- UMR CNRS 6282 BioGéoSciences, Équipe Écologie Évolutive, Université Bourgogne-Franche Comté, Dijon, France
Senescence occurs because of the decline of the strength of selection with age, allowing late-life reduced performances not being counter selected. From there, several phenomena may explain late-life reduced performances, such as the accumulation of deleterious mutations, the expression of pleiotropic genes or the existence of resource trade-offs between early and late performances. This latter phenomenon is at the core of the disposable soma theory of aging, which predicts that growth and early-life reproduction have costs that increase reproductive and actuarial senescence. Whereas the impact of the cost of early reproduction on reproductive and actuarial senescence has been extensively studied, that of the cost of growth remains overlooked and often inconclusive, possibly because of confounding effects associated with the procedures used to manipulate growth rate. Here, we investigated the cost of growth rate and its impact on reproductive senescence and longevity of females of the mealworm beetle, Tenebrio molitor. For this purpose, we generated insects with contrasted growth rates by raising groups of them in conditions below, above and optimal relative humidity (RH: 55, 85 and 70%, respectively) during the larval stage. The resulting adult females then bred, under the same optimal RH conditions, early in life, then later in life and were followed there until death. We found that larvae grown under the highest relative humidity exhibited the highest larval growth rate, thanks to both shorter growth duration and the achievement of heavier pupae mass. Adult females from this favorable growing condition lived longer, were more fecund early in life, but suffered from lower late-life reproductive investment. Our study shows that growth rate, which is highly dependent on the early-life environment, is an important factor modulating adult reproductive senescence, through the occurrence of early-late life trade-offs.
Introduction
Senescence (or aging) is a quasi-universal phenomenon in multicellular organisms, defined by the age-related decrease of life-history features related to individual fitness, mainly survival and reproduction. This phenomenon is nevertheless variable both within and among species (Jones et al., 2014; Flatt and Partridge, 2018). Evolution of aging occurs because the strength of age-specific selection, which is maximal during pre-reproductive development, declines after sexual maturation with advancing adult age (Medawar, 1952; Williams, 1957; Hamilton, 1966; Moorad et al., 2019). Although this decrease of selection with age and the supposed accompanying aging is not an universal rule (Baudisch, 2005; Jones et al., 2014; Wensink et al., 2017), it seems accurate for organisms with finite growth, an age structure and well-defined somatic and germline lines (Charlesworth, 2000). In this context, the antagonistic pleiotropy theory of aging proposes that the expression of genes having early-life beneficial effects will be selected even if they cause deleterious effects later in life (Williams, 1957). Such a trade-off between early and late-life performances is also central to the disposable soma theory of aging (Kirkwood, 1977), which may represent a physiological explanation of the antagonistic pleiotropy theory (Lemaître et al., 2015; Maklakov and Chapman, 2019). The disposable soma theory of aging predicts that, as resource demands for growth or reproduction are high, organisms have reduced options to maintain their own body condition, thereby increasing senescence and reducing future fertility and survival through physiological costs incurred. Therefore, individuals should optimize their allocation of resources between growth, reproduction and somatic maintenance according to their average lifespan or the risk of environmental mortality.
A large number of studies have attempted to challenge the predictions of a trade-off between early-life and late-life performances, through laboratory experiments and field longitudinal studies (Partridge et al., 1999; Metcalfe and Monaghan, 2003; Lee et al., 2013; Lemaître et al., 2015). While there is ample evidence suggesting that strong investment in early reproduction is associated with increased rates of reproductive or actuarial senescence (with differences between sexes, e.g., Lemaître et al., 2015; Jehan et al., 2020), evidence of trade-offs between growth and late-life fitness traits is relatively scarce and controversial. Individuals may draw considerable benefits from rapid growth, as reaching adult size in a minimum of time reduces vulnerability to environmental disturbances and predators while facilitating early entry into reproduction (Dmitriew, 2011). Nevertheless, individual growth has been found rarely maximal even when resource availability is unlimited, suggesting that higher growth rates (enabled by either a fast growth or large adult size) is costly (Metcalfe and Monaghan, 2003). To date, interactions between growth processes and senescence remain scarcely explored. For instance, in the three-spined stickleback, fast-growing individuals exhibit shorter longevity, suggesting that enhanced resource allocation to body development early in life increases actuarial senescence (Lee et al., 2013). In the damselfly Lestes viridis, experimentally-induced rapid larval development (but not growth rate) reduces adult life span by increasing oxidative damage (Janssens and Stoks, 2018). Other studies failed to detect such early-late life trade-offs. This is the case of a study comparing two ecotypes of the snake, Thamnophis elegans, characterized by a contrasted pace of life (fast vs. slow living pace of life) (Sparkman et al., 2007). However, the incomplete knowledge of the environmental conditions experienced early in life by the two ecotypes and the fact that snakes are growing continuously may have prevented the evidence of such a trade-off. In another study, the growth rate of males of the antler fly, Protopiophila litigata, was altered by the experimental manipulation of the larval diet, generating variation in development time, which was positively correlated with lifetime mating rate but not with reproductive senescence (Angell et al., 2020). As a result, more research is needed into the early-late trade-off between growth rate and early reproductive success, as well as their respective impacts on late-life reproductive success and senescence. Our study intends to elucidate these relationships by changing growth rate experimentally.
Modifying the amount of food or the quality of the diet provided to juveniles in order to manipulate growth is a priori reasonable, as the expression of trade-off is often dependent on resource availability (Metcalfe and Monaghan, 2003; Cohen et al., 2019). Nevertheless, this approach may have several drawbacks when confronted with the phenomenon of compensatory growth after periods of starvation (e.g., Metcalfe and Monaghan, 2003; Kecko et al., 2017; Ziegelbecker and Sefc, 2021), or when aging does not depend on early diet (Zajitschek et al., 2009), leading to contradictory or inconclusive results. Moreover, increasing growth rate by setting highly favorable conditions may generate individuals with a higher fitness, called the “silver spoon” effect (Grafen, 1988; Lindström, 1999; Monaghan, 2008), which may prevent detection of senescence (Angell et al., 2020). There is therefore a need to explore further the early-late trade-off between growth rate and reproductive senescence using experimental approaches a priori avoiding resource alteration during growth to manipulate growth rate. We propose here to study this relationship using a biological model where pre-adult growth can be easily discriminated against (holometabolous insect, where immature stages are strictly different from the sexually mature stage), can be manipulated independently of the diet, and for which experimental conditions are close to those of “field” conditions, to circumvent the problem of field-laboratory differences (Metcalfe and Monaghan, 2003).
This biological model is the mealworm beetle Tenebrio molitor. This insect “naturally” lives in stored grain product installations, in which it is considered as a pest. This insect is also attracting considerable interest for its cultivation as a source of food and feed, leading to the development of mass rearing installations (Ribeiro et al., 2018; van Huis, 2021). Living conditions of this insect in the laboratory and “natural” conditions are therefore less different from most other species. Moreover, this insect species seems to fit the preconditions of the disposable soma theory such as finite growth, age structure, and well-separated somatic and germline lines (Charlesworth, 2000). Under the conditions considered to be the most favorable (about 75% RH and 25°C (Punzo and Mutchmor, 1980)), larvae are taken about 17 weeks to reach the pupal stage, this latter stage lasts about ten days (Crosland, personal observations). Adults emerging from pupae can live for several months and do not grow anymore. Individuals are fertile throughout their adult life and this from their fifth day post-eclosion at the earliest. Females exhibit a peak of fertility from day 10 to 20 post-eclosion, which subsequently decreases until death (Dick, 1937; Jehan et al., 2020). To modify the growth rate of T. molitor, we adjusted the relative humidity during its larval development. Relative humidity has already been shown to influence growth duration and adult mass (Leclercq, 1948). Humidity might mediate food absorption and metabolism, independently of the amount or the quality of food provided (Hardouin and Mahoux, 2003; Ribeiro et al., 2018). Three relative humidity levels (55, 75, and 85% RH) were used, reported to be non-detrimental to larval survival (Punzo and Mutchmor, 1980).
We first controlled for the success of the modification of larval growth rate by this process: the higher the relative humidity in the larval environment, the faster the larvae developed and the larger the animals were. Females from larvae reared in these contrasted conditions showed differences in survival (longer longevity in adults from larvae grown at 85% RH). The females that had the higher developmental rate were also those that exhibited a higher fecundity early in life, but not late in life. The trade-off between high early developmental rate and reproductive senescence was demonstrated only in the condition where growth rate was higher (exceeding 1 mg/day).
Materials and methods
Insect culture and larval environmental conditions
Age-controlled experimental larvae were obtained among the progeny of insects from an outbred stock culture (150 females and 100 males of about 14–21 days post-eclosion) kept together for 3 days in a plastic tank (L 56.5 × l 36.5 × h 15 cm) with bran flour in standard laboratory conditions (24 ± 1°C, 70 ± 5% RH and in permanent darkness). When the larvae had reached 4 weeks old (size from 0.8 to 1.2 cm), 180 of them were isolated and haphazardly distributed into three relative humidity conditions: 55 ± 5%, 70 ± 5%, and 85 ± 5% (designated as larval RH thereafter). For all these three conditions, the temperature was set at 24.5 ± 2°C and the larvae were supplied ad libitum with a 9:1 mix of bran flour:fish food (JLB, Novo Malawi). After pupation, all individuals were transferred, still isolated, at 24.5 ± 1°C, 70 ± 5% RH and in permanent darkness. Therefore, only larval development was under different environmental conditions to manipulate growth rates only.
Growth duration and survival
Isolated larvae were observed twice a week until pupation. Development time (or growth duration) was considered from the egg laying date (estimated as the median date of the 3-day period during which their parents reproduced) to the date of pupation. Pupae were weighed (OHAUS Discovery DV114C balance, d = 0.1 mg) to determine their growth rate, which was calculated as the ratio of pupal mass (in mg) to growth duration (in days) from the egg laying date, when body mass was assumed to be null. Because the larvae were kept in the same environment (same tank) before being haphazardly assigned to their respective RH treatments, they were not supposed to have a distinct body mass. After pupation, all individuals were transferred at 24.5 ± 1°C, 70 ± 5% RH and in permanent darkness. Pupae were observed twice a week until eclosion. New-born adults were weighed and sexed. After eclosion, individuals were maintained in the same environmental condition as were the pupae, supplied with the same bran flour-fish food mix than during the larval stage and provided with a little cube of apple (about 4 mm side) weekly as a water source. Survival of individuals was checked weekly until the death of all of them. Individual size was estimated after death by measuring the length of both elytra using a Nikon SMZ 1500 stereo microscope with NIS Elements AR software (version 4.00.03). The length of each elytron was measured from its insertion into the scutellum to its basis. All measures were made by the same person with a precision of 0.01 mm. The average value of the two measures per insect was used as a data point.
Age-specific reproductive performance
Reproduction was analyzed on females only because data on egg production are relevant of their reproductive investment, whereas reproductive investment in males is rather linked to copulation rate, which was not assessed in this study. Reproductive performance of each female beetle was assessed through two reproductive episodes of 10 days: an early one, from day 10 to day 20 post-eclosion, and a late one, from day 40 to day 50 post-eclosion. This early reproductive episode corresponds to the age where females are the most fertile, while the “late” reproduction corresponds to the age period when fertility is declining, but at which mortality is low enough to minimize selective disappearance (Jehan et al., 2020). The reproductive partners (a novel one in each reproductive episode, assigned randomly) were 10-to-20-day old, virgin, males and came from the stock culture. The females were weighed before and after each reproductive episode. During each reproductive episode, a female and her partner were placed in a plastic Petri dish (9 cm diameter) containing a thin layer of bleached flour, a 2 ml tube of water clogged with cotton wool and a piece of apple. To prevent eggs from hatching before being counted, each couple was transferred to a new Petri dish at the middle of the 10-day reproductive period. Eggs were counted after sifting the flour (mesh size: 600 μm). Between the early and the late-life reproductive episodes, and after the late reproductive episode, the experimental individuals were kept isolated, at 24.5 ± 1°C, 70 ± 5% RH and in permanent darkness.
Statistical analysis
Data on larval development and reproduction were analyzed using R (version 4.1.2). Models presented in the results are those minimizing the Akaike’s information criterion (with a ΔAIC ≤ 2) and containing the fewest elements in an exhaustive list of models integrating variables and interactions of interest (Galipaud et al., 2017). Larval RH was coded as a factor (three modalities: 55, 70, and 85% RH).
We first tested whether our three experimental larval RH treatments affected female larval growth duration, pupal mass, growth rate, elytra length, survival (as the adult age at death), and total fecundity (calculated as the sum of eggs laid during the two reproductive episodes, R1 + R2). An ANOVA followed by a Tukey’s HSD test (functions HSD.test, agricolae package – de Mendiburu, 2021) were computed for larval growth duration, elytra length and total fecundity. For pupal mass and larval growth rate, a Kruskal–Wallis test followed by non-parametric pair comparisons (function kruskal, package agricolae—de Mendiburu, 2021) were computed due to an over-dispersion in the females raised at 85% RH. For survival, a Kaplan–Meier analysis (functions Surv and survdiff, survival package—Therneau et al., 2022) was followed by a pairwise log-rank comparison (function pairwise_survdiff, package survminer—Kassambara et al., 2021). All females that reached pupation were included in the analyses of growth duration, pupal mass and growth rate (55% RH: n = 32; 70% RH: n = 29; 85% RH: n = 33). Three females raised at 70% RH and four raised at 55% RH were not included in the analysis of the elytra length because their elytra were damaged. Only females that had no problem during both reproductive episodes (e.g., death of the partner) were considered for the total fecundity analysis (RH were: 55% RH: n = 28; 70% RH: n = 28; 85%: n = 30).
The effect of larval RH on both female egg production and reproductive investment (as the number of eggs laid per milligram of female, i.e., corrected for the mass of individuals before the reproductive episode considered) was analyzed using linear mixed models (lme function, package nlme—Pinheiro et al., 2022). The most complex models included reproductive episode (early vs. late), larval RH (RH: 55, 70, and 85%), growth rate (mg/day), and life expectancy at the beginning of each reproductive episode as main effects, and the two-way interactions between reproductive episode, larval RH, and growth rate. To adjust for selective disappearance, life expectancy at each reproductive episode was included as an independent covariate (Tarwater and Arcese, 2017). Female identity was included as a random factor on the intercept. After model selection with models computed at maximum likelihood, parameters were estimated by the same model but computed by maximizing the restricted log-likelihood (Zuur et al., 2009). Sample sizes for each larval RH were: 55% RH: n = 26; 70% RH: n = 28; 85%: n = 30. Even if our main hypothesis concerned the influence of growth rate on reproduction, we opted to include larval RH in the model selection despite its collinearity with growth rate. Indeed, larval RH seemed to influence more than just the growth rate. A summary of the best models according to AIC is available in Supplementary material (Supplementary Table 1 for the number of eggs and Supplementary Table 2 for the reproductive investment).
In addition, links between growth rate (mg/day), early reproduction (R1), late reproduction (R2), and adult longevity were investigated through a path analysis. The hypotheses tested in the model, according to the disposable soma theory, were: (i) individuals with a higher growth rate would be more productive in early reproduction but less in late one, (ii) individuals with a higher growth rate would live shorter adult life, and (iii) individuals more productive during reproduction would die earlier. From these hypotheses, we constructed four diagrams (see Supplementary Figure 1 in Supplementary material). The significance of relationships between variables and their effects were determined using the package lavaan (function sem) (Rosseel et al., 2022). The best diagram was the one minimizing AIC (with a ΔAIC ≤ 2) and minimizing of the number of links. Five model-fit indices were also considered: a chi-square test with as null hypothesis the adequacy of the model to the data, the Comparative Fit Index, the Tucker–Lewis Index, the Root Mean Square Error of Approximation, and the Standardized Root Mean Square Residual (Rosseel et al., 2022).
Our analysis of reproductive senescence relied on a senescence index: the difference in egg numbers between the late reproductive episode and the early one, corrected by the total fecundity of the female. This correction accounting for the total fecundity allows scaling individuals.
Reproductive senescence, therefore, occurs if this index is negative. Linear models conducted included larval RH, growth rate, and/or age at death as main effects, and the interaction between larval RH and growth rate. Individual age at death was used as an indicator of individual quality. A summary of the best models according to AIC is available in the Supplementary material (Supplementary Table 3).
Results
Effect of larval environment on growth duration, pupal mass, growth rate and elytra length of females Tenebrio molitor
The six traits analyzed, larval growth duration, pupal mass, larval growth rate, adult elytra length, adult survival, and total fecundity, were all significantly affected by the larval RH (growth duration: F2,91 = 102, p < 0.0001; pupal mass: χ2 2 = 51.9, p < 0.0001; growth rate: χ2 2 = 78.3, p < 0.0001; elytra length: F2,88 = 50.6, p < 0.0001; survival: χ2 2 = 17.1, p = 0.0002; total fecundity: F2,83 = 5.11, p = 0.008) (Figure 1). The higher the relative humidity was in the larval RH, the less time the larvae needed to reach pupation (Figure 1A), and the heavier were the pupae (Figure 1B). Because growth rate values were calculated from these two latter measures, they unsurprisingly increase as larval relative humidity increases (Figure 1C). Elytra length was similar between females grown at 55 and 70% RH whereas females grown at 85% RH were larger (Figure 1D). Adult survival was similar between females reared at 55 and 70% RH (55% RH: 129 ± 9 days; 70% RH: 128 ± 8 days). Females grown at 85% RH lived about 30 days longer than females reared at 55 and 70% RH (85% RH: 159 ± 10 days) (Figure 1E). Females reared at 85% RH produced more eggs during the two reproductive episodes cumulated than females grown at lower relative humidity (55% RH: 126 ± 6 eggs; 70% RH: 129 ± 6 eggs; 85% RH: 148 ± 5 eggs) (Figure 1F).
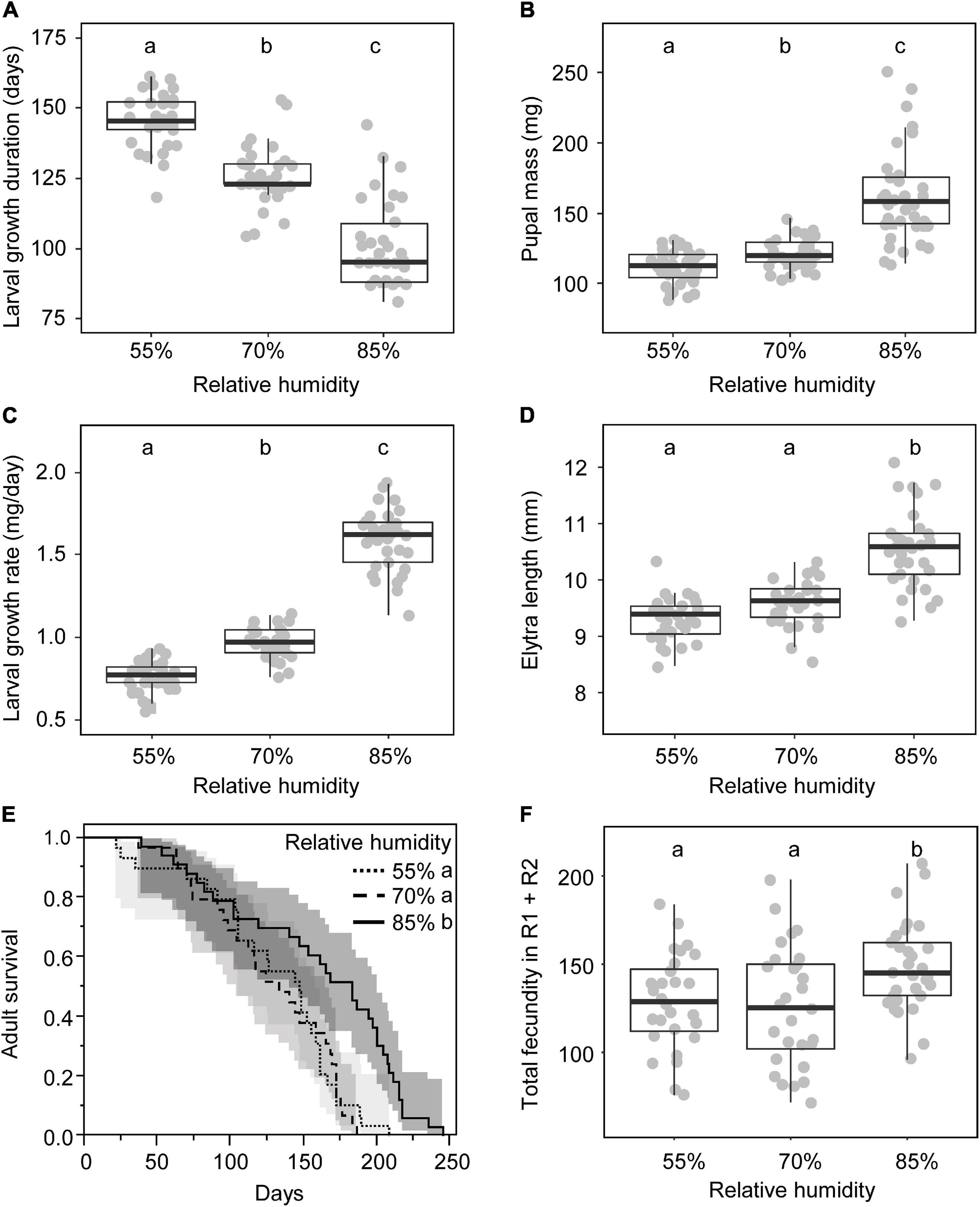
Figure 1. Variation of life-history traits according to conditions of larval growth (relative humidity): growth duration (A), pupal mass (B), growth rate (C), elytra length (D), adult survival (E), and total fecundity (sum of the number of eggs laid during the early and the late reproductive episode) (F). Different letters indicate significant differences assessed by post-hoc tests [Tukey HSD in panels (A,D,F), Kruskal–Wallis with Bonferroni correction in panels (B,C) and Log-rank pairwise tests in panel (E)]. In panels (A–F), gray dots are observations; box plots are median (bold lines), interquartile (boxes) and interdecile (error bars). In panel (E), confidence interval curves are given for 95%.
Reproductive performance
Females laid significantly more eggs during their early reproductive episode than during the late one (76.0 ± 2.4 eggs vs. 58.8 ± 1.9 eggs, respectively) (Table 1 and Figure 2A). In general, the higher the female growth rates during larval stages, the higher the egg production (Table 1 and Figure 2A). However, this increase in female fecundity was contrasted according to the reproductive episodes considered (Table 1 and Figure 2A). Indeed, the positive association between egg production and growth rate was only significant during the early reproductive episode (F = 12.68; d.f. = 1 on 82; p < 0.001) but not during the late one (F = 0.12; d.f. = 1 on 82; p = 0.735) (Figure 2A). In addition, female life expectancy was positively associated with fecundity (Table 1): the most fecund females in the two reproductive episodes measured here were also those that lived the longest (Figure 2B).
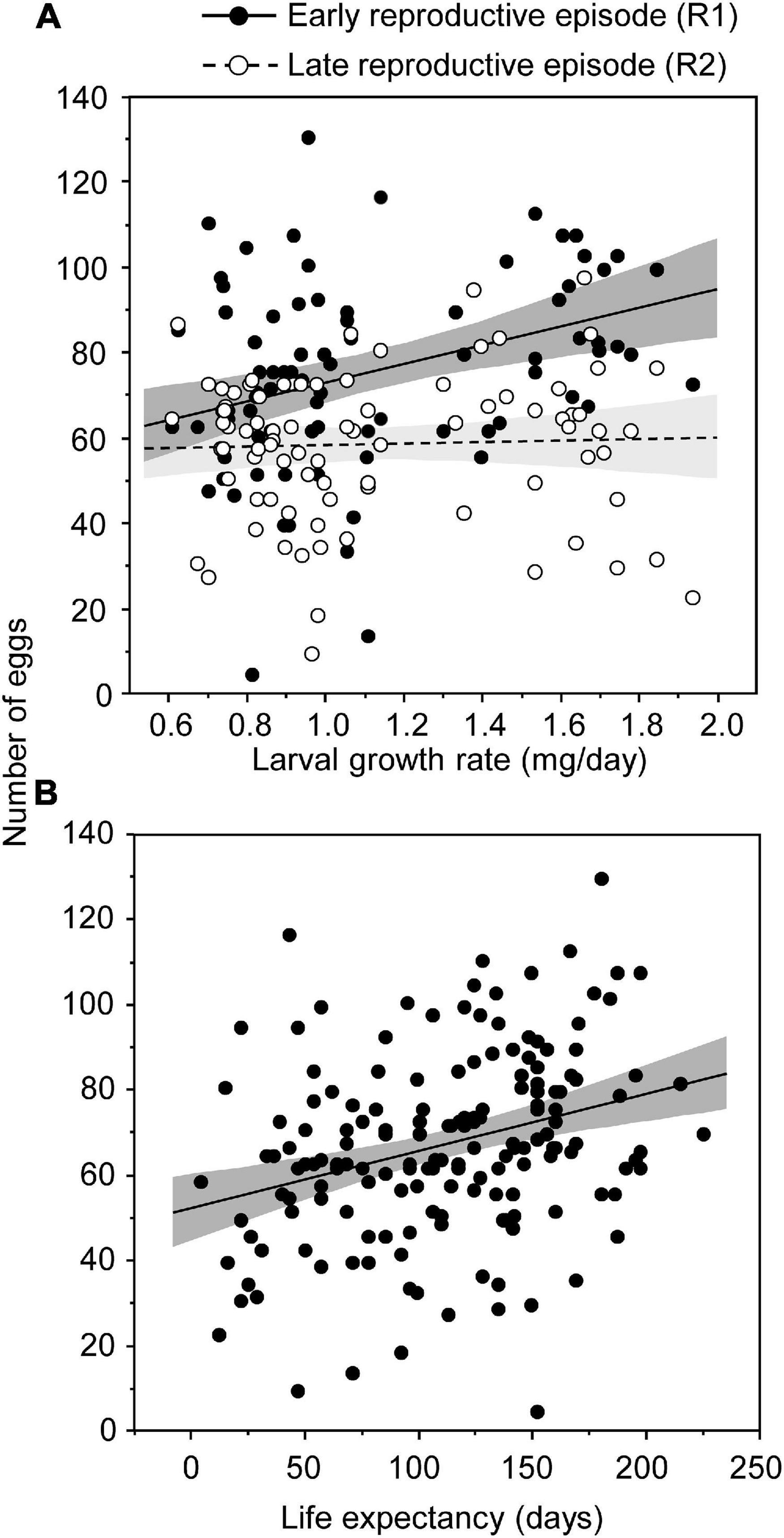
Figure 2. Number of eggs laid by Tenebrio molitor as a function of the female’s growth rate according to the reproductive episode (early reproductive episode, R1: females 10–20 days old post-eclosion; late reproductive episode, R2: females 40–50 days old post-eclosion) (A) and as a function of life expectancy at reproductive episode (B). Each dot represents the measure of a single female during one reproductive episode. Lines are linear regressions with 95% confidence intervals.
Female’s reproductive investment (number of eggs per mg of weight) was significantly higher during the early reproduction episode than during the late one (0.64 ± 0.02 eggs/mg vs. 0.53 ± 0.02 eggs/mg, respectively) (Table 2 and Figure 3A). Growth rate also influenced reproductive investment: the higher the growth rate, the lower the reproductive investment (Table 2 and Figure 3B). Females that lived the longest after reproduction were those that invested the most in reproduction according to their body mass (Table 2 and Figure 3C).
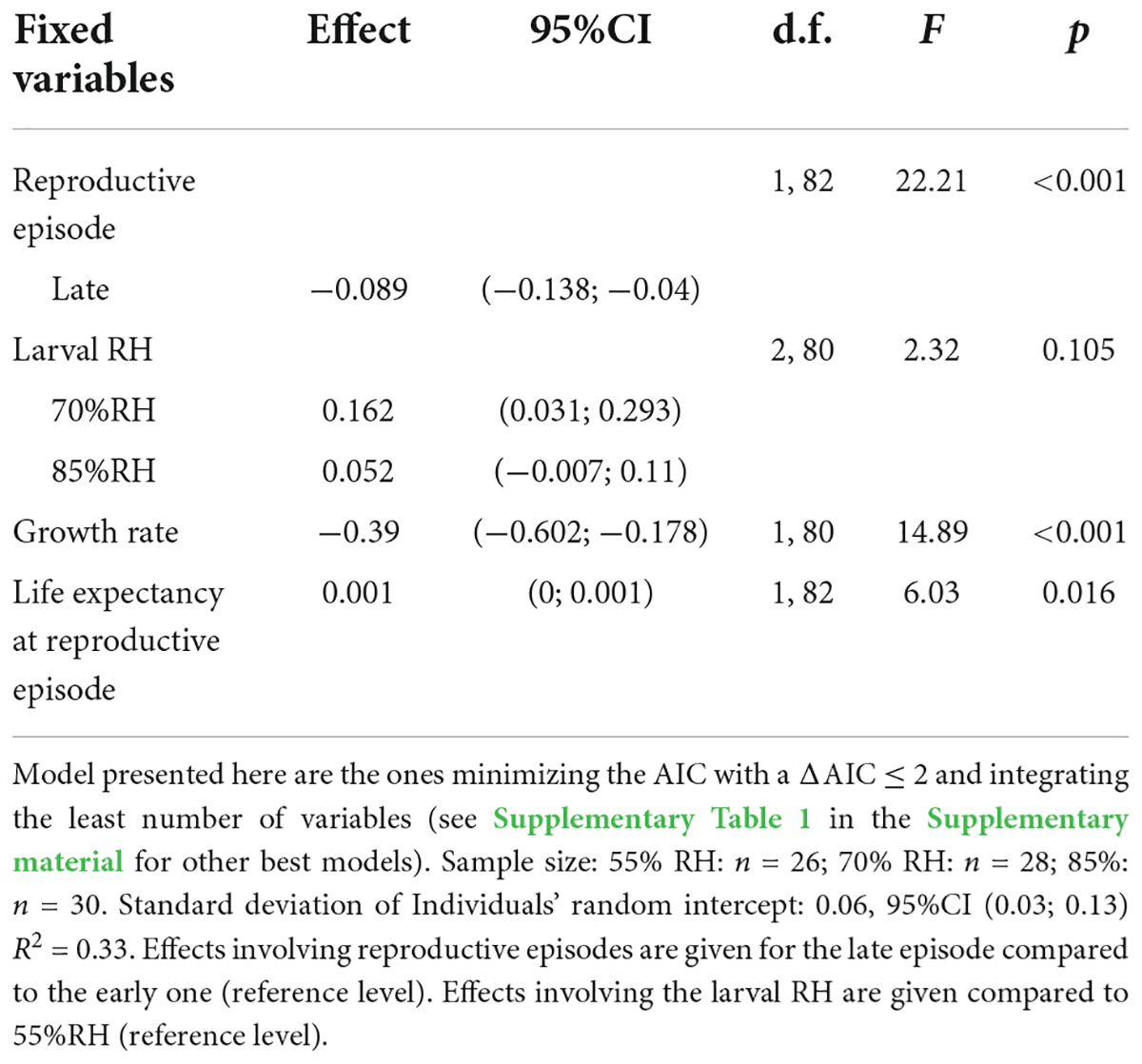
Table 2. Linear mixed model analyzing the reproductive investment (number of eggs per mg of body mass before the reproduction episode) of Tenebrio molitor females.
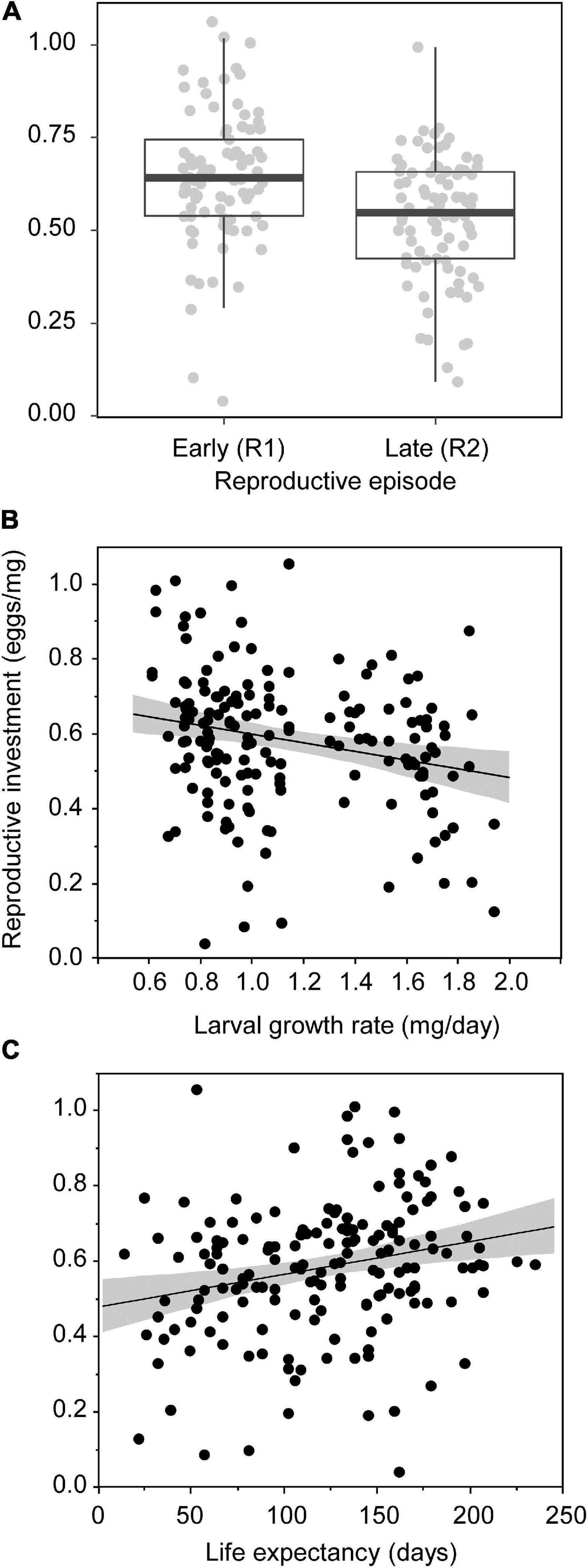
Figure 3. Reproductive investment (number of eggs per mg of body mass) of females Tenebrio molitor, according to reproductive episode (early reproductive episode, R1: females 10–20 days old post-eclosion; late reproductive episode, R2: females 40–50 days old post-eclosion) (A); as a function of the female’s growth rate (B) and as a function of life expectancy at reproductive episode (C). Each dot represents the measure of a single female during one reproductive episode. Lines are linear regressions with 95% confidence intervals.
Causality network between growth rate, early/late fecundity and adult longevity
As shown above in the analysis of reproductive performances, the path analysis revealed that females that grew faster laid more eggs during the early reproductive episode and lived longer (Figure 4). The link between early and late fecundity was not significant. Finally, the more fecund the females were late in their life, the longer they lived (Figure 4).
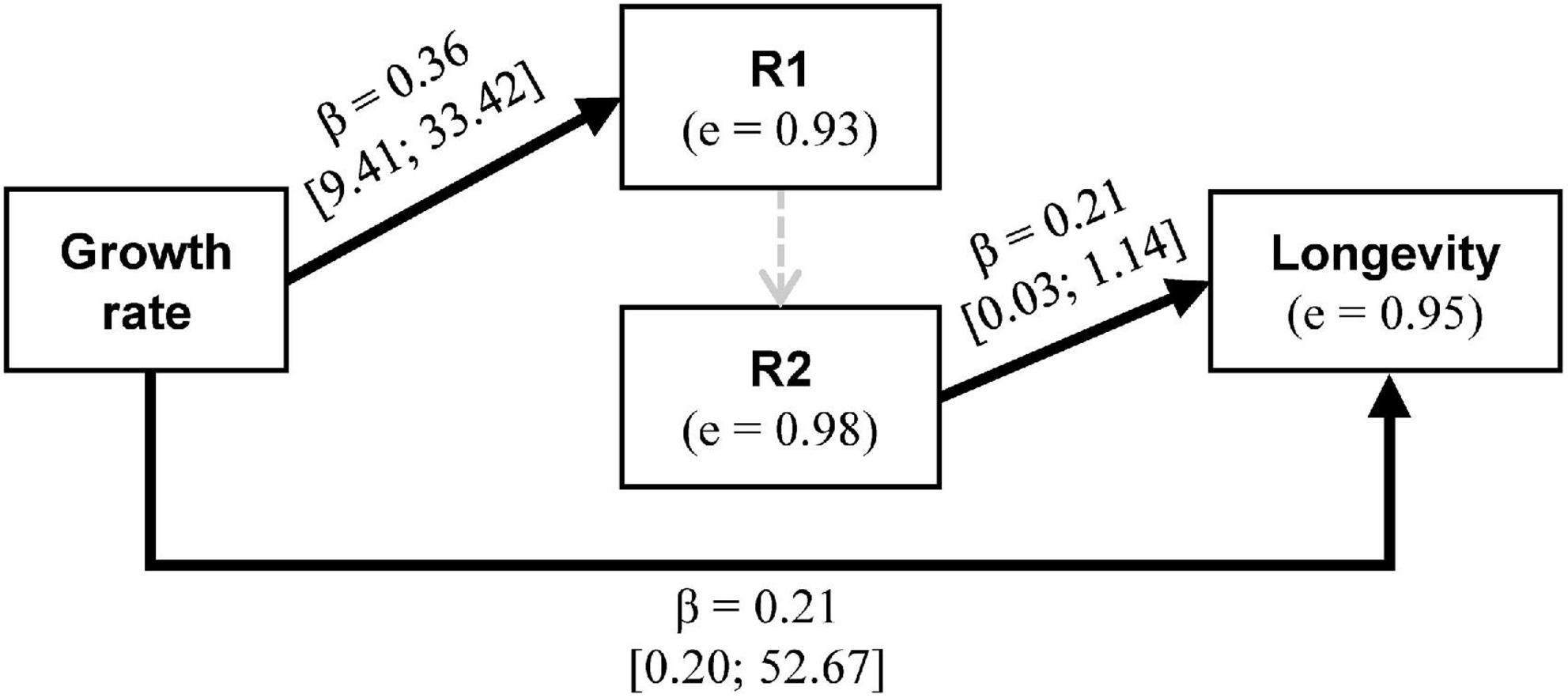
Figure 4. Path analysis diagram describing the direct and indirect effects of growth rate on fecundity and adult longevity (best one of four tested; see Supplementary Figure 1 in Supplementary material for other diagrams). Arrows with solid black lines represent significant (p-value <0.05) links, arrows with dashed lines represent links tested but insignificant. When the relationship was significant, the standardized coefficient (β) and 95% confidence interval of the non-standardized coefficient are given. Errors (e) are given for each endogenous variable as √(1-R2).
Reproductive senescence
The reproductive senescence index was significantly affected by the interaction between growth rate and larval RH (Table 3). There was no significant relationship between growth rate and reproductive senescence in females that grew at 55% (F = 0.65; df = 1; p = 0.430) and 70% RH (F = 3.11; df = 1; p = 0.090) (Figure 5). However, females from larva kept at 85% RH showed stronger reproductive senescence when having grown faster (F = 9.60; d.f. = 1; p = 0.004) (Figure 5).
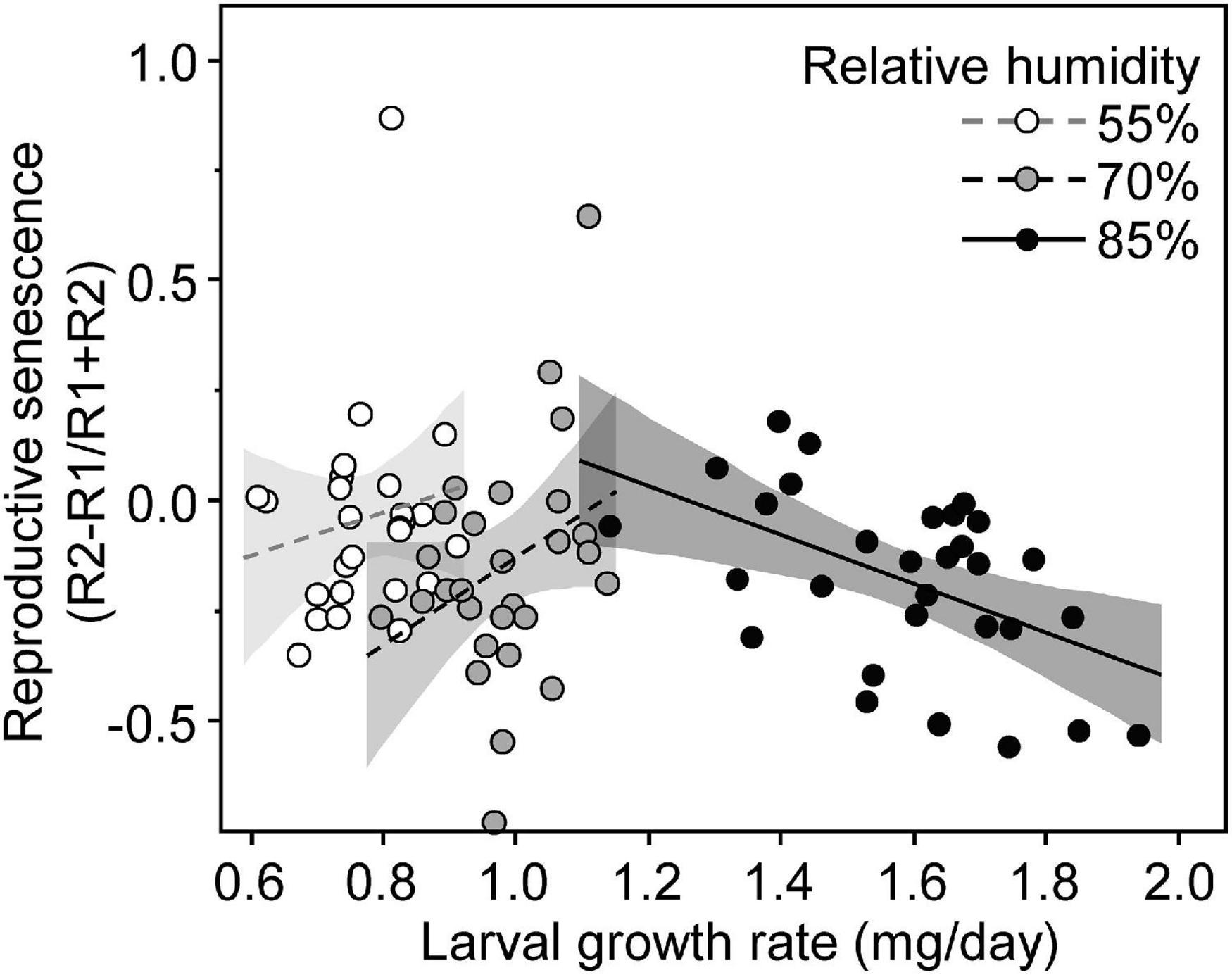
Figure 5. Reproductive senescence of females of Tenebrio molitor as a function of larval growth rate and according to the conditions of larval growth (relative humidity). Reproductive senescence was estimated by the difference between the late number of eggs (R2) and the early number of eggs (R1) corrected by the total number of eggs during the two reproductive episodes (R1 + R2).
Discussion
Higher relative humidity increases larval growth rate in Tenebrio molitor
By manipulating the relative humidity during the larval stages of T. molitor, we successfully manipulated the growth rate. The larvae reared at higher relative humidity developed faster. This enhanced growth rate resulted from both a shorter growth duration and an increase in body mass when reaching the pupal stage. Such a result was expected, as the relative humidity is known to modify T. molitor larval development (Leclercq, 1948). A mechanism proposed but not tested yet to our knowledge, is that higher relative humidity might enhance the capacity to absorb water and so to ingest food, increasing metabolism, irrespectively of the food quality and quantity provided (Hardouin and Mahoux, 2003; Ribeiro et al., 2018). Indeed, although T. molitor is an insect living on dry substrates and is efficient at extracting and saving water, its optimal relative humidity is around 70% (Ribeiro et al., 2018 for a review). Such an effect of relative humidity on body mass might be a potential source of confounding effect when attempting to understand how growth rate is trading-off against future performances and especially future reproduction (Metcalfe and Monaghan, 2003). It is widely known that the body mass of animals, and especially insects, is directly linked with individual body condition and often predicts fecundity (Arrese and Soulages, 2010; Zanchi et al., 2019). However, it seems illusory to think that changing larval growth rate can be done without affecting adult size in T. molitor. We have controlled for this effect of body size in our statistical analyses, but it never directly affected female fertility nor their reproductive investment (see below). Additional experimental methods enabling to generate variation in larval growth rate might provide complementary information regarding early-late life trade-offs, at least to figure out whether they all converge toward the same results. They may involve the modification of the larval environment by other means than the manipulation of the relative humidity, such as manipulating food or temperature. They may also involve the use of different strains of T. molitor differing in their larval growth rate (e.g., inbred lines or lines obtained after from selection experiments). At least, our present study succeeded in generating individuals that have experienced different growth conditions during the larval stages only. The adults were all kept in a common garden. Thus, if, as suspected, changes in larval growth rate between the RH treatment modalities might be caused by metabolic changes, they were due to differences in the larval environment only.
Does increasing growth rate of Tenebrio molitor induce a “silver spoon” effect?
The experimental modification of the environmental relative humidity of larvae affected the survival of T. molitor adult females. There were no differences between individuals reared at 55 and 70% RH, but the ones reared at 85% RH survived longer. The same tendency was observed for the total fecundity. In addition, the longer the females are living after the last reproductive episode, the more eggs they have laid and the more they have invested in reproduction. This observation is congruent with the absence of terminal investment and rather militates for the hypothesis of the use of a reproductive restraint strategy in this insect species, as previously proposed (Jehan et al., 2021, 2020). The higher reproductive performances of long-lived females may also be linked to their individual quality. Indeed, considering the fact that females reared at 85% RH during their larval stages were also those that lived the longest, were the largest, and laid more eggs (see below), they may benefit from a “silver spoon” effect, where individuals born in good conditions have fitness advantages later in life (Grafen, 1988; Lindström, 1999; Monaghan, 2008). Such an effect has already been observed—not consistent with all life-history traits, however—in an experimental study manipulating larval diet in an insect (Angell et al., 2020). Alternatively, high environmental relative humidity may represent cues for a harsher environment, as it could be associated to better conditions for microbial development and therefore higher risk of infection. As a result, females might have adaptively accelerated their reproductive effort (Nettle et al., 2013). However, such an acceleration of reproduction is expected to negatively affect somatic defenses and reduce longevity (Williams, 1957; Kirkwood, 1977; Lemaître et al., 2015; Maklakov and Chapman, 2019). Since our controlled laboratory conditions prevented the development of microbial pathogens and provided ad libitum food, the survival cost of accelerated early reproduction could not be revealed. Further study may need to look at the immunity of the insects in such a condition.
Higher larval growth rate increased reproductive senescence
As expected, the female reproductive performance, in terms of both number of eggs laid and reproductive investment (number of eggs controlled by female body mass), were lower late in life than earlier (Dick, 1937; Jehan et al., 2021). Females that exhibited the highest growth rate laid more eggs during the early reproduction episode, but the larval growth rate did not affect the number of eggs produced during the late one. The index of reproductive senescence we have used, calculated as the difference between the number of eggs laid during the late and the early reproductive episode divided by the total number of eggs laid, provides complementary information. This index has the advantage of taking into account female total fecundity (during both reproductive episodes) and so reduces the influence of individual female quality. According to this index, reproductive senescence was only detected in females reared at 85% RH, namely the fastest-developing ones (because they had the shorter growth duration and the highest pupal mass), exhibiting growth rate values above one mg per day. Our experimental approach did not allowed separating the effect of the onset of senescence and the rate of senescence. Therefore, we cannot tell whether females that developed faster were those that had the earliest of senescence. Nevertheless, our results clearly illustrate the occurrence of pace of life along a slow-fast continuum. Fast-developing females were larger, exhibited higher fecundity early in life, but suffer from steep reproductive senescence later while growing in age. By contrast, slow-developing females were smaller, died earlier, but exhibited stable reproductive performances throughout their lives (at least between the 10 and 50 days of adult life we have measured here). The results of our work are in line with those of two previous studies on insects. Hunt et al. (2004) showed that high-quality cricket males were those that invested the most in reproduction at the expense of reduced longevity. Hooper et al. (2017) showed that high-condition males of a neriid fly were those that had the quickest development, but the fastest reproductive and actuarial senescence compared to low condition males. Since we did not measure the total (lifetime) reproductive success of the females, we cannot exclude that the observed low rate of reproductive senescence among slow-developing females is due to an incomplete reproductive census. However, our protocol, where “only” two reproductive episodes were analyzed—one early in life, when fecundity was predicted to be the highest, and one later in life (from the age of 40 day old onward), when fecundity is known to begin its decline (Jehan et al., 2020)—has the advantage of measuring reproductive senescence before the onset of actuarial senescence. Perhaps recording additional reproductive episodes later in life would allow a comparison of senescence trajectories among females with contrasted growth rates, but such a study would require disentangling senescence from selective disappearance (Vaupel et al., 1979; Nussey et al., 2011), since mortality is rapidly increasing after 60 days of adult life.
Conclusion
By manipulating the relative humidity of the environment of the larvae of the mealworm beetle, T. molitor, we were able to generate variation in growth rate and adult female quality consistent with a “silver spoon” effect (Grafen, 1988). Despite this effect, and in line with the disposable soma theory of aging, we provide unambiguous evidence that growth rate significantly correlates with reproductive senescence. Overall, our study shows that growth rate, which is highly dependent on the early-life environment, is an important factor contributing to adult life history and senescence through the occurrence of early-late life trade-offs.
Data availability statement
The raw data supporting the conclusions of this article will be made available by the authors, without undue reservation.
Author contributions
All authors listed have made a substantial, direct, and intellectual contribution to the work, and approved it for publication.
Funding
This study received support from the National Research Agency (ANR-21-CE02-0023). AC was funded by a grant from the MESRI (French Ministère de l’Enseignement Supèrieur, de la Recherche et de l’Innovation).
Conflict of interest
The authors declare that the research was conducted in the absence of any commercial or financial relationships that could be construed as a potential conflict of interest.
Publisher’s note
All claims expressed in this article are solely those of the authors and do not necessarily represent those of their affiliated organizations, or those of the publisher, the editors and the reviewers. Any product that may be evaluated in this article, or claim that may be made by its manufacturer, is not guaranteed or endorsed by the publisher.
Supplementary material
The Supplementary Material for this article can be found online at: https://www.frontiersin.org/articles/10.3389/fevo.2022.915054/full#supplementary-material
References
Angell, C. S., Oudin, M. J., Rode, N. O., Mautz, B. S., Bonduriansky, R., and Rundle, H. D. (2020). Development time mediates the effect of larval diet on ageing and mating success of male antler flies in the wild. Proc. R. Soc. Lond. B Biol. Sci. 287, 20201876. doi: 10.1098/rspb.2020.1876
Arrese, E. L., and Soulages, J. L. (2010). Insect fat body: Energy, metabolism, and regulation. Annu. Rev. Entomol. 55, 207–225. doi: 10.1146/annurev-ento-112408-085356
Baudisch, A. (2005). Hamilton’s indicators of the force of selection. Proc. Natl. Acad. Sci. U.S.A. 102, 8263–8268. doi: 10.1073/pnas.0502155102
Charlesworth, B. (2000). Fisher, medawar, hamilton and the evolution of aging. Genetics 156, 927–931. doi: 10.1093/genetics/156.3.927
Cohen, A. A., Coste, C. F. D., Li, X.-Y., Bourg, S., and Pavard, S. (2019). Are trade-offs really the key drivers of ageing and life span? Funct. Ecol. 34, 153–166. doi: 10.1111/1365-2435.13444
Dmitriew, C. M. (2011). The evolution of growth trajectories: What limits growth rate? Biol. Rev. 86, 97–116. doi: 10.1111/j.1469-185X.2010.00136.x
Flatt, T., and Partridge, L. (2018). Horizons in the evolution of aging. BMC Biol. 16:93. doi: 10.1186/s12915-018-0562-z
Galipaud, M., Gillingham, M. A. F., and Dechaume-Moncharmont, F.-X. (2017). A farewell to the sum of akaike weights: The benefits of alternative metrics for variable importance estimations in model selection. Methods Ecol. Evol. 8, 1668–1678. doi: 10.1111/2041-210X.12835
Grafen, A. (1988). “On the uses of data on lifetime reproductive success,” in Reproductive success, ed. T. H. Clutton-Brock (Chicago, IL: The University of Chicago Press), 454–471.
Hamilton, W. D. (1966). The moulding of senescence by natural selection. J. Theor. Biol. 12, 12–45. doi: 10.1016/0022-5193(66)90184-6
Hardouin, J., and Mahoux, G. (2003). Zootechnie d’insectes – Elevage et utilisation au bénéfice de l’homme et de certains animaux [WWW Document]. Available online at: https://docplayer.fr/22063218-Zootechnie-d-insectes-elevage-et-utilisation-au-benefice-de-l-homme-et-de-certains-animaux.html (accessed March 15, 22).
Hooper, A. K., Spagopoulou, F., Wylde, Z., Maklakov, A. A., and Bonduriansky, R. (2017). Ontogenetic timing as a condition-dependent life history trait: High-condition males develop quickly, peak early, and age fast. Evolution 71, 671–685. doi: 10.1111/evo.13172
Hunt, J., Brooks, R., Jennions, M. D., Smith, M. J., Bentsen, C. L., and Bussière, L. F. (2004). High-quality male field crickets invest heavily in sexual display but die young. Nature 432, 1024–1027. doi: 10.1038/nature03084
Janssens, L., and Stoks, R. (2018). Rapid larval development under time stress reduces adult life span through increasing oxidative damage. Funct. Ecol. 32, 1036–1045. doi: 10.1111/1365-2435.13068
Jehan, C., Chogne, M., Rigaud, T., and Moret, Y. (2020). Sex-specific patterns of senescence in artificial insect populations varying in sex-ratio to manipulate reproductive effort. BMC Evol. Biol. 20:18. doi: 10.1186/s12862-020-1586-x
Jehan, C., Sabarly, C., Rigaud, T., and Moret, Y. (2021). Late-life reproduction in an insect: Terminal investment, reproductive restraint or senescence. J. Anim. Ecol. 90, 282–297. doi: 10.1111/1365-2656.13367
Jones, O. R., Scheuerlein, A., Salguero-Gómez, R., Camarda, C. G., Schaible, R., Casper, B. B., et al. (2014). Diversity of ageing across the tree of life. Nature 505, 169–173. doi: 10.1038/nature12789
Kassambara, A., Kosinski, M., Biecek, P., and Fabian, S. (2021). survminer: Drawing survival curves using “ggplot2”.
Kecko, S., Mihailova, A., Kangassalo, K., Elferts, D., Krama, T., Krams, R., et al. (2017). Sex-specific compensatory growth in the larvae of the greater wax moth Galleria mellonella. J. Evol. Biol. 30, 1910–1918. doi: 10.1111/jeb.13150
Leclercq, J. (1948). Influence des conditions hygrométriques sur les larves, les nymphes et les adultes de «Tenebrio Molitor» L. Arch. Int. Physiol. 55, 366–376. doi: 10.3109/13813454809144860
Lee, W.-S., Monaghan, P., and Metcalfe, N. B. (2013). Experimental demonstration of the growth rate–lifespan trade-off. Proc. R. Soc. Lond. B Biol. Sci. 280, 20122370. doi: 10.1098/rspb.2012.2370
Lemaître, J.-F., Berger, V., Bonenfant, C., Douhard, M., Gamelon, M., Plard, F., et al. (2015). Early-late life trade-offs and the evolution of ageing in the wild. Proc. R. Soc. Lond. B Biol. Sci. 282, 20150209. doi: 10.1098/rspb.2015.0209
Lindström, J. (1999). Early development and fitness in birds and mammals. Trends Ecol. Evol. 14, 343–348. doi: 10.1016/S0169-5347(99)01639-0
Maklakov, A. A., and Chapman, T. (2019). Evolution of ageing as a tangle of trade-offs: Energy versus function. Proc. R. Soc. Lond. B Biol. Sci. 286, 20191604. doi: 10.1098/rspb.2019.1604
Metcalfe, N. B., and Monaghan, P. (2003). Growth versus lifespan: Perspectives from evolutionary ecology. Exp. Gerontol. 38, 935–940. doi: 10.1016/S0531-5565(03)00159-1
Monaghan, P. (2008). Early growth conditions, phenotypic development and environmental change. Philos. Trans. R. Soc. Lond. B Biol. Sci. 363, 1635–1645. doi: 10.1098/rstb.2007.0011
Moorad, J., Promislow, D., and Silvertown, J. (2019). Evolutionary ecology of senescence and a reassessment of Williams’ ‘extrinsic mortality’ hypothesis. Trends Ecol. Evol. 34, 519–530. doi: 10.1016/j.tree.2019.02.006
Nettle, D., Frankenhuis, W. E., and Rickard, I. J. (2013). The evolution of predictive adaptive responses in human life history. Proc. R. Soc. Lond. B Biol. Sci. 280, 20131343. doi: 10.1098/rspb.2013.1343
Nussey, D., Coulson, T., Delorme, D., Clutton-Brock, T., Pemberton, J., Festa-Bianchet, M., et al. (2011). Patterns of body mass senescence and selective disappearance differ among three species of free-living ungulates. Ecology 92, 1936–1947. doi: 10.2307/23034827
Partridge, L., Prowse, N., and Pignatelli, P. (1999). Another set of responses and correlated responses to selection on age at reproduction in Drosophila melanogaster. Proc. R. Soc. Lond. B Biol. Sci. 266, 255–261. doi: 10.1098/rspb.1999.0630
Pinheiro, J., Bates, D., Debroy, S., Sarkar, D., and R Core Team (2022). nlme: Linear and nonlinear mixed effects models.
Punzo, F., and Mutchmor, J. A. (1980). Effects of temperature, relative humidity and period of exposure on the survival capacity of Tenebrio molitor (Coleoptera: Tenebrionidae). J. Kansas Entomol. Soc. 53, 260–270.
Ribeiro, N., Abelho, M., and Costa, R. (2018). A review of the scientific literature for optimal conditions for mass rearing Tenebrio molitor (Coleoptera: Tenebrionidae). J. Entomol. Sci. 53, 434–454. doi: 10.18474/JES17-67.1
Rosseel, Y., Jorgensen, T. D., Rockwood, N., Oberski, D., Byrnes, J., Vanbrabant, L., et al. (2022). lavaan: Latent variable analysis.
Sparkman, A. M., Arnold, S. J., and Bronikowski, A. M. (2007). An empirical test of evolutionary theories for reproductive senescence and reproductive effort in the garter snake Thamnophis elegans. Proc. Biol. Sci. 274, 943–950. doi: 10.1098/rspb.2006.0072
Tarwater, C. E., and Arcese, P. (2017). Age and years to death disparately influence reproductive allocation in a short-lived bird. Ecology 98, 2248–2254. doi: 10.1002/ecy.1851
Therneau, T. M., Lumley, T., Elizabeth, A., and Cynthia, C. (2022). original S->R port and R maintainer until 2009, survival: Survival analysis.
van Huis, A. (2021). Prospects of insects as food and feed. Org. Agr. 11, 301–308. doi: 10.1007/s13165-020-00290-7
Vaupel, J. W., Manton, K. G., and Stallard, E. (1979). The impact of heterogeneity in individual frailty on the dynamics of mortality. Demography 16, 439–454. doi: 10.2307/2061224
Wensink, M. J., Caswell, H., and Baudisch, A. (2017). The rarity of survival to old age does not drive the evolution of senescence. Evol. Biol. 44, 5–10. doi: 10.1007/s11692-016-9385-4
Williams, G. C. (1957). Pleiotropy, natural selection, and the evolution of senescence. Evolution 11, 398–411. doi: 10.1126/sageke.2001.1.cp13
Zajitschek, F., Hunt, J., Jennions, M. D., Hall, M. D., and Brooks, R. C. (2009). Effects of juvenile and adult diet on ageing and reproductive effort of male and female black field crickets, Teleogryllus commodus. Funct. Ecol. 23, 602–611.
Zanchi, C., Moret, Y., and Gillingham, M. A. F. (2019). Relationship between early body condition, energetic reserves and fitness in an iteroparous insect. bioRxiv [Preprint]. doi: 10.1101/774893
Ziegelbecker, A., and Sefc, K. M. (2021). Growth, body condition and contest performance after early-life food restriction in a long-lived tropical fish. Ecol. Evol. 11, 10904–10916. doi: 10.1002/ece3.7867
Zuur, A. F., Ieno, E. N., Walker, N. J., Saveliev, A. A., and Smith, G. M. (2009). “Mixed effects modelling for nested data,” in Mixed effects models and extensions in ecology with R, statistics for biology and health, eds A. F. Zuur, E. N. Ieno, N. Walker, A. A. Saveliev, and G. M. Smith (New York, NY: Springer), 101–142. doi: 10.1007/978-0-387-87458-6_5
Keywords: aging, cost of growth, disposable soma theory, experimental manipulation, reproductive investment, longevity, holometabolous insect
Citation: Crosland A, Rigaud T, Balourdet A and Moret Y (2022) “Born with a silver spoon in the mouth has bad sides too”: Experimentally increasing growth rate enhances individual quality but accelerates reproductive senescence in females of the mealworm beetle, Tenebrio molitor. Front. Ecol. Evol. 10:915054. doi: 10.3389/fevo.2022.915054
Received: 07 April 2022; Accepted: 28 July 2022;
Published: 12 August 2022.
Edited by:
Christos Rumbos, University of Thessaly, GreeceReviewed by:
Gabriel Pigeon, Université de Sherbrooke, CanadaPhyllis Lee, University of Stirling, United Kingdom
Copyright © 2022 Crosland, Rigaud, Balourdet and Moret. This is an open-access article distributed under the terms of the Creative Commons Attribution License (CC BY). The use, distribution or reproduction in other forums is permitted, provided the original author(s) and the copyright owner(s) are credited and that the original publication in this journal is cited, in accordance with accepted academic practice. No use, distribution or reproduction is permitted which does not comply with these terms.
*Correspondence: Agathe Crosland, YWdhdGhlLmNyb3NsYW5kQHUtYm91cmdvZ25lLmZy