modification map which was invaluable to this project. Thank you to Laura Jones, Tony Barnosky, Rodolfo Dirzo, Avery Hill, Katie Solari, Kelly Chauvin, Diane Gifford-Gonzalez, and M. Allison Stegner for helpful feedback and guidance. Funding was provided through the National Science Foundation’s Graduate Research Fellowship Program (DGE-1656518) and the Paleontological Society’s Currano Scholarship Student Research Award. We additionally acknowledge that this work was conducted on the ancestral lands of the Muwekma Ohlone people and directly relied on the analysis of their cultural materials. We acknowledge and thank them for access to these materials.
Conflict of interest
The authors declare that the research was conducted in the absence of any commercial or financial relationships that could be construed as a potential conflict of interest.
Funding
Funding was provided through the Hadly lab, Stanford University, the National Science Foundation’s Graduate Research Fellowship Program (DGE-1656518), and the Paleontological Society’s Currano Scholarship Student Research Award.
Publisher’s note
All claims expressed in this article are solely those of the authors and do not necessarily represent those of their affiliated organizations, or those of the publisher, the editors and the reviewers. Any product that may be evaluated in this article, or claim that may be made by its manufacturer, is not guaranteed or endorsed by the publisher.
References
Alroy, J. (2010). Fair sampling of taxonomic richness and unbiased estimation of origination and extinction rates. Paleontol. Soc. Pap. 16, 55–80. doi: 10.1017/S1089332600001819
Amorosi, T., Woollett, J., Perdikaris, S., and McGovern, T. (1996). Regional zooarchaeology and global change: problems and potentials. World Archaeol. 28, 126–157.
Anderson, M. K. (2005). Tending the Wild: Native American Knowledge and the Management of California’s Natural Resources. Berkeley, CA: University of California Press.
Andrade, A., de Menezes, J. F. S., and Monjeau, A. (2016). Are owl pellets good estimators of prey abundance? J. King Saud Univ. Sci. 28, 239–244. doi: 10.1016/j.jksus.2015.10.007
Attinello, K. (2021). A Test of the Space-For-Time Substitution Hypothesis: North American Bird Responses to Forest Loss over Space do not Predict Their Responses Over Time. Ottawa: Carleton University Research.
Avenant, N. (2011). The potential utility of rodents and other small mammals as indicators of ecosystem ‘integrity’ of South African grasslands. Wildl. Res. 38, 626–639. doi: 10.1071/WR10223
Balestrieri, A., Gazzola, A., Formenton, G., and Canova, L. (2019). Long-term impact of agricultural practices on the diversity of small mammal communities: a case study based on owl pellets. Environ. Monit. Assess. 191:725. doi: 10.1007/s10661-019-7910-5
Barnosky, A. D. (2014). Palaeontological evidence for defining the Anthropocene. Geol. Soc. Lond. Spec. Publ. 395, 149–165. doi: 10.1144/SP395.6
Barnosky, A. D., Hadly, E. A., Gonzalez, P., Head, J., Polly, P. D., Lawing, A. M., et al. (2017). Merging paleobiology with conservation biology to guide the future of terrestrial ecosystems. Science 355:eaah4787. doi: 10.1126/science.aah4787
Barnosky, A. D., Matzke, N., Tomiya, S., Wogan, G. O. U., Swartz, B., Quental, T. B., et al. (2011). Has the Earth’s sixth mass extinction already arrived? Nature 471, 51–57. doi: 10.1038/nature09678
Barnosky, E. H. (1994). Ecosystem dynamics through the past 2000 years as revealed by fossil mammals from Lamar cave in Yellowstone National Park, USA. Hist. Biol. 8, 71–90. doi: 10.1080/10292389409380472
Biswell, H. H. (1967). “The use of fire in wildland management in California,” in Natural Resources: Quality and Quantity, eds S. V. Ciriacy-Wantrup and J. J. Parsons (Berkeley, CA: University of California Press), 71–87.
Blair, R. B. (1999). Birds and butterflies along an urban gradient: surrogate taxa for assessing biodiversity? Ecol. Appl. 9, 164–170.
Blois, J. L., McGuire, J. L., and Hadly, E. A. (2010). Small mammal diversity loss in response to late-Pleistocene climatic change. Nature 465, 771–774. doi: 10.1038/nature09077
Bocek, B. (1986). Rodent ecology and burrowing behavior: predicted effects on archaeological site formation. Am. Antiq. 51, 589–603. doi: 10.2307/281754
Bocek, B. (1987). Hunter-Gatherer Ecology and Settlement Mobility Along San Francisquito Creek. Doctoral dissertation. Stanford, CA: Stanford University.
Bocek, B., and Reese, E. (1992). Land use History of Jasper Ridge Biological Preserve. Woodside, CA: Jasper Ridge Biological Preserve.
Bolger, D. T., Alberts, A. C., Sauvajot, R. M., Potenza, P., McCalvin, C., Tran, D., et al. (1997). Response of rodents to habitat fragmentation in coastal Southern California. Ecol. Appl. 7, 552–563. doi: 10.2307/2269520
Brashares, J. S., Arcese, P., and Sam, M. K. (2001). Human demography and reserve size predict wildlife extinction in West Africa. Proc. R. Soc. Lond. B Biol. Sci. 268, 2473–2478. doi: 10.1098/rspb.2001.1815
Broughton, J. M. (2002). Prey spatial structure and behavior affect archaeological tests of optimal foraging models: examples from the Emeryville Shellmound vertebrate fauna. World Archaeol. 34, 60–83. doi: 10.1080/00438240220134269
Brown, A. K. (1964). Palo Alto in the Late 1840s from Later Surveys and Other Sources. Stanford, CA: Stanford University, 1853–1997.
Cardillo, M., Mace, G. M., Jones, K. E., Bielby, J., Bininda-Emonds, O. R. P., Sechrest, W., et al. (2005). Multiple causes of high extinction risk in large mammal species. Science 309, 1239–1241. doi: 10.1126/science.1116030
Carraway, L. N., and Verts, B. J. (1991). Neotoma fuscipes. Mamm. Spec. 43, 1–10. doi: 10.2307/3504130
Ceballos, G., Ehrlich, P. R., and Dirzo, R. (2017). Biological annihilation via the ongoing sixth mass extinction signaled by vertebrate population losses and declines. Proc. Nat. Acad. Sci. U.S.A. 114, E6089–E6096. doi: 10.1073/pnas.1704949114
Chaudhary, V., Tietje, W. D., Polyakov, A. Y., Rolland, V., and Oli, M. K. (2021). Factors driving California pocket mice (Chaetodipus californicus) population dynamics. J. Mammal. 102, 1353–1364.
Clark, D., and Bunck, C. (2011). Trends in North American small mammals found in common barn-owl (Tyto alba) dietary studies. Can. J. Zool. 69, 3093–3102. doi: 10.1139/z91-435
Connor, E. F., Hafernik, J., Levy, J., Lee Moore, V., and Rickman, J. K. (2002). Insect conservation in an urban biodiversity hotspot: the San Francisco bay area. J. Insect Conserv. 6, 247–259. doi: 10.1023/A:1024426727504
Cooper, W. S. (1926). Vegetational development upon alluvial fans in the vicinity of Palo Alto, California. Ecology 7, 1–30. doi: 10.2307/1929116
Cowling, R. M., and Bond, W. J. (1991). How small can reserves be? An empirical approach in Cape Fynbos, South Africa. Biol. Conserv. 58, 243–256. doi: 10.1016/0006-3207(91)90094-P
Dalquest, W. W., and Orcutt, D. R. (1942). The biology of the least shrew-mole, Neurotrichus gibbsii minor. Am. Midl. Nat. 27, 387–401. doi: 10.2307/2421007
Damgaard, C. (2019). A critique of the space-for-time substitution practice in community ecology. Trends Ecol. Evol. 34, 416–421. doi: 10.1016/j.tree.2019.01.013
Davis, E. B. (2005). Mammalian beta diversity in the Great Basin, western USA: palaeontological data suggest deep origin of modern macroecological structure. Glob. Ecol. Biogeogr. 14, 479–490. doi: 10.1111/j.1466-822x.2005.00183.x
Dietl, G. P., and Flessa, K. W. (2011). Conservation paleobiology: putting the dead to work. Trends Ecol. Evol. 26, 30–37. doi: 10.1016/j.tree.2010.09.010
Dietl, G. P., Kidwell, S. M., Brenner, M., Burney, D. A., Flessa, K. W., Jackson, S. T., et al. (2015). Conservation paleobiology: leveraging knowledge of the past to inform conservation and restoration. Annu. Rev. Earth Planet. Sci. 43, 79–103. doi: 10.1146/annurev-earth-040610-133349
Dirzo, R., Young, H. S., Galetti, M., Ceballos, G., Isaac, N. J. B., and Collen, B. (2014). Defaunation in the Anthropocene. Science 345, 401–406. doi: 10.1126/science.1251817
Draft Pathways to 30x30 (2022). Available Online at: https://www.californianature.ca.gov/pages/30x30 [accessed February 28, 2022].
Ellis, E. C., Gauthier, N., Klein Goldewijk, K., Bliege Bird, R., Boivin, N., Díaz, S., et al. (2021). People have shaped most of terrestrial nature for at least 12,000 years. Proc. Natl. Acad. Sci. U.S.A. 118:e2023483118. doi: 10.1073/pnas.2023483118
Fox, N. S. (2020). Community Ecology, Stable Isotope Ecology, and Taxonomy of Small Mammal Fossils. Los Angeles, CA: Rancho La Brea.
Fraixedas, S., Roslin, T., Antão, L. H., Pöyry, J., and Laine, A.-L. (2022). Nationally reported metrics can’t adequately guide transformative change in biodiversity policy. Proc. Natl. Acad. Sci. U.S.A. 119:e2117299119. doi: 10.1073/pnas.2117299119
França, F., Louzada, J., Korasaki, V., Griffiths, H., Silveira, J. M., and Barlow, J. (2016). Do space-for-time assessments underestimate the impacts of logging on tropical biodiversity? An Amazonian case study using dung beetles. J. Appl. Ecol. 53, 1098–1105. doi: 10.1111/1365-2664.12657
Friedlander, A. M., Brown, E. K., and Monaco, M. E. (2007). Coupling ecology and Gis to evaluate efficacy of marine protected areas in Hawaii. Ecol. Appl. 17, 715–730. doi: 10.1890/06-0536
Gaston, K., Jackson, S., Cantú-Salazar, L., and Cruz-Piñón, G. (2008). The ecological performance of protected areas. Annu. Rev. Ecol. Evol. Syst. 39, 93–113. doi: 10.1146/annurev.ecolsys.39.110707.173529
Grinnell, J. (1901). Field Notes. MVZA.MSS.0005, Museum of Vertebrate Zoology Archives. Berkeley, CA: University of California.
Gurd, D. B., Nudds, T. D., and Rivard, D. H. (2001). Conservation of mammals in eastern North American wildlife reserves: how small is too small? Conserv. Biol. 15, 1355–1363. doi: 10.1111/j.1523-1739.2001.00188.x
Hadly, E. A. (1996). Influence of late-Holocene climate on northern rocky mountain mammals. Quat. Res. 46, 298–310. doi: 10.1006/qres.1996.0068
Hadly, E. A. (1999). Fidelity of terrestrial vertebrate fossils to a modern ecosystem. Palaeogeogr. Palaeoclimatol. Palaeoecol. 149, 389–409. doi: 10.1371/journal.pone.0018057
Hadly, E. A., Spaeth, P. A., and Li, C. (2009). Niche conservatism above the species level. Proc. Natl. Acad. Sci. USA. 106, 19707–19714. doi: 10.1073/pnas.0901648106
Heske, E. J., Ostfeld, R. S., and Lidicker, W. Z. Jr. (1984). Competitive interactions between Microtus californicus and Reithrodontomys megalotis during two peaks of Microtus abundance. J. Mammal. 65, 271–280. doi: 10.2307/1381166
Heske, E. J., Rosenblatt, D. L., and Sugg, D. W. (1997). Population dynamics of small mammals in an oak woodland-grassland-chaparral habitat mosaic. Southwest. Nat. 42, 1–12.
Hindmarch, S., and Elliott, J. E. (2015). A specialist in the city: the diet of barn owls along a rural to urban gradient. Urban Ecosyst. 18, 477–488. doi: 10.1007/s11252-014-0411-y
Kelly, M., Allen-Diaz, B., and Kobzina, N. (2005). Digitization of a historic dataset: the Wieslander California vegetation type mapping project. Madroño 52, 191–201. doi: 10.3120/0024-9637
Kidwell, S. M. (2007). Discordance between living and death assemblages as evidence for anthropogenic ecological change. Proc. Natl. Acad. Sci. U.S.A. 104, 17701–17706. doi: 10.1073/pnas.0707194104
Kidwell, S. M. (2015). Biology in the Anthropocene: challenges and insights from young fossil records. Proc. Natl. Acad. Sci. U.S.A. 112, 4922–4929. doi: 10.1073/pnas.1403660112
Kirkland, G. L. (1990). Patterns of initial small mammal community change after clearcutting of temperate North American forests. Oikos 59, 313–320. doi: 10.2307/3545141
Kross, S. M., Bourbour, R. P., and Martinico, B. L. (2016). Agricultural land use, barn owl diet, and vertebrate pest control implications. Agric. Ecosyst. Environ. 223, 167–174. doi: 10.1016/j.agee.2016.03.002
Laurance, W. F., and Coan, M. E. (1987). Microhabitat and demographic correlates of tick parasitism in a northern Great Basin small mammal community. Am. Midl. Nat. 1181, 1–9.
Lawrence, G. E. (1966). Ecology of vertebrate animals in relation to chaparral fire in the Sierra Nevada foothills. Ecology 47, 278–291. doi: 10.2307/1933775
Leempoel, K., Hebert, T., and Hadly, E. A. (2020). A comparison of eDNA to camera trapping for assessment of terrestrial mammal diversity. Proc. R. Soc. B 287:20192353.
Legendre, P., and Gallagher, E. D. (2001). Ecologically meaningful transformations for ordination of species data. Oecologia 129, 271–280. doi: 10.1007/s004420100716
Lewis, S., and Maslin, M. (2015). Defining the Anthropocene. Nature 519, 171–180. doi: 10.1038/nature14258
López, J. M., and Chiavazza, H. (2019). Amidst wind, sand and raptors. Small mammal bone remains recovered in open-air archaeological sites from the Monte Desert in Central Western Argentina: taphonomic and palaeoenvironmental implications. Archaeol. Anthropol. Sci. 11, 5149–5169.
López-García, J. M., Blain, H.-A., Morales, J. I., Lorenzo, C., Bañuls-Cardona, S., and Cuenca-Bescós, G. (2013). Small-mammal diversity in Spain during the late Pleistocene to early Holocene: climate, landscape, and human impact. Geology 41, 267–270. doi: 10.1130/G33744.1
Lyman, R. L. (2012). A warrant for applied palaeozoology. Biol. Rev. 87, 513–525. doi: 10.1111/j.1469-185X.2011.00207.x
Lyons, S. K., Smith, F. A., and Brown, J. H. (2004). Of mice, mastodons and men: human-mediated extinctions on four continents. Evol. Ecol. Res. 6, 339–358.
McGill, B. J., Hadly, E. A., and Maurer, B. A. (2005). Community inertia of quaternary small mammal assemblages in North America. Proc. Natl. Acad. Sci. U.S.A. 102, 16701–16706. doi: 10.1073/pnas.0504225102
Meyer, J. M., Leempoel, K., Losapio, G., and Hadly, E. A. (2020). Molecular ecological network analyses: an effective conservation tool for the assessment of biodiversity, trophic interactions, and community structure. Front. Ecol. Evol. 8:588430. doi: 10.3389/fevo.2020.588430
Michelson, A. V., Kidwell, S. M., Boush, L. E. P., and Ash, J. L. (2018). Testing for human impacts in the mismatch of living and dead ostracode assemblages at nested spatial scales in subtropical lakes from the Bahamian archipelago. Paleobiology 44, 758–782. doi: 10.1017/pab.2018.20
Moloney, K. A., Levin, S. A., Chiariello, N. R., and Buttel, L. (1992). Pattern and scale in a serpentine grassland. Theor. Popul. Biol. 41, 257–276. doi: 10.1016/0040-5809(92)90029-S
Myers, N., Mittermeier, R. A., Mittermeier, C. G., Da Fonseca, G. A., and Kent, J. (2000). Biodiversity hotspots for conservation priorities. Nature 403, 853–858.
Naeem, S., Thompson, L. J., Lawler, S. P., Lawton, J. H., and Woodfin, R. M. (1994). Declining biodiversity can alter the performance of ecosystems. Nature 368, 734–737. doi: 10.1038/368734a0
Newmark, W. D. (1987). A land-bridge island perspective on mammalian extinctions in western North American parks. Nature 325, 430–432. doi: 10.1038/325430a0
Oksanen, J. (2019). vegan: Community Ecology Package (R package Version 2.5-5). Available Online at: https://CRAN.R-project.org/package=vegan (accessed April 1, 2022).
Pastron, A. G., Walsh, M. R., and Ericson, J. E. (1988). Archaeological Excavations at CA-SFR-113, the Market Street Shell Midden. San Francisco, CA: Coyote Press.
Pickett, S. T. A. (1989). “Space-for-time substitution as an alternative to long-term studies,” in Long-Term Studies in Ecology: Approaches and Alternatives, ed. G. E. Likens (New York, NY: Springer), 110–135. doi: 10.1007/978-1-4615-7358-6_5
Purger, J. J., and Szép, D. (2022). An attempt to determine the size of the common Barn-owl’s (Tyto alba) hunting area based on its prey composition. Avian Biol. Res. 15, 41–46. doi: 10.1177/17581559211066091
QGIS.org (2022). QGIS Geographic Information System. QGIS Association. Available Online at: http://www.qgis.org (accessed April 1, 2022).
Quinn, N., Kenmuir, S., and Krueger, L. (2018). A California without rodenticides: challenges for commensal rodent management in the future. Vertebr. Pest Conf. 28, 1–11. doi: 10.5070/V42811007
Quinn, R. D. (1990). Habitat Preferences and Distribution of Mammals in California Chaparral. Washington, DC: U.S. Department of Agriculture.
R Core Team (2019). R: A Language and Environment for Statistical Computing. Vienna: R Foundation for Statistical Computing.
Racey, G. D., and Euler, D. L. (1982). Small mammal and habitat response to shoreline cottage development in central Ontario. Can. J. Zool. 60, 865–880. doi: 10.1139/z82-119
Rick, T. C., and Lockwood, R. (2013). Integrating paleobiology, archeology, and history to inform biological conservation: paleobiology, archeology, and history. Conserv. Biol. 27, 45–54. doi: 10.1111/j.1523-1739.2012.01920.x
Rissman, A. R., and Merenlender, A. M. (2008). The conservation contributions of conservation easements: analysis of the San Francisco bay area protected lands spatial database. Ecol. Soc. 13:40.
Roberts, D. W. (2019). labdsv: Ordination and Multivariate Analysis for Ecology. R Package Version 2.0-1. Available Online at: https://CRAN.R-project.org/package=labdsv (accessed April 1, 2022).
Rowe, K. C., Rowe, K. M. C., Tingley, M. W., Koo, M. S., Patton, J. L., Conroy, C. J., et al. (2014). Spatially heterogeneous impact of climate change on small mammals of montane California. Proc. R. Soc. B Biol. Sci. 282:20141857. doi: 10.1098/rspb.2014.1857
Rowe, R. J. (2007). Legacies of land use and recent climatic change: the small mammal fauna in the mountains of Utah. Am. Nat. 170, 242–257. doi: 10.1086/518948
Rowe, R. J., and Terry, R. C. (2014). Small mammal responses to environmental change: integrating past and present dynamics. J. Mammal. 95, 1157–1174. doi: 10.1644/13-MAMM-S-079
Salafsky, N., Salzer, D., Stattersfield, A. J., Hilton-Taylor, C., Neugarten, R., Butchart, S. H. M., et al. (2008). A standard lexicon for biodiversity conservation: unified classifications of threats and actions. Conserv. Biol. 22, 897–911. doi: 10.1111/j.1523-1739.2008.00937.x
Salazar, G. (2020). EcolUtils: Utilities for Community Ecology Analysis. R Package Version 0.1. Available Online at: https://github.com/GuillemSalazar/EcolUtils (accessed April 1, 2022).
Sauvajot, R., Buechner, M., Kamradt, D., and Schonewald, C. (1998). Patterns of human disturbance and response by small mammals and birds in Chaparral near urban development. Urban Ecosyst. 2, 279–297. doi: 10.1023/A:1009588723665
Schap, J. A., Meachen, J. A., and McGuire, J. L. (2021). Microfauna relative abundance since the late pleistocene at natural trap cave, Wyoming, U.S.A. Quat. Int. (in press). doi: 10.1016/j.quaint.2021.11.018
Severson, A. L., Byrd, B. F., Mallott, E. K., Owings, A. C., DeGiorgio, M., de Flamingh, A., et al. (2022). Ancient and modern genomics of the Ohlone Indigenous population of California. Proc. Natl. Acad. Sci. U.S.A. 119:e2111533119. doi: 10.1073/pnas.2111533119
Shaffer, B. S., and Sanchez, J. L. (1994). Comparison of 1/8 “-and 1/4 “-mesh recovery of controlled samples of small-to-medium-sized mammals. Am. Antiq. 59, 525–530.
Simms, S. R. (1987). Behavioral Ecology and Hunter-Gatherer Foraging: an Example from the Great Basin. Bethany, IL: BAR.
Smith, C. S. (2003). Hunter–gatherer mobility, storage, and houses in a marginal environment: an example from the mid-Holocene of Wyoming. J. Anthropol. Archaeol. 22, 162–189.
Smith, F. A., Smith, R. E. E., Lyons, S. K., and Payne, J. L. (2018). Body size downgrading of mammals over the late Quaternary. Science 360, 310–313. doi: 10.1126/science.aao5987
Stanford University (2022). Stanford University. Available Online at: https://facts.stanford.edu/ [accessed April 7, 2022].
Stegner, M. A. (2016). Stasis and change in Holocene small mammal diversity during a period of aridification in Southeastern Utah. Holocene 26, 1005–1019. doi: 10.1177/0959683616632894
Taberlet, P. (1983). An estimation of the average foraging radius of the barn owl Tyto alba based upon rejection pellets analysis. Terre Vie 38, 171–178.
Tammone, M. N., Lacey, E. A., and Pardiñas, U. F. (2020). Dramatic recent changes in small mammal assemblages from Northern Patagonia: a caution for paleoenvironmental reconstructions. Holocene 30, 1579–1590. doi: 10.1177/0959683620941096
Terborgh, J., and Soule, M. (1999). Why we need megareserves: large-scale networks and how to design them. Wild Earth 9, 66–72.
Terry, R. C. (2008). Modeling the effects of predation, prey cycling, and time averaging on relative abundance in raptor-generated small mammal death assemblages. Palaios 23, 402–410.
Terry, R. C. (2009). The dead do not lie: using skeletal remains for rapid assessment of historical small-mammal community baselines. Proc. R. Soc. Lond. B Biol. Sci. 277, 1193–1201. doi: 10.1098/rspb.2009.1984
Terry, R. C. (2010). On raptors and rodents: testing the ecological fidelity and spatiotemporal resolution of cave death assemblages. Paleobiology 36, 137–160. doi: 10.1666/0094-8373-36.1.137
Theobald, D. M. (2013). A general model to quantify ecological integrity for landscape assessments and US application. Landsc. Ecol. 28, 1859–1874. doi: 10.1007/s10980-013-9941-6
Theobald, D. M., Kennedy, C., Chen, B., Oakleaf, J., Baruch-Mordo, S., and Kiesecker, J. (2020). Earth transformed: detailed mapping of global human modification from 1990 to 2017. Earth Syst. Sci. Data 12, 1953–1972.
Viteri, M., and Hadly, E. (2021). Bothersome burrowers: tracking gopher (Thomomys bottae) time-averaging in a late-Holocene site in California. EcoEvoRxiv [Preprint] doi: 10.32942/osf.io/trh2k
Viteri, M. C., Stegner, M. A., and Hadly, E. A. (2021). Assessing the reliability of raptor pellets in recording local small mammal diversity. Quat. Res. 106, 1–10. doi: 10.1017/qua.2021.59
Wake, T. (2012). “Interpreting new samples from famous old sites: mammal remains from the 1999 Emeryville Shellmound excavations (CA-ALA-309 and CA-ALA-310), with comments on fish and birds,” in Exploring Methods of Faunal Analysis: Insights from California Archaeology, eds M. A. Glassow and T. L. Joslin (France: CIOA Press), 4–33.
Walker, R. A. (2009). The Country in the City: The Greening of the San Francisco Bay Area. Seattle, WA: University of Washington Press.
Wolverton, S., and Lyman, R. L. (2012). Conservation Biology and Applied Zooarchaeology. Tucson, AZ: University of Arizona Press.
Yasuda, M., Ishii, N., Okuda, T., and Hussein, N. A. (2003). “Small mammal community: habitat preference and effects after selective logging,” in Pasoh: Ecology of a Lowland Rain Forest in Southeast Asia, eds T. Okuda, N. Manokaran, Y. Matsumoto, K. Niiyama, S. C. Thomas, and P. S. Ashton (Tokyo: Springer), 533–546. doi: 10.1007/978-4-431-67008-7_37
Young, H. S., Dirzo, R., Helgen, K. M., McCauley, D. J., Billeter, S. A., Kosoy, M. Y., et al. (2014). Declines in large wildlife increase landscape-level prevalence of rodent-borne disease in Africa. Proc. Natl. Acad. Sci. U.S.A. 111, 7036–7041. doi: 10.1073/pnas.1404958111
Keywords: small mammal, community ecology, protected areas, raptor pellets, biodiversity, urbanization, archeological record, land modification
Citation: Viteri MC and Hadly EA (2022) Spatiotemporal impacts of the Anthropocene on small mammal communities, and the role of small biological preserves in maintaining biodiversity. Front. Ecol. Evol. 10:916239. doi: 10.3389/fevo.2022.916239
Received: 08 April 2022; Accepted: 14 July 2022;
Published: 15 August 2022.
Edited by:
Gregory Dietl, Cornell University, United StatesReviewed by:
Emily Lindsey, La Brea Tar Pits, United StatesNathaniel Fox, South Dakota School of Mines and Technology Rapid City, United States
Copyright © 2022 Viteri and Hadly. This is an open-access article distributed under the terms of the Creative Commons Attribution License (CC BY). The use, distribution or reproduction in other forums is permitted, provided the original author(s) and the copyright owner(s) are credited and that the original publication in this journal is cited, in accordance with accepted academic practice. No use, distribution or reproduction is permitted which does not comply with these terms.
*Correspondence: Maria C. Viteri, bXZpdGVyaUBzdGFuZm9yZC5lZHU=