- 1Department of Natural History, University Museum, University of Bergen, Bergen, Norway
- 2Department of Archaeology and Cultural History, NTNU University Museum, Trondheim, Norway
- 3Geological Survey of Norway, Trondheim, Norway
- 4Department of Historical and Classical Studies, Norwegian University of Technology and Science, Trondheim, Norway
Human impact on long-term vegetation and biodiversity changes is often discussed on a general level, connecting palynological data to archaeological time periods. In the present paper we present environmental change during the last 2,400 years on a coastal peninsula in Norway using pollen data from three sites: one lake and two bogs, in addition to 621 radiocarbon dates and in-context pollen samples from archaeological sites. Locally, a close relationship between palynological richness reflecting high landscape, habitat and floristic diversity, and the summed probability distribution of radiocarbon dates was found. During the settlement period 400 BCE–550 CE, concordant with maximum number of dates from archaeological contexts, a mosaic landscape containing infields and outfields developed. Cereals were cultivated and animals were grazing in heathlands that could provide both summer grazing and winter fodder. Additionally, seashores and wetlands were used for grazing. Settlement recession from 350 CE and abandonment following the 536 CE climate event, resulted in vegetation successions toward reforestation, abandonment of arable fields, and marshes turning into ombrotrophic peat. At the same time the distance to the sea, and to species rich shoreline meadows, continued to increase due to continuous postglacial land uplift in a flat landscape. A new increase in the summed probability distribution 900–1250 CE, is reflected in expansion of outfield pastures, heathlands in particular, a management that continued up into modern time. The local development is supported by the results on a regional scale, indicating overall climatic and social causes for observed vegetation changes. Both palynological richness and pollen-based landcover reconstructions indicate reforestation and less habitat diversity in the sixth century. On a regional scale, reforestation in the fifteenth century following the late medieval crises, is more pronounced than on the local scale, although both reflect exploitation of outfield resources.
Introduction
Human impact on landscapes, vegetation, and biodiversity is today visible locally as well as globally. During the last century, changes in the agricultural systems, demography, and increased pressure on land-area, have to a large extent changed what were sustainable cultural landscapes created through long-term management systems (Emanuelsson, 2009; Krzywinski et al., 2009). From early agriculture and traditional land-use practices creating new habitats and increased biodiversity, both species and habitat diversity, representing our natural and biocultural heritage, have been declining since the inception of modern agricultural practices and areal changes (Brondizio et al., 2019). Human impact is today considered the main driver of accelerating ecosystem transformations globally (Nogué et al., 2021).
Several paleoecological studies have shown the gradual transition from climate being the main driver of ecosystem change, to increased impact by human activity. In northern Europe this has especially taken place the last 6,000 years, after the introduction of agriculture (Odgaard and Rasmussen, 2000; Gaillard et al., 2010; Nielsen et al., 2012; Birks et al., 2016a; Marquer et al., 2017; Kuosmanen et al., 2018; Alenius et al., 2021). By establishment of farming societies, new habitats developed, and floristic diversity increased (Odgaard, 1999; Berglund et al., 2008; Overland and Hjelle, 2009; Birks et al., 2016a; Fredh et al., 2017; Halvorsen and Hjelle, 2017; Woodbridge et al., 2021). Working on longer time scales, palynological richness is a commonly used diversity measure, and although all pollen or spore types cannot be identified to species level and interspecific variation in pollen production and dispersal exist, a good correlation between species richness and palynological richness has been found (Odgaard, 1999, 2001; Meltsov et al., 2011; Birks et al., 2016b; Reitalu et al., 2019). The influence of human activity on long-term changes in habitat and species diversity, is often discussed on a general level, connecting palynological data to archaeological time periods, reflected in increasing and often stepwise impact from the Stone Age, through the Bronze Age, Iron Age and Middle Ages. With the assumption that establishment of farming societies resulted in opening-up of the landscape and development of new vegetation types through grazing, mowing and cultivation, landscape openness may be considered a proxy to habitat diversity, especially when combined with diversity measures. Here we apply pollen-based landscape reconstructions (Sugita, 2007a,b) which give an overall pattern of landscape openness (Gaillard et al., 2010; Nielsen et al., 2012; Fyfe et al., 2013; Kuneš et al., 2015; Hjelle et al., 2018) and the importance of different habitats in the landscape (Mehl et al., 2015; Alenius et al., 2021). We combine different approaches; palynological richness and landscape reconstructions and relate these to the summed probability distribution (SPD) and kernel density estimate (KDE) of radiocarbon dates (Bronk Ramsey, 2017) from archaeological contexts as the measure of human activity. SPD of radiocarbon dates has shown to be a good proxy for population size, demographic development, and the development of arable farming (Woodbridge et al., 2014; Solheim and Persson, 2018; Tallavaara and Pesonen, 2020; Bergsvik et al., 2021; Solheim, 2021).
Whereas climate and human impact have been drivers of vegetation change during the Holocene, relative sea level changes have also contributed to large changes in land areas. In Norway, strong isostatic land uplift since deglaciation has caused mostly continuous postglacial land emergence from the sea. This created new habitats for light-demanding species at the same time as it influenced potential harbor conditions.
The investigated archaeological sites are dated from 800 BCE to 1350 CE, covering two periods of special interest: The possible climate deterioration 560 CE (Büntgen et al., 2016), and the start of the Little Ice Age. Generally, there was a gradual development toward colder climate, culminating in the Little Ice Age, after the medieval climatic optimum 800–1100 CE (Sejrup et al., 2016). Both periods are also connected to plagues; the Justinian plague in Europe in the mid-sixth century and the late medieval crisis including the Black Death in 1349.
In the present study, we will combine palynological and archaeological data with the aim to connect vegetation development directly to activity and settlement and to discuss human impact on different spatial scales. To reflect differences in the spatial scales, we combine pollen data from one large lake, two bogs and in-context samples from archaeological sites. The pollen data are compared to radiocarbon dates of charcoal from archaeological contexts to link vegetation types, habitat and palynological diversity, and the intensity of human impact through time. The results are discussed in relation to local sea level changes and general climate development. We ask the following questions: Is settlement size and intensity decisive for habitat diversity and palynological richness in the Iron Age? Is the development on a local scale also visible on a more regional level?
Materials and methods
Study area
The study area is at Ørland (Figure 1), on the western part of a peninsula northwest of Trondheim in Central Norway (Ystgaard, 2019). The area is relatively flat having a plateau around 11 m asl with some higher hills reaching up to 150 m asl. The ground consists of a glacial till at depth, overlain by thick deposits of marine and littoral origin. The marine, calcareous sediments give a rich soil well-suited for agriculture, and farms with meadows and fields are characterizing much of the modern landscape, including the excavated fields. The western part was prior to modern farming covered by open heathlands and wetlands, where the two sites Stormyra and Ryggamyra are found, whereas low mountains are found in the central and eastern part, also separating the large lake Eidsvatnet from the study area in the west. Eidsvatnet is surrounded by agricultural land and mixed pine forests which are increasingly dominating the vegetation toward the east.
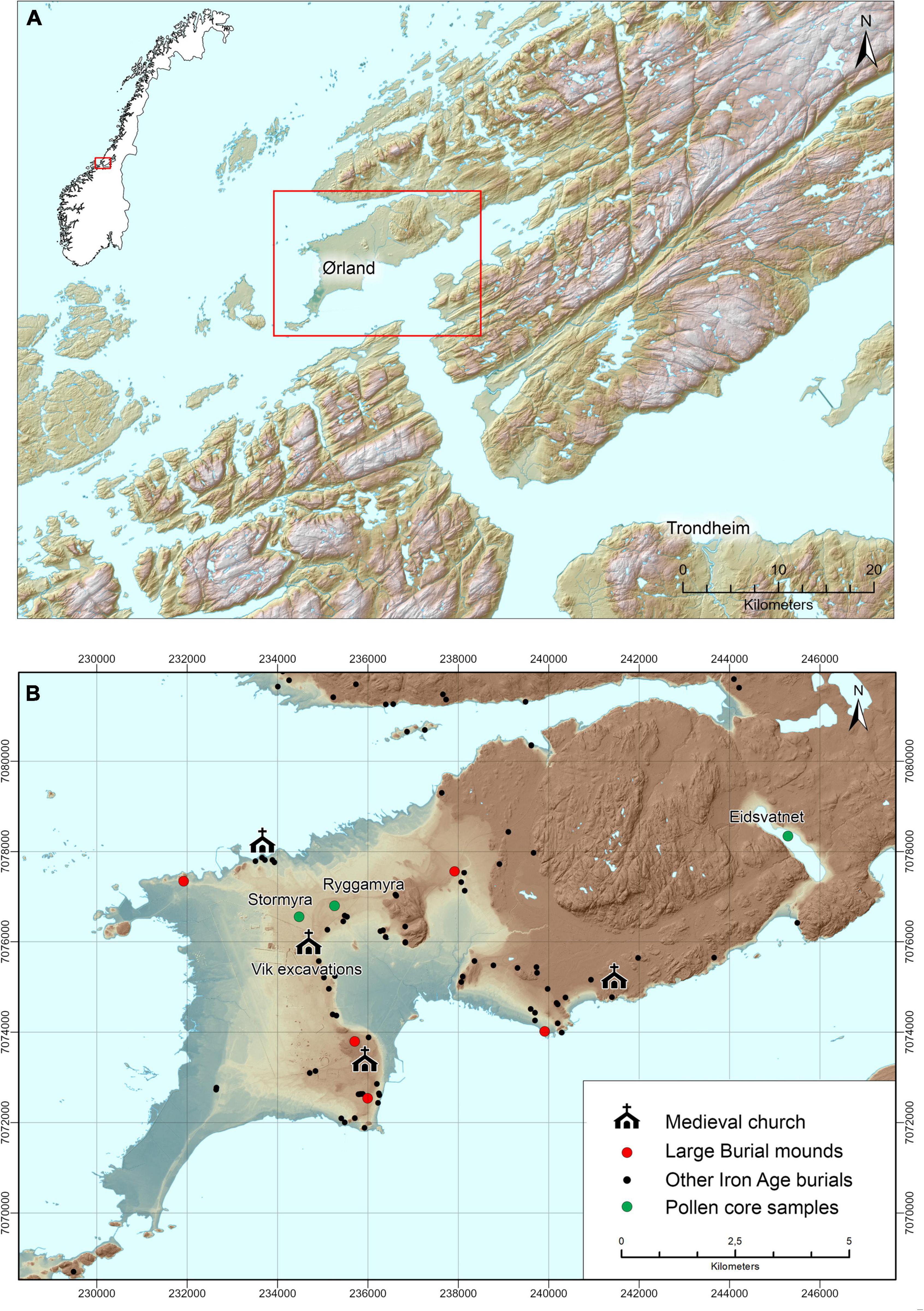
Figure 1. Map of study area. (A) The peninsula with Ørland and its surroundings, (B) detailed map showing the localities for pollen analysis in relation to large burial mounds, Iron Age graves and medieval churches. Illustration: Magnar Mojaren Gran, NTNU University Museum.
The study area is located within the south boreal vegetation zone and has an oceanic climate with mean winter temperature −2°C (January), mean summer temperature 14°C (July) and yearly precipitation 1,000–1,500 mm (Moen, 1999).
In this low-lying and flat landscape, postglacial land uplift has resulted in significant changes in available land for settlements up until medieval time, and still does although slowly, as revealed through investigations of local changes in relative sea level (Romundset and Lakeman, 2019). This development has not only affected the available land, but also the potential harbor area through time. The archaeological excavated area is located within the agricultural land on marine sediments at Vik, c. 11 m asl. and covers 117,000 m2. The distance from the excavation area at Vik to Stormyra, Ryggamyra, and Eidsvatnet, is c. 1, 1.5, and 10.5 km, respectively. The excavations revealed eight concentrations of Iron Age and early medieval settlements (Ystgaard et al., 2019), nine settlement phases were identified, and the importance of the area in these periods is seen also through a high number of burial mounds and four medieval churches in the wider study area (Figure 1B). Based on results from coring of isolation lakes at Ørland peninsula and its surroundings (Romundset and Lakeman, 2019), compared with minimum-limiting dates from archaeological sites, a new shoreline displacement curve (Figure 2A) and reconstructions of the landscape development have been made (Figure 2B). These show the available land during the Iron Age and early medieval settlement phases identified at Vik; the time also covered by the pollen diagrams.
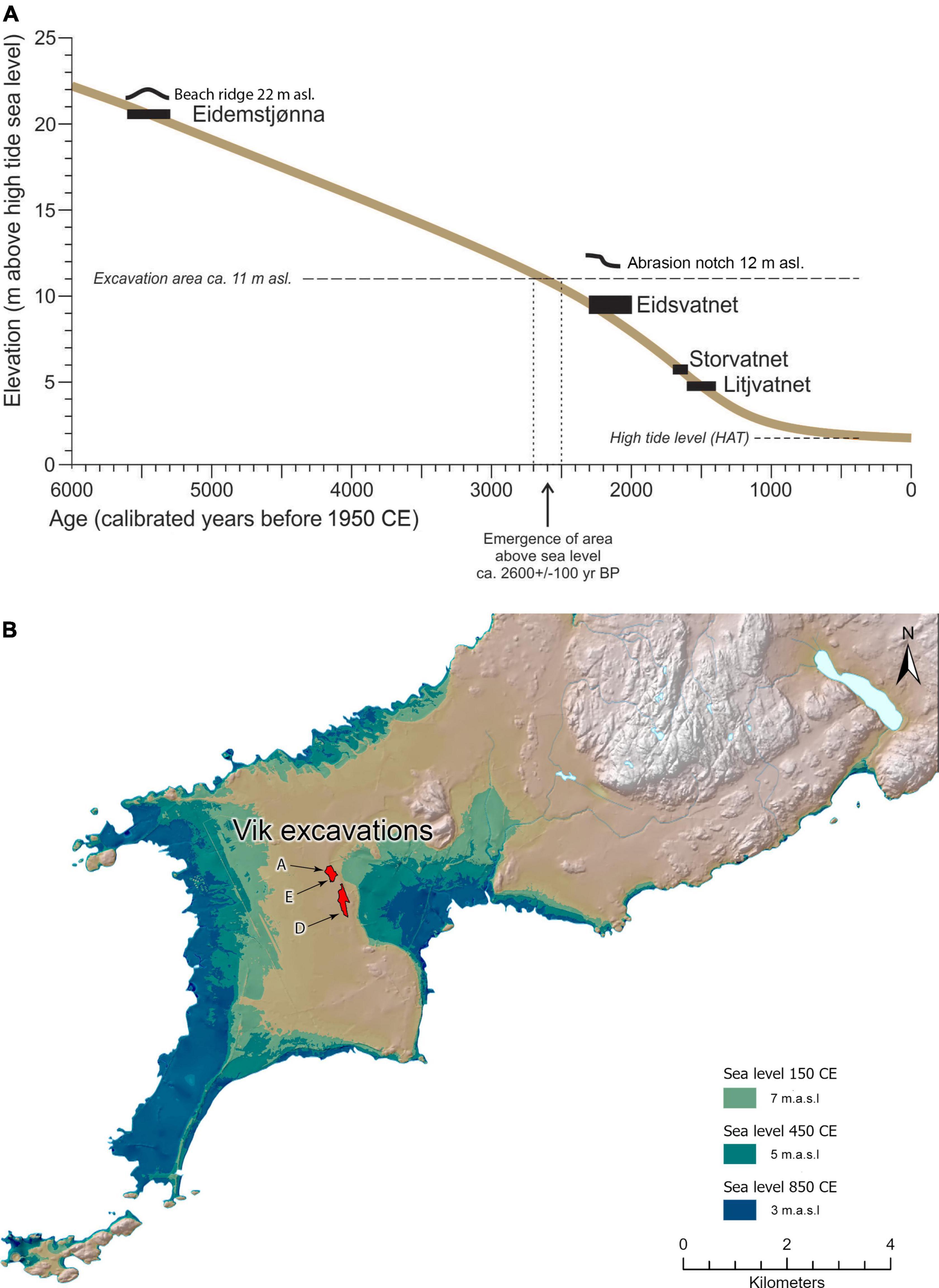
Figure 2. Shoreline changes at Ørland through time. (A) The new shoreline displacement curve for the period 6,000 calibrated years BP to present, (B) the shoreline at Ørland in different settlement phases, with the excavated areas at Vik marked. In-context pollen samples are analyzed from areas A, D, and E. Illustration: Magnar Mojaren Gran, NTNU University Museum.
Pollen data
Three sites are investigated (Figure 1B): the lake Eidsvatnet (63.7388N 9.8375E, 64 ha) and the two bogs Stormyra (63.71558N 9.62232E, 78 ha) and Ryggamyra (63.7185N 9.6355E, 4 ha). Additionally, some pollen samples collected from dated archaeological contexts at Vik (soil profiles and water holes, Figure 2B and Supplementary Figure 1), are included. Field and laboratory methods are described in Overland and Hjelle (2019). Compared to the 2019 publication, one diagram from a large bog, Stormyra, has been analyzed, and analysis of additional samples from Eidsvatnet and Ryggamyra has been carried out. This has resulted in 42 pollen samples from Eidsvatnet (sum pollen 704–1,210, mean 1,041; sum pollen and spores 831–1,341, mean 1,215), 32 from Ryggamyra (sum pollen 107–1058, mean 556; sum pollen and spores 109–1,069, mean 577) and 28 samples from Stormyra (sum pollen 421–1,190, mean 758; sum pollen and spores 437–1,198, mean 796). The three pollen diagrams are zoned based on CONISS cluster analysis (Grimm, 1987) in the Tilia program (Grimm, 2019). The pollen samples from archaeological contexts—agricultural layers, water holes, wells, refuse pits—reflect accumulation within a certain period. These features have, either by radiocarbon dates or stratigraphic relationships, been related to the identified Vik settlement phases based on archaeological data. In several pollen samples the preservation is bad, resulting in large variations in the pollen sums (sum pollen 102–1,152, mean 651; sum pollen and spores 113–1,170, mean 669).
18 radiocarbon dates based on macroscopic plant and animal remains from the three cores (Eidsvatnet 9, Stormyra 4, Ryggamyra 5) were calibrated using Clam age-depth modeling and the IntCal20. 14C calibration curve (Blaauw, 2010; Reimer et al., 2020). The best age estimated make the basis for vegetation reconstructions in 100-year time intervals and comparisons with the summed probability distribution of radiocarbon dates from archaeological contexts.
Pollen diversity through time was investigated by palynological richness, using Hill (1973) number N0 (Birks et al., 2016b; Felde et al., 2018). This estimated number is based on rarefaction, which is the minimum pollen sum for each data set (Birks and Line, 1992). The aim of the investigation was to identify trends over time for each site, and each data set was therefore analyzed separately, based on the lowest sum of pollen and spores for the respective data set. Samples from the same archaeological contexts were combined prior to this analysis. To compare palynological richness during the Vik settlement periods, pollen data from archaeological contexts (individual samples), bogs and lakes were also analyzed using the same sum (n = 109). Additionally, the samples from each of the three sites were combined into 100-year time intervals, to make direct comparisons between the palynological richness, land-cover estimates and summed probability distribution (SPD) of radiocarbon dates. The lowest pollen sum, 406, was used for these analyses. The analysis was carried out in R version 4.1.1 R Core Team, 2021. Also, a DCA (Detrended Correspondence Analysis) was carried out to identify the main gradients in the pollen data. Pollen and spores from terrestrial and vascular plants were included in the analysis.
The Landscape Reconstruction Algorithm (LRA), consisting of two sub-models, was used to reconstruct regional (Sugita, 2007a) and local (Sugita, 2007b) vegetation cover within 100-year time intervals. In REVEALS (Regional Estimates of VEgetation Abundance from Large Sites) the regional vegetation within a radius of 50 km was estimated based on the pollen data from Eidsvatnet. For local vegetation reconstruction, estimates of regional vegetation is needed to be able to differ between regional and local pollen deposition. As Eidsvatnet is found next to pine forests on the eastern part of the peninsula and Stormyra is situated on the open western part, the regional vegetation cover used as input parameter in LOVE (LOcal Vegetation Estimates), was based on pollen data from both Eidsvatnet and Stormyra. When subtracting the background pollen in LOVE, the local vegetation within 1,500 m around Ryggamyra was estimated (see Overland and Hjelle, 2019 for more details). This area also includes the archaeological excavated areas.
22 taxa, contributing to 71–100% of the pollen sums (Eidsvatnet 91–98%, mean 96%; Ryggamyra 76–100%, mean 91%; Stormyra (71–99%, mean 94%), were included in the reconstructions. When testing the Landscape Reconstruction Algorithm in western Norway, the pollen productivity estimates obtained from that region (Hjelle and Sugita, 2012) gave improved estimates of vegetation cover when linked to maps of actual vegetation, compared to using mean values from Europe (Hjelle et al., 2015). We propose that the same is the case for our study region and have used the Norwegian estimates when available. In other cases, mean values from Europe (Mazier et al., 2012) were applied (Table 1).
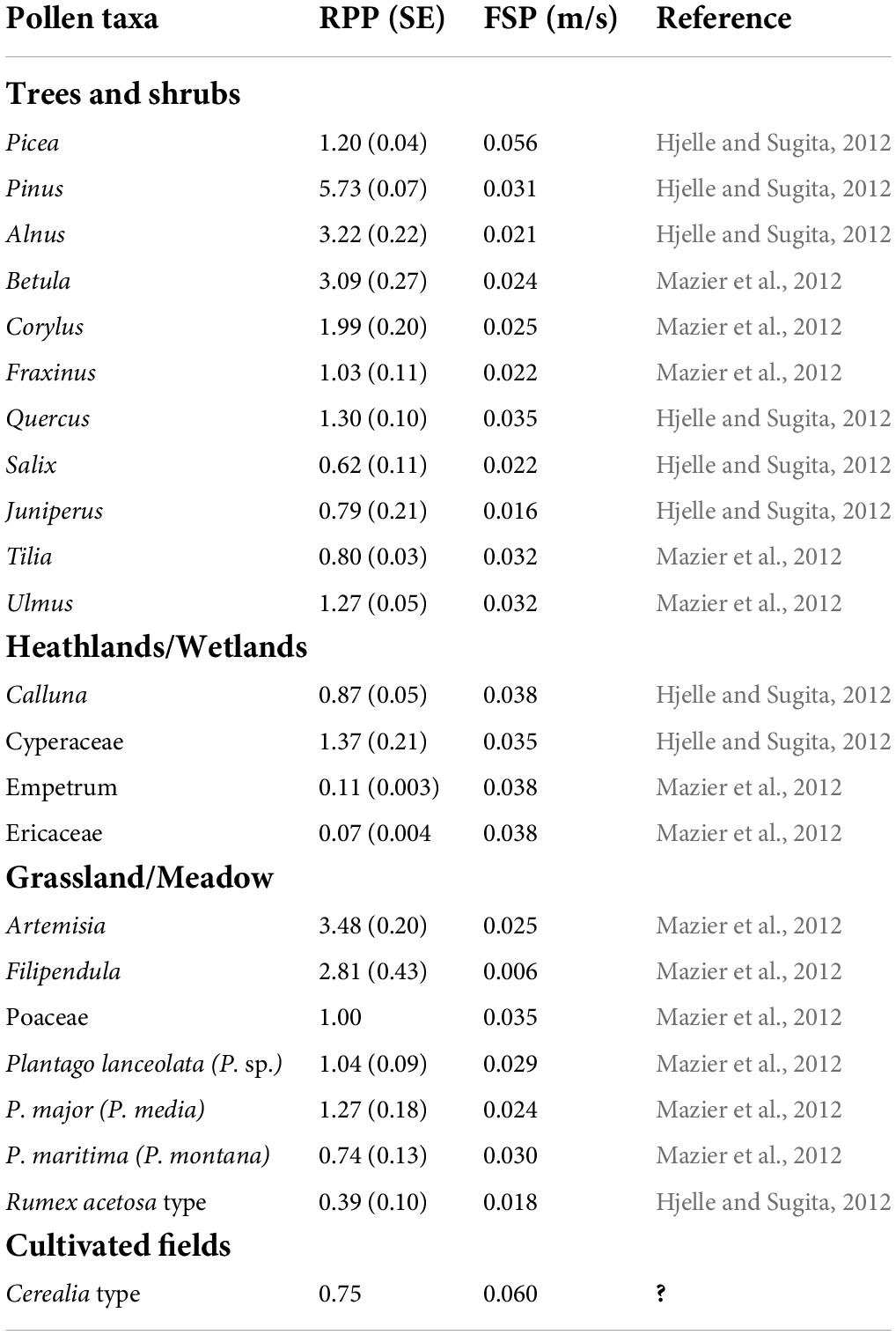
Table 1. Relative pollen productivity (RPP) and their standard errors, fall speed of pollen (FSP), and taxa included in the four land-cover categories.
Archaeological data
Excavations at Vik revealed a dynamic settlement landscape, with settlement emerging as soon as the land rose from the sea and became habitable c. 600 BCE. Seven farms were established, inhabited, and deserted between 500 BCE and 1250 CE. The farm economy was based on mixed farming, combining animal husbandry and crop cultivation with fishing, and foraging for seashells in the nearby sea and along the seashore (Mokkelbost, 2019; Storå et al., 2019). The number of buildings and other features such as waste deposits and cooking pits indicate intensive settlement and high activity c. 400 BCE–350 CE, followed by reduced activity and an almost complete decline between c. 550 CE and 900, and a new settlement establishment phase c. 900–1250 CE, followed by a decline toward the end of the thirteenth century (Ystgaard et al., 2019). Interpretational frames for the fluctuations in activity may be found in over-regional economic and political circumstances (Solberg, 2000; Hedeager, 2008), in supra-regional climatic events (Büntgen et al., 2016; Sejrup et al., 2016; Stamnes, 2016), and in the local transformation of the landscape following the postglacial land uplift (Romundset and Lakeman, 2019), including also local vegetation/habitat changes.
In the present paper, the distribution of 14C dates from archaeological contexts is used as an indicator of settlement intensity, and 621 radiocarbon dates are used in a summed probability distribution (SPD) and kernel density estimate (KDE) to give an indication of time periods of high and low activity (Bronk Ramsey, 2017).
Sampling for 14C dating was highly prioritized, and samples were collected to date and chronologically assess structures such as post holes and cooking pits, contexts such as buildings and waste deposits, and environments such as farmsteads. Well preserved archaeological contexts with as low risk for contamination as possible were prioritized for sampling. Charcoal from species with low own age, were selected for dating. Dates were calibrated using the IntCal20. 14C calibration curve (Reimer et al., 2020). A summed probability distribution and kernel density estimate was created according to Bronk Ramsey (2017). Sources of error connected to the sampling, dating, and summing of the radiocarbon dates relate to taphonomic processes, prehistoric practices, sampling practices, and the calibration curve. The excavated area had been subject to plowing both in prehistoric and modern times, leaving only the lower parts of earth-dug construction parts of cooking pits and buildings. Cooking pits and buildings are parts of cultural practices that change over time. Cooking pits were most widely used in the Iron Age up until the sixth century and indicate feasting practices more than they reflect relative population size (Gundersen et al., 2020). Prehistoric building practice in Scandinavia included earth-dug, roof-carrying constructions during the Bronze and Iron Ages, while new construction principles where roof-carrying constructions rested on foundations above ground were introduced from the sixth century. At approximately the same time, farmsteads were re-located to the sites of present farmsteads. This correlates with the abandonment of the practice of using open-air cooking-pits (Grønnesby, 2016; Sauvage and Mokkelbost, 2016; Gundersen et al., 2020; Løken, 2020). The relation between new building techniques, re-location of settlement, the abandonment of cooking pits and a potential depopulation in the fifth and sixth centuries is a matter of discussion. The excavation focused on settlement prior to 1537 CE, according to regulations in the Norwegian Cultural Heritage Act. Therefore, activities post-dating 1537 CE are not represented in the archaeological dataset. Several of the prehistoric dates fall within the plateau in the calibration curve 800–400 BCE, which hinders a nuanced chronology within this age period (van der Plicht, 2004).
Results
Chronology
The age-depth models indicate a relatively constant sedimentation in Eidsvatnet with estimated ages of 25–108 years between analyzed samples (Figure 3). Also, the accumulation rate in Stormyra is relatively constant with 24–92 years between analyzed samples. In Ryggamyra, there is a marked increase in organic matter at 149 cm (c. 200 BCE), following the change from sedimentation in water to peat formation. This may result in some uncertainties in dates given for samples correlated to Vik settlement phase 2 or 3. Estimated sample ages at Ryggamyra give 21–145 years between analyzed samples. Based on radiocarbon dates from archaeological contexts, mainly post-holes and cooking pits, the botanical data are related to six settlement phases identified at Vik, and three later periods in the historical development at Ørland (Ystgaard et al., 2019, p. 30).
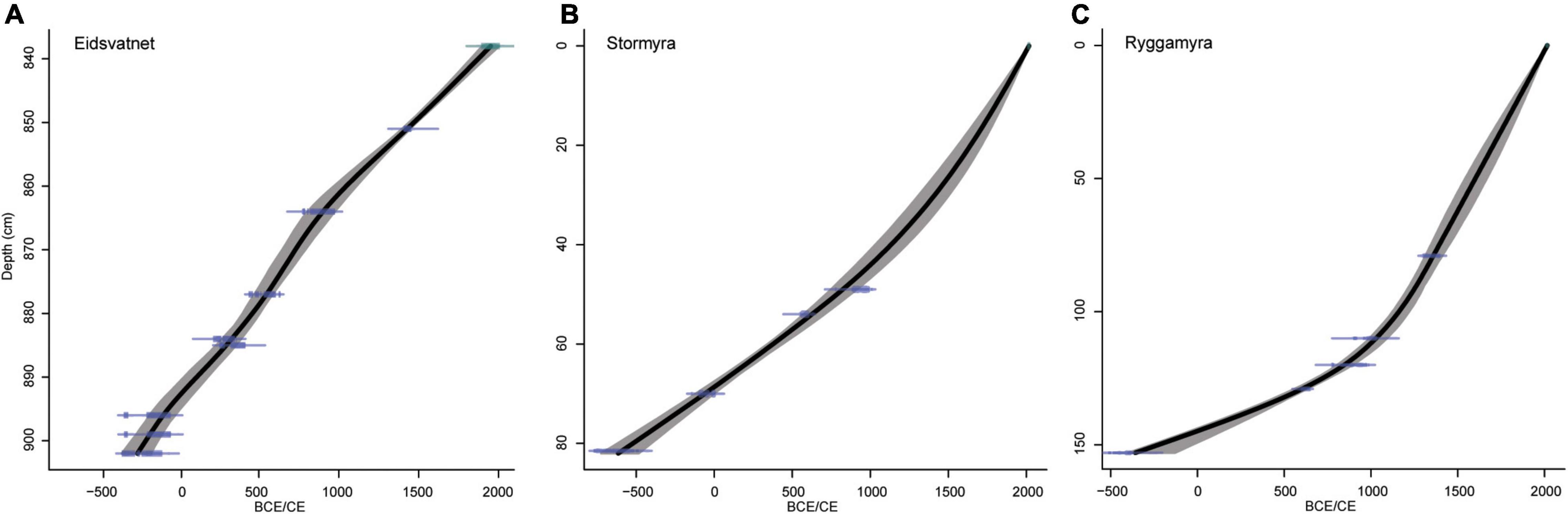
Figure 3. Age-depth models for the three pollen diagrams, (A) Eidsvatnet, (B) Stormyra, (C) Ryggamyra. Smooth spline in CLAM, R-code for classical age-depth modeling version 2.2 (Blaauw, 2010). Calibrations by IntCal20.14C (Reimer et al., 2020), using 95% probability.
Summed probability distribution and kernel density estimate
The summed probability distribution and kernel density estimate of radiocarbon dates show a low, but relatively constant number of dates in phase 1, c. 800–400 BCE (Figure 4). In phase 2, c. 400–50 BCE, the number of dates reach a maximum, followed by a decrease, but still with a high number of dates. The highest number of dates are found in phase 3, the period covering c. 50 BCE–350 CE and especially c. 100–200 CE. Phase 4, c. 350–550 CE, marks a recession phase, but has still quite high number of dates. In phase 5, c. 550–900 CE, and especially around 650 CE, the number of dates is at a minimum. Phase 6, c. 900–1250 CE, reveals a new period with increased number of radiocarbon dates, followed by a decrease toward the end of the thirteenth century.
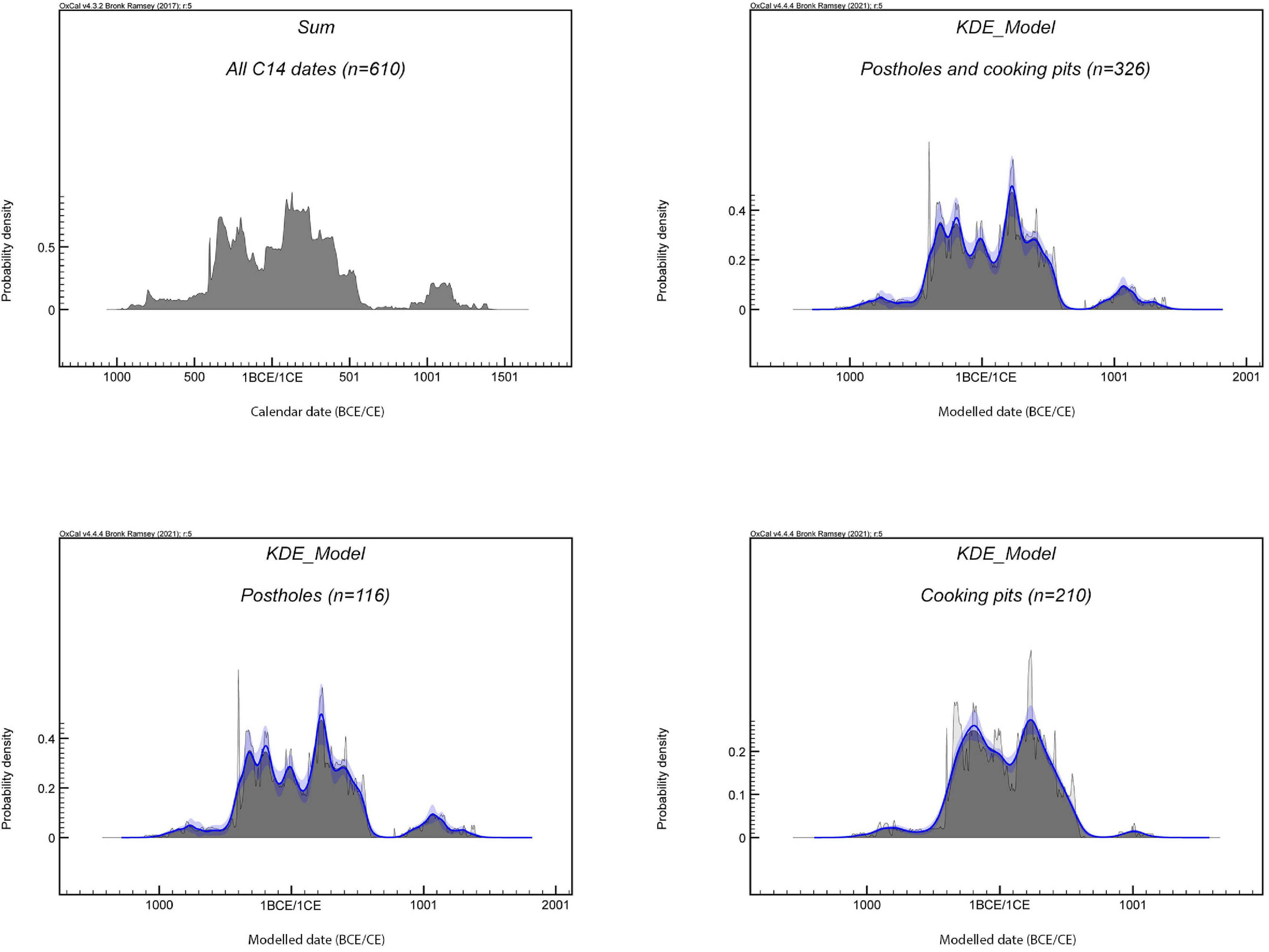
Figure 4. Summed probability distribution (SPD) and Kernel density estimate (KDE) of radiocarbon dates from Vik archaeological excavations.
Pollen and archaeological data
The results of pollen analysis from the three sites (Supplementary Figures 2–4), archaeological contexts (Supplementary Figure 5), and archaeological data are summarized in Table 2. The local pollen assemblage zones (PAZ) are in some cases in accordance with the Vik settlement phases, whereas in other cases changes in vegetation happen independently from the archaeological based zonation at Vik. Below, the results are presented in relation to the settlement phases 1–7.
Phase 1, c. 800–400 BCE (Bronze Age/Pre-Roman Iron Age)
Some settlement traces are found in Vik and the zone is covered by the pollen diagram from Stormyra. Sedge rich wetlands, partly with open water, dominated. Both grazing indicators and cereals are present, indicating the existence of grass-dominated pastures and cultivated fields.
Phase 2, c. 400–50 BCE (Pre-Roman Iron Age)
Farm settlement is documented through buildings and cooking pits. Pollen analysis from agricultural soil reveal (moist) grasslands, cultivated fields and seashore vegetation. The vicinity to the sea as well as a mixture of habitats—wetlands, grasslands and cultivated fields are reflected in Ryggamyra and Stormyra, with increase in grasslands on behalf of deciduous trees/shrubs, dwarf-shrubs and sedges, with time. Eidsvatnet was isolated from the sea during phase 2, and a change from species rich seashore communities to increased influence of the regional forest vegetation is observed. Calluna heathlands existed both locally at Vik, and at the regional level.
Phase 3, c. 50 BCE–350 CE (Pre-Roman/Roman Iron Age)
Settlement concentrations are found in all excavated areas. A mosaic of open vegetation types existed; species rich meadows and fertilized cereal fields dominated the dry ridge, whereas wetlands increased in importance in the outlands north of Vik. Both lime rich grasslands/seashore communities and nutrient-poor heathland/wetlands may have been grazed, and coprophilous fungi indicate presence of animals and probably the use of manure. Hordeum, and probably Cannabis, were cultivated. On a regional level, a first maximum in openness is found at the transition phase 3/phase 4.
Phase 4, c. 350–550 CE (Migration period)
Traces of settlement at Vik is low and the number of charcoal dates are decreasing through the period. Cereal cultivation and a mosaic of vegetation types are still indicated in the pollen data. At Ryggamyra, the highest percentages of sedges are found, and coprophilous fungi indicate grazing activity. Microscopic charcoal has high values both at Ryggamyra and Stormyra, and cultivation of Hordeum is indicated in both pollen diagrams. At a regional level, the high degree of openness and increase in Juniperus, Calluna and grassland taxa may indicate an expansion in the use of outfield areas for grazing.
Phase 5, c. 550–900 CE (Merovingian period/early Viking Age)
No buildings are found at Vik in this period and the number of charcoal dates are at a minimum. Regionally there is a reforestation. In Ryggamyra the values of microscopic charcoal are low, and a succession is initiated from a wetland influenced by agricultural activity to a Betula and Salix shrubland on ombrotrophic peat. In Stormyra there is a slight increase in Betula, a top in Pedicularis and an increase in Sphagnum. There is also a sharp drop in charcoal in the end of the phase, together with a change to more Empetrum dominated heathland at the expense of Calluna, which may be due to a reduction in heathland management.
Phase 6, c. 900–1250 CE (Late Viking Age/early medieval period)
Medieval farm established at Vik, with at least two and possible six houses. Mowing took probably place and there is regional woodland clearance and increase in outfield grazing activity. Ryggamyra sees a reduction in Betula, and increase in dwarf shrubs, particularly in Calluna, and Sphagnum. There is presence of Cerealia and coprophilous fungi, suggesting local outfield grazing, but low levels of charcoal. In Stormyra there is higher values of Calluna and charcoal in the mid part of the phase, then an increase in Betula and a reduction in charcoal in the later part.
Phase 7, c. 1250–1850 CE (High and late medieval period/modern period)
The open landscapes of phase 6 continued into phase 7 until marked changes are observed in the mid-fourteenth century. Regionally the period 1380–1550 CE sees a reforestation probably in the aftermath of the Black Death (1349 CE), followed by new opening-up of the landscape. In Ryggamyra there is an increase in charcoal and coprophilous fungi from c. 1400 CE. Stormyra is a peatland with Cyperaceae and dwarf shrubs.
Palynological richness and gradients in the data
Palynological richness is given individually for the lake, two bog diagrams and for archaeological contexts (Figures 5A–D). To compare palynological richness from the different sites directly, the minimum pollen count of 109 (Ryggamyra) was used (Figure 5E). Estimates based on higher counts give the same pattern but higher estimated richness, as presented in the pollen diagrams from the individual sites. Generally, a higher diversity is estimated at Ryggamyra compared to Stormyra and Eidsvatnet during the settlement phases at Vik, followed by a reduction at Ryggamyra after settlement abandonment around 550 CE reaching a minimum in the ninth century. Stormyra does not show the same connection to the settlement phases. This may be due to the coring point more centrally located in the bog than the coring point at Ryggamyra, resulting in longer distance to and less direct human impact. It may also be that an unknown settlement contemporaneous with the Vik settlement existed at Ryggen, close to Ryggamyra. In contrast to Ryggamyra, the estimated palynological richness at Stormyra increase through settlement phases 1–4 and reaches its maximum, as well as a level comparable to Ryggamyra and Eidsvatnet at the end of phase 4. This indicates that natural bog development was an important driver of diversity changes, but also that decreased human activity in the sixth century, had an effect. A weak decreasing trend is estimated for the last settlement period c. 900–1250 CE. This indicates that it may well be a settlement at Ryggen, simultaneous with the Vik settlement, that is reflected in the estimated diversity and high degree of concordance with SPD at Vik in the Early Iron Age. The estimated palynological richness from Eidsvatnet show less variation than Ryggamyra and Stormyra, with a maximum around 300 CE, 700 CE and from c. 1000–1300 CE when the estimated richness is higher than for the two other sites. This may be connected to the Iron Age and medieval settlements in the larger surroundings of Eidsvatnet (cf. Figure 1B). The highest palynological diversity is estimated for archaeological contexts; a waterhole in area E used as waste pit/latrine, and a waterhole in Field E also containing waste. Both contexts have several pollen sources, reflecting both the utilization of plants and a diverse cultural landscape. The palynological richness from different soil profiles are comparable to the levels in Ryggamyra during the settlement phases.
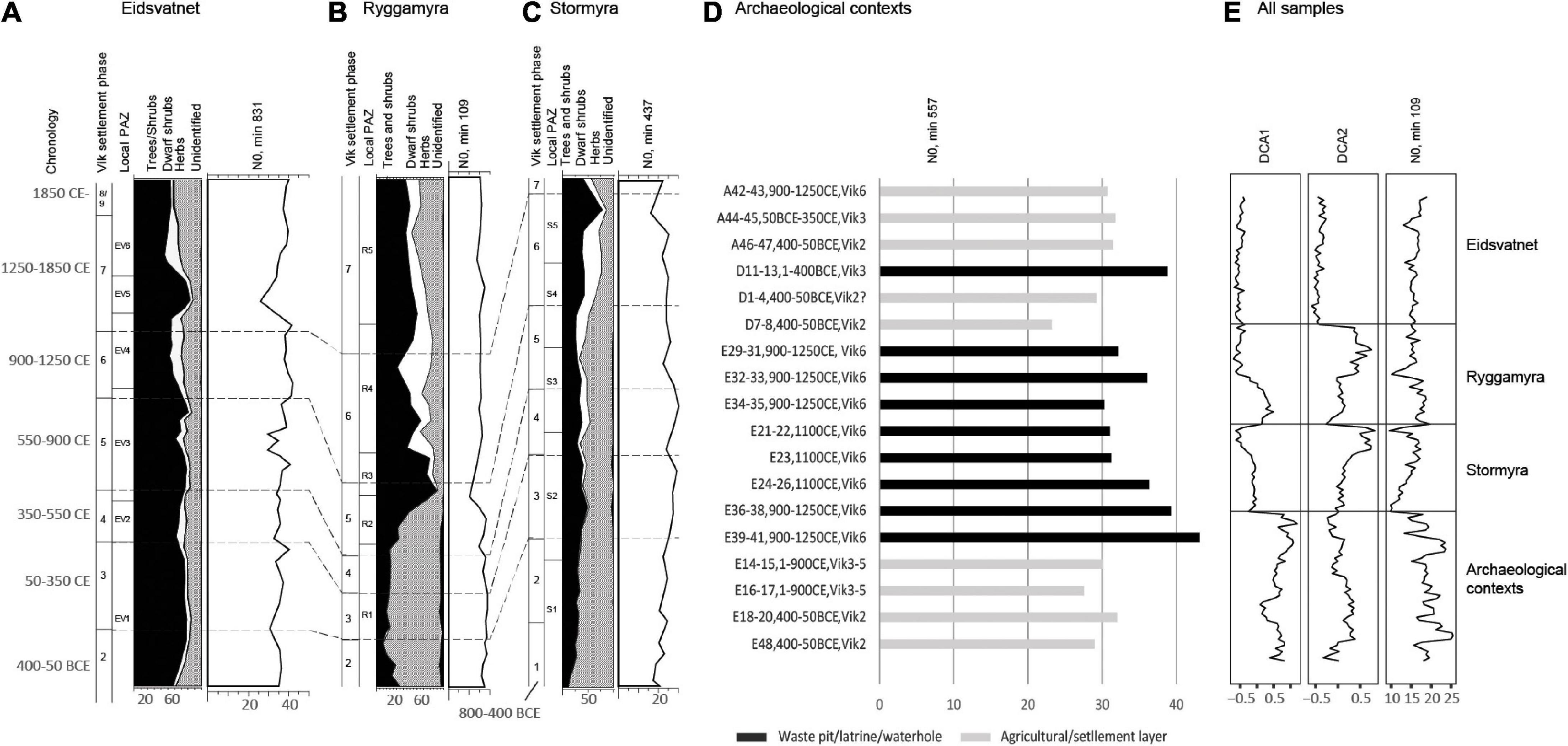
Figure 5. Palynological richness (N0) in relation to settlement phases (A) Eidsvatnet, (B) Stormyra, (C) Ryggamyra, and (D) archaeological contexts. (E) Palynological richness and Detrended Correspondence Analysis (DCA) sample scores for the four datasets treated simultaneously.
The distribution of samples along the first and second DCA axes (Figure 5E), show the complete difference in pollen composition in lake sediments and archaeological contexts. The pollen samples from Eidsvatnet and archaeological contexts are found on different ends of the axes, whereas Ryggamyra and Stormyra are partly overlapping, as well as the samples from the Early Iron Age settlement phase in Ryggamyra and some samples from soil profiles; samples also having comparable palynological richness.
Reconstructions of vegetation cover
The REVEALS-estimated regional vegetation cover based on Eidsvatnet (Figure 6A) differ from the estimates based on Stormyra (Figure 6B). The main difference is the local dominance of Cyperaceae and Vaccinium in the pollen assemblages from Stormyra, resulting in high estimated vegetation cover. Eidsvatnet, with the absence of local plants, have higher representation of tree pollen in all time periods than Stormyra. For reconstruction of the local vegetation based on pollen data from Ryggamyra, geographically closer to Stormyra than to Eidsvatnet, pollen data from both Eidsvatnet and Stormyra were used to estimate the regional vegetation cover (Figure 6C) needed for the model.
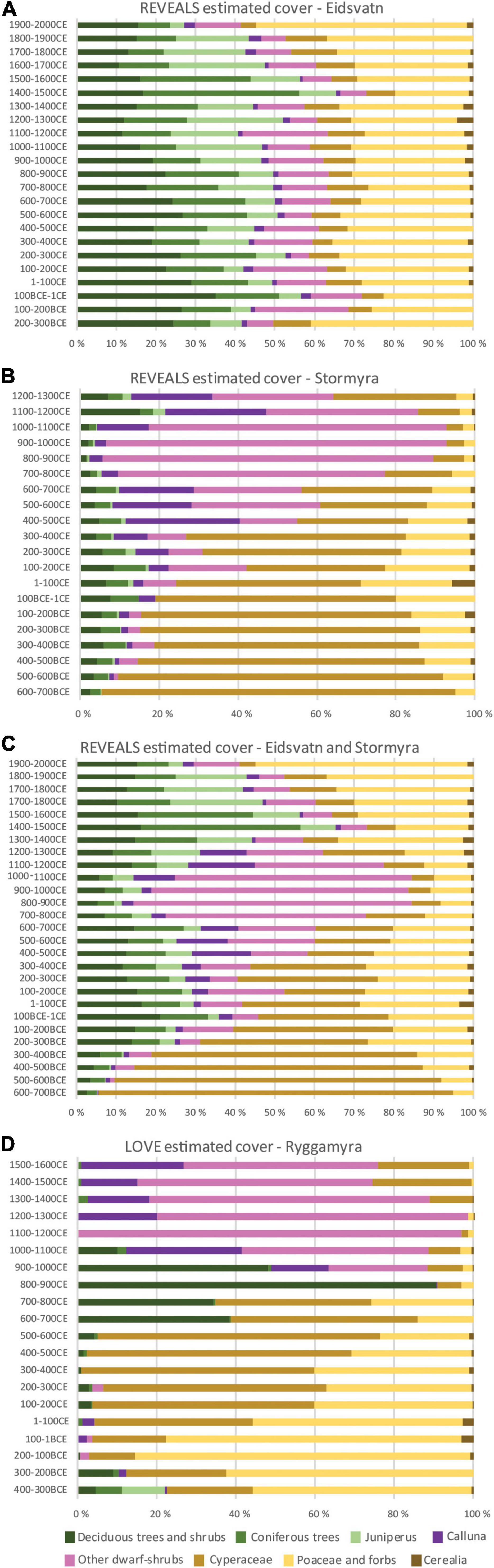
Figure 6. Land cover reconstructions on a regional scale using (A) Eidsvatnet, (B) Stormyra, (C) Eidsvatnet and Stormyra combined, and (D) land cover reconstructions on a local scale. For original pollen data, see Supplementary Figures 2–4.
The reconstruction of vegetation cover on and around Ryggamyra using LRA, indicates c. 20% trees and shrubs 400–300 BCE (phase 2), in a landscape dominated by grasslands. An expansion of open-land communities took place in the following centuries, with maximum cover of grasslands 200–100 BCE. Between 100 BCE and 100 CE (phase 3), maximum cover of cultivated fields is estimated, and an expansion of heathlands is indicated. This reflects a cultural landscape with infields and outfields, with a transition from dryland to more wetland with time (Figure 6D). From c. 5% cover of trees and shrubs 500–600 CE, trees and shrubs reach nearly 40% cover 600–700 CE (phase 5) and contribute to 90% of the land-cover 800–900 CE. Around 900 CE expansion of heathlands starts, reflecting a changed use of the area. Whereas cereal cultivation and meadows characterized the Early Iron Age landscape, coastal heathlands dominated the area from the Late Iron Age onwards.
In contrast to the complete open landscape estimated for Ryggamyra in the Early Iron Age and medieval time, the REVEALS estimated regional vegetation cover indicate presence of woodlands, but with fluctuations through time. Eidsvatnet, reflecting the vegetation cover without over-representation of local taxa, indicate a decrease from nearly 60% cover of trees and shrubs c. 1 CE, to around 40% c. 200 CE (Figure 6A). In this period the cover of both grasslands and cereal fields increased. Reduced openness is indicated 200–300 CE and 500–700 CE, whereas a marked opening of the landscape took place 900–1000 CE and in the following centuries. The most dramatic increase in forest cover took place in the fifteenth century CE, reaching nearly 60%. From the seventeenth century a semi-open landscape (<30% forest cover) with grass dominated meadows and pastures, and heathlands, have existed regionally.
Summed probability distribution in relation to land cover and palynological richness
The period with high number of radiocarbon dates is characterized by maximum cover of meadows, pastures, and cultivated fields (Figure 7A). Concordant with the decrease in SPD c. 550 CE, shrubs and trees quickly expand. The new increase in SPD is followed by expansion of heathlands, whereas a decrease in heathlands and increase in sedge dominated vegetation is indicated after the decrease in SPD in the fourteenth century.
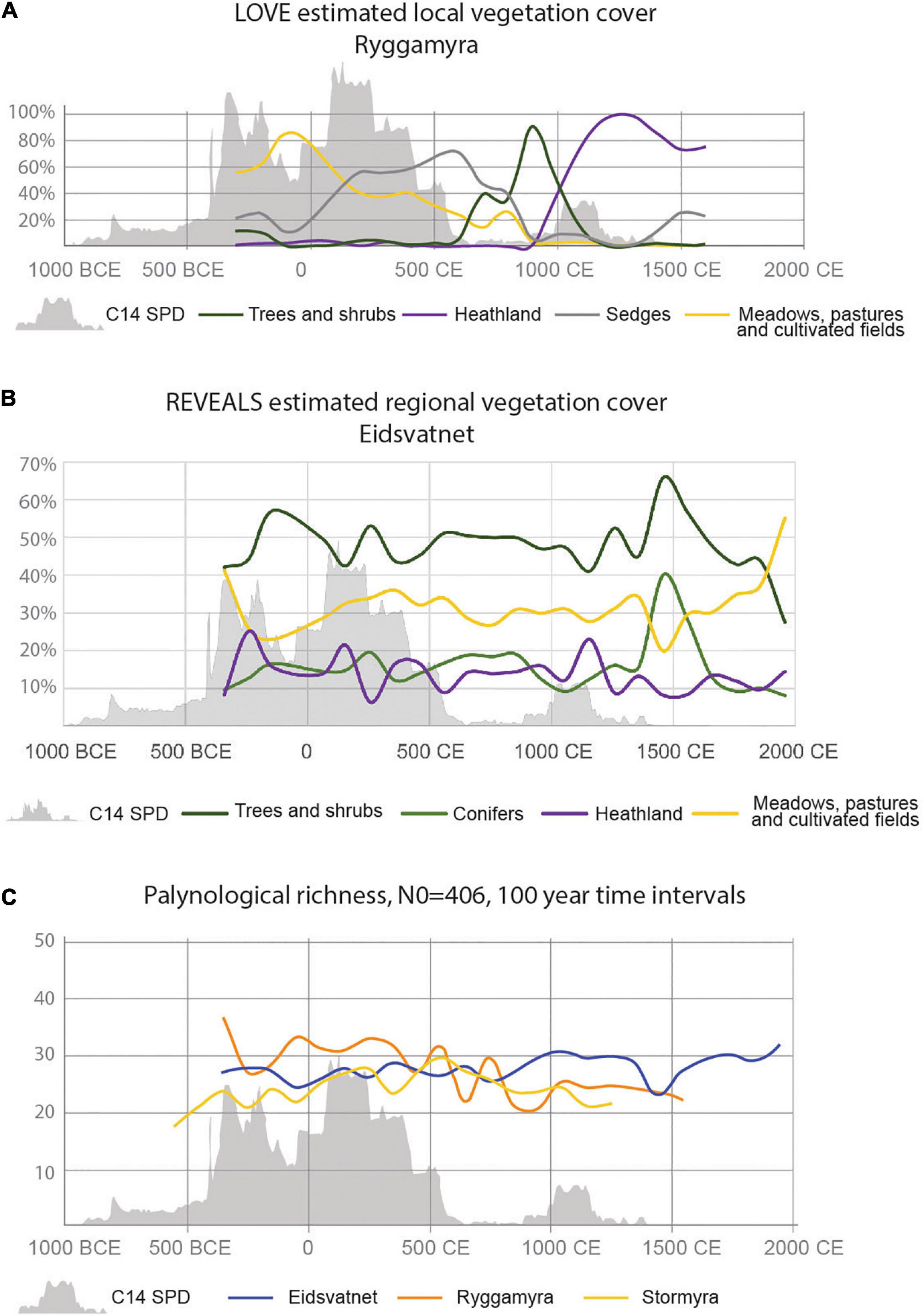
Figure 7. Summed probability distribution (SPD) shown in relation to (A) REVEALS estimated vegetation cover Eidsvatnet, (B) LOVE estimated vegetation cover Ryggamyra, and (C) palynological richness (estimated number) in 100-year time intervals at the different sites.
On a regional level, there is a less clear trend following the development in the SPD from Vik, although trees and shrubs increase in periods with few radiocarbon dates (Figure 7B). The highest cover of grasses and forbs (herbaceous flowering plants that are not grasses or sedges) are found immediately after isolation, c. 300 BCE and reaches a new maximum c. 300 CE. Between c. 600 CE and 900 CE, there are less fluctuations in the vegetation communities than before and after. Meadows, pastures and cultivated fields reach > 30% c. 1350 CE and decrease to 20% cover c. 1450 CE, when a marked increase in conifers take place. A smaller but marked increase in conifer cover is also estimated for the sixth century CE.
The comparison of summed probability distribution of radiocarbon dates and palynological richness at the three sites (Figure 7C), indicate high richness at Ryggamyra and increasing richness at Stormyra during the Early Iron Age settlement period. At Eidsvatnet, the highest richness is observed in the Late Iron Age and medieval time, simultaneous with increase in SPD at Vik and increased settlement in the larger Ørland area.
Discussion
Information gained from different basin types and deposits
The investigation covers the past c. 2,300 years, a time when farming societies were well established in Norway (Solberg, 2000; Diinhoff et al., 2013; Iversen and Petersson (eds), 2016) and to a high degree also shaped the vegetation and landscape in northern Europe (e.g., Odgaard and Rømer (eds), 2009; Kaland, 2014; Marquer et al., 2014, 2017; Fredh et al., 2018; Hjelle et al., 2018; ter Schure et al., 2021). Our results, based on a pollen diagram from one lake, two bogs and different archaeological contexts compared to the summed probability distribution of radiocarbon dates from archaeological contexts, clearly show the local impact of settlements in the Iron Age and early Middle Ages. It demonstrates the different patterns given by data from different basin types and contexts, and the effect of natural processes taking place concordant with the settlement development. The different basins investigated reveal the natural settings; todays open landscape at the western part of the peninsula existed in the Iron Age, probably due to exploitation from the very beginning of dry-land formation that hindered forest development, whereas the eastern part with higher-lying areas had been forested and continued to be so up into modern time. Vicinity to the seashore is reflected in the estimated high cover of meadows/grasslands and high palynological richness following the land-uplift and isolation. With time and with longer distance to the sea, the distance to these species rich communities increased, and distance to human impact as well as types of activity and management became decisive for the vegetation and diversity development.
Estimates of vegetation cover and palynological richness based on the Ryggamyra data reflect the development in the vicinity of the bog and changes in the curves show a high degree of concordance with decrease or increase in number of radiocarbon dates. This conformity with the summed probability distribution/kernel density estimate is in lesser extent found in Eidsvatnet, reflecting the vegetation within a larger area. However, some trends seem to be general and are visible on both spatial scales. These are reflecting a more overall pattern and probably causes of change, e.g., the late medieval crisis (see discussion below). Through the Early Iron Age Vik settlement period, higher palynological richness is estimated from Ryggamyra than from Stormyra and Eidsvatnet, most likely due to short distance to and influence from a contemporaneous settlement at Ryggen, whereas the highest richness in medieval time is estimated for Eidsvatnet. When bog development expanded at Ryggamyra, local vegetation types with relatively low plant diversity developed, resulting in local over-representation in the pollen assemblages and in the estimated cover. In contrast, the lake reflects several vegetation types and activities in the surrounding landscape, without having the local overrepresentation in the pollen assemblages. Since the entomophilous taxa Empetrum and Vaccinium have high values in the two bog diagrams, they were included in the reconstructions. They are, however, overrepresented in the regional and local estimates of vegetation cover (cf. Mazier et al., 2012; Nielsen et al., 2012; Fyfe et al., 2013; Overland and Hjelle, 2019). On the other hand, by including these, the heterogeneity of the heathland communities, not dominated only by Calluna, is illustrated.
The highest richness estimated is from the waterlogged archaeological contexts, interpreted as secondary use of wells and water holes. These pollen assemblages reflect the vegetation at the site and in the vicinity, but also additional sources of pollen, i.e., the use of different plants and the waste produced through habitation and activity. This shows that a high diversity of plants was exploited. The higher diversity and difference in species composition compared to the soil profiles, indicate that plants growing in vegetation types in some distance to the site were collected. Pollen can also be present within the settlement, and in the agricultural layers, as result of for instance animal dung and manuring. Fertilization of cultivated fields by material brought in from the outfields, may be an additional pollen source for the agricultural layers and contribute to similarities with pollen assemblages from Ryggamyra.
Human activity as main driver of habitat and species diversity
During the Vik settlement period, a mixture of cultivated fields, ruderal communities, grazed and may be also mown meadows, existed in the vicinity of the settlement, as well as wetlands and shrub communities at longer distance. This reflects a marked differentiation in use of landscapes; production in infields and exploitation of outfields to optimize available resources, resulting in a mosaic of vegetation types.
The reconstructed vegetation cover at Ryggamyra indicate between 70 and 40% grasslands and fields during the Vik settlement phases, with a decline toward <20% starting around 400 CE, at a time when there also is a reduction in number of radiocarbon dates. From around 100 CE sedge dominated wetlands became more important in the landscape than grasslands. A marked reduction in radiocarbon dates after 550 CE is probably the direct cause of an increase in trees and shrubs. Pioneer taxa such as Salix and Betula quickly expanded into the abandoned ground. The correlation between radiocarbon data from Vik and palynological data from Ryggamyra suggest that a local population decline took place. Also, the wetlands were overgrown, and the succession resulted in 90% trees and shrub cover prior to the next increase in SPD c. 900 CE. At that time clearance took place and the former shrubland turned into grazed heathlands, a vegetation type that at Vik had its maximum extent during the early and high Middle Ages. Although present along the coast from the introduction of animal husbandry around 6,000 years ago, the management system with year-long grazing, burning, and mowing of heather, expanded in the Iron Age and Middle Ages (Kaland, 1986, 2014; Prøsch-Danielsen and Simonsen, 2000; Hjelle et al., 2009, 2012, 2018), clearly also seen at Frøya (Paus, 1982), an island west of the investigation area at Ørland. An estimated reduction in heathland cover in the fifteenth century probably reflects the medieval crisis, with population reduction following the Black Death, probably resulting in less regular burning and maintenance of the heathlands.
As indicated both from the pollen percentage data and the landscape reconstructions, a high diversity of vegetation types existed in the area until c. 550 CE, when wetlands became dominant. The high habitat diversity is also reflected in high palynological richness/species diversity, which decrease when the number of charcoal dates decrease. The minimum around 900 CE at Ryggamyra may, however, be explained by the influence of a high pollen producer such as Betula in the close vicinity to the investigated site. Several investigations have shown that insect pollinated taxa, and thereby a higher number of herbs, are better represented in records from lakes and bogs in periods of open landscapes than in forested periods (e.g., Odgaard, 1999; Overland and Hjelle, 2009; Meltsov et al., 2013). When the number of charcoal dates again increase, also palynological richness increase and stabilize on a lower level than in the Early Iron Age settlement phase. This indicates lower plant diversity in the Late Iron Age and early Middle Ages heathland vegetation compared to the grasslands and cultivated fields in the Early Iron Age, although some grasslands were present also in the Late Iron Age and early Middle Ages. The high diversity in these, probably mown and grazed meadows, is reflected in the palynological richness estimated from Eidsvatnet.
On a regional level the highest cover of grasses and forbs, >40%, is found around 250 BCE in Eidsvatnet, immediately after isolation from the sea and again in historical time reflecting traditional semi-natural grasslands. The high cover in the Pre-Roman Iron Age probably reflects a combination of seashore meadows and human-induced grasslands at that time. If so, this shows the local impact on the pollen assemblages in a large basin which theoretically covers the vegetation within a radius of c. 50 km (Sugita, 1994, 2007a). The dominance of grassland communities is followed by increase in trees and shrubs and fluctuations in communities through time, but without the close relationship to the SPD from the excavation area at Vik; high cover of grasslands is in Eidsvatnet estimated also for periods with low SPD at Vik. On the other hand, the large number of Iron Age burial mounds and four medieval churches in the region reflects well-developed societies which influenced on the vegetation. The importance of these settlements is also visible in the estimated palynological richness. The Late Iron Age and early/high Middle Ages are periods with high diversity reflecting cultivation, mown meadows, outfield pastures including both grasslands and heathlands, as well as more natural communities such as seashore meadows and woodlands. Semi-natural grasslands are one of those habitats depending on grazing, as well as the positive effect of long-time use (Reitalu et al., 2010; Natlandsmyr and Hjelle, 2016). This indicates that human activity was the main driver of biodiversity and habitat diversity both on a local and a regional scale. The results from Vik in the Early Iron Age and Eidsvatnet in the Late Iron Age and Middle Ages, indicate the high diversity in the traditionally managed infield/outfield vegetation communities. Compared to these, the managed heathlands have lower diversity. The increase in heathlands at Vik from 900 CE, and the increase in both grasslands and juniper-rich outfields regionally, both reflect the importance of grazing and the need for fodder. This indicates the value of animal husbandry in the economy. At the same time the estimated cover of cultivated fields also shows the importance of cereal cultivation in the economy.
Crises in the society; vegetation changes from the sixth to the fourteenth centuries
In general, in Norway re-location of settlements and population decrease starts at the end of the fourth century due to a combination of economic, political, climatic stress or the Justinian plague, ending in a marked change in the archaeological data in the sixth century (Solberg, 2000, p. 197; Myhre, 2002; Gjerpe, 2021). Alternative explanations are volcanic eruptions around 536–540 CE, resulting in a dust veil and colder climate, that could be the origin of the myth of the “Fimbul winter”—years with no summer—in the Nordic Mythology (Gräslund and Price, 2012; Büntgen et al., 2016; Nordvig and Riede, 2018). A co-effect from cultural transition and environmental crisis is expected to have taken place, both forming a transition of the settlement pattern (Gundersen, 2019). At Ørland, the period is visible in the archaeological record as abandonment of the excavation areas at Vik; no house remains could be dated to this period and the SPD/KDE curves show low values, which together with the palynological data suggest that a local population decline took place. In the fifth to sixth century Vik, isostatic uplift combined with the very flat local landscape had turned the earlier bay into wetlands. The result was abolition of the settlement’s harbor. When the climate deterioration following the 536 and 540 CE events, struck, Ørland societies were already under pressure, and the climatic events may have acted as a final tipping point for site abandonment (cf. Solheim and Iversen, 2019; Gjerpe, 2021).
Another aspect, the changes in local settlement pattern at Vik may have forced changes in land use practices in later time periods. Reduced human impact following c. 536 CE, in combination with more long-term sea level changes, may have had a pronounced effect on the local hydrological situation, influencing the trophic status of wetlands. This is visible in both Ryggamyra and Stormyra, but most pronounced in Ryggamyra which underwent a succession from minerotrophic wetland to raised bog following the abandonment phase. In Stormyra the period marks a change in vegetation from Cyperaceae rich wetland and Calluna heathland, to more Empetrum and Vaccinium and nutrient poor peat with Pedicularis and Sphagnum. Pollen data, with LOVE estimated vegetation cover, in Ryggamyra suggests that the early Iron Age settlement made use of infield meadows and pastures (grasslands), whereas in the medieval period the settlement relied on heathlands. This change in local agricultural potentials may have been caused by a combination of significant local isostatic uplift making the area Vik landlocked, and increased peat development on soils of poor drainage, that partly could be a response to local abandonment. With settlement abandonment at Vik, and possibly also other places at Ørland, the previous infields turned into successional stages toward forests. Also the regional estimates of vegetation cover indicate an increase in shrubs and pioneer trees followed by increase in coniferous forests.
After dominance of grasslands and cultivated land on a regional level from the tenth to the fourteenth century, there is another abandonment phase in the late medieval period, that has larger effect regionally. An abrupt decrease in meadows and pastures is followed by increase in deciduous trees and especially conifers in the fourteenth/fifteenth centuries, reflecting the climate deterioration (Sejrup et al., 2016) and late medieval crises in the society, (Svensson, 2019) with a marked reforestation following the Black Death in 1349 (Thun and Svarva, 2018). It was not until around 1600 CE that the open land again became dominant.
Conclusion
The combination of palynological and archaeological data indicate a significant human impact on long-term vegetation and biodiversity changes in Ørland. Fluctuations in human and livestock populations correlate to fluctuations in vegetation and biodiversity in many respects.
Archaeological and palynological data indicate a decline in human and livestock populations in the sixth century on both a local scale in Vik and on a regional scale. In the fifth to sixth century a correlation between radiocarbon data (SPD) from Vik and palynological data from Ryggamyra suggest that a local population decline did take place. At the same time isostatic uplift led to the abolition of the settlement’s harbor, suggesting that when the climate deterioration following the 536 CE and 540 events struck, Ørland societies were clearly already under pressure. In the late Middle Ages, a marked forest regeneration took place on a regional scale. Reasons behind these population declines appear as combinations of over-regional societal changes, local geographical changes, as well as supra-regional climatic events, however in different cultural historical settings, as well as geographical/vegetational settings.
Species diversity, shown as estimated palynological richness, on a local level follow the SPD in general. In the Early Iron Age, the species diversity estimated from bog diagrams is at its maximum connected to settlement with infields (meadows, pastures, cultivated fields) and short distance to seashore communities. Low SPD, settlement abandonment and decreasing species diversity is associated with local trees and shrub vegetation. The increase in SPD in the Late Iron Age/medieval time, associated with one farm settlement and heathland management, gave increased species diversity, but to a lower level than in the Early Iron Age. However, the highest species diversity estimated from our data, is the Late Iron Age/medieval water hole at the farm settlement. This reflects high local activity and several pollen sources.
On a regional level, the trend is quite constant species diversity until the Late Iron Age when it increases and reach a maximum around 900 CE. The species diversity remains high until the most pronounced reduction around 1400 CE. Two centuries later, the species diversity reaches its previous high level. Our SPD data reflects local settlement, whereas species diversity estimated from the lake reflect human activity on a regional scale. However, periods with high diversity are connected to a diverse cultural landscape.
From the regional estimated species diversity and estimated forest cover, the late medieval crises are more profound than the 536 CE event.
Although postglacial land uplift and climate changes have influenced settlement, habitat types and food production, human activity has had a final decisive role for the floristic diversity and landscape development.
Data availability statement
The original contributions presented in this study are included in the article/Supplementary Material, further inquiries can be directed to the corresponding author.
Author contributions
KH: developing idea, pollen data, data handling, computing, first draft of manuscript, and writing. AO: pollen data, diagrams, discussions, and writing. MG: radiocarbon data, computing, discussions, and figures. AR: shoreline data and editing. IY: archaeological data, discussions, writing, and leader of Ørland excavation project. All authors contributed to the article and approved the submitted version.
Funding
The excavations, fieldwork, charcoal analysis, and pollen analysis by AO presented in this manuscript were funded by the Norwegian Defence Estate Agency. A workshop devoted to refining the results was supported by the Department of Archaeology and Cultural History at the NTNU University Museum. Open access publication was funded by the University of Bergen.
Conflict of interest
The authors declare that the research was conducted in the absence of any commercial or financial relationships that could be construed as a potential conflict of interest.
Publisher’s note
All claims expressed in this article are solely those of the authors and do not necessarily represent those of their affiliated organizations, or those of the publisher, the editors and the reviewers. Any product that may be evaluated in this article, or claim that may be made by its manufacturer, is not guaranteed or endorsed by the publisher.
Acknowledgments
We are grateful to Vivian A. Felde for providing codes for diversity estimates and ordinations in R, to Shinya Sugita for providing unpublished programs, to Beate Helle for improving several figures, and to two reviewers for valuable comments.
Supplementary material
The Supplementary Material for this article can be found online at: https://www.frontiersin.org/articles/10.3389/fevo.2022.911780/full#supplementary-material
SUPPLEMENTARY FIGURE 1 | Profile drawings for analyzed pollen samples from archaeological contexts. D and E followed by a number refer to the excavation areas at Vik (Figure 2B).
SUPPLEMENTARY FIGURE 2 | Percentage pollen diagram Eidsvatnet.
SUPPLEMENTARY FIGURE 3 | Percentage pollen diagram Stormyra.
SUPPLEMENTARY FIGURE 4 | Percentage pollen diagram Ryggamyra.
SUPPLEMENTARY FIGURE 5 | Pollen samples from archaeological contexts. (A) Samples from agricultural layers in field A (see Figure 2B), (B) samples from field D (see Supplementary Figure 1 for information on context), and (C) samples from field E (see Supplementary Figure 1 for information on context).
References
Alenius, T., Marquer, L., Molinari, C., Heikkilä, M., and Ojala, A. (2021). The environment they lived in: anthropogenic changes in local and regional composition in eastern Fennoscandia during the Neolithic. Veg. Hist. Archaeobot. 30, 489–506.
Berglund, B. E., Persson, T., and Björkman, L. (2008). Late Quaternary landscape and vegetation diversity in a North European perspective. Quat. Int. 184, 187–194. doi: 10.1016/j.quaint.2007.09.018
Bergsvik, K. A., Darmark, K., Hjelle, K. L., Aksdal, J., and Åstveit, L. I. (2021). Demographic developments in Stone Age coastal western Norway by proxy of radiocarbon dates, stray finds and palynological data. Quat. Sci. Rev. 259, 1–18.
Birks, H. J. B., and Line, J. M. (1992). The use of rarefaction analysis for estimating palynological richness from Quaternary pollen-analytical data. Holocene 2, 1–10. doi: 10.1177/095968369200200101
Birks, H. J. B., Felde, V. A., and Seddon, A. W. (2016a). Biodiversit trends within the Holocene. Holocene 26, 994–1001. doi: 10.1177/0959683615622568
Birks, H. J. B., Felde, V. A., Bjune, A. E., Grytnes, J.-A., Seppä, H., and Giesecke, T. (2016b). Does pollen-assemblage richness reflect floristic richness? A review of recent developments and future challenges. Rev. Palaeobot. Palynol. 228, 1–25. doi: 10.1016/j.revpalbo.2015.12.011
Blaauw, M. (2010). Methods and code for ‘classical’ age-modelling of radiocarbon sequences. Quat. Geochronol. 5, 512–518.
Brondizio, E. S., Settele, J., Díaz, S., and Ngo, T. (eds) (2019). IPBES: Global assessment report on biodiversity and ecosystem services of the Intergovernmental Science-Policy Platform on Biodiversity and Ecosystem Services. Bonn: IPBES secretariat, 1148. doi: 10.5281/zenodo.3831673
Bronk Ramsey, C. (2017). Methods for Summarizing Radiocarbon Datasets. Radiocarbon 59, 1809–1833. doi: 10.1017/RDC.2017.108
Büntgen, U., Myglan, V. S., Ljungqvist, F. C., McCormick, M., Di Cosmo, N., Sigl, M., et al. (2016). Cooling and societal change during the Late Antique Little Ice Age from 536 to around 660 AD. Nat. Geosci. 9, 231–236. doi: 10.1038/NGEO2652
Diinhoff, S., Ramstad, M., and Slinning, T. (eds) (2013). Jordbruksbosetningens utvikling på Vestlandet. Kunnskapsstatus, presentasjon av nye resultater og fremtidige problemstillinger. UBAS – Universitetet i Bergen Arkeologiske Skrifter 7. Bergen: Universitetet i Bergen.
Emanuelsson, U. (2009). The Rural Landscape of Europe: how Man has Shaped European Nature. Stockholm: The Swedish Research Council Formas.
Felde, V. A., Grytnes, J. A., Bjune, A. E., Peglar, S. M., and Birks, H. J. B. (2018). Are diversity trends in western Scandinavia influenced by post-glacial dispersal limitations? J. Veg. Sci. 29, 360–370. doi: 10.1111/jvs.12569
Fredh, D., Mazier, F., Bragée, P., Lagerås, P., Rundgren, M., Hammarlund, D., et al. (2017). The effect of local land-use changes on floristic diversity during the past 1000 years in southern Sweden. Holocene 27, 694–711. doi: 10.1177/0959683616670464
Fredh, E. D., Prøsch-Danielsen, L., and Jensen, C. E. (2018). A synthesis of pollen composition in prehistoric cultication layers in Southwestern Norway. Environ. Archaeol. 27, 127–145. doi: 10.1080/14614103.2018.1536499
Fyfe, R., Twiddle, C. L., Sugita, S., Gaillard, M.-J., Barratt, P., Caseldine, C. J., et al. (2013). The Holocene vegetation cover of Britain and Ireland: overcoming problems of scale and discerning patterns of openness. Quat. Sci. Rev. 73, 132–148. doi: 10.1016/j.quascirev.2013.05.014
Gaillard, M.-J., Sugita, S., Mazier, F., Trondman, A.-K., Broström, A., Hickler, T., et al. (2010). Holocene land-cover reconstructions for studies on land cover– climate feedbacks. Clim. Past 6, 483–499. doi: 10.1073/pnas.1908179116
Gräslund, B., and Price, N. (2012). Twilight of the gods? The ‘dust veil event’ of AD 536 in critical perspective. Antiquity 86, 428–443.
Grimm, E. (2019). Tilia version 2.6.1. Available Online at: http://www.tiliait.com (accessed July 15, 2021).
Grimm, E. C. (1987). CONISS: a Fortran 77 program for stratigraphically constrained cluster analysis by the method of incremental sum of squares. Comput. Geosci. 13, 13–35. doi: 10.1016/0098-3004(87)90022-7
Grønnesby, G. (2016). “Hot rocks! Beer brewing on Viking and medieval age farms in Trøndelag,” in The Agrarian Life of the North 2000 BC–AD 1000: Studies in Rural Settlement and Farming in Norway, eds F. Iversen and H. Petersson (Oslo: Cappelen Damm Akademisk), 133–149.
Gundersen, I. M. (2019). The Fimbulwinter theory and the 6th century crisis in the light of Norwegian archaeology: towards a human-environmental approach. Primitive Tider 21, 101–119.
Gundersen, I. M., Rødsrud, C. L., and Martinsen, J. R. P. (2020). “Kokegroper som massemateriale. Regional variasjon i en kulturhistorisk brytningstid,” in Ingen vei utenom, eds C. L. Rødsrud, A. Mjærum, A. A. Stamnes, J. Bergstøl, K. Eriksen, I. M. Gundersen, et al. (Oslo: Cappelen Damm Akademisk), 187–199. doi: 10.23865/noasp.97
Halvorsen, L. S., and Hjelle, K. L. (2017). Prehistoric agriculture in western Norway – Evidence for shifting and permanent cultivation based on botanical investigations from archaeological sites. J. Archaeol. Sci. 13, 682–696. doi: 10.1016/j.jasrep.2017.05.011
Hedeager, L. (2008). “Scandinavia before the Viking Age,” in The Viking world, eds S. Brink and N. Price (Milton Park: Routledge), 35–46.
Hill, M. O. (1973). Diversity and evenness: a unifying notation and its consequences. Ecology 54, 427–432. doi: 10.2307/1934352
Hjelle, K. L., and Sugita, S. (2012). Estimating pollen productivity and relevant source area of pollen using lake sediments in Norway: how does lake size variation affect the estimates? Holocene 22, 313–324. doi: 10.1177/0959683611423690
Hjelle, K. L., Halvorsen, L. S., and Overland, A. (2009). Heathland development and relationship between humans and environment along the coast of western Norway through time. Quat. Int. 220, 133–146. doi: 10.1016/j.quaint.2009.09.023
Hjelle, K. L., Halvorsen, L., Prøsch-Danielsen, L., Sugita, S., Paus, A., Kaland, P. E., et al. (2018). Long-term changes in regional vegetation cover along the west coast of southern Norway: the importance of human impact. J. Veg. Sci. 29, 404–415. doi: 10.1111/jvs.12626
Hjelle, K. L., Mehl, I. K., Sugita, S., and Andersen, G. L. (2015). From pollen percentage to vegetation cover: evaluation of the Landscape Reconstruction Algorithm in western Norway. J. Quat. Sci. 30, 312–324. doi: 10.1002/jqs.2769
Hjelle, K. L., Solem, T., Halvorsen, L. S., and Åstveit, L. I. (2012). Human impact and landscape utilization from the Mesolithic to medieval time traced by high spatial resolution pollen analysis and numerical methods. J. Archaeol. Sci. 39, 1368–1379. doi: 10.1016/j.jas.2011.12.026
Iversen, F., and Petersson, H. (eds) (2016). The Agrarian life of the North 2000 BC – AD 1000. Studies in Rural Settlement and Farming in Norway. Oslo: Cappelen Damm Akademisk.
Kaland, P. E. (1986). “The origin and management of Norwegian coastal heaths as reflected by pollen analysis,” in Anthropogenic Indicators in Pollen Diagrams, ed. K. E. Behre (Rotterdam: A.A. Balkema), 19–36.
Kaland, P. E. (2014). “Heathlands – land-use, ecology and vegetation history as a source for archaeological interpretations,” in Northern worlds – Landscapes, Interactions and Dynamics. Research at the National Museum of Denmark. Proceedings of the Northern worlds Conference Copenhagen 28-30 November 2012, ed. H. C. Gullov (Odense: University Press of Southern Denmark).
Krzywinski, K., O’Connell, M., and Küster, H.-J. (2009). Cultural Landscapes of Europe - Fields of Demeter, Haunts of Pan. Delmenhorst: Aschenbeck and Holstein Verlag.
Kuneš, P., Svobodová-Svidtavská, H., Kolář, J., Hajnalová, M., Abraham, V., Macek, M., et al. (2015). The origin of grasslands in the temperate forest zone of east-central Europe: long-term legacy of climate and human impact. Quat. Sci. Rev. 116, 15–27. doi: 10.1016/j.quascirev.2015.03.014
Kuosmanen, N., Marquer, L., Tallavaara, M., Moliniari, C., Zhang, Y., Alenius, T., et al. (2018). The role of climate, forest fires and human population size in Holocene vegetation dynamics in Fennoscandia. J. Veg. Sci. 29, 382–392. doi: 10.1111/jvs.12601
Løken, T. (2020). Bronze Age and Early Iron Age house and settlement development at Forsandmoen, south-western Norway. AmS-Skrifter 28, 1–300.
Marquer, L., Gaillard, M.-J., Sugita, S., Poska, A., Trondman, A.-K., Mazier, F., et al. (2017). Quantifying the effects of land use and climate on Holocene vegetation in Europe. Quat. Sci. Rev. 171, 20–37. doi: 10.1016/j.quascirev.2017.07.001
Marquer, L., Gaillard, M.-J., Sugita, S., Trondman, A.-K., Mazier, F., Nielsen, A. B., et al. (2014). Holocene changes in vegetation composition in northern Europe: why quantitative pollen-based vegetation reconstructions matter. Quat. Sci. Rev. 90, 199–216. doi: 10.1016/j.quascirev.2014.02.013
Mazier, F., Gaillard, M.-J., Kunes, P., Sugita, S., Trondman, A.-K., and Broström, A. (2012). Testing the effect of site selection and parameter setting on REVEALS-model estimates of plant abundance using the Czech Quaternary Palynological Database. Rev. Palaeobot. Palynol. 187, 38–49.
Mehl, I. K., Overland, A., Berge, J., and Hjelle, K. L. (2015). Cultural landscape development on a west-east gradient in western Norway - potential of the Landscape Reconstruction Algorithm (LRA). J. Archaeol. Sci. 61, 1–16. doi: 10.1016/j.jas.2015.04.015
Meltsov, V., Poska, A., Odgaard, B. V., Sammul, M., and Kull, T. (2011). Palynological richness and pollen sample evenness in relation to local floristic diversity in southern Estonia. Rev. Palaeobot. Palynol. 166, 344–351. doi: 10.1016/j.revpalbo.2011.06.008
Meltsov, V., Poska, A., Reitalu, T., Sammul, M., and Kull, T. L. (2013). The role of landscape structure in determining palynological and floristic richness. Veg. Hist. Archaeobot. 22, 39–49. doi: 10.1007/s00334-012-0358-y
Mokkelbost, M. (2019). “Roman period waste deposits at Ørland, Norway,” in Environment and settlement: Ørland 600 BC – AD 1250. Archaeological excavations at Vik, Ørland main air base, ed. I. Ystgaard (Oslo: Cappelen Damm Akademisk), 195–231.
Myhre, B. (2002). Landbruk, landskap og samfunn 4000 f. Kr., –800 e. Kr., in Norges landbrukshistorie I, 4000 f.Kr.-1350 e. Kr. Jorda blir levevei, eds. Myhre, B., and Øye, I. (Oslo: Det Norske Samlaget), 11–213.
Natlandsmyr, B., and Hjelle, K. L. (2016). Long-term vegetation dynamics and land-use history: providing a baseline for conservation strategies in protected Alnus glutinosa swamp woodlands. For. Ecol. Manag. 372, 78–92.
Nielsen, A. B. (2004). Modelling pollen sedimentation in Danish lakes at c. A.D. 1800: an attempt to validate the POLLSCAPE model. J. Biogeogr. 31, 1693–1709.
Nielsen, A. B., Giesecke, T., Theuerkauf, M., Feeser, I., Behre, K.-E., Beug, H.-J., et al. (2012). Quantitative reconstructions of changes in regional openness in north-central Europe reveal new insights into old questions. Quat. Sci. Rev. 47, 131–149. doi: 10.1016/j.quascirev.2012.05.011
Nogué, S., Santos, A. M. C., Birks, H. J. B., Björck, S., Castilla-Beltráin, A., Connor, S., et al. (2021). The human dimension of biodiversity changes on islands. Science 372, 488–491. doi: 10.1126/science.abd6706
Nordvig, M., and Riede, F. (2018). Are there echoes of the AD 536 event in the Viking Ragnarok Myth? A critical appraisal. Environ. Hist. 24, 303–324. doi: 10.3197/096734018X15137949591981
Odgaard, B. V. (2001). Palaeoecological perspectives on pattern and process in plant diversity and distribution adjustments: a comment on recent developments. Divers. Distrib. 7, 197–201.
Odgaard, B. V., and Rasmussen, P. (2000). Origin and temporal development of macro-scale vegetation patterns in the cultural landscape of Denmark. J. Ecol. 88, 200–221.
Odgaard, B., and Rømer, J. R. (eds) (2009). Danske landbrugslandskaber gennem 2000 år. Fra digevoldinger til støtteordninger. Aarhus: Universitetsforlag, Aarhus.
Overland, A., and Hjelle, K. L. (2009). From forest to open pastures and fields: cultural landscape development in western Norway inferred from two pollen records representing different spatial scales of vegetation. Veg. Hist. Archaeobot. 18, 459–476. doi: 10.1007/s00334-009-0225-7
Overland, A., and Hjelle, K. L. (2019). “Vegetation development at Ørland, and in the region, from c. 260 BC to the present,” in Environment and settlement: Ørland 600 BC – AD 1250. Archaeological excavations at Vik, Ørland main air base, ed. I. Ystgaard (Oslo: Cappelen Damm Akademisk), 69–105.
Paus, A. (1982). Paleo-økololgiske Undersøkelser påFrøya, Sør-Trøndelag. Den vegetasjonshistoriske Utviklingen fra Senistiden og Fram til i dag. Cand. real thesis. Trondheim: Universitetet i Trondheim.
Prøsch-Danielsen, L., and Simonsen, A. (2000). Palaeoecological investigations towards the reconstruction of the history of forest clearance and coastal heathlands in southwestern Norway. Veg. Hist. Archaeobot. 9, 189–204.
R Core Team (2021). R: A Language and Environment for Statistical Computing. Vienna: R Foundation for Statistical Computing.
Reimer, P. J., Austin, W. E. N., Bard, E., Bayliss, A., Blackwell, P. G., Ramsey, C. B., et al. (2020). The IntCal20 Northern Hemisphere radiocarbon age calibration curve (0-55 cal kBP). Radiocarbon 62, 725–757. doi: 10.1017/RDC.2020.41
Reitalu, T., Bjune, A. E., Blaus, A., Giesecke, T., Helm, A., Matthias, I., et al. (2019). Patterns of modern pollen and plant richness across northern Europe. J. Ecol. 2019, 1662–1677. doi: 10.1111/1365-2745.13134
Reitalu, T., Johansson, L. J., Sykes, M. T., Hall, K., and Prentice, H. C. (2010). History matters: village distances, grazing and grassland species diversity. J. Appl. Ecol. 47, 1216–1224. doi: 10.1111/j.1365-2664.2010.01875.x
Romundset, A., and Lakeman, T. R. (2019). “Shoreline displacement at Ørland since 6000 cal. yr BP,” in Environment and settlement: Ørland 600 BC – AD 1250. Archaeological excavations at Vik, Ørland main air base, ed. I. Ystgaard (Oslo: Cappelen Damm Akademisk), 51–67.
Sauvage, R., and Mokkelbost, M. (2016). “Rural buildings from the Viking and Early Medieval Period in central Norway,” in The Agrarian life of the North 2000 BC–AD 1000: Studies in Rural Settlement and Farming in Norway, eds F. Iversen and H. Petersson (Oslo: Cappelen Damm Akademisk), 275–292.
Sejrup, H. P., Seppä, H., McKay, N. P., Kaufman, D. S., Geirsdóttir, Á, de Vernal, A., et al. (2016). North Atlantic-Fennoscandian Holocene climate trends and mechanisms. Quat. Sci. Rev. 147, 365–378. doi: 10.1016/j.quascirev.2016.06.005
Solberg, B. (2000). Jernalderen i Norge: ca. 500 f. Kr.-1030 e. Kr. Oslo: Cappelen akademisk forlag.
Solheim, S. (2021). Timing the emergence and development of arable farming in Southeastern Norway by using summed probability distribution of radiocarbon dates and a Bayesian age model. Radiocarbon 63, 1503–1524. doi: 10.1017/RDC.2021.80
Solheim, S., and Iversen, F. (2019). The mid-6th century crises and their impacts on human activity and settlements in southeastern Norway. Ruralia XII, 423–434.
Solheim, S., and Persson, P. (2018). Early and mid-Holocene coastal settlement and demography in southeastern Norway: comparing distribution of radiocarbon dates and shoreline-dated sites, 8500–2000 cal. BCE. J. Archaeol. Sci. 19, 334–343. doi: 10.1016/j.jasrep.2018.03.007
Stamnes, A. A. (2016). “Effect of temperature change on Iron Age cereal production and settlement patterns in mid-Norway,” in The Agrarian Life of the North 2000 BC–AD 1000: Studies in Rural Settlement and Farming in Norway, eds F. Iversen and H. Petersson (Oslo: Cappelen Damm Akademisk), 27–39.
Storå, J., Ivarsson-Aalders, M., and Ystgaard, I. (2019). “Utilization of animal resources in Roman Iron Age Vik: Zooarchaeology at Ørland,” in Environment and settlement: Ørland 600 BC – AD 1250. Archaeological excavations at Vik, Ørland main air base, ed. I. Ystgaard (Oslo: Cappelen Damm Akademisk), 233–258.
Sugita, S. (1994). Pollen representation of vegetation in Quaternary sediments: theory and method in patchy vegetation. J. Biogegr. 32, 1993–2005.
Sugita, S. (2007a). Theory of quantitative reconstruction of vegetation I: pollen from large sites REVEALS regional vegetation composition. Holocene 17, 229–241. doi: 10.1177/0959683607075837
Sugita, S. (2007b). Theory of quantitative reconstruction II: all you need is LOVE. Holocene 17, 243–257.
Svensson, E. (2019). Crisis or transition? Risk and resilience during the Late Medieval agrarian crisis. Ruralia XII, 171–181.
Tallavaara, M., and Pesonen, P. (2020). Human ecodynamics in the north-west coast of Finland 10,000-2000 years ago. Quat. Int. 549, 26–35. doi: 10.1016/j.quaint.2018.06.032
ter Schure, A. T. M., Bajard, M., Loftsgarden, K., Høeg, H. I., Ballo, E., Bakke, J., et al. (2021). Anthropogenic and environmental drivers of vegetation change in southeastern Norway during the Holocene. Quat. Sci. Rev. 270:107175. doi: 10.1016/j.quascirev.2021.107175
Thun, T., and Svarva, H. (2018). Tree-ring growth shows that the significant population decline in Norway began decades before the Black Death. Dendrochronologia 47, 23–29. doi: 10.1016/j.dendro.2017.12.002
van der Plicht, J. (2004). “Radiocarbon, the calibration curve and Scythian chronology,” in Impact Of The Environment On Human Migration In Eurasia (NATO Science Series IV Earth and Environmental Sciences; Vol. 42), eds E. M. Scott, A. Y. Alekseev, and G. Zaitseva (Dordrecht: Springer).
Woodbridge, J., Fyfe, R. M., Roberts, N., Downey, S., Edinborough, K., and Shennan, S. (2014). The impact of the Neolithic agricultural transition in Britain: a comparison of pollen-based land-cover and archaeological 14C date-inferred population change. J. Archaeol. Sci. 51, 216–224. doi: 10.1016/j.jas.2012.10.025
Woodbridge, J., Fyfe, R., Smith, D., Pelling, R., de Vareilles, A., Batchelor, R., et al. (2021). What drives biodiversity patterns? Using long-term multipisciplinary data to discern centennial-scale change. J. Ecol. 109, 1396–1410. doi: 10.1111/1365-2745.13565
Ystgaard I.(ed.) (2019). Environment and settlement: Ørland 600 BC – AD 1250. Archaeological excavations at Vik, Ørland main air base. Oslo: Cappelen Damm Akademisk.
Keywords: long-term vegetation, biodiversity, archaeology, pollen, summed probability distribution, REVEALS, LOVE, human impact
Citation: Hjelle KL, Overland A, Gran MM, Romundset A and Ystgaard I (2022) Two thousand years of Landscape—Human interactions at a coastal peninsula in Norway revealed through pollen analysis, shoreline reconstruction, and radiocarbon dates from archaeological sites. Front. Ecol. Evol. 10:911780. doi: 10.3389/fevo.2022.911780
Received: 03 April 2022; Accepted: 04 July 2022;
Published: 28 July 2022.
Edited by:
Miikka Tallavaara, University of Helsinki, FinlandReviewed by:
Jutta Lechterbeck, University of Stavanger, NorwayAnneli Poska, Lund University, Sweden
Copyright © 2022 Hjelle, Overland, Gran, Romundset and Ystgaard. This is an open-access article distributed under the terms of the Creative Commons Attribution License (CC BY). The use, distribution or reproduction in other forums is permitted, provided the original author(s) and the copyright owner(s) are credited and that the original publication in this journal is cited, in accordance with accepted academic practice. No use, distribution or reproduction is permitted which does not comply with these terms.
*Correspondence: Kari Loe Hjelle, kari.hjelle@uib.no