- 1Graduate School of Media and Governance, Keio University, Fujisawa, Japan
- 2Institute for Advanced Biosciences, Keio University, Tsuruoka, Japan
- 3Faculty of Environment and Information Studies, Keio University, Fujisawa, Japan
While most ant species establish a colony independently, some socially parasitic ants build the foundation of their colony by invading other ant (host) colonies and utilizing their labor forces. Many socially parasitic ants disguise their cuticular hydrocarbon (CHC) profile, which is also known as signature mixture for nestmate discrimination, when invading the host colony. Since the strategy of chemical disguise is widespread in socially parasitic ants, elucidating the mechanism of chemical disguise will promote knowledge on the evolutionary history of social parasitism. However, detailed knowledge is still lacking, as the relevant information has only originated from circumstantial evidence, which was obtained from ecological observations. In this study, we investigated the mechanism of chemical disguise in a new queen of a temporary socially parasitic spiny ant (Polyrhachis lamellidens) by measuring its CHC profile, performing a tracing assay with labeled substances, and analyzing gene expression levels. First, after rubbing behavior was observed against the host workers, the CHC profile in P. lamellidens shifted to pronounced peaks that closely resembling that of the host workers. We also observed a reduction in aggressive behaviors by the host ant against P. lamellidens after rubbing behavior was performed. In addition, P. lamellidens acquired artificially-applied labeling substances from host workers through their rubbing behaviors, while gene expression profiling showed the expression of CHC synthesis-related genes did not change during this behavior. These results suggest that P. lamellidens directly obtains host CHCs through rubbing behavior, and these host CHCs enables P. lamellidens to remain disguised during colony invasion.
Introduction
There are several hydrocarbons present on ant cuticles, and these hydrocarbons function as semiochemicals (Detrain et al., 1999; Howard and Blomquist, 2005; Sturgis and Gordon, 2012). The cuticular hydrocarbon (CHC) profile of ants varies among different colonies. Ants discriminate between nestmates and foreign enemies (non-nestmates) by recognizing the differences in CHC profiles and behave aggressively toward individuals with different CHC profiles, even if the non-nestmates are the same species. On the other hand, solitary organisms that evade the nestmate discrimination by ants and utilize the workforce of ants are referred to as “myrmecophiles” (Kistner, 1982). In addition, social insects with strategies similar to myrmecophiles are referred “social parasites” (Buschinger, 2009). It is known that some myrmecophile species and socially parasitic ants disguise their own CHC profile to obtain a similar profile to that of the host. This strategy is called “chemical disguise” (e.g., Hojo et al., 2009; Nehring et al., 2016). It is believed that myrmecophiles and socially parasitic ants utilize chemical disguises to avoid being exclusively discriminated as non-nestmates by host ants (Dettner and Liepert, 1994; Lenoir et al., 2001; Akino, 2008). For example, when a newly mated queen in the slave-making parasitic ant Polyergus (Hymenoptera: Formicidae) enters a host colony, she temporarily avoids aggressive behaviors from the host worker by emitting a repellent substance, then she kills the host queen, disguises her CHC profile, and exhibits the CHC profile of the host queen (Zimmerli and Topoff, 1994; Mori et al., 1995; d’Ettorre and Errard, 1998; d’Ettorre et al., 2000; Johnson et al., 2001; Tsuneoka, 2008; Tsuneoka and Akino, 2009, Tsuneoka and Akino, 2012).
There are two methods of chemical disguise, including the chemical camouflage and chemical mimicry methods (Dettner and Liepert, 1994; Lenoir et al., 2001; Akino, 2008). Howard et al. (1990a,b) defined that organisms utilize the methods of “chemical camouflage” to acquire the CHC profile from the host directly, and “chemical mimicry” is utilized to biosynthesize host-like CHC. Some studies have experimentally verified the mechanism of chemical disguise in several myrmecophile species, such as spiders and crickets (Vander Meer and Wojcik, 1982; Howard et al., 1990b; Akino et al., 1996; von Beeren et al., 2011, 2012; Scarparo et al., 2019). The mechanism of chemical disguise was estimated in socially parasitic ants through ecological observations (Lenoir et al., 1997; Bauer et al., 2010). However, there is little information on the quantitative and molecular mechanisms that describe the intricacies of chemical disguise. Since the strategy of chemical disguise is a feature that is unique to socially parasitic ants, we believe that elucidating the molecular basis of the detailed mechanisms will elucidate the evolutionary history of social parasitism.
Polyrhachis lamellidens is an appropriate species to utilize when investigating the detailed mechanism of chemical disguise. There are approximately 700 species in the genus Polyrhachis (Bolton, 2022), of which three socially parasitic species are included. Polyrhachis lama and Polyrhachis loweryi are xenobiosis (Maschwitz et al., 2000, 2003, 2004; Witte et al., 2009), whereas P. lamellidens is the only temporary socially parasitic ant species in the genus Polyrhachis. P. lamellidens utilizes several species of hosts, including Camponotus japonicus and Camponotus obscuripes, and the presumed candidate host, Camponotus kiusiuensis (Yano, 1911; Kohriba, 1963, 1966; Kubota, 1974; Sakai, 1990, 1996, 2000; Japanese Ant Database Group, 2003; Iwai et al., 2021; Kurihara et al., 2022). These host Camponotus species overlap habitat with the initial nesting sites of P. lamellidens [this species changes its nesting site at the end of the social parasitism (Kohriba, 1966; Japanese Ant Database Group, 2003)], and are common species here (Japanese Ant Database Group, 2003). As morphological similarity (e.g., body size) of parasite between its host has been pointed out as the recruitment of social parasitism (Fischer et al., 2020), C. japonicus and C. obscuripes are the only species of similar body size in the habitat of P. lamellidens (Japanese Ant Database Group, 2003). The social parasitism of P. lamellidens begins by the invasion of the host nest by the newly mated queen and murder of the host queen. After a successful killing host queen, the newly mated queen starts oviposition, and all eggs are raised by host workers. As a result, all of the new larvae are only P. lamellidens, and the host workers will continue to work for P. lamellidens until they die. Eventually, the colony will be consisted with only P. lamellidens (Sakai, 1996, 2000; Japanese Ant Database Group, 2003; Iwai et al., 2021). In this species, the newly mated queen performs distinct behaviors when invading the host colony, and these behaviors include straddling the host worker and rubbing its body with her legs (Kohriba, 1963; Kubota, 1974; Sakai, 1990, 2000; Japanese Ant Database Group, 2003; Kurihara et al., 2022) (Figure 1A). This behavior, which is known as a “rubbing behavior,” is unique to P. lamellidens queen that are newly mated, is considered a strategy of chemical disguise, and prevents attacks by the host (Kohriba, 1963); however, little is known about the molecular machinery of this strategy.
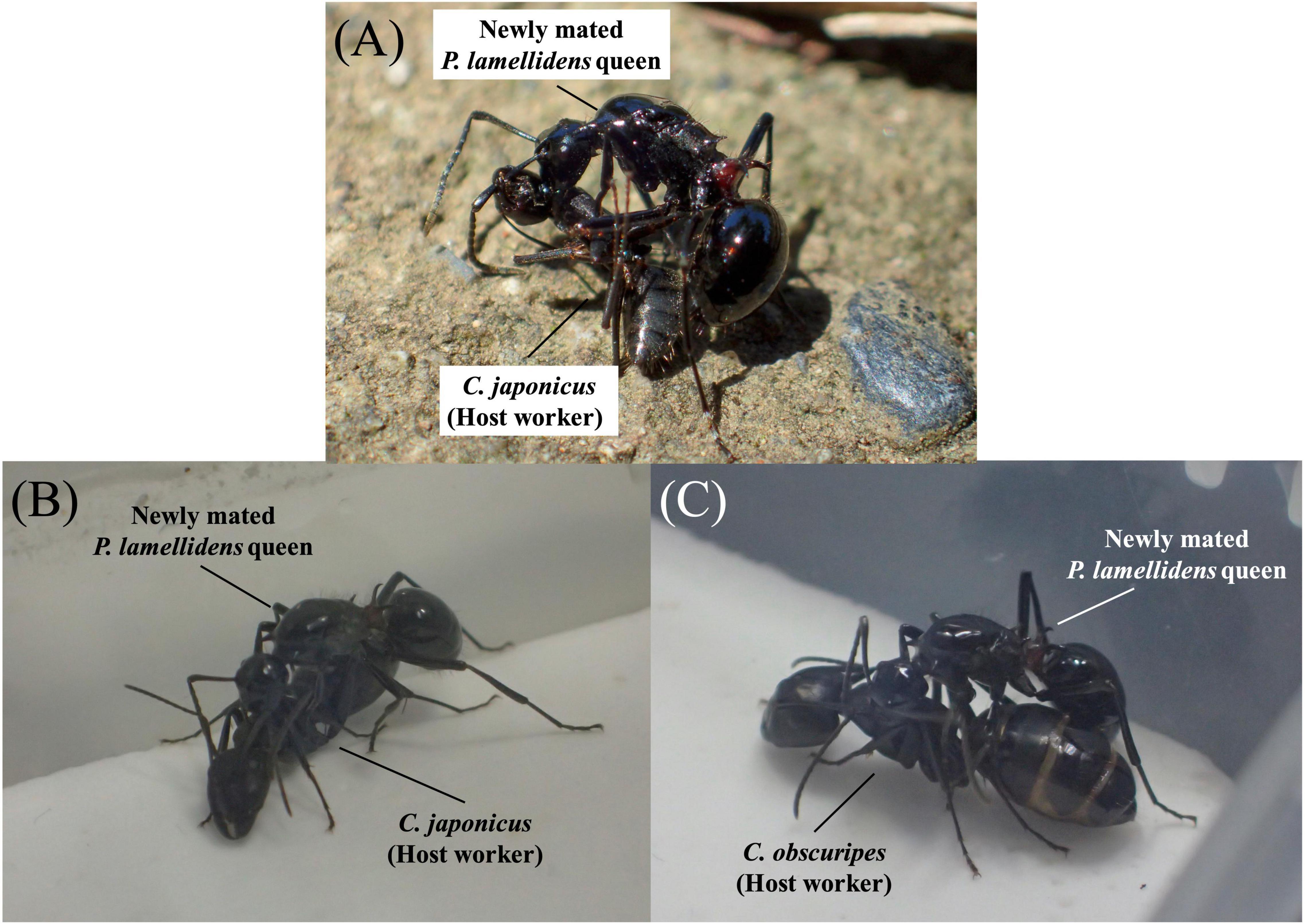
Figure 1. The rubbing behavior of newly mated Polyrhachis lamellidens queens. (A) The newly mated P. lamellidens queen exhibits rubbing behaviour towards its host worker (C. japonicus) in a natural environment at a mixed forest in Nagaoka City, Niigata Prefecture (N 37°25′51′′, E 138°52′53′′). Adapted from Kurihara et al. (2022) (https://doi.org/10.1242/bio.058956). (B) Rubbing behavior against C. japonicus workers under laboratory conditions. (C) Rubbing behavior against Camponotus obscuripes workers under laboratory conditions.
In this study, we quantitatively evaluated the efficiency of rubbing behavior-induced chemical disguise by measuring CHC profiles and conducting behavioral tests, and this was performed to clarify the effect of rubbing behavior on the CHC of P. lamellidens and nestmate recognition of host workers. Furthermore, we elucidated the mechanisms of the disguise strategy of P. lamellidens by performing tracing assays with the labeled substances and by analyzing the expression of related genes, aiming to reveal the behavioral ecology, biochemistry, and molecular biology foundations that explain social parasitism in ants. Therefore, in this study, GC-MS analyses of CHC were performed to quantitatively evaluate the extent to which the rubbing behavior achieves chemical disguise. Through behavioral experiments, we examined the reactions of host workers to the chemical disguise obtained by rubbing behavior. Furthermore, by labeling assays and gene expression profiling, we investigated whether chemical disguise is implemented by camouflage or mimicry.
Materials and Methods
Sampling and Rearing
Ant samples were collected in Niigata, Yamanashi, and Yamagata Prefecture, Japan (2015–2021). Approximately 50 newly mated P. lamellidens queens were collected from a mixed forest in Nagaoka City, Niigata Prefecture (N 37°25′51′′, E 138°52′53′′), and after the queens finished their nuptial flights, their original colony was not known. A P. lamellidens colony was collected from a primeval beech forest in Nirasaki City, Yamanashi Prefecture (N 35°42′49′′, E 138°29′12′′); the colony approximately consisted of three queens, more than 1,000 workers and larvae. C. japonicus and C. obscuripes colonies were collected from a primeval beech forest in Nagaoka City, Niigata Prefecture (N 37°41′42′′, E 138°88′95′′), a mixed forest in Tsuruoka City, Yamagata Prefecture (N 38°33′49′′, E 139°55′31′′), and a mixed forest in Minamikoma District, Yamanashi Prefecture (N 35°46′11′′, E 138°29′43′′); the average colonies consisted of 100 workers for C. japonicus and one queen and 200 workers for C. obscuripes. The host workers used in each experiment were from the same colony each time.
The rearing method was adjusted to the colony size. Newly mated P. lamellidens queens were reared individually in a plastic tub that was 4.5 cm in length, 2.5 cm in width, and 2.0 cm in height and contained moistened tissue. Each collected ant colony was reared separately in a plastic tub (17.5 cm in length, 8.0 cm in width, 3.0 cm in height, or 20.5 cm in length, 10.5 cm in width, 7.0 cm in height) in which plaster was laid and a feeding area was established. Newly mated P. lamellidens queens were fed 5 μl of 50% maple syrup (Maple Farms Japan, Osaka, Japan) every 7–10 days. Ant colonies were also fed 100–5,000 μl of 50% maple syrup every 7–10 days. Worker ants were also fed mealworms, crickets, cockroaches, and/or 8–16 g of Pro Jelly for beetles and stag beetles (Wraios, Saitama, Japan). Newly mated P. lamellidens queens were reared in a cool incubator (Mitsubishi Electric Engineering Company, Tokyo, Japan) under dark (0 L:24 D) conditions at 15–20°C. C. japonicus and C. obscuripes were reared under light-dark conditions (14 L10 D) at 20–30°C. Different rearing conditions were adopted for each species due to our previous rearing experience. In particular, newly mated P. lamellidens queens were observed to be sensitive to high temperatures, which is why we adopted the above rearing method.
Induction of Rubbing Behavior Under Laboratory Conditions
The newly mated P. lamellidens queen and host workers (C. japonicus or C. obscuripes) were placed in plastic cases that contained plaster (5.0 cm × 4.5 cm × 2.5 cm) (Figure 1 and Supplementary Movie 1). To prevent possible attacks by the host worker before the newly mated P. lamellidens queen performed rubbing behaviors, the host worker was anesthetized through freezing (it was exposed to an environment of 4°C for 2 min and −20°C for 3 min) before the experiment. A newly mated P. lamellidens queen could perform the rubbing behavior for 3 days. When the newly mated P. lamellidens queens continued the rubbing behavior for more than 1 day, we added one new host worker (all from the same colony) every day until the third day. Host workers that spent 1 week in the nest were used this experiment. The newly mated P. lamellidens queens that were prepared in this process were used for the various experiments that followed. The individuals that performed rubbing behavior at least once after contact with the host worker were used for later experiments. In the isolation rearing, only the host workers were removed from the case, and they were maintained for 1–9 days after the rubbing behavior was observed. We conducted the rubbing behavior and isolation rearing experiments under light conditions (24 L:0 D) at 20–30°C and did not feed the ants throughout these experiments. As a control, we also prepared a newly mated P. lamellidens queen without exposure to host workers.
Observations of Host Worker Aggressions Against the Newly Mated P. lamellidens Queen by Behavioral Tests
To observe the aggressive behaviors of host workers on the newly mated P. lamellidens queen, all host workers were removed immediately after rubbing behavior had occurred for 3 days, and the newly mated P. lamellidens queen was transferred to a plastic cup that was lined with plaster (7.6 cm in diameter and 3.8 cm in height). Three host workers from the same colony as that used in the rubbing behavior experiment were transferred into a plastic cup that contained a newly mated P. lamellidens queen that already exhibited rubbing. The first 10 behaviors that host workers performed within 1 min after being transferred to the plastic cup were recorded according to the following definitions:
1. Ignore: the ant does not respond aggressively upon contact with the target.
2. Threaten: the ant opens its mandibles or maintains distance from the target.
3. Rush: the ant approaches and quickly bites the target.
4. Bite: the ant continuously bites the target for at least 1 s.
Among these behaviors, “threaten,” “rush,” and “bite” were considered aggressive behaviors. The host workers were selected at random and were used only once in each test. The host workers used for the test were transferred to another case and were not used in subsequent tests.
Application of Labeled Substances for the Tracing Assay
We used the stable isotopes of n-triacontane (C30H62) and n-dotriacontane (C32H66) as labeled substances, and these substances have similar carbon chain lengths to those of the ant CHC (CAS: 93952-07-9, 62369-68-0). Each substance was dissolved individually in hexane (16 mg/mL), 100 μl of the solution was added to a 300 μl micro insert (GLC4010-S630; Shimadzu, Kyoto, Japan), and the solvents were evaporated with nitrogen gas. The solution was stirred with an end-to-end pipette (AS ONE, Osaka, Japan) which are capillary glass tubes, during which nitrogen gas was sprayed to evenly apply the labeled substances over the entire inner wall of the insert. After the solvent was completely volatilized, the labeled substances were applied to the host body surfaces without inducing injury, and this was achieved by placing the host worker into the insert where the labeled substances (C30D62 and C32D66) were applied to the inner wall and gently shaking the hosts for 1 h using a rotator.
Extraction and Analysis of Hydrocarbons
The method for extracting cuticular hydrocarbons was modified from a previous study (Akino and Yamaoka, 2012). A single individual was exposed to 4°C for 2 min and then −20°C for 3 min for anesthetic purposes and was then immediately placed in a disposable glass tube (trunk diameter: 10 mm, total height: 75 mm) that contained 200 μl of hexane mixed with saturated alkane (docosane: C22H46; 10 ng/μl) as an internal standard material. The chemical materials from the body surfaces of ants were extracted by dipping the ants in hexane for 5 min. After separation in 1X PBS, the chemical materials from the postpharyngeal glands were extracted by being dipped in hexane and were sonicating using a BioruptorII (BM Equipment, Tokyo, Japan). Next, 0.5 g of silica gel C-200 (Wako Pure Chemical Industries, Tokyo, Japan) was used to fractionate hydrocarbons from the extracted materials. After washing with hexane, silica gel was placed inside a glass pipette that was installed on the stand. Fractionation was carried out by adding the extract inside the pipette. At this time, 1 ml of hexane was added into the pipette to elute hydrocarbons. The obtained hydrocarbons were concentrated by applying nitrogen gas until the solvent hexane was completely evaporated. Thereafter, 50 μl of hexane was added to the vial, and the concentrated cuticular hydrocarbon was eluted.
The GC-MS system comprised an Agilent 6890N, and an Agilent 5973 MSD was used to measure hydrocarbons. The column used for MS was an HP-5 MS (length 30 m, diameter 0.250 mm, film thickness 0.25 μm; Agilent Technologies, Santa Clara, CA, United States). Two microliters of sample was injected. The splitless mode was adopted for the sample injection port and the apparatus was maintained at 300°C. Helium was used as the carrier gas at a constant flow rate setting of 0.9 ml/min. The oven temperature was set as follows: 40°C for 3 min, 40 to 260°C at 30°C/min, 260 to 300°C at 15°C/min, and 18 min at the final temperature. C7 to C40 saturated alkanes were used as standard substances.
The estimation of cuticular hydrocarbons and statistical analysis were carried out based on peak areas. Equivalent chain length (ECL), the value of fragment and molecular ions, and the pattern of the mass spectrum was used to estimate the kind of each CHC. A previous study (Ozaki et al., 2005) was referred to estimate the kind of each CHC of C. japonicus. The peak areas were transformed according to Zij = ln[Yij/g(Yj)], where Yij is the peak area i for the individual j values and g(Yj) is the geometric mean of all peak areas for the individual j values (Reyment, 1989). Hierarchical clustering analysis and correlation analysis were performed using a standardized data matrix of CHC to investigate the similarity between parasite and host CHC profiles. In the hierarchical clustering analysis, the Euclidean distance between each sample was calculated and clustered by the Ward method. In the correlation analysis, the Pearson correlation coefficient was calculated, and the similarity of the CHC profile between each group was evaluated. Statistical analysis was carried out using R software v 4.2.01 (R Core Team, 2022), and the gplots package was used to create a heatmap2 (Warnes et al., 2016). The concentration of HCs was quantified by using standards (the mixtures of C7 to C40 saturated alkanes) of known concentrations (0.5, 1, 2, 5, and 10 ng/μl) diluted at five levels, and the internal standard (docosane) added in each sample and above standard. After normalizing the peak areas of each standard and sample by using internal standards, a calibration curve was created standard and quantified the concentration of HCs.
Total RNA Extraction
Total RNA extraction was carried out for the whole body (5 larvae, 1 worker, and 1 queen), abdomen (18 newly mated queens), and fat body in the abdomen (17 newly mated queens) of P. lamellidens according to a previous study (Kono et al., 2016). Samples were exposed to liquid nitrogen and were placed in a ZR BashingBead lysis tube (Zymo Research, Irvine, CA, United States) that contained 600 μl of TRIzol Reagent (Life Technologies, Carlsbad, CA, United States). The samples were crushed with a Multi-Beads Shocker at 2,500 rpm for 30 s (Yasui Kikai, Osaka, Japan), and total RNA was extracted with a Direct-zol RNA MiniPrep (Zymo Research, Irvine, CA, United States) without DNase treatment. The extraction of total RNA from the abdomen fat body was performed according to Koto et al. (2019). The abdomen fat body was dissected from the newly mated P. lamellidens queens in ant saline [4.8 mM TES, which contained 127 mM NaCl, 6.7 mM KCl, 2 mM CaCl2, and 3.5 mM sucrose] and placed in BioMasher II (Nippi, Tokyo, Japan) that contained 110 μl TRIzol Reagent. After the tissue was disrupted in BioMasher II, extraction was performed with a DNase treatment using a Direct-zol RNA MicroPrep (Zymo Research, Irvine, CA, United States). We performed a quality check (RIN, quantity, purity ratio) for the total RNA by utilizing TapeStation 2200 RNA Screen Tape (Agilent Technologies, Santa Clara, CA, United States), Qubit Broad Range or High Sensitivity (BR or HS) RNA assay (Life Technologies, Carlsbad, CA, United States), and NanoDrop 2000 (Thermo Fisher Scientific, Waltham, MA, United States).
Library Preparation and cDNA Sequencing
Illumina sequence libraries were prepared with the extracted total RNA using the NEBNext Ultra RNA Library Prep Kit for Illumina (New England BioLabs, Ipswich, MA, United States) or KAPA mRNA HyperPrep Kit (KAPA BIOSYSTEMS, Durham, NC, United States). Libraries that contained the whole body and abdomen were prepared using the NEBNext Ultra RNA Library Prep Kit for Illumina. The mRNAs of the whole body and abdomen were isolated by NEBNext Oligo d(T)25 beads (New England BioLabs, Ipswich, MA, United States) from 100–200 ng of total RNA. ds cDNA was synthesized from the isolated mRNA using ProtoScript II Reverse Transcriptase and NEBNext Second Strand Synthesis Enzyme Mix (New England BioLabs, Ipswich, MA, United States). The synthesized cDNAs were end-repaired by NEBNext End Prep Enzyme Mix and added to NEBNext Adapter for Illumina (New England BioLabs, Ipswich, MA, United States). The addition adapter to cDNA and amplification were achieved through PCR (16 cycles).
The mRNAs of ant fat bodies were isolated by mRNA Capture Beads (KAPA BIOSYSTEMS, Durham, NC, United States) from 100–200 ng of total RNA. ds cDNA was synthesized from the isolated mRNA using KAPA Script and 2nd Strand Synthesis and A-Tailing Enzyme Mix (KAPA BIOSYSTEMS, Durham, NC, United States). The synthesized cDNAs were end-repaired by 2nd Strand Synthesis and A-Tailing Enzyme Mix and Adapter Ligation Master Mix (KAPA BIOSYSTEMS, Durham, NC, United States). The adapter addition to cDNA and amplification were achieved through PCR (16–18 cycles).
The cDNA libraries were sequenced by the NextSeq 500 (Illumina, San Diego, CA, United States) in pairs or single-ends with 150 or 75 cycles of the NextSeq 500/550 High Output Kit v2.0 (Illumina, San Diego, CA, United States). We evaluated the accuracy of sequence reads by FastQC v0.11.93.
De novo Transcriptome Assembly and Gene Prediction
The de novo assembly of the transcriptome sequence reads was performed with Trinity v2.8.5 (Grabherr et al., 2011). We performed a quality check of the constructed transcriptome assembly by evaluating BUSCO v2/v3 (reference gene set: Arthropoda) using gVolante v1.2.1 (Nishimura et al., 2017). We used Augustus v3.2.2 to predict the gene regions that were present on the transcriptome assembly using the Camponotus floridanus gene model, a closely related species (Stanke and Morgenstern, 2005). CD-HIT-EST (Huang et al., 2010) removed the predicted gene regions with overlapping sequences in the cut-off value of 0.9.
Gene Annotation and Expression Analysis
We annotated CHC synthesis-related genes in the transcriptome assembly of P. lamellidens with predicted coding regions. The CHC synthesis-related genes (fatty acid synthase, desaturase, elongase, cytochrome P450 decarbonylase) were annotated using the Drosophila melanogaster genes that were registered in UniProt as a query (Q9VQL6_DROME, M9PB21_DROME, ELOF_DROME, Q7K4Y0_DROME, Q9VG68_DROME, A7DZ97_DROME, and CP4G1_DROME). After searching for candidate genes in the transcriptome assembly of P. lamellidens using BLAST similarity search (E-value < 1E-30; Camacho et al., 2009), the obtained candidate genes and query sequences were subjected to a protein domain search using HMMER v3.1b2 (Eddy, 2011) with the Pfam-A database (E-value ≤ 1E-10). The domain structure was confirmed using DoMosaics v0.95 (Moore et al., 2014). Gene expression was quantified as transcripts per million (TPM) using Kallisto v0.43.0 (Bray et al., 2016). The genes that possessed a domain structure similar to that of D. melanogaster and exhibited a TPM value of 10 or higher in any sample were considered to be CHC synthesis-related genes of P. lamellidens. We used the R package Edge R v3.18.1 with an FDR < 5% for searching differentially expressed genes (DEGs) (Robinson et al., 2010). We also annotated DEGs by a tBLASTn similarity search using the nr database.
RT–qPCR
We synthesized cDNA from 100 ng of total RNA extracted from the fat body in the abdomens using SuperScript III Reverse Transcriptase (Invitrogen, Waltham, MA, United States). qPCR was performed using a KAPA SYBR Fast qPCR Kit (KAPA BIOSYSTEMS, Durham, NC, United States) and LightCycler 96 (Roche, Basel, Switzerland). The primer list is provided in Supplementary Table 1. Preliminary tests confirmed that the amplification efficiency of all primers was similar (1.8 ∼ 2.2). We used the housekeeping genes Gapdh1 and Actin-5C as reference genes. The amplicon lengths of all genes were between 143–163 bp. We calculated the relative expression level of target genes by the E-Method using LightCycler 96 Application software (Roche, Basel, Switzerland).
Protein Expression Analysis
The fat body from a newly mated queen of P. lamellidens was dissected in ant saline. The tissue was crushed in lysis buffer (12 mM sodium deoxycholate, 12 mM sodium N-dodecanoylsarcosinate, and 50 mM ammonium bicarbonate containing 1% protease inhibitor cocktail for general use [Nacalai Tesque, Kyoto, Japan]) followed by sonication for 20 min. The lysate (20 μg protein/50 μl) was reacted with 0.5 μl of 1 M dithiothreitol at 37°C for 30 min followed by 2.5 μl of 1 M iodoacetamide at 37°C for 30 min in the dark. After adding 200 μl of 50 mM ammonium bicarbonate, the sample was digested using 0.6 μg of Lys-C (Wako Pure Chemical Industries, Tokyo, Japan) at 37°C for 3 h followed and 0.5 μg of trypsin (Promega, Madison, WI, United States) at 37°C for 16 h. The digest was acidified with trifluoroacetic acid and sonicated for 10 min. The supernatant was desalted using C18-StageTips (Rappsilber et al., 2003) and dried under reduced pressure.
To perform quantitative analysis of key proteins using LC-MS analysis, a system equipped with a nanoElute and a timsTOFPro (Bruker Daltonics, Bremen, Germany) was used. The digests that were dissolved in 0.1% formic acid 2% acetonitrile (0.4 μg/μl) were injected into a spray needle column (ACQUITY UPLC BEH C18, 1.7 μm, ID = 75 μm, length = 25 cm) and were separated by gradient analysis. Mixtures of (A) formic acid/water (0.1/100, v/v) and (B) formic acid/acetonitrile (0.1/100, v/v) were used as the mobile phase. The composition of the mobile phase (B) was changed to 2–35% in 100 min and 35–80% in 10 min while maintaining a flow rate of 280 nl/min at 60°C.
Tandem MS was performed using a parallel accumulation serial fragmentation (PASEF) scan mode (Meier et al., 2018). Briefly, the peptides were ionized to positive ion at 1,600 V. Trapped ion mobility spectrometry scanning was performed at a 1/K0 range of 0.7–1.2 Vs/cm2 with a ramp time of 100 ms while maintaining the duty cycle at 100%. MS scanning was performed at a mass range of m/z 300–1,200 followed by 10 PASEF-tandem MS scans per cycle (precursor ion charge = 0–5, intensity threshold = 500, target intensity = 2,000, isolation width = 2 Th at m/z 700 and 3 Th at m/z 800, collision energy = 20 eV at 0.6 Vs/cm2, 59 eV at 1.6 Vs/cm2). The raw data and the associated files were then deposited in the ProteomeXchange Consortium via the jPOST partner repository (accession number: JPST001552) (Okuda et al., 2017).
Protein identification and quantitative analysis of CHC synthesis-related proteins were performed using FragPipe v15.0 containing MSFragger v3.2 and Philosopher v3.4.13 (Kong et al., 2017). The protein sequence database was generated from transcriptome assembly, and the decoy reversed sequences were added. We also used the cRAP database4 to detect contaminant proteins.
The mass tolerance of the precursor and the fragment ions was set to 20 ppm and 0.05 Da, respectively. Mass calibration and parameter optimization were performed using the Philosopher algorithm (da Veiga Leprevost et al., 2020). The enzyme was set to trypsin as a specific cleavage, and up to two missed cleavages were allowed in the proteolysis. The allowed peptide lengths and mass ranges were 7–50 residues and 500–5,000 Da, respectively. Carbamidomethylation was set as a fixed modification at the cysteine residue, whereas N-acetylation at the protein N-term and oxidation at the methionine residue were set as variable modifications, and this allowed for up to three sites per peptide. The peptide spectrum matches and the identified peptides/proteins were determined at <1% FDR at the protein level.
Quantitative analysis of each peptide was performed with matches between runs (m/z tolerance = 20 ppm, retention time tolerance = 3 min, ion mobility tolerance = 0.05 Vs/cm2). After removing outlier values for the identified peptides, the intensities of the identified peptide in the data were normalized (the median log2 values were unified). The MaxLFQ algorithm (Cox et al., 2014) was used to compare the levels of protein expression between runs using the normalized intensity values of identified unique peptides.
Results
Induction of Rubbing Behavior Under Laboratory Conditions
To perform quantitative assessments, first, the rubbing behavior of a newly mated P. lamellidens queen (the new queen) was re-enacted in a laboratory environment (Figure 1 and Supplementary Movie 1). The rubbing behavior experiment was carried out in a plaster-lined plastic case (5.0 cm × 4.5 cm × 2.5 cm), and the new queen interacted with the host workers in the case. To reduce the risk of counterattack from the host workers, the host workers were anesthetized before being placed with the new queen (the detailed procedures are described in the “Materials and Methods” section). The rubbing behavior observed in the field is characterized by the queens straddling the host worker and rubbing it with her legs (Figure 1A; Yano, 1911; Kohriba, 1963, 1966; Kubota, 1974; Sakai, 1990, 1996, 2000), and we succeeded in observing the same behavior in the laboratory environment. In addition, this rubbing behavior of the new queen was similar for both known host workers, C. japonicus (Kohriba, 1963; Japanese Ant Database Group, 2003) and C. obscuripes (Iwai et al., 2021) (Figures 1B,C and Supplementary Movie 1).
The Effect of Rubbing Behaviors on Host Workers
A behavioral test was performed to examine how rubbing behaviors changed the nestmate discrimination displayed by host workers. The behavioral test measured the actions of host workers toward the new P. lamellidens queen after 3 days of rubbing behavior was observed on the host worker. At this time, the rubbing behavior was performed on the worker from the same colony as that of the workers used for the behavioral test. When the newly mated P. lamellidens did not perform rubbing behaviors, C. japonicus workers recognized P. lamellidens as a non-nestmate and a high frequency of aggressive behavior was observed (Figure 2A). On the other hand, C. japonicus workers did not attack the new queen that had exhibited rubbing behavior (Figure 2A). This finding suggests that the rubbing behavior enabled the newly mated queen of P. lamellidens to deceive the system of host nestmate recognition.
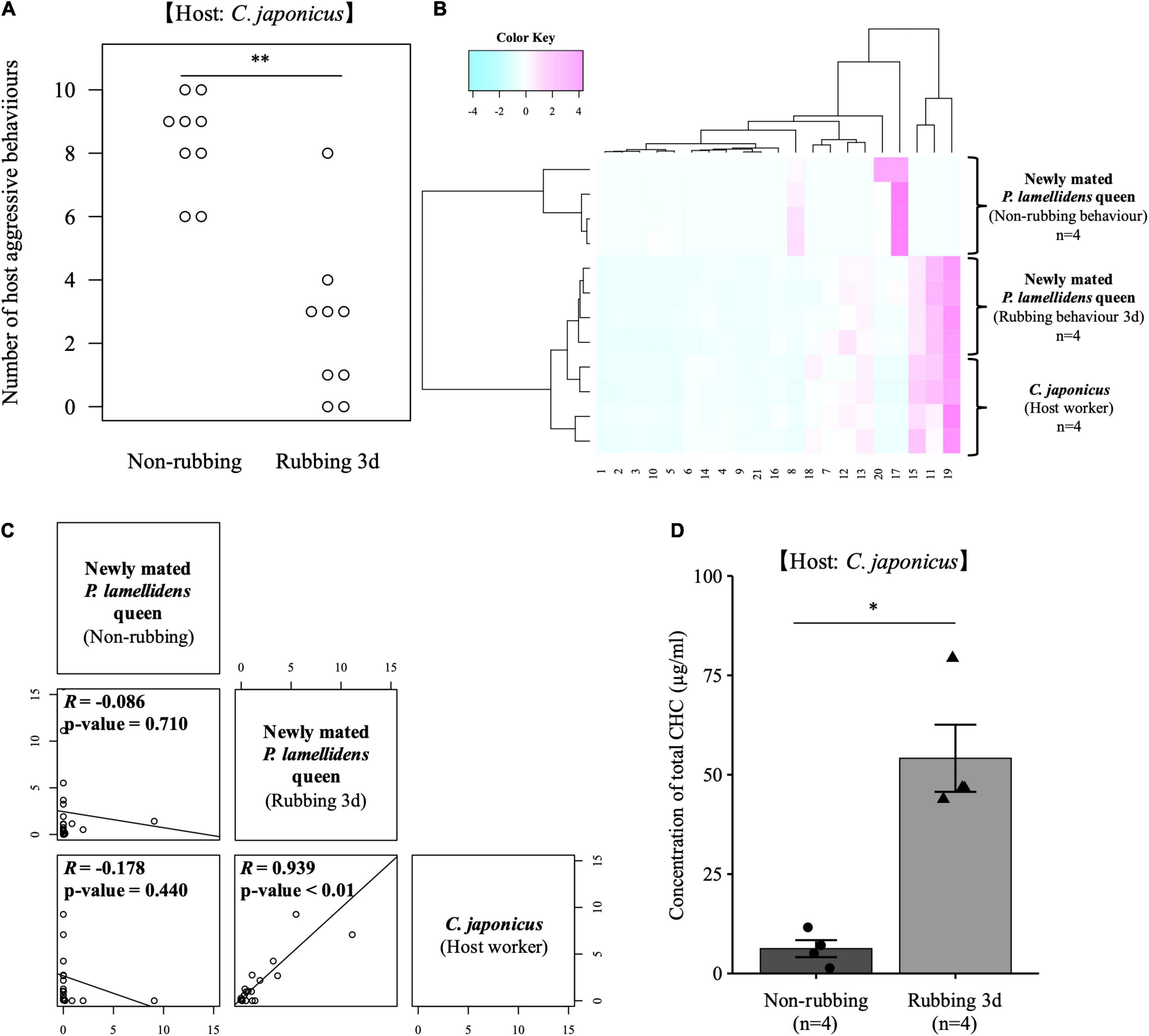
Figure 2. The CHC profile and effect of rubbing behavior on the host worker reaction. We used C. japonicus as a host worker in these experiments. (A) The number of host aggressive behaviors of host workers against newly mated P. lamellidens queens. These host workers were prepared with nine biological replicates, and they belonged to the same colony as that used in the rubbing behavior experiments but with different individuals. Ten actions were observed against the newly mated P. lamellidens queen, and among these actions, “threaten,” “rush,” and “bite” were used to count the number of host aggressive behaviors. Wilcoxon signed rank test, **; significant difference: p-value < 0.01 (the actual value: 0.003). (B) Hierarchical clustering analysis of CHC profiles of newly mated P. lamellidens queens and host workers. This analysis was performed using the standardized value of the peak area that was converted to a Z score. The x-axis indicates the type of estimated hydrocarbons (see Supplementary Table 2). (C) Correlation analysis of CHC profiles of newly mated P. lamellidens queens and host workers. The correlation coefficient was calculated by the Pearson method. R indicates the correlation coefficient. Each plot shows the detected cuticular hydrocarbons. The x-axis and the y-axis show the area values of the standardized average peak area value. (D) The concentration of CHC in newly mated P. lamellidens queens. Mann–Whitney U test, *; significant difference: p-value < 0.05 (the actual value: 0.028), n = 4. Error bar indicates standard error.
To determine how the rubbing behavior altered the response of the host workers, a GC-MS analysis was conducted. The mass spectrometer measured the cuticular hydrocarbons (CHCs) on the body surface of new queens that had or had not exhibited the rubbing behavior, as well as the host workers that were used as the rubbing target. As a result, the CHC profile of the newly mated P. lamellidens queens that had exhibited rubbing behavior had clearly changed to one that closely resembling the host worker profile (Figures 2B,C, Supplementary Figure 1, and Supplementary Table 2, R > 0.7, p-value < 0.01). Interestingly, the total amount of CHCs of the new queen increased due to the rubbing behavior (Figure 2D). The profiles were also confirmed to change even when the target of rubbing behavior was C. obscuripes (Supplementary Figures 2, 3 and Supplementary Table 3). These results suggest that the new queen can disguise her CHC profile and amount of CHCs by rubbing regardless of the host species to avoid host worker aggression.
Tracing Assay With Labeled Cuticular Hydrocarbons
The MS and behavioral tests demonstrated that the rubbing behavior enables the host CHC profile to be disguised. However, whether this chemical disguise is implemented by chemical camouflage or chemical mimicry is unclear. Here, we focused on the result that the total amount of CHCs in the new queen was increased by the rubbing behavior (Figure 2D). Therefore, whether this chemical disguise is implemented by direct deprivation from the body surface of the host worker (chemical camouflage) was verified by a tracing assay in which substances labeled with stable isotopes were applied. The tracking experiments were conducted using two types of hydrocarbons (n-triacontane-d62: C30D62, n-dotriacontane-d66: C32D66), in which the hydrogens of hydrocarbons with a chain length similar to those possessed by the host worker (C. japonicus; Supplementary Table 2) were replaced by stable isotopes. The efficiency of applying labeled substances to the ant body was confirmed with GC-MS measurements, and the labeled substances accounted for approximately 10–20% of the total CHC in the host worker (Supplementary Figure 4A). A similar ratio was also confirmed in the newly mated P. lamellidens queens, which exhibited rubbing behaviors (Supplementary Figure 4B). A new queen could perform rubbing behavior on the labeled host worker for 3 days. By rubbing, the newly mated P. lamellidens queens acquired both the host CHC and the labeled substances on their cuticle from these host workers (Figures 3A–C and Supplementary Figures 4B, 5). In addition, since postpharyngeal glands are involved in the storage of hydrocarbons in ants (Soroker et al., 1994, 1995), we analyzed the hydrocarbon content in the postpharyngeal glands of the newly mated P. lamellidens queens. Host CHC and labeled substances were also detected in the postpharyngeal glands of newly mated P. lamellidens queens (Figure 3D and Supplementary Figure 6). These results suggest that the new queen directly obtains the host CHC through rubbing behavior.
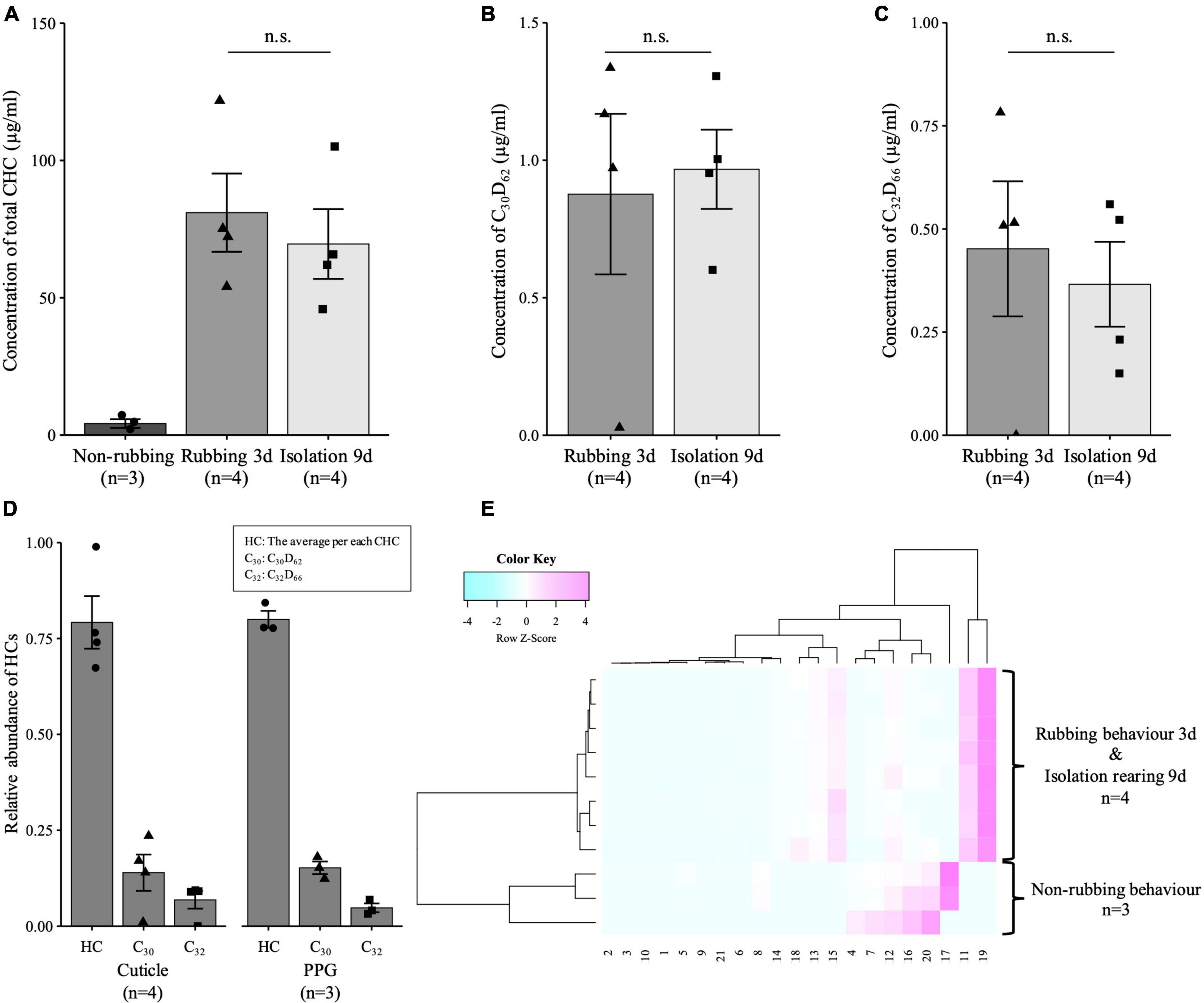
Figure 3. Comparison of CHC and labeled substances in the newly mated P. lamellidens queens. These experiments used C. japonicus as a host worker (A–E). (A) The concentration of CHC in the newly mated P. lamellidens queens [Mann–Whitney U test, n.s., non-significant difference (the actual value: 0.485), n = 4]. (B,C) The concentration of labeled substances (n-triacontane-d62: C30D62, n-dotriacontane-d66: C32D66) in newly mated P. lamellidens queens [Mann–Whitney U test, n.s., non-significant difference (the actual value: 0.885–1.000), n = 4]. (D) The ratio of hydrocarbons (the average per CHC, C30D62, C32D66) on the newly mated P. lamellidens queen cuticle and postpharyngeal glands (PPG) after rubbing behavior. The average ratios of hydrocarbons identified in the cuticle and postpharyngeal glands were correlated [Pearson method, R = 1, p-value < 0.01 (the actual value: 0.000)]. (E) Hierarchical clustering analysis of the CHCs of newly mated P. lamellidens queens. This analysis was performed using the peak area value that was converted to a Z score. The number on the X-axis indicates the type of estimated hydrocarbon (see Supplementary Table 2). All error bars indicate the standard error.
To observe how long the new queen can retain the acquired CHC, including the labeled substances, the new queens were isolated for up to 9 days after rubbing behavior was observed against the labeled host worker, and the amount of CHC and CHC profile were measured. The newly mated P. lamellidens queen maintained the disguised CHC profile for at least 9 days of isolation (Figure 3E and Supplementary Figures 7A,B). Furthermore, the quantity of disguised CHC and labeled substances did not significantly increase or decrease during the 9 days (Figures 3A–C and Supplementary Figure 7C).
Gene and Protein Expression Profiling
The tracing assay with labeled CHC suggested that the chemical disguise of P. lamellidens may be based on a “chemical camouflage” method. We next examined another disguising strategy, “chemical mimicry.” Since chemical mimicry is a biosynthesis-mediated strategy, expression profiling was performed. A reference transcriptome of P. lamellidens was established by extracting the total RNA from the whole body of the larvae, worker, queen, abdomen of the newly mated queen, and fat body of the newly mated queen. cDNA was synthesized from the obtained total RNA and sequenced by an Illumina sequencer. Paired-end and single-end sequencing of 75–150 bp produced a total of 210 M reads (Supplementary Table 4). We assembled these reads using Trinity v2.8.5 and constructed the reference assembly of 23,523 coding regions with a BUSCO completeness of 96.6% (Supplementary Table 5).
The expression levels of the newly mated queen were analyzed by RNA-seq with the P. lamellidens reference assembly, and these analyses were performed for queens without rubbing behavior (non-rubbing behavior) and queens with rubbing behavior to the host workers for 3 days (rubbing 3d), or queens that were isolated from the hosts for 9 days after rubbing behavior was observed (isolation 9d). There are five CHC synthesis-related genes (Figure 4A), and these were expressed on the oenocytes that are contained in the abdomen fat body (Thiele and Camargo-Mathias, 2003; Roma et al., 2006, 2008; Martins and Ramalho-Ortigao, 2012; Koto et al., 2019). Fatty acid synthase is involved in the biosynthesis of CHC precursors, desaturase and elongase in the construction of CHC varieties (it is involved in extending the chain length and inserting double bonds), and fatty acyl-CoA reductase and cytochrome P450 decarbonylase are involved in the final modification of CHC. The expression level of most of the genes that are related to CHC synthesis did not change among the non-rubbing, rubbing 3d, and isolation 9d groups, except for desaturase (Desat) (Figure 4 and Supplementary Tables 6–8). Desaturase (Desat) (g34819.t1), a CHC synthesis-related gene, was changed at the mRNA level but not at the protein level (Figures 4B,C, Supplementary Figures 8A,B, and Supplementary Tables 7, 8). The expression level of cytochrome P450 decarbonylase (Cyp4g1), which is specifically expressed in the oenocyte (Koto et al., 2019), is particularly known to directly correlate with the amount of synthesized CHC. In this study, cytochrome P450 decarbonylase (Cyp4g1) (g28547.t1) was not detected as a differentially expressed gene (DEG) by transcriptome analysis (Figures 4D–G, Supplementary Figures 8C,D, and Supplementary Tables 7, 8). In addition, the expression pattern of cytochrome P450 decarbonylase (Cyp4g1) was examined by RT–qPCR analysis (Supplementary Figure 8D) and a quantitative analysis of key proteins using LC-MS analysis (Figure 4E). These analyses also demonstrated that the expression level of Cyp4g1 was not changed significantly in mRNA and protein level.
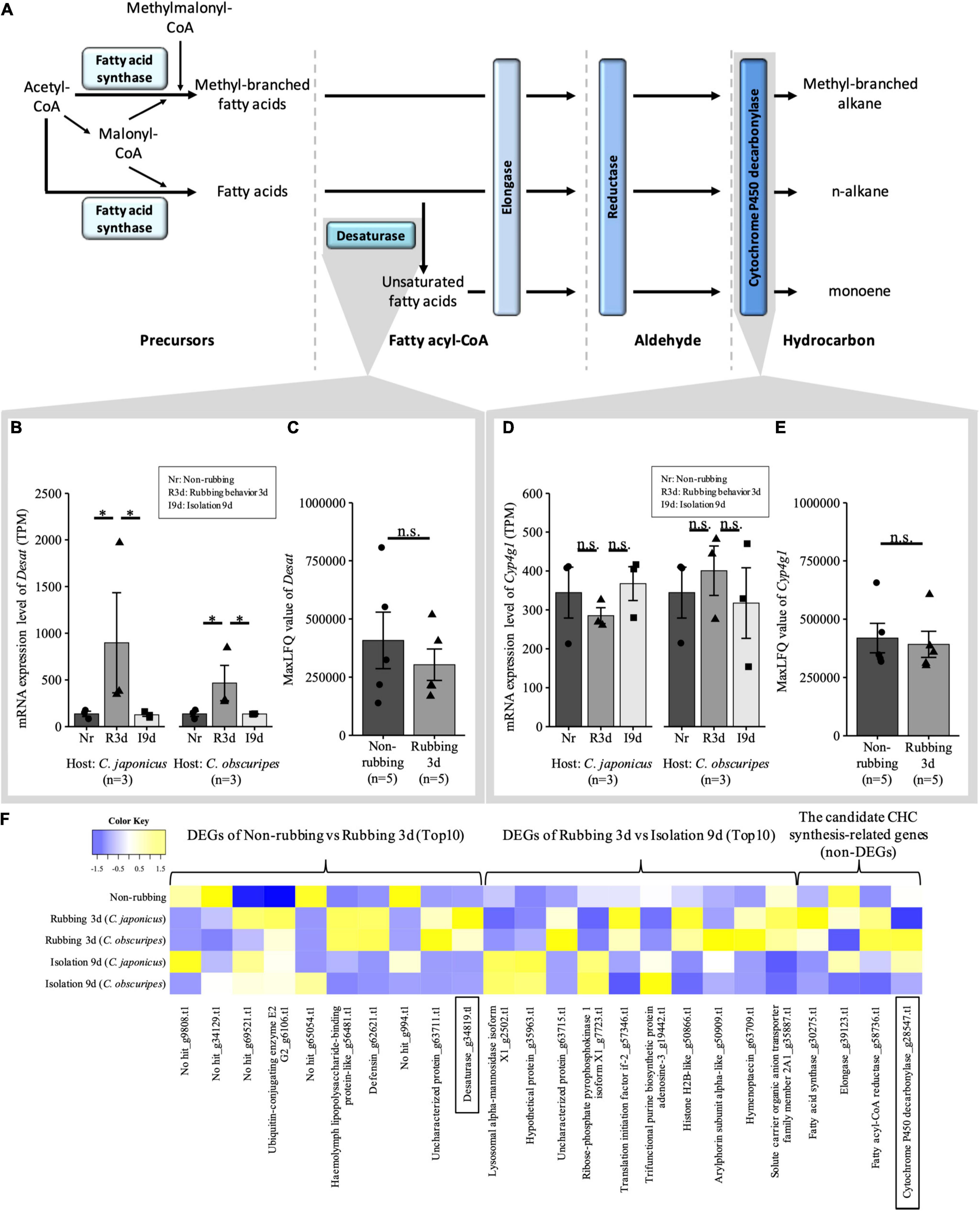
Figure 4. The expression levels of CHC synthesis-related genes in the whole abdomen. (A) The synthesis pathway of CHC (modified from Chung and Carroll, 2015). (B,D) The expression levels of desaturase (Desat): g34819.t1 in mRNA from the whole abdomen by transcriptome (B) and protein from fat body by quantitative analysis of key proteins using LC-MS analysis (C). The expression levels of cytochrome P450 decarbonylase (Cyp4g1): g28547.t1 in mRNA from the whole abdomen by transcriptome (D) and protein from fat body by quantitative analysis of key proteins using LC-MS analysis (E). In mRNA; *, significant difference: FDR < 0.05 (the actual value: 0.000–0.008); n.s., non-significant difference (the actual value: 1.000), n = 3. In protein, one-way ANOVA; n.s., non-significant difference (the actual value: 0.473–0.758), n = 5. (F) The heatmap of expression levels of the top ten differentially expressed genes (DEGs) and the top-hit candidate CHC synthesis-related genes were commonly observed regardless of the host species. This analysis was performed using the TPM that was converted to a Z score. The name of genes referred to in (B–E) are enclosed in squares.
Discussion
Rubbing behavior was shown to disguise the host-like CHC profile and prevent the aggression of hosts, and this was demonstrated through replicating rubbing behavior in the laboratory, testing the nestmate-recognition behavior by hosts, and performing GC-MS analysis with the newly mated P. lamellidens queen against its host worker. In addition, the newly mated P. lamellidens queens acquired and maintained artificially-applied labeling substances from the host workers by performing rubbing behavior, while gene expression profiling showed no change in the expression of cytochrome P450 decarbonylase (Cyp4g1), which is a CHC synthesis-related factor, during rubbing behavior. These results suggest that the newly mated P. lamellidens queens directly acquire the hosts’ CHC through rubbing behavior and deceive the technique of nestmate recognition by hosts through employing chemical disguises.
In a previous rearing study, a reduction in aggressive behavior was observed in host workers toward the newly mated P. lamellidens queen after rubbing behavior was performed (Kohriba, 1963). Our results demonstrate that the effect is due to chemical disguise (Figure 2) and quantitatively indicate that versatility promotes this disguise in both host species (C. japonicus and C. obscuripes), which exhibit different CHC profiles (Figure 2, Supplementary Figures 1–3, and Supplementary Tables 2, 3). In addition, we revealed that the newly mated P. lamellidens queens possessed only a minute amount of CHCs before the rubbing behavior (Figure 2D and Supplementary Tables 2, 3), and this quantity was significantly increased after the rubbing behavior for C. japonicus (Figure 2D). Obtaining a new CHC is natural and simple when the baseline is limited, and this observation also supports the idea of chemical camouflage (acquirement of CHC). Since a low base quantity of CHC has been observed in other social parasitic ants, we expect it to be one of their general strategies (d’Ettorre and Errard, 1998; Johnson et al., 2001; Martin et al., 2007; Tsuneoka and Akino, 2012). The tracing assay with the labeled substances revealed that the newly mated P. lamellidens queen acquires labeled substances from its host workers by performing rubbing behaviors (Figures 3B–D and Supplementary Figures 4B, 5, 6). This result also confirmed that the newly mated P. lamellidens queens directly obtained host CHCs through rubbing behaviors. Previous studies reported that ants exchange CHC through performing trophallaxis and grooming with nestmates, and through these behaviors, the CHC profile becomes standardized within the colony (Soroker et al., 1994, 1995; Dahbi et al., 1999). The newly mated P. lamellidens queen also grooms the host while performing rubbing behaviors, and the queen probably acquires the CHC of hosts during this process (Kubota, 1974).
Isolation rearing revealed that the newly mated queen, which was isolated from its hosts for up to 9 days, could maintain the disguised CHC profile (Figures 3A–C,E and Supplementary Figure 7). This result suggests that the newly mated queen could maintain the amount and profile of the CHC that was acquired from the host. It is known that ants store CHC in the postpharyngeal glands in their heads (Soroker et al., 1994, 1995). We also confirmed that the postpharyngeal glands of newly mated P. lamellidens queens contain host CHC and labeled substances from its labeled host workers after performing rubbing behaviors (Figure 3D and Supplementary Figure 6). The hydrocarbon profile found in the postpharyngeal glands was similar to that of the host CHC, and the labeled substances applied to the host were also detected in the postpharyngeal glands, suggesting that the newly mated P. lamellidens queen also supplies the acquired host CHC to its postpharyngeal glands. Most likely, the newly mated queen enables the disguised CHC profile to be maintained by storing the acquired CHC in the postpharyngeal glands.
Expression analysis was performed to verify the chemical mimicry, and except for the mRNA of desaturase (Desat) (but not the protein level), no changes were observed in the expression of genes in the pathways related to CHC synthesis before and after rubbing behavior (Figure 4, Supplementary Figure 8, and Supplementary Tables 6–8). This result suggests that the biosynthesis of CHC, i.e., chemical mimicry, does not occur in the newly mated P. lamellidens queens. Among the CHC synthesis-related genes, the expression of cytochrome P450 decarbonylase (Cyp4g1) (Koto et al., 2019), which is responsible for the final modification of CHC precursors and has an expressional level that correlates with the amount of CHC synthesized, was not detected at the mRNA or protein level (Figures 4D–F, Supplementary Figures 8C,D, and Supplementary Tables 6–8). Since the expression of cytochrome P450 decarbonylase (Cyp4g1) did not change despite the apparent increase in the amount of CHC after rubbing behavior, we expected that no new biosynthesis of CHC would occur, at least during the rubbing behavior. The expression level of Desaturase (Desat), which is involved in the construction of CHC variants (Chung and Carroll, 2015), changed at the mRNA level but not at the protein level (Figures 4B,C, Supplementary Figures 8A,B, and Supplementary Tables 6–8). Based on the above observations, we expected that the newly mated P. lamellidens queen would achieve chemical disguise by performing chemical camouflage (acquiring CHC) rather than chemical mimicry (CHC biosynthesis) through rubbing behavior.
In general, socially parasitic ants and their host are considered to be phylogenetically closely related, which is known as Emery’s rule (Emery, 1909; Hölldobler and Wilson, 1990). The reason why the application of Emery’s rule to some socially parasitic ants is thought to be because it is easy for the parasitic ants to carry out the chemical disguise (Blatrix and Sermage, 2005; Huang and Dornhaus, 2008; de la Mora et al., 2020). However, some parasitic species deviate from this rule, such as Polyrhachis lama and Polyrhachis loweryi, which host distantly related Diacamma sp. and Rhytidoponera sp (Formicidae: Ponerinae) at the subfamily level (Maschwitz et al., 2000, 2003, 2004; Witte et al., 2009). P. lama is estimated as avoiding the nestmate discrimination of its host by other means (e.g., to use the appeasement substance) without CHC disguise, perhaps because it is phylogenetically quite distant from its host (Witte et al., 2009). P. lamellidens also allows the phylogenetically distant species (C. japonicus) as its host (although they belong to the same clade at the subfamily level), and such socially parasitic ants are expected to have difficulty avoiding the nestmate discrimination of their host without experiencing some special event. The acquisition of host CHC by rubbing behavior, as performed by the newly mated P. lamellidens queen, may be an example. The shampoo ant (Formicoxenus sp.), which allows its host species of a different genus but the same at the subfamily level (Ojeda et al., 2021), is also presumed to acquire the host CHC through the unique behavior similar to the rubbing behavior of P. lamellidens (Lenoir et al., 1997). As in the above examples of Polyrhachis species, how the nestmate discrimination of the host ants is deceived may vary according to the phylogenetic distance between parasite and host. This question could be answered by examining the chemical disguise and its mechanism (chemical camouflage and/or chemical mimicry) through research approaches such as ours (e.g., CHC analysis, tracing assay of the labeled substances, and the gene expression analysis) against several parasitic ants which follow or do not follow the Emery’s rule.
We used a combination of experimental techniques to reveal the chemical disguise strategy of the newly mated P. lamellidens queen in the early stages of social parasitism. In addition, we believe that this study succeeded in elucidating some of the previously obscure mechanisms that are involved in the chemical disguise of socially parasitic ants.
Data Availability Statement
The data of transcriptome analysis presented in this study are deposited in the NCBI repository, accession number PRJNA821370 (BioProject). The data of LC-MS analysis presented in the study are deposited in the JPOST repository, accession number JPST001552. The transcriptome assembly presented in the study are deposited in the NCBI repository, accession number GJVV01000000 (the sequences less than 200 bp in registered assembly were removed). Supplementary Movie 1 can be found at https://figshare.com/articles/media/Supplementary_Movie_1_mp4/19533670/1.
Author Contributions
HI and NK designed the project. HI collected ant specimens, performed transcriptome analysis, and wrote first draft of the manuscript. HI and MM conducted quantitative analysis of key proteins using LC-MS analysis. NK managed the experimental environment. MT and KA managed the resources. All authors commented on previous versions of the manuscript.
Funding
JSPS Fellows (202021677), a Taikichiro Mori Memorial Research Grant, a Nakatsuji Foresight Foundation Research Grant, KAKENHI Grant-in-Aid for Scientific Research (B) (21H02210), and Yamagata Prefecture, and Tsuruoka City.
Conflict of Interest
The authors declare that the research was conducted in the absence of any commercial or financial relationships that could be construed as a potential conflict of interest.
Publisher’s Note
All claims expressed in this article are solely those of the authors and do not necessarily represent those of their affiliated organizations, or those of the publisher, the editors and the reviewers. Any product that may be evaluated in this article, or claim that may be made by its manufacturer, is not guaranteed or endorsed by the publisher.
Acknowledgments
We would like to thank Toshiharu Akino for providing extraction method of CHC; Masataka Wakayama, Noriko Fukuda, and Noriko Kagata for the supporting GC-MS measurement; and Yuki Takai for technical support in RNA sequencing. We would also like to thank Daiki D. Horikawa, Koto Akiko, Keizo Takasuka, Takahiro Masuda, Konosuke Ii, Yu Kurihara, Sora Ishikawa, Phillip Yamamoto, Tomoki Takeda, and Masaru Hojo for their critical suggestions.
Supplementary Material
The Supplementary Material for this article can be found online at: https://www.frontiersin.org/articles/10.3389/fevo.2022.915517/full#supplementary-material
Footnotes
- ^ http://www.R-project.org/
- ^ https://cran.r-project.org/web/packages/gplots/
- ^ http://www.bioinformatics.babraham.ac.uk/projects/fastqc/
- ^ https://www.thegpm.org/crap/
References
Akino, T. (2008). Chemical strategies to deal with ants: a review of mimicry, camouflage, propaganda, and phytomimesis by ants (Hymenoptera: Formicidae) and other arthropods. Myrmecol. News. 11, 173–181.
Akino, T., and Yamaoka, R. (2012). Sample preparation for analyses of cuticular hydrocarbons as semiochemicals. Jpn. J. Appl. Entomol. Zool. 56, 141–149. doi: 10.1303/jjaez.2012.141
Akino, T., Mochizuki, R., Morimoto, M., and Yamaoka, R. (1996). Chemical camouflage of myrmecophilous cricket Myrmecophilus sp. to be integrated with several ant species. Jpn. J. Appl. Entomol. Zool. 40, 39–46. doi: 10.1303/jjaez.40.39
Bauer, S., Böhm, M., Witte, V., and Foitzik, S. (2010). An ant social parasite in-between two chemical disparate host species. Evol. Ecol. 24, 317–332. doi: 10.1007/s10682-009-9308-2
Blatrix, R., and Sermage, C. (2005). Role of early experience in ant enslavement: a comparative analysis of a host and a non-host species. Front. Zool. 2:13. doi: 10.1186/1742-9994-2-13
Bray, N. L., Pimentel, H., Melsted, P., and Pachter, L. (2016). Near-optimal probabilistic RNA-seq quantification. Nat. Biotechnol. 34, 525–527. doi: 10.1038/nbt.3519
Bolton, B. (2022). An Online Catalog of the Ants of the World. Available online at: http://antcat.org (accessed May 18, 2022).
Buschinger, A. (2009). Social parasitism among ants: a review (Hymenoptera: Formicidae). Myrmecol. News. 12, 219–235.
Camacho, C., Coulouris, G., Avagyan, V., Ma, N., Papadopoulos, J., Bealer, K., et al. (2009). BLAST+: architecture and applications. BMC Bioinform. 10:421. doi: 10.1186/1471-2105-10-421
Chung, H., and Carroll, S. B. (2015). Wax, sex and the origin of species: Dual roles of insect cuticular hydrocarbons in adaptation and mating. BioEssays. 37, 822–830. doi: 10.1002/bies.201500014
Cox, J., Hein, M. Y., Luber, C. A., Paron, I., Nagaraj, N., and Mann, M. (2014). Accurate proteome-wide label-free quantification by delayed normalization and maximal peptide ratio extraction, termed MaxLFQ. Mol. Cell. Proteomics. 13, 2513–2526. doi: 10.1074/mcp.M113.031591
da Veiga Leprevost, F., Haynes, S. E., Avtonomov, D. M., Chang, H. Y., Shanmugam, A. K., Mellacheruvu, D., et al. (2020). Philosopher: a versatile toolkit for shotgun proteomics data analysis. Nat. Methods. 17, 869–870. doi: 10.1038/s41592-020-0912-y
Dahbi, A., Hefetz, A., Cerda, X., and Lenoir, A. (1999). Trophallaxis mediates uniformity of colony odor in Cataglyphis iberica ants (Hymenoptera, Formicidae). J. Insect. Behav. 12, 559–567. doi: 10.1023/A:1020975009450
de la Mora, A., Sankovitz, M., and Purcell, J. (2020). Ants (Hymenoptera: Formicidae) as host and intruder: recent advances and future directions in the study of exploitative strategies. Myrmecol. News 30, 53–71. doi: 10.25849/myrmecol.news_030:053
d’Ettorre, P., and Errard, C. (1998). Chemical disguise during colony founding in the dulotic ant Polyergus rufescens Latr.(Hymenoptera, Vespidae). Insect. Soc. Life. 2, 71–77.
d’Ettorre, P., Errard, C., Ibarra, F., Francke, W., and Hefetz, A. (2000). Sneak in or repel your enemy: dufours gland repellent as a strategy for successful usurpation in the slave-maker Polyergus rufescens. Chemoecology 10, 135–142. doi: 10.1007/pl00001815
Detrain, C., Deneubourg, J. L., and Pasteels, J. M. (1999). Information Processing in Social Insects. Bern: Birkhäuser.
Dettner, K., and Liepert, C. (1994). Chemical mimicry and camouflage. Annu. Rev. Entomol. 39, 129–154. doi: 10.1146/annurev.ento.39.1.129
Eddy, S. R. (2011). Accelerated profile HMM searches. PLoS Comput. Biol. 7:e1002195. doi: 10.1371/journal.pcbi.1002195
Emery, C. (1909). Über den ursprung der dulotischen, parasitischen und myrmekophilen ameisen. Biol. Centralbl. 29, 352–362.
Fischer, G., Friedman, N. R., Huang, J. P., Narula, N., Knowles, L. L., Fisher, B. L., et al. (2020). Socially parasitic ants evolve a mosaic of host-matching and parasitic morphological traits. Curr. Biol. 30, 3639–3646. doi: 10.1016/j.cub.2020.06.078
Grabherr, M. G., Haas, B. J., Yassour, M., Levin, J. Z., Thompson, D. A., Amit, I., et al. (2011). Trinity: reconstructing a full-length transcriptome without a genome from RNA-Seq data. Nat. Biotechnol. 29:644. doi: 10.1038/nbt.1883
Hölldobler, B., and Wilson, E. O. (1990). The Ants. Cambridge, CA: The Belknap Press of Harvard University Press.
Hojo, M. K., Wada-Katsumata, A., Akino, T., Yamaguchi, S., Ozaki, M., and Yamaoka, R. (2009). Chemical disguise as particular caste of host ants in the ant inquiline parasite Niphanda fusca (Lepidoptera: Lycaenidae). Proc. R. Soc. B: Biol. Sci. 276, 551–558. doi: 10.1098/rspb.2008.1064
Howard, R. W., and Blomquist, G. J. (2005). Ecological, behavioral, and biochemical aspects of insect hydrocarbons. Annu. Rev. Entomol. 50, 371–393. doi: 10.1146/annurev.ento.50.071803.130359
Howard, R. W., Roger, D. A., and William, B. G. (1990a). Chemical mimicry in an obligate predator of carpenter ants (Hymenoptera: Formicidae). Ann. Entomol. Soc. Am. 83, 607–616. doi: 10.1093/aesa/83.3.607
Howard, R. W., Stanley-Samuelson, D. W., and Akre, R. D. (1990b). Biosynthesis and chemical mimicry of cuticular hydrocarbons from the obligate predator, Microdon albicomatus Novak (Diptera: Syrphidae) and its ant prey, Myrmica incompleta Provancher (Hymenoptera: Formicidae). J. Kans. Entomol. Soc. 63, 437–443.
Huang, M. H., and Dornhaus, A. (2008). A meta-analysis of ant social parasitism: host characteristics of different parasitism types and a test of Emery’s rule. Ecol. Entomol. 33, 589–596.
Huang, Y., Niu, B., Gao, Y., Fu, L., and Li, W. (2010). CD-HIT Suite: a web server for clustering and comparing biological sequences. Bioinformatics 26, 680–682. doi: 10.1093/bioinformatics/btq003
Iwai, H., Kurihara, Y., Kono, N., Tomita, M., and Arakawa, K. (2021). The evidence of temporary social parasitism by Polyrhachis lamellidens (Hymenoptera, Formicidae) in a Camponotus obscuripes colony (Hymenoptera, Formicidae). Insectes. Soc. 68, 375–382. doi: 10.1007/s00040-021-00830-8
Johnson, C. A., Vander Meer, R. K., and Lavine, B. (2001). Changes in the cuticular hydrocarbon profile of the slave-maker ant queen, Polyergus breviceps emery, after killing a Formica host queen (Hymenoptera: Formicidae). J. Chem. Ecol. 27, 1787–1804. doi: 10.1023/A:1010456608626
Kistner, D. H. (1982). The social insects bestiary. Soc. Insects 3, 1–244. doi: 10.1016/b978-0-12-342203-3.50008-4
Kohriba, O. (1963). A parasitic life of Polyrhachis lamellidens F. smith (Hymenoptera, Formicidae), first report. Kontyû. 31, 200–209.
Kohriba, O. (1966). Record of mixed colony of Polyrhachis lamellidens F. Smith and Camponotus japonicus Mayr in the field. Kontyû. 34:316.
Kong, A. T., Leprevost, F. V., Avtonomov, D. M., Mellacheruvu, D., and Nesvizhskii, A. I. (2017). MSFragger: ultrafast and comprehensive peptide identification in mass spectrometry–based proteomics. Nat. Methods. 14, 513–520. doi: 10.1038/nmeth.4256
Kono, N., Nakamura, H., Ito, Y., Tomita, M., and Arakawa, K. (2016). Evaluation of the impact of RNA preservation methods of spiders for de novo transcriptome assembly. Mol. Ecol. Resour. 16, 662–672. doi: 10.1111/1755-0998.12485
Koto, A., Motoyama, N., Tahara, H., McGregor, S., Moriyama, M., Okabe, T., et al. (2019). Oxytocin/vasopressin-like peptide inotocin regulates cuticular hydrocarbon synthesis and water balancing in ants. Proc. Natl. Acad. Sci. U.S.A. 116, 5597–5606. doi: 10.1073/pnas.1817788116
Kurihara, Y., Iwai, H., Kono, N., Tomita, M., and Arakawa, K. (2022). Initial parasitic behaviour of the temporary social parasitic ant Polyrhachis lamellidens can be induced by host-like cuticles in laboratory environment. Biol. Open. 11:bio058956. doi: 10.1242/bio.058956
Lenoir, A., Malosse, C., and Yamaoka, R. (1997). Chemical mimicry between parasitic ants of the genus Formicoxenus and their host Myrmica (hymenoptera. Formicidae). Biochem. Syst. Ecol. 25, 379–389. doi: 10.1016/s0305-1978(97)00025-2
Lenoir, A., d’Ettorre, P., Errard, C., and Hefetz, A. (2001). Chemical ecology and social parasitism in ants. Annu. Rev. Entomol. 46, 573–599. doi: 10.1146/annurev.ento.46.1.573
Martin, S. J., Jenner, E. A., and Drijfhout, F. P. (2007). Chemical deterrent enables a socially parasitic ant to invade multiple hosts. Proc. R. Soc. B: Biol. Sci. 274, 2717–2722. doi: 10.1098/rspb.2007.0795
Martins, G. F., and Ramalho-Ortigao, J. M. (2012). Oenocytes in insects. Invertebr. Surviv. J. 9, 139–152.
Maschwitz, U., Dorow, W. H. O., Buschinger, A., and Kalytta, G. (2000). Social parasitism involving ants of different subfamilies: Polyrhachis lama (Formicinae) an obligatory inquiline of Diacamma sp. (Ponerinae) in Java. Insectes. Soc. 47, 27–35. doi: 10.1007/s000400050005
Maschwitz, U., Go, C., Dorow, W. H. O., Buschinger, A., and Kohout, R. J. (2003). Polyrhachis loweryi (Formicinae): a guest ant parasitizing Rhytidoponera sp. (Ponerinae) in Queensland. Australia. Insectes. Soc. 50, 69–76. doi: 10.1007/s000400300011
Maschwitz, U., Go, C., Kaufmann, E., and Buschinger, A. (2004). A unique strategy of host colony exploitation in a parasitic ant: workers of Polyrhachis lama rear their brood in neighbouring host nests. Naturwissenschaften. 91, 40–43. doi: 10.1007/s00114-003-0487-3
Meier, F., Brunner, A. D., Koch, S., Koch, H., Lubeck, M., Krause, M., et al. (2018). Online parallel accumulation–serial fragmentation (PASEF) with a novel trapped ion mobility mass spectrometer. Mol. Cell. Proteomics. 17, 2534–2545. doi: 10.1074/mcp.TIR118.000900
Moore, A. D., Held, A., Terrapon, N., Weiner, J., and Bornberg-Bauer, E. (2014). DoMosaics: software for domain arrangement visualization and domain-centric analysis of proteins. Bioinformatics. 30, 282–283. doi: 10.1093/bioinformatics/btt640
Mori, A., Dettorre, P., and Moli, F. L. (1995). Host nest usurpation and colony foundation in the European amazon ant. Polyergus rufescens Latr. (Hymenoptera: Formicidae). Insectes. Soc 42, 279–286. doi: 10.1007/bf01240422
Nishimura, O., Hara, Y., and Kuraku, S. (2017). gVolante for standardizing completeness assessment of genome and transcriptome assemblies. Bioinformatics. 33, 3635–3637. doi: 10.1093/bioinformatics/btx445
Nehring, V., Dani, F. R., Calamai, L., Turillazzi, S., Bohn, H., Klass, K. D., et al. (2016). Chemical disguise of myrmecophilous cockroaches and its implications for understanding nestmate recognition mechanisms in leaf-cutting ants. BMC Ecol. 16:35. doi: 10.1186/s12898-016-0089-5
Ojeda, D. I., John, M., Hammond, R. L., Savolainen, R., Vepsäläinen, K., and Kvamme, T. (2021). Phylogeny of the Formicoxenus genus-group (Hymenoptera: Formicidae) reveals isolated lineages of Leptothorax acervorum in the Iberian Peninsula predating the Last Glacial Maximum. bioRxiv [Preprint] doi: 10.1101/2021.11.05.467305
Okuda, S., Watanabe, Y., Moriya, Y., Kawano, S., Yamamoto, T., Matsumoto, M., et al. (2017). jPOSTrepo: an international standard data repository for proteomes. Nucleic. Acids. Res 45, D1107–D1111. doi: 10.1093/nar/gkw1080
Ozaki, M., Wada-Katsumata, A., Fujikawa, K., Iwasaki, M., Yokohari, F., Satoji, Y., et al. (2005). Ant nestmate and non-nestmate discrimination by a chemosensory sensillum. Science. 309, 311–314. doi: 10.1126/science.1105244
R Core Team (2022). R: A language and Environment for Statistical Computing. R software version 4.2.0. [Computer program]. Retrieved from. Available online at: http://www.R-project.org/. (accessed June 7, 2022).
Rappsilber, J., Ishihama, Y., and Mann, M. (2003). Stop and go extraction tips for matrix-assisted laser desorption/ionization, nanoelectrospray, and LC/MS sample pretreatment in proteomics. Anal. Chem. 75, 663–670. doi: 10.1021/ac026117i
Reyment, R. A. (1989). Compositional data analysis. Terra Nova. 1, 29–34. doi: 10.1111/j.1365-3121.1989.tb00322.x
Robinson, M. D., McCarthy, D. J., and Smyth, G. K. (2010). edgeR: a Bioconductor package for differential expression analysis of digital gene expression data. Bioinformatics. 26, 139–140. doi: 10.1093/bioinformatics/btp616
Roma, G. C., Bueno, O. C., and Camargo-Mathias, M. I. (2008). Chemical detection of the proteins and lipids in the fat body cells from workers of Attini ants (Hymenoptera: Formicidae). Cell Biol. Int. 32, 406–416. doi: 10.1016/j.cellbi.2007.12.010
Roma, G. C., Mathias, M. I. C., and Bueno, O. C. (2006). Fat body in some genera of leaf-cutting ants (Hymenoptera: Formicidae). Proteins, lipids and polysaccharides detection. Micron. 37, 234–242. doi: 10.1016/j.micron.2005.10.012
Sakai, H. (1990). Mixed colony of Polyrhachis lamellidens and Camponotus kiusiuensis. Insectarium. 27:31.
Sakai, H. (1996). Living life of Polyrhachis lamellidens - through rearing and field observation -. Insectarium. 33, 232–235.
Scarparo, G., d’Ettorre, P., and Di Giulio, A. (2019). Chemical deception and structural adaptation in Microdon (Diptera. Syrphidae, Microdontinae), a genus of hoverflies parasitic on social insects. J. Chem. Ecol. 45, 959–971. doi: 10.1007/s10886-019-01121-0
Soroker, V., Vienne, C., and Hefetz, A. (1995). Hydrocarbon dynamics within and between nestmates in Cataglyphis niger (Hymenoptera: Formicidae). J. Chem. Ecol. 21, 365–378. doi: 10.1007/bf02036724
Soroker, V., Vienne, C., Hefetz, A., and Nowbahari, E. (1994). The postpharyngeal gland as a “Gestalt” organ for nestmate recognition in the ant Cataglyphis niger. Naturwissenschaften. 81, 510–513. doi: 10.1007/s001140050120
Stanke, M., and Morgenstern, B. (2005). AUGUSTUS: a web server for gene prediction in eukaryotes that allows user-defined constraints. Nucl. Acids. Res 33, W465–W467. doi: 10.1093/nar/gki458
Sturgis, S. J., and Gordon, D. M. (2012). Nestmate recognition in ants (Hymenoptera: Formicidae): a review. Myrmecol. News. 16, 101–110.
Thiele, E., and Camargo-Mathias, M. I. (2003). Morphology, ultramorphology and morphometry of the fat body of virgin females and queens of the ants Pachycondyla striata (Hymenoptera: Formicidae). Sociobiology 42, 243–254.
Tsuneoka, Y. (2008). Host colony usurpation by the queen of the Japanese pirate ant, Polyergus samurai (hymenoptera: formicidae). J. Ethol. 26, 243–247. doi: 10.1007/s10164-007-0055-y
Tsuneoka, Y., and Akino, T. (2009). Repellent effect on host formica workers of queen dufours gland secretion of the obligatory social parasite ant, Polyergus samurai (Hymenoptera: Formicidae). Appl. Entomol. Zool. 44, 133–141. doi: 10.1303/aez.2009.133
Tsuneoka, Y., and Akino, T. (2012). Chemical camouflage of the slave-making ant Polyergus samurai queen in the process of the host colony usurpation (Hymenoptera: Formicidae). Chemoecology 22, 89–99. doi: 10.1007/s00049-012-0101-2
Vander Meer, R. K., and Wojcik, D. P. (1982). Chemical mimicry in the myrmecophilous beetle myrmecaphodius excavaticollis. Science 218, 806–808. doi: 10.1126/science.218.4574.806
von Beeren, C., Hashim, R., and Witte, V. (2012). The social integration of a myrmecophilous spider does not depend exclusively on chemical mimicry. J. Chem. Ecol. 38, 262–271. doi: 10.1007/s10886-012-0083-0
von Beeren, C., Schulz, S., Hashim, R., and Witte, V. (2011). Acquisition of chemical recognition cues facilitates integration into ant societies. BMC Ecol. 11:30. doi: 10.1186/1472-6785-11-30
Warnes, G. R., Bolker, B., Bonebakker, L., Gentleman, R., Liaw, W. H. A., Lumley, T., et al. (2016). gplots: Various R programming tools for plotting data. R package version 3.0. 1. The Comprehensive R Archive Network. [Computer program]. Available online at: https://cran.r-project.org/web/packages/gplots/. (accessed June 7, 2022).
Witte, V., Lehmann, L., Lustig, A., and Maschwitz, U. (2009). Polyrhachis lama, a parasitic ant with an exceptional mode of social integration. Insectes. Soc. 56, 301–307. doi: 10.1007/s00040-009-0024-2
Keywords: myrmecology, social insect, social parasite, chemical disguise, chemical camouflage, chemical mimicry
Citation: Iwai H, Mori M, Tomita M, Kono N and Arakawa K (2022) Molecular Evidence of Chemical Disguise by the Socially Parasitic Spiny Ant Polyrhachis lamellidens (Hymenoptera: Formicidae) When Invading a Host Colony. Front. Ecol. Evol. 10:915517. doi: 10.3389/fevo.2022.915517
Received: 08 April 2022; Accepted: 30 May 2022;
Published: 20 June 2022.
Edited by:
Johannes Stökl, University of Bayreuth, GermanyReviewed by:
Heikki Helanterä, University of Oulu, FinlandJoanne Yew, University of Hawai‘i at Mānoa, United States
Copyright © 2022 Iwai, Mori, Tomita, Kono and Arakawa. This is an open-access article distributed under the terms of the Creative Commons Attribution License (CC BY). The use, distribution or reproduction in other forums is permitted, provided the original author(s) and the copyright owner(s) are credited and that the original publication in this journal is cited, in accordance with accepted academic practice. No use, distribution or reproduction is permitted which does not comply with these terms.
*Correspondence: Nobuaki Kono, Y2ljb25pY2FAc2ZjLmtlaW8uYWMuanA=