- 1College of Geography and Environmental Science, Henan University, Kaifeng, China
- 2Key Laboratory of Geospatial Technology for the Middle and Lower Yellow River Regions, Ministry of Education, Henan University, Kaifeng, China
- 3Regional Planning and Development Center, Henan University, Kaifeng, China
- 4School of Government, Central University of Finance and Economics, Beijing, China
Land-use change is a global issue, and the built-up land expansion has affected the ecological landscape patterns of the major river basins in the world. However, measurement of the ecological risks of potential landscape and identification of the dynamic relationships by natural and human-driven built-up land expansion at different zoning scales are still less understood. Based on multi-period Landsat satellite image data, we combined remote sensing (RS) and geography information systems (GIS) technologies with Spatial Durbin Panel Model to quantitatively analyze the landscape ecological effects under the built-up land expansion in the Yellow River Basin. The results showed that there is spatial heterogeneity in the built-up land expansion and ecological security patterns, with the expansion gravity center gradually spreading from the downstream to the middle and upstream areas, and the most dramatic change in landscape patches of ecological safety patterns occurring around the year 2000. At different zoning scales, there is a spatial spillover effect on the interaction between built-up land expansion and ecological security, with the significance of the regression estimates decreasing from large sample sizes to small sample sizes. Our findings highlighted the importance of spatial heterogeneity at different zoning scales in identifying the dynamic relationship between built-up land expansion and ecological security, scientific planning of land resources, and mitigation of ecological and environmental crises.
Introduction
The impact of human activities on ecosystems has gradually increased in the Anthropocene era and has become a dominant factor in ecosystem evolution (Best, 2019). In the past few decades, the world’s built-up land expansion has exceeded the population growth rate (Flörke et al., 2018), with far-reaching implications for biodiversity and water, carbon, and nitrogen cycles in local and global climate systems (Chen et al., 2020). In 2018, the global built-up land area was nearly 8 km × 105 km, which is 1.5 times of that in 1990 (Gong et al., 2020). In the future, built-up land is expected to continue to expand globally, reaching three times its 2000 level by 2030 (Seto et al., 2012). Nearly 60% of the population lives in urban built-up areas (Narducci et al., 2019). Although the built-up land expansion provides opportunities for human, social, and economic development, the expansion also leads to the loss of more than 80% of natural habitats (Li et al., 2021), threatening the sustainability of human survival (Elmqvist et al., 2019; Zhang et al., 2020b). Therefore, in this “urban age,” understanding how the expansion of built-up land will affect other land covers and how to protect and optimize the pattern of ecological security is essential for resolving social and environmental problems that challenge the sustainable development of human society (Zhou et al., 2020).
Ecological security is considered a basic component of regional security (Ghosh et al., 2021). Global competition for multiple land use has led to a sharp decrease in ecologically safe land (Lambin and Meyfroidt, 2011), which has a significant negative impact on ecosystem services and terrestrial biodiversity (Newbold et al., 2015). In particular, the loss of natural habitats, such as woodlands and water bodies, has significantly increased global greenhouse gas emissions (Tubiello et al., 2015). Land-use change is one of the important factors leading to the loss of ecological security land (Cayuela et al., 2015). The impact of built-up land expansion on ecological processes is currently gaining an increasing amount of attention and debate (Lausch et al., 2015; Guo et al., 2020; Zhang et al., 2020c). Between 1982 and 2016, global land-use change resulted in significant woodland loss: Paraguay lost 34% of its total area of 7.9 km × 104 km; Argentina lost 25% of its total area of 11.3 km × 104 km; and Brazil lost 8% of the forest area of its total area of 38.5 km × 104 km (Song et al., 2018). It is expected that natural habitats will be reduced by 26–58% (Jantz et al., 2015), 39% of ecological security land will be occupied by built-up land and cropland, and 37% will be degraded and segmented from 2005 to 2100 (Ellis et al., 2010). Alcamo et al. (2005) pointed out that by 2050, 10–20% of woodlands and water bodies will be occupied by built-up land and cropland. Therefore, it is increasingly important to explore the impact of built-up land change on natural habitats.
Exploring the impact of past and future built-up land expansion on ecological security has become a key link in landscape analysis (Yang et al., 2020; Stoica et al., 2021). Wihbey (2017) predicted that global built-up land will increase to nearly 300 km × 104 km by 2050. The built-up land not only occupies natural habitats but also threatens local food security and biodiversity (d’Amour et al., 2017; Van Vliet, 2019; McDonald et al., 2020). Furthermore, it affects the regional and global biogeochemical cycles (Huang et al., 2019), resulting in increased pollutant emissions and natural disaster risks (Güneralp et al., 2015). The methods for balancing economic development and the ecological environment in regions experiencing rapid expansion are also being discussed. McDonald et al. (2018) believe that the establishment of ecological priority zones can effectively mitigate biodiversity loss due to the built-up land expansion. To avoid placing more economic assets at risk, it is necessary to prevent and control floods and other disasters within the basin (Mård et al., 2018). Although most scholars have discussed the impact of built-up land expansion on the ecological environment at different levels, it is mostly at the macro level, and studies focused on forest land and water bodies are limited.
The river basin is an important unit for studying the coupling of natural and human processes (Gleeson et al., 2020). Nearly 40% of the global built-up land expansion occurs near river basins, such as the Mississippi River Basin and the Yangtze River Basin (Huang et al., 2021). In addition, built-up land expansion at most of the world’s watershed scales is in line with the S Northam curve model of urbanization (Xu et al., 2020). The model helps developed economies to reduce the changes in the biogeochemical cycle, while the impact of the model on developing economies cannot be overlooked (Wiedmann and Lenzen, 2018). The Yellow River Basin is not only an important ecological barrier area in China, but also, at the same time, an area lagging in economic and social development and the current fight against poverty. The region is facing regional development imbalance, ecological function degradation, ecosystem connectivity, and integrity weakening (Rong et al., 2020). The transformation and development of the region have become an urgent problem that can be solved by finding ways to meet the needs of regional green development with limited land resources.
The issue of scale is central to landscape ecology, and because differences in the physical geography and socioeconomic conditions of regions at different scales can lead to large differences and even reverse outputs, it is necessary to make multiscale attempts to enhance the reliability of research results (Xiao et al., 2022). The expansion of built-up land and its impacts are not limited to the boundaries of administrative divisions but are reflected in different natural ecological backgrounds. Existing studies have examined the expansion of built-up land from the perspective of administrative divisions but lacked a comprehensive understanding of different divisions. Our study investigated the characteristics of built-up land expansion and its impact on ecological security patterns from three zoning perspectives: administrative zoning, eco-geographical zoning, and agricultural natural zoning, and then revealed the spatial spillover effects of the complex relationship between built-up land expansion and ecological security patterns in different regions. New methods and perspectives for studying this relationship are necessary to avoid conflicting and inconsistent outcomes in research, which can lead to the formulation of inappropriate policies. The aim of our research is to examine the relationship between human activities and regional ecological security patterns at the watershed scale using a perspective that provides more confidence and reliable results than traditional perspectives in the field. We argue that regional governance should move from administrative boundaries to multiple zoning perspectives; thus, it is necessary to resolve land-use conflicts through scientific land use planning and management to achieve ecological civilization.
Materials and Methods
Overview of the Study Area
The Yellow River Basin is located in the central and eastern regions of China. It is the birthplace of Chinese civilization (Zhang et al., 2021). In 2019, the ecological protection and high-quality development strategy in the Yellow River Basin provide new directions for watershed management and protection. Promoting the construction of an ecological civilization in the Yellow River Basin is a long-term goal.
The terrain of Yellow River Basin is high in the west and low in the east, with a total area of approximately 79.5 km × 104 km. Among them, the built-up land area increased from 3.9 km × 104 km in 1980 to 6.2 km × 104 km in 2018 (Figure 1). By the end of 2018, the per capita GDP was 60,000 yuan, which was lower than the national average GDP (64,000 yuan). The vegetation coverage of the basin decreased significantly due to climate change and soil erosion, with threatened species accounting for 15–20%, which is higher than the world average (10–15%) (Jing et al., 2020). Since the 1990s, a series of major ecological security protection projects launched by government departments have curbed the degradation trend of ecosystem in some regions. However, the gradual improvement of the ecological environment does not imply that the previous development methods can be restored. We need to pay attention to the ecological security of the basin and seek sustainable land development strategies.
Regional Division
Regional development may have an impact on adjacent areas (Kopp and Allen, 2021). This study aims to analyze the interactive relationship between multiple spatial zoning scales to test the spatial spillover effect of the relationship between built-up land expansion and ecological security.
First, the use of administrative division data improves the accuracy of spatial dependence capture (Liu and Wu, 2021). Prefecture-level cities were chosen as the analysis scale (Figure 2A).
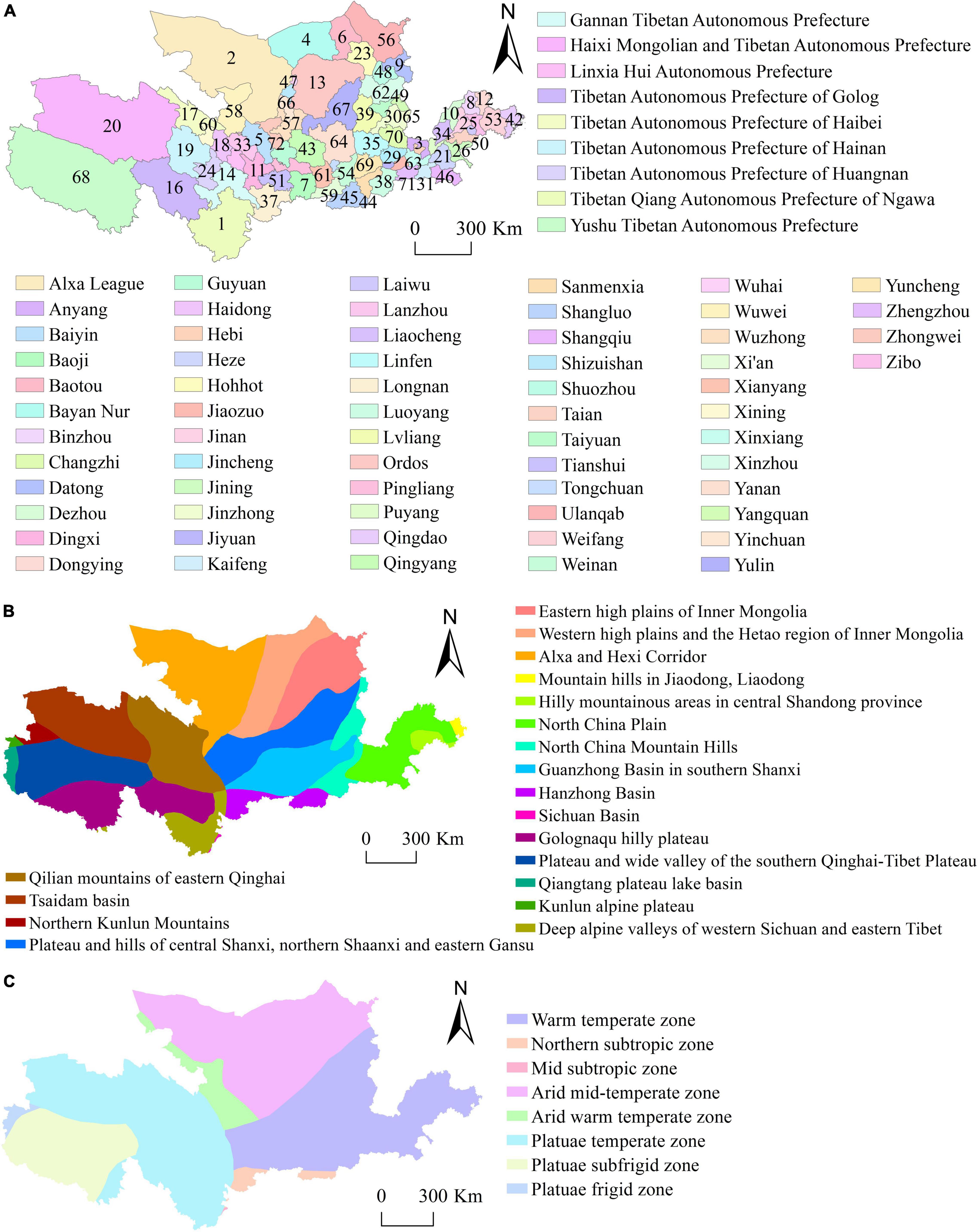
Figure 2. Regional delineation of the study area. (A) Administrative zoning; (B) eco-geographical zoning; (C) agricultural natural zoning.
Second, the Yellow River Basin spans the eastern, central, and western parts of China, with a wide range of climatic, geomorphological, and geological conditions and resource endowments. We selected the eco-geographical zoning scale based on geographic relativity (i.e., geographical differences in nature are not absolute, and geographical changes are often in a state of development and gradual transition) and the main ecosystem elements (i.e., the spatial location of various ecosystem factors changes over time, and their manifestations are diverse, e.g., encompassing plains, hills, and basins) (Figure 2B).
Third, the Yellow River Basin is the largest agricultural base in China, spanning all climate zones in northern China. The agricultural natural zoning scale was selected based on differences in natural climatic conditions and temperatures (Figure 2C).
Variables Selection
The aim of this study was to analyze the impact of built-up land expansion on the ecological security pattern in the Yellow River Basin. Therefore, it is necessary to define variables, introduce data sources, and provide empirical models. The following specific variables were selected for the study:
(1) Explained variables: Built-up land to correlate the surface area variables of built-up land and polygon landscape pattern variables, according to different spatial scales. The area calculated by ArcGIS 10.2 software spatial statistical tool was used as the expansion level of built-up land (referred to as LUE).
(2) Core explanatory variables: The study comprehensively considers the spatial resolution of data and refers to relevant studies (Yang et al., 2018; Bosch et al., 2020) selected five landscape pattern indexes from patch and landscape types to comprehensively characterize the landscape ecological effect of built-up land expansion (Riitters et al., 1995; Table 1).
Model Establishment
Transfer Matrix of Land Use
The land use transfer matrix is a quantitative description of the state and state transfer in a specific period and uses the transfer matrix to measure the dynamic information of land use in that period (Anees et al., 2019). This method is considered one of the most commonly used landscape change detection methods (Ghosh et al., 2016). The calculation formula is as follows:
where S is the land area, a and b are pre-transfer and post-transfer land-use types, respectively (a, b = 1, 2, …, n), and n is the total number of land-use types.
Landscape Expansion Index
Landscape indices are used to express landscape heterogeneity within a region (Kowe et al., 2021). To comprehensively reflect the landscape pattern and characteristics and reduce information redundancy, this study used the traditional landscape index calculation method, based on the Fragstats 4.2 software, to depict the landscape status and landscape pattern fragmentation, heterogeneity, and connectivity from a macro perspective. The specific landscape pattern index is summarized in Table 1. According to the scope of the research area, it has been determined through multiple debugging that the land use data of the Yellow River Basin should be resampled to 200 m to better reflect the changes in the study area.
Spatial Durbin Panel Model
To future reveal the spatial spillover effect of built-up land expansion, we introduced the Spatial Durbin Panel Model (SDPM) for referring to the research results of Elhorst (2014) and Chen et al. (2017). The maximum likelihood method was used to estimate the parameters, which tests the spatial, temporal, and spatiotemporal correlations of the explained variables (Anselin et al., 2008). Based on this, the initial model was set to the SDPM with the following formula:
where yit is the built-up land expansion of region i in period t, and i and j are regional individuals, N is the number of sample units, wij is the spatial weight matrix, α is a constant term, xit is the explanatory variable, β is the regression coefficient of each variable, ρ is a space regression coefficient, wijxit is the spatial lag term, θ is the coefficient of the spatial lag term, μi and λt are individual fixed effects and individual time effects, respectively, and εit is a random error term.
The Likelihood Ratio test and the Wald test are used to verify the original hypothesis: when θ = 0, SDPM is the same as the spatial lag panel model (SLPM), and when θ = -ρβ, SDPM is the same as the spatial error panel model (SEPM). According to this model, spatial econometric regression estimations were conducted for different zoning variables.
The spatial weight matrix (wij) is the core element of the spatial econometric model (Feng et al., 2019). This study selected the adjacency matrix (w) as the benchmark space matrix referring to the study of Lesage and Pace (2014), as follows:
Data Source
To study the impact of built-up land expansion on regional ecological security patterns, the Yellow River Basin panel data (covering 73 prefecture-level and above cities in China) from 1980 to 2018 were selected. Figure 3 shows the overview chart of the article. The basic data used in the study were 100 m resolution land-use datasets from 1980, 1990, 2000, 2010, and 2018. The zoning data were derived from http://www.resdc.cn. The production of the land use data set was based on the remote sensing images of Landsat TM/ETM of each period as the main data source. The built-up land, ecological security pattern land (woodland, water body), and transition land (cropland, grassland, and unused land) at each time point were generated by manual visual interpretation based on the “source–sink” theory (Chen et al., 2006). Among them, the built-up land is the interference source area, the ecological security pattern land is the sink area disturbed by the source area, and the other land is the source-sink transition area. At the same time, to avoid the potential heteroscedasticity of the data and the dependence on the setting of the regression model, all variables were converted into natural logarithms (Table 2).
Results
Evolutionary Characteristics of Spatiotemporal
Spatiotemporal Evolutionary Characteristics of Built-Up Land Expansion
The built-up land in the Yellow River Basin expanded rapidly from 1980 to 2018, with significant spatial and temporal variations (Figure 4). In terms of land-use change (Table 3), the built-up land area expanded by 58.83% from 39,061.56 km2 in 1980 to 62,042.20 km2 in 2018. The difference in the expansion level was greatly affected by the location. The expansion level in the eastern plains was significantly higher than in the western region, and the remaining areas were concentrated in the provincial capital. The expansion was mainly concentrated in provinces of the Lower Yellow River Basin, with the most significant increase between 2010 and 2018 and gradually spreading to the middle and upper regions. Thus, the rapid development of built-up land in the downstream plains was the main feature during this period.
Spatiotemporal Evolutionary of Ecological Security Pattern
The variation range of ecological security land was not significant, and the overall decrease was dominated by patches (Figure 5). The ecological security land increased from 246,358.87 km2 in 1980 to 247,421 km2 in 2018. In space, ecological security land shows a trend of gradual increase from east to west. The western region was mainly characterized by scattered patches reduction, while the eastern region had a relatively significant reduction along the river channel, and the rest of the region had fewer local patches. It was observed that the ecological environment in the basin is still relatively fragile, the ecological security pattern in the upper and middle region is significantly degraded, and the wetland in the downstream area is shrinking.
The landscape is a comprehensive reflection of environmental and anthropogenic activities, revealing the succession law of landscape elements and ecological security patterns in the study area (Keita et al., 2021). In this study, COHESION, LSI, PLAND, LPI, and PD were selected to reflect the landscape characteristics of the ecological security pattern in the basin (Figure 6). During the study period, COHESION was in a horizontal state, indicating a good overall spatial connectivity of the ecological security pattern in the basin. Although the change was minimal, it showed a decreasing trend year by year, indicating that the landscape patches of the ecological security pattern had a potential trend of dispersion. The LSI and COHESION indices trends were basically the same, but the LSI value was always high, indicating a complex landscape shape in the basin. PLAND, LPI, and PD decreased sharply around 2000 and then gradually stabilized, indicating that the ecological security pattern in the basin has gradually decreased since 2000. The most significant change in the pattern of ecological security in the basin was observed around 2000.
Impact of Built-Up Land Expansion on Ecological Security Patterns
The spatial agglomeration state of expansion level was observed to be increasing through a horizontal comparison of the relationship between built-up land expansion and the ecological security pattern index at the city level. Based on this, the SDPM under the time-fixed effect was further determined by combining the significance LR test. The variables with collinearity were eliminated and the four explanatory variables (PLAND, LPI, LSI, and PD) were retained to eliminate the possibility of multiple collinearities among variables (Table 4). In terms of the total effect, the response degree of each variable was: LSI > PLAND > LPI > PD.
In terms of the direct effect, the LPI and LSI coefficients were significantly negative at the 1% level, implying that the LPI and LSI of ecological security pattern significantly slowed down the built-up land expansion. The impact of LPI on ecological security pattern was opposite to that of built-up land expansion, which further verified that the estimation results of this study are highly feasible and reliable. The PD coefficient was significantly positively correlated at the 1% level and belonged to the index describing the overall pattern change in landscape types, implying that the explanatory variable significantly increased the built-up land expansion. The coefficient of PLAND was positive but not significant, indicating that the proportion of patches in the ecological security pattern has little effect on the built-up land expansion. The mutual transformation between built-up land expansion and transition land is higher than that of ecological security land, whereas the relevant measures to protect the ecological security pattern have different impacts on the built-up land. In terms of indirect effects, the coefficients of PLAND, LPI, and LSI were significantly correlated at the levels of 5, 1, and 1%, respectively, indicating that the land-use expansion will affect the local ecological security pattern as well as the ecological security pattern of adjacent areas.
The time lag coefficient of built-up land in the model was significantly positive at the 1% level, that is, the time dependence of built-up land significantly accelerated the expansion in the basin. Although the built-up land expansion in surrounding areas will intensify the expansion of local built-up land in the current stage, it will also force the local government to strengthen land expansion control and improve land-use level in the next stage.
Regional Differences in the Effect of Built-Up Land Expansion on Ecological Safety Patterns
The difference in responses of variables cannot be further identified due to the uncertainty of human factors and natural conditions in administrative divisions. This study used two geographical divisions (i.e., eco-geographical zoning and agricultural natural zoning) to re-estimate and to further explore whether the expansion process of built-up land and ecological security pattern have established spatial heterogeneity at the regional level.
Differences in Eco-Geographic Zoning
Table 5 summarizes the estimation results of the SDPM for the time-fixed effect in eco-geographical zoning. The significance of each variable decreased when compared with the results for the entire watershed (Table 4). However, it is worth noting that the results (coefficients and symbols) of PLAND, LSI, and PD were different from those of the entire basin. First, the direct effect coefficient of PLAND was positive but not significant. The indirect and total effect coefficients were significantly negative at the 1% level, indicating that the proportion of patches in the ecological security pattern effectively slows down the expansion process. Although the patch shape is relatively complex, its effect on inhibiting the disorderly expansion of built-up land is significantly enhanced.
Second, the indirect effect and the total effect coefficient of LSI were significantly positively correlated at the 1 and 5% levels, respectively. The direct effect coefficient was also positive, but not significant, indicating that the more complex the patch shape under eco-geographical zoning, the faster the expansion of surrounding built-up land; the spatial spillover effect is relatively poor on the local area. Third, the direct effect coefficient of PD was positive, the indirect effect and the total effect coefficients were negative, and the three effects were not significant, indicating that the number of landscape patches per unit area was helpful in protecting the local ecological security pattern, but had less effect on the surrounding areas. The direct effect of LPI on the local was higher than that of the whole basin, and the indirect effect and total effect were not significant. The spatial spillover effect was not significant because of the significant regional differences under eco-geographical zoning. Moreover, the time-dependent positive impact of built-up land expansion is no longer as significant as that of the entire basin.
Differences in Agricultural Natural Zoning
Table 6 summarizes the estimation results of the SDPM with time-fixed effects in agricultural natural zoning. Compared with the estimation results presented in Table 4, the regression coefficient of variables improved, whereas the coefficient symbols of PLAND and LSI were inconsistent with the results of the whole basin. In terms of the indirect and total effects, only the PLAND and LSI coefficients were significant at the 5 and 1% levels, respectively, which is consistent with the eco-geographical zoning results, indicating that the effect of built-up land expansion on regional landscape patterns under the agricultural natural zoning differs at different stages of built-up land expansion compared with administrative zoning.
In terms of direct effect, the coefficients of PLAND and LPI were negative, but PLAND was not significant, indicating that the proportion of patches in agricultural natural zoning had no significant effect on the ecological security pattern. However, the effect of the proportion of the largest patch area in the total area is the highest among the three zonings, indicating that this variable had a strong impact on regional ecological security pattern. The LSI and PD coefficients were significantly positively correlated at the 1% level. It was observed that the impact of built-up land expansion on ecological security pattern existed in the change in patch area.
Discussion
Understanding Built-Up Land Expansion From a Watershed Perspective
Rivers around the world provide various ecosystem services and commodities for human livelihoods and are also important bases for global biodiversity (Zipper et al., 2020). Although the Yellow River is only 15% smaller than the Amazon River and the Nile River, the annual discharge and flow rates are relatively low (Wohlfart et al., 2016). We found ecosystem degradation, landscape fragmentation, and increasing landscape diversity and structural complexity in the watershed after years of intensive economic development and natural resources exploitation, which were consistent with the findings of Liu et al. (2018). In addition, the rapid expansion of built-up land in the area will easily trigger the heat island effect (Zhao et al., 2021), which will seriously hinder the high-quality development of the region (Chi et al., 2021; Song et al., 2021). Therefore, there is an urgent need to strengthen the ecological civilization of watersheds and scientifically control the total amount of built-up land to maintain a balance between economic development and ecological security. Our results at the watershed scale were useful for understanding the dynamics of built-up land expansion under different zoning levels on the watershed scale.
Many natural ecological patches have been occupied by urban activities, particularly in the last 10 years, driven by urban development and limited by national arable land protection policies (Zhang et al., 2020d), and some areas are extremely vulnerable to overexploitation of groundwater and large-scale logging (Fang et al., 2020; Lin et al., 2020). Due to the influence of human activities, the density of patches in the basin was significantly and positively correlated with the built-up land expansion (Kuenzer et al., 2014), which is closely related to the long-term maintenance of the artificial environment-dominated mode in traditional planning in China (Zhang et al., 2020a). The most significant areas of built-up land expansion were located in the eastern part of the basin, and the conversion between built-up land and ecological security land is most notable along the river, which is consistent with the trend of urban sprawl in other countries (Middleton and Sternberg, 2013; Berdugo et al., 2020). This implies that the expansion trend of built-up land may have a greater ecological impact on inland river basins. Therefore, more attention should be paid to the built-up land expansion in inland river basins with different types of situations through developmental policy control. In contrast, filling expansion and vertical growth are encouraged to improve land-use efficiency.
Effects of Built-Up Land Expansion on Ecological Security Patterns
In recent years, spatial issues have been extensively explored in regional development (Ertur and Koch, 2007; Cheng et al., 2021). Therefore, it is necessary to consider possible spatial correlations. Studies have shown that when built-up land expansion reaches a certain stage, it accelerates the expansion of adjacent landscape patches and merges into larger patches (Qian et al., 2015). Our study also confirms that the different landscape pattern indexes in different stages of built-up land expansion have different trends. Since the beginning of the 21st century, there have been varying degrees of disorderly development and illegal buildings in the north and south of the Qinling Mountains, resulting in a decrease in PLAND and LPI. In this process, plaque density and connectivity also change accordingly.
The proportion of built-up land in the study area has increased from 1.93 to 3.07% in the past 40 years, which is the most prominent change among the three land-use types based on the “source–sink” theory. However, the contribution of expansion to the loss of ecological security land was very small (0.80%). This is mainly due to the current urban planning scheme implemented by local governments, which correctly guides the direction of spatial development and protects most of the ecological security land (Xiao, 2021). However, because of the influence of the geographical environment, most of the ecological security land does not have the suitability factors for built-up land, which inhibits the expansion in the region (Jiang et al., 2021).
The ecological security model is mainly based on the development of ecosystem service functions and ecological carrying capacity (Liang et al., 2018; Liang et al., 2020). Built-up land expansion has been identified as the most important direct cause of the loss of natural landscape areas (McDonald et al., 2020; Winkler et al., 2021). The landscape pattern changes of ecological security through human activities (urban expansion, zoning creation, agricultural mechanization, etc.) can affect the ecological processes within the natural landscape area (Tanner and Fuhlendorf, 2018) and change the relationship between human beings and the natural environment (Pickard et al., 2017). The changes in landscape types and proportions are mainly reflected in the conversion of ecological land, such as woodlands and water bodies, to built-up land and cropland (Dadashpoor et al., 2019). At the same time, the landscape pattern of the rapid expansion area showed significant and highly dispersed characteristics. Single and continuous natural areas have gradually evolved into complex and discontinuous landscape patches (Li et al., 2017). Therefore, it is important to study the spatiotemporal variation characteristics of ecological security landscape patterns.
Conclusion
Land-use change is a global problem, and the built-up land expansion affects the ecological landscape pattern of the world’s major river basins. In our study, we consider that the development of one region may have an effect on the neighboring regions and that there is spatial heterogeneity between different zones. Therefore, we break away from the limitations of previous studies, which have mostly used administrative zones as boundaries, and explore the spatial spillover effects of the built-up land expansion in the Yellow River Basin on ecological security patterns based on three scales: Administrative zoning, Eco-geographical zoning, and Agricultural natural zoning. We found that the built-up land expanded rapidly, and spread from the downstream region to the middle and upper reaches, and the landscape patches of the ecological security pattern gradually decreased. At different zoning scales, the interactive relationship between built-up land expansion and ecological security showed a certain spatial spillover effect, and the significance of regression estimation gradually decreases from large sample size to small sample size. However, the underlying mechanisms controlling the complex relationship between the built-up land expansion and ecological security patterns within the basin are not elucidated yet and need further research. To optimize the ecological security pattern, in the future basin management, land use planning, and policy should break the limitations of administrative boundaries. Our research provides reference and significance for land development and urban planning regulation of the Yellow River Basin, as well as for other basins in China and other large river basins in developing countries, to seek sustainable urban planning and alleviate environmental pressure.
Data Availability Statement
The original contributions presented in the study are included in the article/supplementary material, further inquiries can be directed to the corresponding authors.
Author Contributions
PZ, DY, and LJ contributed to the conceptualization and writing, reviewing, and editing the manuscript. DY contributed to the methodology and writing the original draft. PZ and YL contributed to the supervision. PZ contributed to project administration and funding acquisition. All authors have read and agreed to the published version of the manuscript.
Funding
This research was funded by the National Natural Science Foundation of China, grant numbers 41601175 and 41801362, the Young Backbone Teachers Foundation from Henan Province, grant numbers 2018GGJS019 and 2020GGJS114, the Program for Innovative Research Talent in University of Henan Province, grant number 20HASTIT017, 2021 Project of Henan Soft Science Funds, grant number 212400410250 and 2020 Philosophy and Social Science Planning Project of Henan Province, grant number 2020BJJ020.
Conflict of Interest
The authors declare that the research was conducted in the absence of any commercial or financial relationships that could be construed as a potential conflict of interest.
Publisher’s Note
All claims expressed in this article are solely those of the authors and do not necessarily represent those of their affiliated organizations, or those of the publisher, the editors and the reviewers. Any product that may be evaluated in this article, or claim that may be made by its manufacturer, is not guaranteed or endorsed by the publisher.
Acknowledgments
We thank the Geographical Science Data Center of The Greater Bay Area for providing the relevant data in this study.
References
Alcamo, J., Van Vuuren, D., and Cramer, W. (2005). Changes in ecosystem services and their drivers across the scenarios. Ecosystems Hum. Well Being 2, 297–373.
Anees, M. M., Sajjad, S., and Joshi, P. K. (2019). Characterizing urban area dynamics in historic city of Kurukshetra, India, using remote sensing and spatial metric tools. Geocartol. Int. 34, 1584–1607. doi: 10.1080/10106049.2018.1499819
Anselin, L., Gallo, J. L., and Jayet, H. (2008). “Spatial Panel Econometrics,” in The Econometrics of Panel Data. Advanced Studies in Theoretical and Applied Econometrics, eds L. Mátyás and P. Sevestre (Berlin: Springer), 625–660. doi: 10.1007/978-3-540-75892-1_19
Berdugo, M., Delgado-Baquerizo, M., and Soliveres, S. (2020). Global ecosystem thresholds driven by aridity. Science 367, 787–790. doi: 10.1126/science.aay5958
Best, J. (2019). Anthropogenic stresses on the world’s big rivers. Nat. Nanotechnol. 12, 7–21. doi: 10.1038/s41561-018-0262-x
Bosch, M., Jaligot, R., and Chenal, J. (2020). Spatiotemporal patterns of urbanization in three Swiss urban agglomerations: insights from landscape metrics, growth modes and fractal analysis. Landsc. Ecol. 35, 879–891. doi: 10.1007/s10980-020-00985-y
Cayuela, H., Lambrey, J., and Vacher, J. P. (2015). Highlighting the effects of land-use change on a threatened amphibian in a human-dominated landscape. Popul. Ecol. 57, 433–443. doi: 10.1007/s10144-015-0483-4
Chen, G. Z., Li, X., and Liu, X. P. (2020). Global projections of future urban land expansion under shared socioeconomic pathways. Nat. Commun. 11:537. doi: 10.1038/s41467-020-14386-x
Chen, L. D., Fu, B. J., and Zhao, W. W. (2006). Source-sink landscape theory and its ecological significance. Acta Ecol. Sin. 26, 1444–1449.
Chen, W. Y., Hu, F. Z. Y., Li, X., and Hua, J. (2017). Strategic interaction in municipal governments’ provision of public green spaces: a dynamic spatial panel data analysis in transitional China. Cities 71, 1–10. doi: 10.1016/j.cities.2017.07.003
Cheng, C., Yang, X., and Cai, H. (2021). Analysis of spatial and temporal changes and expansion patterns in mainland Chinese urban land between 1995 and 2015. Remote Sens. 13:2090. doi: 10.3390/rs13112090
Chi, Q., Zhou, S., and Wang, L. (2021). Exploring on the eco-climatic effects of land use changes in the influence area of the Yellow River Basin from 2000 to 2015. Land 10:601. doi: 10.3390/land10060601
Dadashpoor, H., Azizi, P., and Moghadasi, M. (2019). Land use change, urbanization, and change in landscape pattern in a metropolitan area. Sci. Total Environ. 655, 707–719. doi: 10.1016/j.scitotenv.2018.11.267
d’Amour, C. B., Reitsma, F., and Baiocchi, G. (2017). Future urban land expansion and implications for global croplands. PNAS 114, 8939–8944. doi: 10.1073/pnas.1606036114
Elhorst, J. P. (2014). “Dynamic Spatial Panels: Models, Methods and Inferences,” in Spatial Econometrics. Springer Briefs in Regional Science, ed. Springer (Berlin: Springer), 95–119. doi: 10.1007/978-3-642-40340-8_4
Ellis, E. C., Goldewijk, K. K., and Siebert, S. (2010). Anthropogenic transformation of the biomes, 1700 to 2000. Global Ecol. Biogeogr. 19, 589–606. doi: 10.1111/j.1466-8238.2010.00540.x
Elmqvist, T., Andersson, E., and Frantzeskaki, N. (2019). Sustainability and resilience for transformation in the urban century. Nat. Sustain. 2, 267–273. doi: 10.1038/s41893-019-0250-1
Ertur, C., and Koch, W. (2007). Growth, technological interdependence and spatial externalities: theory and evidence. J. Appl. Economet. 22, 1033–1062. doi: 10.1002/jae.963
Fang, F., Ma, L., and Fan, H. (2020). The spatial differentiation of quality of rural life based on natural controlling factors: a case study of Gansu Province. China J. Environ. Manage. 264:110439. doi: 10.1016/j.jenvman.2020.110439
Feng, Y., Wang, X., and Du, W. (2019). Effects of environmental regulation and FDI on urban innovation in China: a spatial Durbin econometric analysis. J. Clean. Prod. 235, 210–224. doi: 10.1016/j.jclepro.2019.06.184
Flörke, M., Schneider, C., and McDonald, R. I. (2018). Water competition between cities and agriculture driven by climate change and urban growth. Nat. Sustain. 1, 51–58. doi: 10.1038/s41893-017-0006-8
Ghosh, M. K., Kumar, L., and Roy, C. (2016). Mapping long-term changes in mangrove species composition and distribution in the Sundarbans. Forests 7:305. doi: 10.3390/f7120305
Ghosh, S., Chatterjee, N. D., and Dinda, S. (2021). Urban ecological security assessment and forecasting using integrated DEMATEL-ANP and CA-Markov models: a case study on Kolkata Metropolitan Area, India. Sustain. Cities Soc. 68:102773. doi: 10.1016/j.scs.2021.102773
Gleeson, T., Wang-Erlandsson, L., and Zipper, S. C. (2020). The water planetary boundary: interrogation and revision. One Earth 2, 223–234. doi: 10.1016/j.oneear.2020.02.009
Gong, P., Li, X., and Wang, J. (2020). Annual maps of global artificial impervious area (GAIA) between 1985 and 2018. Remote Sens. Environ. 236:111510. doi: 10.1016/j.rse.2019.111510
Güneralp, B., Güneralp, I., and Liu, Y. (2015). Changing global patterns of urban exposure to flood and drought hazards. Global Environ. Chang. 31, 217–225. doi: 10.1016/j.gloenvcha.2015.01.002
Guo, A. D., Yang, J., and Sun, W. (2020). Impact of urban morphology and landscape characteristics on spatiotemporal heterogeneity of land surface temperature. Sustain. Cities Soc. 63:102443. doi: 10.1016/j.scs.2020.102443
Huang, Q., Zhang, H., and van Vliet, J. (2021). Patterns and distributions of urban expansion in global watersheds. Earth’s Future 9:e2021EF002062.
Huang, Q. X., Zhao, X., and He, C. Y. (2019). Impacts of urban expansion on wetland ecosystem services in the context of hosting the Winter Olympics: a scenario simulation in the Guanting Reservoir Basin. China Reg. Environ. Change. 19, 2365–2379. doi: 10.1007/s10113-019-01552-1
Jantz, S. M., Barker, B., and Brooks, T. M. (2015). Future habitat loss and extinctions driven by land-use change in biodiversity hotspots under four scenarios of climate-change mitigation. Conserv. Biol. 29, 1122–1131. doi: 10.1111/cobi.12549
Jiang, L., Zuo, Q., and Ma, J. (2021). Evaluation and prediction of the level of high-quality development: a case study of the Yellow River Basin. China Ecol. Indic. 129:107994. doi: 10.1016/j.ecolind.2021.107994
Jing, M. X., Cai, Fu, Wang, X. J., and Zhao, X. J. (2020). The vegetation coverage spatial characteristics in the Three River source region. J. Arid Land Res. Environ. 34, 141–147.
Keita, M. A., Ruan, R. Z., and An, R. (2021). Spatiotemporal change of urban sprawl patterns in Bamako district in Mali based on time series analysis. J. Urban Sci. 5:4. doi: 10.3390/urbansci5010004
Kopp, D., and Allen, D. (2021). Scaling spatial pattern in river networks: the effects of spatial extent, grain size and thematic resolution. Landsc. Ecol. 36, 2781–2794. doi: 10.1007/s10980-021-01270-2
Kowe, P., Mutanga, O., and Dube, T. (2021). Advancements in the remote sensing of landscape pattern of urban green spaces and vegetation fragmentation. Int. J. Remote Sens. 42, 3797–3832. doi: 10.1080/01431161.2021.1881185
Kuenzer, C., Ottinger, M., and Liu, G. (2014). Earth observation-based coastal zone monitoring of the Yellow River Delta: dynamics in China’s second largest oil producing region over four decades. Appl. Geogr. 55, 92–107. doi: 10.1016/j.apgeog.2014.08.015
Lambin, E. F., and Meyfroidt, P. (2011). Global land use change, economic globalization, and the looming land scarcity. PNAS 108, 3465–3472. doi: 10.1073/pnas.1100480108
Lausch, A., Blaschke, T., and Haase, D. (2015). Understanding and quantifying landscape structure–A review on relevant process characteristics, data models and landscape metrics. Ecol. Model. 295, 31–41. doi: 10.1016/j.ecolmodel.2014.08.018
Lesage, J., and Pace, R. (2014). The biggest myth in spatial econometrics. Econometrics 2, 217–249. doi: 10.3390/econometrics2040217
Li, H., Peng, J., and Yanxu, L. (2017). Urbanization impact on landscape patterns in Beijing City. China 82, 50–60. doi: 10.1016/j.ecolind.2017.06.032
Li, S., Sun, Z. Q., Wang, Y. F., and Wang, Y. X. (2021). Understanding urban growth in Beijing-Tianjin-Hebei region over the past 100 years using old maps and landsat data. Remote Sens. 13:3264. doi: 10.3390/rs13163264
Liang, X., Liu, X., Li, X., Chen, Y., Tian, H., Yao, Y., et al. (2018). Delineating multi-scenario urban growth boundaries with a CA-based FLUS model and morphological method. Landsc. Urban Plan. 177, 47–63. doi: 10.1016/j.landurbplan.2018.04.016
Liang, Z., Wu, S., and Wang, Y. (2020). The relationship between urban form and heat island intensity along the urban development gradients. Sci. Total Environ. 708:135011. doi: 10.1016/j.scitotenv.2019.135011
Lin, M., Biswas, A., and Bennett, E. M. (2020). Socio-ecological determinants on spatiotemporal changes of groundwater in the Yellow River Basin. China. Sci. Total Environ. 731:138725. doi: 10.1016/j.scitotenv.2020.138725
Liu, L. M., and Wu, J. G. (2021). Ecosystem services-human wellbeing relationships vary with spatial scales and indicators: the case of China. Resour. Conserv. Recycl. 172:105662. doi: 10.1016/j.resconrec.2021.105662
Liu, P., Jia, S. J., Han, R. M., and Zhang, H. W. (2018). Landscape pattern and ecological security assessment and prediction using remote sensing approach. J. Sens. 2018, 1–14. doi: 10.1155/2018/1058513
Mård, J., Baldassarre, D. G., and Mazzoleni, M. (2018). Nighttime light data reveal how flood protection shapes human proximity to rivers. Sci. Adv. 4:eaar5779. doi: 10.1126/sciadv.aar5779
McDonald, R. I., Güneralp, B., and Huang, C. W. (2018). Conservation priorities to protect vertebrate endemics from global urban expansion. Biol. Conserv. 224, 290–299. doi: 10.1016/j.biocon.2018.06.010
McDonald, R. I., Mansur, A. V., and Ascensão, F. (2020). Research gaps in knowledge of the impact of urban growth on biodiversity. Nat. Sustain. 3, 16–24. doi: 10.1038/s41893-019-0436-6
Middleton, N. J., and Sternberg, T. (2013). Climate hazards in drylands: a review. Earth Sci. Rev. 126, 48–57. doi: 10.1016/j.earscirev.2013.07.008
Narducci, J., Quintas-Soriano, C., and Castro, A. (2019). Implications of urban growth and farmland loss for ecosystem services in the western United States. Land Use Policy 86, 1–11. doi: 10.1016/j.landusepol.2019.04.029
Newbold, T., Hudson, L. N., and Hill, S. L. L. (2015). Global effects of land use on local terrestrial biodiversity. Nature 520, 45–50. doi: 10.1038/nature14324
Pickard, B. R., Van Berkel, D., and Petrasova, A. (2017). Forecasts of urbanization scenarios reveal trade-offs between landscape change and ecosystem services. Landsc. Ecol. 32, 617–634. doi: 10.1007/s10980-016-0465-8
Qian, M., Pu, L. J., and Zhang, J. (2015). Urban spatial morphology evolution in Suzhou-Wuxi-Changzhou region based on improved landscape expansion index. Sci. Geol. Sin. 35, 314–321.
Riitters, K. H., O’Neill, R. V., and Hunsaker, C. T. (1995). A factor analysis of landscape pattern and structure metrics. Landsc. Ecol. 10, 23–39. doi: 10.1007/bf00158551
Rong, T. Q., Zhang, P. Y., and Jing, W. L. (2020). Carbon dioxide emissions and their driving forces of land use change based on Economic Contributive Coefficient (ECC) and Ecological Support Coefficient (ESC) in the Lower Yellow River Region (1995–2018). Energies 13:2600. doi: 10.3390/en13102600
Seto, K. C., Guneralp, B., and Hutyra, L. R. (2012). Global forecasts of urban expansion to 2030 and direct impacts on biodiversity and carbon pools. PNAS 109, 16083–16088. doi: 10.1073/pnas.1211658109
Song, X. P., Hansen, M. C., and Stehman, S. V. (2018). Global land change from 1982 to 2016. Nature 560, 639–643. doi: 10.1038/s41586-018-0411-9
Song, Y., Wang, M., and Sun, X. (2021). Quantitative assessment of the habitat quality dynamics in Yellow River Basin. China Environ. Monit. Assess. 193:614. doi: 10.1007/s10661-021-09404-4
Stoica, I.-V., Zamfir, D., and Vîrghileanu, M. (2021). Evaluating the territorial impact of built-up area expansion in the surroundings of Bucharest (Romania) through a multilevel approach based on landsat satellite imagery. Remote Sens. 13:3969. doi: 10.3390/rs13193969
Tanner, E. P., and Fuhlendorf, S. D. (2018). Impact of an agri-environmental scheme on landscape patterns. Ecol. Indic. 85, 956–965. doi: 10.1016/j.ecolind.2017.11.043
Tubiello, F. N., Salvatore, M., and Ferrara, A. F. (2015). The contribution of agriculture, forestry and other land use activities to global warming, 1990–2012. Glob Chang Biol. 21, 2655–2660. doi: 10.1111/gcb.12865
Van Vliet, J. (2019). Direct and indirect loss of natural area from urban expansion. Nat. Sustain. 2, 755–763. doi: 10.1038/s41893-019-0340-0
Wiedmann, T., and Lenzen, M. (2018). Environmental and social footprints of international trade. Nat. Geosci. 11, 314–321. doi: 10.1038/s41561-018-0113-9
Wihbey, J. (2017). Boundary Issues: the 2016 atlas of urban expansion indicates global dedensification. City Scape. 19, 21–30.
Winkler, K., Fuchs, R., and Rounsevell, M. (2021). Global land use changes are four times greater than previously estimated. Nat. Commun. 12:2501. doi: 10.1038/s41467-021-22702-2
Wohlfart, C., Kuenzer, C., and Chen, C. (2016). Social–ecological challenges in the Yellow River basin (China): a review. Environ. Earth Sci. 75:1066. doi: 10.1007/s12665-016-5864-2
Xiao, F. J. (2021). Impact of climate change on ecological security of the Yellow River Basin and its adaptation countermeasures. J. Landsc. Res. 13, 51–56. doi: 10.16785/j.issn1943-989x.2021.4.012
Xiao, R., Cao, W., Liu, Y., and Lu, B. (2022). The impacts of landscape patterns spatio-temporal changes on land surface temperature from a multi-scale perspective: a case study of the Yangtze River Delta. Sci. Total Environ. 821:153381. doi: 10.1016/j.scitotenv.2022.153381
Xu, Z., Li, Y., and Chau, S. (2020). Impacts of international trade on global sustainable development. Nat. Sustain. 3, 964–971. doi: 10.1038/s41893-020-0572-z
Yang, J., Zhan, Y. X., and Xiao, X. M. (2020). Investigating the diversity of land surface temperature characteristics in different scale cities based on local climate zones. Urban Clim. 34:100700. doi: 10.1016/j.uclim.2020.100700
Yang, Y., Liu, Y., and Li, Y. (2018). Quantifying spatio-temporal patterns of urban expansion in Beijing during 1985–2013 with rural-urban development transformation. Land Use Policy 74, 220–230. doi: 10.1016/j.landusepol.2017.07.004
Zhang, P. Y., Li, Y. Y., and Jing, W. L. (2020b). Comprehensive assessment of the effect of urban built-up land expansion and climate change on net primary productivity. Complexity 2020, 1–12. doi: 10.1155/2020/8489025
Zhang, P. Y., Yang, D., and Qin, M. Z. (2020c). Spatial heterogeneity analysis and driving forces exploring of built-up land development intensity in Chinese prefecture-level cities and implications for future Urban Land intensive use. Land Use Policy 99:104958. doi: 10.1016/j.landusepol.2020.104958
Zhang, Y., Geng, W. L., and Zhang, P. Y. (2020d). Dynamic changes, spatiotemporal differences and factors influencing the urban eco-efficiency in the lower reaches of the Yellow River. Int. J. Env. Res. Pub. He. 17:7510. doi: 10.3390/ijerph17207510
Zhang, J. Q., Luo, T., Xu, M., and Wang, Y. Y. (2020a). Influence of urban spatial expansion on the regionally ecological security pattern in Min-Delta region. J. Ecol. 40, 5113–5123.
Zhang, Y., Lyu, X., Liu, B., and Wu, D. (2021). Spatial relationships between ecosystem services and socioecological drivers across a large-scale region: a case study in the Yellow River Basin. Sci. Total Environ. 766:142480. doi: 10.1016/j.scitotenv.2020.142480
Zhao, Z. Q., Sharifi, A., Dong, X., Shen, L. S., and He, B. J. (2021). Spatial Variability and Temporal Heterogeneity of Surface Urban Heat Island Patterns and the Suitability of Local Climate Zones for Land Surface Temperature Characterization. Remote Sens. 13:4338. doi: 10.3390/rs13214338
Zhou, L., Dang, X. W., and Mu, H. W. (2020). Cities are going uphill: slope gradient analysis of urban expansion and its driving factors in China. Sci. Total Environ. 775, 1–10. doi: 10.1016/j.scitotenv.2021.145836
Keywords: spillover effect, Spatial Durbin Panel Model, built-up land expansion, ecological constraint, Yellow River Basin
Citation: Yang D, Lou Y, Zhang P and Jiang L (2022) Spillover Effects of Built-Up Land Expansion Under Ecological Security Constraint at Multiple Spatial Scales. Front. Ecol. Evol. 10:907691. doi: 10.3389/fevo.2022.907691
Received: 30 March 2022; Accepted: 26 April 2022;
Published: 26 May 2022.
Edited by:
Jun Yang, Northeastern University, ChinaReviewed by:
Shaohua Wang, Aerospace Information Research Institute (CAS), ChinaXiao Xie, Key Lab for Environmental Computation and Sustainability of Liaoning Province, Institute of Applied Ecology (CAS), China
Bao-Jie He, Chongqing University, China
Copyright © 2022 Yang, Lou, Zhang and Jiang. This is an open-access article distributed under the terms of the Creative Commons Attribution License (CC BY). The use, distribution or reproduction in other forums is permitted, provided the original author(s) and the copyright owner(s) are credited and that the original publication in this journal is cited, in accordance with accepted academic practice. No use, distribution or reproduction is permitted which does not comply with these terms.
*Correspondence: Pengyan Zhang, cGVuZ3lhbnpoQDEyNi5jb20=; Ling Jiang, Y3VmZWppYW5nbGluZ0AxMjYuY29t