- 1Departamento de Botánica, Instituto de Biología, Universidad Nacional Autónoma de México, Mexico City, Mexico
- 2Centro Universitario Regional del Litoral Atlántico (CURLA), La Ceiba, Honduras
The pantropical genus Dalbergia includes more than 250 species. Phylogenetic studies of the group are scarce and have only included two or three species distributed in Mexico. We obtained herbarium samples of Mexican, Central American, and South American species (sourced from MEXU). In addition, sequences of GenBank accessions were used to complement the study. Using internal transcribed spacer (ITS), the matK and rbcL sequences from 384 accessions comprising species from America, Asia, and Africa were sampled to evaluate phylogenetic relationships of Mexican species and infrageneric classifications based on morphological data. Phylogenetic analyses suggest that the genus Dalbergia is monophyletic and originated in South America. The species distributed in Mexico are not a monophyletic clade but are divided into four clades with affinities to South American and Asian species clades. There is no correlation between geography and large-scale phylogeny. The estimated ages of the Mexican and Central American clades ranged from 11.32 Ma (Dalbergia granadillo clade) to 1.88 Ma (Dalbergia ecastaphyllum clade). Multiple long-distance dispersal events should be used to explain the current genus distribution.
Introduction
The subfamily Papilionoideae includes an important clade, the Dalbergiodeae group. The “Dalbergioides” represent a monophyletic group comprising all genera referred to as the tribes Adesmieae and Aeschynomeneae, the subtribe Bryinae of Desmodieae and Dalbergieae except the genera Andira, Hymenolobium, Vatairea, and Vataireopsis (Lavin et al., 2001). This group consists of the subclades Adesmia, Dalbergia, and Pterocarpus, supported and identified mainly on a molecular data basis (chloroplast sequences; the trnK/matK spacer and the trnL intron, Lavin et al., 2001).
The Dalbergia genus is a pantropical group with around 250 species and centers of diversity in Central and South America, Africa, Madagascar, and Asia (Klitgård and Lavin, 2005). In Mexico, Dalbergia comprises 20 species, of which six are endemic (Sousa et al., 2001; Linares and Sousa, 2007; Ricker et al., 2013). Dalbergia, or rosewoods as are generally known, distinguishes because their heartwood is considered of high economic value, owing to its beauty, durability, and excellent physical, mechanical, and acoustic properties (Pittier, 1922). They also produce metabolites, used as antimicrobial (Rutiaga-Quiñones et al., 2010), antifungal (Rutiaga-Quiñones et al., 1995; Barragán-Huerta et al., 2004), antibiotic, antioxidants, and cytotoxic agents (Hamburger et al., 1987; Lianhe et al., 2011; Pérez-Gutiérrez and García-Baez, 2013). In addition, it has been reported that Dalbergia species establish symbiotic relationships with rhizobia for nitrogen fixation. This plays an important role in ecosystems since it improves soil fertility (Rasolomampianina et al., 2005). The species populations are at risk because of its intensive use, habitat loss, and fragmentation as well as slow recruitment rate and growth. Several species of the genus are used as timber species, and they are intensively exploited and subject to international traffic. Conservation of timber species threatened by illegal logging and deforestation is essential. Barcodes of the species could help to monitor species of Dalbergia subject to international traffic and reconstruct a phylogenetic hypothesis of the genus.
Phylogenetic analyses of the tribe Dalbergieae are based on molecular and morphological data (Lavin et al., 2001), placing Dalbergia in the Dalbergia clade as sister to the genus Machaerium Pers. and Aeschynomene L. subgen. Ochopodium. Later on, Ribeiro et al. (2007) concluded that Aeschynomene subgen. Ochopodium Vogel is more closely related than Machaerium to Dalbergia. Vatanparast et al. (2013) made other attempts to resolve the generic relationships between Aeschynomene and other segregate genera (Bryaspis, Geissaspis Wight & Arn. and Kotschya Endl.), but they are weakly resolved still. Cardoso et al. (2020), studying the phylogenetic relationships of Aeschynomene subgen. Ochopodium, find that both, Aeschynomene and Machaerium, are sister taxa of Dalbergia. Ochopodium section was newly circumscribed as Ctenodon, and the genus is particularly diverse in Mexico and Brazil and has a few endemic species in the Andes.
In Mexico, 20 species have been described, 15 of which are potentially threatened by illegal logging (Linares and Sousa, 2007). Due to the characteristics of its wood, they are over-exploited, placing them in danger of extinction (NOM-059 SEMARNAT, 2010). According to the red list of the IUCN (International Union for Conservation of Nature), the species of the most concern are Dalbergia granadillo and D. retusa, as their natural populations are decreasing considerably and are therefore considered Critically Endangered (IUCN). Mexican species inhabit the west and center of the country and the Yucatan peninsula. However, most species grown in the southeast are associated with tropical forests, cloud forests, tropical deciduous and sub-deciduous forests, and pine-oak forests (Standley, 1922) (Table 1). Only three Mexican species were used in previous phylogenetic studies.
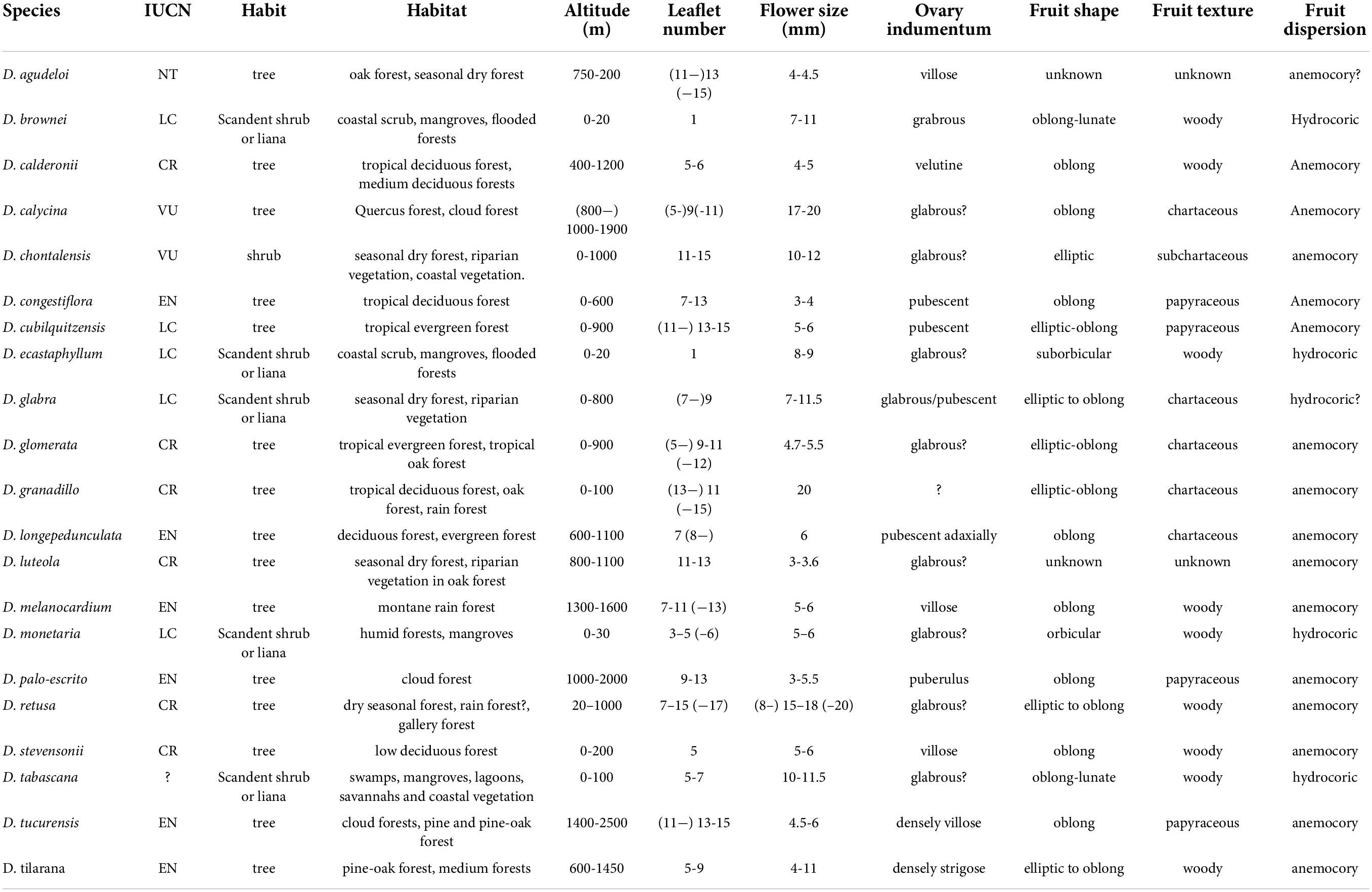
Table 1. Ecological and morphological information of Dalbergia species distributed in Mexico, Central America, and the Caribbean.
Dalbergia species are morphologically variable and possess a wide range of habitat preferences which made it difficult to classify the New and Old World species into natural groups (Bentham, 1860; Prain, 1904; Carvalho, 1989). It is necessary to use several specimens for each species in a broadscale distribution to get a more clear idea of which are the taxonomic circumscription within Dalbergia species. We employed a relatively wide taxonomic sampling using several Dalbergia accessions from species occurring in Mexico and included species from its centers of diversity in America, Africa, and Asia. The objectives of this study were to (1) provide a phylogenetic framework for Mexican Dalbergia species, (2) test up barcode molecular markers in Mexican species, and (3) provide an age of divergence for the Mexican species.
Materials and methods
Taxa sampling and deoxyribonucleic acid sequencing
To obtain an in-depth view of the phylogenetic relationships within the genus, we increased the previous sampling by the addition of Mexican, Central America, and South American species of Dalbergia. We included a total of 287 Dalbergia accessions. Outgroup selection was based on previous phylogenetic studies ensuring that accession sequences from Ctenodon, Machaerium, and Pictetia close relative genera were represented (Supplementary Table 1). A summary of accessions used for species from Mexico, Central America, and the Caribbean is listed in Table 2.
The sample tissue material for DNA extraction was obtained from specimens in the MEXU herbarium. Total genomic DNA was extracted from leaves, flowers, or fruit samples using a modified DNeasy Plant Mini Kit (Qiagen). The target DNA regions, rbcL and matK, were amplified with universal barcoding primers (CBOL Plant Working Group, 2009). In the case of internal transcribed spacer (ITS), AB101 and AB102 primers (Sun et al., 1994) were used. PCR amplification of rbcL, matK, and ITS was carried out on a Gene Amp 2700 (Applied Biosystems, United States) with a Thermo PCR Master Mix kit (Thermo Fisher), using the manufacturer’s instructions. PCR conditions for matK and rbcL were as follows: 2 min initial denaturation at 94°C, 35 cycles (94°C 1 min, 52°C 1 min, and 72°C 1 min), and 10 min of final extension at 72°C. PCR conditions for ITS were as follows: 2 min initial denaturation at 94°C, 35 cycles (94°C 1 min, 53°C 1 min, and 72°C 1 min), and 7 min of final extension at 72°C. Amplified PCR products were checked on 1% agarose gel electrophoresis. Both strands of the clean PCR products were directly sequenced using BigDye Terminator v.3.1 (Thermo Fisher, Foster City, CA, United States) cycle sequencing kit and visualized on an ABI 3730 (Applied Biosystems) at Laboratorio de Secuenciación Genomica de la Biodiversidad y la Salud, Instituto de Biología, using the same primers as for amplification.
Distribution maps
We constructed distribution maps with collection information accessed from Global Biodiversity Information Facility (GBIF.org, 2022).1 We downloaded 11,941 herbaria records for Mexican, Central American, and Caribbean species of Dalbergia sampled in the molecular phylogeny. Data cleaning involved, first, standardizing data, deleting duplicate specimens, deleting records without any geographical coordinates, and any georeference erroneously georeferenced. After that, we used the R package “CoordinateCleaner” (Zizka et al., 2019) for further cleaning about coordinates at sea, country and province centroids, country capitals, urban areas, and around biodiversity institutions, which often come from cultivated individuals or with incorrect data. From the records downloaded, 5840 records were georeferenced, and after filtering and cleaning, 4,014 records were suitable to be used to generate distribution maps by species and phylogenetic clade.
Data analysis
Sequences were edited and assembled using SeqTrace software (Stucky, 2012). All sequences generated in this study were deposited in GenBank (Supplementary Table 1). Edited sequences for each gene region were aligned separately with MAFFT (Katoh et al., 2009). After an initial alignment, the alignments were manually adjusted using AliView (Larsson, 2014) if needed, following the principles described in Kelchner and Clark (1997). In addition, we compiled all ITS, matK, and rbcL sequences publicly available in GenBank for Dalbergia and added our newly generated three loci sequences to that dataset to produce a phylogenetic tree with a denser sampling across Dalbergia. Sequences generated from the same voucher from at least two loci have been used in the combined dataset to reduce the missing data. The combined dataset has 194 accessions and 336 accessions for the unique ITS dataset. A total of 384 accessions were analyzed.
Phylogenetic reconstruction of all the taxa sampled was undertaken using Bayesian inference (BI). We used three datasets: (1) the individual ITS dataset (unique ITS), (2) the plastid data set, and (3) the concatenated dataset. A Bayesian analysis without a molecular clock for the concatenated matrix was inferred with MrBayes. Gene trees for calibration were inferred with BEAST2 (Bouckaert et al., 2019). The GTR + Γ was selected as the best fit model based on the Akaike information criterion (Akaike, 1974) using the software jModelTest 2 (Darriba et al., 2012). The combined analysis for the three markers was run in 20 × 106 generations, sampling every 1,000. For the ITS dataset, 40 × 106 generations were run. Trees were sampled for 1,000 generations, and 20% of them were discarded as burn-in. The convergence of MCMC chain trees was visualized with the Bestiary software (Wirth and Duchene, 2021). Calibrated time trees were estimated using BEAST2 (Bouckaert et al., 2019) with a Yule tree prior model, lognormal relaxed molecular clock, and the node Machaerium-Dalbergia data according to Lavin et al. (2005). The trees were visualized with ggtree for R (Yu, 2020). Alignments in FASTA format can be seen in the Supplementary Material (S2).
Results
Phylogenetic relationships, combined tree
Phylogenetic trees show that Dalbergia is monophyletic (1.0 PP) with a basal clade formed by South American Neotropical species (Dalbergia miscolobium, Dalbergia spruceana, and Dalbergia villosa, sect Dalbergia sensu Carvalho, 1997) resolved sister to the remaining species (Figures 1, 2). The second clade of Asian species, containing two subclades, is then sister to the remaining species. Subclade II-A of Mexican climbing or woody vine species part of Ecastaphyllum sensu Carvalho (1997) (Dalbergia ecastaphyllum, Dalbergia monetaria) is sister to subclade II-B of woody vine Asian species: Dalbergia velutina, Dalbergia pinnata (synonym of Dalbergia tamarindifolia), and Dalbergia rubiginosa (series Polyphyllae, Rubiginosae, Sericeae, and Velutina sensu Prain, 1904).
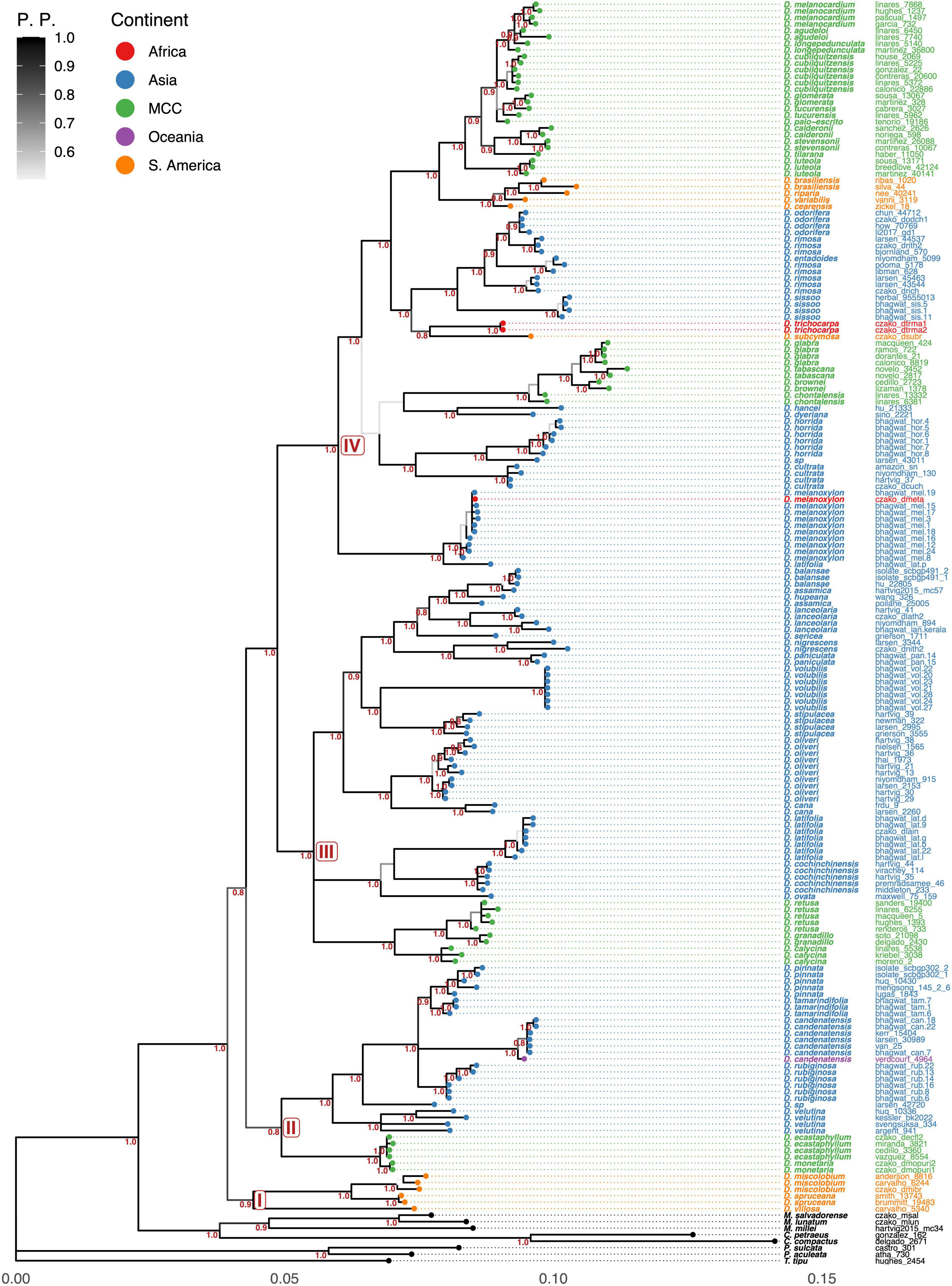
Figure 1. Bayesian combined phylogram of Dalbergia. Under the branches, posterior probabilities (pp) are in red font.
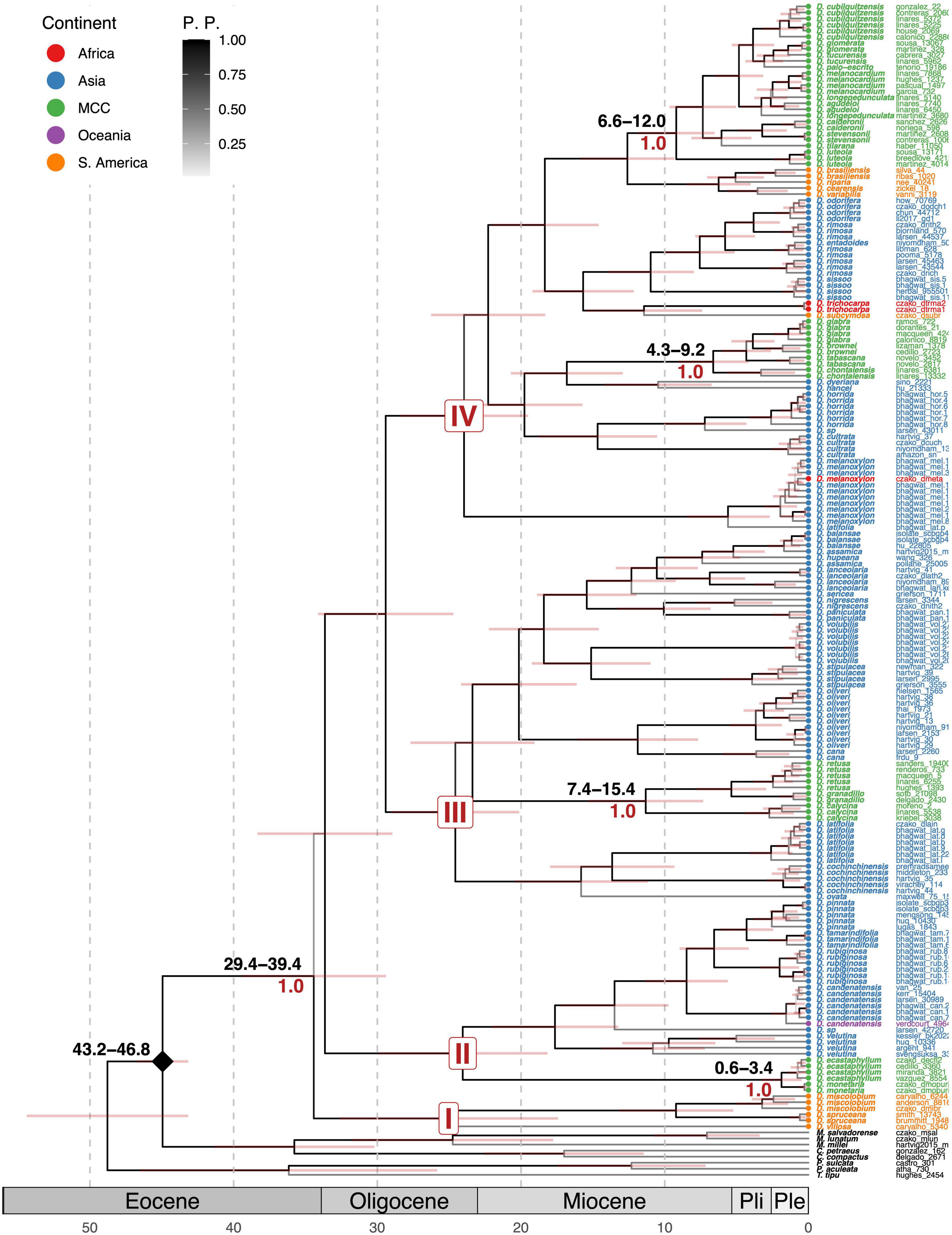
Figure 2. Combined Bayesian calibrated tree of Dalbergia. Above the branches, the estimated age range of the clades is in black font. Local posterior probabilities are shown under branches in red font. Shading bottom bars represent geological epochs. The diamond mark represents the calibration node. The bars on the branches represent the range of the 95% confidence interval in the Bayesian tree.
Clade III contains three subclades (III-A, III-B, and III-C). Subclade III-A contains Asian species Dalbergia ovata (Ovatae), Dalbergia cochinchinensis, and Dalbergia latifolia (serie Latifoliae), both parts of section Miscolobium. Subclade III-B contains Mexican and Central American species: Dalbergia calycina, D. granadillo, D. retusa, and Dalbergia cuscatlanica. Subclade III-C contains Asian species, and the species are part of subgenus Amerimmnon sensu Prain (1904) section Dalbergaria; Dalbergia cana (Canae) is the sister species of Dalbergia oliveri (Lanceolarieae); then, they are the sister group of Dalbergia stipulacea (Stipulaceae)-Dalbergia volubilis (Volubilis), and the group formed by seven species part of Lanceolarieae (Dalbergia lanceolaria subsp. lanceolaria, Dalbergia balansae, Dalbergia huapeana, Dalbergia assamica, Dalbergia nigrescens, and Dalbergia paniculata) and Sericeae (Dalbergia sericea).
Clade IV is formed by five subclades (IV-A, IV-B, IV-C, IV-D, and IV-E). Subclade IV-A is formed by the tree species of Dalbergia latifolia (Latifoliae) and Dalbergia melanoxylon (Phyllanthoides). Subclade IV-B is formed by Dalbergia dyeriana, Dalbergia hancei (Foliaceae), Dalbergia cultrata (Cultratae), and Dalbergia horrida. Subclade IV-C is formed with four climbing Mexican–Central American species (Dalbergia brownei, Dalbergia chontalensis, Dalbergia glabra, and Dalbergia tabascana). Subclade IV-D includes a divergent climbing species Dalbergia subcymosa (Ecastaphyllum sensu Carvalho, 1997) from South America as a sister to the following Asian species: Dalbergia trichocarpa (Madagascar-African tree, unknown sect.), the tree Dalbergia sissoo (serie Sisso), the woody climbers Dalbergia rimosa (serie Rimosae), and Dalbergia entadoides (unknown sect.-serie), and the tree Dalbergia odorifera (unknown sect.-serie). Subclade IV-E resolves a group of South American tree species of Triptolemea sensu Carvalho, 1997 (Dalbergia variabilis = Dalbergia frutescens, Dalbergia cearensis, Dalbergia riparia, and Dalbergia brasiliensis) as the sister group of 10 Mexican–Central American species of trees (Dalbergia agudeloi, Dalbergia melanocardium, Dalbergia palo-escrito, Dalbergia tucurensis, Dalbergia calderonii, Dalbergia stevensonii, Dalbergia cubilquitzensis, Dalbergia glomerata, Dalbergia longepedunculata, and D. calycina).
Phylogenetic relationships, internal transcribed spacer tree
In this tree, we included a larger number of species from Africa (Figure 3). Dalbergia is monophyletic with a basal clade formed by South American Neotropical species (D. miscolobium, D. spruceana, Dalbergia foliolosa, Dalbergia cuiabensis, D. villosa, Dalbergia acuta, Dalbergia revoluta, Dalbergia inundata, and Dalbergia lateriflora) resolved sister to Dalbergia afzeliana from Africa. The second clade is formed by two subclades: one of Mexican climbing or woody vine species (D. ecastaphyllum and D. monetaria) as sister to a subclade of woody vine Asian species (D. pinnata, D. tamarindifolia, D. rubiginosa, Dalbergia candenatensis, Dalbergia rostrata, D. stipulacea, and D. velutina) and an African bush species (Dalbergia microphylla).
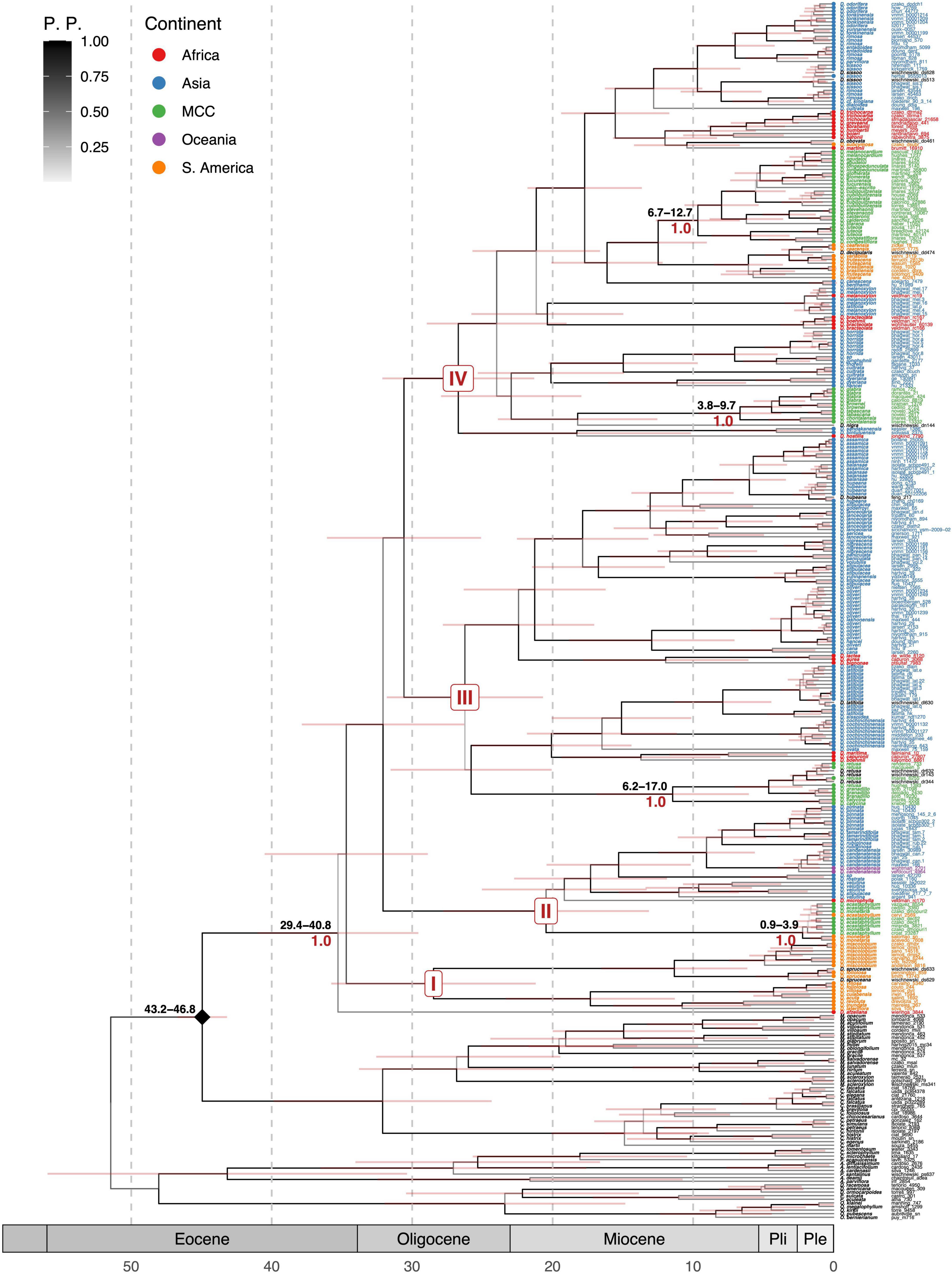
Figure 3. ITS calibrated tree of Dalbergia. Above the branches, the estimated age range of the clades is in black font. Local posterior probabilities are shown under branches in red font. Shading bottom bars represent geological epochs. The diamond mark represents the calibration node. The bars on the branches represent the range of the 95% confidence interval in the Bayesian tree.
Clade III contains three subclades. The first contains Asiatic species (Dalbergia ovata, D. cochinchinensis, Dalbergia sissoides, and D. latifolia) and African tree species (Dalbergia maritima, Dalbergia capuronii, and Dalbergia boehmi). The second one is with Mexican and Central American species (D. calycina, D. granadillo, and D. retusa). The last subclade has a group of African species (Dalbergia lactea, Dalbergia aurea, and Dalbergia bignonae) as sister of a clade with Asian species grouped into three clades: the first one grouping D. cana, D. oliveri, D. hancei, and Dalbergia lakhonensis; second one grouping D. stipulacea, Dalbergia yunnanensis, D. volubilis, D. paniculata, and D. nigrescens; and the third one formed by D. sericea, D. lanceolaria, Dalbergia godefroyi, D. stipulacea, Dalbergia huepeana, D. balansae, and D. assamica.
Clade IV is formed by five subclades. The first subclade is formed by a climber African species Dalbergia hostilis and two Asian species (Dalbergia sandakanensis and Dalbergia bintuluensis). In the second subclade, three climbing and one small tree species from Mexico-Central America (D. brownei, D. chontalensis, D. glabra, and D. tabascana) are nested with D. nigra from Brazil; these species are the sisters of an Asian group formed by D. dyeriana, D. hancei, D. cultrata, Dalbergia thorelii, Dalbergia lunghuhnii, and D. horrida. The third subclade has African species Dalbergia bracteolata and D. boehmii as the sister group of a clade with the tree species Dalbergia latifolia and D. melanoxylon. The fourth subclade has Dalbergia canescens and Dalbergia benthamii from Asia, South American species (D. cearensis, Dalbergia decipularis, D. variabilis = D. frutescens, D. brasiliensis, D. riparia, and Dalbergia frutenscens var. tomentosa), and 12 Mexican–Central American species of trees (D. agudeloi, D. calderonii, Dalbergia congestiflora, D. cubilquitzensis, D. glomerata, D. longepedunculata, Dalbergia luteola, D. melanocardium, D. palo-escrito, Dalbergia tilarana, D. tucurensis, and D. stevensonii). The fifth subclade includes an African group of trees (D. trichocarpa, Dalbergia greveana, Dalbergia abrahamii, Dalbergia humbertii, Dalbergia bojeri, and Dalbergia baronii) with two divergent climbing species (D. subcymosa from South America and Dalbergia martii from Africa) as sister to Asian species. This group of Asiatic species consists of a divergent tree species (D. cultrata) sister to a subclade formed by woody climbers (D. rimosa, Dalbergia cf. kingiana, and Dalbergia dialoides), plus a tree species (D. sissoo), and a mix of trees, lianas, and woody climbers (D. odorifera, Dalbergia tonkinensis, D. yunnanensis, Dalbergia rimosa var. foliacea, D. entadoides, and Dalbergia parviflora).
There are not many obvious relationships conflicting between the nuclear loci tree and the two loci concatenated plastid tree (Figure 4). The backbone of the plastid loci tree is nearly identical to the nuclear ITS tree, with all major nodes and monophyly receiving strong support. In the plastid tree, there are many polytomies but a geographical structuring of the species is observed. The major clades disappear hierarchically but still form a group. The major conflict in the tanglegram is Dalbergia melanoxylum, in ITS tree is a sister species to D. glabra clade, and in plastid tree is part of a polytomy.
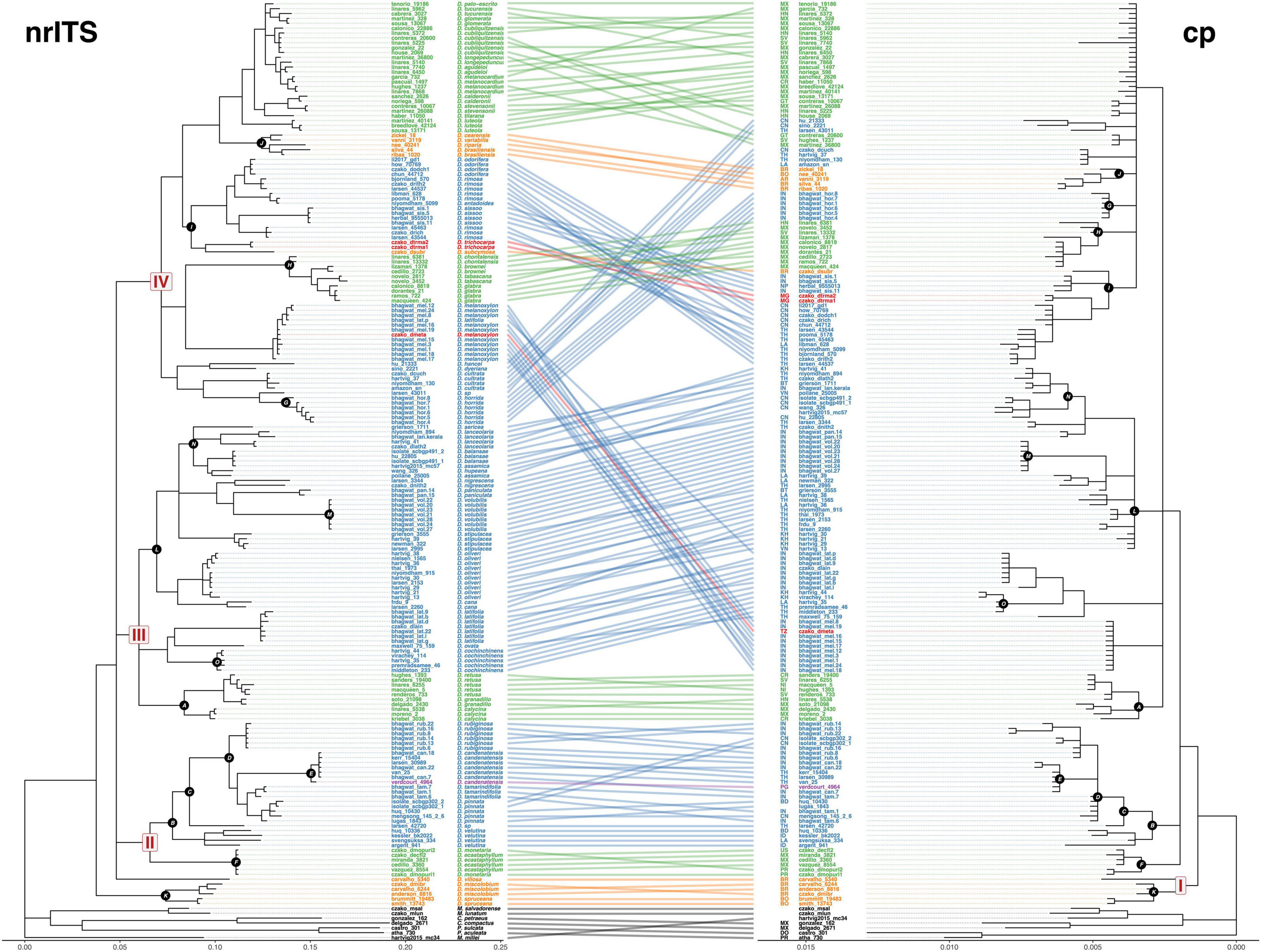
Figure 4. Tanglegram illustrating the discordance between the ITS gene tree (nrITS) and the combined plastid gene tree (cp) for Dalbergia. Links connect identical tips, with nodes rotated to minimize link overlap. Links are colored by geographical distribution. Clades that are similar between the two trees are indicated by black circles with white font. Tipuana tipu was eliminated because only the ITS1 sequence was available.
Geographical distribution of Mexican, Central American, and Caribbean species of Dalbergia
Most tree species of Dalbergia are restricted in distribution with the exception of D. congestiflora. Populations of D. granadillo clade are mostly distributed along the Pacific coast of Mexico from Colima to Panama. Climbing species have more widespread distributions, like D. ecastaphyllum and D. monetaria whose distribution reaches South America and Africa. D. brownei is a climbing species that has managed to spread as far as the coast of Florida. Distribution maps by clade can be found in Figure 5. Distribution maps by species can be found in Figures 1–5 of the Supplementary Material.
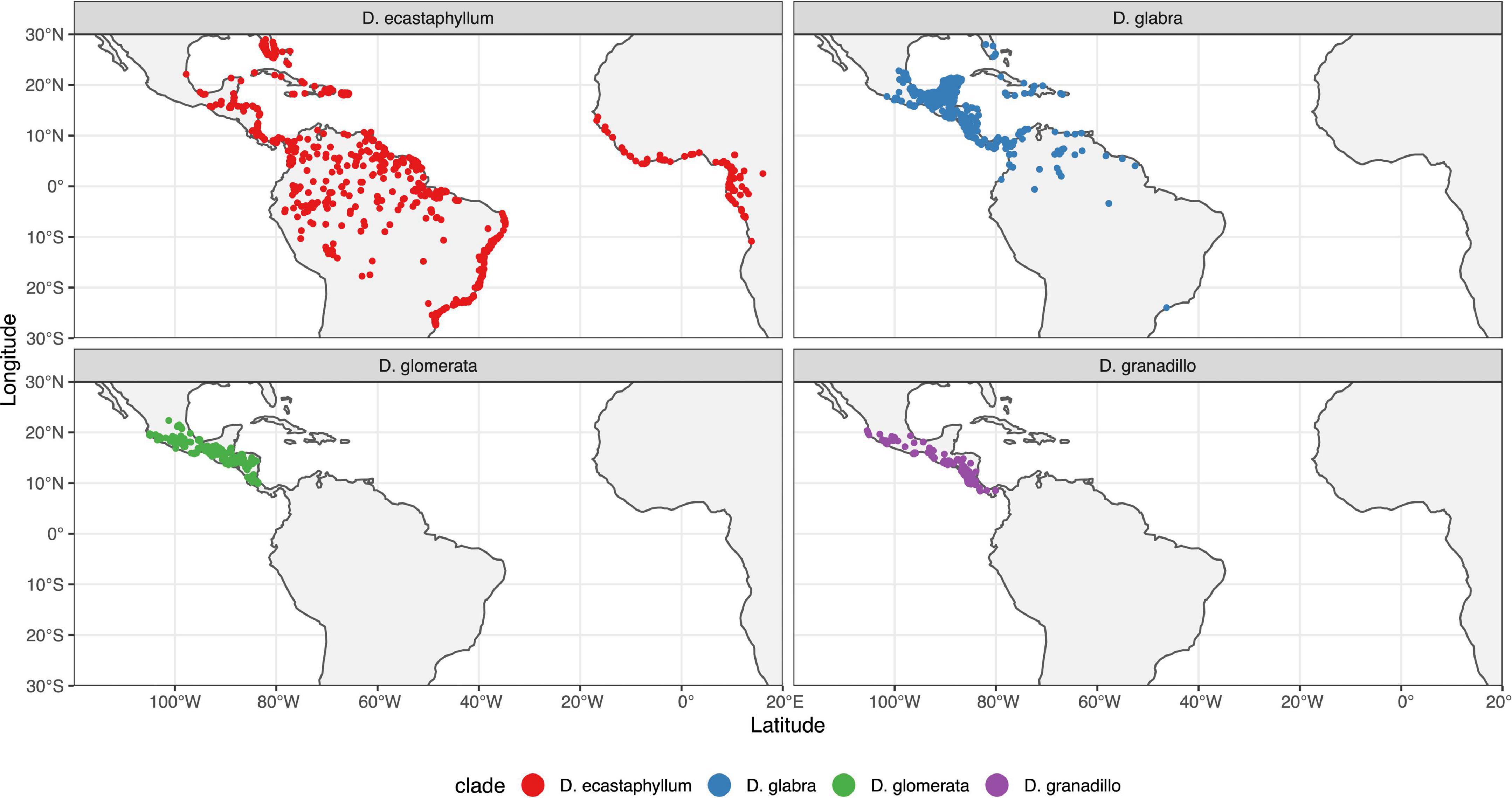
Figure 5. Distribution maps by clade for Dalbergia species from Mexico, Central America, and the Caribbean.
Divergence time estimates
Divergence time estimation provided a robust time-calibrated tree of Dalbergia (Figure 2). The Dalbergia group arose 34.42 Ma during the Oligocene and diversification of the present day occurred during the Miocene to Pleistocene from 34.42 to 1.88 Ma. Dalbergia diverged from their sister genera 44.95 Ma and diversified during the Miocene (24.73–5.23 Ma). The divergence ages for Mexican Dalbergia species are between Quaternary (Pleistocene) and Tertiary (Neogene). The oldest Mexican clade is Dalbergia granadillo with 11.32 Ma (Miocene), D. congestiflora clade with 9.2 Ma (Miocene, Tortonian), D. glabra with 6.63 Ma (Miocene, Zancleane), and D. ecastaphyllum with 1.88 Ma (Pleistocene, Calabrian). Divergence estimations from the combined tree to the ITS tree do not vary considerably (Figure 3). The estimation age for the Dalbergia granadillo clade was 11.5 Ma, for D. glomerata clade was 9.7 Ma, for D. glabra clade was 6.7 Ma, and for D. ecastaphyllum clade was 2.2 Ma.
Discussion
Taxonomy
Bentham (1860) divided the 64 species of Dalbergia known into six series (Triptolemea Americanae, Triptolemea, Sissoae Americanae, Sissoae Gerontogee, Dalbergariae, and Selenolobium). von Taubert (1894) divided the species into four sections (Triptolemaea, Sissoa, Dalbergaria, and Selenolobium). In the Neotropics, the 44 Brazilian species of Dalbergia were divided into five sections by Carvalho (1989, 1997) based on inflorescence and fruit types (Dalbergia, Ecastaphyllum, Pseudoecastaphyllum, Selenobium, and Triptolemea). In Asia, Prain (1904) classified the 86 South-East Asian species of Dalbergia into two subgenera (Amerimnon and Sissoa), five sections (Dalbergaria, Endespermum, Miscolobium, Podiopetalum, and Triptolemea) and 24 series. Finally, Thothathri (1987) categorized the 46 Dalbergia species, present in the Indian subcontinent, into four sections and seven series based on androecium and fruit types.
The sect. Triptolemea, with cymose inflorescences and samaroid legume, and sect. Ecastaphyllum, with racemose or paniculate inflorescences and orbicular to reniform legume sensu Carvalho (1997), are monophyletic. These sister relationships between species have also been found by Ribeiro et al. (2007); Vatanparast et al. (2013), and Hartvig et al. (2015). We also found relationships between D. candenatensis, D. pinnata, and D. velutina as other authors do (Vatanparast et al., 2013; Hartvig et al., 2015) but no as sister species. They are part of the same clade with D. tamarindifolia (sister to D. pinnata), D. rubiginosa, and D. sericea. Niyomdham et al. (1997) recognized that D. pinnata, D. candenatensis, and D. velutina have morphological affinities in the lower calyx tooth as long as or slightly longer than the laterals, standard equal to at least 3/4 of the blade, sometimes exceeding it. Niyomdham et al. (1997) treated D. tamarindifolia as a synonym of D. pinnata, specimens occurring together into the clade in two groups; these results might be indicative of taxonomic differences.
We found that sect. Dalbergia sensu Carvalho (1997) is also monophyletic. Species sampled from section Dalbergiaria sensu Prain (1904) are monophyletic too (series Lanceolarieae, Stipulaceae, and Volubilis). Vatanparast et al. (2013) treated Dalbergia balansae and D. assamica as separate species, but Hartvig et al. (2015) treated both species as a synonym. In this study, we treated the species separately and we included Dalbergia hupeana. Specimens in the phylogenetic analyses occur together; D. hupeana and D. balansae are sister species of D. assamica, as well as D. sericea, D. nigrescens, and D. paniculata. These results might be indicative that D. balansae and D. assamica are different species.
The Latifoliae series (D. latifolia, D. cochinchinensis, and D. ovata) from section Miscolobium (Prain, 1904) is monophyletic, and this group was also found by Vatanparast et al. (2013) and Hartvig et al. (2015). Morphological characters between D. cochinchinensis and D. ovata are lower calyx teeth as long or slightly longer than the lateral ones; standard longer than wide; leaves with (5−) 7-9 leaflets; leaflets acute to acuminate, apiculate, rarely obtuse, or rounded; flowers white to whitish, 5.5–6 mm long; fruits thin, papyraceous, glabrous, light brown (Niyomdham et al., 1997).
Accessions of Dalbergia rimosa are in three different groups in the same subclade. The first group is the sister species to D. odorifera, the second one is sister to D. entadoides, and the third one is basal D. rimosa accessions. The species is distributed in India, Myanmar, South of China, Thailand, Laos, and Vietnam from 200 to 1,300 m in mixed deciduous forests and scrub forests. When we see herbarium specimens from the different country distributions, it is clear that a morphological taxonomic revision must be carried out (e.g., Hooker J.D. sn. from Myanmar, Berhaman et al. SAN 134566 from Malaysia).
Mexican, Central American, and Caribbean species of Dalbergia
Although there is a taxonomic treatment for Flora Mesoamericana (Linares, in press), since Pittier (1922) there have been no attempts at subgeneric-level classifications of Mexican Dalbergia. Richter et al. (1996), citing personal comments by Richter et al. (1996), suggested four groups for the Mexican species. The first consists of D. retusa, Dalbergia hypoleuca, D. granadillo, and Dalbergia lineata, probably D. cuscatlanica and Dalbergia pacifica, all of which are similar in wood structure and metabolites.
The second group comprises Central American and Mexican species, D. tucurensis (including D. cubilquitzensis), D. palo-escrito, D. melanocardium, D. glomerata, D. congestiflora, D. calderonii (including Dalbergia funera), and probably D. stevensoni. No differentiation was detected in the wood of these species, although Richter et al. (1996) underline that D. stevensonii may be different from the rest. The third group, with the species D. calycina and Dalbergia intibucana (nowadays synonyms), were not sampled for Richter’s study because of their lack of commercial value at that time. Finally, the fourth group with D. brownei whose wood parenchyma banding is similar to that found in D. congestiflora and D. funera but different in the uniseriate rays.
Three of the groups hypothesized by Rudd are phylogenetically valid. The first is referred to here as the Dalbergia granadillo clade (because it is the most traded species in the clade). Currently, only the following species are recognized by Linares (in press), Dalbergia granadillo, D. retusa var. retusa, and D. retusa var. cuscatlanica. To the same clade belongs D. calycina which Rudd recognized as a separate group and which is within the clade as the most divergent species. The species are distributed in the seasonally dry forests of the Pacific Coast of Mexico from Jalisco to Oaxaca (D. granadillo), in the seasonally dry forests of Southeastern Honduras, Nicaragua, and Costa Rica (D. retusa var. retusa), and in humid environments of Honduras and El Salvador (D. retusa var. cuscatlanica).
The second group is what we called the Dalbergia glomerata clade. The D. glomerata clade is a group of 12 species distributed in the Pacific Coast of Mexico from Jalisco to Costa Rica, in the Rio Balsas Depression, in the Gulf Coastal plain from Veracruz to North of Chiapas, and in Guatemala and Belize. Species can be found in cloud forests, seasonally dry tropical forests, tropical rainforests, or secondary vegetation. Sister species clade is from South America (D. brasiliensis, D. riparia, D. cearensis, and D. variabilis (D. frutescens)). They are part of Triptolemea and characterized by inflorescence cymose in terminal racemes; fruit oblong to elliptical, samaroid, with reticulate venation more prominent over the seed cavity. Species occur mainly in central and eastern Brazil, with the exception of D. riparia that inhabits the central Amazon Basin and less frequently on the lower Amazon.
Finally, the third group, referred to here as the Dalbergia glabra clade (fourth group for Rudd), includes the species D. brownei, D. chontalensis, D. glabra, and D. tabascana. Rudd does not include the last three species, although, in 1995, she described varieties within D. glabra. The D. glabra clade species are distributed from Veracruz, Mexico, to Honduras on the Atlantic and from Oaxaca, Mexico, to El Salvador in the Pacific Coast. Furthermore, Rudd did not say anything about the species D. ecastaphyllum and D. monetaria (D. ecastaphyllum clade). The D. ecastaphyllum clade is distributed from Florida, United States, to Brazil, passing through Mexico, and in Caribbean islands. Plant distribution records exist in the Western part of Africa. Species inhabits riparian vegetation, coastal dunes, mangrove forests, and mangrove-associated forests.
Time of diversification in Dalbergia
The origin of Dalbergia is probably South America, as the South American species are the earliest divergent. Later, the genus must have migrated to North America (possibly when Central America did not yet exist) and diversified into the four lineages we recognize today. In Figure 6 (Supplementary Material), we can see that all haplotypes are central (in green). The Asian species evolved from the North American ones. There are different lineages between them, probably during the boreotropic (the only issue is that the geological data date this stage in the Eocene, implying that the genus is possibly older), which is in agreement with the fossil record found in America and Europe. The South American lineage of Dalbergia frutescens is a more recent arrival and derives from a southern migration of the Dalbergia glomerata clade (Figure 1 and Supplementary Material).
The Dalbergia granadillo clade must have had an ancestor in mountain areas, tolerant of metamorphic rocky soils and dry conditions, and equivalent to mixed pine-oak forest. The earliest diverged species is D. calycina, a species found in montane areas such as Bochil or in the Cañon del Sumidero, both in Chiapas.
The Dalbergia glomerata clade can be divided into two groups: (1) species related to D. cubilquitzensis (species complex) that inhabit humid environments and are tolerant of limestone and metamorphic soils, and (2) species related to D. congestiflora that inhabit areas with marked seasonality and dryness. Dalbergia stevensonii, which is the most recently diversified species in the clade, has a morphology that resembles species from the seasonal dry forest and not from the humid and flooded area where it is currently distributed.
The Dalbergia glabra clade mostly consists of climbing species. The earliest divergent species, D. chontalensis, is a shrub distributed in floodplains or near low-lying streams. D. brownei is a shrubby, climbing species distributed on coastal dunes and has dispersed as far as Florida. Dalbergia tabascana is another lianoid species that has “specialized” to grow in freshwater swamp areas. The area where it is currently distributed was once a wetland area (San Lorenzo Tenochtitlán). The most recently diversified species (Pleistocene) is D. glabra, the only species in the clade that succeeded in diversifying from seasonal dry forest environments associated with water bodies to rain forests. Populations of this species can be found in the interior of the country but are always associated with water bodies.
The Dalbergia ecastaphyllum clade is the most recent in origin, and the species that comprise it are two lianoid species that inhabit mostly coastal regions. D. monetaria is the most recent species, is tolerant of freshwater bodies, and can therefore be found in different areas of the Amazon and in the African Congo. The fruit is floating and woody and has a “spongy” endocarp.
Most of the ages obtained here are younger than previous estimates (Lavin et al., 2005). Lavin et al. (2005) using a matK phylogenetic reconstruction estimated the age of divergence between Dalbergia sisso and Tipuana tipu in 49.1 ± 0.8 Ma (47.1–51.4 Ma). In the same study, they estimated the age of divergence between D. sissoo and Ormocarpum in 45.6 ± 0.8 Ma (43.9–47.3 Ma). However, in the study of Lavin et al. (2004), the reported Dalbergia estimation age from stem and crown clades is 40.4–43.3 Ma, and they give divergence estimates ranging from 12.7-3.8 to 7-12.2 Ma. Later on, Hung et al. (2020) with transcriptomes data (256 single-copy orthologs, 479,064 bp) established that the Dalbergia miscolobium clade is basal with an age of ± 14.78 Ma (Miocene-Langhian). The divergence ages found for D. cochinchinensis and D. oliveri in Indochina were estimated to be 11.68 Ma (Lower Miocene), which corresponds with the separation of the Thai–Malay Peninsula from Borneo ± 15 Ma ago (Vatanparast et al., 2013). The fossils of Dalbergia found in Europe are from the Miocene such as Dalbergia nostratum (15.97–23.03 Ma; lower Miocene), Dalbergia lucida (5.33–11.61 Ma; late Miocene), or Dalbergia phleboptera (27.82–23.2 Ma; Oligocene–Miocene). For Mexico, fossils of wood from Puebla are dated from the Oligocene (32 Ma, Sainz-Reséndiz, 2011).
Miocene diversification of Dalbergia reflects patterns shown in other tropical genera (Choo et al., 2020; Schley et al., 2022) in accordance with the climatic and ecological changes that occurred in the Tropics during the Miocene.
The combined marker phylogenetic reconstruction indicated that in Mexico, several ancestral independent lineages within Dalbergia might began their diversification consecutively during the Miocene. The ancestors of Mexican Dalbergia clades came from South America and Asia. How species were exchanged from South America to Mexico can be explained by migrations through Central America via the narrow Isthmus of Panama, which existed above sea level from the late Eocene to the Oligocene (38–28 Mya, Montes et al., 2012), and through which the exchanges of flora and fauna may have taken place (Cody et al., 2010). In the warm periods from the Eocene to Miocene through the transport of seeds and after the consolidation of the Isthmus, the contributions of flora must have increased.
For the Dalbergia glomerata clade, the sister group of taxa D. brasiliensis, D. cearensis, and D. variabilis (D. frutescens) have a fruit that could be wind-dispersed, while D. riparia has a fruit that is dispersed by water. The ancestor of the D. glomerata clade could have been wind-dispersed through Panama Isthmus. Physiographic conditions in Mexico at that time must have facilitated the introduction of coastal and low-elevation species through efficient mechanisms of long-distance seed dispersal (e.g., ancestors of Dalbergia glomerata and D. granadillo). These species then evolved in the Sierra Madre del Sur, which was still active during the early Miocene, and, later on, in some areas during the Pleistocene (Ferrari et al., 2005; Moran-Zenteno et al., 2007). Likewise, the complex Trans-Mexican Volcanic Belt generated hundreds of scenarios in Central Mexico from the Miocene to the present (Ferrari et al., 2012) promoting population divergence, and thus speciation.
Ancestors from Asia and Africa must have arrived in America due to long-distance dispersal. How did they arrive could have been by different routes. One of these ways could have been by ocean currents. The tropical Atlantic belts where surface currents and winds are simultaneously favorable for East-West crossing are found between the Congo delta and the Maranhão in Brazil and just North of the Senegal river delta and Northern Brazil and the Guianas. Both streams originate in river deltas in Africa. Parrish (1993) has suggested “rafting” transport of organisms between South America and Africa during the Tertiary and was probably predominantly from East to West rather than the other way around (Renner, 2004). Although these currents may have been different during the warm climates of the Eocene–Miocene, there is no evidence that they were different from those of today. The only current that may have been different is the one in the vicinity of the Isthmus of Panama, before it closed. There are also data that the Rio Grande rise (Southeastern of the coast in Brazil) and the western end of the Walvis Ridge (Southwest African Coast, Cape Town) may have been above water until the Oligocene (Parrish, 1993; Morley, 2000), reducing the distance between continental coasts. Another form of long-distance dispersal using ocean currents may have been by fruit flotation. Currently, we have a clear example with Dalbergia ecastaphyllum and D. monetaria found in America but also in the western portion of Africa and whose fruits are frequent buoyants in marshes and rivers and can remain floating up to nine months (Gunn et al., 1976). There are Dalbergia species reported in the literature as Dalbergia monosperma that are waterborne (Ridley, 1990). Some of the Asian species of Dalbergia have fruits with similar characteristics to D. ecastaphyllum and D. monetaria to be transported, because they have coriaceous to woody fruits with a single seed which would form an air chamber inside, allowing them to float (e.g., Dalbergia albertesii, Dalbergia beccarii, D. horrida, and D. tamarindifolia).
Other options for long-distance transport are migratory birds, but except for some Psittacidae that consume Dalbergia seeds, there are no migratory species that could transport them from Asia to America or vice versa. While only ocean currents are heard as consistent for Dalbergia to be dispersed over long distances, all of the above mechanisms together could shape the diversity encountered in the genus today. Species distribution must also be related to soil type and microbiome. Rasolomampianina et al. (2005) found 68 strains of fixative bacteria in eight endemic Dalbergia species from Madagascar. Some of these strains such as Bradhirhizobium are common in tropical legumes, but the others are specific.
Concluding remarks
The reconstructed evolutionary history of Dalbergia from Mexico and Central America provides insights on how the number of species present in the area may have originated.
Regarding genetic barcodes, the most commonly used for Dalbergia have been ITS, matK, and rbcL, either alone or in different combinations (Bhagwat et al., 2015; Hassold, 2015; Li et al., 2017). Li et al. (2017) recommend the combination ITS + matK + rbcL to identify Dalbergia species. Our results show that, for species from Mexico, Central America, and the Caribbean, the ITS region is acceptable to distinguish at the species level, and in combination with chloroplast markers, we can know the area of provenance. Hassold (2015), in her study with chloroplast markers, indicates that plastid sequences reflect the geographical range and shared haplotypes between species. The data obtained in this study demonstrate that the whole piece of ITS alone can help us to differentiate between Dalbergia species. If the area of provenance is also required, it will be necessary to use chloroplast sequences.
Data availability statement
The datasets presented in this study can be found in online repositories. The names of the repository/repositories and accession number(s) can be found in the article/Supplementary Material.
Author contributions
SS designed the study, collected the materials, conducted the experiments, drafted the manuscript, and secured funding for the project. EP-O conceived and conducted the bioinformatic analyses, reviewed the manuscript, and assisted in the discussions. EM-S assisted in the discussions and reviewed the manuscript. JL provided taxonomic advice, reviewed the manuscript, and assisted in the discussions. LC trained students in the laboratory and performed a first draft assembly of sequences generated by this study. All authors contributed to the article and approved the submitted version.
Funding
This study was supported by a grant from PAPIIT project IA2015 to SS and operative funding to SS from Instituto de Biología.
Acknowledgments
We thank Berenice Mendoza, Mayra Castillo, and José Antonio Contreras-Chijate for their help in the laboratory. Our special gratitude goes to Laura Márquez and Nelly López-Ortiz for sequencing.
Conflict of interest
The authors declare that the research was conducted in the absence of any commercial or financial relationships that could be construed as a potential conflict of interest.
Publisher’s note
All claims expressed in this article are solely those of the authors and do not necessarily represent those of their affiliated organizations, or those of the publisher, the editors and the reviewers. Any product that may be evaluated in this article, or claim that may be made by its manufacturer, is not guaranteed or endorsed by the publisher.
Supplementary material
The Supplementary Material for this article can be found online at: https://www.frontiersin.org/articles/10.3389/fevo.2022.910250/full#supplementary-material
Supplementary Figures 1–5 | Distribution maps by species.
Supplementary Figure 6 | Haplotype network for plastid markers of Dalbergia.
Supplementary Table 1 | List of Dalbergia species and allies used in this study.
Footnotes
References
Akaike, H. (1974). A new look at the statistical model identification. IEEE Trans. Automat. Control 19, 716–723.
Barragán-Huerta, B. E., Peralta-Cruz, J., González-Laredo, R. F., and Karchesy, J. (2004). Neocandenatone, an isoflavan-cinnamylphenol quinone methide pigment from Dalbergia congestiflora. Phytochemistry 65, 925–928. doi: 10.1016/j.phytochem.2003.11.011
Bentham, G. (1860). Synopsis of Dalbergieæ, a tribe of leguminosæ. J. Proc. Linn. Soc. Lond. Bot. 4, 1–128. doi: 10.1111/j.1095-8339.1860.tb02464.x
Bhagwat, R. M., Dholakia, B. B., Kadoo, N. Y., and Balasundaran, M. (2015). Two new potential barcodes to discriminate Dalbergia species. PLoS One 10:e0142965. doi: 10.1371/journal.pone.0142965
Bouckaert, R., Vaughan, T. G., Barido-Sottani, J., Duchêne, S., Fourment, M., Gavryushkina, A., et al. (2019). BEAST 2.5: an advanced software platform for Bayesian evolutionary analysis. PLoS Comput. Biol. 15:e1006650. doi: 10.1371/journal.pcbi.1006650
Cardoso, D. B., Mattos, C. M., Filardi, F. L., Delgado-Salinas, A., Lavin, M., Moraes, P. L., et al. (2020). A molecular phylogeny of the pantropical papilionoid legume Aeschynomene supports reinstating the ecologically and morphologically coherent genus Ctenodon. Neodiversity 13, 1–38. doi: 10.13102/neod.131.1
Carvalho, A. M. (1989). Systematic Studies of the Genus Dalbergia L.f. in Brazil. Reading: University of Reading.
Carvalho, A. M. (1997). A synopsis of the genus Dalbergia (Fabaceae: Dalbergieae) in Brazil. Brittonia 49, 87–109. doi: 10.2307/2807701
CBOL Plant Working Group (2009). A DNA barcode for land plants. Proc. Natl. Acad. Sci. U.S.A. 106, 12794–12797. doi: 10.1073/pnas.0905845106
Choo, L. M., Forest, F., Wieringa, J. J., Bruneau, A., and de la Estrella, M. (2020). Phylogeny and biogeography of the Daniellia clade (Leguminosae: Detarioideae), a tropical tree lineage largely threatened in Africa and Madagascar. Mol. Phylogenet. Evol. 146:106752. doi: 10.1016/j.ympev.2020.106752
Cody, S., Richardson, J. E., Rull, V., Ellis, C., and Pennington, T. (2010). The Great American Biotic Interchange revisited. Ecography 33, 326–332. doi: 10.1111/j.1600-0587.2010.06327.x
Darriba, D., Taboada, G. L., Doallo, R., and Posada, D. (2012). jModelTest 2: more models, new heuristics and high-performance computing. Nat. Methods 9:772. doi: 10.1038/nmeth.2109
Ferrari, L., Orozco-Esquivel, T., Manea, V., and Manea, M. (2012). The dynamic history of the Trans-Mexican Volcanic Belt and the Mexico subduction zone. Tectonophysics 522–523, 122–149. doi: 10.1016/j.tecto.2011.09.018
Ferrari, L., Valencia-Moreno, M., and Bryan, S. (2005). Magmatismo y tectónica en la Sierra Madre Occidental y su relación con la evolución de la margen occidental de Norteamérica. Boletín de la Soc. Geol. Mexicana 57, 343–378. doi: 10.18268/bsgm2005v57n3a5
Gunn, C. R., Dennis, J. V., and Paradine, J. (1976). Gunn World Guide To Tropical Drift Seeds and Fruits. New York, NY: Demeter Press.
Hamburger, M. O., Cordell, G. A., Tantivatana, P., and Ruangrungsi, N. (1987). Traditional Medicinal Plants of Thailand, VIII. Isoflavonoids of Dalbergia candenatensis. J. Nat. Prod. 50, 696–699. doi: 10.1021/np50052a020
Hartvig, I., Czako, M., Kjær, E. D., Nielsen, L. R., and Theilade, I. (2015). The Use of DNA Barcoding in Identification and Conservation of Rosewood (Dalbergia spp.). PLoS One 10:e0138231. doi: 10.1371/journal.pone.0138231
Hassold, S. (2015). Molecular Identification of Malagasy Dalbergia species (rosewoods) for Biodiversity Conservation. Ph.D. thesis. Zürich: ETH Zurich, doi: 10.3929/ethz-a-010782581
Hung, T. H., So, T., Sreng, S., Thammavong, B., Boounithiphonh, C., Boshier, D. H., et al. (2020). Reference transcriptomes and comparative analyses of six species in the threatened rosewood genus Dalbergia. Sci. Rep. 10:17749. doi: 10.1038/s41598-020-74814-2
Katoh, K., Asimenos, G., and Toh, H. (2009). Multiple alignment of DNA sequences with MAFFT. Methods Mol. Biol. 537, 39–64. doi: 10.1007/978-1-59745-251-9_3
Kelchner, S. A., and Clark, L. G. (1997). Molecular evolution and phylogenetic utility of the chloroplast rpl16 intron in Chusquea and the Bambusoideae (Poaceae). Mol. Phylogenet. Evol. 8, 385–397. doi: 10.1006/mpev.1997.0432
Klitgård, B., and Lavin, M. (2005). “Dalbergieae,” in Legumes of the World, eds G. P. Lewis, B. Schrire, B. MacKinder, and M. Lock (Kew, UK: Royal Botanic Gardens), 307–335.
Larsson, A. (2014). AliView: a fast and lightweight alignment viewer and editor for large datasets. Bioinformatics 30, 3276–3278. doi: 10.1093/bioinformatics/btu531
Lavin, M., Herendeen, P. S., and Wojciechowski, M. (2005). Evolutionary rates analysis of leguminosae implicates a rapid diversification of lineages during the tertiary. Syst. Biol. 54, 575–594. doi: 10.1080/10635150590947131
Lavin, M., Schrire, B. P., Lewis, G., Pennington, R. T., Delgado-Salinas, A., et al. (2004). Metacommunity Process Rather than Continental Tectonic History Better Explains Geographically Structured Phytogenies in Legumes. Philos. Trans. Biol. Sci. 359, 1509–1522. doi: 10.1098/rstb.2004.1536
Lavin, M., Wojciechowski, M. F., Richman, A. D., Rotella, J. J., Sanderson, M. J., and Beyra-Matos, A. (2001). Identifying tertiary radiations of fabaceae in the greater antilles: alternatives to cladistic vicariance analysis. Int. J. Plant Sci. 162, S53–S76.
Li, Q., Wu, J., Wang, Y., Lian, X., Wu, F., Zhou, L., et al. (2017). The phylogenetic analysis of Dalbergia (Fabaceae: Papilionaceae) based on different DNA barcodes. Holzforschung 71, 939–949. doi: 10.1515/hf-2017-0052
Lianhe, Z., Li, W., Xing, H., and Zhengxing, C. (2011). Antioxidant activities of seed extracts from Dalbergia odorifera T. Chen. Afr. J. Biotechnol. 10, 11658–11667.
Linares, J., and Sousa, M. (2007). Nuevas especies de Dalbergia (Leguminosae: Papilionoideae: Dalbergieae) en México y Centroamérica. Ceiba 48, 61–82.
Linares, J. L. (in press). Dalbergia. Flora Mesoamericana: Fabaceae a Begoniaceae, Vol. 3. St. Louis, MO: Missouri Botanical Garden.
Montes, C., Cardona, A., McFadden, R., Morón, S. E., Silva, C. A., Restrepo-Moreno, S., et al. (2012). Evidence for middle Eocene and younger land emergence in central Panama: implications for Isthmus closure. GSA Bull. 124, 780–799. doi: 10.1130/B30528.1
Moran-Zenteno, D., Cerca, M., and Keppie, J. D. (2007). “The Cenozoic tectonic and magmatic evolution of southwestern Mexico: advances and problems of interpretation,” in Geology of Mexico: Celebrating the Centenary of the Geological Society of Mexico, eds S. A. Alaniz-Alvarez and A. F. Nieto-Samaniego (Boulder, CO: Geological Society of America), 71–90. doi: 10.1130/2007.2422(03)
Niyomdham, C., Hô, P. H., Dy Phon, P., and Vidal, J. E. (eds) (1997). Flore du Cambodge, du Laos et du Viêtnam. 29 Légumineuses-Papilionoïdées-Dalbergiées. Paris: Muséum National d’Histoire Naturelle.
Pérez-Gutiérrez, R. M., and García-Baez, E. (2013). Cytotoxic activity of isoflavan-cinnamylphenols from Dalbergia congestiflora on HeLa cells. J. Med. Plants Res. 7, 2992–2998.
Pittier, H. (1922). On the Species of Dalbergia of Mexico and Central America. J. Wash. Acad. Sci. 12, 54–64.
Rasolomampianina, R., Bailly, X., Fetiarison, F., Rabevohitra, R., Béna, G., Ramaroson, L., et al. (2005). Nitrogen-fixing nodules from rosewood legume trees (Dalbergia spp.) endemic to Madagascar host seven different genera belonging to α-and β-Proteobacteria. Mol. Ecol. 14, 4135–4146. doi: 10.1111/j.1365-294X.2005.02730.x
Renner, S. S. (2004). Plant dispersal across the tropical Atlantic by wind and sea currents. Int. J. Plant Sci. 165, S23–S33.
Ribeiro, R. A., Lavin, M., Lemos-Filho, J. P., Mendonça-Filho, C. V., Rodrigues dos Santos, F., and Lovato, M. B. (2007). The genus Machaerium (Leguminosae) is more closely related to Aeschynomene sect. Ochopodium than to Dalbergia: inferences from combined sequence data. Syst. Bot. 32, 762–771. doi: 10.1600/036364407783390700
Richter, H. G., Krause, V. J., and Muche, C. (1996). Dalbergia congestiflora Standl.: wood structure and physico-chemical properties compared with other Central American species of Dalbergia. IAWA J. 17, 327–341. doi: 10.1163/22941932-90001583
Ricker, M., Hernández, H. M., Sousa, M., and Ochoterena, H. (2013). Tree and tree-like species of Mexico: Asteraceae, Leguminosae, and Rubiaceae. Rev. Mex. Biodivers. 84, 439–470. doi: 10.7550/rmb.32013
Rutiaga-Quiñones, J. G., Pedraza-Bucio, F. E., and López-Albarrán, P. (2010). Componentes químicos principales de la madera de Dalbergia granadillo Pittier y de Platymiscium lasiocarpum Sandw. Rev. Chapingo Ser. Cienc. For. Ambient. 16, 179–186. doi: 10.5154/R.RCHSCFA.2010.04.023
Rutiaga-Quiñones, J. G., Windeisen, E., and Schumacher, P. (1995). Anti fungal activity of heartwood extracts from Dalbergia granadillo and Enterolobium cyclocarpum. Holz als Rohund Werkstoff 53, 308–308.
Sainz-Reséndiz, B. A. (2011). Descripción e identificación de maderas del Paleógeno de San Juan Atzingo, Puebla, México, Facultad de Estudios Superiores, Iztacala. Ph.D. thesis. México: Universidad Nacional Autónoma de México.
Schley, R. J., Qin, M., Vatanparast, M., Malakasi, P., de la Estrella, M., Lewis, G. P., et al. (2022). Pantropical diversification of padauk trees and relatives was influenced by biome-switching and long-distance dispersal. J. Biogeogr. 49, 391–404. doi: 10.1111/jbi.14310
SEMARNAT (2010). Norma Oficial Mexicana NOM-059-SEMARNAT-2010, Protección ambiental – Especies nativas de México de flora y fauna silvestres – Categorías de riesgo y especificaciones para su inclusión, exclusión o cambio – Lista de especies en riesgo. Diario Oficial de la Federación 30 de diciembre de 2010. Mexico: SEMARNAT.
Sousa, S. M., Ricker, M., and Hernández, H. M. (2001). Tree Species of the Family Leguminosae in Mexico. Harv. Pap. Bot. 6, 339–365.
Stucky, B. J. (2012). SeqTrace: a graphical tool for rapidly processing DNA sequencing chromatograms. J. Biomol. Tech. 23, 90–93. doi: 10.7171/jbt.12-2303-004
Sun, Y., Skinner, D. J., Liang, G. H., and Hulbert, S. H. (1994). Phylogenetic analysis of Sorghum and related taxa using internal transcribed spacers of nuclear ribosomal DNA. Theor. Appl. Gen. 89, 26–32.
Thothathri, K. (1987). Taxonomic Revision of the Tribe Dalbergieae in the Indian Subcontinent. Kolkata: Botanical Survey of India.
Vatanparast, M., Klitgård, B., Frits, A. C., Adema, R., Pennington, T., Yahara, T., et al. (2013). First molecular phylogeny of the pantropical genus Dalbergia: implications for infrageneric circumscription and biogeography. S. Afr. J. Bot. 89, 143–149. doi: 10.1016/j.sajb.2013.07.001
von Taubert, P. (1894). III sb. Papilionatae-Dalbergieae-Lonchocarpinae. Nat. Pflanzenfamilien 3, 341–348.
Wirth, W., and Duchene, S. (2021). Real-Time and Remote MCMC Trace Inspection with Beastiary. bioRxiv [Preprint]. doi: 10.1101/2021.11.21.469478v1
Yu, G. (2020). Using ggtree to visualize data on tree-like structures. Curr. Prot. Bioinformatics 69:e96. doi: 10.1002/cpbi.96
Keywords: barcode, Dalbergia, diversification, Miocene, Mexico
Citation: Sotuyo S, Pedraza-Ortega E, Martínez-Salas E, Linares J and Cabrera L (2022) Insights into phylogenetic divergence of Dalbergia (Leguminosae: Dalbergiae) from Mexico and Central America. Front. Ecol. Evol. 10:910250. doi: 10.3389/fevo.2022.910250
Received: 01 April 2022; Accepted: 28 June 2022;
Published: 28 July 2022.
Edited by:
Jonathan J. Fong, Lingnan University, ChinaReviewed by:
Harald Schneider, Xishuangbanna Tropical Botanical Garden (CAS), ChinaMaria M. Romeiras, University of Lisbon, Portugal
Copyright © 2022 Sotuyo, Pedraza-Ortega, Martínez-Salas, Linares and Cabrera. This is an open-access article distributed under the terms of the Creative Commons Attribution License (CC BY). The use, distribution or reproduction in other forums is permitted, provided the original author(s) and the copyright owner(s) are credited and that the original publication in this journal is cited, in accordance with accepted academic practice. No use, distribution or reproduction is permitted which does not comply with these terms.
*Correspondence: Solange Sotuyo, anNzb3R1eW9AaWIudW5hbS5teA==
†These authors have contributed equally to this work and share first authorship