- 1Marine Institute, Newport, Ireland
- 2Inland Fisheries Ireland, Dublin, Ireland
- 3School of Biological, Earth & Environmental Sciences, University College Cork, Cork, Ireland
Migration is an important ecological trait that allows animals to exploit resources in different habitats, obtaining extra energy for growth and reproduction. The phenology (or timing) of migration is a highly heritable trait, but is also controlled by environmental factors. Numerous studies have reported the advancement of species life-events with climate change, but the rate and significance of such advancement is likely to be species specific, spatially variable and dependent on interactions with population and ecosystem changes. This is particularly true for diadromous fishes which are sentinels of change in both freshwater and marine domains, and are subject to considerable multiple stressors including overfishing and habitat degradation. Here, we describe trends in the migration phenology of three native Irish migratory fishes over half a century, Atlantic salmon (Salmo salar), brown trout (Salmo trutta) and European eel (Anguilla anguilla). The trends were derived from daily counts of 745,263 fish moving upstream and downstream through the fish traps of the Burrishoole catchment, an internationally important monitoring infrastructure allowing a full census of migrating fish. We found that the start of the seaward migration of eel has advanced by one month since 1970. The commencement of the salmon smolt migration has advanced by one week, although the rest of the migration, and the entirety of the trout smolt run has remained stable. The beginning of the upstream migration of trout to freshwater has advanced by 20 days, while the end of the run is more than one month later than in the 1970’s. The greatest phenological shift has been in the upstream migration of adult salmon, with at least half of migrating fish returning between one and two months earlier from the marine environment compared to the 1970’s. The earlier return of these salmon is coincident with reduced marine survival and decreasing body size, indicating considerable oceanic challenges for this species. Our results demonstrate that the impacts of climate change on the phenology of diadromous fish are context-dependent and may interact with other factors. The mobilization of long-term datasets are crucial to parse the ecological impacts of climate change from other anthropogenic stresses.
Introduction
Animal migrations play an important role in the functioning of ecosystems around the world (Bauer and Hoye, 2014), and are vulnerable to changes in climate (Shaw, 2016) as well as habitat alteration, loss of connectivity and changes in resource availability (Moore, 2011). The timing of these recurrent events, which allow individuals and populations to enhance their reproductive success by utilizing seasonally available resources, is largely an evolutionary adaptation, but is controlled by predictable environmental cues, including temperature. A recent review indicates that phenological shifts in migration can have population-level consequences when they affect survival in longer-lived organisms and reproduction in shorter-lived organisms (Iler et al., 2021). Diadromous fish move between freshwater and marine habitats at key life stages, either spawning in freshwater (anadromous fish) or in the ocean (catadromous fish). These species are, therefore, sentinels of change over two domains, and their migrations constitute time periods of significant predation, including that wrought by humans. In many cases of diadromous fish stock collapses, overfishing specifically during migration runs has been identified as being the primary cause (ICES, 2020, 2021; Lennox et al., 2021). Fish migrations are of intrinsic social, cultural and economically value to many societies (Gende et al., 2002; Oke et al., 2020), and changes in these events, either in terms of timing or biomass, can have significant consequences for dependent human communities. These migrations are also a crucial dynamic in the receiving ecosystems, as successful migrants contribute significant amounts of energy to receiving habitats once they die, bringing rich marine resources into the trophic web of freshwater ecosystems in the case of diadromous species (e.g., Reimchen, 2000).
Along the Atlantic coasts of Europe, diadromous fish include several anadromous salmonid species, such as Atlantic salmon (Salmo salar), brown trout (Salmo trutta), and the catadromous European eel (Anguilla anguilla). Catchments may have several native co-existing diadromous species, with migrations to and from the ocean happening at distinct times of the year. A combination of regulating and controlling factors determine the timing of migrations of diadromous fish either to or from the sea (Jonsson and Jonsson, 2009a). Regulating factors affect the physiological processes that are required for successful migration while controlling factors affect the physical process of migration. For example, in salmonids, smoltification occurs prior to the downstream migration of fish to sea, whereby growth hormones, cortisol, and thyroid hormones interact to increase salinity tolerance, bring about behavioral change and transform the fish into streamlined, silver colored individuals, ready for the marine environment (Björnsson, 1997; McCormick et al., 2002). The primary regulating factor controlling this transformation is photoperiod (Hoar, 1988; Byrne et al., 2004), although cumulative water temperature also plays a role by affecting the rate of development and interacting with photoperiod (McCormick et al., 2002; Zydlewski et al., 2005). Controlling factors operate within the smolt run, affecting the physical process of migration. The most obvious of these is water discharge, which allows fish to physically move from one habitat to another in times of flood (Byrne et al., 2003; Carlsen et al., 2004; Harvey et al., 2020), whilst potentially delaying or curtailing migrations in times of drought (Raymond, 1979; Hembrel et al., 2001; Winter et al., 2016). Water temperature in the time period immediately preceding migration also plays a role (Jonsson and Jonsson, 2009b). The regulatory and controlling factors determining the upstream migration of salmonids to freshwater spawning habitats are perhaps more complex, and operate over a wider spatial and temporal scale than smoltification. For Atlantic salmon, and sea trout that spend a winter at sea, the return to freshwater is intrinsically linked to spawning and hence sexual maturation, regulating factors are those that control and maximize reproductive potential for particular populations, including photoperiod, temperature and food availability in the marine environment (Adams and Thorpe, 1989). A combination of these factors, along with genetic factors, will determine the growth of individuals and the available energy for maturation (Thorpe, 2007). Once maturation has been achieved, the main controlling factor enabling upstream migration is water discharge (Jonsson and Jonsson, 2009a), although thresholds are likely to be extremely population specific and linked to catchment level hydrological controls.
Similar to salmonids, the metamorphosis and maturation from yellow to silver eels before the marine migration to the spawning area in the Sargasso Sea includes morphological, anatomical, as well as physiological changes and occurs during the summer immediately before the autumn/winter migrations (Durif et al., 2005; Balm et al., 2007). The regulatory factors controlling this metamorphosis are likely to be similar to those of salmonids, albeit over different time periods (e.g., summer water temperatures vs. winter water temperatures). However, determination of the exact triggers of metamorphosis in European eel is complicated by the unpredictability of the phenomenon, which up until fairly recently was poorly described in a physiological sense (Durif et al., 2005). In contrast, the controlling factors determining migration have been well described for several populations, and include water discharge, moon phase, water temperature and chemical cues such as conductivity (Vøllestad et al., 1986; Sandlund et al., 2017; Monteiro et al., 2020; Arevalo et al., 2021a).
Numerous studies have documented a change in the phenology of migration of diadromous fish in response to climate change (e.g., Kennedy and Crozier, 2010; Kovach et al., 2013; Fealy et al., 2014; Otero et al., 2014; Sandlund et al., 2017; Legrand et al., 2021). Increasing water temperature has been identified as being a principal driver of the phenomenon (Kovach et al., 2013; Arevalo et al., 2020; Teichert et al., 2020) and modeling experiments using future climate projections indicate that earlier runs of fish are increasingly likely (Hedger et al., 2013; Teichert et al., 2020). While it is tempting to attribute any significant changes in migration phenology to the direct effects of climate warming, these species are impacted by interacting multiple stressors, including fishing, land use change, introgression with captive-bred individuals, food web disruption, the presence of dams and the incidence of parasites (Palstra et al., 2007; Belletti et al., 2020; Bolstad et al., 2021; Sobocinski et al., 2021; Tillotson et al., 2021). Shifts in phenology are therefore likely to be context-dependent, but worth exploring as we strive to understand, mitigate and adapt to the interconnected impacts of climate change and biodiversity loss on ecosystems.
Long term ecological datasets allow us to quantify changes in the context of historical interannual variability, attribute changes to drivers and develop management actions in response (Webb et al., 2012). However, datasets of this nature are rare (Woods et al., 2022) and often predate the digital age. A first step in this process, therefore, is collating long term longitudinal data, quantifying any trends that are apparent, and contextualizing them. In this paper, we describe and explore trends in key metrics pertaining to diadromous fish populations on the western edge of the European continent. We describe the decadal trends in migration phenology for Atlantic salmon, brown trout and European eel in the Burrishoole catchment, Co. Mayo, and test for significance over 50 years. Reported trends are then discussed in relation to sentinel climate and hydrological data for the region in question, and documented population changes that have occurred.
Materials and Methods
Study Region and System
Burrishoole is a small (100 km2) upland catchment (53° 56′ N, 9° 35′ W) draining into the North-east Atlantic through Clew Bay (Figure 1). Climatically influenced by the Atlantic Ocean (Jennings et al., 2000; Blenckner et al., 2007), the catchment experiences a temperate, oceanic climate with mild winters and relatively cool summers. Maximum summer air temperatures rarely exceed 20°C, while minimum winter air temperatures are usually between 2 and 4°C. The base geology of the catchment is primarily quartzite and schist, interspersed with veins of volcanic rock, dolomite and wacke in some subcatchments. Soils in the catchment comprise poorly drained gleys, peaty podsols and blanket peats. The combination of the geology and the soils mean that the rivers and lakes are relatively oligotrophic, colored (c. 80 mgl–1 PtCo) due to high levels of dissolved organic carbon (DOC) and have low alkalinity (< 20 mgl–1 CaCO3). The main lakes are slightly acidic (pH = c. 6.7), and the rivers draining the hillsides can be very acidic during rainfall events (pH < 5.0). A diadromous fish research station has been in operation in the Burrishoole catchment in since 1955. The station is located between the brackish coastal lagoon Lough Furnace and the upstream, freshwater Lough Feeagh, which are linked by two channels, the Mill Race River and the Salmon Leap River (Figure 1). Staff of the research station operate four permanent fish traps on these two channels (an upstream and downstream trap on each channel), allowing for a complete census of migratory fish moving between their freshwater and marine habitats. The three migratory species in the catchment are Atlantic salmon (Salmo salar), brown trout (Salmo trutta) and European eel (Anguilla anguilla).
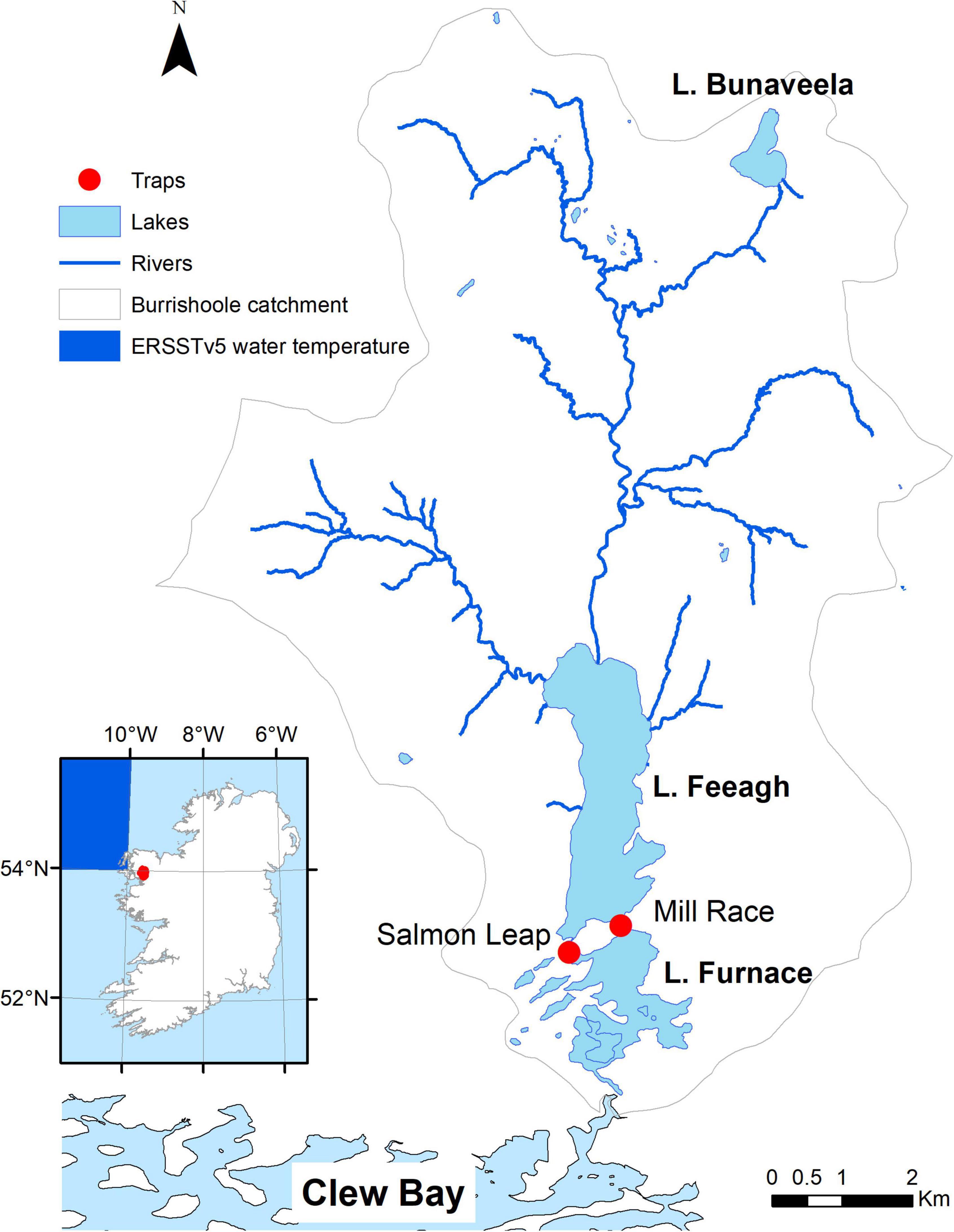
Figure 1. Location of the Burrishoole catchment on the west coast of Ireland. Everything upstream of the red dots (representing the fish traps) is freshwater, while downstream (including lough Furnace) are coastal waters. The Met Éireann weather station are at the same location as the Mill Race trap (red dot). The blue square in the inset map indicates the area for which we extracted the oceanic water temperature.
Atlantic salmon typically migrate out of the freshwater part of the Burrishoole catchment as two-year-old smolts in late spring, numbering from over 16,000 in 1976 to 5,500 in the late 1980’s to the mid-1990’s. Current smolt production is on average 5,789 smolts per annum (3 year average 2017–2019, Marine Institute, 2020), with greater than 90% migrating as 2 + fish (Piggins and Mills, 1985). Most will return to Burrishoole the following summer after spending one winter at sea (1SW). Such fish are termed “grilse”. In contrast, some fish will return as “spring” salmon after spending 2 or 3 winters at sea (2SW or 3SW), returning to freshwater in the earlier part of the year. The spring component of the stock is currently less than 5% of the total run (Marine Institute, 2020) although it comprised a larger component historically (Reed et al., 2016). Reasons for this decline are most likely the result of overfishing, either on the high sea fishery in west Greenland (ICES, 2021), or in Lough Furnace. A commercial draft net fishery in Furnace ceased in 1965, but at the height of its operations in the 1950’s, it caught on average 120 spring fish in a season, along with about 500 grilse (Nixon, 1999). The spring component of the Burrishoole run was also badly affected by an outbreak of Ulcerative Dermal Necrosis in the system from 1967 to the mid-1970s. The drift net fishery for salmon off the Irish coast was a major determinant of the number of salmon returning to freshwater to spawn, as it caught between 60 and 80% of the stock between the 1980’s and the early 2000’s (Ó Maoiléidigh et al., 2004). While the cessation of this fishery in 2007 has reduced one source of mortality, marine survival of salmon continues to decline, and has averaged 7% between 2017 and 2019 in Burrishoole. Returns of grilse to the catchment have ranged from almost 1,800 in 1973 to lows of 252 in 1990 and 279 in 2014. The current return averages 429 fish (3 year average 2017–2019, Marine Institute, 2020).
Sea trout is the name commonly given to the adult anadromous (sea going migratory) form of the European brown trout Salmo trutta. Trout in the Burrishoole system occur as freshwater residents, partially anadromous unsilvered “slob” trout and fully anadromous fish. Two and three year old sea trout smolts run to sea in late spring. Downstream migrations of 0+ and 1+ unsilvered juvenile trout also occur in autumn and early winter. True sea trout (silver color) return to freshwater in the same summer as their first migration to sea (0 + SW, known as finnock) or spend a full winter at sea before returning to freshwater (1 + SW). In Burrishoole, many sea trout return as finnock, with the proportion ranging from 31.6 to 63.5% (Poole et al., 2006). In the 1970s, the average run of sea trout smolts out of Burrishoole was 4506 fish and the number of returning sea trout averaged 2503 (Poole et al., 2006). The population suffered a catastrophic collapse in the late 1980’s, which affected both finnock and older adults, leaving a spawning stock of sea trout of only 155 fish in 1990. The population has failed to recover and the return of adults currently averages 16 fish (3 year average 2017–2019, Marine Institute, 2020). Higher marine mortality of predominantly smolts and 1 + sea age fish from 1990 to 1992 was paralleled by poor growth of the surviving fish (Poole et al., 1996). Sea lice, Lepeophtheirus salmonis (Kroyer), emanating from sea farms, have been implicated as the cause of this mortality, not just in Burrishoole but in many west of Ireland, Scottish and Norwegian systems in close proximity to salmon farms (Gargan et al., 2006). After the stock collapse of 1989–90, and the lack of sea trout returning to freshwater to spawn, the smolt run started to fall and it currently numbers 298 fish (3 year average 2017–2019, Marine Institute, 2020).
Finally, the life cycle of the European eel is still somewhat of a mystery, but current evidence supports the view that eel returning to European continental waters as juveniles originate from a single spawning stock in the Atlantic Ocean, presumably in the Sargasso Sea area where the smallest larvae (Leptocephali) have been found (Palm et al., 2009; Als et al., 2011; Miller et al., 2019). The laterally flattened Leptocephalus transforms into a rounded glass eel off the Irish coast in autumn and enter Irish estuaries from November to February. As temperatures rise, the glass eel become progressively more pigmented and when temperatures pass 9-11°C, many commence active migration into freshwaters as elvers. Once in freshwater, elvers grow into yellow eel which is the life stage in which they spend most of their life [7–58 years in the west of Ireland (Poole et al., 2018; Vaughan et al., 2021)]. Eels mature only once when they undergo a process known as silvering before and during their seaward migration. The numbers of glass eel migrating into European estuaries dropped dramatically in the mid-1980’s and has not recovered since. It is estimated that current glass eel stocks are < 10% of the historical average (ICES, 2020). Silver eel migrations in Burrishoole take place typically from August to November, with 50% of the numbers counted in October (Marine Institute, 2020). Day-to-day variation in the number of eels migrating is associated with conditions that minimize predation risk, such as new moons, floods and the presence of other eel stimulate migration (Sandlund et al., 2017). Numbers of emigrating silver eels fell significantly after 1982 in Burrishoole, from more than 4,000 eels per annum to approximately 2,000 eels per annum (Poole et al., 2018). Along with the decrease in the numbers of migrating silver eels, the proportion of small male eels dropped and the average size of female eels has increased until the 2000’s and has since been decreasing, indicating a change in the stock structure of yellow eel in the catchment (Poole et al., 2018), potentially resulting from decreased eel density (De Leo and Gatto, 1996; Bark et al., 2007; Andersson et al., 2012).
Historical Climate Data
In order to contextualize the fish phenology data presented here, we collated long term climate records of air and water temperature and rainfall for the Burrishoole catchment and the marine environment experienced by fish migrating in and out of Burrishoole. Air temperature and rainfall are recorded every morning at the Newport (Furnace) manual weather station (jointly run by Met Éireann and the Marine Institute, station 833) (Located at the Mill Race, Figure 1). Maximum and minimum temperatures are recorded using mercury thermometers, and average daily temperature is taken as the average of these two readings. Daily rainfall is measured using a five inch (12.7 cm) diameter standard copper rain gauge. Data were downloaded from www.met.ie, licensed under a Creative Commons Attribution 4.0 International (CC BY 4.0) License.
Water temperature is recorded by the Marine Institute at the Mill Race (Dillane et al., 2018) (Figure 1), at a site 60 m along the outflow, at a water depth of <1 m. Recordings are continuous, via in situ paper or sensor based recorders, and compiled daily. A Negretti paper chart recorder, calibrated against a mercury thermometer, was used from 1960 to 2004. From 2004 to 2009, a StowAway TidbiT temperature data logger from Onset (TB132-05 + 37) was used. From 2009 to the present day, data were collected using a temperature sensor on an Orpheus Mini Water Level Recorder from OTT Hydrometry1 and checked for drift against a regularly calibrated Seabird SBE39plus.2 For all data sources, the midnight temperatures were extracted for each day. When sensors were changed in 2004 and 2009, overlapping data were used to confirm that there were no significant jumps in the data that would introduce bias to the long-term trend. We sourced oceanic water temperature data from ERSSTv5 (Huang et al., 2017b) for the grid sea in the North Atlantic closest to the migration route of fish moving in and out of Burrishoole (54°N and 10° W) (Figure 1). This dataset comprises monthly reconstructed sea surface temperatures for each 2°× 2° grid square around the globe (Huang et al., 2017a).
Fish Census Data
All migrating fish are counted individually, by hand, in the Salmon Leap and Mill Race traps and then immediately released either upstream or downstream, depending on their route. Counts are recorded manually on trap record sheets, and subsequently digitized. Annual collation of data occurs each year, and census details are described in the annual reports of the research station (e.g., Marine Institute, 2020). In 2015, a significant effort was made to digitize and quality check any paper records back to 1970 that remained undigitized, and store all records in a standardized format. Five datasets were included in this analysis: downstream movement of trout smolts; downstream movement of salmon smolts; downstream movement of silver eel; upstream movement of wild salmon grilse and upstream movement of adult sea trout. For the purposes of this paper, data were collated describing the number of fish (by species) migrating through the upstream and downstream traps every day between the 1st January 1970 and the 31st December 2020 (see data availability for open access details). There are some additional data describing fish movements through the Mill Race trap between 1959 and 1970, but this was only a partial count, and are not included in this analysis.
There are no long term data describing the upstream migration of juvenile eel to freshwater in Burrishoole, as this life stage is particularly hard to trap (Harrison et al., 2014). A monitoring program has commenced in Burrishoole, which captures a small proportion of the glass eel run each year, but these data are not included here. We chose not to include MSW (multi sea winter salmon) in this data collation, as the numbers of such fish are very small.
During the 1970s, there were some inconsistencies in the recording of salmon and trout upstream movements on the paper records. Throughout the history of the research station, a variable number of hatchery bred salmon are released into Lough Furnace as smolts, to return to the upstream traps as adults. On return to the upstream traps between 1970 and 1979, these salmon were not differentiated into wild or hatchery reared fish, but were instead recorded simply as “grilse.” A retrospective analysis of the annual reports with the original trap and hatchery records indicates that the proportion of hatchery fish was likely to be ∼10%. We therefore chose to include all grilse in the analysis, being cognizant that some of them may be hatchery stock. From 1980, fish were differentiated in the trap records into wild and hatchery stock (based on the presence or absence of an adipose fin, respectively), and hatchery fish were not included in this dataset from 1980 on. For trout, the categorization of fish into “finnock,” “brown trout” and “sea trout” only commenced after 1976, and prior to 1976, upstream trout were simply counted as “trout”. For consistency, and because the proportion of brown trout is small (< 100 fish in any year), we included all upstream migrating trout in this analysis, which therefore includes all finnock, older mature sea trout and brown trout.
The Mill Race channel underwent some structural changes in 2019 and 2020, following flood damage. In these two years, wild salmon were observed holding out in a pool immediately downstream of the trap for several weeks along with large numbers of hatchery fish, rather than moving upstream into Lough Feeagh. These “holding” fish in 2019 and 2020 were particularly noticeable in the last part of the salmon run, with some fish moving upstream during the last week of December (but only in the Mill Race trap). Generally, there is a strong coherence between the run timings in the Salmon Leap and the Mill Race, but the discrepancy in 2019 and 2020 was very obvious (Supplementary Figure 1). We therefore decided to only use the run timing data from the Salmon Leap for characterizing the last part of the salmon run (95% quantile), rather than leaving out 2019 and 2020 altogether. Between 1970 and 2020, the number of salmon moving upstream through the Mill Race dropped from ∼50 to ∼10-20% in recent years, with 11% of the run using the Mill Race in 2019 and 18% in 2020. Additional fish data (biological metrics, stock characteristics) were taken from the annual reports of the Marine Institute or from unpublished Marine Institute data. In particular, we compiled data on adult salmon size from the trap records, extending the time series used in de Eyto et al. (2016) and O’Sullivan et al. (2021) to estimate fecundity. Briefly, wild adult salmon are not all routinely measured (length or weight) as they move upstream through the Burrishoole traps, owing to concerns about stress. However, the lengths of all kelts (salmon returning to sea after spawning) are measured as they migrate downstream, and there is a considerable dataset of female kelt lengths available for the same time period as this phenology dataset. As we do not expect female fish to change in length between upstream and downstream migration, we assume that kelt size is a good proxy for the size of adults returning from the sea. All length measurements of female migrating kelts were extracted from the trap records and compiled here to complement the phenology data.
Statistical Analysis
All data exploration and analyses were undertaken using R v3.6.1 (R Core Team, 2019). All datasets were standardized to the time period 1970–2020. Outliers were removed based on visual inspection and knowledge of expected ranges. Trends were determined using the non-parametric Theil-Sen estimate of trends (Sen’s slope) in the R package openair v2.7.0 (Carslaw and Ropkins, 2012). The advantage of the using the Theil-Sen estimator is that it tends to yield accurate confidence intervals even with non-normal data and heteroscedasticity (non-constant error variance), and is also resistant to outliers (Theil, 1950; Sen, 1968; Ohlson and Kim, 2015). Autocorrelation was checked using the acf function in the R v3.6.1 (R Core Team, 2019). For hydroclimate data (air temperature, water temperature and rainfall), trends were estimated on annual means (avg.time = “year”) and split into seasons where appropriate (type = “season”). We estimated the trends in the number of dry and wet days per year, by assigning days where daily cumulative rainfall was 0 mm day–1 as “dry” days, and where daily cumulative rainfall was greater than the 95% quantile (16.7 mm day–1) as “wet” days. For fish phenology, we calculated the calendar day of the year (DOY) by which quantiles of the number of fish (5%, 33%, 50%, 66%, 95%) had passed through the traps. For example, the 33% quantile is the day of the year by which 33% of annual cohort of a particular species life stage (e.g., salmon smolts) had moved through the relevant trap. By using 5% and 95% as the start and end of the runs, we down weighted the importance of stragglers.
Results
Hydroclimate
In general, the climate of Burrishoole is now warmer and wetter than in previous decades. There was a significant increasing trend in all of the air and water temperatures indicators recorded, apart from the summer water temperature in the Mill Race which has been stable over five decades. The temperature observed in spring has increased faster than other seasons for both air temperature and freshwater (Figure 2). Annual mean air temperature warmed faster (0.25°C decade–1) than water temperatures in the Mill Race (0.18°C decade–1) and in the Atlantic west of Achill island (0.17°C decade–1) (Supplementary Figure 2 and Supplementary Table 1). Annual rainfall has increased significantly over the Burrishoole catchment since 1970, by 47 mm decade–1 (Supplementary Table 1). The trend is significant in summer (+ 17 mm decade–1) and winter (+ 27 mm decade–1), but not in spring and autumn. The increased rainfall translates into a significant trend in the number of wet days recorded in the catchment, which has increased by one day decade–1 (Supplementary Table 1). A wet day in this context is when more than 16.7mm of rain is recorded in 24 h, and so by the end of the time series (2020), there are five more such days per year than at the start of the time period (1970). There was no significant change in the amount of dry days, indicating that increasing drought conditions were not observed in the Burrishoole over this study period.
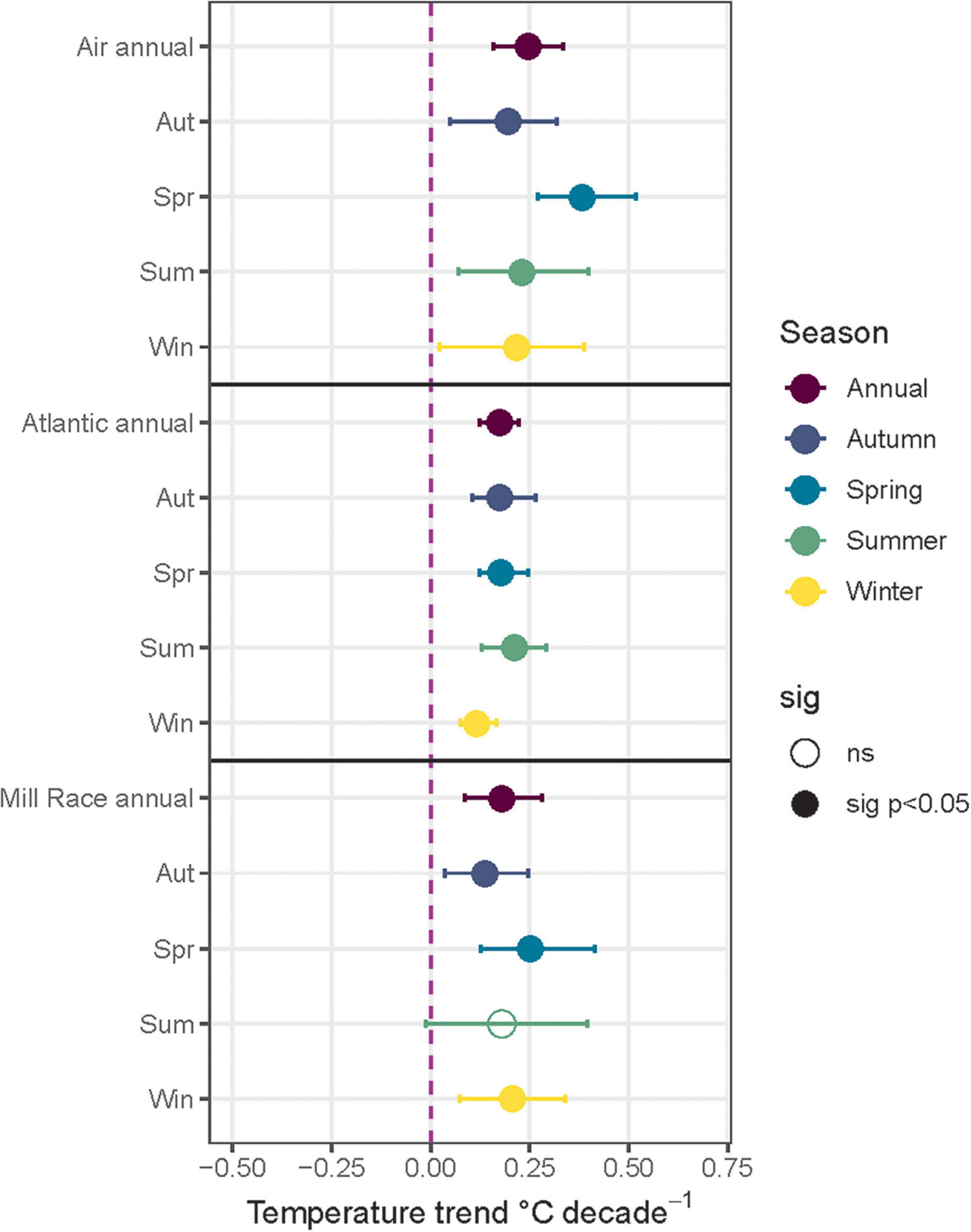
Figure 2. Decadal trends in mean temperature (°C decade–1) measured between 1970 and 2020 at Furnace Meteorological station (air), the Mill race channel (water) and the Atlantic Ocean (water) west of Achill Island (54°N and 10° W). Dots indicate the Theil-Sens estimate of the trend (slope) for each variable, and error bars denote the 95% confidence intervals (c.i.) of the estimate. The dashed purple line indicates a slope of 0, i.e., no trend. Filled circles indicate a significant trend at p < 0.05. Open circles indicate a non-significant trend.
Fish Migration
The dataset used in this analysis includes records of 745,263 fish migrating into and out of the Burrishoole catchment between 1970 and 2020. The dataset comprised 406,171 counts of salmon smolts and 111,456 counts of trout smolts migrating to sea. 155,290 silver eels were counted on their migration out of the Burrishoole catchment toward the Atlantic Ocean. 40,806 and 31,540 adult trout and salmon, respectively, were counted returning to freshwater to spawn. The start of the silver eel downstream migration, from freshwater to the ocean, has advanced over the 5 decades considered here, with the trend in both the 5% and 33% quantile being significant (Figure 3). The day of the year (DOY) by which the 5% of silver eel have migrated has shifted by −7.2 days decade–1 (Table 1), with the start of the run in the 1970s occurring around day 258 (14th Sep), but that of the 2010s occurring more than one month earlier, around day 221 (8th Aug). The 33% quantile also shifted forward by −3.4 days decade–1 (Table 1), from a median of day 276 (2nd Oct) in the 1970s, to 263 (19th Sep) in the 2010s. There were no significant trends in the rest of the eel migration (Supplementary Figure 3).
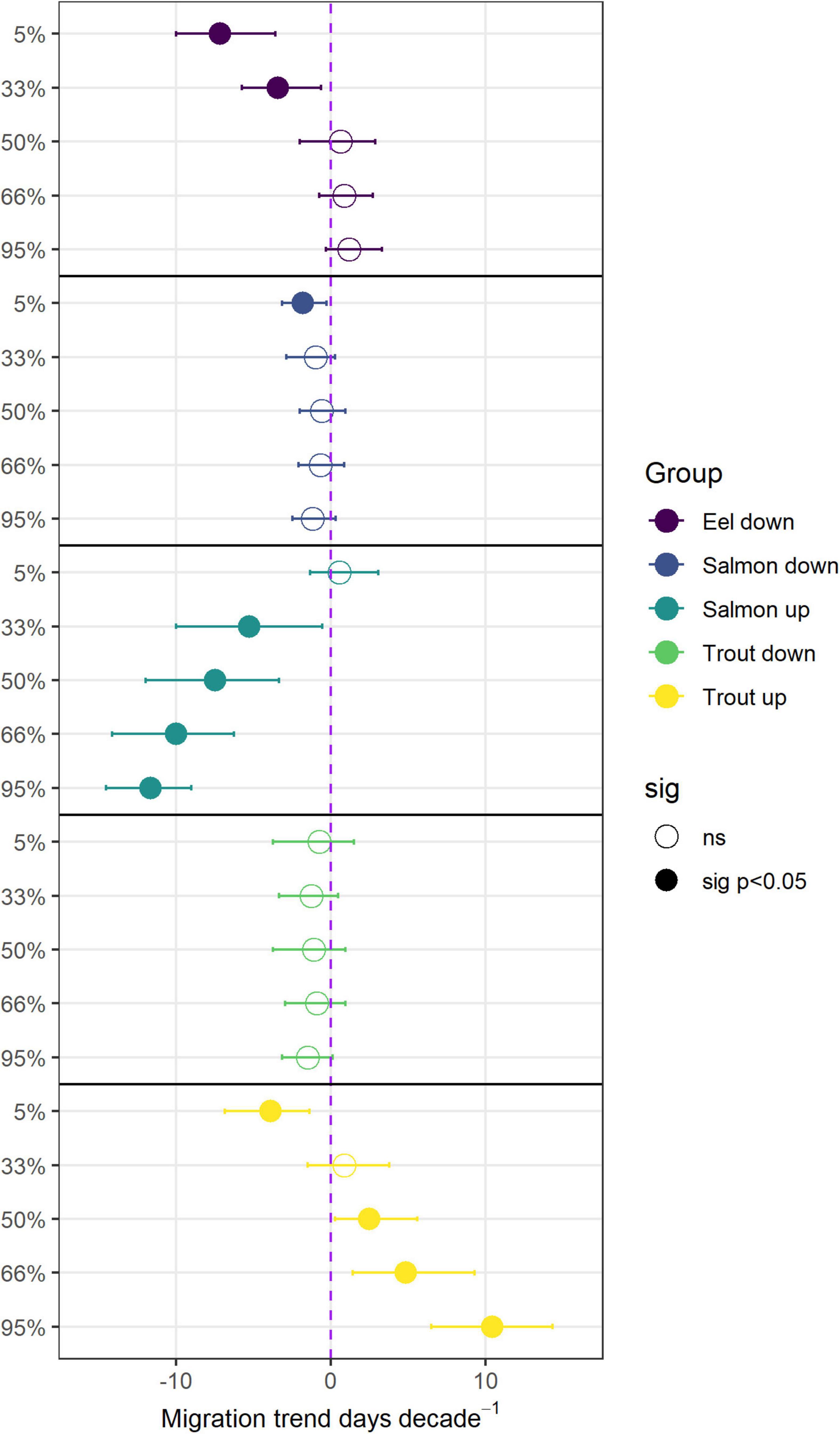
Figure 3. Decadal trends in the migration of diadromous fish through the Burrishoole upstream and downstream traps. Dots are the Theil-Sens estimate of the trend (slope) for each variable, and error bars denote the 95% confidence intervals (c.i.) of the estimate. The dashed purple line indicates a slope of 0, i.e., no trend. Filled circles display a significant trend at p < 0.05 while open circles display a non-significant trend.
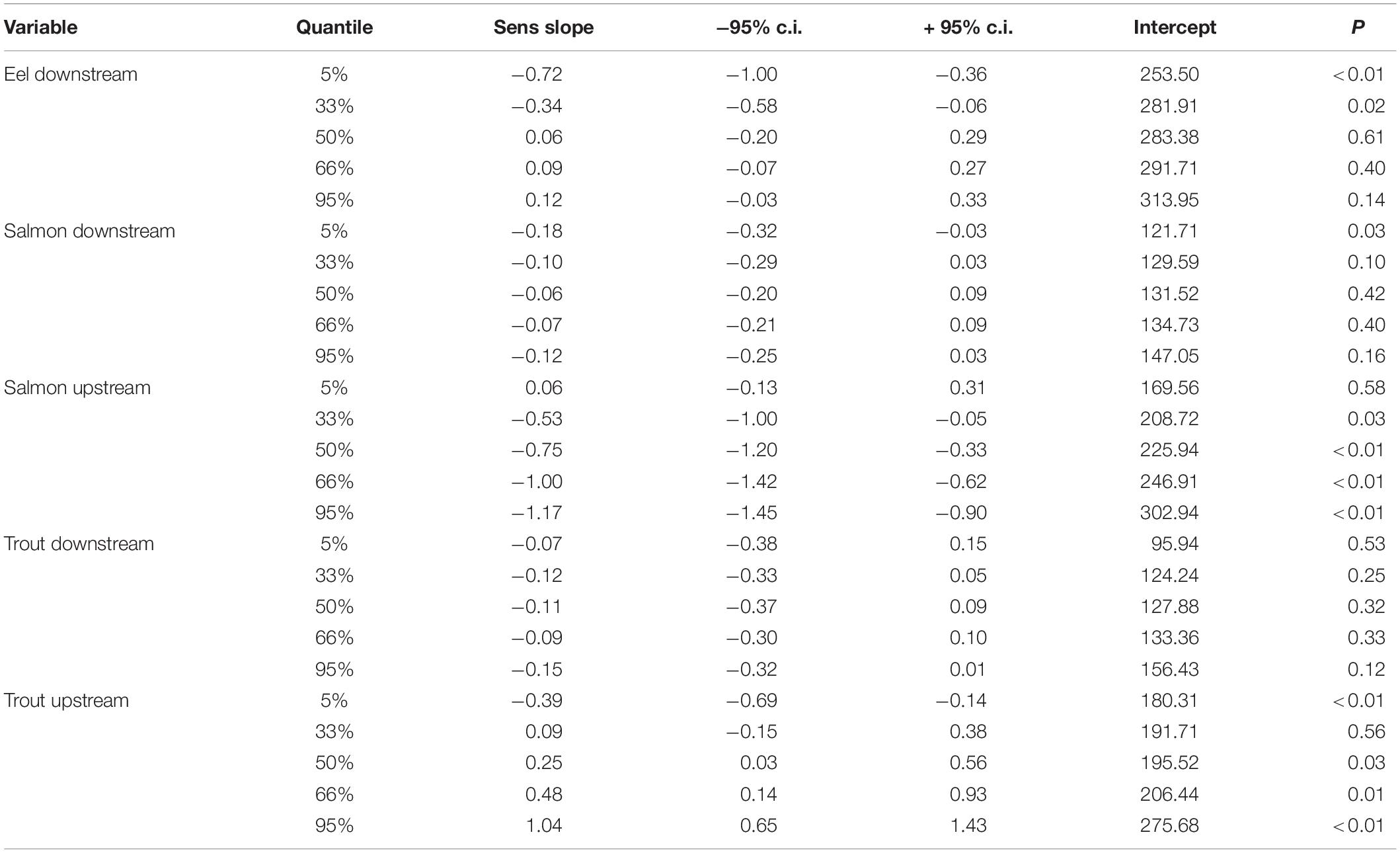
Table 1. Trends (Theil sens estimate of slope) in migration phenology (days year–1) of diadromous fish migrating through the Burrishoole traps 1970–2020, based on the day of the year by which quantiles of the annual run had passed through the relevant (upstream or downstream) traps.
The very start of the salmon downstream migration was the only part of the smolt run where a significant trend was apparent (Figure 3), with fish moving earlier by −1.8 days decade–1 (Table 1). This equates to a shift of about one week over the time series, from a median day of 120 (29th Apr) in the 1970s, to 112 (21st Apr) in the 2010s. All other metrics of the migration phenology of salmon smolts were stable between 1970 and 2020 (Supplementary Figure 4). In contrast, the timing of the upstream spawning migration of salmon moving from the Atlantic to freshwater has changed considerably over these five decades, with all quantiles, apart from 5%, returning earlier every decade (Figure 3). In the 1970s, the median DOY of the end of the run (95%) was around the 19th Oct (day 293), but by the 2010s, the vast majority of fish had migrated through the upstream traps by the 31st Aug (day 244). The trend in the 33%, 50%, 66%, and 95% quantiles was −5.3, −7.5, −10, and −11.7 days decade–1, respectively (Table 1). The start of the upstream migration (5%) has remained fairly constant around day 170 (18th Jun), but the run has constricted with every passing year, such that in the 1970s, the run extended over almost 4 months (117 days), but by the 2010’s, it was spread over only 2 months (73 days) (Figure 4). The size (length) of Burrishoole fish has decreased over time (Figure 5), with the average length of adult female kelts being 62.3 cm in the 1970’s, but only 57.3 by the 2010’s. This trend was significant (-0.11 cm year–1, p < 0.001). When compared to the average size of returning salmon, it is clear that earlier migration back to freshwater from the sea is associated with increasingly smaller fish (Figure 6). Whilst the decrease may seem to be relatively small, when converted to ova number (see Supplementary Figure 5 for details), this decrease in size is linked with a decrease in modeled ova number per fish from >2800 to <2400 (Supplementary Figure 6).
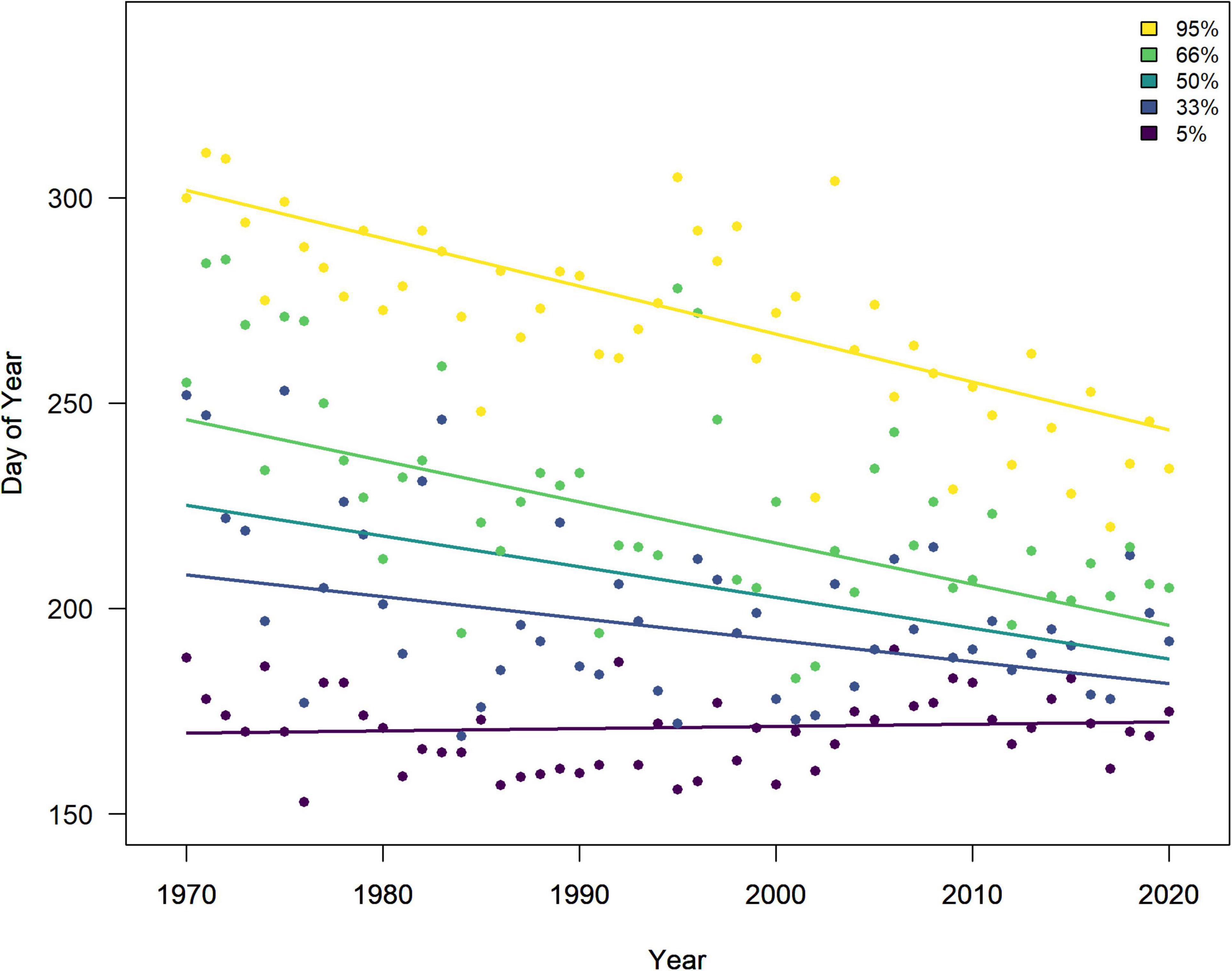
Figure 4. Day of the year by which quantiles of the annual adult salmon run (1970–2020) had moved through the upstream traps (marine − > freshwater) in the Burrishoole catchment. Lines indicate the Theil-Sens estimate of the trend.
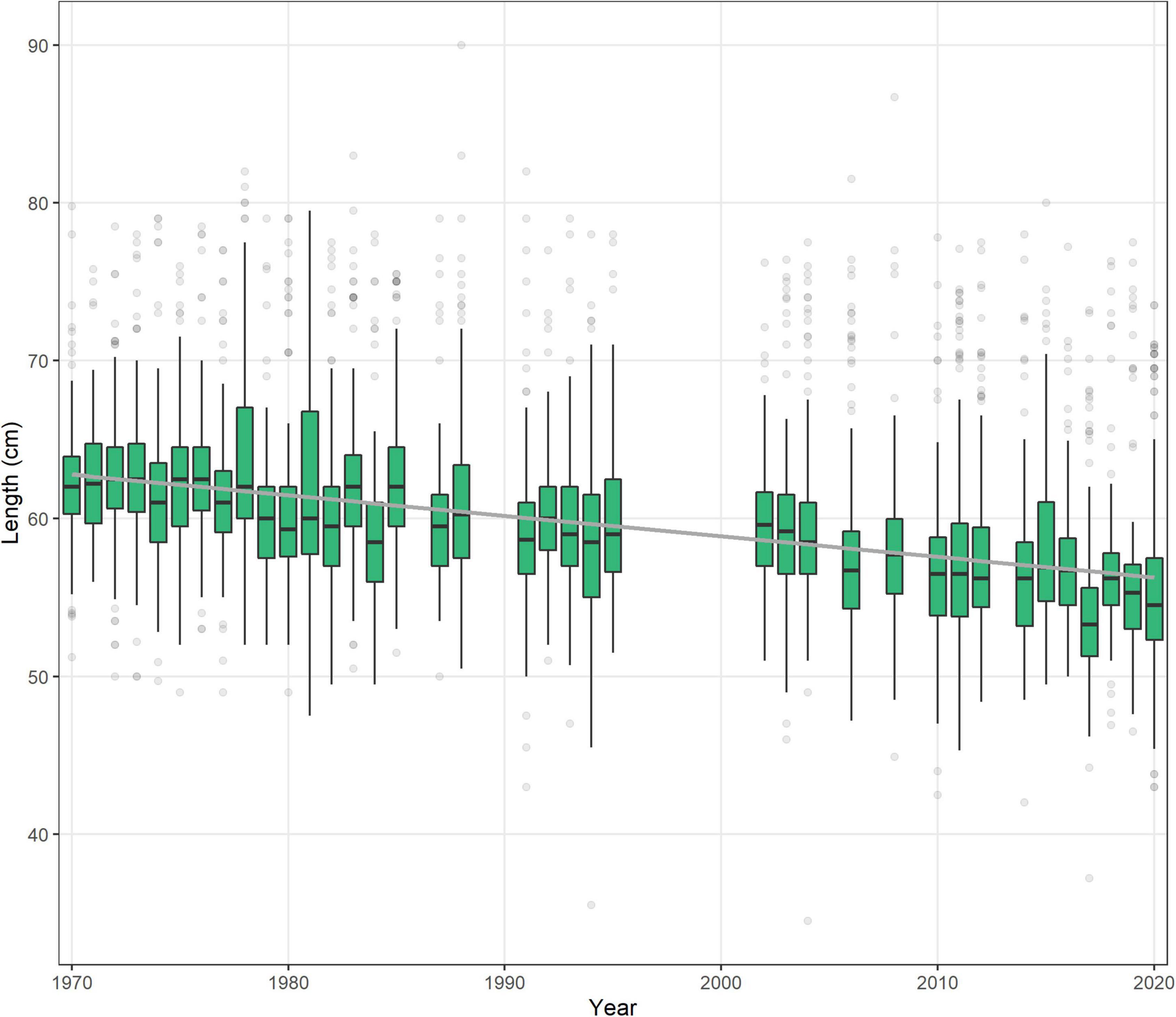
Figure 5. Length of wild female kelts per year measured in the Burrishoole traps between 1970 and 2020. Gray line indicates the linear relationship between year and length of fish.
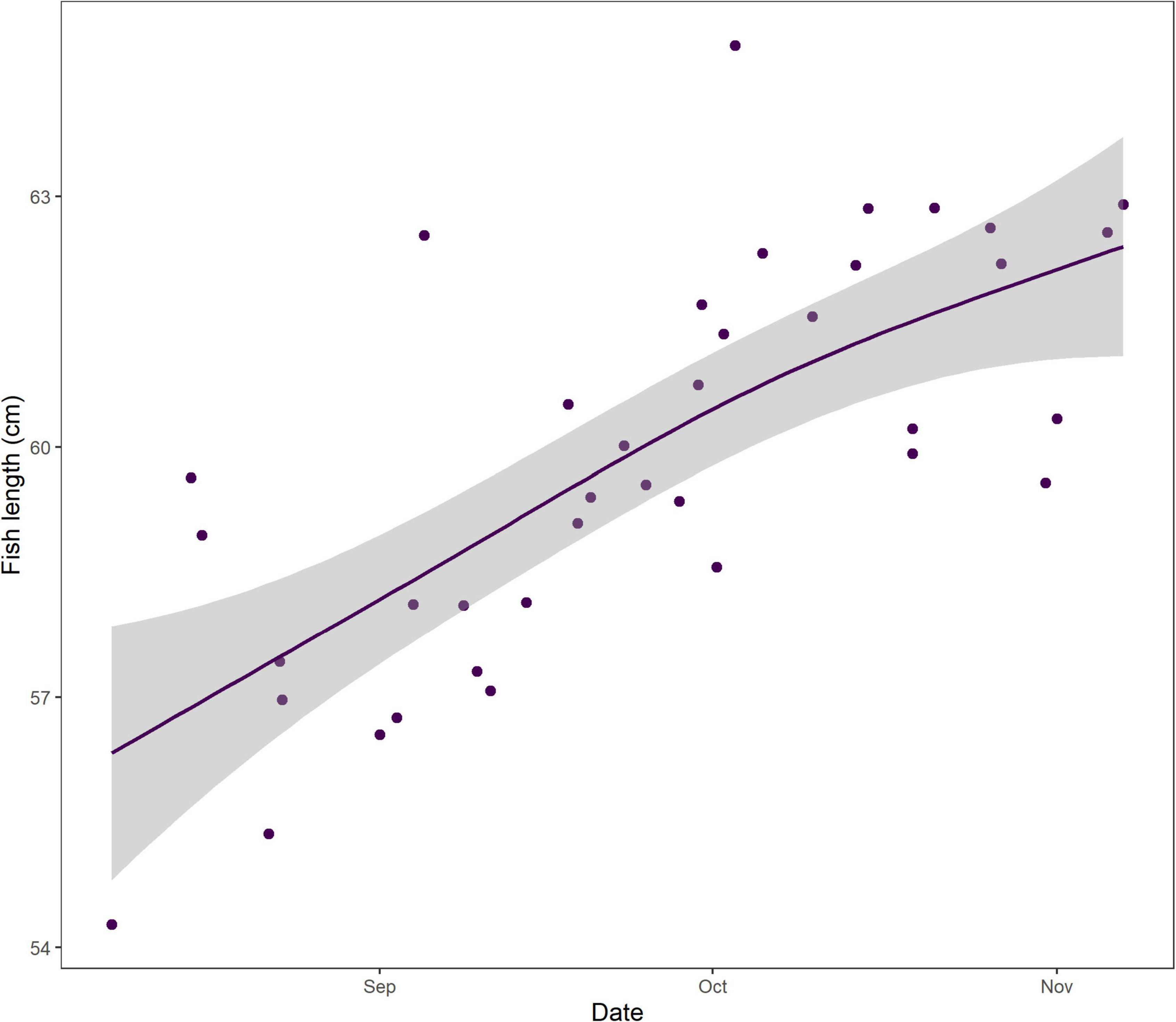
Figure 6. Relationship between the date of the end of the annual salmon run (95% quantile) through the upstream Burrishoole traps, and the average length of wild female grilse kelts of that cohort. The line indicates a GAM of the relationship and the gray areas indicate the 95% confidence interval of the predications.
As with salmon smolts, the phenology of the downstream migration of trout smolts has been stable over the time period 1970–2020 (Supplementary Figure 7), with no significant trends in any of the quantiles (Figure 5 and Table 1). The upstream migration has, however, changed significantly, with 5% of the run returning to freshwater earlier with each passing decade (−3.9 days decade–1), and the latter parts of the run returning later (Figure 3). The trend in the 50%, 66%, and 95% quantiles was 2.5, 4.8, and 10.4 days decade–1, respectively (Table 1), leading to the observation that the end of the upstream trout run in the last decade (2010s) was almost one month later than in the 1970s. (19th Nov vs. 9th Oct) (Supplementary Figure 8). The combination of the earlier start of the run, and the later end of the run means that trout are returning to the Burrishoole traps for a much longer period than they used to, with the run now extending over 158 days in comparison to 107 days at the start of the study period.
In summary, Figure 7 captures the notable trends in migration timing of Burrishoole diadromous fish. The characteristic length of the upstream migrations of salmon and trout have reversed, with a shorter trout migration and longer salmon migration in the 1970’s becoming a drawn out trout migration and a relatively short sharp salmon migration in the 2010’s. The advancement of the earlier portions of the migrations of silver eel and salmon smolts are also apparent. While the median DOY for most cohorts is roughly similar between the start and end of the time series, that of the adult salmon run is markedly earlier, having shifted from the start of September to early July. This shift in the median and second half of the salmon run has progressed in a consistent fashion, with each decade being markedly earlier than the previous (Figure 8).
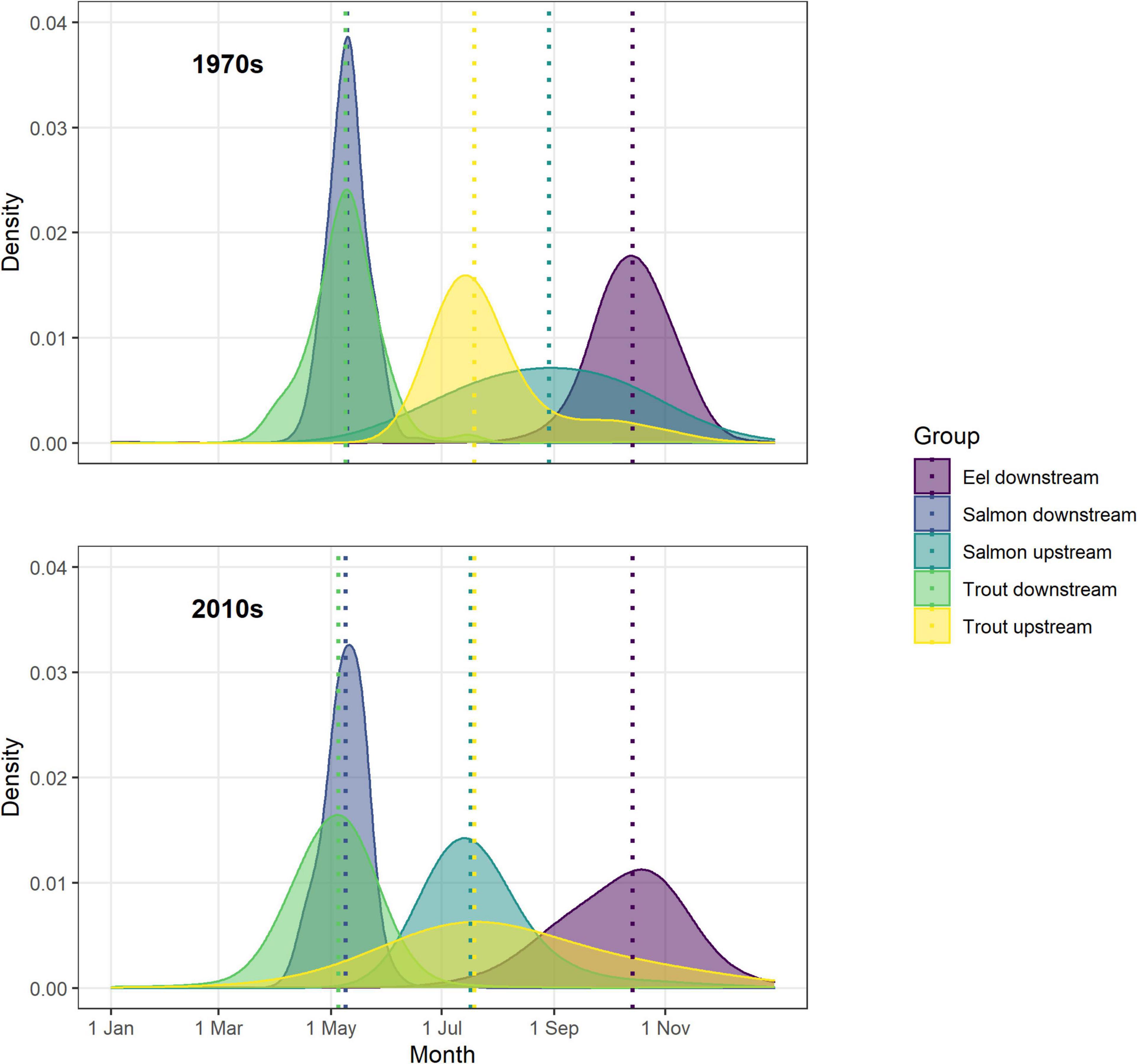
Figure 7. Density plots of counts of fish per day over 10 years for the 1970s (1970–1979) and the 2010s (2010–2019). Densities are expressed per group. Vertical dotted lines indicate the median run time of each group of fish.
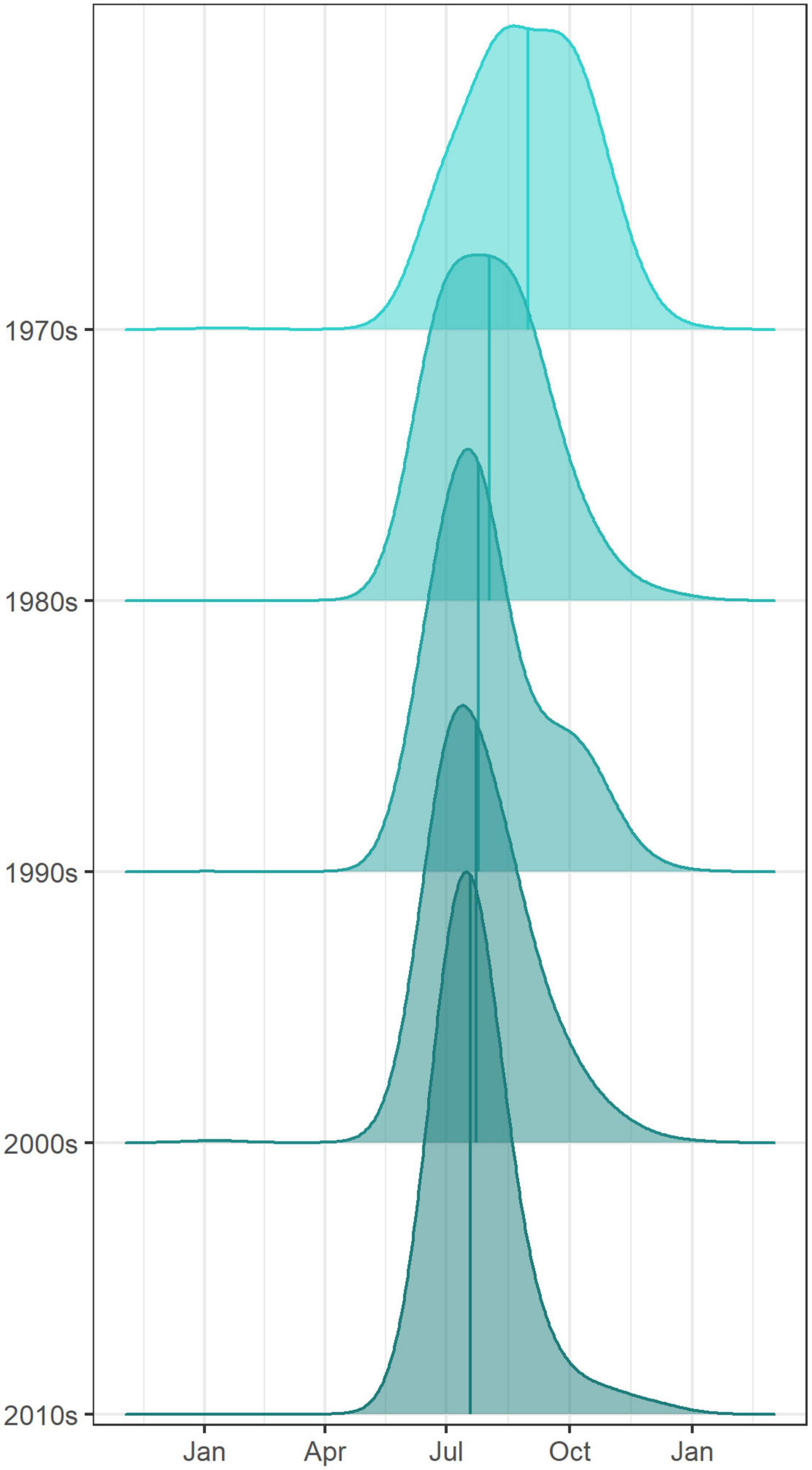
Figure 8. Density plots of adult salmon moving upstream to spawn in the Burrishoole catchment. Densities are calculated from counts of fish per day, accumulated by decade between the 1970s and 2010s. Vertical lines indicate the median run time of each group of fish.
Discussion
Although it is widely accepted that the phenology of animal migration is being impacted by anthropogenic climate change (Cohen et al., 2018), variation in the response of locally adapted populations to regional climates means that it is difficult to generalize, even within species (Sparks et al., 2019). This is particularly relevant to species with broad geographic ranges such as Atlantic salmon and European Eel, where phenological adaptations to climate will depend on life history, migration routes, and hydrological characteristics of their freshwater habitats. The fish populations of Burrishoole are adapted to the “moist and equable” climate of Ireland (Sweeney, 2014), where the moderating influence of the Gulf stream and the North Atlantic current is well documented (McCarthy et al., 2015). Extreme air temperatures, such as those recorded on mainland Europe, are not yet a feature of our climate, and this pattern is carried through to aquatic habitats, where the water temperature of the coast, lakes and rivers is also moderated. Our results show that increasing trends in air temperatures recorded at the meteorological station in the catchment align with those recorded across Ireland (Cámaro García and Dwyer, 2021). In Burrishoole, spring (Mar, Apr, May) is the season with the fastest rate of air temperature warming (0.38°C decade–1). This is biologically important as it is the season where juvenile fish come out of winter torpor and start actively feeding (Jonsson and Jonsson, 2009a). The increasing trend in rainfall recorded in Burrishoole across all seasons is also a feature of Ireland in general (Murphy et al., 2018; Cámaro García and Dwyer, 2021), although we note that the annual trend in Burrishoole is largely driven by the relatively dry period of the early 1970s. Whilst increasing drought is a cause for concern generally for fish populations across the world (Lennox et al., 2019), it is not yet an apparent feature of the climate of the Burrishoole catchment for the time period described here. While the trends in water warming are significant, comparisons of Burrishoole water temperature with those across Europe and the world indicate that the warming is occurring at a lower rate than in comparable ecosystems (O’Reilly et al., 2015; Woolway et al., 2019; Dokulil et al., 2021). This suggests that the position of the catchment at the edge of Europe, by the Atlantic Ocean, has provided some buffering against the thermal extremes associated with global warming recorded in other parts of the world. Nevertheless, the trends in the hydroclimate data described here for the Burrishoole catchment indicate that fish are now living in warmer conditions than in the latter half of the 20th century, and we would perhaps expect changes in their migration phenology as a result, given the overwhelming evidence that water temperature is the one of the main regulatory factors. Our results, however, established varying life stage and species specific phenological changes, highlighting the need for full life cycle mechanistic models to fully understand the role that multiple stressors play in the survival of these species (Woodward et al., 2021).
The advancement of the start of the silver eel from Burrishoole has been previously reported (Sandlund et al., 2017), albeit for a shortened time period (1970-2015). Our results confirm their findings that it is only the start of the run that is advancing significantly, while the timing of second half of the run has remained fairly stable over the years. When considering changing migration phenology of eel, there is likely to be some overlap between factors impacting physiological changes associated with the silvering process, and those impacting growth rate across the whole life span of European eel. There is no doubt that growth rates of European Eel are intrinsically linked with water temperature (Daverat et al., 2012; Vaughan et al., 2021), and that as temperatures warm, eels can grow faster and reach maturity at earlier ages. However, this in itself may not impact the timing of the migration, which is primarily determined by the availability of silver eel ready to migrate. This readiness is controlled by the process of silvering, which is regulated by environmental factors, including temperature, occurring in the months preceding migration (Bruijs and Durif, 2009). The increasing trend in water temperature in the months prior to the silver eel run (winter and spring) hints at the mechanism controlling the observed earlier migration, as the silvering process is thought to start in spring of each year (Durif et al., 2005) and is enhanced by favorable growth conditions, including warmer temperatures. Our results support this theory, with up to 33% of eel being ready to migrate 2-4 weeks earlier than in previous decades.
It is perhaps surprising that it is only the timing of the start of the eel run (5% and 33%) which has advanced, while the timing of the rest of the migration has stayed constant. There are several possibilities here. The first is that the impacts of warming temperatures are not yet great enough to induce a full shift of a migration run which is also constrained by photoperiod (Durif et al., 2005) and moon phase (Sandlund et al., 2017). Secondly, where species are not able to track changes in environmental conditions fast enough to allow timely adaptation (Reed et al., 2011), changes in phenology may lag behind the rates observed in climate variables, resulting in a mismatch that can be ecologically detrimental (Bertrand et al., 2011; Strangeways, 2018; Arevalo et al., 2021a; Song et al., 2021). This is especially true for long-lived species with consequential slow generational turnover, such as European eel. The ecological consequences of such a lag, if found, are still open questions deserving of attention in the future (Kuczynski et al., 2017). The third possibility is that regardless of water temperature, Burrishoole eel are adapted to migrate specifically in these last months of each year, in order to reach the Sargasso Sea at the same time as all other eel migrating from Europe. Durif et al. (2005) hypothesized that it is photoperiod which acts as the main determinant of migration timing, as it would ensure that the genetically panmictic eel migrating from diverse geographical locations should converge at the same time for spawning. In fact, latitudinal observations on eel migration contradict the hypothesis that warmer water temperatures could induce earlier migration, as eels from northern latitudes currently migrate much earlier than their conspecifics at southern latitudes (Davidsen et al., 2011). By leaving earlier, these eels, which have more distance to cover, will likely reach the spawning grounds at the same time as eels from more southern rivers (Bruijs and Durif, 2009). Therefore, early migration in this species might be seen as disadvantageous unless all populations undergo a similar shift. A meta-analysis of all potential migrating times of silver European eel across its range would be informative, in determining whether there is now significant mismatch between various components of the stock that could potentially disperse spawning aggregations. Finally, it has been observed in some catchments that male eel tend to migrate earlier in the year than females (Haraldstad et al., 1985), and therefore it is possible that the observed change in sex ratio (Poole et al., 2018) may have contributed to the advancement in the start of the migration period. However, earlier runs of male eel have not been observed in Burrishoole historically, when high proportions of males were common (Poole et al., 1990). Data from the 2000’s and 2010’s indicate that males and females migrate at the same time.
Like eel migrations, photoperiod is thought to be the dominant regulator factor controlling the timing of smolt migrations in salmonids (Thorpe, 1988), with local temperatures playing a subordinate role (McCormick et al., 2002). Salmon smolts in the north of Europe migrate later than those emanating from rivers further south, and when this spatial trend is corrected for latitude (and hence photoperiod), water temperature plays only a minor controlling role (Otero et al., 2014). It is probable that regional adaptations to a physiological switch based on photoperiod restricts migration timing to a relatively tight window. This “optimum smolt window” described in Russell et al. (2012) enables locally adapted fish to enter marine waters at the perfect time to take advantage of optimal environmental conditions including plentiful food resources. However, the onset and median timing of smolt migrations of salmon across its Atlantic range are generally advancing at a rate of circa three days decade–1 (Russell et al., 2012; Otero et al., 2014), and the authors hypothesized that this was linked to warming trends in air, river and ocean temperatures. Kennedy and Crozier (2010) also reported significantly earlier migrations (across the whole run) for smolts migrating from the River Bush in Northern Ireland, in the order of 3.6 − 4.8 days decade–1 and linked this earlier migration with reduced marine survival. That Burrishoole salmon smolt migration (apart from the first 5% of fish) has not advanced is therefore somewhat surprising. However, Otero et al. (2014) reported significant variation across the 70 sites included in their analysis, and it seems that Burrishoole salmon are one of the exceptions to the general rule. It is also worth noting that at five decades, the Burrishoole time series is one of the longest on record for Atlantic salmon populations.
Although the salmon smolt migration timing has been fairly stable over these five decades, there is nevertheless, a possible survival implication in migrating on approximately the same date. The marine environment is warming steadily, with consequential phenological advancement of food availability and optimum growing and swimming conditions. This mismatch theory has been put forward as one of the possible reasons why marine survival of salmon continues to decline (Friedland, 1998; Hvidsten et al., 2009; Kennedy and Crozier, 2010). Some studies have evaluated this mismatch in terms of the difference in temperature between freshwater and marine habitats (e.g., Kennedy and Crozier, 2010; Arevalo et al., 2021b), but this is not likely to be a cause for concern for Burrishoole fish, as the trend in warming during spring in the Atlantic is quite similar to that of their freshwater habitat (0.18 vs. 0.25°C decade–1). Atlantic water temperatures just before the peak of the smolt run (10 day average leading up to the 9th May) are generally 2°C warmer than those measured in the Mill Race (Supplementary Figure 9), which is far less than the value of 4−6°C put forward by Staurnes et al. (2001) as being problematic. This difference has not increased over the time period of this study, although there is considerable interannual variation. While our results are consistent with the conclusion that the climate change is not yet impacting the average phenology of downstream migration of salmon smolts from Burrishoole, the interannual variation warrants further exploration in a full life cycle evaluation, and may explain why some annual cohorts have much higher survival than others.
The migration timing of trout smolt from Burrishoole are stable over the 50 years considered here. While some of the discussion related to salmon downstream migration is likely relevant, (e.g., the overriding influence of photoperiod), the collapse of the sea trout population must also be considered here. Byrne et al. (2004) documented some changes in the trout downstream migration timing when data from 1971 to 2000 were examined. In their analysis, they found an extended run timing and a shift from discrete defined periods in the 1970’s to more temporally extended runs in the 1990’s. With the benefit of an additional 2 decades of data, we can say that this trend has not continued, and there has been no directional shift in the timing of the smolt run. Since 2000 [the end of the dataset used in Byrne et al. (2004)], the number of sea trout smolts has collapsed even further, with numbers falling by more than 90%, from several thousand in the 1970’s to a couple of hundred in the 2010’s. The almost complete loss of the anadromous portion of the Burrishoole stock means that we are not comparing like with like across the five decades of data described here. The same conclusions must also be applied to the upstream migration phenology of trout, with counts of upstream migrating trout in the 2010’s (mean of 134 year–1) being less than 6% of counts in the 1970’s (mean of 2389 year–1). One of the key recommendations of a recent methodological review of phenology studies by Brown et al. (2016) was that “reanalyses of existing time series (must) state how the existing data sets may limit the inferences about possible climate responses”. In the case of the Burrishoole trout population, it is perhaps necessary to parse the phenology time series into “before” and “after” the collapse in 1989/1990. When we do this, we were left with datasets of 19 (1970-1988) and 30 (1991-2020) years, respectively, and apart from the 95% quantile of trout moving upstream pre-collapse, no significant trends in migration phenology were apparent (Supplementary Table 2).
The trend toward an earlier return time for most of the adult salmon to Burrishoole is the most biologically significant change that we found in our analysis of the data, and is previously unreported for this wild population. Earlier return to freshwater has been reported for Atlantic salmon populations in France (Legrand et al., 2021) and North America (Juanes et al., 2004; Dempson et al., 2017). Earlier spawning migrations of pacific salmon have also been reported (Crozier et al., 2008; Kovach et al., 2013; Oke et al., 2020), and in all the aforementioned studies, changing oceanic conditions were implicated in the shift. The advancement of the median run timing of Burrishoole salmon (0.75 days year–1) was faster than that reported for North American (0.5 days year–1) and French populations (0.29 days year–1). Earlier run timing is not a feature of every Atlantic salmon population, with some studies reporting a later run of fish in some rivers (Valiente et al., 2011; Todd et al., 2012; Bal et al., 2017). Variation in trends in phenology migration within species is to be expected. The complexity of the system (e.g., catchment physiography such as the presence of extended estuaries, coastal lagoons, numerous river branches) into which the returning salmon are migrating has large implications for the run timing into freshwater for spawning. This is further complicated by populations that constitute a large proportion of mixed age adults (1 or multi sea winter individuals). In complex systems, there may be weeks or months between the return to freshwater of differing age classes [1SW vs. MSW (Reed et al., 2016)] or those homing to different parts of a very large catchment such as the River Teno (Vähä et al., 2011). In addition, the method and extent of data collection can cause problems in the calculation of phenological metrics, for example, where data are restricted to fishing within closed seasons (e.g., Todd et al., 2012). Cohen et al. (2018) found that some examples of delays in phenology were associated with short annual records that are prone to sampling error. Generalizing within a species across broad geographical ranges is therefore fraught with difficulties, but this should not dissuade us from the effort, as our results indicate that changes in return timing is biologically important.
Theoretically, salmon should stay in their marine environment for as long as possible, to maximize feeding opportunities, size at maturity and hence reproductive success (Quinn et al., 2016), given that egg number and egg size (females) and competitive ability (males) scale positively with size (Fleming and Reynolds, 2004; de Eyto et al., 2015). The results presented here show that Burrishoole salmon are spending considerably less time at sea than in previous generations, potentially forfeiting valuable feeding opportunities in rich marine waters. As adult salmon do not feed once they return to freshwater, it also means that returning fish will have less energy reserves than those that returned in the 1970’s and 1980’s, with resulting implications for energy reserves (Martin et al., 2015) and hence reproductive potential. Collation of fish size from the records available for this study confirm that the earlier migration to freshwater has a biological consequence, with smaller fish (and hence less ova) in the spawning cohort every decade. Mounting evidence from multiple taxa indicates that a decrease in body size is a universal response of ectotherms to warming temperatures (Daufresne et al., 2009; Horne et al., 2015) in accordance with the ecological rules dealing with temperature–size relationships (Atkinson, 1994). The phenomenon is reported for multiple marine fish species in temperate environments (Brunel and Dickey-Collas, 2010; van Walraven et al., 2010; Ikpewe et al., 2021), including Atlantic salmon in their marine phase (Todd et al., 2012; Bal et al., 2017). Our data provide strong circumstantial evidence that this is related to anthropogenic climate change, being a consistent year on year decline coincident with increasing seawater temperatures.
Modeling work on Pacific salmon has indicated that long term declines in body size has the potential to substantially erode the resilience of affected populations (Rand et al., 2006). Cooke et al. (2004) outline the many competing hypotheses (e.g., energetics, osmoregulatory dysfunction, oceanic conditions, trophic ecology, parasites, disease) that may account for early migration of Pacific salmon back to freshwater, and these are all equally applicable to Atlantic salmon (Olmos et al., 2020; Thorstad et al., 2021). Several large scale projects such as the Missing Salmon Alliance3 (Crozier et al., 2018), Salsea4 and SeaSalar5 are in the process of trying to quantify these causal links. Up until recently, this work has been hampered by a lack of certainty about where the various populations of Atlantic salmon reside during their marine migration. Thanks to technological advances and collaborative efforts, some progress has been made on this very recently (Almodóvar et al., 2020; Gilbey et al., 2021; Rikardsen et al., 2021), allowing targeted hypotheses to now be generated on salmon migration and distribution patterns in the sea. Data such as those described in this paper are crucial in providing definitive answers as to why marine survival of Atlantic salmon continues to decline. The earlier return migration, coupled with smaller body size, point to several fruitful lines of investigation including: physiological challenges associated with growth rates (Tréhin et al., 2021; Vollset et al., 2022), changing ocean currents (Caesar et al., 2021), decreased feeding opportunities (Peyronnet et al., 2008; Utne et al., 2021) and trophic mismatches (e.g., Burthe et al., 2012). All of these potential causative agents are consistent with the impacts of anthropogenic climate change on the ocean, and are likely to interact in synergistic and antagonistic ways with Atlantic salmon stocks. Integration of long time series of observational data into full life cycle models (e.g., Olmos et al., 2019) may allow a more complete mechanistic understanding of the Atlantic salmon decline (Woodward et al., 2021), and therefore allow conservation actions to take a more directed focus.
This analysis was facilitated by a comprehensive review and collation of paper records, which are a feature of many long term records that predate the digital era. The value of such data collection and mobilization cannot be overstated as we enter a period of unprecedented ecological turmoil. Every effort should be made to locate, digitize and share such records according to the FAIR principles (Wilkinson et al., 2016). Our results demonstrate that phenological changes in locally adapted diadromous fish populations are highly context-dependent (varying with species and life stage) and may be driven by multiple interacting stressors. Nevertheless, collating such datasets offer the possibility of meta-analyses which can move our scientific field beyond locally valuable case studies, toward greater understanding of the complex interactions between ecological impacts of climate change and those of other human-caused stresses. For diadromous fish, subject to a multitude of pressures and impacts across habitats, this understanding is essential if we are to successfully prevent the extinction of these iconic species.
Data Availability Statement
The fish dataset generated for this study can be downloaded here: http://data.marine.ie/geonetwork/srv/eng/catalog.search#/metadata/ie.marine.data:dataset.4343. An accompanying readme file and R script for exploration of the data can be found at: https://github.com/IrishMarineInstitute/BurishooleLTER-Public/tree/master/daily_fish_migration_burrishoole_1970_2020.
Ethics Statement
Ethical review and approval was not required for the animal study because the data used in this article were collected by the Irish Marine Institute for stock assessment purposes and therefore does not fall under the EU and Irish directives on animal welfare (2010/63/EU, SI No 543 of 2012). The sampling infrastructure (fish traps) operates under license (Fisheries Acts 1959–2003) from the Department of Agriculture, Food and Marine and by permission of the Minister of Agriculture, Food and Marine.
Author Contributions
EE, SK, and AF conceived this study. EE carried out the analysis and wrote the first draft. All authors contributed to data collection and edited the final submission.
Funding
PMcG was supported by the Marine Institute under the Marine Research Programme 2014–2020 RESPI/BIO/21/01 and by the SFI-DEL Investigators Award 2015 15/IA/3028. AF was funded by the WATExR project, which is part of ERA4CS, an ERA-NET initiated by JPI Climate, and funded by MINECO (ES), FORMAS (SE), BMBF (DE), EPA (IE), RCN (NO), and IFD (DK), with co-funding by the European Union (Grant Number: 690462).
Conflict of Interest
The authors declare that the research was conducted in the absence of any commercial or financial relationships that could be construed as a potential conflict of interest.
Publisher’s Note
All claims expressed in this article are solely those of the authors and do not necessarily represent those of their affiliated organizations, or those of the publisher, the editors and the reviewers. Any product that may be evaluated in this article, or claim that may be made by its manufacturer, is not guaranteed or endorsed by the publisher.
Acknowledgments
The data collection for this work was carried out by the many staff of the Marine Institute (formerly the Salmon Research Agency and the Salmon Research Trust of Ireland). Their diligence and foresight is gratefully acknowledged. Karl Phillips and Joshka Kaufmann provided coding assistance to EE, and Louise Vaughan provided assistance in locating oceanic data. We thank our two reviewers and Tom Reed for constructive comments on previous drafts.
Supplementary Material
The Supplementary Material for this article can be found online at: https://www.frontiersin.org/articles/10.3389/fevo.2022.915854/full#supplementary-material
Footnotes
- ^ http://www.ott.com/products/water-level-1/ott-orpheus-mini-water-level-logger-3/
- ^ https://www.seabird.com/
- ^ https://missingsalmonalliance.org/
- ^ http://salmonatsea.com/
- ^ https://www.seasalar.no/
References
Adams, C. E., and Thorpe, J. E. (1989). Photoperiod and temperature effects on early development and reproductive investment in Atlantic salmon (Salmo salar L.). Aquaculture 79, 403–409. doi: 10.1016/0044-8486(89)90483-3
Almodóvar, A., Nicola, G. G., Ayllón, D., Trueman, C. N., Davidson, I., Kennedy, R., et al. (2020). Stable isotopes suggest the location of marine feeding grounds of South European Atlantic salmon in Greenland. ICES J. Mar. Sci. 77, 593–603. doi: 10.1093/icesjms/fsz258
Als, T. D., Hansen, M. M., Maes, G. E., Castonguay, M., Riemann, L., Aarestrup, K., et al. (2011). All roads lead to home: panmixia of European eel in the Sargasso Sea. Mol. Ecol. 20, 1333–1346. doi: 10.1111/j.1365-294X.2011.05011.x
Andersson, J., Florin, A.-B., and Petersson, E. (2012). Escapement of eel (Anguilla anguilla) in coastal areas in Sweden over a 50-year period. ICES J. Mar. Sci. 69, 991–999. doi: 10.1093/icesjms/fss094
Arevalo, E., Drouineau, H., Tétard, S., Durif, C. M. F., Diserud, O. H., Poole, W. R., et al. (2021a). Joint temporal trends in river thermal and hydrological conditions can threaten the downstream migration of the critically endangered European eel. Sci Rep 11:16927. doi: 10.1038/s41598-021-96302-x
Arevalo, E., Lassalle, G., Tétard, S., Maire, A., Sauquet, E., Lambert, P., et al. (2020). An innovative bivariate approach to detect joint temporal trends in environmental conditions: application to large French rivers and diadromous fish. Sci. Total Environ. 748:141260. doi: 10.1016/j.scitotenv.2020.141260
Arevalo, E., Maire, A., Tétard, S., Prévost, E., Lange, F., Marchand, F., et al. (2021b). Does global change increase the risk of maladaptation of Atlantic salmon migration through joint modifications of river temperature and discharge? Proc. R. Soc. B Biol. Sci. 288:20211882. doi: 10.1098/rspb.2021.1882
Atkinson, D. (1994). Temperature and organism size: a biological law for ectotherms? Adv. Ecol. Res. 25, 1–58. doi: 10.1016/S0065-2504(08)60212-3
Bal, G., Montorio, L., Rivot, E., Prévost, E., Baglinière, J.-L., and Nevoux, M. (2017). Evidence for long-term change in length, mass and migration phenology of anadromous spawners in French Atlantic salmon Salmo salar. J. Fish Biol. 90, 2375–2393. doi: 10.1111/jfb.13314
Balm, S. P., Durif, C., Verstegen, M. W. A., Antonissen, E., Boot, R., Thillart, G., et al. (2007). Silvering of European eel (Anguilla anguilla L.): seasonal changes of morphological and metabolic parameters. Animal Biol. 57, 63–77. doi: 10.1163/157075607780002014
Bark, A., Williams, B., and Knights, B. (2007). Current status and temporal trends in stocks of European eel in England and Wales. ICES J. Mar. Sci. 64, 1368–1378.
Bauer, S., and Hoye, B. J. (2014). Migratory animals couple biodiversity and ecosystem functioning worldwide. Science 344:1242552. doi: 10.1126/science.1242552
Belletti, B., Garcia, de Leaniz, C., Jones, J., Bizzi, S., Börger, L., et al. (2020). More than one million barriers fragment Europe’s rivers. Nature 588, 436–441. doi: 10.1038/s41586-020-3005-2
Bertrand, R., Lenoir, J., Piedallu, C., Riofrío-Dillon, G., de Ruffray, P., Vidal, C., et al. (2011). Changes in plant community composition lag behind climate warming in lowland forests. Nature 479, 517–520. doi: 10.1038/nature10548
Björnsson, B. (1997). The biology of salmon growth hormone: from daylight to dominance. Fish Physiol. Biochem. 17, 9–24. doi: 10.1023/A:1007712413908
Blenckner, T., Adrian, R., Livingstone, D. M., Jennings, E., Weyhenmeyer, G. A., George, D. G., et al. (2007). Large-scale climatic signatures in lakes across Europe: a meta-analysis. Glob. Change Biol. 13, 1314–1326. doi: 10.1111/j.1365-2486.2007.01364.x
Bolstad, G. H., Karlsson, S., Hagen, I. J., Fiske, P., Urdal, K., Sægrov, H., et al. (2021). Introgression from farmed escapees affects the full life cycle of wild Atlantic salmon. Sci. Adv. 7:eabj3397. doi: 10.1126/sciadv.abj3397
Brown, C. J., O’Connor, M. I., Poloczanska, E. S., Schoeman, D. S., Buckley, L. B., Burrows, M. T., et al. (2016). Ecological and methodological drivers of species’ distribution and phenology responses to climate change. Glob. Change Biol. 22, 1548–1560. doi: 10.1111/gcb.13184
Bruijs, M. C. M., and Durif, C. M. F. (2009). “Silver eel migration and behaviour,” in Spawning Migration of the European Eel: Reproduction Index, a Useful Tool for Conservation Management Fish & Fisheries Series, eds G. van den Thillart, S. Dufour, and J. C. Rankin (Dordrecht: Springer). 65–95. doi: 10.1007/978-1-4020-9095-0_4
Brunel, T., and Dickey-Collas, M. (2010). Effects of temperature and population density on von Bertalanffy growth parameters in Atlantic herring: a macro-ecological analysis. Mar. Ecol. Prog. Ser. 405, 15–28. doi: 10.3354/meps08491
Burthe, S., Daunt, F., Butler, A., Elston, D. A., Frederiksen, M., Johns, D., et al. (2012). Phenological trends and trophic mismatch across multiple levels of a North Sea pelagic food web. Mar. Ecol. Prog. Ser. 454, 119–133. doi: 10.3354/meps09520
Byrne, C. J., Poole, R., Dillane, M., Rogan, G., and Whelan, K. F. (2004). Temporal and environmental influences on the variation in sea trout (Salmo trutta L.) smolt migration in the Burrishoole system in the west of Ireland from 1971 to 2000. Fish. Res. 66, 85–94. doi: 10.1016/S0165-7836(03)00146-2
Byrne, C. J., Poole, R., Rogan, G., Dillane, M., and Whelan, K. F. (2003). Temporal and environmental influences on the variation in Atlantic salmon smolt migration in the Burrishoole system 1970–2000. J. Fish Biol. 63, 1552–1564. doi: 10.1111/j.1095-8649.2003.00266.x
Caesar, L., McCarthy, G. D., Thornalley, D. J. R., Cahill, N., and Rahmstorf, S. (2021). Current atlantic meridional overturning circulation weakest in last millennium. Nat. Geosci. 14, 118–120. doi: 10.1038/s41561-021-00699-z
Carlsen, K. T., Berg, O. K., Finstad, B., and Heggberget, T. G. (2004). Diel periodicity and environmental influence on the smolt migration of Arctic charr, Salvelinus alpinus, atlantic salmon, Salmo salar, and brown trout, Salmo trutta, in Northern Norway. Environ. Biol. Fish. 70, 403–413. doi: 10.1023/B:EBFI.0000035438.85321.fa
Carslaw, D., and Ropkins, K. (2012). openair - an R package for air quality data analysis. Environ. Model. Softw. 2, 52–61. doi: 10.1016/j.envsoft.2011.09.008
Cohen, J. M., Lajeunesse, M. J., and Rohr, J. R. (2018). A global synthesis of animal phenological responses to climate change. Nat. Clim. Change 8, 224–228. doi: 10.1038/s41558-018-0067-3
Cooke, S. J., Hinch, S. G., Farrell, A. P., Lapointe, M. F., Jones, S. R. M., Macdonald, J. S., et al. (2004). Abnormal migration timing and high en route mortality of sockeye salmon in the Fraser River, British Columbia. Fisheries 29, 22–33.
Crozier, L. G., Hendry, A. P., Lawson, P. W., Quinn, T. P., Mantua, N. J., Battin, J., et al. (2008). Potential responses to climate change in organisms with complex life histories: evolution and plasticity in Pacific salmon. Evol. Appl. 1:252–270. doi: 10.1111/j.1752-4571.2008.00033.x
Crozier, W., Whelan, K., Buoro, M., Chaput, G., Daniels, J., Grant, S., et al. (2018). Atlantic Salmon Mortality at Sea: Developing an Evidence-Based “Likely Suspects” Framework. Edinburgh: Atlantic Salmon Trust Blue Book.
Daufresne, M., Lengfellner, K., and Sommer, U. (2009). Global warming benefits the small in aquatic ecosystems. PNAS 106, 12788–12793. doi: 10.1073/pnas.0902080106
Daverat, F., Beaulaton, L., Poole, R., Lambert, P., Wickström, H., Andersson, J., et al. (2012). One century of eel growth: changes and implications. Ecol. Freshw. Fish 21, 325–336. doi: 10.1111/j.1600-0633.2011.00541.x
Davidsen, J. G., Finstad, B., ØKland, F., Thorstad, E. B., Mo, T. A., and Rikardsen, A. H. (2011). Early marine migration of European silver eel Anguilla anguilla in northern Norway. J. Fish Biol. 78, 1390–1404. doi: 10.1111/j.1095-8649.2011.02943.x
de Eyto, E., Dalton, C., Dillane, M., Jennings, E., McGinnity, P., O’Dwyer, B., et al. (2016). The response of North Atlantic diadromous fish to multiple stressors, including land use change: a multidecadal study. Can. J. Fish. Aquat. Sci. 73, 1759–1769. doi: 10.1139/cjfas-2015-0450
de Eyto, E., White, J., Boylan, P., Clarke, B., Cotter, D., Doherty, D., et al. (2015). The fecundity of wild Irish Atlantic salmon Salmo salar L. and its application for stock assessment purposes. Fish. Res. 164, 159–169. doi: 10.1016/j.fishres.2014.11.017
De Leo, G. A., and Gatto, M. (1996). Trends in vital rates of the European Eel: evidence for density dependence? Ecol. Appl. 6, 1281–1294. doi: 10.2307/2269607
Dempson, B., Schwarz, C. J., Bradbury, I. R., Robertson, M. J., Veinott, G., Poole, R., et al. (2017). Influence of climate and abundance on migration timing of adult Atlantic salmon (Salmo salar) among rivers in Newfoundland and Labrador. Ecol. Freshw. Fish 26, 247–259. doi: 10.1111/eff.12271
Dillane, M., de Eyto, E., Cooney, J., Hughes, P., Murphy, M., Nixon, P., et al. (2018). Midnight Surface Water Temperatures from the Mill Race. Furnace: Mayo Marine Institute. Available online at: 10/cvft.
Dokulil, M. T., de Eyto, E., Maberly, S. C., May, L., Weyhenmeyer, G. A., and Woolway, R. I. (2021). Increasing maximum lake surface temperature under climate change. Clim. Change 165:56. doi: 10.1007/s10584-021-03085-1
Durif, C., Dufour, S., and Elie, P. (2005). The silvering process of Anguilla anguilla: a new classification from the yellow resident to the silver migrating stage. J. Fish Biol. 66, 1025–1043. doi: 10.1111/j.0022-1112.2005.00662.x
Fealy, R., Allott, N., Broderick, C., de Eyto, E., Dillane, M., Erdil, R. M., et al. (2014). RESCALE: Review and Simulate Climate and Catchment Responses at Burrishoole. Galway. Maynooth: National University of Ireland.
Fleming, I. A., and Reynolds, J. D. (2004). “Salmonid breeding systems,” in Evolution Illuminated: Salmon and their Relatives, eds A. P. Hendry and S. C. Stearns (Oxford: Oxford University Press), 264–294.
Friedland, K. D. (1998). Ocean climate influences on critical Atlantic salmon (Salmo salar) life history events. Can. J. Fish. Aquat. Sci. 55, 119–130. doi: 10.1139/d98-003
Gargan, P., Poole, W. R., and Forde, G. (2006). “A review of the status of Irish Sea trout stocks,” in Sea Trout: Biology, Conservation and Management, ed. N. M. Graeme Harris (Oxford: Blackwell), 25–44.
Gende, S. M., Edwards, R. T., Willson, M. F., and Wipfli, M. S. (2002). Pacific salmon in aquatic and terrestrial ecosystems: pacific salmon subsidize freshwater and terrestrial ecosystems through several pathways, which generates unique management and conservation issues but also provides valuable research opportunities. BioScience 52, 917–928.
Gilbey, J., Utne, K. R., Wennevik, V., Beck, A. C., Kausrud, K., Hindar, K., et al. (2021). The early marine distribution of Atlantic salmon in the North-east Atlantic: a genetically informed stock-specific synthesis. Fish Fish. 22, 1274–1306. doi: 10.1111/faf.12587
Haraldstad, Ø, Vøllestad, L. A., and Jonsson, B. (1985). Descent of European silver eels, Anguilla anguilla L., in a Norwegian watercourse. J. Fish Biol. 26, 37–41. doi: 10.1111/j.1095-8649.1985.tb04238.x
Harrison, A. J., Walker, A. M., Pinder, A. C., Briand, C., and Aprahamian, M. W. (2014). A review of glass eel migratory behaviour, sampling techniques and abundance estimates in estuaries: implications for assessing recruitment, local production and exploitation. Rev. Fish Biol. Fish. 24, 967–983. doi: 10.1007/s11160-014-9356-8
Harvey, A. C., Glover, K. A., Wennevik, V., and Skaala, Ø (2020). Atlantic salmon and sea trout display synchronised smolt migration relative to linked environmental cues. Sci. Rep. 10:3529. doi: 10.1038/s41598-020-60588-0
Hedger, R. D., Sundt-Hansen, L. E., Forseth, T., Ugedal, O., Diserud, O. H., Kvambekk, ÅS., et al. (2013). Predicting climate change effects on subarctic–arctic populations of atlantic salmon (Salmo salar). Can. J. Fish. Aquat. Sci. 70, 159–168. doi: 10.1139/cjfas-2012-0205
Hembrel, B., Arnekleiv, J. V., and L’Abée-Lund, J. H. (2001). Effects of water discharge and temperature on the seaward migration of anadromous browntrout, Salmo trutta, smolts. Ecol. Freshw. Fish 10, 61–64. doi: 10.1111/j.1600-0633.2001.tb00195.x
Hoar, W. S. (1988). “4 the physiology of smolting salmonids,” in Fish Physiology The Physiology of Developing Fish, eds W. S. Hoar and D. J. Randall (Cambridge, MA: Academic Press). 275–343. doi: 10.1016/S1546-5098(08)60216-2
Horne, C. R., Hirst, A. G., and Atkinson, D. (2015). Temperature-size responses match latitudinal-size clines in arthropods, revealing critical differences between aquatic and terrestrial species. Ecol. Lett. 18, 327–335. doi: 10.1111/ele.12413
Huang, B., Thorne, P. W., Banzon, V. F., Boyer, T., Chepurin, G., Lawrimore, J. H., et al. (2017a). Extended Reconstructed Sea Surface Temperature, Version 5 (ERSSTv5). Available online at: https://www.ncei.noaa.gov/access/metadata/landing-page/bin/iso?id=gov.noaa.ncdc:C00927 [Accessed December 15, 2017]
Huang, B., Thorne, P. W., Banzon, V. F., Boyer, T., Chepurin, G., Lawrimore, J. H., et al. (2017b). Extended reconstructed sea surface temperature, version 5 (ERSSTv5): upgrades, validations, and intercomparisons. J. Clim. 30, 8179–8205. doi: 10.1175/JCLI-D-16-0836.1
Hvidsten, N. A., Jensen, A. J., Rikardsen, A. H., Finstad, B., Aure, J., Stefansson, S., et al. (2009). Influence of sea temperature and initial marine feeding on survival of Atlantic salmon Salmo salar post-smolts from the Rivers Orkla and Hals, Norway. J. Fish Biol. 74, 1532–1548. doi: 10.1111/j.1095-8649.2009.02219.x
Ikpewe, I. E., Baudron, A. R., Ponchon, A., and Fernandes, P. G. (2021). Bigger juveniles and smaller adults: changes in fish size correlate with warming seas. J. Appl. Ecol. 58, 847–856. doi: 10.1111/1365-2664.13807
Iler, A. M., CaraDonna, P. J., Forrest, J. R. K., and Post, E. (2021). Demographic consequences of phenological shifts in response to climate change. Ann. Rev. Ecol. Evol. Syst. 52, 221–245. doi: 10.1146/annurev-ecolsys-011921-032939
Jennings, E., Allott, N., McGinnity, P., Poole, R., Quirke, W., Twomey, H., et al. (2000). The North Atlantic oscillation: effects on freshwater systems in Ireland. biology and environment. Proc. R. Ir. Acad. 100B, 149–157.
Jonsson, B., and Jonsson, N. (2009a). A review of the likely effects of climate change on anadromous Atlantic salmon Salmo salar and brown trout Salmo trutta, with particular reference to water temperature and flow. J. Fish Biol. 75, 2381–2447. doi: 10.1111/j.1095-8649.2009.02380.x
Jonsson, B., and Jonsson, N. (2009b). Migratory timing, marine survival and growth of anadromous brown trout Salmo trutta in the River Imsa. Norway. J. Fish Biol. 74, 621–638. doi: 10.1111/j.1095-8649.2008.02152.x
Juanes, F., Gephard, S., and Beland, K. F. (2004). Long-term changes in migration timing of adult Atlantic salmon (Salmo salar) at the southern edge of the species distribution. Can. J. Fish. Aquat. Sci. 61, 2392–2400. doi: 10.1139/f04-207
Kennedy, R. J., and Crozier, W. W. (2010). Evidence of changing migratory patterns of wild Atlantic salmon Salmo salar smolts in the River Bush, Northern Ireland, and possible associations with climate change. J. Fish Biol. 76, 1786–1805. doi: 10.1111/j.1095-8649.2010.02617.x
Kovach, R. P., Joyce, J. E., Echave, J. D., Lindberg, M. S., and Tallmon, D. A. (2013). Earlier migration timing, decreasing phenotypic variation, and biocomplexity in multiple salmonid species. PLoS One 8:e53807. doi: 10.1371/journal.pone.0053807
Kuczynski, L., Chevalier, M., Laffaille, P., Legrand, M., and Grenouillet, G. (2017). Indirect effect of temperature on fish population abundances through phenological changes. PLoS One 12:e0175735. doi: 10.1371/journal.pone.0175735
Legrand, M., Briand, C., Buisson, L., Besse, T., Artur, G., Azam, D., et al. (2021). Diadromous fish modified timing of upstream migration over the last 30 years in France. Freshw. Biol. 66, 286–302. doi: 10.1111/fwb.13638
Lennox, R. J., Alexandre, C. M., Almeida, P. R., Bailey, K. M., Barlaup, B. T., Bøe, K., et al. (2021). The quest for successful Atlantic salmon restoration: perspectives, priorities, and maxims. ICES J. Mar. Sci. 78, 3479–3497. doi: 10.1093/icesjms/fsab201
Lennox, R. J., Crook, D. A., Moyle, P. B., Struthers, D. P., and Cooke, S. J. (2019). Toward a better understanding of freshwater fish responses to an increasingly drought-stricken world. Rev. Fish Biol. Fish. 29, 71–92. doi: 10.1007/s11160-018-09545-9
Martin, B. T., Nisbet, R. M., Pike, A., Michel, C. J., and Danner, E. M. (2015). Sport science for salmon and other species: ecological consequences of metabolic power constraints. Ecol. Lett. 18, 535–544. doi: 10.1111/ele.12433
McCarthy, G. D., Gleeson, E., and Walsh, S. (2015). The influence of ocean variations on the climate of Ireland. Weather 70, 242–245. doi: 10.1002/wea.2543
McCormick, S. D., Shrimpton, J. M., Moriyama, S., and Björnsson, B. T. (2002). Effects of an advanced temperature cycle on smolt development and endocrinology indicate that temperature is not a zeitgeber for smolting in Atlantic salmon. J. Exp. Biol. 205, 3553–3560. doi: 10.1242/jeb.205.22.3553
Miller, M. J., Westerberg, H., Sparholt, H., Wysujack, K., Sørensen, S. R., Marohn, L., et al. (2019). Spawning by the European eel across 2000 km of the Sargasso Sea. Biol. Lett. 15:20180835. doi: 10.1098/rsbl.2018.0835
Monteiro, R. M., Domingos, I., Almeida, P. R., Costa, J. L., Alexandre, C. M., and Quintella, B. R. (2020). Migration and escapement of silver eel males, Anguilla anguilla, from a southwestern European river. Ecol. Freshw. Fish 29, 679–692. doi: 10.1111/eff.12545
Murphy, C., Broderick, C., Burt, T. P., Curley, M., Duffy, C., Hall, J., et al. (2018). A 305-year continuous monthly rainfall series for the island of Ireland (1711–2016). Clim. Past 14, 413–440. doi: 10.5194/cp-14-413-2018
Nixon, S. (1999). Guarding the Silver: A life with Salmon and Sea trout.. Westport, Co. Mayo: Berry Print Group.
Ó Maoiléidigh, N., McGinnity, P., Prévost, E., Potter, E. C. E., Gargan, P., Crozier, W., et al. (2004). Application of pre-fishery abundance modelling and Bayesian hierarchical stock and recruitment analysis to the provision of precautionary catch advice for Irish salmon (Salmo salar L.) fisheries. ICES J. Mar. Sci. 61, 1370–1378. doi: 10.1016/j.icesjms.2004.08.014
Ohlson, J. A., and Kim, S. (2015). Linear valuation without OLS: the Theil-Sen estimation approach. Rev. Account Stud. 20, 395–435. doi: 10.1007/s11142-014-9300-0
Oke, K. B., Cunningham, C. J., Westley, P. A. H., Baskett, M. L., Carlson, S. M., Clark, J., et al. (2020). Recent declines in salmon body size impact ecosystems and fisheries. Nat. Commun. 11:4155. doi: 10.1038/s41467-020-17726-z
Olmos, M., Massiot-Granier, F., Prévost, E., Chaput, G., Bradbury, I. R., Nevoux, M., et al. (2019). Evidence for spatial coherence in time trends of marine life history traits of Atlantic salmon in the North Atlantic. Fish Fish. 20, 322–342. doi: 10.1111/faf.12345
Olmos, M., Payne, M. R., Nevoux, M., Prévost, E., Chaput, G., Du Pontavice, H., et al. (2020). Spatial synchrony in the response of a long range migratory species (Salmo salar) to climate change in the North Atlantic Ocean. Glob. Change Biol. 26, 1319–1337. doi: 10.1111/gcb.14913
O’Reilly, C. M., Sharma, S., Gray, D. K., Hampton, S. E., Read, J. S., Rowley, R. J., et al. (2015). Rapid and highly variable warming of lake surface waters around the globe. Geophys. Res. Lett. 42, 10773–10781. doi: 10.1002/2015GL066235
O’Sullivan, R. J., Aykanat, T., Johnston, S. E., Rogan, G., Poole, R., Prodöhl, P. A., et al. (2021). Captive-bred Atlantic salmon released into the wild have fewer offspring than wild-bred fish and decrease population productivity. Proc. R. Soc. B Biol. Sci. 287:20201671. doi: 10.1098/rspb.2020.1671
Otero, J., L’Abée-Lund, J. H., Castro-Santos, T., Leonardsson, K., Storvik, G. O., Jonsson, B., et al. (2014). Basin-scale phenology and effects of climate variability on global timing of initial seaward migration of Atlantic salmon (Salmo salar). Glob. Change Biol. 20, 61–75. doi: 10.1111/gcb.12363
Palm, S., Dannewitz, J., Prestegaard, T., and Wickström, H. (2009). Panmixia in European eel revisited: no genetic difference between maturing adults from southern and northern Europe. Heredity 103, 82–89. doi: 10.1038/hdy.2009.51
Palstra, A. P., Heppener, D. F. M., van Ginneken, V. J. T., Székely, C., and van den Thillart, G. E. E. J. M. (2007). Swimming performance of silver eels is severely impaired by the swim-bladder parasite Anguillicola crassus. J. Exp. Mar. Biol. Ecol. 352, 244–256. doi: 10.1016/j.jembe.2007.08.003
Peyronnet, A., Friedland, K. D., and Maoileidigh, N. Ó (2008). Different ocean and climate factors control the marine survival of wild and hatchery Atlantic salmon Salmo salar in the north-east Atlantic Ocean. J. Fish Biol. 73, 945–962. doi: 10.1111/j.1095-8649.2008.01984.x
Piggins, D. J., and Mills, C. P. R. (1985). Comparative aspects of the biology of naturally produced and hatchery-reared Atlantic salmon smolts (Salmo salar L.). Aquaculture 45, 321–333. doi: 10.1016/0044-8486(85)90278-9
Poole, W. R., Dillane, M., de Eyto, E., Rogan, G., McGinnity, P., and Whelan, K. (2006). “Characteristics of the burrishoole sea trout population: census, marine survival, enhancement and stock-recruitment relationship, 1971-2003,” in Sea Trout: Biology, Conservation and Management, eds G. Harris and N. Milner (Oxford: Blackwell). 279–306.
Poole, W. R., Diserud, O. H., Thorstad, E. B., Durif, C. M., Dolan, C., Sandlund, O. T., et al. (2018). Long-term variation in numbers and biomass of silver eels being produced in two European river systems. ICES J. Mar. Sci. 75, 1627–1637. doi: 10.1093/icesjms/fsy053
Poole, W. R., Reynolds, J. D., and Moriarty, C. (1990). Observations on the silver eel migrations of the burrishoole river system, Ireland, 1959 to 1988. Int. Revue ges. Hydrobiol. Hydrogr. 75, 807–815. doi: 10.1002/iroh.19900750621
Poole, W. R., Whelan, K. F., Dillane, M. G., Cooke, D. J., and Matthews, M. (1996). The performance of sea trout, Salmo trutta L., stocks from the Burrishoole system western Ireland, 1970–1994. Fish. Manag. Ecol. 3, 73–92. doi: 10.1111/j.1365-2400.1996.tb00131.x
Quinn, T. P., McGinnity, P., and Reed, T. E. (2016). The paradox of “premature migration” by adult anadromous salmonid fishes: patterns and hypotheses. Can. J. Fish. Aquat. Sci. 73, 1015–1030. doi: 10.1139/cjfas-2015-0345
Core Team, R. (2019). R: A Language and Environment for Statistical Computing. R Foundation for Statistical Computing. Vienna: R Core Team.
Rand, P. S., Hinch, S. G., Morrison, J., Foreman, M. G. G., MacNutt, M. J., Macdonald, J. S., et al. (2006). Effects of river discharge, temperature, and future climates on energetics and mortality of adult migrating fraser river Sockeye Salmon. Trans. Am. Fish. Soc. 135, 655–667. doi: 10.1577/T05-023.1
Raymond, H. L. (1979). Effects of dams and impoundments on migrations of Juvenile Chinook Salmon and steelhead from the snake river, 1966 to 1975. Trans. Am. Fish. Soc. 108, 505–529. doi: 10.1577/1548-86591979108<505:EODAIO<2.0.CO;2
Reed, T. E., de Eyto, E., O’Higgins, K., Gargan, P., Roche, W., White, J., et al. (2016). Availability of holding habitat in lakes and rivers affects the incidence of spring (premature) upriver migration by Atlantic salmon. Can. J. Fish. Aquat. Sci. 74, 668–679. doi: 10.1139/cjfas-2016-0191
Reed, T. E., Schindler, D. E., Hague, M. J., Patterson, D. A., Meir, E., Waples, R. S., et al. (2011). Time to evolve? potential evolutionary responses of fraser river sockeye salmon to climate change and effects on persistence. PLoS One 6:e20380E. doi: 10.1371/journal.pone.0020380
Reimchen, T. E. (2000). Some ecological and evolutionary aspects of bear-salmon interactions in coastal British Columbia. Can. J. Zool. 78, 448–457. doi: 10.1139/z99-232
Rikardsen, A. H., Righton, D., Strøm, J. F., Thorstad, E. B., Gargan, P., Sheehan, T., et al. (2021). Redefining the oceanic distribution of Atlantic salmon. Sci. Rep. 11:12266. doi: 10.1038/s41598-021-91137-y
Russell, I. C., Aprahamian, M. W., Barry, J., Davidson, I. C., Fiske, P., Ibbotson, A. T., et al. (2012). The influence of the freshwater environment and the biological characteristics of Atlantic salmon smolts on their subsequent marine survival. ICES J. Mar. Sci. 69, 1563–1573. doi: 10.1093/icesjms/fsr208
Sandlund, O. T., Diserud, O. H., Poole, R., Bergesen, K., Dillane, M., Rogan, G., et al. (2017). Timing and pattern of annual silver eel migration in two European watersheds are determined by similar cues. Ecol. Evol. 7, 5956–5966. doi: 10.1002/ece3.3099
Sen, P. K. (1968). Estimates of the regression coefficient based on kendall’s tau. J. Am. Stat. Assoc. 63, 1379–1389. doi: 10.1080/01621459.1968.10480934
Shaw, A. K. (2016). Drivers of animal migration and implications in changing environments. Evol. Ecol. 30, 991–1007. doi: 10.1007/s10682-016-9860-5
Sobocinski, K. L., Greene, C. M., Anderson, J. H., Kendall, N. W., Schmidt, M. W., Zimmerman, M. S., et al. (2021). A hypothesis-driven statistical approach for identifying ecosystem indicators of coho and Chinook salmon marine survival. Ecol. Indic. 124:107403. doi: 10.1016/j.ecolind.2021.107403
Song, Y., Zajic, C. J., Hwang, T., Hakkenberg, C. R., and Zhu, K. (2021). Widespread mismatch between phenology and climate in human-dominated landscapes. AGU Adv. 2:e2021AV000431. doi: 10.1029/2021AV000431
Sparks, M. M., Falke, J. A., Quinn, T. P., Adkison, M. D., Schindler, D. E., Bartz, K., et al. (2019). Influences of spawning timing, water temperature, and climatic warming on early life history phenology in western Alaska Sockeye salmon. Can. J. Fish. Aquat. Sci. 76, 123–135. doi: 10.1139/cjfas-2017-0468
Staurnes, M., Sigholt, T., Åsgård, T., and Baeverfjord, G. (2001). Effects of a temperature shift on seawater challenge test performance in Atlantic salmon (Salmo salar) smolt. Aquaculture 201, 153–159. doi: 10.1016/S0044-8486(01)00654-8
Strangeways, I. (2018). Phenology: plants and animals as meteorological sensors. Weather 73, 86–89. doi: 10.1002/wea.2993
Sweeney, J. (2014). Regional weather and climates of the British Isles – Part 6: Ireland. Weather 69, 20–27.
Teichert, N., Benitez, J.-P., Dierckx, A., Tétard, S., de Oliveira, E., Trancart, T., et al. (2020). Development of an accurate model to predict the phenology of Atlantic salmon smolt spring migration. Aquat. Cons. Mar. Freshw. Ecosyst. 30, 1552–1565. doi: 10.1002/aqc.3382
Theil, H. (1950). A rank-invariant method of linear and polynominal regression analysis (Parts 1-3). Ned. Akad. Wetensch. Proc. Ser. A 53, 1397–1412.
Thorpe, J. E. (2007). Maturation responses of salmonids to changing developmental opportunities. Mar. Ecol. Prog. Ser. 335, 285–288. doi: 10.3354/meps335285
Thorstad, E. B., Bliss, D., Breau, C., Damon-Randall, K., Sundt-Hansen, L. E., Hatfield, E. M. C., et al. (2021). Atlantic salmon in a rapidly changing environment—Facing the challenges of reduced marine survival and climate change. Aquat. Conserv. 31, 2654–2665. doi: 10.1002/aqc.3624
Tillotson, M. D., Arostegui, M. C., Austin, C. S., Lincoln, A. E., Matsubu, W., McElroy, K. N., et al. (2021). Challenges in the identification and interpretation of phenological shifts: anthropogenic influences on adult migration timing in salmonids. Rev. Fish. Sci. Aquacult. 29, 769–790. doi: 10.1080/23308249.2021.1874292
Todd, C. D., Friedland, K. D., MacLean, J. C., Whyte, B. D., Russell, I. C., Lonergan, M. E., et al. (2012). Phenological and phenotypic changes in Atlantic salmon populations in response to a changing climate. ICES J. Mar. Sci. 69, 1686–1698. doi: 10.1093/icesjms/fss151
Tréhin, C., Rivot, E., Lamireau, L., Meslier, L., Besnard, A.-L., Gregory, S. D., et al. (2021). Growth during the first summer at sea modulates sex-specific maturation schedule in Atlantic salmon. Can. J. Fish. Aquat. Sci. 78, 659–669. doi: 10.1139/cjfas-2020-0236
Utne, K. R., Pauli, B. D., Haugland, M., Jacobsen, J. A., Maoileidigh, N., Melle, W., et al. (2021). Poor feeding opportunities and reduced condition factor for salmon post-smolts in the Northeast Atlantic Ocean. ICES J. Mar. Sci. 78, 2844–2857. doi: 10.1093/icesjms/fsab163
Vähä, J.-P., Erkinaro, J., Niemelä, E., Primmer, C. R., Saloniemi, I., Johansen, M., et al. (2011). Temporally stable population-specific differences in run timing of one-sea-winter Atlantic salmon returning to a large river system. Evol. Appl. 4, 39–53. doi: 10.1111/j.1752-4571.2010.00131.x
Valiente, A. G., Juanes, F., and Garcia-Vazquez, E. (2011). Increasing regional temperatures associated with delays in atlantic salmon sea-run timing at the southern edge of the European Distribution. Trans. Am. Fish. Soc. 140, 367–373. doi: 10.1080/00028487.2011.557018
van Walraven, L., Mollet, F. M., van Damme, C. J. G., and Rijnsdorp, A. D. (2010). Fisheries-induced evolution in growth, maturation and reproductive investment of the sexually dimorphic North Sea plaice (Pleuronectes platessa L.). J. Sea Res. 64, 85–93. doi: 10.1016/j.seares.2009.07.003
Vaughan, L., Brophy, D., O’Toole, C., Graham, C. Ó, Maoiléidigh, N., and Poole, R. (2021). Growth rates in a European eel Anguilla anguilla (L., 1758) population show a complex relationship with temperature over a seven-decade otolith biochronology. ICES J. Mar. Sci. 78, 994–1009. doi: 10.1093/icesjms/fsaa253
Vøllestad, L. A., Jonsson, B., Hvidsten, N. A., Næsje, T. F., Haraldstad, Ø, and Ruud-Hansen, J. (1986). Environmental factors regulating the seaward migration of European Silver Eels (Anguilla anguilla). Can. J. Fish. Aquat. Sci. 43, 1909–1916. doi: 10.1139/f86-236
Vollset, K. W., Urdal, K., Utne, K., Thorstad, E. B., Sægrov, H., Raunsgard, A., et al. (2022). Ecological regime shift in the Northeast Atlantic Ocean revealed from the unprecedented reduction in marine growth of Atlantic salmon. Sci. Adv. 8:eabk2542. doi: 10.1126/sciadv.abk2542
Webb, L. B., Whetton, P. H., Bhend, J., Darbyshire, R., Briggs, P. R., and Barlow, E. W. R. (2012). Earlier wine-grape ripening driven by climatic warming and drying and management practices. Nat. Clim Change 2, 259–264. doi: 10.1038/nclimate1417
Wilkinson, M. D., Dumontier, M., Aalbersberg, Ij. J, Appleton, G., Axton, M., Baak, A., et al. (2016). The FAIR Guiding Principles for scientific data management and stewardship. Sci. Data 3:160018. doi: 10.1038/sdata.2016.18
Winter, E. R., Tummers, J. S., Aarestrup, K., Baktoft, H., and Lucas, M. C. (2016). Investigating the phenology of seaward migration of juvenile brown trout (Salmo trutta) in two European populations. Hydrobiologia 775, 139–151. doi: 10.1007/s10750-016-2720-z
Woods, T., Kaz, A., and Giam, X. (2022). Phenology in freshwaters: a review and recommendations for future research. Ecography 2022:e05564. doi: 10.1111/ecog.05564
Woodward, G., Morris, O., Barquín, J., Belgrano, A., Bull, C., de Eyto, E., et al. (2021). Using food webs and metabolic theory to monitor, model, and manage Atlantic Salmon—a keystone species under threat. Front. Ecol. Evol. 9:912. doi: 10.3389/fevo.2021.675261
Woolway, R. I., Weyhenmeyer, G. A., Schmid, M., Dokulil, M. T., de Eyto, E., Maberly, S. C., et al. (2019). Substantial increase in minimum lake surface temperatures under climate change. Clim. Change 155, 81–94. doi: 10.1007/s10584-019-02465-y
Keywords: salmon, trout, eel, freshwater, marine
Citation: de Eyto E, Kelly S, Rogan G, French A, Cooney J, Murphy M, Nixon P, Hughes P, Sweeney D, McGinnity P, Dillane M and Poole R (2022) Decadal Trends in the Migration Phenology of Diadromous Fishes Native to the Burrishoole Catchment, Ireland. Front. Ecol. Evol. 10:915854. doi: 10.3389/fevo.2022.915854
Received: 08 April 2022; Accepted: 17 June 2022;
Published: 05 July 2022.
Edited by:
Karin Charlotta Harding, University of Gothenburg, SwedenReviewed by:
José Lino Vieira De Oliveira Costa, University of Lisbon, PortugalFinnbar Lee, The University of Auckland, New Zealand
Copyright © 2022 de Eyto, Kelly, Rogan, French, Cooney, Murphy, Nixon, Hughes, Sweeney, McGinnity, Dillane and Poole. This is an open-access article distributed under the terms of the Creative Commons Attribution License (CC BY). The use, distribution or reproduction in other forums is permitted, provided the original author(s) and the copyright owner(s) are credited and that the original publication in this journal is cited, in accordance with accepted academic practice. No use, distribution or reproduction is permitted which does not comply with these terms.
*Correspondence: Elvira de Eyto, ZWx2aXJhLmRlZXl0b0BtYXJpbmUuaWU=